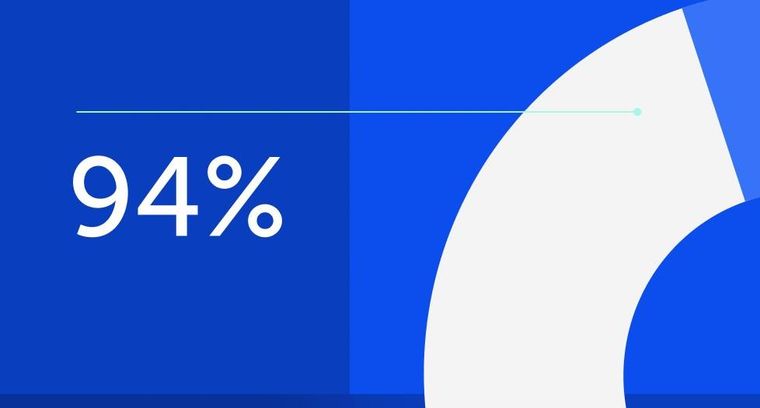
94% of researchers rate our articles as excellent or good
Learn more about the work of our research integrity team to safeguard the quality of each article we publish.
Find out more
ORIGINAL RESEARCH article
Front. Microbiol., 10 February 2025
Sec. Microorganisms in Vertebrate Digestive Systems
Volume 16 - 2025 | https://doi.org/10.3389/fmicb.2025.1526362
Probiotics as antibiotic alternatives are unstable for use under stress in clinical applications. To explore the influence of catecholamine hormones on probiotic bacterial inhibition and antimicrobial activity, we tested the effects of norepinephrine (NE) on Levilactobacillus in vitro and in a mouse model. The in vitro results showed that in the presence of NE, 80% of Levilactobacillus strains showed increased growth rate and more than 80% of the strains indicated lower antimicrobial activity at 22 h. Furthermore, in the mouse model, NE weakens the protective effect of L. brevis 23,017 on Escherichia coli infection, which is shown by the decreased ability of antibacterial colonization, antioxidation, and anti-inflammation, and downregulating the expression of antioxidant genes and intestinal mucosal barrier-related genes. At the same time, the addition of NE modulates the bacterial microbiota richness and diversity in the intestine, disrupting the balance of intestinal probiotics. These findings provide evidence that NE reduces the probiotic ability of Levilactobacillus and illustrates the plasticity of the probiotics in response to the intestinal microenvironment under stress.
In 1992, Lyte and Ernst introduced the concept of “microbial endocrinology” to assess the effect of bacterial growth under stress hormones, thus showing the two-way interaction between microorganisms and humans’ neuroendocrine factors (Lyte and Ernst, 1992). In past research, stress could increase neuroendocrine hormones, particularly glucocorticoids and catecholamines (Felten and Olschowka, 1987; Lymperopoulos et al., 2008; Webster Marketon and Glaser, 2008). Catecholamine hormones, including epinephrine, norepinephrine (NE), and dopamine, can affect the growth of many pathogenic bacteria in vitro (Coulanges et al., 1997; Nakano et al., 2007; Sandrini et al., 2014; Gao et al., 2019). Some studies have also shown that the virulence and biofilm formation of bacteria, such as Salmonella (Hiller et al., 2019) and Escherichia coli (E. coli) (Vlisidou et al., 2004), can be regulated by catecholamines, and this affects the outcome of infection by these bacteria on numerous hosts. However, there are few reports on the effect of catecholamine hormones on probiotics. Combined with proteomic analysis, Scardaci et al. assessed that in vitro norepinephrine (NE) treatment of Enterococcus faecalis enhanced the abundance of proteins involved in adhesion and immune stimulation (Scardaci et al., 2021). Cambronel et al. (2020) proposed that in E. faecalis, catecholamine hormones can promote adherence to eukaryotic cells and biofilm formation, and structural modeling and molecular docking confirmed that E. faecalis contains adrenergic sensors that interact with epinephrine and NE. However, the aforementioned studies were only based on bioinformatics analysis and in vitro tests to investigate the molecular level or biofilm and adhesion of probiotics.
Some researchers have also conducted a few in vivo experiments. Dong et al. discovered that NE increased the ability of Aeromonas hydrophila to proliferate in the lungs of mice (Dong et al., 2016). Some studies have also shown that catecholamine hormones can increase the pathogenicity of Salmonella in mouse, chicken, and bovine models (Williams et al., 2006; McCuddin et al., 2008; Methner et al., 2008; Pullinger et al., 2010b; Pullinger et al., 2010a), Yersinia ruckeri toward rainbow trout (Torabi Delshad et al., 2019), A. hydrophila in crucian (Gao et al., 2019), Vibrio harveyi and Vibrio campbellii in juvenile shrimp (Pande et al., 2014; Yang et al., 2014), and Pseudomonas aeruginosa toward Galleria mellonella larvae (Cambronel et al., 2019). Nevertheless, these studies have only looked at pathogenic microorganisms, and no scholars have yet explored the effect of catecholamine hormones on probiotics in vivo. We hypothesize that this interaction of host signals with Levilactobacillus strains may affect their bacterial properties and influence their probiotic effects. A better understanding of microbial endocrinology in the field of probiotics will allow a more comprehensive interpretation of how stress hormones are involved in the colonization of the microbiota and the ecological balance of the gut or other organs, which may help in the development of new therapies with medical and economic benefits.
In our research, we tried to evaluate the effect of NE on the probiotic properties Levilactobacillus based on the body injury caused by E. coli, which could potentially provide ideas to improve the unstable application effects of Levilactobacillus as antibiotic alternatives. For this purpose, (i) we simulated the internal environment (serum-SAPI medium) in vitro to investigate the effects of NE on the growth rate, viable bacteria count, bacterial inhibition capacity, and acid production capacity of Levilactobacillus strains; (ii) we selected the most suitable probiotic Levilactobacillus strains for investigating the effect of NE on the probiotic properties of Levilactobacillus in a mouse model; (iii) we established a BALB/c mouse model with E. coli standard strain infection and Levilactobacillus treatment; and (iv) we observed the intestinal pathological sections, oxidative damage, expression of inflammatory factors, intestinal mucosal barrier-related factors, Nrf2 and TLR4 and their downstream genes, and microbial amplicon sequencing and biological information analysis. Our findings shed light on the involvement of stress-related hormones in probiotics’ positive effects on the organism.
The E. coli CVCC230 strain was purchased from the China Veterinary Drug Supervision Institute. Nalidixic acid was used to induce the CVCC230 strain to be resistant to 50 μg/mL nalidixic acid with the method in the reference (Daudelin et al., 2011). Levilactobacillus brevis L5, 23010, 23017, 27197, 27053, 21060, 27058 and 23027 were isolated by our laboratory (Cui et al., 2018; Shi et al., 2019). As in almost all previous studies, the serum-SAPI medium was used in this study, which simulates the internal environment of the host (Lyte and Ernst, 1992; Freestone et al., 1999; Bearson et al., 2008; Li et al., 2009; Inaba et al., 2016).
The effect of NE (Sigma-Aldrich, USA) on the growth of Levilactobacillus strains was carried out according to the method of Inaba et al. (2016). The bacteria were cultivated in serum-SAPI medium in a ratio of 1:100 as the Levilactobacillus groups and those added to NE (100 μM) as the Levilactobacillus + NE groups. We selected 100 μM NE for in vitro testing based on the concentrations in reference (Cogan et al., 2007; Gao et al., 2019; Scardaci et al., 2021). The bacterial suspension was collected at time periods of 2 h, 4 h, 6 h, 8 h, 12 h, 24 h, 28 h, 32 h, and 48 h. At the same time, live bacterial counts were also recorded.
The antimicrobial activity of NE on Levilactobacillus strains was evaluated using an agar-well diffusion test, as described in the reference (Shi et al., 2019). The Levilactobacillus culture solution and 100 μM NE were added to the serum-SAPI medium as the experimental group, and the Levilactobacillus culture solution alone was used as the control group. The antimicrobial activity was assessed against E. coli (CVCC230), Staphylococcus aureus (CVCC26003), and Pseudomonas aeruginosa (ATCC9027).
The co-culture of the probiotic L. brevis 23,017 and pathogenic bacteria E. coli CVCC230 was carried out according to Yang et al. (2015), with slight modifications. They were suspended in sterile phosphate-buffered saline (PBS) before being co-inoculated in the serum-SAPI medium at a cell density of 1 × 106 CFU/mL and incubated aerobically for 24 h at 37°C. The samples were then serially diluted and plated on eosin-methylene blue (EMB) agar containing 50 μg/mL nalidixic acid to enumerate the number of pathogen colonies. EMB medium is a selective medium for E. coli, which appears as purple-black colonies with a green metallic luster when E. coli cultured in EMB medium.
The titratable acidity of the culture supernatant was determined by acid–base titration, following the methods described in reference (Navrátilova et al., 2022). The culture supernatant was collected at time periods of 2 h, 4 h, 6 h, 8 h, 12 h, 24 h, 28 h, 36 h, and 48 h. Hydrogen peroxide production was determined according to Eschenbach et al. (1989).
The Ethical Committee of the Institute approved all scientific experiments. All applicable international and national guidelines for the care and use of animals in experiments were followed. Approval (NEAUEC-20, 3 March 2020) was obtained from the Institutional Committee of Northeast Agricultural University for animal experiments. SPF BALB/c female mice (6–8 weeks of age) were purchased from the Laboratory Animal Centre, the Second Affiliated Hospital of Harbin Medical University (Harbin, China). The mice were maintained in a controlled environment and had free access to rodent food and tap water during a 12-h cycle of light and darkness.
In order to select the concentration and route of administration of NE, we first performed pre-experiments. The mice were divided randomly into five groups (n = 5/group), and they were vaccinated orally five different concentrations of NE, 16.9 mg/kg/day (100 μM), 8.45 mg/kg/day (50 μM), 4.23 mg/kg/day (25 μM), 1.69 mg/kg/day (12.5 μM), and 0 mg/kg/day (0 μM). The mice were kept under observation and the disease activity index (DAI) was recorded every 6 h and the NE administration route that causes the most significant clinical symptoms was identified. DAI is the sum of individual scores recorded for blood stool, stool consistency, and weight loss (Shinde et al., 2019). According to the method in literature (Cooper et al., 1993), the body weight of mice was recorded twice a day at the same time, and the average value was taken. In brief, scores were determined by reference.
Based on the previous in vitro test data, we selected L. brevis 23,017 for animal testing. Before bacterial inoculation, the animals were given 0.25 mL of a 0.2 mol/L sodium bicarbonate solution. A total of 25 mice were randomly divided into 5 groups (5 mice in each) in a completely random design. Dietary treatments included the control group with oral PBS, the NE group with oral NE, the CVCC230 group with oral E. coli CVCC230, the 23,017 + CVCC230 group with oral L. brevis 23,017 and E. coli CVCC230, and the 23,017 + NE + CVCC230 group with oral L. brevis 23,017 cultured in the medium containing NE and E. coli CVCC230. The specific mouse infection model is shown in Figure 1. After 15 days of treatment, the mice in each group were sacrificed and used for the subsequent analysis. The blood was collected from the eyeballs, and the serum was separated and stored in a − 70°C freezer for further analysis. The duodenum tissues were immediately collected for experiments and fixed in 10% formalin for histological evaluation. The small intestine was collected for biological information analysis.
The bacterial enumeration of the duodenum was determined by the modified method of Nour et al. (2021). The entire duodenum was sampled for postmortem clinical assessment of parameters. The EMB containing 50 μg/mL nalidixic acid was used for counting agar.
According to the previous pathological tissue staining protocol (Trajković et al., 2007), the tissues were sectioned and images were obtained at 100× and 400× magnifications, respectively.
The antioxidant capacities of duodenum were determined using assay kits according to the manufacturer’s instructions (Nanjing Jiancheng Bioengineering Institute, Nanjing, China). The activities of superoxide dismutase (SOD), glutathione peroxidase (GSH-Px), and total antioxidant capacity (T-AOC) were expressed as U per mg protein. The activities of malondialdehyde (MDA) were expressed as nmol per mg protein.
The levels of interleukin-6 (IL-6), interleukin-10 (IL-10), interleukin-1β (IL-1β), and myeloperoxidase (MPO) were measured via an enzyme-linked immunosorbent assay (ELISA) kit according to the manufacturer’s instructions (Boster Biological Technology, China).
Based on the method of Gao et al. (2019), the detection of intestinal mucosal barrier-related functional genes was implemented with a slight modification. The RNA was extracted from the duodenum tissue using an RNA extraction kit (TransGen Biotech, Beijing, China) and then reverse-transcribed into cDNA using ReverTra Ace® (TOYOBO, Shanghai, China) following the manufacturer’s protocol. The cDNA was used for quantification and expression of various genes, including inflammation-related genes, Nrf2, and their downstream genes and intestinal mucosal barrier-related genes. Furthermore, the real-time quantitative PCR reactions were carried out using the Applied Biosystems® 7,500 Real-Time PCR System (Analytik Jena AG, Germany) according to the manufacturer’s instructions. Actin β-action was taken as the reference gene. Primers for this study were synthesized with the company (Comate Bioscience Co., Ltd., Jilin, China). The primer sequence sets used are provided in Supplementary Table S1. The relative mRNA levels were quantified using the 2−ΔΔCt method (Livak and Schmittgen, 2001).
Fecal pellets and contents of small intestine were collected from mice in different groups, and the microbial amplicon sequencing was conducted by Genesky Biotechnologies Inc. (Shanghai, China). Alpha diversity was used to analyze the complexity of species diversity in each sample, which included Chao1, ACE, Shannon, and Simpson.
All the data are expressed as ±standard error of the mean. The SPSS 22.0 software (SPSS Inc., Chicago, IL, USA) was utilized for statistical analyses. The one-way ANOVA with the least significant difference (LSD) post-hoc test was used to identify the significant differences between groups. p < 0.05 was considered to be statistically significant.
To investigate whether NE has an effect on the growth of Levilactobacillus strains, we examined the growth rate and viable bacterial counts in ten strains of Levilactobacillus in vitro. In 0–48 h, the addition of NE resulted in an increase in the final culture density of 8 (80%) Levilactobacillus strains over control cultures (Figure 2A; Supplementary Figure S1) in addition to the 27,197 and 23,027 groups.
Figure 2. The effect of norepinephrine (NE) on the growth of Levilactobacillus. (A) Heatmap representing the OD600 value of Levilactobacillus growth within 0–48 h. Asterisks indicate strains with NE enhanced growth at 0–48 h. (B) Heatmap of the number of viable bacteria of Levilactobacillus growth within 0–48 h, the unit is colony-forming units per milliliter (107 CFU/mL). Asterisks indicate strains with NE enhanced growth at 0–24 h.
According to Figure 2B and Supplementary Figure S2, the growth trend of the average Levilactobacillus viable count is similar to the trend of the bacterial growth curve. In 0–24 h, the addition of NE resulted in an increase in the viable count of 9 (90%) Levilactobacillus strains over control cultures. In general, the results showed that NE has a promotive effect on the growth of most Levilactobacillus strains.
To explore the effect of NE on the inhibitory effect of probiotic Levilactobacillus strains on E. coli, S. aureus, and P. aeruginosa in vitro, we examined their antimicrobial activity at 8 h and 22 h. As shown in Figure 3, at 8 h for the Levilactobacillus strains tested, NE inhibited the antimicrobial activity of 3 (30%) strains against E. coli, with 2 of them being significantly inhibited; NE inhibited 3 (43%) strains against S. aureus, with 1 of them was significantly inhibited; and NE inhibited 7 (70%) strains against P. aeruginosa, with 4 of them being significantly inhibited. At 22 h, NE inhibited the antimicrobial activity of 8 (80%) Levilactobacillus strains against E. coli, with 6 of them being significantly inhibited; NE inhibited 6 (86%) Levilactobacillus strains against S. aureus, with 5 of them being significantly inhibited; and NE inhibited the 10 (100%) Levilactobacillus strains against P. aeruginosa, with 6 of them being significantly inhibited. In summary, the addition of NE reduced the antimicrobial activity of the majority of the Levilactobacillus strains.
Figure 3. Effect of NE on antibacterial ability of Levilactobacillus to E. coli, Staphylococcus aureus and Pseudomonas aeruginosa in 8 h and 24 h. Different colors represent different strains, blank columns represent Levilactobacillus acted alone, and columns with slashes represent Levilactobacillus groups acting with NE. Experiments were repeated three times, *, **, respectively, represent significant differences (p < 0.05), significant differences (p < 0.01).
To simulate the intestinal environment in which the three factors NE, L. brevis 23,017, and E. coli CVCC230 coexist in the intestine and to investigate the effect of catecholamine on the mixed bacteria, we performed co-culture experiments. Figure 4A and Supplementary Figure S3 depict the viable counts of E. coli. In the early phase (0–24 h), in most groups (50%), mixed cultures were higher than controls, and this proportion increased to 70% after 24 h. Overall, in the co-culture system, the addition of NE increased the number of viable E. coli.
Figure 4. The effect of NE on the antimicrobial and acid production capacity of Levilactobacillus. (A) Effect of NE on the number of viable Escherichia coli (E. coli) bacteria under co-culture conditions, heatmap of the number of viable bacteria of E. coli (107 CFU/mL). Asterisks indicate that co-culture with NE enhanced the number of viable bacteria of E. coli. (B) Effect of NE on acid production of Levilactobacillus, heatmap of the pH values of culture supernatant. Asterisks indicate that co-culture with NE enhanced the acid production capacity.
To investigate the reason why NE reduces the antimicrobial activity of Levilactobacillus strains, we examined their acid production capacity. As shown in Figure 4B and Supplementary Figure S4, for 60% of the tested strains, Levilactobacillus strains grown with NE generated higher concentrations of acid than those grown without it. However, for the ATCC4356, 27,197, 27,053, and 21,060 strains, the capacity of acid production was similar with or without NE. The addition of NE did not affect the ability of Levilactobacillus strains to produce hydrogen peroxide (data not shown).
Mice orally administered with 8.45 mg/kg/day (50 μM) NE showed depression and loss of appetite within 48 h. Symptoms were relieved after 72 h, and normal diet was resumed. The mice in the high-concentration group of 16.9 mg/kg/day (100 μM) showed depression, trembling bodies, no eating or drinking, loss of appetite, weight loss, and even death within 72 h. Therefore, the 8.45 mg/kg/day (50 μM) concentration of NE was considered optimal.
To investigate whether NE regulates the competition between Levilactobacillus and pathogenic bacteria for colonization sites, we examined the viable E. coli count. The viable E. coli count was recorded as log10 CFU/g. E. coli CVCC230 was not detected in the livers of each group of mice. In duodenum tissues, the 23,017 + CVCC230 (6.55 ± 0.19) group and the 23,017 + NE + CVCC230 (7.15 ± 0.13) group significantly reduced the number of E. coli compared to the CVCC230 group (7.32 ± 0.12), while the number of E. coli increased in the 23,017 + NE + CVCC230 group compared to the 23,017 + CVCC230 group. In colon tissues, the 23,017 + CVCC230 (5.38 ± 0.15) group and the 23,017 + NE + CVCC230 (5.95 ± 0.17) group also reduced the number of E. coli in comparison to the CVCC230 (6.98 ± 0.16) group, while the level of duodenum tissues in the number of E. coli was increased in comparison to the colon tissues, proving that the duodenum may be the main colonization site. We deduced that the addition of NE gives E. coli an advantage in the competition with Levilactobacillus for colonization sites.
To investigate the effect of NE on the capability of L. brevis 23,017 to protect the integrity of intestinal tissue, we performed a histological evaluation. As shown in Figure 5, microscopic duodenum damage observed in the CVCC230 group was significantly higher than that in the untreated control and NE groups (Figures 5C,H). There is a large area of tissue shedding, interstitial congestion, and other pathological changes. However, the histopathological signatures were ameliorated by L. brevis 23,017 monotherapies (Figures 5D,I), which obviously ameliorated the deterioration of mucosal tissue caused by E. coli CVCC230. Moreover, the degree of mucosal inflammation deteriorated in mice that were given the probiotic and NE acting together, with observations of increased mucosal lesions, vasodilation, and increased inflammatory infiltration (Figures 5E,J). That is, the addition of NE reduced the level of cellular integrity protection effect of L. brevis 23,017 on intestinal tissues.
Figure 5. Histological image of duodenum. (A,F) Group control; (B,G) Group NE; (C,H) Group CVCC230; (D,I): Group 23,017 + CVCC230; (E,J): Group 23,017 + NE + CVCC230. (A–E) Histological image of duodenum (100×); (F–J) Histological image of duodenum (400×). The red arrows in the figure mark the areas where the damage mainly occurs.
To determine whether NE acts on Levilactobacillus strains to influence their ability in reducing oxidative stress, we measured some indicators of the antioxidant enzyme system. As shown in Table 1, the CVCC230 group in the mice exhibited significantly elevated SOD (p < 0.05), GSH-Px (p < 0.05), and T-AOC activity in the duodenum in comparison to the control group. The 23,017 + CVCC230 group resulted in significantly elevated SOD, GSH-Px (p < 0.05), and T-AOC activity compared to the CVCC230 group, while the level of MDA is the opposite. At the same time, the levels of SOD (p < 0.05), GSH-Px (p < 0.05), and T-AOC are significantly reduced, while the level of MDA is elevated in comparison to the 23,017 + CVCC230 group. Taken together, these data show that the addition of NE increases oxidative damage induced by E. coli in the organism, indicating that NE decreased the antioxidant capacity of L. brevis 23,017.
To reflect the effect of NE on the ability of Levilactobacillus in modulating the inflammatory response at serological levels, serum IL-6, IL-1β, IL-10, and MPO were tested (Figure 6). In the CVCC230 group, the expressions of IL-6 (p < 0.01), IL-1β (p < 0.05), IL-10, and MPO (p < 0.01) were upregulated compared to the control group. The mice in the 23,017 + CVCC230 group were found to have downregulated levels of IL-6 (p < 0.01), IL-10 (p < 0.05), and MPO (p < 0.01) compared to the CVCC230 group, with increased expression of IL-1β. The expressions of IL-10 (p < 0.05) and MPO (p < 0.05) were upregulated, while those of IL-6 (p < 0.05) and IL-1β (p < 0.01) were downregulated in the 23,017 + NE + CVCC230 group compared to the 23,017 + CVCC230 group. Overall, the addition of NE affects the ability of L. brevis 23,017 in modulating the inflammatory response at serological levels.
Figure 6. Detection of inflammatory response at serological levels by Elisa method, abscissas represent groups and ordinates represent the concentration of IL-6, IL- IL-1β, IL-10 and MPO. Experiments were repeated three times, *, **, respectively, represent significant differences (p < 0.05), significant differences (p < 0.01).
To reflect the effect of NE on the ability of Levilactobacillus in modulating the inflammatory response at mRNA levels, IL-6, IL-1β, and TNF-α were tested (Figure 7A). IL-6 (p < 0.01), IL-1β (p < 0.01), and TNF-α (p < 0.01) were all upregulated in the NE-treated group compared to the control group. Compared to the control group, the expressions of IL-1β and TNF-α were downregulated, and the level of IL-6 was unchanged in the CVCC230 group. IL-6 (p < 0.01) and IL-1β (p < 0.05) expressions were upregulated and TNF-α expression was downregulated in the 23,017 + CVCC230 group compared to the CVCC230 group. Compared to the 23,017 + CVCC230 group, the expressions of IL-6 and IL-1β were downregulated and the expression of TNF-α was upregulated in the 23,017 + NE + CVCC230 group. Overall, the addition of NE affects the ability of L. brevis 23,017 in modulating the inflammatory response at mRNA levels.
Figure 7. (A) Detection of inflammatory response at mRNA levels. (B) Detection of mRNA levels of Nrf2 and its downstream genes. Real-time qPCR reactions were used to detect, and β-actin was seen as house-keeping gene. The abscissas represent groups, the ordinates represent 2−ΔΔCt value. Experiments were repeated three times, *, **, respectively, represent significant differences (p < 0.05), significant differences (p < 0.01).
To determine the potential role of NE in the antioxidant effect of Levilactobacillus, we detected the mRNA levels of Nrf2 and its downstream genes, an important transcription factor of the oxidative stress response (Figure 7B). As expected, treatment with CVCC230 significantly downregulated the Nrf2 (p < 0.01), HO-1 (p < 0.05), and NQO1 expressions compared to the control group, while after preventive Levilactobacillus treatment, the levels were significantly upregulated (p < 0.01). In contrast, preventive NE and Levilactobacillus treatments induced downregulation of the Nrf2 (p < 0.01), HO-1, and NQO1 (p < 0.01) expressions compared to the 23,017 + CVCC230 group. These results show that NE inhibits the expression of Nrf2 and its downstream genes in mRNA levels.
To investigate whether the action of NE on Levilactobacillus strains affects their ability to protect the integrity of the intestinal mucosa, we examined the mRNA levels of intestinal mucosal barrier-related genes. As shown in Figure 8, the mRNA expression profiles of CRS4C, Cryptdin-1, ZO-1, mucin-2, and iNOS in the CVCC230 group were decreased when compared to those of control mice, whereas OCLN (p < 0.01), TLR2 (p < 0.01), and TLR4 were increased. The mRNA expression profiles of CRS4C (p < 0.01), Cryptdin-1 (p < 0.01), ZO-1 (p < 0.01), mucin-2 (p < 0.01), OCLN, TLR4, and iNOS in the 23,017 + CVCC230 group were significantly elevated when compared to the CVCC230 group, while TNF-α and TLR2 (p < 0.01) expressions were downregulated. Compared to the 23,017 + CVCC230 group, the expressions of ZO-1, mucin-2, and TLR2 (p < 0.01) in the 23,017 + NE + CVCC230 group were increased, while the expressions of CRS4C, Cryptdin-1, OCLN, TLR4, and iNOS were decreased. In conclusion, NE affects the ability of L. brevis 23,017 in enhancing the expression of intestinal mucosal barrier-related genes.
Figure 8. Detection of genes related to the intestinal mucosal barrier at mRNA levels. Real-time qPCR reactions were used to detect, and β-actin was seen as house-keeping gene. The abscissas represent groups, the ordinates represent 2−ΔΔCt value. Experiments were repeated three times, *, **, respectively, represent significant differences (p < 0.05), significant differences (p < 0.01).
To investigate whether NE affects the function of Levilactobacillus in regulating intestinal health, we performed an analysis of the microbial composition of the intestinal flora (Figure 9). The 11 dominant bacterial phyla (with mean relative abundance >1%) are shown in Figure 9A, and at the genus level (Figure 9B), 16 dominant bacterial genera (average relative abundance >1%) could be identified. In comparison of the relative abundance of Levilactobacillus in each group, the relative abundance of Levilactobacillus was the highest in the NE group, followed by the normal control group. The members of the Levilactobacillus family were in low abundance in the 23,017 + NE + CVCC230 group as compared to the 23,017 + CVCC230 group. From the above results, we can see that NE induces an increase in the relative abundance of probiotics in the intestinal flora, and oral administration of NE affects the balance of intestinal flora.
Figure 9. (A) The intestinal microbiota at the phylum level were analyzed using 16S rRNA sequencing results. The horizontal axis represents the sample, and the vertical axis represents the relative richness value. (B) The intestinal microbiota at the genus level were analyzed using 16S rRNA sequencing results. The horizontal axis represents the sample, and the vertical axis represents the relative richness value.
To investigate whether NE affects the function of Levilactobacillus in regulating intestinal flora species diversity, we measured the richness and diversity of the intestine’s bacterial microbiota. The α diversity indices of the samples are shown in Table 2. The values of Chao1, ACE, and Simpson were increased in the CVCC230 and 23,017 + CVCC230 groups compared to the control group, while the value of Shannon was decreased. The values of Chao1, ACE, and Shannon in the 23,017 + NE + CVCC230 group were increased when compared to the 23,017 + CVCC230 group, while Simpson was decreased. There is no significant difference between each group. Overall, this result indicates that the 23,017 + NE + CVCC230 group had the highest species richness and the highest community diversity.
Microbial endocrinology is a relatively young scientific field, and for decades, scientists have studied the activity of catecholamines only in terms of host immune response, vasoconstriction, and so on. The direct effects on bacteria have been explored mostly in pathogenic bacteria and very few in probiotics (Boukerb et al., 2021). Many clinical diseases are induced by enterotoxigenic E. coli (ETEC), and several stress hormones, especially catecholamines, can aggravate the condition and cause anorexia, intestinal inflammation, and intestinal flora imbalance (McCracken et al., 1999; Bomba et al., 2014). Currently, treatment for E. coli infection relies primarily on antibiotics, but they can cause many side effects. As an antibiotic alternative, Levilactobacillus is extremely widespread in nature and plays a vital role in resistance to pathogen infection and keeping the gastrointestinal tract healthy (Round and Mazmanian, 2009; Liu et al., 2014; Ritze et al., 2014; Dowarah et al., 2017; Vinasco et al., 2019). However, it has different effects in different individuals, or in laboratory and clinical applications. Therefore, in this study, we tried for the first time to analyze the effects of NE on the probiotic properties of Levilactobacillus strains in vivo to resolve the inconsistent effect of the application of Levilactobacillus. This study fills some of the gaps in microbial endocrinology with respect to probiotics and is important for both the theoretical basis and clinical application of the study of stress hormone interactions with probiotics.
When the Levilactobacillus strains were tested in vitro in the presence of NE, 80% of the strains showed an increase in growth rate and the number of viable bacteria. This is in general agreement with majority of the previous studies of pathogenic Gram-positive bacteria (Belay and Sonnenfeld, 2002; Lyte et al., 2003; Sandrini et al., 2014; Boyanova, 2017). We speculate that NE-induced growth of Levilactobacillus strains may be related to iron homeostasis and gene transcription. The study by Bearson et al. indicated that Salmonella Typhimurium changes the biosynthesis of numerous cellular pathways to increase its growth rate in serum-SAPI minimal media to utilize the increased availability of iron provided by NE (Bearson et al., 2008; Boukerb et al., 2021). Iron sequestration is mainly due to the mammalian ferric-iron-binding proteins transferrin in serum and lactoferrin in mucosal secretions (Mietzner and Morse, 1994). Many studies demonstrating the influence of catecholamines on bacterial growth used a serum-like medium containing transferrin (Tf) and lactoferrin (Lf). Serum-SAPI minimal media more accurately simulate a stressful and bacteriostatic environment for the bacteria, and so more precisely they resemble the conditions that the bacteria may experience within the host (Mietzner and Morse, 1994; Freestone et al., 2003; Lyte, 2004). In several other studies, NE inhibits the growth of Prevotella intermedius (Jentsch et al., 2013) and Porphyromonas pulposus (Calil et al., 2014). The difference between promotion and inhibition may be related to the concentration of hormones (Roberts et al., 2002; Hegde et al., 2009; Jentsch et al., 2013; Calil et al., 2014), but we only selected the optimal concentration for investigation based on the clues provided by previous experiments. In the next step, we selected representative numbers of concentrations to investigate. Overall, our study demonstrates that NE has a beneficial effect on the growth of Levilactobacillus strains, and this study fills the gaps in microbial endocrinology in the study of probiotic Levilactobacillus strains.
The antimicrobial activity of more than 80% of the Levilactobacillus strains against three indicator bacteria at 22 h was decreased due to the presence of NE. This study investigates the effect of catecholamine hormones on the antimicrobial activity of probiotic Levilactobacillus strains. According to the results of the preliminary pre-experiment, we found that the effect of Levilactobacillus strains on E. coli, S. aureus, and P. aeruginosa was stronger, so we explored the effect of NE on the antimicrobial activity of Levilactobacillus strains using three indicator bacteria in this experiment. Metabolites of Levilactobacillus strains such as organic acids, hydrogen peroxide, antimicrobial peptides, and bactericidal proteins are the main substances that exert antibacterial effects (Awaisheh and Ibrahim, 2009; Gupta and Srivastava, 2014). Some studies have shown that catecholamines can play a role in free radical generation (Borisenko et al., 2000), and bacteria may promote adrenaline oxidation to adrenochrome and produce superoxide (Toulouse et al., 2019; Reiske et al., 2020). We hypothesized that if NE also reacted in this way, it would affect the probiotic viability of Levilactobacillus. We imply that NE may reduce the antibacterial effect of Levilactobacillus strains by affecting their metabolites. The results provide a theoretical basis for the next application of Levilactobacillus in practice.
By simulating the coexistence of three factors in the intestine in vivo, the interaction increases the number of viable bacteria of E. coli. Our previous study has shown that NE promotes the growth of E. coli CVCC230. Although we found that NE inhibited the anti-E. coli ability of Levilactobacillus strains and significantly increased the viable E. coli count in the three-factor mixed environment, it was impossible to conclude that NE reduced the antimicrobial activity of Levilactobacillus against E. coli CVCC230 in this environment as the competition for nutrients in the medium could also contribute to the higher counts. The role of NE in the organism is very complex and needs to be analyzed from multiple perspectives. Our discussion of the coexistence of the three factors in the intestine is not comprehensive enough, and we wish to provide clues for the next studies in the microbial endocrinology field, in vitro or in vivo.
In the presence of NE, the acid production capacity of 60% Levilactobacillus strains was enhanced without any effect on the hydrogen peroxide production capacity. Past studies have shown that the primary antimicrobial activity of certain strains depends on the production of acid or hydrogen peroxide (Blajman et al., 2015). However, we speculate that the diminished antimicrobial activity of NE on Levilactobacillus strains is not acting through these two characteristics in this study. NE probably reduces the antimicrobial capacity of Levilactobacillus strains by affecting the secretion of antimicrobial peptides or antimicrobial compounds (Awaisheh and Ibrahim, 2009; Ren et al., 2018). We attempted to elucidate the mechanism of NE action on Levilactobacillus strains through in vitro experiments, and our study provides a basis for the exploration of microbial endocrinology in Levilactobacillus.
The results of intestinal colonization and observation of pathological sections demonstrated that giving NE and L. brevis 23,017 together attenuated the inhibitory effect of E. coli CVCC230 colonization and increased mucosal lesions, vasodilatation, and inflammatory infiltration relative to the group given L. brevis 23,017 alone. The study of Cambronel et al. showed that catecholamine hormones influenced the adhesion of both pathogenic and probiotic Enterococcus faecalis (Cambronel et al., 2020). It has also been suggested that catecholamine hormones may have opposite effects on the ability of different pathogenic bacteria to adhere (Gonzales et al., 2013). We speculate that NE may have affected the adhesion and competition for attachment sites of L. brevis 23,017 and weakened its inhibitory effect on E. coli colonization. Previously, it was investigated that NE at the cellular level enhanced the probiotic properties of Enterococcus faecalis, such as resistance to bile salts, autoaggregation, and biofilm formation (Scardaci et al., 2021). However, our results demonstrate that NE negatively regulates the probiotic effect of L. brevis 23,017. In the context of the microbiota–gut axis communication, the activities of the organism at all levels are complex (Lyte, 2016), and our study is important to elucidate the interactions between symbiotic bacteria and their hosts.
The detection of oxidative stress-related markers concluded that NE decreased the antioxidant capacity of L. brevis 23,017. Many past studies have indicated that treatment with Levilactobacillus showed higher antioxidant capacity than that of other groups; the same was true for us (Ge et al., 2021). Previous studies have shown that the autotrophic antioxidant enzyme systems, chelated metal ions, and regulation of the intestinal bacteria group are the primary ways that the antioxidant mechanism of Levilactobacillus is exhibited (Li et al., 2012). We speculate that NE may also affect the antioxidant capacity of L. brevis 23,017 in these ways, and we intend to continue investigating the mechanisms at the gene level or signaling pathway in the next step. Overall, this again confirms that NE can reduce the probiotic effects of Levilactobacillus strains.
The assay of inflammatory factors at serological levels showed that NE acting on L. brevis 23,017 may promote an anti-inflammatory environment by downregulating the IL-6 and IL-1β levels and upregulating the levels of IL-10 and MPO transcripts. The NE group had significantly higher levels of inflammatory factor mRNA expression, and the relative tendency in the 23,017 group was completely opposite to that in the 23,017 + NE group, demonstrating that the addition of NE inhibited the regulation of intestinal inflammatory factors by L. brevis 23,017. The results obtained from our assay of serum and intestinal levels of inflammatory factors did not show a significant trend toward consistency. As reported in a previous study, Mu et al. indicated that the transcriptional level was not consistent in the intestine, spleen, and serum with Levilactobacillus treatment, suggesting gut-specific immunosuppression (Mu et al., 2017). Even though in some circumstances IL-6 signaling has been characterized as anti-inflammatory, it also plays a crucial role in increasing inflammation and immunity (Hirano et al., 2000; Vardam et al., 2007; Silver and Hunter, 2010; Rose-John, 2012). Moreover, many pro-tumor actions can be supported by stimulation of IL-6 trans signaling, which also has the ability to boost adaptive immunity against tumors (Fisher et al., 2014). Together, these findings suggest that NE has a role in the ability of L. brevis 23,017 to modulate the inflammatory response, but the exact pattern of action still needs to be explored.
In the mouse duodenum, NE on L. brevis 23,017 decreased Nrf2 and its downstream gene expression. Nrf2 is a transcription factor that helps regulate the cellular oxidative stress (Ma, 2013). It is an important regulator of cellular defense mechanisms against xenobiotics and oxidative stress (Tonelli et al., 2018). As a part of the gut microbiota, Levilactobacillus benefits in regulating oxidative stress in tissues and cells, and the results of Long et al. (2021) are similar to ours and show that L. fermentum can also promote high-level expression of Nrf2 and other downstream antioxidant factors such as HO-1 and NQO1. Previous research has shown that Nrf2 signaling pathways mediate the defense mechanisms against oxidative stress and inflammation (Fuentes et al., 2015; Li et al., 2018). Therefore, we speculate that NE acting on L. brevis 23,017 may reduce the antioxidant effect by regulating the expression of Nrf2 and its downstream genes then affect their protein expression. The addition of NE reduced the L. brevis 23,017 enhancement of intestinal mucosal barrier-related gene expression in vivo in the mouse. Catecholamines, particularly NE, are abundantly present in the intestinal mucosa during stressful conditions (Eisenhofer et al., 2004). Previous research has demonstrated that Levilactobacillus can improve intestinal mucosal status and increase the expression of intestinal mucosal barrier-associated proteins (Chaudière and Ferrari-Iliou, 1999; Mao et al., 2016), which is consistent with our study. However, NE acting on L. brevis 23,017 downregulated the expression of antimicrobial peptides CRS4C and Cryptdin-1 and the tight junction protein OCLN. We speculate that NE affects the probiotic effects of L. brevis 23,017 by affecting its ability to regulate intestinal mucosal secretion.
The effect of oral NE modulates the balance of intestinal flora, causing a decrease in the relative quantity of probiotic bacteria and an increase in the relative abundance of other harmful bacteria. NE may act on numerous gut microorganisms. According to the in vitro part of this study, NE attenuated the inhibitory effects of L. brevis 23,017 on E. coli, S. aureus, and P. aeruginosa. Since previous studies have shown that catecholamine hormones have a promoting effect on E. coli growth and virulence, we hypothesize that one of the reasons why the addition of NE causes an imbalance in the intestinal flora may be due to a reduction in the inhibitory effect of Levilactobacillus on the harmful microorganisms in the intestinal tract. The development and changes of the intestinal microbiota have a non-negligible impact on the development and treatment of many diseases (Linninge et al., 2019). Previous studies have shown that Levilactobacillus strains can regulate the disease process by maintaining the balance of intestinal flora (Mu et al., 2017). We suggest that the imbalance of intestinal flora regulated by NE action on Levilactobacillus and the change of intestinal flora will also regulate the immune response of the body, leading to the occurrence of stress, creating a vicious circle until it affects systemic immunity. The highest relative abundance of Levilactobacillus was obtained by the assay of intestinal flora in the NE group, followed by the normal control group. This was validated against the promotion of bacterial growth by in vitro levels of NE, demonstrating that NE also enhanced the growth of Levilactobacillus under complex conditions in the in vivo organism. Overall, the addition of NE modulates the balance of intestinal flora.
This study was only a basic one hoping to provide some clues for microbial endocrinology. Owing to the complexity of NE activity in the organism and the fact that the addition of NE may also affect some other gut microorganisms in the organism, we have examined only the changes in the organism that may be induced by the concomitant administration of NE and L. brevis. Hopefully, some evidence can be given that in animals, the addition of norepinephrine also reduces the probiotic effect of lactobacilli on the body. Studies have shown that catecholamine receptor antagonists are therapeutically important in the treatment of conditions such as hypertension, and adrenergic and dopaminergic antagonists block NE, epinephrine, and Dopa responses in bacteria (Freestone et al., 2008). Whether we can prevent the disease by blocking the catecholamine hormones in the body is the next major direction of our research. In the next step, we will use this study as a basis to investigate the effects of different types of receptor inhibitors and catecholamine hormones under different environmental conditions and adjust the assay indicators and methods to carry out more in-depth studies.
In conclusion, this is the first report on how probiotics and catecholamine hormones interact in a mouse model. The results showed that NE promoted growth while suppressing the antimicrobial capacity of Levilactobacillus strains, and the viable count of E. coli was increased in the presence of Levilactobacillus strains and neurohormone coexistence. In addition, the administration of NE in the ETEC-infected mouse model reduced the ability of L. brevis 23,017 in inhibiting pathogenic bacterial colonization of the intestine, inhibiting intestinal inflammatory cell infiltration, antioxidant capacity, protection of the intestinal mucosal barrier, and maintaining intestinal flora homeostasis. Our study is the first report to investigate the effect of catecholamine hormones on the properties of probiotics in vivo and may provide a new direction to address the unstable clinical application of Levilactobacillus strains and improve the application of Levilactobacillus as an antibiotic alternative.
The original contributions presented in the study are publicly available. This data can be found here: www.ncbi.nlm.nih.gov, accession number PRJNA1212890.
The animal study was approved by the Institutional Committee of Northeast Agricultural University. The study was conducted in accordance with the local legislation and institutional requirements.
LN: Conceptualization, Data curation, Investigation, Methodology, Software, Writing – original draft. MG: Investigation, Methodology, Supervision, Validation, Writing – original draft. YL: Data curation, Formal analysis, Writing – original draft. CW: Methodology, Writing – original draft. CZ: Data curation, Formal analysis, Writing – original draft. HD: Investigation, Methodology, Software, Writing – original draft. HL: Investigation, Methodology, Writing – original draft. FW: Conceptualization, Data curation, Formal analysis, Funding acquisition, Investigation, Methodology, Project administration, Resources, Software, Supervision, Validation, Visualization, Writing – original draft, Writing – review & editing. JG: Conceptualization, Data curation, Formal analysis, Funding acquisition, Investigation, Methodology, Project administration, Resources, Software, Supervision, Validation, Visualization, Writing – original draft, Writing – review & editing.
The author(s) declare that financial support was received for the research, authorship, and/or publication of this article. This research was supported by the National Natural Science Foundation of China (Grant No. 31672532), Earmarked Fund for China Agriculture Research System (CARS36), and the SIPT Project of Northeast Agricultural University.
We would like to thank Dr. Dexing Ma for assisting with pathological analysis.
The authors declare that the research was conducted in the absence of any commercial or financial relationships that could be construed as a potential conflict of interest.
The authors declare that no Gen AI was used in the creation of this manuscript.
All claims expressed in this article are solely those of the authors and do not necessarily represent those of their affiliated organizations, or those of the publisher, the editors and the reviewers. Any product that may be evaluated in this article, or claim that may be made by its manufacturer, is not guaranteed or endorsed by the publisher.
The Supplementary material for this article can be found online at: https://www.frontiersin.org/articles/10.3389/fmicb.2025.1526362/full#supplementary-material
Awaisheh, S. S., and Ibrahim, S. A. (2009). Screening of antibacterial activity of lactic acid bacteria against different pathogens found in vacuum-packaged meat products. Foodborne Pathog. Dis. 6, 1125–1132. doi: 10.1089/fpd.2009.0272
Bearson, B. L., Bearson, S. M. D., Uthe, J. J., Dowd, S. E., Houghton, J. O., Lee, I., et al. (2008). Iron regulated genes of Salmonella enterica serovar Typhimurium in response to norepinephrine and the requirement of fep DGC for norepinephrine-enhanced growth. Microbes Infect. 10, 807–816. doi: 10.1016/j.micinf.2008.04.011
Belay, T., and Sonnenfeld, G. (2002). Differential effects of catecholamines on in vitro growth of pathogenic bacteria. Life Sci. 71, 447–456. doi: 10.1016/S0024-3205(02)01683-1
Blajman, J., Gaziano, C., Zbrun, M. V., Soto, L., Astesana, D., Berisvil, A., et al. (2015). In vitro and in vivo screening of native lactic acid bacteria toward their selection as a probiotic in broiler chickens. Res. Vet. Sci. 101, 50–56. doi: 10.1016/j.rvsc.2015.05.017
Bomba, L., Minuti, A., Moisá, S. J., Trevisi, E., Eufemi, E., Lizier, M., et al. (2014). Gut response induced by weaning in piglet features marked changes in immune and inflammatory response. Funct. Integr. Genomics 14, 657–671. doi: 10.1007/s10142-014-0396-x
Borisenko, G. G., Kagan, V. E., Hsia, C. J., and Schor, N. F. (2000). Interaction between 6-hydroxydopamine and transferrin: “let my iron go”. Biochemistry 39, 3392–3400. doi: 10.1021/bi992296v
Boukerb, A. M., Cambronel, M., Rodrigues, S., Mesguida, O., Knowlton, R., Feuilloley, M. G. J., et al. (2021). Inter-kingdom signaling of stress hormones: sensing, transport and modulation of bacterial physiology. Front. Microbiol. 12:690942. doi: 10.3389/fmicb.2021.690942
Boyanova, L. (2017). Stress hormone epinephrine (adrenaline) and norepinephrine (noradrenaline) effects on the anaerobic bacteria. Anaerobe 44, 13–19. doi: 10.1016/j.anaerobe.2017.01.003
Calil, C. M., Oliveira, G. M., Cogo, K., Pereira, A. C., Marcondes, F. K., and Groppo, F. C. (2014). Effects of stress hormones on the production of volatile sulfur compounds by periodontopathogenic bacteria. Braz. Oral Res. 28, 1–8. doi: 10.1590/1807-3107BOR-2014.vol28.0008
Cambronel, M., Nilly, F., Mesguida, O., Boukerb, A. M., Racine, P.-J., Baccouri, O., et al. (2020). Influence of Catecholamines (epinephrine/norepinephrine) on biofilm formation and adhesion in pathogenic and probiotic strains of. Front. Microbiol. 11:1501. doi: 10.3389/fmicb.2020.01501
Cambronel, M., Tortuel, D., Biaggini, K., Maillot, O., Taupin, L., Réhel, K., et al. (2019). Epinephrine affects motility, and increases adhesion, biofilm and virulence of Pseudomonas aeruginosa H103. Sci. Rep. 9:20203. doi: 10.1038/s41598-019-56666-7
Chaudière, J., and Ferrari-Iliou, R. (1999). Intracellular antioxidants: from chemical to biochemical mechanisms. Food Chem. Toxicol. 37, 949–962. doi: 10.1016/S0278-6915(99)00090-3
Cogan, T. A., Thomas, A. O., Rees, L. E. N., Taylor, A. H., Jepson, M. A., Williams, P. H., et al. (2007). Norepinephrine increases the pathogenic potential of Campylobacter jejuni. Gut 56, 1060–1065. doi: 10.1136/gut.2006.114926
Cooper, H. S., Murthy, S. N., Shah, R. S., and Sedergran, D. J. (1993). Clinicopathologic study of dextran sulfate sodium experimental murine colitis. Lab. Investig. 69, 238–249
Coulanges, V., Andre, P., Ziegler, O., Buchheit, L., and Vidon, D. J. (1997). Utilization of iron-catecholamine complexes involving ferric reductase activity in Listeria monocytogenes. Infect. Immun. 65, 2778–2785. doi: 10.1128/iai.65.7.2778-2785.1997
Cui, X., Shi, Y., Gu, S., Yan, X., Chen, H., and Ge, J. (2018). Antibacterial and Antibiofilm activity of lactic acid Bacteria isolated from traditional artisanal Milk cheese from Northeast China against Enteropathogenic Bacteria. Probiotics Antimicrob. Proteins 10, 601–610. doi: 10.1007/s12602-017-9364-9
Daudelin, J.-F., Lessard, M., Beaudoin, F., Nadeau, E., Bissonnette, N., Boutin, Y., et al. (2011). Administration of probiotics influences F4 (K88)-positive enterotoxigenic Escherichia coli attachment and intestinal cytokine expression in weaned pigs. Vet. Res. 42:69. doi: 10.1186/1297-9716-42-69
Dong, Y., Liu, J., Pang, M., Du, H., Wang, N., Awan, F., et al. (2016). Catecholamine-stimulated growth of requires the ton B2 energy transduction system but is independent of the Amonabactin Siderophore. Front. Cell. Infect. Microbiol. 6:183. doi: 10.3389/fcimb.2016.00183
Dowarah, R., Verma, A. K., and Agarwal, N. (2017). The use of as an alternative of antibiotic growth promoters in pigs: a review. Anim. Nutr. 3, 1–6. doi: 10.1016/j.aninu.2016.11.002
Eisenhofer, G., Kopin, I. J., and Goldstein, D. S. (2004). Catecholamine metabolism: a contemporary view with implications for physiology and medicine. Pharmacol. Rev. 56, 331–349. doi: 10.1124/pr.56.3.1
Eschenbach, D. A., Davick, P. R., Williams, B. L., Klebanoff, S. J., Young-Smith, K., Critchlow, C. M., et al. (1989). Prevalence of hydrogen peroxide-producing Lactobacillus species in normal women and women with bacterial vaginosis. J. Clin. Microbiol. 27, 251–256. doi: 10.1128/jcm.27.2.251-256.1989
Felten, S. Y., and Olschowka, J. (1987). Noradrenergic sympathetic innervation of the spleen: II. Tyrosine hydroxylase (TH)-positive nerve terminals form synapticlike contacts on lymphocytes in the splenic white pulp. J. Neurosci. Res. 18, 37–48. doi: 10.1002/jnr.490180108
Fisher, D. T., Appenheimer, M. M., and Evans, S. S. (2014). The two faces of IL-6 in the tumor microenvironment. Semin. Immunol. 26, 38–47. doi: 10.1016/j.smim.2014.01.008
Freestone, P. P. E., Haigh, R. D., and Lyte, M. (2008). Catecholamine inotrope resuscitation of antibiotic-damaged staphylococci and its blockade by specific receptor antagonists. J. Infect. Dis. 197, 1044–1052. doi: 10.1086/529202
Freestone, P. P., Haigh, R. D., Williams, P. H., and Lyte, M. (1999). Stimulation of bacterial growth by heat-stable, norepinephrine-induced autoinducers. FEMS Microbiol. Lett. 172, 53–60. doi: 10.1111/j.1574-6968.1999.tb13449.x
Freestone, P. P. E., Haigh, R. D., Williams, P. H., and Lyte, M. (2003). Involvement of enterobactin in norepinephrine-mediated iron supply from transferrin to enterohaemorrhagic Escherichia coli. FEMS Microbiol. Lett. 222, 39–43. doi: 10.1016/S0378-1097(03)00243-X
Fuentes, F., Paredes-Gonzalez, X., and Kong, A.-N. T. (2015). Dietary Glucosinolates Sulforaphane, Phenethyl Isothiocyanate, Indole-3-Carbinol/3, 3’-Diindolylmethane: anti-oxidative stress/inflammation, Nrf 2, epigenetics/Epigenomics and Cancer Chemopreventive efficacy. Curr Pharmacol. Rep. 1, 179–196. doi: 10.1007/s40495-015-0017-y
Gao, J., Xi, B., Chen, K., Song, R., Qin, T., Xie, J., et al. (2019). The stress hormone norepinephrine increases the growth and virulence of Aeromonas hydrophila. Microbiology 8:e00664. doi: 10.1002/mbo3.664
Ge, Q., Yang, B., Liu, R., Jiang, D., Yu, H., Wu, M., et al. (2021). Antioxidant activity of Lactobacillus plantarum NJAU-01 in an animal model of aging. BMC Microbiol. 21:182. doi: 10.1186/s12866-021-02248-5
Gonzales, X. F., Castillo-Rojas, G., Castillo-Rodal, A. I., Tuomanen, E., and López-Vidal, Y. (2013). Catecholamine norepinephrine diminishes lung epithelial cell adhesion of Streptococcus pneumoniae by binding iron. Microbiology 159, 2333–2341. doi: 10.1099/mic.0.065607-0
Gupta, R., and Srivastava, S. (2014). Antifungal effect of antimicrobial peptides (AMPs LR14) derived from Lactobacillus plantarum strain LR/14 and their applications in prevention of grain spoilage. Food Microbiol. 42, 1–7. doi: 10.1016/j.fm.2014.02.005
Hegde, M., Wood, T. K., and Jayaraman, A. (2009). The neuroendocrine hormone norepinephrine increases Pseudomonas aeruginosa PA14 virulence through the las quorum-sensing pathway. Appl. Microbiol. Biotechnol. 84, 763–776. doi: 10.1007/s00253-009-2045-1
Hiller, C. C., Lucca, V., Carvalho, D., Borsoi, A., Borges, K. A., Furian, T. Q., et al. (2019). Influence of catecholamines on biofilm formation by Salmonella Enteritidis. Microb. Pathog. 130, 54–58. doi: 10.1016/j.micpath.2019.02.032
Hirano, T., Ishihara, K., and Hibi, M. (2000). Roles of STAT3 in mediating the cell growth, differentiation and survival signals relayed through the IL-6 family of cytokine receptors. Oncogene 19, 2548–2556. doi: 10.1038/sj.onc.1203551
Inaba, M., Matsuda, N., Banno, H., Jin, W., Wachino, J.-I., Yamada, K., et al. (2016). In vitro reduction of antibacterial activity of tigecycline against multidrug-resistant Acinetobacter baumannii with host stress hormone norepinephrine. Int. J. Antimicrob. Agents 48, 680–689. doi: 10.1016/j.ijantimicag.2016.09.022
Jentsch, H. F. R., März, D., and Krüger, M. (2013). The effects of stress hormones on growth of selected periodontitis related bacteria. Anaerobe 24, 49–54. doi: 10.1016/j.anaerobe.2013.09.001
Li, B., Evivie, S. E., Lu, J., Jiao, Y., Wang, C., Li, Z., et al. (2018). Lactobacillus helveticus KLDS1.8701 alleviates d-galactose-induced aging by regulating Nrf-2 and gut microbiota in mice. Food Funct. 9, 6586–6598. doi: 10.1039/C8FO01768A
Li, W., Lyte, M., Freestone, P. P., Ajmal, A., Colmer-Hamood, J. A., and Hamood, A. N. (2009). Norepinephrine represses the expression of tox a and the siderophore genes in Pseudomonas aeruginosa. FEMS Microbiol. Lett. 299, 100–109. doi: 10.1111/j.1574-6968.2009.01739.x
Li, S., Zhao, Y., Zhang, L., Zhang, X., Huang, L., Li, D., et al. (2012). Antioxidant activity of Lactobacillus plantarum strains isolated from traditional Chinese fermented foods. Food Chem. 135, 1914–1919. doi: 10.1016/j.foodchem.2012.06.048
Linninge, C., Xu, J., Bahl, M. I., Ahrné, S., and Molin, G. (2019). Lactobacillus fermentum and Lactobacillus plantarum increased gut microbiota diversity and functionality, and mitigated, in a mouse model. Benef. Microbes 10, 413–424. doi: 10.3920/BM2018.0074
Liu, H., Zhang, J., Zhang, S., Yang, F., Thacker, P. A., Zhang, G., et al. (2014). Oral administration of Lactobacillus fermentum I5007 favors intestinal development and alters the intestinal microbiota in formula-fed piglets. J. Agric. Food Chem. 62, 860–866. doi: 10.1021/jf403288r
Livak, K. J., and Schmittgen, T. D. (2001). Analysis of relative gene expression data using real-time quantitative PCR and the 2(-Delta Delta C (T)) method. Methods 25, 402–408. doi: 10.1006/meth.2001.1262
Long, X., Sun, F., Wang, Z., Liu, T., Gong, J., Kan, X., et al. (2021). CQPC08 protects rats from lead-induced oxidative damage by regulating the Keap 1/Nrf 2/ARE pathway. Food Funct. 12, 6029–6044. doi: 10.1039/D1FO00589H
Lymperopoulos, A., Rengo, G., Zincarelli, C., Soltys, S., and Koch, W. J. (2008). Modulation of adrenal catecholamine secretion by in vivo gene transfer and manipulation of G protein-coupled receptor kinase-2 activity. Mol. Ther. 16, 302–307. doi: 10.1038/sj.mt.6300371
Lyte, M. (2004). Microbial endocrinology and infectious disease in the 21st century. Trends Microbiol. 12, 14–20. doi: 10.1016/j.tim.2003.11.004
Lyte, M. (2016). Microbial endocrinology: an ongoing personal journey. Adv. Exp. Med. Biol. 874, 1–24. doi: 10.1007/978-3-319-20215-0_1
Lyte, M., and Ernst, S. (1992). Catecholamine induced growth of gram negative bacteria. Life Sci. 50, 203–212. doi: 10.1016/0024-3205(92)90273-R
Lyte, M., Freestone, P. P. E., Neal, C. P., Olson, B. A., Haigh, R. D., Bayston, R., et al. (2003). Stimulation of Staphylococcus epidermidis growth and biofilm formation by catecholamine inotropes. Lancet 361, 130–135. doi: 10.1016/S0140-6736(03)12231-3
Ma, Q. (2013). Role of nrf 2 in oxidative stress and toxicity. Annu. Rev. Pharmacol. Toxicol. 53, 401–426. doi: 10.1146/annurev-pharmtox-011112-140320
Mao, X., Gu, C., Hu, H., Tang, J., Chen, D., Yu, B., et al. (2016). Dietary Lactobacillus rhamnosus GG supplementation improves the mucosal barrier function in the intestine of weaned piglets challenged by porcine rotavirus. PLoS One 11:e0146312. doi: 10.1371/journal.pone.0146312
McCracken, B. A., Spurlock, M. E., Roos, M. A., Zuckermann, F. A., and Gaskins, H. R. (1999). Weaning anorexia may contribute to local inflammation in the piglet small intestine. J. Nutr. 129, 613–619. doi: 10.1093/jn/129.3.613
McCuddin, Z. P., Carlson, S. A., and Sharma, V. K. (2008). Experimental reproduction of bovine Salmonella encephalopathy using a norepinephrine-based stress model. Vet. J. 175, 82–88. doi: 10.1016/j.tvjl.2006.12.014
Methner, U., Rabsch, W., Reissbrodt, R., and Williams, P. H. (2008). Effect of norepinephrine on colonisation and systemic spread of Salmonella enterica in infected animals: role of catecholate siderophore precursors and degradation products. Int. J. Med. Microbiol. 298, 429–439. doi: 10.1016/j.ijmm.2007.07.013
Mietzner, T. A., and Morse, S. A. (1994). The role of iron-binding proteins in the survival of pathogenic bacteria. Annu. Rev. Nutr. 14, 471–493. doi: 10.1146/annurev.nu.14.070194.002351
Mu, Q., Zhang, H., Liao, X., Lin, K., Liu, H., Edwards, M. R., et al. (2017). Control of lupus nephritis by changes of gut microbiota. Microbiome 5:73. doi: 10.1186/s40168-017-0300-8
Nakano, M., Takahashi, A., Sakai, Y., Kawano, M., Harada, N., Mawatari, K., et al. (2007). Catecholamine-induced stimulation of growth in Vibrio species. Lett. Appl. Microbiol. 44, 649–653. doi: 10.1111/j.1472-765X.2007.02136.x
Navrátilova, P., Borkovcova, I., Stastkova, Z., Bednarova, I., and Vorlova, L. (2022). Effect of cephalosporin antibiotics on the activity of yoghurt cultures. Food Secur. 11:2751. doi: 10.3390/foods11182751
Nour, M. A., El-Hindawy, M. M., Abou-Kassem, D. E., Ashour, E. A., Abd El-Hack, M. E., Mahgoub, S., et al. (2021). Productive performance, fertility and hatchability, blood indices and gut microbial load in laying quails as affected by two types of probiotic bacteria. Saudi. J. Biol. Sci. 28, 6544–6555. doi: 10.1016/j.sjbs.2021.07.030
Pande, G. S. J., Suong, N. T., Bossier, P., and Defoirdt, T. (2014). The catecholamine stress hormones norepinephrine and dopamine increase the virulence of pathogenic Vibrio anguillarum and Vibrio campbellii. FEMS Microbiol. Ecol. 90, 761–769. doi: 10.1111/1574-6941.12432
Pullinger, G. D., Carnell, S. C., Sharaff, F. F., van Diemen, P. M., Dziva, F., Morgan, E., et al. (2010b). Norepinephrine augments Salmonella enterica-induced enteritis in a manner associated with increased net replication but independent of the putative adrenergic sensor kinases Qse C and Qse E. Infect. Immun. 78, 372–380. doi: 10.1128/IAI.01203-09
Pullinger, G. D., van Diemen, P. M., Carnell, S. C., Davies, H., Lyte, M., and Stevens, M. P. (2010a). 6-hydroxydopamine-mediated release of norepinephrine increases faecal excretion of Salmonella enterica serovar Typhimurium in pigs. Vet. Res. 41:68. doi: 10.1051/vetres/2010040
Reiske, L., Schmucker, S. S., Steuber, J., Toulouse, C., Pfaffinger, B., and Stefanski, V. (2020). Interkingdom cross-talk in times of stress: Salmonella Typhimurium grown in the presence of Catecholamines inhibits porcine immune functionality in vitro. Front. Immunol. 11:572056. doi: 10.3389/fimmu.2020.572056
Ren, D., Zhu, J., Gong, S., Liu, H., and Yu, H. (2018). Antimicrobial characteristics of lactic acid Bacteria isolated from homemade fermented foods. Biomed. Res. Int. 2018, 1–9. doi: 10.1155/2018/5416725
Ritze, Y., Bárdos, G., Claus, A., Ehrmann, V., Bergheim, I., Schwiertz, A., et al. (2014). Lactobacillus rhamnosus GG protects against non-alcoholic fatty liver disease in mice. PLoS One 9:e80169. doi: 10.1371/journal.pone.0080169
Roberts, A., Matthews, J. B., Socransky, S. S., Freestone, P. P. E., Williams, P. H., and Chapple, I. L. C. (2002). Stress and the periodontal diseases: effects of catecholamines on the growth of periodontal bacteria in vitro. Oral Microbiol. Immunol. 17, 296–303. doi: 10.1034/j.1399-302X.2002.170506.x
Rose-John, S. (2012). IL-6 trans-signaling via the soluble IL-6 receptor: importance for the pro-inflammatory activities of IL-6. Int. J. Biol. Sci. 8, 1237–1247. doi: 10.7150/ijbs.4989
Round, J. L., and Mazmanian, S. K. (2009). The gut microbiota shapes intestinal immune responses during health and disease. Nat. Rev. Immunol. 9, 313–323. doi: 10.1038/nri2515
Sandrini, S., Alghofaili, F., Freestone, P., and Yesilkaya, H. (2014). Host stress hormone norepinephrine stimulates pneumococcal growth, biofilm formation and virulence gene expression. BMC Microbiol. 14:180. doi: 10.1186/1471-2180-14-180
Scardaci, R., Varese, F., Manfredi, M., Marengo, E., Mazzoli, R., and Pessione, E. (2021). Enterococcus faecium NCIMB10415 responds to norepinephrine by altering protein profiles and phenotypic characters. J. Proteome 231:104003. doi: 10.1016/j.jprot.2020.104003
Shi, Y., Cui, X., Gu, S., Yan, X., Li, R., Xia, S., et al. (2019). Antioxidative and probiotic activities of lactic acid Bacteria isolated from traditional artisanal Milk cheese from Northeast China. Probiotics Antimicrob. Proteins 11, 1086–1099. doi: 10.1007/s12602-018-9452-5
Shinde, T., Perera, A. P., Vemuri, R., Gondalia, S. V., Karpe, A. V., Beale, D. J., et al. (2019). Synbiotic supplementation containing whole plant sugar cane fibre and probiotic spores potentiates protective synergistic effects in mouse model of IBD. Nutrients 11:818. doi: 10.3390/nu11040818
Silver, J. S., and Hunter, C. A. (2010). Gp 130 at the nexus of inflammation, autoimmunity, and cancer. J. Leukoc. Biol. 88, 1145–1156. doi: 10.1189/jlb.0410217
Tonelli, C., Chio, I. I. C., and Tuveson, D. A. (2018). Transcriptional regulation by Nrf 2. Antioxid. Redox Signal. 29, 1727–1745. doi: 10.1089/ars.2017.7342
Torabi Delshad, S., Soltanian, S., Sharifiyazdi, H., and Bossier, P. (2019). Effect of catecholamine stress hormones (dopamine and norepinephrine) on growth, swimming motility, biofilm formation and virulence factors of Yersinia ruckeri in vitro and an in vivo evaluation in rainbow trout. J. Fish Dis. 42, 477–487. doi: 10.1111/jfd.12934
Toulouse, C., Schmucker, S., Metesch, K., Pfannstiel, J., Michel, B., Starke, I., et al. (2019). Mechanism and impact of catecholamine conversion by Vibrio cholerae. Biochim. Biophys. Acta Bioenerg. 1860, 478–487. doi: 10.1016/j.bbabio.2019.04.003
Trajković, S., Dobrić, S., Jaćević, V., Dragojević-Simić, V., Milovanović, Z., and Dordević, A. (2007). Tissue-protective effects of fullerenol C60 (OH)24 and amifostine in irradiated rats. Colloids Surf. B Biointerfaces 58, 39–43. doi: 10.1016/j.colsurfb.2007.01.005
Vardam, T. D., Zhou, L., Appenheimer, M. M., Chen, Q., Wang, W.-C., Baumann, H., et al. (2007). Regulation of a lymphocyte-endothelial-IL-6 trans-signaling axis by fever-range thermal stress: hot spot of immune surveillance. Cytokine 39, 84–96. doi: 10.1016/j.cyto.2007.07.184
Vinasco, K., Mitchell, H. M., Kaakoush, N. O., and Castaño-Rodríguez, N. (2019). Microbial carcinogenesis: lactic acid bacteria in gastric cancer. Biochim. Biophys. Acta Rev. Cancer 1872:188309. doi: 10.1016/j.bbcan.2019.07.004
Vlisidou, I., Lyte, M., van Diemen, P. M., Hawes, P., Monaghan, P., Wallis, T. S., et al. (2004). The neuroendocrine stress hormone norepinephrine augments Escherichia coli O157: H7-induced enteritis and adherence in a bovine ligated ileal loop model of infection. Infect. Immun. 72, 5446–5451. doi: 10.1128/IAI.72.9.5446-5451.2004
Webster Marketon, J. I., and Glaser, R. (2008). Stress hormones and immune function. Cell. Immunol. 252, 16–26. doi: 10.1016/j.cellimm.2007.09.006
Williams, P. H., Rabsch, W., Methner, U., Voigt, W., Tschäpe, H., and Reissbrodt, R. (2006). Catecholate receptor proteins in Salmonella enterica: role in virulence and implications for vaccine development. Vaccine 24, 3840–3844. doi: 10.1016/j.vaccine.2005.07.020
Yang, Q., Anh, N. D. Q., Bossier, P., and Defoirdt, T. (2014). Norepinephrine and dopamine increase motility, biofilm formation, and virulence of Vibrio harveyi. Front. Microbiol. 5:584. doi: 10.3389/fmicb.2014.00584
Keywords: Levilactobacillus, catecholamine hormone, antimicrobial activity, probiotic effects, microbial endocrinology
Citation: Niu L, Gao M, Li Y, Wang C, Zhang C, Duan H, Li H, Wang F and Ge J (2025) Effects of the stress hormone norepinephrine on the probiotic properties of Levilactobacillus: antibacterial colonization, anti-inflammation, and antioxidation. Front. Microbiol. 16:1526362. doi: 10.3389/fmicb.2025.1526362
Received: 12 November 2024; Accepted: 16 January 2025;
Published: 10 February 2025.
Edited by:
Yi Xu, Hefei University of Technology, ChinaReviewed by:
Atte Johannes Von Wright, University of Eastern Finland, FinlandCopyright © 2025 Niu, Gao, Li, Wang, Zhang, Duan, Li, Wang and Ge. This is an open-access article distributed under the terms of the Creative Commons Attribution License (CC BY). The use, distribution or reproduction in other forums is permitted, provided the original author(s) and the copyright owner(s) are credited and that the original publication in this journal is cited, in accordance with accepted academic practice. No use, distribution or reproduction is permitted which does not comply with these terms.
*Correspondence: Fang Wang, d2FuZ2ZhbmcwNkBjYWFzLmNu; Junwei Ge, Z2VqdW53ZWlAbmVhdS5lZHUuY24=
†These authors have contributed equally to this work
Disclaimer: All claims expressed in this article are solely those of the authors and do not necessarily represent those of their affiliated organizations, or those of the publisher, the editors and the reviewers. Any product that may be evaluated in this article or claim that may be made by its manufacturer is not guaranteed or endorsed by the publisher.
Research integrity at Frontiers
Learn more about the work of our research integrity team to safeguard the quality of each article we publish.