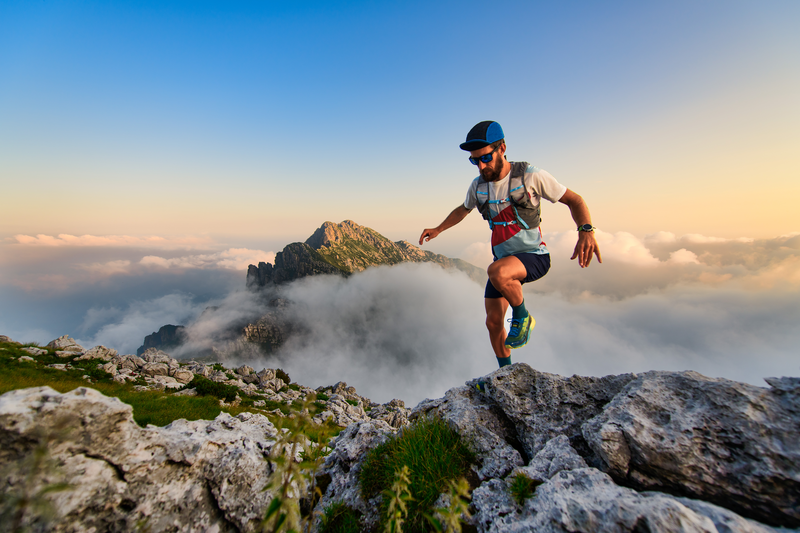
94% of researchers rate our articles as excellent or good
Learn more about the work of our research integrity team to safeguard the quality of each article we publish.
Find out more
ORIGINAL RESEARCH article
Front. Microbiol. , 24 February 2025
Sec. Microorganisms in Vertebrate Digestive Systems
Volume 16 - 2025 | https://doi.org/10.3389/fmicb.2025.1522191
This article is part of the Research Topic Probiotics for Global Health: Advances, Applications and Challenges View all 23 articles
The global prevalence of allergic rhinitis (AR) remains high, posing challenges due to its chronic nature and propensity for recurrence. Gut microbiota dysbiosis contributes to immune dysregulation, impacting AR pathogenesis. Limosilactobacillus reuteri (L. reuteri) has great potential in regulating immune function to alleviate AR symptoms. However, the specific active components and mechanisms underlying its therapeutic effects in AR remain incompletely clarified. This study aimed to explore the potential mechanisms of L. reuteri and its metabolites in alleviating AR. The AR mouse model was constructed using ovalbumin (OVA). The analysis of hematoxylin–eosin staining (HE staining) and enzyme-linked immunosorbent assay (ELISA) suggested that L. reuteri alleviated nasal inflammation, suppressed aberrant Th2 immune responses, and modulated the balance of Treg and Th17 cytokines. The 16S rRNA sequencing and untargeted metabolic analysis revealed that L. reuteri restored gut microbiota composition and significantly increased the abundance of Ligilactobacillus and the metabolite luteolin (LO). Through ELISA and Western blotting analysis, LO treatment restored the Th1/Th2 and Treg/Th17 cytokine balance and suppressed the MAPK/STAT3 signaling pathway in AR mice. The study highlights LO as a key metabolite contributing to the anti-inflammatory effects of L. reuteri, suggesting potential avenues for future therapeutic strategies in AR management.
Allergic rhinitis (AR) is a common chronic inflammatory condition affecting the upper respiratory tract (Bousquet et al., 2020). Over the past few decades, significant changes in environmental exposures and lifestyles have contributed to the increasing prevalence of AR, thereby adversely affecting individuals’ quality of life. It is estimated that approximately 500 million individuals worldwide are affected by AR (Ozdoganoglu and Songu, 2012). The AR prevalence in Asia ranges approximately 5–35%, depending on the method of diagnosis. In Europe, the most recent estimates put AR prevalence at around 25% (Wise et al., 2023). AR is classified as a type I hypersensitivity reaction, triggered by exposure to allergens in susceptible individuals (Bernstein et al., 2016). The production of immunoglobulin E (IgE) and the polarization of T helper type 2 (Th2) cell responses are key pathogenic factors in AR (Okubo et al., 2020). Clinical symptoms typically manifest as episodic and intermittent attacks, which may occur seasonally or persist throughout the year, and commonly include sneezing, rhinorrhea, nasal pruritus, and nasal congestion (Schuler and Montejo, 2019; Sur and Plesa, 2015). AR primarily arises from the secretion of IL-4, IL-5, and IL-13 by Th2 cells, which affects the balance between T helper type 1 (Th1) and Th2 cells, thereby triggering IgE production (Abdel-Gadir et al., 2019). Conversely, Th1 cells mitigate AR symptoms by secreting anti-inflammatory agents such as IFN-γ, which suppress the Th2 immune response (Takahashi et al., 2006).
Currently, intranasal corticosteroids are the most effective treatment (Sur and Plesa, 2015). Prolonged use of these medications can lead to side effects such as headache, nasal bleeding, and dryness, which not only diminish quality of life (QOL) but also increase health risks (Liang et al., 2020). The high costs and adverse effects of these drugs are significant. Thus, developing more affordable drugs or treatments with fewer side effects is crucial for helping patients to manage their illnesses and improve their lives.
The intestinal microbiota refers to microorganisms residing in the intestinal tract, where beneficial species such as Bifidobacterium and Lactobacillus contribute positively to human health. The intestinal microbiota acts as a crucial signaling center, integrating environmental stimuli with genetic and immune signals to significantly influence host metabolism, immunity, and resistance to infection (Thaiss et al., 2016). Lactobacillus, one of the earliest discovered probiotics, has been widely used in various foods and dietary supplements (Gangaiah et al., 2022). Studies have shown that Lactobacillus can restore the intestinal microenvironment and effectively regulate immune homeostasis (Zhou et al., 2020). A study found that administering Lactobacillus paracasei (LP-33) for 6 weeks to children with perennial allergic rhinitis was similarly effective to cetirizine, with nearly all children experiencing significant symptom improvement (Ahmed et al., 2019). Lactobacillus plantarum GUANKE effectively alleviated AR symptoms by maintaining a balanced ratio of Th1 and Th2 immune cells and regulating chemokine production (Han et al., 2023). Limosilactobacillus reuteri (L. reuteri) is one of the most extensively studied probiotic strains (Rao et al., 2021). L. reuteri CCFM1072 and CCFM1040 were found to reduce airway inflammation in mice with allergic asthma, suppress Th2 and Th17 immune responses, promote Treg response, and enhance gut microbiota diversity and metabolism (Li et al., 2022). Another study showed that L. reuteri significantly reduced serum concentrations of IgE and Th2 cytokines while increasing the production of the important microbial metabolite butyric acid by remodeling the intestinal microbial structure (Chen et al., 2018).
However, the precise metabolites and underlying mechanisms through which L. reuteri exerts its ameliorative effects on AR remain elusive. The exploration of gut microbiome-metabolite interactions could shed light on the connections between the gut and human health, such as mental health, autoimmune disorders, and chronic inflammation. Based on gut microbiome-metabolite interactions, this study studied the effects of L. reuteri and its metabolite luteolin (LO) on allergic rhinitis. This study aims to delve deeper into how L. reuteri regulates gut microbiota to modulate immune responses and how associated metabolites contribute to its therapeutic effects on AR. We confirmed that L. reuteri alleviates nasal inflammation symptoms, suppresses aberrant Th2 immune responses, balances Treg/Th17 cytokines, and restores gut microbiota composition in AR mice through ELISA and 16S rRNA sequencing analysis. Additionally, untargeted metabolic analysis revealed that L. reuteri increases the metabolite luteolin, further enhancing its role in AR.
The L. reuteri strain was obtained from China Center for Type Culture Collection (CCTCC). The strain was cultured in deMan Rogosa Sharpe (MRS) broth at 37°C under anaerobic conditions for 12 to 16 h.
Specific-pathogen-free (SPF) BALB/c female mice (6 weeks old, 16–20 g, SiPeiFu Biotechnology Company, Suzhou, China) were housed in an environmentally controlled animal facility. Mice were maintained on a 12-h light/12-h dark cycle to simulate natural circadian rhythms and preserve physiological homeostasis. The temperature indoors ranged from 20 to 26°C, with humidity maintained between 40 and 70% to prevent environmental stress. This animal study protocol was approved by the Ethics Committee of Huadong Medical Institute of Biotechniques (No.2024NKY012).
The AR mice model was established through ovalbumin (OVA) induction. After a week adaptation period, mice were randomly assigned to three groups (n = 6): the negative control (NC) group, the OVA group, and the L. reuteri (LR) group. In brief, OVA-induced mice were sensitized via intraperitoneal injection with a mixture of 100 μg ovalbumin (OVA; Sigma, St. Louis, MO) and 4 mg aluminum hydroxide (Solarbio, Beijing, China) in 200 μL PBS on days 1, 3, 5, 7, 9, 11, and 13. Subsequently, mice were intranasally challenged with 10% OVA (10 μL per nasal cavity) daily from day 15 to 21. During OVA induction, mice in the LR group received daily oral administration of 0.2 mL L. reuteri suspension containing 1 × 109 colony forming units for 3 weeks. The NC group were gavaged with PBS all the time. After the final OVA challenge, nasal sneezing and nose scratching frequency were recorded for 10 min, concurrent with fecal sample collection.
Mice were randomly assigned to five groups (n = 8): the NC group, the OVA group, and three experimental groups receiving different doses of luteolin (5-LO: 5 mg/kg, 10-LO: 10 mg/kg, 20-LO: 20 mg/kg). To induce an allergic response, mice were intraperitoneally injected with a solution containing 100 μg ovalbumin and 4 mg aluminum hydroxide on days 1, 3, 5, 7, 9, 11, and 13. From day 15 to day 28, mice underwent intranasal stimulation with 10% OVA (Dong et al., 2021). During intranasal OVA stimulation, mice in the LO group was administered intraperitoneally luteolin (Merced, California, United States), dissolved in 0.2 mL normal saline, once daily from day 15 to 28. After the final OVA challenge, nose-scratching frequency was observed and recorded for 10 min.
After sacrificing the mice, nasal mucosal tissues were fixed in 4% paraformaldehyde (Beyotime, Shanghai, China) to preserve morphology. The fixed tissues underwent ethanol dehydration and paraffin embedding. Paraffin blocks were sectioned into 5 μm thickness using a Minux S700A automatic rotary slicer (RWD Life Sciences, Shenzhen, China). Sections were dewaxed in xylene, rehydrated in ethanol, and stained with hematoxylin (Biyuntian, Shanghai, China) for 20–30 s, followed by a distilled water rinse. Excess stain was removed with hydrochloric acid ethanol for 5 s (Pician, Shanghai, China), and sections were briefly treated with ammonia for 5 s (Solarbio, Beijing, China) to enhance nuclear clarity. Following eosin staining (20 s), sections were dehydrated, cleared with xylene, and examined under a CX23 microscope at 400x magnification (Olympus, Tokyo, Japan). Microscopic analysis enabled the observation of cell morphology, structure, and nasal mucosal lesions.
ELISA was performed to quantify OVA-sIgE in serum, along with IL-4, IL-5, IL-13, IFN-γ, IL-17, IL-10, RORγt, and Foxp3 levels in both nasal lavage fluid (NALF) and serum samples (Lapuda, Nanjing, China). Samples were preprocessed for uniformity and stability before being adding to 96-well plates coated with specific antibodies. Biotinlabeled secondary antibodies were applied, followed by further incubation and washing steps. A streptavidin-HRP conjugate was added to bind biotin and introduce HRP enzymatic activity. Addition of a chromogenic substrate initiated an HRP-catalyzed oxidation reaction, generating colored products whose absorbance at 450 nm was measured using a microplate reader (Promega, Madison, WI). Antigen concentrations were determined from a standard curve.
Fecal samples were collected and subjected to DNA extraction using a Fast DNA Spin Kit (MP Biomedicals, Santa Ana, CA, United States). The hypervariable region V3-V4 of the bacterial 16S rRNA gene were amplified with primer pairs 338F: 5′- ACTCCTAC GGGAGGCAGCA-3′ and 806R: 5′- GGACTACHVGGGTWTCT AAT-3′. PCR products were checked on agarose gel and purified through the Omega DNA purification kit (Omega Inc., Norcross, GA, United States). The purified PCR products were collected and the paired ends (2 × 250 bp) was performed on the Illumina Novaseq 6,000 platform (Fang et al., 2022). Identification and removal of primer sequences was process by Cutadapt (Bolger et al., 2014). PE reads obtained from previous steps were assembled by USEARCH and followed by chimera removal using UCHIME. The high-quality reads generated from above steps were used in the following analysis. Sequences with similarity >97% were clustered into the same operational taxonomic unit (OTU) by USEARCH and the OTUs conuts less than 2 in all samples were filtered (Edgar, 2013). Clean reads then were conducted on feature classification to output an ASVs (amplicon sequence variants) by dada2, and the ASVs counts less than 2 in all samples were filtered. Taxonomy annotation of the OTUs was performed based on the Naive Bayes classifier in QIIME2 using the SILVA database with a confidence threshold of 70% (Quast et al., 2013). The Alpha diversity was calculated and displayed by the QIIME2 and R software, respectively. Beta diversity was determined to evaluate the degree of similarity of microbial communities from different samples using QIIME. Principal coordinate analysis (PCoA) and heatmaps were used to analyze the beta diversity. Furthermore, we employed linear discriminant analysis effect size (LEfSe) to test the significant taxonomic difference among group. A logarithmic LDA score of 4.0 was set as the threshold for discriminative features (Segata et al., 2011).
This study employed untargeted metabolic analysis using LC/MS (Mir et al., 2015), which included a Waters Acquity I-Class PLUS liquid chromatograph coupled with a Waters Xevo G2-XS QTOF mass spectrometer. A Waters Acquity UPLC HSS T3 column (1.8 μm, 2.1 × 100 mm) was utilized, with mobile phase A composed of 0.1% formic acid in aqueous solution and mobile phase B composed of 0.1% formic acid in acetonitrile, for both positive and negative ion modes. Data acquisition was performed in MSe mode on the Waters Xevo G2-XS QTOF mass spectrometer controlled by MassLynx V4.2 software. Compound classification and pathway information were obtained from databases such as KEGG, HMDB, and lipid maps. Differential analysis was conducted using grouping information, followed by T-test analysis to determine compound significance (p-value).
For targeted metabolism investigation of flavones in supernatants from L. reuteri cultures, metabolites were extracted and analyzed using a Waters ACQUITY I-Class ultra-performance liquid chromatograph equipped with an ACQUITY UPLC HSS T3 column (100 mm × 2.1 mm, 1.8 μm particle size, Waters). Prior to UHPLC–MS/MS analysis, standard solutions of target compounds were introduced (Liu et al., 2013). Multiple parent ion-daughter ion pairs were optimized for MRM parameters, selecting the most intense ion pair for quantitative analysis and others for qualitative analysis of target compounds.
WB was used to assess p-STAT3 (1:1000, ab68153), and STAT3 (1:1000, ab267373), and MAPK signaling pathway-associated proteins including p38 (1:1000, #9212), p-p38 (1:1000, #4511). Nasal mucosa samples were weighed and homogenized, and proteins were extracted using a total protein extraction kit (Beyotime, Shanghai, China). Protein concentration was determined using the BCA assay (Vazyme, Nanjing, China). The samples were then loaded into a 10% SDS-PAGE gel for separation and subsequently transferred to a polyvinylidene fluoride (PVDF) membrane. The membranes were blocked with 5% skim milk at room temperature for 1 h, then incubated. Primary antibodies, diluted in TBST at recommended concentrations, were incubated with the membranes overnight at 4°C. After washing with TBST, the membranes were incubated with secondary antibodies at room temperature for 1 h. Protein bands were visualized using a detection reagent (Thermo Fisher Scientific, Waltham, MA, United States), and Image J software was used to quantify band intensity.
In this study, measurement data described as means ± standard deviation underwent statistical analyses using GraphPad Prism 9.0 (GraphPad Software, La Jolla, CA). For the comparison of two sets of data, an independent sample t-test was used; whereas for the comparison between multiple groups, a one-way analysis of variance (one-way ANOVA) was performed. In all statistical analyses, a p-value less than 0.05 was used as the criterion for statistically significant differences. * p < 0.05, ** p < 0.01, *** p < 0.001, and **** p < 0.0001.
To assess the impact of L. reuteri strains on the pathological manifestations of AR, a model was established through the induction of OVA. The experimental design was depicted in Figure 1A. The OVA group exhibited significant rhinitis symptoms characterized by frequent nasal scratching and sneezing compared to the NC group. However, these symptoms were reduced in the LR group (Figures 1B,C). Compared to the NC group, the OVA-sIgE serum level in the OVA group exhibited a statistically significant elevation (p < 0.0001). Notably, in the LR group, a marked decrease in the OVA-sIgE serum level was observed (Figures 1D, p < 0.0001). Moreover, in order to assess pathological changes in mice, the nasal mucosal tissues were subjected to HE staining. The OVA treatment resulted in a significant inflammatory cell infiltration in the nasal cavity of mice, compared to the NC group. L. reuteri strain treatments reduced inflammatory cell infiltration and tissue edema compared to the OVA group (Figure 1E).
Figure 1. L. reuteri alleviated pathological symptoms in OVA-induced AR mice. (A) The experimental design of OVA-induced AR mice with dietary supplement of L. reuteri. (B,C) The frequency of nasal scratching and sneezing. (D) The serum level of OVA-sIgE was measured through ELISA. (E) HE staining showed the inflammatory cell infiltration of the nasal mucosal tissue (magnification: ×400). Data were presented as means ± SD. Results were analyzed by one-way ANOVA, * p < 0.05, ** p < 0.01, *** p < 0.001, **** p < 0.0001. n = 6 for each group. NC, negative control; OVA, ovalbumin; LR, L. reuteri; AR, allergic rhinitis; ELISA, enzyme-linked immunosorbent assay; HE, hematoxylin–eosin.
To assess the immunomodulatory impact of L. reuteri administration in mice, we evaluated whether the treatment with L. reuteri modulates the Th1/Th2 and Treg/Th17 immune responses in the AR mice. Obviously, the OVA group exhibited significantly elevated levels of IL-4, IL-5, IL-13 (indicators of Th2 cell activity) in both serum and NALF compared to the NC group (p < 0.0001). In the LR group, these cytokine levels were significantly reduced (Figures 2A–F). In the OVA group, the level of IFN-γ (an indicator of Th1 cell activity) was significantly reduced both in the serum and NALF compared to the NC group, but was not significantly increased in the LR group (Figures 2G,H). The level of IL-17 (an indicator of Th17 cell activity) was significantly increased both in the serum and NALF in the OVA group compared to the NC group and significantly decreased in the serum in the LR group (Figures 2I,J). As depicted in Figures 2K,L, the level of IL-10 (an indicator of Treg cell activity) in the OVA group was significantly decreased compared to the NC group (p < 0.01). Furthermore, a marked elevation of IL-10 was observed in the LR group, reaching a highly significant level (p < 0.0001).
Figure 2. L. reuteri balanced Th1/Th2 and Treg/Th17 cytokine levels in OVA-induced AR mice. (A–F) The levels of IL-4, IL-5, IL-13 in both serum and NALF. (G,H) The level of IFN-γ was measured through ELISA. (I,J) The level of IL-17 was significantly decreased in the LR group. (K,L) The level of IL-10 was significantly increased in the LR group. Data were presented as means±SD. Results were analyzed by one-way ANOVA; ns: not significant, **p < 0.01; ***p < 0.001; ****p < 0.0001. NC, negative control; OVA, ovalbumin; LR, L. reuteri; AR, allergic rhinitis; NALF, nasal lavage fluid; ELISA, enzyme-linked immunosorbent assay.
To explore the differences of gut microbiota among the NC, OVA, and LR groups, a comprehensive analysis was conducted using 16S rRNA sequencing analysis on mouse fecal samples. The alpha diversity was assessed using the Shannon index to evaluate species diversity within each group. Compared to the NC group, the Shannon index was reduced in the OVA group. However, there was a notable increase in the Shannon index in the LR group (Figure 3A). The beta diversity analysis was employed to compare the differences in microbial community composition and structure. The PCoA revealed significant differences in microbial community composition between the NC and OVA groups. Additionally, microbial composition also varied between the OVA and LR groups (Figure 3B,). Figure 3C showed the composition of species abundance between the groups at the genus level. The LefSe further evaluated the differences in species abundance between groups to identify statistically significant biomarkers. We identified differential bacteria, such as Ligilactobacillus, Blautia, Parabacteroides, and Akkermansia (Figure 3D). Furthermore, the correlation heatmap was created to assess the relationship between different bacterial genera and changes in Th1 and Th2 cytokine levels between the OVA and LR groups. We found that Prevotellaceae_UCG_001 and Odoribacter were positively correlated with IL-4, IL-5, IL-13 cytokines, whereas a positive correlation between the Ligilactobacillus and IFN-γ cytokines (Figure 3E). The analysis of the relative abundance of individual bacterial genera revealed that supplementation with L. reuteri significantly increased the abundance of Ligilactobacillus (Figures 3F, p < 0.01). This increased abundance of Ligilactobacillus in the LR group was strongly positively associated with Th1 cytokines. In contrast, the genus-level abundance of Prevotellaceae_UCG_001 and Odoribacter was decreased in the LR group (Figures 3G,H). The increased abundance of these bacteria in the OVA group was strongly positively associated with Th2 cytokines. However, the abundance of Akkermansia increased almost exclusively in the LR group (Figure 3I).
Figure 3. Gut microbiota changed and associated with immune response. (A) The Shannon index indicated the change of alpha diversity in OVA-induced AR mice. (B) PCoA analysis indicated the change of beta diversity in OVA-induced AR mice. (C) The composition of species abundance between the groups at the genus level. (D) The LefSe analysis assessed the species abundance composition differences between groups. (E) The correlation between different bacteria genera and Th1 and Th2 cytokine levels between the OVA and LR groups. (F–I) The genus-level abundance of change of Ligilactobacillus, Prevotellaceae_UCG_001, Odoribacter and Akkermansia. Data were presented as means ± SD. Results were analyzed by one-way ANOVA; ns, not significant, ** p < 0.01. NC, negative control; OVA, ovalbumin; LR, L. reuteri; AR, allergic rhinitis; PCoA, principal coordinates analysis; LEfSe, linear discriminant analysis effect size.
To further explore the effective substances of L. reuteri treatment for alleviating AR symptoms, we conducted an untargeted metabolic analysis on mouse fecal samples. The principal component analysis (PCA) was performed to assess the variation between different groups. As shown in Figure 4A, the metabolite profiles of the NC and OVA groups were significantly different, and there was also a notable variation between the OVA and LR groups. Through orthogonal projections to latent structures-discriminant analysis (OPLS-DA), the LR group was identified as a valid model for screening differential metabolites compared to the OVA group (Figure 4B). We created the hierarchical clustering heatmap of the differential metabolites and observed clear differences in flavonoid compounds between the OVA and LR groups, including luteolin, vaccarin, daidzein, pelargonidin, and senegenin (Figure 4C). The abundance of the differential metabolite luteolin between the LR and OVA groups was significantly increased in the LR group (Figures 4D, p < 0.001). Additionally, we performed a pure culture of L. reuteri and conducted flavonoids-targeted metabolic analysis on the supernatant of L. reuteri culture medium, revealing the production of a small amount of luteolin (Figure 4E).
Figure 4. L. reuteri increased production of metabolite luteolin in OVA-induced AR mice. (A) The PCA analysis indicated differences in metabolic composition among all groups. (B) The LR group was considered a valid model for screening differential metabolites compared to the OVA group. (C) The differential metabolites in the OVA and LR group, such as Luteolin. (D) The abundance of the differential metabolite luteolin. (E) A small amount of luteolin was produced in the L. reuteri supernatant. Data were presented as means ± SD. Results were analyzed by one-way ANOVA; ns, not significant, *** p < 0.001. NC, negative control; OVA, ovalbumin; LR, L. reuteri; AR, allergic rhinitis; PCA, principal component analysis.
Figure 5A illustrated the effects of LO treatment in a mouse model of AR. The mice in the OVA group exhibited a significantly higher frequency of nose scratching compared to the NC group. However, the frequency of nose scratching was reduced in the 10-LO and 20-LO groups (Figure 5B, p < 0.01). Additionally, mice exposed to OVA showed a significantly elevated serum level of OVA-sIgE in the OVA group. Luteolin treatment effectively ameliorated OVA-induced upregulation of OVA-sIgE, with the most pronounced reduction observed in the 20-LO group (Figure 5C, p < 0.0001). HE staining revealed that OVA treatment caused epithelial cell shedding and increased inflammatory cell infiltration in the nasal mucosa, effects which were mitigated by LO treatment (Figure 5D).
Figure 5. Luteolin improved pathological symptoms in OVA-induced AR mice. (A) The experimental design of OVA-induced AR mice with LO treatment. (B) The frequency of nasal scratching. (C) The serum level of OVA-sIgE was measured through ELISA. (D) HE staining showed the inflammatory cell infiltration of the nasal mucosal tissue (magnification: ×400). Data were presented as means ± SD. Results were analyzed by one-way ANOVA, ns, not significant, *p < 0.05; **p < 0.01; ***p < 0.001; ****p < 0.0001. n = 8 for each group. NC, negative control; OVA, ovalbumin; LO, luteolin; AR, allergic rhinitis; ELISA, enzyme-linked immunosorbent assay; HE, hematoxylin–eosin.
In the OVA group, we observed a significant increase in the concentrations of IL-4, IL-5, and IL-13 in both NALF and serum compared to the NC group (p < 0.0001). In contrast, the levels of these cytokines in the LO-5, LO-10, and LO-20 groups were markedly lower than those in the OVA group (Figures 6A–F). The serum level of IFN-γ was not significantly elevated in the LO-5 and LO-10 groups, but was significantly higher in the LO-20 group compared to the OVA group (Figure 6G). Additionally, the level of IFN-γ in NALF was significantly increased in the 10-LO and LO-20 groups (Figure 6H). Th2 cytokine levels decreased significantly with increasing doses of luteolin, whereas Th1 cytokines increased notably at higher doses. Notably, in the OVA group, IL-17 levels were significantly elevated in both serum and NALF compared to the NC group. This increase was significantly reduced in the LO-20 group (Figures 6I,J). Moreover, IL-10 levels in serum and NALF were significantly higher in the LO-20 group (Figures 6K,L).
Figure 6. Luteolin balanced Th1/Th2 and Treg/Th17 cell cytokine in OVA-induced AR mice. (A–F) The levels of IL-4, IL-5, IL-13 in both serum and NALF. (G,H) The level of IFN-γ in serum and NALF was significantly increased in the LO-20 group. (I,J) The level of IL-17 was significantly decreased in the LO-20 group. (K,L) The level of IL-10 was significantly increased in the LO-20 group. Data were presented as means ± SD. Results were analyzed by one-way ANOVA; ns, not significant, *p < 0.05; **p < 0.01; ***p < 0.001; ****p < 0.0001. NC, negative control; OVA, ovalbumin; LO, luteolin; AR, allergic rhinitis; NALF, Nasal Lavage Fluid.
RORγt is a major transcription factor for IL-17, while Foxp3 is a key marker of Treg cells. To determine if RORγt promotes Th17 polarization in AR mice, we measured its concentration in NALF by ELISA. In the OVA group, Foxp3 levels were significantly reduced in NALF, whereas they were increased in the 20-LO group (Figure 7A). RORγt levels in the OVA groups were notably higher compared to the NC group, and the increase was significantly reversed in the 20-LO group (Figure 7B). To further investigate the mechanisms, we assessed the expression of the Th17-related STAT3 signaling pathway by measuring p-STAT3 and STAT3 levels. The western blotting analysis revealed that p-STAT3 protein expression in the nasal mucosa was significantly higher in the OVA group compared to the NC group (Figure 7C). LO treatment effectively reduced p-STAT3 protein expression (Figures 7D,E). The p-STAT3/STAT3 ratios in the 20-LO group showed a significant decrease (Figure 7F). This suggests that the OVA-induced AR model presented an activated STAT3 signaling pathway. Next, we examined the inhibitory effects of luteolin on the MAPK signaling pathway activation. Western blot analysis was used to investigate protein expression within the MAPK signaling pathway, which is involved in the inflammatory response. Phosphorylation of p38 was significantly higher in the nasal mucosa of the OVA group compared to the NC group and was notably reduced in the 20-LO group (Figures 7G–I). Additionally, the p-p38/p38 ratios in the 20-LO group showed a significant decrease (Figure 7J).
Figure 7. Luteolin suppressed MAPK/STAT3 signaling pathway. (A) The Foxp3 levels were increased in NALF in the 20-LO group. (B) The NALF level of RORγt was measured through ELISA after LO treatment. (C–F) Western blot was used to examine the proteins (STAT3, p-STAT3) in the nasal mucosa of OVA-induced AR mice after LO treatment. GAPDH was the loading control. (G–I) Western blot was investigated protein expression along the MAPK signaling pathway (p38, p-p38). (J) The p-p38/p38 ratios in the 20-LO groups was decreased. Data were presented as means ± SD. Results were analyzed by one-way ANOVA; ns: not significant, *p < 0.05; **p < 0.01; ***p < 0.001; ****p < 0.0001. NC, negative control; OVA, ovalbumin; LO, luteolin; Foxp3, forkhead box P3; RORγt, retinoic acid receptor-related orphan nuclear receptor; NALF, Nasal Lavage Fluid; ELISA, enzyme-linked immunosorbent assay; AR, allergic rhinitis; GAPDH, glyceralde-hyde-3-phosphate dehydrogenase.
This study comprehensively demonstrates that treatment with L. reuteri effectively alleviates the symptoms of AR. It not only rectifies immune dysregulation but also modulates the imbalance of the intestinal microbiota. Notably, luteolin, a metabolite identified during the L. reuteri treatment process in AR mice, has been found to play a significant role. This highlights the importance of the gut - microbiota - immune axis in the pathogenesis of AR and the potential of microbiota - derived metabolites in treating allergic diseases.
Targeting the gut microbiota may serve as a promising preventive and therapeutic strategy for AR. The pathogenesis of AR remains highly complex and not fully understood. Although multiple studies have demonstrated the significant potential of L. reuteri in managing allergic disorders (Chen et al., 2018; Fang et al., 2022), the precise underlying mechanisms are yet to be fully elucidated.
Traditional drug treatment for AR, such as antihistamines and glucocorticoids, can alleviate symptoms, but there are different degrees of side effects, long-term use may also affect the immune balance of the body (Chen et al., 2020; Ren et al., 2017). Most previous studies focused on the pathogenesis of AR. For example, Mengze Ding et al. demonstrated Mahuang Fuzi Xixin decoction suppressed nasal epithelial pyroptosis by inhibiting the NLRP3/Caspase-1/GSDMD-N signaling pathway (Ding et al., 2024).
However, this study explores a new biological therapy path from the regulation of human microecology. This study integrates microbiome and metabolomics analysis, demonstrating that dietary supplementation with L. reuteri can modulate the immune response, reshape gut microflora composition, and increase the production of the metabolite luteolin, thereby alleviating allergic nasal inflammation and AR symptoms.
L. reuteri strains show promise in alleviating AR symptoms through immune modulation. Multiple studies have demonstrated a beneficial effect of L. reuteri on allergic diseases. In a study of multiple probiotics on asthma, L. reuteri was the most effective in reducing airway inflammation, total IgE and Th2-associated pro-inflammatory cytokines, and regulating specific microbial genera to prevent asthma (Choi et al., 2021). In an animal experiment, L. reuteri Fn041, isolated from breast milk, adjusted Th1/Th2 cytokine ratios, promoted Treg cell production, and altered gut microbiota by increasing the abundance of Lactobacillus and Akkermansia abundance in atopic dermatitis mice (Zhao et al., 2022). In our study, we found L. reuteri treatment enhanced gut microbiota diversity and increased the abundance of certain beneficial bacteria, such as Ligilactobacillus. Ligilactobacillus, a gastrointestinal lactobacillus and probiotic, which likely plays a role in L. reuteri-treated organisms. Additionally, we found the abundance of Akkermansia genus increased only in the LR group, consistent with previous reports.
While many studies have demonstrated the efficacy of probiotics in alleviating AR, few have investigated the specific metabolites involved in related mechanisms. Changes in specific microbiota can significantly impact gut microbiota metabolism. Lactobacillus metabolites are rich and diverse, including short-chain fatty acids, bactericins, polysaccharides, and so on (Liu et al., 2022). Each component may play a unique role in immune regulation. Thus, we conducted untargeted metabolic analysis of fecal samples from mice. L. reuteri treatment not only modulated the composition of intestinal microbiota but also increased the production of flavonoid compounds in the intestine, notably luteolin. In this study, we explored the potential medicinal value of luteolin, and compared with single-component drugs, the combination of L. reuteri metabolites may produce synergistic effects.
Luteolin, a flavonoid found in various plants such as vegetables, medicinal herbs, and fruits (Imran et al., 2019), has been shown to possess anti-allergic activity (Khan, 2014; Ueda et al., 2002). As a member of the flavonoid family, luteolin exhibits anti-inflammatory functions that may have clinical applications for patients with cancer, neurobehavioral disorders, or intestinal inflammation (Franza et al., 2021). Flavonoids interact with the gut microbiota by modulating its composition, thereby serving as precursors for the fermentation into additional bioactive compounds with anti-inflammatory properties (Loo et al., 2023).
LO treatment significantly reduced colon injury, inhibited the inflammatory response, and altered the structure and composition of the gut microbiota in ulcerative colitis rats (Li et al., 2021). Dong et al. (2021) discovered that LO effectively alleviated inflammation and restored the Th1/Th2 imbalance in AR rats by modulating the TLR4/NF-κB signaling pathway. In our study, Luteolin’s immunomodulatory properties may alleviate AR symptoms by balancing Th1/Th2 and Treg/Th17 cells. It can also modulate immune function by inhibiting the MAPK/STAT3 signaling pathway.
A study suggested that LO restored the balance between Treg and Th17 cells in a mouse model, where the levels of OVA-sIgE, IL-17A, and RORγt were elevated, while IL-10 and Foxp3 levels were reduced (Yang et al., 2023). A comprehensive review article explored the latest research on luteolin’s role as a natural immunomodulator, particularly focusing on its influence on inflammatory signaling mechanisms, including its impact on NF-κB, MAPK, and JAK/STAT pathways (Hussain et al., 2023). LO also exhibited a therapeutic effect on neutrophilic asthma by suppressing the secretion of IL-36γ, thereby modulating MAPK signaling pathways (Qiao et al., 2023). Network pharmacology showed that 251 luteolin against osteosarcoma targets and 8 hub targets including AKT1, ALB, CASP3, IL6, JUN, STAT3, TNF, and VEGFA (Huang et al., 2023).
The STAT3 signaling pathway is closely associated with the development of Th17 and Treg cells (Piao et al., 2020). Santana et al. (2019) showed sakuranetin inhibited of MAPK and STAT3-SOCS3 to attenuate chronic allergic airway inflammation in mice. Weixiong Chen et al. showed She-Chuang-Si-Wu-Tang alleviates inflammation and itching symptoms in a psoriasis mouse model by regulating the Th17/IL-17 axis via the STAT3/MAPK pathways (Chen et al., 2024). Our study demonstrated that LO treatment effectively reduced the p-p38/p38 and p-STAT3/STAT3 protein expression ratios. This suggests that microbially metabolized luteolin may influence immune function by balancing Th17 and Treg cells and regulating the MAPK/STAT3 signaling pathway.
The oral administration of L. reuteri can regulate the intestinal microecology, so that L. reuteri and its metabolites can indirectly act on the nasal mucosal immune system. The cross-organ regulation approach breaks the previous limitation of local treatment of nasal cavity, treats the whole body as a unified immune whole, and provides an innovative strategy for the treatment of complex diseases associated with multiple organs. L. reuteri can modulate the immune response, reshape gut microflora composition. We found that Prevotellaceae_UCG_001 and Odoribacter were positively correlated with IL-4, IL-5, IL-13 cytokines, whereas a positive correlation between the Ligilactobacillus and IFN-γ cytokines. The increase in specific microbial abundance, and the alteration of intestinal microflora structure or metabolism may be related to the Th2 response. The negative correlation between reduced microbial abundance and Th2 response warrants further investigation. This could provide more effective personalized medicine treatments for specific microbial imbalances or metabolic dysfunction.
The mechanisms by which gut microorganisms and their metabolites exert their effects need further exploration, which may reveal more effective therapeutic strategies through metabolite interactions. L. reuteri may activate signaling pathways by increasing luteolin expression, inhibiting the aberrant Th2-type immune response. Though direct evidence is lacking, luteolin’s properties provide a plausible mechanism. Luteolin’s immunomodulatory properties suggest a mechanism for alleviating AR symptoms by restoring the Th1/Th2 and Treg/Th17 cell balance. Moreover, luteolin has the potential to modulate immune function by inhibiting MAPK/STAT3 signaling pathway.
We will further delve into the pharmacological mechanisms of luteolin in the future, providing evidence that L. reuteri treatment and its flavonoid compounds may be effective alternative strategies for alleviating AR. Future research should aim to investigate various metabolites to comprehensively understand their combined interactions. Additionally, animal and clinical studies should further evaluate the effects of probiotics and their metabolites on intestinal microflora to provide a more holistic view. Moreover, the findings from this study highlight the potential of probiotics as a complementary or alternative treatment for allergies, warranting further investigations into their long-term safety and efficacy in human subjects.
The datasets presented in this study can be found in online repositories. The names of the repository/repositories and accession number(s) can be found in the article/supplementary material.
The animal study was approved by the Ethics Committee of Huadong Medical Institute of Biotechniques. The study was conducted in accordance with the local legislation and institutional requirements.
MZ: Data curation, Formal analysis, Investigation, Methodology, Validation, Writing – original draft, Writing – review & editing. XS: Methodology, Writing – original draft. XY: Data curation, Writing – review & editing. LX: Resources, Writing – review & editing. XZ: Investigation, Methodology, Writing – original draft. RZ: Investigation, Writing – original draft. HL: Software, Writing – original draft. YW: Data curation, Writing – original draft. FX: Resources, Writing – review & editing. TZ: Methodology, Writing – review & editing. CT: Methodology, Writing – original draft. ZW: Software, Writing – original draft. ZZ: Software, Writing – original draft. JZ: Resources, Writing – review & editing. QC: Data curation, Writing – review & editing. ZY: Conceptualization, Supervision, Visualization, Writing – review & editing. YC: Funding acquisition, Project administration, Supervision, Writing – review & editing.
The author(s) declare that financial support was received for the research, authorship, and/or publication of this article. The present study was supported by the China Postdoctoral Science Foundation (grant no. 2017 M613438) and by the Strengthening Health through Science and Education Program of Nanjing Commission of Health (no. SZDZK202002).
The authors declare that the research was conducted in the absence of any commercial or financial relationships that could be construed as a potential conflict of interest.
The authors declare that no Gen AI was used in the creation of this manuscript.
All claims expressed in this article are solely those of the authors and do not necessarily represent those of their affiliated organizations, or those of the publisher, the editors and the reviewers. Any product that may be evaluated in this article, or claim that may be made by its manufacturer, is not guaranteed or endorsed by the publisher.
Abdel-Gadir, A., Stephen-Victor, E., Gerber, G. K., Noval Rivas, M., Wang, S., Harb, H., et al. (2019). Microbiota therapy acts via a regulatory T cell MyD88/RORgammat pathway to suppress food allergy. Nat. Med. 25, 1164–1174. doi: 10.1038/s41591-019-0461-z
Ahmed, M., Billoo, A. G., and Iqbal, K. (2019). Efficacy of probiotic in perennial allergic rhinitis under five year children: A randomized controlled trial. Pak J Med Sci 35, 1538–1543. doi: 10.12669/pjms.35.6.744
Bernstein, D. I., Schwartz, G., and Bernstein, J. A. (2016). Allergic rhinitis: mechanisms and treatment. Immunol. Allergy Clin. N. Am. 36, 261–278. doi: 10.1016/j.iac.2015.12.004
Bolger, A. M., Lohse, M., and Usadel, B. (2014). Trimmomatic: a flexible trimmer for Illumina sequence data. Bioinformatics 30, 2114–2120. doi: 10.1093/bioinformatics/btu170
Bousquet, J., Anto, J. M., Bachert, C., Baiardini, I., Bosnic-Anticevich, S., Walter Canonica, G., et al. (2020). Allergic rhinitis. Nat Rev Dis Primers 6:95. doi: 10.1038/s41572-020-00227-0
Chen, F., He, D., and Yan, B. (2020). Apigenin attenuates allergic responses of ovalbumin-induced allergic rhinitis through modulation of Th1/Th2 responses in experimental mice. Dose Response 18:4799. doi: 10.1177/1559325820904799
Chen, W., Liang, J., He, S., Liang, Q., Tian, W., Lu, A., et al. (2024). She-Chuang-Si-Wu-Tang alleviates inflammation and itching symptoms in a psoriasis mouse model by regulating the Th17/IL-17 Axis via the STAT3/MAPK pathways. J. Inflamm. Res. 17, 5957–5975. doi: 10.2147/JIR.S472417
Chen, J. C., Tsai, C. C., Hsieh, C. C., Lan, A., Huang, C. C., and Leu, S. F. (2018). Multispecies probiotics combination prevents ovalbumin-induced airway hyperreactivity in mice. Allergol Immunopathol (Madr) 46, 354–360. doi: 10.1016/j.aller.2018.02.001
Choi, S., Jung, M. A., Hwang, Y. H., Pyun, B. J., Lee, J. Y., Jung, D. H., et al. (2021). Anti-allergic effects of Asarum heterotropoides on an ovalbumin-induced allergic rhinitis murine model. Biomed. Pharmacother. 141:111944. doi: 10.1016/j.biopha.2021.111944
Ding, M., Wei, X., Liu, C., and Tan, X. (2024). Mahuang Fuzi Xixin decoction alleviates allergic rhinitis by inhibiting NLRP3/Caspase-1/GSDMD-N-mediated pyroptosis. J. Ethnopharmacol. 327:118041. doi: 10.1016/j.jep.2024.118041
Dong, J., Xu, O., Wang, J., Shan, C., and Ren, X. (2021). Luteolin ameliorates inflammation and Th1/Th2 imbalance via regulating the TLR4/NF-kappaB pathway in allergic rhinitis rats. Immunopharmacol. Immunotoxicol. 43, 319–327. doi: 10.1080/08923973.2021.1905659
Edgar, R. C. (2013). UPARSE: highly accurate OTU sequences from microbial amplicon reads. Nat. Methods 10, 996–998. doi: 10.1038/nmeth.2604
Fang, Z., Pan, T., Wang, H., Zhu, J., Zhang, H., Zhao, J., et al. (2022). Limosilactobacillus reuteri attenuates atopic dermatitis via changes in gut Bacteria and indole derivatives from tryptophan metabolism. Int. J. Mol. Sci. 23:7735. doi: 10.3390/ijms23147735
Franza, L., Carusi, V., Nucera, E., and Pandolfi, F. (2021). Luteolin, inflammation and cancer: special emphasis on gut microbiota. Biofactors 47, 181–189. doi: 10.1002/biof.1710
Gangaiah, D., Mane, S. P., Tawari, N. R., Lakshmanan, N., Ryan, V., Volland, A., et al. (2022). In silico, in vitro and in vivo safety evaluation of Limosilactobacillus reuteri strains ATCC PTA-126787 & ATCC PTA-126788 for potential probiotic applications. PLoS One 17:e0262663. doi: 10.1371/journal.pone.0262663
Han, H., Chen, G., Zhang, B., Zhang, X., He, J., Du, W., et al. (2023). Probiotic Lactobacillus plantarum Guanke effectively alleviates allergic rhinitis symptoms by modulating functions of various cytokines and chemokines. Front. Nutr. 10:1291100. doi: 10.3389/fnut.2023.1291100
Huang, R., Xu, M., Guo, W., Cheng, M., Dong, R., Tu, J., et al. (2023). Network pharmacology and experimental verification-based strategy for exploring the mechanisms of luteolin in the treatment of osteosarcoma. Cancer Cell Int. 23:213. doi: 10.1186/s12935-023-03046-x
Hussain, M. S., Gupta, G., Goyal, A., Thapa, R., Almalki, W. H., Kazmi, I., et al. (2023). From nature to therapy: Luteolin’s potential as an immune system modulator in inflammatory disorders. J. Biochem. Mol. Toxicol. 37:e23482. doi: 10.1002/jbt.23482
Imran, M., Rauf, A., Abu-Izneid, T., Nadeem, M., Shariati, M. A., Khan, I. A., et al. (2019). Luteolin, a flavonoid, as an anticancer agent: A review. Biomed. Pharmacother. 112:108612. doi: 10.1016/j.biopha.2019.108612
Khan, D. A. (2014). Allergic rhinitis and asthma: epidemiology and common pathophysiology. Allergy Asthma Proc. 35, 357–361. doi: 10.2500/aap.2014.35.3794
Li, B., Du, P., Du, Y., Zhao, D., Cai, Y., Yang, Q., et al. (2021). Luteolin alleviates inflammation and modulates gut microbiota in ulcerative colitis rats. Life Sci. 269:119008. doi: 10.1016/j.lfs.2020.119008
Li, L., Fang, Z., Lee, Y. K., Zhao, J., Zhang, H., Peng, H., et al. (2022). Efficacy and safety of Lactobacillus reuteri CCFM1040 in allergic rhinitis and asthma: A randomized, placebo-controlled trial. Front Nutr 9:862934. doi: 10.3389/fnut.2022.862934
Liang, K. L., Yu, S. J., Huang, W. C., and Yen, H. R. (2020). Luteolin attenuates allergic nasal inflammation via inhibition of Interleukin-4 in an allergic rhinitis mouse model and peripheral blood from human subjects with allergic rhinitis. Front. Pharmacol. 11:291. doi: 10.3389/fphar.2020.00291
Liu, P., Hu, T., Kang, C., Liu, J., Zhang, J., Ran, H., et al. (2022). Research advances in the treatment of allergic rhinitis by probiotics. J Asthma Allergy 15, 1413–1428. doi: 10.2147/JAA.S382978
Liu, R., Peng, Y., Li, X., Wang, Y., Pan, E., Guo, W., et al. (2013). Identification of plasma metabolomic profiling for diagnosis of esophageal squamous-cell carcinoma using an UPLC/TOF/MS platform. Int. J. Mol. Sci. 14, 8899–8911. doi: 10.3390/ijms14058899
Loo, Y. T., Howell, K., Suleria, H., Zhang, P., Liu, S., and Ng, K. (2023). Flavones interact with fiber to affect fecal bacterial communities in vitro. Food Chem. 404:134721. doi: 10.1016/j.foodchem.2022.134721
Mir, S. A., Rajagopalan, P., Jain, A. P., Khan, A. A., Datta, K. K., Mohan, S. V., et al. (2015). LC-MS-based serum metabolomic analysis reveals dysregulation of phosphatidylcholines in esophageal squamous cell carcinoma. J. Proteome 127, 96–102. doi: 10.1016/j.jprot.2015.05.013
Okubo, K., Kurono, Y., Ichimura, K., Enomoto, T., Okamoto, Y., Kawauchi, H., et al. (2020). Japanese guidelines for allergic rhinitis 2020. Allergol. Int. 69, 331–345. doi: 10.1016/j.alit.2020.04.001
Ozdoganoglu, T., and Songu, M. (2012). The burden of allergic rhinitis and asthma. Ther. Adv. Respir. Dis. 6, 11–23. doi: 10.1177/1753465811431975
Piao, C. H., Song, C. H., Lee, E. J., and Chai, O. H. (2020). Saikosaponin A ameliorates nasal inflammation by suppressing IL-6/ROR-gammat/STAT3/IL-17/NF-kappaB pathway in OVA-induced allergic rhinitis. Chem. Biol. Interact. 315:108874. doi: 10.1016/j.cbi.2019.108874
Qiao, X. R., Feng, T., Zhang, D., Zhi, L. L., Zhang, J. T., Liu, X. F., et al. (2023). Luteolin alleviated neutrophilic asthma by inhibiting IL-36gamma secretion-mediated MAPK pathways. Pharm. Biol. 61, 165–176. doi: 10.1080/13880209.2022.2160770
Quast, C., Pruesse, E., Yilmaz, P., Gerken, J., Schweer, T., Yarza, P., et al. (2013). The SILVA ribosomal RNA gene database project: improved data processing and web-based tools. Nucleic Acids Res. 41, D590–D596. doi: 10.1093/nar/gks1219
Rao, N. S., Lundberg, L., Palmkron, S., Hakansson, S., Bergenstahl, B., and Carlquist, M. (2021). Flow cytometric analysis reveals culture condition dependent variations in phenotypic heterogeneity of Limosilactobacillus reuteri. Sci. Rep. 11:23567. doi: 10.1038/s41598-021-02919-3
Ren, M., Tang, Q., Chen, F., Xing, X., Huang, Y., and Tan, X. (2017). Mahuang Fuzi Xixin decoction attenuates Th1 and Th2 responses in the treatment of ovalbumin-induced allergic inflammation in a rat model of allergic rhinitis. J Immunol Res 2017:8254324. doi: 10.1155/2017/8254324
Santana, F. P. R., Da Silva, R. C., Grecco, S. D. S., Pinheiro, A., Caperuto, L. C., Arantes-Costa, F. M., et al. (2019). Inhibition of MAPK and STAT3-SOCS3 by Sakuranetin attenuated chronic allergic airway inflammation in mice. Mediat. Inflamm. 2019:1356356. doi: 10.1155/2019/1356356
Schuler, C. F., and Montejo, J. M. (2019). Allergic rhinitis in children and adolescents. Pediatr. Clin. N. Am. 66, 981–993. doi: 10.1016/j.pcl.2019.06.004
Segata, N., Izard, J., Waldron, L., Gevers, D., Miropolsky, L., Garrett, W. S., et al. (2011). Metagenomic biomarker discovery and explanation. Genome Biol. 12:R60. doi: 10.1186/gb-2011-12-6-r60
Sur, D. K., and Plesa, M. L. (2015). Treatment of allergic rhinitis. Am. Fam. Physician 92, 985–992 Available at: https://www.aafp.org/afp/2015/1201/p985.html
Takahashi, N., Kitazawa, H., Iwabuchi, N., Xiao, J. Z., Miyaji, K., Iwatsuki, K., et al. (2006). Immunostimulatory oligodeoxynucleotide from Bifidobacterium longum suppresses Th2 immune responses in a murine model. Clin. Exp. Immunol. 145, 130–138. doi: 10.1111/j.1365-2249.2006.03111.x
Thaiss, C. A., Zmora, N., Levy, M., and Elinav, E. (2016). The microbiome and innate immunity. Nature 535, 65–74. doi: 10.1038/nature18847
Ueda, H., Yamazaki, C., and Yamazaki, M. (2002). Luteolin as an anti-inflammatory and anti-allergic constituent of Perilla frutescens. Biol. Pharm. Bull. 25, 1197–1202. doi: 10.1248/bpb.25.1197
Wise, S. K., Damask, C., Roland, L. T., Ebert, C., Levy, J. M., Lin, S., et al. (2023). International consensus statement on allergy and rhinology: allergic rhinitis - 2023. Int Forum Allergy Rhinol 13, 293–859. doi: 10.1002/alr.23090
Yang, Y., Wang, L., Wang, S., Wang, Y., Du, Y., and Fan, Y. (2023). Luteolin restored Treg/Th17 balance to ameliorate allergic rhinitis in a mouse model. Immunopharmacol. Immunotoxicol. 45, 461–468. doi: 10.1080/08923973.2023.2166527
Zhao, Y., Qi, C., Li, X., Lu, M., Zhang, H., Zhou, J., et al. (2022). Prevention of atopic dermatitis in mice by Lactobacillus reuteri Fn041 through induction of regulatory T cells and modulation of the gut microbiota. Mol. Nutr. Food Res. 66:e2100699. doi: 10.1002/mnfr.202100699
Keywords: allergic rhinitis, Limosilactobacillus reuteri, gut microbiota, metabolite, luteolin, immune regulation
Citation: Zhang M, Sun X, Yu X, Xu L, Zhang X, Zhang R, Lu H, Wang Y, Xue F, Zhang T, Tang C, Wu Z, Zhang Z, Zhu J, Cui Q, Yang Z and Cheng Y (2025) Limosilactobacillus reuteri regulates gut microbiota and increases the effective metabolite luteolin to inhibit MAPK/STAT3 signaling pathway to alleviate allergic rhinitis. Front. Microbiol. 16:1522191. doi: 10.3389/fmicb.2025.1522191
Received: 05 November 2024; Accepted: 11 February 2025;
Published: 24 February 2025.
Edited by:
Arun K. B., Christ University, IndiaReviewed by:
Zhangyong Song, Southwest Medical University, ChinaCopyright © 2025 Zhang, Sun, Yu, Xu, Zhang, Zhang, Lu, Wang, Xue, Zhang, Tang, Wu, Zhang, Zhu, Cui, Yang and Cheng. This is an open-access article distributed under the terms of the Creative Commons Attribution License (CC BY). The use, distribution or reproduction in other forums is permitted, provided the original author(s) and the copyright owner(s) are credited and that the original publication in this journal is cited, in accordance with accepted academic practice. No use, distribution or reproduction is permitted which does not comply with these terms.
*Correspondence: Qian Cui, cuiqianok1@163; Zhan Yang, breezezhan@126.com; You Cheng, chengyou3086@163.com
Disclaimer: All claims expressed in this article are solely those of the authors and do not necessarily represent those of their affiliated organizations, or those of the publisher, the editors and the reviewers. Any product that may be evaluated in this article or claim that may be made by its manufacturer is not guaranteed or endorsed by the publisher.
Research integrity at Frontiers
Learn more about the work of our research integrity team to safeguard the quality of each article we publish.