- Department of Biological Sciences, University of Southern California, Los Angeles, CA, United States
Underlying the thick sediment layer in ocean basins, the flow of seawater through the cracked and porous upper igneous crust supports a previously hidden and largely unexplored active subsurface microbial biome. Subseafloor crustal systems offer an enlarged surface area for microbial habitats and prolonged cell residence times, promoting the evolution of novel microbial lineages in the presence of steep physical and thermochemical gradients. The substantial metabolic potential and dispersal capabilities of microbial communities within these systems underscore their crucial role in biogeochemical cycling. However, the intricate interplay between fluid chemistry, temperature variations, and microbial activity remains poorly understood. These complexities introduce significant challenges in unraveling the factors that regulate microbial distribution and function within these dynamic ecosystems. Using synthesized data from previous studies, this work describes how the ocean crustal biosphere functions as a continuous-flow biogechemical reactor. It simultaneously promotes the breakdown of surface-derived organic carbon and the creation of new, chemosynthetic material, thereby enhancing element recycling and ocean carbon productivity. Insights gained from the qualitative analysis of the extent of biogeochemical microbial activity and diversity across the temperature and chemical gradients that characterize these habitats, as reviewed herein, challenge traditional models of global ocean carbon productivity and provide the development of a new conceptual framework for understanding the quantitative metabolic potential and broad dispersal of the crustal microbial biome.
Introduction
The biogeochemical role of microbes deeply buried beneath the seafloor is far more important than presumed possible 80 years ago (Zobell and Anderson, 1936; Zobell, 1938). Over the past decades, comprehensive studies of subseafloor sedimentary microbes have revealed not only cell abundances that match previous estimates in seawater and in surface sediments (Kallmeyer et al., 2012) but most importantly, have demonstrated the viability of these microbes (Morono et al., 2011; Trembath-Reichert et al., 2017; Imachi et al., 2019) and their essential role in operating and maintaining global biogeochemical cycles (Parkes et al., 2014). We now understand that beneath the sediment layer, fluids moving through the basaltic ocean crust hold a similar amount of organic carbon, stored within living prokaryotic biomass (~1.6 Gt C, Bar-On et al., 2018). The volume of the ocean crust biosphere represents nearly 2% of the volume of the oceans (Johnson and Pruis, 2003). Conditions along the active fluid flow paths that characterize this habitat indicate that the crustal biosphere is the most favorable of deep-subsurface habitats and is likely a very active site of element cycling (Johnson et al., 2006). Furthermore, this aquifer is hydrothermally active and interactions with the overlaying sediments and ocean seawater facilitate the free exchange of fluid, chemicals, biological material, and heat, which likely have a large impact on the variability of seawater chemical composition and global biogeochemical cycling (Edwards et al., 2011).
The upper crustal reservoir as a sub-surface microbial biosphere
Basaltic ocean crust is formed at the axis of spreading mid-ocean ridges (MORs, Figure 1). As new ocean floor is formed and moves away from the spreading center, it is cooled by the interaction with seawater. Aging crustal porewaters remain generally isolated within buried upper oceanic basement, subjected to increasing temperatures as plates move away from spreading ridges. The accumulation of overlaying sediments on the MOR flanks and ocean basins prevents continued advective heat loss and results in strong hydrothermal gradients, which drive the rapid—on the order of m/day (Neira et al., 2016)—and largely lateral flow of low temperature (~5–65°C) fluids. Local circulation patterns are largely controlled by differences in pressure between cool (recharging) bottom seawater and warm (discharging) crustal fluids occurring at permeable igneous outcrops that penetrate the thick sediment cover (Winslow and Fisher, 2015; Winslow et al., 2016; Lauer et al., 2018). In contrast to the diffusion-dominated overlying sediments, the advective flow of hydrothermal fluids within the basaltic crust provides a pathway for the fast transport of solutes and particles including microbial cells, carbon and nutrients and generates small-scale variability in conditions supporting crustal biomes (Edwards et al., 2012a). The microbial biosphere, presumably located within the uppermost part of the igneous crust (Heberling et al., 2010), has likely been present since microbes first inhabited the oceanic crust around 3.5 billion years ago (Furnes et al., 2004). Quantitative knowledge of the extent of its metabolic potential and contribution to active global biogeochemical cycling, however, remains largely speculative (Orcutt et al., 2011b), as direct access to uncontaminated fluids in old ocean crust remains a major challenge.
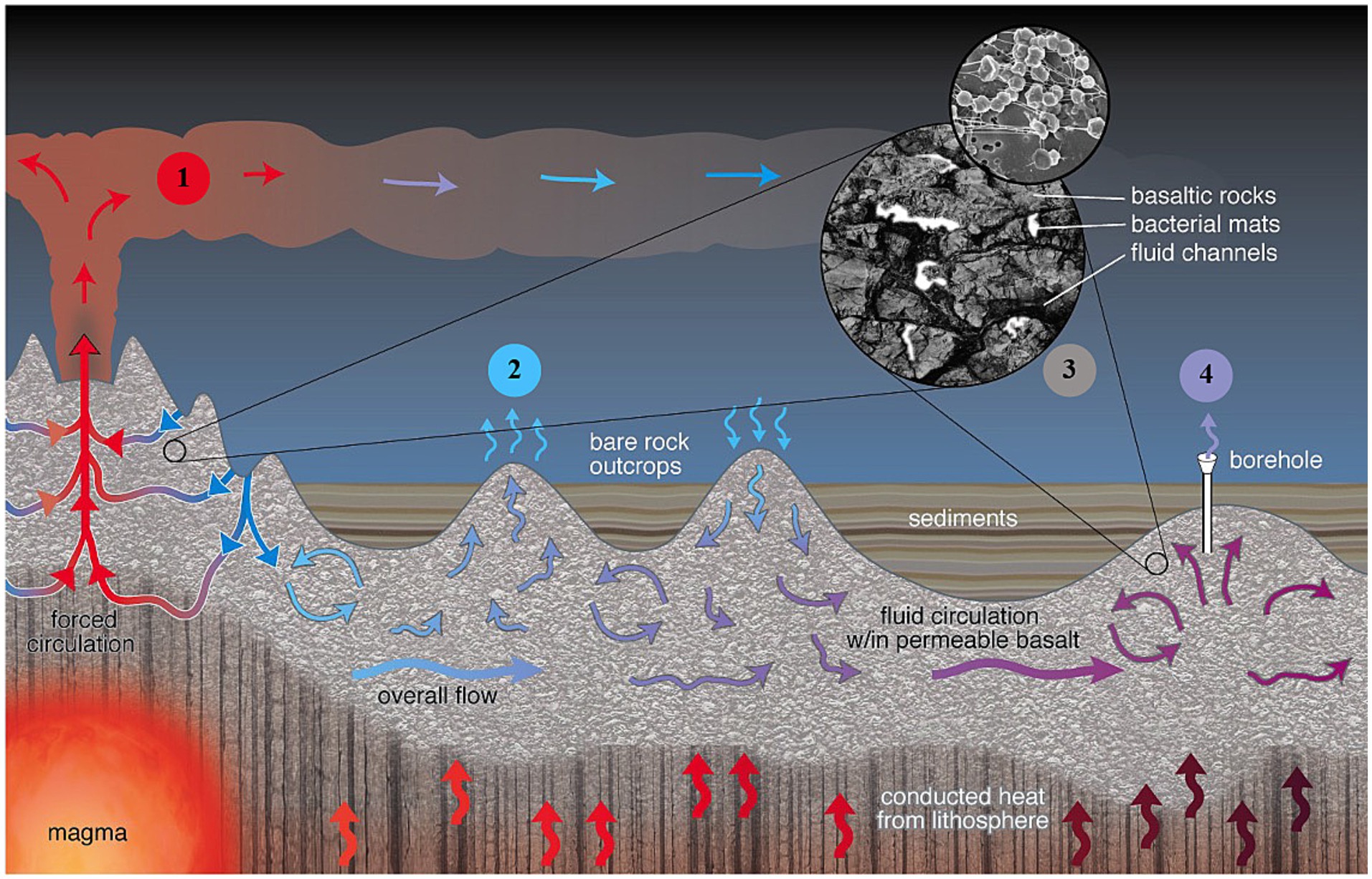
Figure 1. Several key access “windows” provide entry points to the crustal biosphere: ❶ Hydrothermal vents at mid-ocean ridges (MORs), featuring both diffuse and focused high-temperature flows (up to ~400°C) and event plumes associated with seafloor eruptions; ❷ Warm water seeps (<150°C) found at exposed rocky discharge zones on seamounts; ❸ Borehole cores extracted from sediment and basement rock layers; and ❹ Borehole CORK (Circulation Obviation Retrofit Kit) observatories, installed in boreholes, which offer the most precise control over sample placement, depth, and quality. These observatories provide unparalleled access to discrete depths in deep basement environments, enabling in situ sampling and experimentation with the highest degree of accuracy.
Access “windows” to crustal biosphere
The active circulation of hydrothermal fluids between open surfaces of the reservoir and overlying seawater provides multiple access “windows” to the subseafloor biosphere and, therefore, the opportunity to obtain high integrity samples from this challenging environment (Figure 1, #1–4). Most studies of subseafloor crustal microbiology (Figure 1, #1) have focused on MOR spreading areas, i.e., hydrothermal vents (Thorseth et al., 2001; Edwards et al., 2003; Lysnes et al., 2004), event plumes associated with seafloor eruptions (Meyer et al., 2013), and other settings where volcanism occurs (Fisk et al., 2003; Santelli et al., 2008). Active subseafloor microbial communities in venting fluids from MOR spreading axes are indicative of their local geologic setting and resultant geochemistry (Trembath-Reichert et al., 2019). These communities, however, do not reflect conditions in other crustal environments. The high-temperature focused fluid flows that characterized these MOR field sites tap the deepest and hottest (400°C) sub-crustal zone and can be considered end members with respect to the range of conditions that determine the nature of subseafloor crustal biomes (Elderfield et al., 1999). Most of the upper oceanic crust remains relatively cool, with temperatures below 150°C (Mottl and Wheat, 1994). Additionally, MOR flanks contribute more substantially to the global output of the upper crustal reservoir compared to the hotter axial regions, which play a comparatively minor role (Mottl, 2003). Recent research supports this disparity, showing that while axial zones release high-temperature fluids from hydrothermal activity near magma chambers, the ridge flanks exhibit a significantly greater overall heat flux through widespread low-temperature hydrothermal circulation (Urabe et al., 2015).
Exposed rocky discharge seamounts along MOR flanks in the other hand, are characterized by warm (<150°C) diffuse water seeps (Wheat et al., 2017) and offer an alternative access to the near surface basement biosphere (Figure 1, #2). Important surveys in exiting fluids of both heat and chemical fluxes as well as microbial communities have shown a high diversity of microorganisms in a continuum of near seafloor to deep subsurface biosphere communities (Hara et al., 2005; Huber et al., 2006; Lee et al., 2015). However, due in part to their generally diffuse flow there is a high mixing rate with overlying sediments (Meyers et al., 2014; Zinke et al., 2018) and, consequently, these sites have been studied to relatively limited extent.
At present, drilling represents the only access into the upper crustal reservoir for older, heavily sedimented crust (Figure 1, #3). Reports on the phylogenetic distribution of microbial communities within drilled marine basalts have revealed a cosmopolitan community with many members but vastly different from that in crustal fluids (Mason et al., 2007, 2009, 2010; Lever et al., 2013; Goordial et al., 2021). The extraction of borehole rock cores has no control over the placement, depth and quality of the samples (Lever, 2013), which leaves open the possibility of seawater or sediment contamination.
The best opportunities for co-located and simultaneous microbiological in situ sampling and experimentation have been provided by Borehole Circulation Obviation Retrofit Kit (CORK) observatories installed at Ocean Drilling Program (ODP/IODP) boreholes (e.g. Edwards et al., 2014; Fisher et al., 2005; Fisher et al., 2011) (Figure 1, #4). CORK’s critical attributes include the ability to penetrate through sediments into basement and isolate different horizons within the borehole, e.g., depth, degree of fracturing and differential flow rates. CORK observatories allow permanent access to samples through individual CORK installations as well as arrays of CORKs. Furthermore, in situ sampling and experimentation is possible using both downhole and seafloor fluid sampling systems. In downhole applications, flow-through osmotic pumping systems (FLOCS) represent an important technological advancement, providing a reliable fluid sampling solution. FLOCS are powerful steadfast osmotic pumps operating without any moving parts or electronics by bringing a reservoir of super-saturated salt solution to equilibrium (Orcutt et al., 2010; Edwards et al., 2012b). They are highly versatile and provide the pumping power to collect samples in manipulative experiments, such as colonization chambers that integrate various relevant mineral and control surfaces into the in-line flow path of the osmo-samplers (Orcutt et al., 2011a). On the other hand, technological advancements on seafloor applications, such as the GeoMICROBE sled (Cowen et al., 2012), represent significant progress in the ability to conduct long-term monitoring and in-depth analysis of microbial and chemical processes deep beneath the ocean floor. This innovative autonomous sensor and accompanying fluid sampling systems were designed to extract large volumes of fluid from crustal aquifers for surface analysis, highlighting a leap forward in crustal research capabilities (Lin et al., 2020). This article emphasizes investigations into the microbial community in the crustal subseafloor using borehole CORK observatories because they have yielded the most extensive knowledge on the crustal microbial biosphere (Orcutt et al., 2020) and stablished the foundation for present studies on the microbial community’s metabolic potential and role in global biogeochemical cycles.
Emerging crustal microbiology
Microbial communities in crustal fluids exhibit spatial heterogeneity (Jungbluth et al., 2013) and inter-annual variability (Jungbluth et al., 2014) reflecting the dynamic and diverse environments that characterize the hydrogeological active upper igneous crust. Shared distinct microbial lineages, however, are consistent with the inferred hydrogeologic connectivity of these systems and the idea of permanent residency in the crustal subseafloor (Jungbluth et al., 2016). When analyzing datasets from different crustal habitats, the nature of the crustal environment (distinguished by either planktonic communities or mineral-attached communities), the prevailing redox conditions, and the geochemical evolution of these habitats collectively reveal patterns of global biogeographic distribution (Smith et al., 2016; Zhang et al., 2016b; Ramírez et al., 2019; Orcutt et al., 2021). Time-course studies of the genetic makeup and evolutionary trajectories of recovered metagenome-assembled genomes (MAGs) in subsurface crustal fluids (Anderson et al., 2022) have shown rapid allele frequency shifts linked to gene flow and recombination between microbial populations, which are mainly associated with stochastic events such as dispersal and mixing of populations throughout the aquifer. Despite this dynamism, however, temporal and spatial trends reconstructed from MAGs (Tully et al., 2018) have shown a significant amount of functional redundancy in the subseafloor microbial populations, which do not correspond to changes in the composition of the community over time. This implies global microbial functional stability in the ubiquitous crustal subseafloor biosphere.
Driven by geochemical redox gradients created by the continued interaction of seawater with the basaltic rock at different spatial and temporal scales, microbes in these crustal environments have developed physiological and metabolic strategies to exploit the specific conditions of their environment. In young, oxic, and low-temperature crustal environments –key to most global hydrothermal fluid circulation in the ocean—the metabolic reconstruction of recovered MAGs (Tully et al., 2018) has revealed microorganisms representative of heterotrophic and autotrophic lifestyles. These versatile microbes, however, are also poised to exploit hypoxic and anoxic conditions with alternative electron acceptors, i.e., nitrate and sulfate. Furthermore, complementary time-series metatranscriptomic data have confirmed that microbial communities in this environment are populated by motile mixotrophic and organotrophic bacteria active under both oxic and anoxic conditions (Seyler et al., 2021). In old, anoxic, and warm crustal environments –representative of situations that are common in all ocean basins where hydrothermal circulation is isolated from the deep ocean– the MAG of a versatile heterotrophic microbial population has been recovered capable of oxidizing reduced carbon species with nitrate, iron, and sulfur compounds as potential electron acceptors (Boyd et al., 2019). Comparative analysis of single-amplified genomes (SAGs) representative of other persistent lineages also suggests the heterotrophic potential of anaerobic crustal communities to respire organic carbon with sulfate and nitrate (Carr et al., 2019) and other external terminal electron acceptors such as iron and sulfur oxides consistent with the mineralogy of these environments (Booker et al., 2023). Further genomic evidence has also identified MAGs representative of chemosynthetic microbial communities residing in mineral biofilms (Smith et al., 2019). These communities are sustained by water-rock reactions in a process involving the use molecular hydrogen to convert inorganic carbon from seawater into organic matter, with additional energy supplementation derived from amino acids or peptides present in the mineral-attached biofilms (Smith et al., 2021). Altogether, this suggests the presence of highly dynamic microbial communities capable of rapid adaptation to varying redox conditions within the basaltic crustal fluids. Moreover, the transcription of genes related to biofilm formation and motility suggests an adaptation for spatial relocation in response to environmental shifts, crucial for accessing diverse nutrient and energy sources.
The subseafloor crustal system as a continuous-flow biogeochemical reactor
The biogeochemical state of the subseafloor crustal reservoir is influenced by several factors. The age of the crust affects the geochemical extent of water-rock interaction, while the thermal state of the aquifer determines the nature and scale of metabolic reactions that can occur. Fluids along the flow paths of the hydrogeologically active upper oceanic crust exhibit a geochemical reaction consistent with a decline in the redox potential of oxidants and concentrations of organic material (Lin et al., 2012). By leveraging compositional geochemical data of basement fluids relative to bottom seawater, comprehensive thermodynamic modeling of diverse redox reactions has shown a geochemical shift toward lower free energy availability with increasing residence time and temperature (Robador et al., 2015). Despite this energetic constraint, direct heat measurements indicative of the change in enthalpy associated with total microbial activity have demonstrated the high metabolic potential of microbes to respire under oxic and anoxic conditions (Robador et al., 2016). This is observed in fluids characteristic of both young, oxygenated, and warm, highly reacted end-member areas of the upper oceanic crust, respectively. Furthermore, extensive studies have demonstrated the widespread potential of microbial activity in crustal fluids, with high rates of autotrophy and heterotrophy comparable to those found in surface environments (Meyer et al., 2016; Robador et al., 2016; Zhang et al., 2016a; Trembath-Reichert et al., 2021). These findings suggest that the upper crustal reservoir, functioning as a subsurface microbial biosphere, may be as metabolically active as the overlying ocean.
Additionally, the rate at which fluid flows through the crust influences the replenishment of reactants and the removal of byproducts, thus affecting the concentration of chemical species. During the geochemical evolution of fluids, redox species are removed, further shaping the chemical composition. This geochemical evolution of crustal fluids strongly suggests in situ microbial consumption within the basement (Orcutt et al., 2013; Robador et al., 2015). However, sediment pore water data from near sediment-basement interface (Elderfield et al., 1999) indicate that deep sediments must also serve as a sink for basement electron acceptors, which diffuse into the overlaying depleted sediments. This dynamic exchange supplies essential electron acceptors, such as oxygen and sulfate, to deeper sediment layers, which is crucial for sustaining microbial metabolisms within the sediment. Deep sediment porewater profiles demonstrate that oxygen diffuses upward from the underlying basalt and nitrate accumulates in the overlying sediments (Ziebis et al., 2012). This observation strongly implicates the process of nitrification as both an autotrophic sink of porewater oxygen and a source of nitrate, which, in turn, may support heterotrophy via denitrification where oxygen is absent and, potentially, autotrophic growth via methanogenesis. Furthermore, diffusion of sulfate from crustal fluids into overlying sediments, can form a transition zone where sulfate meets in situ-produced methane, thereby stimulating microbial heterotrophic respiration, i.e., anaerobic methane oxidation coupled to sulfate reduction (Engelen et al., 2008; Fichtel et al., 2012, 2015). These microbial processes, in turn, accelerate the breakdown of organic carbon deposits. Concurrently, the sediments supply electron donors, including dissolved organic carbon (DOC), to the basement ecosystem. Higher sediment pore water DOC likely diffuses into the basement, making it a sink for both seawater and sediment DOC. This reciprocal exchange results in the alteration of DOC compounds with respect to seawater (LaRowe et al., 2017) and underscores the complex interplay between geochemical processes and microbial life in deep subsurface crustal environments, driving both the degradation and the recycling of organic materials (Shah Walter et al., 2018; Lin et al., 2019). The ocean crustal biosphere, therefore, functions to simultaneously degrade surface-derived organic carbon and to export new, chemosynthetic material (McCarthy et al., 2011; Lin et al., 2015). As such, the ocean crustal biosphere acts as a biogeochemical reactor, facilitating the transformation and transport of elements through various states and locations. By breaking down organic carbon from surface sources and synthesizing new materials through chemosynthesis, it promotes element recycling and enhances ocean carbon productivity. Given that the entire volume of seawater is estimated to cycle through the ocean crust approximately every 100,000 years (Johnson and Pruis, 2003), this continuous-flow biogeochemical reactor plays a crucial role in driving the cycling of key elements, supporting diverse microbial communities, and sustaining essential ecological processes in the ocean. The complex interactions and metabolic activities within the ocean crustal biosphere underscore its critical role in maintaining the balance of both oceanic and global biogeochemical cycles.
Conclusion
The subseafloor crustal system plays a crucial role in the biogeochemical cycling within mid-ocean ridge flanks by redistributing mass and energy between deep sediments and basement aquifers and back into the ocean. Although quantitative estimates of microbial contributions to global biogeochemical cycles are limited by difficult access and sparse sampling, the widespread metabolic potential of this biosphere suggests that incorporating the crustal system into current global biogeochemical models will be crucial for accurately representing the full ocean carbon cycle. The ocean crustal biosphere plays a dual role, capturing the intricate processes of carbon degradation and synthesis occurring beneath the ocean floor. Recognizing its impact on global biogeochemical cycles will allow for a more comprehensive understanding of carbon mineralization and recycling, enhancing the accuracy of predictions related to ocean productivity and ecological dynamics. Moreover, studying the ocean crustal biosphere has significant implications for astrobiology, as it serves as an analog for potential life on other planetary bodies (Jones et al., 2018). Understanding how microbial life thrives in these extreme conditions on Earth offers valuable insights into the potential for life in similar environments elsewhere in the solar system and beyond. This connection highlights the broader importance of studying these systems, not only to improve our biogeochemical models but also to advance our search for life beyond Earth.
Author contributions
AR: Writing – original draft, Writing – review & editing.
Funding
The author(s) declare that no financial support was received for the research, authorship, and/or publication of this article.
Conflict of interest
The author declares that the research was conducted in the absence of any commercial or financial relationships that could be construed as a potential conflict of interest.
The author(s) declared that they were an editorial board member of Frontiers, at the time of submission. This had no impact on the peer review process and the final decision.
Publisher’s note
All claims expressed in this article are solely those of the authors and do not necessarily represent those of their affiliated organizations, or those of the publisher, the editors and the reviewers. Any product that may be evaluated in this article, or claim that may be made by its manufacturer, is not guaranteed or endorsed by the publisher.
References
Anderson, R., Graham, E., Huber, J., and Tully, B. (2022). Microbial populations are shaped by dispersal and recombination in a low biomass subseafloor habitat. MBio 13:e0035422. doi: 10.1128/mbio.00354-22
Bar-On, Y. M., Phillips, R., and Milo, R. (2018). The biomass distribution on earth. Proc. Natl. Acad. Sci. USA 115, 6506–6511. doi: 10.1073/pnas.1711842115
Booker, A. E., D’angelo, T., Adams-Beyea, A., Brown, J. M., Nigro, O., Rappé, M. S., et al. (2023). Life strategies for Aminicenantia in subseafloor oceanic crust. ISME J. 17, 1406–1415. doi: 10.1038/s41396-023-01454-5
Boyd, J. A., Jungbluth, S. P., Leu, A. O., Evans, P. N., Woodcroft, B. J., Chadwick, G. L., et al. (2019). Divergent methyl-coenzyme M reductase genes in a deep-subseafloor Archaeoglobi. ISME J. 13, 1269–1279. doi: 10.1038/s41396-018-0343-2
Carr, S. A., Jungbluth, S. P., Eloe-Fadrosh, E. A., Stepanauskas, R., Woyke, T., Rappé, M. S., et al. (2019). Carboxydotrophy potential of uncultivated Hydrothermarchaeota from the subseafloor crustal biosphere. ISME J. 13, 1457–1468. doi: 10.1038/s41396-019-0352-9
Cowen, J. P., Copson, D. A., Jolly, J., Hsieh, C. C., Lin, H. T., Glazer, B. T., et al. (2012). Advanced instrument system for real-time and time-series microbial geochemical sampling of the deep (basaltic) crustal biosphere. Deep-Sea Res. I Oceanogr. Res. Pap. 61, 43–56. doi: 10.1016/j.dsr.2011.11.004
Edwards, K. J., Bach, W., and Rogers, D. R. (2003). Geomicrobiology of the ocean crust: a role for chemoautotrophic Fe-bacteria. Biol. Bull. 204, 180–185. doi: 10.2307/1543555
Edwards, K., Fisher, A., and Wheat, C. G. (2012a). The deep subsurface biosphere in igneous ocean crust: frontier habitats for microbiological exploration. Front. Microbiol. 3:8. doi: 10.3389/fmicb.2012.00008
Edwards, K.J., Wheat, C.G., Orcutt, B.N., Hulme, S., Becker, K., and Jannasch, H., and Al., E. (2012b). “Design and deployment of borehole observatories and experiments during IODP expedition 336 mid-Atlantic ridge flank at north pond” in Proc. IODP, 336. (eds.) K.J. Edwards, W. Bach, A. Klaus & E. Scientists. Integrated Ocean Drilling Program Management International, Inc., Tokyo, Japan.
Edwards, A. T., Bach, T., and Klaus, K.the IODP Expedition 336 Scientific Party. (2014). Mid-Atlantic Ridge microbiology: initiation of long-term coupled microbiological, geochemical, and hydrological experimentation within the seafloor at North Pond, western flank of the Mid-Atlantic Ridge. IODP Prel. Rept. 336. doi: 10.2204/iodp.pr.336.2012
Edwards, K. J., Wheat, C. G., and Sylvan, J. B. (2011). Under the sea: microbial life in volcanic oceanic crust. Nat. Rev. Microbiol. 9, 703–712. doi: 10.1038/nrmicro2647
Elderfield, H., Wheat, C. G., Mottl, M. J., Monnin, C., and Spiro, B. (1999). Fluid and geochemical transport through oceanic crust: a transect across the eastern flank of the Juan de Fuca ridge. Earth Planet. Sci. Lett. 172, 151–165. doi: 10.1016/s0012-821x(99)00191-0
Engelen, B., Ziegelmueller, K., Wolf, L., Kopke, B., Gittel, A., Cypionka, H., et al. (2008). Fluids from the oceanic crust support microbial activities within the deep biosphere. Geomicrobiol J. 25, 56–66. doi: 10.1080/01490450701829006
Fichtel, K., Logemann, J., Fichtel, J., Rullkötter, J., Cypionka, H., and Engelen, B. (2015). Temperature and pressure adaptation of a sulfate reducer from the deep subsurface. Front. Microbiol. 6:1078. doi: 10.3389/fmicb.2015.01078
Fichtel, K., Mathes, F., Koenneke, M., Cypionka, H., and Engelen, B. (2012). Isolation of sulfate-reducing bacteria from sediments above the deep-subseafloor aquifer. Front. Microbiol. 3:65. doi: 10.3389/fmicb.2012.00065
Fisher, A. T., Wheat, C. G., Becker, K., Davis, E. E., Jannasch, H., Schroeder, D., et al. (2005). Scientific and technical design and deployment of long-term, subseafloor observatories for hydrogeologic and related experiments, IODP Expedition 301, eastern flank of Juan de Fuca Ridge. In Fisher, A.T., Urabe, T., Klaus, A., and the Expedition 301 Scientists, Proc. IODP, 301: College Station TX (Integrated Ocean Drilling Program Management International, Inc.). doi: 10.2204/iodp.proc.301.103.2005
Fisher, A. T., Tsuji, T., and Petronotis, K.the Expedition 327 Scientists. (2011). Site U1362. Proc. IODP, 327: Tokyo (Integrated Ocean Drilling Program Management International, Inc.). doi: 10.2204/iodp.proc.327.103.2011
Fisk, M. R., Storrie-Lombardi, M. C., Douglas, S., Popa, R., Mcdonald, G., and Di Meo-Savoie, C. (2003). Evidence of biological activity in Hawaiian subsurface basalts. Geochem. Geophys. Geosyst. 4:1103. doi: 10.1029/2002GC000387
Furnes, H., Banerjee, N., Muehlenbachs, K., Staudigel, H., and Wit, M. J. (2004). Early life recorded in Archean pillow lavas. Science 304, 578–581. doi: 10.1126/science.1095858
Goordial, J., D’angelo, T., Labonté, J. M., Poulton, N. J., Brown, J. M., Stepanauskas, R., et al. (2021). Microbial diversity and function in shallow subsurface sediment and oceanic lithosphere of the Atlantis massif. MBio 12, e0049021–e0000421. doi: 10.1128/mBio.00490-21
Hara, K., Kakegawa, T., Yamashiro, K., Maruyama, A., Ishibashi, J.-I., Marumo, K., et al. (2005). Analysis of the archaeal sub-seafloor community at Suiyo seamount on the Izu-Bonin arc. Adv. Space Res. 35, 1634–1642. doi: 10.1016/j.asr.2005.04.111
Heberling, C., Lowell, R. P., Liu, L., and Fisk, M. R. (2010). Extent of the microbial biosphere in the oceanic crust. Geochem. Geophys. Geosyst. 11. doi: 10.1029/2009gc002968
Huber, J. A., Johnson, H. P., Butterfield, D. A., and Baross, J. A. (2006). Microbial life in ridge flank crustal fluids. Environ. Microbiol. 8, 88–99. doi: 10.1111/j.1462-2920.2005.00872.x
Imachi, H., Tasumi, E., Takaki, Y., Hoshino, T., Schubotz, F., Gan, S. C., et al. (2019). Cultivable microbial community in 2-km-deep, 20-million-year-old subseafloor coalbeds through similar to 1000 days anaerobic bioreactor cultivation. Sci. Rep. 9:2305. doi: 10.1038/s41598-019-38754-w
Johnson, H. P., Baross, J. A., and Bjorklund, T. A. (2006). On sampling the upper crustal reservoir of the NE Pacific Ocean. Geofluids 6, 251–271. doi: 10.1111/j.1468-8123.2006.00151.x
Johnson, H. P., and Pruis, M. J. (2003). Fluxes of fluid and heat from the oceanic crustal reservoir. Earth Planet. Sci. Lett. 216, 565–574. doi: 10.1016/s0012-821x(03)00545-4
Jones, R. M., Goordial, J. M., and Orcutt, B. N. (2018). Low energy subsurface environments as extraterrestrial analogs. Front. Microbiol. 9:1605. doi: 10.3389/fmicb.2018.01605
Jungbluth, S. P., Bowers, R. M., Lin, H.-T., Cowen, J. P., and Rappé, M. S. (2016). Novel microbial assemblages inhabiting crustal fluids within mid-ocean ridge flank subsurface basalt. ISME J. 10, 2033–2047. doi: 10.1038/ismej.2015.248
Jungbluth, S. P., Grote, J., Lin, H.-T., Cowen, J. P., and Rappe, M. S. (2013). Microbial diversity within basement fluids of the sediment-buried Juan de Fuca ridge flank. ISME J. 7, 161–172. doi: 10.1038/ismej.2012.73
Jungbluth, S., Lin, H.-T., Cowen, J., Glazer, B. T., and Rappe, M. (2014). Phylogenetic diversity of microorganisms in subseafloor crustal fluids from boreholes 1025C and 1026B along the Juan de Fuca ridge flank. Front. Microbiol. 5:119. doi: 10.3389/fmicb.2014.00119
Kallmeyer, J., Pockalny, R., Adhikari, R. R., Smith, D. C., and D’hondt, S. (2012). Global distribution of microbial abundance and biomass in subseafloor sediment. Proc. Natl. Acad. Sci. USA 109, 16213–16216. doi: 10.1073/pnas.1203849109
Larowe, D. E., Koch, B. P., Robador, A., Witt, M., Ksionzek, K., and Amend, J. P. (2017). Identification of organic compounds in ocean basement fluids. Org. Geochem. 113, 124–127. doi: 10.1016/j.orggeochem.2017.07.017
Lauer, M. R., Fisher, A., and Winslow, D. M. (2018). Three-dimensional models of hydrothermal circulation through a seamount network on fast-spreading crust. Earth Planet. Sci. Lett. 501, 138–151. doi: 10.1016/j.epsl.2018.08.025
Lee, M. D., Walworth, N. G., Sylvan, J. B., Edwards, K. J., and Orcutt, B. N. (2015). Microbial communities on seafloor basalts at dorado outcrop reflect level of alteration and highlight global lithic clades. Front. Microbiol. 6:1470. doi: 10.3389/fmicb.2015.01470
Lever, M. A. (2013). Functional gene surveys from ocean drilling expeditions a review and perspective. FEMS Microbiol. Ecol. 84, 1–23. doi: 10.1111/1574-6941.12051
Lever, M. A., Rouxel, O., Alt, J. C., Shimizu, N., Ono, S. H., Coggon, R. M., et al. (2013). Evidence for microbial carbon and sulfur cycling in deeply buried ridge flank basalt. Science 339, 1305–1308. doi: 10.1126/science.1229240
Lin, H.-T., Amend, J. P., Larowe, D. E., Bingham, J.-P., and Cowen, J. P. (2015). Dissolved amino acids in oceanic basaltic basement fluids. Geochim. Cosmochim. Acta 164, 175–190. doi: 10.1016/j.gca.2015.04.044
Lin, H. T., Cowen, J. P., Olson, E. J., Amend, J. P., and Lilley, M. D. (2012). Inorganic chemistry, gas compositions and dissolved organic carbon in fluids from sedimented young basaltic crust on the Juan de Fuca ridge flanks. Geochim. Cosmochim. Acta 85, 213–227. doi: 10.1016/j.gca.2012.02.017
Lin, H.-T., Hsieh, C.-C., Repeta, D. J., and Rappé, M. S. (2020). Sampling of basement fluids via circulation obviation retrofit kits (CORKs) for dissolved gases, fluid fixation at the seafloor, and the characterization of organic carbon. MethodsX 7:101033. doi: 10.1016/j.mex.2020.101033
Lin, H.-T., Repeta, D. J., Xu, L., and Rappé, M. S. (2019). Dissolved organic carbon in basalt-hosted deep subseafloor fluids of the Juan de Fuca ridge flank. Earth Planet. Sci. Lett. 513, 156–165. doi: 10.1016/j.epsl.2019.02.008
Lysnes, K., Thorseth, I. H., Steinsbu, B. O., Ovreas, L., Torsvik, T., and Pedersen, R. B. (2004). Microbial community diversity in seafloor basalt from the Arctic spreading ridges. FEMS Microbiol. Ecol. 50, 213–230. doi: 10.1016/j.femsec.2004.06.014
Mason, O. U., Di Meo-Savoie, C. A., Van Nostrand, J. D., Zhou, J. Z., Fisk, M. R., and Giovannoni, S. J. (2009). Prokaryotic diversity, distribution, and insights into their role in biogeochemical cycling in marine basalts. ISME J. 3, 231–242. doi: 10.1038/ismej.2008.92
Mason, O. U., Nakagawa, T., Rosner, M., Van Nostrand, J. D., Zhou, J. Z., Maruyama, A., et al. (2010). First investigation of the microbiology of the deepest layer of ocean crust. PLoS One 5:e15399. doi: 10.1371/journal.pone.0015399
Mason, O. U., Stingl, U., Wilhelm, L. J., Moeseneder, M. M., Di Meo-Savoie, C. A., Fisk, M. R., et al. (2007). The phylogeny of endolithic microbes associated with marine basalts. Environ. Microbiol. 9, 2539–2550. doi: 10.1111/j.1462-2920.2007.01372.x
Mccarthy, M. D., Beaupre, S. R., Walker, B. D., Voparil, I., Guilderson, T. P., and Druffel, E. R. M. (2011). Chemosynthetic origin of C-14-depleted dissolved organic matter in a ridge-flank hydrothermal system. Nat. Geosci. 4, 32–36. doi: 10.1038/ngeo1015
Meyer, J. L., Akerman, N. H., Proskurowski, G., and Huber, J. A. (2013). Microbiological characterization of post-eruption "snowblower" vents at axial seamount, Juan de Fuca Ridge. Front. Microbiol. 4:153. doi: 10.3389/fmicb.2013.00153
Meyer, J. L., Jaekel, U., Tully, B., Glazer, B. T., Wheat, C. G., Lin, H. T., et al. (2016). A distinct and active bacterial community in cold oxygenated fluids circulating beneath the western flank of the mid-Atlantic ridge. Sci. Rep. 6:22541. doi: 10.1038/srep22541
Meyers, M. E. J., Sylvan, J. B., and Edwards, K. J. (2014). Extracellular enzyme activity and microbial diversity measured on seafloor exposed basalts from Loihi seamount indicate the importance of basalts to global biogeochemical cycling. Appl. Environ. Microbiol. 80, 4854–4864. doi: 10.1128/AEM.01038-14
Morono, Y., Terada, T., Nishizawa, M., Ito, M., Hillion, F., Takahata, N., et al. (2011). Carbon and nitrogen assimilation in deep subseafloor microbial cells. Proc. Natl. Acad. Sci. USA 108, 18295–18300. doi: 10.1073/pnas.1107763108
Mottl, M. J. (2003). “Partitioning of energy and mass fluxes between mid-ocean ridge axes and flanks at high and low temperature” in Energy and Mass Transfer in Marine Hydrothermal Systems. eds. P. Halbach, V. Tunnicliffe, and J. R. Hein (Berlin: Dahlem University Press), 271–286.
Mottl, M. J., and Wheat, C. G. (1994). Hydrothermal circulation through mid ocean ridge flanks: fluxes of heat and magnesium. Geochim. Cosmochim. Acta 58, 2225–2237. doi: 10.1016/0016-7037(94)90007-8
Neira, N. M., Clark, J. F., Fisher, A. T., Wheat, C. G., Haymon, R. M., and Becker, K. (2016). Cross-hole tracer experiment reveals rapid fluid flow and low effective porosity in the upper oceanic crust. Earth Planet. Sci. Lett. 450, 355–365. doi: 10.1016/j.epsl.2016.06.048
Orcutt, B. N., Bach, W., Becker, K., Fisher, A. T., Hentscher, M., Toner, B. M., et al. (2011a). Colonization of subsurface microbial observatories deployed in young ocean crust. ISME J. 5, 692–703. doi: 10.1038/ismej.2010.157
Orcutt, B., D'angelo, T., Jungbluth, S.P., Huber, J.A., and Sylvan, J.B. (2020). "Microbial life in oceanic crust". OSF [Preprint]. doi: 10.31219/osf.io/2wxe6
Orcutt, B. N., D'angelo, T., Wheat, C. G., and Trembath-Reichert, E. (2021). Microbe-mineral biogeography from multi-year incubations in oceanic crust at north pond, mid-Atlantic ridge. Environ. Microbiol. 23, 3923–3936. doi: 10.1111/1462-2920.15366
Orcutt, B. N., Sylvan, J. B., Knab, N. J., and Edwards, K. J. (2011b). Microbial ecology of the Dark Ocean above, at, and below the seafloor. Microbiol. Mol. Biol. Rev. 75, 361–422. doi: 10.1128/mmbr.00039-10
Orcutt, B., Wheat, C. G., and Edwards, K. J. (2010). Subseafloor Ocean crust microbial observatories: development of FLOCS (FLow-through Osmo colonization system) and evaluation of borehole construction materials. Geomicrobiol J. 27, 143–157. doi: 10.1080/01490450903456772
Orcutt, B. N., Wheat, C. G., Rouxel, O., Hulme, S., Edwards, K. J., and Bach, W. (2013). Oxygen consumption rates in subseafloor basaltic crust derived from a reaction transport model. Nat. Commun. 4:2539. doi: 10.1038/ncomms3539
Parkes, R. J., Cragg, B., Roussel, E., Webster, G., Weightman, A., and Sass, H. (2014). A review of prokaryotic populations and processes in sub-seafloor sediments, including biosphere: geosphere interactions. Mar. Geol. 352, 409–425. doi: 10.1016/j.margeo.2014.02.009
Ramírez, G. A., Garber, A. I., Lecoeuvre, A., D’angelo, T., Wheat, C. G., and Orcutt, B. N. (2019). Ecology of subseafloor crustal biofilms. Front. Microbiol. 10:1983. doi: 10.3389/fmicb.2019.01983
Robador, A., Jungbluth, S. P., Larowe, D. E., Bowers, R., Rappe, M., Amend, J. P., et al. (2015). Activity and phylogenetic diversity of sulfate-reducing microorganisms in low-temperature subsurface fluids within the upper oceanic crust. Front. Microbiol. 5:748. doi: 10.3389/fmicb.2014.00748
Robador, A., Larowe, D. E., Jungbluth, S. P., Lin, H. T., Rappe, M. S., Nealson, K. H., et al. (2016). Nanocalorimetric characterization of microbial activity in deep subsurface oceanic crustal fluids. Front. Microbiol. 7:454. doi: 10.3389/fmicb.2016.00454
Santelli, C. M., Orcutt, B. N., Banning, E., Bach, W., Moyer, C. L., Sogin, M. L., et al. (2008). Abundance and diversity of microbial life in ocean crust. Nature 453, 653–656. doi: 10.1038/nature06899
Seyler, L. M., Trembath-Reichert, E., Tully, B. J., and Huber, J. A. (2021). Time-series transcriptomics from cold, oxic subseafloor crustal fluids reveals a motile, mixotrophic microbial community. ISME J. 15, 1192–1206. doi: 10.1038/s41396-020-00843-4
Shah Walter, S. R., Jaekel, U., Osterholz, H., Fisher, A. T., Huber, J. A., Pearson, A., et al. (2018). Microbial decomposition of marine dissolved organic matter in cool oceanic crust. Nat. Geosci. 11, 334–339. doi: 10.1038/s41561-018-0109-5
Smith, A. R., Fisk, M. R., Thurber, A. R., Flores, G. E., Mason, O. U., Popa, R., et al. (2016). Deep crustal communities of the Juan de Fuca ridge are governed by mineralogy. Geomicrobiol J. 34, 147–156. doi: 10.1080/01490451.2016.1155001
Smith, A. R., Kieft, B., Mueller, R., Fisk, M. R., Mason, O. U., Popa, R., et al. (2019). Carbon fixation and energy metabolisms of a subseafloor olivine biofilm. ISME J. 13, 1737–1749. doi: 10.1038/s41396-019-0385-0
Smith, A. R., Mueller, R., Fisk, M. R., and Colwell, F. S. (2021). Ancient metabolisms of a thermophilic subseafloor bacterium. Front. Microbiol. 12:764631. doi: 10.3389/fmicb.2021.764631
Thorseth, I. H., Torsvik, T., Torsvik, V., Daae, F. L., and Pedersen, R. B. (2001). Diversity of life in ocean floor basalt. Earth Planet. Sci. Lett. 194, 31–37. doi: 10.1016/S0012-821X(01)00537-4
Trembath-Reichert, E., Butterfield, D. A., and Huber, J. A. (2019). Active subseafloor microbial communities from Mariana back-arc venting fluids share metabolic strategies across different thermal niches and taxa. ISME J. 13, 2264–2279. doi: 10.1038/s41396-019-0431-y
Trembath-Reichert, E., Morono, Y., Ijiri, A., Hoshino, T., Dawson, K. S., Inagaki, F., et al. (2017). Methyl-compound use and slow growth characterize microbial life in 2-km-deep subseafloor coal and shale beds. Proc. Natl. Acad. Sci. USA 114, E9206–E9215. doi: 10.1073/pnas.1707525114
Trembath-Reichert, E., Shah Walter, S. R., Ortiz, M. A. F., Carter, P. D., Girguis, P. R., and Huber, J. A. (2021). Multiple carbon incorporation strategies support microbial survival in cold subseafloor crustal fluids. Sci. Adv. 7:eabg0153. doi: 10.1126/sciadv.abg0153
Tully, B. J., Wheat, C. G., Glazer, B. T., and Huber, J. A. (2018). A dynamic microbial community with high functional redundancy inhabits the cold, oxic subseafloor aquifer. ISME J. 12, 1–16. doi: 10.1038/ismej.2017.187
Urabe, T., Ishibashi, J.-I., Sunamura, M., Okino, K., Takai, K., and Suzuki, K. (2015) In: Subseafloor biosphere linked to hydrothermal systems: TAIGA concept, edited by J.-I. Ishibashi, K. Okino and M. Sunamura. Springer Japan 2015.
Wheat, C. G., Fisher, A. T., Mcmanus, J., Hulme, S. M., and Orcutt, B. N. (2017). Cool seafloor hydrothermal springs reveal global geochemical fluxes. Earth Planet. Sci. Lett. 476, 179–188. doi: 10.1016/j.epsl.2017.07.049
Winslow, D. M., and Fisher, A. T. (2015). Sustainability and of outcrop-to-outcrop hydrothermal circulation. Nat. Commun. 6:7567. doi: 10.1038/ncomms8567
Winslow, D. M., Fisher, A. T., Stauffer, P. H., Gable, C. W., and Zyvoloski, G. A. (2016). Three-dimensional modeling of outcrop-to-outcrop hydrothermal circulation on the eastern flank of the Juan de Fuca ridge. J. Geophys. Res. Solid Earth 121, 1365–1382. doi: 10.1002/2015jb012606
Zhang, X., Fang, J., Bach, W., Edwards, K. J., Orcutt, B. N., and Wang, F. (2016a). Nitrogen stimulates the growth of subsurface basalt-associated microorganisms at the Western flank of the mid-Atlantic ridge. Front. Microbiol. 7:633. doi: 10.3389/fmicb.2016.00633
Zhang, X., Feng, X., and Wang, F. (2016b). Diversity and metabolic potentials of subsurface crustal microorganisms from the Western flank of the mid-Atlantic ridge. Front. Microbiol. 7:363. doi: 10.3389/fmicb.2016.00363
Ziebis, W., Mcmanus, J., Ferdelman, T., Schmidt-Schierhorn, F., Bach, W., Muratli, J., et al. (2012). Interstitial fluid chemistry of sediments underlying the North Atlantic gyre and the influence of subsurface fluid flow. Earth Planet. Sci. Lett. 323-324, 79–91. doi: 10.1016/j.epsl.2012.01.018
Zinke, L. A., Reese, B. K., Mcmanus, J., Wheat, C. G., Orcutt, B. N., and Amend, J. P. (2018). Sediment microbial communities influenced by cool hydrothermal fluid migration. Front. Microbiol. 9:1249. doi: 10.3389/fmicb.2018.01249
Zobell, C. E. (1938). Studies on the bacterial flora of marine bottom sediments. J. Sediment. Res. 8, 10–18. doi: 10.1306/d4268fd6-2b26-11d7-8648000102c1865d
Keywords: subseafloor biosphere, crustal microbiology, biogeochemical cycling, hydrothermal circulation, carbon productivity
Citation: Robador A (2024) The subseafloor crustal biosphere: Ocean’s hidden biogeochemical reactor. Front. Microbiol. 15:1495895. doi: 10.3389/fmicb.2024.1495895
Edited by:
Mark Alexander Lever, The University of Texas at Austin, United StatesReviewed by:
Michael Hügler, Technologiezentrum Wasser, GermanyCopyright © 2024 Robador. This is an open-access article distributed under the terms of the Creative Commons Attribution License (CC BY). The use, distribution or reproduction in other forums is permitted, provided the original author(s) and the copyright owner(s) are credited and that the original publication in this journal is cited, in accordance with accepted academic practice. No use, distribution or reproduction is permitted which does not comply with these terms.
*Correspondence: Alberto Robador, cm9iYWRvcmFAdXNjLmVkdQ==