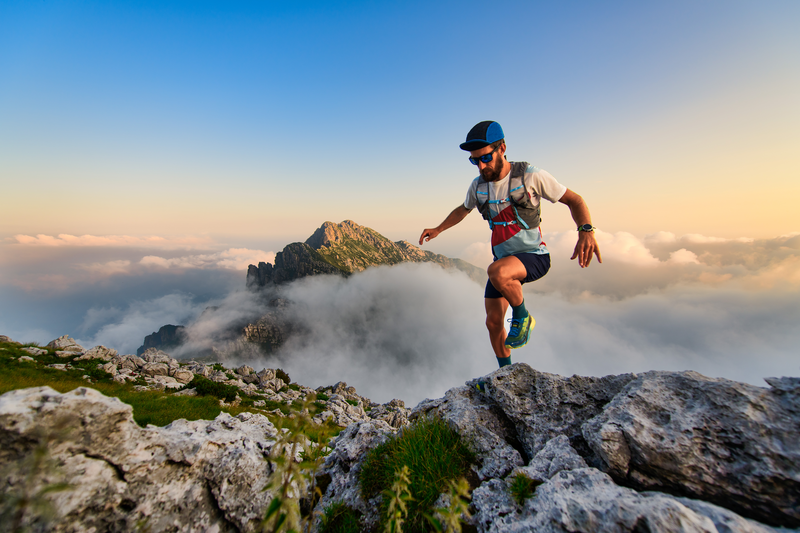
95% of researchers rate our articles as excellent or good
Learn more about the work of our research integrity team to safeguard the quality of each article we publish.
Find out more
ORIGINAL RESEARCH article
Front. Microbiol. , 02 December 2024
Sec. Microbiotechnology
Volume 15 - 2024 | https://doi.org/10.3389/fmicb.2024.1487165
This article is part of the Research Topic The Role Of Microbial Communities In Environmental Engineering Systems View all 3 articles
The biomass of native microorganisms in food waste (FW) suitable for accelerated composting is initially low and requires time for adaptation. Adding of efficient hydrolytic microorganisms should be able to enhance compost-specific microbial activity, adjust microbial community structure, and potentially hasten FW biodegradation. This study aimed to identify bacterial and fungal strains with growth characteristics suitable for accelerating FW composting. Over 7 weeks, FW was composted in a pilot-scale test, either inoculated at the start or on day 28 with three different mixtures of 10 autochthonous Bacillus and Penicillium spp. strains known for their high hydrolytic activity. The effects of inoculation were assessed by measuring the rate of carbon dioxide (CO2) and ammonia (NH3) production and also the increase in temperature due to spontaneous exothermic activity of the enhanced microbial population degrading FW. Inoculation with Bacillus spp., particularly B. amyloliquefaciens and B. subtilis, at the beginning of composting increased CO2 production nearly 3-fold while maintaining stable ammonia production and temperature. The high concentration of Bacillus relative to native FW microorganisms led to dominant fermentation processes even in the presence of oxygen, resulting in moderate heat release and elevated production of volatile organic compounds. Introducing Penicillium spp. at a later stage (day 28) increased CO2 production nearly 2-fold, along with higher NH3 levels and temperature. These findings highlight the significance of inoculation timing and microbial composition in regulating metabolic pathways during FW composting degradation, offering insights for designing effective microbial formulations for composting.
Food waste (FW) is generated at all stages of the food supply chain, from the farms to the ultimate consumers (Parfitt et al., 2010). FW consists of unharvested crops, trimmings and peels, by-products, stale or expired products, damaged goods, unused ingredients, leftovers, and packaging waste. About 1.3 billion tons of FW are generated globally each year, and these amounts are expected to be increasing for the next 25 years (Li et al., 2020). Composting is an efficient way to transform food waste into an organic fertilizer for plant growth, providing a recycling option instead of a one-way process (Wang et al., 2024).
FW are characterized by heterogeneous and variable composition and high moisture content of 65–80% (Awasthi et al., 2017; Cerda et al., 2018). The biomass of intrinsic composting-specific FW microorganisms is low in the early stages of composting and it takes some time for these organisms to proliferate (Xi et al., 2015). Lactic acid microorganisms of the order Lactobacillales predominate in the initial FW (Mironov et al., 2021). These bacteria consume readily available organic compounds (primarily sugars) by fermentation and release organic acids, which leads to a decrease in substrate pH and slower microbial degradation (Brinton, 1998; Wang et al., 2024). In addition, even at high temperature (60°C), pathogenic mesophilic microorganisms Salmonella sp., Pseudomonas aeruginosa have been found in the active phase of FW composting (Droffner et al., 1995). Therefore, processing of FW remains a challenge.
Artificial addition of ‘IM − introduced microorganisms’ can rapidly adjust the microbiota structure and improve microbial activity (Fang et al., 2019; Li et al., 2020). The use of IM reduces the initial acidification of FW (Song et al., 2018; Nakasaki et al., 2013; Nakasaki and Hirai, 2017), shortens composting time (Wang et al., 2024; Barrena et al., 2006), waste volume (Duan et al., 2020; Cerda et al., 2018) and malodour emissions (Fan et al., 2018; Liu et al., 2024), and improves the quality of the finished product (Voběrková et al., 2017; Ballardo et al., 2017).
As IM for FW composting, bacteria of genera are most often used Bacillus (Song et al., 2018; Awasthi et al., 2017; Duan et al., 2020; Wang et al., 2024; Zhang et al., 2024), Geobacillus (Sarkar et al., 2010; Fang et al., 2019), as well as fungi of the genera Trichoderma, Aspergillus (Awasthi et al., 2014; Heidarzadeh et al., 2019) and white-rot fungi (Voběrková et al., 2017). The use of microorganisms isolated from composted waste and most adapted to the conditions is promising (Sarkar et al., 2010; Awasthi et al., 2017). For example, representatives of the genera Bacillus, Caldibacillus, Aspergillus and Penicillium were the dominant degraders in the FW aerobic composting (Li et al., 2020; Mironov et al., 2021).
The concentration of IM in the waste is usually brought to values of ∼105–108 CFU g−1 FW (Ke et al., 2010; Song et al., 2018). Probably, these values depend on the type of IM. Thus, a concentration of ∼105 CFU g−1 was used for actinomycete of the genus Thermoactinomyces (Ke et al., 2010), fungal compositions of the genera Trichoderma and Aspergillus (Awasthi et al., 2014), and bacterial-fungal compositions (Xi et al., 2015). For mesophilic the acid-consuming yeast of the genus Pichia, a concentration of 107 CFU g−1 was used (Nakasaki et al., 2013). For bacterial inoculants (mainly members of the genus Bacillus), concentrations of 106–107 CFU g−1 were used for isolated cultures (Zhang et al., 2024; Rastogi et al., 2019; Ballardo et al., 2017) and 108 CFU g−1 for consortia (Song et al., 2018). The concentration of autochthonous microbiota in source wastes before composting can be 106–107 CFU g−1 and 109 CFU g−1 during the period of highest decomposition rate (Awasthi et al., 2014; Manu et al., 2017; Mironov et al., 2021). Probably, the effect of IM depends on the quantitative and qualitative composition of the waste’s own microbiota.
IM inoculation is mainly performed at the start of composting (Xie et al., 2023; Awasthi et al., 2017), but there are also reports of inoculation at the end of the thermophilic stage (Voběrková et al., 2017) and in several stages (Zhu et al., 2023; Xi et al., 2015). Probably, multistage inoculation can be more effective than single-stage inoculation due to the possibility of using specific microorganisms adapted to the current stage of the process (availability of substances, temperature, pH, oxygen availability). And it also avoids the competition of the inoculum with the waste’s own microorganisms (Xi et al., 2015). Thus, despite the existing research results, the issues of screening microorganisms to enhance aerobic composting of FW are relevant. There is still insufficient information on how the timing of inoculation and the quantitative ratio between the introduced and autochthonous microbiota affect FW degradation.
IM enhance composting either directly through the activity of the inoculum cultures themselves or indirectly by influencing their own waste microbiota. Wang et al. (2024) conclude that inoculum had a significant effect on the succession of the bacterial community, which caused the enhancement of composting. Inoculation can improve the community structure and functional diversity of microorganisms and enhance the growth of dominant microorganisms (Xi et al., 2015). Analyzing the succession of FW microbial community under the influence of IM can provide insight into the mechanisms of inoculant action.
In the absence of a standardized methodology for evaluating the action of IM in composting, it is difficult to assess the effect. It is useful to express the response to inoculation of microorganisms by indicators that are well suited to instrumental evaluation, e.g., self-heating temperature, CO2 and ammonia production dynamics. The measurement of CO2 production quantifies the degradation of organic matter during composting (Sánchez-García et al., 2015). This indicates progressive stabilization of the material, as CO2 production is often used as a respirometric index that reflects microbial activity. Most NH3 emissions are associated with ammonification, which can reveal the rate of decomposition of organic nitrogen, such as protein (Wang et al., 2024). In turn, temperature shows the rate of organic matter degradation and is related to microbial metabolic activity during composting.
Thus, this study aimed to screen active autochthonous microorganisms for rapid decomposition of raw FW under controlled aerobic composting conditions. The effect of inoculation was evaluated on the basis of data on the dynamics of CO2, ammonia production and temperature change. Experimental studies were carried out on non-sterile raw FW under pilot-scale test composting conditions.
Our strategy of stepwise screening for potential FW degraders included the following steps (Supplementary Figure 1): (1) obtaining biological material from composted FW; (2) obtaining parent and pure cultures of microorganisms; (3) testing their metabolic activity; (4) composing microbial formulations and testing the cultures for antagonism; (5) evaluating the effects of inoculation during FW composting in a pilot-scale test, and (6) investigating the mechanism of action of the effective composting composition.
To obtain enrichment cultures of microorganisms, samples of composted waste (food waste, wood chips, excess sludge from biological treatment facilities of dairy production, and mechanically sorted organic fraction of solid municipal waste) were collected at the “Grunt Eco” industrial composting plant (Moscow oblast’; 55°32′26″N, 38°4′46″E).
For microbiological analysis, 1 g samples of composted waste were resuspended in 10 mL of sterile tap water with 5-mm glass beads (Sigmund Linder GmbH, Germany) and shaken for 30 s on Bio Vortex V1 (Biosan, Latvia). Next, series of 10-fold dilutions were prepared and plated on reinforced clostridial agar (g L−1): yeast extract 13.0, peptone 10.0, NaCl 5.0, sodium acetate 3.0, glucose 5.0, starch 1.0, cysteine hydrochloride 0.5; pH 7.0 and incubated for 3–4 days at temperatures of 28 and 55°C. The choice of the medium was determined by its composition resembling FW in the representation of various organic compounds. The same medium was used to isolate pure microbial cultures from the enrichments.
The isolated pure cultures were tested for their ability to utilize a variety of carbon compounds: starch, milk proteins, cellulose, and lipids. A similar method for the isolation and selection of cultures for composting compositions was described by Kausar et al. (2011) and Awasthi et al. (2017). For cultures growing on various substrates, the ratio of the hydrolysis zone diameter to the colony diameter was used as an estimate of the culture’s substrate-degrading activity (Hankin et al., 1971; Hankin and Anagnostakis, 1975). Specifically, proteolytic activity was determined on modified Eikman agar of the following composition (g L−1): peptone 5.0, yeast extract 3.0, skimmed milk powder 1.0, agar 15.0; pH 7.0. Amylolytic activity was assessed on the Starch–mineral salt–agar medium described by Kausar et al. (2011) (g L−1): soluble starch 10.0, K2HPO4 1.0, MgSO4 1.0, NaCl 1.0, (NH4)2SO4 2.0, CaCO3 2.0, FeSO4 0.001, MnCl2 0.001, agar 20.0; pH 7.0. The zones of starch hydrolysis were visualized using iodine solution (5% alcohol solution diluted 10 times with water). Cellulolytic activity was determined using a modified Hetchinson–Clayton medium (g L−1): carboxymethylcellulose 2.0, NaNO3 2.5, (NH4)2SO4 2.0, K2HPO4 1.0, MgSO4·7H2O 0.3, NaCl 0.1, CaCl2·4H2O 0.1, FeCl3·6H2O 0.01, agar 17.0; рН 7.2. Cellulose degradation was visualized using a 0.1% (w/w) aqueous Congo red solution. Lipolytic activity was determined using a medium with the following composition (g L−1): Tween-80 10.0, peptone 10.0; NaCl 5.0, CaCl2·2H2O 0.1, agar 20.0. The results were analyzed by measuring the diameter of an opaque zone of calcium salts of fatty acids released from Tween-80 around the colonies.
Bacterial and fungal isolates were identified based on 16S rRNA and ITS gene sequences. For this purpose, the microorganisms were pre-grown in 50 mL vials in liquid LB. Once the desired culture density was reached, the culture was washed from the medium and concentrated in Eppendorf microcentrifuge tubes. DNA was isolated from biomass as described in Boulygina et al. (2002). The concentration of the resulting DNA preparations was 30–50 μg mL−1. RNA was present in trace amounts (less than 1% by electrophoretic analysis). For each of the samples studied, PCR and subsequent sequencing of the amplified 16S rRNA gene fragments were performed using universal primer systems to detect both eubacteria (11f–1492r) (Lane, 1991) and fungi (ITS5) (White et al., 1990). PCR products were analyzed by electrophoresis in 2% agarose gel at an electric field strength of 6 V cm−1. PCR products were isolated and purified from fusible agarose using WizardPCRPreps reagent kit (Promega, USA) according to the manufacturer’s recommendations. Sequencing of the obtained PCR gene fragments was performed according to the Sanger method (Sanger et al., 1977) on an ABI 3730 (3500XL) genetic analyzer (AppliedBiosystems, Inc., USA) using BigDyeTerminatorv.3.1 reagent set (AppliedBiosystems, Inc., USA) at the Core Facility “Bioengineering” of the Research Center of Biotechnology RAS. The primary similarity analysis of the obtained nucleotide sequences was performed using the BLAST software package (Camacho et al., 2009).
Microbial cultures were selected for inoculum compositions based on their highest hydrolytic activity, and their main morphological features (colony and cell morphology) and physiological characteristics (temperature range of growth) were described.
To avoid antagonism between strains included in the same composition, their mutual influence was assessed using the cross-streak method (Egorov, 2004; Lertcanawanichakul and Sawangnop, 2008). The first culture was seeded with a streak on the agar surface in a Petri dish. After the colonies appeared, the second culture was seeded perpendicularly to the streak, and the dishes were placed in a thermostat at 28°C for 24 h. Culture inhibition was recorded if the colony size decreased in comparison to normal growth of that culture.
To prepare inoculum compositions, the selected bacterial cultures were first grown separately in 150 mL of liquid LB medium (g L−1: triptone 10.0, yeast extract 5.0, NaCl 5.0, agar 15.0) at 28°C and 130 rpm for 2 days. The cultures were washed from the medium, resuspended in sterile tap water, and mixed in equal amounts (v/v) as described by Awasthi et al. (2017). The resulting inoculum (300 mL) was added to the composting substrate with a volume of 10 L and an average wet sample weight of 4,500 g (average dry matter weight, 1,230 g; average organic matter weight, 1,070 g). To assess the effect of inoculation dose, suspensions with cell titers of 106 CFU mL−1 and 109 CFU mL−1 of each culture were used. The total concentration of cells added to composting substrate was 4 × 105 CFU and 1 × 108 CFU g−1 raw weight FW of the original moisture content. The control substrate was supplemented with 300 mL of tap water. The substrate was thoroughly mixed to ensure uniform distribution of the inoculum.
To obtain the inoculum of the fungal composition, the selected fungi were cultivated on Czapek agar (g L−1: sucrose 30.0, NaNO3 3.0, K2HPO4 1.0, MgSO4·7H2О 0.5, FeSO4·7H2O 0.01, agar 15.0) until a mycelium with spores appeared. After 7 days of cultivation, fungal spores were collected from Petri dishes and suspended in sterile water to a concentration of 106 CFU mL−1. The inoculum of the fungal composition was prepared by mixing the spore suspensions and added to FW to obtain a final spore concentration of 104 CFU g−1 raw weight FW.
Experimental composting of non-sterile food waste was carried out in 2 variants. In option I (START), raw food waste was inoculated and then composted for 28 days. Uninoculated raw food waste was composted as a control experiment. In option II (RESTART), pre-composted food waste from the control experiment after 28 days was supplemented with tap water to an optimum moisture content of about 60% (wt/wt) and air-cooled for 1–2 h to ambient temperature so as to equalize the experimental conditions. The substrate thus obtained was inoculated and composted for 21 days. The experiments were conducted under laboratory conditions at a temperature of 22.1 ± 2.8°C and a humidity of 32.2 ± 4.1%.
The inoculum compositions were separately applied at 2 stages of composting: START or RESTART.
The effect of the inoculum compositions on the composting efficiency was assessed based on CO2 formation (principal criterion), NH3 formation, and composting temperature Tav (auxiliary criteria). Criteria: (i) the average CO2 formation during the composting period should be greater than in the control without inoculation (Test >Control); (ii) the average NH3 formation during the composting period must be greater than or equal to the control without inoculation (Test ≥Control); (iii) mean temperature Tav should be greater than or equal to the mean temperature in the control without inoculation (Test ≥Control). The results were graded as “+” if the average values of these parameters were higher in the experiment than in the control or equal; overwise, they were graded as “–.” If the difference between the experimental and the control values was more than 2-fold or more than 5°C, the result was graded as significant: “++” (or “– –”).
FW substrate obtained in a waste processing facility consisted of expired products in typical proportions (wt%): potato, 18.0; cabbage, 18.0; apple, 7.2; orange, 7.2; banana, 7.2; minced meat, 3.6; fish, 1.4; bread, 7.2; cottage cheese, 1.4; and chicken egg, 0.7. The solid filler materials included (wt%) waste paper, 11.0; plastic, 10.6; textiles, 0.7; and wood chips, 5.8. The components were homogenized into fragment of ≤10 mm on an IK-07E fodder shredder (Avtomash, Russia) and mixed thoroughly in a BSE-63 concrete mixing machine (Kalibr, Russia). For each series of experiments, 80 L of solid raw FW were prepared as the substrate for the START experiment. The physicochemical parameters of the substrates (analyzed as described in Section 2.2.3) are presented in Table 1.
Composting was carried out in the pilot-scale test system for 49 days in 8 identical chambers according to the scheme (Figure 1) with a total volume of 200 dm3. For each variant, 2 reaction vessels of 10 dm3 each were designed simultaneously. The test system fully reproduced the conditions of spontaneous temperature change under control of aeration regime and heat loss compensation. The design and performance characteristics of the pilot-scale test system were in accordance with Mironov et al. (2024). The number of repetitions of each variant of the experiments was a minimum of 2 and a maximum of 8 (in the case of the best composition). The dynamics of the biological and physicochemical parameters of the substrate: temperature (T), moisture content, рН, total microbial count (TMC, CFU), germination index (GI), C/N ratio, and organic matter (OM), were analyzed as described in work (Mironov et al., 2021). The content of CO2, NH3, CH4, and H2S was analyzed with a MAG-6C-1 gas analyzer (Eksis, Russia), and volatile organic compounds (VOCs) were analyzed by chromatography combined with vapor preconcentration using the solid phase microextraction (SPME) technique as described (Mironov et al., 2023).
Figure 1. Schematic image of a test (laboratory) setup simulating the conditions of aerobic solid phase biodegradation: chamber (1); reaction vessel (2); temperature sensors (3, 4); temperature meter-regulator (5); gas analyzer (6); computer (7); blower (8); gas sensors (9); sampling port for solid phase micro extraction (SPME) (10); flask (11); heating element (12); rotameter (13); atmospheric air (14); waste air (15).
To calculate TMC, series of 10-fold dilutions were seeded on modified LB agar (g L−1): yeast extract 2.0, peptone 5.0, glucose 1.0, NaCl 1.0, tap water; рН 7.0. The Petri dishes were incubated for 3–4 days at 30 and 55°C. TMC values were obtained by counting the colonies and converting the results to colony forming units per 1 g of substrate (CFU g−1).
For NGS profiling of the microbial community, samples were taken from the reaction vessels inoculated with the effective composition and the uninoculated control at the beginning of composting (initial FW community) and on day 15 of the process. Due to substrate heterogeneity, the material was taken from different parts of the reaction vessel into a sterile Falcon tube and homogenized manually. The composition of the bacterial community was analyzed based on the abundance of 16S rRNA genes. DNA isolation, PCR, and Illumina MiSeq high-throughput sequencing of the 16S rRNA gene region were performed using conventional techniques as described (Mironov et al., 2021). For each sample, DNA isolation was performed without replication; for each DNA sample, library preparation and sequencing were performed in duplicate. All 16S rRNA gene sequence reads were then processed using the SILVAngs 1.3 pipeline (Quast et al., 2013) with default settings: 98% similarity threshold was used to generate operational taxonomic unit (OTU) tables; the minimum similarity to the nearest relative used for classification was 93%.
To evaluate the effectiveness of the inoculation, the dynamics of the principal (CO2 formation) and auxiliary (Tav and NH3 formation) parameters was monitored for 28 days of START and 21 days of RESTART experiments. Gas composition was determined on days 0–1, 4–8, 11–15, 18–22, and 25–28 for START and on days 0–1, 4–8, 11–15, 18–21 for RESTART. All data were the means from at least 2 parallel experiments. Moisture content, pH, OM, C/N ratio, VOCs, and TMC were measured in 2 replicates for each sample. For NGS profiling of the microbial community, samples were collected from 2 parallel experiments. Community composition was determined in four samples of each substrate from 2 parallel experiments.
The distributions of the experimental data on the dynamics of the principal (CO2 emission) and auxiliary (Tav and NH3 emission) criteria were tested for normality using the Shapiro–Wilk test (significance level, 0.05). It was found that most data did not a have a normal distribution. Therefore, the Kruskal–Wallis test (KWt) for 2 or more samples was used as a nonparametric method (Ruxton and Beauchamp, 2008; Chen et al., 2023). For KWt, the null hypothesis is stochastic homogeneity and the alternative hypothesis is stochastic heterogeneity (Ruxton and Beauchamp, 2008). The following assumptions were made: variables are continuous and independent after randomization, distributions in each group have a similar shape.
Results were presented or as means and standard errors of the mean (SE) for CO2, NH3 and Tav and as means and standard deviations (SD) for all other data. Statistical analysis of the data was performed with MS Excel (Microsoft, USA).
Altogether, 75 pure cultures of microorganisms were isolated from the composted waste, including 66 bacterial and 9 fungal cultures. Among them, 26 cultures showed the ability to hydrolyze one or more biopolymers (starch, cellulose, proteins) or lipids. The 10 cultures with the highest hydrolytic activity were selected for 2 bacterial compositions (B1 and B2) and the fungal composition F (Table 2).
Table 2. Characterization and metabolic activity of isolated cultures during degradation of different substrates: ratio between hydrolysis zone diameter and colony diameter (mean ± SD).
Composition B1 included five cultures of the genus Bacillus. It has been repeatedly observed that Bacillus members dominate among easily cultivated microorganisms of composted waste (Blanc et al., 1997; Mironov et al., 2021). These cultures were isolated at the high-temperature stage of composting (45–60°C), but grew actively at 30°C, which indicated their thermotolerance (Moreno et al., 2021). Bacteria of composition B1 were capable of degrading proteins, starch, cellulose, and partially lipids.
Composition B2 was prepared from 2 cultures: Bacillus subtilis and Bacillus amyloliquefaciens. The temperature range of their growth was 20–55°C. Both cultures were capable of hydrolyzing starch and cellulose (Figure 2), but differed in their ability to utilize milk proteins as the only carbon source. In addition, both cultures were incapable of lipid hydrolysis (Figure 2C). Previously, it was found that Bacillus cultures can grow under anaerobic and microaerophilic conditions through mixed acid fermentation (with formation of acetate, lactate, formate, CO2 and other compounds) and anaerobic respiration (nitrate and fumarate) (Hoffmann et al., 1995; Clements et al., 2002).
Figure 2. Metabolic activity of microorganisms (1) Bacillus subtilis and (2) Bacillus amyloliquefaciens of composition B2: (А) proteolytic; (B) amylolytic; (C) lipolytic; (D) cellulolytic.
Fungal composition F included four cultures of the genus Penicillium; 2 cultures were identified at the genus level, and the other 2 were a mix of Penicillium crustosum and Penicillium chrysogenum. These cultures were isolated at high-temperature composting conditions (45–60°C). Under laboratory conditions, they grew at 20–35°C and actively degraded lipids (Tween–80), starch, cellulose, and proteins.
Using the cross-streak method, it was shown that the cultures included in compositions B1, B2, and F did not inhibit each other’s growth. The colony size of the test cultures did not decrease compared to their normal growth (Figure 3).
Figure 3. Compatibility of pure cultures shown by the perpendicular stroke method: (A1–A3) composition B1, (B1) composition B2 and (C1–C2) composition F.
The results of evaluation of different compositions based on the principal (CO2) and auxiliary (NH3, and Tav) criteria are summarized in Table 3.
Table 3. Comparative evaluation of the criteria based on the mass of CO2 and NH3 and the temperature of self-heating Tav during composting (mean ± SE).
The effect of inoculum concentration was assessed using B1 suspensions with total cell titers of 4 × 105 and 108 CFU g−1 FW, which corresponded to the lowest and highest concentrations previously used in inoculation studies (Barrena et al., 2006; Awasthi et al., 2017; Song et al., 2018; Duan et al., 2020; Rastogi et al., 2019). The dynamics of CO2, NH3, and Tav for the START and RESTART variants are shown in Supplementary Figures 2, 3, respectively.
Introduction of composition B1 at a low cell concentration (105 CFU g−1) led to a decrease in biodegradation intensity: CO2 formation in the substrate with B1 (105) was significantly (four-fold) lower than in the control, with no significant differences in NH3 production and Tav. Apparently, at this concentration, the introduced hydrolytic microorganisms had difficulty competing with the original microbial community of the waste.
The use of composition B1 with a concentration of 108 CFU g−1 in the START variant led to a significant decrease (by 10.6°C) in the average temperature over the entire period, without differences in the formation of CO2 and NH3.
Based on the principal and auxiliary criteria, composition B2 consisting of 2 Bacillus cultures exhibited significant advantages over B1 and F when applied in the START variant. Inoculation with composition B2 at 108 CFU g−1 resulted in the highest (2.8-fold) increase in CO2 formation, while maintaining an acceptable level of NH3 formation and the Tav values as in the uninoculated control. Inoculation with B1 and F resulted in a significant decrease in Tav and CO2 production, respectively.
In the RESTART experiments, there was no response to the application of B1. In the case of B2, CO2 production increased by 2.4 times and the autoheating Tav decreased by 2.9°C compared to the control without inoculum, while ammonia emission was similar. Inoculation with composition F increased CO2 emission by 1.8 times, Tav by 5.6°C, and NH3 emission by 3.1 times compared to the control. Thus, B2 and F showed similar positive results in the RESTART variant.
Composition B2 was selected for detailed analysis as the most effective one. Inoculation with B2 led to changes in the principal composting parameters (Figure 4) and in the succession of the bacterial community (Figure 5).
Figure 4. START with B2: profiles of (A) CO2, (В) NH3, (С) temperature, (D) TMC, (E) pH, (F) VOCs total, (G) terpenes, (H) aldehydes, (I) alcohols, (J) aliphatic acids, (K) acetic acid, (L) acetone: τ, composting time; B2, with inoculation; C, control; out, outside temperature; B2-30, С-30, B2-55, С-55, TMC for temperatures 30 and 55°C.
Figure 5. The composition of the bacterial community at the level of genera based on the results of 16S rRNA gene sequencing of microbiota of the substrates (genera representing more than 1% of the community are represented, the remainder are grouped as “other”): Initial community – the initial bacterial community of food waste, С (15 days) and B2 (15 days) are the bacterial communities of the control and inoculated B2 substrates, respectively, on day 15 of composting.
The dynamics of the principal and auxiliary criteria are presented in Figure 4A–C. TMCs decreased significantly on day 3 by about 1.5 orders of magnitude in the control and by 3.0 orders of magnitude in the inoculated substrate (Figure 4D). The decrease in microbial counts was due to a drastic change in the growth conditions at the start of composting, which led to the death of some species and the promoted the growth of others. The TMC decrease was more pronounced in the inoculated sample, because the supplemented Bacillus entered in an active competition with other members of the community, suppressing the development of some groups of microorganisms. Subsequently, TMC values gradually recovered. On days 6–15, TMCs in the control and inoculated substrate remained stable, amounting to 107–108 CFU g−1. Under thermophilic conditions, TMCs increased to ~109 CFU g−1 by day 28, which was close to the initial numbers in the FW substrate.
The dynamics of pH values after B2 inoculation and in the control were similar (Figure 4E). The pH decrease to 4.7–5.3 on days 3 and 9 occurred almost simultaneously. The increase in pH was observed on day 12 in the control and on day 15 in the inoculated variant. At the end of the experiment, pH was close to neutral in both variants.
Gas chromatography of the exhaust air detected a total of 121 VOCs. They were represented by 18–29% ketones (acetone, etc.), 23–26% terpenes (D-limonene, β-pinene, etc.), 7–10% aldehydes (acetaldehyde, propanal, heptaldehyde, etc.), 6% alcohols (methanol, ethanol, isopropanol), 4% amines (ethylamine, etc.), and 2–3% aliphatic acids (acetic, propionic, butyric, etc.). The highest formation of VOCs in variant B2 was observed in the first 2 weeks (Figure 4F). In total, 1.6 times more VOCs were detected from the substrate with B2 inoculum than in the control experiment over a period of 28 days: 390 ± 30 and 251 ± 15 conventional units (CU) × 103, respectively. The maximum VOC formation occurred on day 8 of composting with inoculate B2 and on day 11 in the control, amounting to 33 ± 4 and 14 ± 1 CU × 103, respectively.
The maximum of terpene emission was detected in the exhaust air from B2 on day 22 in the control on day 18: 5.9 ± 0.4 and 5.2 ± 0.7 CU × 103, respectively (Figure 4G). The maximum of aldehydes was detected on day 3, and their total emission was nearly independent of the addition of inoculum B2 (23.1 ± 3.3 and 27.2 ± 4.1 CU × 103) (Figure 4H). Similarly, the formation of amines was not related to the inoculation (for a total of 28 days, 13.5 ± 1.9 vs. 11.1 ± 1.2 CU × 103), with the maximum occurring on day 2.
Acetone (Figure 4L) was detected mainly from day 2 to day 14. Altogether, twice as much acetone was formed in the B2 variant than in the control over 28 days: 111.9 ± 15.9 vs. 55.3 ± 8.7 CU × 103, respectively. Aliphatic C2–C8 acids were mainly detected from day 1 to day 14, and their total amount formed over 28 days was 2.1 times higher in the B2 variant than in the control (10.1 ± 1.3 vs. 4.8 ± 0.8 CU × 103) (Figure 4J). They were mainly represented by acetic, butyric, valerian, and propionic acids. Acetic acid was detected in maximum concentrations from day 5 to day 14 and its total amount in the B2 variant was 2.5 times higher than in the control (1,136 ± 99 vs. 463 ± 40 CU) (Figure 4K).
Alcohols were represented by methanol, ethanol, and isopropanol and were mainly detected from day 2 to day 14 (Figure 4I). In the B2 variant, their levels were 34% higher than in the control (total 24.8 ± 3.9 vs. 18.5 ± 3.1 CU × 103).
The formation of CH4 reached the highest values on day 1 both in the control of 1.2 ± 0.8 vol. % and with the inoculum of 1.7 ± 1.1 vol. %, with average values for the observation period of 0.23 ± 0.06 and 0.33 ± 0.05 vol. %, respectively. Hydrogen sulfide was detected in the exhaust air at relatively low concentrations in different time periods, averaging 0.06 ± 0.02 mg m−3 in the control and 0.29 ± 0.08 mg m−3 in the inoculated compost.
The original waste and the composted material obtained after 28 days of incubation with and without inoculum did not inhibit plant growth and development as assessed by GI: it was 73.7 ± 12.4% for original FW, 116.5 ± 21.2% for the control compost on day 28, and 128.2 ± 18.8% for the B2 compost. OM losses after 28 days of composting were more significant in the substrate with B2 inoculation (38%) than in the control (28%).
On day 15, the relative abundance of the genus Bacillus in FW inoculated with B2 was 21% higher than in FW without inoculation: 54.9 ± 2.9% and 33.8 ± 1.8%, respectively (Figure 5). The increase in the presence of this genus was probably the result of inoculation and subsequent survival of the introduced microorganisms under the composting conditions.
The inoculum concentration significantly affected the composting of FW in the START variant. Application of composition B1 at a higher titer of 108 CFU g−1 enhanced biodegradation (assessed by CO2 emission) in comparison to using a lower titer. Our results are consistent with those from earlier studies: Fang et al. (2019) reported successful inoculation of raw sludge with thermophilic bacteria of the genus Geobacillus with a titer of 106 CFU g−1 and Nakasaki et al. (2013) found that inoculation had a significant accelerating effect on composting when the cell concentration was higher than 105 CFU g−1. In our study, an inoculum with a low concentration of 4 × 105 CFU g−1 had a negative effect on the composting intensity. A similar effect was reported by Ballardo et al. (2017) and Xi et al. (2015), who used multi-stage inoculation to avoid attenuation of the process due to competition. However, Song et al. (2018), managed to accelerate degradation of carbohydrates during FW composting by applying a consortium of autochthonous microorganisms at a concentration of 105 CFU g−1. In our study, the proportions between the cell numbers of the inoculated and autochthonous FW microbiota were 1: 2500 and 1: 10 for inoculation using concentrations of 4 × 105 and 108 CFU g−1, respectively.
In the START variant, inoculation with the bacterial composition B1 at 108 CFU g−1 or the fungal composition F at 104 CFU g−1 resulted in a significant decrease in Tav in the case of B1 and CO2 formation in the case of F. However, the test for antagonism did not detect any growth suppression effects among cultures of composition B1. It is possible that their relationships changed during composting, where the environment is more aggressive, competition is more intense, and various growth factors are limited in comparison to in vitro cultivation conditions, which could diminish the effect of the inoculation. A similar effect was observed by Voběrková et al. (2017) and Xie et al. (2023), when the compound microbial agents were inoculated, CO2 formation was reduced by 20–30%. The fungal composition F, which consisted of four Penicillium members with a pronounced ability to decompose lipids, decelerated FW biodegradation when applied at the beginning of composting. Apparently, these cultures, although isolated under conditions of high-temperature composting, were not active at elevated temperatures during the experiment. It is also possible that the cultures of composition F entered into an antagonistic interaction with the autochthonous microbiota of the substrate, which led to a decrease in the biodegradation rate. Similarly, in the study by Heidarzadeh et al. (2019), introduction of Aspergillus niger at the beginning of composting negatively affected the loss of total organic carbon and temperature.
Inoculation with composition B2 at 108 CFU g−1 at the START stage augmented the intensity of composting in comparison to the control experiment. Composition B2 was represented by 2 cultures of the genus Bacillus with hydrolytic activity against starch, cellulose, and proteins. A 2.8-fold increase in CO2 formation and a 10% increase in OM losses were observed, while NH3 formation levels remained acceptable and Tav was the same as in the uninoculated control. Our results are consistent with the data from Ke et al. (2010) and Nakasaki et al. (2013): it was shown that inoculation increased CO2 formation during composting of both model and real FW. High carbon losses following inoculation with B. subtilis were also observed by Duan et al. (2020). Similar results of high titres were obtained by Zhu et al. (2023), Nakasaki and Hirai (2017) and Zhang et al. (2024), who used inoculants at a concentration of 107–108 CFU g−1 raw waste.
After 28 days, the substrate with B2 was by 11% more effective in stimulating germination of the test plants than the control. This was probably due to a decrease in the level of unstable OM, which inhibits germination. The observed increase in GI and OM uptake following microbial inoculation of FW is consistent with data from Manu et al. (2017) and Li et al. (2020).
Following inoculation in the RESTART variant, positive effects of similar magnitude were observed for 2 compositions: bacterial B2 and fungal F. In the variant with B2, CO2 emission increased 2.4-fold, but at the same time the average temperature decreased by 2.9°C. Inoculation with composition F increased CO2 formation 1.8-fold, Tav by 5.6°C, and ammonia emission 3.1-fold in comparison to the control. Liu et al. (2024) also observed that a microbial agent stimulated NH3 emission at the early stages of composting. A positive effect on waste inoculation with Bacillus cultures after a high-temperature period was also shown by Ballardo et al. (2017). If we compare the START and RESTART variants, the hydrolytic bacteria of composition B2 produced a more pronounced effect when applied at the beginning of the experiment rather than at the cooling stage. Probably, the conditions of pre-composted FW did not correspond to the optimum for their growth, for instance, due to a lower residual content of easily degradable biopolymers (such as starch or pectin). During the 28 days of substrate composting, a considerable portion of easily degradable compounds was consumed, as observed previously (Mironov et al., 2021). The maximum of CO2 formation in observed in RESTART, both in the control and with the inoculum, can be related to the mixing of the substrate, which intensified the hydrolysis of the available biopolymers.
Inoculation with the fungal composition F at RESTART may be more effective because during the late stage of composting fungi are able to degrade water-insoluble biopolymers, while the amounts of more readily available nutrients in FW become limited. The effectiveness of fungal inoculation at the late stages of composting (cooling and maturation) was also demonstrated by Voběrková et al. (2017) and Xi et al. (2015). The observed increase in the average temperature by 5.6°C in the presence of composition F was probably due to the high lipolytic activity of Penicillium sp. Similar results were previously reported by Awasthi et al. (2014) for composting with an initial concentration of 106 CFU g−1 of autochthonous FW microorganisms. Inoculation with Aspergillus and Trichoderma fungi in concentrations similar to ours (104 and 106 spores g−1) led to a significant increase in temperature and enhanced mineralization of the waste. A comparative evaluation of the effect of inoculation in the composting process is presented in Supplementary Table 1.
During the first day of composting, substrate degradation occurred via both the pathways that involved O2 and those that did not. On the one hand, aerobic degradation probably took place in a liquid film on the surface of solid particles with access to oxygen through air pores. On the other hand, fermentation occurred within solid substrate particles in places inaccessible to oxygen diffusion. The microbial community of FW inoculated with B2 was diverse and dynamic.
Food waste has a high content of easily degradable organic compounds. Large amounts of available polysaccharides of plant origin can undergo enzymatic hydrolysis. The original microbial community of FW was dominated (>60%) by lactic acid bacteria (LAB) of the genera Leuconostoc, Lactococcus, and Latilactobacillus. Obviously, on day 1, LAB were active both in the substrate with B2 and in the control, and the main process was monosaccharide fermentation producing lactic acid, ethanol, and CO2.
The observed accumulation of acetic acid under the mixed aerobic-anaerobic conditions was unsurprising, since it is a common product of several bacterial metabolic pathways. In particular, it is produced as a result of mixed acid fermentation by facultatively anaerobic microorganisms, as well as by strictly anaerobic members of the genus Clostridium. During FW composting, acetate is mainly derived from pyruvate generated by glycolysis (Song et al., 2018). Under aerobic conditions, acetic acid may be a product of incomplete oxidation of glucose or ethanol, which is transformed to acetaldehyde and then to acetic acid. In our experiment, significant amounts of alcohols, mainly ethanol, were detected in the exhaust air during the first 2 weeks; their level was by 34% higher in the B2 variant than in the control.
Abundance of easily degradable compounds in the FW substrate together with a high concentration of B2 inoculum supported intense microbial respiratory activity with moderate heat release and pH decrease during the first 10 days. Significant levels of acetic, butyric, isovaleric, and propionic acids were detected in the exhaust air during this period. According to Nakasaki et al. (2013), acetic, propionic, butyric, and lactic acids are the four dominant organic acids that cause pronounced acidification and slow down the composting process. Song et al. (2018) specified that it may be acetic and propionic acids that cause excessive acidification at the initial stage of FW composting.
In addition to these principal pathways, an alternative pathway may have contributed to acetate formation. Won et al. (2021) showed that acetic acid was a major metabolic product in Bacillus spp. growing in the presence of oxygen and with excess of glucose and other easily metabolized carbohydrates. This phenomenon is known as “overflow metabolism” or “the bacterial Crabtree effect”: when glucose uptake in aerobic bacteria exceeds a critical threshold, the carbon flow is directed primarily toward acetate formation. This effect may also occur during composting as a response to substrate excess (Reinhardt, 2002). The phenomenon of overflow metabolism was described for the bacterial consortia of activated sludge flocs from sewage treatment plants, when the specific uptake of glucose exceeded the critical rate corresponding to the maximum respiration rate (Thierie and Penninckx, 2004). Szenk et al. (2017) report the demonstration by Escherichia coli of a similar effect: at high growth rates, it switches from respiration to fermentation. Fermentation processes provide the lowest energy production. Probably related to this is the moderate heating of the B2 substrate with inoculation.
Acetate can also be formed by heterotrophic homoacetogens through carbohydrate fermentation with acetogenesis. Acetate, like other organic acids, is oxidized by microorganisms to carbon dioxide and water. Song et al. (2018) reported that inoculation of FW with a microbial consortium containing members of the genera Bacillus, Lactobacillus, Aeribacillus, and Pseudomonas can increase the diversity of microbes producing enzymes that catalyze the reactions of acetic and propionic acid degradation, as well as the abundance of these key enzymes.
A further group of compounds available at the beginning of composting were proteins. The original FW community had a high proteolytic potential, as demonstrated by active ammonification. Protein hydrolysis produced amino acids, which were further degraded to the end products ammonia, carbon dioxide and water.
The totality of aerobic and anaerobic reactions of OM decomposition at the initial stage of FW composting produced a large amount of carbon dioxide: up to 3.73 g CO2 day−1 kg−1 OM, and an equivalent amount of oxygen was required. Due to spontaneous heat release, the temperature increased from 22 to 30°C, which was optimal for the development of LAB; oxygen consumption and CO2 formation reached the first local maximum. Inoculation with composition B2 resulted in a 2.3-fold increase in CO2 formation and a 56% increase in VOC formation on day 1. The detected VOCs were mainly aliphatic acids, terpenes, alcohols, and amines.
Jakobsen (1992) observed that CO2 and H2O formation can be a limiting factor for composting reactions if their excess is not removed from the substrate at the appropriate rate. If all the CO2 is removed due to air replacement, the reaction proceeds rapidly.
Apparently, under conditions of high moisture content (72.6 wt%) and O2 deficit (~10 vol. %) observed on days 1–3, anaerobic processes accounted for a larger share of OM decomposition reactions, and energy production was moderate. Since the temperature was not high enough, water and CO2 were not completely removed from the substrate with the air flow and reacted in the solution to form carbonic acid. As a consequence, only a part of the emitted CO2 could be detected in the exhaust air. During this period, the balance between O2 absorption and CO2 emission was not observed. According to Li et al. (2020), when moisture content exceeds 70%, it is difficult to maintain air circulation inside the reactor and FW tends to produce acidic decay gas as a result of anaerobic fermentation.
The accumulation of CO2 prevented the dissolution of oxygen, and microbial activity decreased, while acetic and other organic acids were not completely degraded and probably accumulated in the substrate. By day 3, pH decreased to 4.9–5.3 and TMCs also decreased by 103–102 CFU g−1. The decrease was more pronounced in inoculated FW substrates. These results were consistent with observations from previous studies (Sarkar et al., 2010; Mironov et al., 2021).
On days 4 to 6, FW substrates were characterized by relatively low CO2 emissions (1.5–2.2 g CO2 day−1 kg−1 ОМ), moderate temperature (39.2–43.1°C), and acidic pH of 5.1. Low pH values due to organic acid formation were reported previously by Awasthi et al. (2017, 2014) and Manu et al. (2017). Similar temperature dynamics was observed without inoculation (Fang et al., 2019) and after inoculation with Bacillus spp. at the initial composting stage (Li et al., 2020). During this period, NH3 emission from the substrate remained below the detection limit, which could be due to its chemical binding. Ammonia dissolved in water to form ammonium hydroxide or (in the presence of CO2) ammonium carbonate. Organic acids reacted with NH4OH to form ammonium salts.
During days 7–14, the temperature increased from 40 to 60°C. At the same time, the solubility of CO2 and NH3 in water decreased 1.5- to 2-fold, which led to a decrease in the formation of ammonium carbonate. In addition, the gradual increase in temperature first caused carbonic acid decomposition into water and CO2 and then induced ammonium carbonate decomposition into ammonia and CO2. This enhanced the emission of CO2 (to its second local maximum) and NH3 (to a lower extent) from the substrate. Ammonium hydroxide is more resistant to temperature rise than ammonium carbonate, and its accumulation led to gradual alkalinization of the substrate.
During days 10–12, active decomposition of organic acids and their salts, including ammonium, led to an increase in pH values to 7.4–7.6. Our results are consistent with the data of Reinhardt (2002), who showed that temperatures above 50°C were detected only together with a significant decrease of acetic acid concentrations. Apparently, this is why ammonia was detected in the exhaust air from day 12 onwards. Our results agree with previous observations of microbial activity leading to an increase in pH with growing temperature, volatilization of organic acids, and decomposition of organic nitrogen of FW with formation of ammonium (Li et al., 2020).
Interestingly, while inoculation with B2 led to a significant 2.8-fold increase in CO2 emission, the temperature dynamics did not change, and the pH dynamics was similar to the control, except that the acidic period lasted 3 days longer. Our result differs from the data reported by Wang et al. (2024), who observed an increase in temperature of the inoculated compost. However, their composting experiment was started under thermostated conditions at 50°С. While providing an advantage to thermophilic microorganisms, this protocol deviates considerably from the real-life composting process, which usually begins under normal conditions (18–25°C) and sometimes even at low temperatures of 8–12°С. Nevertheless, the authors concluded that the enhancing in composting was due to an autochthonous microbiota enhanced by an exogenous consortium.
Probably, a slowdown in the decomposition of organic acids and moderate heat generation were consequences of insufficient CO2 replacement by O2. The high activity of B2 microorganisms caused oxygen deficiency, which limited the development of aerobes and favored fermentation processes, as evidenced by an increase in the emission of CO2, VOCs, CH4, and H2S without an increase in heat generation. The highest VOC levels in the exhaust air were detected during the first 14–15 days. Furthermore, inoculation with B2 resulted in a 2.5-fold increase in VOC production. This rise was due to an increase in the content of alcohols, terpenes, aliphatic acids, and ketones (acetone) generated during decomposition of plant components of FW.
During the first 2 weeks, there was a pronounced change in the dominant microorganisms of the compost communities. Apparently, LAB were unable to compete with active development of other bacterial genera, mainly Bacillus, which accounted for 54.9% of the total B2 community by day 15. Quantitatively similar results were obtained by Wang et al. (2024) for FW inoculation with commercial Bacillus-containing preparations. In comparison to the control, inoculation with B2 led to a more pronounced increase in the proportion of Bacillus members (54.9 vs. 33.8% in the control by day 15) and promoted the growth of anaerobic hydrolytic bacteria of the genus Tepidimicrobium (4.1 vs. 1.18%) and members of Lactobacillus (1.63 vs. 0.0%). The replacement of Leuconostoc (53.1% of the original community) by Lactobacillus was probably related to the increase in temperature to 60°C, which is consistent with the results reported by Fisgativa et al. (2018).
It is known that acetone, ethanol, acetate, propionate, and butyrate can be generated by bacteria, including Bacillus, Caldibacillus, and Tepidimicrobium spp., through fermentation (Hoffmann et al., 1995; Clements et al., 2002). In particular, high levels of hydrolytic activity were demonstrated for strictly anaerobic bacteria of the genus Tepidimicrobium and facultatively anaerobic members of Caldibacillus (Wushke et al., 2017; Shikata et al., 2018). For example, Tepidimicrobium ferriphilum (order Clostridiales) is a moderately thermophilic anaerobic bacterium with a growth temperature range of 26–62°C and an optimum of 50°C (Slobodkin et al., 2006). The pH range of its growth is 5.5–9.5, with an optimum at 7.5–8.0. The composting conditions in our experiments were similar to these ranges, while Tepidimicrobium and Caldibacillus were represented a significant share of the FW community. Noteworthy, members of the order Clostridiales were previously identified in FW subjected to pretreatment under strict anaerobic conditions (Fisgativa et al., 2018). These results indicate that the microbial community of FW inoculated with composition B2 was oxygen-deficient for at least the first 14 days. Anaerobic conditions can develop even within very small particles, since oxygen has a limited capacity of transferring from the gaseous to the liquid phase (Reinhardt, 2002). The presence of facultative anaerobic bacteria of the genera Lactobacillus and Anoxybacillus in substrate B2 on day 15 of composting also supports this notion.
Limitations: Firstly, we used one aeration rate and did not increase it when using inoculum, which could affect the oxygen availability. Secondly, we used one FW moisture value, which was consistent with real values but higher than the recommended optimum values for composting (60 wt%). Thirdly, the cultures were washed from the medium, thus reducing the potential positive effect on composting of metabolic products accumulated during cultivation. However, our results are based on a large amount of experimental data from a significant number of parallel experiments on non-sterile raw FW of variable composition, which provides solid evidence for their use in composting acceleration.
To sum up, bacterial and fungal compositions from autochthonous cultures can be used together to intensify composting according to their growth characteristics. The composition of bacteria of the genus Bacillus is better introduced into the initial food waste – in a wetter and more rich in easily degradable organics substrate, and the composition of fungi of the genus Penicillium – at the cooling stage for degradation of difficult to degrade compounds.
The introduction of Bacillus at a low cell concentration of 4 × 105 CFU g−1 of FW caused a decrease in the biodegradation rate, which was attributed to the difficulty in overcoming competition with the native waste microbial community.
The use of Bacillus inoculum with a concentration of 108 CFU g−1 FW may be an effective way to increase the degradation rate of raw FW at moderate composting temperatures. A high titer of Bacillus inoculum during composting reduces oxygen availability and changes the degradation scenario of FW toward a higher proportion of anaerobic metabolism. This should be taken into account when using such composts and create conditions where the oxygen diffusion rate is more intense than the microbial activity. This may also be interesting to use as a process preceding anaerobic fermentation and increasing its efficiency, given that hydrolysis may be the limiting stage of fermentation.
Thus, this study expands the understanding of the influence of inoculation parameters and composition of hydrolytically active microbial compositions on the biodegradation of FW during composting. The results can be used for targeted design of microbial compositions.
The datasets presented in this study can be found in NCBI repository under the following accession numbers: Bacillus subtilis BS2022 (PRJNA979896); B. inaquosorum GMPCOW1 (OR166016); B. spizizenii GMPCOW4 (OR187152); B. xiamenensis GMPCOW3 (OR187099); B. velezensis GMPCOW2 (OR185556); B. amyloliquefaciens BAM2022 (PRJNA979896); Penicillium sp. strain GMPCOW5 (OR187303); Penicillium sp. strain GMPCOW6 (OR187306); P. crustosum+P. chrysogenum GMPCOWM1 (PRJNA997142).
VM: Conceptualization, Data curation, Formal analysis, Funding acquisition, Investigation, Methodology, Project administration, Resources, Software, Validation, Visualization, Writing – original draft, Writing – review & editing. VZ: Data curation, Formal analysis, Investigation, Resources, Software, Validation, Writing – original draft, Writing – review & editing. KE: Data curation, Formal analysis, Validation, Investigation, Software, Writing – review & editing. WB: Conceptualization, Investigation, Methodology, Supervision, Writing – original draft, Writing – review & editing.
The author(s) declare that financial support was received for the research, authorship, and/or publication of this article. This article was made with the support of the Ministry of Science and Higher Education of the Russian Federation in accordance with agreement No. 075-15-2022-318, dated 20 April 2022. The grant was provided for state support for the creation and development of a World-class Scientific Center “Agrotechnologies for the Future.”
The authors are grateful to A. Gannesen, A. Vanteeva and E. Bochkova for the assistance with the obtaining isolates. Thank you to all reviewers who participated in the review and to D. Timchenko for linguistic assistance in the preparation of this manuscript.
WB was employed by Woods End Agricultural Institute Inc.
The remaining authors declare that the research was conducted in the absence of any commercial or financial relationships that could be construed as a potential conflict of interest.
All claims expressed in this article are solely those of the authors and do not necessarily represent those of their affiliated organizations, or those of the publisher, the editors and the reviewers. Any product that may be evaluated in this article, or claim that may be made by its manufacturer, is not guaranteed or endorsed by the publisher.
The Supplementary material for this article can be found online at: https://www.frontiersin.org/articles/10.3389/fmicb.2024.1487165/full#supplementary-material
Awasthi, M. K., Pandey, A. K., Khan, J., Bundela, P. S., Wong, J. W., and Selvam, A. (2014). Evaluation of thermophilic fungal consortium for organic municipal solid waste composting. Bioresour. Technol. 168, 214–221. doi: 10.1016/j.biortech.2014.01.048
Awasthi, M. K., Selvam, A., Lai, K. M., and Wong, J. W. C. (2017). Critical evaluation of post-consumption food waste composting employing thermophilic bacterial consortium. Bioresour. Technol. 245, 665–672. doi: 10.1016/j.biortech.2017.09.014
Ballardo, C., Barrena, R., Artola, A., and Sánchez, A. (2017). A novel strategy for producing compost with enhanced biopesticide properties through solid-state fermentation of biowaste and inoculation with Bacillus thuringiensis. Waste Manag. 70, 53–58. doi: 10.1016/j.wasman.2017.09.041
Barrena, R., Pagans, E., Faltys, G., and Sánchez, A. (2006). Effect of inoculation dosing on the composting of source-selected organic fraction of municipal solid wastes. J. Chem. Technol. Biotechnol. 81, 420–425. doi: 10.1002/jctb.1418
Blanc, M., Marilley, L., Beffa, T., and Aragno, M. (1997). Rapid identification of heterotrophic, thermophilic, spore-forming Bacteria isolated from hot composts. Int. J. Syst. Bacteriol. 47, 1246–1248. doi: 10.1099/00207713-47-4-1246
Boulygina, E. S., Kuznetsov, B. B., and Marusina, A. I. (2002). A study of nucleotide sequences of nifH genes of some Methanotrophic Bacteria. Microbiology 71, 425–432. doi: 10.1023/A:1019893526803
Brinton, W. F. (1998). Volatile Organic Acids In Compost: Production and Odorant Aspects. Compost Science and Utilization, 6, 75–82. doi: 10.1080/1065657X.1998.10701912
Camacho, C., Coulouris, G., Avagyan, V., Ma, N., Papadopoulos, J., Bealer, K., et al. (2009). BLAST+: architecture and applications. BMC Bioinformatics 10:421. doi: 10.1186/1471-2105-10-421
Cerda, A., Artola, A., Font, X., Barrena, R., Gea, T., and Sanchez, A. (2018). Composting of food waste: status and challenges. Bioresour. Technol. 248, 57–67. doi: 10.1016/j.biortech.2017.06.133
Chen, Z., Ma, M., Li, T., Wang, H., and Li, C. (2023). Long sequence time-series forecasting with deep learning: A survey. Inf. Fusion 97:101819. doi: 10.1016/j.inffus.2023.101819
Clements, L. D., Miller, B. S., and Streips, U. N. (2002). Comparative growth analysis of the facultative anaerobes Bacillus subtilis, Bacillus licheniformis, and Escherichia coli. Syst. Appl. Microbiol. 25, 284–286. doi: 10.1078/0723-2020-00108
Droffner, M. L., Brinton, W. F., and Evans, E. (1995). Evidence for the prominence of well characterized mesophilic bacteria in thermophilic (50–70°C) composting environments. Biomass Bioenergy 8, 191–195. doi: 10.1016/0961-9534(95)00002-O
Duan, M., Zhang, Y., Zhou, B., Qin, Z., Wu, J., Wang, Q., et al. (2020). Effects of Bacillus subtilis on carbon components and microbial functional metabolism during cow manure–straw composting. Bioresour. Technol. 303:122868. doi: 10.1016/j.biortech.2020.122868
Egorov, N. S. (2004). Osnovy uchenija Ob antibiotikax [fundamentals of the doctrine of antibiotics]. Moscow: Moscow State University Publishing House.
Fan, Y. V., Klemeš, J. J., Lee, C. T., and Ho, C. S. (2018). Efficiency of microbial inoculation for a cleaner composting technology. Clean Techn. Environ. Policy 20, 517–527. doi: 10.1007/s10098-017-1439-5
Fang, Y., Jia, X., Chen, L., Lin, C., Zhang, H., and Chen, J. (2019). Effect of thermotolerant bacterial inoculation on the microbial community during sludge composting. Can. J. Microbiol. 65, 750–761. doi: 10.1139/cjm-2019-0107
Fisgativa, H., Tremier, A., Saoudi, M., Roux, S. L., and Dabert, P. (2018). Biochemical and microbial changes reveal how aerobic pre-treatment impacts anaerobic biodegradability of food waste. Waste Manag. 80, 119–129. doi: 10.1016/j.wasman.2018.09.011
Hankin, L., and Anagnostakis, S. L. (1975). The use of solid media for detection of enzyme production by fungi. Mycologia 67, 597–607. doi: 10.1080/00275514.1975.12019782
Hankin, L., Zucker, M., and Sands, D. C. (1971). Improved solid medium for the detection and enumeration of pectolytic bacteria. Appl. Environm. Microbiol. 22, 205–209. doi: 10.1128/am.22.2.205-209.1971
Heidarzadeh, M. H., Amani, H., and Javadian, B. (2019). Improving municipal solid waste compost process by cycle time reduction through inoculation of aspergillus Niger. J. Environ. Health Sci. Eng. 17, 295–303. doi: 10.1007/s40201-019-00348-z
Hoffmann, T., Troup, B., Szabo, A., Hungerer, C., and Jahn, D. (1995). The anaerobic life of Bacillus subtilis: cloning of the genes encoding the respiratory nitrate reductase system. FEMS Microbiol. Lett. 131, 219–225. doi: 10.1111/j.1574-6968.1995.tb07780.x
Jakobsen, S. T. (1992). Chemical reactions and air change during decomposition of organic matters. Resour. Conserv. Recycl. 6, 259–266. doi: 10.1016/0921-3449(92)90035-Z
Kausar, H., Sariah, M., Mohd Saud, H., Zahangir Alam, M., and Razi Ismail, M. (2011). Isolation and screening of potential actinobacteria for rapid composting of rice straw. Biodegradation 22, 367–375. doi: 10.1007/s10532-010-9407-3
Ke, G. R., Lai, C. M., Liu, Y. Y., and Yang, S. S. (2010). Inoculation of food waste with the thermo-tolerant lipolytic actinomycete Thermoactinomyces vulgaris A31 and maturity evaluation of the compost. Bioresour. Technol. 101, 7424–7431. doi: 10.1016/j.biortech.2010.04.051
Lane, D. J. (1991). “16S/23S rRNA Sequencing” in Nucleic acid techniques in bacterial systematic. eds. E. Stackebrandt and M. Goodfellow (New York: John Wiley and Sons), 115–175.
Lertcanawanichakul, M., and Sawangnop, S. (2008). A comparison of two methods used for measuring the antagonistic activity of Bacillus species. Walailak J. Sci. Tech. 5, 161–171. doi: 10.2004/wjst.v5i2.86
Li, Y., Chen, Z., Peng, Y., Zheng, K., Ye, C., Wan, K., et al. (2020). Changes in aerobic fermentation and microbial community structure in food waste derived from different dietary regimes. Bioresour. Technol. 317:123948. doi: 10.1016/j.biortech.2020.123948
Liu, N., Liu, Z., Wang, K., Zhao, J., Fang, J., Liu, G., et al. (2024). Comparison analysis of microbial agent and different compost material on microbial community and nitrogen transformation genes dynamic changes during pig manure compost. Bioresour. Technol. 395:130359. doi: 10.1016/j.biortech.2024.130359
Manu, M. K., Kumar, R., and Garg, A. (2017). Performance assessment of improved composting system for food waste with varying aeration and use of microbial inoculum. Bioresour. Technol. 234, 167–177. doi: 10.1016/j.biortech.2017.03.023
Mironov, V., Trofimchuk, E., and Plutalova, A. (2024). Degradation of high concentrations of commercial polylactide packaging on food waste composting in pilot-scale test. Bioresour. Technol. 410:131288. doi: 10.1016/j.biortech.2024.131288
Mironov, V., Vanteeva, A., and Merkel, A. (2021). Microbiological activity during co-composting of food and agricultural waste for soil amendment. Agronomy 11, 928–950. doi: 10.3390/agronomy11050928
Mironov, V., Zhukov, V., Moldon, I., Zagustina, N., Shchelushkina, A., Ostrikova, V., et al. (2023). Pollutant emissions from municipal biowaste composting: comparative analysis and contribution of N-containing organic compounds. Energies 16:7271. doi: 10.3390/en16217271
Moreno, J., López-González, J. A., Arcos-Nievas, M. A., Suárez-Estrella, F., Jurado, M. M., Estrella-González, M. J., et al. (2021). Revisiting the succession of microbial populations throughout composting: A matter of thermotolerance. Sci. Total Environ. 773:145587. doi: 10.1016/j.scitotenv.2021.145587
Nakasaki, K., Araya, S., and Mimoto, H. (2013). Inoculation of Pichia kudriavzevii RB1 degrades the organic acids present in raw compost material and accelerates composting. Bioresour. Technol. 144, 521–528. doi: 10.1016/j.biortech.2013.07.005
Nakasaki, K., and Hirai, H. (2017). Temperature control strategy to enhance the activity of yeast inoculated into compost raw material for accelerated composting. Waste Manag. 65, 29–36. doi: 10.1016/j.wasman.2017.04.019
Parfitt, J., Barthel, M., and Macnaughton, S. (2010). Food waste within food supply chains: quantification and potential for change to 2050. Philos. Trans. R. Soc. B 365, 3065–3081. doi: 10.1098/rstb.2010.0126
Quast, C., Pruesse, E., Yilmaz, P., Gerken, J., Schweer, T., Yarza, P., et al. (2013). The SILVA ribosomal RNA gene database project: improved data processing and web-based tools. Nucleic Acids Res. 41, D590–D596. doi: 10.1093/nar/gks1219
Rastogi, M., Nandal, M., and Nain, L. (2019). Additive effect of cow dung slurry and cellulolytic bacterial inoculation on humic fractions during composting of municipal solid waste. Int. J. Recycl. Org. Waste Agric. 8, 325–332. doi: 10.1007/s40093-019-0277-3
Reinhardt, T. (2002). “Organic acids as a decisive limitation to process dynamics during composting of organic matter” in Microbiology of composting. eds. H. Insam, N. Riddech, and S. Klammer (Berlin. Heidelberg: Springer).
Ruxton, G. D., and Beauchamp, G. (2008). Some suggestions about appropriate use of the Kruskal–Wallis test. Anim. Behav. 76, 1083–1087. doi: 10.1016/j.anbehav.2008.04.011
Sánchez-García, M., Alburquerque, J. A., Sánchez-Monedero, M. A., Roig, A., and Cayuela, M. L. (2015). Biochar accelerates organic matter degradation and enhances N mineralisation during composting of poultry manure without a relevant impact on gas emissions. Bioresour. Technol. 192, 272–279. doi: 10.1016/j.biortech.2015.05.003
Sanger, F., Nicklen, S., and Coulson, A. R. (1977). DNA sequencing with chain-terminating inhibitors. Proc. Natl. Acad. Sci. USA 84, 5463–5467. doi: 10.1073/pnas.74.12.546
Sarkar, S., Banerjee, R., Chanda, S., Das, P., Ganguly, S., and Pal, S. (2010). Effectiveness of inoculation with isolated Geobacillus strains in the thermophilic stage of vegetable waste composting. Bioresour. Technol. 101, 2892–2895. doi: 10.1016/j.biortech.2009.11.095
Shikata, A., Sermsathanaswadi, J., Thianheng, P., Baramee, S., Tachaapaikoon, C., Waeonukul, R., et al. (2018). Characterization of an anaerobic, thermophilic, Alkaliphilic, high lignocellulosic biomass-degrading bacterial community, ISHI-3, isolated from biocompost. Enzym. Microb. Technol. 118, 66–75. doi: 10.1016/j.enzmictec.2018.07.001
Slobodkin, A. I., Tourova, T. P., Kostrikina, N. A., Lysenko, A. M., German, K. E., Bonch-Osmolovskaya, E. A., et al. (2006). Tepidimicrobium ferriphilum gen. Nov., sp. nov., a novel moderately thermophilic, Fe(III)-reducing bacterium of the order Clostridiales. Int. J. Syst. Evol. Microbiol. 56, 369–372. doi: 10.1099/ijs.0.63694-0
Song, C., Li, M., Qi, H., Zhang, Y., Liu, D., Xia, X., et al. (2018). Impact of anti-acidification microbial consortium on carbohydrate metabolism of key microbes during food waste composting. Bioresour. Technol. 259, 1–9. doi: 10.1016/j.biortech.2018.03.022
Szenk, M., Dill, K. A., and de Graff, A. M. R. (2017). Why do fast-growing Bacteria enter overflow metabolism? Testing the membrane real estate hypothesis. Cell Syst. 5, 95–104. doi: 10.1016/j.cels.2017.06.005
Thierie, J., and Penninckx, M. J. (2004). Possible occurrence of a Crabtree effect in the production of lactic and butyric acids by a floc-forming bacterial consortium. Curr. Microbiol. 48, 224–229. doi: 10.1007/s00284-003-4169-3
Voběrková, S., Vaverková, M. D., Burešová, A., Adamcová, D., Vršanská, M., Kynický, J., et al. (2017). Effect of inoculation with white-rot fungi and fungal consortium on the composting efficiency of municipal solid waste. Waste Manag. 61, 157–164. doi: 10.1016/j.wasman.2016.12.039
Wang, Q., Li, N., Jiang, S., Li, G., Yuan, J., Li, Y., et al. (2024). Composting of post-consumption food waste enhanced by bioaugmentation with microbial consortium. Sci. Total Environ. 907:168107. doi: 10.1016/j.scitotenv.2023.168107
White, T. J., Bruns, T., Lee, S., and Taylor, J. (1990). “Amplification and direct sequencing of fungal ribosomal RNA genes for phylogenetics” in PCR protocols: a guide to methods and applications. eds. M. A. Innis, D. H. Gelfand, J. J. Sninsky, and T. J. White (Cambridge: Academic Press).
Won, H. I., Watson, S. M., Ahn, J.-S., Endres, J. L., Bayles, K. W., and Sadykov, M. R. (2021). Inactivation of the Pta-AckA pathway impairs fitness of Bacillus anthracis during overflow metabolism. J. Bacteriol. 203:9. doi: 10.1128/jb.00660-20
Wushke, S., Spicer, V., Zhang, X. L., Fristensky, B., Krokhin, O. V., Levin, D. B., et al. (2017). Understanding aerobic/anaerobic metabolism in Caldibacillus debilis through a comparison with model organisms. Syst. Appl. Microbiol. 40, 245–253. doi: 10.1016/j.syapm.2017.03.004
Xi, B., He, X., Dang, Q., Yang, T., Li, M., Wang, X., et al. (2015). Effect of multi-stage inoculation on the bacterial and fungal community structure during organic municipal solid wastes composting. Bioresour. Technol. 196, 399–405. doi: 10.1016/j.biortech.2015.07.069
Xie, T., Zhang, Z., Zhang, D., Wei, C., Lin, Y., Feng, R., et al. (2023). Effect of hydrothermal pretreatment and compound microbial agents on compost maturity and gaseous emissions during aerobic composting of kitchen waste. Sci. Total Environ. 854:158712. doi: 10.1016/j.scitotenv.2022.158712
Zhang, X., Zhang, D., Yan, Y., Wang, R., Chi, Y., Zhang, D., et al. (2024). Enhancing aerobic composting performance of high-salt oily food waste with Bacillus safensis YM1. Bioresour. Technol. 397:130475. doi: 10.1016/j.biortech.2024.130475
Keywords: hydrolytic microorganisms, composting, anaerobic metabolism, Bacillus, Penicillium
Citation: Mironov V, Zhukov V, Efremova K and Brinton WF (2024) Enhancing aerobic composting of food waste by adding hydrolytically active microorganisms. Front. Microbiol. 15:1487165. doi: 10.3389/fmicb.2024.1487165
Received: 27 August 2024; Accepted: 31 October 2024;
Published: 02 December 2024.
Edited by:
Keli Zhao, Zhejiang Agriculture and Forestry University, ChinaCopyright © 2024 Mironov, Zhukov, Efremova and Brinton. This is an open-access article distributed under the terms of the Creative Commons Attribution License (CC BY). The use, distribution or reproduction in other forums is permitted, provided the original author(s) and the copyright owner(s) are credited and that the original publication in this journal is cited, in accordance with accepted academic practice. No use, distribution or reproduction is permitted which does not comply with these terms.
*Correspondence: Vladimir Mironov, NzM5MDUzMEBnbWFpbC5jb20=
Disclaimer: All claims expressed in this article are solely those of the authors and do not necessarily represent those of their affiliated organizations, or those of the publisher, the editors and the reviewers. Any product that may be evaluated in this article or claim that may be made by its manufacturer is not guaranteed or endorsed by the publisher.
Research integrity at Frontiers
Learn more about the work of our research integrity team to safeguard the quality of each article we publish.