- 1Fishery Institute of Anhui Academy of Agricultural Sciences, Hefei, China
- 2Key Laboratory of Aquaculture & Stock Enhancement in Anhui Province, Hefei, China
Cyanobacterial blooms (CBs) present significant challenges to Chinese mitten crab (CMC) culture, posing hazards to the aquatic microbial ecology. However, the current focus on the microbial ecological changes within the CMC culture system under the influence of CBs is somewhat insufficient. There’s an urgent need to analyze the microbial ecosystem of the CMC culture system under CBs. This study employed 16S rRNA gene amplicon sequencing to investigate the dynamics of the environmental microbial community in both the rice-crab co-culture (RC) and crab monoculture (CM) models. The results revealed that cyanobacteria reached high levels in the CM water in July, while they began to increase in the RC water in August. Notably, OTU147 (uncultured bacterium g_Planktothrix NIVA-CYA 15), identified as the dominant taxon associated with CBs, showed a significant linear relationship with TP, NO2−-N, and the N:P ratio. TP, TN, NO2−-N, and CODMn had a more pronounced impact on the structure of bacterial communities and cyanobacterial taxa in the water. The bacterial community structure involved in carbon metabolism displayed temporal succession in the water. The co-occurrence network of the bacterial community primarily consisted of Chloroflexi, Proteobacteria, and Firnicutes in the sediment, and Actinobacteria, Proteobacteria, Chloroflexi, and Bacteroidota in the water. In contrast, the co-occurrence network included different peripheral species in the sediment and water. Keystone species were predominantly represented by OTU22 (uncultured actinobacterium g_ hgcI clade) and OTU12 (uncultured Opitutae bacterium g_ norank) in the RC water, and by OTU25 (uncultured bacterium g_ Limnohabitans) in the CM water. TP, TN, NO2−-N, and CODMn were identified as the primary environmental factors influencing these keystone taxa within the culture water. In conclusion, this study on the microbial ecology of the CMC culture system under the influence of CBs provides valuable insights that can be instrumental in subsequent management efforts.
Introduction
The Chinese mitten crab (Eriocheir sinensis, CMC) is a vital economic species in China, with an annual output that has ranged between 700,000 and 800,000 tons in recent years. However, challenges including extreme weather, eutrophication of culture water, and the decay of aquatic plants have led to frequent occurrences of cyanobacterial blooms (CBs) within the CMC culture system. The state of the culture environment is critical for the successful progress of CMC culture, and CBs pose a significant threat to the industry’s development. Microbial communities, which can rapidly respond to environmental changes, play a pivotal role in predicting and characterizing aquaculture diseases and the quality of culture systems (Zhou et al., 2012; Xiong et al., 2014, 2015; Huang et al., 2018). For instance, Wang et al. (2020) found that T-2 toxin challenge increased pathogenic bacteria of the gut microbiota and might induce disruption of microbiota balance in CMCs. The occurrence of CBs can trigger variations in the bacterial community structure of the water, highlighting the microbial community’s role as a bridge connecting the environment and aquatic animals (Huang et al., 2018). Investigating changes in the microbial community in the CMC culture system will enhance our ability to conduct aquaculture activities successfully.
CBs can induce dynamic changes in both benthic and planktonic microbial communities in the aquatic environment. Understanding how microbial communities respond to the emergence and dissipation of CBs is crucial for preventing and managing these blooms and mitigating the associated risks. Currently, there is limited research on how microorganisms involved in environmental material cycling and energy flow within CMC culture ponds respond to CBs. Most research has focused on characterizing changes in the composition and structure of the microbial community within the CMC culture environment during CB events, such as significant alterations in the water’s bacterial community structure at different stages of CBs (Zhu et al., 2019). Several environmental factors significantly influence cyanobacterial dynamics in CMC culture environments. Excessive nutrient levels, particularly nitrogen and phosphorus, promote cyanobacterial growth, and higher temperatures accelerate their proliferation. These environmental factors do not act in isolation; they interact and collectively affect cyanobacterial bloom formation in crab culture environments. For instance, research by Li et al. (2019) demonstrated that the N:P ratio, water temperature (WT), and total phosphorus (TP) were key environmental drivers influencing the complex development of cyanobacterial communities in crab ponds in Lake Guchenghu, China. It is essential to further analyze how the bacterial community in the CMC culture system responds to changes induced by CBs. Such analysis will facilitate the development of strategies to prevent and mitigate the harmful effects of CBs.
Microbial communities in the natural environment do not exist independently; instead, they form complex networks through predation, competition, and mutualistic symbiosis to maintain the stability of their structure and function (Faust and Raes, 2012). The importance of maintaining microbial community networks in aquatic environments is self-evident. Co-occurrence network analysis has been used to explain microbial community interactions, and related studies have found that keystone species contribute to regulating the microbial community structure and function (Ma et al., 2016). For example, when compared to irrigation canals, sediment microbial communities in aquatic ponds exhibit more stable interactions, with microorganisms of relatively low relative abundances potentially acting as key players in sediment ecosystems (Xu et al., 2022). Microbial communities in different types of CMC culture systems respond differently to cyanobacteria, and various CBs determine which microorganisms survive, establish specific microbial metabolic pathways, and influence their activities (Li et al., 2019; Ma et al., 2021). Therefore, our objective is to gain insights into the associations between microbial communities in different types of systems and their network co-occurrence patterns under CB conditions, aiming to elucidate the co-occurrence patterns of microbial communities and identify keystone species within these ecosystems.
In this study, the impact of CB on bacterial communities in both the water and sediment of CMC culture systems, specifically the rice-crab co-culture and crab monoculture models, was assessed using 16S rRNA gene amplification and sequencing. The primary objectives were to: (1) Investigate the alterations in the composition of microbial communities in both the water and sediment of culture environments under the influence of CB. (2) Characterize the differentiation of bacterial communities resulting from physicochemical factors between different CMC culture modes under CB. (3) Examine the variations in carbon metabolism within microbial communities in both the water and sediment under CB. (4) Compare the differences in microbial co-occurrence patterns between the two types of culture environments under the influence of CB.
Materials and methods
Experimental materials
This experiment was conducted in the Tengfei Aquatic Products Professional Cooperative Juvenile Crab Cultivation Core Demonstration Area, located in the Xianzhou district of Xuancheng, Anhui province, China (latitude: 118°45’ N, longitude: 31°11’ S). The experiment was divided into two models: Rice-crab co-culture (RC) and crab monoculture models (CM).
Basic information
In the experimental ponds, there had been CBs occurrence in the past years, and it is well known that the probability of CB occurrence is very high in these ponds. Under this background, we carried out RC in three of these ponds and CM in the adjacent three ponds. CBs in the CM system occurred in July, while in the RC system occurred in August. The whole sampling process can also be divided into non-BC outbreak phase, BC outbreak phase and BC recession phase according to the bloom process of OTU 147 (uncultured bacterium g_Planktothrix NIVA-CYA 15) (Supplementary Figure S2C). Each pond was covered an area of approximately 1,334 m2. On March 5, 2022, Alternanthera philoxeroides was planted throughout the entire pond in the CM model, whereas in the RC model, it was only planted in the peripheral trench. On May 7, 2022, rice (Brand: Youliangyou 2,152) was transplanted using rice planting technology in the RC group. Megalopa larvae were released after the rice transplant in both modes, with each field stocked with 2.4 × 105 megalopa, each averaging about 0.008 g in weight. The rice was harvested at the end of October. CMCs were harvested in February of the following year. The average yield of RC was 146.33 ± 4.02 kg/ 667 m2, and that of CM was 165.33 ± 3.63 kg/667 m2.
Sample collection
Sediment (S) and water (W) samples were collected from June to mid-November, excluding October, for subsequent monitoring. Sampling locations for water or sediment samples of each time point were repetition in each pond. Physicochemical factors of the culture water were continuously monitored. One liter of water from the middle layer of the pond was collected using a diagonal two-point method. Subsequently, microorganisms in the water were captured after passing through a 2 μm filter membrane. These microorganisms were then placed in sterilized centrifuge tubes, stored in refrigerators at −80°C for subsequent DNA extraction, and finally used for 16S rRNA gene sequencing analysis. Sediment samples, with a surface layer height of 5 cm, were collected using the diagonal two-point method. These samples were placed in sterilized centrifuge tubes and stored in a refrigerator at −80°C for subsequent DNA extraction for 16S rRNA gene sequencing analysis and heavy metal index detection.
The water quality index detection methods are as follows: Ammonia nitrogen (NH4+-N) was detected using Nessler reagent spectrophotometry. Nitrite nitrogen (NO2−-N) was detected using spectrophotometry. Nitrate nitrogen (NO3−-N) was detected using ultraviolet spectrophotometry. Soluble orthophosphate (TP) was detected using molybdenum antimony spectrophotometry. Total nitrogen (TN) was determined using ultraviolet spectrophotometry after digestion with alkaline potassium persulfate. Total phosphorus was detected using ammonium molybdate spectrophotometry. Chemical oxygen demand (CODMn) was determined using permanganate as an oxidant.
The methods for detecting heavy metals, pH, and organic matter in sediment are as follows: Arsenic (As) content was tested in accordance with the DZ/T0279.13–2016 standard. Mercury (Hg) content was tested following the DZ/T0279.17–2016 standard. Organic matter content (TOC) was determined based on the DZ/T0279.27–2016 standard. pH was tested as per the DZ/T0279.34–2016 standard. Cadmium (Cd) content was tested following the DZ/T0279.5–2016 standard. Chromium (Cr), Copper (Cu), Nickel (Ni), Lead (Pb), and Zinc (Zn) contents were analyzed in accordance with the HJ780-2015 standard.
DNA extraction and high-throughput sequencing
The SDS method was employed to extract total DNA from water and sediment samples. DNA quality and concentration were assessed using a Nanodrop ND-2000 (Nano Drop Technologies, United States). For amplification of the V3-V4 region of the 16S rRNA gene, primer sets 341F (5’-CCTAYGGGRBGCASCAG-3′) and 806R (5’-GGACTNNGGGTATCTAAT-3′) were selected. The amplification was performed using a Bio-Rad T 100 thermocycler (Bio-Rad, United States). The PCR product of each sample was purified using a PCR fragment purification kit (Thermo Scientific, United States), and the concentration of the purified PCR product was measured with an Agilent 2,100 bioanalyzer (Agilent, United States). A library was created by pooling equimolar amounts of PCR amplicons from each sample using the TruSeq DNA PCR-Free Library Preparation Kit (Illumina, United States). The library was quantified using a Qubit 2.0 fluorometer (Life Technologies, United States) and sequenced using the NovaSeq 6,000 system (Illumina, United States). The sequencing data have been deposited as accession number PRJNA1030144 in the NCBI BioProject database.
The microbial community analysis
The Similarity Percentages (SIMPER) method was utilized to analyze the primary differences in bacterial community composition by using PAST4 software. To investigate the impact of physicochemical factors on the bacterial community structure in both sediment and water, we employed the vegan package within the R environment for Redundancy Analysis (RDA). Furthermore, we used the varpart function (VPA) from the “vegan” package in the R environment to assess the importance of environmental variables throughout the entire culture process.
Phylogenetic investigation of communities by reconstruction of unobserved states (PICRUSt2)1 was used to predict the carbon metabolism function of bacterial community in the water, and further analyzed by comparing with KEGG orthology. To assess structural changes in gene functions related to carbon metabolism, we utilized Non-Metric Multidimensional Scaling (NMDS). Additionally, we used Analysis of Similarities (ANOSIM) to evaluate structural differences in gene functions related to carbon metabolism at adjacent CB stages.
To explore the differences in co-occurrence patterns of bacterial communities under different culture modes, spearman correlation was employed to examine the relationships between OTUs in sediment and water, respectively. We applied Spearman correlations to filter nodes and edges with significant associations using the “Hmisc” package (Harrell, 2019) within the R environment. To calculate network topology parameters, including nodes, edges, clustering coefficient, average path length, degree, closeness centrality, betweenness centrality, and eigencentrality, we used Gephiv 0.9.2. Furthermore, to analyze the differences in node topology parameters within bacterial community networks of the two different culture modes, we employed the Wilcox.test in the “ggsigif” package (Ahlmann-Eltze and Patil, 2021) in the R environment.
To further explore the keystone species within the microbial community in both sediment and water, we calculated the within-module connectivity (Zi) and inter-module connectivity (Pi) of the bacterial community in sediment and water using the “microeco” package in the R environment (Liu et al., 2021). We performed Spearman analysis using the “corrplot” package (Wei et al., 2017) to assess the physicochemical factors that impact these keystone species.
Results
Bacterial community succession characteristics under different CMC culture modes
At the phylum level, the bacterial communities in the sediment under both culture modes were primarily composed of Chloroflexi (CM: 27.13% vs. RC: 23.52%), Proteobacteria (13.21% vs. 14.86%), and Firmicutes (CM: 11.53% vs. RC: 12.56%) (Figure 1A). In contrast, the bacterial communities in the water were primarily composed of Acidobacteriota (CM: 27.90% vs. RC: 33.06%), Proteobacteria (CM: 21.86% vs. RC: 27.74%), and Cyanobacteria (CM: 29.30% vs. RC: 13.04%) (Figure 1B). Notably, the abundance of Cyanobacteria reached a peak of 52.91% in the CM system in July, while it began to increase in the RC system in August, with a relative abundance of 47.87% (Figure 1B). At the genus level, the bacterial communities in the sediment were predominantly composed of norank Anaerolineaceae (CM: 10.42% vs. RC: 8.67%), Bacillus (CM: 3.04% vs. RC: 3.41%), and norank Vicinamibacterales (CM: 2.89% vs. RC: 3.08%) (Supplementary Figure S1). Their composition remained relatively stable over time, and their relative abundances were similar between the two culture modes (Supplementary Figure S1). In contrast, the bacterial communities in the water were mainly composed of Planktothrix NIVA.CYA 15 (CM: 22.56% vs. RC: 10.35%), hgcI clade (CM: 6.57% vs. RC: 6.15%), Mycobacterium (CM: 4.26% vs. RC: 7.03%), and Rhodoluna (CM: 1.81% vs. RC: 5.93%) (Supplementary Figure S1).
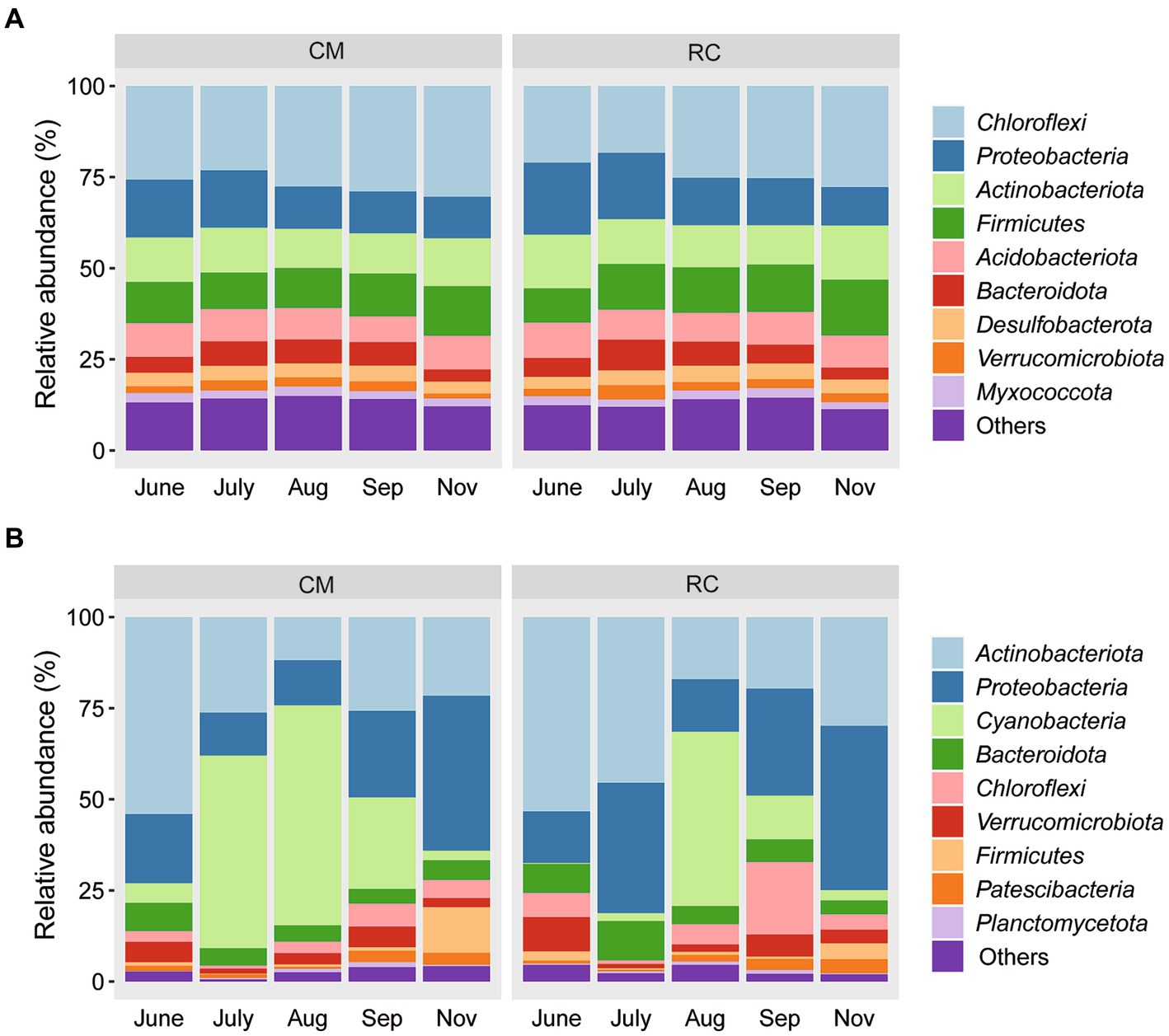
Figure 1. The composition of bacterial communities in the CMC culture environment at phylum level. (A) Sediment. (B) Water.
According to the SIMPER analysis, 12 OTUs, including OTU685 (Bacillus selenatarsenatis), OTU374 (unclassified f_Nocardioidaceae), and OTU2690 (uncultured gamma proteobacterium g_norank f_Steroidobacteriaceae), were identified as the primary bacterial communities responsible for the differences between the two sediment modes (Figure 2A). Additionally, eight OTUs, such as OTU147 (uncultured bacterium g_Planktothrix NIVA-CYA 15), OTU5 (uncultured bacterium g_Rhodoluna), and OTU1 (uncultured Canditatus Planktophila sp.), were the main bacterial taxa contributing to the distinctions between the two water modes (Figure 2B).
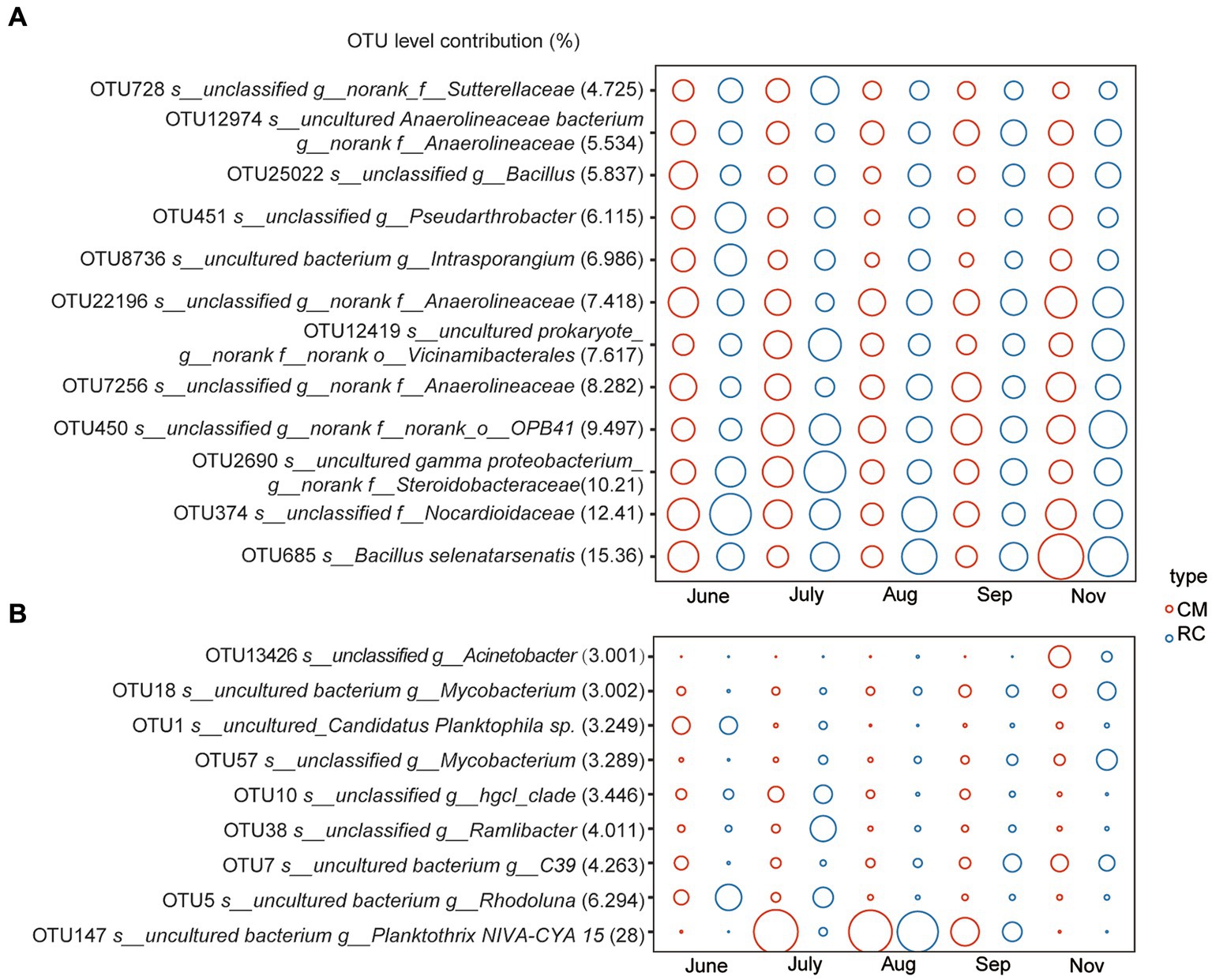
Figure 2. Similarity of bacteria community between RC and CM culture modes based on SIMPER analysis. (A) Sediment. (B) Water.
Physicochemical factor driven variation of bacterial communities under different CMC culture modes
TOC, Cd, Cr, Cu, Ni, and Zn exhibited a significant impact on the structure of sediment bacterial communities. In contrast, TP, TN, NO2−-N, and CODMn had a more pronounced influence on the structure of bacterial communities in the water (Figure 3). Further analysis revealed that TP, NO2−-N, and CODMn also had a significant impact on cyanobacterial taxa (Figure 3). When assessing the variation in sediment bacterial communities, it was found that heavy metals and TOC together explained 8.94% of the variance, with heavy metals accounting for 7.4% and TOC contributing 1.54% in the VPA analysis (Figure 3). On the other hand, the explanatory power of TP on the variation of water bacterial communities was notably high, at 21.61%, surpassing the impact of other physicochemical factors and CODMn (Figure 3A). The cumulative explanatory effect of these physicochemical factors on cyanobacterial taxa in the water amounted to 29.09%, with N-related physicochemical factors explaining 7.24% and CODMn contributing 1.10% (Supplementary Figure S2). Furthermore, a linear relationship was observed between OTU147 (uncultured bacterium g_Planktothrix NIVA-CYA 15) and TP, NO2−-N, and the N:P ratio (Supplementary Figure S2).
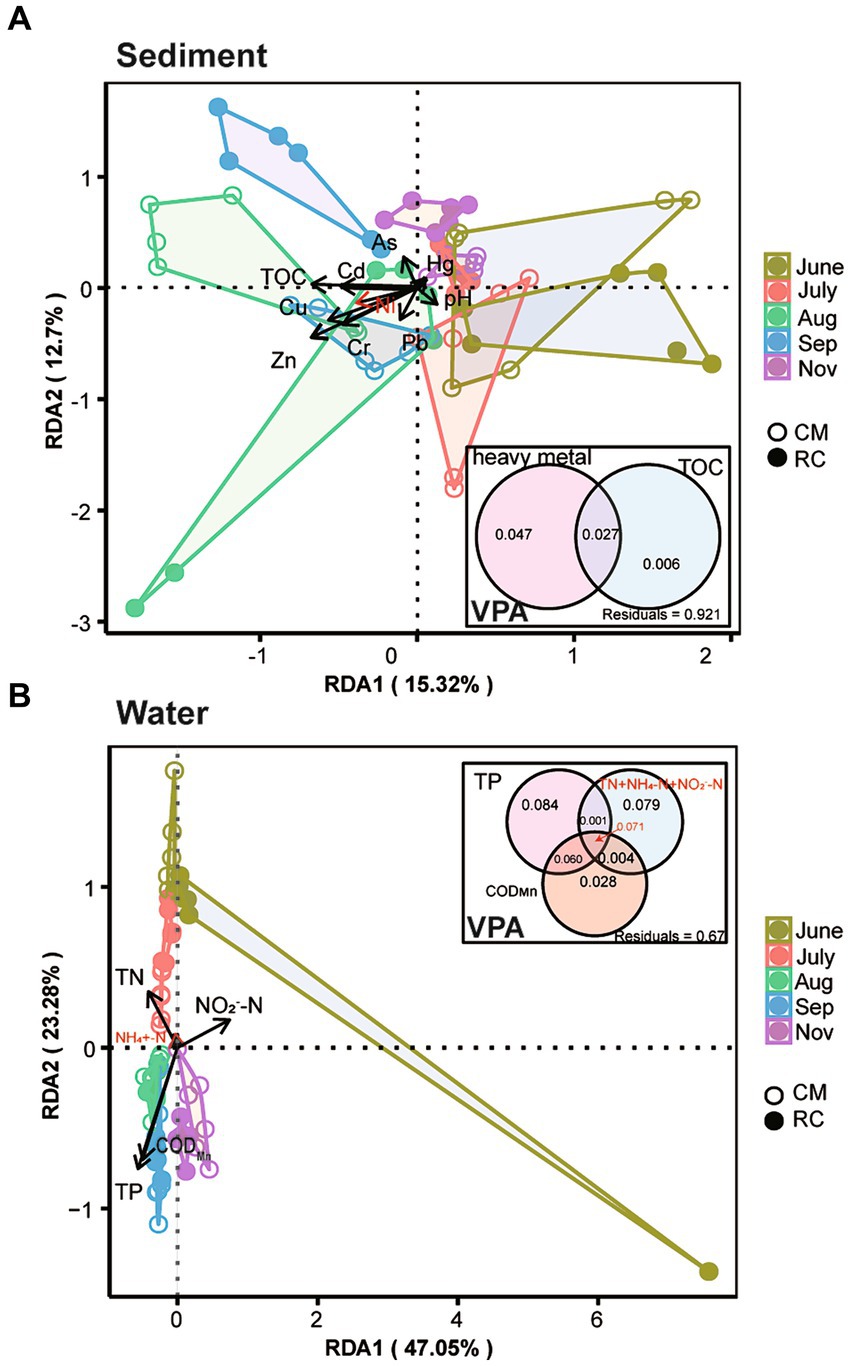
Figure 3. Impact of environmental factors on the bacterial community structure of the CMC culture environment based on RDA and VPA analysis. (A) Sediment. (B) Water.
Differences of carbon metabolic of bacterial communities under the two culture modes
Using PICRUSt2, a total of 284 genes related to carbon metabolic functions were identified within the bacterial communities of sediments throughout the entire process. Additionally, there were 278 genes associated with carbon metabolic functions within the bacterial communities of water. In total, 365 KEGG orthology genes related to carbon metabolism were identified (Supplementary Table S1). Genes related to carbon metabolism, with relative abundances exceeding 30,000, were selected for further analysis. Through NMDS analysis, the structure of carbon metabolism-related genes in sediment bacterial communities exhibited a scattered distribution (Figure 4A). Subsequent analysis using PERMANOVA indicated that there was no significant difference in the bacterial communities of carbon metabolism-related genes between the two culture modes over time (R2 = 0.06072, p = 0.1648). On the other hand, NMDS analysis revealed that the structure of carbon metabolism-related genes in water bacterial communities displayed characteristics of succession over time (Figure 4B). Further analysis using PERMANOVA showed a significant difference in the bacterial communities of carbon metabolism-related genes between the two culture modes over time (R2 = 0.10592, p < 0.001).
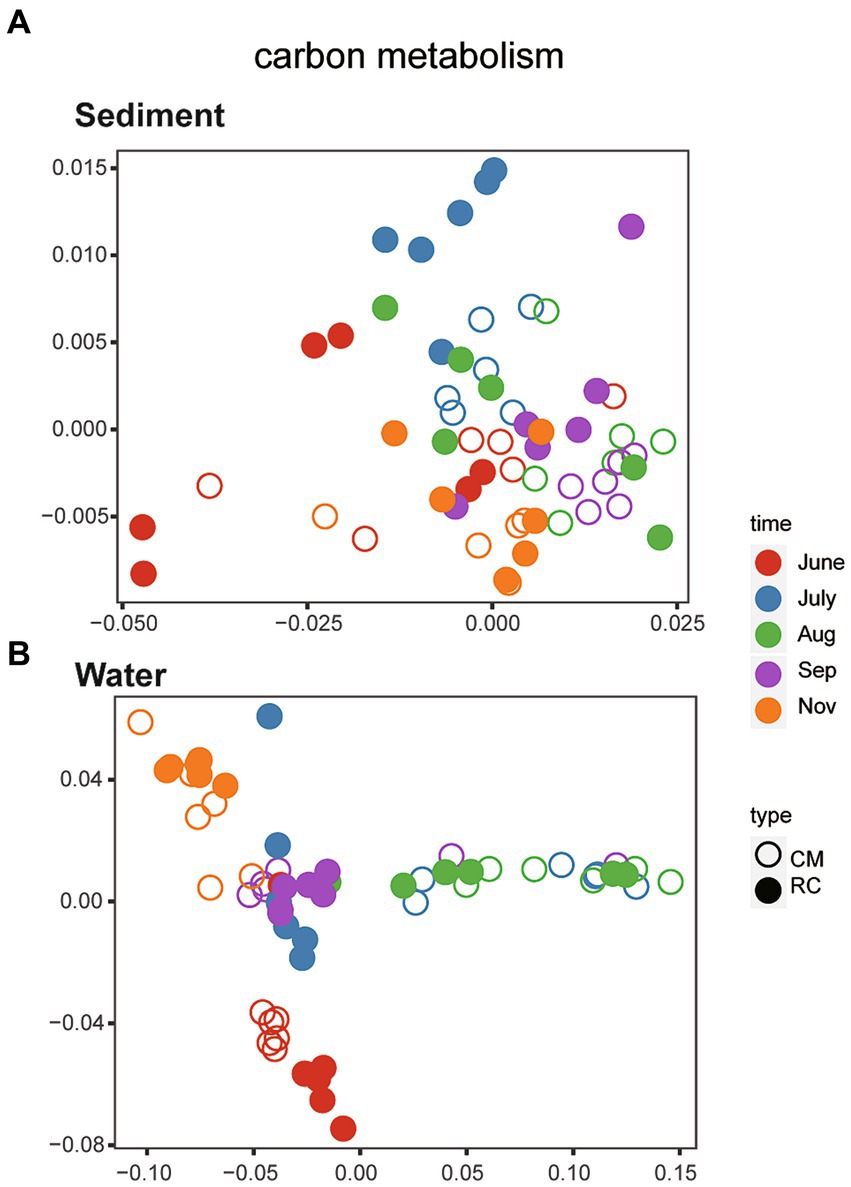
Figure 4. The structure of bacteria community function of Carbon metabolism. (A) Sediment. (B) Water.
Network characteristics of bacterial communities under different CMC culture modes
The sediment bacterial community network under the RC mode comprised 63 nodes and 218 edges, while the CM mode had relatively fewer nodes and edges (Figure 5A). Additionally, the average clustering coefficient of the RC mode was higher than that of the CM mode, while the average path length was lower than that of the CM mode (Table 1). The positive correlation ratio of sediment bacterial communities in the RC mode was 78.44%, which was higher than that of the CM mode (Figure 5A). In both culture modes, the predominant components were Chloroflexi, Proteobacteria, Firnicutes, and Actinobacteriota (Figure 5A). Significant differences in closeness centrality between nodes in the bacterial community networks were observed between the two culture modes (Supplementary Figure S3). ZiPi analysis indicated that microbial communities in both culture modes were primarily composed of peripherals (Figure 5C).
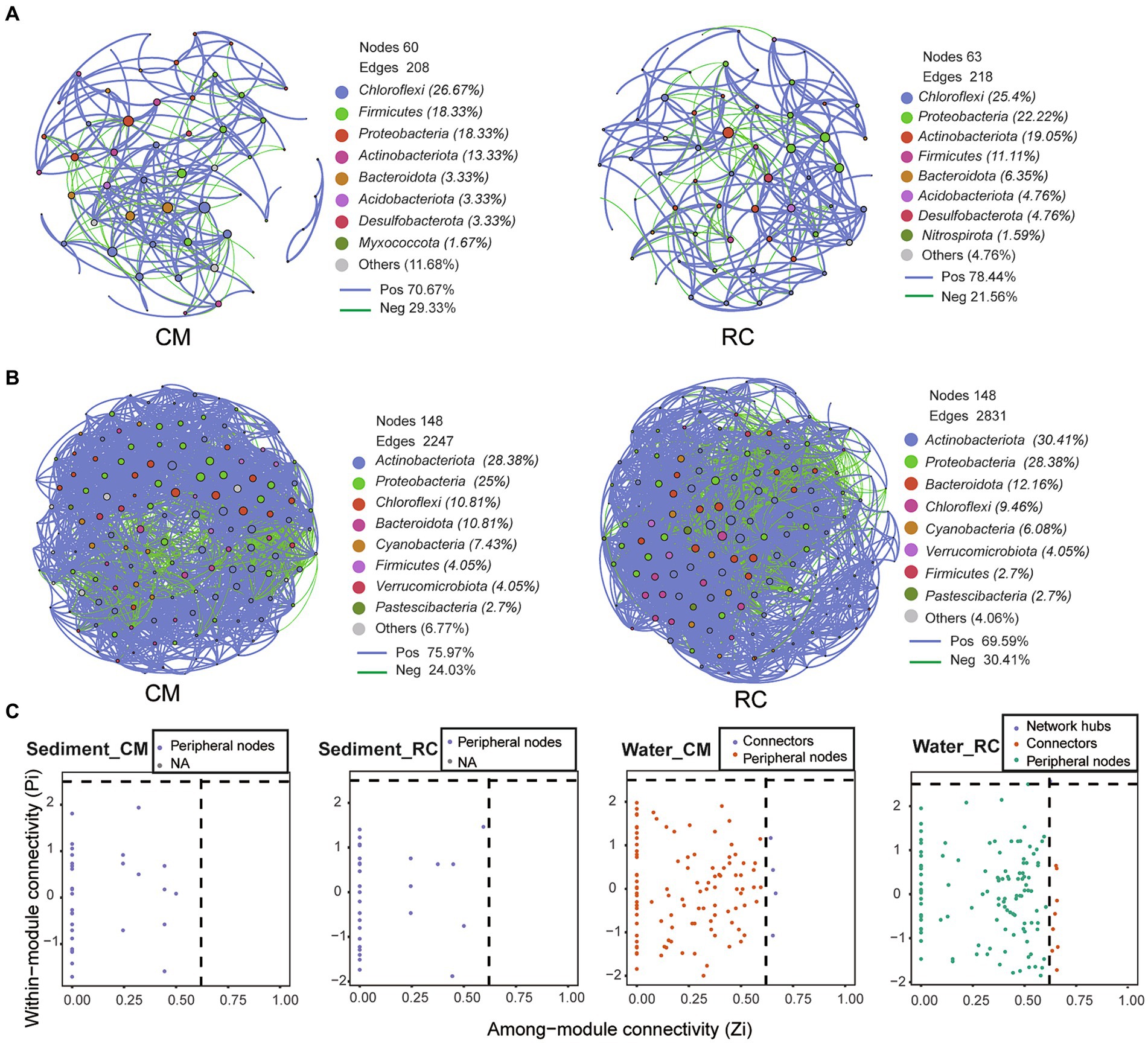
Figure 5. The co-occurrence network of bacterial community in the CMC culture environment. (A) Network graphs of sediment. (B) Network graphs of water. (C) ZiPi plot. In Zipi plots, nodes were categorized into four functional types based on their topological characteristics. These categories included: Connectors: Nodes with high connectivity within two modules (Zi > 2.5 and Pi<0.6). Module hubs: Nodes with high connectivity between two modules (Zi < 2.5 and Pi>0.62). Network hubs: Nodes with high connectivity (Zi > 2.5 and Pi>0.62). Peripherals: Nodes that do not exhibit high connectivity (Zi < 2.5 and Pi<0.62) either within or between modules.
The water bacterial community network under the RC mode consisted of 148 nodes and 2,831 edges, while the CM mode had relatively fewer edges (Figure 5B). Additionally, the average clustering coefficient of the RC mode was lower than that of the CM mode, while the average path length was higher than that of the CM mode (Table 1). The positive correlation ratio of water bacterial communities in the RC mode was 59.59%, which was lower than that of the CM mode. In both modes, the primary components were Actinobacteria, Proteobacteria, Chloroflexi, and Bacteroidota (Figure 5B). Significant differences in degree and closeness centrality between nodes in the bacterial community networks were observed between the two modes (Supplementary Figure 3).
ZiPi analysis indicated that microbial communities in both modes were primarily composed of peripherals (Figure 5C). In the RC mode, microbial taxa with network hubs and connects were considered keystone taxa, including OTU22 (uncultured actinobacterium g_ hgcI clade) and OTU12 (uncultured Opitutae bacterium g_ norank) (Figure 6). In the CM mode, microbial taxa with connects were also keystone taxa, mainly including OTU25 (uncultured bacterium g_ Limnohabitans) (Figure 6). Furthermore, a correlation analysis between keystone taxa and environmental factors revealed that TP, TN, NO2−-N, and CODMn in pond water were factors affecting keystone taxa. These same physicochemical factors also influenced keystone taxa in the RC mode water (Figure 6).
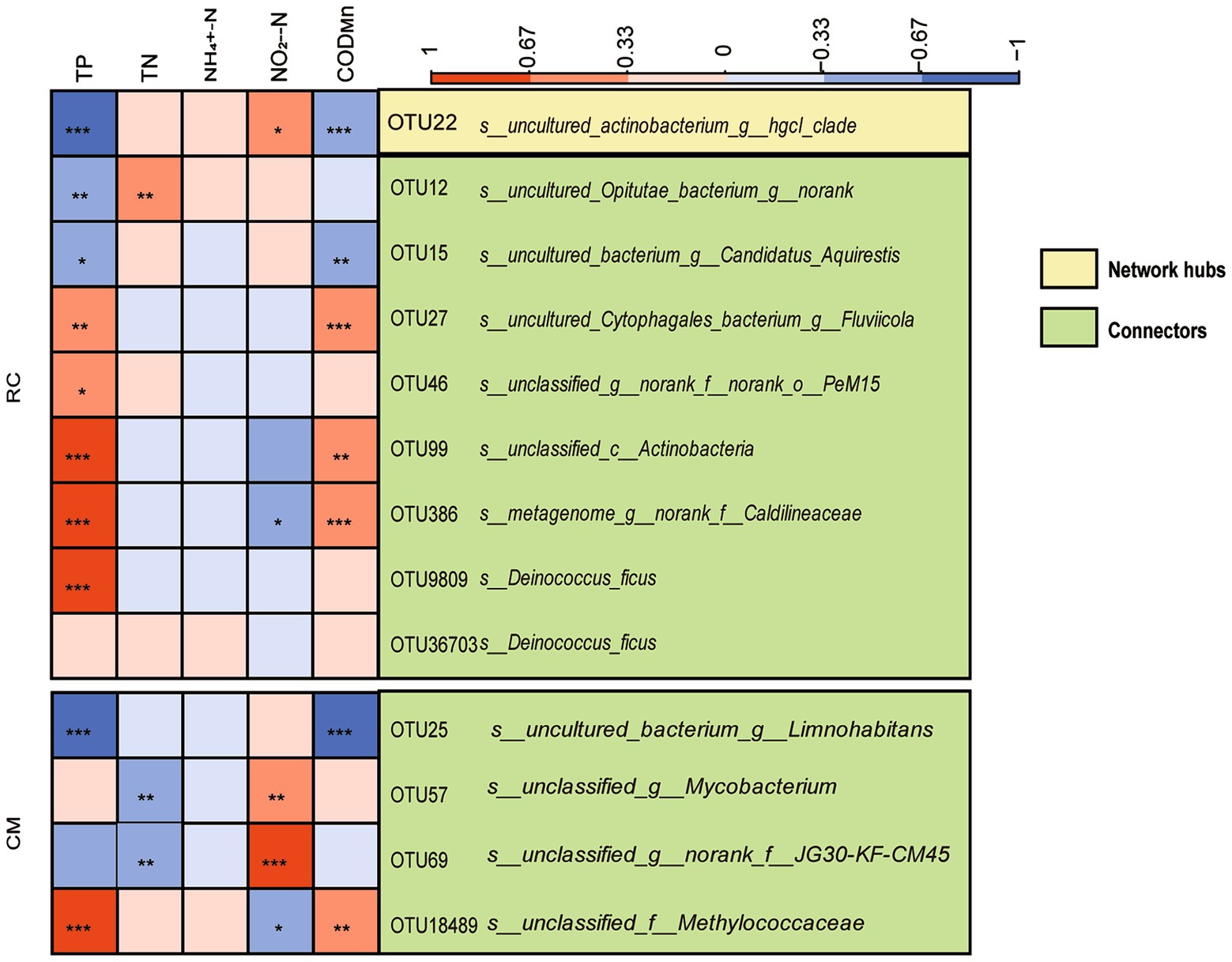
Figure 6. The relationships of keystone species of bacterial community and environmental factors in the CMC culture water. Notes: *denotes a correlation with significance p < 0.05, **denotes a correlation with significance p < 0.01, ***denotes a correlation with significance p < 0.001.
Discussion
The trend of Planktothrix blooms in CMC culture systems has been increasingly severe. Pond culture systems, with Planktothrix dominating, experienced a bloom as early as July. Typically, Planktothrix blooms are known to occur in late summer (Millie et al., 2009; Ma et al., 2021). This trend highlights the advancing nature of CB and their potentially negative impacts on CMC culture. To our surprise, the RC system demonstrated lower bloom intensity and timing compared to the CM mode (Supplementary Figure S1). This suggests that the RC system possesses more robust self-regulation, and its ecological value is enhanced by employing rice as an alternative to Alternanthera philoxeroides in CMC culture.
CB has a significant influence on the composition and variation of bacterial communities in CMC culture water (Figure 1). This observation aligns with findings in CMC culture systems in lakes (Li et al., 2019). Notably, the bacterial community composition in CMC culture water exhibits significant variations under CB conditions, and different functional taxa display distinct succession patterns. For instance, in our study, Planktothrix, a dominant cyanobacterium at the genus level, proliferated in the CMC culture system. OTU147, belonging to Planktothrix, emerged as the dominant taxon responsible for the differences in water composition between the two culture modes. It has been reported that toxic Planktothrix can lead to surface water odor pollution (Su et al., 2017). Thus, the impact of OTU147 (uncultured bacterium g_Planktothrix NIVA-CYA 15) on CMC farming is significant and should not be underestimated (Figure 2).
One of the concerning aspects of CBs is their potential to cause the accumulation of pathogenic bacteria. The occurrence of Planktothrix has led to the accumulation of Mycobacterium (Figure 2), posing a health threat to humans (Dong et al., 2021) through water exposure. Therefore, considering the advancing Planktothrix blooms and the concurrent increase in pathogenic bacteria, it is imperative to emphasize the secondary hazards associated with Planktothrix blooms in CMC culture. Preventative and control measures are of paramount importance.
Several environmental factors, such as nutrients, have been shown to influence Planktothrix bloom (Cheng et al., 2022). In our study, we found that TN, TP, NO2−-N, and CODMn had a significant impact on the bacterial community composition in the water (Figure 3). Furthermore, we observed a significant positive correlation between the Planktothrix bloom and the levels of TN, TP, and CODMn (Supplementary Figure S2). This finding aligns with a study conducted in crab ponds in Guchenghu, where the N:P ratio, WT, and TP were identified as significant factors affecting cyanobacterial development (Li et al., 2019), which is in line with our research.
The substantial input of nitrogen and phosphorus into the water has been identified as a key factor in promoting Planktothrix formation (Davis et al., 2015; Wang et al., 2023). In our study, we noticed that the TP content in RC water was lower than that in CM water during the same time period, while the intensity of Planktothrix blooms in the RC system was lower than that in the CM culture system (Supplementary Figure S1). This suggests that, in RC models, rice roots may have a greater tendency to utilize available phosphorus compared to Alternanthera philoxeroides.
Planktothrix, as carbon-fixing microorganisms, plays a critical role in converting atmospheric carbon into organic carbon in the water (Zhang et al., 2020). Our further analysis indicated that COM drives the succession of Planktothrix blooms. Prior to the rice harvest, the CODMn content in the RC system was lower than that in the CM system. During this time, CB in the CM system was more severe than in the RC system. However, after the rice harvest, the CODMn content in the RC system increased compared to the CM system. This change could be attributed to the reduced nutrient absorption by rice roots in the water of the RC system and the presence of remaining roots in the culture system, leading to increased eutrophication and organic matter in the system. However, as temperatures decreased during this period, the intensity of CB in the culture system tended to decline, and the microbial community that relies on organic matter was no longer dominated by Planktothrix. As a result, the adverse impact on the culture system was relatively reduced. Thus, we conclude that Planktothrix blooms in the CMC culture system are linked to increases in physicochemical factors such as TP and CODMn (Supplementary Figure S2).
The RC system exhibits more intricate relationships between bacterial communities in sediment and water compared to the CM mode. This complexity may arise from the more intricate ecological environment created by rice cultivation in the RC system. Higher clustering coefficients and shorter average path lengths are indicative of more pronounced small-world effects, as outlined by Collins and Chow (1998). Our research findings suggest that the bacterial community in sediment, forming a small-world network, is more stable under the RC mode. In contrast, the bacterial community in water, also forming a small-world network, appears to be relatively fragile. The positive correlation ratio between sediment bacterial communities under the RC mode is significantly higher than that under the CM mode (Figure 5). This suggests that cooperation within sediment bacterial communities under the RC mode is more robust, and frequent collaboration among bacteria may enhance their ability to adapt to environmental changes. Conversely, the negative correlation ratio between water bacterial communities under the RC mode is higher than that under the CM mode (Figure 5). This indicates that competition is more intense within water bacterial communities under the RC mode. In summary, our data reveals that, when compared to the CM modes, the complex ecosystems of the RC mode can lead to more stable microbial networks, providing a degree of resilience against Planktothrix blooms.
In addition, our data shows that Planktothrix blooms have an impact on the bacterial community structure and carbon metabolism (Figure 4). Several keystone taxa, primarily consisting of OTU22 (uncultured actinobacterium g_ hgcI clade) and OTU12 (uncultured Opitutae bacterium g_ norank) in our study (Figure 6), require carbon and nitrogen sources for their growth. Members of Opitutae contain nitrogen fixation genes (Wertz et al., 2012), while Roseiflexus requires carbon sources for growth and is strictly phototrophic (Wertz et al., 2012). Fluviicola may indicate good water quality (Ji et al., 2018) and exhibit efficient performance in nitrogen and carbon removal (Chen et al., 2020). In RC mode, the microbial taxa serving as keystone species are predominantly composed of OTU25 (uncultured bacterium g_ Limnohabitans) (Figure 6), among which Limnohabitans plays a role in nitrogen cycling and cyanobacteria degradation (Zhu et al., 2020). The taxon of norank_f_JG30-KF-CM45 is involved in denitrification.TN, NO2−-N, and CODMn are factors affecting the keystone taxa in CMC culture water (Figure 6). This indicates that the keystone microbial taxa in CMC culture water actively participate in carbon and nitrogen cycling processes. These keystone taxa can compete for additional carbon and nitrogen sources in the water to support their growth, ensuring the stable operation of the entire aquaculture system. Moreover, these keystone taxa possess the ability to degrade cyanobacteria, thereby mitigating the harm caused by cyanobacterial blooms to some extent.
Conclusion
In summary, our research offers valuable insights into the mechanisms driving microbial community changes in the CMC culture system during Planktothrix blooms from a microbial ecology perspective. We observed that both the timing and intensity of Planktothrix blooms in the RC mode were lower than those in the CM mode. The RC system exhibits more complex relationships among bacterial communities when compared to the CM mode. The occurrence of Planktothrix blooms in the CMC culture system is associated with increased physicochemical factors, such as TP and CODMn, and alterations in the bacterial community structure related to carbon metabolism. Furthermore, the keystone taxa identified in our study are reliant on carbon and nitrogen sources for their growth and possess the capacity to degrade cyanobacteria. This research offers valuable environmental microbial data to support the management of cyanobacterial outbreaks in CMC culture.
Data availability statement
The datasets presented in this study can be found in online repositories. The names of the repository/repositories and accession number(s) can be found in the article/Supplementary material.
Ethics statement
The animal study was approved by all applicable international, national, and/or institutional guidelines for the care and use of animals followed the National Institutes of Health Guide for the Care and Use of Laboratory Animals. The study was conducted in accordance with the local legislation and institutional requirements.
Author contributions
LT: Conceptualization, Data curation, Formal analysis, Investigation, Methodology, Software, Writing – original draft, Writing – review & editing. LJ: Conceptualization, Data curation, Funding acquisition, Project administration, Supervision, Visualization, Writing – original draft. JH: Conceptualization, Funding acquisition, Project administration, Supervision, Validation, Visualization, Writing – original draft, Writing – review & editing. YM: Conceptualization, Data curation, Formal analysis, Investigation, Methodology, Writing – original draft, Writing – review & editing. DG: Supervision, Visualization, Writing – review & editing. HY: Supervision, Validation, Visualization, Writing – review & editing. ZH: Supervision, Validation, Visualization, Writing – review & editing. WH: Supervision, Validation, Visualization, Writing – review & editing. PT: Conceptualization, Data curation, Formal analysis, Investigation, Methodology, Writing – original draft, Writing – review & editing.
Funding
The author(s) declare that financial support was received for the research, authorship, and/or publication of this article. This research was supported by Ministry of Agriculture and Rural Affairs of the People's Republic of China earmarked fund (grant number: CARS-48) and Anhui Province innovation platform of major science and technology projects (grant number: S202305a12020001).
Acknowledgments
Thanks to all participants of Xuanzhou District Fisheries Technology Extension Center, Xuancheng City, Anhui Province, China in conducting the experiment.
Conflict of interest
The authors declare that the research was conducted in the absence of any commercial or financial relationships that could be construed as a potential conflict of interest.
Publisher’s note
All claims expressed in this article are solely those of the authors and do not necessarily represent those of their affiliated organizations, or those of the publisher, the editors and the reviewers. Any product that may be evaluated in this article, or claim that may be made by its manufacturer, is not guaranteed or endorsed by the publisher.
Supplementary material
The Supplementary material for this article can be found online at: https://www.frontiersin.org/articles/10.3389/fmicb.2024.1327520/full#supplementary-material
Footnotes
References
Ahlmann-Eltze, C., and Patil, I. (2021). Ggsignif: R package for displaying significance brackets for'ggplot2'. doi: 10.31234/osf.io/7awm6
Cheng, J., Ma, L., Zhao, W., and Liu, Y. (2022). Effects of environmental factors on the growth and 2-MIB production of Planktothrix sp. J. Hydroecol. 43:7. doi: 10.15928/j.1674-3075.202008180236
Chen, S., Chen, W., Wu, S., Wang, X., and Wang, J. (2020). Effects of external resistance on denitrification and carbon removal performance of a microbial fuel cell used in simulated groundwater. Fresenius Environ. Bull. 29, 5464–5471.
Davis, T. W., Bullerjahn, G. S., Tuttle, T., McKay, R. M., and Watson, S. B. (2015). Effects of increasing nitrogen and phosphorus concentrations on phytoplankton community growth and toxicity during planktothrix blooms in Sandusky Bay, Lake Erie. Environ. Sci. Technol. 49, 7197–7207. doi: 10.1021/acs.est.5b00799
Dong, W., Nie, X., Zhu, H., Liu, Q., Shi, K., You, L., et al. (2021). Mycobacterial fatty acid catabolism is repressed by FdmR to sustain lipogenesis and virulence. Proc. Natl. Acad. Sci. 118:e2019305118. doi: 10.1073/pnas.2019305118
Faust, K., and Raes, J. (2012). Microbial interactions: from networks to models. Nat. Rev. Microbiol. 10, 538–550. doi: 10.1038/nrmicro2832
Harrell, F. E. . (2019). Package ‘hmisc’. CRAN2018, 2019, 235-236. Available at: https://cran.uib.no/web/packages/Hmisc/Hmisc.pdf
Huang, F., Pan, L., Song, M., Tian, C., and Gao, S. (2018). Microbiota assemblages of water, sediment, and intestine and their associations with environmental factors and shrimp physiological health. Appl. Microbiol. Biotechnol. 102, 8585–8598. doi: 10.1007/s00253-018-9229-5
Ji, B., Qin, H., Guo, S., Chen, W., Zhang, X., and Liang, J. (2018). Bacterial communities of four adjacent fresh lakes at different trophic status. Ecotoxicol. Environ. Saf. 157, 388–394. doi: 10.1016/j.ecoenv.2018.03.086
Li, H., Chen, H., Gu, X., Mao, Z., Zeng, Q., and Ding, H. (2019). Dynamics of cyanobacteria and related environmental drivers in freshwater bodies affected by mitten crab culturing: a study of lake guchenghu, China. Water 11:2468. doi: 10.3390/w11122468
Liu, C., Cui, Y., Li, X., and Yao, M. (2021). Microeco: an R package for data mining in microbial community ecology. FEMS Microbiol. Ecol. 97:fiaa255. doi: 10.1093/femsec/fiaa255
Ma, B., Wang, H., Dsouza, M., Lou, J., He, Y., Dai, Z., et al. (2016). Geographic patterns of co-occurrence network topological features for soil microbiota at continental scale in eastern China. ISME J. 10, 1891–1901. doi: 10.1038/ismej.2015.261
Ma, Q., Wang, Y., Li, C., Fan, P., Zhou, J., and Fang, W. (2021). Influence of cyanobacterial bloom dominated by Planktothrix sp. and Cylindrospermopsis raciborskii on microflora structure of intestine, gill and culture enviroment of cultured Eriocheir sinensis. Mairne Fish. 43, 595–606. doi: 10.3969/j.issn.1004-2490.2021.05.009
Millie, D. F., Fahnenstiel, G. L., Dyble Bressie, J., Pigg, R. J., Rediske, R. R., Klarer, D. M., et al. (2009). Late-summer phytoplankton in western Lake Erie (Laurentian Great Lakes): bloom distributions, toxicity, and environmental influences. Aquat. Ecol. 43, 915–934. doi: 10.1007/s10452-009-9238-7
Su, M., Jia, D., Yu, J., Vogt, R. D., Wang, J., An, W., et al. (2017). Reducing production of taste and odor by deep-living cyanobacteria in drinking water reservoirs by regulation of water level. Sci. Total Environ. 574, 1477–1483. doi: 10.1016/j.scitotenv.2016.08.134
Wang, C., Wang, X., Huang, Y., Bu, X., and Chen, L. (2020). Effects of dietary T-2 toxin on gut health and gut microbiota composition of the juvenile Chinese mitten crab (Eriocheir sinensis). Fish Shellfish Immunol. 106, 574–582. doi: 10.1016/j.fsi.2020.08.019
Wang, S., Zhang, P., Zhang, D., and Chang, J. (2023). Evaluation and comparison of the benthic and microbial indices of biotic integrity for urban lakes based on environmental DNA and its management implications. J Environ Manage. 341:118026. doi: 10.1016/j.jenvman.2023.118026
Wei, T., Simko, V., Levy, M., Xie, Y., Jin, Y., and Zemla, J. (2017). Package ‘corrplot’. Statistician 56:e24.
Wertz, J. T., Kim, E., Breznak, J. A., Schmidt, T. M., and Rodrigues, J. L. M. (2012). Genomic and physiological characterization of the verrucomicrobia isolate diplosphaera colitermitum gen. Nov., sp. nov., reveals microaerophily and nitrogen fixation genes. Appl. Environ. Microbiol. 78, 1544–1555. doi: 10.1128/AEM.06466-11
Xiong, J., Wang, K., Wu, J., Qiuqian, L., Yang, K., Qian, Y., et al. (2015). Changes in intestinal bacterial communities are closely associated with shrimp disease severity. Appl. Microbiol. Biotechnol. 99, 6911–6919. doi: 10.1007/s00253-015-6632-z
Xiong, J., Zhu, J., and Zhang, D. (2014). The application of bacterial indicator phylotypes to predict shrimp health status. Appl. Microbiol. Biotechnol. 98, 8291–8299. doi: 10.1007/s00253-014-5941-y
Xu, M., Xu, R., Shen, X., Gao, P., Xue, Z., Huang, D., et al. (2022). The response of sediment microbial communities to temporal and site-specific variations of pollution in interconnected aquaculture pond and ditch systems. Sci. Total Environ. 806:150498. doi: 10.1016/j.scitotenv.2021.150498
Zhang, X., Ye, X., Chen, L., Zhao, H., Shi, Q., Xiao, Y., et al. (2020). Functional role of bloom-forming cyanobacterium Planktothrix in ecologically shaping aquatic environments. Sci. Total Environ. 710:136314. doi: 10.1016/j.scitotenv.2019.136314
Zhou, J., Fang, W., Yang, X., Zhou, S., Hu, L., Li, X., et al. (2012). A nonluminescent and highly virulent Vibrio harveyi strain is associated with “bacterial white tail disease” of Litopenaeus vannamei shrimp. PLoS One 7:e29961. doi: 10.1371/journal.pone.0029961
Zhu, C., Zhang, J., Nawaz, M. Z., Mahboob, S., Al-Ghanim, K. A., Khan, I. A., et al. (2019). Seasonal succession and spatial distribution of bacterial community structure in a eutrophic freshwater Lake, Lake Taihu. Sci. Total Environ. 669, 29–40. doi: 10.1016/j.scitotenv.2019.03.087
Keywords: cyanobacterial blooms (CB), Chinese mitten crab, crab culture models, bacterial community, environmental factors, co-occurrence network, keystone taxa, carbon metabolism
Citation: Tong L, Jun L, He J, Min Y, Guoqing D, Yuting H, Huaxing Z, Huan W and Tingshuang P (2024) Differences in environmental microbial community responses under rice-crab co-culture and crab monoculture models under cyanobacterial bloom. Front. Microbiol. 15:1327520. doi: 10.3389/fmicb.2024.1327520
Edited by:
Huan Li, Lanzhou University, ChinaReviewed by:
Yi Luo, Huazhong Agricultural University, ChinaYafei Duan, South China Sea Fisheries Research Institute, China
Xianyong Bu, Ocean University of China, China
Copyright © 2024 Tong, Jun, He, Min, Guoqing, Yuting, Huaxing, Huan and Tingshuang. This is an open-access article distributed under the terms of the Creative Commons Attribution License (CC BY). The use, distribution or reproduction in other forums is permitted, provided the original author(s) and the copyright owner(s) are credited and that the original publication in this journal is cited, in accordance with accepted academic practice. No use, distribution or reproduction is permitted which does not comply with these terms.
*Correspondence: Pan Tingshuang, cGFudGluZ3NodWFuZ0AxNjMuY29t