- 1SANKEN (The Institute of Scientific and Industrial Research), Osaka University, Osaka, Japan
- 2Graduate School of Pharmaceutical Sciences, Osaka University, Osaka, Japan
- 3Institute for Advanced Co-Creation Studies, Osaka University, Osaka, Japan
- 4Center for Infectious Disease Education and Research, Osaka University, Osaka, Japan
Fatty acids salts exert bactericidal and bacteriostatic effects that inhibit bacterial growth and survival. However, bacteria can overcome these effects and adapt to their environment. Bacterial efflux systems are associated with resistance to different toxic compounds. Here, several bacterial efflux systems were examined to determine their influence on fatty acid salt resistance in Escherichia coli. Both acrAB and tolC E. coli deletion strains were susceptible to fatty acid salts, while plasmids carrying acrAB, acrEF, mdtABC, or emrAB conferred drug resistance to the ΔacrAB mutant, which indicated complementary roles for these multidrug efflux pumps. Our data exemplify the importance of bacterial efflux systems in E. coli resistance to fatty acid salts.
Introduction
Fatty acid salts exhibit amphipathic properties and antibacterial activities which are mediated by (i) increased membrane permeability and leakage, (ii) disrupted electron transport chain and oxidative phosphorylation uncoupling, and (iii) inhibited nutrient uptake and membrane enzyme activity (Yoon et al., 2018). While bacteria employ several strategies to resist the antibacterial actions of fatty acid salts (Miller et al., 1977; Chamberlain et al., 1991; Desbois and Smith, 2010), the resistance mechanisms remain unclear, therefore, it is important to comprehend how bacteria evade/abrogate the bactericidal effects of fatty acid salts.
Bacterial drug resistance is associated with drug efflux pumps which reduce drug accumulation in cells (Nikaido, 1996; Zgurskaya and Nikaido, 2000). These pumps are classified into six categories based on sequence similarity with other protein families: major facilitator superfamily (MFS), resistance-nodulation-cell division (RND), small multidrug resistance (SMR), multidrug and toxic compound extrusion (MATE), ATP-binding cassette (ABC), and proteobacterial antimicrobial compound efflux families (PACE; Putman et al., 2000; Hassan et al., 2018). The elucidation of bacterial genome sequences has greatly facilitated the identification of putative drug resistance genes in Gram-negative bacteria, including Escherichia coli (Nishino and Yamaguchi, 2001). Of note, the RND family has a major role in both intrinsic and acquired multidrug resistance in Gram-negative bacteria (Venter et al., 2015). RND efflux pumps require two proteins to function: a membrane fusion protein (periplasmic adaptor protein) and an outer membrane protein, e.g., the major drug efflux pump AcrB of the RND family requires the membrane fusion protein AcrA and the outer membrane protein TolC to function (Nishino et al., 2003; Alav et al., 2021; Zwama and Nishino, 2021).
In some bacteria, multidrug efflux pumps are believed to play vital functions overcoming the antibacterial effects of fatty acid salts (Ma et al., 1995; Gunn, 2000; Rosenberg et al., 2003; Prouty et al., 2004; Lennen et al., 2013; Wotzka et al., 2019; Henderson et al., 2021; Yoneda et al., 2022). In this study, we evaluated multidrug efflux pump functions toward fatty acid salt resistance using various E. coli strains deficient in or overexpressing genes encoding multidrug efflux pumps. Using this strategy, we identified multidrug efflux pumps and mechanisms involved in bacterial resistance to fatty acid salts.
Materials and methods
Strains and plasmids used in this study
Study strains and plasmids are shown (Table 1). Escherichia coli strains were derived from the MG1655 wild-type (WT) strain (Blattner et al., 1997). To construct E. coli gene deletion mutants, gene disruption strategies were performed according to Datsenko and Wanner (2000) using the primers listed in Table 2. Plasmids carrying acrAB, acrD, acrEF, mdtABC, mdtEF, emrAB, macAB, emrE, mdfA, or mdtK were constructed as previously described (Nishino et al., 2003).
Measurement of the minimum inhibitory concentrations of fatty acid salts
To examine multidrug efflux pumps in E. coli fatty acid salt resistance, antibacterial activities were examined on LB plates containing sodium hexanoate (C6) at concentrations from 78 to 40,000 μg/ml, sodium octanoate (C8) at concentrations from 78 to 40,000 μg/ml, sodium decanoate (C10) at concentrations from 0.63 to 40,000 μg/ml, and sodium dodecanoate (C12) at concentrations from 0.08 to 5,000 μg/ml (Sigma-Aldrich, St Louis, MO, United States; Table 3). Plates were prepared using the 2-fold agar dilution technique (Nishino and Yamaguchi, 2004). To determine minimum inhibitory concentrations (MICs), bacteria were cultured overnight at 37°C in LB, diluted in the same medium, and tested at a final inoculum of 105 colony forming units/spot using McFarland turbidity standards (Eiken Chemical, Tokyo, Japan) and a multipoint inoculator (Sakuma Seisakusyo, Tokyo, Japan). Plates were incubated at 37°C for 20 h.
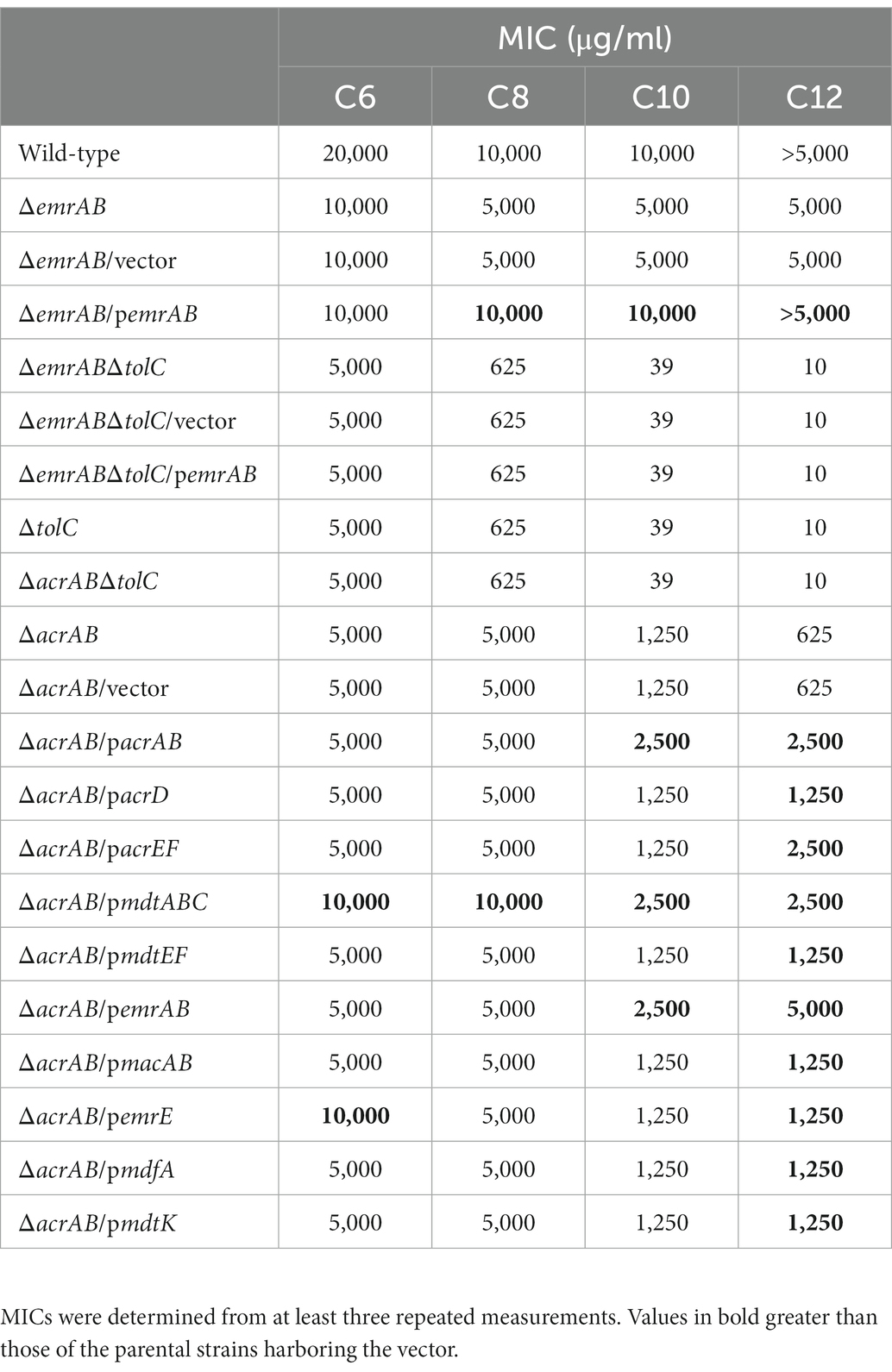
Table 3. Escherichia coli strain susceptibility to sodium hexanoate (C6), sodium octanoate (C8), sodium decanoate (C10), and sodium dodecanoate (C12) using minimum inhibitory concentrations (MIC).
Measurement of the bacterial growth in the presence of sodium dodecanoate (C12)
Single E. coli colonies were inoculated into 2 ml of LB. Bacteria were cultured overnight at 37°C, diluted in the same medium, and tested at a final inoculum concentration of 105 colony forming units/μl in 200 μl of LB broth containing sodium dodecanoate (C12; 1,000 μg/ml) using McFarland turbidity standards (Eiken Chemical, Tokyo, Japan). Then, liquid cultures were incubated and shaken at 37°C in NUNC Edge 96-well plates (Thermo Scientific, MA, United States). Bacterial growth was measured by OD600nm readings using the Infinite M200 PRO plate reader (Tecan, Männedorf, Switzerland).
Results and discussion
In order to investigate the involvement of multidrug efflux pumps in the fatty acid salt susceptibility of E. coli, MIC measurements were carried out as described in the section materials and methods. Strains lacking acrAB (coding for the bacterial efflux system) or tolC (outer membrane channel gene) were more susceptible to fatty acid salts when compared with the WT strain (Table 3). These observations showed that the antibacterial activity of fatty acid salts increased with carbon atom numbers in salts, i.e., MIC values for ΔtolC in E. coli decreased as carbon atoms increased (Table 3). Both ΔtolC and ΔacrABΔtolC mutants were more sensitive to sodium octanoate (C8), sodium decanoate (C10), and sodium dodecanoate (C12) than the ΔacrAB mutant, suggesting TolC was needed for fatty acid salt resistance as it functioned not only with AcrAB but also with other multidrug efflux pumps in a protein complex in E. coli (Zgurskaya et al., 2011; Lennen et al., 2013).
Furthermore, plasmids carrying drug efflux pump genes belonging to RND (acrAB, acrD, acrEF, mdtABC, and mdtEF), MFS (emrAB and mdfA), ABC (macAB), SMR (emrE), and MATE (mdtK) families were transformed into the ΔacrAB E. coli strain, and susceptibility to fatty acid salts measured (Table 3). When acrAB, acrEF, mdtABC, or emrAB were overexpressed in the ΔacrAB strain, a 4–8-fold increase in resistance to sodium dodecanoate (C12) was observed. The complementation of acrAB on the plasmid in the ΔacrAB strain did not completely reconstitute the resistance of the wild-type strain. This is often the case with some antimicrobial compounds, since the expression of acrAB in the wild-type strain is quite high (Nishino et al., 2003). In particular, emrAB expression conferred the highest resistance level (Table 3) and the fastest growth rate (Figure 1). Thus, multiple efflux pumps had complementary roles with AcrAB in generating fatty acid salt resistance in E. coli.
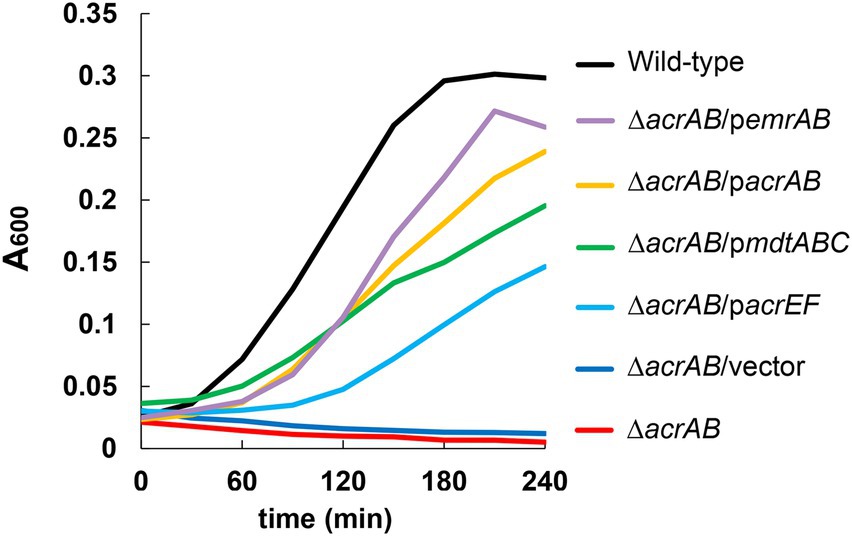
Figure 1. Effects of multidrug efflux pumps on the growth of Escherichia coli in the presence of 1,000 μg/ml of sodium dodecanoate (C12). The growth of the acrAB mutants with plasmids carrying multidrug efflux pump genes. One of the three experiments that have the similar results is shown.
Our finding that AcrAB belonging to the RND family is major efflux pump to contribute the resistance to fatty acid salts is consistent with previous reports (Rosenberg et al., 2003; Lennen et al., 2013). In a previous report provided by Rosenberg et al., they showed the mechanism of acrAB induction by decanoate is mediated with Rob (XylS/AraC family regulator). In future, the detailed mechanism of fatty acid salts resistance we found should be further investigated. Additionally, we could demonstrate that the efflux pump EmrAB, a class of MFS, was specifically involved in fatty acid salt sensitivity in E. coli. Of note, considering the previous findings, EmrAB is one of pumps to complement with AcrAB across Gram-negative bacteria, including E. coli and Salmonella enterica (Lennen et al., 2013; Yoneda et al., 2022). In S. enterica, EmrAB could increase fatty acid salt resistance without TolC, therefore, it was suggested that other genes were involved in the resistance regulated by EmrAB (Yoneda et al., 2022). We showed that the deletion of emrAB increased susceptibility to fatty acid salts, and the complementation of emrAB on the plasmid in the ΔemrAB strain reconstituted the resistance of the wild-type strain in E. coli (Table 3). However, the susceptibility of ΔemrABΔtolC, ΔemrABΔtolC/vector, and ΔemrABΔtolC/pemrAB strains did not change from that of ΔtolC strain, indicating that, unlike in S. enterica, EmrAB requires TolC for fatty acid salt efflux in E. coli (Table 3). EmrA and EmrB are 89.7 and 95.7% identical in their amino acid sequences between E. coli and S. enterica (Nishino et al., 2006). However, the difference in the structure of EmrAB between two species is unknown and it is difficult to predict the difference related to interaction with TolC from their amino acid sequences because not only changes in specific amino acids, but also the overall structure of EmrAB is involved in TolC interaction. On the other hand, it has been shown that the substrates for EmrAB are different between E. coli and S. enterica (Lomovskaya and Lewis, 1992; Nishino and Yamaguchi, 2001; Nishino et al., 2006), therefore, there may be difference in the structure of EmrAB between two species. It is also possible that there are other unknown outer membrane proteins in S. enterica that could be used by EmrAB.
Infecting bacteria into the host cells must be resistant to antimicrobial compounds. Fatty acid salts are host-derived antimicrobial molecules (Henderson et al., 2021). Therefore, each efflux pump has the function to efflux fatty acid salts to protect E. coli from accumulating these compounds.
In conclusion, we provide valuable insights on how multidrug efflux pumps confer fatty acid salt resistance in E. coli.
Data availability statement
The original contributions presented in the study are included in the article/supplementary material, further inquiries can be directed to the corresponding author.
Author contributions
TY, SY, and SI conceived the experiments. MH-N and KN designed the study. SY, SI, M-HN, and KN constructed strains. TY, SY, SI, M-HN, and KN analyzed data. TY and KN wrote the manuscript. KN supervised the project. All authors contributed to the article and approved the submitted version.
Funding
This study was supported by a research grant from Kakenhi (18K19451, 21H03542, 21K16318, and 22K19831) from the Japan Society for the Promotion of Science, Core Research for Evolutional Science and Technology (JPMJCR20H9) from the Japan Science and Technology Agency, Japan Agency for Medical Research and Development, the Takeda Science Foundation, the Nippon Foundation-Osaka University Project for Infectious Disease Prevention, International Joint Research Promotion Program of Osaka University, and Research Program for CORE2-A lab, Network Joint Research Center for Materials and Devices and Dynamic Alliance for Open Innovation Bridging Human, Environment, and Materials from the Ministry of Education, Culture, Sports, Science and Technology of Japan.
Conflict of interest
TY is employed by Novartis Pharmaceutical K.K which provided Ph.D. support to TY However, the company did not participate in study design, collection, analysis, and interpretation of data, and writing the manuscript. TY and SI were graduate students at the Graduate School of Pharmaceutical Sciences, Osaka University, and conducted research at SANKEN (The Institute of Scientific and Industrial Research), Osaka University. SY, MH-N, and KN were employed by SANKEN (The Institute of Scientific and Industrial Research), Osaka University, Osaka, Japan.
Publisher’s note
All claims expressed in this article are solely those of the authors and do not necessarily represent those of their affiliated organizations, or those of the publisher, the editors and the reviewers. Any product that may be evaluated in this article, or claim that may be made by its manufacturer, is not guaranteed or endorsed by the publisher.
References
Alav, I., Kobylka, J., Kuth, M. S., Pos, K. M., Picard, M., Blair, J. M., et al. (2021). Structure, assembly, and function of tripartite efflux and type 1 secretion Systems in Gram-Negative Bacteria. Chem. Rev. 121, 5479–5596. doi: 10.1021/acs.chemrev.1c00055
Blattner, F. R., Plunkett, G. 3rd, Bloch, C. A., Perna, N. T., Burland, V., Riley, M., et al. (1997). The complete genome sequence of Escherichia coli K-12. Science 277, 1453–1462. doi: 10.1126/science.277.5331.1453
Chamberlain, N. R., Mehrtens, B. G., Xiong, Z., Kapral, F. A., Boardman, J. L., and Rearick, J. I. (1991). Correlation of carotenoid production, decreased membrane fluidity, and resistance to oleic acid killing in Staphylococcus aureus 18Z. Infect. Immun. 59, 4332–4337. doi: 10.1128/iai.59.12.4332-4337.1991
Datsenko, K. A., and Wanner, B. L. (2000). One-step inactivation of chromosomal genes in Escherichia coli K-12 using PCR products. Proc. Natl. Acad. Sci. U. S. A. 97, 6640–6645. doi: 10.1073/pnas.120163297
Desbois, A. P., and Smith, V. J. (2010). Antibacterial free fatty acids: activities, mechanisms of action and biotechnological potential. Appl. Microbiol. Biotechnol. 85, 1629–1642. doi: 10.1007/s00253-009-2355-3
Gunn, J. S. (2000). Mechanisms of bacterial resistance and response to bile. Microbes Infect. 2, 907–913. doi: 10.1016/S1286-4579(00)00392-0
Hassan, K. A., Liu, Q., Elbourne, L. D. H., Eijkelkamp, B. A., Ahmad, I., Sharples, D., et al. (2018). Pacing across the membrane: the novel PACE family of efflux pumps is widespread in gram-negative pathogens. Res. Microbiol. 169, 450–454. doi: 10.1016/j.resmic.2018.01.001
Henderson, P. J. F., Maher, C., Elbourne, L. D. H., Eijkelkamp, B. A., Paulsen, I. T., and Hassan, K. A. (2021). Physiological functions of bacterial "multidrug" efflux pumps. Chem. Rev. 121, 5417–5478. doi: 10.1021/acs.chemrev.0c01226
Lennen, R. M., Politz, M. G., Kruziki, M. A., and Pfleger, B. F. (2013). Identification of transport proteins involved in free fatty acid efflux in Escherichia coli. J. Bacteriol. 195, 135–144. doi: 10.1128/JB.01477-12
Lomovskaya, O., and Lewis, K. (1992). Emr, an Escherichia coli locus for multidrug resistance. Proc. Natl. Acad. Sci. U. S. A. 89, 8938–8942. doi: 10.1073/pnas.89.19.8938
Ma, D., Cook, D. N., Alberti, M., Pon, N. G., Nikaido, H., and Hearst, J. E. (1995). Genes acrA and acrB encode a stress-induced efflux system of Escherichia coli. Mol. Microbiol. 16, 45–55. doi: 10.1111/j.1365-2958.1995.tb02390.x
Miller, R. D., Brown, K. E., and Morse, S. A. (1977). Inhibitory action of fatty acids on the growth of Neisseria gonorrhoeae. Infect. Immun. 17, 303–312. doi: 10.1128/iai.17.2.303-312.1977
Nikaido, H. (1996). Multidrug efflux pumps of gram-negative bacteria. J. Bacteriol. 178, 5853–5859. doi: 10.1128/jb.178.20.5853-5859.1996
Nishino, K., Latifi, T., and Groisman, E. A. (2006). Virulence and drug resistance roles of multidrug efflux systems of salmonella enterica serovar Typhimurium. Mol. Microbiol. 59, 126–141. doi: 10.1111/j.1365-2958.2005.04940.x
Nishino, K., Yamada, J., Hirakawa, H., Hirata, T., and Yamaguchi, A. (2003). Roles of 229 TolC-dependent multidrug transporters of Escherichia coli in resistance to b9 lactams. Antimicrob. Agents Chemother. 47, 3030–3033. doi: 10.1128/AAC.47.9.3030-3033.2003
Nishino, K., and Yamaguchi, A. (2001). Analysis of a complete library of putative drug transporter genes in Escherichia coli. J. Bacteriol. 183, 5803–5812. doi: 10.1128/JB.183.20.5803-5812.2001
Nishino, K., and Yamaguchi, A. (2004). Role of histone-like protein H-NS in multidrug resistance of Escherichia coli. J. Bacteriol. 186, 1423–1429. doi: 10.1128/JB.186.5.1423-1429.2004
Prouty, A. M., Brodsky, I. E., Falkow, S., and Gunn, J. S. (2004). Bile-salt-mediated induction of antimicrobial and bile resistance in salmonella typhimurium. Microbiology 150, 775–783. doi: 10.1099/mic.0.26769-0
Putman, M., Van Veen, H. W., and Konings, W. N. (2000). Molecular properties of bacterial multidrug transporters. Microbiol. Mol. Biol. Rev. 64, 672–693. doi: 10.1128/MMBR.64.4.672-693.2000
Rosenberg, E. Y., Bertenthal, D., Nilles, M. L., Bertrand, K. P., and Nikaido, H. (2003). Bile salts and fatty acids induce the expression of Escherichia coli AcrAB multidrug efflux pump through their interaction with rob regulatory protein. Mol. Microbiol. 48, 1609–1619. doi: 10.1046/j.1365-2958.2003.03531.x
Venter, H., Mowla, R., Ohene-Agyei, T., and Ma, S. (2015). RND-type drug efflux pumps from gram-negative bacteria: molecular mechanism and inhibition. Front. Microbiol. 06:377. doi: 10.3389/fmicb.2015.00377
Wotzka, S. Y., Kreuzer, M., Maier, L., Arnoldini, M., Nguyen, B. D., Brachmann, A. O., et al. (2019). Escherichia coli limits salmonella Typhimurium infections after diet shifts and fat-mediated microbiota perturbation in mice. Nat. Microbiol. 4, 2164–2174. doi: 10.1038/s41564-019-0568-5
Yoneda, T., Sakata, H., Yamasaki, S., Hayashi-Nishino, M., and Nishino, K. (2022). Analysis of multidrug efflux transporters in resistance to fatty acid salts reveals a TolC-independent function of EmrAB in salmonella enterica. PLoS One 17:e0266806. doi: 10.1371/journal.pone.0266806
Yoon, B. K., Jackman, J. A., Valle-Gonzalez, E. R., and Cho, N. J. (2018). Antibacterial free fatty acids and monoglycerides: biological activities, experimental testing, and therapeutic applications. Int. J. Mol. Sci. 19:1114. doi: 10.3390/ijms19041114
Zgurskaya, H. I., Krishnamoorthy, G., Ntreh, A., and Lu, S. (2011). Mechanism and function of the outer Membrane Channel TolC in multidrug resistance and physiology of Enterobacteria. Front. Microbiol. 2:189. doi: 10.3389/fmicb.2011.00189
Zgurskaya, H. I., and Nikaido, H. (2000). Multidrug resistance mechanisms: drug efflux across two membranes. Mol. Microbiol. 37, 219–225. doi: 10.1046/j.1365-2958.2000.01926.x
Keywords: bacteria, Escherichia coli, fatty acid salts, multidrug efflux pump, resistance
Citation: Yamasaki S, Yoneda T, Ikawa S, Hayashi-Nishino M and Nishino K (2023) Investigating multidrug efflux pumps associated with fatty acid salt resistance in Escherichia coli. Front. Microbiol. 14:954304. doi: 10.3389/fmicb.2023.954304
Edited by:
Hidetada Hirakawa, Gunma University, JapanReviewed by:
Ilyas Alav, University of Birmingham, United KingdomAriel Langevin, Ginkgo BioWorks, United States
Copyright © 2023 Yamasaki, Yoneda, Ikawa, Hayashi-Nishino and Nishino. This is an open-access article distributed under the terms of the Creative Commons Attribution License (CC BY). The use, distribution or reproduction in other forums is permitted, provided the original author(s) and the copyright owner(s) are credited and that the original publication in this journal is cited, in accordance with accepted academic practice. No use, distribution or reproduction is permitted which does not comply with these terms.
*Correspondence: Kunihiko Nishino, ✉ bmlzaGlub0BzYW5rZW4ub3Nha2EtdS5hYy5qcA==
†These authors have contributed equally to this work