- 1Guangdong Laboratory for Lingnan Modern Agriculture, College of Marine Sciences, South China Agricultural University, Guangzhou, Guangdong, China
- 2College of Veterinary Medicine, South China Agricultural University, Guangzhou, Guangdong, China
- 3Southern Marine Science and Engineering Guangdong Laboratory, Zhuhai Yueshun Aquaculture Co., Ltd., Zhuhai, China
- 4Laboratory for Marine Biology and Biotechnology, Qingdao, China
- 5Qingdao National Laboratory for Marine Science and Technology, Qingdao, China
The effect of structure of gut microbes on the health of host has attracted increasing attention. Sea bass Lateolabrax japonicus is an important farmed fish in China. The relationship of the dynamic changes of intestinal bacterial communities in L. japonicus and the cultural water environment is very important for healthy culture. Here, the diversity and abundance of the gut microbial communities of L. japonicus were evaluated during the culture using 16S rRNA Illumina sequencing. Both the opportunistic pathogens Aeromonas (1.68%), Vibrio (1.59%), and Acinetobacter (1.22%); and the potential probiotics Lactobacillus (2.27%), Bacillus (1.16%), and Lactococcus (0.37%) were distributed in the gut of L. japonicus. The increasing concentration of nitrogen of water environments with the increase of culture time significantly correlated with shifts in the microbial community structure: 40.04% of gut microbial changes due to nitrogen concentration. Higher concentrations of nitrogen showed a significantly negative correlation with intestinal probiotics in L. japonicus. The results indicate that the abundance of intestinal bacteria of L. japonicus is mainly driven by the changes of environmental factors (e.g., nitrogen), and it’s very important that the linking environmental parameters with bacterial data of guts could be used as an early warning indicator in L. japonicus heath culture.
Introduction
Gut microorganisms play a crucial role in the growth, development and health of the host, and the guts of fish also have a large number of bacteria (Jandhyala et al., 2015; Diwan et al., 2022). These bacterial communities are consist of probiotics, opportunistic bacteria, and neutral bacteria (Lv et al., 2021). Probiotics, one of important components of intestinal microorganisms, can exert immunoregulatory function, inhibit the proliferation of intestinal pathogens, and protect the integrity of the intestinal mucosal barrier (Cristofori et al., 2021). The gut is also a reservoir of pathogens. Normally, enteric pathogens are at a low abundance under interaction with intestinal probiotics (Vargas-Albores et al., 2021). However, the abundances of beneficial bacteria and pathogenic bacteria were different in diseased fish (Xiao et al., 2021; Yang et al., 2022), indicating that gut microbes might be closely related to the health of the host. It’s useful for controlling the diseases outbreak of fish according to the composition of the gut microbiota (Xiong et al., 2019; Das et al., 2022; Medina-Félix et al., 2022).
Fish live in the complex water environments, and the relationship between its gut microbes and the water environment is crucial for healthful culture (Jing et al., 2021). Deterioration of the water environment can cause various diseases of aquatic animals (Lieke et al., 2020). Dynamic changes of gut microbes of fish are influenced by both the fish themselves and environmental factors of water (Chen et al., 2022). Understanding the impact of the water environment on gut microbes is important to prevent aquaculture diseases, increase yields, and improve food quality (Wei et al., 2021; Chen et al., 2022).
Sea bass Lateolabrax japonicus is an important farmed fish in China. In 2021, the production of the farmed L. japonicus in China exceeded 199,000 tons (Fisheries Bureau of the Ministry of Agriculture, 2022). South China Sea is the major production areas, and the annual production of sea bass accounts for more than half of China (Zhao et al., 2018b; Zhang et al., 2022). At present, pond culture is the main culture method of L. japonicus in China. However, the water environment of the pond culture is relatively independent and has poor self-purification ability (Meerhoff and de los Ángeles González-Sagrario, 2022). Undigested feed, excreta and biological carcasses increase the concentration of nitrogen and phosphorus of water, and exceed the self-purification ability of water during culture (Liu et al., 2021). Bad water environment would directly lead to slow growth, yield reduction, disease outbreak, and even death of cultured fish (Boyd, 2017; Das et al., 2022).
Basis on this information, the structure of gut microbes of the farmed L. japonicus, and the relationship between gut microbes and the environmental factors of water were analyzed in this study, which would provide a theoretical basis for healthy culture of L. japonicus.
Materials and methods
Sample collection
The experimental pool was located in Zhuhai, China (113°14’57”E, 22°7’39.71”N), with an area of about 9,720 square meters (135 m × 72 m) and an average water depth of 2 m. The experimental sea bass was fed twice a day at a ratio of 3% body weight with puffed formula feed (Guangdong Yuehai Feed Group Co., Ltd., Zhanjiang, China).
The breeding cycle of sea bass in Guangdong, China is 1 year, and the environmental factors and climates were different in the four seasons. The times of sampling were set in the four seasons (January, April, August, and October). For each sampling, 1.8 m of water below the water surface was collected using a 2.5 L plexiglas water collector, filtered using 0.45 μM filtering membrane, and then used for analysis. Sixteen L. japonicus were randomly collected from the pond, and the length and the weight per fish were measurements. Contents from the second half of the gut of six L. japonicus were collected, and immediately frozen in liquid nitrogen for further analysis.
Determination of water physicochemical factors
Temperature and pH of the water were measured using a pH-meter (Sartorius, Göttingen, Germany). Total phosphorus, phosphate, total nitrogen, nitrate, nitrite, and ammonium nitrogen of cultured water were determined according to standard methods using UV-VIS spectrophotometer (P general, Beijing, China) (Zou and Cheng, 2002).
DNA extraction, PCR and sequencing
Microbial DNA of 100 mg of intestinal contents was extracted using the DNeasy Power Soil Pro Kit (QIANEN, Germany) according to the manufacturer’s instructions. DNA integrity was assessed using 2% agarose gel electrophoresis. DNA purity and concentration were tested using NanoDrop2000 (Thermo Fisher, USA). A total of 10 ng of genomic DNA was added to the polymerase chain reaction (PCR) system. PCR was performed to amplify the V4 region of the 16S rDNA gene using primers 515F (5’-GTGCCAGCMGCCGCGG-3’) and 806R (5’-GGACTACHVGGGTWTCTAAT-3’) with barcode. Each sample was performed in triplicates. PCR products were detected by 2% agarose gel electrophoresis, recovered using the AxyPrep DNA Gel Recovery Kit (AXYGEN, China), and quantified with QuantiFluor™ –ST blue fluorescence quantification system (Promega, USA). High-throughput sequencing was performed on the Illumina MiSeq platform (Illumina, USA) according to standard protocols developed by Majorbio Bio-Pharm Technology Co, Ltd (Shanghai, China).
Bioinformatic analysis
The raw data obtained were stitched into sequences, and the sequences were filtered. A total of 97% similarity sequences were sorted into the same OTU using Uparse 7.0.1090 software. The representative sequences of each OTU were aligned according to the silva database1 and classified using RDP Classifier. Principal co-ordinates analysis (PCoA), analysis of similarities (ANOSIM), Canonical correspondence analysis (CCA), variance partitioning analysis (VPA) and mantel test analysis were performed by the vegan 2.4-2 package of R 4.1.2 software. Bacterial taxonomic tree was carried out by Mega X. Species abundance heatmaps were carried out with the gplots 2.17.0 package of R 4.1.2. Collinear relation diagram is carried out by Circos 0.69-8 software. Bacterial FAPROTAX Function was predicted by FAPROTAX 1.2.6 software. Bacterial PICRUSt2 Function was predicted by PICRUSt2 2.2.0 software. Network analysis was carried out by QIIME 1.9.1 software. Diversity indices and bacterial abundances of different groups were compared using one-way ANOVA. Statistical significance was set at P < 0.05.
Results
Growth of L. japonicus and water physicochemical factors
Culture cycle of L. japonicus in South China was about 1 year, so the growth of L. japonicus and the changes of environmental factors in the four seasons were explored. As expected, the body length and body weight of L. japonicus were increased gradually with the time of culture (Figures 1A, B). Higher temperatures of water occurred in August, and lower water temperatures occurred in January (Figure 1C). There was little difference of water temperature in April and October. This result was consistent with the climatic conditions in the experimental locality. In the early stage of culture (April), the concentration of both nitrogen and phosphorus of cultured water were at a low level. With the increase of culture time, the concentration of total nitrogen, nitrate and ammonium nitrogen showed an increasing trend. The concentration of total phosphorus and phosphate was the highest in August. The highest concentration of nitrite was in October.
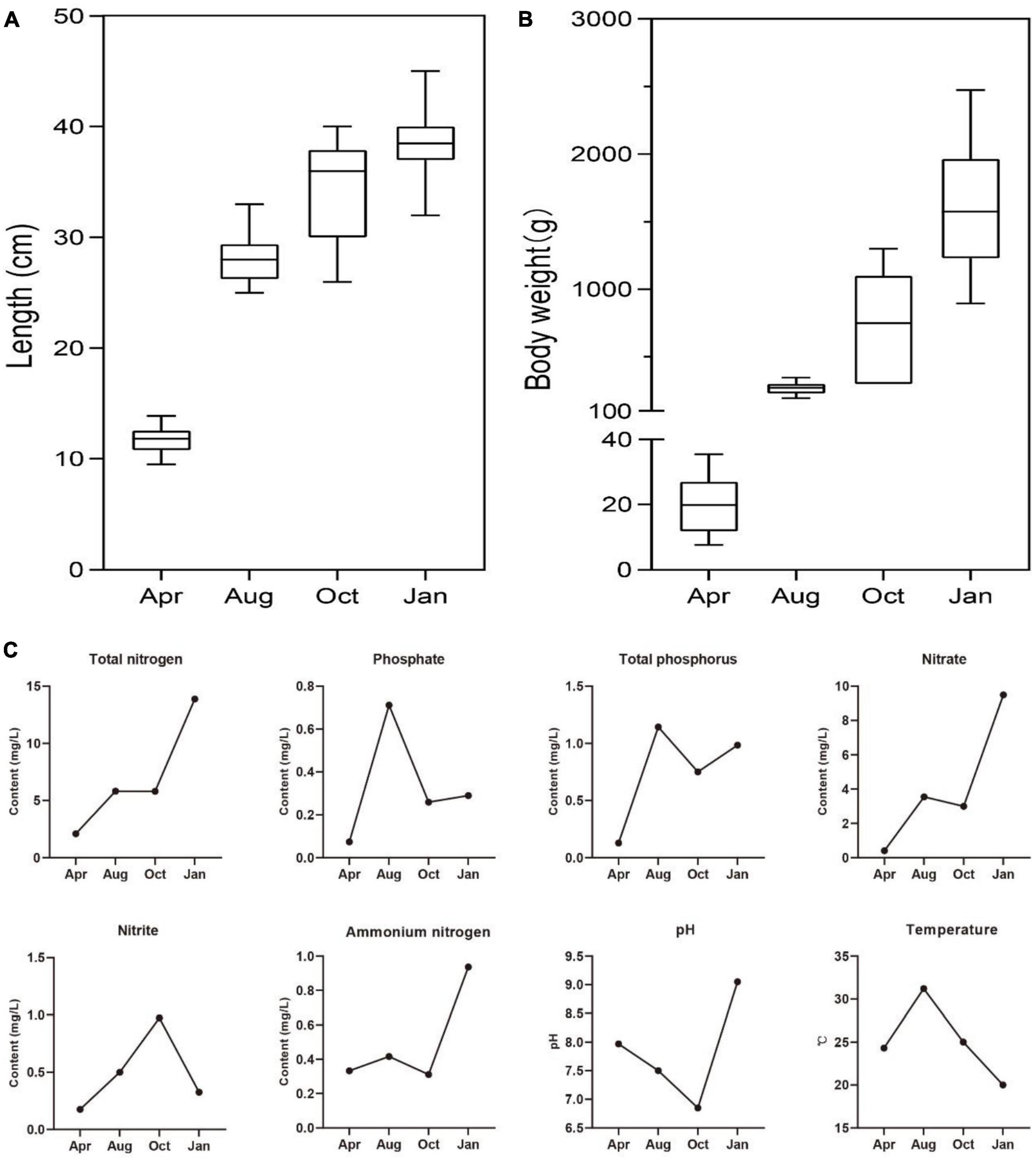
Figure 1. The growth status of farmed sea bass and the physicochemical factors of water. (A) The changes of sea bass body length. (B) The changes of sea bass body weight. (C) The changes of total phosphorus, phosphate, total nitrogen, nitrate, nitrite, ammonium nitrogen, pH, temperature in water.
Diversity of gut microbes
A total of 2,642,153 sequences were obtained from 24 samples, with an average length of 256 bp. A total of 2,609,399 of these sequences are high-quality valid sequences, with an average coverage rate of 98.76%. Shannon, Simpson, Chao and Sobs rarefaction curves also indicate that all sample sequences have sufficient coverage.
In order to analyze the diversity and abundance of gut microbial communities of L. japonicus at differential cultured times, PCoA was performed using weighted UniFrac distances. As shown in Figure 2A, There was no significant difference of the microbial community between January and August (ANOSIM, P > 0.05), but significant difference occurred in the remaining time points (ANOSIM, P < 0.05).
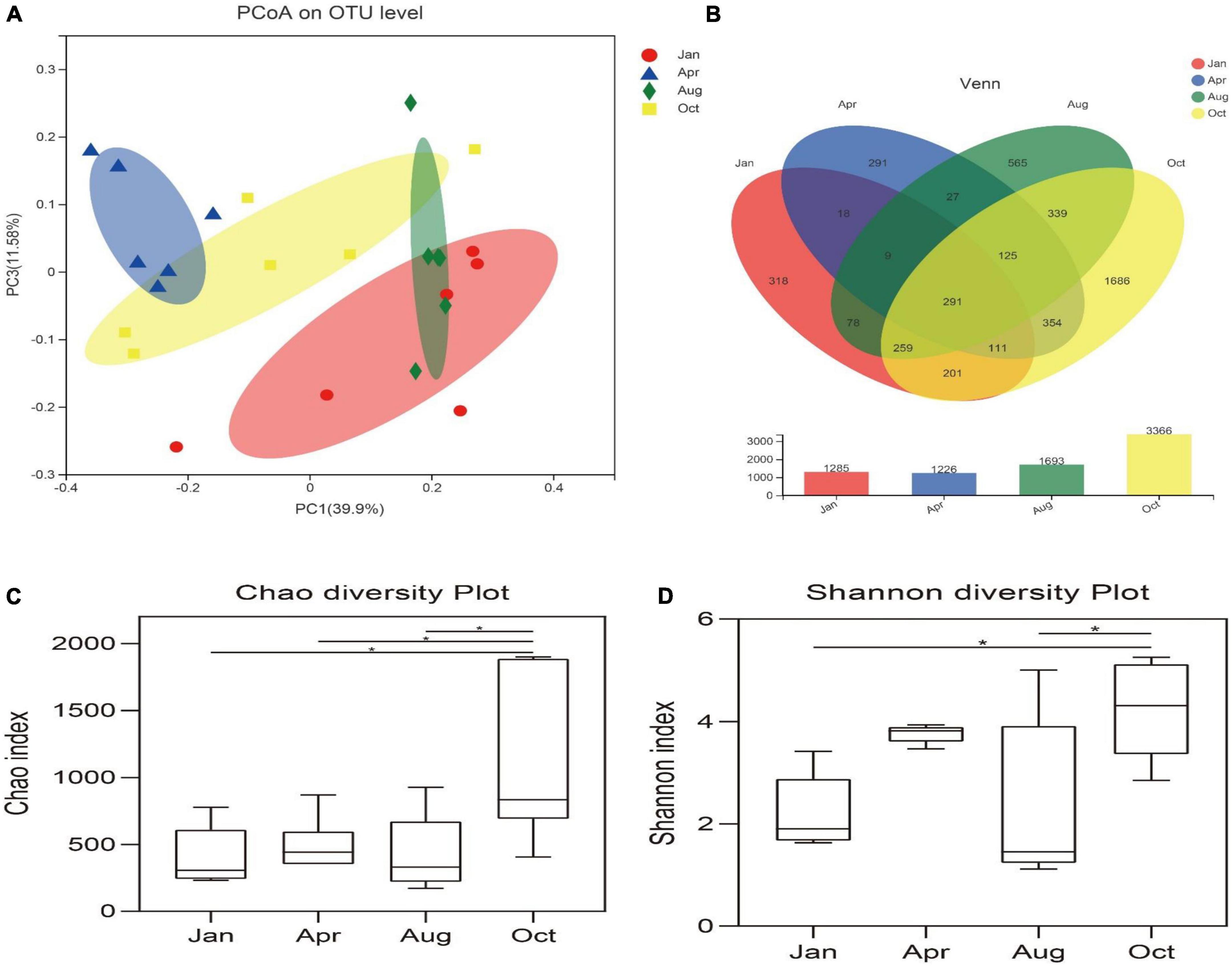
Figure 2. Diversity of gut microbes. (A) Principal co-ordinates analysis (PCoA) was performed at the OUT level based on weighted UniFrac distances for all samples. Different colored dots in the figure represent different groups. The x-axis and y-axis (PCoA1 and PCoA3) represent 33.9 and 11.58% of the variance, respectively. (B) Venn diagram represents sharing and unique OUT in Jan, Apr, Aug, Oct. Histograms represent the total number of OUT for each group. (C) Chao index indicates community distribution abundance. (D) Shannon index indicates diversity in community distribution. *p < 0.05.
The alpha diversity of the gut microbes was conducted using the Chao index, Shannon index and the number of OTUs. Based on 97% homology, high-quality valid sequences can be divided into 4,672 OUTs (Figure 2B). The Chao index of the gut microbes in October was significantly higher than that of other groups (P < 0.05), and there was no significant difference in the Chao index between other time points (P > 0.05) (Figure 2C). The Shannon index of the gut microbes in October was significantly higher than that in January and August (P < 0.05) (Figure 2D).
Composition and changes of gut microbes in L. japonicus
All 4,672 OUTs can be divided into 58 phylum, 160 classes, 356 orders, 612 family, and 1,318 genus. At the phylum classification level, the gut microbes of L. japonicus were mainly composed of Firmicutes (48.70%), Proteobacteria (22.65%), Cyanobacteria (10.75%), Actinobacteriota (9.71%), Bacteroidota (1.65%), and Planctomycetota (1.27%) (Figure 3A). Compared with that of April, the abundance of Cyanobacteria (P < 0.01), Actinobacteriota (P < 0.05) and Planctomycetota (P < 0.05) in October was significantly decreased. There were no significant differences in other major bacterial phyla between groups. As for genus, the predominant bacteria were Romboutsia (25.85%), Achromobacter (8.95%), Weissella (5.47%), Candidatus Arthromitus (4.92%), Mycobacterium (2.33%), Lactobacillus (2.27%), Aeromonas (1.68%), Vibrio (1.59%), Acinetobacter (1.22%), and Bacillus (1.16%) (Figure 3B). At the OUT level, the gut microbes were mainly from 13 OUTs. As shown in Figure 3C, OTU1035 (Candidatus Arthromitus), OUT1594 (Chloroplast) were undetectable in January. OTU1035 was significantly enriched in April and August. OTU2915 (Romboutsia) was significantly enriched in January and August. OTU1585 (Chloroplast) and OTU1594 were only significantly enriched in April.
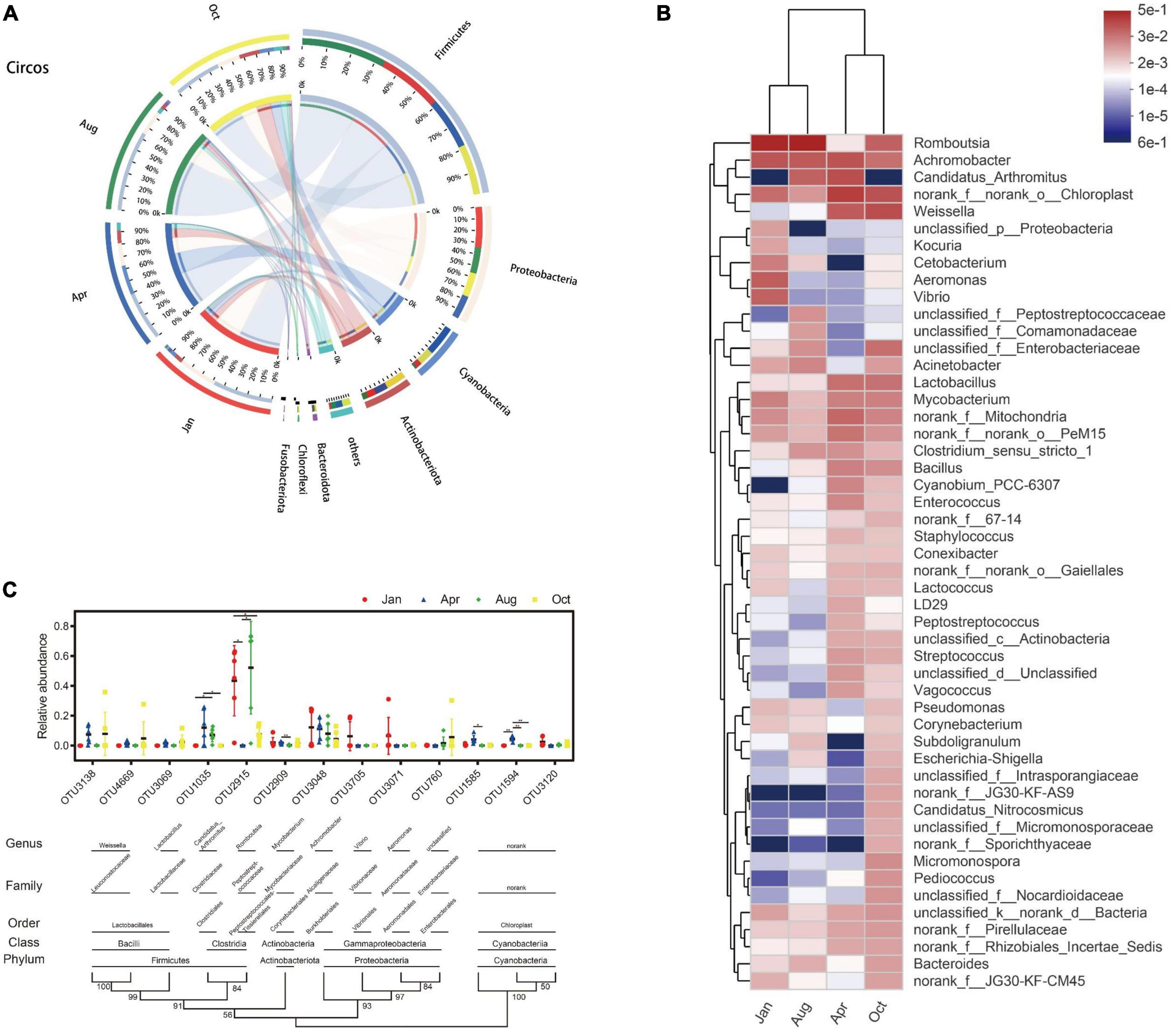
Figure 3. Composition and dynamic changes of gut microbes. (A) Collinear relation diagram between bacteria phylum and each group. Only phyla with an average abundance greater than 1% are presented, and the rest are merged into others. (B) Heatmap of bacterial genus level in each group. Only the top 50 abundance bacterial genera are shown in the figure. Bacterial genera and groups were clustered using the average algorithm. The color of the color blocks was used to indicate the relative abundance of each genus. (C) Classification and change of main OUT in each group. Only OUT with mean abundances greater than 1% are shown in the figure. OUT presented in the figure is clustered using the N-J algorithm. The values of the cluster tree represent the degree of reliability of this branch. Above the cluster tree are species classifications corresponding to OUT. Scatter plots were used to represent the abundance of OUT and mean ± standard error were presented. *p < 0.05, **p < 0.01.
The relationship between environmental factors and gut microbes
The effect of environmental factors of culture water on gut microbes was conducted by CCA. As shown in Figure 4A, The concentrations of total phosphorus, phosphate, total nitrogen, nitrate, nitrite or ammonium nitrogen had significant effects on gut microbes in L. japonicus (P < 0.01). VPA was used to further quantify the effect of the concentration of nitrogen and phosphorus on the gut microbes. The results showed that the concentration of nitrogen and phosphorus could explain 40.04% of the changes of gut microbes: 18.65% were explained by both nitrogen and phosphorus, 21.39% by nitrogen alone, and 0% by phosphorus alone (Figure 4B). Analysis of mantel test was used to reveal the correlation between the environment factors and the gut microbial function. As shown in Figure 4C, The FAPROTAX function of gut microbes had a significant correlation with the environment factors total phosphorus, phosphate, total nitrogen and nitrate (P < 0.01 or P < 0.05); the PICRUSt2 function of gut microbes was only significantly correlated with the phosphate (P < 0.01); the OUT of gut microbes was significantly correlated with total phosphorus, phosphate, total nitrogen, nitrate and nitrite (P < 0.01).
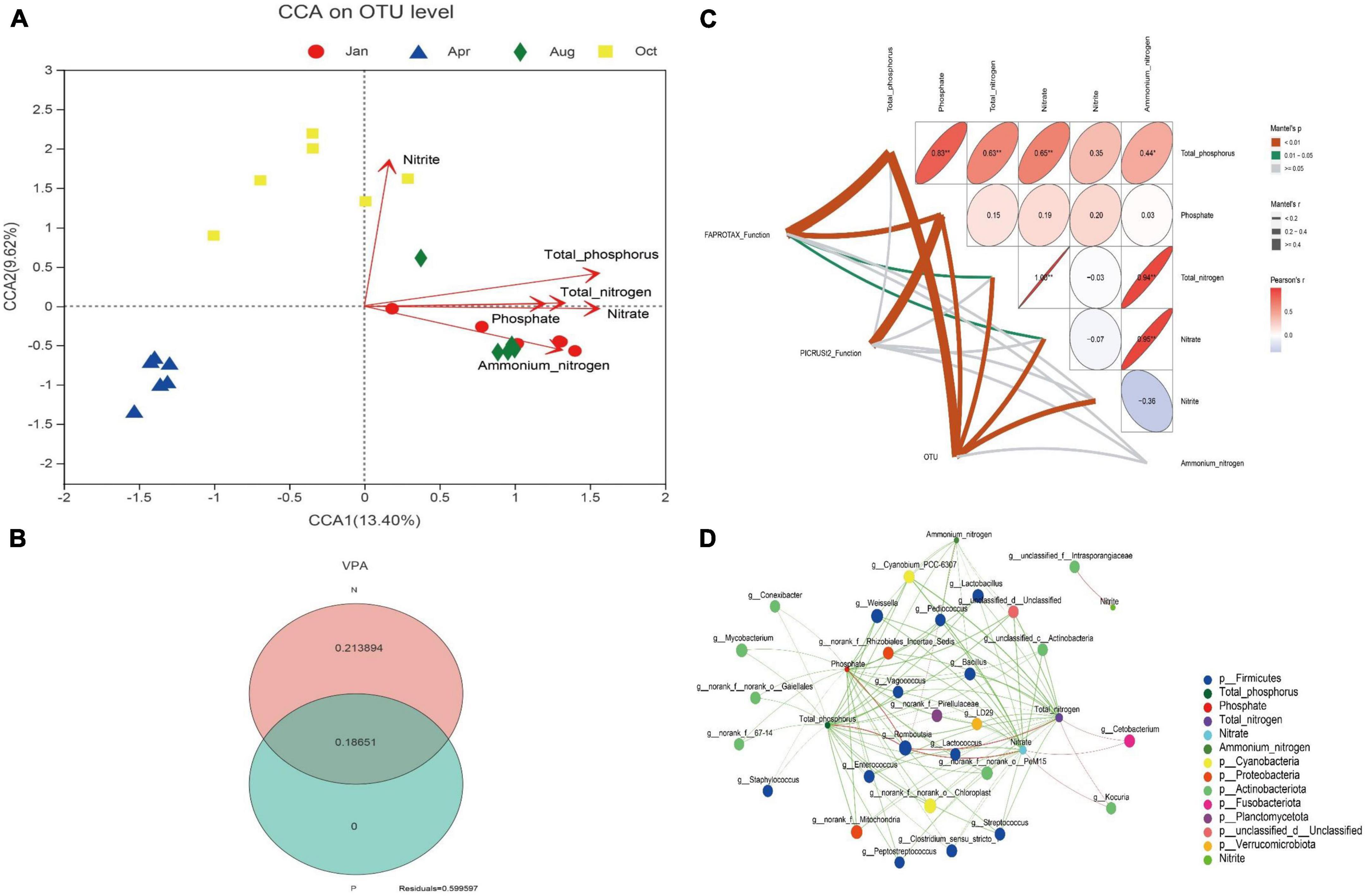
Figure 4. Relationship between nitrogen and phosphorus with gut microbes. (A) Canonical correspondence analysis (CCA) plot for OUT level. Different colored dots in the figure represent different groups. Red arrows are corresponding environmental factors. The length of arrows can represent the relative influence of environmental factors on OTU data. (B) Variance partitioning analysis (VPA) venn diagram. N indicates the explanation degree of changes in gut microbiota composition by total nitrogen, nitrate, nitrite and ammonium nitrogen in water. P indicates the explanation degree of changes in gut microbiota composition by total phosphorus and phosphate. (C) Mantel test analysis plot. Matrices represent correlations between environmental factors. In the matrix, the direction of the ellipse represents positive or negative correlation. The color and value of the ellipse represent the pearson correlation coefficient. *p < 0.05, **p < 0.01. The width of the line between FAPROTAX Function, PICRUSt2 function, and OUT to the environmental factor in the matrix represents the magnitude of their pearson correlation coefficient. The color of the line represents the significance of the correlation. (D) Network analysis plot. Pearson correlation coefficients were calculated between the top 50 bacterial genera in mean abundance and environmental factors. Nodes with absolute correlation coefficients greater than 0.5 and p < 0.05 were plotted. The circles in the figure represent different environmental agents or bacterial genera. The size of the circle represents the magnitude of the bacterial genus abundance or environmental factor values. Different colors represent different bacterial phyla or environmental agents. The color of the line indicates positive or negative correlation. Red indicates positive correlation, and green indicates negative correlation. The width of the line indicates the magnitude of the correlation coefficient.
To further search for bacterial genera associated with environmental factors, network analysis was performed. As shown in Figure 4D, Only one unclassified genus Intrasporangiaceae was significantly correlated with the concentration of nitrite (P < 0.05); Romboutsia was the only genus hat had a significant positive correlation with total phosphorus, phosphate, total nitrogen, and nitrate of water (P < 0.05); Kocuria and Cetobacterium had significant positive correlation with total nitrogen and nitrate (P < 0.05); and the other intestinal bacteria genera, especially Lactobacillus, Bacillus, and Lactococcus, were significantly negatively correlated with nitrogen and phosphorus (P < 0.05). The further analysis found that, the abundance of Lactobacillus, Bacillus and Lactococcus decreased by 96% (July), 98% (October), and 41% (January), respectively, compared with that of April (the beginning of the culture).
Discussion
Gut microbes play an important role in the biological processing and the health of the hosts. In this study, the diversity, dynamic changes of gut bacteria and the environmental factors of water were explored during the L. japonicus culture.
The intestines of fish are important repositories of bacteria (Zhao et al., 2018a). Understanding the species of intestinal bacteria (e.g., pathogenic bacteria) of fish are important for its healthy culture (Tarnecki et al., 2017; Ou et al., 2021). This study showed that Aeromonas (1.68%), Vibrio (1.59%), and Acinetobacter (1.22%) are major members of gut microbes in farmed L. japonicus. The presence of the opportunistic pathogens is a non-negligible threat to L. japonicus. Aeromonas is widely distributed in aquatic ecosystems, and the gut of various aquatic animals (Pessoa et al., 2019). Some Aeromonas (e.g., A. hydrophila) have been proven to be significant pathogen to fish, and cause fish disease when fish was a low immunity or stressful conditions (Nhinh et al., 2021). Vibrio is one of the most important pathogens of fish, and causes huge economic losses to the fish industry every year (Sheikh et al., 2022). Members of the Vibrio have a strong ability to generate biofilms, and therefore Vibrio possess strong tolerance to antimicrobials and the host immune system (Arunkumar et al., 2020). Previous studies have shown that Acinetobacter only causes the infection of humans (Carvalheira et al., 2021). But now, some members of the Acinetobacter genus can cause the diseases of fish (Cao et al., 2016; Kuebutornye et al., 2020). Potential pathogens in the gut microbiota can cause damage when host immunity is low (Pérez-Sánchez et al., 2018).
Microorganisms in the surrounding environment are one of the important sources of gut microbes in fish (Sun et al., 2019). Aeromonas, Vibrio, and Acinetobacter are common residents in water environment (Diwan et al., 2022). Our study showed that these opportunistic pathogens are also able to colonize the intestinal tract of L. japonicus. Normally, the intestinal mucosal immunity and mucosal barrier of fish can control the composition of intestinal microorganisms and prevent the massive proliferation and invasion of fish pathogens (Okumura and Takeda, 2018). However, when the environment changes dramatically or outbreaks occur during aquaculture, these pathogenic bacteria will proliferate abnormally and further aggravate the condition. In the past, multiple antibiotics were extensively used response to bacterial infections during culture. However, the abuse of antibiotics leads to serious food safety, environmental pollution, and bacterial resistance problems. Therefore, it is particularly important to use a more environmentally friendly and safe method to shape the gut microbes of L. japonicus to reduce bacterial diseases during L. japonicus culture (Shao et al., 2021).
Probiotics are widely used for enhancing immunity, helping digestion, improving water quality, and promoting growth and reproduction (Banerjee and Ray, 2017; Yilmaz et al., 2022). In this study, the potential probiotics Lactobacillus (2.27%), Bacillus (1.16%), and Lactococcus (0.37%) were detected from the intestines of L. japonicus, indicating that these bacteria have good colonization ability, and can be applied in its culture. Lactobacillus and Lactococcus are both lactic acid-producing bacteria and are important for enhancing immunity and disease resistance in animals (Van Doan et al., 2020). Bacillus as a probiotic has been shown to improve feed utilization thereby promoting growth and enhancing immunity to combat disease, while improving aquaculture water quality (Kuebutornye et al., 2019; Hlordzi et al., 2020).
With the increase of farming time in L. japonicus pond, the concentration of nitrogen and phosphorus of the water also increased continuously. In the middle and late stage of culture, the food intake of L. japonicus increased rapidly, and the faces at the bottom continued to accumulate, resulting the enrichment of nitrogen and phosphorus in the water. Previous studies have shown that fish only absorb about 20% of the phosphorus in the feed, and majority of phosphorus in the feed was released into the water (Hua and Bureau, 2006; Kong et al., 2020). High concentration of phosphorus of water can cause the overgrowth of algae and weeds, as well as oxygen depletion and toxin production (Kong et al., 2020). The enrichment of ammonia nitrogen of water might change the pH of fish blood and reduce the ability of blood to carry oxygen (Xu et al., 2021). High concentration of ammonia nitrogen of culture water will lead to oxidative stress of fish, resulting in a decrease of antioxidant enzymes and the activity of superoxide dismutase (Xu et al., 2021). Meanwhile, ammonia stress can directly damage the gut of fish and cause immuno-suppression (Xu et al., 2021). The gut mucosal immunity of fish is an important means to control the composition of gut microbes (Brown et al., 2013; Yoo et al., 2020). When fish were cultured in a high concentration of nitrogen-phosphorus, the composition and function of the gut microbiota would be affected (Li et al., 2021). Analysis of VPA here showed that nitrogen of water was able to explain 40.04% of the changes of gut microbes, and 18.65% of the changes the gut microbes was explained by both nitrogen and phosphorus of water. The gut microbiota has multiple metabolic and biosynthetic functions, so it is more important to study the function of gut microbes (Torsvik and Øvreås, 2002; Bosco and Noti, 2021). Mantel test analysis showed a significant correlation between phosphate and the PICRUSt2 function of the gut microbiota (P < 0.01). FAPROTAX mainly predicts the metabolic function of gut microbes to substances such as nitrogen and phosphorus in the environment. FAPROTAX function was significantly correlated with total phosphorus, phosphate, total nitrogen and nitrate (P < 0.01 or P < 0.05). Therefore, the function of gut microbes of L. japonicus can be changed by regulating environmental factors of pond.
Network analysis showed that the concentrations of nitrogen and phosphorus of water were significantly negatively correlated with most bacterial genera including the probiotics Lactobacillus, Bacillus, and Lactococcus. With the increasing of nitrogen and phosphorus during culture, the abundance of potential probiotics decreased. Therefore, the enrichment of nitrogen and phosphorus in the aqueous environment is not conducive to the colonization of intestinal probiotics. Feeding probiotics in L. japonicus pond culture should ensure that nitrogen and phosphorus in water are at a low level. Romboutsia can be isolated in the gut of a variety of animals both aquatic and terrestrial (Gerritsen et al., 2019). It has been shown that Romboutsia are significantly enriched in fish fed fishmeal (Habte-Tsion et al., 2022). Romboutsia was the only genus which showed a significant positive correlation with nitrogen and phosphorus in this study (P < 0.05). Romboutsia may therefore be a manifestation of L. japonicus adaptation to nitrogen-phosphorus enriched environments. Studying the interaction between Romboutsia and the host is important for exploring the effects of nitrogen and phosphorus on the host.
Conclusion
The diversity and abundance of gut bacterial communities of L. japonicus were studied, and its dynamic changes response to the environmental factors of water were elucidated. Both the opportunistic pathogens (Aeromonas, Vibrio, and Acinetobacter); and the potential probiotics (Lactobacillus, Bacillus, and Lactococcus) were distributed in the gut of L. japonicus. The environmental factors of water affect the diversity of bacterial communities. The concentration of nitrogen and phosphorus significantly drive the composition and function of gut microbes during culture, and higher concentration of nitrogen adversely could reduce the ratio of probiotics of gut bacterial communities in L. japonicus. The results would help us explore the relationship of environmental parameters of water and bacterial communities of guts, and would be used as an early warning indicator in L. japonicus heath culture.
Data availability statement
The data presented in this study are deposited in the NCBI, accession number: PRJNA898861.
Ethics statement
This animal study was reviewed and approved by the Ethics Committee of South China Agricultural University.
Author contributions
Q-WQ, H-YS, and J-DC conceived the research. ZZ, Y-MX, J-HL, WH, and S-TW performed the experiments. ZZ wrote the manuscript. X-HH and Y-HH edited the manuscript. X-YZ contributed sampling or data analysis pipelines. All authors reviewed and approved the manuscript.
Funding
This research was supported by grants from the Key-Area Research and Development Program of Guangdong Province (2021B0202040002), National Key R&D Program of China (2018YFD0900500), Natural Science Foundation of Guangdong, China (2022A1515010648), National Natural Science Foundation of China (U20A20102 and 31930115), the China Agriculture Research System of MOF and MARA (CARS-47-G16), Science and Technology Planning Project of Guangdong Province, China (2020B1212060058 and LAMB20221004), State Key Laboratory of Applied Microbiology Southern China (Grant No. SKLAM001-2021), Science and Technology Planning Project of Guangdong Province, China (2020B1212060058), Guangdong Provincial Key Laboratory of Applied Marine Biology (LAMB20221004), and Foundation of Laboratory of Lingnan Modern Agriculture Project (NT2021008).
Acknowledgments
We thank all of the members of the Zhuhai Yueshun Aquaculture Co., Ltd., Zhuhai, China for their assistance in field sample collection.
Conflict of interest
X-YZ and Q-WQ were employed by Zhuhai Yueshun Aquaculture Co., Ltd.
The remaining authors declare that the research was conducted in the absence of any commercial or financial relationships that could be construed as a potential conflict of interest.
Publisher’s note
All claims expressed in this article are solely those of the authors and do not necessarily represent those of their affiliated organizations, or those of the publisher, the editors and the reviewers. Any product that may be evaluated in this article, or claim that may be made by its manufacturer, is not guaranteed or endorsed by the publisher.
Footnotes
References
Arunkumar, M., LewisOscar, F., Thajuddin, N., Pugazhendhi, A., and Nithya, C. (2020). In vitro and in vivo biofilm forming Vibrio spp: A significant threat in aquaculture. Process Biochem. 94, 213–223. doi: 10.1016/j.procbio.2020.04.029
Banerjee, G., and Ray, A. K. (2017). The advancement of probiotics research and its application in fish farming industries. Res. Vet. Sci. 115, 66–77. doi: 10.1016/j.rvsc.2017.01.016
Bosco, N., and Noti, M. (2021). The aging gut microbiome and its impact on host immunity. Genes Immun. 22, 289–303. doi: 10.1038/s41435-021-00126-8
Boyd, C. E. (2017). “General relationship between water quality and aquaculture performance in ponds,” in Fish diseases: Prevention and control strategies, ed. G. Jeney (London: Elsevier), 147–166. doi: 10.1016/B978-0-12-804564-0.00006-5
Brown, E. M., Sadarangani, M., and Finlay, B. B. (2013). The role of the immune system in governing host-microbe interactions in the intestine. Nat. Immunol. 14, 660–667. doi: 10.1038/ni.2611
Cao, H., Ye, W., He, S., Li, Y., and Yang, Y. (2016). Acinetobacter lwoffii: An emerging pathogen for red head disease in farmed channel catfish Ictalurus punctatus. Israeli J. Aquac. Bamidgeh 68:20799. doi: 10.46989/001c.20799
Carvalheira, A., Silva, J., and Teixeira, P. (2021). Acinetobacter spp. in food and drinking water–a review. Food Microbiol. 95:103675. doi: 10.1016/j.fm.2020.103675
Chen, C.-Z., Li, P., Liu, L., and Li, Z.-H. (2022). Exploring the interactions between the gut microbiome and the shifting surrounding aquatic environment in fisheries and aquaculture: A review. Environ. Res. 214:114202. doi: 10.1016/j.envres.2022.114202
Cristofori, F., Dargenio, V. N., Dargenio, C., Miniello, V. L., Barone, M., and Francavilla, R. (2021). Anti-inflammatory and immunomodulatory effects of probiotics in gut inflammation: A door to the body. Front. Immunol. 12:578386. doi: 10.3389/fimmu.2021.578386
Das, S. K., Mandal, A., and Khairnar, S. O. (2022). Aquaculture resources and practices in a changing environment. Sustain. Agric. Syst. Technol. 169–199. doi: 10.1002/9781119808565.ch8
Diwan, A. D., Harke, S. N., and Panche, A. N. (2022). Aquaculture industry prospective from gut microbiome of fish and shellfish: An overview. J. Anim. Physiol. Anim. Nutr. 106, 441–469. doi: 10.1111/jpn.13619
Fisheries Bureau of the Ministry of Agriculture (2022). China fishery statistics yearbook 2022. Beijing: China Agriculture Press.
Gerritsen, J., Hornung, B., Ritari, J., Paulin, L., Rijkers, G. T., Schaap, P. J., et al. (2019). A comparative and functional genomics analysis of the genus Romboutsia provides insight into adaptation to an intestinal lifestyle. BioRxiv [Preprint]. doi: 10.1101/845511
Habte-Tsion, H., Riche, M., Mejri, S., Bradshaw, D., Wills, P. S., Myers, J. J., et al. (2022). The effects of fish meal substitution by clam meal on the growth and health of Florida pompano (Trachinotus carolinus). Sci. Rep. 12, 1–16. doi: 10.1038/s41598-022-11675-x
Hlordzi, V., Kuebutornye, F. K., Afriyie, G., Abarike, E. D., Lu, Y., Chi, S., et al. (2020). The use of Bacillus species in maintenance of water quality in aquaculture: A review. Aquac. Rep. 18:100503. doi: 10.1016/j.aqrep.2020.100503
Hua, K., and Bureau, D. (2006). Modelling digestible phosphorus content of salmonid fish feeds. Aquaculture 254, 455–465. doi: 10.1016/j.aquaculture.2005.10.019
Jandhyala, S. M., Talukdar, R., Subramanyam, C., Vuyyuru, H., Sasikala, M., and Reddy, D. N. (2015). Role of the normal gut microbiota. World J. Gastroenterol. 21:8787. doi: 10.3748/wjg.v21.i29.8787
Jing, X., Su, S., Zhang, C., Zhu, J., Hou, Y., Li, Z., et al. (2021). Dynamic changes in microbial community structure in farming pond water and their effect on the intestinal microbial community profile in juvenile common carp (Cyprinus carpio L.). Genomics 113, 2547–2560. doi: 10.1016/j.ygeno.2021.05.024
Kong, W., Huang, S., Yang, Z., Shi, F., Feng, Y., and Khatoon, Z. (2020). Fish feed quality is a key factor in impacting aquaculture water environment: Evidence from incubator experiments. Sci. Rep. 10, 1–15. doi: 10.1038/s41598-019-57063-w
Kuebutornye, F. K., Abarike, E. D., and Lu, Y. (2019). A review on the application of Bacillus as probiotics in aquaculture. Fish Shellfish Immunol. 87, 820–828. doi: 10.1016/j.fsi.2019.02.010
Kuebutornye, F. K., Abarike, E. D., Lu, Y., Hlordzi, V., Sakyi, M. E., Afriyie, G., et al. (2020). Mechanisms and the role of probiotic Bacillus in mitigating fish pathogens in aquaculture. Fish Physiol. Biochem. 46, 819–841. doi: 10.1007/s10695-019-00754-y
Li, J., Fang, L., Liang, X.-F., Guo, W., Lv, L., and Li, L. (2021). Influence of environmental factors and bacterial community diversity in pond water on health of Chinese perch through gut microbiota change. Aquac. Rep. 20:100629. doi: 10.1016/j.aqrep.2021.100629
Lieke, T., Meinelt, T., Hoseinifar, S. H., Pan, B., Straus, D. L., and Steinberg, C. E. (2020). Sustainable aquaculture requires environmental-friendly treatment strategies for fish diseases. Rev. Aquac. 12, 943–965. doi: 10.1111/raq.12365
Liu, X., Shao, Z., Cheng, G., Lu, S., Gu, Z., Zhu, H., et al. (2021). Ecological engineering in pond aquaculture: A review from the whole-process perspective in China. Rev. Aquac. 13, 1060–1076. doi: 10.1111/raq.12512
Lv, Z., Xiong, D., Shi, J., Long, M., and Chen, Z. (2021). The interaction between viruses and intestinal microbiota: A review. Curr. Microbiol. 78, 3597–3608. doi: 10.1007/s00284-021-02623-5
Medina-Félix, D., Garibay-Valdez, E., Vargas-Albores, F., and Martínez-Porchas, M. (2022). Fish disease and intestinal microbiota: A close and indivisible relationship. Rev. Aquac. 15, 820–839. doi: 10.1111/raq.12762
Meerhoff, M., and de los Ángeles González-Sagrario, M. (2022). Habitat complexity in shallow lakes and ponds: Importance, threats, and potential for restoration. Hydrobiologia 849, 3737–3760. doi: 10.1007/s10750-021-04771-y
Nhinh, D. T., Le, D. V., Van, K. V., Huong Giang, N. T., Dang, L. T., and Hoai, T. D. (2021). Prevalence, virulence gene distribution and alarming the multidrug resistance of Aeromonas hydrophila associated with disease outbreaks in freshwater aquaculture. Antibiotics 10:532. doi: 10.3390/antibiotics10050532
Okumura, R., and Takeda, K. (2018). Maintenance of intestinal homeostasis by mucosal barriers. Inflamm. Regen. 38, 1–8. doi: 10.1186/s41232-018-0063-z
Ou, W., Yu, G., Zhang, Y., and Mai, K. (2021). Recent progress in the understanding of the gut microbiota of marine fishes. Mar. Life Sci. Technol. 3, 434–448. doi: 10.1007/s42995-021-00094-y
Pérez-Sánchez, T., Mora-Sánchez, B., and Balcázar, J. L. (2018). Biological approaches for disease control in aquaculture: Advantages, limitations and challenges. Trends Microbiol. 26, 896–903. doi: 10.1016/j.tim.2018.05.002
Pessoa, R. B. G., de Oliveira, W. F., Marques, D. S. C., dos Santos Correia, M. T., de Carvalho, E. V. M. M., and Coelho, L. C. B. B. (2019). The genus Aeromonas: A general approach. Microb. Pathog. 130, 81–94. doi: 10.1016/j.micpath.2019.02.036
Shao, Y., Wang, Y., Yuan, Y., and Xie, Y. (2021). A systematic review on antibiotics misuse in livestock and aquaculture and regulation implications in China. Sci. Total Environ. 798:149205. doi: 10.1016/j.scitotenv.2021.149205
Sheikh, H., John, A., Musa, N., Alfatama, M., and Fadhlina, A. (2022). Vibrio spp. and their Vibriocin as a Vibriosis control measure in aquaculture. Appl. Biochem. Biotechnol. 194, 4477–4491. doi: 10.1007/s12010-022-03919-3
Sun, F., Wang, Y., Wang, C., Zhang, L., Tu, K., and Zheng, Z. (2019). Insights into the intestinal microbiota of several aquatic organisms and association with the surrounding environment. Aquaculture 507, 196–202. doi: 10.1016/j.aquaculture.2019.04.026
Tarnecki, A. M., Burgos, F. A., Ray, C. L., and Arias, C. R. (2017). Fish intestinal microbiome: Diversity and symbiosis unravelled by metagenomics. J. Appl. Microbiol. 123, 2–17. doi: 10.1111/jam.13415
Torsvik, V., and Øvreås, L. (2002). Microbial diversity and function in soil: From genes to ecosystems. Curr. Opin. Microbiol. 5, 240–245. doi: 10.1016/S1369-5274(02)00324-7
Van Doan, H., Hoseinifar, S. H., Ringø, E., Ángeles Esteban, M., Dadar, M., Dawood, M. A., et al. (2020). Host-associated probiotics: A key factor in sustainable aquaculture. Rev. Fish. Sci. Aquac. 28, 16–42. doi: 10.1080/23308249.2019.1643288
Vargas-Albores, F., Martínez-Córdova, L. R., Hernández-Mendoza, A., Cicala, F., Lago-Lestón, A., and Martínez-Porchas, M. (2021). Therapeutic modulation of fish gut microbiota, a feasible strategy for aquaculture? Aquaculture 544:737050. doi: 10.1016/j.aquaculture.2021.737050
Wei, D., Xing, C., Hou, D., Zeng, S., Zhou, R., Yu, L., et al. (2021). Distinct bacterial communities in the environmental water, sediment and intestine between two crayfish-plant coculture ecosystems. Appl. Microbiol. Biotechnol. 105, 5087–5101. doi: 10.1007/s00253-021-11369-w
Xiao, F., Liao, L., Xu, Q., He, Z., Xiao, T., Wang, J., et al. (2021). Host–microbiota interactions and responses to grass carp reovirus infection in Ctenopharyngodon idellus. Environ. Microbiol. 23, 431–447. doi: 10.1111/1462-2920.15330
Xiong, J.-B., Nie, L., and Chen, J. (2019). Current understanding on the roles of gut microbiota in fish disease and immunity. Zool. Res. 40:70.
Xu, Z., Cao, J., Qin, X., Qiu, W., Mei, J., and Xie, J. (2021). Toxic effects on bioaccumulation, hematological parameters, oxidative stress, immune responses and tissue structure in fish exposed to ammonia nitrogen: A review. Animals 11:3304. doi: 10.3390/ani11113304
Yang, Y., Lin, H., Xing, Y., Wen, J., Fang, W., Zhang, J., et al. (2022). Response signatures of intestinal microbiota and gene transcription of the tiger grouper Epinephelus fuscoguttatus to nervous necrosis virus infection. Aquaculture 550:737848. doi: 10.1016/j.aquaculture.2021.737848
Yilmaz, S., Yilmaz, E., Dawood, M. A., Ringø, E., Ahmadifar, E., and Abdel-Latif, H. M. (2022). Probiotics, prebiotics, and synbiotics used to control Vibriosis in fish: A review. Aquaculture 547:737514. doi: 10.1016/j.aquaculture.2021.737514
Yoo, J. Y., Groer, M., Dutra, S. V. O., Sarkar, A., and McSkimming, D. I. (2020). Gut microbiota and immune system interactions. Microorganisms 8:1587. doi: 10.3390/microorganisms8101587
Zhang, Z., Fang, X., and Sun, L. (2022). Comparison for ecological economic performance of Chinese sea perch (Lateolabrax maculatus) under different aquaculture systems. Aquac. Fish. 7, 683–692. doi: 10.1016/j.aaf.2021.02.004
Zhao, Y., Peng, W., Guo, H., Chen, B., Zhou, Z., Xu, J., et al. (2018b). Population genomics reveals genetic divergence and adaptive differentiation of Chinese sea bass (Lateolabrax maculatus). Mar. Biotechnol. 20, 45–59. doi: 10.1007/s10126-017-9786-0
Zhao, Y., Duan, C., Zhang, X., Chen, H., Ren, H., Yin, Y., et al. (2018a). Insights into the gut microbiota of freshwater shrimp and its associations with the surrounding microbiota and environmental factors. J. Microbiol. Biotechnol. 28, 946–956. doi: 10.4014/jmb.1709.09070
Keywords: gut microbes, bacterial community, environmental factors, Lateolabrax japonicus, sea bass ponds
Citation: Zhu Z, Xu Y-M, Liang J-H, Huang W, Chen J-D, Wu S-T, Huang X-H, Huang Y-H, Zhang X-Y, Sun H-Y and Qin Q-W (2023) Relationship of environmental factors in pond water and dynamic changes of gut microbes of sea bass Lateolabrax japonicus. Front. Microbiol. 14:1086471. doi: 10.3389/fmicb.2023.1086471
Received: 15 December 2022; Accepted: 14 March 2023;
Published: 30 March 2023.
Edited by:
Ram Krishan Negi, University of Delhi, IndiaReviewed by:
Ajaya Kumar Rout, Central Inland Fisheries Research Institute (ICAR), IndiaKai Peng, Guangdong Academy of Agricultural Sciences (GDAAS), China
Copyright © 2023 Zhu, Xu, Liang, Huang, Chen, Wu, Huang, Huang, Zhang, Sun and Qin. This is an open-access article distributed under the terms of the Creative Commons Attribution License (CC BY). The use, distribution or reproduction in other forums is permitted, provided the original author(s) and the copyright owner(s) are credited and that the original publication in this journal is cited, in accordance with accepted academic practice. No use, distribution or reproduction is permitted which does not comply with these terms.
*Correspondence: Hong-Yan Sun, aG9uZ3lhbmx1Y2t5QHNjYXUuZWR1LmNu; Qi-Wei Qin, cWlucXdAc2NhdS5lZHUuY24=
†These authors have contributed equally to this work