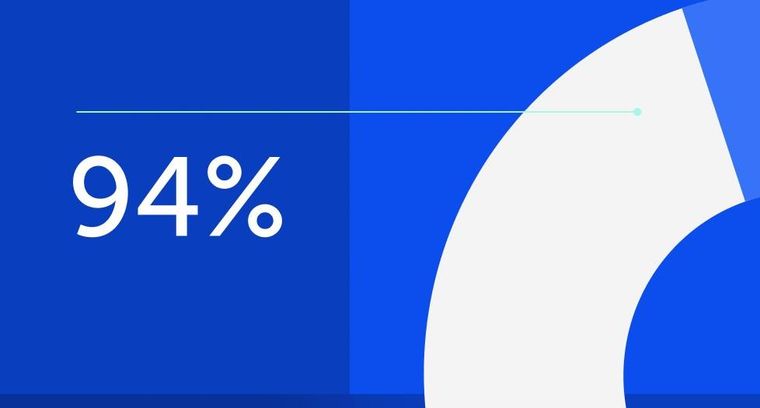
94% of researchers rate our articles as excellent or good
Learn more about the work of our research integrity team to safeguard the quality of each article we publish.
Find out more
ORIGINAL RESEARCH article
Front. Microbiol., 04 November 2022
Sec. Microbe and Virus Interactions with Plants
Volume 13 - 2022 | https://doi.org/10.3389/fmicb.2022.974526
This article is part of the Research TopicEndophytic Fungi: Secondary Metabolites and Plant Biotic and Abiotic Stress ManagementView all 14 articles
Benzoin resin, produced by the native Indonesian trees Styrax sumatrana and Styrax benzoin, has been incorporated into medical practices to treat wounds, erythema, and many other conditions for centuries. Endophytic fungi that reside within medicinal plants have antimicrobial, antioxidant, and α-glucosidase inhibitory capacities, contributing to plant health and derivative products. In this study, we determined the antifungal, antioxidant, and α-glucosidase inhibitory capacities of endophytic fungal isolates from three different tissues (leaves, bark, and stems) of S. sumatrana and S. benzoin trees. The genera of fungal isolates were determined by phylogenetic analysis of internal transcribed spacer sequences. A total of 58 fungal isolates were classified into 15 different fungal genera from eight taxonomic orders—Hypocreales, Botryosphaeriales, Glomerellales, Diaphortales, Pleosporales, Eurotiales, Xylariales, and Mucorales—with a pattern of host species specificity. Among these isolates, Trichoderma sp. 6407 consistently exhibited high inhibition of the growth of plant pathogens Fusarium sp., Trichoderma viride, and Aspergillus niger. With respect to antioxidant activity, Phyllosticta sp. 6454 consistently showed 2,2-diphenyl-1-picrylhydrazyl inhibition (37.59 ± 0.05%), 2,2′-azino-bis (3-ethylbenzothiazoline-6-sulphonic acid)-based antioxidant activity (25.04 ± 0.27 mgTE/g), and α-glucosidase inhibitory activity (52.15 ± 10.08%). Neopestalotiopsis sp. 6431 was notably potent in 2,2-diphenyl-1-picrylhydrazyl inhibition (49.65 ± 0.80%), ferric reducing antioxidant power-based antioxidant activity (197.49 ± 8.65 mgTE/g), and α-glucosidase inhibitory activity (52.88 ± 4.93%). This study revealed that Trichoderma sp. 6407, Phyllosticta sp. 6454, and Neopestalotiopsis sp. 6431 exhibited antifungal, antioxidant, and α-glucosidase inhibitory activities.
Humankind has long used fragrances for health, beauty, and ceremonial purposes. The burning of fragrant incense has been observed in numerous cultures and religious ceremonies (Nir, 2004; Smit, 2004; Baum, 2013; Ergin, 2014; le Maguer, 2015; Milburn, 2016; Gershon, 2019). Ancient Greek, early Christian, Jewish, and Islamic societies used or use various fragrances, including balsamic resin, in their ritual practices (Haran, 1960; Nir, 2004; Smit, 2004; Ergin, 2014; le Maguer, 2015; Gershon, 2019). Balsamic resin has been traditionally used in Chinese culture for ceremonial and medicinal purposes, and the tradition has spread to other Asian countries (Milburn, 2016). Such practices have made the substance a valuable trading commodity (Kashio and Johnson, 2001; Ergin, 2014; le Maguer, 2015).
Styrax trees produce a balsamic resin known as benzoin resin (Kashio and Johnson, 2001). Styrax sumatrana and Styrax benzoin are native Indonesian trees that are widely cultivated in North Sumatra, Indonesia, where their resin has been traditionally used for herbal remedies and cultural ceremonies. For such applications, benzoin resin is valuable as a nontimber forest product (Nurwahyuni et al., 2021). Benzoin resin and its derivatives are incorporated into incense, cosmetics, and pharmaceutical products for their anti-inflammatory, antioxidant, and antimicrobial properties (Sharif et al., 2016; Hidayat et al., 2018, 2019b). The radical scavenging activity of resin from S. sumatrana has been reported to have high potency as an antioxidant; hence, it is a good candidate as a natural antioxidant resource (Nurwahyuni et al., 2021). Despite supporting the livelihood of 70% of local people in North Sumatra, Indonesia (Iswanto et al., 2016), and their value as a natural antioxidant resource, Styrax plantations for benzoin resin have gradually decreased because of land conversion (Saputra and Lee, 2021). Such limitations in plantation areas necessitate innovations in the sustainable production of benzoin resins and their derivatives.
In most plants, endophytic fungi that colonize the inner tissue play significant ecological roles, such as strengthening plant defenses against pathogens and abiotic stressors (Arnold et al., 2003; Schardl et al., 2004; Mejía et al., 2008). Particularly, in medicinal plants, these fungi have been reported to have distinctive relationships with their hosts; they influence the plant’s secondary metabolite production and antioxidant enzyme functioning and even incorporate their own metabolites into the host plant’s tissues, which consequently enhances the plant’s ability to withstand stress (Zhao et al., 2010; Ogbe et al., 2020). However, whether benzoin resin is synthesized and accumulated by endophytic fungi that reside within these plants remains an intriguing question.
Naturally sourced antioxidants have desirable properties that may reduce the use of chemically synthesized additives in food products (Brewer, 2011). The carcinogenic potential and other health risks of synthetic additives have limited their use and instigated the search for natural antioxidants (Capitani et al., 2013). A similar trend has been observed for cosmetics and pharmaceutical products. If the capacity of such functional substances from endophytic fungi is better than that of the host plant, endophytic fungi could be a more manageable and sustainable source option. Endophytic fungi isolated from medicinal plants, such as Pinus roxburghii, Ginko biloba, Rauwolfia tetraphylla, and agarwood-producing trees Aquilaria and Gyrinops have been reported to have antimicrobial and antioxidant capacities (Xiao et al., 2013; Bhardwaj et al., 2015; Alurappa and Chowdappa, 2018). However, information on endophytic fungi from S. sumatrana and S. benzoin remains scarce (Elfiati et al., 2021; Slamet et al., 2021). Considering the well-known role of endophytic fungi in enhancing plant defense against pathogens and the antioxidant function of benzoin resin (Hidayat et al., 2018), this study aimed to determine the antifungal, antioxidant, and alpha-glucosidase inhibitory activities of endophytic fungi isolated from the leaves, stems, and bark of S. sumatrana and S. benzoin trees.
Asymptomatic (healthy) 25- to 30-year-old S. benzoin and S. sumatrana trees with heights between 15 and 20 m and breast-high diameters between 15 and 25 cm were selected for this study (Slamet et al., 2021). Fragments were collected from plantations in North Sumatra Province, Indonesia, at elevations of 800–1,000 m asl and temperatures between 15 and 24°C. Tree fragments or plant organs (leaves, stems, or bark) were surface sterilized, and their inner parts were cut and planted aseptically on four chloramphenicol-supplemented isolation media: potato dextrose agar, Pachlewski, yeast dextrose agar, and yeast malt extract (Atlas, 2004; Hidayat et al., 2019a, 2021a); thereafter, successful endophytic fungal isolation was validated (Hidayat et al., 2019a,2021a). The obtained fungal isolates were deposited in the Indonesian Tropical Forest Culture Collection.
Genomic DNA was extracted from a 7-day-old mycelial culture grown in potato dextrose broth with a DNA Wizard Kit (Promega, Madison, WI, USA) using the manufacturer’s method. Polymerase chain reaction (PCR) with Go Taq Green Master Mix (Promega) and ITS1 and ITS4 primers (White et al., 1990) was used to amplify the internal transcribed spacer (ITS) region of fungal DNA. PCR products were subjected to Sanger sequencing (1st BASE Sequencing Service, Singapore) and sequences were aligned using the Basic Local Alignment Search Tool from the National Center for Biotechnology Information database to identify closely related genera. The closest genera and number of isolates are referred to sequentially hereinafter as the isolate identities. A phylogenetic tree was constructed using Mega 11 (Tamura et al., 2021) and the neighbor-joining method (Saitou and Nei, 1987; Tamura et al., 2004). All sequences were deposited in the NCBI database with accession numbers ON796950 to ON97007 (Supplementary Table 1).
Single linkage with combined rescaled distance was calculated to observe the clustering of endophytic fungal genera based on the combination of host tree species and plant organs. Binary values were standardized before analysis. A dendrogram was constructed using hierarchical cluster analysis in IBM SPSS Statistics 25 (IBM Corp., Armonk, NY, USA) to determine relationships among endophytic fungi based on their origins. Squared Euclidean distance was used to express the cluster distance (Mufarhatun et al., 2021).
A dual culture assay was used to screen fungal isolates for their ability to suppress mycelial growth of the plant pathogenic fungi Fusarium sp. (INTROF CC 0509) and Trichoderma viride, and Aspergillus niger. T. viride, and A. niger cultures were obtained from IPB University Culture Collection. Five-millimeter-diameter agar discs of endophytic and pathogenic fungal cultures were co-inoculated 3 cm apart on potato dextrose agar plates (90 mm diameter) and incubated at 25°C for 7 days. The percentage of inhibition (%I) was calculated using the following formula:
where Jc is the pathogen’s radial outward growth (control) and Jt is its radial growth in the direction of the endophytic fungi (Hajieghrari et al., 2010). Each treatment was repeated five times.
Prior to phytochemical assays for bioactive compounds, fungal extracts were prepared according to the method described by Hidayat et al. (2019a), with three replicates for each assay. Antioxidant activities were determined using three approaches: 2,2-diphenyl-1-picrylhydrazyl (DPPH), 2,2′-azino-bis (3-ethylbenzothiazoline-6-sulphonic acid) (ABTS), and ferric reducing antioxidant power (FRAP).
Determination of antioxidant activity via DPPH depletion followed the protocol described by Hidayat et al. (2021b). The effective concentration at which 50% of the radicals were scavenged (IC50 value) was obtained by interpolation from the linear regression analysis (Dewi et al., 2013). Concentrations of DPPH in ppm (based on IC50 estimation, E) were ranked as follows: E < 50, very strong; 50 ≤ E < 100, strong; 100 ≤ E < 150, moderate; 150 ≤ E < 200, weak; and ≥200, undetected.
Measurement of antioxidant activity using the ABTS approach was based on Trolox equivalent antioxidant capacity. The activity was expressed as Trolox equivalent in fungal extracts (mg TE/g), where the capacity of the sample to neutralize ABTS radicals is equivalent to that of Trolox (Wang et al., 2013). ABTS radical solution was prepared as follows: ABTS solution (7 mM) was mixed with potassium persulfate (140 mM) at a 62.5:1 ratio for the stock solution. The mixture was then kept in a dark room at 25°C for 16 h. For measurement, an ABTS radical solution with a 0.7 absorbance value at 734 nm was prepared. The assay was performed by mixing sample solution (5 μL, 1 mg/mL) with 200 μL of ABTS radical solution. The mixture was kept in a dark room for 6 min before absorbance reading at 734 nm. A Trolox solution with a range of concentrations was used to construct the standard curve.
Antioxidant capacity was determined using the FRAP approach according to the methods described by Dudonné et al. (2009) with slight modifications. FRAP reagent was prepared with 300 mM acetate buffer (pH 3.6), 10 mM 2,4,6-tri(2-pyridyl)-s-triazine, and 20 mM FeCl3 in a 10:1:1 ratio. Fungal extracts (40 μL) were then mixed with 1,200 μL FRAP reagent and incubated at 37°C for 30 min. FRAP values were based on the standard Trolox calibration curve and expressed in mg TE/g.
Measurement of total flavonoid content was performed according to the protocol described by Tambe and Bhambar (2014) with some modifications. A volume of 0.1 mL of the sample (1,000 μg/mL) was added to 2.6 mL distilled water and 0.15 mL 5% NaNO2, mixed, and incubated for 5 min. The mixture was combined with 0.15 mL 10% AlCl3 10%, stirred, and incubated for 6 min before being combined with 2 mL NaOH 1 N, mixed, and incubated at 25°C for 30 min. Absorbance was measured at 510 nm. Quercetin at concentrations of 50, 100, 250, 500, and 1,000 μg/mL was used to construct the standard curve. Flavonoid content was expressed as quercetin equivalents (mg QE/g).
Total phenolic content was measured as previously described (Tambe and Bhambar, 2014) with some modifications. A volume of 0.1 mL of the sample (1,000 μg/mL) was combined with 1.4 mL distilled water and 0.25 mL Folin–Ciocalteau reagent. The mixture was incubated for 8 min, combined with 0.75 mL 20% Na2CO3, stirred, and incubated for 2 h at 27°C. Absorbance was measured at 750 nm wavelength. Three replicates of gallic acid at concentrations of 20, 40, 60, 80, and 100 μg/mL were used to construct the standard curve. Phenolic content was expressed as gallic acid equivalents (mg GAE/g).
Fungal extracts were evaluated for α-glucosidase inhibitory activity as described in our previous report (Dewi et al., 2013). Inhibition of α-glucosidase activity was determined by the reaction of α-glucosidase with the p-nitrophenyl-α-D-glucopyranoside substrate, resulting in the formation of p-nitrophenol (405 nm). Each assay was performed in triplicate, and the results are expressed as percentages of inhibition [% inhibition = (AB–AS)/AB × 100%], where AB is the absorbance of the blank solution and AS is the absorbance of the sample. Values are presented as the mean ± standard deviation.
Secondary metabolites from the fungal extracts were determined using a Xevo G2-XS Quadrupole Time of Flight Mass Spectrometer (Waters Corp., Milford, MA, USA) equipped with an electrospray ionization source coupled to a Waters Acquity Ultra Performance LC system. Approximately, 1.5 mg of fungal extract was added to MeOH (LiChrosolv, hypergrade for LC–MS, Merck KGaA, Darmstadt, Germany) and sonicated for 10 min until completely dissolved. Samples were then filtered through a 0.22-μm polytetrafluoroethylene syringe filter (Waters Corp.) to obtain a final concentration of 1 mg/mL. LC–HRMS analysis was performed as described previously (Dewi et al., 2022). Bioactive compounds were identified and analyzed using the LC-HRMS and UNIFI software version 1.5. Peaks were tentatively assigned with the comparison to water built-in library.
All results are presented as the mean of triplicate measurements and the standard deviation.
Fifty-eight fungal isolates were obtained from the bark, stems, and leaves of S. sumatrana and S. benzoin trees. Thirty-eight isolates were obtained from 14 bark, eight stem, and nine leaf samples of S. sumatrana grown in four types of isolating media. Twenty isolates from four bark, nine stem, and seven leaf samples of S. benzoin were obtained using the same set of agar media.
The genera of all 58 isolates were determined based on ITS sequences. The closest matching genera based on sequence similarity are shown in Figure 1. Almost all isolates belonged to the phylum Ascomycota, except Lichtheimia sp. 6410, which belonged to the phylum Mucoromycota. The 58 fungi identified in this study were classified into 15 genera belonging to 12 families that belong to eight orders: Hypocreales, Botryosphaeriales, Glomerellales, Diaphortales, Pleosporales, Eurotiales, Xylariales, and Mucorales (Figure 1). Fusarium, Pestalotiopsis, and Neopestalotiopsis were the most common genera, with 20, 9, and 8 isolates, respectively.
Figure 1. Neighbor-joining phylogenetic tree of 58 endophytic fungi isolated from Styrax sumatrana and Styrax benzoin and their respective reference based on fungal internal transcribed spacer sequence. Fungi from this study are presented as their isolate number followed by their National Center for Biotechnology Information accession number, whereas for reference sequences, species name, and accession number are given (Supplementary Table 1).
Six possible combinations of two variables (host species and host organ) from which endophytic fungal genera originated are presented in Figure 2. A shorter Euclidean distance indicates a closer relationship; that is, the two variables share more similarities. Conversely, a longer Euclidean distance indicates greater heterogeneity and fewer similarities. Clustering analysis of endophytic fungal species based on the combination of plant organs and host plant species revealed two large groups. Host plant species seemed to be more important than plant organs in determining the grouping. Fungal communities residing in the leaves, bark, and stem of S. sumatrana tended to have more similarities and were thus grouped into one distinct cluster. The other cluster consisted of fungal communities residing in the leaves, bark, and stem of S. benzoin. A slightly different pattern was observed in each host plant species: in S. benzoin, fungal diversities in bark and stem organs showed more similarities to those residing in leaves, whereas in S. sumatrana, fungal diversities in bark and leaves showed more similarities to each other than to those in the stem.
Figure 2. Single-linkage dendrogram with combined rescaled distance clusters. Endophytic fungi species were clustered based on their origins, plant organ (bark, stem, and leaf), and host plant species (Styrax sumatrana and Styrax benzoin).
All 58 endophytic fungal isolates exhibited in vitro growth inhibition of three plant-pathogenic fungi. The percentage inhibition ranged from 10.27 to 78.59%, 18.33 to 82.67%, and 6.31 to 73.57% for Fusarium sp., T. viride, and A. niger, respectively (Supplementary Table 2). Three most inhibiting isolates against Fusarium sp. were Trichoderma sp. 6395, Trichoderma sp. 6411, and Trichoderma sp. 6407, with averages of 78.59 ± 1.03%, 74.41 ± 3.61%, and 73.57 ± 7.56% inhibition, respectively. Trichoderma sp. 6407, Fusarium sp. 6413, and Fusarium sp. 6445 were the most inhibiting against T. viride, with 82.67 ± 4.94%, 76.00 ± 2.79%, and 69.00 ± 5.35% inhibition, respectively. A. niger, Trichoderma sp. 6407, Neofusicoccum sp. 6399, and Trichoderma sp. 6396 were the most inhibiting (73.57 ± 7.56%, 62.66 ± 3.25%, and 59.77 ± 10.50%, respectively) (Table 1).
Table 1. The high antagonistic activity of endophytic fungal isolates against plant pathogenic fungi, Fusarium sp, Trichoderma viride, and Aspergillus niger using dual culture assay*.
Corroborating Wheeler and Hocking (1993), Trichoderma sp. 6407, which inhibited all three pathogenic fungi, displayed interaction type B against T. viride, where both fungi inhibited each other’s growth and created less than 2 mm space between their colonies (Figure 3A, left). Both Fusarium spp. 6413 and 6445 exhibited interaction type C against T. viride, where the growth of each endophytic fungus was lower than that of the pathogen, followed by slower fungal growth when a barrier between colonies was apparent (Figure 3A, center and right). Neofusicoccum sp. 6399 and A. niger pathogen appeared to have type D interaction, where both cultures inhibited each other and created a space of 2 mm or more between colonies (Figure 3B, center). Interaction type E was observed in Trichoderma sp. 6395 and pathogenic Fusarium sp. dual cultures, where the pathogen’s growth was smaller and covered by endophytic fungi; however, the growth of both fungi decreased, and a barrier between colonies was apparent (Figure 3C, left). Interaction type F was observed in the remaining dual cultures, where pathogen growth was smaller than that in the endophytic fungal cultures, which later covered the pathogen’s colony (Figure 3C, center and right, and Figure 3B, left and right).
Figure 3. Inhibition of pathogenic fungi (placed on the left side of the plate); inhibition of (A) Trichoderma viride, (B) Aspergillus niger, and (C) Fusarium sp. by endophytic fungi (placed on the right side of the plate) in dual culture plates. The three highest inhibition percentages for each pathogen were obtained from isolates (left to right): (A) 6407, 6413, and 6445; (B) 6407, 6399, and 6396; (C) 6395, 6411, and 6407.
Antioxidant activities of the endophytic fungal extracts were measured using DPPH, ABTS, and FRAP assays (Supplementary Table 2). Seven isolates with the highest values were exclusive for each method. The remaining three isolates were able to perform among the highest levels for the two methods. Neopestalotiopsis sp. 6431 extract performed the best in DPPH inhibition, with an average of 49.65 ± 0.80% or IC50 estimated concentration of 100.71 ppm, followed by Trichoderma sp. 6395, Phyllosticta sp. 6454, and Fusarium sp. 6430 extracts, tied at 37.59% or IC50 estimated concentration of 133.02 ppm. As previously mentioned, Trichoderma sp. 6395 was the most inhibiting in the dual culture assay against pathogenic Fusarium sp.
Estimated IC50 concentrations were all categorized as medium. For ABTS-based antioxidant activity, isolate 6412 extract led to an average of 28.41 ± 0.04 mg TE/g, closely followed by Fusarium sp. 6430 and Phyllosticta sp. 6454 extracts, with averages of 27.74 ± 0.34 and 25.04 ± 0.27 mg TE/g, respectively. The last two isolates were among the highest achievers of DPPH inhibition. Neopestalotiopsis sp. 6431, Neopestalotiopsis sp. 6450, and Neofusicoccum sp. 6399 were the three isolates with the highest antioxidant activities for the FRAP method: 197.49 ± 8.65, 167.39 ± 8.57, and 152.05 ± 32.40 mg TE/g, respectively. Moreover, the Neopestalotiopsis sp. 6431 extract exhibited the highest activity for both the DPPH and FRAP methods (Table 2).
Table 2. Fungal isolates with high antioxidant activity determined by 2,2-diphenyl-1-picrylhydrazyl (DPPH), 2,2′ -azino-bis (3-ethylbenzothiazoline-6-sulphonic acid) (ABTS), and ferric reducing antioxidant power (FRAP) assay*.
An entirely different set of isolates, Colletotrichum sp. 6439, Fusarium sp. 6456, and Fusarium sp. 6444, produced extracts that contained the three highest concentrations of flavonoid compounds (155,79 ± 7.47, 108.86 ± 94.86, and 88.86 ± 14.49 mg QE/g, respectively) (Table 3). As for the total phenolic compounds, the three highest producers were fungal extracts from Phyllosticta sp. 6454, Neopestalotiopsis sp. 6431, and Fusarium sp. 6430 (110.87 ± 18.52, 43.02 ± 1.25, and 41.33 ± 1.50 mg GAE/g, respectively). These isolates were also the most inhibiting in the DPPH-based antioxidant capacity assay (Table 2).
Table 3. Fungal isolates with high content of flavonoid by using aluminum chloride assay, and phenol by using Folin–Ciocalteau reagent assay*.
α-glucosidase inhibitory activity of the 58 endophytic fungal isolates ranged from 0.00 to 65.00% (Supplementary Table 2). Fungal extracts of Pestalotiopsis sp. 6416, Neopestalotiopsis sp. 6431, and Phyllosticta sp. 6454 were the most inhibiting at 65.00 ± 0.28%, 52.88 ± 4.93%, and 52.15 ± 10.08%, respectively (Table 4). Phyllosticta sp. 6454 repeatedly resurfaced among the top three isolates for DPPH inhibition, ABTS-based antioxidant capacity, phenolic content, and α-glucosidase inhibitory activity. Similarly, Neopestalotiopsis sp. 6431 was the top performer in four assays: DPPH inhibition, FRAP-based antioxidant capacity, phenolic content, and α-glucosidase inhibitory activity (Tables 2–4).
Table 4. Fungal isolates with high α-glucosidase inhibition determined by p-nitrophenyl-α-D-glucopyranoside as substrate*.
Based on the LC-HRMS results from base line (Figure 4A) and fungal crude extracts (Figures 4B–D), the crude extract of Trichoderma sp. 6407, which showed prominent antifungal activities, had eight peaks in total, with four main peaks indicating the active compounds (Figure 4B). The first four major peaks occurred at a retention time (tR) of 7.87 min, with a molecular ion (m/z) value of 279.2321 [M+H]+, and molecular formula C18H30O2. Bioactive compounds with such properties were predicted to be methyl hydroxysterpurate ethylidene acetal or xylarinorditerpene Q (Figures 4B, 5A1). The second four major peaks occurred at tR = 8.67 min, with a m/z value of 319.2251 [M+H]+, and molecular formula C18H32O3, which could be (1S,2S)-3-oxo-2-pentylcyclopentane-1-octanoic acid or 3,7-dimethyl-9-(-2,2,5,5-tetramethyl-1,3-dioxolan-4-yl)nona-1,6-dien-3-ol (Figures 4B, 5A2). The third four major peaks occurred at tR = 9.98 min, with an m/z value of 395.3310 [M+H]+, and molecular formula C28H42O, which were predicted to be ergosta-4,6,8,22E-tetraen-11β-OL (Figures 4B, 5A3). The last detected major peak occurred at tR = 10.91 min, with a predicted m/z value of 281.2459 [M+H]+, and molecular formula C18H32O2, which were approximated to be 4-Me-6E,8E-16:2 methyl ester or 4-methyl-7,11-heptadecadienoic acid (Figures 4B, 5A4).
Figure 4. Liquid chromatography–mass spectrometry chromatogram of baseline (A), extracted isolates Trichoderma sp. 6407 (B), Phyllosticta sp. 6454 (C), and Neopestalotiopsis sp. 6431 (D).
Figure 5. Mass chromatogram of metabolite compounds from extracted isolates Trichoderma sp. 6407 (A1–4), Phyllosticta sp. 6454 (B1–4), and Neopestalotiopsis sp. (C1–3).
Several ionic predictions and molecular formulas for Phyllosticta sp. 6454 extracts, which showed antioxidant and α-glucosidase activity, were identified (Figures 4C, 5B). The LC–HRMS results showed at least 10 peaks for active compounds. The four largest peaks had a tR of 7.43, 8.25, 10.13, and 10.19 min (Figure 4C), with the following respective m/z values and molecular formulas: m/z = 463.1601 [M+Na]+ and molecular formula C27H24N2O4 (predicted to be SF2809-IV (4-hydroxy-3-[[3-(2-hydroxyethyl)-1H-indol-2-yl]-(4-hydroxyphenyl)methyl]-1-methylquinolin-2-one) compound); m/z = 475.3167 [M+Na]+ and molecular formula C30H44O3 (predicted to be igniarine); m/z = 337.1663 [M+H]+ and molecular formula C18H24O6 (predicted to be corynechromone or cytosprone); and m/z = 808.3502 [M+Na]+ and molecular formula C37H51N7O12 (Figures 5B1–4).
For the active compounds in Neopestalotiopsis sp. 6431 extracts, which may be responsible for the exhibited antioxidant activities, at least nine peaks were predicted (Figure 4D). The three largest peaks were detected at a tR of 3.36, 10.92, and 11.05 min (Figure 4D), with the following respective m/z values and molecular formulas: m/z = 211.1445 [M+H]+ and molecular formula C11H18N2O2; m/z = 808.3502 [M+Na]+ and molecular formula C37H51N7O12; and m/z = 626.3495 [M+Na]+ and molecular formula C26H49N7O9 (Figures 5C1–3).
All the closest matches of the isolates belong to the phylum Ascomycota, except for Lichtheimia, which belongs to Mucoromycota. The dominance of Ascomycota in endophytic fungal assemblages has also been observed elsewhere (Hamzah et al., 2018; Zhou et al., 2018; Nguyen et al., 2021). This study complements previous reports on endophytic fungi isolated from S. sumatrana and S. benzoin and adds the newly reported genera Lichtheimia, Penicillium, Pseudopestalotiopsis, and Colletotrichum from S. sumatrana and Botryosphaeria from S. benzoin (Hidayat et al., 2021a; Slamet et al., 2021). All of the closest-matched genera have been previously reported as endophytes (Shetty et al., 2016; Huang et al., 2020). Various factors are involved in the dynamics of endophytic fungal communities, including macroenvironmental factors such as season, geographic location (Mishra et al., 2012; Slamet et al., 2021), and water availability (Costa et al., 2018). Each microenvironmental biotic factor, including host species, host tissue/organ (Moricca et al., 2012; Slamet et al., 2021), and other coexisting endophytes or pathogens (Sicard et al., 2007), adds to the community dynamics. These complex mechanisms may act as selection pressures for endophytic fungi, leading to host species and/or host organ specificities (Slamet et al., 2021).
Clustering the endophytic fungal genera based on the plant host species and plant organs demonstrated a specific pattern only for the plant host species. Endophytic fungal communities in the bark and stems of S. benzoin clustered within the shortest Euclidean distance, indicating higher genus similarity and homogeneity between them. The fungal community in the leaves of the same host species was clustered at a longer distance but was still within the same cluster. Although these organs have different structures (Romero, 2014), the closer proximity of the bark and stem may accommodate fungal mycelia extending between these two organs and consequently having more shared species. In the other cluster, the fungal community residing in the bark and leaf organs of S. sumatrana shared more similarities than those residing in the stems of the same host plant species. This pattern of similarities indicates the tendency of fungal genus specificity to be based on host plant species. These observations are based on the genus taxonomic rank, which is limited to a less restrictive interpretation. Multi-locus taxonomy identification and/or an inclusive metagenomic approach is recommended for further studies to scrutinize these mechanisms.
Trichoderma sp. 6407, isolated from the stems of S. sumatrana, consistently showed the highest antifungal activity against all tested pathogenic fungi. Plant-protective members of the Trichoderma genus, including T. pubescens, produce a group of polypeptide antibiotics that may contribute to their antagonistic potential against fungal diseases in grapevine trunks (Degenkolb et al., 2006). Other species of Trichoderma have been shown to reduce disease severity by inhibiting the growth of the pathogenic fungus Rhizoctonia solani (Molan et al., 2008). The predicted methyl hydroxysterpurate ethylidene acetal or xylarinorditerpene Q compounds from Trichoderma sp. 6407 extracts were previously reported to be isolated from endophytic fungi and also have antifungal properties (Xie and Li, 1992; Wu et al., 2014; Chen et al., 2020). The predicted (1S,2S)-3-oxo-2-pentylcyclopentane-1-octanoic acid or 3,7-dimethyl-9-(-2,2,5,5-tetramethyl-1,3-dioxolan-4-yl)nona-1,6-dien-3-ol compounds have also been reported to be produced by endophytic fungi (Miersch et al., 1999; Lin et al., 2016). The prediction ergosta-4,6,8,22E-tetraen-11β-OL compound has previously been reported to be isolated from the fruiting body of Coprinus setulosus (Ma et al., 2018), whereas, the postulated 4-Me-6E,8E-16:2 methyl ester or 4-methyl-7,11-heptadecadienoic acid compounds were reported to be isolated from liquid cultures of Clonostachys rosea and Sporothrix sp. and have the potential to inhibit the growth of MFC-7 cancer cells, F. oxysporum f. sp. lycospersici, T. viride, and Bacillus subtilis (Choudhury et al., 1994; Dias et al., 2015). In this study, the metabolites produced by Trichoderma sp. 6407 displayed four major peaks; however, other minor peaks still have the potential to represent other active compounds. Considering the vital role of endophytic fungi in strengthening plant defense against pathogens and promoting overall health (Mejía et al., 2008), purification and elucidation of the active compounds produced by Trichoderma sp. 6407 and further investigation of antifungal mechanisms are required to optimize their biocontrol potential.
Phyllosticta sp. 6454, which was isolated from the bark of S. sumatrana, is characterized by its high antioxidant and α-glucosidase activity. This genus is a widely distributed fungal endophyte and is found in 70 plant families (Wikee et al., 2013). Despite its widespread distribution, information regarding its antioxidant capacity is limited. Endophytic Phyllosticta sp. isolated from the medicinal plant Guazuma tomentosa has been reported to have antioxidant properties, with phenol and flavonoid contents that are one-sixth and one-fourteenth, respectively, of those observed in Phyllosticta sp. 6454 (Srinivasan et al., 2010). The presumed SF2809-IV (4-hydroxy-3-[[3-(2-hydroxyethyl)-1H-indol-2-yl]-(4-hydroxyphenyl)methyl]-1-methylquinolin-2-one) compound from Phyllosticta sp. 6454 extracts were previously isolated from Dactylosporangium sp., and some of its derivatives have been shown to inhibit recombinant human chymase activity (Tani et al., 2004). Secondary metabolites from Phyllosticta with antimicrobial activities have been previously reported (Taher et al., 2022) and may be related to the phenol and flavonoid contents of Phyllosticta sp. 6454 extracts.
Neopestalotiopsis sp. 6431, isolated from the stems of S. benzoin, also exhibited high antioxidant activity. Neopestalotiopsis is a common endophytic fungal genus (Hamzah et al., 2018; Azuddin et al., 2021) and was among the most commonly isolated genera in this study. This genus was also previously discovered in the bark of S. benzoin (Ilyas et al., 2019). A member of the genus Neopestalotiopsis has been reported to produce antimicrobial and antioxidant agents, such as eugenol, myristaldehyde, lauric acid, and caprylic acid (Tanapichatsakul et al., 2019). However, based on the LC–HRMS results of the present study, Neopestalotiopsis sp. 6431 may have produced different secondary metabolites.
Fusarium sp. 6430 isolated from the stems of S. sumatrana was the most frequently isolated genus in this study and displayed high antioxidant activity. This genus has been reported to exude diverse bioactive compounds and exert biocontrol functions to enhance plant health. Secondary metabolites, aza-anthraquinones, isolated from an endophytic F. solani strain, the crude extract of which shows antimicrobial and antioxidant activities, have been reported to be potent bioactive compounds for anticancer and antimicrobial agents (Khan et al., 2018). Fusarium sp. evinced the production of a new antifungal and antimalarial cyclodepsipeptide, known as fusaripeptide (Ibrahim et al., 2018). The observed antioxidant activity and phenol content of Phyllosticta sp. 6454, Neopestalotiopsis sp. 6431, and Fusarium sp. 6430 suggests that these isolates are strong candidates for natural antioxidant sources. Future studies, including methods of purifying bioactive compounds, are required to further optimize their potential as bioresources.
Antioxidants are compounds that inhibit the initiation or propagation of chain oxidation reactions. The chemical structure of antioxidants, source of free radicals, and physicochemical properties of different sample preparations can provide different test results for antioxidant activity (Karamać et al., 2005). Therefore, it is necessary to analyze the antioxidant activity of a specific sample type. In this study, antioxidant testing using DPPH, ABTS, and FRAP assays was conducted. The DPPH assay measures the ability of compounds to donate hydrogen to a stable DPPH• molecule, resulting in the formation of a purple color (520 nm). In contrast, the ABTS cation radical (ABTS•+), which absorbs light at 743 nm, changes to stable ABTS by accepting hydrogen from antioxidant compounds, resulting in solution decolorization (Chu et al., 2000). This method is based on a reduction reaction in an acidic atmosphere to a yellow Fe3+ (potassium hexacyanoferrate) complex compound to a bluish-green Fe2+ complex compound owing to electrons donated by antioxidant compounds (Craft et al., 2012).
Furthermore, we investigated the α-glucosidase inhibitory activity of the fungal extracts in this study. Phenolic compounds are antioxidants and can also inhibit natural α-glucosidase enzymes because they inhibit carbide enzymes owing to their ability to bind proteins (Zhang et al., 2015). This assay is based on the formation of p-nitrophenol, which results from the cleavage of p-nitrophenyl-α-D-glucopyranose at 410 nm.
These findings lay the groundwork for further studies that will identify the compounds responsible for the observed antioxidant and α-glucosidase inhibitory activities and their underlying mechanisms, which will potentially guide the optimization of their production.
The datasets presented in this study can be found in online repositories. The names of the repository/repositories and accession number(s) can be found in the article/Supplementary material.
DE, SF, LR, AA, RD, HR, MT, MR, AS, and AH contributed to the conceptualization, methodology, experiment, validation, analysis, resources, writing, review, and editing of the manuscript. All authors have read and agreed to the published version of the manuscript.
This research was funded by the Directorate of Research and Community Service of the Ministry of Research and Technology/National Research and Innovation Agency, Indonesia, Number: 61/UN5.2.3.1/PPM/KP-DRPM/2021 dated March 17, 2021.
We acknowledge the Indonesian Ministry of Environment and Forestry for facilitating and supporting this research. We are grateful to the reviewers for their helpful comments on an earlier draft of the manuscript.
The authors declare that the research was conducted in the absence of any commercial or financial relationships that could be construed as a potential conflict of interest.
All claims expressed in this article are solely those of the authors and do not necessarily represent those of their affiliated organizations, or those of the publisher, the editors and the reviewers. Any product that may be evaluated in this article, or claim that may be made by its manufacturer, is not guaranteed or endorsed by the publisher.
The Supplementary Material for this article can be found online at: https://www.frontiersin.org/articles/10.3389/fmicb.2022.974526/full#supplementary-material
Alurappa, R., and Chowdappa, S. (2018). Antimicrobial activity and phytochemical analysis of endophytic fungal extracts isolated from ethno-pharmaceutical plant Rauwolfia tetraphylla L. J. Pure Appl. Microbiol. 12, 317–332. doi: 10.22207/JPAM.12.1.38
Arnold, A. E., Mejía, L. C., Kyllo, D., Rojas, E. I., Maynard, Z., Robbins, N., et al. (2003). Fungal endophytes limit pathogen damage in a tropical tree. Proc. Natl Acad. Sci. U.S.A. 100, 15649–15654. doi: 10.1073/PNAS.2533483100
Atlas, R. M. (2004). Handbook of microbiological media. Great Britain: Taylor & Francis. doi: 10.1201/9781420039726
Azuddin, N. F., Mohd, M. H., Rosely, N. F. N., Mansor, A., and Zakaria, L. (2021). Molecular phylogeny of endophytic fungi from rattan (Calamus castaneus griff.) spines and their antagonistic activities against plant pathogenic fungi. J. Fungi 7:301. doi: 10.3390/JOF7040301
Baum, J. M. (2013). From incense to idolatry: The reformation of olfaction in late medieval german ritual. Sixteenth Century J. 44, 323–344.
Bhardwaj, A., Sharma, D., Jadon, N., and Agrawal, P. K. (2015). Antimicrobial and phytochemical screening of endophytic fungi isolated from spikes of Pinus roxburghii. Arch. Clin. Microbiol. 6, 1–9.
Brewer, M. S. (2011). Natural antioxidants: Sources, compounds, mechanisms of action, and potential applications. Comput. Rev. Food Sci. Food Saf. 10, 221–247. doi: 10.1111/J.1541-4337.2011.00156.X
Capitani, C. D., Hatano, M. K., Marques, M. F., and Castro, I. A. (2013). Effects of optimized mixtures containing phenolic compounds on the oxidative stability of sausages. Food Sci. Technol. Int. 19, 69–77. doi: 10.1177/1082013212442184
Chen, H. P., Zhao, Z. Z., Cheng, G. G., Zhao, K., Han, K. Y., Zhou, L., et al. (2020). Immunosuppressive nor-isopimarane diterpenes from cultures of the fungicolous fungus Xylaria longipes HFG1018. J. Nat. Prod. 83, 401–412. doi: 10.1021/acs.jnatprod.9b00889
Choudhury, S. R., Traquair, J. A., and Jarvis, W. R. (1994). 4-methyl-7,11-heptadecadienal and 4-methyl-7,11-heptadecadienoic acid: New antibiotics from Sporothrix flocculosa and Sporothrix rugulosa. J. Nat. Prod. 57, 700–704. doi: 10.1021/np50108a003
Chu, Y. H., Chang, C. L., and Hsu, H. F. (2000). Flavonoid content of several vegetables and their antioxidant activity. J. Sci. Food Agric. 80, 561–566. doi: 10.1002/(SICI)1097-0010(200004)80:5<561::AID-JSFA574<3.0.CO;2-#
Costa, D., Tavares, R. M., Baptista, P., and Lino-Neto, T. (2018). Diversity of fungal endophytic community in Quercus suber L. under different climate scenarios. Revista de Ciências Agrárias 41, 22–30. doi: 10.19084/RCA.17063
Craft, B. D., Kerrihard, A. L., Amarowicz, R., and Pegg, R. B. (2012). Phenol-based antioxidants and the in vitro methods used for their assessment. Comput. Rev. Food Sci. Food Saf. 11, 148–173. doi: 10.1111/J.1541-4337.2011.00173.X
Degenkolb, T., Gräfenham, T., Berg, A., Nirenberg, H. I., Gams, W., and Brückner, H. (2006). Peptaibiomics: Screening for polypeptide antibiotics (peptaibiotics) from plant-protective Trichoderma species. Chem. Biodivers. 3, 593–610. doi: 10.1002/CBDV.200690063
Dewi, R. T., Tachibana, S., and Darmawan, A. (2013). Effect on α-glucosidase inhibition and antioxidant activities of butyrolactone derivatives from Aspergillus terreus MC751. Med. Chem. Res. 23, 454–460. doi: 10.1007/S00044-013-0659-4
Dewi, R. T., Primahana, G., Septama, A. W., Angelina, M., Meilawati, L., Fajriah, S., et al. (2022). Quality control standardization of indonesian noni fruit (Morinda citrifolia) extract and evaluation of their angiotensin-converting enzyme inhibitory activity. Pharmacia 69, 709–717. doi: 10.3897/pharmacia.69.e86854
Dias, A. C. D. S., Ruiz, N., Couzinet-Mossion, A., Bertrand, S., Duflos, M., Pouchus, Y. F., et al. (2015). The marine-derived fungus Clonostachys rosea, source of a rare conjugated 4-me-6E,8E-hexadecadienoic acid reducing viability of MCF-7 breast cancer cells and gene expression of lipogenic enzymes. Mar. Drugs 13, 4934–4948. doi: 10.3390/md13084934
Dudonné, S., Vitrac, X., Coutiére, P., Woillez, M., and Mérillon, J. M. (2009). Comparative study of antioxidant properties and total phenolic content of 30 plant extracts of industrial interest using DPPH, ABTS, FRAP, SOD, and ORAC assays. J. Agric. Food Chem. 57, 1768–1774. doi: 10.1021/JF803011R
Elfiati, D., Susilowati, A., and Hidayat, A. (2021). Isolation of endophytic fungi from styrax sumatrana tree from humbang hasundutan, north sumatra. Earth Environ. Sci. 782:2045. doi: 10.1088/1755-1315/782/4/042045
Ergin, N. (2014). The fragrance of the divine: Ottoman incense burners and their context. Art Bull. 96, 70–97. doi: 10.1080/00043079.2014.877306
Gershon, L. (2019). Smells like divine spirit. Available online at: https://daily.jstor.org/smells-like-divine-spirit/ (accessed March 27, 2022).
Hajieghrari, B., Torabi-Giglou, M., Mohammadi, M. R., and Davari, M. (2010). Biological potantial of some Iranian trichoderma isolates in the control of soil borne plant pathogenic fungi. Afr. J. Biotechnol. 7, 967–972. doi: 10.4314/ajb.v7i8.58586
Hamzah, T. N. T., Lee, S. Y., Hidayat, A., Terhem, R., Faridah-Hanum, I., and Mohamed, R. (2018). Diversity and characterization of endophytic fungi isolated from the tropical mangrove species, rhizophora mucronata, and identification of potential antagonists against the soil-borne fungus, Fusarium solani. Front. Microbiol. 9:1707. doi: 10.3389/FMICB.2018.01707
Haran, M. (1960). The uses of incense in the ancient israelite ritual. Vetus Testamentum 10, 129. doi: 10.2307/1516131
Hidayat, A., Iswanto, A. H., Susilowati, A., and Rachmat, H. H. (2018). Radical scavenging activity of kemenyan resin produced by an Indonesian native plant, Styrax sumatrana. J. Korean Wood Sci. Technol. 46, 346–354. doi: 10.5658/WOOD.2018.46.4.346
Hidayat, A., Susilowati, A., Faulina, S. A., Elfiati, D., Imanuddin, R., and Turjaman, M. (2021a). Diversity of endophytic fungi isolated from benzoin-producing tree styrax sumatrana. Earth Environ. Sci. 762, 12002. doi: 10.1088/1755-1315/762/1/012002
Hidayat, A., Turjaman, M., Qamyari, R., Imanuddin, R., Tohir, D., Rahmanto, R. G. H., et al. (2021b). Bioactive composition, antifungal, antioxidant, and anticancer potential of agarwood essential oil from decaying logs (Gyrinops spp.) of papua Island (indonesia). J. Appl. Pharm. Sci. 11, 070–078. doi: 10.7324/JAPS.2021.1101010
Hidayat, A., Turjaman, M., Faulina, S. A., Ridwan, F., Aryanto, Najmulah, et al. (2019a). Antioxidant and antifungal activity of endophytic fungi associated with agarwood trees. J. Korean Wood Sci. Technol. 47, 459–471. doi: 10.5658/WOOD.2019.47.4.459
Hidayat, N., Yati, K., Krisanti, E. A., and Gozan, M. (2019b). Extraction and antioxidant activity test of black sumatran incense. AIP Conference Proc. 2193, 30017. doi: 10.1063/1.5139354
Huang, L. Q., Niu, Y. C., Su, L., Deng, H., and Lyu, H. (2020). The potential of endophytic fungi isolated from cucurbit plants for biocontrol of soilborne fungal diseases of cucumber. Microbiol. Res. 231, 126369. doi: 10.1016/J.MICRES.2019.126369
Ibrahim, S. R. M., Abdallah, H. M., Elkhayat, E. S., Musayeib, N. M., Asfour, H. Z., Zayed, M. F., et al. (2018). Fusaripeptide a: New antifungal and anti-malarial cyclodepsipeptide from the endophytic fungus Fusarium sp. J. Asian Nat. Prod. Res. 20, 75–85. doi: 10.1080/10286020.2017.1320989
Ilyas, M., Praptiwi, Wulansari, D., Fathoni, A., and Agusta, A. (2019). An assemblages of fungal endophytes isolated from medicinal plants collected from toba and samosir, north sumatra. Earth Environ. Sci. 308:012070. doi: 10.1088/1755-1315/308/1/012070
Iswanto, A. H., Susilowati, A., Azhar, I., Riswan, S., Tarigan, J. E., et al. (2016). Physical and mechanical properties of local styrax woods from north tapanuli in indonesia. J. Korean Wood Sci. Technol. 44, 539–550. doi: 10.5658/WOOD.2016.44.4.539
Karamać,, M., Kosińska, A., and Pegg, R. B. (2005). Comparison of radical-scavenging activities for selected phenolic acids. Polish J. Food Nutr. Sci. 55, 165–170.
Kashio, M., and Johnson, D. V. (2001). Monograph on benzoin (balsamic resin from styrax species. food and agriculture organization of the united nations. regional office for asia and the pacific. Bangkok: RAP. Publication, 21.
Khan, N., Afroz, F., Begum, M. N., Roy Rony, S., Sharmin, S., Moni, F., et al. (2018). Endophytic Fusarium solani: A rich source of cytotoxic and antimicrobial napthaquinone and aza-anthraquinone derivatives. Toxicol. Rep. 5, 970–976. doi: 10.1016/J.TOXREP.2018.08.016
le Maguer, S. (2015). “The incense trade during the islamic period,” in Proceedings of the seminar for arabian studies, Vol. 45, (Oxford: Archaeopress Publishing Ltd.), 175–183.
Lin, X., Yu, M., Lin, T., and Zhang, L. (2016). Secondary metabolites of Xylaria sp., an endophytic fungus from Taxus mairei. Nat. Prod. Res. 30, 2442–2447. doi: 10.1080/14786419.2016.1198350
Ma, Q. Y., Yang, S., Huang, S. Z., Kong, F. D., Xie, Q. Y., Dai, H. F., et al. (2018). Ergostane steroids from coprinus setulosus. Chem. Nat. Compd. 54, 710–713. doi: 10.1007/s10600-018-2451-7
Mejía, L. C., Rojas, E. I., Maynard, Z., van Bael, S., Arnold, A. E., Hebbar, P., et al. (2008). Endophytic fungi as biocontrol agents of Theobroma cacao pathogens. Biol. Control 46, 4–14. doi: 10.1016/J.BIOCONTROL.2008.01.012
Miersch, O., Bohlmann, H., and Wasternack, C. (1999). Jasmonates and related compounds from Fusarium oxysporum. Phytochemistry 50, 517–523. doi: 10.1016/S0031-9422(98)00596-2
Milburn, O. (2016). Aromas, scents, and spices: Olfactory culture in China before the arrival of buddhism. J. Am. Orient. Soc. 136, 441–464. doi: 10.7817/JAMERORIESOCI.136.3.0441/0
Mishra, A., Gond, S. K., Kumar, A., Sharma, V. K., Verma, S. K., Kharwar, R. N., et al. (2012). Season and tissue type affect fungal endophyte communities of the Indian medicinal plant Tinospora cordifolia more strongly than geographic location. Microb. Ecol. 64, 388–398. doi: 10.1007/S00248-012-0029-7
Molan, Y. Y., Kamel, A., and El-Hussieni, S. (2008). Bicontrol of R. solani AG – 4 with piperitone product from Cymbopogon proximus and comparison with bicontrol agents (Trichoderma Spp). Alex. Sci. Exch. J. 29, 16–25. doi: 10.21608/ASEJAIQJSAE.2008.3178
Moricca, S., Ginetti, B., and Ragazzi, A. (2012). Species- and organ-specificity in endophytes colonizing healthy and declining Mediterranean oaks. Phytopathol. Mediterr. 51, 587–598.
Mufarhatun, N., Hilwan, I., and Rachmat, H. H. (2021). Identification of red meranti group (Shorea spp., dipterocarpaceae) saplings based on variations in the morphological features of quantitative leaves. J Penelitian Hutan Konservasi Alam 18, 137–149. doi: 10.20886/JPHKA.2021.18.2.137-149
Nguyen, M. H., Shin, K. C., and Lee, J. K. (2021). Fungal community analyses of endophytic fungi from two oak species, quercus mongolica and quercus serrata, in Korea. Mycobiology 49, 385–395. doi: 10.1080/12298093.2021.1948175/SUPPL_FILE/TMYB_A_1948175_SM3053.XLSX
Nir, R. (2004). The aromatic fragrances of paradise in the greek life of adam and eve and the christian origin of the composition. Novum Testamentum 46, 20–45. doi: 10.1163/156853604772719739
Nurwahyuni, I., Situmorang, M., and Sinaga, R. (2021). Identification of mother plant for in vitro propagation of sumatra benzoin as a strategy to improve non-timber forest product. J. Physics Conf. Ser. 1811, 12018. doi: 10.1088/1742-6596/1811/1/012018
Ogbe, A. A., Finnie, J. F., and van Staden, J. (2020). The role of endophytes in secondary metabolites accumulation in medicinal plants under abiotic stress. Soc. Afr. J. Bot. 134, 126–134. doi: 10.1016/J.SAJB.2020.06.023
Saitou, N., and Nei, M. (1987). The neighbor-joining method: A new method for reconstructing phylogenetic trees. Mol. Biol. Evol. 4, 406–425. doi: 10.1093/OXFORDJOURNALS.MOLBEV.A040454
Saputra, M. H., and Lee, H. S. (2021). Evaluation of climate change impacts on the potential distribution of Styrax sumatrana in north sumatra, indonesia. Sustainability 13:462. doi: 10.3390/SU13020462
Schardl, C. L., Leuchtmann, A., and Spiering, M. J. (2004). Symbioses of grasses with seedborne fungal endophytes. Annu. Rev. Plant Biol. 55, 315–340. doi: 10.1146/ANNUREV.ARPLANT.55.031903.141735
Sharif, A., Nawaz, H., Rehman, R., Mushtaq, A., and Rashid, U. (2016). A review on bioactive potential of benzoin resin. IJCBS 10, 106–110.
Shetty, K. G., Rivadeneira, D., Jayachandran, K., and Walker, D. (2016). Isolation and molecular characterization of the fungal endophytic microbiome from conventionally and organically grown avocado trees in south florida. Mycol. Prog. 15, 977–986. doi: 10.1007/s11557-016-1219-3
Sicard, D., Pennings, P. S., Grandclément, C., Acosta, J., Kaltz, O., and Shykoff, J. A. (2007). Specialization and local adaptation of a fungal parasite on two host plant species as revealed by two fitness traits. Evolution 61, 27–41. doi: 10.1111/J.1558-5646.2007.00003.X
Slamet, W. Y., Faulina, S. A., Hidayat, A., Susilowati, A., Elfiati, D., Rahayu, L. M., et al. (2021). Diversity of endophytic fungal species from styrax benzoin found in benzoin-producing locations in north sumatra. Earth Environ. Sci. 914:12041. doi: 10.1088/1755-1315/914/1/012041
Smit, P. B. (2004). Incense revisited: Reviewing the evidence for incense as a clue to the christian provenance of the greek life of adam and eve. Novum Testamentum 46, 369–375. doi: 10.1163/1568536042650595
Srinivasan, K., Jagadish, L. K., Shenbhagaraman, R., and Muthumary, J. (2010). Antioxidant activity of endophytic fungus Phyllosticta sp. isolated from Guazuma tomentosa. J. Phytol. 2, 37–41.
Taher, M. A., Tan, W. N., Chear, N. J. Y., Leong, C. R., Rashid, S. A., and Tong, W. Y. (2022). Metabolites characterisation of endophytic Phyllosticta fallopiae L67 isolated from aloe vera with antimicrobial activity on diabetic wound microorganisms. Nat. Prod. Res. 2022, 1–6. doi: 10.1080/14786419.2022.2103127
Tambe, V. D., and Bhambar, R. S. (2014). Estimation of total phenol, tannin, alkaloid and flavonoid in Hibiscus tiliaceus Linn. wood extracts. RRJPP 2, 41–47.
Tamura, K., Nei, M., and Kumar, S. (2004). Prospects for inferring very large phylogenies by using the neighbor-joining method. Proc. Natl. Acad. Sci. U.S.A. 101, 11030–11035. doi: 10.1073/PNAS.0404206101
Tamura, K., Stecher, G., and Kumar, S. (2021). MEGA11: Molecular evolutionary genetics analysis version 11. Mol. Biol. Evol. 38, 3022–3027. doi: 10.1093/MOLBEV/MSAB120
Tanapichatsakul, C., Khruengsai, S., Monggoot, S., and Pripdeevech, P. (2019). Production of eugenol from fungal endophytes Neopestalotiopsis sp. and Diaporthe sp. isolated from Cinnamomum loureiroi leaves. PeerJ 7:e6427. doi: 10.7717/PEERJ.6427/SUPP-3
Tani, M., Harimaya, K., Gyobu, Y., Sasaki, T., Takenouchi, O., Kawamura, T., et al. (2004). SF2809 compounds, novel chymase inhibitors from Dactylosporangium sp. 2. Struct. Eluc. J Antibiot. 57, 89–96. doi: 10.7164/antibiotics.57.89
Wang, S. S., Wang, D. M., Pu, W. J., and Li, D. W. (2013). Phytochemical profiles, antioxidant and antimicrobial activities of three Potentilla species. BMC Complement. Altern. Med. 13:1–11. doi: 10.1186/1472-6882-13-321
Wheeler, K. A., and Hocking, A. D. (1993). Interactions among xerophilic fungi associated with dried salted fish. J. Appl. Bacteriol. 74, 164–169. doi: 10.1111/J.1365-2672.1993.TB03010.X
White, T. J., Bruns, T., Lee, S., and Taylor, J. (1990). “Amplification and direct sequencing of fungal ribosomal RNA genes for phylogenetics,” in PCR protocols, a guide to methods and applications, (Academic Press), 315–322. *editor. doi: 10.1016/B978-0-12-372180-8.50042-1
Wikee, S., Lombard, L., Crous, P. W., Nakashima, C., Motohashi, K., Chukeatirote, E., et al. (2013). Phyllosticta capitalensis, a widespread endophyte of plants. Fungal Divers. 60, 91–105. doi: 10.1007/S13225-013-0235-8
Wu, S. H., He, J., Li, X. N., Huang, R., Song, F., Chen, Y. W., et al. (2014). Guaiane sesquiterpenes and isopimarane diterpenes from an endophytic fungus Xylaria sp. Phytochemistry 105, 197–204. doi: 10.1016/j.phytochem.2014.04.016
Xiao, Y., Li, H. X., Li, C., Wang, J. X., Li, J., Wang, M. H., et al. (2013). Antifungal screening of endophytic fungi from ginkgo biloba for discovery of potent anti-phytopathogenic fungicides. FEMS Microbiol. Lett. 339, 130–136. doi: 10.1111/1574-6968.12065
Xie, J. L., and Li, L. P. (1992). New metabolites from the fungus Stereum purpureum. Chin. J. Chem. 10, 537–543. doi: 10.1002/cjoc.19920100610
Zhang, H., Wang, G., Beta, T., and Dong, J. (2015). Inhibitory properties of aqueous ethanol extracts of propolis on alpha-glucosidase. Evid. Based Complement. Alternat. Med. 2015:383. doi: 10.1155/2015/587383
Zhao, J., Zhou, L., Wang, J., Shan, T., Zhao, J., Zhou, L., et al. (2010). Endophytic fungi for producing bioactive compounds originally from their host plants. Curr. Res. Technol. Educ. Trop. Appl. Microbiol. Microbial. Biotechnol. 1, 567–576.
Zhou, J., Diao, X., Wang, T., Chen, G., Lin, Q., Yang, X., et al. (2018). Phylogenetic diversity and antioxidant activities of culturable fungal endophytes associated with the mangrove species Rhizophora stylosa and R. mucronata in the South China sea. PLoS One. 13:e0197359. doi: 10.1371/JOURNAL.PONE.0197359
Keywords: medicinal plant, benzoin resin, antifungal, antioxidant, Trichoderma, Phyllosticta, Neopestalotiopsis
Citation: Elfiati D, Faulina SA, Rahayu LM, Aryanto A, Dewi RT, Rachmat HH, Turjaman M, Royyani MF, Susilowati A and Hidayat A (2022) Culturable endophytic fungal assemblages from Styrax sumatrana and Stryax benzoin and their potential as antifungal, antioxidant, and alpha-glucosidase inhibitory resources. Front. Microbiol. 13:974526. doi: 10.3389/fmicb.2022.974526
Received: 21 June 2022; Accepted: 03 October 2022;
Published: 04 November 2022.
Edited by:
Naser Safaie, Tarbiat Modares University, IranReviewed by:
Debdulal Banerjee, Vidyasagar University, IndiaCopyright © 2022 Elfiati, Faulina, Rahayu, Aryanto, Dewi, Rachmat, Turjaman, Royyani, Susilowati and Hidayat. This is an open-access article distributed under the terms of the Creative Commons Attribution License (CC BY). The use, distribution or reproduction in other forums is permitted, provided the original author(s) and the copyright owner(s) are credited and that the original publication in this journal is cited, in accordance with accepted academic practice. No use, distribution or reproduction is permitted which does not comply with these terms.
*Correspondence: Deni Elfiati, ZGVuaTFAdXN1LmFjLmlk; Asep Hidayat, YXNoZXBoaWRheWF0MTJAZ21haWwuY29t
†These authors have contributed equally to this work
Disclaimer: All claims expressed in this article are solely those of the authors and do not necessarily represent those of their affiliated organizations, or those of the publisher, the editors and the reviewers. Any product that may be evaluated in this article or claim that may be made by its manufacturer is not guaranteed or endorsed by the publisher.
Research integrity at Frontiers
Learn more about the work of our research integrity team to safeguard the quality of each article we publish.