- College of Resources and Environmental Sciences, China Agricultural University, Beijing, China
Nitrification inhibitor (NI) is often claimed to be efficient in mitigating nitrogen (N) losses from agricultural production systems by slowing down nitrification. Increasing evidence suggests that ammonia-oxidizing archaea (AOA) and ammonia-oxidizing bacteria (AOB) have the genetic potential to produce nitrous oxide (N2O) and perform the first step of nitrification, but their contribution to N2O and nitrification remains unclear. Furthermore, both AOA and AOB are probably targets for NIs, but a quantitative synthesis is lacking to identify the “indicator microbe” as the best predictor of NI efficiency under different environmental conditions. In this present study, a meta-analysis to assess the response characteristics of AOB and AOA to NI application was conducted and the relationship between NI efficiency and the AOA and AOB amoA genes response under different conditions was evaluated. The dataset consisted of 48 papers (214 observations). This study showed that NIs on average reduced 58.1% of N2O emissions and increased 71.4% of soil concentrations, respectively. When 3, 4-dimethylpyrazole phosphate (DMPP) was applied with both organic and inorganic fertilizers in alkaline medium soils, it had higher efficacy of decreasing N2O emissions than in acidic soils. The abundance of AOB amoA genes was dramatically reduced by about 50% with NI application in most soil types. Decrease in N2O emissions with NI addition was significantly correlated with AOB changes (R2 = 0.135, n = 110, P < 0.01) rather than changes in AOA, and there was an obvious correlation between the changes in concentration and AOB amoA gene abundance after NI application (R2 = 0.037, n = 136, P = 0.014). The results indicated the principal role of AOB in nitrification, furthermore, AOB would be the best predictor of NI efficiency.
Introduction
Nitrification is a crucial process in the nitrogen (N) cycle, involving the oxidization of ammonium () to nitrate () through nitrite (). The process supplies significant amounts of N to be taken up by growing crops. However, unabsorbed N is lost to the atmosphere or the soil below the root zone. These unwanted losses of N have significant implications for the environment, for example leaching and greenhouse gas emissions (GHG), particularly nitrous oxide (N2O). N2O is a potent GHG which greatly contributes to global climate change, it has a 265-fold higher global warming potential than CO2 (IPCC, 2014) and it is involved in the destruction of the protective ozone layer (Ravishankara et al., 2009), which has become one of society’s most important challenges (Desloover et al., 2012).
The application of nitrification inhibitor (NI) is a promising technology to reduce N losses in different kinds of soil systems. In agriculture, several chemical compounds were designed to delay the steps of microbial oxidation of to in the soil to decrease N2O emissions, such as 3, 4-dimethylpyrazole phosphate (DMPP), dicyandiamide (DCD), and 2-chloro-6-(trichloromethyl) pyridine (nitrapyrin). Of these, DMPP is the most efficient commercial compound, which is applied as dihydrogen phosphate salt to reduce its loss through evaporation. NIs target the first step, i.e., the enzyme ammonia monooxygenase (AMO) in the case of DMPP (and other N-containing inhibitors) presumably through reversible complexation of the enzyme’s Cu center (McCarty, 1999; Beeckman et al., 2018). Ammonia-oxidizing archaea (AOA) and bacteria (AOB) both perform the first step of nitrification and are probably targets for NIs. The impact of NIs in delaying nitrification and reducing N2O emissions has been widely reported (Huang et al., 2014; Cai and Akiyama, 2017; Xu et al., 2019). However, the effectiveness of NIs varies greatly within different soils (Shi et al., 2017; Zhu et al., 2019), fertilizers (Pereira et al., 2010), and moisture content (Chen et al., 2010; Hu et al., 2015a; Fan et al., 2019). Soil temperature is another key factor controlling NI efficiency, which can subside after 1 week at 35°C (Barth et al., 2008; Chen et al., 2015). Furthermore, many studies focused on the impact and contribution of soil microorganisms on N2O emissions (Chen et al., 2019; Lazcano et al., 2021; Yang et al., 2021; You et al., 2022). However, there is still a lack of direct evidence on whether soil microbial community, especially AOA and AOB, affects NI efficacy (Chen et al., 2010; Guardia et al., 2018; Lam et al., 2018).
Within the major N-cycling microbes, AOA and AOB are important functional strains, and both carry the amoA gene which encodes AMO (Xia et al., 2018). Due to its strong conserved nature, the amoA gene is often used as a biomarker for exploring ammonia-oxidizing microorganisms (Schleper and Nicol, 2010). This has certain advantages in analyzing the genetic diversity of ammonia-oxidizing microorganisms. The differences in cellular biochemistry and physiology between AOA and AOB lead to their different ecological niches in different agroecosystems in terms of sensitivity to soil pH, soil texture, N forms, moisture, temperature and other conditions (Morimoto et al., 2011; Prosser and Nicol, 2012; Hu et al., 2015b). Hu et al. (2013) showed that the increase of nitrification activity in most acidic soils was positively correlated with the increase of AOA quantity, but not with AOB. In general, AOB dominates nitrification in neutral and alkaline soils, while AOA is more suitable to the acidic environment (Lu et al., 2012; Li et al., 2018). Increasing the concentration will enhance the nitrification activity of AOB (Okano et al., 2014), while AOA prefers an environment with a lower concentration. For example, a low pH environment is favorable for the formation of and changes the utilization of by AOB (Ying et al., 2017). Therefore, different edaphic and environmental conditions would influence AOA and AOB nitrification activity, and in turn affect the response of AOA and AOB to NI application.
To date, most studies on the inhibitory effect of NIs on AOA and AOB have focused either on the change of the amoA gene population (Prosser and Nicol, 2008; Kleineidam et al., 2011) or on the change of the AOA and AOB community (Zhang et al., 2012a; Liu et al., 2015). There is very little research available with respect to the “indicator microbe,” AOA or AOB, as the best predictor of NI efficiency under different environmental conditions. In acidic soils, AOA played a dominant role in nitrification and N2O production (Liu et al., 2016; Gu et al., 2019; Zhou et al., 2020), but NIs especially DMPP showed a lower inhibitory effect in acidic soils. Furthermore, many studies showed that NIs effectively decreased the AOB population, but not AOA (Gong et al., 2013; Liu et al., 2015, 2017; Dong et al., 2018; Yin et al., 2021). Hence, it was hypothesized that AOB are more sensitive to NIs than AOA and NIs would work through selectively inhibiting AOB.
Using a meta-analytical approach, results of 48 individual studies were combined to estimate the variations of NI efficiency in different edaphic and experimental conditions. Moreover, the general trends in the response of AOA and AOB abundance to NI addition were explored. Lastly, the efficiency of NIs was investigated by looking at the relationship among AOA or AOB amoA gene abundance and N2O emissions. This approach will help to identify the “indicator microbe,” AOA or AOB, as the best predictor of NI efficiency under different environmental conditions.
Materials and methods
Data compilation
The databases used for the data collection included Web of Science, WanFang digital database and China knowledge Resource Integrated to search for relevant studies published between 2010 and 2021. The key search terms were: nitrification inhibitors, nitrification, N2O, amoA gene, AOA, AOB, ammonia-oxidizing. The number of studies selected at various stages is shown in the flow diagram in Figure 1. After screening the literature, the database consisted of 214 selected pairwise comparisons reported in 48 studies (Supplementary Table 1), which met predetermined quality criteria (studies with replication, with detailed information, and performed under greenhouse, field and controlled laboratory conditions) (Abalos et al., 2022). All the studies included pairwise comparisons in which treated soil (with NI addition) was compared to an untreated control (without NI addition). Furthermore, the collated observations which were screened should measure the abundance of the amoA functional gene for AOB and AOA and the studied ecosystem type belonged to pastoral or agricultural environments.
In these present analyses, to take full advantage of published results, multiple experimental treatments from the same study were included (e.g., treatments that varied by N fertilizer type). However, only one measurement from each experimental replicate was included to maximize independence among measurements (Carey et al., 2016). For instance, the highest concentration was selected from the studies where concentration was measured multiple times from the same treatment.
The mitigation of cumulative N2O emissions and the changes in concentrations were considered as the evaluation variables of the NI inhibitory effect. The change of amoA gene abundance was reflected as the influence of NIs on microorganisms. Of all the 214 observations in the present study, observations 155, 174, 166, 147 concerned N2O yield, concentration, AOB amoA and AOA amoA gene abundance, respectively. Data on soil physical and chemical properties and experimental conditions were also collected from the original literature to analyze their influence on NI efficacy. Soil pH, soil organic matter (SOM), soil texture, soil moisture content (water filled pore space, WFPS and water holding capacity, WHC), soil temperature (TEMP), N fertilizer type, N application rate (NR), and NIs type were chosen to assess how edaphic conditions and management measures influenced NI efficacy. The following were the categorical variables classified into different groups:
– Soil pH: (1) soil pH ≤ 6, (2) 6 < soil pH < 8, (3) soil pH ≥ 8
– SOM (g kg–1): (1) SOM ≤ 20, (2) 20 < SOM ≤ 40, (3) SOM > 40
– Soil texture: (1) coarse (sand, loamy sand, sandy loam, loam, silt loam, and silt), (2) medium (sandy clay loam, clay loam, and silty clay loam), (3) fine (sandy clay, silty clay, and clay)
– WFPS and WHC: (1) WFPS and WHC ≤ 40%, (2) 40% < WFPS and WHC ≤ 60%, (3) 60% < WFPS and WHC ≤ 80%, (4) WFPS and WHC > 80%
– TEMP (°C): (1) TEMP ≤ 20, (2) 20 < TEMP ≤ 25, (3) TEMP > 25
– N fertilizer type: (1) based fertilizer (including ammonium chloride (NH4Cl), ammonium nitrate (NH4NO3) and ammonium sulfate [(NH4)2SO4)], (2) organic fertilizer (including livestock manure and urine), (3) urea, (4) both (combination of organic and inorganic fertilizer)
– NR (kg N ha–1): (1) NR ≤ 100, (2) 100 < NR ≤ 150, (3) NR > 150
– NIs type: (1) DMPP, (2) DCD, (3) nitrapyrin and others
Data analysis
The natural logarithmic response ratio (lnRR) as an effect size for each observation was calculated as Equation (1) (Luo et al., 2006):
where Xt is the average value of index X from NIs treatments and Xc is the average from the control treatments.
The results were expressed by using the conversion equation according to Equation (2) as percentage change:
A positive percentage change indicated increases in N2O yield, concentration, and amoA gene abundance after NI addition, while a negative percentage change indicated decreases in these variables (Sha et al., 2020). Replication-based weighting was used to avoid the effect of extreme weightings, using the following Equation (3) (Groenigen et al., 2011):
where nt and nc were the number of replications in the treatment group and control group, respectively.
The mean effect size of environmental and management variables on NI efficacy was calculated by a random-effect model and 95% of confidence intervals (CIs) were produced by a bootstrapping procedure with 4,999 iterations (Sha et al., 2020). In the present meta-analysis, Metawin 2.1 software (Rosenberg et al., 2000) was applied to perform all the calculations. If the 95% CIs did not overlap zero, the effects of NIs on the evaluation variables were considered significant. When the 95% CIs of each categorical group did not overlap, there were significantly different from each other. For each categorical variable, total heterogeneity (Qt) was segmented into within-group (Qw) and between-group (Qb). Qb/Qt describes the proportion of total variation explained by each modifier. The P-value is the probability value for randomization tests (999 permutations) with sample size as the weighting function, calculated only for the Qb values. A particular categorical variable was considered to have a significant impact on the response ratio when Qb was significant (P < 0.05) and was larger than the critical value (Carey et al., 2016). The heterogeneity in different categorical groups for each explanatory variable was also reported in Tables 1, 2. Of all observations (from the 48 studies) included in this meta-analysis, 113 and 110 measured the effect of NIs on N2O emissions, and AOA and AOB amoA gene abundance simultaneously. Of those, 133 and 136 measured NI effects on concentration changes in addition to AOA and AOB amoA gene abundances, respectively. Based on these observations, a regression analysis was conducted in Origin 9.0 to explore the relationship between the effects of NIs on concentration, N2O emission and amoA gene abundance.
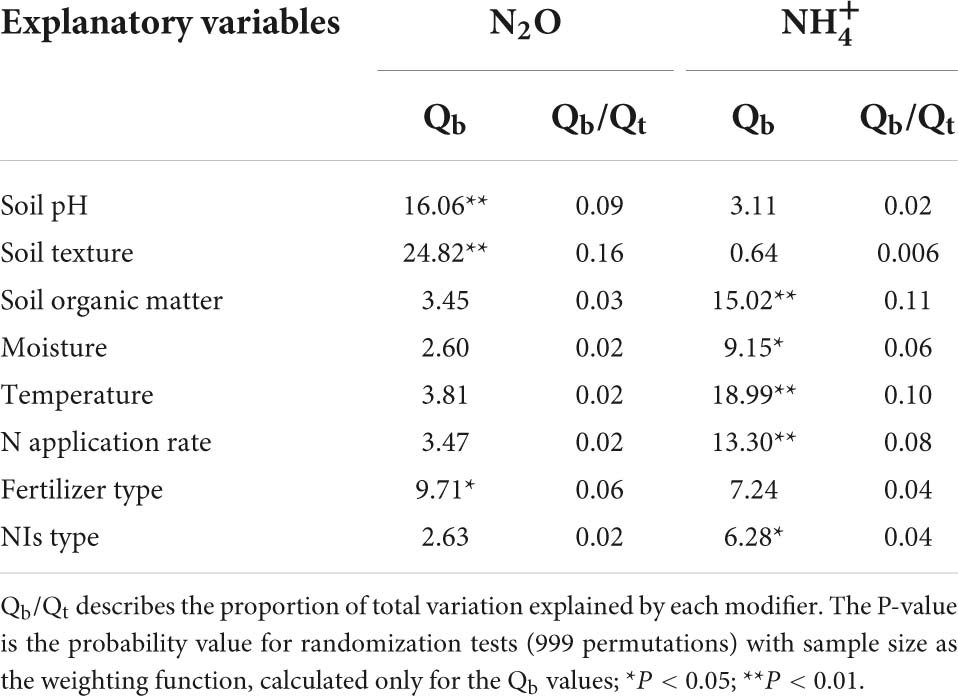
Table 1. Between-group heterogeneity (Qb) illustrating the effects of NIs additions on N2O emission and concentration across categorical modifiers.
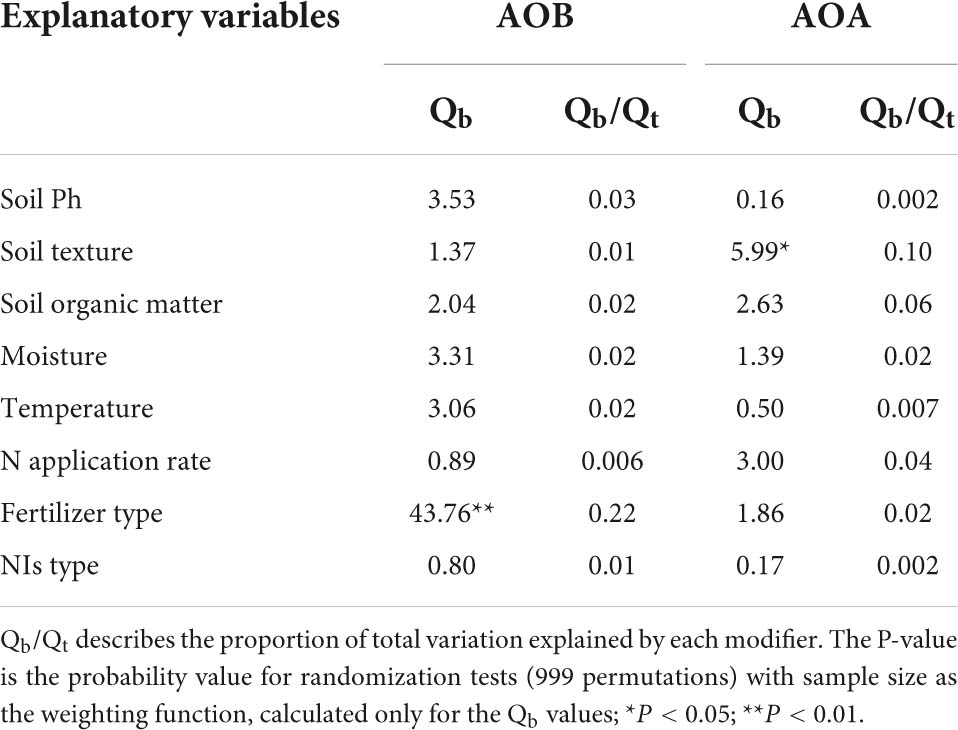
Table 2. Between-group heterogeneity (Qb) illustrating the effects of NIs additions on ammonia oxidizer across categorical modifiers.
Results
Inhibitory effect of nitrification inhibitors on nitrous oxide emissions
NIs effectively decreased N2O emissions across all experimental and edaphic conditions. For the efficacy of NIs, soil pH, soil texture and fertilizer type were the best explanatory variables (Table 1 and Figures 2A, 3A). N2O emissions were reduced by 54.9, 51.4, 77.4% by NIs in acidic, neutral and alkaline soils, respectively (Figure 2A), indicating that NIs performed better in alkaline soils than in neutral and acidic soils. The efficacy of NIs on reducing N2O emissions reached 75.2% in medium soil (Figure 2A, 95% CIs ranged 8.0–10.62%) while it only reached 46.9 and 47.7% in coarse and fine soils, respectively. The combined application of NIs with both organic and fertilizer or urea (at a relatively high N rate above 100 kg N ha–1) performed better (71.7%) than the combined application of NIs with organic or inorganic fertilizer alone (43.8 and 52.2%) (Figure 3A). Of all observations, DMPP was the best NI to mitigate N2O emissions (63.3%).
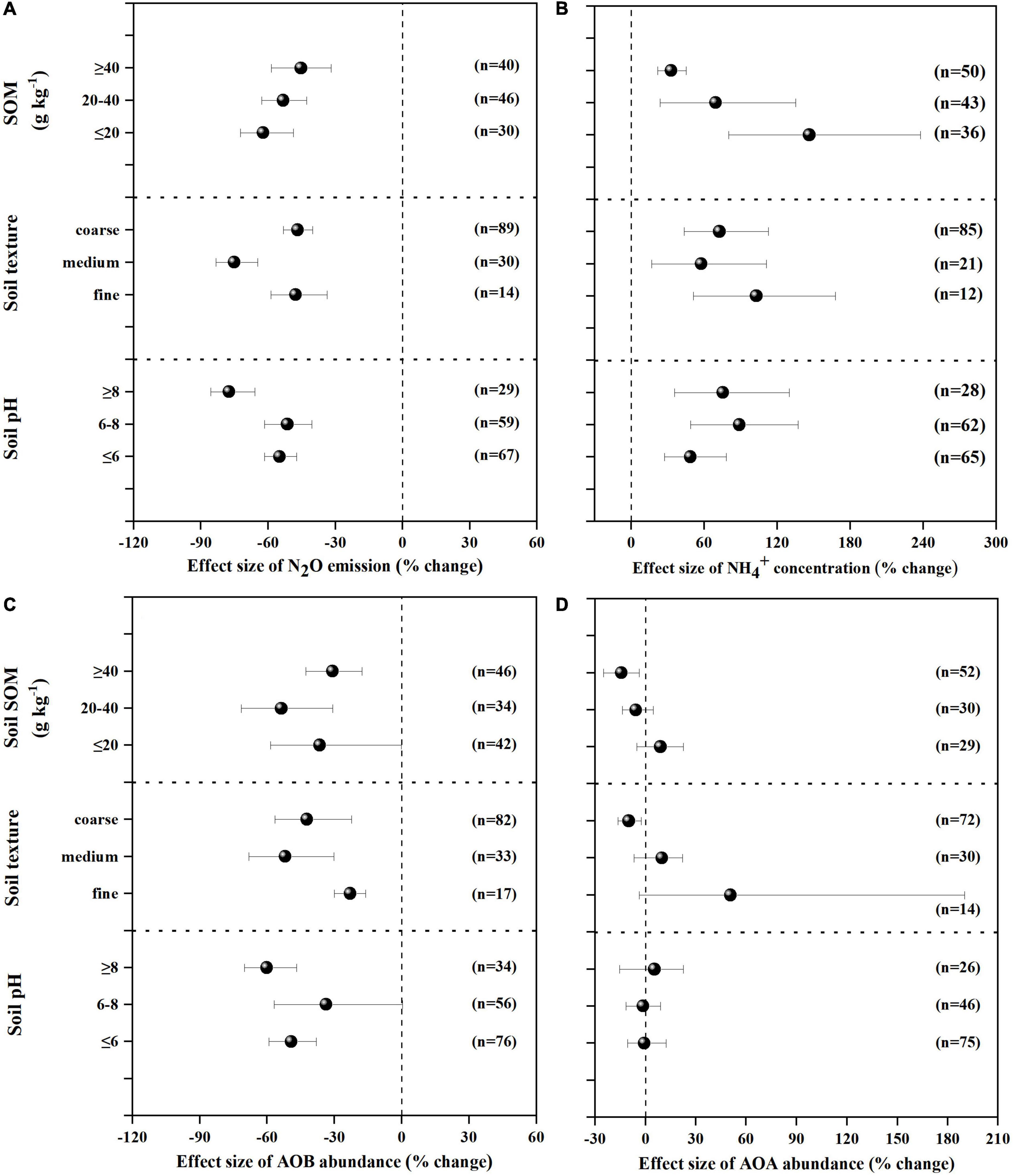
Figure 2. Mean response ratios (% change) and bootstrapped 95% Confidence Intervals (CI) for the effects of soil properties on the N2O emissions (A), concentration (B), AOB gene abundance (C) and AOA gene abundance (D) after NIs application. Values in parentheses represent the number of observations.
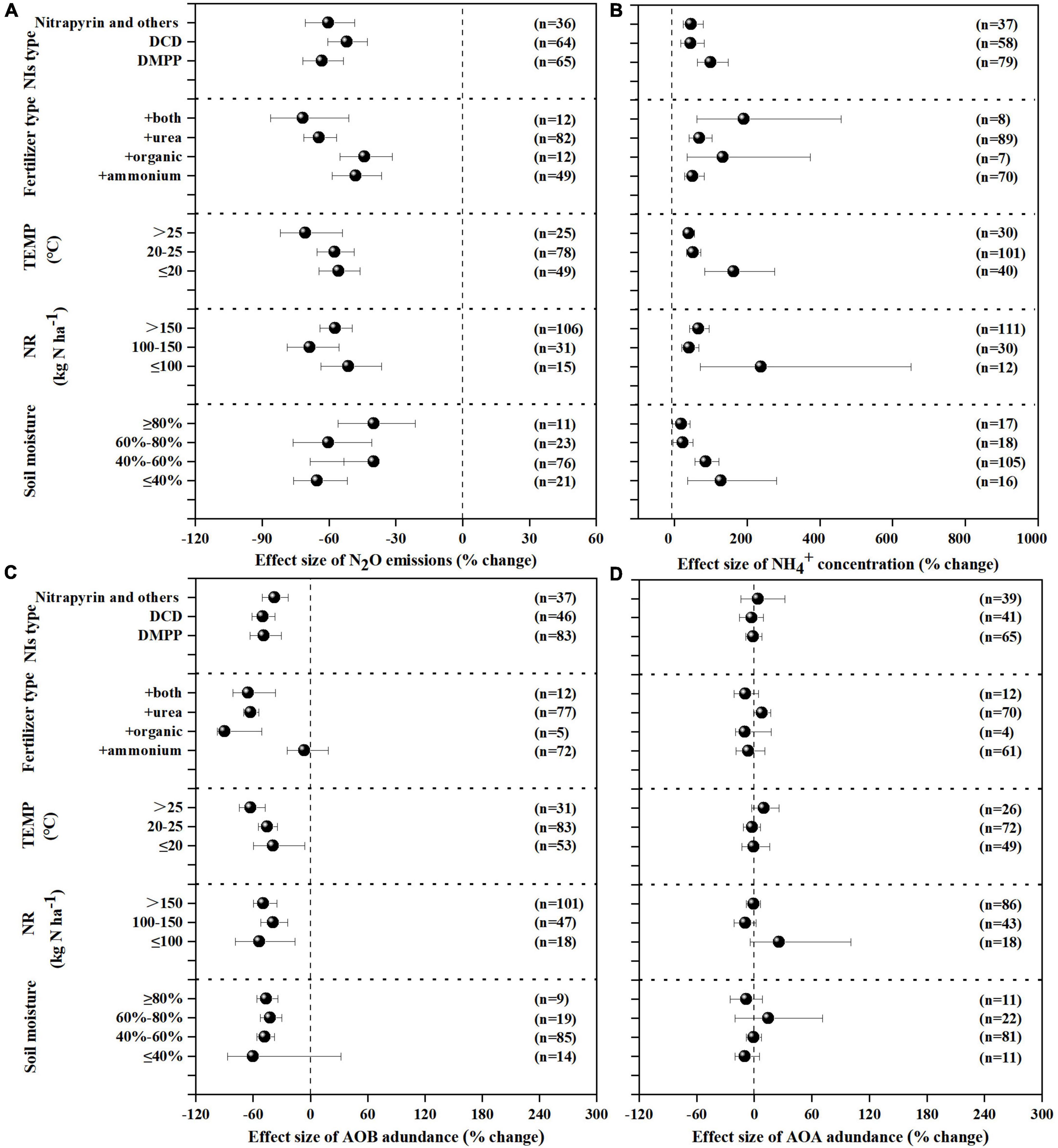
Figure 3. Mean response ratios (% change) and bootstrapped 95% Confidence Intervals (CI) for the effects of experiment conditions on the response of N2O (A), (B), AOB (C), AOA (D) after NIs treatment. Values in parentheses represent the number of observations.
Effect of nitrification inhibitors on concentration
concentration was increased by 71.4% on average with NI application across all experimental and edaphic conditions. NIs had a stronger ability to restrain the oxidization of in soil with low SOM (below 20 g kg–1) when the soil WHC/WFPS was lower than 40% (Figures 2B, 3B). The effect of NIs in slowing nitrification was better when the temperature was lower than 20°C (Figure 3B and Table 1, P < 0.01). Different NIs showed different efficacies in inhibiting nitrification, and DMPP was the most effective inhibitor compared with others (98.9% average change, 95% CIs range 36.5–48.8%, Figure 3B and Table 1, P < 0.05). The greater soil retention by DMPP was associated with a lower N application rate (below 100 kg N ha–1) (Figure 3B and Table 1, P < 0.05). In addition, concentration in alkaline and neutral soils was more responsive to NI addition than in acid soils.
Effect of nitrification inhibitors on ammonia-oxidizing bacteria and archaea
AOB amoA gene abundance negatively responded to NI addition (Figures 2C, 3C). The response ratio was always lower than or equal to zero, the magnitude significantly depended on fertilizer type (P < 0.01; Table 2). The efficacy of NIs on reducing AOB amoA gene abundance reached up to 90.08% when NIs were applied with organic fertilizer, which was higher than in NI application combined with inorganic fertilizer alone and with both organic and inorganic fertilizers (Figure 3C). However, no significant differences were observed in the response of AOB gene abundance to NIs across most of the categorical variables (P > 0.05; Table 2), including soil pH, NI type, SOM, moisture, TEMP, and NR.
The response ratio of AOA amoA gene abundance was always slightly lower than or equal to zero. It was observed that only soil texture had significant impact on the responses of AOA to NIs (P < 0.05; Table 2). Under certain experimental and edaphic conditions, NIs increased AOA amoA gene abundances (Figures 2D, 3D). Notably, AOA amoA gene abundance positively responded to NIs in medium and fine soils (P < 0.05; Table 2). Furthermore, when soil moisture was between 60 and 80% WHC/WFPS or NR was below 100 kg N ha–1, NIs could increase AOA amoA gene abundance (Figure 3D).
Relationship between nitrous oxide emissions, the efficiency of nitrification inhibitors and amoA gene response
The response ratio of AOB was significantly and positively correlated with N2O emissions (N2O emission[lnR] = 0.42 × AOB[lnR]−0.78, R2 = 0.14, P < 0.01; Figure 4A). In contrast, there was no significant correlation observed between the response ratio of AOA and N2O emissions (N2O emission[lnR] = −0.06 × AOA[lnR]−1.05, R2 < 0.00, P = 0.71; Figure 4A). There was an obvious correlation between the changes in concentration and AOB amoA gene abundance after NI application ( concentration[lnR] = −0.17 × AOB[lnR] + 0.47, R2 = 0.04, P = 0.014; Figure 4B).
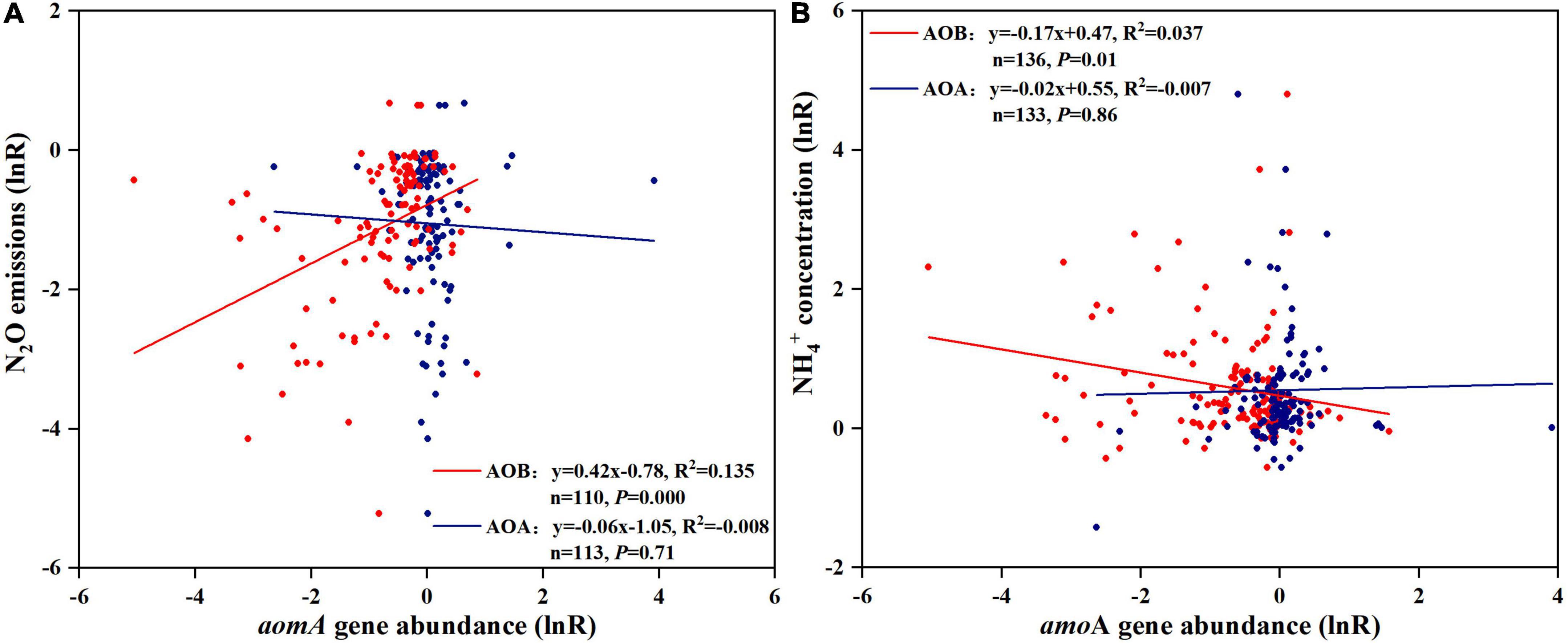
Figure 4. Relationship between effect size (lnR) of N2O emissions (A) or concentration (B) and effect size (lnR) of AOA and AOB amoA gene abundance. Line is the best-fit regression, where AOB-NIs effectiveness is the red line and AOA-NIs effectiveness is the blue line. Each symbol represents one observation; red point, AOB, blue point, AOA.
Discussion
Effect of edaphic and experimental conditions on nitrification inhibitor efficacy
Soil pH was an important explanatory variable for NI efficacy in reducing N2O emissions (Cui et al., 2021). The results from the current meta-analysis showed that NIs had different effects on decreasing N2O emissions under different soil pH (Figure 2A and Table 1, P < 0.01), and NIs efficacy had a positive response to soil pH. Firstly, it may be attributed to NIs being retained for longer in alkaline soils (pH ≥ 8). Soil pH has been considered as one of the most important factors controlling NI efficacy, because pH has potential to impact the degradation rate of the NIs in soils. Cui et al. (2021) showed that DMPP performed better in alkaline soil compared to acid soil conditions, which may be caused by the shorter half-life time of DMPP in acidic soil compared to alkaline soil. DMPP undergoes degradation in soil through chemical reaction steps, potentially involving reactive oxygen species (ROS) generated through abiotic and/or biotic processes (Sidhu et al., 2021), which would possibly be affected by pH. Secondly, soil pH played a vital role in controlling N2O emissions from soils (Morkved et al., 2007). Wang Y. et al. (2018) demonstrated that soil pH was negatively correlated with N2O emission, indicating less N2O emission from alkaline soils. In the current research, the inhibition efficacy of NIs on reducing N2O emissions increased with soil pH, indicating that NIs were more effective in alkaline soils. It may be also attributed to the less N2O emissions from alkaline soils.
SOM and soil texture were also considered as main factors affecting NI performance (Jarvis et al., 2007). Previous studies have reported a negative correlation between NI efficacy and SOM and clay content (Barth et al., 2008; Zhu et al., 2019). High SOM and clay content could easily adsorb NIs, which would influence their availability and effectiveness (Zhang et al., 2020; Cui et al., 2021). Furthermore, SOM could be used by soil microorganisms as energy, carbon (C), and N source, which improve microbial bioactivity, leading to accelerated biodegradation of NIs (Fisk et al., 2015). Clay had a protective effect on nitrifying oxidizers (Neufeld and Knowles, 1999). Higher clay content might make microorganisms less susceptible to being affected by inhibitors, thus weakening NIs inhibitory effects. Therefore, the current study found that NIs delayed ammonia oxidation and inhibited N2O emissions more efficiently in medium soils with lower SOM.
Soil temperature had a significant effect on NIs inhibition on nitrification (P < 0.01). Temperature influenced the rate of nitrification, which might affect the inhibitory effect of NIs on nitrification and retention (Mathieu et al., 2006). Irigoyen et al. (2003) reported that the nitrification rate accelerated at 20°C, but slowed down when the temperature reached up to 30°C. A lower temperature (≤ 20°C) was favorable for improving the efficacy of NIs on delaying the nitrification rate, which may also be attributed to the rapid decomposition of NIs by microorganisms in high temperature (Irigoyen et al., 2003; Wang X. et al., 2018). Yu et al. (2015) found that the increased concentration by DMPP at 15°C was 56 times higher than that at 25°C due to better persistence of the molecule of DMPP at 15°C. Hauser and Haselwandter (1990) also demonstrated that the degradation rate of DCD reached its highest between 25°C and 33°C. The above studies were consistent with the results in this study, in which the addition of NIs increased concentration in temperatures below 20°C.
Kirschke et al. (2019) found that the effect of NIs on nitrification was negatively correlated with soil moisture, which was consistent with this study. The probable reason was that higher water content may increase the distance between NI and because of faster diffusion of than that of NIs (Azam et al., 2001). On the other hand, the soil was supposed to be hypoxic at high water content (80% WFPS), inducing denitrification occurrence and dominance (Menéndez et al., 2008). Nitrification dominated at 40% WFPS, which was conducive to the effect of NIs on retention (Menéndez et al., 2012). This would also explain the negative correlation between the effect of NIs on retention and soil moisture in the current study.
The combined application of NIs with the appropriate N fertilizers could improve their efficacy (Vinzent et al., 2018). The present results showed that the combined application of NIs with organic fertilizer could enhance NIs inhibitory effect on N2O emissions. On the one hand, the application of organic fertilizer significantly improved soil pH, which could prolong the retention time of NIs and thus improve the efficacy of NIs in inhibiting N2O emissions (Zhang et al., 2012b). On the other hand, as observed in the current study, the efficacy of NIs in reducing AOB amoA gene abundance was highest when NIs were applied with organic fertilizer, thus N2O emission mitigation by NIs reaching its maximum. NR significantly influenced the effect size of NIs on retention. Better NI efficacy in increasing concentration could be observed at a lower N application rate (≤100 kg N ha–1). This is in accordance with previous findings by Rowlings et al. (2016), which revealed that N application which was less than the conventional rate could increase DMPP performance. Inappropriate N application rates may result in a large N surplus, providing adequate substrate of for ammonia volatilization and thus reducing the efficacy of the NI in increasing concentration (Nauer et al., 2018).
Response of ammonia oxidizers to nitrification inhibitors
AOB amoA gene abundance negatively responded to NI addition under different edaphic and experimental conditions. However, in contrast to AOB, AOA amoA gene abundance responded positively to NI addition in medium and fine soils. Fan et al. (2019) found an increase in AOA abundance after DMPP application in the tested soils, which was consistent with our results. Our results were also in good agreement with the study by Hink et al. (2018) and Fan et al. (2022), which reported that AOA growth were accelerated while AOB were inhibited with NIs. The growth of AOA might be promoted by organic compounds (Tourna et al., 2011; Ai et al., 2013), and it is possibly because that NIs such as acetylene and DMPP could be available C substrates for AOA (Florio et al., 2014; Hink et al., 2017). Compared to coarse soils, fine and medium soils showed a generally higher accumulation potential of SOM which provided sufficient C and N substrates for AOA proliferation (Kögel-Knabner et al., 2008; Dieckow et al., 2009). In line with results of this study, Shen et al. (2013) illustrated that most of the NIs appeared to have no effect on AOA in agricultural soils. Shi et al. (2016) also discovered that DMPP could strongly influence the metabolic activity of AOB by using DNA-stable isotope probing (SIP) but did not influence AOA. The potential physiological or metabolic differences between AOA and AOB (Prosser and Nicol, 2012) may explain the different responses of AOB and AOA to NIs. Furthermore, the most commonly used inhibitors suppressed microbial activity by chelating Cu active sites in AMO, and the periplasmic AmoB, a subunit of ammonia monooxygenase, presumably contains a copper-catalyzed active site (Monaghan et al., 2013; Beeckman et al., 2018). Lawton et al. (2014) found that archaea AmoB is a non-active enzyme and NIs tend to chelate on the active site of AOB to inhibit its activity, which indicated that the structural difference of the AmoB subunit and the ecophysiological differences also possibly lead to the variation in sensitivity among AOA and AOB to NIs (Tolar et al., 2017).
Fertilizer forms significantly affected the response of AOB to NIs, rather than the response of AOA (Tao et al., 2017). The results from the current study demonstrated that NIs showed the best performance in slowing down AOB growth in the case of organic fertilizer application, however, there was no difference observed on AOA abundance with NI application under different fertilizer forms. Wang et al. (2014) found an obvious stimulating effect of manure fertilization on the efficacy of NIs in reducing the population of AOB rather than AOA in a paddy soil, which was confirmed by the results of the current analysis. The application of organic fertilizer would provide an ideal alkaline environment for NIs to reduce AOB amoA gene abundance, which may be attributed to better activity and sensitivity of AOB to NI addition under alkaline conditions. But AOA adapted to low pH conditions (i.e., have a pH optima below 7; Hatzenpichler, 2012).
The best-fit regression in this study showed that N2O mitigation and concentration increase by NIs was positively correlated with the decrease of AOB-amoA gene abundance by NI application but not AOA-amoA. This supported the hypothesis that AOB are more sensitive to NIs than AOA and NIs would work through selectively inhibiting AOB. Previous studies illustrated that although the number of AOA far exceeds that of AOB in most terrestrial ecosystems, the N2O production capacity of AOB was 10–1,000 times higher than that of AOA (Leininger et al., 2006; Jung et al., 2011; Xia et al., 2011; Gu et al., 2019). The main reason for that was that AOB-related N2O was produced via nitrifier-denitrification and incomplete NH2OH oxidation (Shaw et al., 2006; Wu et al., 2018), while the N2O produced by AOA could not be attributed to nitrifier-denitrification, due to a lack of NO reductase (Tourna et al., 2011; Jung et al., 2014; Stieglmeier et al., 2014). Kozlowski et al. (2016) showed direct evidence that N2O produced by AOA was attributed to abiotic reactions of released NO under anoxic conditions, in which Nitrososphaera viennensis EN76(T) (a Thaumarchaeon) was used as a test AOA. There was an obvious correlation between and AOB (P < 0.05; Figure 4B), indicating the high inhibitory effect of NIs on nitrification through inhibiting AOB, which was consistent with the results reported by Zerulla et al. (2001) and Di and Cameron (2011). The obvious correlation between concentration and AOB also revealed the dominate role of AOB in nitrification. Although the relationship between AOA amoA gene abundance and N2O emissions, concentration after NI application was found to be insignificant in this study, AOA was also important for nitrification in soils. AOA had been shown to play an integral role in soil nitrification of some unmanaged soils (Huang et al., 2011; Isobe et al., 2015), with the greatest contribution likely occurring in N-limited scenarios. As observed in this study, AOB was more sensitive to NIs than AOA, even in soils where AOA were more abundant.
Conclusion
Soil pH, soil texture, SOM, soil temperature, and N application rate were identified to be the factors most affecting the efficacy of NIs. There was a significant positive correlation between NIs efficacy on decreasing N2O emissions, increasing concentration and AOB amoA gene abundance reduction after NIs. Taken together, for both soil and experimental conditions, AOB plays a key role in nitrification and NIs specifically inhibit AOB rather than AOA, which indicates AOB would be the best predictor of NI efficiency. These results would provide a scientific basis for better modeling and N management strategies to reduce N2O emissions and improve N use efficiency in agricultural systems.
Data availability statement
The original contributions presented in this study are included in the article/Supplementary Material, further inquiries can be directed to the corresponding author.
Author contributions
JL: data curation, writing – original draft preparation and review and editing, conceptualization, and validation. QF: methodology, software, and writing – original draft preparation. JiY: formal analysis, investigation, and resources. YM and JuY: writing – reviewing and editing. RL: supervision, project administration, funding acquisition, conceptualization, and writing – reviewing and editing. All authors contributed to manuscript revision, read, and approved the submitted version.
Funding
This research was supported by the National Natural Science Foundation of China (grant no. 42007031).
Conflict of interest
The authors declare that the research was conducted in the absence of any commercial or financial relationships that could be construed as a potential conflict of interest.
Publisher’s note
All claims expressed in this article are solely those of the authors and do not necessarily represent those of their affiliated organizations, or those of the publisher, the editors and the reviewers. Any product that may be evaluated in this article, or claim that may be made by its manufacturer, is not guaranteed or endorsed by the publisher.
Supplementary material
The Supplementary Material for this article can be found online at: https://www.frontiersin.org/articles/10.3389/fmicb.2022.962146/full#supplementary-material
References
Abalos, D., Rittl, T. F., Recous, S., Thiébeau, P., Topp, C. F. E., Groenigen, K. J., et al. (2022). Predicting field N2O emissions from crop residues based on their biochemical composition: a meta-analytical approach. Sci. Total Environ. 812:152532. doi: 10.1016/j.scitotenv.2021.152532
Ai, C., Liang, G., Sun, J., Wang, B., He, P., and Zhou, W. (2013). Different roles of rhizosphere effect and long-term fertilization in the activity and community structure of ammonia oxidizers in a calcareous fluvo-aquic soil. Soil Biol. Biochem. 57, 30–42. doi: 10.1016/j.soilbio.2012.08.003
Azam, F., Benckiser, G., Müller, C., and Ottow, J. C. G. (2001). Release, movement and recovery of 3, 4-dimethlpyrazole phosphate(DMPP), ammonium and nitrate from stabilized nitrogen fertilizer granules in a soil under laboratory conditions. Biol. Fertil. Soils 34, 118–125. doi: 10.1007/s003740100384
Barth, G., Tucher, S. V., and Schmidhalter, U. (2008). Effectiveness of 3, 4-dimethylpyrazole phosphate as nitrification inhibitor in soil as influenced by inhibitor content, application form, and soil matric potential. Pedosphere 18, 378–385. doi: 10.1016/S1002-0160(08)60028-4
Beeckman, F., Motte, H., and Beeckman, T. (2018). Nitrification in agricultural soils: impact, actors and mitigation. Curr. Opin. Biotechnol. 50, 166–173. doi: 10.1016/j.copbio.2018.01.014
Cai, Y., and Akiyama, H. (2017). Effects of inhibitors and biochar on nitrous oxide emissions, nitrate leaching, and plant nitrogen uptake from urine patches of grazing animals on grasslands: a meta-analysis. Soil Sci. Plant Nutr. 63, 405–414. doi: 10.1080/00380768.2017.1367627
Carey, C. J., Dove, N. C., Beman, J. M., Hart, S. C., and Aronson, E. L. (2016). Meta-analysis reveals ammonia-oxidizing bacteria respond more strongly to nitrogen addition than ammonia-oxidizing archaea. Soil Biol. Biochem. 99, 158–166. doi: 10.1016/j.soilbio.2016.05.014
Chen, D., Suter, H. C., Islam, A., and Edis, R. (2010). Influence of nitrification inhibitors on nitrification and nitrous oxide (N2O) emission from a clay loam soil fertilized with urea. Soil Biol. Biochem. 42, 660–664. doi: 10.1016/j.soilbio.2009.12.014
Chen, H., Yin, C., Fan, X., Ye, M., Peng, H., Li, T., et al. (2019). Reduction of N2O emission by biochar and/or 3,4-dimethylpyrazole phosphate (DMPP) is closely linked to soil ammonia oxidizing bacteria and nosZI-N2O reducer populations. Sci. Total Environ. 694:133658. doi: 10.1016/j.scitotenv.2019.133658
Chen, Q., Qi, L., Bi, Q., Dai, P., Sun, D., Sun, C., et al. (2015). Comparative effects of 3,4-dimethylpyrazole phosphate (DMPP) and dicyandiamide (DCD) on ammonia-oxidizing bacteria and archaeaina vegetable soil. Appl. Microbiol. Biotechnol. 99, 477–487. doi: 10.1007/s00253-014-6026-7
Cui, L., Li, D., Wu, Z., Xue, Y., Xiao, F., Zhang, L., et al. (2021). Effects of Nitrification inhibitors on soil nitrification and ammonia volatilization in three soils with different pH. Agronomy 11, 1674. doi: 10.3390/agronomy11081674
Desloover, J., Vlaeminck, S. E., Verstraete, W., and Boon, N. (2012). Strategies to mitigate N2O emissions from biological nitrogen removal systems. Curr. Opin. Biotechnol. 23, 474–482. doi: 10.1016/j.copbio.2011.12.030
Di, H., and Cameron, K. C. (2011). Inhibition of ammonium oxidation by a liquid formulation of 3,4-Dimethylpyrazole phosphate (DMPP) compared with a dicyandiamide (DCD) solution in six new Zealand grazed grassland soils. J. Soils Sediments 11, 1032–1039. doi: 10.1007/s11368-011-0372-1
Dieckow, J., Bayer, C., Conceição, P. C., Zanatta, J. A., Martin-Neto, L., Milori, D. B. M., et al. (2009). Land use, tillage, texture and organic matter stock and composition in tropical and subtropical Brazilian soils. Eur. J. Soil Sci. 60, 240–249. doi: 10.1111/j.1365-2389.2008.01101.x
Dong, D., Kou, Y., Yang, W., Chen, G., and Xu, H. (2018). Effects of urease and nitrification inhibitors on nitrous oxide emissions and nitrifying/denitrifying microbial communities in a rainfed maize soil: a 6-year field observation. Soil Tillage Res. 180, 82–90. doi: 10.1016/j.still.2018.02.010
Fan, X., Chen, H., Yan, G., Ye, M., Yin, C., Li, T., et al. (2022). Niche Differentiation Among Canonical Nitrifiers and N2O Reducers Is Linked to Varying Effects of Nitrification Inhibitors DCD and DMPP in Two Arable Soils. Microb. Ecol. [Epub ahead of print]. doi: 10.1007/s00248-022-02006-8
Fan, X., Yin, C., Chen, H., Ye, M., Zhao, Y., Li, T., et al. (2019). The efficacy of 3, 4-dimethylpyrazole phosphate on N2O emissions is linked to niche differentiation of ammonia oxidizing archaea and bacteria across four arable soils. Soil Biol. Biochem. 130, 82–93. doi: 10.1016/j.soilbio.2018.11.027
Fisk, L. M., Maccarone, L. D., Barton, L., and Murphy, D. V. (2015). Nitrapyrin decreased nitrification of nitrogen released from soil organic matter but not amoA gene abundance at high soil temperature. Soil Biol. Biochem. 88, 214–223. doi: 10.1016/j.soilbio.2015.05.029
Florio, A., Clark, I. M., Hirsch, P. R., Jhurreea, D., and Benedetti, A. (2014). Effects of the nitrification inhibitor 3,4-dimethylpyrazole phosphate (DMPP) on abundance and activity of ammonia oxidizers in soil. Biol. Fertil. Soils 50, 795–807. doi: 10.1007/s00374-014-0897-8
Gong, P., Zhang, L., Wu, Z., Chen, Z., and Chen, L. (2013). Responses of ammonia-oxidizing bacteria and archaea in two agricultural soils to nitrification inhibitors DCD and DMPP: a pot experiment. Pedosphere 23, 729–739. doi: 10.1016/S1002-0160(13)60065-X
Groenigen, K. J. V., Osenberg, C. W., and Hungate, B. A. (2011). Increased soil emissions of potent greenhouse gases under increased atmospheric CO2. Nature 475, 214–216. doi: 10.1038/nature10176
Gu, Y., Mi, W., Xie, Y., Ma, Q., Wu, L., Hu, Z., et al. (2019). Nitrapyrin affects the abundance of ammonia oxidizers rather than community structure in a yellow clay paddy soil. J. soils Sediments 19, 872–882. doi: 10.1007/s11368-018-2075-3
Guardia, G., Marsden, K. A., Vallejo, A., Jones, D. L., and Chadwick, D. R. (2018). Determining the influence of environmental and edaphic factors on the fate of the nitrification inhibitors DCD and DMPP in soil. Sci. Total Environ. 624, 1202–1212. doi: 10.1016/j.scitotenv.2017.12.250
Hatzenpichler, R. (2012). Diversity, physiology, and niche differentiation of ammonia-oxidizing archaea. Appl. Environ. Microbiol. 78, 7501–7510. doi: 10.1128/aem.01960-12
Hauser, M., and Haselwandter, K. (1990). Degradation of dicyandiamide by soil bacteria. Soil Biol. Biochem. 22, 113–114. doi: 10.1016/0038-0717(90)90069-c
Hink, L., Gubry-Rangin, C., Nicol, G. W., and Prosser, J. I. (2018). The consequences of niche and physiological differentiation of archaeal and bacterial ammonia oxidisers for nitrous oxide emissions. ISME J. 12, 1084–1093. doi: 10.1038/s41396-017-0025-5
Hink, L., Nicol, G. W., and Prosser, J. I. (2017). Archaea produce lower yields of N2O than bacteria during aerobic ammonia oxidation in soil. Environ. Microbiol. 19, 4829–4837. doi: 10.1111/1462-2920.13282
Hu, H., Chen, D., and He, J. (2015b). Microbial regulation of terrestrial nitrous oxide formation understanding the biological pathways for prediction of emission rates. FEMS Microbiol. Rev. 39, 729–749. doi: 10.1093/femsre/fuv021
Hu, H., Zhang, L., Dai, Y., Di, H., and He, J. (2013). pH-dependent distribution of soil ammonia oxidizers across a large geographical scale as revealed by high-throughput pyrosequencing. J. Soils Sediments 13, 1439–1449. doi: 10.1007/s11368-013-0726-y
Hu, H., Zhang, L., Yuan, C., Zheng, Y., Wang, J., Chen, D., et al. (2015a). The large-scale distribution of ammonia oxidizers in paddy soils is driven by soil pH, geographic distance, and climatic factors. Front. Microbiol. 6:938. doi: 10.3389/fmicb.2015.00938
Huang, R., Wu, Y., Zhang, J., Zhong, W., Jia, Z., and Cai, Z. (2011). Nitrification activity and putative ammonia-oxidizing archaea in acidic red soils. J. Soils Sediments 12, 420–428. doi: 10.1007/s11368-011-0450-4
Huang, T., Gao, B., Hu, X., Lu, X., Well, R., Christie, P., et al. (2014). Ammonia-oxidation as an engine to generate nitrous oxide in an intensively managed calcareous Fluvo-aquic soil. Sci. Rep. 4:3950. doi: 10.1038/srep03950
IPCC (2014). “Climate change 2014: synthesis report,” in Contribution of Working Groups I, II and III to the Fifth Assessment Report of the Intergovernmental Panel on Climate Change, eds R. K. Pachauri and L. A. Meyer (Geneva: IPCC), 151.
Irigoyen, I., Muro, J., Azpilikueta, M., Aparicio-Tejo, P., and Lamsfus, C. (2003). Ammonium oxidation kinetics in the presence of nitrification inhibitors DCD and DMPP at various temperatures. Soil Res. 41, 1177–1183. doi: 10.1071/sr02144
Isobe, K., Ohte, N., Oda, T., Murabayashi, S., Wei, W., Senoo, K., et al. (2015). Microbial regulation of nitrogen dynamics along the hillslope of a natural forest. Front. Environ. Sci. 2:63. doi: 10.3389/fenvs.2014.00063
Jarvis, N., Larsbo, M., Roulier, S., Lindahl, A., and Persson, L. (2007). The role of soil properties in regulating non-equilibrium macropore flow and solute transport in agricultural topsoils. Eur. J. Soil Sci. 58, 282–292. doi: 10.1111/j.1365-2389.2006.00837.x
Jung, M. Y., Park, S. J., Min, D., Kim, J. S., Rijpstra, W. I. C., Damsté, J. S. S., et al. (2011). Enrichment and Characterization of an Autotrophic Ammonia-Oxidizing Archaeon of Mesophilic Crenarchaeal Group I.1a from an Agricultural Soil. Appl. Environ. Microbiol. 77, 8635–8647. doi: 10.1128/aem.05787-11
Jung, M. Y., Well, R., Min, D., Giesemann, A., Park, S. J., Kim, J. G., et al. (2014). Isotopic signatures of N2O produced by ammonia-oxidizing archaea from soils. ISME J. 8, 1115–1125. doi: 10.1038/ismej.2013.205
Kirschke, T., Spott, O., and Vetterlein, D. (2019). Impact of urease and nitrification inhibitor on and dynamic in soil after urea spring application under field conditions evaluated by soil extraction and soil solution sampling. J. Plant Nutr. Soil Sci. 182, 441–450. doi: 10.1002/jpln.201800513
Kleineidam, K., Kosmrlj, K., Kublik, S., Palmer, I., Pfab, H., Ruser, R., et al. (2011). Influence of the nitrification inhibitor 3, 4-dimethylpyrazole phosphate (DMPP) on ammonia-oxidizing bacteria and archaea in rhizosphere and bulk soil. Chemosphere 84, 182–186. doi: 10.1016/j.chemosphere.2011.02.086
Kögel-Knabner, I., Guggenberger, G., Kleber, M., Kandeler, E., Kalbitz, K., Scheu, S., et al. (2008). Organo-mineral associations in temperate soils: integrating biology, mineralogy, and organic matter chemistry. J. Plant Nutr. Soil Sci. 171, 61–82. doi: 10.1002/jpln.200700048
Kozlowski, J. A., Stieglmeier, M., Schleper, C., Klotz, M. G., and Stein, L. Y. (2016). Pathways and key intermediates required for obligate aerobic ammonia-dependent chemolithotrophy in bacteria and Thaumarchaeota. ISME J. 10, 1836–1845. doi: 10.1038/ismej.2016.2
Lam, S. K., Suter, S., Walker, C., Davies, R., Mosier, A. R., et al. (2018). Using urease and nitrification inhibitors to decrease ammonia and nitrous oxide emissions and improve productivity in a subtropical pasture. Sci. Total Environ. 644, 1531–1535. doi: 10.1016/j.scitotenv.2018.07.092
Lawton, T. J., Ham, J. W., Sun, T. L., and Rosenzweig, A. C. (2014). Structural conservation of the B subunit in the ammonia monooxygenase/particulate methane monooxygenase superfamily. Proteins 82, 2263–2267. doi: 10.1002/prot.24535
Lazcano, C., Zhu-Barker, X., and Decock, C. (2021). Effects of organic fertilizers on the soil microorganisms responsible for N2O emissions: a review. Microorganisms 9:983. doi: 10.3390/microorganisms9050983
Leininger, S., Urich, T., Schloter, M., Schwark, L., Qi, J., Nicol, G. W., et al. (2006). Archaea predominate among ammonia-oxidizing prokaryotes in soils. Nature 442, 806–809. doi: 10.1038/nature04983
Li, Y., Chapman, S. J., Nicol, G. W., and Yao, H. (2018). Nitrification and nitrifiers in acidic soils. Soil Biol. Biochem. 116, 290–301. doi: 10.1016/j.soilbio.2017.10.023
Liu, R., Hayden, H. L., Hu, H., He, J., Suter, H., and Chen, D. (2017). Effects of the nitrification inhibitor acetylene on nitrous oxide emissions and ammonia-oxidizing microorganisms of different agricultural soils under laboratory incubation conditions. Appl. Soil Ecol. 119, 80–90. doi: 10.1016/j.apsoil.2017.05.034
Liu, R., Hayden, H., Suter, H., He, J., and Chen, D. (2015). The effect of nitrification inhibitors in reducing nitrification and the ammonia oxidizer population in three contrasting soils. J. Soils Sediments 15, 1113–1118. doi: 10.1007/s11368-015-1086-6
Liu, R., Hayden, H., Suter, H., Hu, H., Lam, S. K., He, J., et al. (2016). The effect of temperature and moisture on the source of N2O and contributions from ammonia oxidizers in an agricultural soil. Biol. Fertil. Soils 53, 141–152. doi: 10.1007/s00374-016-1167-8
Lu, L., Han, W., Zhang, J., Wu, Y., Wang, B., Lin, X., et al. (2012). Nitrification of archaeal ammonia oxidizers in acid soils is supported by hydrolysis of urea. ISME J. 6, 1978–1984. doi: 10.1038/ismej.2012.45
Luo, Y., Hui, D., and Zhang, D. (2006). Elevated CO2 stimulates net accumulations of carbon and nitrogen in land ecosystems: a meta-analysis. Ecology 87, 53–63. doi: 10.1890/04-1724
Mathieu, O., Hénault, C., Lévêque, J., Baujard, E., Milloux, M. J., and Andreux, F. (2006). Quantifying the contribution of nitrification and denitrification to the nitrous oxide flux using 15N tracers. Environ. Pollut. 144, 933–940. doi: 10.1016/j.envpol.2006.02.005
McCarty, G. W. (1999). Modes of action of nitrification inhibitors. Biol. Fertil. Soils 29, 1–9. doi: 10.1007/s003740050518
Menéndez, S., Barrena, I., Setien, I., González-Murua, C., and Estavillo, J. M. (2012). Efficiency of nitrification inhibitor DMPP to reduce nitrous oxide emissions under different temperature and moisture conditions. Soil Biol. Biochem. 53, 82–89. doi: 10.1016/j.soilbio.2012.04.026
Menéndez, S., López-Bellido, R. J., Benítez-Vega, J., González-Murua, C., López-Bellido, L., and Estavillo, J. M. (2008). Long-term effect of tillage, crop rotation and N fertilization to wheat on gaseous emissions under rainfed Mediterranean conditions. Eur. J. Agron. 28, 559–569. doi: 10.1016/j.eja.2007.12.005
Monaghan, R. M., Smith, L. C., and de Klein, C. A. M. (2013). The effectiveness of the nitrification inhibitor dicyandiamide (DCD) in reducing nitrate leaching and nitrous oxide emissions from a grazed winter forage crop in southern New Zealand. Agric. Ecosyst. Environ. 175, 29–38. doi: 10.1016/j.agee.2013.04.019
Morimoto, S., Hayatsu, M., Hoshino, Y. T., Nagaoka, K., Yamazaki, M., Karasawa, T., et al. (2011). Quantitative analyses of ammonia-oxidizing archaea (AOA) and ammonia-oxidizing bacteria (AOB) in fields with different soil types. Microbes Environ. 26, 248–253. doi: 10.1264/jsme2.me11127
Morkved, P. T., Dorsch, P., and Bakken, L. R. (2007). The N2O product ratio of nitrification and its dependence on long-term changes in soil pH. Soil Biol. Biochem. 39, 2048–2057. doi: 10.1016/j.soilbio.2007.03.006
Nauer, P. A., Fest, B. J., Visser, L., and Arndt, S. K. (2018). On-farm trial on the effectiveness of the nitrification inhibitor DMPP indicates no benefits under commercial Australian farming practices. Agric. Ecosyst. Environ. 253, 82–89. doi: 10.1016/j.agee.2017.10.022
Neufeld, J. D., and Knowles, R. (1999). Inhibition of nitrifiers and methanotrophs from an agricultural humisol by allylsulfide and its implications for environmental studies. Appl. Environ. Microbiol. 65, 2461–2465. doi: 10.1128/aem.65.6.2461-2465.1999
Okano, Y., Hristova, K. R., Leutenegger, C. M., Jackson, L. E., Denison, R. F., Gebreyesus, B., et al. (2014). Application of real-time PCR to study effects of ammonium on population size of ammonia-oxidizing bacteria in soil. Appl. Environ. Microbiol. 70, 1008–1016. doi: 10.1128/aem.70.2.1008-1016.2004
Pereira, J., Fangueiro, D., Chadwick, D. R., Misselbrook, T. H., Coutinho, J., and Trindade, H. (2010). Effect of cattle slurry pre-treatment by separation and addition of nitrification inhibitors on gaseous emissions and N dynamics: a laboratory study. Chemosphere 79, 620–627. doi: 10.1016/j.chemosphere.2010.02.029
Prosser, J. I., and Nicol, G. W. (2008). Relative contributions of archaea and bacteria to aerobic ammonia oxidation in the environment. Environ. Microbiol. 10, 2931–2941. doi: 10.1111/j.1462-2920.2008.01775.x
Prosser, J. I., and Nicol, G. W. (2012). Archaeal and bacterial ammonia-oxidisers in soil: the quest for niche specialisation and differentiation. Trends Microbiol. 20, 523–531. doi: 10.1016/j.tim.2012.08.001
Ravishankara, A. R., Daniel, J. S., and Portmann, R. W. (2009). Nitrous Oxide (N2O): the Dominant Ozone-Depleting Substance Emitted in the 21st Century. Science 326, 123–125. doi: 10.1126/science.1176985
Rosenberg, M. S., Adams, D. C., and Gurevitch, J. (2000). MetaWin (Version 2). Sunderland: Sinauer Associates.
Rowlings, D. W., Scheer, C., Liu, S., and Grace, P. R. (2016). Annual nitrogen dynamics and urea fertilizer recoveries from a dairy pasture using 15N: effect of nitrification inhibitor DMPP and reduced application rates. Agric. Ecosyst. Environ. 216, 216–225. doi: 10.1016/j.agee.2015.09.025
Schleper, C., and Nicol, G. W. (2010). Ammonia-oxidising archaea: physiology, ecology and evolution. Adv. Microb. Physiol. 57, 1–41. doi: 10.1016/b978-0-12-381045-8.00001-1
Sha, Z., Ma, X., Wang, J., Lu, T., Li, Q., Misselbrook, T., et al. (2020). Effect of N stabilizer on fertilizer-N fate in soil-crop system: a meta-analysis. Agric. Ecosyst. Environ. 290:106763. doi: 10.1016/j.agee.2019.106763
Shaw, L. J., Nicol, G. W., Smith, Z., Fear, J., Prosser, J. I., and Baggs, E. M. (2006). Nitrosospira spp. can produce nitrous oxide via a nitrifier denitrification pathway. Environ. Microbiol. 8, 214–222. doi: 10.1111/j.1462-2920.2005.00882.x
Shen, T., Stieglmeier, M., Dai, J., Urich, T., and Schleper, C. (2013). Responses of the terrestrial ammonia-oxidizing archaeon Ca. Nitrososphaera viennensis and the ammonia-oxidizing bacterium Nitrosospira multiformis to nitrification inhibitors. FEMS Microbiol. Lett. 344, 121–129. doi: 10.1111/1574-6968.12164
Shi, X., Hu, H., Muller, C., He, J., Chen, D., and Suter, H. (2016). Effects of the nitrification inhibitor 3,4-dimethylpyrazole phosphate on nitrification and nitrifiers in two contrasting agricultural soils. Appl. Environ. Microbiol. 82, 5236–5248. doi: 10.1128/aem.01031-16
Shi, X., Hu, H., Zhu-Barker, X., Hayden, H., Wang, J., Suter, H., et al. (2017). Nitrifier-induced denitrification is an important source of soil nitrous oxide and can be inhibited by a nitrification inhibitor 3, 4-dimethylpyrazole phosphate. Environ. Microbiol. 19, 4851–4865. doi: 10.1111/1462-2920.13872
Sidhu, P. K., Taggert, B. I, Chen, D., and Wille, U. (2021). Degradation of the Nitrification Inhibitor 3,4-Dimethylpyrazole Phosphate in Soils: indication of Chemical Pathways. Agric. Sci. Technol. 1, 540–549. doi: 10.1021/acsagscitech.1c00150
Stieglmeier, M., Mooshammer, M., Kitzler, B., Wanek, W., Zechmeister-Boltenstern, S., Richter, A., et al. (2014). Aerobic nitrous oxide production through N-nitrosating hybrid formation in ammonia-oxidizing archaea. ISME J. 8, 1135–1146. doi: 10.1038/ismej.2013.220
Tao, R., Wakelin, S. A., Liang, Y., and Chu, G. (2017). Response of ammonia-oxidizing archaea and bacteria in calcareous soil to mineral and organic fertilizer application and their relative contribution to nitrification. Soil Biol. Biochem. 114, 20–30. doi: 10.1016/j.soilbio.2017.06.027
Tolar, B., Herrmann, J., Bargar, J. R., Bedem, H. V. D., Wakatsuki, S., and Francis, C. A. (2017). Integrated structural biology and molecular ecology of N-cycling enzymes from ammonia-oxidizing archaea. Environ. Microbiol. Rep. 9, 484–491. doi: 10.1111/1758-2229.12567
Tourna, M., Stieglmeier, M., Spang, A., Könneke, M., Schintlmeister, A., Urich, T., et al. (2011). Nitrososphaera viennensis, an ammonia oxidizing archaeon from soil. Proc. Natl. Acad. Sci. U.S.A. 108, 8420–8425. doi: 10.1073/pnas.1013488108
Vinzent, B., Fuss, R., Maidl, F. X., and Hülsbergen, K. J. (2018). N2O emissions and nitrogen dynamics of winter rapeseed fertilized with different N forms and a nitrification inhibitor. Agric. Ecosyst. Environ. 259, 86–97. doi: 10.1016/j.agee.2018.02.028
Wang, J., Zhang, L., Lu, Q., Raza, W., Huang, Q., and Shen, Q. (2014). Ammonia oxidizer abundance in paddy soil profile with different fertilizer regimes. Appl. Soil Ecol. 84, 38–44. doi: 10.1016/j.apsoil.2014.06.009
Wang, X., Yan, B., Fan, B., Shi, L., and Liu, G. (2018). Temperature and soil microorganisms interact to affect Dodonaea viscosa growth on mountainsides. Plant Ecol. 219, 759–774. doi: 10.1007/s11258-018-0832-4
Wang, Y., Guo, J., Vogt, R. D., Mulder, J., Wang, J., Zhang, X., et al. (2018). Soil pH as the chief modifier for regional nitrous oxide emissions: new evidence and implications for global estimates and mitigation. Glob. Change Biol. 24, e617–e626. doi: 10.1111/gcb.13966
Wu, D., Zhao, Z., Han, X., Meng, F., Wu, W., Zhou, M., et al. (2018). Potential dual effect of nitrification inhibitor 3, 4-dimethylpyrazole phosphate on nitrifier denitrification in the mitigation of peak N2O emission events in North China Plain cropping system. Soil Biol. Biochem. 121, 147–153. doi: 10.1016/j.soilbio.2018.03.010
Xia, F., Wang, J., Zhu, T., Zou, B., Rhee, S. K., and Quan, Z. (2018). Ubiquity and Diversity of Complete Ammonia Oxidizers (Comammox). Appl. Environ. Microbiol. 84:e01390–18. doi: 10.1128/aem.01390-18
Xia, W., Zhang, C., Zeng, X., Feng, Y., Weng, J., Lin, X., et al. (2011). Autotrophic growth of nitrifying community in an agricultural soil. ISME J. 5, 1226–1236. doi: 10.1038/ismej.2011.5
Xu, J., Zhu, T., Xue, W., Ni, D., Sun, Y., Yang, J., et al. (2019). Influences of nitrification inhibitor 3, 4-dimethylpyrazole phosphate (DMPP) and application method on nitrogen dynamics at the centimeter-scale. Eur. J. Soil Biol. 90, 44–50. doi: 10.1016/j.ejsobi.2018.12.004
Yang, L., Zhu, G., Ju, X., and Liu, R. (2021). How nitrification-related N2O is associated with soil ammonia oxidizers in two contrasting soils in China? Sci. Total Environ. 770:143212. doi: 10.1016/j.scitotenv.2020.143212
Yin, C., Fan, X., Chen, H., Jiang, Y., Ye, M., Yan, G., et al. (2021). 3, 4-Dimethylpyrazole phosphate is an effective and specific inhibitor of soil ammonia-oxidizing bacteria. Biol. Fertil. Soils 57, 753–766. doi: 10.1007/s00374-021-01565-1
Ying, J., Li, X., Wang, N., Lan, Z., He, J., and Bai, Y. (2017). Contrasting effects of nitrogen forms and soil pH on ammonia oxidizing microorganisms and their responses to long–term nitrogen fertilization in a typical steppe ecosystem. Soil Biol. Biochem. 107, 10–18. doi: 10.1016/j.soilbio.2016.12.023
You, L., Ros, G. H., Chen, Y., Yang, X., Cui, Z., Liu, X., et al. (2022). Global meta-analysis of terrestrial nitrous oxide emissions and associated functional genes under nitrogen addition. Soil Biol. Biochem. 165:108523. doi: 10.1016/j.soilbio.2021.108523
Yu, Q., Ma, J., Zou, P., Lin, H., Sun, W., Yin, J., et al. (2015). Effects of combined application of organic and inorganic fertilizers plus nitrification inhibitor DMPP on nitrogen runoff loss in vegetable soils. Environ. Sci. Pollut. Res. 22, 472–481. doi: 10.1007/s11356-014-3366-x
Zerulla, W., Barth, T., Dressel, J., Erhardt, K., von-Locquenghien, K. H., Pasda, G., et al. (2001). 3,4-Dimethylpyrazole phosphate (DMPP)-a new nitrification inhibitor for agriculture and horticulture. Biol. Fertil. Soils 34, 79–84. doi: 10.1007/s003740100380
Zhang, A., Liu, Y., Pan, G., Hussain, Q., Li, L., Zheng, J., et al. (2012b). Effect of biochar amendment on maize yield and greenhouse gas emissions from a soil organic carbon poor calcareous loamy soil from Central China Plain. Plant Soil 351, 263–275. doi: 10.1007/s11104-011-0957-x
Zhang, L., Hu, H., Shen, J., and He, J. (2012a). Ammonia-oxidizing archaea have more important role than ammonia-oxidizing bacteria in ammonia oxidation of strongly acidic soils. ISME J. 6, 1032–1045. doi: 10.1038/ismej.2011.168
Zhang, Z., Gao, Q., Yang, J., Li, L., Li, Y., Liu, J., et al. (2020). Effect of soil organic matter on adsorption of nitrification inhibitor nitrapyrin in black soil. Commun. Soil Sci. Plant Anal. 51, 883–895. doi: 10.1080/00103624.2020.1744636
Zhou, X., Wang, S., Ma, S., Zheng, X., Wang, Z., and Lu, C. (2020). Effects of commonly used nitrification inhibitors-dicyandiamide (DCD), 3,4-dimethylpyrazole phosphate (DMPP), and nitrapyrin-on soil nitrogen dynamics and nitrifiers in three typical paddy soils. Geoderma 380:114637. doi: 10.1016/j.geoderma.2020.114637
Keywords: nitrous oxide, DMPP, ammoxidation, edaphic conditions, microorganism
Citation: Lei J, Fan Q, Yu J, Ma Y, Yin J and Liu R (2022) A meta-analysis to examine whether nitrification inhibitors work through selectively inhibiting ammonia-oxidizing bacteria. Front. Microbiol. 13:962146. doi: 10.3389/fmicb.2022.962146
Received: 06 June 2022; Accepted: 29 June 2022;
Published: 19 July 2022.
Edited by:
Yong Li, Zhejiang University, ChinaReviewed by:
Hong Pan, Shandong Agricultural University, ChinaXiaoping Fan, Zhejiang University, China
Copyright © 2022 Lei, Fan, Yu, Ma, Yin and Liu. This is an open-access article distributed under the terms of the Creative Commons Attribution License (CC BY). The use, distribution or reproduction in other forums is permitted, provided the original author(s) and the copyright owner(s) are credited and that the original publication in this journal is cited, in accordance with accepted academic practice. No use, distribution or reproduction is permitted which does not comply with these terms.
*Correspondence: Rui Liu, rliu@cau.edu.cn
†These authors have contributed equally to this work and share first authorship