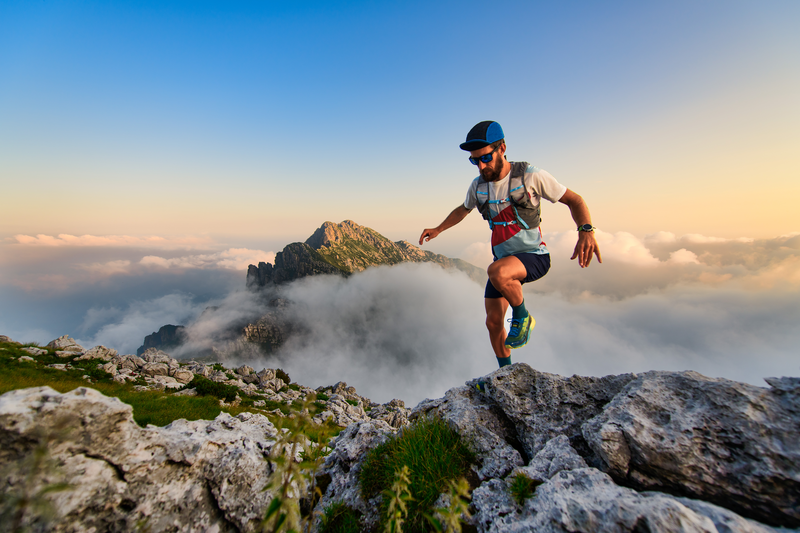
95% of researchers rate our articles as excellent or good
Learn more about the work of our research integrity team to safeguard the quality of each article we publish.
Find out more
ORIGINAL RESEARCH article
Front. Microbiol. , 07 July 2022
Sec. Aquatic Microbiology
Volume 13 - 2022 | https://doi.org/10.3389/fmicb.2022.950857
This article is part of the Research Topic Microbes from Marine Distinctive Environments View all 19 articles
Two new indole diketopiperazines (1–2) obtained from the fermentation culture of a deep-sea-derived fungus Aspergillus chevalieri MCCC M23426, were characterized, together with nine biogenetic related compounds (3–11). The structures of 1–2 were assigned based on NMR, MS, NMR calculation, DP4+ analysis, and ECD calculation. The bioactive assay showed that compounds 1, 5–7 significantly inhibited the growth of Staphylococcus aureus. Meanwhile, compound 8 potently reduced the cell viability of gastric cancer cell MKN1 with an IC50 value of 4.6 μM.
Indole diketopiperazines (indole DKPs) are a group of natural products with diketopiperazine backbones. The backbones are formed through the condensation of certain amino acids with L-tryptophan. There are multiple candidates for this amino acid, such as L-valine, L-tryptophan, L-alanine, or L-proline. After being modified by various tailoring enzymes the structures of the products can be very diverse. One family worth mentioning is the echinulin family which usually shared the same structure moiety of a cyclo-L-Trp-L-Ala with a prenyl group attached to C-2. The additional prenyl substituents and the diketopiperazine moiety with diverse oxidative states distinguish the members of this family from each other. Most of the reported echinulins were produced by fungi. Due to their diversity in structures and their broad biological and pharmacological activities, echinulins are of great interest to natural medicinal chemists. Several echinulins were reported to be promising leads. Neoechinulin A, isoechinulin A, and variecolorin G can scavenge free radicals such as DPPH. What is more, neoechinulin A also shows anti-inflammatory activity. It can function as a cytoprotective agent and can protect PC12 cells from peroxynitrite-induced death (Nies and Li, 2021).
Our previous work has shown that marine microorganisms are promising producers of bioactive compounds. The secondary metabolites of a deep-sea-derived Aspergillus chevalieri strain were studied in this work. The genus Aspergillus is very productive in bioactive compounds. Several bioactive products of Aspergillus chevalieri were reported. One new nonadride enantiomer named ent-epiheveadride was recently obtained from the marine-derived fungus Aspergillus chevalieri PSU-AMF79, together with five known dioxopiperazine derivatives (Ningsih et al., 2022).
In earlier research, sixteen metabolites were separated from the endolichenic fungus Aspergillus chevalieri SQ-8 (Lin et al., 2021). Seven of these compounds are C7-alkylated salicylaldehyde derivatives and nine are prenylated indole alkaloids. Interestingly, two indole DKPs were reported to be generated by the deep-sea cold-seep-derived fungus Aspergillus chevalieri CS-122 (Yan et al., 2022).
In this study, the extract of a deep-sea-derived Aspergillus chevalieri strain showed great potential in antibacterial and cytotoxic activities. Two new indole DKPs, 1–2, were obtained from the extract by biological activity-tracking, together with nine biogenetic related compounds (3–11) (Figure 1). Their structures were determined by multiple spectroscopic methods and their bioactivities were also evaluated.
A Chirascan spectrometer (Applied Photophysics) was used to detect optical rotations of new compounds in MeOH. A UV-1800 spectrophotometer (Shimadzu, Kyoto, Japan) was used to collect UV (Ultra Violet) data for new compounds dissolved in MeOH. NMR spectra were collected on a Bruker Avance 850 MHz spectrometer. A Waters Xevo G2 Q-TOF mass spectrometer was utilized to obtain accurate molecular weight. MPLC (Medium Pressure Liquid Chromatography) was undertaken using different packing materials such as silica gel (200–300 mesh, 300–400 mesh, Tsingtao Marine Chemical Co., Ltd., Tsingtao, China), RP-C18 (ODS-A, 50 μm, YMC, Kyoto, Japan), and Sephadex LH-20 (GE Healthcare Bio-Science AB, Pittsburgh, PA, United States). HPLC (High-Performance Liquid Chromatography) was conducted on an Agilent 1260 system using an RP-C18 column (5 μm, 20 × 250 mm, YMC, Kyoto, Japan) with HPLC grade MeOH and MeCN as mobile phases.
Strain R10-1G1 of Aspergillus chevalieri was identified by ITS sequence homology [100% similarity with Aspergillus chevalieri NRRL 78 (TYPE material) with a Genbank accession No. NR_135340 (max score 955, e value 0.0, query cover 100%)]. A voucher specimen was deposited at the Marine Culture Collection of China (MCCC) with the preservation number MCCC M23426. The strain was streaked on potato dextrose agar (PDA) slants and stored at 4 °C until use.
Details about the fermentation, extraction, and isolation were provided in the supporting information (Supplementary Material, “Fermentation, Extraction, and Isolation”).
5-prenylcryptoechinulin A (1): white amorphous solid; UV λmax (methanol) nm (log ε): 230 (4.71), 275 (4.45); 1H NMR and 13C NMR data are shown in Table 1; HR-ESI-MS: m/z 456.2650 [M – H]– (Calcd. 456.2651 for C29H34N3O2, Δ – 0.2 ppm).
9-epi-didehydroechinulin (2): white amorphous solid; UV λmax (methanol) nm (log ε): 230 (4.56), 275 (4.14); 1H NMR and 13C NMR data are shown in Table 1; HR-ESI-MS: m/z 482.2784 [M + Na]+ (Calcd. 482.2783 for C29H37N3O2Na, Δ + 0.2 ppm).
The conformer rotamer ensemble sampling tool (crest) (Pracht et al., 2020) was utilized to generate candidate conformers and DFT calculations were performed using the Gaussian 16 program (Frisch et al., 2016). The shielding constants were calculated by the GIAO method with TMS (Willoughby et al., 2014). More details about the computations and DP4+ analysis were provided in the supporting information (Supplementary Material, “NMR Calculation”) (Wang et al., 2018a,2020a,2020b).
The human gastric cancer cell line MKN1 was purchased from American Type Culture Collection (ATCC, LGC Standards SLU, Barcelona, Spain). The in vitro assay was carried out to investigate the effects of compounds 1-11 on the gastric cancer cells. The cell viability (CCK8 assays), colony formation, and cell apoptosis (Annexin V-FITC Apoptosis Staining/Detection Kit, BD, United States) were all evaluated and the details were described previously (Wang et al., 2014, 2018c,2019a). The protocol for antibacterial assay has also been described previously (Wang et al., 2018a,b, 2019b).
Compound 1 was isolated in the form of a colorless amorphous solid. Inspection of the HR-ESI-MS at m/z 456.2650 [M – H]– (calcd. 456.2651 for C29H34N3O2, Δ – 0.2 ppm, Supplementary Figure 1) allowed the establishment of its molecular formula as C29H35N3O2 with 14 degrees of unsaturation. The HSQC and 13C/DEPT135 spectra evidenced six methyl groups (C-18, 19, 24, 25, 29, and 30), five methylene groups (C-17, 20, 21, and 26), six methine groups (C-4, 7, 8, 16, 22, and 27), and ten quaternary carbons (C-2, 3, 5, 6, 3a, 7a, 9, 10, 12, 13, 15, 23, and 28) (Supplementary Figures 2, 3). Interpretation of UV absorptions at λmax 230 and 275 nm delineated the amide and conjugated indole moieties in 1 (Supplementay Figure 4). Cross peaks of H-4/C-6 and 7a, NH/C-2, 3, 3a and 7a, and H-7/C-5 and 3a, constructed the indole moiety. Cross peaks of H-8/C-2 and 3a (Figure 2 and Supplementary Figure 5) in HMBC spectra placed the C-8–C-9–C-10 unit at C-3. Detailed inspection of NMR data of 1 proved that 1 might be an indole diketopiperazine, structurally close to cryptoechinulin A (Supplementary Figures 6, 7). Additional signals were deduced to be a prenyl group connecting to C-5, based on the apparent single peaks at δH 7.07 and 7.17 (coupling constants were small and negligible). This was also supported by the HMBC correlation of H-22/C-5 (Table 1 and Supplementary Figure 8). As the NMR data was insufficient to support the configuration of C-8, NMR calculations at MPW1PW91-SCRF/6–31+G(d,p)//B3LYP/6-31G(d) for two candidate isomers (8Z)-1 and (8E)-1 were carried out. Through the comparison of the key computation parameters, as shown in Table 2, the calculated data of (8Z)-1 matched well with their experimental counterparts. In addition, the 100% DP4+ probability of (8Z)-1 (Table 2, Supplementary Tables 1–15, and Supplementary Figure 9) also supported this deduction. The structure of 1 was established and named 5-prenylcryptoechinulin A.
Compound 2 was isolated as a colorless amorphous solid. The UV spectra of 1 and 2 were similar which demonstrated that they shared the same backbone (Supplementary Figure 10). The molecular formula C29H37N3O2 with 13 degrees of unsaturation (Supplementary Figure 11) indicated that one double bond was absent in 2 compared to 1, and 2 was a hydrogenated derivative of 1. The correlations of H2-8 to C-2 and C-3a and of H-9 to C-3, C-10 and C-13 in the HMBC spectrum allowed the elucidation of the hydrogenated bond C-8-C-9 (Figure 2 and Supplementary Figures 12–16). The 1H-NMR signals at δ 7.14 (d, J = 1.5 Hz) and 6.81 (d, J = 1.5 Hz) indicated the presence of a 4,6 or 5,7 disubstituted benzene moiety (Table 1 and Supplementary Figure 17). The HMBC correlation of H-4/C-3 further placed the two prenyls at C-5 and C-7, respectively (Figure 2 and Supplementary Figure 15), excluding the possibility of 4,6-disubstitution. Next, TDDFT calculation of the ECD spectrum at Cam-B3LYP/Def2SVP (Supplementary Tables 16–22) revealed the stereochemistry of C-9 as R (Figure 3A), as the theoretical ECD curve was in agreement with the experimental one. The molecular orbital (MO) analysis was carried out for the optimal conformer of 2a (65.2%). The positive Cotton effect (CE) at ∼282 nm of 2 was thus related to electron transition from MO124 (HOMO) to MO125 (LUMO). The negative CE at ∼251 nm could be ascribed to the electron transition from MO124 (HOMO) to MO126 (LUMO+1). The positive CE at ∼219 nm was caused by the electron transition from MO124 (HOMO) to MO127 (LUMO+2) (Figure 3C). Finally, compound 2 was assigned as 9-epi-didehydroechinulin.
Figure 3. (A,B) Calculated and experimental ECD spectra of 2 and 3. (C,D) Key molecular orbitals (MOs) involved in the important transitions of 2 and 3.
Compound 3 was obtained as a colorless amorphous solid. Detailed analysis of the NMR (Supplementary Figures 18–23) and HR-ESI-MS data revealed that 3 shared the same planar structure as the known compound cryptoechinuline D, which was firstly isolated from Aspergillus amstelodami in 1976 (Gatti et al., 1976). The relative stereochemistry of cryptoechinuline D was previously determined to be 12S*, 28R*, and 31R* (Gao et al., 2013). In their work, two epimers of cryptoechinuline D were obtained by chiral HPLC and assigned by CD spectra as (12R, 28S, 31S) and (12S, 28R, 31R), respectively. However, after carefully examining the stereochemistry of C-12, 28, and 31 of cryptoechinuline D, we found that the relative stereochemistry reported by Gao (Gao et al., 2013) was wrong and actually should be assigned as 12S*, 28S*, 31R*. To unambiguously establish the relative configuration of compound 3 in the current study, we simplified the candidate structures for DFT calculations as 3a–d (Figure 4) and carried out the NMR calculation. By comparison of the calculated 1H NMR and 13C NMR of C-12, 13, 28, 29, 30, 31, 32, 33, the calculated 3c, corresponding to (12S*, 28S*, 31R*)-cryptoechinuline D, was found to be in better agreement with the experimental data, as indicated by the correlation coefficient (R2), the mean absolute error (MAE), and the corrected mean absolute error (CMAE) (Table 2). Subsequently, TDDFT ECD calculation at the CAM-B3LYP-SCRF/def2-SVP (IEFPCM) level of theory was performed to elucidate the absolute configuration of compound 3 as 12S, 28S, 31R (Figure 3B and Supplementary Tables 23–27). The MO analysis of the optimal conformer of (12S, 28S, 31R)-cryptoechinuline D (35.9%) revealed that the negative CE at ∼250 nm in the experimental curve of 3 was related to the electron transition from MO164 (HOMO-1) to MO168 (LUMO+2). The negative CE at ∼206 nm could be ascribed to the electron transition from MO159 (HOMO-6) to MO167 (LUMO+1) (Figure 3D). Finally, compound 3 was assigned as (12S, 28S, 31R)-cryptoechinulin D.
Figure 4. Linear regression analysis between the experimental and calculated NMR data of simplified conformers of 3a–d at C-12, 13, 28, 29, 30, 31, 32, 33.
The known compounds (4–11) were identified as neoechinuline (4) (Barbetta et al., 1969), cryptoechinulin A (5) (Cardillo et al., 1974), neoechinulin B (6) (Dossena et al., 1974), 7-prenylneoechinulin B (7) (Glisic et al., 2015; Nies and Li, 2021), neoechinulin D (8) (Dossena et al., 1975), variecolorin H (9) (Wang et al., 2007), cryptoechinulin C (10) (Cardillo et al., 1975), and neoechinulin A (11) (Cardillo et al., 1975), respectively, by comparing their spectroscopic data with those reported in the literature.
We first evaluated the antibacterial activities of 1–11 using strains of Staphylococcus aureus CICC 10384, Vibrio parahaemolyticus VP-HL, Vibrio parahaemolyticus ATCC 17802, and Escherichia coli CICC 10302. At the concentration of 250 μM, compounds 1, 5–7 displayed selective antibacterial activities against Staphylococcus aureus CICC 10384 with an inhibition rate of over 90%, while compounds 8 and 10 exhibited moderate and weak antibacterial activities with an inhibition rate of 76 and 41%, respectively. Compound 7 showed potent antibacterial activity against Staphylococcus aureus CICC 10384 with a MIC value of 62.5 μM (positive control: chloramphenicol, MIC: 15.5 μM). We then evaluated the cytotoxicity of 1–11 against the gastric cancer cell line MKN1. Compounds 6 and 8 exhibited growth inhibition effects with IC50 values of 20.7 and 4.6 μM, respectively. Other compounds displayed weak cytotoxic activities with IC50 values over 100 μM (positive control: cisplatin, IC50: 8.8 μM).
Indole DKPs are an important group of bioactive metabolites usually derived from fungi (Ma et al., 2016). Aspergillus is the most important genera in producing indole DKPs with great diversity in structures. Seven new prenylated indole DKPs were isolated from Aspergillus fumigatus (Wang et al., 2008). Three new indole DKPs were obtained from the Aspergillus taichungensis ZHN-7-07 (Cai et al., 2015). Three new indole DKPs were reported to be produced by Aspergillus ochraceus (Wen et al., 2018). A pair of enantiomeric indole DKPs were isolated from the mangrove endophytic fungus Aspergillus sp. SK-28 (Cai et al., 2019). Three new indole DKPs were generated by a soft coral-associated epiphytic fungus Aspergillus sp. EGF 15-0-3 (Wei et al., 2020). New indole DKPs were also obtained from the mangrove rhizosphere soil-derived fungus, Aspergillus effuses H1-1 (Gao et al., 2013), the terrestrial-derived endophytic fungus Aspergillus sp. (Lhamo et al., 2015), the soil-derived fungus Aspergillus ochraceopetaliformis (Mostafa et al., 2021), the marine-derived fungus Aspergillus sp. Z3 (Li et al., 2022), the marine endophytic fungus Aspergillus sp. YJ191021 (Yang et al., 2021), the deep-sea-derived fungus Aspergillus sp. FS445 (Liu et al., 2021), and so on. In this work, two new indole DKPs were purified from the fermentation of the Aspergillus chevalieri MCCC M23426. This further demonstrated that the capacity of the genera Aspergillus in producing novel indole DKPs is yet to be fully explored.
In addition to their structural diversity, indole DKPs also exhibit a variety of activities (Jia et al., 2018). The cytotoxic activities of different indole DKPs on MOLT-4, A549, HL-60 (Cai et al., 2015), and BEL-7420 cell lines (Wang et al., 2008), on P388, HL-60, BEL-7402, and A-549 cell lines (Gao et al., 2013), on the prostate cancer PC3 cell line (Li et al., 2022), as well as on the NCI-H1975 gefitinib resistance (NCI-H1975/GR) cell lines (Wei et al., 2020) were evaluated. An indole diketopiperazine named asperthrins A was reported to possess antifungal and antibacterial activities against Vibrio anguillarum, Xanthomonas oryzae pv. Oryzicola, and Rhizoctonia solani (Yang et al., 2021). Another indole diketopiperazine named penilline D was assayed for its cytotoxic, antibacterial, and enzyme inhibitory activities against acetylcholinesterase (AChE) and pancreatic lipase (PL) but no significant activity was observed (Hu et al., 2021). Other reported activity assessments include anti-inflammatory effects (Wen et al., 2018), NO production inhibitory activity (Liu et al., 2021), radical scavenging activity against DPPH radicals (Zou et al., 2014), plant growth regulation (Zhang et al., 2013), and antifouling activity against the barnacle Balanus reticulatus (Cai et al., 2019). In this study, the inhibition effects of the new indole DKPs on several bacterial strains including the Staphylococcus aureus, and their cytotoxic activity against the gastric cancer cell MKN1, were evaluated. Although a variety of models have been applied to evaluate the bioactivities of indole DKPs, the activities observed were usually not significant. In-depth structure-activity relationship studies of these compounds, as well as structural modifications, may bring new chances for finding new structures with better bioactivities.
The cooccurrence of the echinulin family alkaloids also indicated some clues about their biosynthesis. Recent research heterologously expressed the putative echinulin biosynthetic gene cluster from Aspergillus ruber in Aspergillus nidulans (Nies and Li, 2021). Their work proved that EchPT2 catalyzes the prenylation steps and EchP450 catalyzes the formation of the double bond between C10 and C11. Compound 1 is the prenylated product of compound 5 at C5 whereas compound 7 is the prenylated product of compound 6 at C7. It is possible that these prenylation steps are also catalyzed by EchPT2. It was reported that preechinulin can be converted by the cytochrome P450 enzyme EchP450 to form neoechinulin A with a double bond and it can also be converted by EchPT2 to form prenylated products (Nies and Li, 2021). Here in this work, the obtained compounds 2 and 6 were closely related to a previously reported compound dihydroneochinulin B (Gao et al., 2013). The difference between compound 6 and dihydroneochinulin B lies in the double bond between C8 and C9. Compound 2 can be taken as the bioconversion product of dihydroneochinulin B after two prenylation steps at C5 and C7. It is possible that dihydroneochinulin B can be converted either by EchP450 to form compound 6 or by EchPT2 to produce compound 2.
In the current research, we have isolated two new indole DKPs (1–2) and nine biogenetically related compounds (3–11) from the deep-sea-derived Aspergillus chevalieri strain. Their structures were elucidated by extensive spectroscopic methods, NMR, and ECD calculations. Compounds 1, and 5–7 selectively inhibited the growth of Staphylococcus aureus at the concentration of 250 μM. Meanwhile, compound 8 potently reduced the cell viability of gastric cancer cell MKN1 with an IC50 value of 4.6 μM. The present study demonstrates that the fungal indole DKPs are promising candidates for the discovery of new lead compounds for antimicrobial and anticancer therapy.
The datasets presented in this study can be found in online repositories. The names of the repository/repositories and accession number(s) can be found in the article/Supplementary Material.
DL, JX, XG, JL, and WW: investigation. QL and ZS: resources. JX, BZ, and WW: data analysis. JX, WW, and J-JQ: writing—original draft preparation. ZS, SL, and DZ: writing-revision. All authors have read and agreed to the published version of the manuscript.
This research was supported by the Natural Science Foundation of Fujian Province (2021J01509), the Scientific Research Foundation of Third Institute of Oceanography SOA (2018021), Deep-Sea Habitats Discovery Project (DY-XZ-04), the National Natural Science Foundation of China (41906104), COMRA program (DY135-B2-01 and DY135-B2-05), and the Scientific Research Foundation of Third Institute of Oceanography MNR (2019021).
The authors declare that the research was conducted in the absence of any commercial or financial relationships that could be construed as a potential conflict of interest.
All claims expressed in this article are solely those of the authors and do not necessarily represent those of their affiliated organizations, or those of the publisher, the editors and the reviewers. Any product that may be evaluated in this article, or claim that may be made by its manufacturer, is not guaranteed or endorsed by the publisher.
We are grateful to all the members of Wang Lab for their contribution to this manuscript.
The Supplementary Material for this article can be found online at: https://www.frontiersin.org/articles/10.3389/fmicb.2022.950857/full#supplementary-material
Barbetta, M., Casnati, G., Pochini, A., and Selva, A. (1969). Neoechinuline: a new indole metabolite from aspergillus amstelodami. Tetrahedron Lett. 10, 4457–4460. doi: 10.1016/S0040-4039(01)88723-2
Cai, R., Jiang, H., Xiao, Z., Cao, W., Yan, T., Liu, Z., et al. (2019). (–)- and (+)-asperginulin A, a pair of indole diketopiperazine alkaloid dimers with a 6/5/4/5/6 pentacyclic skeleton from the mangrove endophytic fungus Aspergillus sp. SK-28. Org. Lett. 21, 9633–9636. doi: 10.1021/acs.orglett.9b03796
Cai, S., Sun, S., Peng, J., Kong, X., Zhou, H., Zhu, T., et al. (2015). Okaramines S-U, three new indole diketopiperazine alkaloids from Aspergillus taichungensis ZHN-7-07. Tetrahedron 71, 3715–3719. doi: 10.1016/j.tet.2014.09.019
Cardillo, R., Fuganti, C., Gatti, G., Ghiringhelli, D., and Grasselli, P. (1974). Molecular structure of cryptoechinuline A, a new metabolite of Aspergillus amstelodami, isolated during investigations on echinuline biosynthesis. Tetrahedron Lett. 15, 3163–3166. doi: 10.1016/S0040-4039(01)91850-7
Cardillo, R., Fuganti, C., Ghiringhelli, D., Grasselli, P., and Gatti, G. (1975). Stereochemical course of the α,β-desaturation of L-tryptophan in the biosynthesis of cryptoechinuline A in Aspergillus amstelodami. J. Chem. Soc. Chem. Commun. 4, 778–779. doi: 10.1039/C39750000778
Dossena, A., Marchelli, R., and Pochini, A. (1974). New metabolites of Aspergillus amstelodami related to the biogenesis of neoechinulin. J. Chem. Soc. Chem. Commun. 3, 771–772. doi: 10.1039/c39740000771
Dossena, A., Marchelli, R., and Pochini, A. (1975). Neoechinulin D, a new isoprenylated dehydrotryptophyl metabolite from Aspergillus amstelodami. Experientia 31, 1249.
Frisch, M. J., Trucks, G. W., Schlegel, H. B., Scuseria, G. E., Robb, M. A., Cheeseman, J. R., et al. (2016). Gaussian 16 Rev. C.01. Wallingford, CT.
Gao, H., Zhu, T., Li, D., Gu, Q., and Liu, W. (2013). Prenylated indole diketopiperazine alkaloids from a mangrove rhizosphere soil-derived fungus Aspergillus effuses H1-1. Arch. Pharm. Res. 36, 952–956. doi: 10.1007/s12272-013-0107-5
Gatti, G., Cardillo, R., Fuganti, C., and Ghiringhelli, D. (1976). Structure determination of two extractives from Aspergillus amstelodami by nuclear magnetic resonance spectroscopy. J. Chem. Soc. Chem. Commun. 5, 435–436.
Glisic, S., Veljkovic, N., Stanojevic, M., Gemovic, B., Perovic, V., Radosevic, D., et al. (2015). Natural products as promising therapeutics for treatment of influenza disease. Curr. Pharm. Des. 21, 5573–5588. doi: 10.2174/1381612821666151002113426
Hu, Y.-W., Chen, W.-H., Song, M.-M., Pang, X.-Y., Tian, X.-P., Wang, F.-Z., et al. (2021). Indole diketopiperazine alkaloids and aromatic polyketides from the Antarctic fungus Penicillium sp. SCSIO 05705. Nat. Prod. Res. doi: 10.1080/14786419.2021.1973460 [Epub ahead of print].
Jia, B., Ma, Y., Chen, D., Chen, P., and Hu, Y. (2018). Studies on structure and biological activity of indole diketopiperazine alkaloids. Prog. Chem. 30, 1067–1081. doi: 10.7536/PC171231
Lhamo, S., Wang, X.-B., Li, T.-X., Wang, Y., Li, Z.-R., Shi, Y.-M., et al. (2015). Three unusual indole diketopiperazine alkaloids from a terrestrial-derived endophytic fungus, Aspergillus sp. Tetrahedron Lett. 56, 2823–2826. doi: 10.1016/j.tetlet.2015.04.058
Li, X., Xu, J., Wang, P., and Ding, W. (2022). Novel indole diketopiperazine stereoisomers from a marine-derived fungus Aspergillus sp. Mycology-Intern. J. Fung. Bio. doi: 10.1080/21501203.2022.2069173 [Epub ahead of print].
Lin, L.-B., Gao, Y.-Q., Han, R., Xiao, J., Wang, Y.-M., Zhang, Q., et al. (2021). Alkylated salicylaldehydes and prenylated indole alkaloids from the endolichenic fungus Aspergillus chevalieri and their bioactivities. J. Agric. Food Chem. 69, 6524–6534. doi: 10.1021/acs.jafc.1c01148
Liu, Z., Chen, Y., Li, S., Hu, C., Liu, H., and Zhang, W. (2021). Indole diketopiperazine alkaloids from the deep-sea-derived fungus Aspergillus sp. FS445. Nat. Prod. Res. doi: 10.1080/14786419.2021.1925271 [Epub ahead of print].
Ma, Y. M., Liang, X. A., Kong, Y., and Jia, B. (2016). Structural diversity and biological activities of indole diketopiperazine alkaloids from fungi. J. Agric. Food Chem. 64, 6659–6671. doi: 10.1021/acs.jafc.6b01772
Mostafa, A. A., Dennis, A., Ahmed, A.-R. S., Ioanna, C., Nikolas, F., Mohamed, S., et al. (2021). New indole diketopiperazine alkaloids from a soil-derived fungus Aspergillus ochraceopetaliformis. Planta Med. 87, 1316–1317. doi: 10.1055/s-0041-1736992
Nies, J., and Li, S. M. (2021). Prenylation and dehydrogenation of a C2-reversely prenylated diketopiperazine as a branching point in the biosynthesis of echinulin family alkaloids in Aspergillus ruber. ACS Chem. Biol. 16, 185–192. doi: 10.1021/acschembio.0c00874
Ningsih, B. N. S., Rukachaisirikul, V., Phongpaichit, S., Preedanon, S., Sakayaroj, J., and Muanprasat, C. (2022). A nonadride derivative from the marine-derived fungus Aspergillus chevalieri PSU-AMF79. Nat. Prod. Res. doi: 10.1080/14786419.2022.2039651 [Epub ahead of print].
Pracht, P., Bohle, F., and Grimme, S. (2020). Automated exploration of the low-energy chemical space with fast quantum chemical methods. Phys. Chem. Chem. Phys. 22, 7169–7192. doi: 10.1039/c9cp06869d
Wang, F., Fang, Y., Zhu, T., Zhang, M., Lin, A., Gu, Q., et al. (2008). Seven new prenylated indole diketopiperazine alkaloids from holothurian-derived fungus Aspergillus fumigatus. Tetrahedron 64, 7986–7991. doi: 10.1016/j.tet.2008.06.013
Wang, W., Chen, R., Luo, Z., Wang, W., and Chen, J. (2018a). Antimicrobial activity and molecular docking studies of a novel anthraquinone from a marine-derived fungus Aspergillus versicolor. Nat. Prod. Res. 32, 558–563. doi: 10.1080/14786419.2017.1329732
Wang, W., Cheng, J. W., Qin, J. J., Hu, B., Li, X., Nijampatnam, B., et al. (2019a). MDM2-NFAT1 dual inhibitor, MA242: effective against hepatocellular carcinoma, independent of p53. Cancer Lett. 459, 156–167. doi: 10.1016/j.canlet.2019.114429
Wang, W., Liao, Y., Chen, R., Hou, Y., Ke, W., Zhang, B., et al. (2018b). Chlorinated azaphilone pigments with antimicrobial and cytotoxic activities isolated from the deep sea derived fungus Chaetomium sp. NA-S01-R1. Mar. Drugs 16:61. doi: 10.3390/md16020061
Wang, W., Liao, Y., Zhang, B., Gao, M., Ke, W., Li, F., et al. (2019b). Citrinin monomer and dimer derivatives with antibacterial and cytotoxic activities isolated from the deep sea-derived fungus Penicillium citrinum NLG-S01-P1. Mar. Drugs 17:46. doi: 10.3390/md17010046
Wang, W., Qin, J. J., Voruganti, S., Nijampatnam, B., Velu, S. E., Ruan, K. H., et al. (2018c). Discovery and characterization of dual inhibitors of MDM2 and NFAT1 for pancreatic cancer therapy. Cancer Res. 78, 5656–5667. doi: 10.1158/0008-5472.CAN-17-3939
Wang, W., Qin, J. J., Voruganti, S., Wang, M. H., Sharma, H., Patil, S., et al. (2014). Identification of a new class of MDM2 inhibitor that inhibits growth of orthotopic pancreatic tumors in mice. Gastroenterol. 147, 893–902.e2. doi: 10.1053/j.gastro.2014.07.001
Wang, W., Yang, J., Liao, Y. Y., Cheng, G., Chen, J., Cheng, X. D., et al. (2020a). Cytotoxic nitrogenated azaphilones from the deep-sea-derived fungus Chaetomium globosum MP4-S01-7. J. Nat. Prod. 83, 1157–1166. doi: 10.1021/acs.jnatprod.9b01165
Wang, W., Yang, J., Liao, Y. Y., Cheng, G., Chen, J., Mo, S., et al. (2020b). Aspeterreurone A, a cytotoxic dihydrobenzofuran-phenyl acrylate hybrid from the deep-sea-derived fungus Aspergillus terreus CC-S06-18. J. Nat. Prod. 83, 1998–2003. doi: 10.1021/acs.jnatprod.0c00189
Wang, W.-L., Lu, Z.-Y., Tao, H.-W., Zhu, T.-J., Fang, Y.-C., Gu, Q.-Q., et al. (2007). Isoechinulin-type alkaloids, variecolorins A-L, from halotolerant Aspergillus variecolor. J. Nat. Prod. 70, 1558–1564. doi: 10.1021/np070208z
Wei, X., Feng, C., Wang, S.-Y., Zhang, D.-M., Li, X.-H., and Zhang, C.-X. (2020). New indole diketopiperazine alkaloids from soft coral-associated epiphytic fungus Aspergillus sp. EGF 15-0-3. Chem. Biodivers. 17:e2000106. doi: 10.1002/cbdv.202000106
Wen, H., Liu, X., Zhang, Q., Deng, Y., Zang, Y., Wang, J., et al. (2018). Three new indole diketopiperazine alkaloids from Aspergillus ochraceus. Chem. Biodivers. 15:e1700550. doi: 10.1002/cbdv.201700550
Willoughby, P. H., Jansma, M. J., and Hoye, T. R. (2014). A guide to small-molecule structure assignment through computation of ((1)H and (1)(3)C) NMR chemical shifts. Nat. Protoc. 9, 643–660. doi: 10.1038/nprot.2014.042
Yan, L.-H., Li, P.-H., Li, X.-M., Yang, S.-Q., Liu, K.-C., Wang, B.-G., et al. (2022). Chevalinulins A and B, proangiogenic alkaloids with a spiro[bicyclo[2.2.2]octane-diketopiperazine] skeleton from deep-sea cold-seep-derived fungus Aspergillus chevalieri CS-122. Org. Lett. 24, 2684–2688. doi: 10.1021/acs.orglett.2c00781
Yang, J., Gong, L., Guo, M., Jiang, Y., Ding, Y., Wang, Z., et al. (2021). Bioactive indole diketopiperazine alkaloids from the marine endophytic fungus Aspergillus sp. YJ191021. Mar. Drugs 19:157. doi: 10.3390/md19030157
Zhang, Q., Wang, S.-Q., Tang, H.-Y., Li, X.-J., Zhang, L., Xiao, J., et al. (2013). Potential allelopathic indole diketopiperazines produced by the plant endophytic aspergillus fumigatus using OSMAC method. J. Agric. Food Chem. 61, 11447–11452. doi: 10.1021/jf403200g
Keywords: Aspergillus, cytotoxic, antibacterial, NMR, indole diketopiperazine
Citation: Lv D, Xia J, Guan X, Lai Q, Zhang B, Lin J, Shao Z, Luo S, Zhangsun D, Qin J-J and Wang W (2022) Indole Diketopiperazine Alkaloids Isolated From the Marine-Derived Fungus Aspergillus chevalieri MCCC M23426. Front. Microbiol. 13:950857. doi: 10.3389/fmicb.2022.950857
Received: 23 May 2022; Accepted: 08 June 2022;
Published: 07 July 2022.
Edited by:
Shan He, Ningbo University, ChinaReviewed by:
Haifeng Wang, Shenyang Pharmaceutical University, ChinaCopyright © 2022 Lv, Xia, Guan, Lai, Zhang, Lin, Shao, Luo, Zhangsun, Qin and Wang. This is an open-access article distributed under the terms of the Creative Commons Attribution License (CC BY). The use, distribution or reproduction in other forums is permitted, provided the original author(s) and the copyright owner(s) are credited and that the original publication in this journal is cited, in accordance with accepted academic practice. No use, distribution or reproduction is permitted which does not comply with these terms.
*Correspondence: Dongting Zhangsun, emhhbmdzdW5kdEAxNjMuY29t; Jiang-Jiang Qin, anFpbkB1Y2FzLmFjLmNu; Weiyi Wang, d3l3YW5nQHRpby5vcmcuY24=
†These authors have contributed equally to this work
Disclaimer: All claims expressed in this article are solely those of the authors and do not necessarily represent those of their affiliated organizations, or those of the publisher, the editors and the reviewers. Any product that may be evaluated in this article or claim that may be made by its manufacturer is not guaranteed or endorsed by the publisher.
Research integrity at Frontiers
Learn more about the work of our research integrity team to safeguard the quality of each article we publish.