- 1ICMR-Vector Control Research Centre, Medical Complex, Puducherry, India
- 2Indian Council of Medical Research, Ramalingaswami Bhawan, New Delhi, India
Wolbachia, an intracellular maternally transmitted endosymbiont, has been shown to interfere with the replication of dengue virus in Aedes aegypti mosquitoes. The Wolbachia-transinfected Ae. aegypti has been currently released in many countries to test its effectiveness in preventing the transmission of dengue virus. ICMR-Vector Control Research Centre in collaboration with World Mosquito Program Monash University, Australia, has generated two new Wolbachia-introgressed Ae. aegypti Puducherry (Pud) lines via backcrossing Ae. aegypti females of Australian (Aus) strains, infected with wMel and wAlbB Wolbachia with wild-type Ae. aegypti Puducherry (Pud) males. Wolbachia infections are known to induce a fitness cost and confer benefit on the host mosquito populations that will influence spread of the Wolbachia into native wild mosquito populations during the field release. Hence, the induced fitness cost or benefit/advantage in the two newly generated Ae. aegypti (Pud) lines was assessed in the laboratory in comparison with the wild-type Ae. aegypti (Pud) strain. In addition, maternal transmission (MT) efficiency, induced cytoplasmic incompatibility (CI), and insecticide resistance status of the two (Pud) lines were determined to assess the likely frequency of wMel and wAlbB infections in the native wild population after field invasion. The study shows that wMel and wAlbB infections did not induce any fitness cost on the two newly generated (Pud) lines. Rather, in terms of wing length, fecundity, egg hatch rate, and adult survival, the Wolbachia introgression conferred fitness benefits on the (Pud) lines compared to uninfected Wolbachia free wild Ae. aegypti population. wMel and wAlbB exhibited a high maternal transmission (99–100%) and induced nearly complete (98–100%) cytoplasmic incompatibility. Both the (Pud) lines were resistant to deltamethrin, malathion, DDT, and temephos, and the level of resistance was almost the same between the two lines as in the wild type. Overall, the stable association of wMel and wAlbB established with Ae. aegypti and the reproductive advantages of the (Pud) lines encourage a pilot release in the field for population replacement potential.
Introduction
Dengue is the fastest spreading arboviral infection of humans accounting for a considerable disease burden across the tropics. It is estimated that approximately 390 million dengue infections and 96 million cases occur worldwide annually (World Health Organization [WHO], 2022). In India, it is endemic in 28 States and six union territories and is the leading cause of febrile illness (Ganeshkumar et al., 2018). A total of 913,817 cases of dengue and 1,490 deaths were reported in the country during 2015–2021 (National Vector Borne Disease Control Programme [NVBDCP], 2022). Dengue virus (DENV) is transmitted by infective bite primarily of Aedes aegypti females and secondarily of Aedes albopictus. No specific drugs are currently available to treat the dengue patients. The safety concerns with the currently available vaccines prevented their use for prophylaxis in the public health program (Wilder-Smith et al., 2010; Deng et al., 2020). Control of the vector mosquito is thus the only option to prevent and interrupt the transmission of dengue virus. Use of chemical larvicides, biological control agents, thermal fogging, and source reduction activities are the ongoing practical measures of vector control today, but these measures have yielded only a limited success in reducing dengue cases not only in India (Jain and Sharma, 2017), but also in other countries (Kay and Vu, 2005; Garcia et al., 2019). In addition, these interventions require repeated applications and are expensive and difficult to carry out in urban areas (Iturbe-Ormaetxe et al., 2011). This has necessitated the testing of alternative methods to achieve effective control of dengue in India.
One such method is the use of Wolbachia-based strategy to prevent the transmission of dengue and other arboviral infections. Wolbachia is an endosymbiotic intracellular gram-negative bacterium that is naturally prevalent in about 51% (CI: 48–57) of insect species (Weinert et al., 2015) and 39.5% of the 147 mosquito species screened (Bourtzis et al., 2014). Wolbachia pipientis (wPip) is the strain first isolated from the mosquito species Culex pipiens (Hertig and Wolbach, 1924). Other mosquito species known to be naturally infected with Wolbachia are Culex quinquefasciatus (Dumas et al., 2013), Aedes fluviatilis (Moreira et al., 2009), and Ae. albopictus (Sinkins et al., 1995). Recent studies have reported the presence of Wolbachia in Ae. aegypti with a low frequency and density (Teo et al., 2017; Bennett et al., 2019; Carvajal et al., 2019; Kulkarni et al., 2019), but the evidence is not compelling (Ross et al., 2020).
Wolbachia alters reproductive fitness of arthropod vectors through selective male killing, parthenogenesis, feminization of male embryos, and cytoplasmic incompatibility (CI) (Werren et al., 2008). Of these, CI is the prominent and is the only phenotype found in mosquitoes (Sinkins, 2004). It also alters the vector competence of transinfected arthropod vectors for the transmission of arboviruses through competition for resources, such as cholesterol (Hedges et al., 2008; Glaser and Meola, 2010), pre-activation of the immune system (immune-priming), induction of the phenol-oxidase cascade, and stimulation of microRNA-dependent immune pathways, that are essential for host defense against viruses (Sim et al., 2014; Johnson, 2015).
Some Wolbachia strains have many traits that induce fitness costs on their host mosquito populations (Allman et al., 2020) and that will affect its spread among the native wild population during field release. The most pathogenic Wolbachia strain wMelPop generates large deleterious effects on adult longevity, egg viability, and reproductive potential (McMeniman and O’Neill, 2010; Almeida et al., 2011; Caragata et al., 2016). However, these deleterious effects are less pronounced or absent with wMel and wAlbB strains of Wolbachia (Axford et al., 2016).
Transinfection of Ae. aegypti with Wolbachia strains, wMelPop, wMel, and wAlbB, has initially been shown to significantly reduce its vector competence, particularly to dengue virus under laboratory conditions (Moreira et al., 2009; Frentiu et al., 2010; Ant et al., 2018). In small-scale field releases of transinfected Ae. aegypti, Wolbachia was found to spread in high frequencies among the native Ae. aegypti populations (O’Neill et al., 2018; Indriani et al., 2020; Ryan et al., 2020) and suppress replication of dengue virus in mosquitoes (Ferguson et al., 2015). Wolbachia-infected Ae. aegypti mosquitoes can be deployed for either population replacement or population suppression. Both approaches rely on CI induced by Wolbachia. The population replacement approach involves the release of both male and female Wolbachia-infected mosquitoes that reduce virus transmission in Ae. aegypti populations. Population suppression approach involves the release of only males that cannot produce viable offspring when they mate with wild females.
Field trials have been carried out releasing Wolbachia-infected males in Singapore (National Environmental Agency [NEA], 2022), California (Crawford et al., 2020), Australia (Beebe et al., 2021), and Puerto Rico (Martín-Park et al., 2022) for population suppression. Currently, the field release of wMel-infected Ae. aegypti for population replacement is now underway in 11 countries to evaluate its effectiveness in controlling dengue (World Mosquito Program [WMP], 2022). This strain has successfully been established in Australia (O’Neill et al., 2018; Ryan et al., 2020), Brazil (Pinto et al., 2021), Indonesia (Utarini et al., 2021), and Vietnam (Hien et al., 2022), while wAlbB was successfully established among wild mosquito population in Malaysia for population replacement (Nazni et al., 2019). In a recent randomized control trial in Yogyakarta city, Indonesia, field releases of wMel-infected Ae. aegypti mosquitoes significantly reduced the virologically confirmed dengue cases by 77.1% in the intervention clusters compared to the control clusters (Utarini et al., 2021). In other city-wide field trials, 76% reduction in dengue cases was observed in Indonesia (Indriani et al., 2020), more than 70% reduction in Brazil (Pinto et al., 2021), 86% reduction in Vietnam (Burki, 2020), and 96% reduction in Australia (O’Neill et al., 2018; Ryan et al., 2020).
Indian Council of Medical Research-Vector Control Research Centre (ICMR-VCRC), Puducherry, India, in collaboration with the World Mosquito Program (WMP) [formerly known as Eliminate Dengue Program (EDP)] from Monash University, Australia, has generated two new Wolbachia-introgressed Ae. aegypti Puducherry (Pud) lines that carry wMel and wAlbB Wolbachia strains via backcrossing wMel and wAlbB Ae. aegypti Australia (Aus) females with Ae. aegypti Puducherry (Pud) wild males over six generations. The two Indian Ae. aegypti (Pud) lines infected with wMel or wAlbB Wolbachia strains were developed with an aim of testing them, in future, in the field to select the suitable strain for population replacement for Indian conditions.
Before launching a field release of Wolbachia-introgressed Ae. aegypti, the critical issue that needs to be considered is the fitness of mosquitoes since these mosquitoes must compete effectively with the Ae. aegypti wild population to facilitate the efficient invasion of Wolbachia into the wild mosquito population through near-complete maternal transmission (MT) coupled with strong CI, but without producing any fitness cost (Fraser et al., 2017). Apart from these factors, male mating competitiveness and vector competence are other important factors which affect the successful establishment of the inherited Wolbachia infections in the wild population. In this study, the fitness of the two newly generated Ae. aegypti (Pud) lines infected with wMel and wAlbB infections was assessed in comparison with that of the wild-type Ae. aegypti (Pud) line in terms of life-history traits, such as wing length, fecundity, egg hatch rate, and adult survival. In addition, MT efficiency, induced CI, and insecticide resistance status of the (Pud) lines were determined.
Materials and methods
Mosquito strains and colony maintenance
Mosquito strains
The eggs of the two Ae. aegypti Australian (Aus) lines, one infected by embryonic microinjection with wMel Wolbachia isolated from Drosophila melanogaster (Walker et al., 2011) and the other infected by microinjection with wAlbB Wolbachia infection from Ae. albopictus (Xi et al., 2005) imported from the World Mosquito Program (WMP), Australia, were used to raise colonies of the two (Pud) lines. Eggs of wild-type Ae. aegypti (Pud) line were collected using ovitraps from different sites in Puducherry, reared to adults, fed with human blood, and allowed for oviposition. The F1 eggs were reared to adults, which were identified and confirmed to be Ae. aegypti (Barraud, 1934). The F1 generation adults were used for backcrossing with the Wolbachia-infected Ae. aegypti (Aus) lines and other laboratory studies on fitness characteristics, MT, CI, sensitivity to heat stress, male mating competitiveness, and population replacement. Prior to the experiments, the wild Ae. aegypti (Pud) were screened by PCR assays to ensure that they are free from natural Wolbachia infection (Noda et al., 1997).
Generation of Wolbachia-infected Indian strains
Backcrossing was done in three replicates. Into each replicate cage containing 250 wMel or wAlbB Ae. aegypti (Aus) females, 250 wild-type Ae. aegypti (Pud) males were released for mating [Backcross I (BC 1)]. Five days after mating, the females were fed with human blood and allowed to oviposit, and the eggs were collected and stored. One-week-old eggs obtained from backcross I (BC I) were hatched replicate wise and reared to adults. The adult female progeny from BC I were backcrossed with wild-type F1 males (BC II). In total, for each Wolbachia strain, six backcrossing were done. After the sixth backcrossing, the resultant Ae. aegypti colonies were designated as the wMel Ae. aegypti (Pud) and wAlbB Ae. aegypti (Pud) lines. At every generation, females of the (Pud) lines were outcrossed (250 females and 225 males of respective line) with 10% (25) wild-type (Pud) males to minimize the selection pressure due to continuous rearing under laboratory conditions over many generations.
Colony maintenance
wMel- and wAlbB-infected Ae. aegypti (Aus) lines, w Mel-, and wAlbB-infected Ae. aegypti (Pud) lines and uninfected Ae. aegypti (Pud) line were reared in the insectary at 27 ± 2°C temperature and 80 ± 10% relative humidity with a photoperiod of 12L and 12D. Colonies of 500 adults (1:1 sex ratio) were maintained in BugDorm cages (W30 x D30 x H30 cm) (BugDorm Stores, Australia). To provide nutrition and hydration, the adult mosquitoes were provided with 10% sucrose solution kept in sugar cups. Five-day-old females were fed with human blood obtained from blood bank (Pondicherry AIDS Control Society, Government of Puducherry, India) through artificial membrane feeding system following the SOP on blood feeding (World Mosquito Program [WMP], 2018a). Three days after blood feeding, oviposition cups (plastic polycarbonate cups, 200 ml capacity) lined inside with filter paper and half-filled tap water were placed in the adult mosquito cages for 2 days. On the third day, the egg papers were removed from the oviposition cups and allowed to dry at room temperature (27°C ± 2°C; 80 ± 10% RH) for 2 days. Once the egg papers were dried, they were transferred to plastic ziplock bags and stored in a sealed container at 27°C ± 2°C and 80% RH using saturated KCL solution (Ross et al., 2017; World Mosquito Program [WMP], 2017). The tap water supplied by the municipality was used for both egg hatching and larval rearing. The quality of the tap water was checked periodically (every 6 months) by subjecting it to physical, chemical, and bacteriological analysis (Water Testing Laboratory, Level II + Category, Government of Puducherry). Synchronous hatching of eggs was done using cooled boiled (deoxygenated) water containing brewer’s yeast (0.2 g/l) in an airtight container (Judson, 1960; Imam et al., 2014; World Mosquito Program [WMP], 2017). One day after hatching, approximately 150 first instar larvae were transferred to enamel trays (45 cm L × 30 cm W × 5 cm H) containing 3 L of tap water (one larva/20 ml). The larvae were fed with fish food (TetraMin Tropical Tablets) at the rate of 2.0 mg/larva. Five days later, pupae were collected; male and female pupae were separated manually and transferred to separate containers (500 ml capacity) that were kept inside one-cubic foot BugDorm cages for adult emergence.
Wing length
Mosquito wing size is used to estimate adult body size (Joshi et al., 2014), and body size is considered as an indicator of fitness characteristics of mosquitoes (Xue et al., 2010). Accordingly, the wing size was measured as a part of assessing the fitness of the colonized wMel Ae. aegypti (Pud) and wAlbB Ae. aegypti (Pud) lines. One-day-old 25 unfed males and 25 unfed females of each of the Ae. aegypti (Pud) lines from first outcross generation (OCG1) and wild-type Ae. aegypti (Pud) line (F1) were killed by freezing at −20°C for 10 min. Subsequently, the right wing of each mosquito was removed, placed on a microslide, and covered with a cover glass. Wings were freed from scales by carefully sliding the cover glass over the microslide. The length from the axillary incision (Alular notch) to the wing tip was measured using a stereomicroscope (Olympus SZ61) attached with a digital camera (Olympus DP22) and measurement software (Cell Sens Entry 1.13).
Fecundity and egg hatch rate
Fecundity and egg hatch rate are the two major reproductive biological characteristics, often used to assess the fitness of colonized mosquito populations. Fifty wMel Ae. aegypti (Pud) females (OCG1) were crossed with 50 wMel Ae. aegypti (Pud) males (OCG1) in six replicates. Similarly, replicate cages were set up with wAlbB Ae. aegypti (Pud) (OCG1) line and also wMel Ae. aegypti (Aus) (F12) and wAlbB Ae. aegypti (Aus) (F12) lines. For comparison, 50 Ae. aegypti (Pud) wild females (F1) were crossed with 50 wild males (F1) in six replicates. In each replicate, 5-day-old females were fed and the blood-fed mosquitoes were allowed to oviposit. The mortality of females was scored daily until oviposition. The number of eggs laid in each replicate was counted using stereomicroscope, and from this count, the average number of eggs laid by a single female was estimated. Eggs obtained from each replicate (n = 1,000) were hatched (vide Colony maintenance), and the number of first instar larvae hatched in each replicate was counted to determine the hatch rate.
Adult survival
Adult survival of wMel and wAlbB Ae. aegypti (Pud) lines (OCG9), the wMel, and wAlbB Ae. aegypti (Aus) parental lines (F19 generation), and the wild-type Ae. aegypti (Pud) line (F1 generation) was estimated, simultaneously using batches of 100 adult mosquitoes (1:1 sex ratio), replicated six times. One-week-old eggs of these lines were hatched, and the larvae were reared to pupae (vide Colony maintenance). Male and female pupae were separated. In each replicate, 50 male and 50 female pupae were kept in enamel bowls (300-ml capacity) containing 200 mL of tap water and placed in BugDorm cages (W30 × D30 × H30 cm) for emergence. Adults were provided with 10% sucrose solution. Females were fed weekly on human blood for the entire duration of the experiment. Each time, the same batch of human blood was used for feeding all lines and in all replicates. Three days after each blood feeding, a 300-ml cup containing 150 ml of tap water lined with a filter paper was kept inside the cage for oviposition. The adults were maintained in the insectary at 27 ± 2°C temperature and 80 ± 10% relative humidity. Mortality was scored for males and females daily until all adult mosquitoes had died.
Maternal transmission
Wolbachia is transferred maternally from an infected female mosquito to her progeny. Maternal transmission is one of the key parameters that influence Wolbachia functions in the population replacement process (Hoffmann et al., 1990). Fifty each of wMel and wAlbB Ae. aegypti (Pud) females were allowed separately to mate with 50 Ae. aegypti (Pud) wild males in three replicates to confirm the maternal transmission of Wolbachia to their progeny. For comparison, the wMel and wAlbB Ae. aegypti (Aus) females were allowed separately to mate with the wild-type Ae. aegypti (Pud) males. In each replicate, 5-day-old females were fed with the same source of human blood and allowed to oviposit, and the parental adults were screened for Wolbachia frequency by real time-PCR assays. In each replicate, eggs (progeny) were reared to adults and 160 females from each replicate were screened for the presence of Wolbachia. DNA from individual mosquito was extracted by homogenizing in squash buffer. The homogenate was briefly centrifuged, and the supernatant containing DNA was used for the Wolbachia diagnosis following the Diagnostics SOP (World Mosquito Program [WMP], 2018b). wMel was screened using primers and probes specifically targeting the Wolbachia surface protein (WSP) gene (WspTM2_FW: 5′-CATTGGTGTTGGTGTTGGTG-3′; WspTM2_RV: 5′-ACA CCAGCTTTTACTTGACCAG-3′; WspTM_Probe: 5′- LC640- TCCTTTGGAACCCGCTGTGA ATGA-lowaBlack-3′), and wAlbB was identified using primers and probes directed to wAlbB specific Ankyrin repeat domain gene (wAlbB_16009_ FW: 5′-AGTAGTGCAGCGAGTCT-3′; wAlbB 16009_RV: 5′-TGGAGGAAGAGTTCACTGTGC-3′; wAlbB 16009_Probe: 5′-FAM-ZEN-AATTATCCCCTACCA AAGCAATTAAGATAGAAT-IowaBlack-3′). Gene encoding ribosomal protein (RPS17) of Ae. aegypti was used as internal positive control (Rps17_FW: 5′-TCCGTGGTATC TCCATCAAGCT-3′; Rps17_RV: 5′-CACTTCCGGCACGTA GTTGTC-3′; Rps17_TaqM_Probe: 5′-HEX-CAGGAGGAGGA ACGTGAGCGCAG-BHQ1-3′). The frequency of Wolbachia was calculated as the percentage of positives among the total number of mosquitoes tested. The experiment was repeated three times with successive outcrossed generations (OCG1, OCG2, and OCG3).
Cytoplasmic incompatibility
Wolbachia-induced cytoplasmic incompatibility is the most commonly occurring reproductive manipulation phenotype that leads to the production of sterile offspring. The crosses between uninfected wild (Pud) females and wMel-infected Ae. aegypti (Pud) males were set up in three replicates. Similarly, the wild-type (Pud) females were allowed to mate with the wAlbB-infected Ae. aegypti (Pud) males. The experiments were repeated three times with successive outcrossed generations (OCG1, OCG2, and OCG3). The eggs collected from the CI crosses were floated for hatching, and the hatch rates were estimated to determine the cytoplasmic incompatibility.
Insecticide resistance
In areas reported with dengue infection, insecticides are used to reduce the vector (Ae. aegypti) population. For considering the field release of Wolbachia-infected Ae. aegypti (Pud) lines, it is essential that the (Pud) lines match with the wild population of Ae. aegypti in terms of insecticide susceptibility/resistance status. Therefore, the insecticide susceptibility/resistance status of newly generated wMel and wAlbB Ae. aegypti (Pud) lines was determined through WHO tube assays using impregnated papers at the discriminating concentration of deltamethrin 0.03%, malathion 0.08%, and DDT 4%, the insecticides commonly used in the public health program, in comparison with wild Ae. aegypti (Pud) line. The tube assays were done as per the WHO guidelines (World Health Organization [WHO], 2016). The assays were replicated three times with different batches of mosquitoes. Furthermore, intensity assays were carried out at 5X concentrations (5 times higher than the diagnostic concentration) of deltamethrin (0.15%) and malathion (4%) (World Health Organization [WHO], 2016). Susceptibility/resistance status of the larvae of Ae. aegypti (Pud) lines and of the wild Ae. aegypti to temephos, an organophosphorus larvicide used in the control program, was also determined at the diagnostic concentration (0.02 ppm) following the WHO guidelines (World Health Organization [WHO], 2005).
Data analysis
Data on wing length, fecundity, and egg hatch (fertility) rates were expressed as mean ± SE. The difference in the wing length between the lines was analyzed using one-way ANOVA as the data on wing length followed normal distribution, and the Bonferroni test was used for pair-wise comparison. The data on fecundity were analyzed using negative binomial regression (variance greater than mean), and the fertility was analyzed using Poisson regression (variance equal to mean) to find out the differences between the strains. Kaplan–Meier survival curve plots were made separately for males and females of each line. The mean duration of 50% adult survival between the lines was analyzed using the log-rank test. Cox-proportional hazard regression model was used to find out the death-risk rate between the lines separately for males and females, and the hazard ratio (HR) with 95% confidence interval was reported. The dose–response data from insecticide susceptibility bioassays were analyzed using probit regression analysis to determine LC50 and LC99.9 values. P-value < 0.05 was considered as statistically significant. The statistical software STATA 14.2 (Texas, United States) and SPSS 16.0 were used for data analysis.
Results
Wing length
The mean wing length of wMel Ae. aegypti (Pud) females (n = 25) was 3.03 ± 0.02 mm and that of the males (n = 25) was 2.34 ± 0.01 mm. The mean wing length of wAlbB (Pud) females and males was 3.07 ± 0.01 mm and 2.35 ± 0.01 mm, respectively, and those of wild (Pud) females and males were 2.96 ± 0.02 mm and 2.30 ± 0.01 mm, respectively. Analysis of variance showed that the wing length of males [F = 5.65, df (2.72), p = 0.005] and females [F = 14.26, df (2.72), p < 0.001] varied significantly between the lines. Pair-wise comparisons showed that the wing length of females of both the introgressed Ae. aegypti (Pud) lines was significantly greater than that of wild Ae. aegypti (Pud) females (wMel (Pud) female: p = 0.004; wAlbB (Pud) female: p < 0.001). In case of males, wAlbB (Pud) line showed greater wing length than that of wild Ae. aegypti (Pud) (p = 0.005) line. Wing length in males (p = 0.836) and in females (p = 0.176) did not differ significantly between the two Ae. aegypti (Pud) lines.
Fecundity and egg hatch rate
The fecundity (average number of eggs laid by a female) of wAlbB Ae. aegypti (Pud) line was significantly [Incidence rate (IR) = 1.23, p < 0.001] greater than those of the wild-type Ae. aegypti (Pud) and wMel Ae. aegypti (Pud) lines (Figure 1). When compared to wild Ae. aegypti (Pud), the fecundity of wMel Ae. aegypti (Pud), wMel Ae. aegypti (Aus), and wAlbB Ae. aegypti (Aus) did not differ significantly (p > 0.05) (Figure 1).
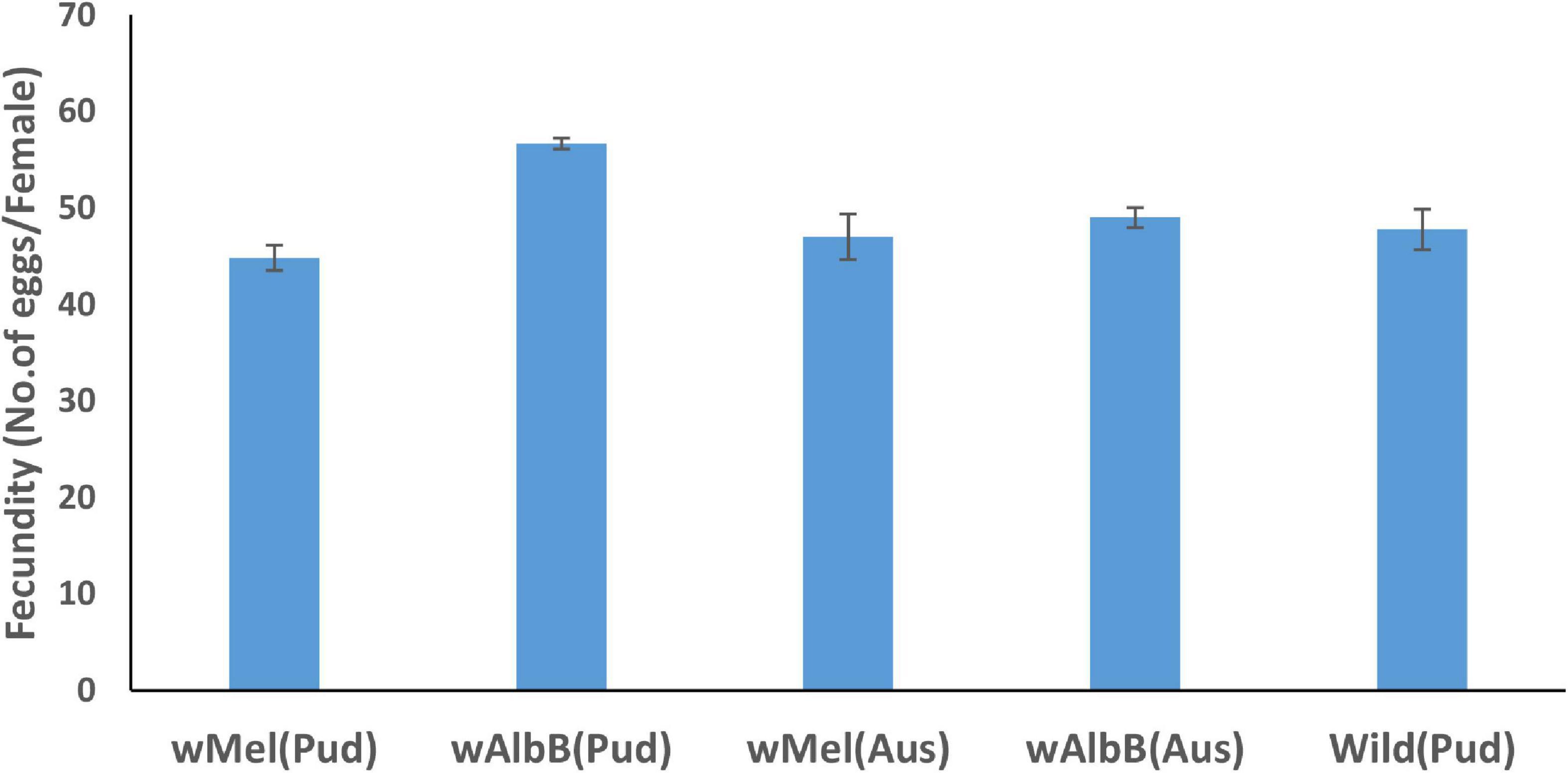
Figure 1. Fecundity (average number of eggs per female ± SE) of Wolbachia-infected Ae. aegypti females and uninfected wild Ae. aegypti (Pud) females.
The egg hatch rate of wAlbB (Aus) line was significantly (IR = 1.04, p = 0.035) greater than that of wild-type (Pud) line (Figure 2). The egg hatch rates of wMel (Pud) (IR = 1.03, p = 0.08), wAlbB (Pud) (IR = 1.03, p = 0.068), and wMel (Aus) (IR = 0.97, p = 0.136) lines did not differ significantly when compared to the wild-type (Pud) line (Figure 2).
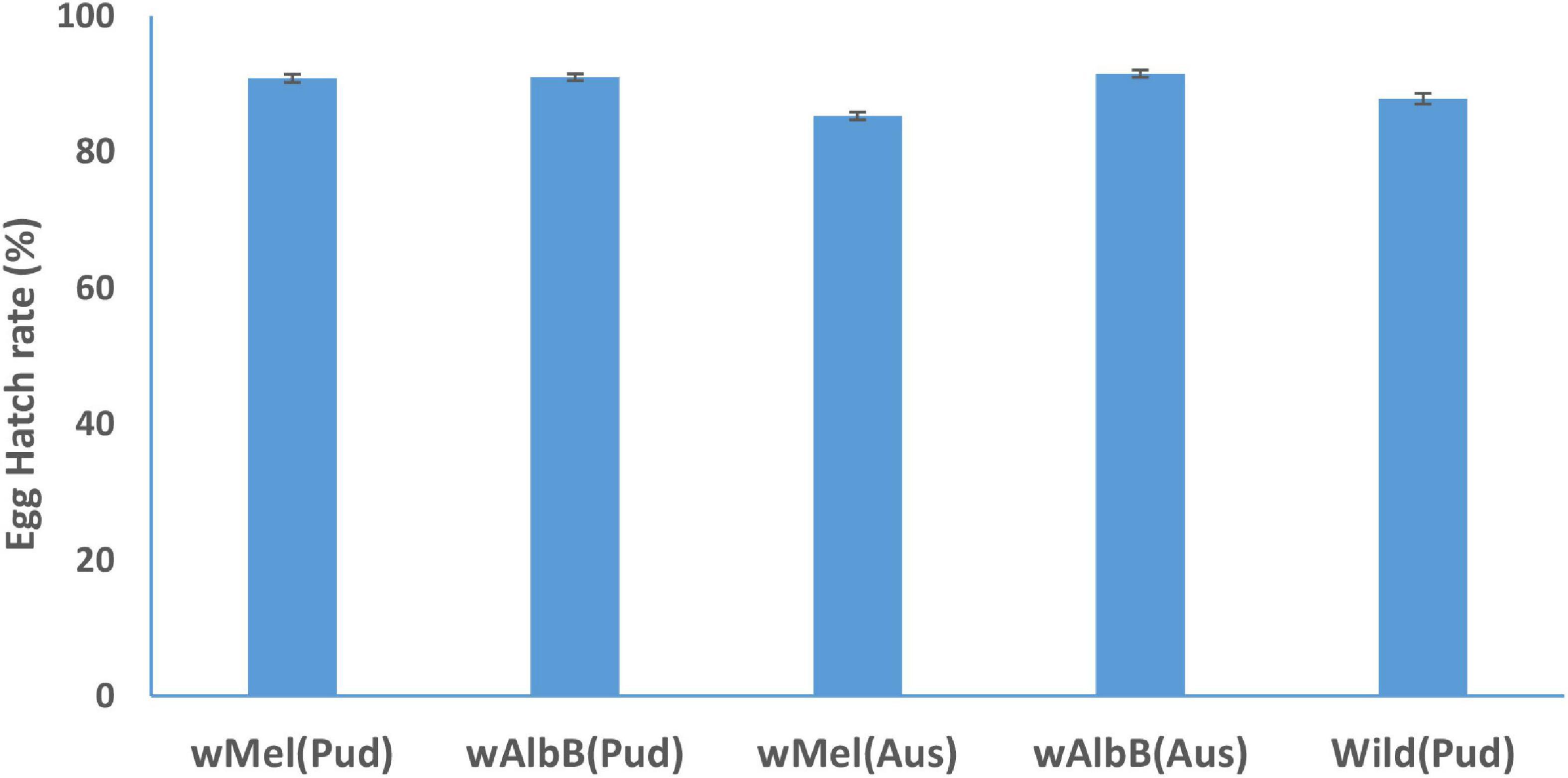
Figure 2. Hatch rate (± SE) of eggs of Wolbachia-infected Ae. aegypti females and uninfected wild Ae. aegypti (Pud) females.
Adult survival
The effects of wMel and wAlbB infections on the survival of Ae. aegypti (Pud) lines over time were examined. In wMel Ae. aegypti (Pud) line, 50% of males survived up to 25 days and females up to 35 days (Figures 3A,B), whereas in wMel (Aus) line, 50% of males and 50% of females survived up to 21 and 29 days, respectively (Figures 3A,B).
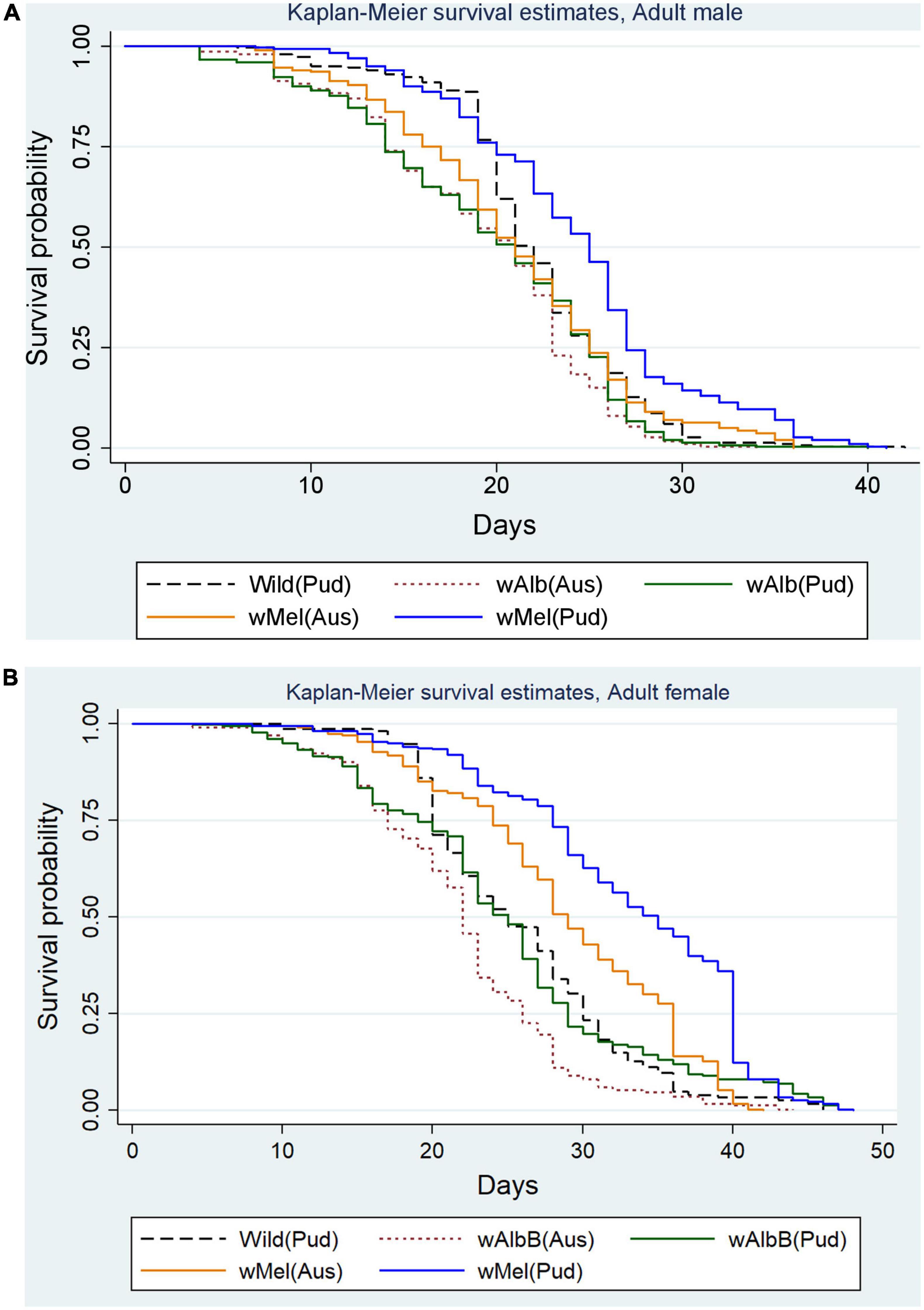
Figure 3. (A) Survival of Wolbachia-infected Ae. aegypti males in comparison with uninfected wild Ae. aegypti (Pud) males. (B) Survival of Wolbachia-infected Ae. aegypti females in comparison with uninfected wild Ae. aegypti (Pud) females.
In wAlbB (Pud) line, 50% of adult males survived up to 21 days and females up to 25 days and the corresponding values for wAlbB (Aus) line were 21 days for males and 22 days for females. In the case of wild (Pud) line, survival of 50% males was up to 22 days and females up to 25 days (Figures 3A,B).
The mean duration of 50% survival of the wMel Ae. aegypti (Pud) males and females was significantly greater than those of the other Wolbachia-infected and the uninfected wild Ae. aegypti males and females (p < 0.001; using log-rank test). Overall, the survival curves of males (p < 0.001, log-rank test) and females (p < 0.001, log-rank test) of the five lines varied significantly. Among the adult Ae. aegypti males, the risk of death was significantly lower in the wMel Ae. aegypti (Pud) males (HR = 0.66, 95% CI: 0.56–0.78, p < 0.001) than that of the wild Ae. aegypti (Pud) males. The risk of death was significantly higher in the wAlbB Ae. aegypti (Pud) line (HR = 1.25, 95% CI: 1.06–1.47, p = 0.007) and the wAlbB Ae. aegypti (Aus) line (HR = 1.43, 95% CI: 1.22–1.68, p < 0.001), and the risk of death was higher but not significant in the wMel Ae. aegypti (Aus) line (HR = 1.06, 95% CI: 0.90–1.24, p = 0.505) when compared to that of the wild Ae. aegypti (Pud) line. Among the adult females, the risk of death was significantly lower in the wMel Ae. aegypti (Pud) females (HR = 0.44, 95% CI: 0.38–0.52, p < 0.001) and the wMel Ae. aegypti (Aus) females (HR = 0.73, 95% CI: 0.62–0.86, p < 0.001) than that of the wild Ae. aegypti (Pud) females. The risk of death was lower, but not significantly different in wAlbB Ae. aegypti (Pud) line (HR = 0.92, 95% CI: 0.78–1.08, p = 0.301) than that of the wild Ae. aegypti (Pud) line. The risk of death was significantly higher in the wAlbB Ae. aegypti (Aus) line (HR = 1.65, 95% CI: 1.40–1.94, p < 0.001) than that of the wild Ae. aegypti (Pud) line.
Maternal transmission
The maternal transmission efficiency of the wMel and wAlbB (Pud) lines was studied in comparison with that of the Wolbachia-infected (Aus) parental lines by testing the frequency of infected adult progeny produced by an infected female. In all the replicates of wMel and wAlbB Ae. aegypti (Pud) lines, a near-complete maternal transmission was observed with a frequency of Wolbachia in the adult progeny ranging from 99 to 100% (Table 1), while for wMel and wAlbB Ae. aegypti (Aus) lines, the frequency of Wolbachia in the adult progeny ranged from 96 to 100% (Table 2).
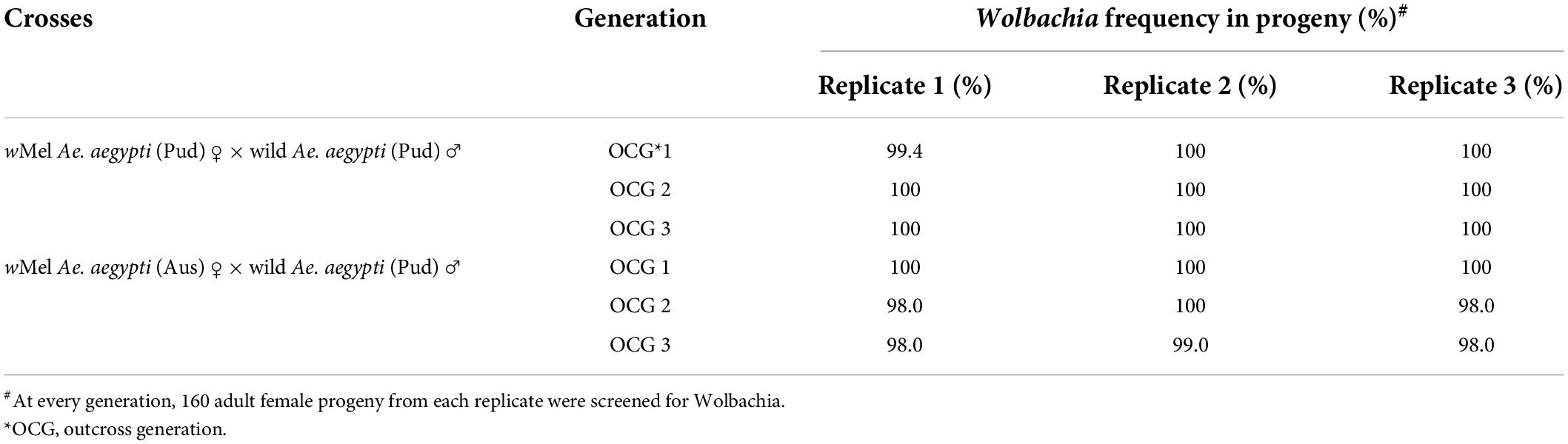
Table 1. Maternal transmission of Wolbachia assessed from the progeny of crosses between wMel-infected Ae. aegypti females and uninfected wild Ae. aegypti males.
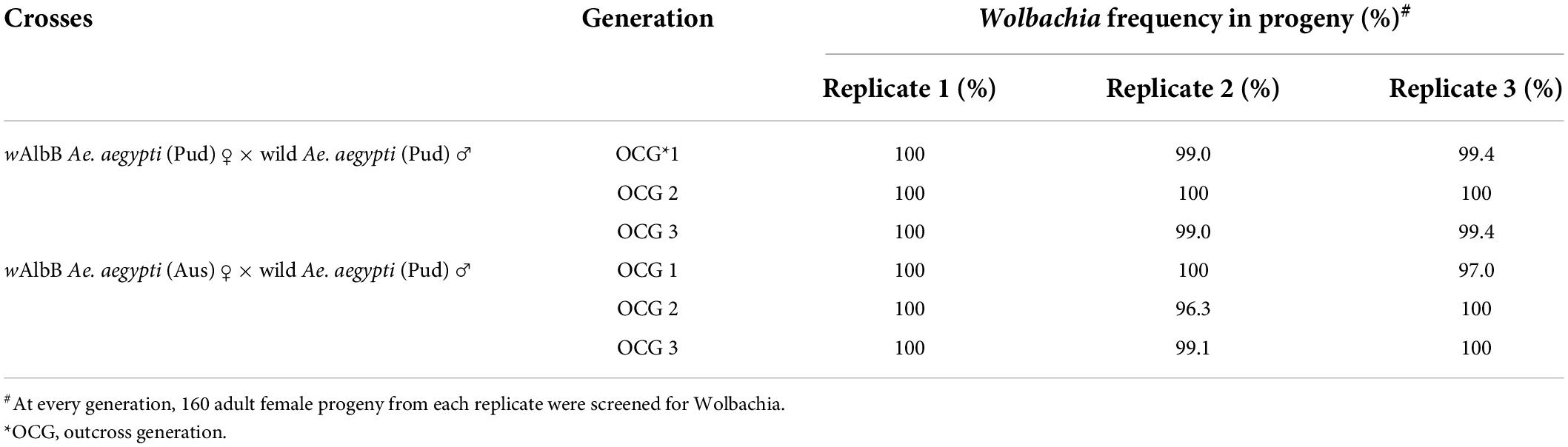
Table 2. Maternal transmission of Wolbachia assessed from the progeny of crosses between wAlbB-infected Ae. aegypti females and uninfected wild Ae. aegypti males.
Cytoplasmic incompatibility
Cytoplasmic incompatibility was determined by measuring the egg hatch rate from crosses between the uninfected wild Ae. aegypti (Pud) females and the Wolbachia-infected males. Egg hatch rate of the crosses between uninfected wild Ae. aegypti (Pud) females and wMel-infected Ae. aegypti (Pud) males was only 0.2–1.2% in the first outcross generation (OCG 1). In outcross generations 2 and 3, no viable offspring were obtained indicating a complete sterility (Table 3). Similarly, the crosses between the uninfected wild Ae. aegypti (Pud) females and the wAlbB-infected Ae. aegypti (Pud) males resulted in complete sterility as there was no viable offspring in all the three generations tested (Table 4).
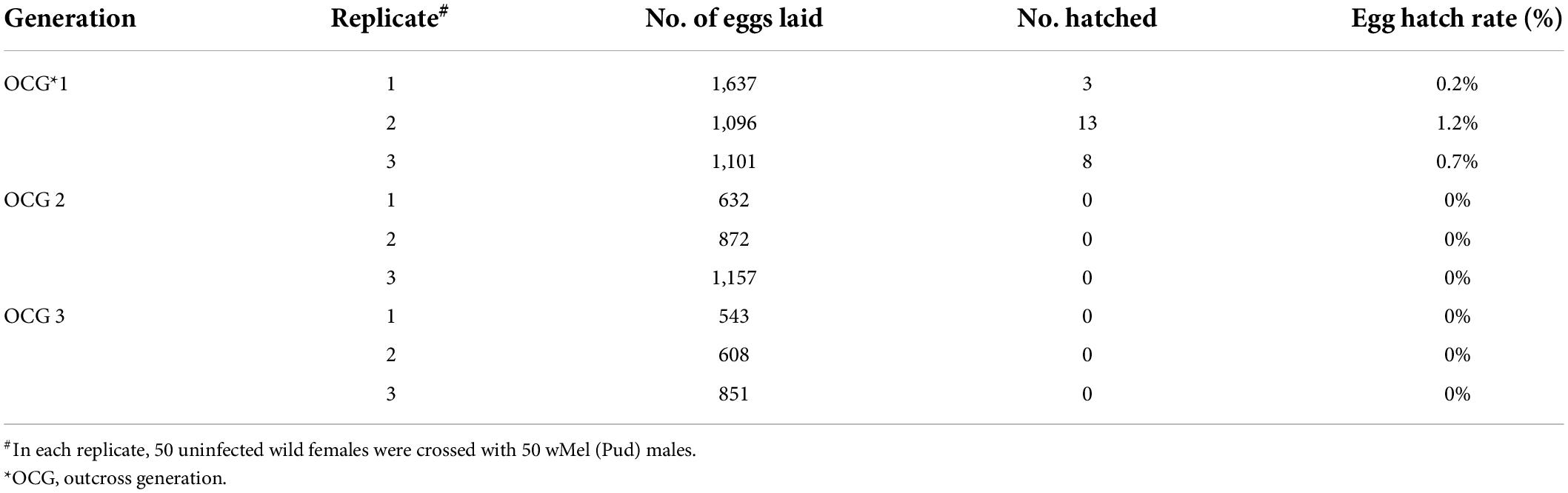
Table 3. Induced cytoplasmic incompatibility observed in crosses between uninfected wild Ae. aegypti (Pud) females and wMel-infected Ae. aegypti (Pud) males.
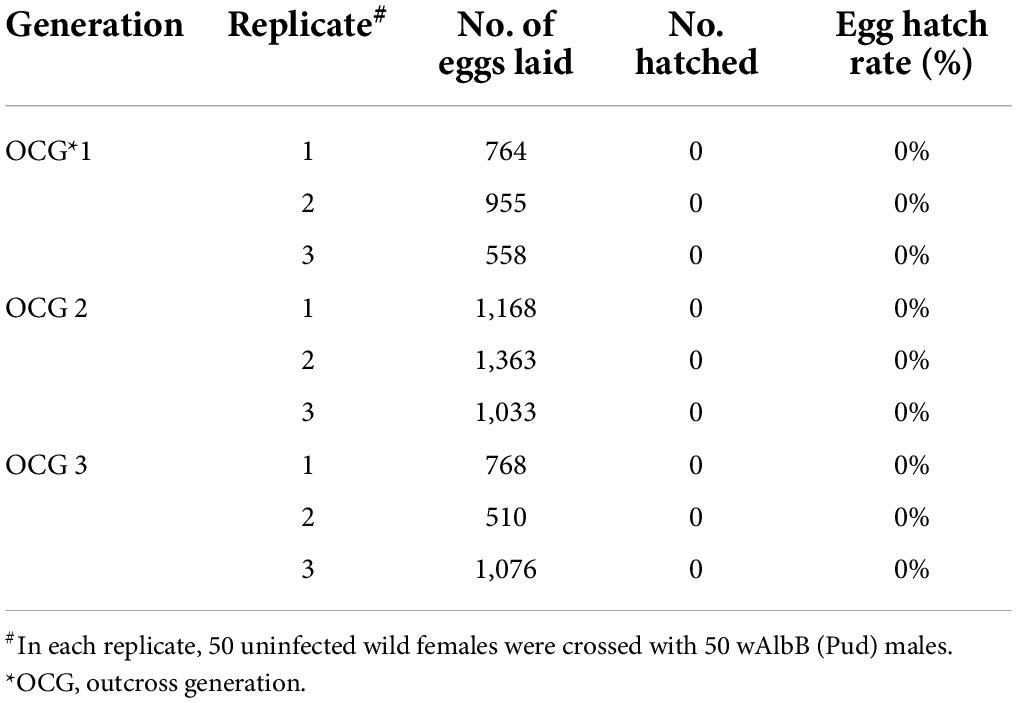
Table 4. Induced cytoplasmic incompatibility observed in crosses between uninfected wild Ae. aegypti (Pud) females and wAlbB-infected Ae. aegypti (Pud) males.
Insecticide resistance
When exposed to the discriminating concentration of DDT (4%) and malathion (0.8%), the percent mortality of both the Ae. aegypti (Pud) lines and the wild Ae. aegypti females ranged from 0.0 to 5.7% (Table 5). Against deltamethrin 0.03%, the percent mortality of the (Pud) lines and the wild females ranged from 81.4 to 83.6%. The percent mortality of the Wolbachia-introgressed Ae. aegypti (Pud) lines and of the wild Ae. aegypti (Pud) females was almost 100% on exposure to 5x discriminating concentration of malathion and deltamethrin (Table 5). At the diagnostic concentration of temephos (0.02 mg/L), the percent mortality of larvae of both the Wolbachia-introgressed Ae. aegypti (Pud) lines and the wild Ae. aegypti (Pud) line ranged from 61.0 to 70.0%.
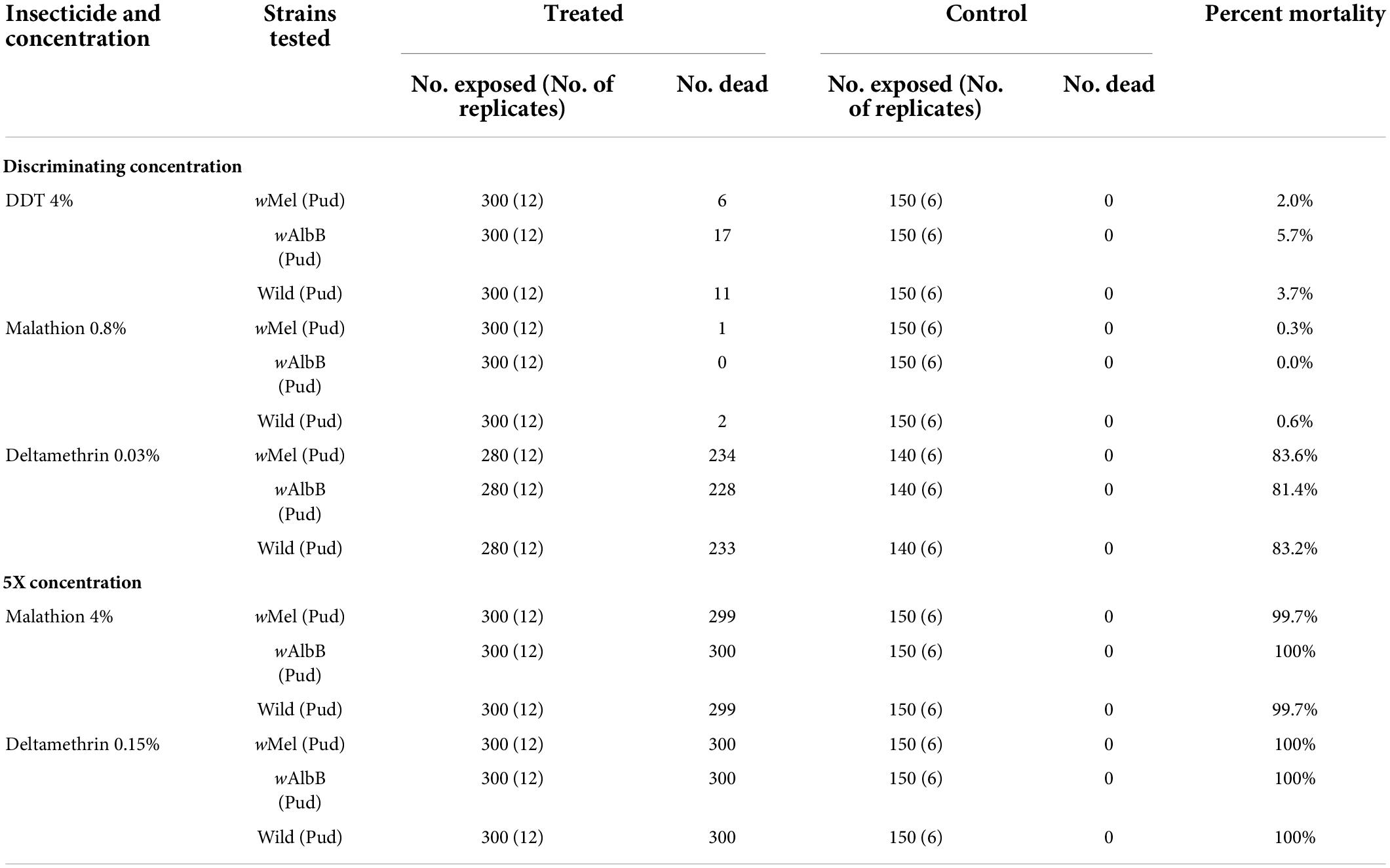
Table 5. Percent mortality of wMel and wAlbB Ae. aegypti (Pud) lines and wild Ae. aegypti (Pud) females on exposure to discriminating/5x concentrations of public health insecticides.
Discussion
ICMR-VCRC in collaboration with WMP has generated two new Ae. aegypti (Pud) lines introgressed with the wMel and wAlbB Wolbachia (Aus) strains for field release and testing. Wolbachia strains have many traits that induce a fitness cost and confer fitness benefit on their host mosquito populations. The effects of Wolbachia infections on the biological/reproductive fitness characteristics, such as fecundity, egg hatch rate, egg viability, locomotor ability, blood feeding, adult survival, and male mating competitiveness, have been documented (Evans et al., 2009; Turley et al., 2009; Walker et al., 2011; Yeap et al., 2011; Carvalho et al., 2020). Fitness of the Wolbachia-introgressed Ae. aegypti (Pud) lines are the key factors that would determine their rapid spread into native wild populations when tested in the field. The Wolbachia-introgressed (Pud) lines must have at the minimum a similar level of fitness characteristics compared to that of the native wild Ae. aegypti populations. This study examined the effects of the wMel and wAlbB Wolbachia infections on the fitness of the newly generated Ae. aegypti (Pud) lines with reference to the wild Ae. aegypti (Pud) populations. The maternal transmission efficiency, cytoplasmic incompatibility, and insecticide resistance status of the (Pud) lines were also investigated. It is to be noted that the fitness characteristics that were measured in the laboratory represent correlates of field fitness although they were not the measures of actual fitness, which can be determined only in the field. However, the data generated on the fitness measurements showed that the backcrossed lines are not heavily compromised in terms of these correlates and as such appear suitable to be used as release lines.
The fitness characteristics, such as wing length, fecundity, fertility, and longevity, are highly sensitive to variations in the micro-environment and feeding regimen. Hence, the experiments were carried out with all the five lines under controlled temperature (27 ± 2°C), relative humidity (80%), and rearing conditions (including egg storage, egg hatching, larval rearing, and larval and adult feeding regimens). In terms of physical fitness, that is, wing length, wMel and wAlbB infections produced a fitness benefit in the two Ae. aegypti (Pud) lines. The wing length of females of both Wolbachia-introgressed (Pud) lines was significantly greater than that of the wild (Pud) line. Between the two Wolbachia-introgressed (Pud) lines, no significant difference in the wing length was observed. In a previous study, no significant difference in the wing length was observed between wAlbB-infected and uninfected Ae. aegypti males and females (Axford et al., 2016).
The wAlbB (Pud) line had 16% fecundity advantage over the uninfected wild females. In the case of wMel (Pud) line, there was a slight reduction in fecundity (1.06%), but it was not significant compared to that of the wild-type females. The two (Pud) lines showed a comparable egg hatch rate to that of the wild (Pud) line. Joubert et al. (2016) observed that wMel-infected females laid significantly a larger number of eggs than the uninfected females. On the contrary, no significant difference in fecundity was observed between the wAlbB-infected and the uninfected Ae. aegypti females (Axford et al., 2016; Joubert et al., 2016). The reason for such variations in the results of the studies could be attributed to the different strains of Wolbachia-introgressed into Ae. aegypti lines with different genomic backgrounds. It has been reported that the same Wolbachia strain may have a different effect on the fitness of a strain depending on the host background (Carvalho et al., 2020).
In this study, both sexes of the wMel-infected Ae. aegypti (Pud) survived longer than the wild Ae. Aegypti, indicating a fitness benefit conferred by the wMel infection. The survival of the wAlbB-infected (Pud) females and males was comparable to that of the wild Ae. aegypti (Pud) line. As a result, in terms of adult survival, the wMel (Pud) line could be in an advantageous position than the wAlbB (Pud) line. In an earlier study, out of the four Wolbachia strains tested namely, wMel, wAlbA, wAlbB, and wAu, wMel was the only infection that did not cause a significant reduction in adult female longevity (Ant et al., 2018). wMel- and wAlbB-infected Ae. aegypti males and females lived longer than the uninfected males and females. When the two infected strains are compared, wMel males and females survived longer than the wAlbB-infected males and females (Axford et al., 2016). It has been reported that the survival rate of Ae. albopictus females infected with wAlbA and superinfected with wAlbA and wAlbB was higher than that of the uninfected females (Dobson et al., 2002, 2004). There was a 50% reduction in survival rates in wMelPoP-infected Ae. aegypti line, whereas it was only 10% in the wMel-infected Ae. aegypti line, indicating that the wMel infection induced a less fitness cost on adult survival than the life-shortening wMelPoP strain (Riegler et al., 2005; Walker et al., 2011). Joubert et al. (2016) reported a higher mean survival time for the wMel- and wAlbB-infected Ae. aegypti females than the uninfected females, as observed in this study. Increased adult survival is a fitness advantage conferred by an avirulent wMel strain that could facilitate the introgression of the wMel infection into the wild populations upon field releases. In the city-wide field trials, wMel had invaded wild mosquito populations successfully (O’Neill et al., 2018; Indriani et al., 2020; Ryan et al., 2020) and the infection remained stable in the release areas (Frentiu et al., 2014; Hoffmann et al., 2014), whereas the field release of a virulent wMelPoP strain in Vietnam failed to invade the wild population due to its life-shortening deleterious effect (Nguyen et al., 2015). In addition to the above factors, male mating competitiveness is one of the important life-history traits that influence the successful establishment of the inherited Wolbachia infections into the wild population (Carvalho et al., 2020).
The maternal transmission of Wolbachia strain and its ability to induce cytoplasmic incompatibility are the two essential features that must be conserved while considering a line for field release (Fraser et al., 2017). In this study, a near-complete (99–100%) maternal transmission of wMel and wAlbB infections was observed in the (Pud) lines. A perfect (100%) cytoplasmic incompatibility was observed in all the crosses between wild-type Ae. aegypti (Pud) females and the wAlbB or wMel Ae. aegypti (Pud) males, except in the first generation of wMel Ae. aegypti (Pud) line, where there was an egg hatch rate (viable progeny) of 0.2–1.2%, and on screening, 13 (54.2%) of the emerged adults (n = 24) were found positive for Wolbachia. This was, however, well below the acceptable level of < 3% (World Mosquito Program [WMP], 2019). As indicated by the results, CI is thus expected to provide a reproductive advantage to the Wolbachia-infected females over the uninfected females resulting in the spread of Wolbachia among local population (Joshi et al., 2014). In the laboratory experiments carried out elsewhere, wMel-transinfected Ae. aegypti displayed perfect cytoplasmic incompatibility and maternal transmission (Riegler et al., 2005; Walker et al., 2011; Joubert et al., 2016). Similar observations were made in wAlbB-infected Ae. albopictus (Xi et al., 2005; Axford et al., 2016; Joubert et al., 2016), the secondary vector of dengue and chikungunya viruses. Aedes albopictus has been responsible for outbreaks of dengue in India (Tewari et al., 2004; Thenmozhi et al., 2007; Kumari et al., 2011) and also in Madagascar (Ratsitorahina et al., 2008), Hawaii (Effler et al., 2005), Mauritius (Issack et al., 2010), and China (Xu et al., 2007).
Field-released mosquito strains require adequate protection against the insecticides used by the public health program to ensure their survival after the field release. It has been shown that the field releases of susceptible lines into wild populations that are resistant were unable to compete with the wild population and failed to result in Wolbachia establishment, whereas field releases of lines that are similar in resistant status to the wild population led to the successful spread of Wolbachia infection among the local wild population of Ae. aegypti (Garcia et al., 2020). The determination of insecticide resistance profile of the wild-type Ae. aegypti (Pud) is also equally important since that will help in deciding where to collect the wild-type mosquitoes for backcrossing experiments to raise the local Wolbachia-infected (Pud) lines that will have similar insecticide resistance status as that of wild mosquito population.
In this study, on exposure to discriminating 1X and 5X concentrations, the two Wolbachia-introgressed (Pud) lines showed similar level of resistance to deltamethrin and malathion compared to the wild-type Ae. aegypti, indicating that the Wolbachia-infected Ae. aegypti (Pud) lines would tolerate the insecticide pressure in the field after release. The 100% mortality on exposure to 5X concentrations of deltamethrin and malathion indicated a low level of resistance intensity in the two (Pud) lines, as well as in the wild type to the two commonly used insecticides during emergencies in the control program. At the diagnostic concentration of 0.02 mg/L (World Health Organization [WHO], 1981), the resistance level to temephos was similar in both the (Pud) lines and the wild type.
Summary
The effects of wMel and wAlbB infections on Ae. aegypti (Pud) lines were assessed in terms of their physical (wing length) and reproductive fitness. The Wolbachia-infected Ae. aegypti (Pud) lines had greater wing length than the wild uninfected line. With respect to reproductive fitness, wAlbB-infected Ae. aegypti (Pud) had an advantage of 16% higher fecundity, while wMel Ae. aegypti (Pud) showed a comparable fecundity with the uninfected wild females. Both the wMel- and wAlbB-infected Ae. aegypti (Pud) lines presented a higher egg hatch rate than the uninfected wild (Pud) line. The wMel-infected Ae. aegypti (Pud) line lived significantly longer than the wAlbB-infected (Pud) line and the uninfected (Pud) wild line. Both the wMel and wAlbB strains displayed a complete maternal transmission and induced a strong cytoplasmic incompatibility. The two Ae. aegypti (Pud) lines showed a similar level of insecticide resistance compared to the uninfected wild Ae. aegypti (Pud) line. The study results showed that the wMel and wAlbB infections in the two newly generated Ae. aegypti (Pud) lines produced strong cytoplasmic incompatibility, perfect maternal transmission, and favorable biological/reproductive fitness benefits, indicating the suitability of the two (Pud) lines for examination under field release trials. Studies on population replacement and male mating competitiveness have been completed (unpublished results), and the results are in favor of this conclusion.
Data availability statement
The original contributions presented in this study are included in the article/supplementary material, further inquiries can be directed to the corresponding authors.
Author contributions
PJ, KG, and SS contributed to conception and design of the study. CS, KG, DP, AS, and VA conducted the experiments. DP and SD performed Wolbachia diagnosis. CS and MR supervised the experiment. CS organized the database and wrote the first draft of the manuscript. BV performed the statistical analysis. KG and DP wrote the sections of the manuscript. All authors contributed to manuscript revision, read, and approved the submitted version.
Funding
This work was supported by Indian Council of Medical Research (ICMR), Department Health Research, Ministry Health & Family Welfare, Govt. of India.
Acknowledgments
We thank the Indian Council of Medical Research (ICMR) for providing financial support. We gratefully acknowledge the World Mosquito Program for supplying eggs of Wolbachia-infected Ae. aegypti (Aus) lines. We wish to thank Scott L. O’Neill, Breeanna Mclean, Edwige Rances, and Jacqui Montgomery of the World Mosquito Program for providing technical support and guidance with the experiments. We would also like to thank M. Stalin, N. Ramesh, R. Nivethaa, S. Sathiyapriya, and other project technical staff for maintenance of Wolbachia-infected Aedes aegypti colonies and M. Krishnaraja for Wolbachia diagnosis.
Conflict of interest
The authors declare that the research was conducted in the absence of any commercial or financial relationships that could be construed as a potential conflict of interest.
Publisher’s note
All claims expressed in this article are solely those of the authors and do not necessarily represent those of their affiliated organizations, or those of the publisher, the editors and the reviewers. Any product that may be evaluated in this article, or claim that may be made by its manufacturer, is not guaranteed or endorsed by the publisher.
References
Allman, M. J., Fraser, J. E., Ritchie, S. A., Joubert, D. A., Simmons, C. P., and Flores, H. A. (2020). Wolbachia’s Deleterious Impact on Aedes aegypti Egg Development: The Potential Role of Nutritional Parasitism. Insects 11:735. doi: 10.3390/insects11110735
Almeida, F. D., Moura, A. S., Cardoso, A. F., Winter, C. E., Bijovsky, A. T., and Suesdek, L. (2011). Effects of Wolbachia on fitness of Culex quinquefasciatus (Diptera; Culicidae). Infect. Genet. Evol. 11, 2138–2143. doi: 10.1016/j.meegid.2011.08.022
Ant, T. H., Herd, C. S., Geoghegan, V., Hoffmann, A. A., and Sinkins, S. P. (2018). The Wolbachia strain wAu provides highly efficient virus transmission blocking in Aedes aegypti. PLoS Pathog. 14:e1006815. doi: 10.1371/journal.ppat.1006815
Axford, J. K., Ross, P. A., Yeap, H. L., Callahan, A. G., and Hoffmann, A. A. (2016). Fitness of wAlbB Wolbachia Infection in Aedes aegypti: Parameter Estimates in an Outcrossed Background and Potential for Population Invasion. Am. J. Trop. Med. Hyg. 94, 507–516. doi: 10.4269/ajtmh.15-0608
Barraud, P. J. (1934). The Fauna of British India, including Ceylon and Burma. Diptera. Vol. V. Family Culicidae. Tribes Megarhinini and Culicini. London: Taylor and Francis, 1–510.
Beebe, N. W., Pagendam, D., Trewin, B. J., Boomer, A., Bradford, M., Ford, A., et al. (2021). Releasing incompatible males drives strong suppression across populations of wild and Wolbachia-carrying Aedes aegypti in Australia. Proc. Natl. Acad. Sci. U.S.A. 118:e2106828118. doi: 10.1073/pnas.2106828118
Bennett, K. L., Gómez-Martínez, C., Chin, Y., Saltonstall, K., McMillan, W. O., Rovira, J. R., et al. (2019). Dynamics and diversity of bacteria associated with the disease vectors Aedes aegypti and Aedes albopictus. Sci. Rep. 9:12160. doi: 10.1038/s41598-019-48414-8
Bourtzis, K., Dobson, S. L., Xi, Z., Rasgon, J. L., Calvitti, M., Moreira, L. A., et al. (2014). Harnessing mosquito-Wolbachia symbiosis for vector and disease control. Acta Trop. 132, S150–S163. doi: 10.1016/j.actatropica.2013.11.004
Burki, T. (2020). Wolbachia, a bacterium fighting on our side. Lancet Infect. Dis. 20, 662–663. doi: 10.1016/S1473-3099(20)30384-4
Caragata, E. P., Dutra, H., and Moreira, L. A. (2016). Exploiting Intimate Relationships: Controlling Mosquito-Transmitted Disease with Wolbachia. Trends Parasitol. 32, 207–218. doi: 10.1016/j.pt.2015.10.011
Carvajal, T. M., Hashimoto, K., Harnandika, R. K., Amalin, D. M., and Watanabe, K. (2019). Detection of Wolbachia in field-collected Aedes aegypti mosquitoes in metropolitan Manila, Philippines. Parasit. Vectors 12:361. doi: 10.1186/s13071-019-3629-y
Carvalho, D. O., Torres-Monzon, J. A., Koskinioti, P., Dilrukshi Wijegunawardana, N. A., Liang, X., Pillwax, G., et al. (2020). Aedes aegypti lines for combined sterile insect technique and incompatible insect technique applications: The importance of host genomic background. Entomol. Exp. Appl. 168, 560–572. doi: 10.1111/eea.12892
Crawford, J. E., Clarke, D. W., Criswell, V., Desnoyer, M., Cornel, D., Deegan, B., et al. (2020). Efficient production of male Wolbachia-infected Aedes aegypti mosquitoes enables large-scale suppression of wild populations. Nat. Biotechnol. 38, 482–492. doi: 10.1038/s41587-020-0471-x
Deng, S. Q., Yang, X., Wei, Y., Chen, J. T., Wang, X. J., and Peng, H. J. (2020). A Review on Dengue Vaccine Development. Vaccines 8:63. doi: 10.3390/vaccines8010063
Dobson, S. L., Marsland, E. J., and Rattanadechakul, W. (2002). Mutualistic Wolbachia infection in Aedes albopictus: Accelerating cytoplasmic drive. Genetics 160, 1087–1094. doi: 10.1093/genetics/160.3.1087
Dobson, S. L., Rattanadechakul, W., and Marsland, E. J. (2004). Fitness advantage and cytoplasmic incompatibility in Wolbachia single- and super infected Aedes albopictus. Heredity 93, 135–142. doi: 10.1038/sj.hdy.6800458
Dumas, E., Atyame, C. M., Milesi, P., Fonseca, P. D., Shaikevich, E. V., Unal, S., et al. (2013). Population structure of Wolbachia and cytoplasmic introgression in a complex of mosquito species. BMC Evol. Biol. 13:181. doi: 10.1186/1471-2148-13-181
Effler, P. V., Pang, L., Kitsutani, P., Vorndam, V., Nakata, M., Ayers, T., et al. (2005). Dengue fever, Hawaii, 2001-2002. Emerg. Infect. Dis. 11, 742–749. doi: 10.3201/eid1105.041063
Evans, O., Caragata, E. P., McMeniman, C. J., Woolfit, M., Green, D. C., Williams, C. R., et al. (2009). Increased locomotor activity and metabolism of Aedes aegypti infected with a life-shortening strain of Wolbachia pipientis. J. Exp. Biol. 212, 1436–1441. doi: 10.1242/jeb.028951
Ferguson, N. M., Kien, D. T., Clapham, H., Aguas, R., Trung, V. T., Chau, T. N., et al. (2015). Modeling the impact on virus transmission of Wolbachia-mediated blocking of dengue virus infection of Aedes aegypti. Sci. Transl. Med. 7:279ra37. doi: 10.1126/scitranslmed.3010370
Fraser, J. E., DeBruyne, J. T., Iturbe-Ormaetxe, I., Stepnell, J., Burns, R. L., Flores, H. A., et al. (2017). Novel Wolbachia-transinfected Aedes aegypti mosquitoes possess diverse fitness and vector competence phenotypes. PLoS Pathog. 13:e1006751. doi: 10.1371/journal.ppat.1006751
Frentiu, F. D., Robinson, J., Young, P. R., McGraw, E. A., and O’Neill, S. L. (2010). Wolbachia-mediated resistance to dengue virus infection and death at the cellular level. PLoS One 5:e13398. doi: 10.1371/journal.pone.0013398
Frentiu, F. D., Zakir, T., Walker, T., Popovici, J., Pyke, A. T., van den Hurk, A., et al. (2014). Limited dengue virus replication in field-collected Aedes aegypti mosquitoes infected with Wolbachia. PLoS Negl. Trop. Dis. 8:e2688. doi: 10.1371/journal.pntd.0002688
Ganeshkumar, P., Murhekar, M. V., Poornima, V., Saravanakumar, V., Sukumaran, K., Anandaselvasankar, A., et al. (2018). Dengue infection in India: A systematic review and meta-analysis. PLoS Negl. Trop. Dis. 12:e0006618. doi: 10.1371/journal.pntd.0006618
Garcia, G. A., Hoffmann, A. A., Maciel-de-Freitas, R., and Villela, D. (2020). Aedes aegypti insecticide resistance underlies the success (and failure) of Wolbachia population replacement. Sci. Rep. 10:63. doi: 10.1038/s41598-019-56766-4
Garcia, G. A., Sylvestre, G., Aguiar, R., da Costa, G. B., Martins, A. J., Lima, J., et al. (2019). Matching the genetics of released and local Aedes aegypti populations is critical to assure Wolbachia invasion. PLoS Negl. Trop. Dis. 13:e0007023. doi: 10.1371/journal.pntd.0007023
Glaser, R. L., and Meola, M. A. (2010). The native Wolbachia endosymbionts of Drosophila melanogaster and Culex quinquefasciatus increase host resistance to West Nile virus infection. PLoS One 5:e11977. doi: 10.1371/journal.pone.0011977
Hedges, L. M., Brownlie, J. C., O’Neill, S. L., and Johnson, K. N. (2008). Wolbachia and virus protection in insects. Science 322:702. doi: 10.1126/science.1162418
Hertig, M., and Wolbach, S. B. (1924). Studies of Rickettsia-like microorganisms in insects. J. Med. Res. 44, 329–374.7.
Hien, N. T., Anh, D. D., Le, N. H., Yen, N. T., Phong, T. V., Nam, V. S., et al. (2022). Environmental factors influence the local establishment of Wolbachia in Aedes aegypti mosquitoes in two small communities in central Vietnam. Gates Open Res. 5:147. doi: 10.12688/gatesopenres.13347.2
Hoffmann, A. A., Iturbe-Ormaetxe, I., Callahan, A. G., Phillips, B. L., Billington, K., Axford, J. K., et al. (2014). Stability of the wMel Wolbachia Infection following invasion into Aedes aegypti populations. PLoS Negl. Trop. Dis. 8:e3115. doi: 10.1371/journal.pntd.0003115
Hoffmann, A. A., Turelli, M., and Harshman, L. G. (1990). Factors affecting the distribution of cytoplasmic incompatibility in Drosophila simulans. Genetics 126, 933–948. doi: 10.1093/genetics/126.4.933
Imam, H., Zarnigar, Sofi, G., and Seikh, A. (2014). The basic rules and methods of mosquito rearing (Aedes aegypti). Trop. Parasitol. 4, 53–55. doi: 10.4103/2229-5070.129167
Indriani, C., Tantowijoyo, W., Rancès, E., Andari, B., Prabowo, E., Yusdi, D., et al. (2020). Reduced dengue incidence following deployments of Wolbachia-infected Aedes aegypti in Yogyakarta, Indonesia: A quasi-experimental trial using controlled interrupted time series analysis. Gates Open Res. 4:50. doi: 10.12688/gatesopenres.13122.1
Issack, M. I., Pursem, V. N., Barkham, T. M., Ng, L. C., Inoue, M., and Manraj, S. S. (2010). Reemergence of dengue in Mauritius. Emerg. Infect. Dis. 16, 716–718. doi: 10.3201/eid1604.091582
Iturbe-Ormaetxe, I., Walker, T., and O’ Neill, S. L. (2011). Wolbachia and the biological control of mosquito-borne disease. EMBO Rep. 12, 508–518. doi: 10.1038/embor.2011.84
Jain, S., and Sharma, S. K. (2017). Challenges & options in dengue prevention & control: A perspective from the 2015 outbreak. Indian J. Med. Res. 145, 718–721. doi: 10.4103/ijmr.IJMR_1325_16
Johnson, K. N. (2015). The impact of Wolbachia on virus Infection in Mosquitoes. Viruses 7, 5705–5717. doi: 10.3390/v7112903
Joshi, D., McFadden, M. J., Bevins, D., Zhang, F., and Xi, Z. (2014). Wolbachia strain wAlbB confers both fitness costs and benefit on Anopheles stephensi. Parasit. Vectors 7:336. doi: 10.1186/1756-3305-7-336
Joubert, D. A., Walker, T., Carrington, L. B., De Bruyne, J. T., Kien, D. H., Hoang, N., et al. (2016). Establishment of a Wolbachia superinfection in Aedes aegypti mosquitoes as a potential approach for future resistance management. PLoS Pathog. 12:e1005434 doi: 10.1371/.journal.ppat.1005434
Judson, C. L. (1960). The Physiology of Hatching of Aedine Mosquito Eggs: Hatching Stimulus. Ann. Entomol. Soc. Am. 53, 688–691. doi: 10.1093/aesa/53.5.68
Kay, B., and Vu, S. N. (2005). New strategy against Aedes aegypti in Vietnam. Lancet 365, 613–617. doi: 10.1016/S0140-6736(05)17913-6
Kulkarni, A., Yu, W., Jiang, J., Sanchez, C., Karna, A. K., Martinez, K., et al. (2019). Wolbachia pipientis occurs in Aedes aegypti populations in New Mexico and Florida, USA. Ecol. Evol. 9, 6148–6156. doi: 10.1002/ece3.5198
Kumari, R., Kumar, K., and Chauhan, L. S. (2011). First dengue virus detection in Aedes albopictus from Delhi, India: Its breeding ecology and role in dengue transmission. Trop. Med. Int. Health 16, 949–954. doi: 10.1111/j.1365-3156.2011.02789.x
Martín-Park, A., Che-Mendoza, A., Contreras-Perera, Y., Pérez-Carrillo, S., Puerta-Guardo, H., Villegas-Chim, J., et al. (2022). Pilot trial using mass field-releases of sterile males produced with the incompatible and sterile insect techniques as part of integrated Aedes aegypti control in Mexico. PLoS Negl. Trop. Dis. 16:e0010324. doi: 10.1371/journal.pntd.0010324
McMeniman, C. J., and O’Neill, S. L. (2010). A virulent Wolbachia infection decreases the viability of the dengue vector Aedes aegypti during periods of embryonic quiescence. PLoS Negl. Trop. Dis. 4:e748. doi: 10.1371/journal.pntd.0000748
Moreira, L. A., Iturbe-Ormaetxe, I., Jeffery, J. A., Lu, G., Pyke, A. T., Hedges, L. M., et al. (2009). A Wolbachia symbiont in Aedes aegypti limits infection with dengue, Chikungunya, and Plasmodium. Cell 139, 1268–1278. doi: 10.1016/j.cell.2009.11.042
National Environmental Agency [NEA] (2022). Wolbachia-Aedes Mosquito Suppression Strategy. Singapore: National Environmental Agency.
National Vector Borne Disease Control Programme [NVBDCP] (2022). National Vector Borne Disease Control Programme. Available Online at: https://nvbdcp.gov.in/ (accessed May 11, 2022).
Nazni, W. A., Hoffmann, A. A., NoorAfizah, A., Cheong, Y. L., Mancini, M. V., Golding, N., et al. (2019). Establishment of Wolbachia strain wAlbB in Malaysian populations of Aedes aegypti for dengue control. Curr. Biol. 29, 4241–4248. doi: 10.1016/j.cub.2019.11.007
Nguyen, T. H., Nguyen, H. L., Nguyen, T. Y., Vu, S. N., Tran, N. D., Le, T. N., et al. (2015). Field evaluation of the establishment potential of wMelPop Wolbachia in Australia and Vietnam for dengue control. Parasit. Vectors 8:563. doi: 10.1186/s13071-015-1174-x
Noda, H., Munderloh, U. G., and Kurtti, T. J. (1997). Endosymbionts of ticks and their relationship to Wolbachia spp. and tick-borne pathogens of humans and animals. Appl. Environ. Microbiol. 63, 3926–3932. doi: 10.1128/aem.63.10.3926-3932.1997
O’Neill, S. L., Ryan, P. A., Turley, A. P., Wilson, G., Retzki, K., Iturbe-Ormaetxe, I., et al. (2018). Scaled deployment of Wolbachia to protect the community from dengue and other Aedes transmitted arboviruses. Gates Open Res. 2:36. doi: 10.12688/gatesopenres.12844.3
Pinto, S. B., Riback, T., Sylvestre, G., Costa, G., Peixoto, J., Dias, F., et al. (2021). Effectiveness of Wolbachia-infected mosquito deployments in reducing the incidence of dengue and other Aedes-borne diseases in Niterói, Brazil: A quasi-experimental study. PLoS Negl. Trop. Dis. 15:e0009556. doi: 10.1371/journal.pntd.0009556
Ratsitorahina, M., Harisoa, J., Ratovonjato, J., Biacabe, S., Reynes, J. M., Zeller, H., et al. (2008). Outbreak of dengue and Chikungunya fevers, Toamasina, Madagascar, 2006. Emerg. Infect. Dis. 14, 1135–1137. doi: 10.3201/eid1407.071521
Riegler, M., Sidhu, M., Miller, W. J., and O’Neill, S. L. (2005). Evidence for a global Wolbachia replacement in Drosophila melanogaster. Curr. Biol. 15, 1428–1433. doi: 10.1016/j.cub.2005.06.069
Ross, P. A., Axford, J. K., Richardson, K. M., Endersby-Harshman, N. M., and Hoffmann, A. A. (2017). Maintaining Aedes aegypti Mosquitoes Infected with Wolbachia. J. Vis. Exp. 14:56124. doi: 10.3791/56124
Ross, P. A., Callahan, A. G., Yang, Q., Jasper, M., Arif, M., Afizah, A. N., et al. (2020). An elusive endosymbiont: Does Wolbachia occur naturally in Aedes aegypti? Ecol. Evol. 10, 1581–1591. doi: 10.1002/ece3.6012
Ryan, P. A., Turley, A. P., Wilson, G., Hurst, T. P., Retzki, K., Brown-Kenyon, J., et al. (2020). Establishment of wMel Wolbachia in Aedes aegypti mosquitoes and reduction of local dengue transmission in Cairns and surrounding locations in northern Queensland, Australia. Gates Open Res. 3:1547. doi: 10.12688/gatesopenres.13061.2
Sim, S., Jupatanakul, N., and Dimopoulos, G. (2014). Mosquito immunity against arboviruses. Viruses 6, 4479–4504. doi: 10.3390/v6114479
Sinkins, S. P. (2004). Wolbachia and cytoplasmic incompatibility in mosquitoes. Insect Biochem. Mol. Biol. 34, 723–729. doi: 10.1016/j.ibmb.2004.03.025
Sinkins, S. P., Braig, H. R., and O’Neill, S. L. (1995). Wolbachia pipientis: Bacterial density and unidirectional cytoplasmic incompatibility between infected populations of Aedes albopictus. Exp. Parasitol. 8, 284–291. doi: 10.1006/expr.1995.1119
Teo, C., Lim, P., Voon, K., and Mak, J. W. (2017). Detection of dengue viruses and Wolbachia in Aedes aegypti and Aedes albopictus larvae from four urban localities in Kuala Lumpur, Malaysia. Trop. Biomed. 34, 583–597.
Tewari, S. C., Thenmozhi, V., Katholi, C. R., Manavalan, R., Munirathinam, A., and Gajanana, A. (2004). Dengue vector prevalence and virus infection in a rural area in south India. Trop. Med. Int. Health 9, 499–507. doi: 10.1111/j.1365-3156.2004.01103.x
Thenmozhi, V., Hiriyan, J. G., Tewari, S. C., Philip Samuel, P., Paramasivan, R., Rajendran, R., et al. (2007). Natural vertical transmission of dengue virus in Aedes albopictus (Diptera: Culicidae) in Kerala, a southern Indian state. Jpn. J. Infect. Dis. 60, 245–249.
Turley, A. P., Moreira, L. A., O’Neill, S. L., and McGraw, E. A. (2009). Wolbachia infection reduces blood-feeding success in the dengue fever mosquito, Aedes aegypti. PLoS Negl. Trop. Dis. 3:e516. doi: 10.1371/journal.pntd.0000516
Utarini, A., Indriani, C., Ahmad, R. A., Tantowijoyo, W., Arguni, E., Ansari, M. R., et al. (2021). Efficacy of Wolbachia-Infected Mosquito Deployments for the Control of Dengue. N. Engl. J. Med. 384, 2177–2186. doi: 10.1056/NEJMoa2030243
Walker, T., Johnson, P. H., Moreira, L. A., Iturbe-Ormaetxe, I., Frentiu, F. D., McMeniman, C. J., et al. (2011). The wMel Wolbachia strain blocks dengue and invades caged Aedes aegypti populations. Nature 476, 450–453.
Weinert, L. A., Araujo-Jnr, E. V., Ahmed, M. Z., and Welch, J. J. (2015). The incidence of bacterial endosymbionts in terrestrial arthropods. Proc. Biol. Sci. 282:20150249. doi: 10.1098/rspb.2015.0249
Werren, J. H., Baldo, L., and Clark, M. E. (2008). Wolbachia: Master manipulators of invertebrate biology. Nat. Rev. Microbiol. 6, 741–751. doi: 10.1038/nrmicro1969
Wilder-Smith, A., Ooi, E. E., Vasudevan, S. G., and Gubler, D. J. (2010). Update on dengue: Epidemiology, virus evolution, antiviral drugs, and vaccine development. Curr. Infect. Dis. Rep. 12, 157–164. doi: 10.1007/s11908-010-0102-7
World Health Organization [WHO] (1981). Instructions For Determining The Susceptibility Or Resistance Of Mosquito Larvae To Insecticides. WHO/VBC/81.8076p. Geneva: World Health Organization.
World Health Organization [WHO] (2005). Guidelines For Laboratory And Field Testing Of Mosquito Larvicides. WHO/CDS/WHOPES/GCDPP/2005.1339p. Geneva: World Health Organization.
World Health Organization [WHO] (2016). Test Procedures For Insecticide Resistance Monitoring In Malaria Vector Mosquitoes, 2nd Edn. Geneva: World Health Organization.
World Health Organization [WHO] (2022). Dengue and Severe Dengue. Geneva: World Health Organization.
World Mosquito Program [WMP] (2017). Lab Entomology. SOP on Wolbachia colony maintenance. Colombo: World Mosquito Program.
World Mosquito Program [WMP] (2018a). Mosquito Production. SOP on Blood Feeding Using Beewax Pots. Colombo: World Mosquito Program.
World Mosquito Program [WMP] (2018b). Diagnostics. SOP on Screening Wolbachia (wMel and wAlbB) in adult mosquitoes using triplex qPCR. Colombo: World Mosquito Program.
World Mosquito Program [WMP] (2019). Mosquito Production. SOP on testing Maternal inheritance, Cytoplasmic incompatibility, Fertility and Fecundity. Colombo: World Mosquito Program.
World Mosquito Program [WMP] (2022). Protecting Communities From Disease. Available Online at: https://www.worldmosquitoprogram.org/ (accessed April 15, 2022).
Xi, Z., Khoo, C. C., and Dobson, S. L. (2005). Wolbachia establishment and invasion in an Aedes aegypti laboratory population. Science 310, 326–328. doi: 10.1126/science.1117607
Xu, G., Dong, H., Shi, N., Liu, S., Zhou, A., Cheng, Z., et al. (2007). An outbreak of dengue virus serotype 1 infection in Cixi, Ningbo, People’s Republic of China, 2004, associated with a traveler from Thailand and high density of Aedes albopictus. Am. J. Trop. Med. Hyg. 76, 1182–1188.
Xue, R. D., Barnard, D. R., and Muller, G. C. (2010). Effects of body size and nutritional regimen on survival in adult Aedes albopictus (Diptera: Culicidae). J. Med. Entomol. 47, 778–782. doi: 10.1603/me09222
Keywords: Wolbachia, wMel, wAlbB, fitness cost, dengue, Aedes aegypti
Citation: Sadanandane C, Gunasekaran K, Panneer D, Subbarao SK, Rahi M, Vijayakumar B, Athithan V, Sakthivel A, Dinesh S and Jambulingam P (2022) Studies on the fitness characteristics of wMel- and wAlbB-introgressed Aedes aegypti (Pud) lines in comparison with wMel- and wAlbB-transinfected Aedes aegypti (Aus) and wild-type Aedes aegypti (Pud) lines. Front. Microbiol. 13:947857. doi: 10.3389/fmicb.2022.947857
Received: 19 May 2022; Accepted: 11 July 2022;
Published: 05 August 2022.
Edited by:
Antonios Alekos Augustinos, Hellenic Agricultural Organization “DEMETER,” GreeceReviewed by:
Danilo Carvalho, International Atomic Energy Agency, AustriaKaterina Nikolouli, International Atomic Energy Agency, Austria
Copyright © 2022 Sadanandane, Gunasekaran, Panneer, Subbarao, Rahi, Vijayakumar, Athithan, Sakthivel, Dinesh and Jambulingam. This is an open-access article distributed under the terms of the Creative Commons Attribution License (CC BY). The use, distribution or reproduction in other forums is permitted, provided the original author(s) and the copyright owner(s) are credited and that the original publication in this journal is cited, in accordance with accepted academic practice. No use, distribution or reproduction is permitted which does not comply with these terms.
*Correspondence: Candasamy Sadanandane, cs_anandane@yahoo.com; Manju Rahi, drmanjurahi@gmail.com