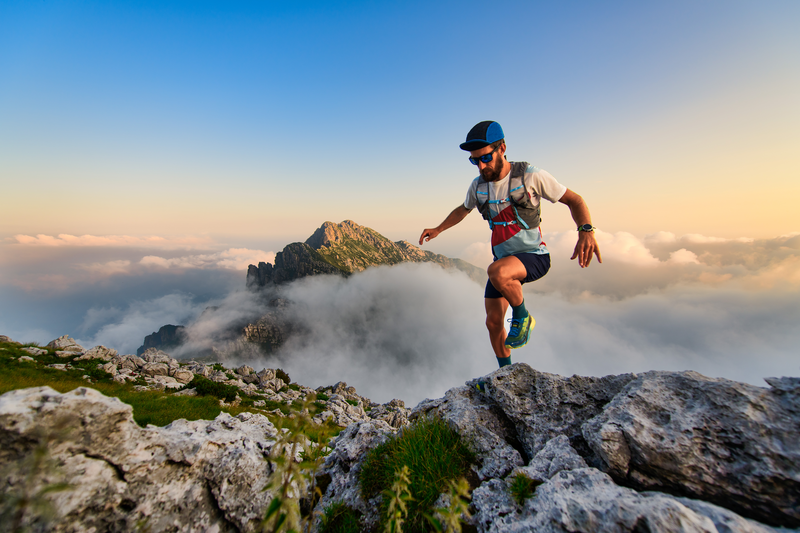
95% of researchers rate our articles as excellent or good
Learn more about the work of our research integrity team to safeguard the quality of each article we publish.
Find out more
ORIGINAL RESEARCH article
Front. Microbiol. , 06 May 2022
Sec. Antimicrobials, Resistance and Chemotherapy
Volume 13 - 2022 | https://doi.org/10.3389/fmicb.2022.858821
This article is part of the Research Topic Antibiotic Resistance in Aquatic Systems, Volume II View all 7 articles
The rapid and ongoing spread of carbapenemase-producing Enterobacteriaceae has led to a global health threat. However, a limited number of studies have addressed this problem in the marine environment. We investigated their emergence in the coastal waters of the central Adriatic Sea (Croatia), which are recipients of submarine effluents from two wastewater treatment plants. Fifteen KPC-producing Enterobacteriaceae (nine Escherichia coli, four Klebsiella pneumoniae and two Citrobacter freundii) were recovered, and susceptibility testing to 14 antimicrobials from 10 classes showed that four isolates were extensively drug resistant (XDR) and two were resistant to colistin. After ERIC and BOX-PCR typing, eight isolates were selected for whole genome sequencing. The E. coli isolates belonged to serotype O21:H27 and sequence type (ST) 2795, while K. pneumoniae isolates were assigned to STs 37 and 534. Large-scale genome analysis revealed an arsenal of 137 genes conferring resistance to 19 antimicrobial drug classes, 35 genes associated with virulence, and 20 plasmid replicons. The isolates simultaneously carried 43–90 genes encoding for antibiotic resistance, while four isolates co-harbored carbapenemase genes blaKPC-2 and blaOXA-48. The blaOXA-48 was associated with IncL-type plasmids in E. coli and K. pneumoniae. Importantly, the blaKPC-2 in four E. coli isolates was located on ~40 kb IncP6 broad-host-range plasmids which recently emerged as blaKPC-2 vesicles, providing first report of these blaKPC-2-bearing resistance plasmids circulating in E. coli in Europe. This study also represents the first evidence of XDR and potentially virulent strains of KPC-producing E. coli in coastal waters and the co-occurrence of blaKPC-2 and blaOXA-48 carbapenemase genes in this species. The leakage of these strains through submarine effluents into coastal waters is of concern, indicating a reservoir of this infectious threat in the marine environment.
Antibiotic resistance is one of the greatest threats to global health nowadays, leading to the higher mortality rates and increased economic costs (Pulingam et al., 2021). The natural environment has been recognized as one of its major reservoirs (Amarasiri et al., 2020), as antibiotic-resistant human pathogens have been detected in coastal marine areas (Šamanić et al., 2021), rivers (Ekwanzala et al., 2020), lakes (Su et al., 2020) and shellfish (Maravić et al., 2013). Effluents from the wastewater treatment plants (WWTPs) have been evidenced as particularly significant routes for dissemination of antibiotic resistance in the natural environment (Ekwanzala et al., 2020), especially the hospital effluents through which the emerging opportunistic pathogens directly enter from the hospital to the aquatic environment (Ekwanzala et al., 2019).
In recent decades, the rapid spread of Gram-negative bacteria resistant to the most potent β-lactam antibiotics, the carbapenems, and the continuous emergence of new resistant strains have raised the global alarm. In 2017, the World Health Organization defined priority categories for emerging multidrug-resistant pathogens for which new antimicrobials are urgently needed, with carbapenem-resistant Enterobacteriaceae (CRE) identified as critical (WHO, 2017). Carbapenem resistance in these bacteria arises mainly from the production of carbapenemases, of which KPC, SME, IMI and NMC belonging to Ambler class A, IMP, VIM and NDM metallo-β-lactamases (MBLs) belonging to class B, and OXA-48 and its derivatives belonging to class D have been detected worldwide (Bonomo et al., 2018; Brolund et al., 2019). In Croatia, CRE isolates are being increasingly reported in hospitals, mainly due to the rapid spread of KPC-producing Enterobacteriaceae first in the northwest (Jelić et al., 2016) and later in the southern coastal regions (Bedenić et al., 2021).
Considering the importance of CRE for public health and the One Health approach, we aimed to study for the first time their occurrence in the coastal waters of the eastern Adriatic, focusing on the area influenced by the submarine sewage outlets of two WWTPs, which could serve as potential routes for the introduction of these bacteria into the coastal marine environment. The isolated CRE were analyzed by PCR for the presence of carbapenemase-encoding genes, after which eight KPC-2-producing Enterobacteriaceae isolates were subjected to high-throughput DNA sequencing. We then performed a detailed search of the obtained genome sequences, focusing on the antibiotic resistance genes (ARGs), virulence factors and plasmid replicons. The isolates were also assigned to sequence types (STs) using the multi-locus sequence typing (MLST) scheme and their serotype was determined. This study led to the first identification of potentially virulent CRE in the marine environment in Croatia, evidencing a transmission route through submarine outfalls and a new reservoir of these opportunistic pathogens in Croatia outside hospital settings.
Fifteen Enterobacteriaceae isolates were recovered in June, July, and September 2020 as part of the project aimed to study the impact of treated submarine effluents in the coastal waters of the central Adriatic Sea in Croatia. Details of the sampling procedure and locations have been described previously (Kvesić et al., 2021). Briefly, the study focused on submarine effluents from the two WWTPs, the Katalinića brig and the Stupe-Stobreč, which mechanically treat wastewater from the wider Split area at an average flow rate of 35,000 and 30,000 m3/day, respectively, and discharge it through submarine outfalls into the coastal waters of the Brač and Split channels (Figure 1). While the Stobreč WWTP processes only municipal wastewater, the Katalinića brig WWTP treats municipal wastewater and stormwater runoff. The Katalinića brig WWTP also collects wastewater from the University Hospital Centre Split, the largest medical center in southern Croatia with 1,400 beds serving a population of approximately 500,000, which increases sharply in the summer months during the tourist season. The submarine outfalls of the Katalinića brig (43°29′22.7 ″N, 16°27′11.2 ″E) and the Stobreč WWTP (43°28′53.6 ″N 16°31′04.3 ″E) are located at a depth of 42 and 37 m, respectively.
Figure 1. Sampling sites (represented by squares) at the submarine outfalls of the two WWTPs, central Adriatic Sea, Croatia.
Water samples were collected from the boat using a Niskin sampler, transferred to sterile 1 L bottles, protected from light, and transported to the laboratory for further analysis within 4 h at 4°C.
One hundred milliliters of the water samples were filtered through 0.2 μm pore size MCE membrane filters (GE Healthcare, United Kingdom), which were then placed on CHROMID® Carba agar (bioMérieux, France) and incubated for 48 h. This chromogenic medium selects for the growth of CRE and allows the typical pink to the burgundy appearance of Escherichia coli colonies and blue-green to blue-grey of Klebsiella, Enterobacter, Serratia and Citrobacter spp. Incubation was carried out at 42°C to suppress the growth of autochthonous environmental species that are unable to grow under mesophilic conditions. Based on colony morphology, all putative Enterobacteriaceae isolates were cultivated in pure culture on MacConkey agar (Biolife, Italy) at 37°C for 18 h and identified to species level using MALDI-TOF MS (Microflex LT mass spectrometer and MALDI Biotyper 4.1.80, Bruker Daltonics, Germany).
The isolates were tested for susceptibility to 14 antibiotics using Etest strips (AB Biodisk, Sweden) except for colistin (CL) whose susceptibility was tested using the broth microdilution method. The tests were performed, and the minimum inhibitory concentrations (MICs) were interpreted based on the European Committee on Antimicrobial Susceptibility Testing (EUCAST) guidelines (EUCAST, 2020). The MIC value of CL was recorded as the lowest concentration showing no visually detectable bacterial growth in the 96-well microtiter plates and was the consensus value of the experiment performed in triplicate. The antibiotics tested (except CL) and their maximum concentrations were as follows: piperacillin/tazobactam (TZP, 256 μg), piperacillin (PIP, 256 μg), ceftazidime (CAZ, 256 μg), cefotaxime (CTX, 32 μg), cefepime (FEP, 256 μg), aztreonam (ATM, 256 μg), imipenem (IMP, 32 μg), meropenem (MER, 32 μg), ertapenem (ETP, 32 μg), ciprofloxacin (CIP, 32 μg), gentamicin (GEN, 256 μg), tetracycline (TET, 256 μg), and trimethoprim-sulfamethoxazole (SXT, 1/19 μg). E. coli ATCC 25922 was used as a control. According to Magiorakos et al. (2012), multidrug-resistant (MDR) phenotype was defined as acquired non-susceptibility to at least one antibiotic from three or more classes, while the extensively drug-resistant (XDR) phenotype was designated as non-susceptibility to at least one agent from all but two or fewer antibiotic classes (i.e., remaining susceptible to only one or two categories).
Isolates were further tested for the presence of class C AmpC β-lactamases with AmpC Etest (AB Biodisk) and carbapenemases with MBL Etest (AB Biodisk) and Rapidec Carba NP test (bioMérieux) according to the manufacturer’s instructions. The production of extended-spectrum β-lactamases (ESBLs) was tested using clavulanic acid (CLA) combination discs. The phenotype consistent with the production of ESBLs was defined by an increase in zone diameter of ≥5 mm for CAZ and/or CTX in combination with CLA compared to its zone when tested alone (EUCAST, 2013).
Genomic DNA was extracted using the NucleoSpin Microbial DNA kit (Macherey-Nagel, United Kingdom) and the concentration and quality of DNA were analyzed using the NanoDrop® Spectrophotometer 1000 (Thermo Scientific, United States). Multiplex PCR assays were performed to screen for the presence of carbapenemase genes encoding class A KPC and class B IMP, VIM and NDM using the primers and PCR conditions described previously (Poirel et al., 2011). Isolates were screened by standard PCR for the presence of the mcr-1 gene, which encodes plasmid-mediated colistin resistance (Liu et al., 2016). The amplified fragments were separated on a 1% (w/v) agarose gel, purified using the ReliaPrep™ DNA Clean-Up and Concentration System (Promega, United States) and subjected to Sanger sequencing of both strands in Macrogen Europe service (Netherlands). The obtained nucleotide sequences were compared with the homologous sequences from the GenBank database using the BLASTn algorithm.1
To exclude the possibility of clonal relatedness between the isolates of the same species, enterobacterial repetitive intergenic consensus (ERIC) and BOX PCR analyses were performed using the primers and conditions previously described (Araújo et al., 2014).
Genomic DNA was sent to Novogene (Cambridge, United Kingdom) for whole genome sequencing (WGS) and bioinformatics analysis of the raw sequencing data. DNA libraries were prepared using the NEBNext® DNA Library Prep Kit (Illumina, United States) and, after a quality check, were subjected to pair-end sequencing on the Illumina NovaSeq 6000 platform with a read length of 150 bp at each end. The obtained reads were subjected to further quality control. Then, the clean reads were mapped to the reference genomes to detect and annotate single nucleotide polymorphism (SNP), structural variants (SV) and copy number variation (CNV) according to the mapping results. FASTQ files containing clean sequences were further analyzed using tools available at the Center for Genomic Epidemiology,2 including multi-locus sequence typing (MLST) with MLST 2.0, sequence type (ST) with SerotypeFinder 2.0, presence of virulence genes (VirulenceFinder 2.0), resistance genes (ResFinder 4.1), and plasmid replicons (PlasmidFinder 2.1). In addition, antibiotic resistance and virulence profiling was performed using the ARESdb cloud platform introduced by Ferreira et al. (2020).3 This involved searching for marker sequences with coverage of ≥60% and identity of ≥90% to those cataloged in ARESdb (Ferreira et al., 2021).
To link carbapenemase KPC-2 and OXA-48 encoding genes to specific Inc. plasmid groups, plasmids were reconstructed with SPAdes v3.13.1 from trimmed (trimmomatic v0.39) sequencing reads, after which the replicon types and resistance markers were cross referenced to the de novo assemblies (Kudirkiene et al., 2018). Plasmid Finder v2.1 determined replicon types from the WGS assemblies generated by SPAdes. Because plasmid reconstruction from short-read sequencing is challenging and assembly typically results in many fragmented contigs per genome of unclear origin, plasmidSPAdes tool was used to identify as much as plasmid contigs. The algorithm in plasmidSPAdes predicted which contigs belong to plasmid DNA and assigned those contigs into components. Components containing specific plasmid replicons and their combinations from a selected strain were further used to search against NCBI nr database using BlastN for the most similar plasmids. De novo assembled plasmids were aligned against ARESdb with thresholds set at >60% query coverage and > 90% alignment identity to detect resistance markers. Further analysis of the generated assemblies was conducted using the Proksee server to create circular alignments of the reads to the reference plasmids available in the NCBI database.4
This study investigated the emergence and antibiotic resistance of CRE in submarine effluent-receiving coastal waters of central Adriatic to contribute to the global surveillance of these opportunistic pathogens outside of hospital settings.
Twenty-two isolates that exhibited characteristic pink or blue-green colony morphology were recovered on selective CHROMID® Carba agar (bioMérieux). By MALDI-TOF MS, seven isolates were identified as Enterococcus faecium and excluded from further investigation. The remaining 15 isolates belonged to the Enterobacteriaceae family (nine E. coli, four Klebsiella pneumoniae and two Citrobacter freundii) and were obtained from water samples collected in June, July, and September 2020 near the submarine outfall of the Katalinića Brig WWTP. All but one isolate showed resistance to at least one carbapenem antibiotic. Among them, four isolates (one E. coli and three K. pneumoniae) were designated extensively drug resistant (XDR), while nine isolates (seven E. coli, one K. pneumoniae and one C. freundii) were multidrug resistant (MDR). Although two K. pneumoniae isolates (C1 and C2) were resistant to colistin, the mcr-1 gene was not detected by PCR. The detailed antibiotic resistance profiles of the Enterobacteriaceae isolates are shown in Table 1.
Table 1. Antibiotic resistance profiles of 15 KPC-producing Enterobacteriaceae isolates recovered in this studya.
The Rapidec Carba NP test indicated carbapenemase production in all 15 isolates. PCR screening and Sanger sequencing further confirmed the presence of the carbapenemase gene blaKPC-2 in all but one C. freundii isolate (CF2), which carried blaKPC-29. This carbapenemase gene is derived from the ancestral allele blaKPC-3, and its expression does not affect the activity of carbapenems (Hobson et al., 2020). However, the CF2 isolate remained sensitive to all beta-lactam antibiotics tested, including cephalosporins (Table 1), casting doubt on the full expression of this gene in this isolate.
Based on the ERIC and BOX profiles (Supplemental Figure S1), eight isolates (four E. coli, three K. pneumoniae, and one C. freundii) that exhibited the most diverse profiles were subjected to WGS.
The draft genome sizes of the isolates ranged from 5.1 to 5.8 Mb, with diverse sizes of N50, and numbers of coding sequences and contigs (Table 2). A total of 137 genes were identified mediating intrinsic or acquired resistance to 19 antimicrobial drug classes, including penicillins, cephamycins, cephalosporins, carbapenems, penems, monobactams, fluoroquinolones, aminoglycosides, macrolides, phenicols, quinolones, sulfonamides, trimethoprim, rifampicin, tetracyclines, fosfomycin, nitroimidazoles, peptides, and aminocoumarin (Supplemental Table S1). The isolates were found to harbor between 43 and 90 gene markers associated with resistance phenotypes, with the highest number detected in E. coli genomes (Table 3). Most of these genes were associated with intrinsic resistance mechanisms such as regulation and transport by resistance-nodulation-cell division (RND) and major facilitator superfamily (MFS) antibiotic efflux pumps or porin uptake (Supplemental Table S1). Moreover, the isolates harbored three to nine bla genes, out of which four isolates (E. coli M12, M14, and M20 and K. pneumoniae C2) simultaneously possessed two carbapenemase-encoding genes, blaKPC-2 and blaOXA-48. In addition, eight sequenced genomes possessed a total of 20 resistance-associated plasmid replicons and 35 genes involved in bacterial virulence (Table 3; Supplemental Table S1).
The four blaKPC-carrying E. coli isolates subjected to WGS were all of serotype O21:H27 and ST2795. Furthermore, the isolates shared a set of 26–30 virulence-related genes (Table 3), including those encoding the outer membrane usher protein (FimD), flagellar biosynthesis protein (FlhA), and the locus of enterocyte effacement (LEE) encoding the type III secretion system effector protein (EspX1). A number of other genes involved in pathogenicity were discovered as well, including glutamate decarboxylase (gad), long polar fimbriae (lpfA), tellurium resistance protein (terC), toxin-antitoxin systems (yafQ, pemK, pemI), type 1 fimbriae (S - fimbrial adhesion minor subunit; genes sfaH and sfaG), small toxic polypeptide (ldrD), polyamine transport protein D (potD), flagellar fli genes, laminin-binding fimbriae (elfG) and carbon starvation protein A (cstA), pointing to the virulence potential of these isolates.
The further similarity between these strains was observed in their plasmid replicon content, with Inc replicons of plasmids FIB(K) and P6 detected in all four strains. Nevertheless, each strain exhibited a unique plasmid replicon pattern, comprising 6–10 replicon types per genome (Table 3). More diversity was observed among the ARGs, of which strains M12, M14, M17, and M20 possessed a total of 90, 79, 82, and 79 genes associated with the regulation or acquisition of antibiotic resistance (Table 3; Supplemental Table S1). Nine bla genes were identified, including the carbapenemase encoding genes blaKPC-2 and blaOXA-48, and ESBL genes blaGES-1, blaGES-2, blaOXA-2, blaOXA-10, and blaCTX-M-3. Three strains (M12, M14 and M20) co-harbored blaKPC-2 and blaOXA-48. Among others, ARGs mediating resistance to trimethoprim (dfrA14), quinolones (qnrVC4, qnrS1, qnrB6), aminoglycosides (ant(3″)-Ii-aac(6′)-IId, ant(3″)-Ia, aph(3″)-Ib) and sulphonamide (sul1, sul2) were continuously detected.
Moreover, further analysis of the genomes identified the blaKPC-2 gene in IncP6 plasmid contigs of 38,767, 14,644, 25,016, and 14,644 bp in E. coli M12, M14, M17, and M20, respectively.
BlastN search against NCBI nr database revealed that a 38,767-bp contig from E. coli M12 had query coverage of 91% and nucleotide identity of 99.75%, 99.75%, and 99.68% with IncP6 plasmids deposited in GenBank: p121SC21-KPC2 from Spanish wastewater C. freundii (Genbank accession no. LT992437; Yao et al., 2017), pKOX3-P5- KPC from a clinical Klebsiella oxytoca in China (GenBank accession no. KY913901; Wang et al., 2017), and pWW14A-KPC2 from wastewater Klebsiella quasipneumoniae in Argentina (Ghiglione et al., 2021). Hybrid plasmids pM12-KPC2, pM14-KPC2, pM17-KPC2, and pM20-KPC2 were reconstructed to a size of ~40 kb and compared to plasmids of both environmental and clinical origin previously reported in the literature (Dai et al., 2016; Wang et al., 2017; Yao et al., 2017; Pérez-Vazquez et al., 2019; Ghiglione et al., 2021; Figure 2). Analysis of the genetic environment of blaKPC-2 revealed that this gene is located within a ΔISKpn6/blaKPC-2-ΔblaTEM-1-ISKpn27 sequence within a Tn3-based transposon interrupted by an ISApu-flanked element (Figure 2), consistent with previous reports (Dai et al., 2016; Ghiglione et al., 2021).
Figure 2. Comparison of plasmids pM12-KPC2, pM14-KPC2, pM17-KPC2, and pM20-KPC2 with IncP6 blaKPC-2-bearing plasmids of environmental and clinical origin described in the literature: pCF121SC21-KPC2 from Spanish wastewater Citrobacter freundii (Genbank accession no. LT992437; Yao et al., 2017), pKOX3-P5-KPC from clinical Klebsiella oxytoca in China (GenBank accession no. KY913901; Wang et al., 2017), pWW14A-KPC2 from wastewater Klebsiella quasipneumoniae in Argentina (GenBank accession no. CP080103; Ghiglione et al., 2021), pWW19C-KPC2 from wastewater Enterobacter asburiae in Argentina (GenBank accession no. CP080110; Ghiglione et al., 2021), p10265-KPC from clinical Pseudomonas aeruginosa in China (GenBank accession no. KU578314; Dai et al., 2016), and pKPC-cd17 from Aeromonas sp. from hospital environment in United States (GenBank accession no. CP026224) which is 100% identical to the blaKPC-2-carrying plasmid in Klebsiella oxytoca from Spanish hospitals (Pérez-Vazquez et al., 2019). The plasmid pCF121SC21-KPC2 was taken as a reference plasmid (black circle).
The blaOXA-48 was associated with IncL-like plasmids in E. coli, but the hybrid blaOXA-48-bearing plasmids could not be reconstituted (the contigs containing this carbapenemase gene were 2,231-bp long in all four OXA-48-positive E. coli and K. pneumoniae isolates). However, the IS1R element flanking the OXA-48-encoding gene was detected in E. coli M12 isolate.
Three KPC-2-producing K. pneumoniae isolates concurrently carried a total of 62, 58, and 62 genes mediating resistance to multiple antibiotics, respectively (Table 3; Supplemental Table S1). Isolates 5a and M11 were affiliated to ST37, while C2 belonged to ST534. Moreover, isolates 5a and M11 exhibited the same antibiogram and XDR phenotype (Table 1), and a similar ARGs content (Table 3; Supplemental Table S1). However, ERIC and BOX-PCR typing excluded their clonality, and WGS data analysis revealed that these differed by the aac(6′)-Ib-cr gene, conferring fluoroquinolone and aminoglycoside resistance, which was found in the genome of strain 5a and not M11. On the other hand, K. pneumoniae C2 harbored less ARGs than the former two strains but was resistant to 13 out of 14 tested antimicrobial drugs, remaining susceptible only to trimethoprim-sulfamethoxazole. Nevertheless, identification of sul1 gene in its genome could eventually result in nonsusceptibility even to this antibiotic. Unfortunately, we were not able to reconstruct blaKPC-2-and blaOXA-48-bearing plasmids in K. pneumoniae isolates due to the short-read genome sequences.
Moreover, some virulence-related genes have been concurrently detected in all three K. pneumoniae genomes (Table 3), codifying for type 1 fimbriae (fimD, sfaG), ferrous ion transport (feoB), ethanolamine-ammonia lyase (eutB), extracellular matrix production (sinR), flagellae (fliY), polyamine transport (potD), and nickel and cobalt resistance (cnrA), respectively. The C2 strain harbored additional three virulence genes encoding the toxin-antitoxin system (pemK, pemI) and carbon starvation protein A (cstA; Table 3).
KPC-2-producing C. freundii CF1 belonged to ST128 (Table 3). Compared to E. coli and K. pneumoniae isolates, this strain showed the least diversity of virulence markers, mainly harboring the genes encoding the flagellar apparatus (fli), polyamine transport protein D (potD), ethanolamine ammonia lyase (eutB) and carbon starvation protein A (cstA; Table 3). Furthermore, this strain contained the fewest ARGs, 43 in total (Table 3; Supplemental Table S1), and was sensitive to all tested antimicrobial agents (Table 1).
All four blaKPC-carrying E. coli isolates that were subjected to WGS belonged to the serotype O21:H27 and ST2795, which was previously identified in the United Kingdom.5 The strains carried several genes involved in pathogenicity or other function. For instance, we identified genes encoding the outer membrane usher protein FimD and flagellar biosynthesis protein FlhA associated with the urinary pathogenic E. coli (UPEC), and the effector protein EspX1 of the type III secretion system common to enterohemorrhagic E. coli (EHEC), all of which were previously detected in wastewater from WWTPs (Zhi et al., 2019). Furthermore, gad gene, also detected in all four E. coli genomes, is commonly involved in resistance to gastric acid, allowing E. coli to survive in the acidic host environment (Mates et al., 2007). The lpfA gene, encoding for the long polar fimbriae, was found to be associated with the gut colonization and the attachment to Peyer’s patches in mice (Cordonnier et al., 2017), and was identified in adherent-invasive E. coli enrolled in the pathogenesis of Crohn’s disease (Chassaing et al., 2011). Both genes were also identified in the KPC-producing E. coli from the riverine environments (Bleichenbacher et al., 2020). Moreover, terC gene, encoding the heavy metal resistance, is found to be significantly correlated with the presence of other virulence factors in the pathogenic strains of E. coli isolated from humans, animals, and food (Orth et al., 2006).
The PemK/pemI type II toxin-antitoxin system was also detected in the genomes of blaKPC-positive E. coli isolates from this study. This module is consisted of a stable toxin and an unstable antitoxin that degrades under stress conditions, enabling the toxin to inhibit the basic cellular processes. Notably, it has been associated with the bacterial persistence in inhospitable conditions, phage inhibition and biofilm formation (Ramage et al., 2009; Hernandez-Ramirez et al., 2017), as well as the IncF plasmid maintenance, conjugation, and spreading (Walling and Butler, 2016; Diaz-Orejas et al., 2017). This system was previously identified in hypermucoviscous carbapenem-resistant E. coli within and outside the hospital environment (Woodford et al., 2009; Mathers et al., 2015; Zurfluh et al., 2018).
Moreover, total of nine carbapenem-resistant E. coli isolates from this study were found to harbor blaKPC-2 gene. It is important to note that the environmental E. coli carrying this carbapenemase gene have rarely been reported, and they have all been recovered from the river water (Poirel et al., 2012; Xu et al., 2015; Yang et al., 2017). To the best of our knowledge, this is the first identification of KPC-producing E. coli in coastal marine waters. More importantly, the co-occurrence of blaKPC-2 and blaOXA-48 in E. coli has not been previously reported in the literature. So far, the blaKPC gene in E. coli has been mainly reported in countries with a high prevalence of KPC-producing K. pneumoniae, indicating the possibility of interspecies gene transfer with K. pneumoniae serving as a blaKPC reservoir (Grundmann et al., 2017). In this regard, we should take into consideration a high prevalence of KPC-producing K. pneumoniae in University Hospital Split (Bedenić et al., 2021) and the fact that the isolates were recovered from the water samples collected near the submarine outfall of the WWTP that treats the hospital wastewater. Nevertheless, this presumption should be carefully addressed in future research, focusing on the genetic environment of the hospital KPC-producing strains. To the best of our knowledge, there are no available data on KPC-producing E. coli or K. pneumoniae from the University Hospital Split analyzed by WGS. The molecular characterization of plasmids harbouring blaKPC-2 gene in Croatia was performed for clinical K. pneumoniae, including those from University Hospital Split that were found to carry this gene on IncFII plasmids (Jelić et al., 2016; Bedenić et al., 2021), or those untyped by PCR-based replicon typing (PBRT; D’Onofrio et al., 2020), as well as in case of river K. pneumoniae that harboured blaKPC-2 gene on IncFII plasmids (Jelić et al., 2019). Notably, blaKPC-2 −bearing IncP6 plasmids were not previously reported in Croatia.
Moreover, all four blaKPC-2 −bearing plasmids from E. coli isolates in this study were of ~40 kb and highly similar to the IncP6 blaKPC-2-containing plasmids from wastewater C. freundii in Spain (Yao et al., 2017), clinical K. oxytoca in China (Wang et al., 2017) and wastewater K. quasipneumoniae in Argentina (Ghiglione et al., 2021), pointing to their global circulation. In comparison to plasmids from other incompatibility (Inc) groups, the KPC-2-encoding gene has been rarely detected in IncP6 resistance plasmids (Yao et al., 2017). However, recent studies confirmed that emergence of blaKPC-2 gene on mobilizable IncP6 broad-host-range plasmids enhanced its dissemination among different members of Enterobacteriaceae in clinical settings and the environment (Pérez-Vazquez et al., 2019; Ghiglione et al., 2021). This study provides further evidence to this speculation, documenting for the first time the blaKPC-2 association with IncP6 plasmids in E. coli in Europe.
Furthermore, 6–10 plasmid replicons were detected in E. coli genomes. Among them, IncN and Col-type replicons have been previously associated with the occurrence of blaKPC in human E. coli from the global surveillance studies (Stoesser et al., 2017). In addition, three out of four analysed E. coli genomes contained the blaOXA-48 carbapenemase gene associated with IncL plasmids. Notably, OXA-48-producing Enterobacteriaceae have widely disseminated in Croatian hospitals over the past years (Bedenić et al., 2018), with OXA-48-positive K. pneumoniae reported at different wards in University Hospital Split. Since IncL-like plasmids were found to enable the transferability of blaOXA-48 in E. coli strains from northern Croatia (Bedenić et al., 2018; Drenjančević et al., 2019), findings from this study further enhance their relevance as reservoirs of blaOXA-48 in Croatia.
Moreover, Ambler class A GES-type ESBLs which were identified in KPC-2-producing E. coli, including GES-1 (isolates M14 and M20) and GES-2 (isolate M12) may have additionally enhanced their nonsusceptibility to beta-lactams as these enzymes effectively hydrolyse penicillins and expanded-spectrum cephalosporins (Castanheira et al., 2021). GES-2, in comparison to GES-1, also displayes hydrolytic activity against imipenem (Poirel et al., 2001).
In addition, other resistance determinants identified in the genome of our E. coli isolates, including the aminoglycoside resistance gene aph(3″)-Ib, trimethoprim resistance gene drfA17 and sulfonamide resistance gene sul2, were previously identified in clinical E. coli from Croatia (Bedenić et al., 2018).
In this study, we found that two blaKPC-2-positive isolates (5a and M11) belong to ST37. Notably, MDR K. pneumoniae strains of the same lineage, bearing the blaKPC-2 (Bedenić et al., 2012) and blaOXA-48 (Jelić et al., 2018) carbapenemase genes were previously reported in Croatian hospitals, but not in the natural environment. KPC-producing K. pneumoniae of other STs were previously isolated from aquatic environments (Ekwanzala et al., 2019), including ST258 in river water in Croatia (Jelić et al., 2019). Therefore, this study reports the first identification of KPC-producing K. pneumoniae in the marine environment in Croatia. Both of K. pneumoniae ST37 isolates in this study harbored replicons of plasmids known to enable the spread of ARGs in Enterobacteriaceae. Namely, multiple IncF replicons (FIIK, FIB, FIA, and/or FII) were previously identified in K. pneumoniae and other Enterobacteriaceae (Carattoli, 2009; Huang et al., 2012). On the other hand, K. pneumoniae strain C2 was affiliated to ST534, which was previously detected in the hospital environment in Israel (Adler et al., 2015). Our strain additionally harbored IncL and IncR-type plasmid replicons which have been previously described as vehicles of blaKPC-2 (Garbari et al., 2015) as well as blaOXA-48 in Croatia (Bedenić et al., 2018; Drenjančević et al., 2019).
K. pneumoniae 51, M11, and C2 isolates produced the Ambler class A GES-5 variant, which confers low carbapenemase activity in addition to penicillins and cephalosporins (Gomi et al., 2018; Castanheira et al., 2021), although GES-5-positive isolates with elevated MICs for imipenem, meropenem, and ertapenem have also been reported (Literacka et al., 2020). In this regard, production of GES-5 may have increased the resistance to carbapenems and other beta-lactams in our isolates. It should be noted that the epidemiology of GES producers is poorly understood, as GES carbapenemase-producing Enterobacteriaceae often stay unreported by resulting falsely negative in the Carba NP test due to the relatively weak activity toward carbapenems (Gomi et al., 2018; Literacka et al., 2020). Nevertheless, hospital outbreaks due to carbapenem-resistant GES-5-positive K. pneumoniae have recently been reported in Portugal (Mendes et al., 2022) and Poland (Literacka et al., 2020), highlighting their clinical relevance. Apart from the carbapenemases, changes in membrane permeability and activity of membrane efflux pumps may have also contributed to carbapenem resistance in K. pneumoniae isolates from this study. Namely, it was observed that a mutant ompK36 porin gene, like the one detected in these three carbapenemase-producing K. pneumoniae isolates, increases nonsusceptibility to this group of antibiotics (Wong et al., 2019). In addition, 7 mutations (P161R, G164A, F172S, R173G, L195V, F197I, and K201M) detected in transcriptional regulator gene acrR in C2 isolate were previously shown to highly increase the expression of a major multidrug efflux pump AcrAB-TolC (Sato et al., 2020) that effectively extrude multiple antimicrobials among which carbapenems (Chetri et al., 2019).
Moreover, the environmental K. pneumoniae isolates from our study shared similar determinants of resistance to other classes of antibiotics, which were previously described in K. pneumoniae clinical isolates from Croatia (Bedenić et al., 2018), such as the aminoglycoside and fluoroquinolone resistance gene aac(6’)Ib-cr, disinfectant resistance genes oqxA and oqxB, sulfonamide resistance gene sul1, fosA encoding fosfomycin resistance and ESBL gene blaCTX-M. Moreover, an amino acid substitution R256G was detected in PmrB protein sequence in 5a and M11 strains, which has been previously associated with colistin resistance in Enterobacteriaceae (Cheng et al., 2015), as well as in case of CL-resistant and carbapenemase-producing hospital K. pneumoniae in Croatia (D’Onofrio et al., 2020). Namely, variations in the PmrB protein, which is a part of the two-component regulatory system PmrA/PmrB enrolled in modification of lipopolysaccharide (LPS) structure, lead to the neutralization of its negative charge and consequently, the reduced susceptibility to cationic peptide antibiotics such as CL. However, as no increased MIC for CL was observed in these isolates, we speculate that the combined action of multiple mechanisms is likely needed to induce resistance to this antibiotic (Cheng et al., 2015). On the other hand, K. pneumoniae C2 was resistant to CL (MIC 32 μg/ml), yet no variations in PmrB were found. Limited number of studies have pointed to the underestimated role of the energy-driven efflux pump of peptide antibiotics in K. pneumoniae, involving two pumps, AcrAB-TolC and KpnEF (Binsker et al., 2021). It was observed that AcrR deficient mutant strains can successfully extrude polymyxin B, another peptide antibiotic, out of the cell using AcrAB-TolC pump (Padilla et al., 2010). More recent study of Naha et al. (2020) revealed that nonmutated and increasingly expressed RamA, a positive regulator of AcrAB-TolC pump, mediates alterations of LPS which along with the upregulation of the pump have contributed to the CL-resistant phenotype in clinical K. pneumoniae. RamA-mediated changes of lipid A moiety have been previously shown to decrease susceptibility to CL in this pathogen (De Majumdar et al., 2015). Therefore, it is likely that a functional AcrAB-TolC system and RamA could also be involved in nonsuceptibility to CL in K. pneumoniae C2, but this should be addressed more carefully in the future research.
Moreover, several common virulence-related genes were simultaneously detected in all three K. pneumoniae genomes, among which those coding the type 1 fimbriae (fimD, sfaG) and ferrous ion transport (feoB). According to Struve et al. (2008) type 1 fimbriae are significantly enrolled in K. pneumoniae infections of urinary tract. The FeoB is the component of the major prokaryotic ferrous ion transport (Feo) system, and the main protein enabling the iron uptake through the lipid bilayer in almost all bacteria (Cartron et al., 2006; Lau et al., 2007). Nevertheless, in this study it was only detected in K. pneumoniae, which could be explained by the fact that a single species can adjust its iron import depending on the type of infection (acute or chronic) and the availability of iron in its environment (Cornelis and Dingemans, 2013). On the other hand, the C2 isolate, like E. coli, additionally harbored two virulence genes (pemK, and pemI) encoding the PemK/PemI type II toxin-antitoxin system that has been previously described in hypermucoviscous carbapenem-resistant K. pneumoniae (Fu et al., 2018; Bleriot et al., 2020).
C. freundii CF1 isolate was affiliated to ST128, which was first described by Bonnin et al. (2020) in an isolate from the rectal swab of a French patient. To date, KPC-producing Citrobacter spp. have been isolated from hospital effluents (Zhang et al., 2012), river sediments (Xu et al., 2018), and the recreational areas (Montezzi et al., 2015), but none of them belonged to ST128. To the best of our knowledge, KPC-2-producing C. freundii of the ST128 lineage has not been previously reported. It was unexpected that this carbapenem-sensitive isolate resulted positive by CarbaNP test. However, although rare, there are previous reports of KPC-producing Enterobacterales showing unusual carbapenems susceptibility profile while testing positive by CarbaNP (Shinde et al., 2017; Cury et al., 2020), suggesting low gene expression.
Furthermore, we found that the strain CF1 also carried the blaCMY-159, a variant gene identified only recently (Piotrowska et al., 2019) that encodes for the eponymous AmpC beta-lactamase of the CMY family intrinsic to Citrobacter spp. In a later study, this bla gene was detected in a Citrobacter sp. isolate resistant to cefotaxime, ceftazidime, cefepime and aztreonam, which is similar to the CF1 susceptibility profile. Overall, the majority of the 43 gene markers involved in the antibiotic resistance in this strain was associated with the activity of intrinsic antibiotic efflux including ATP-binding cassette (ABC), RND or MFS pumps. Notably, missense mutations of the AcrAB-TolC efflux pump regulators marR (Y137H) and soxR (T38S) which were detected in this strain were previously found to increase pump expression, leading to the multidrug resistance, among which to beta-lactams and ciprofloxacin (Al-Farsi et al., 2020). Giving the beta-lactam resistance profile of the CF1 isolate (sensitive to carbapenems and cefepime, but resistant to aztreonam and third generation cephalosporins) we can speculate that the mutation-driven expression of the AcrAB-TolC pump did not influence the activity against carbapenems. This would be a case when coincided with the membrane permeability defects resulted from porin loss or porin structural changes (Pages et al., 2008; Vardakas et al., 2012; Sadeghi, 2019), which have not been detected in CF1 isolate.
Moreover, among the 10 virulence-related genes detected in CF1 genome, the major virulence factors of this pathogen such as Shiga-like and heat-stable toxins, or the cholera toxin B subunit homolog (Bai et al., 2012) were not identified, thus we can speculate about the low virulence potential of this isolate (Pepperell et al., 2002).
This study reports the introduction of XDR and carbapenemase-producing potentially virulent strains of Enterobacteriaceae into the Croatian marine environment through the submarine outfall of the treated wastewater located at a depth of 42 m. Among other antibiotic resistance and virulence determinants previously assigned exclusively to clinical strains, we report for the first time KPC-producing E. coli in coastal waters and the co-occurrence of blaKPC-2 and blaOXA-48 carbapenemase genes in this species. While blaOXA-48 was located on an IncL-type plasmids in this species, blaKPC-2 was harbored by recently described broad-host-range IncP6 resistance plasmids, providing first record of their circulation in E. coli and highlighting their importance in the epidemiology of this globally disseminated carbapenemase encoding gene. Leakage of these highly resistant strains into coastal waters through the submarine outlet is of serious concern as it provides a route for their continuous introduction into the marine environment and a reservoir for their further spread.
The genomic sequences are deposited in the NCBI Sequence Read Archive (BioProject number PRJNA768347) under accession numbers listed in Table 2. The sequence of plasmid pM12-KPC2 from E. coli was deposited in GenBank under accession number CP093216.
MK and AM: conceptualization and writing—original draft preparation. MDŽ and IŠ: formal analysis. AN, AM, IGB, and MT: validation. MK and AN: investigation. IŠ, JK and AM: resources and funding acquisition. AM: data curation, visualization, and supervision. All authors writing--review and editing and contributed to the article and approved the submitted version.
This research was funded by the Croatian Science Foundation (grant number UIP-2019-04-9778), project STIM-REI (KK.01.1.1.01.0003) through the European Regional Development Fund—the Operational Programme Competitiveness and Cohesion 2014–2020 (KK.01.1.1.01), Croatian Academy of Sciences and Arts, and the annual funds for institutional financing of scientific activity from Ministry of Science and Education of Republic of Croatia. The project CAAT “Coastal Auto-purification Assessment Technology” funded by European Union from European Structural and Investment Funds 2014–2020 (KK.01.1.1.04.0064).
The authors declare that the research was conducted in the absence of any commercial or financial relationships that could be construed as a potential conflict of interest.
All claims expressed in this article are solely those of the authors and do not necessarily represent those of their affiliated organizations, or those of the publisher, the editors and the reviewers. Any product that may be evaluated in this article, or claim that may be made by its manufacturer, is not guaranteed or endorsed by the publisher.
The authors acknowledge the Public Health Institute in Zadar, Croatia, for sample collection.
The Supplementary Material for this article can be found online at: https://www.frontiersin.org/articles/10.3389/fmicb.2022.858821/full#supplementary-material
Supplementary Table S1 | 480 Molecular characteristics of eight whole-genome sequenced KPC-producing isolates.
Adler, A., Hussein, O., Ben-David, D., Masarwa, S., Navon-Venezia, S., Schwaber, M. J., et al. (2015). Persistence of Klebsiella pneumoniae ST258 as the predominant clone of carbapenemase-producing Enterobacteriaceae in post-acute-care hospitals in Israel, 2008-13. J. Antimicrob. Chemother. 70, 89–92. doi: 10.1093/jac/dku333
Al-Farsi, H. M., Camporeale, A., Ininbergs, K., Al-Azri, S., Al-Muharrmi, Z., Al-Jardani, A., et al. (2020). Clinical and molecular characteristics of carbapenem non-susceptible Escherichia coli: a nationwide survey from Oman. PLoS One 15:e0239924. doi: 10.1371/journal.pone.0239924
Amarasiri, M., Sano, D., and Suzuki, S. (2020). Understanding human health risks caused by antibiotic resistant bacteria (ARB) and antibiotic resistance genes (ARG) in water environments: current knowledge and questions to be answered. Crit. Rev. Environ. Sci. Technol. 50, 2016–2059. doi: 10.1080/10643389.2019.1692611
Araújo, S., Henriques, I. S., Leandro, S. M., Alves, A., Pereira, A., and Correia, A. (2014). Gulls identified as major source of fecal pollution in coastal waters: A microbial source tracking study. Sci. Total Environ. 470-471, 84–91. doi: 10.1016/j.scitotenv.2013.09.075
Bai, L., Xia, S., Lan, R., Liu, L., Ye, C., Wang, Y., et al. (2012). Isolation and characterization of cytotoxic, aggregative Citrobacter freundii. PLoS One 7:e33054. doi: 10.1371/journal.pone.0033054
Bedenić, B., Mazzariol, A., Plečko, V., Bošnjak, Z., Barl, P., Vraneš, J., et al. (2012). First report of KPC-producing Klebsiella pneumoniae in Croatia. J. Chemother. 24, 237–239. doi: 10.1179/1973947812Y.0000000017
Bedenić, B., Sardelić, S., Bogdanić, M., Zarfel, G., Beader, N., Šuto, S., et al. (2021). Klebsiella pneumoniae carbapenemase (KPC) in urinary infection isolates. Arch. Microbiol. 203, 1825–1831. doi: 10.1007/s00203-020-02161-x
Bedenić, B., Slade, M., Starčević, L. Ž., Sardelić, S., Vranić-Ladavac, M., Benčić, A., et al. (2018). Epidemic spread of OXA-48 beta-lactamase in Croatia. J. Med. Microbiol. 67, 1031–1041. doi: 10.1099/jmm.0.000777
Binsker, U., Käsbohrer, A., and Hammerl, J. A. (2021). Global colistin use: a review of the emergence of resistant Enterobacterales and the impact on their genetic basis. FEMS Microbiol. Rev. 46:fuab049. doi: 10.1093/femsre/fuab049
Bleichenbacher, S., Stevens, M. J. A., Zurfluh, K., Perreten, V., Endimiani, A., Stephan, R., et al. (2020). Environmental dissemination of carbapenemase-producing Enterobacteriaceae in rivers in Switzerland. Environ. Pollut. 265:115081. doi: 10.1016/j.envpol.2020.115081
Bleriot, I., Blasco, L., Delgado-Valverde, M., Gual-de-Torrella, A., Ambroa, A., Fernandez-Garcia, L., et al. (2020). Mechanisms of tolerance and resistance to chlorhexidine in clinical strains of Klebsiella pneumoniae producers of carbapenemase: role of new type II toxin-antitoxin system, PemIK. Toxins 12:566. doi: 10.3390/toxins12090566
Bonnin, R. A., Jousset, A. B., Gauthie, R. L., Emeraud, C., Girlich, D., Sauvadet, A., et al. (2020). First occurrence of the OXA-198 carbapenemase in enterobacterales. Antimicrob. Agents Chemother. 64, e01471–e01419. doi: 10.1128/AAC.01471-19
Bonomo, R. A., Burd, E. M., Conly, J., Limbago, B. M., Poirel, L., Segre, J. A., et al. (2018). Carbapenemase-producing organisms: a global scourge. Clin. Infect. Dis. 66, 1290–1297. doi: 10.1093/cid/cix893
Brolund, A., Lagerqvist, N., Byfors, S., Struelens, M. J., Monnet, D. L., Albiger, B., et al. (2019). Worsening epidemiological situation of carbapenemase-producing Enterobacteriaceae in Europe; assessment by national experts from 37 countries. Euro Surveill. 24:1900123. doi: 10.2807/1560-7917.ES.2019.24.9.1900123
Carattoli, A. (2009). Resistance plasmid families in Enterobacteriaceae. Antimicrob. Agents Chemother. 53, 2227–2238. doi: 10.1128/aac.01707-08
Cartron, M. L., Maddocks, S., Gillingham, P., Craven, C. J., and Andrews, S. C. (2006). Feo – transport of ferrous iron into bacteria. Biometals 19, 143–157. doi: 10.1007/s10534-006-0003-2
Castanheira, M., Simner, P. J., and Bradford, P. A. (2021). Extended-spectrum β-lactamases: An update on their characteristics, epidemiology and detection. JAC Antimicrob. Resist. 3:dlab092. doi: 10.1093/jacamr/dlab092
Chassaing, B., Rolhion, N., de Vallée, A., Salim, S. Y., Prorok-Hamon, M., Neut, C., et al. (2011). Crohn disease-associated adherent-invasive E. coli bacteria target mouse and human Peyer's patches via long polar fimbriae. J. Clin. Investig. 121, 966–975. doi: 10.1172/JCI44632
Cheng, Y. H., Lin, T. L., Pan, Y. J., Wang, Y. P., Lin, Y. T., and Wang, J. T. (2015). Colistin resistance mechanisms in Klebsiella pneumoniae strains from Taiwan. Antimicrob. Agents Chemother. 59, 2909–2913. doi: 10.1128/aac.04763-14
Chetri, S., Bhowmik, D., Paul, D., Pandey, P., Chanda, D. D., Chakravarty, A., et al. (2019). AcrAB-TolC efflux pump system plays a role in carbapenem non-susceptibility in Escherichia coli. BMC Microbiol. 19, 210–217. doi: 10.1186/s12866-019-1589-1
Cordonnier, C., Etienne-Mesmin, L., Thévenot, J., Rougeron, A., Renier, S., Chassaing, B., et al. (2017). Enterohemorrhagic Escherichia coli pathogenesis: role of long polar fimbriae in Peyer’s patches interactions. Sci. Rep. 7:44655. doi: 10.1038/srep44655
Cornelis, P., and Dingemans, J. (2013). Pseudomonas aeruginosa adapts its iron uptake strategies in function of the type of infections. Front. Cell. Infect. Microbiol. 3:75. doi: 10.3389/fcimb.2013.00075
Cury, A. P., Girardello, R., da Silva Duarte, A. J., and Rossi, F. (2020). KPC-producing Enterobacterales with uncommon carbapenem susceptibility profile in Vitek 2 system. Int. J. Infect. Dis. 93, 118–120. doi: 10.1016/j.ijid.2020.01.016
D’Onofrio, V., Conzemius, R., Varda-Brkić, D., Bogdan, M., Grisold, A., Gyssens, I. C., et al. (2020). Epidemiology of colistin-resistant, carbapenemase-producing Enterobacteriaceae and Acinetobacter baumannii in Croatia. Infect. Genet. Evol. 81:104263. doi: 10.1016/j.meegid.2020.104263
Dai, X., Zhou, D., Xiong, W., Feng, J., Luo, W., Luo, G., et al. (2016). The IncP-6 plasmid p10265-KPC from Pseudomonas aeruginosa carries a novel DISEc33-associated blaKPC-2 gene cluster. Front. Microbiol. 7:310. doi: 10.3389/fmicb.2016.00310
De Majumdar, S., Yu, J., Fookes, M., McAteer, S. P., Llobet, E., Finn, S., et al. (2015). Elucidation of the RamA regulon in Klebsiella pneumoniae reveals a role in LPS regulation. PLoS Pathog. 11:e1004627. doi: 10.1371/journal.ppat.1004627
Diaz-Orejas, R., Espinosa, M., and Yeo, C. C. (2017). The importance of the expendable: toxin-antitoxin genes in plasmids and chromosomes. Front. Microbiol. 8:1479. doi: 10.3389/fmicb.2017.01479
Drenjančević, D., Presečki-Stanko, A., Kopić, J., Talapko, J., Zarfel, G., and Bedenić, B. (2019). Hidden carbapenem resistance in OXA-48 and extended-spectrum β-lactamase-positive Escherichia coli. Microb. Drug Resist. 25, 696–702. doi: 10.1089/mdr.2018.0309
Ekwanzala, M. D., Dewar, J. B., Kamika, I., and Momba, M. N. B. (2019). Tracking the environmental dissemination of carbapenem-resistant Klebsiella pneumoniae using whole genome sequencing. Sci. Total Environ. 691, 80–92. doi: 10.1016/j.scitotenv.2019.06.533
Ekwanzala, M. D., Dewar, J. B., Kamika, I., and Momba, M. N. B. (2020). Comparative genomics of vancomycin-resistant Enterococcus spp. revealed common resistome determinants from hospital wastewater to aquatic environments. Sci. Total Environ. 719:137275. doi: 10.1016/j.scitotenv.2020.137275
EUCAST (2013). EUCAST guidelines for detection of resistance mechanisms and specific resistances of clinical and/or epidemiological importance. Version 1.0. EUCAST, 2013. Available at: https://www.eucast.org (Accessed 24 June 2020).
EUCAST (2020). Breakpoint Tables for Interpretation of MICs and Zone Diameters. Version 10.0. EUCAST, 2020. Available at: https://www.eucast.org (Accessed 24 June 2020).
Ferreira, I., Beisken, S., Lueftinger, L., Weinmaier, T., Klein, M., Bacher, J., et al. (2020). Species identification and antibiotic resistance prediction by analysis of whole-genome sequence data by use of ARESdb: an analysis of isolates from the Unyvero lower respiratory tract infection trial. J. Clin. Microbiol. 58, e00273–e00220. doi: 10.1128/JCM.00273-20
Ferreira, I., Lepuschitz, S., Beisken, S., Fiume, G., Mrazek, K., Frank, B. J. H., et al. (2021). Culture-free detection of antibiotic resistance markers from native patient samples by hybridization capture sequencing. Microorganisms 9:1672. doi: 10.3390/microorganisms9081672
Fu, L., Tang, L., Wang, S., Liu, Q., Liu, Y., Zhang, Z., et al. (2018). Co-location of the blaKPC-2, blaCTX-M-65, rmtB and virulence relevant factors in an IncFII plasmid from a hypermucoviscous Klebsiella pneumoniae isolate. Microb. Pathog. 124, 301–304. doi: 10.1016/j.micpath.2018.08.055
Garbari, L., Busetti, M., Dolzani, L., Petix, V., Knezevich, A., Bressan, et al. (2015). pKBuS13, a KPC-2-encoding plasmid from Klebsiella pneumoniae sequence type 833, carrying Tn4401b inserted into an Xer site-specific recombination locus. Antimicrob. Agents Chemother. 59, 5226–5231. doi: 10.1128/AAC.04543-14
Ghiglione, B., Haim, M. S., Penzotti, P., Brunetti, F., Di Conza, J., Figueroa-Espinosa, R., et al. (2021). Characterization of emerging pathogens carrying blaKPC-2 gene in IncP-6 plasmids isolated from urban sewage in Argentina. Front. Cell. Infect. Microbiol. 11:722536. doi: 10.3389/fcimb.2021.722536
Gomi, R., Matsuda, T., Yamamoto, M., Chou, P. H., Tanaka, M., Ichiyama, S., et al. (2018). Characteristics of carbapenemase-producing Enterobacteriaceae in wastewater revealed by genomic analysis. Antimicrob. Agents Chemother. 62, e02501–e02517. doi: 10.1128/AAC.02501-17
Grundmann, H., Glasner, C., Albiger, B., Aanensen, D. M., Tomlinson, C. T., Tambić Andrašević, A., et al. (2017). Occurrence of carbapenemase-producing Klebsiella pneumoniae and Escherichia coli in the European survey of carbapenemase-producing Enterobacteriaceae (EuSCAPE): a prospective, multinational study. Lancet Infect. Dis. 17, 153–163. doi: 10.1016/S1473-3099(16)30257-2
Hernandez-Ramirez, K. C., Chavez-Jacobo, V. M., Valle-Maldonado, M. I., Patino-Medina, J. A., Diaz-Perez, S. P., Jacome-Galarza, I. E., et al. (2017). Plasmid pUM505 encodes a toxin-antitoxin system conferring plasmid stability and increased Pseudomonas aeruginosa virulence. Microb. Pathog. 112, 259–268. doi: 10.1016/j.micpath.2017.09.060
Hobson, C. A., Bonacorsi, S., Jacquier, H., Choudhury, A., Magnan, M., Cointe, A., et al. (2020). KPC beta-lactamases are permissive to insertions and deletions conferring substrate spectrum modifications and resistance to ceftazidime-avibactam. Antimicrob. Agents Chemother. 64, e01175–e01120. doi: 10.1128/AAC.01175-20
Huang, X. Z., Frye, J. G., Chahine, M. A., Glenn, L. M., Ake, J. A., Su, W., et al. (2012). Characteristics of plasmids in multi-drug-resistant Enterobacteriaceae isolated during prospective surveillance of a newly opened hospital in Iraq. PLoS One 7:e40360. doi: 10.1371/journal.pone.0040360
Jelić, M., Butić, I., Plecko, V., Cipris, I., Jajić, I., Bejuk, D., et al. (2016). KPC-producing Klebsiella pneumoniae isolates in Croatia: a nationwide survey. Microb. Drug Resist. 22, 662–667. doi: 10.1089/mdr.2015.0150
Jelić, M., Hrenović, J., Dekić, S., Goić-Barišić, I., and Tambić Andrašević, A. (2019). First evidence of KPC-producing ST258 Klebsiella pneumoniae in river water. J. Hosp. Infect. 103, 147–150. doi: 10.1016/j.jhin.2019.04.001
Jelić, M., Škrlin, J., Bejuk, D., Košćak, I., Butić, I., Gužvinec, M., et al. (2018). Characterization of isolates associated with emergence of OXA-48-producing Klebsiella pneumoniae in Croatia. Microb. Drug Resist. 24, 973–979. doi: 10.1089/mdr.2017.0168
Kudirkiene, E., Andoh, L. A., Ahmed, S., Herrero-Fresno, A., Dalsgaard, A., Obiri-Danso, K., et al. (2018). The use of a combined bioinformatics approach to locate antibiotic resistance genes on plasmids from whole genome sequences of Salmonella enterica serovars from humans in Ghana. Front. Microbiol. 9:1010. doi: 10.3389/fmicb.2018.01010
Kvesić, M., Kalinić, H., Dželalija, M., Šamanić, I., Andričević, R., and Maravić, A. (2021). Microbiome and antibiotic resistance profiling in submarine effluent-receiving coastal waters in Croatia. Environ. Pollut. 292:118282. doi: 10.1016/j.envpol.2021.118282
Lau, H. Y., Clegg, S., and Moore, T. A. (2007). Identification of Klebsiella pneumoniae genes uniquely expressed in a strain virulent using a murine model of bacterial pneumonia. Microb. Pathog. 42, 148–155. doi: 10.1016/j.micpath.2007.01.001
Literacka, E., Izdebski, R., Urbanowicz, P., Żabicka, D., Klepacka, J., Sowa-Sierant, et al. (2020). Spread of Klebsiella pneumoniae ST45 producing GES-5 carbapenemase or GES-1 extended-spectrum β-lactamase in newborns and infants. Antimicrob. Agents Chemother. 64, e00595–e00520. doi: 10.1128/AAC.00595-20
Liu, Y. Y., Wang, Y., Walsh, T. R., Yi, L. X., Zhang, R., Spencer, J., et al. (2016). Emergence of plasmid-mediated colistin resistance mechanism MCR-1 in animals and human beings in China: a microbiological and molecular biological study. Lancet Infect. Dis. 16, 161–168. doi: 10.1016/S1473-3099(15)00424-7
Magiorakos, A. P., Srinivasan, A., Carey, R. B., Carmeli, Y., Falagas, M. E., Giske, C. G., et al. (2012). Multidrug-resistant, extensively drug-resistant and pandrug-resistant bacteria: an international expert proposal for interim standard definitions for acquired resistance. Clin. Microbiol. Infect. 18, 268–281. doi: 10.1111/j.1469-0691.2011.03570.x
Maravić, A., Skočibušić, M., Šamanić, I., Fredotović, Ž., Cvjetan, S., Jutronić, M., et al. (2013). Aeromonas spp. simultaneously harbouring blaCTX-M-15, blaSHV-12, blaPER-1 and blaFOX-2 in wild-growing Mediterranean mussel (Mytilus galloprovincialis) from Adriatic Sea; Croatia. Int. J. Food Microbiol. 166, 301–308. doi: 10.1016/j.ijfoodmicro.2013.07.010
Mates, A. K., Sayed, A. K., and Foster, J. W. (2007). Products of the Escherichia coli acid fitness island attenuate metabolite stress at extremely low pH and mediate a cell density-dependent acid resistance. J. Bacteriol. 189, 2759–2768. doi: 10.1128/JB.01490-06
Mathers, A. J., Peirano, G., and Pitout, J. D. D. (2015). Escherichia coli ST131: the quintessential example of an international multiresistant high-risk clone. Adv. Appl. Microbiol. 90, 109–154. doi: 10.1016/bs.aambs.2014.09.002
Mendes, G., Ramalho, J. F., Bruschy-Fonseca, A., Lito, L., Duarte, A., Melo-Cristino, J., et al. (2022). Whole-genome sequencing enables molecular characterization of non-clonal group 258 high-risk clones (ST13, ST17, ST147 and ST307) among Carbapenem-resistant Klebsiella pneumoniae from a tertiary University Hospital Centre in Portugal. Microorganisms 10, 416. doi: 10.3390/microorganisms10020416
Montezzi, L. F., Campana, E. H., Corrêa, L. L., Justo, L. H., Paschoal, R. P., da Silva, I. L., et al. (2015). Occurrence of carbapenemase-producing bacteria in coastal recreational waters. Int. J. Antimicrob. Agents 45, 174–177. doi: 10.1016/j.ijantimicag.2014.10.016
Naha, S., Sands, K., Mukherjee, S., Roy, C., Rameez, M. J., Saha, B., et al. (2020). KPC-2-producing Klebsiella pneumoniae ST147 in a neonatal unit: clonal isolates with differences in colistin susceptibility attributed to AcrAB-TolC pump. Int. J. Antimicrob. Agents 55:105903. doi: 10.1016/j.ijantimicag.2020.105903
Orth, D., Grif, K., Dierich, M. P., and Würzner, R. (2006). Variability in tellurite resistance and the ter gene cluster among Shiga toxin-producing Escherichia coli isolated from humans, animals and food. Res. Microbiol. 158, 105–111. doi: 10.1016/j.resmic.2006.10.007
Padilla, E., Llobet, E., Doménech-Sánchez, A., Martínez-Martínez, L., Bengoechea, J. A., and Albertí, S. (2010). Klebsiella pneumoniae AcrAB efflux pump contributes to antimicrobial resistance and virulence. Antimicrob. Agents Chemother. 54, 177–183. doi: 10.1128/AAC.00715-09
Pages, J. M., James, C. E., and Winterhalter, M. (2008). The porin and the permeating antibiotic: a selective diffusion barrier in Gram-negative bacteria. Nat. Rev. Microbiol. 6, 893–903. doi: 10.1038/nrmicro1994
Pepperell, C., Kus, J. V., Gardam, M. A., Humar, A., and Burrows, L. L. (2002). Low-virulence Citrobacter species encode resistance to multiple antimicrobials. Antimicrob. Agents Chemother. 46, 3555–3560. doi: 10.1128/AAC.46.11.3555-3560.2002
Pérez-Vazquez, M., Oteo-Iglesias, J., Sola-Campoy, P. J., Carrizo-Manzoni, H., Bautista, V., Lara, N., et al. (2019). Characterization of carbapenemase-producing Klebsiella oxytoca in Spain, 2016–2017. Antimicrob. Agents Chemother. 63, e02529–e02518. doi: 10.1128/AAC.02529-18
Piotrowska, M., Kowalska, S., and Popowska, M. (2019). Diversity of β-lactam resistance genes in Gram-negative rods isolated from a municipal wastewater treatment plant. Ann. Microbiol. 69, 591–601. doi: 10.1007/s13213-019-01450-1
Poirel, L., Barbosa-Vasconcelos, A., Simoes, R. R., Da Costa, P. M., Liu, W., and Nordmann, P. (2012). Environmental KPC-producing Escherichia coli isolates in Portugal. Antimicrob. Agents Chemother. 56, 1662–1663. doi: 10.1128/AAC.05850-11
Poirel, L., Walsh, T. R., Cuvillier, V., and Nordmann, P. (2011). Multiplex PCR for detection of acquired carbapenemase genes. Diagn. Microbiol. Infect. Dis. 70, 119–123. doi: 10.1016/j.diagmicrobio.2010.12.002
Poirel, L., Weldhagen, G. F., Naas, T., De Champs, C., Dove, M. G., and Nordmann, P. (2001). GES-2, a class A β-lactamase from Pseudomonas aeruginosa with increased hydrolysis of imipenem. Antimicrob. Agents Chemother. 45, 2598–2603. doi: 10.1128/AAC.45.9.2598-2603.2001
Pulingam, T., Parumasivam, T., Gazzali, A. M., Sulaiman, A. M., Chee, J. Y., Lakshmanan, M., et al. (2021). Antimicrobial resistance: prevalence, economic burden, mechanisms of resistance and strategies to overcome. Eur. J. Pharm. Sci. 170:106103. doi: 10.1016/j.ejps.2021.106103
Ramage, H. R., Connolly, L. E., and Cox, J. S. (2009). Comprehensive functional analysis of mycobacterium tuberculosis toxin-antitoxin systems: implications for pathogenesis, stress responses, and evolution. PLoS Genet. 5:e1000767. doi: 10.1371/journal.pgen.1000767
Sadeghi, M. (2019). Molecular characterization of multidrug-resistant Escherichia coli isolates in Azerbaijan hospitals. Microb. Drug Resist. 25, 1287–1296. doi: 10.1089/mdr.2019.0006
Šamanić, I., Kalinić, H., Fredotović, Ž., Dželalija, M., Bungur, A.-M., and Maravić, A. (2021). Bacteria tolerant to colistin in coastal marine environment: detection; microbiome diversity and antibiotic resistance genes’ repertoire. Chemosphere 281:130945. doi: 10.1016/j.chemosphere.2021.130945
Sato, T., Wada, T., Nishijima, S., Fukushima, Y., Nakajima, C., Suzuki, Y., et al. (2020). Emergence of the novel aminoglycoside Acetyltransferase variant aac (6′)-Ib-D179Y and Acquisition of Colistin Heteroresistance in Carbapenem-resistant Klebsiella pneumoniae due to a disrupting mutation in the DNA repair enzyme MutS. MBio 11, e01954–e01920. doi: 10.1128/mBio.01954-20
Shinde, S., Gupta, R., Raut, S. S., Nataraj, G., and Mehta, P. R. (2017). Carba NP as a simpler, rapid, cost-effective, and a more sensitive alternative to other phenotypic tests for detection of carbapenem resistance in routine diagnostic laboratories. J. Lab. Physicians 9, 100–103. doi: 10.4103/0974-2727.199628
Stoesser, N., Sheppard, A. E., Peirano, G., Anson, L. W., Pankhurst, L., Sebra, R., et al. (2017). Genomic epidemiology of global Klebsiella pneumoniae carbapenemase (KPC)-producing Escherichia coli. Sci. Rep. 7:5917. doi: 10.1038/s41598-017-06256-2
Struve, C., Bojer, M., and Krogfelt, K. A. (2008). Characterization of Klebsiella pneumoniae type 1 fimbriae by detection of phase variation during colonization and infection and impact on virulence. Infect. Immun. 76, 4055–4065. doi: 10.1128/IAI.00494-08
Su, S., Li, C., Yang, J., Xu, Q., Qiu, Z., Xue, B., et al. (2020). Distribution of antibiotic resistance genes in three different natural water bodies-A Lake; river and sea. Int. J. Environ. Res. Publ. Health. 17, 552. doi: 10.3390/ijerph17020552
Vardakas, K. Z., Tansarli, G. S., Rafailidis, P. I., and Falagas, M. E. (2012). Carbapenems versus alternative antibiotics for the treatment of bacteraemia due to Enterobacteriaceae producing extended-spectrum beta-lactamases: a systematic review and meta-analysis. J. Antimicrob. Chemother. 67, 2793–2803. doi: 10.1093/jac/dks301
Walling, L. R., and Butler, J. S. (2016). Structural determinants for antitoxin identity and insulation of cross talk between homologous toxin-antitoxin systems. J. Bacteriol. 198, 3287–3295. doi: 10.1128/JB.00529-16
Wang, J., Yuan, M., Chen, H., Chen, X., Jia, Y., Zhu, X., et al. (2017). First report of Klebsiella oxytoca strain simultaneously producing NDM-1, IMP-4, and KPC-2 Carbapenemases. Antimicrob. Agents Chemother. 61, e00877–e00817. doi: 10.1128/AAC.00877-17
WHO, (2017). Essential medicines and health products: Global priority list of antibiotic-resistant bacteria to guide research, discovery, and development of new antibiotics. Available at: http://www.who.int/medicines/publications/globalpriority-list-antibiotic-resistant-bacteria (Accessed 7 July 2021).
Wong, J. L., Romano, M., Kerry, L. E., Kwong, H. S., Low, W. W., Brett, S. J., et al. (2019). OmpK36-mediated carbapenem resistance attenuates ST258 Klebsiella pneumoniae in vivo. Nat. Commun. 10:3957. doi: 10.1038/s41467-019-11756-y
Woodford, N., Carattoli, A., Karisik, E., Underwood, A., Ellington, M. J., and Livermore, D. M. (2009). Complete nucleotide sequences of plasmids pEK204, pEK499, and pEK516, encoding CTX-M enzymes in three major Escherichia coli lineages from the United Kingdom, all belonging to the international O25:H4-ST131 clone. Antimicrob. Agents Chemother. 53, 4472–4482. doi: 10.1128/AAC.00688-09
Xu, G., Jiang, Y., An, W., Wang, H., and Zhang, X. (2015). Emergence of KPC-2-producing Escherichia coli isolates in an urban river in Harbin, China. World J. Microbiol. Biotechnol. 31, 1443–1450. doi: 10.1007/s11274-015-1897-z
Xu, H., Wang, X., Yu, X., Zhang, J., Guo, L., Huang, C., et al. (2018). First detection and genomics analysis of KPC-2-producing Citrobacter isolates from river sediments. Environ. Pollut. 235, 931–937. doi: 10.1016/j.envpol.2017.12.084
Yang, F., Huang, L., Li, L., Yang, Y., Mao, D., and Luo, Y. (2017). Discharge of KPC-2 genes from the WWTPs contributed to their enriched abundance in the receiving river. Sci. Total Environ. 581-582(581–582), 136–143. doi: 10.1016/j.scitotenv.2016.12.063
Yao, Y., Lazaro-Perona, F., Falgenhauer, L., Valverde, A., Imirzalioglu, C., Dominguez, L., et al. (2017). Insights Into a novel blaKPC-2-encoding IncP-6 plasmid reveal Carbapenem-resistance circulation in several Enterobacteriaceae species From wastewater and a hospital source in Spain. Front. Microbiol. 8:1143. doi: 10.3389/fmicb.2017.01143
Zhang, X., Lu, X., and Zong, Z. (2012). Enterobacteriaceae producing the KPC-2 carbapenemase from hospital sewage. Diagn. Microbiol. Infect. Dis. 73, 204–206. doi: 10.1016/j.diagmicrobio.2012.02.007
Zhi, S., Banting, G., Stothard, P., Ashbolt, N. J., Checkley, S., Meyer, K., et al. (2019). Evidence for the evolution, clonal expansion and global dissemination of water treatment-resistant naturalized strains of Escherichia coli in wastewater. Water Res. 156, 208–222. doi: 10.1016/j.watres.2019.03.024
Zurfluh, K., Stevens, M. J. A., Stephan, R., and Nüesch-Inderbinen, M. (2018). Complete and assembled genome sequence of an NDM-5- and CTX-M-15-producing Escherichia coli sequence type 617 isolated from wastewater in Switzerland. J. Glob. Antimicrob. Resist. 15, 105–106. doi: 10.1016/j.jgar.2018.08.015
Keywords: carbapenemase-producing Enterobacteriaceae, marine environment, coastal waters, Croatia, KPC, OXA-48
Citation: Kvesić M, Šamanić I, Novak A, Fredotović Ž, Dželalija M, Kamenjarin J, Goić Barišić I, Tonkić M and Maravić A (2022) Submarine Outfalls of Treated Wastewater Effluents are Sources of Extensively- and Multidrug-Resistant KPC- and OXA-48-Producing Enterobacteriaceae in Coastal Marine Environment. Front. Microbiol. 13:858821. doi: 10.3389/fmicb.2022.858821
Received: 20 January 2022; Accepted: 06 April 2022;
Published: 06 May 2022.
Edited by:
Satoru Suzuki, Ehime University, JapanReviewed by:
Milena Dropa, University of São Paulo, BrazilCopyright © 2022 Kvesić, Šamanić, Novak, Fredotović, Dželalija, Kamenjarin, Goić Barišić, Tonkić and Maravić. This is an open-access article distributed under the terms of the Creative Commons Attribution License (CC BY). The use, distribution or reproduction in other forums is permitted, provided the original author(s) and the copyright owner(s) are credited and that the original publication in this journal is cited, in accordance with accepted academic practice. No use, distribution or reproduction is permitted which does not comply with these terms.
*Correspondence: Ana Maravić, ana.maravic@pmfst.hr
Disclaimer: All claims expressed in this article are solely those of the authors and do not necessarily represent those of their affiliated organizations, or those of the publisher, the editors and the reviewers. Any product that may be evaluated in this article or claim that may be made by its manufacturer is not guaranteed or endorsed by the publisher.
Research integrity at Frontiers
Learn more about the work of our research integrity team to safeguard the quality of each article we publish.