- 1College of Animal Science, Guizhou University, Guiyang, China
- 2Key Laboratory of Animal Genetics, Breeding and Reproduction in the Plateau Mountainous Region, Ministry of Education, Guizhou University, Guiyang, Guizhou, China
The present study investigated the effects of Lentilactobacillus buchneri, Saccharomyces cerevisiae, and a mixture of the two on the cellulose degradation and microbial community of cellulase-treated Pennisetum sinese (CTPS) during biological pretreatment. The CTPS was stored without additives (CK) or with L. buchneri (L), yeast (Y, S. cerevisiae), and their mixture (LY) under anaerobic conditions for 60 days. All inoculants enhanced the anaerobic fermentation of CTPS. In relative to L, inoculations with Y and LY decreased the cellulose level of fermented-CTPS by 8.90 ~ 17.13%. Inoculation with L inhibited the growth of Weissella cibaria during anaerobic storage. However, inoculations with LY increased the relative abundance of the homofermentative bacterium Lactiplantibacillus plantarum by 6.04%. Therefore, inoculating S. cerevisiae reduced the adverse effects of L. buchneri-stimulated fermentation on cellulose degradation by altering the bacterial community during anaerobic storage of P. sinese. This work provides a new insight for the subsequent anaerobic digestion of P. sinese.
Introduction
Pennisetum sinese, widely known as King grass, a widely used forage crop in the world, is a fast-growing gramineous grass with large biomass and has recently gained increasing attention as an energy crop (Li et al., 2018a). Anaerobic digestion (AD) is known as a widely used and cost-effective way to convert agriculture biomass into biogas (Wu et al., 2019). However, the majority of P. sinese are used for forage utilization and only a few are used for biomass energy production, which is because the lignocellulose in P. sinese is difficult to be decomposed during AD (Patinvoh et al., 2017). Therefore, pretreatment of P. sinese to improve the efficiency of AD is an essential step.
Until now, biological pretreatment of lignocellulose has been extensively investigated, such as enzymatic, fungal, composting and ensiling pretreatment (Wang et al., 2020). Among them, the ensiling pretreatment process is again accompanied by the addition of microorganisms, enzymes. Cellulase enzyme is a biological additive often used to pretreat high-fiber plant materials due to their ability to degrade structural carbohydrates to soluble sugars, which act as substrates for subsequent lactic acid bacteria anaerobic fermentation (Colombatto et al., 2004). However, multiple factors, such as enzyme type, concentration, and activity, application method, target substrate, and attached microorganisms of raw materials, govern cellulase activity (Li et al., 2018b). Moreover, the application of cellulase to P. sinese does not always present desirable efficiency due to the enzyme instability during pretreatment (Moharrery et al., 2009). Therefore, the degradation of cellulose by cellulases under microbial conditions is controversial. However, the role of microorganisms in enzyme-driven fiber degradation has rarely been reported. Exploring the effect of cellulase degradation of cellulose under microbial conditions will help to improve the efficiency of subsequent AD of P. sinese.
Yeast and lactic acid bacteria (LAB) inoculants have been used as additives during the ensilage of fresh materials, as they preserve the nutritional qualities of the material effectively. Matano et al. (2013) found that yeast mitigated the irreversible adsorption of cellulase onto crystalline cellulose and increased cellulase activity. It means that the presence of yeast may increase the degradation of cellulose during biological pretreatment. However, the role of yeast in promoting cellulose degradation during biological pretreatment has been rarely explored. Meanwhile, Lentilactobacillus buchneri is a heterotrophicus LAB often used as a bacterial inoculant in ensiling pretreatment due to its ability to produce volatile fatty acids, such as acetate and propionate from lactate (Kung et al., 2018). This conversion increases biogas emissions during AD. Stokes (1992) found that the interaction of LAB with cellulase is antagonistic. This implies that inoculation withe LAB maybe inhibits the degradation of cellulose. However, LAB inoculants are almost invariably applied with enzyme additives, making it difficult to differentiate between bacterial and enzyme-mediated ensiling responses (Xu et al., 2017). Few studies have been conducted to investigate the changes in the fiber fraction during biological pretreatment with a combination of cellulose, yeast, and LAB. Moreover, the fiber composition not just has an impact on the efficiency of AD, but also on the subsequent methane production, as cellulose can be degraded and converted to methane (Fujiwara et al., 2022). Therefore, it is essential to investigate the combined effect of microorganisms and cellulases to improve the AD efficiency of P. sinese.
The present study investigated the effects of L. buchneri, Saccharomyces cerevisiae and their mixtures as pretreatment inoculations on the fiber fraction and bacterial community of cellulase-treated P. sinese (CTPS). We hypothesized that the inoculation of L. buchneri might affect cellulase activity and inhibit cell wall degradation in P. sinese while adding S. cerevisiae would undo this effect.
Materials and methods
Sample preparation
For this study, P. sinese was harvested from the yellow cow breeding base in Fenggang County (27°42′ N, 106°55′ E), Zunyi City, Guizhou Province, China. The P. sinese plants were chopped cm to 1–3 cm in length and randomly divided into four blocks with 25 replicates per treatment. The forage from each block was first pretreated with cellulase (F; 2 × 102 μ/g FM, Shanghai Macklin Biochemical Co., Ltd., Shanghai, China, activity, 50 U/mg) and subsequently treated as follows: (i) no additive (CK); (ii) L. buchneri (L; 105 cfu/g FM, Xi’an Jushengyuan Biotechnology Co., Shaanxi, China); (iii) yeast (Y; S. cerevisiae, 2 × 105 cfu/g FM, Xi’an Jushengyuan Biotechnology Co., Shaanxi, China); and their mixture (LY). The inoculations were diluted with sterile water and sprayed evenly on the cellulase-treated P. sinese (CTPS), and CK treated with equal quantities of sterile water. Then, 100 g of CTPS uniformly mixed with each inoculant was manually loaded into a 500 ml Storage Jar (4 treatments ×25 Storage Jar), connected to a collection bag and sealed the jars after tightly vacuumed with an evacuator (SHZ-III type water Circulating Vacuum Pump, Yarong Biochemical Instrument Factory, Shanghai, China). Five Storage Jar per treatment were opened and sampled after 7, 14, 30, 45, and 60 days of storage. A total of 100 samples (4 treatments × 5 storage periods × 5 replicates) were collected and analyzed for fermentation quality, chemical composition and bacterial community composition during fermentation.
Chemical composition analysis
Each storage sample of 10 g was uniformly mixed with 90 ml of sterile water in a laboratory juicer for 1 min and filtered through four layers of gauze. The filtrate was then centrifuged at 4,500 × g for 15 min at 4°C. High-performance liquid chromatography (HPLC) was used to evaluate the concentration of butyric, propionic, acetic, and lactic acids (Li et al., 2019). The method of Broderick and Kang (1980) was used to determine the concentration of ammoniacal nitrogen. A pH meter was used to determine the pH of the sample solution.
Each storage sample (70 g) was dried at 65°C for a constant weight to determine the dry matter (DM) content, and then ground through 0.20 mm-mesh sieves for analysis of chemical components. Crude protein (CP) content was determined following the AOAC (1990) method. Neutral detergent fiber (NDF) and acid detergent fiber (ADF) content were determined according to the method described by Van Soest et al. (1991). The method of McDonald et al. (1991) was used to ascertain the content of water-soluble carbohydrates (WSC).
Bacterial community analysis
To determine the identities of the species present, we used the CTAB method to extract the total genomic DNA from each storage sample. After purification, DNA samples were diluted to 1 ng ml−1 with sterilized water. We amplified the full-length 16S ribosomal RNA (rRNA) gene using specific, barcoded primers (1514R and 27F; Yan et al., 2019). Polymerase Chain Reaction (PCR) amplification was conducted using TransStart®FastPfu DNA Polymerase (TransGen Biotech Co., Ltd., Beijing, China) and the PCR products purified using the QIAquick Gel Extraction Kit (QIAGEN LLC., Germantown, MD, United States). DNA libraries were generated with the SMRTbell Template Prep Kit (PacBio, Menlo Park, CA, United States) and sequenced using the PacBio Sequel system. In order to annotate taxonomic information and assess phylogenetic relationships with the Silva SSUrRNA Database, we used Novogene Bio-Technology Co., Ltd. (Beijing, China) to process raw sequences. Functional prediction, principal coordinates analysis (PCoA), and alpha diversity of the microbial community were assessed using the NovoMagic platform (Novogene Bio-Technology Co., Ltd., Beijing, China).
Statistical analysis
Data of changes in chemical composition, microbial population and bacterial community indices during storage was repeatedly compared with Duncan’s test, using the SPSS program version 26.0 (IBM Corp., Armonk, NY, United States). Differences were considered statistically significant only when the probability level was lower than 0.05 (p < 0.05). In addition, Spearman correlation was analyzed among bacterial community compositions and anaerobic fermentation parameters.
Results and discussion
Chemical composition of CTPS before and after anaerobic storage
Table 1 shows the chemical composition of fresh P. sinese before fermentation. The DM of P. sinese was 20.74%, and the NDF and ADF content were 61.34% DM and 35.22% DM, respectively. The WSC content was 5.65% DM, which is close to the WSC content (5.73% DM) reported by Li et al. (2018a). Typically, the WSC of the fresh material acts as an important substrate for silage fermentation, and a material with a WSC content >5% DM contributes to good silage quality (Cai et al., 1998). Thus, the WSC content of fresh P. sinese was sufficient for silage fermentation.
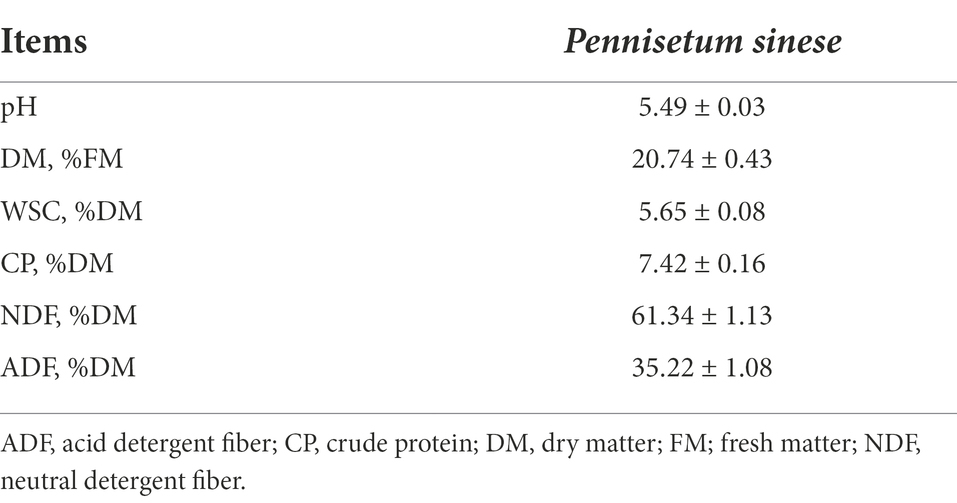
Table 1. Chemical composition of cellulase-treated Pennisetum sinese (CTPS) Pennisetum sinese before anaerobic fermentation.
Table 2 shows the chemical composition of CTPS after 60 days of fermentation. The DM content did not show differed significantly between the samples. The degradation of CP was mainly initiated by microbial and plant enzymes. In this experiment, the various CTPS samples after fermentation with different inoculants showed no significant difference in the CP content. This is due to the failure of high pH (>4.2) to inhibit microbial degradation of CP. Meanwhile, L and Y inoculated CTPS had higher NDF and ADF contents than CK (CTPS was not inoculated with microbial fermentation) after 60 days of fermentation, this may be attributed to the consumption of available nutrients stimulating an increase in NDF and ADF during anaerobic fermentation (Li et al., 2022). However, the LY-treated CTPS had the lowest NDF (53.49% DM) and ADF (29.70% DM) content, probably due to the LY inoculum promoting cell wall degradation by cellulase. Exogenous cellulolytic enzymes are usually added to the woody fiber material before ensiling. These cellulases degrade cellulose and produce WSC to facilitate anaerobic fermentation. In CTPS, the L treatment (30.77% DM) and Y treatment (28.03% DM) samples had higher cellulose content than CK treated samples (26.33% DM). We attribute this phenomenon to the different effects of L. buchneri and S. cerevisiae on cellulase. Interestingly, the LY-treated (25.50% DM) samples showed a different result from it. Earlier, Chen et al. (2020) reported that a mixture of Lactiplantibacillus plantarum, L. buchneri and cellulase promoted the degradation of fiber fractions. However, Stokes (1992) found that inoculation with a multispecies homofermentative LAB culture (L. plantarum, Levilactobacillus brevis, Pediococcus acidilactici, Streptococcus cremoris, and Streptococcus diacetylactis) was antagonistic to cellulase. This indicated that different microorganisms have different effects on cellulase. Therefore, we believe that the phenomenon observed in this study may be due to the inoculated S. cerevisiae and L. buchneri affecting the cellulase activity, which further affects the degradation of the fiber fraction. The higher ADL content in the samples treated with LY supports this fact.
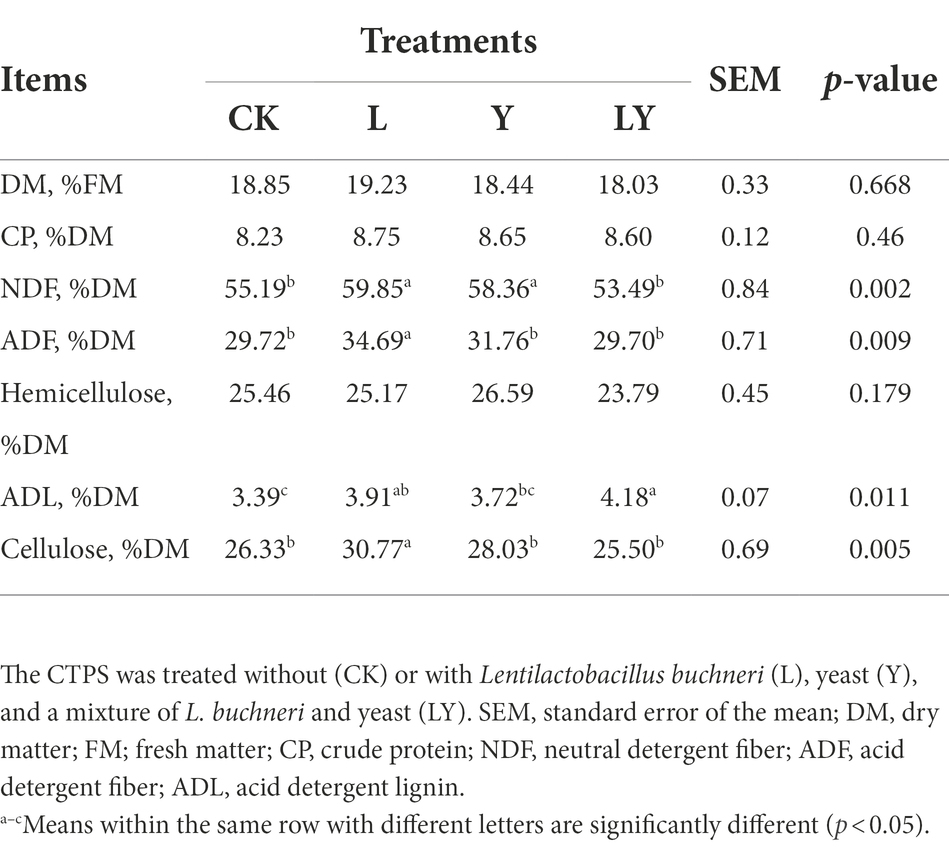
Table 2. Chemical composition of cellulase-treated Pennisetum sinese (CTPS) after 60 days of anaerobic fermentation.
Fermentation profile of CTPS during anaerobic storage
We further analyzed the fermentation characteristics of CTPS during storage (Table 3). The interactive effect of pH was not significant during the anaerobic fermentation. However, the pH of the L-treated CTPS was the lowest (4.34) after 60 days of fermentation, which was strongly related to the production of lactic acid by the inoculant L. buchneri. Similarly, Zielinska et al. (2015) reported that L. buchneri inoculation decreases the pH of alfalfa silage.
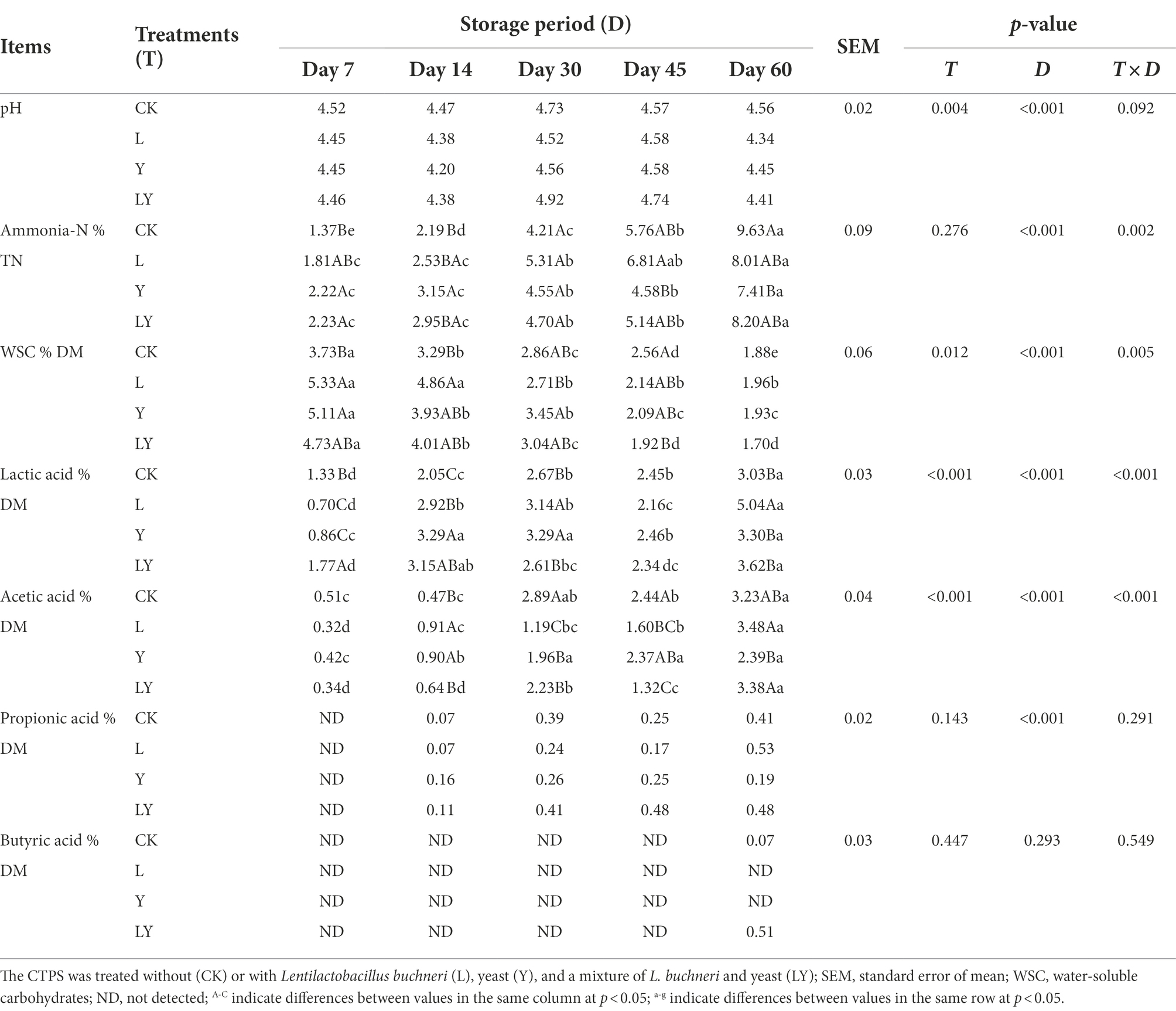
Table 3. Fermentation profile of cellulase-treated Pennisetum sinese (CTPS) during anaerobic storage.
The WSC content is a limiting factor for fermentation. Generally, a minimum WSC content of about 3% DM is necessary to successfully preserve material (Zhang et al., 2010). However, WSC, the primary substrate for microbial growth, decreases gradually with fermentation progress. CK treated CTPS had the fastest decrease in WSC content at 7 days of anaerobic storage (5.65% DM to 3.73% DM), and the predominant inoculants inhibited the consumption of WSC by undesirable bacteria during the anaerobic storage. Guo et al. (2014) found more residual WSC in Lactobacillus-treated and fibrolytic enzyme-treated forages, which was Lactobacillus inhibits the consumption of WSC by undesirable bacteria. Besides, the inoculants delayed the decrease in WSC content of anaerobic fermentation compared with CK. Similarly, Li et al. (2022) also reported that the LAB inoculation delayed the decline in WSC. A high level of ammonia-N (>10% of total N) in sample indicates excessive protein breakdown, usually caused by a slight decrease in pH and/or Clostridium fermentation (Kung et al., 2018). In the present study, ammonia-N increased with the fermentation progress. The lowest ammonia-N level was detected in the Y-treated CTPS compared with the control and other additives, indicating good preservation with S. cerevisiae as the inoculant. This may be the result of the inoculated S. cerevisiae inhibiting the growth of NH3-N producing bacteria, thus reducing the NH3-N content in the silage. However, the mechanism underlying the slow degradation of protein to ammonia in the presence of S. cerevisiae is unclear.
Lactic acid is considered responsible for the decrease in pH. In this study, lactic acid increased in all CTPS samples, followed by a decrease after 45 days of anaerobic storage, with a maximum at the subsequent time. The changes in lactic acid concentration explain the fluctuation in pH. Notably, at 7 days, the LY-treated CTPS had the highest lactic acid content (1.77% DM). Earlier, Li et al. (2017) also reported that the combination of LAB and cellulase increased the concentration of lactic acid, while Mu et al. (2020) explained that cellulase indirectly provided LAB fermentable sugars by degrading cellulose, subsequently increasing the lactic acid level. Additionally, Carvalho et al. (2021) found that a few yeasts had the potential to produce amylase, cellulase, and protease. Therefore, we attributed the higher lactic acid level in the LY-treated CTPS to the cellulases. We also believe these cellulases led to the lowest cellulose content in the LY-treated CTPS at 60 days. However, the lactic acid level of the LY-treated CTPS lowered than the L-treated CTPS at 60 days, which may be due to the continuous consumption of lactic acid by inoculated yeast (Dolci et al., 2011). Meanwhile, the acetic acid content gradually increased with increasing fermentation time, consistent with Zhou et al. (2016). Studies have shown that the inoculation of heterologous L. buchneri increases fermentation’s acetic acid level (Huang et al., 2021). However, in the L-treated CTPS after 60 days of anaerobic storage, the acetic acid content was not significantly different from the CK-treated CTPS (p > 0.05), probably due to the higher lactic acid that inhibited acetic acid production (Lin et al., 2021). Meanwhile, the low acetic acid levels in the Y-treated CTPS at 60 days could be attributed to the continuous consumption of acetic acid in other bacteria (Ogunade et al., 2017), which will subsequently burden bioenergy production. Studies have proven the presence of propionic acid bacteria that convert glucose and lactic acid to propionic acid and acetic acid (Li et al., 2022). Therefore, the propionic acid produced after 7 days of fermentation in CTPS may be due to the action of these propionic acid bacteria. Under unfavorable conditions, certain undesirable microorganisms, such as Clostridium, convert lactic acid to butyric acid (Steinbrenner et al., 2019). The butyric acid in the LY and CK-treated CTPS may be due to the fermentation of Clostridium. These results suggest that the LY-treated CTPS increased lactic acid content by enhanced cellulose degradation in pre-fermentation, however, the mechanisms behind this need to be explored.
Bacterial diversity of CTPS during anaerobic storage
Furthermore, we analyzed the alpha diversity of bacteria found in CTPS after anaerobic fermentation (Table 4). The coverage value of all samples was above 99%, indicating that sequencing adequately captured most of the bacterial communities. The observed species number increased in CTPS during the 60 days anaerobic fermentation. Similarly, Yan et al. (2019) reported an increase in species after anaerobic fermentation of ryegrass. Shannon’s and Simpson’s diversity indices varied similarly among the treatments. The alpha diversity at 60 days was the lowest in the LY-treated CTPS, probably due to the combined effect of S. cerevisiae and L. buchneri. Usually, a low bacterial community diversity is due to the increased abundance of dominant bacteria (Wayne Polley et al., 2007). Meanwhile, ACE and Chao1 are used to evaluate the richness of the microbial community. The richness indices increased in all samples after 60 days of storage, consistent with Ren et al. (2019), who reported an increase in the bacterial richness during anaerobic fermentation of top sugarcane.
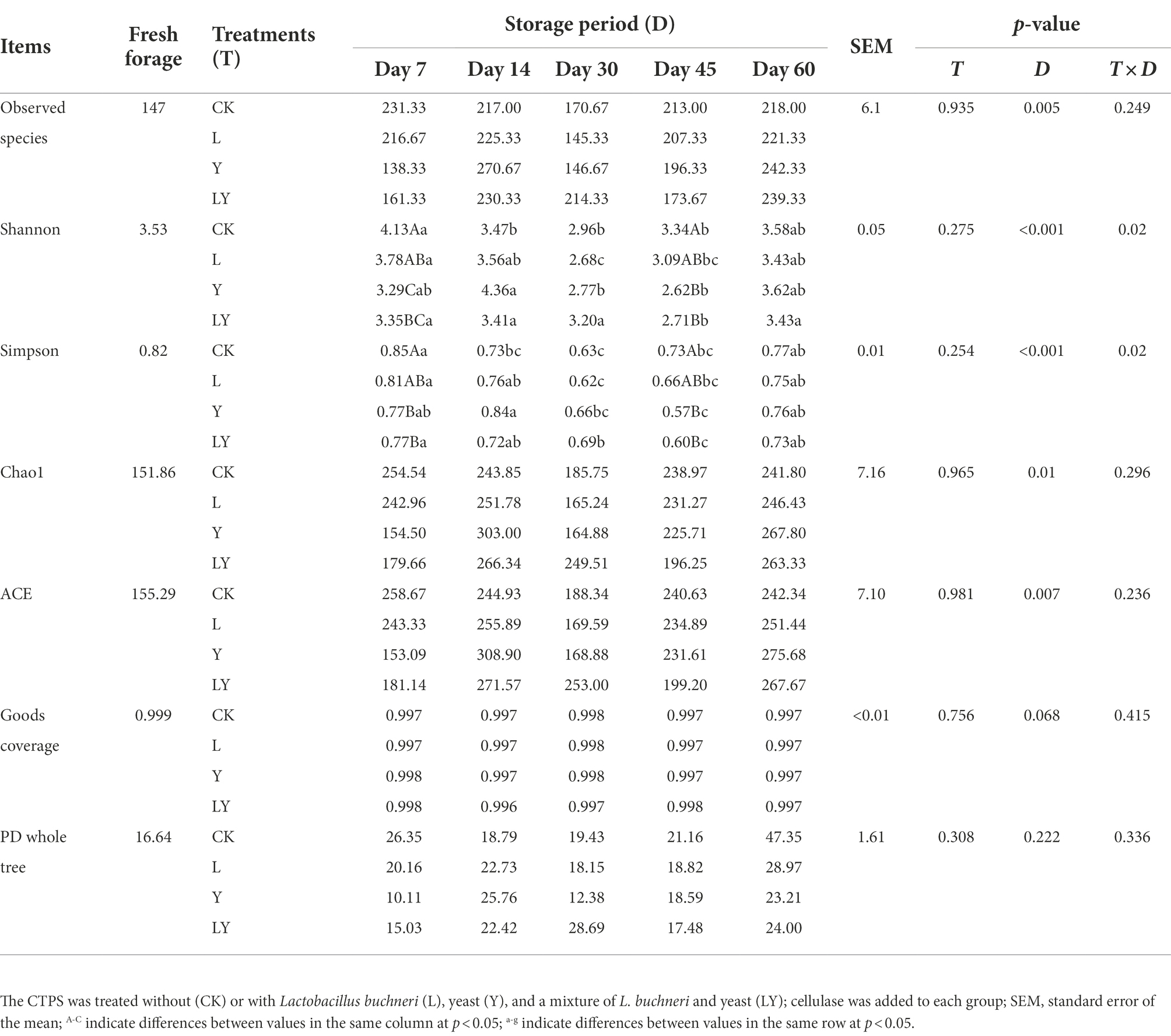
Table 4. Bacterial alpha diversity of cellulase-treated Pennisetum sinese (CTPS) during anaerobic storage.
Bacterial community composition of CTPS during anaerobic storage
Changes in the bacterial community at the genus and species levels during the anaerobic storages are shown in Figure 1 and Table 5. At the genus level, the relative abundance of Lactiplantibacillus and Levilactobacillus increased with increasing storage time, but that of Weissella gradually decreased. Previous studies have shown that Weissella causes fermentation in the early stages, the predominant bacteria gradually shift to Lactobacillus, which is more tolerant to low pH (Cai et al., 1998). In the present study, the LY-treated CTPS had the highest relative abundance of Weissella (14.04%) after 7 days of anaerobic storage, which was also detected after 60 days of storage. The presence of Weissella at the late fermentation stage may be due to the high pH. In the present study, some Bacillus (relative richness >3.0%) was detected in the CK and LY treated CTPS in pre-fermentation. Bacillus accelerates lignocellulose degradation and promotes the stabilization and resource utilization of compost by secreting enzymes (Niu and Li, 2022). Bacillus probably correlated with the high lactic acid concentration of CK-and LY-treated CTPS in the pre-storage period. In addition, Acinetobacter also showed a high abundance in this study. It has been found that Acinetobacter can utilize acetic acid to survive in an anaerobic environment, and its abundance may increase with increasing acetic acid content (Ogunade et al., 2017). Thus, the acetic acid increase may be responsible for the higher abundance of Acinetobacter, the bacterium causes aerobic spoilage via the oxidation of lactic acid and acetic acid (Dolci et al., 2011). The high abundance of Acinetobacter during fermentation may also be responsible for the elevated pH after 30 days of fermentation.
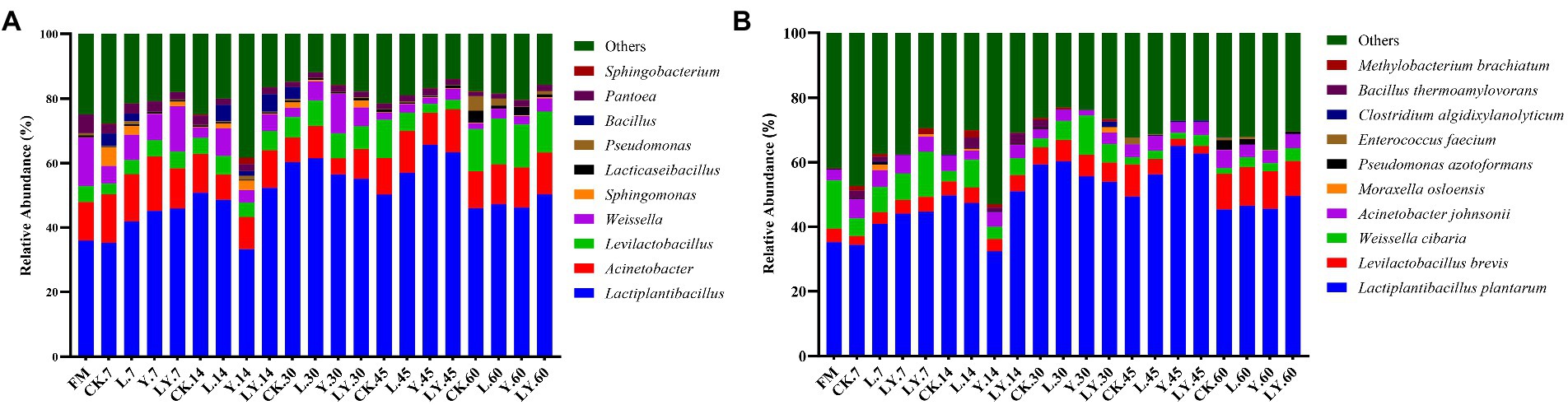
Figure. 1. The relative abundance of dominant genera (A) and species (B) in cellulase-treated Pennisetum sinese (CTPS) after anaerobic storage. CK, control; L, Lentilactobacillus buchneri; Y, yeast; LY, a mixture of L. buchneri and yeast.
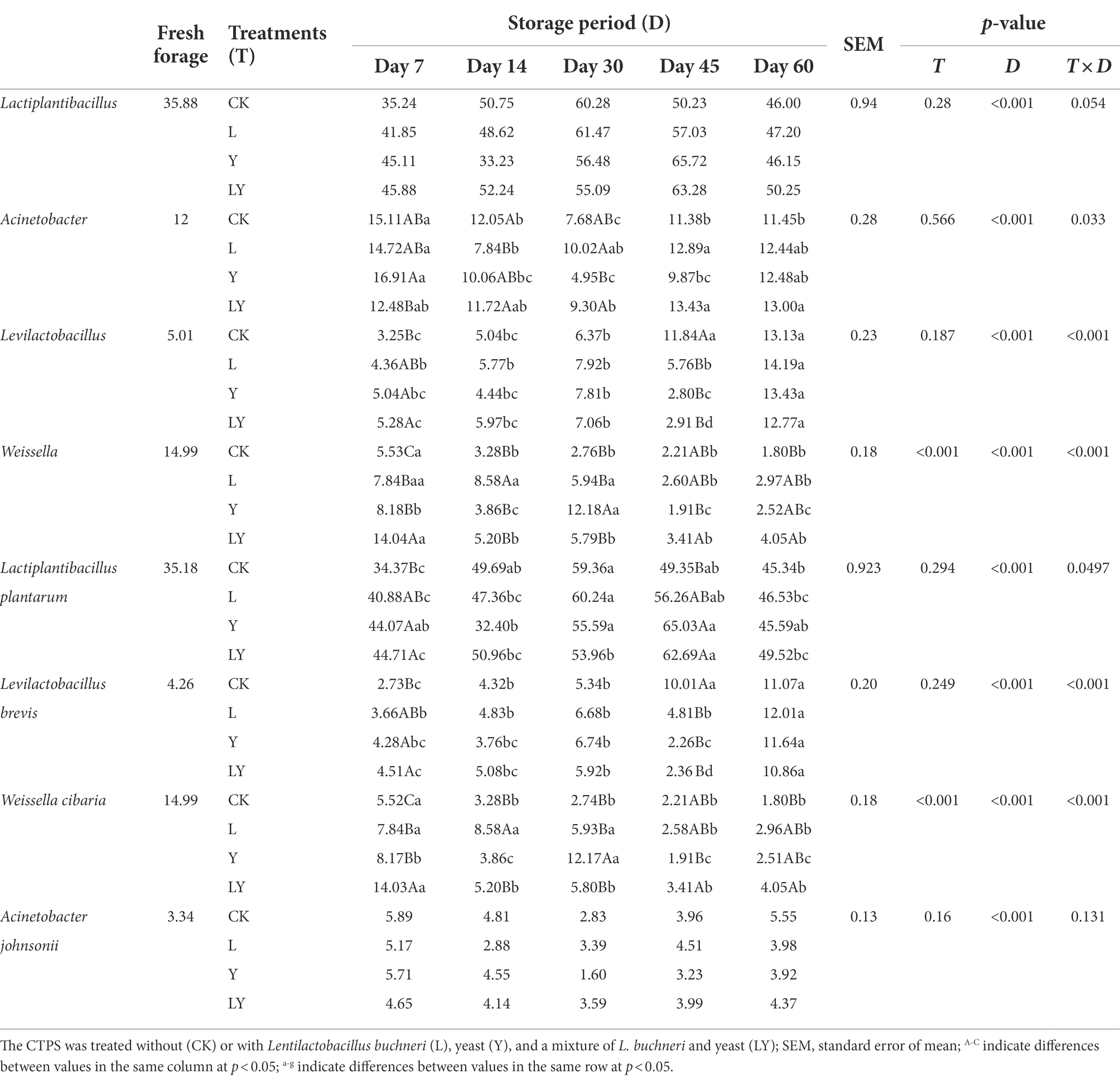
Table 5. Relative abundance (%) of top four bacteria at different taxonomic levels in cellulase-treated Pennisetum sinese (CTPS) before and after anaerobic storage.
The top three abundant bacteria at the species level were L. plantarum, L. brevis, and Weissella cibaria. However, the study did not detect L. buchneri during storage, probably because the L. buchneri used in this study exerted low competition at the beginning of storage, which was replaced by L. plantarum. The high relative abundance of L. plantarum in storage supported this fact. Generally, L. plantarum promotes rapid fermentation that produces lactic acid, preventing further breakdown of the sugars and proteins (Yan et al., 2019). Thus, the lower relative abundance of L. plantarum in the CK-treated CTPS after 7 days of anaerobic storage explains the WSC content. Additionally, we found that L. brevis increased during storage in each treatment group. The Y treatment significantly increased the abundance of L. brevis at 7 days compared to the CK treatment, which may be due to the inoculated S. cerevisiae enhancing cellulose degradation by affecting the cellulase activity, thus providing more substrate for L. brevis growth. L. brevis is a heterologous fermenting bacterium that produces lactic acid and acetic acid as the primary end products through WSC metabolism (Tohno et al., 2012). The abundance of L. brevis also explains the increase in acetic acid content. Meanwhile, the relative abundance of W. cibaria, a heterologous fermenting bacterium, decreased with storage time in overall treated samples. L. plantarum significantly decreased the relative abundance of Weissella, which is usually outcompeted by Lactobacillus spp. as the pH declines during ensiling (Keshri et al., 2019). Interestingly, the LY-treated CTPS had the highest relative abundance of W. cibaria after storage for 60 days. This dominant Weissella resulted in the lowest alpha diversity in the LY-treated CTPS group at 60 days. Teixeira et al. (2021) reported that W. cibaria could ferment prebiotic fibers, which is related to β-glucosidase activity, suggesting that the W. cibaria during anaerobic storage could promote cellulose degradation. Therefore, we attribute the low cellulose content of the LY-treated CTPS in this study to the degradation of cellulose by W. cibaria. Unfortunately, the activity of cellulase was not determined in the current work. In summary, inoculation with L. buchneir and S. cerevisiae increased the abundance of L. brevis and W. cibaria, which enhanced cellulose degradation.
Functional prediction of bacterial community in CTPS during anaerobic storage
Figure 2A and Table 6 show the predicted functions of the bacterial community during anaerobic fermentation. Chemoheterotrophy and fermentation are ways in which microorganisms utilize organic matter, and their abundance represent the intensity of microbial activity. In the present, the functional group chemoheterotrophy was most abundant in the bacterial community, followed by fermentation and nitrogen-related cycling. The CTPS samples inoculated with LY, L, and Y demonstrated improved fermentation function at 7, 14, and 30 days, respectively. This improvement in function is attributed to the high relative abundance of W. cibaria (Table 5). Studies have proven that the presence of W. cibaria promotes anaerobic fermentation (Cai et al., 1998). In addition, higher nitrite ammonification, nitrogen respiration, and nitrite respiration were detected in the groups with or without the inoculants, which explains the persistent production of ammonia-N.
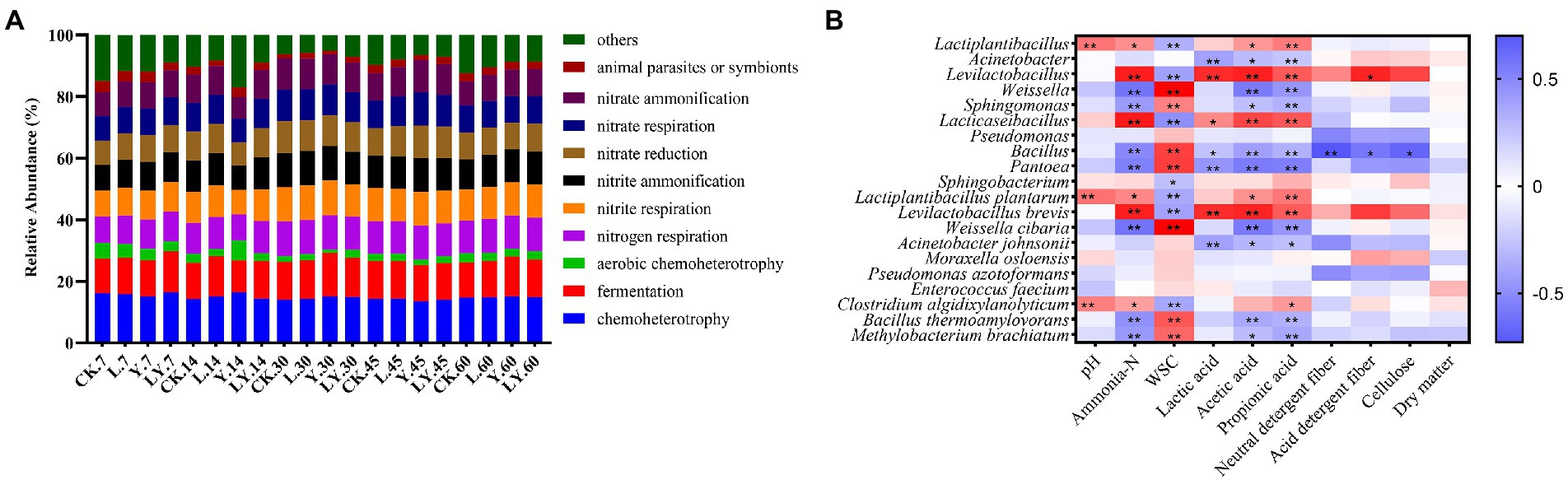
Figure. 2. The functional prediction of bacteria (A) in cellulase-treated Pennisetum sinese (CTPS) during anaerobic storage and Spearman correlations between the fermentation parameters and the relative abundance of top 10 bacterial genera and species (B). CK, control; L, Lentilactobacillus buchneri; Y, yeast; LY, a mixture of L. buchneri and yeast; WSC, water-soluble carbohydrates.
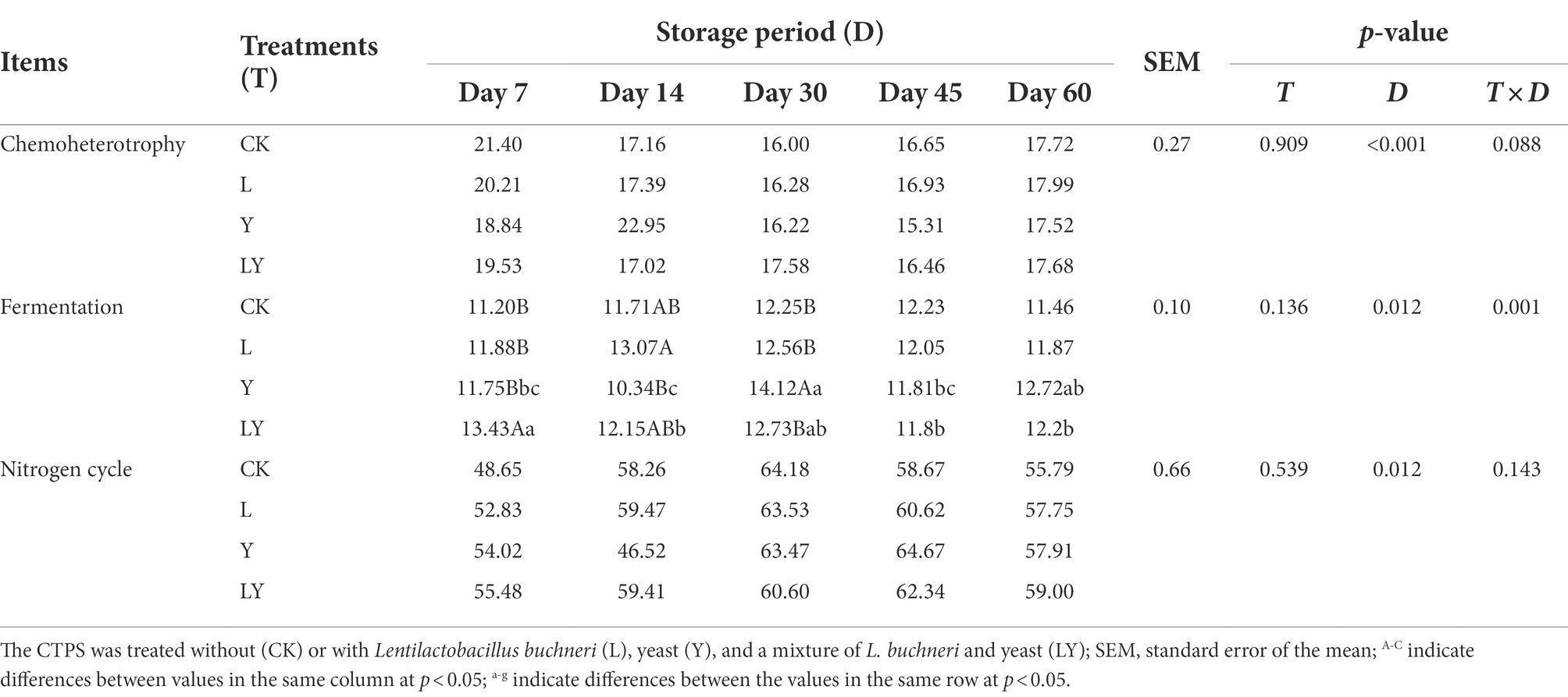
Table 6. Chemoheterotrophy and fermentation changes in functional prediction of bacteria in cellulase-treated Pennisetum sinese (CTPS) during anaerobic storage.
Finally, Spearman correlations between fermentation parameters and kinetics of the top ten genera and species during the fermentation of CTPS were calculated (Figure 2B). The analysis revealed that the pH was positively correlated with Clostridium and L. plantarum, suggesting that the variation in pH was not due to L. plantarum alone. Meanwhile, Weissella correlated negatively with ammonia-N but positively with WSC, implying that the presence of Weissella could preserve the nutritional quality of the anaerobic fermentation. Lactic acid and acetic acid showed positive correlations with L. brevis. Therefore, we inferred that L. brevis was the main component responsible for producing acetic acid during storage. Meanwhile, Bacillus showed a significant negative correlation with NDF, ADF, and cellulose, indicating the role of Bacillus in cellulose degradation, consistent with the reports on the ability of Bacillus to secrete cellulase (Niu and Li, 2022). Furthermore, to explore the effect of inoculants on the bacterial community we performed an analysis of bacterial PCoA. The PCoA plots illustrated that all inoculation promoted the change in the bacterial community during anaerobic fermentation (Figure 3). The LY-treated CTPS remodeled the bacterial community during storage significantly better than the other samples, indicating that S. cerevisiae resulted in a different effect on L. buchneri-mediated anaerobic fermentation.
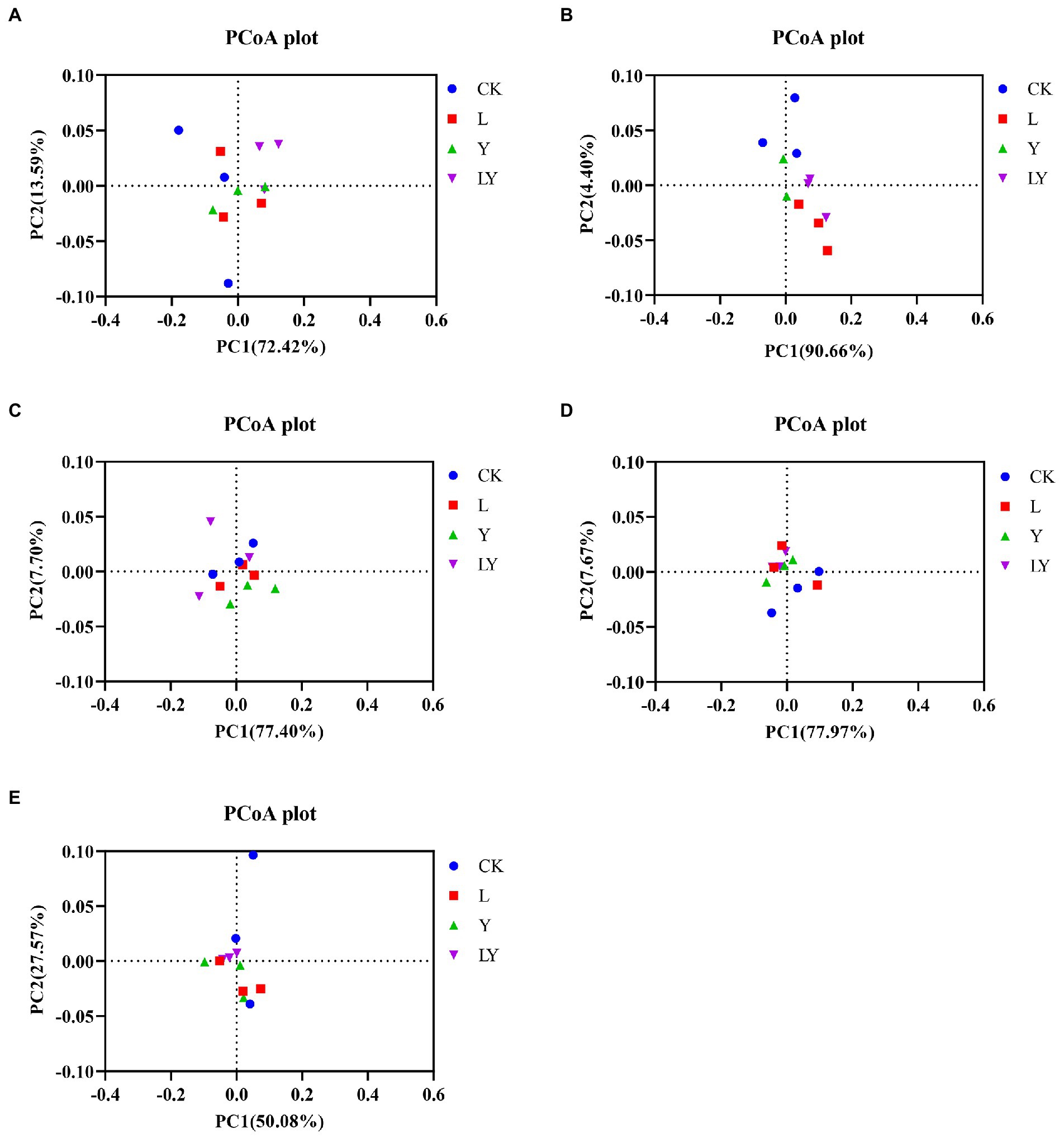
Figure. 3. PCoA plot of the bacterial community structure of cellulase-treated Pennisetum sinese (CTPS) after anaerobic storage for 7 days (A), 14 days (B), 30 days (C), 45 days (D), and 60 days (E). CK, control; L, Lentilactobacillus buchneri; Y, yeast; LY, a mixture of L. buchneri and yeast.
Conclusion
This study evaluated the effects of L. buchneri, S. cerevisiae, and their mixtures on cellulase treated P. sinese (CTPS) silage quality and PM microbial community. Among the several additives evaluated. S. cerevisiae appeared to be a potential inoculant, and its co-addition with LAB reduced the cellulose content of CTPS during anaerobic storage by increasing W. cibaria and L. brevis. This study showed that the combined addition of S. cerevisiae and L. buchneri reduced the cellulose content during anaerobic storage of CTPS.
Data availability statement
The datasets presenting in this article are deposited in NCBI (https://www.ncbi.nlm.nih.gov/) repository, accession number PRJNA887958.
Author contributions
CL: data curation, formal analysis, visualization, writing—original draft, writing—review and editing. XT, ML, GL, XH, LL, MZ: investigation. YX: investigation, resources. PL: conceptualization, methodology, validation, writing—review and editing, supervision, funding acquisition. CC: project administration, funding acquisition. All authors contributed to the article and approved the submitted version.
Funding
This project was supported by the National Key Research and Development Program of China (2021YFD1300300) and the basic scientific research funds of Guizhou Province (2022).
Acknowledgments
We also thank Hong Sun, Yulong Zheng, Qiming Cheng, and Chunmei Wang for the help during sampling.
Conflict of interest
The authors declare that the research was conducted in the absence of any commercial or financial relationships that could be construed as a potential conflict of interest.
Publisher’s note
All claims expressed in this article are solely those of the authors and do not necessarily represent those of their affiliated organizations, or those of the publisher, the editors and the reviewers. Any product that may be evaluated in this article, or claim that may be made by its manufacturer, is not guaranteed or endorsed by the publisher.
References
AOAC. (1990). Official Methods of Analysis. Association of Official Analytical Chemists, Arlington, VA.
Broderick, G. A., and Kang, J. H. (1980). Automated simultaneous determination of ammonia and total amino acids in ruminal fluid and in vitro media. J. Dairy Sci. 63, 64–75. doi: 10.3168/jds.S0022-0302(80)82888-8
Cai, Y., Benno, Y., Ogawa, M., Ohmomo, S., Kumai, S., and Nakase, T. (1998). Influence of lactobacillus spp. from an inoculant and of Weissella and Leuconostoc spp. from forage crops on silage fermentation. Appl. Environ. Microbiol. 64, 2982–2987. doi: 10.1128/AEM.64.8.2982-2987.1998
Carvalho, J. K., Panatta, A. A. S., Silveira, M. A. D., Tav, C., Johann, S., Rodrigues, M. L. F., et al. (2021). Yeasts isolated from a lotic continental environment in Brazil show potential to produce amylase, cellulase and protease. Biotechnol. Rep. 30:e00630. doi: 10.1016/j.btre.2021.e00630
Chen, L., Li, P., Gou, W., You, M., Cheng, Q., Bai, S., et al. (2020). Effects of inoculants on the fermentation characteristics and in vitro digestibility of reed canary grass (Phalaris arundinacea L.) silage on the Qinghai-Tibetan plateau. Anim. Sci. J. 91:e13364. doi: 10.1111/asj.13364
Colombatto, D., Mould, F. L., Bhat, M. K., Phipps, R. H., and Owen, E. (2004). In vitro evaluation of fibrolytic enzymes as additives for maize (Zea mays L.) silage. I. Effects of ensiling temperature, enzyme source and addition level. Anim. Feed Sci. Technol. 111, 111–128. doi: 10.1016/j.anifeedsci.2003.08.010
Dolci, P., Tabacco, E., Cocolin, L., and Borreani, G. (2011). Microbial dynamics during aerobic exposure of corn silage stored under oxygen barrier or polyethylene films. Appl. Environ. Microbiol. 77, 7499–7507. doi: 10.1128/AEM.05050-11
Fujiwara, M., Koyama, M., Akizuki, S., Ban, S., and Toda, T. (2022). Influence of lignocellulosic components on the anaerobic digestibility of aquatic weeds: comparison with terrestrial crops. Ind. Crop. Prod. 178:114576. doi: 10.1016/j.indcrop.2022.114576
Guo, G., Yuan, X., Li, L., Wen, A., and Shao, T. (2014). Effects of fibrolytic enzymes, molasses and lactic acid bacteria on fermentation quality of mixed silage of corn and hulless-barely straw in the Tibetan plateau. Grassl. Sci. 60, 240–246. doi: 10.1111/grs.12060
Huang, Z., Wang, M., Ke, W., and Guo, X. (2021). Screening of high 1,2-Propanediol production by lactobacillus buchneri strains and their effects on fermentation characteristics and aerobic stability of whole-plant corn silage. Agriculture 11:590. doi: 10.3390/agriculture11070590
Keshri, J., Chen, Y., Pinto, R., Kroupitski, Y., Weinberg, Z. G., and Sela Saldinger, S. (2019). Bacterial dynamics of wheat silage. Front. Microbiol. 10:1532. doi: 10.3389/fmicb.2019.01532
Kung, L., Shaver, R. D., Grant, R. J., and Schmidt, R. J. (2018). Silage review: interpretation of chemical, microbial, and organoleptic components of silages. J. Dairy Sci. 101, 4020–4033. doi: 10.3168/jds.2017-13909
Li, D., Ni, K., Zhang, Y., Lin, Y., and Yang, F. (2018b). Influence of lactic acid bacteria, cellulase, cellulase-producing Bacillus pumilus and their combinations on alfalfa silage quality. J. Integr. Agr. 17, 2768–2782. doi: 10.1016/S2095-3119(18)62060-X
Li, J., Yuan, X., Dong, Z., Mugabe, W., and Shao, T. (2018a). The effects of fibrolytic enzymes, cellulolytic fungi and bacteria on the fermentation characteristics, structural carbohydrates degradation, and enzymatic conversion yields of Pennisetum sinese silage. Bioresour. Technol. 264, 123–130. doi: 10.1016/j.biortech.2018.05.059
Li, P., Zhang, Y., Gou, W., Cheng, Q., Bai, S., and Cai, Y. (2019). Silage fermentation and bacterial community of bur clover, annual ryegrass and their mixtures prepared with microbial inoculant and chemical additive. Anim. Feed Sci. Tech. 247, 285–293. doi: 10.1016/j.anifeedsci.2018.11.009
Li, P., Zhao, W., Yan, L., Chen, L., Chen, Y., Gou, W., et al. (2022). Inclusion of abandoned rhubarb stalk enhanced anaerobic fermentation of alfalfa on the Qinghai Tibetan plateau. Bioresour. Technol. 347:126347. doi: 10.1016/j.biortech.2021.126347
Li, M., Zhou, H. L., Zi, X. J., and Cai, Y. M. (2017). Silage fermentation and ruminal degradation of stylo prepared with lactic acid bacteria and cellulase. Anim. Sci. J. 88, 1531–1537. doi: 10.1111/asj.12795
Lin, M., Feng, L., Cheng, Z., and Wang, K. (2021). Effect of ethanol or lactic acid on volatile fatty acid profile and microbial community in short-term sequentially transfers by ruminal fermented with wheat straw in vitro. Process Biochem. 102, 369–375. doi: 10.1016/j.procbio.2020.12.018
Matano, Y., Hasunuma, T., and Kondo, A. (2013). Simultaneous improvement of saccharification and ethanol production from crystalline cellulose by alleviation of irreversible adsorption of cellulase with a cell surface-engineered yeast strain. Appl. Microbiol. Biot. 97, 2231–2237. doi: 10.1007/s00253-012-4587-x
McDonald, P., Henderson, N., and Heron, S. (1991). The biochemistry of silage. Chalcombe Publications, Marlow.
Moharrery, A., Hvelplund, T., and Weisbjerg, M. R. (2009). Effect of forage type, harvesting time and exogenous enzyme application on degradation characteristics measured using in vitro technique. Anim. Feed Sci. Tech. 153, 178–192. doi: 10.1016/j.anifeedsci.2009.06.001
Mu, L., Xie, Z., Hu, L., Chen, G., and Zhang, Z. (2020). Cellulase interacts with lactobacillus plantarum to affect chemical composition, bacterial communities, and aerobic stability in mixed silage of high-moisture amaranth and rice straw. Bioresour. Technol. 315:123772. doi: 10.1016/j.biortech.2020.123772
Niu, J., and Li, X. (2022). Effects of microbial inoculation with different indigenous bacillus species on physicochemical characteristics and bacterial succession during short-term composting. Fermentation 8:152. doi: 10.3390/fermentation8040152
Ogunade, I. M., Jiang, Y., Kim, D. H., Cervantes, A. A. P., Arriola, K. G., Vyas, D., et al. (2017). Fate of Escherichia coli O157:H7 and bacterial diversity in corn silage contaminated with the pathogen and treated with chemical or microbial additives. J. Dairy Sci. 100, 1780–1794. doi: 10.3168/jds.2016-11745
Patinvoh, R. J., Osadolor, O. A., Chandolias, K., Sárvári Horváth, I., and Taherzadeh, M. J. (2017). Innovative pretreatment strategies for biogas production. Bioresour. Technol. 224, 13–24. doi: 10.1016/j.biortech.2016.11.083
Ren, F., He, R., Zhou, X., Gu, Q., Xia, Z., Liang, M., et al. (2019). Dynamic changes in fermentation profiles and bacterial community composition during sugarcane top silage fermentation: a preliminary study. Bioresour. Technol. 285:121315. doi: 10.1016/j.biortech.2019.121315
Steinbrenner, J., Nägele, H., Buschmann, A., Hülsemann, B., and Oechsner, H. (2019). Testing different ensiling parameters to increase butyric acid concentration for maize silage, followed by silage separation and methane yield potential of separated solids residues. Bioresour. Technol. Rep. 7:100193. doi: 10.1016/j.biteb.2019.100193
Stokes, M. R. (1992). Effects of an enzyme mixture, an inoculant, and their interaction on silage fermentation and dairy production. J. Dairy Sci. 75, 764–773. doi: 10.3168/jds.S0022-0302(92)77814-x
Teixeira, C. G., Fusieger, A., Milião, G. L., Martins, E., Drider, D., Nero, L. A., et al. (2021). Weissella: an emerging bacterium with promising health benefits. Probiotics Antimicrob. Proteins 13, 915–925. doi: 10.1007/s12602-021-09751-1
Tohno, M., Kobayashi, H., Nomura, M., Kitahara, M., Ohkuma, M., Uegaki, R., et al. (2012). Genotypic and phenotypic characterization of lactic acid bacteria isolated from Italian ryegrass silage. Anim. Sci. J. 83, 111–120. doi: 10.1111/j.1740-0929.2011.00923.x
Van Soest, P. J., Robertson, J. B., and Lewis, B. A. (1991). Methods for dietary fiber, neutral detergent fiber, and non-starch-polysaccharides in relation to animal nutrition. J. Dairy Sci. 74, 3583–3597. doi: 10.3168/jds.S0022-0302(91)78551-2
Wang, C., Zhang, J., Hu, F., Zhang, S., Lu, J., and Liu, S. (2020). Bio-pretreatment promote hydrolysis and acidification of oilseed rape straw: roles of fermentation broth and micro-oxygen. Bioresour. Technol. 308:123272. doi: 10.1016/j.biortech.2020.123272
Wayne Polley, H., Wilsey, B. J., and Derner, J. D. (2007). Dominant species constrain effects of species diversity on temporal variability in biomass production of tallgrass prairie. Oikos 116, 2044–2052. doi: 10.1111/j.2007.0030-1299.16080.x
Wu, D., Li, L., Zhao, X., Peng, Y., Yang, P., and Peng, X. (2019). Anaerobic digestion: a review on process monitoring. Renew. Sust. Energ. Rev. 103, 1–12. doi: 10.1016/j.rser.2018.12.039
Xu, Z., He, H., Zhang, S., Guo, T., and Kong, J. (2017). Characterization of feruloyl esterases produced by the four lactobacillus species: L. amylovorus, L. acidophilus, L. farciminis and L. fermentum, isolated from ensiled corn Stover. Front. Microbiol. 8, 1–11. doi: 10.3389/fmicb.2017.00941
Yan, Y., Li, X., Guan, H., Huang, L., Ma, X., Peng, Y., et al. (2019). Microbial community and fermentation characteristic of Italian ryegrass silage prepared with corn Stover and lactic acid bacteria. Bioresour. Technol. 279, 166–173. doi: 10.1016/j.biortech.2019.01.107
Zhang, J., Kawamoto, H., and Cai, Y. (2010). Relationships between the addition rates of cellulase or glucose and silage fermentation at different temperatures. Anim. Sci. J. 81, 325–330. doi: 10.1111/j.1740-0929.2010.00745.x
Zhou, Y., Drouin, P., and Lafrenière, C. (2016). Effect of temperature (5-25°C) on epiphytic lactic acid bacteria populations and fermentation of whole-plant corn silage. J. Appl. Microbiol. 121, 657–671. doi: 10.1111/jam.13198
Keywords: biological pretreatment, lactic acid bacteria, yeast, bacterial community, cellulose, Pennisetum sinese
Citation: Liao C, Tang X, Li M, Lu G, Huang X, Li L, Zhang M, Xie Y, Chen C and Li P (2022) Effect of lactic acid bacteria, yeast, and their mixture on the chemical composition, fermentation quality, and bacterial community of cellulase-treated Pennisetum sinese silage. Front. Microbiol. 13:1047072. doi: 10.3389/fmicb.2022.1047072
Edited by:
Amr Fouda, Al-Azhar University, EgyptReviewed by:
Sadat Mohamed Rezk Khattab, Kyoto University, JapanQing Zhang, South China Agricultural University, China
Copyright © 2022 Liao, Tang, Li, Lu, Huang, Li, Zhang, Xie, Chen and Li. This is an open-access article distributed under the terms of the Creative Commons Attribution License (CC BY). The use, distribution or reproduction in other forums is permitted, provided the original author(s) and the copyright owner(s) are credited and that the original publication in this journal is cited, in accordance with accepted academic practice. No use, distribution or reproduction is permitted which does not comply with these terms.
*Correspondence: Ping Li, bHB5em1Ac2luYS5jbg==