- Department of Laboratory Medicine, The Affiliated Hospital of Southwest Medical University, Luzhou, China
Carbapenem-resistant Acinetobacter baumannii (CRAB) has become one of the leading causes of healthcare-associated infections globally, particularly in intensive care units (ICUs). Cross-transmission of microorganisms between patients and the hospital environment may play a crucial role in ICU-acquired CRAB colonization and infection. The control and treatment of CRAB infection in ICUs have been recognized as a global challenge because of its multiple-drug resistance. The main concern is that CRAB infections can be disastrous for ICU patients if currently existing limited therapeutic alternatives fail in the future. Therefore, the colonization, infection, transmission, and resistance mechanisms of CRAB in ICUs need to be systematically studied. To provide a basis for prevention and control countermeasures for CRAB infection in ICUs, we present an overview of research on CRAB in ICUs, summarize clinical infections and environmental reservoirs, discuss the drug resistance mechanism and homology of CRAB in ICUs, and evaluate contemporary treatment and control strategies.
Introduction
The incidence of drug-resistant organism infection is currently increasing in hospitals and other clinical care settings, particularly in ICUs. A place that provides life support for critically ill or unconscious patients, ICU is the cornerstone of life extension for critically ill patients. However, because of a delayed immune response, reduced host defense, and use of invasive devices—central venous catheterizations, mechanical ventilation, and urinary tract catheterizations—patients in ICUs have an increased risk of infection. The morbidity and mortality of such infections have been reduced by the extensive use of antibiotics in recent decades. However, with the rise of the use of antibiotics in the treatment of microbial infections, increased selection pressures promote the emergence and dissemination of drug-resistant pathogens (Lipsitch and Samore, 2002; Esposito and Leone, 2007; Baker et al., 2018).
A significant positive association between antibiotic resistance rates and antibiotic consumption has been determined, together with a rising trend in antimicrobial resistance (Agodi et al., 2015). Carbapenems were once recognized as a pillar of treatment for clinical critical infections, but with their widespread use, resistance to carbapenems has increased as well. The emergence and dissemination of carbapenem-resistant non-fermenting Gram-negative bacilli (NFGNB) in ICUs pose a substantial threat in hospitals (Agarwal et al., 2017; Kousouli et al., 2019). Among these bacteria, CRAB is increasingly becoming one of the leading causes of healthcare-associated infections (HAIs), particularly in ICUs (Blanco et al., 2018; Busani et al., 2019; Chen et al., 2019; Tomczyk et al., 2019). In the Global Priority List of Antibiotic-Resistant Bacteria published by the World Health Organization (WHO) in 2017, CRAB was classified as among those bacteria for which antibiotics are most urgently needed (Tacconelli et al., 2018).
CRAB has been associated primarily with respiratory tract infections in ICUs, particularly ventilator-associated pneumonia (VAP; Nhu et al., 2014; Karruli et al., 2021; Khalil et al., 2021; Said et al., 2021; Pogue et al., 2022). Although no definitive agreement has been reached on the links between CRAB infections and an increased risk of mortality, CRAB infections have exhibited a significant association with the length of ICU stay, increased patient costs, and antibiotic use (Phu et al., 2017; Kousouli et al., 2019; Zhen et al., 2020; Liu Y. et al., 2020; Ejaz et al., 2021). Polymyxin currently remains effective as a treatment method for CRAB infections in ICUs (Garnacho-Montero et al., 2015; Sana et al., 2021). However, on an individual-patient basis, the use of polymyxin remains rather limited because of nephrotoxicity and neurotoxicity (Nazer et al., 2015b; Katip and Oberdorfer, 2021; Liu et al., 2021; Zhang N. et al., 2021). The emergence of polymyxin resistance in A. baumannii has also been reported (Cheah et al., 2016a,b; Carrasco et al., 2021). Under these conditions, the control and treatment of CRAB in ICUs can potentially face new challenges and have prompted growing concern in the medical community.
Therefore, evidence-based interventions to strengthen prevention and control initiatives are urgently needed. Funding, research, and development of new antimicrobials should pay increased attention to CRAB infections in ICUs. This review focuses on CRAB infections in ICUs and its transmission, mechanisms of resistance, treatment alternatives, and control strategies to provide a basis for prevention and control countermeasures for CRAB infections in ICUs.
CRAB in ICUs
CRAB infections of patients in ICUs
WHO estimates that about 30% of ICU patients are affected by at least one HAI in high-income countries; meanwhile, the frequency is at least two-fold to three-fold higher in middle- and low-income countries (World Health Organization, 2011). NFGNB is the leading cause of HAI, among which A. baumannii is an opportunistic pathogen that causes hospital-acquired septicemia, pneumonia, and urinary tract infections, particularly in ICUs (Antunes et al., 2014; Harding et al., 2018). Notably, as CRAB isolates in growing numbers have been isolated from patients, the prevalence and risk factors of CRAB infections have received increasing attention.
VAP, a severe complication, remains to be the most common infection acquired in ICUs (Kalanuria et al., 2014; Kalil et al., 2016). The pathogens responsible for VAP and their resistance mechanisms in ICUs are difficult to identify. The emergence and popularity of CRAB, which causes pulmonary infection in ICUs, have been reported in numerous publications. One multicenter prospective study found that multidrug-resistant Gram-negative bacteria, including A. baumannii, K. pneumoniae, and P. aeruginosa, are frequently associated with VAP in ICUs (Bandić-Pavlović et al., 2020). In a study conducted over a period of 46 months, Lambiase et al. demonstrated that A. baumannii isolated from patients with VAP in ICUs were resistant to carbapenem with imipenem MIC ≥ 16 μg/ml (Lambiase et al., 2012).
A retrospective study further found that the sputum separation rate of CRAB from ICUs was markedly higher than those from non-ICUs, and the resistance rate of CRAB showed a significantly rising trend (He et al., 2020). Similarly, 80% of CRAB in ICUs were isolated from sputum specimens, and CRAB comprised more than 50% of carbapenem-resistant Gram-negative bacilli (Karuniawati et al., 2013; Lăzureanu et al., 2016). Alternatively, another study showed that 58 of 61 A. baumannii isolates exhibited MICs with imipenem or meropenem≥16 μg/ml, and pulmonary infection was the most common site (26 of 36 cases; Mammina et al., 2012). As is widely known, the use of mechanical ventilation is strongly associated with the incidence of VAP. Therefore, when lung infection due to CRAB occurs in ICUs, the use of ventilators should be paid more attention than other wards to prevent cross-infection. However, beyond the use of mechanical ventilation, independent risk factors for CRAB causing pulmonary infections have been identified, such as previous stays in other departments, longer ICU stay, and previous use of carbapenems (Nazer et al., 2015a; Djordjevic et al., 2016). That is to say, a comprehensive infection control strategy is required to effectively control the emergence and spread of CRAB in ICUs.
Zhou et al. reported that the high mortality associated with bloodstream infections (BSIs) caused by A. baumannii has become a major clinical concern (Zhou et al., 2019). As such, increased attention should be paid to patients with CRAB bacteremia, apart from those with pulmonary CRAB infections. Invasive procedures and excessive use of antibiotics, particularly in patients with compromised immunity, are risk factors independently correlated with CRAB bacteremia (Kim et al., 2012; Shirmohammadlou et al., 2018). Previous studies have also found that colonization in the respiratory tract and gastrointestinal tract by CRAB is a crucial step before nosocomial infection (Lazareva et al., 2014; Bado et al., 2018; Kiddee et al., 2018; Maamar et al., 2018). Identifying risk factors and providing targeted interventions may become effective approaches to reducing the incidence of CRAB-causing HAIs.
Environmental contamination of CRAB in ICUs
A. baumannii can persist in the environment for long-term periods. A. baumannii, which ubiquitously and continuously persists in the hospital setting is one of the main sources of HAIs (Sunenshine et al., 2007). Ng et al. reported that environmental CRAB contamination was detected in nearly two-thirds of the rooms housing patients with CRAB (Ng et al., 2018). Previous studies have also indicated that cross-contamination of multidrug-resistant bacteria, specifically CRAB in ICUs, may occur via the air (Shimose et al., 2016), high-density electroencephalogram material (Weiss et al., 2016), Velcro on blood pressure cuffs (Alfandari et al., 2014), medical devices, furniture, and gloves (Raro et al., 2017). Uwingabiye et al. also observed genetic similarity between environmental and clinical CRAB isolates in 96.4% of all isolates (Uwingabiye et al., 2017). Given the intersection between patients and the environment, increasing studies have been devoted to the study of the extensive environmental colonization of CRAB in ICUs.
CRAB from environmental (environment and healthcare workers from ICUs) and clinical samples has been isolated and analyzed in several studies (Royer et al., 2015; Raro et al., 2017; Jain et al., 2019; Al-Hamad et al., 2020; Liu W. et al., 2020; Wang et al., 2021), and it was found that the overwhelming majority of CRAB isolated from clinical and environmental samples produced OXA-23, but no clone was specifically responsible for both environmental colonization and ICU infections. However, the experimental results of pulsed-field gel electrophoresis (PFGE) have revealed the spread of carbapenem-resistant isolates via cross-transmission among the environment and patients (Royer et al., 2015; Jain et al., 2019). Similarly, Salehi et al. (2018) and Raro et al. (2017) analyzed the isolates of A. baumannii from patients, staff, and the environment, and emphasized the circulation of CRAB as a nosocomial pathogen in different wards of hospitals, particularly in ICUs. Shenoy et al. also identified highly contaminated areas and confirmed the role of environmental reservoirs by investigating five clinical CRAB infection cases (Shenoy et al., 2020). It should be noted that although environmental contamination of CRAB in ICUs has gained increasing attention, research about whether specific clone was responsible for both environmental colonization and ICU infections is still lacking.
Further, a prospective surveillance study for 8 months has shown that more than half of the CRAB strains originating from the air are clonally associated with the clinical strains of nine patients in two medical ICUs with 20 beds total (Yakupogullari et al., 2016). The results of this study (Yakupogullari et al., 2016) suggested that infected patients can spread CRAB in large quantities to the air of an ICU, and these strains can still infect new patients after several months. This conclusion was verified by another study in China (Jiang et al., 2018). That is to say, special infection control measures may be required to prevent the airborne spread of CRAB in ICUs.
Colonization is usually regarded as a fundamental ecological process. Bacterial colonization of the surfaces is almost ubiquitous, especially in healthcare settings. In addition, the colonization of gastrointestinal tract, respiratory tract, urinary tract, and axilla is also receiving growing interest from researchers. Previous studies have found that colonization is an essential step in pathogen infections (Chipolombwe et al., 2016; Weiser et al., 2018; Tanimoto et al., 2019). Besides, numerous studies have shown that colonization is an important risk factor for subsequent infection (Huang et al., 2006; Haber et al., 2007; Martin et al., 2016; Gorrie et al., 2017). Studying the relationship between colonization and infections may bring new insights into disease prevention and treatment.
Interestingly, several studies have found that air and environmental surface contamination of CRAB were significantly greater among patients with respiratory tract colonization and gastrointestinal colonization than in other types of patients (Rosa et al., 2014; Shimose et al., 2016). Moreover, in a retrospective cohort study, significant associations were observed between CRAB colonization and clinical infections (Latibeaudiere et al., 2015). Namely, respiratory tract colonization and gastrointestinal colonization also play prominent roles in CRAB infections in ICU patients. Meanwhile, the relationship between environmental contamination and pathogen infections also cannot be neglected in general wards (Al-Hamad et al., 2020). Overall, environmental reservoirs of CRAB play a pivotal role in the HAIs of CRAB. An intensive study of environmental CRAB is beneficial to the control and elimination of CRAB infections in ICUs. The figure below shows the transmission relationship of CRAB among patients, health care workers, and the environment in ICUs (Figure 1).
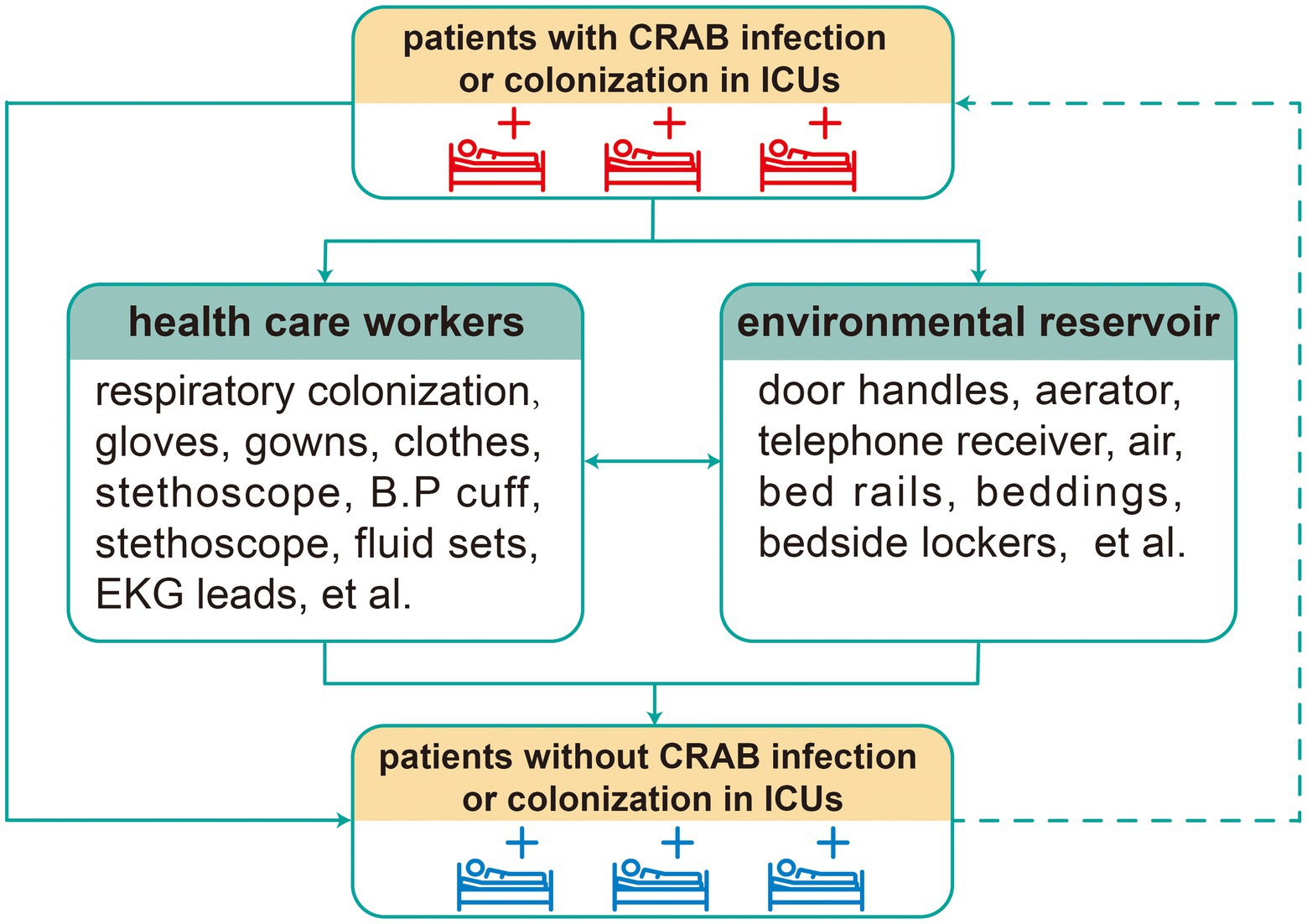
Figure 1. Transmission relationship of CRAB in ICUs. Solid arrow: bacterial infection and dissemination; dotted arrow: patients recently infected and colonized with CRAB are viewed as the new transmission source.
Drug resistance mechanism and homology of CRAB isolated from ICUs
The increase in drug-resistant bacterial infections leads to a heavy burden on healthcare systems globally. The study of drug resistance mechanisms is the first step to overcoming the infection of drug-resistant bacteria. CRAB has been classified by the WHO as one of the 12 top priority resistant bacteria presenting the most serious threat to public health (Tacconelli et al., 2018). As described previously, CRAB has been a major cause of HAIs (Sunenshine et al., 2007). Accordingly, a significant understanding of the mechanism underlying CRAB resistance is of major importance for drug development and clinical therapy.
An increasing number of researchers have conducted studies on drug resistance mechanisms and the homology of CRAB isolated from ICUs (Table 1). Details of the Table 1 reveal that most studies on the molecular characterization of CRAB in ICUs have been conducted in Asia and Africa, primarily in the developing world. Although the antibiotic resistance genes (ARGs) in the majority of studies have been dominated by blaOXA-23-like, differences in ARGs and CRAB molecular typing have been found between different countries and regions.
These literature reviews reflect the high diversity of the ARGs of CRAB isolated from ICUs globally. Zhang X. et al. (2021) investigated the phylogenetic relationships of 105 CRAB isolates from an ICU of a Chinese hospital and found that CRAB isolates contained 17 unique ARGs. And whole-genome sequencing (WGS) revealed the presence of blaADC-25, blaOXA-23, and blaOXA-66 in all strains, which belonged to Sequence type (ST) 2 (Zhang X. et al., 2021). A previous study indicated that carbapenem resistance was dominantly driven by the dissemination of CRAB isolates carrying blaOXA-23, belonging to ST2 (Mammina et al., 2012). A study conducted in Pakistan also found that the ST2 clone-harboring blaNDM-1 and blaOXA-23, which are widely distributed in ICUs, could prompt increased mortality (Ejaz et al., 2021). Notably, a study in another Chinese hospital in Shanghai reported the presence of blaOXA-23 in all CRAB strains (Wang et al., 2021), and it was found that the predominant clone of CRAB was ST208, which was consistent with the results obtained by Qian et al. (2015).
Unlike these studies, Zhang et al. found patterns of blaOXA-23 (93.4%), ISAba1/blaOXA-51-like (27.5%), blaOXA-24 (2.2%), blaOXA-58 (2.2%), and blaNDM-1 (8.8%) in CRAB strains (Zhang Y. et al., 2021). On the basis of their results (Zhang Y. et al., 2021), the ISAba1/blaOXA-51-like and blaOXA-23-like might be more relevant to resistance in CRAB. Although the ARGs in CRAB varied in different regions, blaOXA-23 was always the principal ARG in CRAB (Mahamat et al., 2016; Neves et al., 2016; Salehi et al., 2019). The blaOXA-23 is mostly found on plasmids, and Corvec et al. (2007) found that ISAba1 and ISAba4 which were detected upstream of the blaOXA-23 gene, provided promoter sequences for its expression.
In addition to blaOXA-23, ARGs such as blaOXA-24/40, blaOXA-51, blaOXA-58, and blaOXA-72 also play prominent roles in the drug resistance of CRAB (Schultz et al., 2016; Mavroidi et al., 2017; Chen et al., 2018b; Salehi et al., 2019; Zhang Y. et al., 2021). The blaOXA-24-like genes have been identified as chromosomally encoded. And Azizi et al. found that the isolates of A. baumannii with both blaOXA-23 and blaOXA-24 had strong biofilm-forming capability (Azizi et al., 2015). It is well known that biofilms can facilitate the development of antibiotic resistance by limiting bacterial exposure to antibiotics. Apart from the aforementioned genes, biofilms and AdeABC efflux-pump genes have also been detected in CRAB isolated from ICUs (Mavroidi et al., 2017; Chen et al., 2018a; Khalil et al., 2021). However, there are relatively few studies on biofilms and efflux-pump of CRAB in ICUs. It seems highly likely that the biofilms and efflux-pump of CRAB have not gained enough attention in ICUs.
The emergence of CRAB may be promoted by the adaptation and dissemination of a diverse group of successful clones. In a retrospective study of the drug resistance and distribution of pathogens isolated from the ICUs of 12 hospitals, Liu et al. found homology in the PFGE typing of CRAB (Liu W. et al., 2020). Moreover, Salehi et al. demonstrated that nine cross-existing clones consisting of eight cluster types and one ST were present between two hospitals (Salehi et al., 2019). Likewise, in South Africa, a study involving two hospitals found that ST106, ST229, ST258, and ST208 were established in both hospitals; meanwhile, ST339, ST502, and the novel ST1552 were established in Hospital B only, whereas ST848 was established in Hospital A only (Lowe et al., 2018). ST2 was identified as the most predominant isolate in Italy in several studies (Mammina et al., 2012; Mezzatesta et al., 2014; Schultz et al., 2016). It seems quite different from the distribution in other areas. In addition, resistance mechanisms and molecular epidemiology in the CRAB isolates varied between the general wards and ICUs. This result is supported by the PCR detection results of resistance genes, PFGE, and multilocus sequence typing (MLST) analysis in a recent study (Liu and Liu, 2021). The diverse ST types in different countries and regions have been listed in Table 1. Although CRAB infections have been reported all over the world, the lack of reports of molecular characterization targeted CRAB in ICUs is noticeable in some regions. Thus, further studies are needed to provide further evidence.
Overall, despite a certain degree of homology in CRAB from different ICUs, a high genetic diversity could not be overlooked. Further studies and investigations on the homology and drug resistance mechanism of CRAB in ICUs have important implications for reversing and reducing drug resistance, as well as developing control and treatment strategies.
Strategies and advice
Treatment strategies for CRAB infections
Guidelines for the treatment of CRAB infections
As previously mentioned, CRAB infections have become increasingly prevalent worldwide. The increasing healthcare burden caused by CRAB in ICUs has directed widespread attention to the treatment and control of CRAB. However, limited therapeutic options, as well as the long study period and task difficulty involved in new drug development, have prompted the increased interest among researchers devoting themselves to evaluating and improving treatment regimens on the basis of existing drugs. As clinical treatment options continued to be evaluated and studied, the European Society of Clinical Microbiology and Infectious Diseases (ESCMID) set guidelines in 2021 for the treatment of multidrug-resistant Gram-negative bacilli infections (Paul et al., 2022). The Infectious Diseases Society of America (IDSA) also recently updated the guidance document on the treatment of AmpC β-lactamase-producing Enterobacterales, CRAB, and infections caused by Stenotrophomonas maltophilia (Tamma et al., 2022). Both guidelines include therapeutic choices for CRAB infections. Subtle differences between these two guidance documents are observed despite the similarity of the majority of treatment strategies for CRAB. The current study presents a summary in Figure 2.
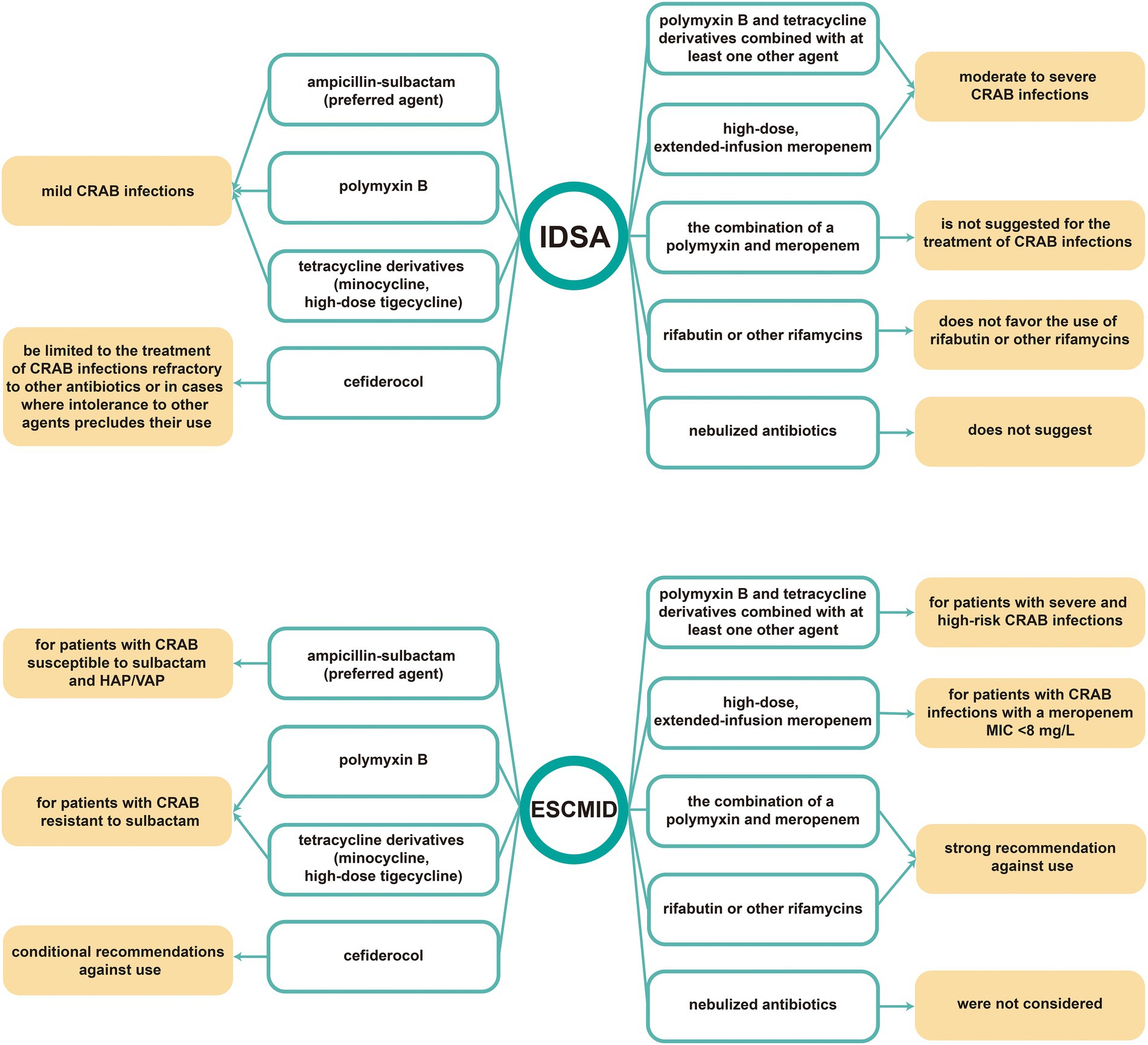
Figure 2. Treatment guidelines of CRAB infections for 2022 IDSA guidance and 2021 ESCMID guidance. IDSA: Infectious Diseases Society of America, ESCMID: European Society of Clinical Microbiology and Infectious Diseases.
In addition to the two guidelines by IDSA and ESCMID, similar guidelines including the treatment strategies related to CRAB infections have also been published in other regions and countries, such as China, Italy, and Arab countries of the Middle East (Guan et al., 2016; Al Salman et al., 2020; Tiseo et al., 2022). These recommendations present the distribution of diverse social and healthcare structures of different regions, as well as compensates for the deficiencies of international consensus guidelines. It is worth noting that, Table 1 lists China, Italy, and Arab countries of the Middle East as the main regions reporting CRAB infections in ICUs. In the consensus statement in Arab countries of the Middle East, A. baumannii infections are divided into two parts: (1) bacteremia and nosocomial pneumonia; (2) complicated urinary tract infection, and complicated skin and soft tissue infection. The treatment schemes, including first-choice therapy and duration, can vary based on the type of infection (Al Salman et al., 2020). In addition, three recommendations are presented in the guidance document in Italy with the following brief overview: (1) Consultation with specialists is recommended; (2) Rigorous monitoring of renal function is strongly recommended when colistin is administered; (3) Although cefiderocol represents a high-potential alternative for patients with CRAB infections, further studies need to be conducted to estimate the use of cefiderocol (Tiseo et al., 2022). In 2016, the Chinese consensus statement on the antimicrobial treatment of extensively drug-resistant Gram-negative bacilli (XDR-GNB) infections was released (Guan et al., 2016). This statement included the treatment strategies of XDR-GNB and was regarded as a reference for the treatment of CRAB infections. In 2019, the recommendations for antimicrobial treatment of CRAB infections were explicitly proposed in “Technical Guidelines for Prevention and Control of Carbapenem-resistant Gram-Negative Bacilli Infection in China” (Hu, 2019). An overview of these three guidelines is presented in Table 2 for a more intuitive comparison. The Table 2 shows that compared with the guidelines in Arab countries of the Middle East and China, the guidance document in Italy contains no detailed treatment strategies. Medical institutions in Italy were likely to comply with the guideline-recommended treatment strategies in ESCMID.
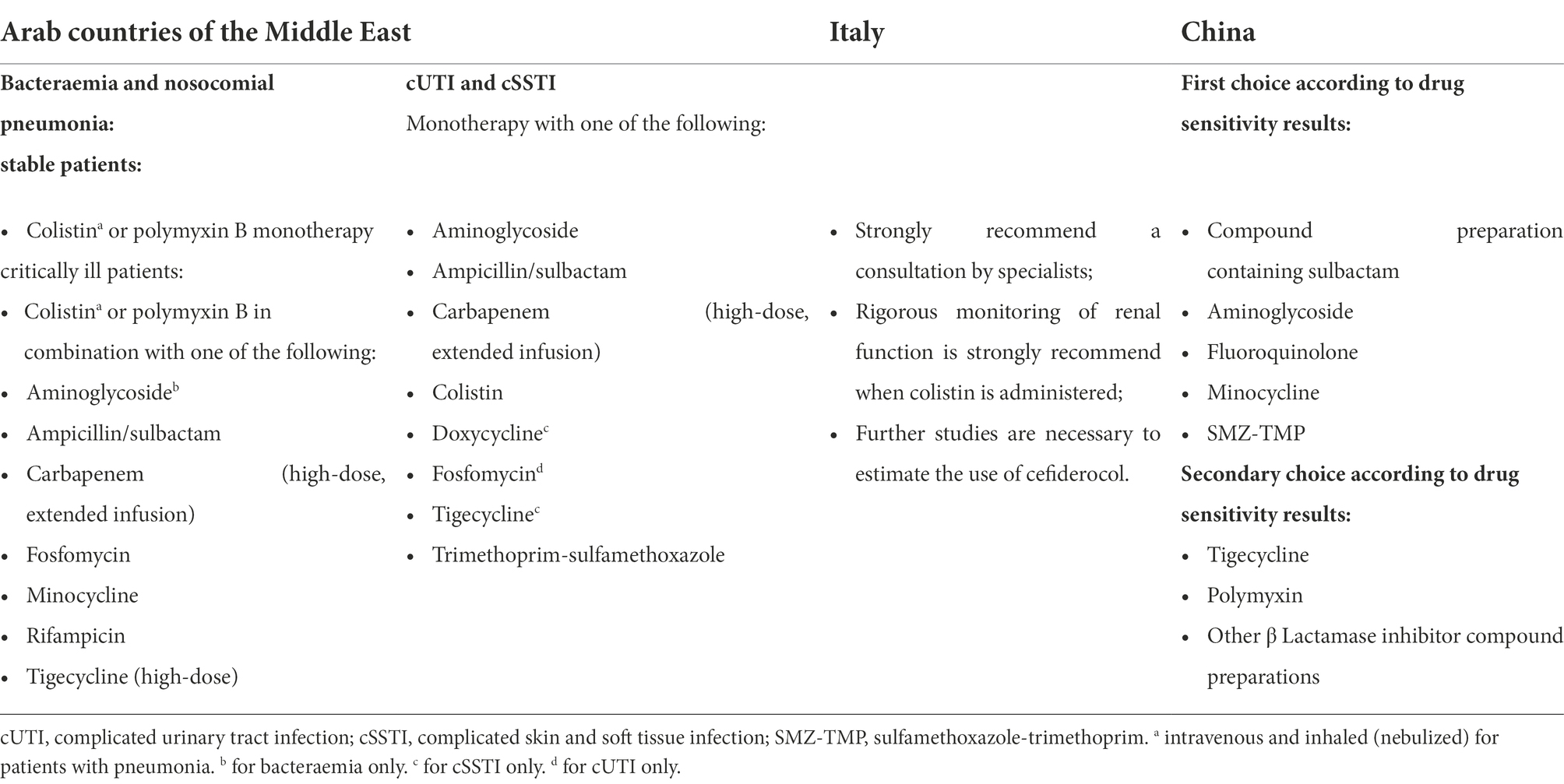
Table 2. Overview of the recommendations for antimicrobial treatment of CRAB infections in Arab countries in the Middle East, Italy, and China.
In summary, although many regions have proposed treatment guidelines and consensus on CRAB, the recommendations for the antimicrobial treatment of CRAB infections differed widely among different areas on the basis of the variations in antimicrobial availability, local preferences, and resistance patterns. Moreover, these recommendations need to be updated periodically in accordance to the evolution and spread of antibiotic resistance, and the advent of novel therapeutic strategies.
Other studies for treatment strategies
Despite the growing availability of guidelines, numerous questions about the treatment of CRAB infections arise. Ehrentraut et al. found that the use of colistin without drug concentration monitoring might be unsafe for critically ill patients, and treatment in accordance with guidelines does not ensure efficient target levels (Ehrentraut et al., 2020). The high frequency of isolation of CRAB in ICUs requires accurate antimicrobial susceptibility testing (AST), particularly to colistin, to ensure treatment precision (Sacco et al., 2021). Accordingly, drug concentration monitoring and the accurate application of AST are indispensable when treating with colistin; in addition, the safety assessment of colistin monotherapy requires further study. Moreover, in treating the BSIs of carbapenem-resistant NFGNB, high-dosage tigecycline (TGC) therapy was not superior to standard TGC dosing, and TGC-based combination antimicrobial therapy was not superior to monotherapy (Qu et al., 2021). Thus, whether TGC is suitable for the treatment of CRAB infections requires further research and verification.
In addition to the treatment alternatives included in these guidelines, other treatment strategies have also been recently examined in response to the development of antibiotic resistance. Phage therapy is regarded as a promising selection for treating pulmonary bacterial infections. Wu et al. reported that using a pre-optimized two-phage cocktail in ICUs can cause a significant decrease in CRAB burden (Wu et al., 2021). Other treatment strategies in recent years also included N-acetylcysteine plus antibiotics (Oliva et al., 2021), trimethoprim–sulfamethoxazole (Raz-Pasteur et al., 2019), and other sulbactam-based combinations (Qu et al., 2020). These therapeutic strategies may be further studied in the future. However, integrated strategies for the effective prevention and control of infection caused by drug-resistant bacteria have to be urgently developed because of the evolution and spread of antibiotic resistance, as well as existing challenges and limitations in pharmaceutical research.
Infection control strategies
Awareness of infection prevention and control has increased with intensified research in epidemiology. Agodi et al. indicated that infection control measures are equally important as the cautious use of antibiotics (Agodi et al., 2015). In 2017, WHO published the first global guidelines for the prevention and control of carbapenem-resistant Enterobacteriaceae–A. baumannii–P. aeruginosa in health care facilities, including eight evidence-based recommendations distilled by leading global experts (World Health Organization, 2017). Subsequently, the WHO global report on infection prevention and control provided a global situation analysis for policymakers at different levels, which can be used to facilitate the development of disease control strategies (World Health Organization, 2022). Accordingly, a growing number of healthcare workers have devoted themselves to the prevention and control of drug-resistant bacteria infections in ICUs. Thus, measures taken for CRAB infection control in ICUs are further discussed in the succeeding section.
Control of carbapenem use
The emergence, persistence, and dissemination of CRAB in ICUs limit therapeutic efficacy in critically ill patients. Antibiotic resistance is an ancient natural mechanism (Munita and Arias, 2016), but recent antibiotic usage effectively imposes selection pressure on ARGs. Selection pressure due to the use of antibiotics leads to the emergence, persistence, and dissemination of clinical resistant strains. Up to half of antibiotic courses might be inappropriate for use in ICUs (Barnes et al., 2017). Short-term carbapenem restriction effectively reduces the incidence of CRAB in ICUs (Ogutlu et al., 2014; Abdallah et al., 2019). Further, Munoz-Price et al. reported that every additive carbapenem-defined daily dose increased the risk of CRAB by 5.1% (Munoz-Price et al., 2016). Djordjevic et al. showed that previous use of carbapenems was a risk factor for CRAB infections in ICUs, and also demonstrated that appropriate policy of antibiotic utilization was an important measure that may decrease the incidence of such infections (Djordjevic et al., 2016). Therefore, controlling the usage of carbapenems to a certain degree can reduce the emergence and spread of CRAB in ICUs. However, the inevitable use of carbapenems prompts the need for stronger evidence to support and guide the use of antibiotics.
Early epidemiological screening for CRAB
As described previously (2.1), colonization by CRAB is a crucial step before nosocomial infection. Patients with CRAB intestinal colonization are more likely to develop CRAB infections, and admission screening of fecal carriage can prevent its spread (Maamar et al., 2018; Qiao et al., 2020). Culture processes and polymerase chain reaction (PCR) have long been regarded as the most common means of epidemiological screening for pathogens. Technological advancements have led to the development of new techniques to assist clinicians in early epidemiological screening for pathogens.
Investigators have detected A. baumannii and carbapenem resistance by loop-mediated isothermal amplification (LAMP) assay and found that LAMP was expected to act as an effective mean for early detection (Garciglia-Mercado et al., 2021; Sharma and Gaind, 2021). Rapid screening using LAMP assay followed by early intervention has also been reported to potentially decrease the transmission rates of CRAB in ICUs (Yamamoto et al., 2019). Li et al. optimized and evaluated the LAMP method, and their results showed that in A. baumannii detection, the sensitivity of LAMP was tenfold higher than that of PCR (Li et al., 2015). Garciglia-Mercado et al. reached a similar conclusion (Garciglia-Mercado et al., 2020). In addition to the LAMP assay, WGS was a valuable tool in epidemiological studies (Venditti et al., 2019). However, although the cost of sequencing has significantly decreased in recent years, WGS in epidemiological investigations remains extremely costly. Therefore, LAMP can potentially be used as an available screening method in epidemiologic investigations of CRAB, balancing sensitivity with the cost of detection.
Interventions
On the basis of the screening results, adequate control and disinfection measures can effectively limit the transmission of CRAB in ICUs. Chung et al. observed a 51.8% reduction in CRAB infection rates after daily chlorhexidine bathing in ICUs with CRAB endemicity (Chung et al., 2015). Other studies also indicated that daily bathing with 2% chlorhexidine gluconate can reduce CRAB cross-transmission among patients in ICUs with high CRAB endemicity (Hong et al., 2018; Metan et al., 2020; Suh et al., 2021). Moreover, environmental cleaning, isolation, and enhanced contact precautions are also integrated into strategies preventing CRAB cross-transmission among patients in ICUs because of the environmental reservoirs of CRAB.
Numerous studies have devoted themselves to preventing and addressing the environmental contamination and cross-transmission of CRAB in ICUs in recent years (Karampatakis et al., 2020; Dickstein et al., 2021; Jung et al., 2021; Kelly et al., 2021). First, the most significant point is that healthcare personnel education should be promoted to strengthen awareness and preparedness. Karampatakis et al. associated the increased infection rates for CRAB with work programs and behavioral factors (Karampatakis et al., 2020). Similarly, Kousouli et al. identified reduced compliance with hand hygiene and participation in educational courses as the most significant factor for CRAB bloodstream infection (Kousouli et al., 2018). A study in China found that after targeted surveillance, further implementation of infection control, including staff education, hand hygiene, and environmental cleaning can effectively prevent the spread of nosocomial CRAB infections (Zhao et al., 2019).
Second, new admissions should be separated from patients with colonization and infection, and their treatment should be handled by different medical staff members. An et al. isolated and grouped patients by CRAB culture results. They observed that the rate of CRAB infections and the use of colistin significantly decreased during the study period (An et al., 2017). Further, single-person isolation in ICUs was found to be an efficient method to prevent the transmission and hospital-acquired infections of CRAB (Guth et al., 2016; Jung et al., 2021).
Third, adequate environmental cleaning and disinfection also decrease the risk of transmission and infection of CRAB. Ben-Chetrit et al. showed that after environmental cleaning and hand hygiene, CRAB acquisition in ICUs considerably decreased from 54.6 to 1.9 (year 1) and 5.6 cases (year 2)/1,000 admissions (Ben-Chetrit et al., 2018). Various cleaning and disinfectant techniques have been widely used in health care settings to minimize HAIs. The use of phages as environmental sanitizers has been considered an alternative approach to removing bacterial contamination from the environment. Among the techniques reported, the use of phages as environmental sanitizers successfully decreased the rates of CRAB infection in ICUs (Ho et al., 2016; Chen et al., 2022). Steam technology (Oztoprak et al., 2019), terminal cleaning with sodium troclosene (Dickstein et al., 2021), installation of heat and moisture exchangers (Thatrimontrichai et al., 2020), ultraviolet-C, and aerosolized hydrogen peroxide (Kelly et al., 2021) have been successfully applied in environmental disinfection. By contrast, another study reported that strengthened environmental cleaning exhibited no association with the incidence (p = 0.156) and colonization pressure (p = 0.825) of CRAB in ICUs (Seok et al., 2021). The argument of whether decreasing the use of ventilators is more important than environmental cleaning was presented. A synthesis of the results of these studies indicates that controlling the use of ventilators for environmental disinfection may achieve significantly improved outcomes in infection control.
Figure 3 recapitulates the treatment and infection control of CRAB in ICUs. Collectively, developing an integrated system to monitor the microbial profiles, usage of antibiotics, and resistance profiles in ICUs, as well as combining multiple interventions, is necessary for infection control of CRAB in ICUs.
Conclusion
CRAB has progressed as a leading cause of HAIs worldwide, particularly in ICUs. Its spread and multiple-drug resistance considerably impedes the treatment of critically ill patients. On the basis of epidemiology and antibiotic resistance, the combined application of multiple interventions can effectively control the emergence and spread of CRAB, as well as provides hope for the control of CRAB infections in ICUs. Certainly, the implementation of control measures is of crucial importance and has to be extended to other wards for the eradication of CRAB from hospitals.
Author contributions
All authors participated in drafting and revising the review, contributed to the article, and approved the submitted version.
Funding
This study was supported by Sichuan Science and Technology Program (2022YFQ0093 and 2021YFH001).
Conflict of interest
The authors declare that the research was conducted in the absence of any commercial or financial relationships that could be construed as a potential conflict of interest.
Publisher’s note
All claims expressed in this article are solely those of the authors and do not necessarily represent those of their affiliated organizations, or those of the publisher, the editors and the reviewers. Any product that may be evaluated in this article, or claim that may be made by its manufacturer, is not guaranteed or endorsed by the publisher.
References
Abdallah, M., Badawi, M., Alzaagi, I., Issa, K. N., Rasheed, A., and Alharthy, A. (2019). Effect of short-term carbapenem restriction on the incidence of non-pseudomonal multi-drug resistant gram-negative bacilli in an intensive care unit. J. Chemother. 31, 261–266. doi: 10.1080/1120009x.2019.1601802
Agarwal, S., Kakati, B., Khanduri, S., and Gupta, S. (2017). Emergence of Carbapenem resistant non-fermenting gram-negative bacilli isolated in an ICU of a tertiary care hospital. J. Clin. Diagn. Res. 11, Dc04–Dc07. doi: 10.7860/jcdr/2017/24023.9317
Agodi, A., Auxilia, F., Barchitta, M., Brusaferro, S., D'Errico, M. M., Montagna, M. T., et al. (2015). Antibiotic consumption and resistance: results of the SPIN-UTI project of the GISIO-SItI. Epidemiol. Prev. 39, 94–98.
Al Salman, J., Al Dabal, L., Bassetti, M., Alfouzan, W. A., Al Maslamani, M., Alraddadi, B., et al. (2020). Management of infections caused by WHO critical priority gram-negative pathogens in Arab countries of the Middle East: a consensus paper. Int. J. Antimicrob. Agents 56:106104. doi: 10.1016/j.ijantimicag.2020.106104
Alfandari, S., Gois, J., Delannoy, P. Y., Georges, H., Boussekey, N., Chiche, A., et al. (2014). Management and control of a carbapenem-resistant Acinetobacter baumannii outbreak in an intensive care unit. Med. Mal. Infect. 44, 229–231. doi: 10.1016/j.medmal.2014.03.005
Al-Hamad, A., Pal, T., Leskafi, H., Abbas, H., Hejles, H., Alsubikhy, F., et al. (2020). Molecular characterization of clinical and environmental carbapenem resistant Acinetobacter baumannii isolates in a hospital of the eastern region of Saudi Arabia. J. Infect. Public Health 13, 632–636. doi: 10.1016/j.jiph.2019.08.013
An, J. H., Kim, Y. H., Moon, J. E., Jeong, J. H., Kim, S. H., Kang, S. J., et al. (2017). Active surveillance for carbapenem-resistant Acinetobacter baumannii in a medical intensive care unit: can it predict and reduce subsequent infections and the use of colistin? Am. J. Infect. Control 45, 667–672. doi: 10.1016/j.ajic.2017.01.016
Antunes, L. C. S., Visca, P., and Towner, K. J. (2014). Acinetobacter baumannii: evolution of a global pathogen. Pathog Dis 71, 292–301. doi: 10.1111/2049-632X.12125
Aruhomukama, D., Najjuka, C. F., Kajumbula, H., Okee, M., Mboowa, G., Sserwadda, I., et al. (2019). Bla VIM-and blaOXA-mediated carbapenem resistance among Acinetobacter baumannii and Pseudomonas aeruginosa isolates from the Mulago hospital intensive care unit in Kampala. Uganda. BMC Infect Dis 19:853. doi: 10.1186/s12879-019-4510-5
Azizi, O., Shakibaie, M. R., Modarresi, F., and Shahcheraghi, F. (2015). Molecular detection of class-D OXA Carbapenemase genes in biofilm and non-biofilm forming clinical isolates of Acinetobacter baumannii. Jundishapur J. Microbiology 8:e21042. doi: 10.5812/jjm.21042
Bado, I., Papa-Ezdra, R., Delgado-Blas, J. F., Gaudio, M., Gutiérrez, C., Cordeiro, N. F., et al. (2018). Molecular characterization of Carbapenem-resistant Acinetobacter baumannii in the intensive care unit of Uruguay's university hospital identifies the first rmtC gene in the species. Microb. Drug Resist. 24, 1012–1019. doi: 10.1089/mdr.2017.0300
Baker, S., Thomson, N., Weill, F. X., and Holt, K. E. (2018). Genomic insights into the emergence and spread of antimicrobial-resistant bacterial pathogens. Science New York, N.Y. 360, 733–738. doi: 10.1126/science.aar3777
Bandić-Pavlović, D., Zah-Bogović, T., Žižek, M., Bielen, L., Bratić, V., Hrabač, P., et al. (2020). Gram-negative bacteria as causative agents of ventilator-associated pneumonia and their respective resistance mechanisms. J. Chemother. 32, 344–358. doi: 10.1080/1120009x.2020.1793594
Barnes, S. L., Rock, C., Harris, A. D., Cosgrove, S. E., Morgan, D. J., and Thom, K. A. (2017). The impact of reducing antibiotics on the transmission of multidrug-resistant organisms. Infect. Control Hosp. Epidemiol. 38, 663–669. doi: 10.1017/ice.2017.34
Ben-Chetrit, E., Wiener-Well, Y., Lesho, E., Kopuit, P., Broyer, C., Bier, L., et al. (2018). An intervention to control an ICU outbreak of carbapenem-resistant Acinetobacter baumannii: long-term impact for the ICU and hospital. Crit. Care 22:319. doi: 10.1186/s13054-018-2247-y
Blanco, N., Harris, A. D., Rock, C., Johnson, J. K., Pineles, L., Bonomo, R. A., et al. (2018). Risk factors and outcomes associated with multidrug-resistant Acinetobacter baumannii upon intensive care unit admission. Antimicrob. Agents Chemother. 62:17. doi: 10.1128/AAC.01631-17
Busani, S., Serafini, G., Mantovani, E., Venturelli, C., Giannella, M., Viale, P., et al. (2019). Mortality in patients with septic shock by multidrug resistant bacteria: risk factors and impact of sepsis treatments. J. Intensive Care Med. 34, 48–54. doi: 10.1177/0885066616688165
Carrasco, L. D. D. M., Dabul, A. N. G., Boralli, C. M. D. S., Righetto, G. M., Carvalho, I. S. E., Dornelas, J. V., et al. (2021). Polymyxin resistance among XDR ST1 Carbapenem-resistant clone expanding in a teaching hospital. Front. Microbiol. 12:622704. doi: 10.3389/fmicb.2021.622704
Cheah, S.-E., Johnson, M. D., Zhu, Y., Tsuji, B. T., Forrest, A., Bulitta, J. B., et al. (2016a). Polymyxin resistance in Acinetobacter baumannii: genetic mutations and Transcriptomic changes in response to clinically relevant dosage regimens. Sci. Rep. 6:26233. doi: 10.1038/srep26233
Cheah, S.-E., Li, J., Tsuji, B. T., Forrest, A., Bulitta, J. B., and Nation, R. L. (2016b). Colistin and Polymyxin B dosage regimens against Acinetobacter baumannii: differences in activity and the emergence of resistance. Antimicrob. Agents Chemother. 60, 3921–3933. doi: 10.1128/AAC.02927-15
Chen, Y., Ai, L., Guo, P., Huang, H., Wu, Z., Liang, X., et al. (2018a). Molecular characterization of multidrug resistant strains of Acinetobacter baumannii isolated from pediatric intensive care unit in a Chinese tertiary hospital. BMC Infect. Dis. 18:614. doi: 10.1186/s12879-018-3511-0
Chen, L.-K., Chang, J.-C., Chu, H.-T., Chen, Y.-T., Jiang, H.-L., Wang, L.-S., et al. (2022). Preoptimized phage cocktail for use in aerosols against nosocomial transmission of carbapenem-resistant Acinetobacter baumannii: a 3-year prospective intervention study. Ecotoxicol. Environ. Saf. 236:113476. doi: 10.1016/j.ecoenv.2022.113476
Chen, Y. P., Liang, C. C., Chang, R., Kuo, C. M., Hung, C. H., Liao, T. N., et al. (2019). Detection and colonization of multidrug resistant organisms in a regional teaching Hospital of Taiwan. Int. J. Environ. Res. Public Health 16:1104. doi: 10.3390/ijerph16071104
Chen, Y., Yang, Y., Liu, L., Qiu, G., Han, X., Tian, S., et al. (2018b). High prevalence and clonal dissemination of OXA-72-producing Acinetobacter baumannii in a Chinese hospital: a cross sectional study. BMC Infect. Dis. 18:491. doi: 10.1186/s12879-018-3359-3
Chipolombwe, J., Török, M. E., Mbelle, N., and Nyasulu, P. (2016). Methicillin-resistant Staphylococcus aureus multiple sites surveillance: a systemic review of the literature. Infect Drug Resist 9, 35–42. doi: 10.2147/IDR.S95372
Chung, Y. K., Kim, J. S., Lee, S. S., Lee, J. A., Kim, H. S., Shin, K. S., et al. (2015). Effect of daily chlorhexidine bathing on acquisition of carbapenem-resistant Acinetobacter baumannii (CRAB) in the medical intensive care unit with CRAB endemicity. Am. J. Infect. Control 43, 1171–1177. doi: 10.1016/j.ajic.2015.07.001
Corvec, S., Poirel, L., Naas, T., Drugeon, H., and Nordmann, P. (2007). Genetics and expression of the carbapenem-hydrolyzing oxacillinase gene blaOXA-23 in Acinetobacter baumannii. Antimicrob. Agents Chemother. 51, 1530–1533. doi: 10.1128/AAC.01132-06
Dickstein, Y., Eluk, O., Warman, S., Aboalheja, W., Alon, T., Firan, I., et al. (2021). Wall painting following terminal cleaning with a chlorine solution as part of an intervention to control an outbreak of carbapenem-resistant Acinetobacter baumannii in a neurosurgical intensive care unit in Israel. J. Infect. Chemother. 27, 1423–1428. doi: 10.1016/j.jiac.2021.05.017
Djordjevic, Z. M., Folic, M. M., Folic, N. D., Gajovic, N., Gajovic, O., and Jankovic, S. M. (2016). Risk factors for hospital infections caused by carbapanem-resistant Acinetobacter baumannii. J. Infect. Dev. Ctries. 10, 1073–1080. doi: 10.3855/jidc.8231
Ehrentraut, S. F., Muenster, S., Kreyer, S., Theuerkauf, N. U., Bode, C., Steinhagen, F., et al. (2020). Extensive therapeutic drug monitoring of Colistin in critically ill patients reveals undetected risks. Microorganisms 8:415. doi: 10.3390/microorganisms8030415
Ejaz, H., Ahmad, M., Younas, S., Junaid, K., Abosalif, K. O. A., Abdalla, A. E., et al. (2021). Molecular epidemiology of extensively-drug resistant Acinetobacter baumannii sequence type 2 Co-harboring blaNDM and blaOXA from clinical origin. Infect Drug Resist 14, 1931–1939. doi: 10.2147/idr.S310478
Esposito, S., and Leone, S. (2007). Antimicrobial treatment for intensive care unit (ICU) infections including the role of the infectious disease specialist. Int. J. Antimicrob. Agents 29, 494–500. doi: 10.1016/j.ijantimicag.2006.10.017
Garciglia-Mercado, C., Gaxiola-Robles, R., Ascencio, F., Grajales-Muñiz, C., Soriano Rodríguez, M. L., Silva-Sanchez, J., et al. (2021). Antibacterial effect of acetic acid during an outbreak of carbapenem-resistant Acinetobacter baumannii in an ICU (II). J. Infect. Dev. Ctries. 15, 1167–1172. doi: 10.3855/jidc.11693
Garciglia-Mercado, C., Gaxiola-Robles, R., Ascencio, F., Silva-Sanchez, J., Estrada-Garcia, M. T., and Gomez-Anduro, G. (2020). Development of a LAMP method for detection of carbapenem-resistant Acinetobacter baumannii during a hospital outbreak. J. Infect. Dev. Ctries. 14, 494–501. doi: 10.3855/jidc.11692
Garnacho-Montero, J., Dimopoulos, G., Poulakou, G., Akova, M., Cisneros, J. M., De Waele, J., et al. (2015). Task force on management and prevention of Acinetobacter baumannii infections in the ICU. Intensive Care Med. 41, 2057–2075. doi: 10.1007/s00134-015-4079-4
Gorrie, C. L., Mirceta, M., Wick, R. R., Edwards, D. J., Thomson, N. R., Strugnell, R. A., et al. (2017). Gastrointestinal carriage is a major reservoir of Klebsiella pneumoniae infection in intensive care patients. Clinical Infectious Diseases: an Official Publication of the Infectious Diseases Society of America 65, 208–215. doi: 10.1093/cid/cix270
Guan, X., He, L., Hu, B., Hu, J., Huang, X., Lai, G., et al. (2016). Laboratory diagnosis, clinical management and infection control of the infections caused by extensively drug-resistant gram-negative bacilli: a Chinese consensus statement. Clin. Microbiol. Infect. 22, S15–S25. doi: 10.1016/j.cmi.2015.11.004
Guo, J., and Li, C. (2019). Molecular epidemiology and decreased susceptibility to disinfectants in carbapenem-resistant Acinetobacter baumannii isolated from intensive care unit patients in Central China. J. Infect. Public Health 12, 890–896. doi: 10.1016/j.jiph.2019.06.007
Guth, C., Cavalli, Z., Pernod, C., Lhopital, C., Wey, P., Gerome, P., et al. (2016). Prompt control of an imported carbapenem-resistant Acinetobacter baumanii outbreak in a French intensive care unit. Medecine Et Sante Tropicales 26, 110–112. doi: 10.1684/mst.2015.0517
Haber, N., Paute, J., Gouot, A., Sevali Garcia, J., Rouquet, M. L., Sahraoui, L., et al. (2007). Incidence and clinical characteristics of symptomatic urinary infections in a geriatric hospital. Medecine Et Maladies Infectieuses 37, 664–672. doi: 10.1016/j.medmal.2006.12.004
Harding, C. M., Hennon, S. W., and Feldman, M. F. (2018). Uncovering the mechanisms of Acinetobacter baumannii virulence. Nat. Rev. Microbiol. 16:148. doi: 10.1038/nrmicro.2017.148
He, S., Li, Z., Yang, Q., Quan, M., Zhao, L., and Hong, Z. (2020). Resistance trends among 1, 294 nosocomial Acinetobacter baumannii strains from a tertiary general Hospital in China, 2014-2017. Clin. Lab. 66:629. doi: 10.7754/Clin.Lab.2019.190629
Ho, Y.-H., Tseng, C.-C., Wang, L.-S., Chen, Y.-T., Ho, G.-J., Lin, T.-Y., et al. (2016). Application of bacteriophage-containing aerosol against nosocomial transmission of Carbapenem-resistant Acinetobacter baumannii in an intensive care unit. Plo S One 11:e0168380. doi: 10.1371/journal.pone.0168380
Hong, J., Jang, O. J., Bak, M. H., Baek, E. H., Park, K. H., Hong, S. I., et al. (2018). Management of carbapenem-resistant Acinetobacter baumannii epidemic in an intensive care unit using multifaceted intervention strategy. Korean J. Intern. Med. 33, 1000–1007. doi: 10.3904/kjim.2016.323
Hu, B. (2019). Technical guidelines for prevention and control of carbapenem-resistant gram-negative bacilli infection in China. Chinese J. Nosocomiology 29, 2075–2080. doi: 10.11816/cn.ni.2019-191088
Huang, Y.-C., Chou, Y.-H., Su, L.-H., Lien, R.-I., and Lin, T.-Y. (2006). Methicillin-resistant Staphylococcus aureus colonization and its association with infection among infants hospitalized in neonatal intensive care units. Pediatrics 118, 469–474. doi: 10.1542/peds.2006-0254
Jain, M., Sharma, A., Sen, M. K., Rani, V., Gaind, R., and Suri, J. C. (2019). Phenotypic and molecular characterization of Acinetobacter baumannii isolates causing lower respiratory infections among ICU patients. Microb. Pathog. 128, 75–81. doi: 10.1016/j.micpath.2018.12.023
Jiang, M., Mu, Y., Li, N., Zhang, Z., and Han, S. (2018). Carbapenem-resistant from air and patients of intensive care units. Pol. J. Microbiol. 67, 333–338. doi: 10.21307/pjm-2018-040
Jung, J., Choe, P. G., Choi, S., Kim, E., Lee, H. Y., Kang, C. K., et al. (2021). Reduction in the acquisition rate of carbapenem-resistant Acinetobacter baumannii (CRAB) after room privatization in an intensive care unit. J. Hosp. Infect. 121, 14–21. doi: 10.1016/j.jhin.2021.12.012
Kalanuria, A. A., Ziai, W., and Mirski, M. (2014). Ventilator-associated pneumonia in the ICU. Crit. Care 18:208. doi: 10.1186/cc13775
Kalil, A. C., Metersky, M. L., Klompas, M., Muscedere, J., Sweeney, D. A., Palmer, L. B., et al. (2016). Management of Adults with Hospital-acquired and Ventilator-associated Pneumonia: 2016 clinical practice guidelines by the Infectious Diseases Society of America and the American Thoracic Society. Clin. Infect. Dis. 63:353. doi: 10.1093/cid/ciw353
Karampatakis, T., Tsergouli, K., Iosifidis, E., Antachopoulos, C., Mouloudi, E., Karyoti, A., et al. (2020). Forecasting models of infections due to carbapenem-resistant gram-negative bacteria in an intensive care unit in an endemic area. J Glob Antimicrob Resist 20, 214–218. doi: 10.1016/j.jgar.2019.06.019
Karruli, A., Boccia, F., Gagliardi, M., Patauner, F., Ursi, M. P., Sommese, P., et al. (2021). Multidrug-resistant infections and outcome of critically ill patients with coronavirus disease 2019: a single center experience. Microb. Drug Resist. 27, 1167–1175. doi: 10.1089/mdr.2020.0489
Karuniawati, A., Saharman, Y. R., and Lestari, D. C. (2013). Detection of carbapenemase encoding genes in Enterobacteriace, Pseudomonas aeruginosa, and Acinetobacter baumanii isolated from patients at intensive care unit Cipto Mangunkusumo hospital in 2011. Acta Med. Indones. 45, 101–106.
Katip, W., and Oberdorfer, P. (2021). Clinical efficacy and nephrotoxicity of Colistin alone versus Colistin plus Vancomycin in critically ill patients infected with Carbapenem-resistant Acinetobacter baumannii: a propensity score-matched analysis. Pharmaceutics 13:162. doi: 10.3390/pharmaceutics13020162
Kelly, S., Schnugh, D., and Thomas, T. (2021). Effectiveness of ultraviolet-C vs aerosolized hydrogen peroxide in ICU terminal disinfection. J. Hosp. Infect. 121, 114–119. doi: 10.1016/j.jhin.2021.12.004
Khalil, M. A. F., Ahmed, F. A., Elkhateeb, A. F., Mahmoud, E. E., Ahmed, M. I., Ahmed, R. I., et al. (2021). Virulence characteristics of biofilm-forming Acinetobacter baumannii in clinical isolates using a galleria mellonella model. Microorganisms 9:365. doi: 10.3390/microorganisms9112365
Kiddee, A., Assawatheptawee, K., Na-Udom, A., Treebupachatsakul, P., Wangteeraprasert, A., Walsh, T. R., et al. (2018). Risk factors for gastrointestinal colonization and Acquisition of Carbapenem-Resistant Gram-Negative Bacteria among patients in intensive care units in Thailand. Antimicrob. Agents Chemother. 62:18. doi: 10.1128/aac.00341-18
Kim, S. Y., Jung, J. Y., Kang, Y. A., Lim, J. E., Kim, E. Y., Lee, S. K., et al. (2012). Risk factors for occurrence and 30-day mortality for carbapenem-resistant Acinetobacter baumannii bacteremia in an intensive care unit. J. Korean Med. Sci. 27, 939–947. doi: 10.3346/jkms.2012.27.8.939
Kousouli, E., Zarkotou, O., Polimeri, K., Themeli-Digalaki, K., and Pournaras, S. (2019). Impact of bloodstream infections caused by carbapenem-resistant gram-negative pathogens on ICU costs, mortality and length of stay. Infect Prev Pract 1:100020. doi: 10.1016/j.infpip.2019.100020
Kousouli, E., Zarkotou, O., Politi, L., Polimeri, K., Vrioni, G., Themeli-Digalaki, K., et al. (2018). Infection control interventions affected by resource shortages: impact on the incidence of bacteremias caused by carbapenem-resistant pathogens. Eur. J. Clin. Microbiol. Infect. Dis. 37, 43–50. doi: 10.1007/s10096-017-3098-1
Lambiase, A., Piazza, O., Rossano, F., Del Pezzo, M., Tufano, R., and Catania, M. R. (2012). Persistence of carbapenem-resistant Acinetobacter baumannii strains in an Italian intensive care unit during a forty-six month study period. New Microbiol. 35, 199–206.
Latibeaudiere, R., Rosa, R., Laowansiri, P., Arheart, K., Namias, N., and Munoz-Price, L. S. (2015). Surveillance cultures growing carbapenem-resistant Acinetobacter baumannii predict the development of clinical infections: a retrospective cohort study. Clin. Infect. Dis. 60, 415–422. doi: 10.1093/cid/ciu847
Lazareva, A. V., Katosova, L. K., Kryzhanovskaya, O. A., Ponomarenko, O. A., Karaseva, O. V., Gorelik, A. L., et al. (2014). Monitoring and antibiotic resistance profile of tracheal aspirate microbiota in ICU children with severe craniocerebral trauma. Antibiot Khimioter 59, 8–15.
Lăzureanu, V., Poroșnicu, M., Gândac, C., Moisil, T., Bădițoiu, L., Laza, R., et al. (2016). Infection with Acinetobacter baumannii in an intensive care unit in the Western part of Romania. BMC Infect. Dis. 16:95. doi: 10.1186/s12879-016-1399-0
Li, P., Niu, W., Li, H., Lei, H., Liu, W., Zhao, X., et al. (2015). Rapid detection of Acinetobacter baumannii and molecular epidemiology of carbapenem-resistant a. baumannii in two comprehensive hospitals of Beijing, China. Front. Microbiol. 6:997. doi: 10.3389/fmicb.2015.00997
Lipsitch, M., and Samore, M. H. (2002). Antimicrobial use and antimicrobial resistance: a population perspective. Emerg. Infect. Dis. 8, 347–354. doi: 10.3201/eid0804.010312
Liu, X., Chen, Y., Yang, H., Li, J., Yu, J., Yu, Z., et al. (2021). Acute toxicity is a dose-limiting factor for intravenous polymyxin B: a safety and pharmacokinetic study in healthy Chinese subjects. J. Infect. 82, 207–215. doi: 10.1016/j.jinf.2021.01.006
Liu, B., and Liu, L. (2021). Molecular epidemiology and mechanisms of Carbapenem-resistant Acinetobacter baumannii isolates from ICU and respiratory department patients of a Chinese university hospital. Infect Drug Resist 14, 743–755. doi: 10.2147/idr.S299540
Liu, Y., Wang, Q., Zhao, C., Chen, H., Li, H., Wang, H., et al. (2020). Prospective multi-center evaluation on risk factors, clinical characteristics and outcomes due to carbapenem resistance in Acinetobacter baumannii complex bacteraemia: experience from the Chinese antimicrobial resistance surveillance of nosocomial infections (CARES) Network. J. Med. Microbiol. 69, 949–959. doi: 10.1099/jmm.0.001222
Liu, W., Yang, Y., Zhang, K., Hai, Y., Li, H., Jiao, Y., et al. (2020). Drug resistance of healthcare-associated pathogenic bacteria and carbapenem-resistant Acinetobacter baumannii homology in the general intensive care unit. Ann Palliat Med 9, 1545–1555. doi: 10.21037/apm-19-632
Lowe, M., Ehlers, M. M., Ismail, F., Peirano, G., Becker, P. J., Pitout, J. D. D., et al. (2018). Acinetobacter baumannii: epidemiological and Beta-lactamase data from two tertiary academic hospitals in Tshwane, South Africa. Front Microbiol 9:1280. doi: 10.3389/fmicb.2018.01280
Maamar, E., Alonso, C. A., Ferjani, S., Jendoubi, A., Hamzaoui, Z., Jebri, A., et al. (2018). NDM-1-and OXA-23-producing Acinetobacter baumannii isolated from intensive care unit patients in Tunisia. Int. J. Antimicrob. Agents 52, 910–915. doi: 10.1016/j.ijantimicag.2018.04.008
Mahamat, A., Bertrand, X., Moreau, B., Hommel, D., Couppie, P., Simonnet, C., et al. (2016). Clinical epidemiology and resistance mechanisms of carbapenem-resistant Acinetobacter baumannii, French Guiana, 2008-2014. Int. J. Antimicrob. Agents 48, 51–55. doi: 10.1016/j.ijantimicag.2016.03.006
Mammina, C., Palma, D. M., Bonura, C., Aleo, A., Fasciana, T., Sodano, C., et al. (2012). Epidemiology and clonality of carbapenem-resistant Acinetobacter baumannii from an intensive care unit in Palermo, Italy. BMC Res Notes 5:365. doi: 10.1186/1756-0500-5-365
Martin, R. M., Cao, J., Brisse, S., Passet, V., Wu, W., Zhao, L., et al. (2016). Molecular epidemiology of colonizing and infecting isolates of. MSphere 1:e00261. doi: 10.1128/mSphere.00261-16
Mavroidi, A., Katsiari, M., Palla, E., Likousi, S., Roussou, Z., Nikolaou, C., et al. (2017). Investigation of extensively drug-resistant Bla OXA-23-producing Acinetobacter baumannii spread in a Greek hospital. Microb. Drug Resist. 23, 488–493. doi: 10.1089/mdr.2016.0101
Metan, G., Zarakolu, P., Otlu, B., Tekin, İ., Aytaç, H., Bölek, E., et al. (2020). Emergence of colistin and carbapenem-resistant Acinetobacter calcoaceticus-Acinetobacter baumannii (CCR-Acb) complex in a neurological intensive care unit followed by successful control of the outbreak. J. Infect. Public Health 13, 564–570. doi: 10.1016/j.jiph.2019.09.013
Mezzatesta, M. L., Caio, C., Gona, F., Cormaci, R., Salerno, I., Zingali, T., et al. (2014). Carbapenem and multidrug resistance in gram-negative bacteria in a single Centre in Italy: considerations on in vitro assay of active drugs. Int. J. Antimicrob. Agents 44, 112–116. doi: 10.1016/j.ijantimicag.2014.04.014
Munita, J. M., and Arias, C. A. (2016). Mechanisms of antibiotic resistance. Microbiol Spectr 4:2015. doi: 10.1128/microbiolspec.VMBF-0016-2015
Munoz-Price, L. S., Rosa, R., Castro, J. G., Laowansiri, P., Latibeaudiere, R., Namias, N., et al. (2016). Evaluating the impact of antibiotic exposures as time-dependent variables on the acquisition of Carbapenem-resistant Acinetobacter baumannii. Crit. Care Med. 44, e949–e956. doi: 10.1097/ccm.0000000000001848
Nazer, L. H., Kharabsheh, A., Rimawi, D., Mubarak, S., and Hawari, F. (2015a). Characteristics and outcomes of Acinetobacter baumannii infections in critically ill patients with cancer: a matched case-control study. Microb. Drug Resist. 21, 556–561. doi: 10.1089/mdr.2015.0032
Nazer, L. H., Rihani, S., Hawari, F. I., and Le, J. (2015b). High-dose colistin for microbiologically documented serious respiratory infections associated with carbapenem-resistant Acinetobacter baummannii in critically ill cancer patients: a retrospective cohort study. Infect Dis (Lond) 47, 755–760. doi: 10.3109/23744235.2015.1055586
Neves, F. C., Clemente, W. T., Lincopan, N., Paião, I. D., Neves, P. R., Romanelli, R. M., et al. (2016). Clinical and microbiological characteristics of OXA-23-and OXA-143-producing Acinetobacter baumannii in ICU patients at a teaching hospital. Brazil. Braz J Infect Dis 20, 556–563. doi: 10.1016/j.bjid.2016.08.004
Ng, D. H. L., Marimuthu, K., Lee, J. J., Khong, W. X., Ng, O. T., Zhang, W., et al. (2018). Environmental colonization and onward clonal transmission of carbapenem-resistant (CRAB) in a medical intensive care unit: the case for environmental hygiene. Antimicrob. Resist. Infect. Control 7:51. doi: 10.1186/s13756-018-0343-z
Nhu, N. T. K., Lan, N. P. H., Campbell, J. I., Parry, C. M., Thompson, C., Tuyen, H. T., et al. (2014). Emergence of carbapenem-resistant Acinetobacter baumannii as the major cause of ventilator-associated pneumonia in intensive care unit patients at an infectious disease hospital in southern Vietnam. J. Med. Microbiol. 63, 1386–1394. doi: 10.1099/jmm.0.076646-0
Odih, E. E., Irek, E. O., Obadare, T. O., Oaikhena, A. O., Afolayan, A. O., Underwood, A., et al. (2022). Rectal colonization and nosocomial transmission of Carbapenem-resistant in an intensive care unit, Southwest Nigeria. Front. Medicine 9:846051. doi: 10.3389/fmed.2022.846051
Ogutlu, A., Guclu, E., Karabay, O., Utku, A. C., Tuna, N., and Yahyaoglu, M. (2014). Effects of Carbapenem consumption on the prevalence of Acinetobacter infection in intensive care unit patients. Ann. Clin. Microbiol. Antimicrob. 13:7. doi: 10.1186/1476-0711-13-7
Oliva, A., Bianchi, A., Russo, A., Ceccarelli, G., Cancelli, F., Aloj, F., et al. (2021). Effect of N-Acetylcysteine administration on 30-day mortality in critically ill patients with septic shock caused by Carbapenem-resistant Klebsiella pneumoniae and Acinetobacter baumannii: a retrospective case-control study. Antibiotics (Basel) 10:271. doi: 10.3390/antibiotics10030271
Oztoprak, N., Kizilates, F., and Percin, D. (2019). Comparison of steam technology and a two-step cleaning (water/detergent) and disinfecting (1,000 resp. 5,000 ppm hypochlorite) method using microfiber cloth for environmental control of multidrug-resistant organisms in an intensive care unit. GMS Hyg. Infect. Control. 14:Doc15. doi: 10.3205/dgkh000330
Paul, M., Carrara, E., Retamar, P., Tängdén, T., Bitterman, R., Bonomo, R. A., et al. (2022). European Society of Clinical Microbiology and Infectious Diseases (ESCMID) guidelines for the treatment of infections caused by multidrug-resistant gram-negative bacilli (endorsed by European society of intensive care medicine). Clin. Microbiol. Infect. 28, 521–547. doi: 10.1016/j.cmi.2021.11.025
Phu, V. D., Nadjm, B., Duy, N. H. A., Co, D. X., Mai, N. T. H., Trinh, D. T., et al. (2017). Ventilator-associated respiratory infection in a resource-restricted setting: impact and etiology. J. Intensive Care 5:69. doi: 10.1186/s40560-017-0266-4
Pogue, J. M., Zhou, Y., Kanakamedala, H., and Cai, B. (2022). Burden of illness in carbapenem-resistant Acinetobacter baumannii infections in US hospitals between 2014 and 2019. BMC Infect. Dis. 22:36. doi: 10.1186/s12879-021-07024-4
Qian, Y., Dong, X., Wang, Z., Yang, G., and Liu, Q. (2015). Distributions and types of multidrug-resistant Acinetobacter baumannii in different departments of a general hospital. Jundishapur J Microbiol 8:e22935. doi: 10.5812/jjm.22935
Qiao, F., Huang, W., Gao, S., Cai, L., Zhu, S., Wei, L., et al. (2020). Risk factor for intestinal carriage of carbapenem-resistant Acinetobacter baumannii and the impact on subsequent infection among patients in an intensive care unit: an observational study. BMJ Open 10:e035893. doi: 10.1136/bmjopen-2019-035893
Qu, J., Feng, C., Li, H., and Lv, X. (2021). Antibiotic strategies and clinical outcomes for patients with carbapenem-resistant gram-negative bacterial bloodstream infection. Int. J. Antimicrob. Agents 57:106284. doi: 10.1016/j.ijantimicag.2021.106284
Qu, J., Yu, R., Wang, Q., Feng, C., and Lv, X. (2020). Synergistic antibacterial activity of combined antimicrobials and the clinical outcome of patients with Carbapenemase-producing Acinetobacter baumannii infection. Front. Microbiol. 11:541423. doi: 10.3389/fmicb.2020.541423
Ramadan, R. A., Gebriel, M. G., Kadry, H. M., and Mosallem, A. (2018). Carbapenem-resistant Acinetobacter baumannii and Pseudomonas aeruginosa: characterization of carbapenemase genes and E-test evaluation of colistin-based combinations. Infect Drug Resist 11, 1261–1269. doi: 10.2147/idr.S170233
Raro, O. H. F., Gallo, S. W., Ferreira, C. A. S., and Oliveira, S. D. (2017). Carbapenem-resistant Acinetobacter baumannii contamination in an intensive care unit. Rev. Soc. Bras. Med. Trop. 50, 167–172. doi: 10.1590/0037-8682-0329-2016
Raz-Pasteur, A., Liron, Y., Amir-Ronen, R., Abdelgani, S., Ohanyan, A., Geffen, Y., et al. (2019). Trimethoprim-sulfamethoxazole vs. colistin or ampicillin-sulbactam for the treatment of carbapenem-resistant Acinetobacter baumannii: a retrospective matched cohort study. J Glob Antimicrob Resist 17, 168–172. doi: 10.1016/j.jgar.2018.12.001
Rosa, R., Depascale, D., Cleary, T., Fajardo-Aquino, Y., Kett, D. H., and Munoz-Price, L. S. (2014). Differential environmental contamination with Acinetobacter baumannii based on the anatomic source of colonization. Am. J. Infect. Control 42, 755–757. doi: 10.1016/j.ajic.2014.03.016
Royer, S., Faria, A. L., Seki, L. M., Chagas, T. P., Campos, P. A., Batistão, D. W., et al. (2015). Spread of multidrug-resistant Acinetobacter baumannii and Pseudomonas aeruginosa clones in patients with ventilator-associated pneumonia in an adult intensive care unit at a university hospital. Braz. J. Infect. Dis. 19, 350–357. doi: 10.1016/j.bjid.2015.03.009
Sacco, F., Visca, P., Runci, F., Antonelli, G., and Raponi, G. (2021). Susceptibility testing of Colistin for: how far are we from the truth? Antibiotics (Basel, Switzerland) 10:48. doi: 10.3390/antibiotics10010048
Said, K. B., Alsolami, A., Khalifa, A. M., Khalil, N. A., Moursi, S., Osman, A., et al. (2021). A multi-point surveillance for antimicrobial resistance profiles among clinical isolates of gram-negative bacteria recovered from major Ha'il hospitals, Saudi Arabia. Microorganisms 9:024. doi: 10.3390/microorganisms9102024
Salehi, B., Ghalavand, Z., Mohammadzadeh, M., Maleki, D. T., Kodori, M., and Kadkhoda, H. (2019). Clonal relatedness and resistance characteristics of OXA-24 and-58 producing carbapenem-resistant Acinetobacter baumannii isolates in Tehran. Iran. J Appl Microbiol 127, 1421–1429. doi: 10.1111/jam.14409
Salehi, B., Goudarzi, H., Nikmanesh, B., Houri, H., Alavi-Moghaddam, M., and Ghalavand, Z. (2018). Emergence and characterization of nosocomial multidrug-resistant and extensively drug-resistant Acinetobacter baumannii isolates in Tehran. Iran. J Infect Chemother 24, 515–523. doi: 10.1016/j.jiac.2018.02.009
Sana, F., Hussain, A., Hussain, W., Zaman, G., Abbas, M. W., Imtiaz, A., et al. (2021). Frequency and clinical Spectrum of multidrug resistant Acinetobacter Baumannii as a significant nosocomial pathogen in intensive care unit patients. J. Ayub Med. Coll. Abbottabad 33, S752–s756.
Schultz, M. B., Pham Thanh, D., Do HoanN, T., Wick, R. R., Ingle, D. J., Hawkey, J., et al. (2016). Repeated local emergence of carbapenem-resistant Acinetobacter baumannii in a single hospital ward. Microb Genom 2:e000050. doi: 10.1099/mgen.0.000050
Seok, H., Jeon, J. H., Jung, J. H., Ahn, S. H., Seo, M., Cho, H. K., et al. (2021). Does enhanced environmental cleaning reduce carbapenem-resistant Acinetobacter baumannii colonization in the intensive care unit? Int. J. Infect. Dis. 109, 72–76. doi: 10.1016/j.ijid.2021.06.065
Sharma, A., and Gaind, R. (2021). Development of loop-mediated isothermal amplification assay for detection of clinically significant members of Acinetobacter calcoaceticus-baumannii complex and associated Carbapenem resistance. Front. Mol. Biosci. 8:659256. doi: 10.3389/fmolb.2021.659256
Shenoy, E. S., Pierce, V. M., Sater, M. R. A., Pangestu, F. K., Herriott, I. C., Anahtar, M. N., et al. (2020). Community-acquired in name only: a cluster of carbapenem-resistant Acinetobacter baumannii in a burn intensive care unit and beyond. Infect. Control Hosp. Epidemiol. 41, 531–538. doi: 10.1017/ice.2020.15
Shimose, L. A., Masuda, E., Sfeir, M., Berbel Caban, A., Bueno, M. X., de Pascale, D., et al. (2016). Carbapenem-resistant Acinetobacter baumannii: concomitant contamination of air and environmental surfaces. Infect. Control Hosp. Epidemiol. 37, 777–781. doi: 10.1017/ice.2016.69
Shirmohammadlou, N., Zeighami, H., Haghi, F., and Kashefieh, M. (2018). Resistance pattern and distribution of carbapenemase and antiseptic resistance genes among multidrug-resistant Acinetobacter baumannii isolated from intensive care unit patients. J. Med. Microbiol. 67, 1467–1473. doi: 10.1099/jmm.0.000826
Suh, J. W., Kim, N. H., Lee, M. J., Lee, S. E., Chun, B. C., Lee, C. K., et al. (2021). Real-world experience of how chlorhexidine bathing affects the acquisition and incidence of vancomycin-resistant enterococci (VRE) in a medical intensive care unit with VRE endemicity: a prospective interrupted time-series study. Antimicrob. Resist. Infect. Control 10:160. doi: 10.1186/s13756-021-01030-6
Sunenshine, R. H., Wright, M.-O., Maragakis, L. L., Harris, A. D., Song, X., Hebden, J., et al. (2007). Multidrug-resistant Acinetobacter infection mortality rate and length of hospitalization. Emerg. Infect. Dis. 13, 97–103. doi: 10.3201/eid1301.060716
Tacconelli, E., Carrara, E., Savoldi, A., Harbarth, S., Mendelson, M., Monnet, D. L., et al. (2018). Discovery, research, and development of new antibiotics: the WHO priority list of antibiotic-resistant bacteria and tuberculosis. Lancet Infect. Dis. 18, 318–327. doi: 10.1016/S1473-3099(17)30753-3
Tamma, P. D., Aitken, S. L., Bonomo, R. A., Mathers, A. J., van Duin, D., and Clancy, C. J. (2022). Infectious Diseases Society of America guidance on the treatment of amp C β-lactamase-producing Enterobacterales, Carbapenem-resistant Acinetobacter baumannii, and Stenotrophomonas maltophilia infections. Clin. Infect. Dis. 74, 2089–2114. doi: 10.1093/cid/ciab1013
Tanimoto, Y., Tamai, S., Matsuzaki, T., Takeuchi, N., Noju, T., Yanagida, S., et al. (2019). Diffusely adherent strains isolated from healthy carriers suppress cytokine secretions of epithelial cells stimulated by inflammatory substances. Infect. Immun. 87, e00683–e00618. doi: 10.1128/IAI.00683-18
Thatrimontrichai, A., Pannaraj, P. S., Janjindamai, W., Dissaneevate, S., Maneenil, G., and Apisarnthanarak, A. (2020). Intervention to reduce carbapenem-resistant in a neonatal intensive care unit. Infect. Control Hosp. Epidemiol. 41, 710–715. doi: 10.1017/ice.2020.35
Tiseo, G., Brigante, G., Giacobbe, D. R., Maraolo, A. E., Gona, F., Falcone, M., et al. (2022). Diagnosis and management of infections caused by multidrug-resistant bacteria: guideline endorsed by the Italian Society of Infection and Tropical Diseases (SIMIT), the Italian Society of Anti-Infective Therapy (SITA), the Italian Group for Antimicrobial Stewardship (GISA), the Italian Association of Clinical Microbiologists (AMCLI) and the Italian Society of Microbiology (SIM). Int. J. Antimicrob. Agents 60:106611. doi: 10.1016/j.ijantimicag.2022.106611
Tomczyk, S., Zanichelli, V., Grayson, M. L., Twyman, A., Abbas, M., Pires, D., et al. (2019). Control of Carbapenem-resistant Enterobacteriaceae, Acinetobacter baumannii, and Pseudomonas aeruginosa in healthcare facilities: a systematic review and reanalysis of quasi-experimental studies. Clin. Infect. Dis. 68, 873–884. doi: 10.1093/cid/ciy752
Uwingabiye, J., Lemnouer, A., Roca, I., Alouane, T., Frikh, M., Belefquih, B., et al. (2017). Clonal diversity and detection of carbapenem resistance encoding genes among multidrug-resistant isolates recovered from patients and environment in two intensive care units in a Moroccan hospital. Antimicrob. Resist. Infect. Control 6:99. doi: 10.1186/s13756-017-0262-4
Venditti, C., Vulcano, A., D'Arezzo, S., Gruber, C. E. M., Selleri, M., Antonini, M., et al. (2019). Epidemiological investigation of an Acinetobacter baumannii outbreak using core genome multilocus sequence typing. J Glob Antimicrob Resist 17, 245–249. doi: 10.1016/j.jgar.2018.11.027
Wang, X., Du, Z., Huang, W., Zhang, X., and Zhou, Y. (2021). Outbreak of multidrug-resistant Acinetobacter baumannii ST208 producing OXA-23-like Carbapenemase in a Children's Hospital in Shanghai. China. Microb Drug Resist 27, 816–822. doi: 10.1089/mdr.2019.0232
Weiser, J. N., Ferreira, D. M., and Paton, J. C. (2018). Streptococcus pneumoniae: transmission, colonization and invasion. Nat. Rev. Microbiol. 16, 355–367. doi: 10.1038/s41579-018-0001-8
Weiss, N., Faugeras, F., Rohaut, B., Leconte, J., Lafeuille, E., Brossier, F., et al. (2016). Multidrug-resistant bacteria transmitted through high-density EEG in ICU. Seizure 37, 65–68. doi: 10.1016/j.seizure.2016.03.005
World Health Organization (2011). Report on the burden of endemic health care-associated infection worldwide. World Health Organization. Available at: https://apps.who.int/iris/handle/10665/80135
World Health Organization (2017). Guidelines for the prevention and control of carbapenem-resistant Enterobacteriaceae, Acinetobacter baumannii and Pseudomonas aeruginosa in health care facilities. World health Organization. Available at: https://apps.who.int/iris/handle/10665/259462
World Health Organization (2022). Global report on infection prevention and control. World Health Organization. Available at: https://apps.who.int/iris/handle/10665/354489
Wu, N., Dai, J., Guo, M., Li, J., Zhou, X., Li, F., et al. (2021). Pre-optimized phage therapy on secondary Acinetobacter baumannii infection in four critical COVID-19 patients. Emerg Microbes Infect 10, 612–618. doi: 10.1080/22221751.2021.1902754
Yakupogullari, Y., Otlu, B., Ersoy, Y., Kuzucu, C., Bayindir, Y., Kayabas, U., et al. (2016). Is airborne transmission of Acinetobacter baumannii possible: a prospective molecular epidemiologic study in a tertiary care hospital. Am. J. Infect. Control 44, 1595–1599. doi: 10.1016/j.ajic.2016.05.022
Yamamoto, N., Hamaguchi, S., Akeda, Y., Santanirand, P., Chaihongsa, N., Sirichot, S., et al. (2019). Rapid screening and early precautions for carbapenem-resistant Acinetobacter baumannii carriers decreased nosocomial transmission in hospital settings: a quasi-experimental study. Antimicrob. Resist. Infect. Control 8:110. doi: 10.1186/s13756-019-0564-9
Zhang, Y., Ding, F., Luo, Y., Fan, B., Tao, Z., Li, Y., et al. (2021). Distribution pattern of carbapenemases and solitary contribution to resistance in clinical strains of Acinetobacter baumannii. Ann Palliat Med 10, 9184–9191. doi: 10.21037/apm-21-1805
Zhang, X., Li, F., Awan, F., Jiang, H., Zeng, Z., and Lv, W. (2021). Molecular epidemiology and clone transmission of Carbapenem-resistant Acinetobacter baumannii in ICU rooms. Front. Cell. Infect. Microbiol. 11:633817. doi: 10.3389/fcimb.2021.633817
Zhang, N., Zhu, L., Ouyang, Q., Yue, S., Huang, Y., Qu, S., et al. (2021). Visualizing the potential impairment of Polymyxin B to central nervous system through MR susceptibility-weighted imaging. Front. Pharmacol. 12:784864. doi: 10.3389/fphar.2021.784864
Zhao, Y., Hu, K., Zhang, J., Guo, Y., Fan, X., Wang, Y., et al. (2019). Outbreak of carbapenem-resistant Acinetobacter baumannii carrying the carbapenemase OXA-23 in ICU of the eastern Heilongjiang Province. China. BMC Infect Dis 19:452. doi: 10.1186/s12879-019-4073-5
Zhen, X., Stålsby Lundborg, C., Sun, X., Gu, S., and Dong, H. (2020). Clinical and economic burden of Carbapenem-resistant infection or colonization caused by Klebsiella pneumoniae, Pseudomonas aeruginosa, Acinetobacter baumannii: a multicenter study in China. Antibiotics (Basel) 9:514. doi: 10.3390/antibiotics9080514
Zhou, Y., Wu, X., Zhang, X., Hu, Y., Yang, X., Yang, Z., et al. (2015). Genetic characterization of ST195 and ST365 Carbapenem-resistant Acinetobacter baumannii harboring blaOXA-23 in Guangzhou. China. Microbial Drug Resistance 21, 386–390. doi: 10.1089/mdr.2014.0183
Keywords: carbapenem-resistant Acinetobacter baumannii, intensive care unit, infection, environmental contamination, resistance mechanism, homology, treatment and control strategies
Citation: Jiang Y, Ding Y, Wei Y, Jian C, Liu J and Zeng Z (2022) Carbapenem-resistant Acinetobacter baumannii: A challenge in the intensive care unit. Front. Microbiol. 13:1045206. doi: 10.3389/fmicb.2022.1045206
Edited by:
Lucinda Janete Bessa, Egas Moniz - Cooperativa de Ensino Superior, CRL, PortugalReviewed by:
Adam Valcek, Vrije University Brussel, BelgiumNicolas Kieffer, Universidad Complutense de Madrid, Spain
Reem Mostafa Hassan, Cairo University, Egypt
Copyright © 2022 Jiang, Ding, Wei, Jian, Liu and Zeng. This is an open-access article distributed under the terms of the Creative Commons Attribution License (CC BY). The use, distribution or reproduction in other forums is permitted, provided the original author(s) and the copyright owner(s) are credited and that the original publication in this journal is cited, in accordance with accepted academic practice. No use, distribution or reproduction is permitted which does not comply with these terms.
*Correspondence: Zhangrui Zeng, zengzhangrui@swmu.edu.cn; Jinbo Liu, liujb7203@swmu.edu.cn
†These authors have contributed equally to this work and share last authorship