- 1Department of Environment and Health, School of Public Health, Cheeloo College of Medicine, Shandong University, Jinan, Shandong, China
- 2Department of Thoracic Surgery, Qilu Hospital of Shandong University, Jinan, Shandong, China
- 3Department of Clinical and Experimental Medicine, Linköping University, Linköping, Sweden
- 4Department of Blood Transfusion, Qilu Hospital of Shandong University, Jinan, Shandong, China
Animal farms have become one of the most important reservoirs of carbapenem-resistant Klebsiella spp. (CRK) owing to the wide usage of veterinary antibiotics. “One Health”-studies observing animals, the environment, and humans are necessary to understand the dissemination of CRK in animal breeding areas. Based on the concept of “One-Health,” 263 samples of animal feces, wastewater, well water, and human feces from 60 livestock and poultry farms in Shandong province, China were screened for CRK. Five carbapenem-resistant Klebsiella pneumoniae (CRKP) and three carbapenem-resistant Klebsiella quasipneumoniae (CRKQ) strains were isolated from animal feces, human feces, and well water. The eight strains were characterized by antimicrobial susceptibility testing, plasmid conjugation assays, whole-genome sequencing, and bioinformatics analysis. All strains carried the carbapenemase-encoding gene blaNDM-5, which was flanked by the same core genetic structure (IS5-blaNDM-5-bleMBL-trpF-dsbD-IS26-ISKox3) and was located on highly related conjugative IncX3 plasmids. The colistin resistance gene mcr-8.1 was carried by three CRKP and located on self-transmissible IncFII(K)/IncFIA(HI1) and IncFII(pKP91)/IncFIA(HI1) plasmids. The genetic context of mcr-8.1 consisted of IS903-orf-mcr-8.1-copR-baeS-dgkA-orf-IS903 in three strains. Single nucleotide polymorphism (SNP) analysis confirmed the clonal spread of CRKP carrying-blaNDM-5 and mcr-8.1 between two human workers in the same chicken farm. Additionally, the SNP analysis showed clonal expansion of CRKP and CRKQ strains from well water in different farms, and the clonal CRKP was clonally related to isolates from animal farms and a wastewater treatment plant collected in other studies in the same province. These findings suggest that CRKP and CRKQ are capable of disseminating via horizontal gene transfer and clonal expansion and may pose a significant threat to public health unless preventative measures are taken.
Introduction
The prevalence of antibiotic-resistant bacteria (ARB) and antibiotic-resistant genes (ARGs) has the potential to cause a global health crisis in the 21st century. Carbapenem-resistant Klebsiella spp. (CRK) are especially concerning owing to their resistance to carbapenems, one of the last resort antibiotics used against gram-negative bacteria, and ability to cause a variety of community- or hospital-acquired infections such as pneumonia, urinary tract infections, bloodstream infections, and septic shock. Carbapenem-resistant Klebsiella pneumoniae (CRKP), which is widely reported in Europe, Asia, Africa, and other regions (Navon-Venezia et al., 2017), is listed in the critical priority tier of pathogens and was recognized as the highest priority in new antibiotic development by the World Health Organization (WHO) in 2017 (Tacconelli et al., 2018). However, according to the China Antimicrobial Surveillance Network (CHINET),1 the prevalence of clinical meropenem resistance in K. pneumoniae has increased rapidly in China, from 2.9% in 2005 to 24.4% in 2021, posing a massive challenge to public health.
China is the largest consumer of veterinary antimicrobials, accounting for 45% of global use in 2017, and is expected to remain the largest consumer in 2030 (Tiseo et al., 2020). Thus, ARB and ARGs have been widely spread in animal farms, such as in animal feces (Tang et al., 2019), groundwater (Gu et al., 2022), aerosol (Yang et al., 2021), and animal foods (Wang et al., 2022). Although carbapenems are not approved for use on animal farms in China, the widespread use of other antibiotics such as fluoroquinolones, sulfonamides, and tetracyclines can co-select carbapenem-resistant bacteria (BIOHAZ, 2013). In China, CRK has been detected in animal farms, such as in animal feces and raw milk in dairy cattle farms in Jiangsu province (He et al., 2017), chicken cloaca and environment (sewage trenches, corridor floors, drooping boards, feeding troughs, and nipple drinkers) in broiler farms in Hebei province (Zhai et al., 2020), duck feces in Guangdong province (Ma et al., 2020), and pig anal swabs from a pig farm in Hunan province (Zhao et al., 2021b). However, most CRK studies on animal farms in China have two major shortcomings. First, the studies mainly focused on a single type of animal farms (He et al., 2017; Ma et al., 2020; Zhao et al., 2021b). Objectively understanding the true prevalence of CRK on different types of animal farms is difficult. Second, the studies are mostly limited to animals and the environment (Zhai et al., 2020; Gu et al., 2022), and the CRK transmission among animals, the environment, and humans is not entirely clear.
Thus, our study randomly selected 60 animal farms, including 20 chicken, 20 pig, and 20 bovine farms, in a typical region of the animal farming industry with a long breeding time and stable animal breeding patterns in Shandong province in July 2019. Based on the perspective of “One-Health,” animal feces, wastewater (excluding chicken farms), well water, and human feces were collected from each farm to screen for CRK. This study aimed to characterize CRK in terms of antimicrobial susceptibility, antibiotic resistance genes, virulence genes and multilocus sequence typing (MLST), investigate the prevalence of CRK in different types of farms in the same animal breeding area, and explore the transmission of CRK among animals, the environment, and humans.
Materials and methods
Sampling site and collection samples
The study site is in Eastern China, has a population of approximately 1,194,365, covers an area of 2,414 km2, and has a long breeding time and stable animal breeding patterns. The primary animal husbandry in this area consists of breeding chickens, pigs, and bovines. We selected 60 livestock and poultry farms (Figure 1), including pig farms (n = 20), chicken farms [(n = 20); laying hen farms (n = 11) and broiler farms (n = 9)], and bovine farms [(n = 20); dairy cattle farms (n = 13) and beef farms (n = 7)]. In July 2019, a total of 263 samples were collected, with 75 from chicken farms, 86 from pig farms, and 102 from bovine farms. The following samples types were collected: 66 animal feces, 37 wastewater, 107 worker feces, and 53 well water samples. The number and type of samples are summarized in Supplementary Table S1. Worker feces was collected using a Copan Liquid Amies Elution Swab (ESwab; Copan, Brescia, Italy). Well water (500 ml) from each farm well and wastewater (500 ml) from each farm sewage outlet (excluding chicken farms) were collected in sterile glass bottles. Approximately 30 g of each fresh animal fecal sample was collected in a sterile plastic bottle with a sterile spoon. All samples were temporarily placed in iceboxes (4–8°C) upon collection and transported to the laboratory at the end of the sampling day. The well water and wastewater samples were filtered through 0.45 μm sterile membrane filters (Millipore, Billerica, United States). After filtration, the membranes were placed in 5 ml of sterile brain heart infusion broth (BHI; Oxoid, Basingstoke, United Kingdom) with 20% glycerin. 2.0 g of each animal fecal sample was homogenized in 5 ml of sterile BHI broth (Oxoid, Basingstoke, United Kingdom) with 20% glycerin. All samples were stored at −80°C until cultivation.
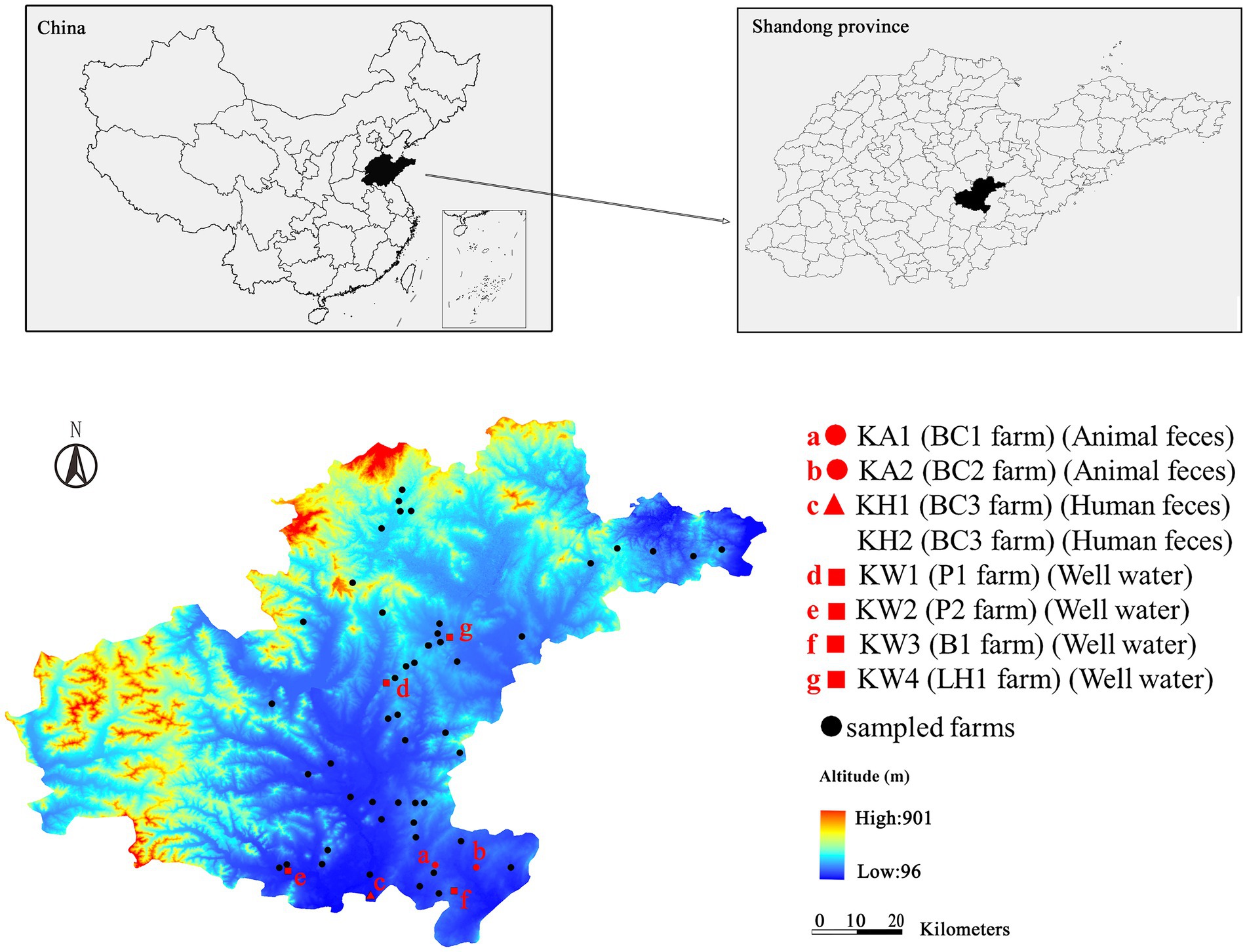
Figure 1. Map showing the location of the CRK-negative farms (denoted by black dots) and CRK-positive farms (denoted by red dots). The farms denoted “a” and “b” represent the farms in which CRK was isolated from animal feces. The red triangles (c) represent the farm in which CRK was isolated from human feces. Red squares (d, e, f, and g) represent the farms in which CRK was isolated from well water. BC1, BC2 and BC3 are broiler chicken farms; P1 and P2 are pig farms; B1 is a beef farm; LH1 is a laying hen farm.
Isolation and identification for carbapenem-resistant Klebsiella spp.
For all samples, a pre-enrichment step in the BHI broth (Oxoid, Basingstoke, United Kingdom) was performed in a constant temperature shaker (Boxun, Shanghai, China) at 37°C overnight. Enriched solutions (50 μl) were evenly spread on MacConkey agar (Landbridge, Beijing, China) supplemented with 2 mg/l meropenem (Meilun, Dalian, China) to screen for carbapenem-resistant isolates. Colonies were selected based on colony morphotypes and repeatedly streaked on the MacConkey agar to obtain pure isolates (Zhao et al., 2021a). The isolates were tested for the presence of blaNDM, blaKPC, blaOXA-48, blaVIM and blaIMP carbapenemase genes using PCR, as previously described (Poirel et al., 2011). The PCR products were verified using Sanger sequencing (Biosune, Shanghai, China). Matrix-assisted laser desorption/ionization time-of-flight mass spectrometry (MALDI-TOF/MS; Bruker Daltonik GmbH, Bremen, Germany) was used to identify the carbapenem-resistant isolates. The CRK isolates were selected for further research.
Antimicrobial susceptibility testing
The minimum inhibitory concentrations (MICs) of the 12 antibiotics were determined for CRK. 12 antibiotics were tested at a series of concentrations, and the maximum concentrations of amoxicillin/clavulanate, piperacillin/tazobactam, cefotaxime, ceftazidime, meropenem, imipenem, amikacin, ciprofloxacin, tetracycline, tigecycline, colistin and nitrofurantoin were 128/64 mg/l, 128/4 mg/l, 256 mg/l, 256 mg/l, 256 mg/l, 256 mg/l, 256 mg/l, 64 mg/l, 128 mg/l, 32 mg/l, 64 mg/l, 128 mg/l, respectively; the minimum concentrations were 0.0625 mg/l for 12 antibiotics. The MICs of tigecycline and colistin were determined using the broth microdilution method, and others were determined using the agar dilution method according to the Clinical Laboratory Standards Institute (CLSI). The results were interpreted according to the CLSI (document M100-S31), excluding tigecycline, for which MICs were interpreted following the ecological cut-off (ECOFF) of the European Committee on Antimicrobial Susceptibility Testing (EUCAST) SOP 10.2. Escherichia coli ATCC 25922 was used as the quality control strain. Multidrug resistance was defined as non-susceptibility to at least one agent in three or more antimicrobial categories (Magiorakos et al., 2012).
The transmissibility of plasmids
Sodium azide-resistant E. coli J53 was used as the recipient strain, and CRK was used as the donor strain to evaluate the horizontal transferability of the plasmids mediating carbapenem resistance, colistin resistance, and tigecycline resistance by filter mating. The donor strain and recipient strain were mixed at a ratio of 2:1 and incubated at 37°C for 12 h. Transconjugants carrying carbapenemase gene, colistin resistance gene, and tigecycline resistance gene were selected by cultivation on LB agar supplemented with 100 mg/l sodium azide and 2 mg/l meropenem, 100 mg/l sodium azide and 2 mg/l colistin, 100 mg/l sodium azide and 4 mg/l tigecycline for 18 h at 37°C, respectively. Transconjugants carrying resistance genes were verified using PCR. Moreover, plasmids of tigecycline-resistant strain (KA2) were extracted using the M5 BAC/PAC large plasmid extraction kit (Mei5, Beijing, China) according to the manufacturer’s instructions. XL10-Gold chemically competent E. coli cells (Angyu, Shanghai, China) were used as the recipient strain to evaluate the transformation of plasmids that mediate tigecycline resistance using the heat shock method, as previously described (Hassan et al., 2022). Transformants were selected by cultivation on LB agar supplemented with 4 mg/l tigecycline for 18 h at 37°C.
Whole-genome sequencing
All CRK were subjected to whole-genome sequencing on the Illumina NovaSeq 6,000-PE150 platform (Illumina, San Diego, United States) combined with the PacBio Sequel platform (Berry Genomics Co. Ltd.). Hybrid genome assembly with both short and long reads was performed using Unicycler v0.5.0 (Wick et al., 2017). The sequences were annotated using Prokka v1.12 (Seemann, 2014) and RAST v2.0 (Aziz et al., 2008). Antibiotic resistance genes, plasmid replicons, and MLST were identified at the Center for Genomic Epidemiology2 using ResFinder 4.1, PlasmidFinder 2.1, and MLST 2.0, respectively. Virulence genes were analyzed using the VFDB analyzer3 and capsule polysaccharide-based serotyping (K-type) was performed using the BIGSdb Klebsiella Pasteur MLST database.4 The Basic Local Alignment Search Tool (BLAST) at the National Center for Biotechnology Information (NCBI)5 was used to analyze the plasmid alignments. Alignment analysis of amino acid sequences of DNA gyrase (gyrA and gyrB) and topoisomerase IV (parC and parE) was performed using Clustal Omega (Sievers et al., 2011). Genetic relatedness of the isolates was determined by single nucleotide polymorphism (SNP) analysis using CSI Phylogeny 1.4 at the Center for Genomic Epidemiology (Kaas et al., 2014). A distance of ≤15 SNPs was defined as clonally related strains (Schürch et al., 2018). Visualization of the genome comparison of the plasmids harboring blaNDM-5 and mcr-8.1 was performed using BLAST Ring Image Generator (BRIG; Alikhan et al., 2011). All genome sequences in this study have been deposited in NCBI Genome database under BioProject PRJNA834640. The blaNDM-5-carrying plasmids of strains KA1, KA2, KH1, KH2, KW1, KW2, KW3, and KW4 have been deposited into GenBank under accession nos. CP102896.1, CP102875.1, CP102881.1, CP102887.1, CP102900.1, CP102890.1, CP102892.1, and CP102905.1, respectively. The mcr-8.1-carrying plasmids of strains KA2, KH1 and KH2 have been deposited into GenBank under accession nos. CP102872.1, CP102880.1, and CP102886.1, respectively.
Results
Prevalence of carbapenem-resistant Klebsiella spp.
Eight CRK were isolated from 263 samples, five of which were K. pneumoniae and three were K. quasipneumoniae. Five CRK (KA1, KA2, KH1, KH2, and KW4) were isolated from chicken farms (6.7%, 5/75), two (KW1 and KW2) from pig farms (2.3%, 2/86) and one (KW3) from bovine farms (0.98%, 1/102). KH1 and KH2 were isolated from the same chicken farm, whereas the other strains were obtained from different farms. In terms of sample types, four CRK (KW1-KW4) were isolated from well water, two (KA1 and KA2) from animal feces, two (KH1 and KH2) from human feces, and no CRK was isolated from wastewater (Supplementary Table S1).
Phenotypic and genotypic antibiotic resistance
Antibiotic susceptibility testing showed that all CRK were multidrug-resistant and resistant to amoxicillin-clavulanic acid, piperacillin/tazobactam, cefotaxime, ceftazidime, meropenem, imipenem, and tetracycline. Most isolates were resistant to ciprofloxacin (62.5%), whereas resistance to colistin (50%), amikacin (37.5%), nitrofurantoin (37.5%), and tigecycline (12.5%) was observed to a lesser degree (Table 1). Eight CRK harbored the carbapenemase gene blaNDM-5, accounting for high-level resistance to meropenem (≥128 mg/l) and imipenem (≥64 mg/l). In addition to the carbapenemase gene, CKR carried various classes of resistance genes and the most prevalent ARGs (prevalence ≥50%), including oqxAB (n = 8), fosA (n = 8), tet (A) (n = 6), aadA2 (n = 5), floR (n = 5), qnrS1 (n = 4), and aac(6′)-Ib-cr (n = 4; Supplementary Table S2). Three strains (KA2, KH1, and KH2) carried the colistin resistance mcr-8.1 and armA genes which encode a 16S rRNA-methylase, accounting for high-grade aminoglycoside resistance. KH1 and KH2 were resistant to all tested antibiotics except tigecycline, and KA2 was resistant to all tested antibiotics, including tigecycline. However, no known tigecycline resistance genes were not found on KA2 strain. Plasmid conjugation assay and transformation assay found that the plasmids pKA2-2, pKA2-3-mcr8.1, pKA2-5, and pKA2-6-NDM5 could be successfully transferred from KA2 to the recipient strain E. coli J53, while the transconjugants and transformants were remain sensitive to tigecycline, indicating a novel or more complex resistant mechanism in this strain.
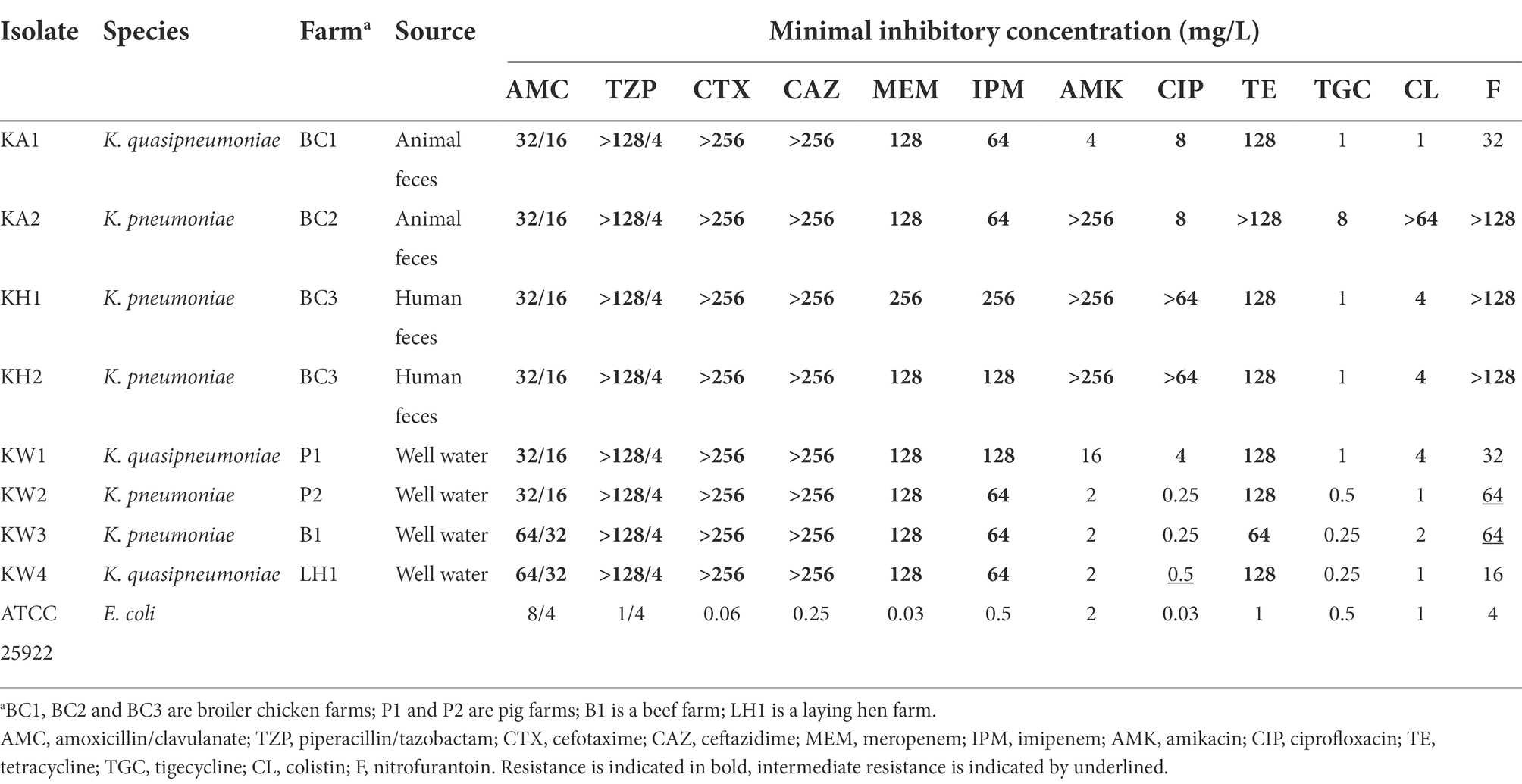
Table 1. Antimicrobial susceptibility profiles of carbapenem-resistant Klebsiella spp. strains from animal farms in Shandong, China.
As the strains showed a high resistance rate (62.5%, 5/8) to ciprofloxacin, the resistance mechanisms of ciprofloxacin were analyzed. Comparison of the amino acid sequence of DNA gyrase (gyrA and gyrB) and topoisomerase IV (parC and parE) between the CRK isolated in this study and the reference sequence in the NCBI database showed that the amino acid substitutions of quinolone resistance-determining regions (QRDRs) were observed at position 83 (Ser → IIe) of gyrA and position 80 (Ser → IIe) of parC in KA2, KH1, and KH2 (Supplementary Table S3). Three strains were resistant to ciprofloxacin, two of which had high-level ciprofloxacin resistance (MICs >64 mg/l). Several studies have confirmed that amino acid co-substitution in the QRDRs of gyrA and parC in Klebsiella spp. were associated with high-level resistance to ciprofloxacin (Minarini and Darini, 2012; Kareem et al., 2021; Zhan et al., 2021), which is consistent with our results. Additionally, all CRK, including ciprofloxacin-resistant strains, carried three or more fluoroquinolone resistance genes, including oqxAB (100%, 8/8), qnrS (50%, 4/8), qnrB (50%, 4/8), and aac(6′)-Ib-cr (50%, 4/8; Supplementary Table S3). Even in the absence of QRDRs mutations, one strain carrying the fluoroquinolone resistance genes qnrB2, aac(6′)-Ib-cr, and oqxAB, and another strain carrying qnrS1 and oqxAB showed non-susceptibility to ciprofloxacin. The qnr genes encode proteins belonging to the pentapeptide repeat protein family that play a role in binding and protecting topoisomerase IV and DNA gyrase from repression by ciprofloxacin (Kim et al., 2009). The aac(6′)-Ib-cr gene encodes a variant aminoglycoside acetyltransferase that reduces the activity of ciprofloxacin by N-acetylation at the amino nitrogen on its piperazinyl substituent (Robicsek et al., 2006). Mirzaii et al. (2018), Zhan et al. (2021), and Reo et al. (2022) reported that Klebsiella spp. strains carrying fluoroquinolone resistance genes, but without mutations in the QRDRs, were resistant to ciprofloxacin. These findings indicate that fluoroquinolone resistance genes can mediate ciprofloxacin resistance in Klebsiella spp.
Virulence genes of carbapenem-resistant Klebsiella spp.
In this study, all strains possessed the type 1 fimbrial gene cluster fimABCDEFGHI, type 3 fimbrial gene cluster mrkABCDFHIJ, enterobactin-encoding genes entABCDEFS and its transport protein-encoding genes fepABCDG, and salmochelin-encoding gene iroE, but carried no genes related to hypervirulent Klebsiella, such as iroB, iucA, rmpA and rmpA2 (Thomas et al., 2018; Supplementary Table S2).
Genotypic characterization of carbapenem-resistant Klebsiella spp.
The silico analysis of the general molecular characteristics of the isolates are summarized in Supplementary Table S2. The eight strains belonged to four different sequence types (ST): ST526 (KA1, KW1, and KW4), ST37 (KH1 and KH2), ST766 (KW2 and KW3), and ST495 (KA2). Three types of capsular serotypes KL22KL37-wzi385, KL28-wzi84, and KL15KL17KL51KL52-wzi50 were identified in KH1 and KH2, KW1 and KW4, and KW2 and KW3, respectively, and KA1 and KA2 had poor match confidence against any known serotype and were determined to be non-typeable (Supplementary Table S2). The genome of each strain possessed a circular chromosome with sizes ranging from 5,225,745 bp to 5,320,447 bp. Furthermore, the genomes of KA1, KA2, KH1, KH2, KW1, KW2, KW3, and KW4 contained four, seven, five, five, four, one, one, and five circular plasmids, respectively. As shown in Supplementary Table S2, various classes of resistance genes were detected on the chromosomes and plasmids of the strains. Incompatible IncX3 type plasmids (45,122–52,085 bp) carrying blaNDM-5 were found on all strains. A hybrid incompatible IncFII(pKP91)/IncFIA(HI1) plasmid carrying mcr-8.1 was detected on KA2, and IncFII(K)/IncFIA(HI1) plasmids carrying mcr-8.1 were detected on KH1 and KH2.
Characterization and comparative genomics of plasmids carrying blaNDM-5 and mcr-8.1
blaNDM-5-carrying IncX3 plasmids were successfully transferred from all strains to the recipient strain E. coli J53 via conjugation assays. blaNDM-5 was located on the 46,161 bp IncX3 plasmid among strains KA1, KW2, and KW3, designated pKA1-3-NDM5, pKW2-1-NDM5, and pKW3-1-NDM5, respectively. Plasmid sequence comparison showed that the three IncX3 plasmids shared 100% nucleotide identity and 100% coverage with each other, and were identical to several other IncX3 plasmids found in Enterobacteriaceae isolates in China, such as the plasmid pNDM5_IncX3 (GenBank: KU761328.1) from K. pneumoniae isolated from a patient in Suzhou city, pNDM-SCCRK18-72 (GenBank: MN565271.1) from an E. coli isolated from chicken in Sichuan province, and pEC24-NDM-5 (GenBank: CP060888.1) from an E. coli isolated from a patient in Zhejiang province. blaNDM-5 was flanked by the same genetic structure as that of Tn3-IS3000-ISAba125-IS5-blaNDM-5-bleMBL-trpF-dsbD-IS26-ISKox3 (Figure 2A). In strains KA2, KW1, and KW4, blaNDM-5 was located on 45,244, 45,122 and 45,122 bp IncX3 plasmids, designated pKA2-6-NDM5, pKW1-2-NDM5, and pKW4-2-NDM5, respectively. The three IncX3 plasmids showed 97% coverage and 99.99% nucleotide identity with pKA1-3-NDM5. Tn3, IS3000, and IS5 were located upstream of blaNDM-5, whereas bleMBL, trpF, dsbD, IS26, and ISKox3 were located downstream (Figure 2A). KH1 and KH2 contained a 52,085 bp IncX3 plasmid carrying blaNDM-5, which shared 99.99% nucleotide identity and 97% coverage with pKA1-3-NDM5. The environment of blaNDM-5 was same with strains KA2, KW1, and KW4 (Figure 2B). Although the size and structure of IncX3 plasmids carrying blaNDM-5 differed slightly among strains, all IncX3 plasmids contained a highly conserved region around blaNDM-5, consisting of IS5-blaNDM-5-bleMBL-trpF-dsbD-IS26-ISKox3.
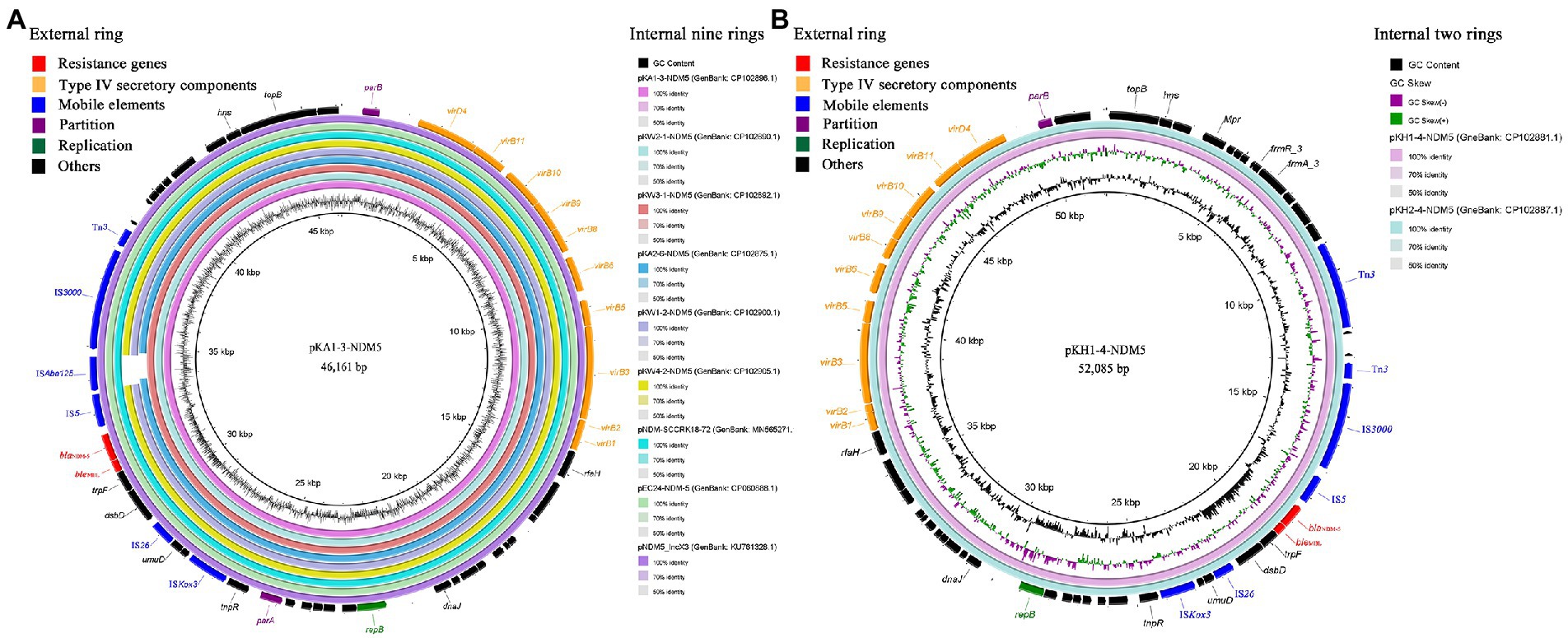
Figure 2. The blast and annotation of blaNDM-5-carrying IncX3 plasmid genomes (constructed by BRIG). Genes are color-coded depending on functional annotations. (A) The nine internal rings represent different blaNDM-5-carrying IncX3 plasmids from this study and GenBank database. The external ring represents the annotation of plasmid pKA1-3-NDM5. (B) The two internal rings represent plasmid pKH1-4-NDM5 and pKH2-4-NDM5. The external ring represents the annotation of plasmid pKH1-4-NDM5.
The mcr-8.1 harboring plasmids were successfully transferred into the recipient strain E. coli J53 from three CRKP via conjugation assays. In strain KA2, mcr-8.1 was located on a 118,931 bp plasmid, designated pKA2-3-mcr8.1, which contained IncFII(pKP91) and IncFIA(HI1) replicons. In addition to mcr-8.1, resistance genes blaTEM-1B, blaCTX-M-1, and bleO were identified on the plasmid. The plasmid BLAST query in the GenBank database showed that the plasmid pKA2-3-mcr8.1 exhibited the highest degree of sequence homology with plasmids pKP19-3,138-3 (GenBank: CP090619.1) from K. pneumoniae and pKP46-mcr8 (GenBank: CP088125.1) from K. pneumoniae, with over 99% identity and 81% coverage (Figure 3A). In strains KH1 and KH2, mcr-8.1 was located on 100,448 bp plasmids, designated pKH1-3-mcr8.1 and pKH2-3-mcr8.1, both of which harbor IncFII(K) and IncFIA(HI1) replicons. The two plasmids shared over 99% nucleotide identity and 94% coverage with the plasmids pKP3 (GenBank: OL804387.1) and pKP91 (GenBank: MG736312.1), which were isolated from chicken anal swab and pig feces, respectively, in Shandong province, China (Figure 3B), indicating that this type of plasmid may have been widely spread in this region. The genetic context of mcr-8.1 in the isolates was similar and was composed of IS903-orf-mcr-8.1-copR-baeS-dgkA-orf-IS903. mcr-8.1 was flanked by the complete insertion sequence IS903, which may facilitate the transmission of mcr-8.1 among animals, the environment, and humans in this animal breeding area. Additionally, the mcr-8.1-carrying IncFII(pKP91)/IncFIA(HI1) and IncFII(K)/IncFIA(HI1) plasmids carried two genes coding the response regulator transcription factor CopR and HAMP domain-containing histidine kinase Baes. The combination of these two protein families usually constitutes a two-component system involved in colistin resistance in Enterobacteriaceae.
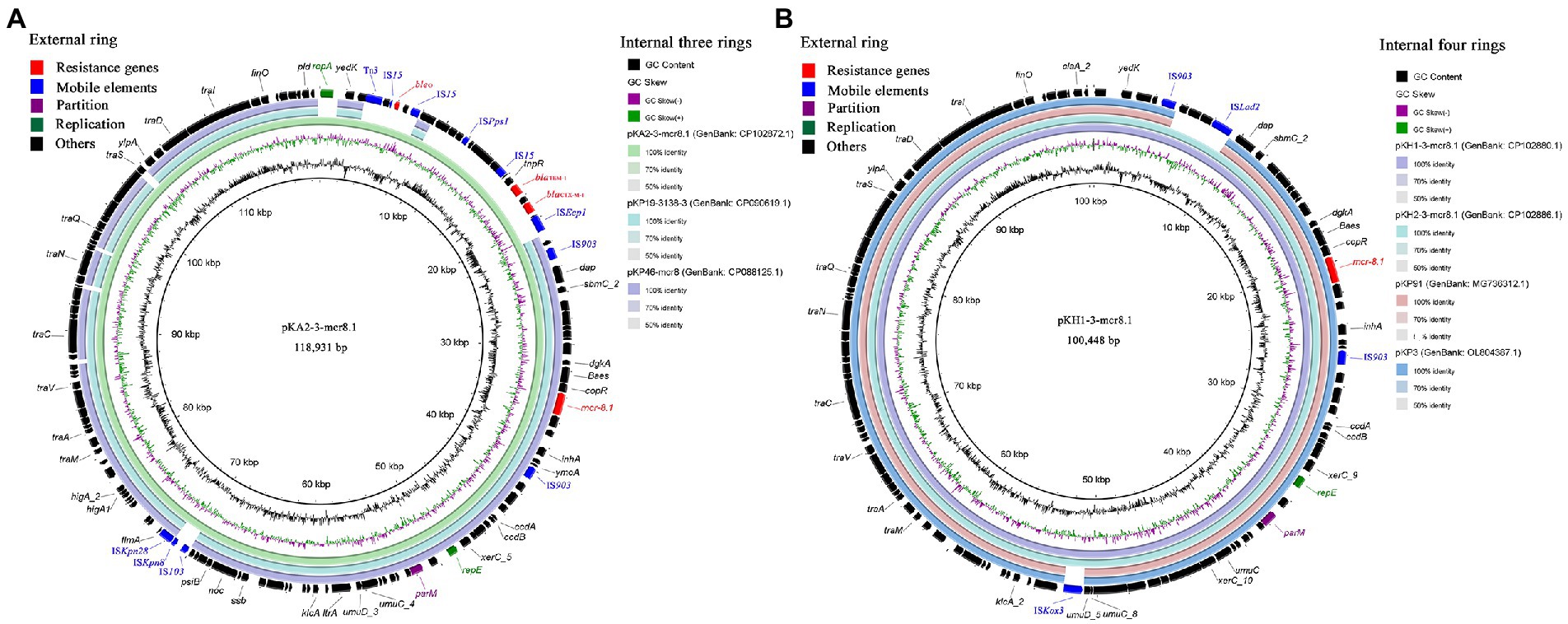
Figure 3. The blast and annotation of mcr-8.1-carrying plasmid genomes (constructed by BRIG). Genes are color-coded depending on functional annotations. (A) The three internal rings represent plasmids pKA2-3-mcr8.1, pKP19-3,138-3 (GenBank: CP090619.1) and pKP46-mcr8 (GenBank: CP088125.1). The external ring represents the annotation of plasmid pKA2-3-mcr8.1. (B) The four internal rings represent different mcr-8.1-carrying plasmids from this study and GenBank database. The external ring represents the annotation of plasmid pKH1-3-mcr8.1.
Genetic relationship analysis
SNP-based phylogenetic analysis revealed that the eight CRK were grouped into four clusters. The first cluster included KW2 and KW3, isolated from the well water of farms P2 and B1, respectively, with four SNPs differences. The second cluster consisted of KA1, KW1, and KW4, among which KW1 and KW4 differed by only six SNPs and were isolated from the well water of farms P1 and LB1. The third cluster included only KA2. The fourth cluster included KH1 and KH2 isolated from two human fecal samples in farm BC3, with two SNPs differences (Figure 4; Supplementary Table S4).
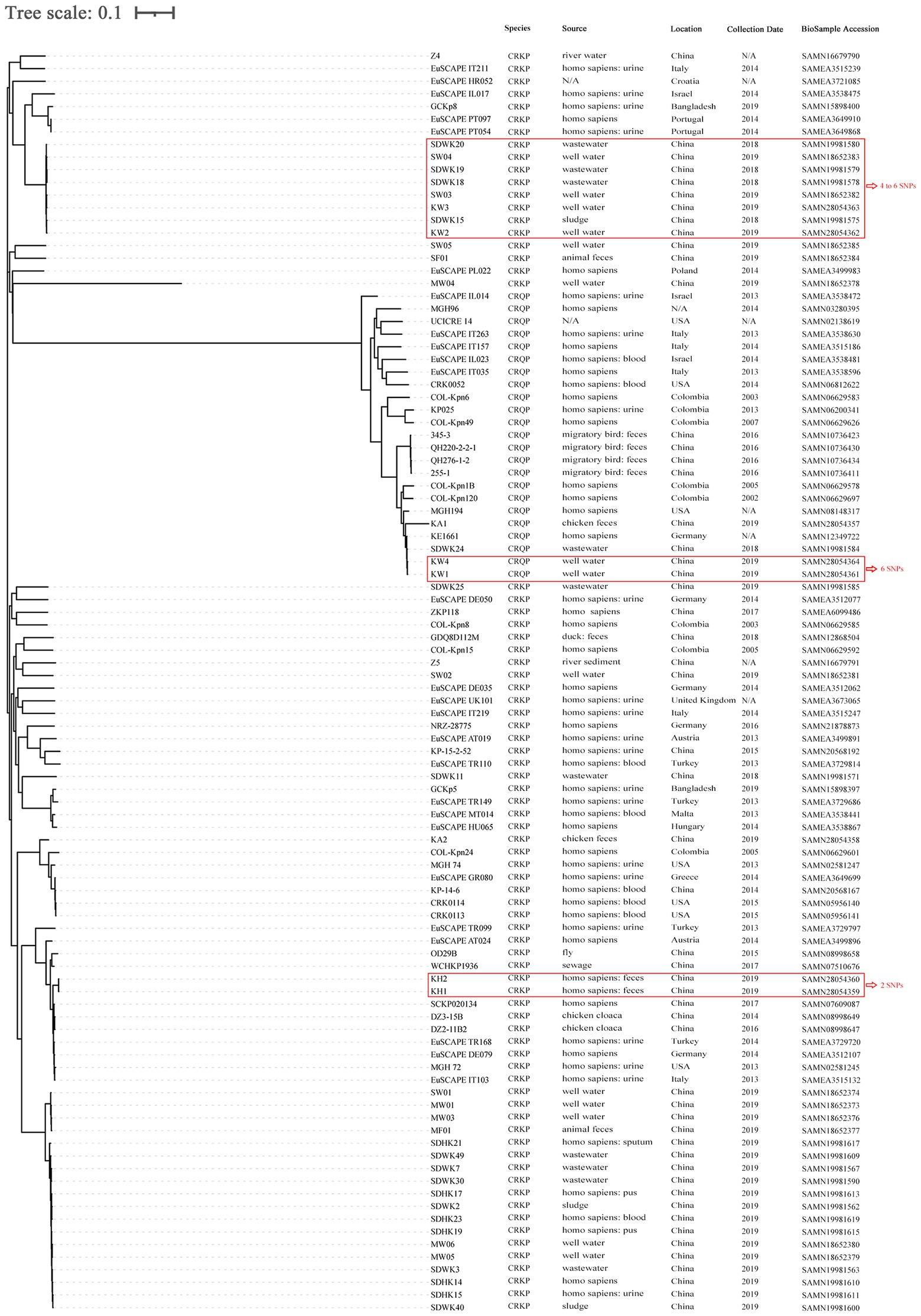
Figure 4. SNP-based phylogenetic tree of 8 carbapenem-resistant Klebsiella spp. isolated from animal breeding area in this study, and another 72 carbapenem-resistant Klebsiella pneumoniae (CRKP) and 20 carbapenem-resistant Klebsiella quasipneumoniae (CRKQ) in NCBI database. The sample identifier, location, collection date and source were indicated for each isolate. The number of SNPs denoted in red outside the rectangles indicate the number of SNPs differing between the isolates. K. pneumoniae MGH 78578 (GenBank: NC_009648.1) genome sequence was used as reference for construction of the phylogenetic trees based on SNP differences. N/A, not available.
Moreover, the genetic relationships between these strains, and 72 CRKP and 20 carbapenem-resistant Klebsiella quasipneumoniae (CRKQ) stains from the GenBank database were characterized (Figure 4). The sample identifier (ID), location, collection date, and source were indicated for each strain. The results suggest that CRKP and CRKQ were wide spread among humans, animals, and different environmental media (well water, sewage, sludge, river water, and river sediment) in multiple countries, and humans were the most common hosts of CRKP and CRKQ. KW2 and KW3 were clonally related to four CRKP from sludge and sewage in a wastewater treatment plant (WWTT), including K. pneumoniae strains SDWK15, SDWK18, SDWK19, and SDWK20, and two CRKP (K. pneumoniae strains SW03 and SW04) from well water in two animal breeding areas (differed by 4–6 SNPs). KW1 and KW4 were similar to K. quasipneumoniae strain KE1661 from a human rectal swab in Germany (differed by 89 and 87 SNPs, respectively) and K. quasipneumoniae strain SDWK24 from the abovementioned WWTT (differed by 91 and 91 SNPs, respectively; Supplementary Table S4). The WWTT and animal breeding areas are in different counties of Shandong province, more than 200 km and 150 km from this study site, respectively.
Discussion
As animal farms have become one of the most important reservoirs of CRK, it is important to investigate the prevalence and dissemination of CRK in animal breeding areas. In this study, a total of eight blaNDM-5-carrying CRK were isolated from animal feces, well water, and worker feces from seven animal farms. Four CRK were isolated from animal feces and human feces in chicken farms, whereas no CRK was isolated from animal feces and human feces in pig and bovine farms. A possible explanation for this may be the use of antibiotics on the farms. Antibiotics are usually used to prevent and treat diseases in larger quantities and at higher frequencies in chicken farms than in pig and bovine farms, because of the higher breeding density and shorter production cycles of chicken farms, especially broiler farms (Zhao et al., 2010). Several studies have shown results consistent with our research. For example, Gu et al. observed that the carbapenem-resistant Enterobacteriaceae (CRE) detection rate in animal fecal samples was higher in chicken farms compared to bovine and pig farms in northern China (Gu et al., 2022). Qian et al. found that the diversity and abundance of ARGs were higher in chicken feces than in bovine feces sampled from the Shaanxi province in China (Qian et al., 2018). In this study, four CRK were isolated from well water in chicken, pig, and bovine farms, suggesting that groundwater has been contaminated by CRK in this animal breeding area and groundwater may have become a potential CRK reservoir. In addition to CRK, other carbapenem-resistant Enterobacteriaceae species have been detected in well water in animal breeding areas in China. Sun et al. isolated one blaKPC-2-carrying Raoultella ornithinolytica from well water in a pig breeding area in Shandong province (Sun et al., 2017). Zou et al. detected one blaNDM-1-carrying Raoultella ornithinolytica in well water in Weifang city of Shandong province (Zou et al., 2020). Gu et al. conducted a large-scale study in animal breeding areas of six countries in Inner Mongolia and Shandong province, and detected several CRE from well water, including K. pneumoniae, Enterobacter spp., Citrobacter sedlakii, and K. michiganensis (Gu et al., 2022). The results indicate that the groundwater in animal breeding areas is vulnerable to CRE contamination, and it is necessary to strengthen the groundwater monitoring and control of CRE in breeding areas.
Since the IncX3 plasmid pNDM-MGR194 carrying blaNDM-5 was first identified in K. pneumoniae from a patient in India (Krishnaraju et al., 2015), pNDM-MGR194-like plasmids have been found in Enterobacteriaceae worldwide (Alexander et al., 2015; Tian et al., 2020; Chakraborty et al., 2021; Costa et al., 2021). Additionally, previous studies have shown a high capacity of the IncX3 plasmid to mediate the dissemination of blaNDM-5 in Enterobacteriaceae in various environments. Tian et al. reported that the horizontal gene transfer (HGT) of blaNDM-5 among different Enterobacteriaceae species was mainly mediated by IncX3 plasmids in a pediatric hospital in Shanghai (Tian et al., 2020). Gu et al. confirmed that blaNDM-5-carrying IncX3 plasmids are widely disseminated among different Enterobacteriaceae genera among animals and the environment in animal breeding areas in Inner Mongolia and Shandong province (Gu et al., 2022). Zhao et al. reported that blaNDM-5 could disseminate among humans and the environment via IncX3 plasmids in an intensive vegetable cultivation area in Shandong province (Zhao et al., 2021a). In this study, blaNDM-5 was located on highly related conjugative IncX3 plasmids among all strains and flanked by the same core genetic structure: IS5-blaNDM-5-bleMBL-trpF-dsbD-IS26-ISKox3, which confirms that the IncX3 plasmid may serve as a major vehicle for the blaNDM-5 dissemination among animals, the environment, and humans in this animal breeding area.
KH1 and KH2 from two fecal samples from workers at farm BC3 and KA2 from animal feces at farm BC2 carried the colistin resistance gene mcr-8.1 and were resistant to colistin. Colistin is effective against most gram-negative bacteria and is considered a last-resort antibiotic for treating serious infections caused by multidrug-resistant bacteria, particularly CRE (Li et al., 2006; Petrosillo et al., 2019). However, with the emergence and prevalence of CRE, extensive use of colistin has led to the emergence of colistin resistance. In 2016, Liu et al. reported the plasmid-mediated colistin resistance gene mcr-1 in Enterobacteriaceae isolated from animals and humans in China (Liu et al., 2016). In the past 5 years, mcr gene variants (mcr-1 to mcr-10) have been identified in different bacteria isolated from animals, the environment, and humans worldwide (Hussein et al., 2021; Portes et al., 2022). Since Wang et al. reported that mcr-8.1 is located on a conjugative IncFII-type plasmid in K. pneumoniae in 2018 (Wang et al., 2018), K. pneumoniae has become one of the main hosts of mcr-8.1 in animals and humans. Plasmids are considered to play an important role in the dissemination of mcr-8.1 genes, because the majority of mcr-8.1 carriers are on plasmids. Mcr-8.1 has been reported to be located on various plasmids replicon types, especially IncFII, IncFIA, IncFIB, IncQ, IncR, and IncA/C replicon plasmids in Enterobacteriaceae (Hadjadj et al., 2019; Farzana et al., 2020; Wu et al., 2020). In this study, mcr-8.1 was located on the hybrid incompatible plasmid groups IncFII(pKP91)/IncFIA(HI1) and IncFII(K)/IncFIA(HI1), supporting the notion that plasmids are the main factor in disseminating mcr-8.1.
Clonal spread of colistin-resistant CRKP has been reported most frequently in hospitals (Battikh et al., 2016; Jonathan et al., 2017; Rocha et al., 2022). However, several studies have reported the clonal spread of colistin-resistant CRKP in animal farms. For example, Zhai et al. and Sun et al. reported the clonal spread of colistin-resistant CRKP among chickens and the environment in a poultry farm in Hebei province, China and among chickens in a chicken farm in Shandong province, China, respectively (Sun et al., 2020; Zhai et al., 2020). In this study, SNP analysis confirmed the clonal spread of ST37 K. pneumoniae carrying blaNDM-5 and mcr-8.1 between human workers in the same chicken farm. However, because the types of samples were limited, the route of dissemination could not be determined in this study, and further research should clarify the route of clonal spread and provide effective control strategies for the dissemination of colistin-resistant CRKP in animal farms. Additionally, two pairs of clonally related CRKP and CRKQ were isolated from well water in four different farms located at a distance of more than 20 km, revealing that clonal spread also has significant implications for the dissemination of blaNDM-5 in the animal breeding area. Unexpectedly, the pair of clonal CRKP was clonally related to six strains from animal farms and a WWTT collected in other studies in the same province, suggesting that blaNDM-5-carrying K. pneumoniae are capable of disseminating within or between farms in the same county, even in different counties within a province, via clonal expansion. Although the exact route of long-distance transmission of CRKP is uncertain, population movement and travel (Schwartz and Morris, 2018), migration of birds (Wang et al., 2017), and the live poultry trade (Wang et al., 2019) may promote the cross-regional transmission of CRKP.
Conclusion
In this study, we investigated the prevalence of CRK by randomly selecting 20 chicken, 20 pig, and 20 bovine farms from a typical region of the animal farming industry in China. Based on the “One-Health” concept, the transmission of CRK among animals, the environment and humans were discussed. All CRK carried blaNDM-5, which was located on highly related conjugative IncX3 plasmids. Mcr-8.1 was carried by three CRK and located on self-transmissible IncFII(K)/ IncFIA(HI1) and IncFII(pKP91)/IncFIA(HI1) plasmids. Two pairs of clonal CRK from well water and one pair of clonal colistin-resistant CRK from human workers were identified. Thus, both HGT via plasmids and clonal expansion play a significant role in disseminating blaNDM-5 and mcr-8.1 among animals, the environment, and humans in the animal breeding area. The results highlight that systematical monitoring and large-scale investigation of the prevalence and spread of CRK with a “One Health”-perspective in animal breeding areas in Eastern China are urgently needed to enable effective measures for controlling the further spread of CRK.
Data availability statement
The datasets presented in this study can be found in online repositories. The names of the repository/repositories and accession number(s) can be found in the article/Supplementary material.
Ethics statement
Ethical approval to conduct this study was granted by the Ethics Committee for Shandong University [Ethics permission number: 20191202]. The patients/participants provided their written informed consent to participate in this study. Ethical review and approval was not required for the animal study because We only collected animal feces from the animal farms and did not contact animals.
Author contributions
CY: sampling, experiments conducting, data formal analysis, and writing—original draft preparation. BB and JH: writing—reviewing and editing. HZo, CG, LZ, CM, and HZh sampling. XM and XL: conceptualization, supervision, and writing-reviewing. All authors contributed to the article and approved the submitted version.
Funding
This work was supported by the National Key Research and Development Program of China [2020YFC1806904] and the National Natural Science Foundation of China (grant nos. 8197120700 and 41771499).
Conflict of interest
The authors declare that the research was conducted in the absence of any commercial or financial relationships that could be construed as a potential conflict of interest.
Publisher’s note
All claims expressed in this article are solely those of the authors and do not necessarily represent those of their affiliated organizations, or those of the publisher, the editors and the reviewers. Any product that may be evaluated in this article, or claim that may be made by its manufacturer, is not guaranteed or endorsed by the publisher.
Supplementary material
The Supplementary material for this article can be found online at: https://www.frontiersin.org/articles/10.3389/fmicb.2022.1030490/full#supplementary-material
Footnotes
2. ^http://www.genomicepidemiology.org/services/
3. ^http://www.mgc.ac.cn/cgi-bin/VFs/v5/main.cgi
References
Alexander, M. W., David, L. P., Caffery, M., Sowden, D., and Hanna, E. S. (2015). Draft genome sequence of NDM-5-producing Escherichia coli sequence type 648 and genetic context of blaNDM-5 in Australia. Genome Announc. 3, e00194–e00115. doi: 10.1128/genomeA.00194-15
Alikhan, N.-F., Petty, N. K., Ben Zakour, N. L., and Beatson, S. A. (2011). BLAST ring image generator (BRIG): simple prokaryote genome comparisons. BMC Genomics 12:402. doi: 10.1186/1471-2164-12-402
Aziz, R. K., Bartels, D., Best, A. A., DeJongh, M., Disz, T., Edwards, R. A., et al. (2008). The RAST server: rapid annotations using subsystems technology. BMC Genomics 9:75. doi: 10.1186/1471-2164-9-75
Battikh, H., Harchay, C., Dekhili, A., Khazar, K., Kechrid, F., Zribi, M., et al. (2016). Clonal spread of Colistin-resistant Klebsiella pneumoniae coproducing KPC and VIM Carbapenemases in neonates at a Tunisian university hospital. Microb. Drug Resist. 23, 468–472. doi: 10.1089/mdr.2016.0175
BIOHAZ (2013). Scientific opinion on Carbapenem resistance in food animal ecosystems. EFSA J. 11:501. doi: 10.2903/j.efsa.2013.3501
Chakraborty, T., Sadek, M., Yao, Y., Imirzalioglu, C., Stephan, R., Poirel, L., et al. (2021). Cross-border emergence of Escherichia coli producing the Carbapenemase NDM-5 in Switzerland and Germany. J. Clin. Microbiol. 59, e02238–e02220. doi: 10.1128/JCM.02238-20
Costa, A., Figueroa-Espinosa, R., Gaudenzi, F., Lincopan, N., Fuga, B., Ghiglione, B., et al. (2021). Co-occurrence of NDM-5 and RmtB in a clinical isolate of Escherichia coli belonging to CC354 in Latin America. Front. Cell. Infect. Microbiol. 11:852. doi: 10.3389/fcimb.2021.654852
Farzana, R., Jones Lim, S., Barratt, A., Rahman Muhammad, A., Sands, K., Portal, E., et al. (2020). Emergence of Mobile Colistin resistance (mcr-8) in a highly successful Klebsiella pneumoniae sequence type 15 clone from clinical infections in Bangladesh. mSphere 5, e00023–e00020. doi: 10.1128/mSphere.00023-20
Gu, C., Li, X., Zou, H., Zhao, L., Meng, C., Yang, C., et al. (2022). Clonal and plasmid-mediated dissemination of environmental carbapenem-resistant Enterobacteriaceae in large animal breeding areas in northern China. Environ. Pollut. 297:118800. doi: 10.1016/j.envpol.2022.118800
Hadjadj, L., Baron, S. A., Olaitan, A. O., Morand, S., and Rolain, J.-M. (2019). Co-occurrence of variants of mcr-3 and mcr-8 genes in a Klebsiella pneumoniae isolate from Laos. Front. Microbiol. 10:720. doi: 10.3389/fmicb.2019.02720
Hassan, A. M., Johar, A. A., Kassem, I. I., and Eltai, N. O. (2022). Transmissibility and persistence of the plasmid-borne Mobile Colistin resistance gene, mcr-1, harbored in poultry-Associated E. coli. Antibiotics 11:774. doi: 10.3390/antibiotics11060774
He, T., Wang, Y., Sun, L., Pang, M., Zhang, L., and Wang, R. (2017). Occurrence and characterization of blaNDM-5-positive Klebsiella pneumoniae isolates from dairy cows in Jiangsu, China. J. Antimicrob. Chemother. 72, 90–94. doi: 10.1093/jac/dkw357
Hussein, N. H., Al-Kadmy, I. M. S., Taha, B. M., and Hussein, J. D. (2021). Mobilized colistin resistance (mcr) genes from 1 to 10: a comprehensive review. Mol. Biol. Rep. 48, 2897–2907. doi: 10.1007/s11033-021-06307-y
Jonathan, A. O., Doumith, M., Davies, F., Mookerjee, S., Dyakova, E., Gilchrist, M., et al. (2017). Emergence and clonal spread of colistin resistance due to multiple mutational mechanisms in carbapenemase-producing Klebsiella pneumoniae in London. Sci. Rep. 7:12711. doi: 10.1038/s41598-017-12637-4
Kaas, R. S., Leekitcharoenphon, P., Aarestrup, F. M., and Lund, O. (2014). Solving the problem of comparing whole bacterial genomes across different sequencing platforms. PLoS One 9:e104984. doi: 10.1371/journal.pone.0104984
Kareem, S. M., Al-Kadmy, I. M., Kazaal, S. S., Mohammed Ali, A. N., Aziz, S. N., Makharita, R. R., et al. (2021). Detection of gyrA and parC mutations and prevalence of plasmid-mediated quinolone resistance genes in Klebsiella pneumoniae. Infect. Drug Resist. 14, 555–563. doi: 10.2147/idr.s275852
Kim, H. B., Park Chi, H., Kim Chung, J., Kim, E.-C., Jacoby George, A., and Hooper David, C. (2009). Prevalence of plasmid-mediated quinolone resistance determinants over a 9-year period. Antimicrob. Agents Chemother. 53, 639–645. doi: 10.1128/AAC.01051-08
Krishnaraju, M., Kamatchi, C., Jha, A., Devasena, N., Vennila, R., Sumathi, G., et al. (2015). Complete sequencing of an IncX3 plasmid carrying blaNDM-5 allele reveals an early stage in the dissemination of the blaNDM gene. Indian J. Microbiol. 33, 30–38. doi: 10.4103/0255-0857.148373
Li, J., Nation, R. L., Turnidge, J. D., Milne, R. W., Coulthard, K., Rayner, C. R., et al. (2006). Colistin: the re-emerging antibiotic for multidrug-resistant gram-negative bacterial infections. Lancet Infect. Dis. 6, 589–601. doi: 10.1016/S1473-3099(06)70580-1
Liu, Y.-Y., Wang, Y., Walsh, T. R., Yi, L.-X., Zhang, R., Spencer, J., et al. (2016). Emergence of plasmid-mediated colistin resistance mechanism MCR-1 in animals and human beings in China: a microbiological and molecular biological study. Lancet Infect. Dis. 16, 161–168. doi: 10.1016/S1473-3099(15)00424-7
Ma, Z., Liu, J., Yang, J., Zhang, X., Chen, L., Xiong, W., et al. (2020). Emergence of blaNDM-carrying IncX3 plasmid in Klebsiella pneumoniae and Klebsiella quasipneumoniae from duck farms in Guangdong Province, China. J. Glob. Antimicrob. Resist. 22, 703–705. doi: 10.1016/j.jgar.2020.07.001
Magiorakos, A. P., Srinivasan, A., Carey, R. B., Carmeli, Y., Falagas, M. E., Giske, C. G., et al. (2012). Multidrug-resistant, extensively drug-resistant and pandrug-resistant bacteria: an international expert proposal for interim standard definitions for acquired resistance. Clin. Microbiol. Infect. 18, 268–281. doi: 10.1111/j.1469-0691.2011.03570.x
Minarini, L. A. R., and Darini, A. L. C. (2012). Mutations in the quinolone resistance-determining regions of gyrA and parC in Enterobacteriaceae isolates from Brazil. Braz. J. Microbiol. 43, 1309–1314. doi: 10.1590/S1517-83822012000400010
Mirzaii, M., Jamshidi, S., Zamanzadeh, M., Marashifard, M., Malek Hosseini, S. A. A., Haeili, M., et al. (2018). Determination of gyrA and parC mutations and prevalence of plasmid-mediated quinolone resistance genes in Escherichia coli and Klebsiella pneumoniae isolated from patients with urinary tract infection in Iran. J. Glob. Antimicrob. Resist. 13, 197–200. doi: 10.1016/j.jgar.2018.04.017
Navon-Venezia, S., Kondratyeva, K., and Carattoli, A. (2017). Klebsiella pneumoniae: a major worldwide source and shuttle for antibiotic resistance. FEMS Microbiol. Rev. 41, 252–275. doi: 10.1093/femsre/fux013
Petrosillo, N., Taglietti, F., and Granata, G. (2019). Treatment options for Colistin resistant Klebsiella pneumoniae: present and future. J. Clin. Med. 8:934. doi: 10.3390/jcm8070934
Poirel, L., Walsh, T. R., Cuvillier, V., and Nordmann, P. (2011). Multiplex PCR for detection of acquired carbapenemase genes. Diagn. Microbiol. Infect. Dis. 70, 119–123. doi: 10.1016/j.diagmicrobio.2010.12.002
Portes, A. B., Rodrigues, G., Leitão, M. P., Ferrari, R., Conte Junior, C. A., and Panzenhagen, P. (2022). Global distribution of plasmid-mediated colistin resistance mcr gene in salmonella: a systematic review. J. Appl. Microbiol. 132, 872–889. doi: 10.1111/jam.15282
Qian, X., Gu, J., Sun, W., Wang, X.-J., Su, J.-Q., and Stedfeld, R. (2018). Diversity, abundance, and persistence of antibiotic resistance genes in various types of animal manure following industrial composting. J. Hazard. Mater. 344, 716–722. doi: 10.1016/j.jhazmat.2017.11.020
Reo, O., Shigemura, K., Osawa, K., Yang, Y.-M., Maeda, K., Tanimoto, H., et al. (2022). Impact on quinolone resistance of plasmid-mediated quinolone resistance gene and mutations in quinolone resistance-determining regions in extended spectrum beta lactamase-producing Klebsiella pneumoniae isolated from urinary tract infection patients. Pathog. Dis. 80:ftac030. doi: 10.1093/femspd/ftac030
Robicsek, A., Strahilevitz, J., Jacoby, G. A., Macielag, M., Abbanat, D., Hye Park, C., et al. (2006). Fluoroquinolone-modifying enzyme: a new adaptation of a common aminoglycoside acetyltransferase. Nat. Med. 12, 83–88. doi: 10.1038/nm1347
Rocha, V. F. D., Barbosa, M. S., Leal, H. F., Silva, G. E. O., Sales, N. M. M. D., Monteiro, A. D. S. S., et al. (2022). Prolonged outbreak of Carbapenem and Colistin-resistant Klebsiella pneumoniae at a large tertiary Hospital in Brazil. Front. Microbiol. 13:770. doi: 10.3389/fmicb.2022.831770
Schürch, A. C., Arredondo-Alonso, S., Willems, R. J. L., and Goering, R. V. (2018). Whole genome sequencing options for bacterial strain typing and epidemiologic analysis based on single nucleotide polymorphism versus gene-by-gene–based approaches. Clin. Microbiol. Infect. 24, 350–354. doi: 10.1016/j.cmi.2017.12.016
Schwartz, K. L., and Morris, S. K. (2018). Travel and the spread of drug-resistant bacteria. Curr. Infect. Dis. Rep. 20, 1–10. doi: 10.1007/s11908-018-0634-9
Seemann, T. (2014). Prokka: rapid prokaryotic genome annotation. Bioinformatics 30, 2068–2069. doi: 10.1093/bioinformatics/btu153
Sievers, F., Wilm, A., Dineen, D., Gibson, T. J., Karplus, K., Li, W., et al. (2011). Fast, scalable generation of high-quality protein multiple sequence alignments using Clustal omega. Mol. Syst. Biol. 7:539. doi: 10.1038/msb.2011.75
Sun, P., Bi, Z., Nilsson, M., Zheng, B., Berglund, B., Stålsby Lundborg, C., et al. (2017). Occurrence of blaKPC-2, blaCTX-M, and mcr-1 in Enterobacteriaceae from well water in rural China. Antimicrob. Agents Chemother. 61, e02569–e02516. doi: 10.1128/AAC.02569-16
Sun, S., Gao, H., Liu, Y., Jin, L., Wang, R., Wang, X., et al. (2020). Co-existence of a novel plasmid-mediated efflux pump with colistin resistance gene mcr in one plasmid confers transferable multidrug resistance in Klebsiella pneumoniae. Emerg. Microbes Infect. 9, 1102–1113. doi: 10.1080/22221751.2020.1768805
Tacconelli, E., Carrara, E., Savoldi, A., Harbarth, S., Mendelson, M., Monnet, D. L., et al. (2018). Discovery, research, and development of new antibiotics: the WHO priority list of antibiotic-resistant bacteria and tuberculosis. Lancet Infect. Dis. 18, 318–327. doi: 10.1016/S1473-3099(17)30753-3
Tang, B., Chang, J., Cao, L., Luo, Q., Xu, H., Lyu, W., et al. (2019). Characterization of an NDM-5 carbapenemase-producing Escherichia coli ST156 isolate from a poultry farm in Zhejiang, China. BMC Microbiol. 19:82. doi: 10.1186/s12866-019-1454-2
Thomas, A. R., Olson, R., Fang, C.-T., Stoesser, N., Miller, M., MacDonald, U., et al. (2018). Identification of biomarkers for differentiation of Hypervirulent Klebsiella pneumoniae from Classical K. pneumoniae. J. Clin. Microbiol. 56, e00776–e00718. doi: 10.1128/JCM.00776-18
Tian, D., Wang, B., Zhang, H., Pan, F., Wang, C., Shi, Y., et al. (2020). Dissemination of the blaNDM-5 gene via IncX3-type plasmid among Enterobacteriaceae in children. mSphere 5, e00699–e00619. doi: 10.1128/mSphere.00699-19
Tiseo, K., Huber, L., Gilbert, M., Robinson, T. P., and Van Boeckel, T. P. (2020). Global trends in antimicrobial use in food animals from 2017 to 2030. Antibiotics 9:918. doi: 10.3390/antibiotics9120918
Wang, Y., Hu, Y., Cao, J., Bi, Y., Lv, N., Liu, F., et al. (2019). Antibiotic resistance gene reservoir in live poultry markets. J. Infect. 78, 445–453. doi: 10.1016/j.jinf.2019.03.012
Wang, J., Tang, B., Lin, R., Zheng, X., Ma, J., Xiong, X., et al. (2022). Emergence of mcr-1- and blaNDM-5-harbouring IncHI2 plasmids in Escherichia coli strains isolated from meat in Zhejiang, China. J. Glob. Antimicrob. Resist. 30, 103–106. doi: 10.1016/j.jgar.2022.06.002
Wang, X., Wang, Y., Zhou, Y., Li, J., Yin, W., Wang, S., et al. (2018). Emergence of a novel mobile colistinresistance gene, mcr-8, in NDM-producing Klebsiella pneumoniae. Emerg. Microbes Infect. 7, 1–9. doi: 10.1038/s41426-018-0124-z
Wang, Y., Zhang, R., Li, J., Wu, Z., Yin, W., Schwarz, S., et al. (2017). Comprehensive resistome analysis reveals the prevalence of NDM and MCR-1 in Chinese poultry production. Nat. Microbiol. 2:16260. doi: 10.1038/nmicrobiol.2016.260
Wick, R. R., Judd, L. M., Gorrie, C. L., and Holt, K. E. (2017). Unicycler: resolving bacterial genome assemblies from short and long sequencing reads. PLoS Comput. Biol. 13:e1005595. doi: 10.1371/journal.pcbi.1005595
Wu, B., Wang, Y., Ling, Z., Yu, Z., Shen, Z., Zhang, S., et al. (2020). Heterogeneity and diversity of mcr-8 genetic context in chicken-associated Klebsiella pneumoniae. Antimicrob. Agents Chemother. 65, e01872–e01820. doi: 10.1128/AAC.01872-20
Yang, F., Gao, Y., Zhao, H., Li, J., Cheng, X., Meng, L., et al. (2021). Revealing the distribution characteristics of antibiotic resistance genes and bacterial communities in animal-aerosol-human in a chicken farm: from one-health perspective. Ecotoxicol. Environ. Saf. 224:112687. doi: 10.1016/j.ecoenv.2021.112687
Zhai, R., Fu, B., Shi, X., Sun, C., Liu, Z., Wang, S., et al. (2020). Contaminated in-house environment contributes to the persistence and transmission of NDM-producing bacteria in a Chinese poultry farm. Environ. Int. 139:105715. doi: 10.1016/j.envint.2020.105715
Zhan, Q., Xu, Y., Wang, B., Yu, J., Shen, X., Liu, L., et al. (2021). Distribution of fluoroquinolone resistance determinants in Carbapenem-resistant Klebsiella pneumoniae clinical isolates associated with bloodstream infections in China. BMC Microbiol. 21:164. doi: 10.1186/s12866-021-02238-7
Zhao, Q., Berglund, B., Zou, H., Zhou, Z., Xia, H., Zhao, L., et al. (2021a). Dissemination of blaNDM-5 via IncX3 plasmids in carbapenem-resistant Enterobacteriaceae among humans and in the environment in an intensive vegetable cultivation area in eastern China. Environ. Pollut. 273:116370. doi: 10.1016/j.envpol.2020.116370
Zhao, L., Dong, Y. H., and Wang, H. (2010). Residues of veterinary antibiotics in manures from feedlot livestock in eight provinces of China. Sci. Total Environ. 408, 1069–1075. doi: 10.1016/j.scitotenv.2009.11.014
Zhao, Y., Liu, L., Wang, S., Tian, M., Qi, J., Li, T., et al. (2021b). Draft genome sequence analysis of a novel MLST (ST5028) and multidrug-resistant Klebsiella quasipneumoniae subsp. similipneumoniae (Kp4) strain 456S1 isolated from a pig farm in China. J. Glob. Antimicrob. Resist. 24, 275–277. doi: 10.1016/j.jgar.2021.01.006
Zou, H., Berglund, B., Xu, H., Chi, X., Zhao, Q., Zhou, Z., et al. (2020). Genetic characterization and virulence of a carbapenem-resistant Raoultella ornithinolytica isolated from well water carrying a novel megaplasmid containing blaNDM-1. Environ. Pollut. 260:114041. doi: 10.1016/j.envpol.2020.114041
Keywords: carbapenem-resistant Klebsiella spp., animal breeding area, blaNDM-5, mcr-8.1, plasmid, clonal expansion
Citation: Yang C, Han J, Berglund B, Zou H, Gu C, Zhao L, Meng C, Zhang H, Ma X and Li X (2022) Dissemination of blaNDM-5 and mcr-8.1 in carbapenem-resistant Klebsiella pneumoniae and Klebsiella quasipneumoniae in an animal breeding area in Eastern China. Front. Microbiol. 13:1030490. doi: 10.3389/fmicb.2022.1030490
Edited by:
Dongchang Sun, Zhejiang University of Technology, ChinaReviewed by:
Biao Tang, Zhejiang Academy of Agricultural Sciences, ChinaXiaofei Jiang, Fudan University, China
Copyright © 2022 Yang, Han, Berglund, Zou, Gu, Zhao, Meng, Zhang, Ma and Li. This is an open-access article distributed under the terms of the Creative Commons Attribution License (CC BY). The use, distribution or reproduction in other forums is permitted, provided the original author(s) and the copyright owner(s) are credited and that the original publication in this journal is cited, in accordance with accepted academic practice. No use, distribution or reproduction is permitted which does not comply with these terms.
*Correspondence: Xianjun Ma, 18560087567@163.com; Xuewen Li, lxw@sdu.edu.cn