- 1College of Horticulture, Gansu Agriculture University, Lanzhou, China
- 2Institute of Biology, Gansu Academy of Sciences, Lanzhou, China
As one of the important sources of human nutrition, Brassicaceae vegetables are widely grown worldwide. Black rot caused by Xanthomonas campestris pv. campestris (Xcc) seriously affects the quality and yield of Brassicaceae vegetables. Therefore, it is important to study control methods of Xcc for Brassicaceae vegetable production. This paper reviews the physical, chemical, and biological control methods of Xcc in Brassicaceae vegetables developed in recent years, and the underlying mechanisms of control methods are also discussed. Based on our current knowledge, future research directions for Xcc control are also proposed. This review also provides a reference basis for the control of Xcc in the field cultivation of Brassicaceae vegetables.
Introduction
The Brassicaceae comprises approximately of 3,700 species, including vegetable, forage, and oil-seed brassicas. Moreover, the planting area of Brassicaceae vegetables is expanding rapidly year by year. However, Brassicaceae vegetables are susceptible to infection by numerous fungal and bacterial pathogens, including Xanthomonas campestris pv. campestris (Xcc) (Li et al., 2019). Xcc causes black rot (BR) and is one of the most destructive and yield-limiting diseases of Brassicaceae vegetables (Vicente and Holub, 2013; Kong et al., 2021; Koroleva et al., 2021). Since BR was discovered in 1894 in the USA, it has spread rapidly around the world and is now found in major Brassicaceae vegetable producing areas (Garman, 1894; Jensen et al., 2010). Seedborne Xcc can survive in crop debris or crucifer weeds, and it is especially damaging to Brassicaceae vegetables. Under conditions with average temperatures between 25–30°C and with sufficient rainfall or heavy dew, Xcc enters the host plant vascular system through hydathodes or wounds caused by machinery or insects (Vicente and Holub, 2013). Except for some cases in which no symptoms occur (latent infection), the bacterium causes a systemic vascular disease, the typical symptoms of which are vein blackening, leaf tissue necrosis, and V-shaped chlorotic lesions (Marroni and Germani, 2014). However, symptoms of BR may differ among different Brassicaceae vegetables. These symptoms reduce the quality and value of the Brassicaceae, a crop in which the leaves are the major commercial product (Vicente and Holub, 2013). Indeed, in some cases, Brassicaceae crops can be entirely lost to BR (Jensen et al., 2005). Hence, it is important to study the methods and mechanisms of BR resistance of Brassicaceae vegetables.
Several agricultural practices and culture methods, such as planting material free of Xcc (seeds or transplants), crop rotation, and eliminating other possible sources of inoculum, such as residues of infected crops and Brassicaceae weeds, have been reported to control BR (Krauthausen et al., 2011; Ryan et al., 2011; Barzman et al., 2015). However, these methods are all preventative, and cannot control BR once the plant is infected (Vicente and Holub, 2013; Holtappels et al., 2021). Planting BR resistant varieties is the most economical and effective approach to control this disease. Although some Brassicaceae, such as radish (Raphanus sativus) and Chinese cabbage (Brassica rapa), BR resistant varieties have been developed, the vast majority of Brassicaceae vegetables still lack effective BR resistant varieties. Unfortunately, the large number of physiological races of Xcc and the differences in the genome types of Brassicaceae make it difficult to cultivate BR resistant varieties of cabbage and other vegetables. At present, Xcc isolates have been differentiated into 11 physiological races based on comparisons of their whole genome sequences, and physiological races 1 and 4 are the two main races that damage Brassicaceae vegetables (Vicente et al., 2001; Qian et al., 2005; Vicente and Holub, 2013; Singh et al., 2016; Cruz et al., 2017). From a production point of view, cultivating varieties resistant to race 1 and race 4 is the main direction of breeding for resistance to BR. However, sources resistant to race 1 and 4 are very rare in genome C (Brassica oleracea), while they are relatively common in A and B genomes (B. rapa and Brassica nigra), which has seriously restricted the breeding of BR resistance varieties of cabbage and other genome C-type plants (Afrin et al., 2018). Therefore, the study of other control methods, especially highly efficient broad-spectrum and pollution-free control methods, has important production guiding value for Brassicaceae vegetable production (Lamichhane et al., 2017).
A large number of in vivo and in vitro studies have shown that there are many physical methods, chemical treatments, and biological control methods that can inhibit the activity or pathogenicity of Xcc. These studies provide references for the pollution-free control of Brassicaceae vegetables BR. However, there is no review of the physical (hot water, ultraviolet light), chemical (pesticides, chemical substances), and biological (plant aqueous extracts, Xcc antagonistic bacteria, biological products) methods for Xcc control. The purpose of the present review is to provide a theoretical basis for the control of BR in the production of Brassicaceae vegetables by analyzing reported control methods and control mechanisms, and to predict future potential control methods for Xcc.
Physical control of Xanthomonas campestris pv. campestris
Hot water
As a common and effective strategy that is widely used in seed disinfection in production, soaking seeds in 45–55°C hot water can economically and effectively kill microbes that are latent or stuck to the inside and outside of seeds. As a bacterium, Xcc can spread over long distances via seeds. The usual lethal temperature of Xcc is 40°C; therefore, Xcc in seeds can be killed by hot water treatment at higher temperatures. Hot water was first observed to kill the Xcc in the 1920s (Walker, 1923). After 50 or 52°C hot water treatment of cauliflower (Brassica oleracea L. var. botrytis L.) seeds, the incidence of BR in the field decreased significantly (Sharma, 1981). Soaking in 52°C hot water for 0.5 h had the best control effect (Shah et al., 1985). Subsequent 3-year and 1-year trials also showed that hot water treatment (50°C for 20 min) of cauliflower (B. oleracea L. var. botrytis L.) seeds decreased Xcc activity (Shah et al., 1985; Van Der Wolf and Van Der Zouwen, 2010). In addition to killing Xcc in the seeds, the process of soaking allows dry seeds to quickly absorb the water needed for germination. However, soaking seeds in hot water not only had a control effect on Xcc, but also strongly decreased the germination rate of treated Chinese cabbage (B. rapa) seeds (Velasco et al., 2013). It is possible that the temperature of the water used for soaking was too high or the soaking time was too long, resulting in seed damage.
Nanoparticles
As a high-tech invention in the 21st century, nanoparticles have been widely used in agricultural production, plant protection, plant nutrition, and other fields. For Xcc control, 800 mg/L Gly-Cu(OH)2 nanoparticles (mean diameter of 240 nm) increased the Xcc resistance of Chinese cabbage (B. rapa pekinensis), and had a better effect than 400–800 mg/L of Kocide, a fungicide/bactericide (Dong et al., 2020). In addition to nano-copper, nano-silver has also been found to prevent Xcc infection. The growth of Xcc could be inhibited at 0.02 μg/ml, and >0.1 μg/ml nano-silver nanoparticles (AgNPs) could destroy the Xcc cell membrane (Gan et al., 2010). The antibacterial effect of AgNPs depended on their size and concentration (Velasco et al., 2013; Pecenka et al., 2021). Nanoparticles can replace the hydrogen bonds between thymidine and purine, and cytosine and guanine in the double helix structure of DNA by combining with DNA in the cell membrane and cell wall of bacteria, resulting in changes to the molecular structure of bacterial DNA, inhibiting the synthesis of DNA, RNA, and proteins, thereby inactivating pathogenic bacteria (Duran et al., 2010).
Other physical methods
In addition to soaking seeds and using nanoparticles, other physical methods can also be used in Xcc control. Using different ultraviolet light (UV-C) doses (1.3–7.5 kJ/m2), study have showed that low doses of UV-C treatment of seeds could elicit resistance to Xcc and improve the quality and growth of cabbage (Brassica oleracea var. capitata). UV-C at 3.6 kJ/m2 was effective in reducing BR and the population density of Xcc in infected cabbage leaves (Brown et al., 2001). UV-C can change the genetic material of pathogenic bacteria and change cellular transcription characteristics, such that the organism loses the ability to synthesize proteins and its reproduction ability (Hamkalo, 1972). In addition to UV-C pre-treatment before sowing, because Xcc enters the plant through wounds or/and the hydathodes on the leaves, BR can also be controlled by spraying the agents to form a protective film on the leaves during the growth period. Xcc infection is particularly harmful because of the formation of biofilms, which contain degradative extracellular enzymes and other virulence factors (Dow et al., 2003). By forming an insulating layer on cabbage (B. oleracea var. capitata) leaves, a novel carbon-based biomaterial, Se/C (formed by adding with a very small amount of selenium into carbon), could restrain Xcc infection (Cao et al., 2020).
Chemical control of Xanthomonas campestris pv. campestris
Pesticides
Pesticides are widely used to prevent and control plant diseases (Lamichhane et al., 2017). Studies have shown that some pesticides can provide control of Xcc. Seed dipping in 100 ppm streptocycline significantly reduced the incidence of BR on cauliflower (B. oleracea L. var. botrytis L.) by seed dipping (Sharma, 1981). The 0.1% streptocycline seed treatment had a good control effect on Xcc in a 3-year cauliflower trial (Shah et al., 1985). By comparing the Xcc control efficiency of streptomycin, oxytetracycline, chloramphenicol, rifampicin, and mancozeb at 200 ppm, it was found that streptomycin provided 100% BR control in cauliflower, followed by oxytetracycline and chloramphenicol, while mancozeb was ineffective (Lenka and Ram, 1997). In addition, 16 ml/kg Metham-sodium could significantly reduce the counts of the Xcc in cauliflower stems, siliques, and seeds, and reduced the incidence of Xcc symptoms. Moreover, the effectiveness of control was also related to the depth of the application of the Metham-sodium into the soil (Kritzman and Ben-yephet, 1990). By analyzing the germination rate and disease symptoms of Chinese cabbage seeds artificially inoculated with Xcc and soaked in streptocycline and 18 other pesticides, it was observed that the anti-Xcc effect of 200 g/ml streptocycline was higher than that of mercuric chloride, Mancozeb, carbendazim, 2-methoxyethylmercury chloride, and ampicillin; while the anti-Xcc effects of chlortetracycline, oxytetracycline, and chloramphenicol were higher than that of streptocycline at 200 g/ml (Bhat and Masoodi, 2000). Similarly, studies on cabbage (B. oleracea var. capitata) and kale (Brassica oleracea var. acephala) showed that pesticides had a good control effect on BR. Kocide and Actigard significantly suppressed the incidence of BR on cabbage (Langston and Cummings, 2003). Lime sulfur could not only prevent BR, but also improved the nutritional quality of kale (B. oleracea var. acephala) in non-inoculated conditions (Nunez et al., 2018). Although the above studies showed that many chemical pesticides can control BR, the molecular mechanisms of resistance to these agents have not been determined.
By spraying Validamycin-A (VMA) and acibenzolar-S-methyl (ASM) on cabbage (Brassica oleracea var. capitata) leaves, studies investigated the BR control mechanism of the two agents, and found that 62.5 g/L VMA effectively inhibited the production of Xcc extracellular polysaccharide (EPS), and injection of EPS from Xcc on medium containing VMA produced fewer lesions, suggesting that VMA might inhibit the multiplication of Xcc and affect the quantity and quality of EPS (Ishikawa et al., 2004). Unlike EPS, ASM could decrease Xcc symptoms and oxidative stress by increasing the activities of superoxide dismutase, peroxidase, and ascorbate peroxidase (Amaral et al., 2019), while the activities of chitinase, β-1,3-glucanase, and polyphenol oxidase, and the concentrations of hydrogen peroxide and malondialdehyde, decreased (Fontana et al., 2021).
Chemical substances
Soaking the seeds with chemicals
Pretreatment of seeds with chemical substances can also prevent Xcc infection. Soaking seeds for 16 h in a 10–20 g/kg calcium hypochlorite slurry reduced Xcc undetectable levels in cabbage (B. oleracea var. capitata) seeds (Schultz et al., 1986). Soaking seeds for 30 min using 3% hydrogen peroxide also killed 100% of Xcc in cabbage (B. oleracea var. capitata) seeds (Sanna et al., 2022). Pre-treatment of Xcc-contaminated cabbage (B. oleracea var. capitata) seeds with CAC-717 (a new disinfectant produced by applying an electric field and water flow to distilled water containing calcium hydrogen carbonate to produce mesoscopic crystals) significantly reduced Xcc cell numbers recovered from the seeds compared with using distilled water (Sakudo et al., 2020).
CuSO4
CuSO4 is widely used in disease control because of its low cost and broad-spectrum antimicrobial activities (Chen et al., 2021). The first study of CuSO4 on Xcc showed that CuSO4 was ineffective in Xcc control (Lenka and Ram, 1997). However, the Xcc killing effects of t-butyl hydroperoxide and hydrogen peroxide increased after the addition of 100 mM CuSO4 to Silva-Buddenhagen medium (Patikarnmonthon et al., 2010). The incidence of BR in kale (B. oleracea var. acephala) was also reduced after Bordeaux mixture (an inorganic copper bactericide mainly composed of CuSO4) was applied, and the nutritional value also improved in non-inoculated kale (B. oleracea var. acephala) (Nunez et al., 2018). Cu(OH)2 has also been found to improve broccoli (B. oleracea L. var. botrytis L.) BR resistance (Krauthausen et al., 2011). The above studies indicated that Cu2+ was the main factor that increased Xcc resistance, in which Cu2+ kills bacteria mainly by denaturing and solidifying bacterial proteins (Cooksey, 1990).
Other chemical substances
In addition to the use of sterilizing chemicals for seed immersion disinfection, chemical treatment during the growing period can also enhance plant resistance to Xcc invasion. After spraying benzoic acid, the BR resistance of cauliflower (B. oleracea L. var. botrytis L.) increased (Krauthausen et al., 2011). Foliar spraying of p-coumaric acid (pCA) promoted the accumulation of specific hydroxycinnamic acids, pCA, ferulic acid, sinapic acid epigallocatechin and epigallocatechinin in Xcc-inoculated Chinese cabbage (B. rapa) (Islam et al., 2018). pCA primed the JA-signaling mediated induction of phenylpropanoid biosynthesis to produce Xcc resistance in oilseed rape (Brassica napus), in which the expression of phenylpropanoid biosynthesis-related genes was upregulated during pre-treatment with pCA (Islam et al., 2019). Using 10 kinds of epigenetic modulating chemicals (Azacytidine, -Oryzanol, Lomeguatrib, RG108, Zebularine, Cambinol, CAY10602, Sirtinol, SRT1720 Hydrochloride, Suramine), Baranek et al. found the use of DNA demethylating chemicals unambiguously caused a durable decrease in Xcc virulence via altered sirtuin activity, even after its re-isolation from infected Chinese cabbage (B. rapa) (Baranek et al., 2021). Xantho-oligosaccharides not only inhibited the growth of Xcc, but also reduced the production of xanthan, which is associated with the virulence of Xcc (Qian et al., 2006). These studies suggested that the incidence of BR could be reduced by application chemical substances that enhance the phenylpropane synthesis pathway in plants or reduce Xcc toxicity.
Biological control of Xanthomonas campestris pv. campestris
Aqueous extracts
Many chemical substances and pesticides are expensive and harmful to the environment, thus it is important to explore environmentally friendly BR control methods for pollution-free Brassicaceae vegetable production (Adesola et al., 2021; Mouna et al., 2021). Therefore, mostly in laboratory studies, plant extracts can be explored as candidates for the management of BR. Studies have shown that extracts from many plants have killing effects on Xcc in plants and/or growth on culture medium. Tiwari et al. found that Datura metel, Allium sativum, Zingiber officinale, Parthenium hysterophorus, and Spiranthus indicus could kill Xcc (Tiwari et al., 2004). And 250–1,000 mg/L of Mikania glomerata alcoholic extract could inhibit Xcc growth in vitro (Vigoschultz et al., 2006). Furthermore, 0.5 and 0.1 mg/ml of methanolic, hydroalcoholic, and hydroalcoholic maltodextrin M. glomerata extracts showed bacteriostatic and bactericidal effects by altering the membrane permeability and biofilm formation of Xcc (Fontana et al., 2021). Using in vitro and in vivo tests of 20 kinds of botanical extracts, it was concluded that the extracts of A. sativum, Azadirachta indica, Tamarix aphylla, Vernonia anthelmentica, Plumbago zelanicum, and Tegetes erecta significantly suppressed the growth of Xcc and resulted in better seed germination and plant vigor (Sain, 2007). Research on the bacteriostatic activity of 20 kinds of botanical extracts also showed that the leaf extracts of Acacia arabicae, Acacia fernesiana, Acacia catechu, Achyranthus asper, Aegle marmelos, Asparagus racemosus, A. indica, Callistemon lanceolatus, and Camellia sinensis showed an inhibitory effect against Xcc (Bhardwaj and Laura, 2009). Xcc was also kill by the petal extracts of T. erecta, Chrysanthemum coronarium, A. fernesiana, Anthocephalus cadamba, Bombax malabaricum, Lathyrus odoratus, Rosa damascena, and Thevetia nerifolia (Bhardwaj and Bhardwaj, 2011). These studies indicated that the anti-Xcc activity was not only related to the plant material, but also to the plant parts. Meanwhile, extracts from the same plant using different solvents also had different antibacterial properties. The water extracts of Ocimum gratissimum and Tylophora asthmatica were effective in inhibiting the growth of Xcc, while the alcohol extract of O. gratissimum was the most effective in inhibiting the growth of Xcc, followed by that of Calotropis gigentea, T. asthmatica, Ocimum sanctum, Nigella sativa, and Ruta graveolens (Kumar et al., 2018). In addition, the Trichoderma atroviride metabolite, 6-pentyl-α-pyrone, also could inhibit Xcc by increasing the antibiofilm activity (Papaianni et al., 2020). Most of these studies only analyzed the antibacterial activity of the total extracts from the whole plant or some organs and did not study the components related to BR resistance (Table 1). The resistant secondary metabolites such as flavonoids, phenolic acids, alkaloids, isothiocyanates, tannins, and saponins in these plants might be the components of Xcc resistance.
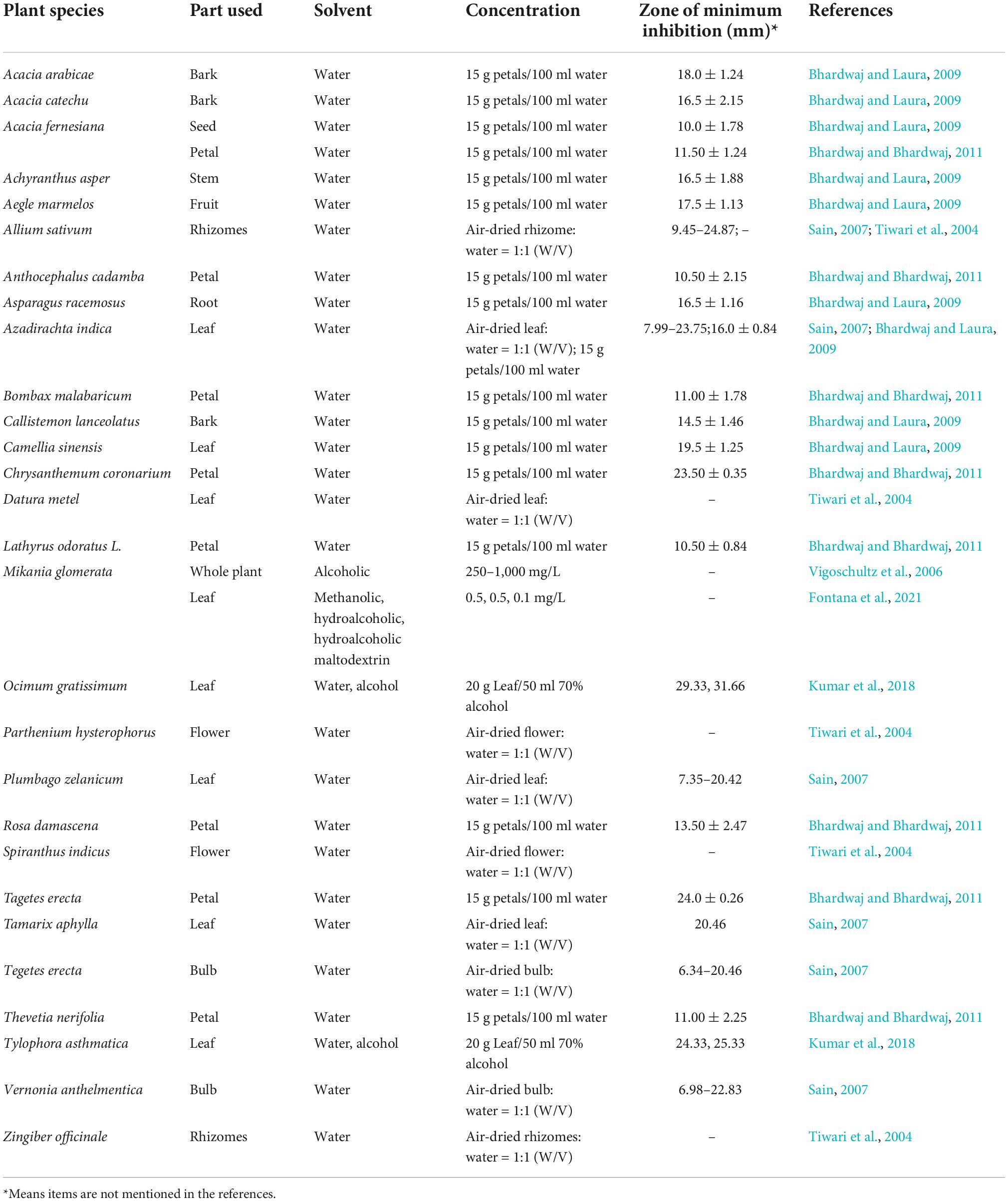
Table 1. Effects of extracts from different crops and different tissues on the antibacterial ability of Xcc.
In addition to the above medicinal plants and non-Brassicaceae plants, a study on cress (Lepidium sativum), salad rocket (Eruca sativa), broccoli (Brassica olerecea L. var. italica), white cabbage (B. olerecea L. var. capitata), and tronchuda cabbage (B. oleracea L. var. tronchuda cv. Tronchuda) showed that the hydrolytic products of glucosinolates could inhibit Xcc growth, although there were no significant correlations between Xcc infection and total phenolics (Aires et al., 2011). However, using the methanolic extracts of Chinese cabbage (B. rapa) leaves, it was concluded that the glucosinolates (Gluconapin, and gluconapin-isothiocyanate) and phenolics had inhibitory effects on the development of Xcc, and there was a significant positive correlation between their concentration and the inhibitory effect (Velasco et al., 2013). In addition to extracts derived from plants using solvents, Piper hispidum leaves essential oils (trans-a-bisabolene, b-pinene, a-pinene, allo-aromadendrene, (-) spatulenol, and L-linalool), obtained by hydrodistillation in a Clevenger-type apparatus, also showed weak antibacterial activity against Xcc (Sánchez Pérez et al., 2014). The thymol and carvacrol in Lippia gracilis essential oils are the main anti-Xcc components (Rafael et al., 2019). Most of these studies only studied the antibacterial activity and BR resistance of relevant plant extracts, and did not study their antibacterial mechanism; however, they also provided a theoretical basis and data support for the subsequent development of plant-derived pesticides for BR control.
Bacillus
Bacillus are gram-positive rhizobacteria with broad-spectrum bactericidal activity, which secrete a variety of enzymes and antibiotics to inhibit the growth of other bacteria and can prevent and control a variety of plant diseases (Babin et al., 2022; Jing et al., 2022). Previous studies identified that there is an antagonistic interaction between Xcc and Bacillus species in the natural ecosystem. Studies on the resistance of Bacillus to Xcc mainly include in vitro bacteriostatic experiments and resistance mechanism studies on Brassicaceae crops. In vitro tests using Bacillus isolates found that Bacillus amyloliquefaciens, Bacillus subtilis, and Bacillus pumilus isolates could inhibit the growth of Xcc to varying degrees (Sain, 2007; Wulff et al., 2010), with B. amyloliquefaciens being the most effective (Wulff et al., 2010; Li’aini et al., 2017). B. subtilis, B. pumilus, Bacillus megaterium, Bacillus cereus, Bacillus velezensis, Paenibacillus, and Bacillus thuringiensis also showed antibiotic activity against the Xcc (Luna et al., 2002; Ghazalibiglar et al., 2016; Liu et al., 2016a; Jelusic et al., 2021). In addition, there were significant correlations between the mean diameter of the inhibition zone, the type of bacteria, and the type of culture medium (Issazadeh et al., 2012; Silva et al., 2018). The gas chromatography-mass spectrometry (GC-MS) and high performance liquid chromatography (HPLC)-electrospray ionization (ESI)-quantitative time of flight (qTOF)/mass spectrometry (MS) results for the benzene extracts and ethyl acetate chemical compositions of two Bacillus strains showed that they contained numerous antimicrobial volatile organic compounds (e.g., alkenes, benzenes, carboxylic acids, indoles, and pyrazines), and antimicrobial metabolites (lipopeptides, and/or antibiotics) (Jelusic et al., 2021). Moreover, the biosynthesis of surfactin, kurstakin, bacillomycin, and iturin are involved in the killing process of Xcc, and the contents of these antibacterial substances are closely related to resistance to Xcc (Wulff et al., 2010; Jelusic et al., 2021; Macha et al., 2021).
In vivo tests concluded that the Bacillus and B. amyloliquefaciens could significantly reduce the incidence and severity of BR in the foliage, stems, and heads of cabbage (B. oleracea var. capitata) and oilseed rape (B. napus) (Sain, 2007; Massomo et al., 2010; Wulff et al., 2010; Li’aini et al., 2017; Jelusic et al., 2021). Not only can Bacillus strains prevent Xcc, but also their cell-free culture medium exerts a killing effect on Xcc. Paenibacillus, B. velezensis, and B. megaterium cell-free supernatants were found to be effective against Xcc in cabbage (B. oleracea var. capitata), kale (B. oleracea var. acephala), and oilseed rape (B.napus) (Liu et al., 2016a; Silva et al., 2018; Jelusic et al., 2021). Besides, the control effect of Bacillus is not only related to the species of bacteria, but also to the species of plants and their growing environment. By evaluated the control efficiency of B. subtilis against Xcc using cabbage (B. oleracea var. capitata), cauliflower (B. oleracea L. var. botrytis L.), oilseed rape (B. napus) and broccoli (B. oleracea L. var. botrytis L.) grown in three consecutive growing seasons and on two types of soil, it found that B. subtilis was effective in broccoli, but not in cabbage and rape during the main rainy season in clay loam soil, and a limited biological control effect was still observed when these crops were grown in sandy loam soil (Wulff et al., 2002). Moreover, root application had the best effect compared with seed soaking, leaf or foliage (cotyledons) spraying and soil drenching (Massomo et al., 2010). In addition to reducing the incidence of BR, B. velezensis, B. mojavensis, and Paenibacillus could also improve crop growth (shoot fresh weight, shoot dry weight, root fresh weight, and root dry weight) and yield (Sain, 2007; Wulff et al., 2010; Ghazalibiglar et al., 2015, 2016; Liu et al., 2016b). A study on the anti-Xcc mechanism of B. thuringiensis showed that RpfF is essential for the production of six diffusible signal factor family signals in Xcc, which employ the same signaling pathways to regulate their biological functions in Xcc and have similar effects on reduction of cell division, sporulation, and antibiotic resistance of B. thuringiensis. Abrogation of RpfF decreased the competitive capability of Xcc against B. thuringiensis on the surface of Chinese cabbage (B. rapa) leaves (Deng et al., 2016).
Pseudomonas
Non-pathogenic Pseudomonas is active in the plant rhizosphere, and belongs to the PGPR (Plant Growth-promoting Rhizobacteria). Pseudomonas can not only promote plant growth by producing active substances or mineral elements, but also inhibits or hinders the development of pathogenic microorganisms in the root zone by producing metabolic substances or via competition (Muthukumar et al., 2022). Studies have shown that Pseudomonas, similar to Bacillus, also has a controlling effect on Xcc. In vitro tests, using different kinds of PGPRs found that P. aeruginosa KA19 and AP218 could significantly inhibit the growth of Xcc (Mishra and Arora, 2012; Liu et al., 2016a). Moreover, Pseudomonas orientalis X2-1P obtained from the oilseed rape (B. napus) phyllosphere was also found to be effective against Xcc in vitro when applied as a whole culture (Jelusic et al., 2021; Table 2). In vivo, P. aeruginosa KA19 was effective in reducing Brassica campestris BR lesions via foliar spray or by combined seed soaking and soil drenching in the greenhouse (Mishra and Arora, 2012). The application of P. orientalis X2-1P cell-free supernatant was also found to be effective against Xcc (Jelusic et al., 2021). In addition, Pseudomonas fluorescens can induce Xcc resistance in cabbage (B. oleracea var. capitata) seedlings (Umesha and Roohie, 2017). GC-MS and HPLC-MS analyses indicated that there were numerous antimicrobial volatile organic compounds (e.g., alkenes, benzenes, carboxylic acids, indoles, and pyrazines, etc.), lipopeptides, and/or antibiotics in P. orientalis ethyl acetate and benzene extracts (Jelusic et al., 2021; Table 3).
Bacteriophages
Bacteriophages are viruses that infect bacteria universally in the environment (Fischetti, 2008). They can not only “obligate hunt” and “precision target” the pathogenic bacteria in soil, reducing their survival competitiveness, but also can adjust the structure of the rhizosphere soil flora, restore community diversity, and increase the abundance of beneficial bacteria in the community (Da Silva et al., 2018; Holtappels et al., 2021). The initial use of bacteriophages to control plant disease dates back almost a century: they were first used to control Xcc in 1924 (Mallmann and Hemstreet, 1924). Bacteriophages significantly reduced the concentration of Xcc in infected seed extracts, and the disease rate after bacteriophage treatment was reduced by 2.0–4.1 times in cabbage (B. oleracea var. capitata) seedlings compared with that in the control (Ha et al., 2017; Holtappels et al., 2022). A bacteriophage suspension was also effective in controlling Xcc in cabbage (B. oleracea var. capitata) and cauliflower (B. oleracea L. var. botrytis L.) (Marroni and Germani, 2014; Nagai et al., 2017). Considering the different pathogenicities of Xcc in different physiological races, the BR could control by releasing weakly virulent Xcc. Potentially, weakly virulent Xcc could be deployed to activate defense mechanisms and increase the persistence of bacteriophages specific to the target Xcc on Brassica campestris, B. nigra, Brassica geniculata, and R. sativus leaf surfaces (Schaad and Ignatov, 2019). A study on the anti-Xcc mechanism showed that the Xcc-specific bacteriophage Xccφ1 could inhibit Xcc by interfering with the genes (rpf, gumB, clp, and manA) involved in the formation of biofilms (Papaianni et al., 2020)
Other bacteria
Studies have shown that in addition to Bacillus, Pseudomonas, and bacteriophages, certain other bacteria can also inhibit or kill Xcc. Acinetobacter lactucae QL-1 effectively attenuated Xcc virulence through quorum quenching in radish (R. sativus), and co-inoculation of Xcc and QL-1 significantly reduced the BR incidence and severity (Ye et al., 2019a). Using two novel diffusible signal factor degrading strains, Cupriavidus HN-2 and Burkholderia anthina HN-8, which were isolated from contaminated soil. It was found that HN-2 and HN-8 could substantially reduce BR disease severity caused by Xcc in radish (R. sativus) and Chinese cabbage (B. rapa) (Ye et al., 2019b,2020; Table 3).
Other biological control methods
In addition to plant extracts and Xcc antagonism, biological products can also help resist Xcc by improving plant resistance. The application of biofertilizer formed by the fermentation of cow manure and sucrose molasse reduced kale (B. oleracea var. acephala) BR severity by 56% in the field, and increased phenylalanine ammonia lyase, catalase, and peroxidase activities and the lignin content (Nunez et al., 2018). Hence, application of biofertilizer is a promising technique to control BR of Brassicaceae vegetables and also improves their nutritional quality and yield. Milk-based products (raw milk and whey) also reduced kale (B. oleracea var. acephala) BR severity by 44% in the field; meanwhile, the antioxidant activity, crude protein, and fiber contents increased after spraying raw milk on the Xcc-inoculated leaves (Nunez et al., 2018).
Future work
Black rot (caused by Xcc) is one of the most important diseases of Brassicaceae vegetables worldwide, which seriously threatens the production of Brassicaceae vegetables and causes significant economic losses to growers (Cho et al., 2012; Kuznetsov et al., 2020; Pang et al., 2020). Research on the resistance methods and mechanisms of BR are important for the production of Brassicaceae vegetables. To control BR, researchers and growers have studied disease resistance breeding, disease resistance methods, and disease resistance mechanisms (Ryan et al., 2011; Barzman et al., 2015). However, because of the large number of Xcc physiological races and the diversity of Brassicaceae vegetable genome types, currently, BR resistant materials are relatively rare, and lack practicality and diversity (Vicente et al., 2001; Vicente and Holub, 2013; Singh et al., 2016; Cruz et al., 2017). Therefore, there is an urgent need to study the disease resistance methods and mechanism of Brassicaceae vegetables.
Similar to most other plant disease control methods, BR control is mainly achieved by improving plant resistance and reducing the pathogenicity of Xcc. At present, a variety of antibiotics and pesticides, such as streptocycline, oxytetracycline, and chloramphenicol have been screened using in vitro antibacterial tests in culture medium and in vivo tests (Sharma, 1981; Shah et al., 1985; Lenka and Ram, 1997). However, the complexity of BR prevention and control means most of these studies remain in the laboratory stage and chemical pesticides are less frequently used in practical production (Langston and Cummings, 2003; Lamichhane et al., 2017). The chemical pesticides currently in use not only pollute the environment, but also easily cause Xcc to form chemical resistance because of their small number. Therefore, pesticides should be used alternately in the field application process (seed soaking, leaf spraying, root irrigation) to improve the effectiveness of BR control. At the same time, new pesticides with low toxicity and high control efficiency should be developed. In addition to chemical pesticides, research on disease resistance without environmental pollution should mainly focus on the use of hot water or chemical agents for seed disinfection, the use of chemical agents or fertilizers to improve plant resistance, and the use of Xcc antagonism (Figure 1).
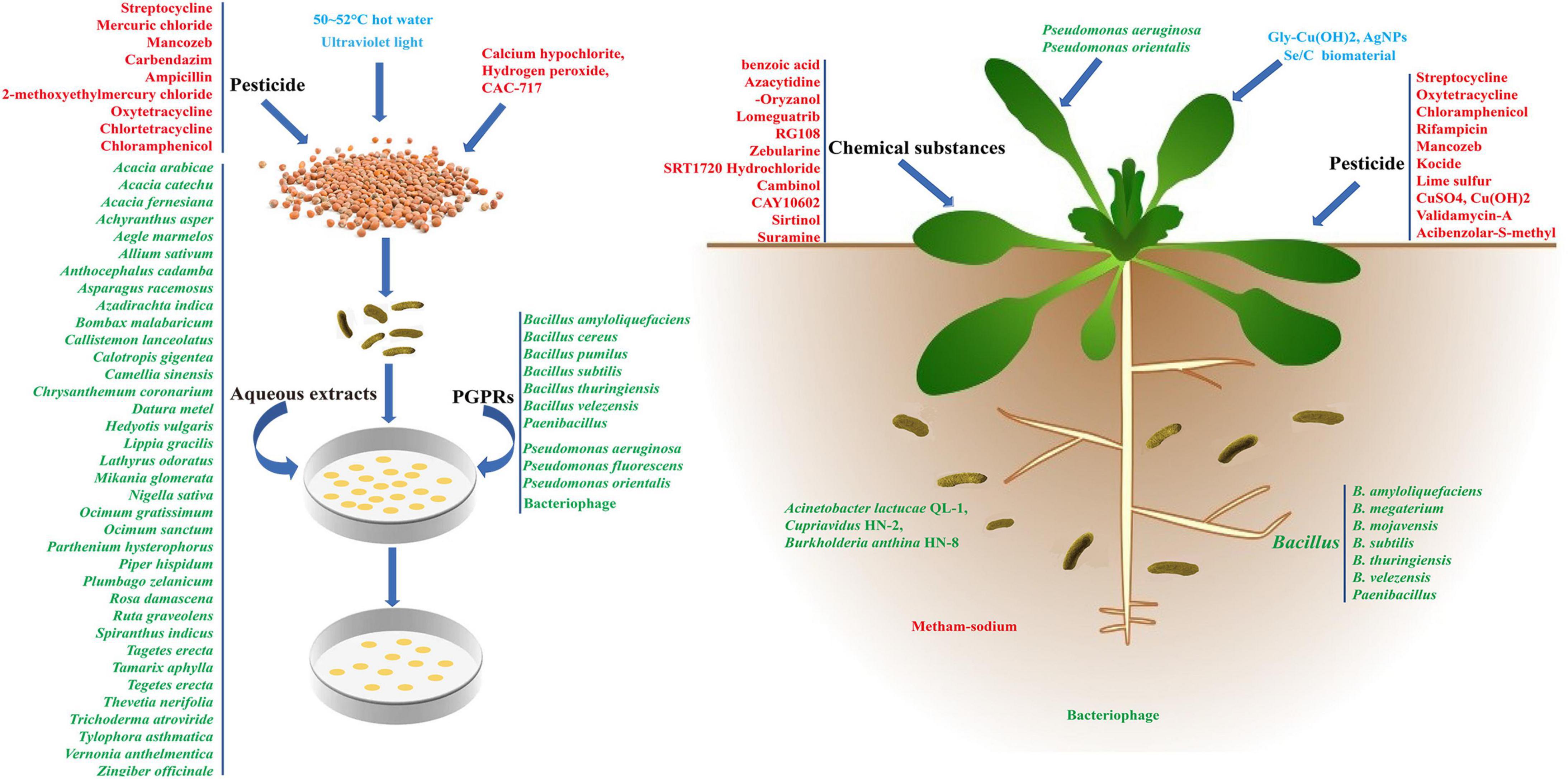
Figure 1. The control process of Xanthomonas campestris pv. campestris (Xcc) by different physical, chemical, biological methods and related agents. The blue, red, and green fonts represent different physical, chemical, and biological Xcc prevention methods, respectively.
As a simple and efficient control method, physical control can effectively inhibit Xcc infection. The maximum temperature usually tolerated by Xcc is 40°C; therefore, Xcc pathogens can be eliminated by soaking the seeds in 50–52°C hot water, or Xcc-contaminated seeds can be sterilized using appropriate doses of ultraviolet light (Shah et al., 1985; Brown et al., 2001; Van Der Wolf and Van Der Zouwen, 2010; Velasco et al., 2013). Xcc can also be prevented by entering the plant via stomata or wounds on plant leaves by forming biofilms on the surface of plant leaves (Velasco et al., 2013; Cao et al., 2020; Pecenka et al., 2021). In addition, sterilizing nanomaterials can also be attached to the leaf surface, resulting in the death of Xcc in the plant via its inability to metabolize energy (Gan et al., 2010; Afrin et al., 2018; Pecenka et al., 2021). Although there are relatively few physical control methods at present, they have the characteristics of simple operation and lack of pollution; therefore, it is believed that more physical methods of BR control, especially nanomaterials, will be developed and used in Brassicaceae vegetable production in the future. In addition, some chemicals can be used for seed soaking or leaf spraying because of their sterilizing activity (Patikarnmonthon et al., 2010; Sakudo et al., 2020). Certain exogenous chemicals have similar functions to hormones, which can improve the resistance of plants to Xcc by enhancing their antioxidant capacity and improving their disease-resistant secondary metabolites; other chemicals can reduce the harm caused by Xcc by affecting its membrane stability (Qian et al., 2006; Baranek et al., 2021).
Compared with physical control and chemical control, there are more studies and reports on biological control, which suggests that researchers are more inclined to use environmentally sound methods for BR control. In the future, the potential biological control of BR will mainly focus on the use of plant extracts and Xcc antagonism. Compared with chemical agents, plant extracts have the advantages of a wide range of sources and less environmental pollution. A large number of crop extracts from Acacia, Tylophora, Ocimum, and other genera have been reported to show a controlling effect on Xcc (Tiwari et al., 2004; Sain, 2007; Bhardwaj and Laura, 2009; Kumar et al., 2018; Fontana et al., 2021; Table 1). Moreover, the antimicrobial activities of extracts from different parts of the same plant are also different (Bhardwaj and Laura, 2009; Bhardwaj and Bhardwaj, 2011; Table 4). The components of these plant extracts are complex; therefore, it is difficult to determine the exact substances or particular components of a class of substances that exert the antibacterial activities of the extracts. If the effective antibacterial substances cannot be determined, the study of its antibacterial mechanism becomes impossible. It is hoped that with developments in HPLC, HPLC-MS, and GC-MS technology, we can better identify the specific Xcc resistant substances and components in these extracts. A study showed that thymol and carvacrol from Lippia gracilis essential oils could control BR (Rafael et al., 2019). Therefore, the effective thymol and carvacrol could be extracted from L. gracilis and used as biopesticides, or could be chemically synthesized and used in Brassicaceae vegetable production. In addition, the glucosinolates and phenolics in Brassicaceae vegetables are closely associated with BR disease resistance, especially the gluconapin and its metabolite gluconapin-isothiocyanate (Aires et al., 2011; Velasco et al., 2013). Therefore, we can reduce the loss caused by BR by planting varieties with relatively high contents of Xcc-resistant glucosinolate and phenolics in production. Meanwhile, the glucosinolate content in Brassicaceae vegetables can be improved by regulating Gluconapin and other BR resistance glucosinolate biosynthesis pathways through genetic engineering, thereby improving resistance to BR.
Among the methods developed to control BR, the use of Xcc-antagonists is the most convenient and environmentally friendly method reported so far. In addition to inhibiting Xcc and improving crop growth index and yield, soil beneficial bacteria such as Bacillus, Pseudomonas, and other PGPRs can also improve soil microbial community structure and increase the abundance of beneficial bacteria in soil (Sain, 2007; Wulff et al., 2010; Liu et al., 2016a; Ha et al., 2017; Li’aini et al., 2017; Holtappels et al., 2022; Tables 2, 3). Crop species and soil structure also affect the control effect of PGPR genera; thus, microbial agents suitable for different crops and soil types can be developed. During planting, growers can apply the best microbial agents according to the soil characteristics of crops and planting environment. Combinations of physical, chemical, and biological control methods could also be used to reduce chemical resistance and adaptability on the premise of improving the effectiveness of BR control.
With the development of biotechnology, chemical synthesis, plant extract separation technology, and other technologies, more BR resistant varieties and efficient and environmentally friendly disease-resistance methods will be developed for use in production to reduce the losses caused by Xcc.
Author contributions
ZCL and JY designed the manuscript. BX, BZ, ZZL, and ZY downloaded and summarized the references. ZCL, HW, and JW completed the manuscript. JL, SL, and SW revised the manuscript. All authors contributed to the article and approved the submitted version.
Funding
This research was funded by the Education Science and Technology Innovation Project of Gansu Province (GSSYLXM-02), the Gansu Top Leading Talent Plan (GSBJLJ-2021-14), and the central government guides local special projects for science and technology development (ZCYD-2021-6).
Conflict of interest
The authors declare that the research was conducted in the absence of any commercial or financial relationships that could be construed as a potential conflict of interest.
Publisher’s note
All claims expressed in this article are solely those of the authors and do not necessarily represent those of their affiliated organizations, or those of the publisher, the editors and the reviewers. Any product that may be evaluated in this article, or claim that may be made by its manufacturer, is not guaranteed or endorsed by the publisher.
References
Adesola, R. O. E., Itodo, A. E., Salami, O., and Abdulazeez, M. D. (2021). Aqueous extracts of Ginger (Zingiber officinale roscoe) and garlic (Allium satium L.) bulbs: Phytochemical screening and in vivo antitrypanosomal effect. Nat. Sci. 37, 135–150.
Afrin, K. S., Rahim, M. A., Jong-In, P., Sathishkumar, N., Hoy-Taek, K., and Ill-Sup, N. (2018). Identification of NBS-encoding genes linked to black rot resistance in cabbage (Brassica oleracea var. capitata). Mol. Biol. Rep. 5, 773–785. doi: 10.1007/s11033-018-4217-5
Aires, A., Dias, C. S. P., Carvalho, R., Oliveira, M. H., Monteiro, A. A., Simoes, M. V., et al. (2011). Correlations between disease severity, glucosinolate profiles and total Phenolics and Xanthomonas campestris pv. campestris inoculation of different Brassicaceae. Sci. Hortic. 129, 503–510. doi: 10.1016/j.scienta.2011.04.009
Amaral, L. S., Debona, D., Costa, L. C., Silva, A. L. R., Oliveira, J. R., and Rodrigues, F. A. (2019). Biochemical insights into basal and induced resistance in cabbage to black rot. J. Phytopathol. 167, 390–403. doi: 10.1111/jph.12808
Babin, A., Lemauf, S., Rebuf, C. P., and Marylène Gatti, J. L. (2022). Effects of Bacillus thuringiensis kurstaki bioinsecticide on two non-target Drosophila larval endoparasitoid wasps. Entomol. General. 42, 611–620. doi: 10.1127/entomologia/2022/1452
Baranek, M., Kováčová, V., Gazdik, F., Spetik, M., Eichmeier, A., Pulawska, J., et al. (2021). Epigenetic modulating chemicals significantly affect the virulence and genetic characteristics of the bacterial plant pathogen Xanthomonas campestris pv. campestris. Genes 12:804. doi: 10.3390/genes12060804
Barzman, M., Bàrberi, P., Birch, A. N. E., Boonekamp, P., Dachbrodt-Saaydeh, S., Graf, B., et al. (2015). Eight principles of integrated pest management. Agron. Sustain. Dev. 35, 1199–1215. doi: 10.1007/s13593-015-0327-9
Bhardwaj, S. K., and Laura, J. S. (2009). Antibacterial activity of some plant-extracts against plant pathogenic bacteria Xanthomonas campestris pv. campestris. Indian J. Agric. Res. 43, 26–31.
Bhardwaj, S. S., and Bhardwaj, SK (2011). Potential Use of some petal extracts against Xanthomonas campestris pv. campestris. Our Nat. 9, 100–106. doi: 10.3126/on.v9i1.5739
Bhat, N. A., and Masoodi, S. D. (2000). Efficacy of various antibiotics against Xanthomonas campestris pv. campestris the casual pathogen of black rot of cabbage. Appl. Biol. Res. 2, 161–163.
Brown, J. E., Lu, T. Y., Stevens, C., Khan, V. A., Lu, J. Y., Wilson, C. L., et al. (2001). The effect of low dose ultraviolet light-C seed treatment on induced resistance in cabbage to black rot (Xanthomonas campestris pv. campestris). Crop Prot. 20, 873–883. doi: 10.1016/S0261-2194(01)00037-0
Cao, H. Y. Y., Chen, X. J., Liu, J., Chen, C., Yuan, S. Z., and Yu, L. (2020). Synthesis of selenium-doped carbon from glucose: An efficient antibacterial material against Xcc. Chin. Chem. Lett. 31, 1887–1889. doi: 10.1016/j.cclet.2020.01.027
Chen, L., Zhang, H., Zhao, S., Xiang, B., and Yao, Z. (2021). Lipopeptide production by Bacillus atrophaeus strain B44 and its biocontrol efficacy against cotton Rhizoctoniosis. Biotechnol. Lett. 43, 1183–1193. doi: 10.1007/s10529-021-03114-0
Cho, Y., Srivastava, A., Ohm, R. A., Lawrence, C. B., Wang, K. H., Grigoriev, I. V., et al. (2012). Transcription factor Amr1 induces melanin biosynthesis and suppresses virulence in Alternaria brassicicola. PLoS Pathog. 8:e1002974. doi: 10.1371/journal.ppat.1002974
Cooksey, D. (1990). Genetics of bactericide resistance in plant pathogenic bacteria. Ann. Rev. Phytopathol. 28, 201–219. doi: 10.1146/annurev.py.28.090190.001221
Cruz, J., Tenreiro, R., and Cruz, L. (2017). Assessment of diversity of Xanthomonas campestris pathovars affecting cruciferous plants in Portugal and disclosure of two novel X. campestris pv. campestris races. J. Plant Pathol. 99, 403–414.
Da Silva, S. R., Roach, D., and Castle, A. (2018). Framing the future with bacteriophages in agriculture. Viruses 10:218. doi: 10.3390/v10050218
Deng, Y., Wu, J., Yin, W., Li, P., Zhou, J., Chen, S., et al. (2016). Diffusible signal factor family signals provide a fitness advantage to Xanthomonas campestris pv. campestris in interspecies competition. Environ. Microbiol. 18, 1534–1545. doi: 10.1111/1462-2920.13244
Dong, H. X., Renci, X., Liang, Y., Tang, G., Yang, J. L., Tang, J. Y., et al. (2020). Development of glycine-copper(ii) hydroxide nanoparticles with improved biosafety for sustainable plant disease management. RSC Adv. 36, 21222–21227. doi: 10.1039/d0ra02050h
Dow, J. M., Crossman, L., Findlay, K., He, Y. Q., Feng, J. X., and Tang, J. L. (2003). Biofilm dispersal in Xanthomonas campestris is controlled by cell–cell signaling and is required for full virulence to plants. Proc. Natl. Acad. Sci. U.S.A. 100, 10995–11000. doi: 10.1073/pnas.1833360100
Duran, N., Marcato, P. D., de Conti, R., Alves, O. L., Costa, F. T. M., and Brocchi, M. (2010). Potential use of silver nanoparticles on pathogenic bacteria, their toxicity and possible mechanisms of action. J. Brazilian Chem. Soc. 21, 949–959. doi: 10.1590/S0103-50532010000600002
Fischetti, V. (2008). Bacteriophage lysins as effective antibacterials. Curr. Opin. Microbiol. 11, 393–400. doi: 10.1016/j.mib.2008.09.012
Fontana, R., Caproni, A., Buzzi, R., Sicurella, M., Buratto, M., Salvatori, F., et al. (2021). Effects of Moringa oleifera leaf extracts on Xanthomonas campestris pv. campestris. Microorganisms 9:2244. doi: 10.3390/microorganisms9112244
Gan, L., Xu, W.-Y., Jiang, M. S., Bing, H. E., and Man, S. U. (2010). A study on the inhibitory activities of Nano-silver to Xanthomonas.campestris pv.campestris. Acta Agric. Univ. Jiangxiensis 32, 493–497.
Ghazalibiglar, H., Hampton, J. G., van Zijll de Jong, E., and Holyoake, A. (2015). Enhanced growth of cabbage seedlings by a Paenibacillus isolate in the presence of Xanthomonas campestris pv. campestris. N Z Plant Prot. 68, 172–178. doi: 10.1080/09583157.2015.1129052
Ghazalibiglar, H., Hampton, J. G., Van, Z., and Holyoake, A. (2016). Evaluation of Paenibacillus spp. isolates for the biological control of black rot in Brassica oleracea var. capitata (cabbage). Biocontrol Sci. Technol. 26, 504–515.
Ha, V. T. N., Dzhalilov, F. S.-U., and Ignatov, A. N. (2017). Biological properties of bacteriophages specific to blackrot pathogen of brassicas Xanthomonas campestris pv. campestris. Известия Тимирязевской сельскохозяйственной академии, 28–36.
Hamkalo, B. (1972). “Chapter 3-The effects of ultraviolet radiations on bacteria,” in Concepts in radiation cell biology, ed. G. L. Whitson (Cambridge, MA: Academic Press), 91–121.
Holtappels, D., Fortuna, K., Lavigne, R., and Wagemans, J. (2021). The future of phage biocontrol in integrated plant protection for sustainable crop production. Curr. Opin. Biotechnol. 68, 60–71. doi: 10.1016/j.copbio.2020.08.016
Holtappels, D., Fortuna, KJ, Moons, L., Broeckaert, N., Backer, L. E., Venneman, S., et al. (2022). The potential of bacteriophages to control Xanthomonas campestris pv. campestris at different stages of disease development. Microb. Biotechnol. 15, 1762–1782. doi: 10.1111/1751-7915.14004
Ishikawa, R., Suzuki Nishimoto, M., Fukuchi, A., and Matsuura, K. (2004). Effective control of cabbage black rot by Validamycin A and its effect on extracellular polysaccharide-production of Xanthomonas campestris pv. campestris. J. Pestic. Sci. 29, 209–213. doi: 10.1584/jpestics.29.209
Islam, M. L., Lee, B. R., Das, P. R., La, V. H., Jung, H. I., and Kim, T. H. (2018). Characterization of p-Coumaric acid-induced soluble and cell wall-bound phenolic metabolites in relation to disease resistance to Xanthomonas campestris pv. campestris in Chinese cabbage. Plant Physiol. Biochem. 125, 172–177. doi: 10.1016/j.plaphy.2018.02.012
Islam, M. L., BokRye, L., La, V. H., Lee, H., Jung, W. J., Bae, D. W., et al. (2019). p-Coumaric acid induces jasmonic acid-mediated phenolic accumulation and resistance to black rot disease in Brassica napus. Physiol. Mol. Plant Pathol. 106, 270–275. doi: 10.1016/j.pmpp.2019.04.001
Issazadeh, K., Rad, S. K., Zarrabi, S., and Rahimibashar, M. R. (2012). Antagonism of Bacillus species against Xanthomonas campestris pv. campestris and Pectobacterium carotovorum subsp. carotovorum. Afr. J. Microbiol. Res. 6, 1615–1620. doi: 10.5897/AJMR12.075
Jelusic, A., Popović, T., Dimkic, I., Mitrovic, P., Peeters, K., Visnjevec, A. M., et al. (2021). Changes in the winter oilseed rape microbiome affected by Xanthomonas campestris pv. campestris and biocontrol potential of the indigenous Bacillus and Pseudomonas isolates. Biol. Control 160:104695. doi: 10.1016/j.biocontrol.2021.104695
Jensen, B. M., Massomo, S. M. S, Swai, I. S., Hockenhull, J., and Andersen, S. B. (2005). Field evaluation for resistance to the black rot pathogen Xanthomonas campestris pv. campestris in cabbage (Brassica oleracea). Eur. J. Plant Pathol. 113, 297–308. doi: 10.1007/s10658-005-2799-y
Jensen, B. D., Vicente, J. G., Manandhar, H. K., and Roberts, S. J. (2010). Occurrence and diversity of Xanthomonas campestris pv. campestris in vegetable Brassica fields in Nepal. Plant Dis. 94, 298–305. doi: 10.1094/PDIS-94-3-0298
Jing, Z., Shaobin, C., Tz, A., Ying, W., Jma, B., Lza, B., et al. (2022). Characterization and antibacterial modes of action of bacteriocins from Bacillus coagulans CGMCC 9951 against Listeria monocytogenes. Food Sci. Technol. 160:113272. doi: 10.1016/j.lwt.2022.113272
Kong, C., Guo, C., Yang, L. M., Zhuang, M., Zhang, Y. Y., Wang, Y., et al. (2021). Germplasm screening and inheritance analysis of resistance to cabbage black rot in a worldwide collection of cabbage (Brassica oleracea var. capitata) resources. Sci. Hortic. 288:110234. doi: 10.1016/j.scienta.2021.110234
Koroleva, S. D., Sa, Polyakova, N. V., and Pistun, O. G. (2021). Evaluation of valuation of inbred lines and hybrid combinations of white cabbage for resistance to black rot (Xanthomonas campestris pv. campestris). Sci. Res. Produc. Mag. 1, 53–58. doi: 10.33775/1684-2464-2021-50-1-53-58
Krauthausen, H. J., Laun, N., and Wohanka, W. (2011). Methods to reduce the spread of the black rot pathogen, Xanthomonas campestris pv. campestris, in brassica transplants. J. Dis. Prot. 118, 7–16. doi: 10.1007/BF03356375
Kritzman, G., and Ben-yephet, Y. (1990). Control by metham-sodium of Xanthomonas campestris pv. campestris and the pathogen’s survival in soil. Phytoparasitica 18, 217–227. doi: 10.1007/BF02980991
Kumar, N. K., Sanath Kumar, V. B., Manjunatha, S. E., Padmaja, A. S., and Pankaja, N. S. (2018). Antimicrobial activity of medicinal plants against Xanthomonas campestris pv. campestris causing black rot of cabbage. Int. J. Commun. Sys. 6, 1871–1874.
Kuznetsov, M., Scherbakov, A., Gorelnikova, E., and Chervyakova, N. (2020). Biochemical identification of patovars of the pathogen of vascular bacteriosis of the cruciferous Xanthomonas campestris pv. campestris. Sci. Life 15, 82–88. doi: 10.35679/1991-9476-2020-15-1-82-88
Lamichhane, J. M., Bischoff-Schaefer, M., Bluemel, S., Dachbrodt-Saaydeh, S., Dreux, L., Jansen, J. P., et al. (2017). Identifying obstacles and ranking common biological control research priorities for Europe to manage most economically important pests in arable, vegetable and perennial crops. Pest Manag. Sci. 73, 14–21. doi: 10.1002/ps.4423
Langston, B. D. Jr., and Cummings, M. P. (2003). Evaluation of bactericides and plant defense activators for control of black rot of cabbage. Plant Pathol. 1, 2594–2595.
Lenka, S., and Ram, S. (1997). A note on the efficacy in vivo of various antibiotics and fungicide chemicals against Xanthomonas campestris pv. campestris causing black rot of cauliflower. Orissa J. Hortic. 25, 90–92.
Li’aini, A. S., Lin, Y.-H., Huang, T.-C., and Sulistyowati, L. (2017). Application of Bacillus amyloliquefaciens to control black rot disease on cabbage caused by Xanthomonas campestris pv. campestris. Phytomedicine 59, 39–44.
Li, G., Lv, H., Zhang, S., Zhang, S., Li, F., Zhang, H., et al. (2019). TuMV management for Brassica crops through host resistance: Retrospect and prospects. Plant Pathol. 68, 1035–1044. doi: 10.1111/ppa.13016
Liu, K., Garrett, C., Fadamiro, H., and Kloepper, J. W. (2016a). Antagonism of black rot in cabbage by mixtures of plant growth-promoting rhizobacteria (PGPR). Biocontrol 61, 605–613. doi: 10.1007/s10526-016-9742-3
Liu, K., Carol, C., Kloepper, J. W., and McInroy, J. A. (2016b). Induction of systemic resistance in Chinese cabbage against black rot by plant growth-promoting rhizobacteria. Biol. Control 105, 8–13. doi: 10.1016/j.biocontrol.2016.04.007
Luna, C l, Mariano, R. L. R., and Souto-Maior, A. M. (2002). Production of a biocontrol agent for crucifers black rot disease. Brazilian J. Chem. Eng. 19, 133–140. doi: 10.1590/S0104-66322002000200007
Macha, H., Marešová, H., Jurikova, T., Svecova, M., Benada, O., Skriba, A., et al. (2021). Killing effect of Bacillus velezensis FZB42 on a Xanthomonas campestris pv. campestris (Xcc) strain newly isolated from cabbage Brassica oleracea convar. capitata (L.): A metabolomic study. Microorganisms 9:1410. doi: 10.3390/microorganisms9071410
Mallmann, W. L., and Hemstreet, C. (1924). Isolation of an inhibitory substance from plants. J. Agric. Res. 28, 599–602. doi: 10.1017/S036839310013336X
Marroni, I., and Germani, J. (2014). New technique to create a suspension containing bacteriophages and how it can be used to control cabbage leaf spot caused by Xanthomonas campestris pv. campestris. Agric. Sci. 5, 286–297. doi: 10.4236/as.2014.54031
Massomo, S. M. S., Mortensen, C. N., Mabagala, R. B., Newman, M. A., and Hockenhull, J. (2010). Biological control of black rot (Xanthomonas campestris pv. campestris) of cabbage in Tanzania with Bacillus strains. J. Phytopathol. 152, 98–105. doi: 10.1111/j.1439-0434.2003.00808.x
Mishra, S., and Arora, N. K. (2012). Evaluation of rhizospheric Pseudomonas and Bacillus as biocontrol tool for Xanthomonas campestris pv campestris. World J. Microbiol. Biotechnol. 28, 693–702. doi: 10.1007/s11274-011-0865-5
Mouna, M., Bourouba, K., Assia, B., and Lebbal, S. (2021). Insecticidal effect of two aqueous extracts from the leaves of Salvia officinalis and Eucalyptus camaldulensis against Aphis fabae. J. Entomol. Res. 45, 268–279. doi: 10.5958/0974-4576.2021.00043.8
Muthukumar, A., Suthin Raj, T., Prabhukarthikeyan, S. R., Naveen, K., and Keerthana, U. (2022). “Pseudomonas and Bacillus: A biological tool for crop protection,” in New future developments in microbial biotechnology bioengineering, 145–148. doi: 10.1016/B978-0-323-85577-8.00006-8
Nagai, H., Miyake, N., Kato, S., Maekawa, D., Inoue, Y., and Takikawa, Y. (2017). Improved control of black rot of broccoli caused by Xanthomonas campestris pv. campestris using a bacteriophage and a nonpathogenic Xanthomonas sp. strain. J. Gen. Plant Pathol. 83, 373–381. doi: 10.1007/s10327-017-0745-4
Nunez, A. R., Rodríguez, G. A. A., Monteiro, F. P., Faria, A. F., Silva, J. C. P., Monteiro, A. C. A., et al. (2018). Bio-based products control black rot (Xanthomonas campestris pv. campestris) and increase the nutraceutical and antioxidant components in kale. Sci. Rep. 8:10199. doi: 10.1038/s41598-018-28086-6
Patikarnmonthon, N., Nawapan, S., Buranajitpakorn, S., Charoenlap, N., Mongkolsuk, S., and Vattanaviboon, P. (2010). Copper ions potentiate organic hydroperoxide and hydrogen peroxide toxicity through different mechanisms in Xanthomonas campestris pv. campestris. FEMS Microbiol. Lett. 313, 75–80. doi: 10.1111/j.1574-6968.2010.02124.x
Pang, W., Liang, Y., Zhan, Z. X., Li, X. N., and Piao, Z. Y. (2020). Development of a sinitic clubroot differential set for the pathotype classification of Plasmodiophora brassicae. Front. Plant Sci. 11:568771. doi: 10.3389/fpls.2020.568771
Papaianni, M., Ricciardelli, A., Fulgione, A., d’Errico, G., Zoina, A., Lorito, M., et al. (2020). Antibiofilm activity of a Trichoderma metabolite against Xanthomonas campestris pv. campestris, alone and in association with a phage. Microorganisms 8:620. doi: 10.3390/microorganisms8050620
Pecenka, J., Bytešníková, Z., Kiss, T., Penazova, E., Baranek, M., Eichmeier, A., et al. (2021). Silver nanoparticles eliminate Xanthomonas campestris pv. campestris in cabbage seeds more efficiently than hot water treatment. Mater. Today Commun. 27:102284. doi: 10.1016/j.mtcomm.2021.102284
Qian, F., An, L., He, X., Han, Q., and Li, X. (2006). Antibacterial activity of Xantho-oligosaccharide cleaved from xanthan against phytopathogenic Xanthomonas campestris pv. campestris. Proc. Biochem. 41, 1582–1588. doi: 10.1016/j.procbio.2006.03.003
Qian, W. J., Jia, Y., Ren, S. X., He, Y. Q., Feng, J. X., Lu, F., et al. (2005). Comparative and functional genomic analyses of the pathogenicity of phytopathogen Xanthomonas campestris pv. campestris. Genome Res. 15, 757–767. doi: 10.1101/gr.3378705
Rafael, S. M., de Oliveira, M. G. O., Juliana, O. M., Arie, F. B., Cristiane, B. C., Ricardo, S., et al. (2019). Antimicrobial activity of Lippia gracilis essential oils on the plant pathogen Xanthomonas campestris pv. campestris and their effect on membrane integrity. Pestic. Biochem. Physiol. 160, 40–48. doi: 10.1016/j.pestbp.2019.06.014
Ryan, R., Vorhölter, F. J., Potnis, N., Jones, J. B., Van Sluys, M. A., Bogdanove, A. J., et al. (2011). Pathogenomics of Xanthomonas: Understanding bacterium–plant interactions. Nat. Rev. Microbiol. 9, 344–355. doi: 10.1038/nrmicro2558
Sain, S. (2007). Evaluation of botanicals and PGPRs against Xanthomonas campestris pv. campestris, an incitant of black rot of cauliflower. J. Eco Friendly Agric. 2, 178–182.
Sakudo, A., Furusaki, K., Onishi, R., and Onodera, T. (2020). Electrically charged disinfectant containing calcium hydrogen carbonate mesoscopic crystals as a potential measure to control Xanthomonas campestris pv. campestris on cabbage seeds. Microorganisms 8:1066. doi: 10.3390/microorganisms8101606
Sánchez Pérez, Y., Correa Vidal, T. M., Machado, Y. A., Cotilla Pelier, L., Berroa Navarro, G., and Pino Pérez, O. (2014). Composición química del aceite esencial de Piper hispidum Sw. y actividad antimicrobiana sobre Xanthomonas albilineans (Ashby) Dowsony Xanthomonas campestris pv. campestris (Pammel) Dowson. Revista de Protección Vegetal 29, 185–191.
Sanna, M., Gilardi, G., Gullino, M. L., and Mezzalama, M. (2022). Evaluation of physical and chemical disinfection methods of Brassica oleracea seeds naturally contaminated with Xanthomonas campestris pv. campestris. J. Plant Dis. Prot. 5, 1145–1152. doi: 10.1007/s41348-022-00635-2
Schaad, N. W., and Ignatov, A. N. (2019). “Role of cruciferous weeds in the epidemiology and biological control of seed borne Xanthomonas campestris,” in Proceedings of the 4th international symposium on biological control of bacterial plant diseases biocontrol.
Schultz, T., Gabrielson, R. L., and Olson, S. (1986). Control of Xanthomonas campestris pv. campestris in crucifer seed with slurry treatments of calcium hypochlorite. Plant Dis. 70, 1027–1030. doi: 10.1094/PD-70-1027
Shah, A., Srivastava, K. K., Roy, A. J., and Bora, S. S. (1985). Control of black rot disease of cauliflower by seed treatment. Prog. Hortic. 17, 72–74.
Sharma, S. (1981). Control of black rot of cauliflower by hot water seed treatment and field sprays with streptocycline. Indian J. Mycol. Plant Pathol. 11, 17–20.
Silva, R. M., Bl, Santos, D. R., Vasconcelo-Rodrigues, I. S., Talamini, V., Fernandes, M. F., et al. (2018). Using antagonistic soil bacteria and their cell-free filtrates to control the black rot pathogen Xanthomonas campestris pv. campestris. J. Phytopathol. 166, 494–501. doi: 10.1111/jph.12709
Singh, D., Rathaur, P. S., and Vicente, J. G. (2016). Characterization, genetic diversity and distribution of Xanthomonas campestris pv. campestris races causing black rot disease in cruciferous crops of India. Plant Pathol. 65, 1411–1418. doi: 10.1111/ppa.12508
Tiwari, R. C., Chandravanshi, S, and Ojha, B. M. (2004). Evaluation of some medicinal plant species for their antibacterial activity against Xanthomonas campestris pv. campestris, the black rot pathogen of cabbage. Indian Phytopath. 57, 308–311.
Umesha, S., and Roohie, R. K. (2017). Role of Pseudomonas fluorescens and INA against black rot of cabbage. J. Phytopath. 165, 265–275. doi: 10.1111/jph.12558
Velasco, P., Lema, M., Francisco, M., Soengas, P., and Cartea, M. E. (2013). In vivo and in vitro effects of secondary metabolites against Xanthomonas campestris pv. campestris. Molecules 18, 11131–11143. doi: 10.3390/molecules180911131
Vicente, J., Conway, J., Roberts, S. J., and Taylor, J. D. (2001). Identification and origin of Xanthomonas campestris pv. campestris races and related pathovars. Phytopathol. 91, 492–499. doi: 10.1094/PHYTO.2001.91.5.492
Vicente, J. G., and Holub, B. (2013). Xanthomonas campestris pv. campestris (cause of black rot of crucifers) in the genomic era is still a worldwide threat to brassica crops. Mol. Plant Pathol. 14, 2–18. doi: 10.1111/j.1364-3703.2012.00833.x
Vigoschultz, S., Stangarlin, J. R., Franzener, G., Portz, R. L., Kuhn, O. J., and Schwan-Estrada, K. R. F. (2006). Avaliacao da eficacia da tintura etanolica de guaco (Mikania glomerata) no controle da podridao negra (Xanthomonas campestris pv. campestris) em couve-flor. Anais Da Escola Superior De Agricultura Luiz De Queiroz 27, 515–524. doi: 10.5433/1679-0359.2006v27n4p515
Van Der Wolf, J. M., and Van Der Zouwen, P. S. (2010). Colonization of cauliflower blossom (Brassica oleracea) by Xanthomonas campestris pv. campestris, via fies (Calliphora vomitoria) can result in seed infestation. J. Phytopathol. 158, 726–732. doi: 10.1111/j.1439-0434.2010.01690.x
Wulff, E. G., Mguni, C. M., Mansfeld-Giese, K., Fels, J., Lübeck, M., and Hockenhull, J. (2010). Biochemical and molecular characterization of Bacillus amyloliquefaciens, B. subtilis and B. pumilus isolates with distinct antagonistic potential against Xanthomonas campestris pv. campestris. Plant Pathol. 51, 574–584. doi: 10.1046/j.1365-3059.2002.00753.x
Wulff, E. G., Mguni, C. M., Mortensen, C. N., Keswani, C. L., and Hockenhull, J. (2002). Biological control of black rot (Xanthomonas campestris pv. campestris) of brassicas with an antagonistic strain of Bacillus subtilis in Zimbabwe. Eur. J. Plant Pathol. 108, 317–325. doi: 10.1023/A:1015671031906
Ye, T., Zhang, W., Feng, Z., Fan, X., Xu, X., Mishra, S., et al. (2020). Characterization of a novel quorum-quenching bacterial strain, Burkholderia anthina HN-8, and its biocontrol potential against black rot disease caused by Xanthomonas campestris pv. Campestris 8:1485. doi: 10.3390/microorganisms8101485
Ye, T., Zhou, T., Fan, X. H., Bhatt, P., Zhang, L. H., and Chen, S. H. (2019a). Acinetobacter lactucae strain QL-1, a novel quorum quenching candidate against bacterial pathogen Xanthomonas campestris pv. campestris. Front. Microbiol. 10:2867. doi: 10.3389/fmicb.2019.02867
Keywords: cruciferous, black rot, Xanthomonas campestris pv. campestris, antibacterial activity, disease resistance
Citation: Liu Z, Wang H, Wang J, Lv J, Xie B, Luo S, Wang S, Zhang B, Li Z, Yue Z and Yu J (2022) Physical, chemical, and biological control of black rot of brassicaceae vegetables: A review. Front. Microbiol. 13:1023826. doi: 10.3389/fmicb.2022.1023826
Received: 20 August 2022; Accepted: 01 November 2022;
Published: 23 November 2022.
Edited by:
Md. Motaher Hossain, Bangabandhu Sheikh Mujibur Rahman Agricultural University, BangladeshReviewed by:
Md Tabibul Islam, Virginia Tech, United StatesJohn Graham Hampton, Lincoln University, New Zealand
Copyright © 2022 Liu, Wang, Wang, Lv, Xie, Luo, Wang, Zhang, Li, Yue and Yu. This is an open-access article distributed under the terms of the Creative Commons Attribution License (CC BY). The use, distribution or reproduction in other forums is permitted, provided the original author(s) and the copyright owner(s) are credited and that the original publication in this journal is cited, in accordance with accepted academic practice. No use, distribution or reproduction is permitted which does not comply with these terms.
*Correspondence: Jihua Yu, eXVqaWh1YWdnQDE2My5jb20=