- 1Department of Microbiology and Parasitology, Faculty of Medical Science, Naresuan University, Phitsanulok, Thailand
- 2Department of Microbiology and Immunology, Faculty of Tropical Medicine, Mahidol University, Bangkok, Thailand
- 3Centre of Excellence in Medical Biotechnology, Faculty of Medical Science, Naresuan University, Phitsanulok, Thailand
- 4Center of Excellence for Biodiversity, Faculty of Sciences, Naresuan University, Phitsanulok, Thailand
- 5Merck-Stiftungsprofessur für Molekulare Biotechnologie, Fachbereich Biowissenschaften, Goethe Universität Frankfurt, Frankfurt am Main, Germany
- 6Buchmann Institute for Molecular Life Sciences, Goethe University Frankfurt, Frankfurt am Main, Germany
Photorhabdus and Xenorhabdus are symbiotic with entomopathogenic nematodes (EPNs) of the genera Heterorhabditis and Steinernema, respectively. These bacteria produce several secondary metabolites including antimicrobial compounds. The objectives of this study were to isolate and identify EPNs and their symbiotic bacteria from Mae Wong National Park, Thailand and to evaluate the antibacterial activities of symbiont extracts against drug resistant bacteria. A total of 550 soil samples from 110 sites were collected between August 2014 and July 2015. A total of EPN isolates were obtained through baiting and White trap methods, which yielded 21 Heterorhabditis and 3 Steinernema isolates. Based on molecular identification and phylogenetic analysis, the most common species found in the present study was P. luminescens subsp. akhurstii associated with H. indica. Notably, two species of EPNs, H. zealandica and S. kushidai, and two species of symbiotic bacteria, X. japonica and P. temperata subsp. temperata represented new recorded organisms in Thailand. Furthermore, the association between P. temperata subsp. temperata and H. zealandica has not previously been reported worldwide. Disk diffusion, minimal inhibitory concentration, and minimal bactericidal concentration analyses demonstrated that the crude compound extracted by ethyl acetate from P. temperata subsp. temperata could inhibit the growth of up to 10 strains of drug resistant bacteria. Based on HPLC-MS analysis, compound classes in bacterial extracts were identified as GameXPeptide, xenoamicin, xenocoumacin, mevalagmapeptide phurealipids derivatives, and isopropylstilbene. Together, the results of this study provide evidence for the diversity of EPNs and their symbiotic bacteria in Mae Wong National Park, Thailand and demonstrate their novel associations. These findings also provide an important foundation for further research regarding the antimicrobial activity of Photorhabdus bacteria.
Introduction
Photorhabdus and Xenorhabdus are symbiotic with entomopathogenic nematodes (EPNs) of the genera Heterorhabditis and Steinernema, respectively (Hominick, 2002). The infective juvenile stages (IJs) of EPNs are distributed within natural and agricultural soils worldwide and are successfully utilized for the biological control of insect pests (Hominick, 2002). Photorhabdus and Xenorhabdus belong to Family Enterobacteriaceae, which are Gram negative, rod shaped, and motile via peritrichous flagella. These bacteria are carried in the intestines of IJs that invade into the hemocoel of an insect host through the mouth, spiracles, and anus whereupon they are released from the IJ intestines into the hemolymph (Wang and Gaugler, 1998). The complex between bacterium and nematode destroys the immune response of the larval insect host and leads to its death it within 24–48 h. Consequently, the body of insect cadaver exhibits a red, beige, or black color and slow putrefaction. The EPNs develop through one to three generations in the host cadaver by feeding on the bacteria and dead insect tissue. Upon depletion of the food resources, the IJs emerge from the host cadaver to search for a new host (Wang and Gaugler, 1998).
Notably, Photorhabdus and Xenorhabdus can produce several secondary metabolites including insecticidal and antimicrobial compounds such as benzylideneacetone, phenethylamines, indole, xenocoumacins and 3,5-dihydroxy-4-isopropylstilbene (McInerney et al., 1991; Eleftherianos et al., 2007). Several studies on the bioactive compounds of Xenorhabdus and Photorhabdus spp. against various microorganisms have demonstrated their antibacterial (Akhurst, 1982), antimicrobial (Genhui, 1996), and antiparasitic activities (Grundmann et al., 2014).
Photorhabdus and Xenorhabdus have been reported from all continents of the world except Antarctica, with approximately 30 species (Hominick, 2002; Tailliez et al., 2010; Ferreira et al., 2013a,b, 2014). In turn, worldwide descriptions of EPNs include approximately 26 species of Heterorhabditis and 100 species of Steinernema (Adams et al., 2006; Li et al., 2012; Thanwisai et al., 2012; Cimen et al., 2014, 2015; Malan et al., 2014; Nthenga et al., 2014; Phan et al., 2014; Vitta et al., 2015). In Thailand, at least two species of Xenorhabdus including X. stockiae and X. miraniensis have been described (Tailliez et al., 2010; Thanwisai et al., 2012) as well as two species with five subspecies of Photorhabdus; i.e., P. luminescens subsp. akhurstii, P. luminescens subsp. hainanensis, P. luminescens subsp. laumondii, P. asymbiotica subsp. australis, and P. luminescens subsp. namnaonensis from across the country (Maneesakorn et al., 2011; Thanwisai et al., 2012; Glaeser et al., 2017). In addition, nine species of EPNs including S. siamkayai, S. minutum, S. websteri, S. khoisanae, S. scarabaei, H. indica, H. bacteriophora, H. baujardi, and H. gerrardi have been reported across the country (Stock, 1998; Maneesakorn et al., 2010, 2011; Thanwisai et al., 2012; Vitta et al., 2015, 2017). Distributions of EPNs and their bacterial symbionts can be influenced by climate and other ecological communities. The majority of soil locations from Thailand that EPNs have previously been isolated comprise roadside verge, fruit crops, rice fields, and the banks of rivers and ponds (Tangchitsomkid and Sontirat, 1998; Thanwisai et al., 2012; Vitta et al., 2015); however, there are no reports regarding the survey of EPNs and their symbiotic bacteria in the National Park of Thailand.
The main objective of our study was to isolate and identify EPNs and their symbiotic bacteria, Xenorhabdus and Photorhabdus, from Mae Wong National Park, Kamphaeng Phet Province, Thailand. We also evaluated the antibacterial activities of identified Xenorhabdus and Photorhabdus extracts against drug resistant bacteria using the disk diffusion method as well as minimal inhibitory concentration (MIC) and minimal bactericidal concentration (MBC) analyses.
Materials and Methods
Soil Sampling
The soil collection procedures in Mae Wong Nation Park were approved and permitted by the Department of National Parks, Wildlife and Plant Conservation, Thailand (Permission number 0907.4/7432). A total of 550 soil samples were randomly collected from 110 different sites in Mae Wong National Park, Kamphaeng Phet Province, Thailand. Soil samples were collected between August 2014 and July 2015. The process for soil collection followed Thanwisai et al. (2012). Site locations, soil temperatures, pH, and moisture were recorded. The samples were transported to the Department of Microbiology and Parasitology, Faculty of Medical Science, Naresuan University, Phitsanulok Province, Thailand for isolation of EPNs.
Isolation and Identification of EPNs
Entomopathogenic nematodes were obtained from soil samples using the baiting technique with Galleria mellonella larvae (Bedding and Akhurst, 1975). After 5 days as bait, the dead G. mellonella were removed from the soil and were subsequently placed on White traps (White, 1927), in which the emerging IJs were then collected and stored at -20°C for further DNA extraction. The IJs (200–300 nematodes) from each isolate were used for DNA extraction as described (Thanwisai et al., 2012). To identify species of EPNs, polymerase chain reaction (PCR) was performed using the primers TW81_F (5′-GTT TCC GTA GGT GAA CCT GC-3′) and AB28_R (5′-ATA TGC TTA AGT TCA GCG GGT-3′) to amplify a region of the internal transcribed spacer locus (ITS) for Heterorhabditis as well as 539_F (5′- GGA TTT CCT TAG TAA CTG CGA GTG-3′) and 535_R (5′-TAG TCT TTC GCC CCT ATA CCC TT-3′) to amplify a region of 28S rDNA for Steinernema (Stock et al., 2001). The PCR components (30 μl) consisted of 7.5 μl DNA extracted solution, 0.6 μl dNTPs (200 μM), 1.2 μl each primer (5 μM), 4.2 μl MgCl2 (25 mM), 3 μl 10X buffer, 0.3 μl 5 U/ml Tag polymerase, and 12 μl distilled water. The cycling conditions for ITS were as follows: 1 cycle of 95°C for 5 min followed by 35 cycles of 94°C for 1 min, 50°C for 30 s, 72°C for 1 min, and a final extension at 72°C for 7 min. The cycling conditions for 28S rDNA were as follows: 1 cycle of 95°C for 5 min followed by 35 cycles of 94°C for 1 min, 55°C for 30 s, 72°C for 45 s, and a final extension at 72°C for 7 min. Both reactions were performed in the Applied Biosystems thermal cycler (Applied BiosystemsTM VeritiTM thermal cycler, Pittsburgh, PA, United States). PCR products were checked by electrophoresis over 30 min, 100 V on a 1.2% TBE-buffered agarose gel stained with ethidium bromide. PCR products were purified using a NucleoSpin Gel and PCR Clean-up column (Macerey-Nagel Ltd., Düren, Germany) as recommended by the manufacturer. The purified PCR products were sequenced at Macrogen Inc. Service (Seoul, Korea).
Isolation and Identification of Photorhabdus and Xenorhabdus
Photorhabdus and Xenorhabdus were isolated from the hemolymph of the dead G. mellonella infected with EPNs. Hemolymph was streaked on nutrient bromothymol blue triphenyltetrazolium chloride agar (NBTA) and incubated at room temperature in the dark for 4 days. Green and blue colonies were sub-cultured for further genomic DNA extractions. A single colony was then transferred to a 15 ml centrifuge tube containing 3 ml Luria-Bertani (LB) broth and shaken at 180 rpm for 18–24 h. Genomic DNA of each bacterial isolate was extracted from 3 ml culture using a genomic DNA mini kit (Blood/culture cell; Geneaid Biotech Ltd., New Taipei, Taiwan) as recommend by the manufacturer.
To identify Xenorhabdus and Photorhabdus, the Recombinase A gene (recA) was amplified using primers recA_F (5′-GCT ATT GAT GAA AAT AAA CA-3′) and recA_R (5′-RAT TTT RTC WCC RTT RTA GCT-3′) (Tailliez et al., 2010). The PCR reagents was mixed in a final volume of 30 μl, which consisted of 3 μl DNA extract, 0.6 μl dNTPs (200 μM), 1.2 μl each primer (5 μM), 4.2 μl MgCl2 (25 mM), 3 μl 10X reaction buffer, and 0.3 μl 5 U/ml Taq polymerase plus 12 μl distilled water. The cycling conditions for Xenorhabdus were as follows: 1 cycle of 94°C for 5 min followed by 30 cycles of 94°C for 1 min, 50°C for 1 min, 72°C for 2 min, and a final extension at 72°C for 7 min. The cycling conditions for Photorhabdus were as follows: 1 cycle of 94°C for 5 min followed by 30 cycles of 94°C for 1 min, 50°C for 45 s, 72°C for 1.30 min, and a final extension at 72°C for 7 min. PCR product analysis, purification and sequencing were performed as for EPN characterization.
Phylogenetic Analysis
Sequence assembly and editing were performed using SeqmanII DNASTAR Inc., (Madison, WI, United States). Sequences were aligned using Clustal W (Thompson et al., 1994) and compared with sequences from other known species in GenBank using the BLASTN algorithm. Phylogenetic analyses of sequence data were performed using MEGA 6.0 (Tamura et al., 2013). A bootstrap consensus tree was inferred from 1000 replicates.
Preparation of Drug Resistant Bacteria
We used 12 strains of drug-resistant bacteria including Acinetobacter baumannii (three clinical strains), Escherichia coli (one clinical strain), E. coli ATCC® 35218 (one strain), Klebsiella pneumoniae ATCC® 700603 (one strain), Enterococcus faecalis ATCC® 51299 (one strain), Pseudomonas aeruginosa (one clinical strain), P. aeruginosa ATCC® 27853 (one strain), Staphylococcus aureus (two clinical isolates), and S. aureus ATCC® 20475 (one strain) for screening and assessment using the disk diffusion method. The clinical isolates of A. baumannii, E. coli, P. aeruginosa, and S. aureus were obtained from patients with positive samples processed by the diagnostic microbiology laboratory at a hospital in Thailand between February 2011 and December 2014 (Supplementary Table S1). Speciation of bacteria were determined using Standard Operating Procedures supplied by the Department of Medical Science, Ministry of Public Heath, Thailand and Clinical and Laboratory Standards Institute (CLSI) guidelines (M100-S24 and M100-S25), respectively. Each bacterial strain was streaked on Mueller Hinton agar (MHA) and incubated at 37°C for 24 h. A single colony was picked, dissolved in phosphate buffered saline solution, and the concentration adjusted to 0.08–0.13 (0.5 McFarland standard) (Seier-Petersen et al., 2014) according to spectrophotometric measurement at a wavelength of 600 nm.
Preparation of Whole Cell Suspension and Cell-Free Supernatant
We utilized 24 isolates of symbiotic bacteria including 20 isolates of P. luminescens subsp. akhurstii, 2 isolates of X. stockiae, 1 isolate of X. Japonica, and 1 isolate of P. temperata subsp. temperata for screening. A bacterial stock of each isolate was streaked on NBTA medium and incubated at room temperature in the dark for 4 days. A single colony was transferred and grown in 25 ml LB broth and shaken at 180 rpm for 24 h. The concentration of the whole cell suspension was adjusted to 107–108 CFU/ml using phosphate buffered saline solution. To prepare cell-free supernatant, 1 ml whole cell suspension was centrifuged at 12,074 g (Centurion, United States) for 5 min. The supernatant was then filtered using a 0.22-μm filter. The flow-through was used as the cell-free supernatant (Bussaman et al., 2012).
Screening of Photorhabdus and Xenorhabdus Isolates
To screen Xenorhabdus (3 isolates) and Photorhabdus (21 isolates) against drug resistant bacteria, 20 μl whole cell suspension and of cell-free supernatant were dropped onto a sterile 6-mm paper disk. The disk was then allowed to dry for 3–5 min and transferred onto the surface of MHA plates that had been plated with 12 strains of drug resistant bacteria. Antibiotic disks were used as positive controls. The MHA plates were incubated at 37°C for 24 h. The diameter of the inhibition zone was measured in millimeter units.
Bacterial Extracts
Xenorhabdus (two isolates) and Photorhabdus (nine isolates) that could inhibit the growth of at least one strain of drug resistant bacteria during screening were then subcultured on NBTA medium for further extraction of crude compounds. The plates were incubated at room temperature in the dark for 4 days. A single colony of each isolate was transferred and grown by shaking in a 1000-ml flask containing 500 ml LB at 180 rpm for 72 h. To extract the crude bioactive compound, 1000 ml ethyl acetate was added to the culture and mixed well. The flask was then allowed to stand at room temperature for 24 h. The extraction from each isolate was performed three times to maximize the level of crude compound. All bacterial extracts were concentrated using a rotary vacuum evaporator (Buchi, Flawil, Switzerland). The condensed extracts of all bacterial isolates were weighted and stored at -20°C until used.
Disk Diffusion Method
Bacterial extracts from each isolate were dissolved in 1 ml dimethyl sulfoxide (DMSO) Then, 10 μl solution was dropped onto a sterile 6-mm disk, which was placed on the MHA plated with drug resistant bacteria. The plates were then incubated at 37°C for 24 h. Antibiotic disks and DMSO were used as a positive and negative control, respectively. The diameter in millimeter of the clear zone was measured using a ruler.
Minimal Inhibitory Concentrations (MIC)
The MIC of nine bacterial extracts was determined using the broth twofold serial dilution method in 96-well micro titer plates. Initial concentrations of all P. luminescens and P. temperata extracts were dissolved in DMSO to generate 500 and 220 mg/ml solutions, respectively. Then, 100 μl of each extract was mixed with 100 μl MH broth in a well of the micro titer plates followed by twofold serial dilution. To each well, 100 μl bacterial suspension (1 × 108 cell/ml) was added and mixed well. Plates were incubated at 37°C for 24 h. Turbidity of each well was observed visually. The clear wells in the micro titer plates with the lowest concentration from each extract were considered as representing the MIC.
Minimal Bactericidal Concentrations (MBC)
To determine the minimum bactericidal concentration (MBC), 10 μl of the bacterial suspension from micro titer plates from the MIC experiment was dropped onto MHA in triplicate to observe viability following aerobic incubation at 37°C for 24 h. The MBC was read by determining the lowest concentration of the bacterial extracts that reduced the viability of the initial bacterial inoculum by ≥99.9%.
HPLC-MS Analysis of Bacterial Extracts
For the analysis of natural products present in the ethyl acetate extract, the extracts were evaporated to dryness and dissolved in a 1/10 culture volume of methanol. HPLC-MS analysis was performed using a Dionex Ultimate 3000 system coupled to a Bruker AmaZon X mass spectrometer and an Acquity UPLC BEH C18 1.7 μm RP column (Waters) with an acetonitrile (0.1% formic acid) in H2O (0.1% formic acid) gradient ranging from 5 to 95% over 16 min at a flow rate of 0.4 ml/min at 40°C. Chromatograms were analyzed using Bruker Compass Data Analysis 4.3.
Results
Isolation of EPNs
A total of 550 soil samples collected from 110 locations in Mae Wong National Park, Kamphaeng Phet Province, Thailand yielded 24 EPNs (4.36%) belonging to Heterorhabditis and Steinernema consisting of 21 and 3 isolates, respectively (Figure 1). Most EPNs were isolated from loam with a soil temperature range of 14–31°C (mean 22.8°C), soil pH range of 6.0–7.0 (mean 6.7), soil moisture range of 1.0–7.5 (mean 2.0), and an above mean sea level range of 224–1275 m (mean 359.7 m).
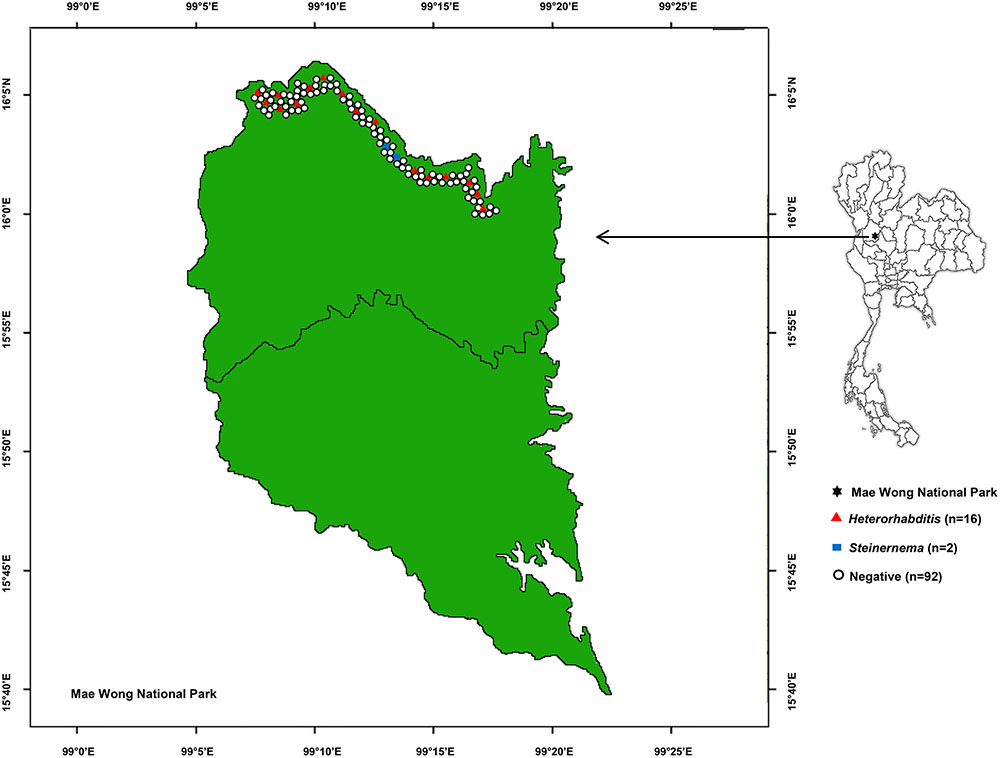
FIGURE 1. Map of 110 soil sampling sites in Mae Wong National Park, Kamphaeng Phet Province, Thailand with positive or negative yields of Heterorhabditis and Steinernema.
Identification and Phylogeny of EPNs
In total, 16 EPN isolates were identified by BLASTN analysis of sequence obtained from a region of the 28S rRNA gene for Steinernema and from the ITS locus for Heterorhabditis. Genomic DNA could not be obtained from the remaining eight isolates owing to contamination by protozoa and fungi.We identified 13 Heterorhabditis isolates as H. indica (seven isolates with 99% identity), H. baujardi (Five isolates with 99% identity), and H. zealandica (one isolate with 100% identity). Phylogenetic tree analysis of Heterorhabditis isolates (Accession No. KY471364–KY471376) obtained in the present study (13 isolates) and Heterorhabditis (20 identified species downloaded from the NCBI database) revealed 3 main groups: group 1 consisted of an isolate of H. indica (KF247222.1) and 7 isolates of Heterorhabditis from the present study; group 2 contained H. baujardi (AF548768.1) and 5 isolates of Heterorhabditis; and group 3 consisted of one isolate of H. zealandica (EF530041.1) and one isolate of Heterorhabditis from our study (Figure 2). In addition, the 3 isolates of Steinernema (Accession No. KY454617–KY454619) were identified as S. websteri (2 isolates, 99% identity) and S. kushidai (1 isolate, 99% identity). The former were closely related to S. websteri (AY841762.1) and the latter was most closely related to S. kushidai (AF331897.1) (Figure 3).
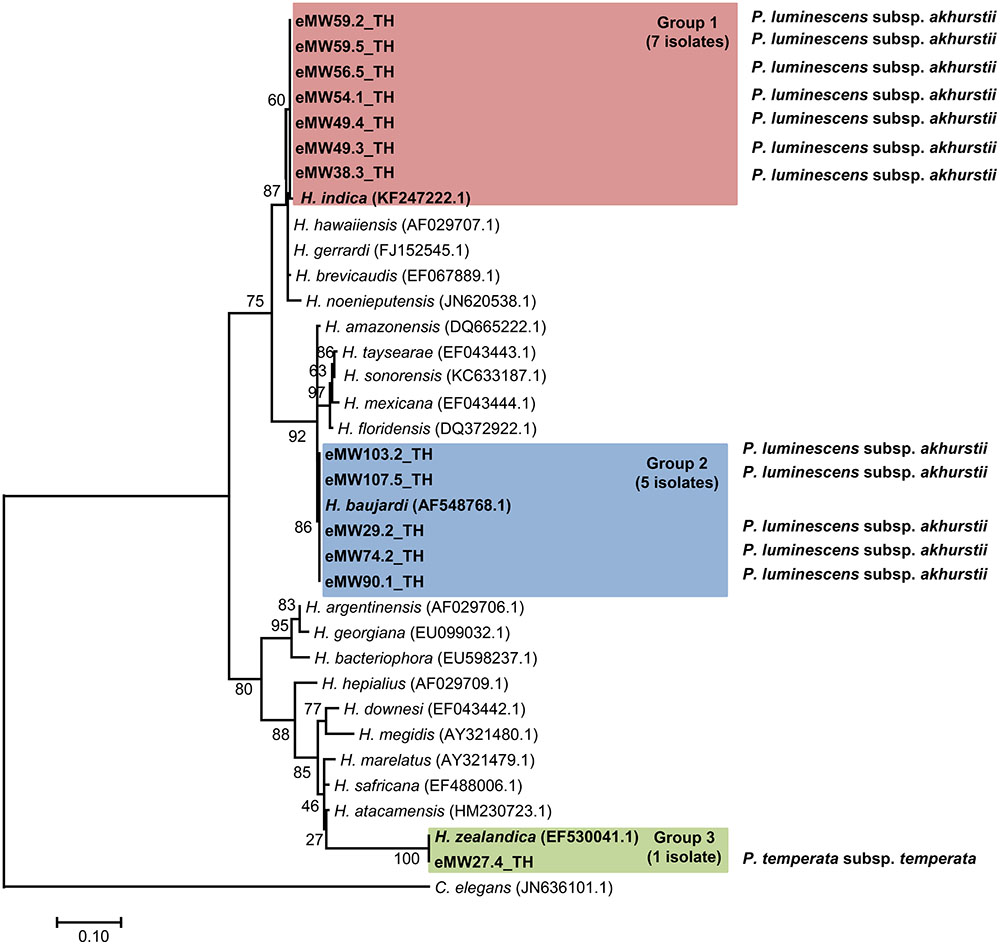
FIGURE 2. Maximum likelihood tree for Heterorhabditis. The phylogenetic tree was based on 634 bp of a partial region of the internal transcribed spacer (ITS) for 13 Heterorhabditis isolates from Mae Wong National Park, Kamphaeng Phet Province, Thailand together with 20 sequences of the Heterorhabditis ITS regions downloaded from NCBI. Caenorhabditis elegans was used as the out-group.
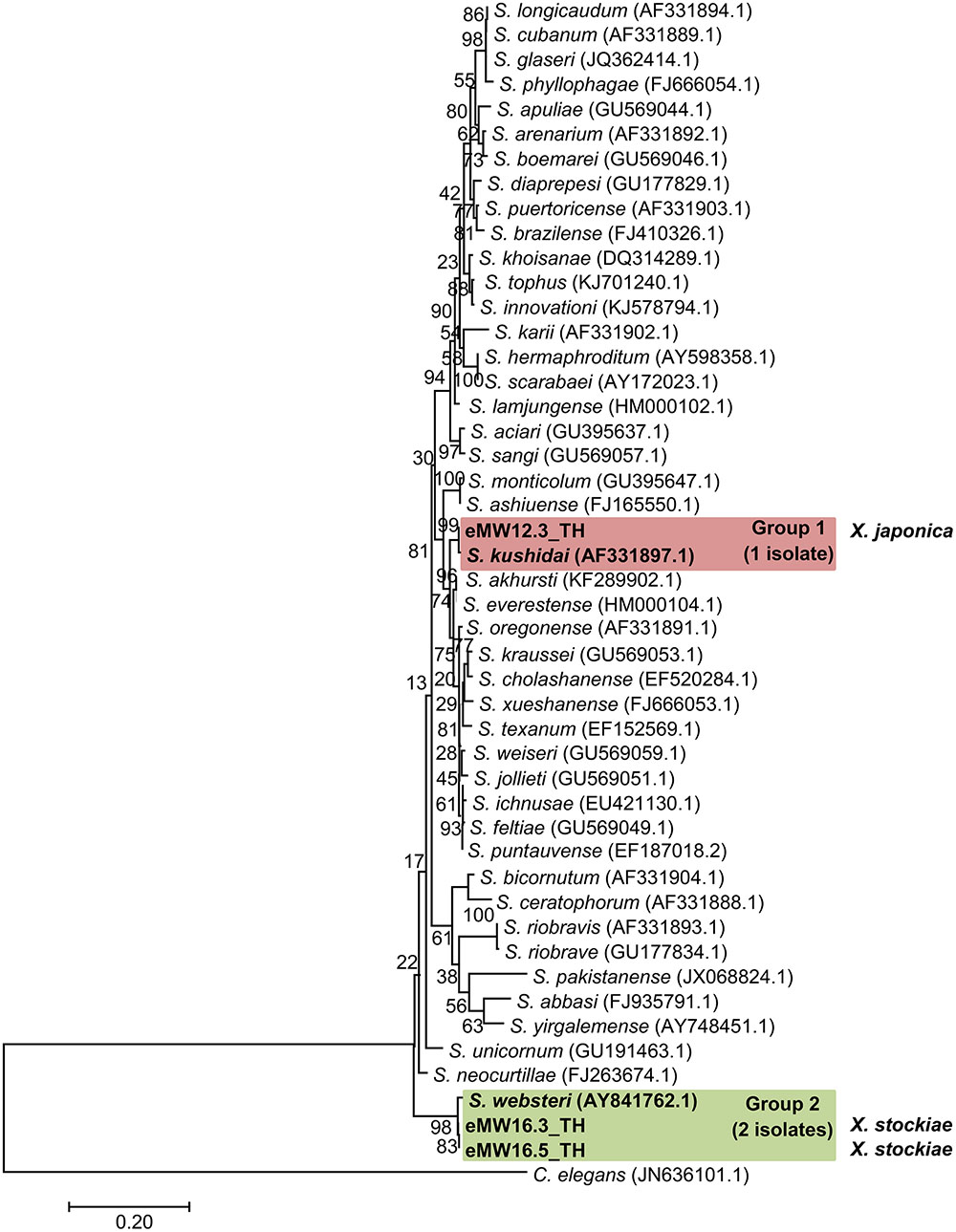
FIGURE 3. Maximum likelihood tree for Steinernema. The phylogenetic tree was based on 634 bp of a partial region of the 28S rRNA gene for 3 Steinernema isolates from Mae Wong National Park, Kamphaeng Phet Province, Thailand together with 44 sequences of a region of the Steinernema 28S rRNA gene downloaded from NCBI. C. elegans was used as the out-group.
Identification and Phylogeny of Photorhabdus and Xenorhabdus
Preliminary identification of symbiotic bacteria at the genus level was based on colony morphology on NBTA agar. In total, 24 EPNs presented as Photorhabdus (green colony) on NBTA agar (21 isolates) and Xenorhabdus (blue colony) on NBTA agar (3 isolates). BLASTN analysis of 588 bp recA sequences revealed that 20 isolates of P. luminescens subsp. akhurstii and 1 isolate of P. temperata subsp. temperata (Accession No. KY436903–KY436923) could be identified with 97–99% identity. Phylogenetic tree analysis of the 21 Photorhabdus isolates demonstrated 2 distinct groups. Group 1 was composed of 20 isolates that were closely related to P. luminescens subsp. akhurstii (FJ862005.1) and group 2 contained only a single isolate that was related to P. temperata subsp. temperata (FJ862014.0) (Figure 4). In turn, the three isolates of Xenorhabdus (Accession No. KY404049–KY404051) were identified as Xenorhabdus stockiae (two isolates) with 97% identity and X. japonica (one isolate) with 98% similarity. Figure 5 shows the phylogenetic tree of Xenorhabdus, which was divided into two groups. Group 1 contained only one study isolate and a recA sequence of X. japonica (FJ823400.1). Group 2 included two study isolates that were closely related to X. stockiae (FJ823425.1).
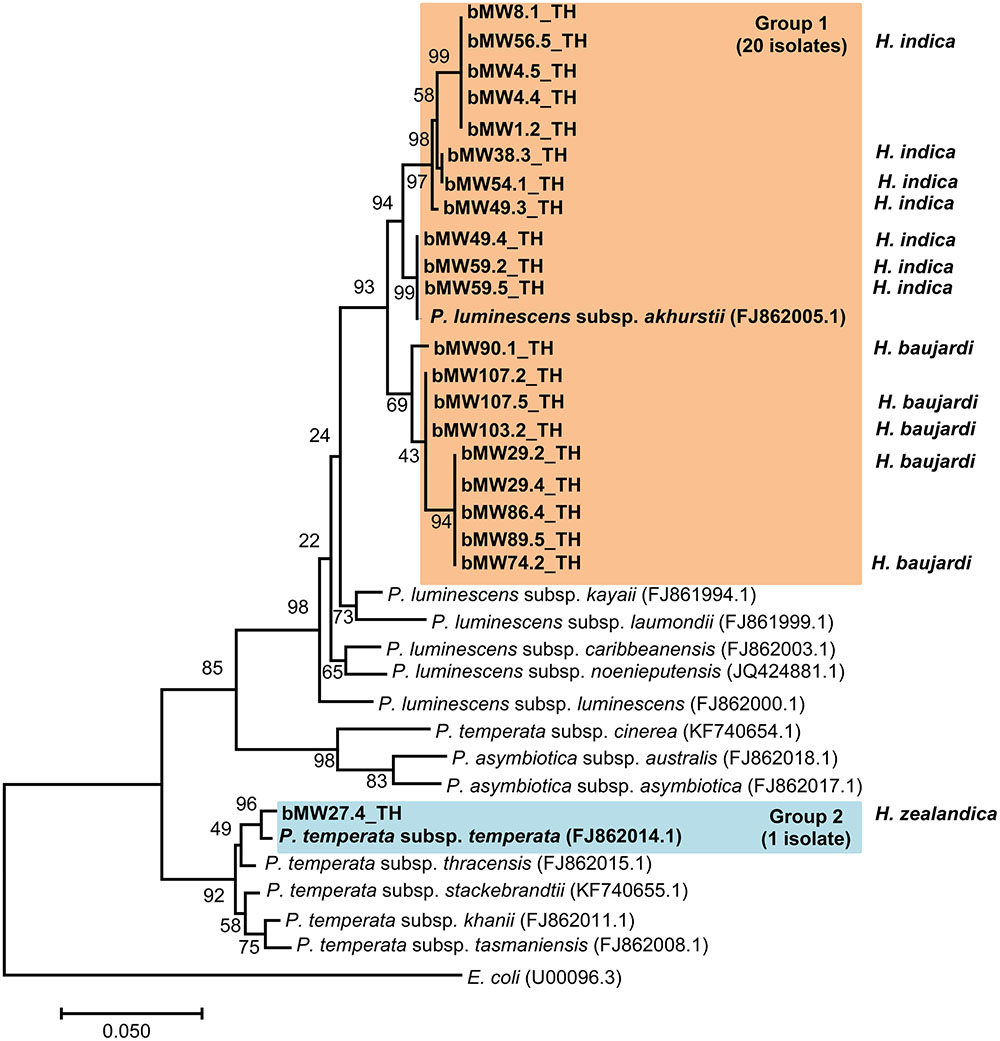
FIGURE 4. Maximum likelihood tree for Photorhabdus. The phylogenetic tree was based on 588 bp of the partial recA gene for 21 Photorhabdus isolated from Mae Wong National Park, Kamphaeng Phet Province, Thailand together with 14 sequences of the Photorhabdus recA gene downloaded from NCBI.
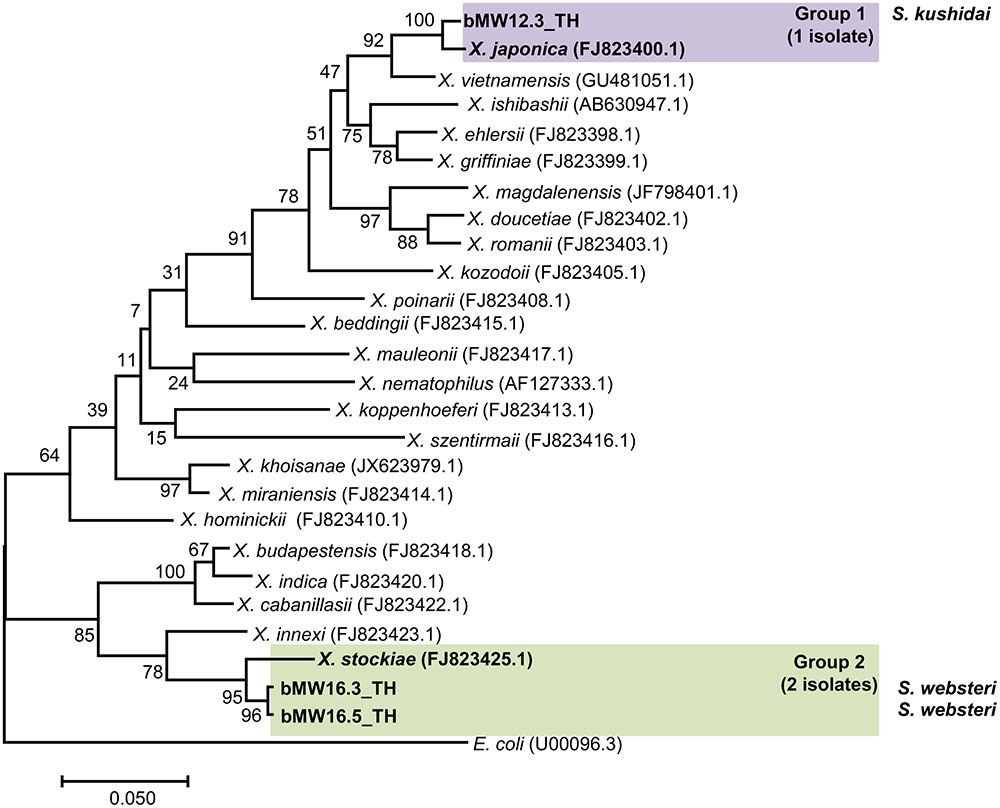
FIGURE 5. Maximum likelihood tree for Xenorhabdus. The phylogenetic tree was based on a 588 bp region of recA for 3 Xenorhabdus isolates from Mae Wong National Park, Kamphaeng Phet Province, Thailand together with 23 sequences of the Xenorhabdus recA gene downloaded from NCBI.
Antimicrobial Activity
A clear zone indicating growth inhibition of drug resistant bacteria was demonstrated after exposure to the bacterial extraction from 2 isolates of Xenorhabdus and nine isolates of Photorhabdus (Figures 6, 7 and Table 1). The active isolates were P. luminescens subsp. akhurstii (bMW1.2_TH, bMW8.1_TH, bMW49.3_TH, bMW56.5_TH, bMW59.2_TH, bMW59.5_TH, bMW90.1_TH, and bMW103.2_TH along with P. temperata subsp. temperata bMW27.4_TH). The most potent Photorhabdus isolate was bMW27.4_TH P. temperata subsp. temperata, which could inhibit up to 10 strains of drug resistant bacteria AB320 A. baumannii (extensively drug resistant or XDR), AB321 A. baumannii (mutidrug resistant or MDR), AB322 A. baumannii (MDR), S. aureus ATCC® 20475, PB36 S. aureus (methicillin-resistant S. aureus or MRSA), PB57 S. aureus (MRSA), E. coli ATCC® 35218, PB1 E. coli (ESBL+MDR), PB30 P. aeruginosa (MDR), and E. faecalis ATCC® 51299. All Photorhabdus isolates could inhibit S. aureus ATCC® 20475, PB36 S. aureus (MRSA), and PB57 S. aureus (MRSA). In addition, the bacterial extraction from bMW27.4_TH P. temperata subsp. temperata could inhibit the growth of S. aureus ATCC® 20475 with a clear zone larger than 25 mm. However, both isolates of X. stockiae (bMW16.3_TH and bMW16.5_TH) inhibited only the growth of P. aeruginosa.
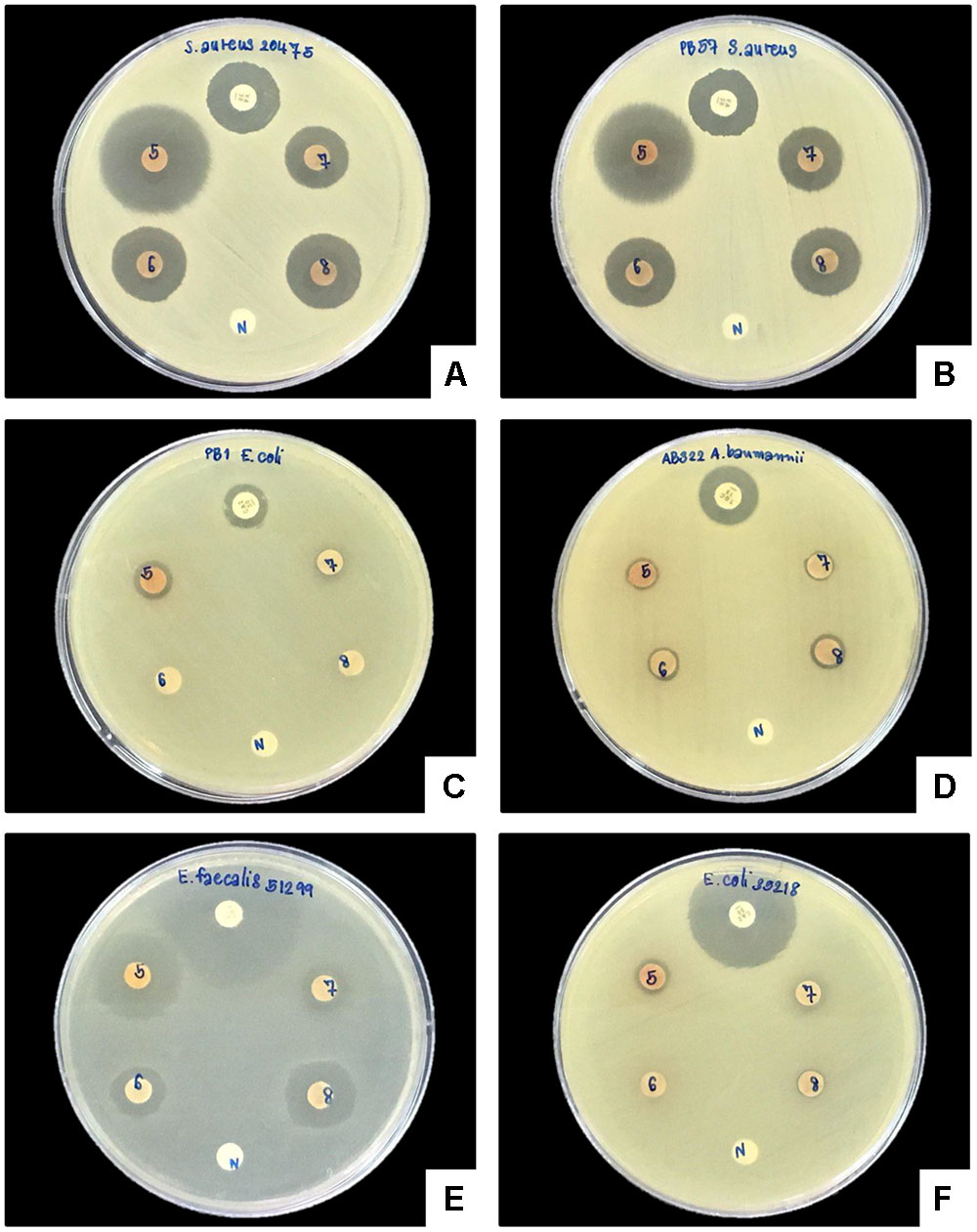
FIGURE 6. Disk diffusion method of bacterial extracts against six drug resistant bacteria. Clear zone of S. aureus ATCC® 20475 (A), PB57 S. aureus (MRSA; B), PB1 E. coli (ESBL+MDR; C), AB322 A. baumannii (MDR; D), E. faecalis ATCC® 51299 (E), and E. coli ATCC® 35218 (F) after exposure to bacterial extracts from bMW27.4_TH P. temperata subsp. temperata (5), bMW49.3_TH P. luminescens subsp. akhurstii (6), bMW56.5_TH P. luminescens subsp. akhurstii (7), bMW59.2_TH P. luminescens subsp. akhurstii (8), Antibiotic disks (P) and Negative control (N).
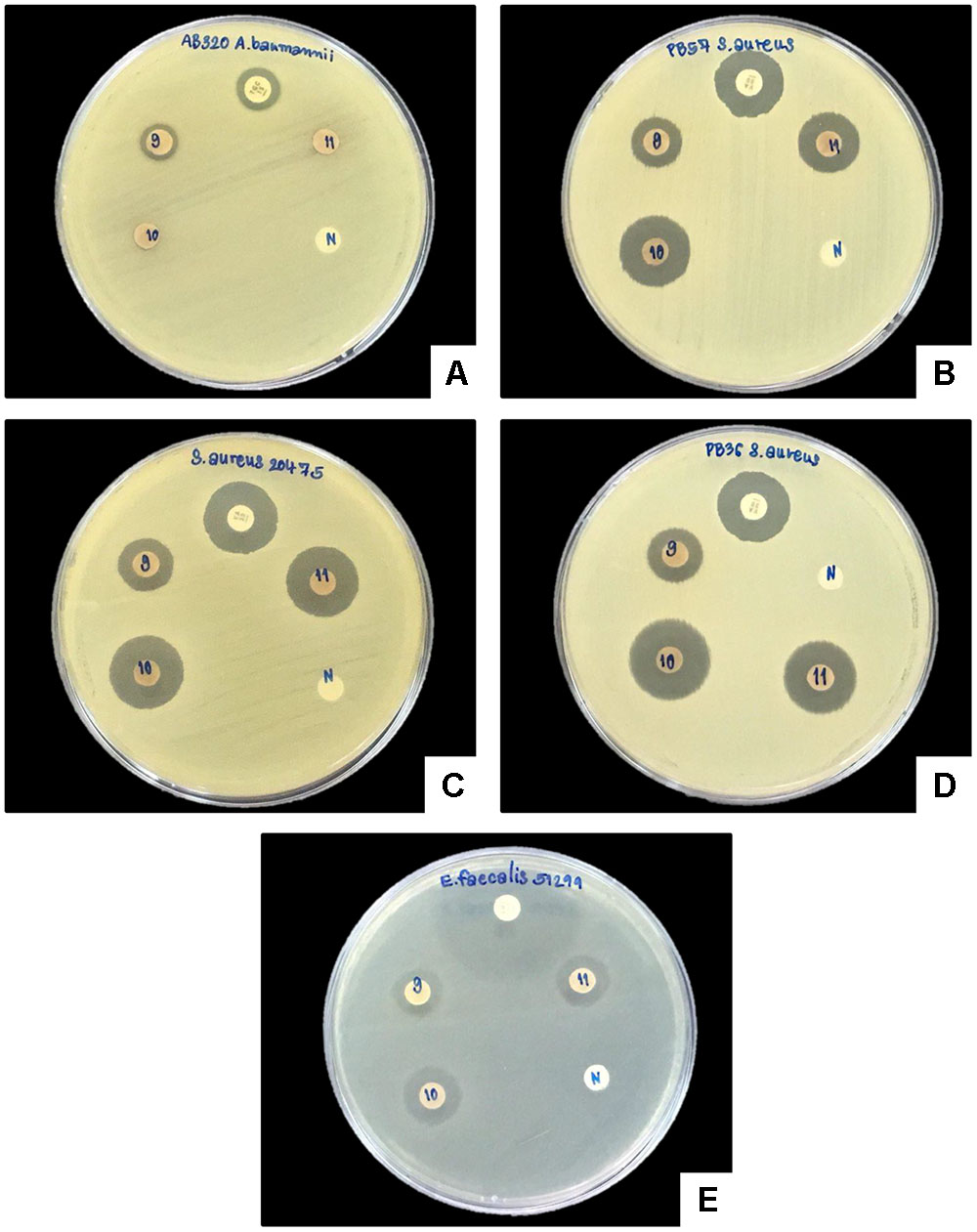
FIGURE 7. Disk diffusion method of bacterial extracts against five drug resistant bacteria. Clear zone of AB320 A. baumannii (XDR; A), PB57 S. aureus (MRSA; B), S. aureus ATCC® 20475 (C), PB- S. aureus (MRSA; D) and E. faecalis ATCC® 51299 (E) after exposure to bacterial extracts from bMW59.5_TH P. luminescens subsp. akhurstii (9), bMW90.1_TH P. Luminescens subsp. akhurstii (10), bMW103.2_TH P. luminescens subsp. akhurstii (11), Antibiotic disks (P) and Negative control (N).
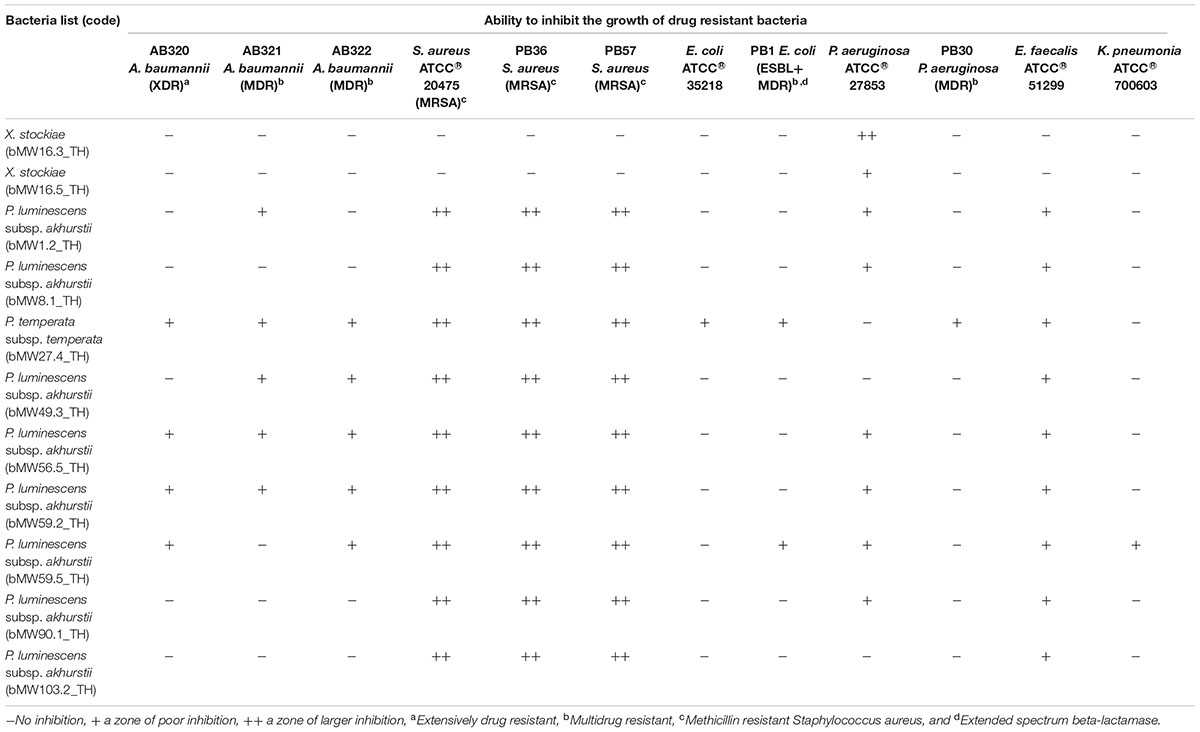
TABLE 1. Antibacterial activity of Photorhabdus extracts against drug resistant bacteria as assessed by disk diffusion.
Based on MIC and MBC testing, eight bacterial extracts from all P. luminescens subsp. akhurstii isolates were evaluated against three strains of drug resistant bacteria including S. aureus ATCC® 20475, PB36 S. aureus (MRSA), and PB57 S. aureus (MRSA). In addition, one bacterial extract from a P. temperata subsp. temperata isolate was evaluated against five strains of drug resistant bacteria including S. aureus ATCC® 20475, PB36 S. aureus (MRSA), PB57 S. aureus (MRSA), E. coli ATCC® 35218, and PB30 P. aeruginosa (MDR). The growth of drug resistant bacteria was inhibited by Photorhabdus extracts with MICs ranging from 7.81 to 0.86 mg/ml. PB36 S. aureus (MRSA) was the most susceptible to all extracts with MICs ranging from 1.95 to 0.86 mg/ml (Table 2) and MBCs ranging from 0.86 to 15.625 mg/ml (Supplementary Figures S1–S4).
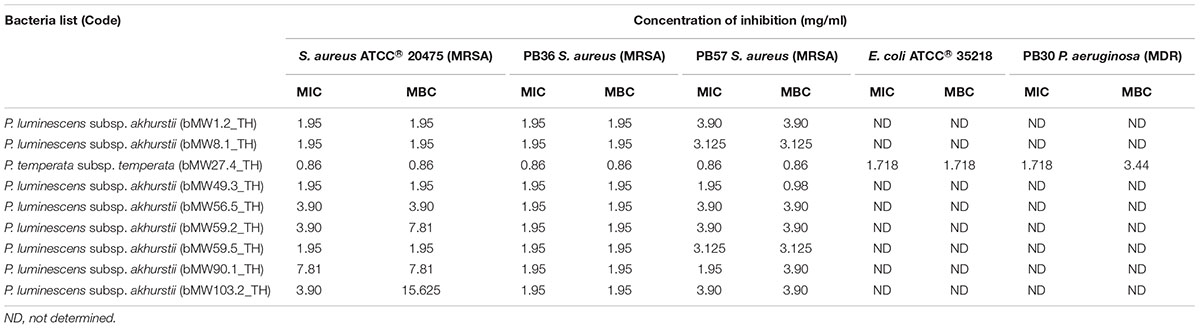
TABLE 2. Antibacterial activity of Photorhabdus extracts against drug resistant bacteria as assessed by minimal inhibitory concentration and minimal bactericidal concentration (mg/ml).
HPLC-MS Analysis
Ethyl acetate extracts were checked by HPLC-MS for known compound classes (Supplementary Figure S5 and Table S2). All isolates of Xenorhabdus and Photorhabdus produced GameXPeptide derivatives. All of the Photorhabdus spp. produced isopropylstilbene and all Xenorhabdus isolates produced xenoamicin derivatives. Six isolates of P. luminescens subsp. akhurstii produced xenocoumacin derivatives. Additionally, mevalagmapeptide and phurealipids derivatives were found in many of the isolates (Supplementary Table S2).
Discussion
In the present study, we surveyed soil samples in search of EPNs associated with Xenorhabdus and Photorhabdus in Mae Wong National Park, Kamphaeng Phet Province, Thailand. Our findings are consistent with previous reports (Thanwisai et al., 2012; Vitta et al., 2015, 2017), which showed that the common species of EPNs in Thailand are H. indica and S. websteri whereas H. baujardi represents a minor identified species, thus confirming that H. indica and S. websteri are abundant in Thailand. In addition, the current study represents the first recorded identification of H. zealandica and S. kushidai in Thailand. H. zealandica was first isolated from Heteronychus arator in Auckland, New Zealand (Poinar, 1990). S. kushidai was first isolated from field soil in Shizuoka Prefecture, Japan (Kushida et al., 1987). H. zealandica and S. kushidai each were identified as a single isolate in the present study, suggesting that these two species may have a low level of distribution and be restricted to near forested areas (National Parks). This conclusion is further suggested by the lack of H. zealandica and S. kushidai isolates recovered from roadside verge, fruit crops, rice fields, or the banks of rivers and ponds in Thailand in prior surveys (Thanwisai et al., 2012; Vitta et al., 2015).
Here, we reported that X. stockiae, X. japonica, P. luminescens subsp. akhurstii, and P. temperata subsp. temperata were dominated by P. luminescens subsp. akhurstii. This is consistent with the results of previous studies in Thailand, which recorded two species of Xenorhabdus including X. stockiae and X. miraniensis and two species (Five subspecies) of Photorhabdus including P. luminescens subsp. laumondii, P. luminescens subsp. akhurstii, P. luminescens subsp. hainaensis, P. asymbiotica subsp. australis, and P. luminescens subsp. namnaonensis throughout the country (Tailliez et al., 2010; Maneesakorn et al., 2011; Thanwisai et al., 2012; Glaeser et al., 2017). Of these, X. miraniensis, P. asymbiotica subsp. australis, P. luminescens subsp. laumondii, and P. luminescens subsp. hainanensis were not identified in our study. This may be due to low distribution of these bacteria. In contrast, a new record of X. japonica and P. temperata subsp. temperata in our country was established in the current study. X. japonica associated with S. kushidai have previously been reported from Japan (Nishimura et al., 1994); this Steinernema-Xenorhabdus complex is similar to our finding. In addition, a previous study showed that P. temperata subsp. temperata is symbiotically associated with H. megidis (Tailliez et al., 2010). In contrast, the current study instead demonstrated P. temperata subsp. temperata and H. zealandica as a novel association. H. zealandica from South Africa has been previously reported to be symbiotic with Photorhabdus heterorhabditis (Ferreira et al., 2014), which suggests that P. temperata subsp. temperata manifests a broad host range with Heterorhabditis spp.
Antibiotics have the potential to prevent or treat infectious agents that can cause patient morbidity and mortality. Accordingly, the development of antibiotic resistance in infectious bacteria is of substantial concern. Our study demonstrated that crude extracts from P. temperata subsp. temperata and P. luminescens subsp. akhurstii showed good antimicrobial activity against drug resistant bacteria. The most common bacteria exhibiting susceptibility to Photorhabdus extract were S. aureus ATCC® 20475, PB36 S. aureus (MRSA), and PB57 S. aureus (MRSA). This may be due to the ability of Photorhabdus to produce several secondary metabolites including insecticidal compounds and antimicrobials such as isopropylstilbene, ethylstilbene, epoxystilbene, photobactin, and ulbactin E (Bode, 2009). In support of the antibacterial activity of this bacterium, P. temperata showed many bioactivities, e.g., insecticidal, antioxidant, and antibacterial activities (Jang et al., 2012; Ullah et al., 2014, 2015). Phthalic acid or 1, 2-benzenedicarboxylic acid purified from P. temperata M1021 showed antibacterial activity with MIC values ranged between 0.1 and 0.5 M (Ullah et al., 2014). In addition, benzaldehyde purified from P. temperata M1021 showed antibacterial activity with MIC values ranging between 6 and 10 mM and antifungal activity with MIC values between 8 and 10 mM (Ullah et al., 2015).
Notably, in the present study P. temperata subsp. temperata could inhibit the growth of 10 strains of drug resistant bacteria, consistent with previous reports that this bacterium inhibited the growth of Salmonella Typhimurium KCTC 1926 and Micrococcus luteus KACC 10488 (Jang et al., 2012). This confirmed that P. temperata is the broad activity against bacterial pathogens. P. temperata may therefore serve as a suitable alternative choice for application as a biocontrol agent in drug industries. In addition, this indicates that several antimicrobial compounds or effective secondary metabolites may be produced by this bacterium that might be used for the production of broad spectrum antimicrobial agents.
Furthermore, several isolates of P. luminescens subsp. akhurstii also exhibited good bactericidal activity in the current study against several strains of drug resistant bacteria. P. luminescens subsp. akhurstii previously showed nematicidal and antibacterial activities (Sharma et al., 2002; Qiu et al., 2009, 2016). Lumicins, novel bacteriocins produced by P. luminescens subsp. akhurstii strain W14, showed bioactivity against other Photorhabdus and E. coli (Sharma et al., 2002). The current findings thus represent a starting point for understanding the antimicrobial activity of Photorhabdus. Further research related to the facilities of Photorhabdus and identifying the active compounds in the extract from. Photorhabdus culture would likely be valuable to facilitate the control of infectious disease in an effective and sustainable manner.
In comparison, X. stockiae, a symbiotic bacterium found abundantly in Thailand, appears to have less bactericidal effects against drug-resistant bacteria. Previous studies show that X. stockiae and their secreted products have good effects against mushroom mites and bacteria that cause mastitis in cows such as S. aureus, S. intermedius, Streptococcus agalactiae, E. faecalis, K. pneumoniae, and E. coli (Bussaman and Rattanasena, 2016; Namsena et al., 2016). In addition, nanoparticles of extracellular metabolites from X. stockiae (KT835471) showed bactericidal effect against six different pathogens (Chandrakasan et al., 2017).
Overall, based on the MIC and MBC of Xenorhabdus and Photorhabdus against drug resistant bacteria, we found that different species and isolates of these bacteria gave variable effects on different species and strains of drug resistant bacteria. This may arise because of the ability of each symbiotic bacteria to produce effective metabolites or the susceptibility of drug resistant bacteria to the respective metabolites produced by each symbiote.
Based on the analysis of ethyl acetate extracts by HPLC-MS, all isolates produced GameXPeptide derivatives, a compound known to be common among the genera but with an unknown biological activity (Bode et al., 2012; Nollmann et al., 2015a). Six isolates produced xenocoumacin derivatives (Reimer et al., 2009) or amicoumacin derivatives (Park et al., 2016) being potent antibiotics, while all of the Photorhabdus spp. produced isopropylstilbene that has multiple biological activities including antibiotic activity (Li et al., 1995; Buscató et al., 2013). All Xenorhabdus isolates produced xenoamicin derivatives showing weak antiprotozoal activity (Zhou et al., 2013). Additionally, we identified mevalagmapeptide (Bode et al., 2015, 2012) and the juvenile hormone epoxide hydrolase inhibitors phurealipids (Nollmann et al., 2015b) in many of the isolates.
Conclusion
We have extended the basic knowledge regarding EPNs and their symbiotic bacteria, Xenorhabdus and Photorhabdus, in Mae Wong National Park of Thailand. The common symbiotic species in the study area are H. indica associated with P. luminescens subsp. akhurstii and S. websteri associated with X. stockiae. H. zealandica, and S. kushidai represent newly recorded EPNs in Thailand with low distribution. X. japonica and P. temperata subsp. temperata reflect new observations of symbiotic bacteria in Thailand. Furthermore, the association between P. temperata subsp. temperata and H. zealandica has not been previously reported in any location. Based on MIC and MBC, all isolates of Xenorhabdus and Photorhabdus have bactericidal activity with variable effect on different species or strains of drug resistant bacteria. Nevertheless, P. temperata subsp. temperata represents the best symbiotic bacteria for inhibiting the growth of AB320 A. baumannii (extensively drug resistant or XDR), AB321 A. baumannii (multidrug resistant or MDR), AB322 A. baumannii (MDR), S. aureus ATCC® 20475, PB36 S. aureus (methicillin-resistant S. aureus or MRSA), PB57 S. aureus (MRSA), E. coli ATCC® 35218, PB1 E. coli (ESBL+MDR), PB30 P. aeruginosa (MDR), and E. faecalis ATCC® 51299. P. temperata subsp. temperata may be of interest for further study regarding its antimicrobial activity. Our findings enhance the understanding of the distribution of EPN-bacteria complexes and provide a foundation for subsequent research toward identifying potential antimicrobial compounds that may represent effective and sustainable resources for combating drug resistant microbial infections.
Author Contributions
Conceived and designed the experiments: AT, AV, SS, and NC. Performed the experiments: PM, TY, CF, MS, and THY. Analyzed the data: AT, AV, and PM. Contributed reagents/materials/analysis tools: AT, AV, SS, and NC. Chemical analysis of bacterial extracts: NT and HB. Wrote the paper: PM, AT, AV, SS, and HB.
Funding
The National Research Council of Thailand (Grant Number R2557A059) for AV. Naresuan University (Grant Number R2559B089) for AT.
Conflict of Interest Statement
The authors declare that the research was conducted in the absence of any commercial or financial relationships that could be construed as a potential conflict of interest.
Acknowledgments
Many thanks are extended to Mr. Suthon Weingdow, Head of Mae Wong National Park and his staff for their facility in soil collection. We would also like to thank Miss Pichamon Janthu, Miss Wipanee Meesil, Miss Ponsuwan Aeiwong, Miss Kamonphan S. Ruangyan, Miss Chutima Sarai, and Mr. Pannawat Thimpoo for their assistance in soil collection.
Supplementary Material
The Supplementary Material for this article can be found online at: http://journal.frontiersin.org/article/10.3389/fmicb.2017.01142/full#supplementary-material
References
Adams, B. J., Fodor, A., Koppenhöfer, H. S., Stäckebrandt, E., Stock, S. P., and Klein, M. G. (2006). Biodiversity and systematics of nematode–bacterium entomopathogens. Biol. Cont. 37, 32–49. doi: 10.1016/j.biocontrol.2005.11.008
Akhurst, R. J. (1982). Antibiotic activity of Xenorhabdus spp. bacteria symbiotically associated with insect pathogenic nematodes of the families Heterorhabdiidae and Steinernematidae. J. Gen. Microbiol. 128, 3061–3065. doi: 10.1099/00221287-128-12-3061
Bedding, R. A., and Akhurst, R. J. (1975). A simple technique for the detection of insect parasitic rhabditid nematode in soil. Nematologica 21, 109–110. doi: 10.1163/187529275X00419
Bode, E., Brachmann, A. O., Kegler, C., Simsek, R., Dauth, C., Zhou, Q., et al. (2015). Simple “on-demand” production of bioactive natural products. Chembiochem 16, 1115–1119. doi: 10.1002/cbic.201500094
Bode, H. B. (2009). Entomopathogenic bacteria as a source of secondary metabolites. Curr. Opin. Chem. Biol. 13, 224–230. doi: 10.1016/j.cbpa.2009.02.037
Bode, H. B., Reimer, D., Fuchs, S. W., Kirchner, F., Dauth, C., Kegler, C., et al. (2012). Determination of the absolute configuration of peptide natural products by using stable isotope labeling and mass spectrometry. Chem. Eur. J. 18, 2342–2348. doi: 10.1002/chem.201103479
Buscató, E. L., Büttner, D., Brüggerhoff, A., Klingler, F.-M., Weber, J., Scholz, B., et al. (2013). From a multipotent stilbene to soluble epoxide hydrolase inhibitors with antiproliferative properties. ChemMedChem 8, 919–923. doi: 10.1002/cmdc.201300057
Bussaman, P., and Rattanasena, P. (2016). Additional property of Xenorhabdus stockiae for inhibiting cow Mastitis-causing bacteria. Biosci. Biotech. Res. Asia 13, 1871–1878. doi: 10.13005/bbra/2342
Bussaman, P., Sa-Uth, C., Rattanasena, P., and Chandrapatya, A. (2012). Acaricidal activities of whole cell suspension, cell-free supernatant and crude cell extract of Xenorhabdus stokiae against mushroom mite (Luciaphorus sp.). J. Zhejiang Univ. Sci. B 13, 261–266. doi: 10.1631/jzus.B1100155
Chandrakasan, G., Seetharaman, P., Gnanasekar, S., Kadarkarai, M., and Sivaperumal, S. (2017). Xenorhabdus stockiae KT835471-mediated feasible biosynthesis of metal nanoparticles for their antibacterial and cytotoxic activities. Artif. Cells Nanomed. Biotechnol. 31, 1–10. doi: 10.1080/21691401.2017.128249
Cimen, H., Lee, M. M., Hatting, J., Hazir, S., and Stock, S. P. (2014). Steinernema tophus sp. n. (Nematoda: Steinernematidae), a new entomopathogenic nematode from South Africa. Zootaxa 3821, 337–353. doi: 10.11646/zootaxa.3821.3.3
Cimen, H., Lee, M. M., Hatting, J., Hazir, S., and Stock, S. P. (2015). Steinernema innovation n. sp. (Panagrolaimomorpha: Steinernematidae), a new entomopathogenic nematode species from South Africa. J. Helminthol. 89, 415–417. doi: 10.1017/S0022149X14000182
Eleftherianos, I., Boundy, S., Joyce, S. A., Aslam, S., Marshall, J. W., Cox, R. J., et al. (2007). An antibiotic produced by an insect-pathogenic bacterium suppresses host defenses through phenoloxidase inhibition. Proc. Natl. Acad. Sci. U.S.A. 104, 2419–2424. doi: 10.1073/pnas.0610525104
Ferreira, T., Van Reenen, C. A., Endo, A., Spröer, C., Malan, A. P., and Dicks, L. M. (2013a). Description of Xenorhabdus khoisanae sp. nov., the symbiont of the entomopathogenic nematode Steinernema khoisanae. Int. J. Syst. Evol. Microbiol. 63, 3220–3224. doi: 10.1099/ijs.0.049049-0
Ferreira, T., Van Reenen, C. A., Endo, A., Tailliez, P., Pagès, S., Spröer, C., et al. (2014). Photorhabdus heterorhabditis sp. nov., a symbiont of the entomopathogenic nematode Heterorhabditis zealandica. Int. J. Syst. Evol. Microbiol. 64, 1540–1545. doi: 10.1099/ijs.0.059840-0
Ferreira, T., Van Reenen, C. A., Pagès, S., Tailliez, P., Malan, A. P., and Dicks, L. M. (2013b). Photorhabdus luminescens subsp. noenieputensis subsp. nov., a symbiotic bacterium associated with a novel Heterorhabditis species related to Heterorhabditis indica. Int. J. Syst. Evol. Microbiol. 63, 1853–1858. doi: 10.1099/ijs.0.044388-0
Genhui, C. (1996). Antimicrobial Activity of the Nematodes Symbionts, Xenorhabdus and Photorhabdus (Enterobacteriaceae), and the Discovery of Two Groups of Antimicrobial Substances, Nemotophin and Xenoxides. Ph.D. thesis, Simon Fraser University, Burnaby, BC.
Glaeser, S. P., Tobias, N. J., Thanwisai, A., Chantratita, N., Bode, H. B., and Kämpfer, P. (2017). Photorhabdus luminescens subsp. namnaonensis subsp. nov., isolated from Heterorhabditis baujardi nematodes in Nam Nao district of central Thailand. Int. J. Syst. Evol. Microbiol. 67, 1046–1051. doi: 10.1099/ijsem.0.001761
Grundmann, F., Kaiser, M., Schiell, M., Batzer, A., Kurz, M., Thanwisai, A., et al. (2014). Antiparasitic chaiyaphumines from entomopathogenic Xenorhabdus sp. PB61.4. J. Nat. Prod. 77, 779–783. doi: 10.1021/np4007525
Hominick, W. M. (2002). “Biogeography,” in Entomopathogenic Nematology, ed. R. Gaugler (Wallingford: CABI Publishing), 115–143. doi: 10.1079/9780851995670.0115
Jang, E. K., Ullah, I., Lim, J. H., Lee, I. J., Kim, J. G., and Shin, J. H. (2012). Physiological and molecular characterization of a newly identified entomopathogenic bacteria, Photorhabdus temperata M1021. J. Microbiol. Biotechnol. 22, 1605–1612. doi: 10.4014/jmb.1203.03068
Kushida, T., Mamiya, Y., and Mitsuhashi, J. (1987). Pathogenicity of newly detected Steinernema sp. (Nematode) to scarabaeid larvae injurious to tree seedlings. J. Appl. Entomol. Zool. 31, 144–149. doi: 10.1303/jjaez.31.144
Li, J., Chen, G., Wu, H., and Webster, J. M. (1995). Identification of two pigments and a hydroxystilbene antibiotic from Photorhabdus luminescens. Appl. Environ. Microbiol. 61, 4329–4333.
Li, X., Liu, Q. Z., Nermut’, J., Půža, V., and Mráček, Z. (2012). Heterorhabditis beicherriana n. sp. (Nematoda: Heterorhabditidae), a new entomopathogenic nematode from the Shunyi district of Beijing, China. Zootaxa 3569, 25–40.
Malan, A. P., Knoetze, R., and Tiedt, L. (2014). Heterorhabditis noenieputensis n. sp. (Rhabditida: Heterorhabditidae), a new entomopathogenic nematode from South Africa. J. Helminthol. 88, 139–151. doi: 10.1017/S0022149X12000806
Maneesakorn, P., An, R., Daneshvar, H., Taylor, K., Bai, X., Adams, B. J., et al. (2011). Phylogenetic and cophylogenetic relationships of entomopathogenic nematodes (Heterorhabditis: Rhabditida) and their symbiotic bacteria (Enterobacteriaceae). Mol. Phylogenet. Evol. 59, 271–280. doi: 10.1016/j.ympev.2011.02.012
Maneesakorn, P., Grewal, P. S., and Chandrapatya, A. (2010). Steinernema minutum sp. nov. (Rhabditida: Steinernamatidae): a new entomopathogenic nematode from Thailand. Int. J. Nematol. 20, 27–42.
McInerney, B. V., Taylor, W. C., Lacey, M. J., Akhurst, R. J., and Gregson, R. P. (1991). Biologically active metabolites from Xenorhabdus spp., Part 2. Benzopyran-1-one derivatives with gastroprotective activity. J. Nat. Prod. 54, 785–795. doi: 10.1021/np50075a006
Namsena, P., Bussaman, P., and Rattanasena, P. (2016). Bioformulation of Xenorhabdus stockiae PB09 for controlling mushroom mite, Luciaphorus perniciosus Rack. Bioresour. Bioprocess 3:19. doi: 10.1186/s40643-016-0097-5
Nishimura, Y., Hagiwara, A., Suzuki, T., and Yamanaka, S. (1994). Xenorhabdus japonicus sp. nov. associated with the nematode Steinernema kushidai. World J. Microbiol. Biotechnol. 10, 207–210. doi: 10.1007/BF00360889
Nollmann, F. I., Dauth, C., Mulley, G., Kegler, C., Kaiser, M., Waterfield, N. R., et al. (2015a). Insect-specific production of new GameXPeptides in Photorhabdus luminescens TTO1, widespread natural products in entomopathogenic bacteria. ChemBioChem 16, 205–208. doi: 10.1002/cbic.201402603
Nollmann, F. I., Heinrich, A. K., Brachmann, A. O., Morisseau, C., Mukherjee, K., Casanova-Torres, Á. M., et al. (2015b). A Photorhabdus natural product inhibits insect juvenile hormone epoxide hydrolase. ChemBioChem 16, 766–771. doi: 10.1002/cbic.201402650
Nthenga, I., Knoetze, R., Berry, S., Tiedt, L. R., and Malan, A. P. (2014). Steinernema sacchari n. sp. (Rhabditida: Steinernematidae), a new entomopathogenic nematode from South Africa. Nematology 16, 475–494. doi: 10.1163/15685411-00002780
Park, H., Perez, C., Perry, E., and Crawford, J. (2016). Activating and attenuating the amicoumacin antibiotics. Molecules 21:E824. doi: 10.3390/molecules21070824
Phan, K. L., Mráček, Z., Půža, V., Nermut’, J., and Jarošová, A. (2014). Steinernema huense sp. n., a new entomopathogenic nematode (Nematoda: Steinernematidae) from Vietnam. Nematology 16, 761–775. doi: 10.1163/15685411-00002806
Poinar, G. O. (1990). “Taxonomy and biology of Steinernematidae and Heterorhabditidae,” in Entomopathogenic Nematodes in Biological Control, eds R. Gaugler and H. K. Kaya (Boca Raton, FL: CRC Press), 23–61.
Qiu, X., Han, R., Yan, X., Liu, M., Cao, L., Yoshiga, T., et al. (2009). Identification and characterization of a novel gene involved in the trans-specific nematicidal activity of Photorhabdus luminescens LN2. Appl. Environ. Microbiol. 75, 4221–4223. doi: 10.1128/AEM.02967-08
Qiu, X., Wu, C., Cao, L., Ehlers, R. U., and Han, R. (2016). Photorhabdus luminescens LN2 requires rpoS for nematicidal activity and nematode development. FEMS Microbiol. Lett 363:fnw035. doi: 10.1093/femsle/fnw035
Reimer, D., Luxenburger, E., Brachmann, A. O., and Bode, H. B. (2009). A new type of pyrrolidine biosynthesis is involved in the late steps of Xenocoumacin production in Xenorhabdus nematophila. ChemBioChem 10, 1997–2001. doi: 10.1002/cbic.200900187
Seier-Petersen, M. A., Jasni, A., Aarestrup, F. M., Vigre, H., Mullany, P., Roberts, A. P., et al. (2014). Effect of subinhibitory concentrations of four commonly used biocides on the conjugative transfer of Tn916 in Bacillus subtilis. J. Antimicrob Chemother. 69, 343–348. doi: 10.1093/jac/dkt370
Sharma, S., Waterfield, N., Bowen, D., Rocheleau, T., Holland, L., James, R., et al. (2002). The lumicins: novel bacteriocins from Photorhabdus luminescens with similarity to the uropathogenic-specific protein (USP) from uropathogenic Escherichia coli. FEMS Microbiol. Lett. 214, 241–249. doi: 10.1111/j.1574-6968.2002.tb11354.x
Stock, S. P. (1998). Steinernema siamkayai n. sp. (Rhabditida: Steinernematidae), an entomopathogenic nematode from Thailand. Syst. Parasitol. 41, 105–113. doi: 10.1023/A:1006087017195
Stock, S. P., Campbell, J. F., and Nadler, S. A. (2001). Phylogeny of Steinernema Travassos 1927 (Cephalobina: Steinernematidae) inferred from ribosomal DNA sequences and morphological characters. J. Parasitol. 87, 877–889. doi: 10.2307/3285148
Tailliez, P., Laroui, C., Ginibre, N., Paule, A., Pages, S., and Boemare, N. (2010). Phylogeny of Photorhabdus and Xenorhabdus based on universally conserved protein-coding sequences and implications for the taxonomy of these two genera. Proposal of new taxa: X. vietnamensis sp. nov., P. luminescens subsp.caribbeanensis subsp. nov., P. luminescens subsp. hainanensis subsp. nov., P. temperata subsp. khanii subsp. nov., P. temperata subsp. tasmaniensis subsp. nov., and the reclassification of P. luminescens subsp. thracensis as P. temperata subsp. thracensis comb. nov. Int. J. Syst. Evol. Microbiol. 60, 1921–1937. doi: 10.1099/ijs.0.014308-0
Tamura, K., Stecher, G., Peterson, D., Filipski, A., and Kumar, S. (2013). MEGA6: molecular evolutionary genetics analysis version 6.0. Mol. Biol. Evol. 30, 2725–2729. doi: 10.1093/molbev/mst197
Tangchitsomkid, N., and Sontirat, S. (1998). Occurrence of entomopathogenic nematodes in Thailand. Kasetsart J. Nat. Sci. 32, 347–354. doi: 10.1016/j.ympev.2011.02.012
Thanwisai, A., Tandhavanant, S., Saiprom, N., Waterfield, N. R., Ke Long, P., Bode, H. B., et al. (2012). Isolation of entomopathogenic nematodes and associated Xenorhabdus/Photorhabdus spp. in Thailand. PLoS ONE 7:e43835. doi: 10.1371/journal.pone.0043835
Thompson, J. D., Higgin, D. G., and Gibson, T. J. (1994). CLUSTAL W: improving the sensitivity of progressive multiple sequence alignment through sequence weighting, position-specific gap penalties and weight matrix choice. Nucleic Acids Res. 22, 4673–4680. doi: 10.1093/nar/22.22.4673
Ullah, I., Khan, A. L., Ali, L., Khan, A. R., Waqas, M., Hussain, J., et al. (2015). Benzaldehyde as an insecticidal, antimicrobial, and antioxidant compound produced by Photorhabdus temperata M1021. J. Microbiol. 53, 127–133. doi: 10.1007/s12275-015-4632-4
Ullah, I., Khan, A. L., Ali, L., Khan, A. R., Waqas, M., Lee, I. J., et al. (2014). An insecticidal compound produced by an insect-pathogenic bacterium suppresses host defenses through phenoloxidase inhibition. Molecules 19, 20913–20928. doi: 10.3390/molecules191220913
Vitta, A., Fukruksa, C., Yimthin, T., Deelue, K., Sarai, C., Polseela, R., et al. (2017). Preliminary survey of entomopathogenic nematodes in upper northern Thailand. Southeast Asian J. Trop. Med. Public Health 48, 18–26.
Vitta, A., Yimthin, T., Fukruksa, C., Wongpeera, W., Yotpanya, W., Ploseela, R., et al. (2015). Distribution of entomopathogenic nematodes in lower Northern Thailand. Southeast Asian J. Trop. Med. Public Health 46, 564–573.
Wang, Y., and Gaugler, R. (1998). Host and penetration site location by entomopathogenic nematode against Japanese beetle larvae. J. Invertebr. Pathol. 72, 313–318. doi: 10.1006/jipa.1998.4805
White, G. F. (1927). A method for obtaining infective nematode larvae from cultures. Science 66, 302–303. doi: 10.1126/science.66.1709.302-a
Keywords: Xenorhabdus, Photorhabdus, entomopathogenic nematodes, antimicrobial activity, drug-resistant bacteria
Citation: Muangpat P, Yooyangket T, Fukruksa C, Suwannaroj M, Yimthin T, Sitthisak S, Chantratita N, Vitta A, Tobias NJ, Bode HB and Thanwisai A (2017) Screening of the Antimicrobial Activity against Drug Resistant Bacteria of Photorhabdus and Xenorhabdus Associated with Entomopathogenic Nematodes from Mae Wong National Park, Thailand. Front. Microbiol. 8:1142. doi: 10.3389/fmicb.2017.01142
Received: 21 February 2017; Accepted: 06 June 2017;
Published: 28 June 2017.
Edited by:
Octavio Luiz Franco, Universidade Católica de Brasília, BrazilReviewed by:
Osmar Nascimento Silva, Universidade Católica Dom Bosco, BrazilAtte Von Wright, University of Eastern Finland, Finland
Copyright © 2017 Muangpat, Yooyangket, Fukruksa, Suwannaroj, Yimthin, Sitthisak, Chantratita, Vitta, Tobias, Bode and Thanwisai. This is an open-access article distributed under the terms of the Creative Commons Attribution License (CC BY). The use, distribution or reproduction in other forums is permitted, provided the original author(s) or licensor are credited and that the original publication in this journal is cited, in accordance with accepted academic practice. No use, distribution or reproduction is permitted which does not comply with these terms.
*Correspondence: Aunchalee Thanwisai, YXVuY2hhbGVldEBudS5hYy50aA==