- 1Department of Dermatology, The Second Affiliated Hospital of Xi’an Jiaotong University, Xi’an, China
- 2State Key Laboratory for Strength and Vibration of Mechanical Structures, Frontier Institute of Science and Technology, Xi’an Jiaotong University, Xi’an, China
- 3Xi’an Key Laboratory of Sustainable Energy Materials Chemistry, Frontier Institute of Science and Technology, Xi’an Jiaotong University, Xi’an, China
Wound repair remains a clinical challenge and bacterial infection is a common complication that may significantly delay healing. Therefore, proper and effective wound management is essential. The photosensitizer-based therapies mainly stimulate the photosensitizer to generate reactive oxygen species through appropriate excitation source irradiation, thereby killing pathogenic microorganisms. Moreover, they initiate local immune responses by inducing the recruitment of immune cells as well as the production of proinflammatory cytokines. In addition, these therapies can stimulate the proliferation, migration and differentiation of skin resident cells, and improve the deposition of extracellular matrix; subsequently, they promote the re-epithelialization, angiogenesis, and tissue remodeling. Studies in multiple animal models and human skin wounds have proved that the superior sterilization property and biological effects of photosensitizer-based therapies during different stages of wound repair. In this review, we summarize the recent advances in photosensitizer-based therapies for enhancing tissue regeneration, and suggest more effective therapeutics for patients with skin wounds.
Introduction
Skin is the most important barrier of human body to resist external invasion. Wound healing after skin injury consists of a serial of intricate processes involving multiple cells, cytokines, inflammatory responses (1). It is a dynamic and continuous process, with overlapping stages, including hemostasis, inflammation, proliferation, and remodeling (2, 3). Inflammation is the initial stage of wound healing, and it occurs almost simultaneously with hemostasis. As the inflammatory response overlaps, various cytokines and growth factors activate and induce the proliferation, migration, and differentiation of repair cells, resulting in a series of biological behaviors, including epithelialization, angiogenesis and collagen accumulation (3). With the differentiation of fibroblasts into myofibroblasts, continuous tissue remodeling is carried out to participate in wound contraction and restore the continuity of skin (2). Wound healing can be affected by many factors, among which the most common challenge is bacterial infection. Bacteria infection could prolong the inflammation period and interfere with re-epithelialization and collagen deposition, affecting wound healing (4–7). For a long time, antibiotics have been empirically used to prevent wound infections. Drug-resistant bacteria is increasing globally, and new strategies that can simultaneously control infection and promote wound healing without developing drug-resistant bacteria are urgently needed. Recently, several studies have clearly demonstrated the beneficial effect of the photosensitizer-based therapies in the healing of infected wounds (8–11).
Photodynamic therapy (PDT) is the most common type of the photosensitizer-based therapies. It was accidentally discovered in 1900 by Oscar Raab. He recognized that micro-organisms were killed when exposed to light, but not when they were kept in the dark (12). Later on, this approach was clinically used in cancer therapy and antimicrobial application. In the past few decades, the research has overwhelmingly focused on the treatment of cancer and it has become a safe modality of tumor ablation for multiple cancer indications. To date, the currently approved dermatological indications of PDT include actinic keratosis, Bowen’s disease (squamous cell carcinoma in situ), and basal cell carcinoma (13). Anti-tumor regimens require lethal doses of photosensitizer (PS) and light, while low-dose treatment have immunomodulatory, antibacterial, and regenerative properties (14, 15). In recent years, the interest in the anti-infective effects of PDT has been revived. It is proposed as a therapy for bacterial, fungal, and viral infections, in which case it is described as antimicrobial PDT (aPDT), or photodynamic inactivation (16). As a new treatment option for inflammatory and infectious diseases, it has been successfully used in skin diseases, such as acne, viral warts, and leishmaniasis (14, 17). Topical PDT is widely used in skin diseases, and it is also an effective method for skin rejuvenation due to the immunomodulatory and antimicrobial effects (17–22).
The types, principles, advantages, and disadvantages of the photosensitizer-based therapies
The photosensitizer-based therapies include the following two main approaches: PDT and photothermal therapy (PTT) (Figure 1). In PTT, photothermal agents absorb energy when irradiated with light of a specific wavelength and transform into the excited singlet state. Then they undergo vibrational relaxation and return to the ground state, during which process enhance the heating in the local area (23). Unlike the local thermal damage caused by PTT, PDT induces cytotoxicity based on the production of reactive oxygen species (ROS). PDT involves three important components: light, PS and molecular oxygen. The PS is preferentially absorbed by rapidly dividing cells (such as microorganisms and tumor cells), and converted into excited singlet state after light irradiation. It partially returns to the ground state by emitting fluorescence or non-radiative form of decay, and can also undergo intersystem crossing to the excited triplet state. The generation of ROS is subsequently promoted by two types of chemical reactions: type I mechanism refers to the PS-induced generation of free radicals and free radical ions through electron transfer reactions; type II mechanism involves the reaction of the PS with molecular oxygen, where the energy is transferred to the triplet ground state molecular oxygen to generate singlet oxygen (11, 15, 23–26).
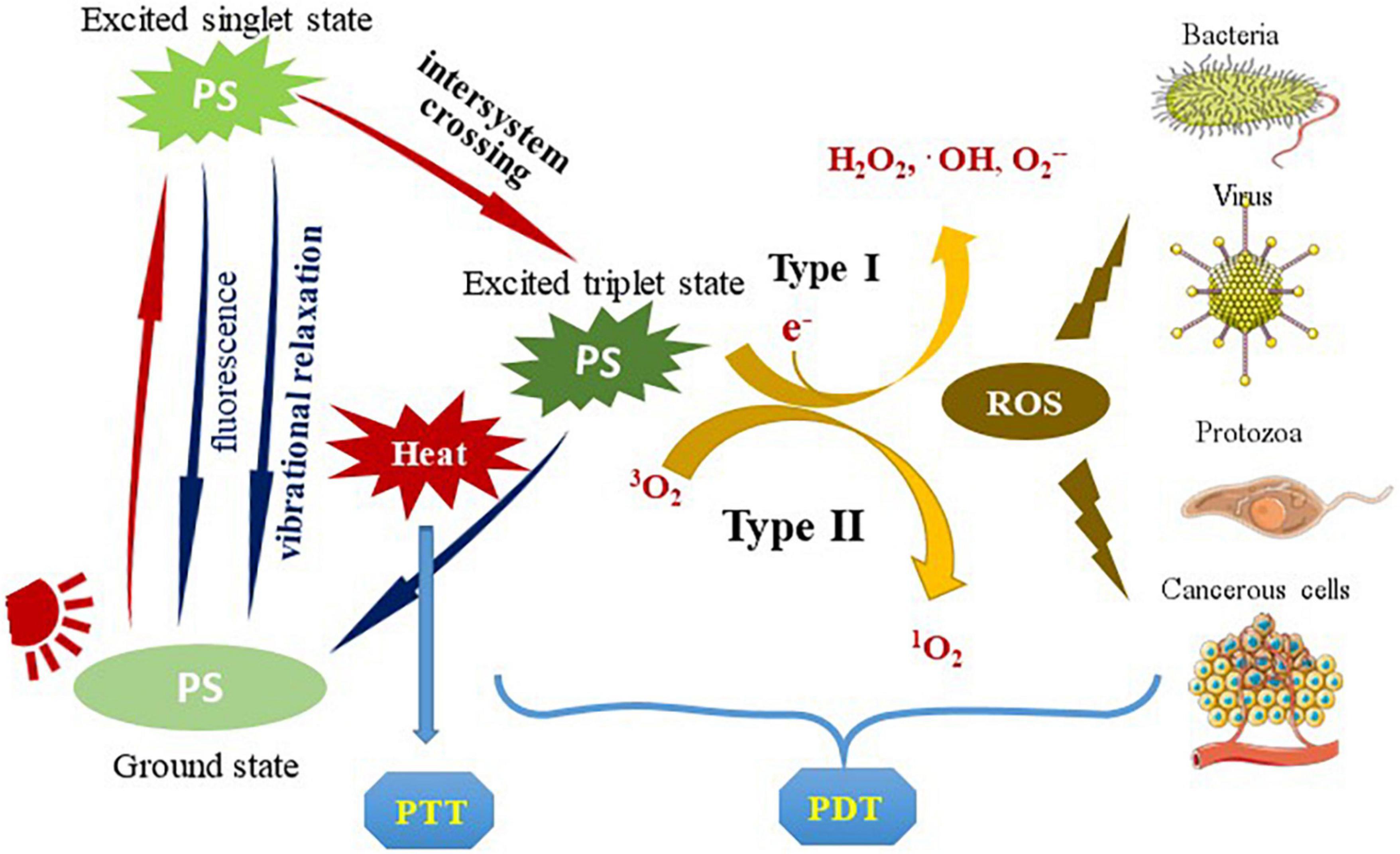
Figure 1. The types and principles of the photosensitizer-based therapies. PS is transformed into an excited singlet state after being irradiated by light. Partially return to the ground state by emitting fluorescence or non-radiative decay, or it can undergo intersystem crossing to the excited triplet state. It partially returns to the ground state by a radiative or non-radiative process, which is usually accompanied by photothermal conversion (used for PTT). The generation of ROS is subsequently promoted by two types of chemical reactions: type I mechanism generates free radicals and radical ions through electron transfer reactions; type II mechanism involves the reaction of PS with molecular oxygen, where the energy is transferred to the triplet ground state molecular oxygen to generate singlet oxygen. ROS acts on pathogenic microorganisms or tumor cells to produce photodynamic effects (used for PDT). PS, photosensitizer; PTT, photothermal therapy; ROS, reactive oxygen species; PDT, photodynamic therapy.
Properties, such as non-invasiveness, spatiotemporal selectivity, the possibility of the parallel application with other treatment, and good therapeutic effect make PDT and PTT become new options for tumor treatment (23). Compared with common antimicrobials, aPDT offers several advantages, including multi-target process, broad antibacterial spectrum, selective cytotoxicity against bacteria, and high bactericidal efficiency. Generally, irradiation is limited to the infected site with no systemic toxicity (24, 27). Another important advantage is that aPDT is independent toward the pattern of bacterial resistance to antibiotics, showing great potential for resistant bacteria (27, 28).
However, there are some side effects. The penetration of light through biological tissue is limited, meaning that PTT and PDT are generally ineffective for deep lesions. PTT may lead to a heat-shock response (23). PDT is generally well tolerated. Pain is the main adverse effect, which can be alleviated by adjusting the irradiation regimen (29). Other phototoxic lesions also manifest as erythema, edema, and sometimes urticaria, which usually subside quickly within a few days (14, 23, 30). Persistent erythema may develop for several months after treatment (31). Erosions, infection, and sterile pustules are also uncommon adverse effects (32, 33). Long-term adverse effects, such as pigment changes, scarring, are less common (31, 34). However, these drawbacks do not prevent PDT from becoming a promising non-invasive treatment for tumor and infection.
Anti-infection effects of the photosensitizer-based therapies
Antibiotic resistance is increasing globally. As a non-antibiotic process, the photosensitizer-based therapies are considered as attractive alternative treatment for microorganism eradication. ROS and singlet oxygen generated during the photodynamic process can lead to death of microorganisms by damaging cell membranes, changing the structure of microsomes and impairing certain functions, such as protein cross-linking and oxidative damage to nucleic acids (25, 27, 35, 36). A biofilm is a sessile microbial community that shelters microbes from a variety of environmental assaults, and biofilm-related pathogens can tolerate up to 1000-fold higher levels of antimicrobial agents (26, 37–39). In the subsequent sections, we describe the effective elimination of microorganisms, including bacteria, fungi, protozoa, and viruses, and their biofilms by photosensitizer-based therapies.
On human skin samples ex vivo, aPDT with SAPYR [2-((4-pyridinyl)methyl)-1H-phenalen-1-one chloride] (1 mM, 20 mW/cm2 for 25 min, light dose of 30 J/cm2) reduced the viability of methicillin-resistant Staphylococcus aureus (MRSA) by 3.9 ± 0.3 log10 orders. aPDT can effectively and safely kill bacteria on the skin surface, and it may have the potential to decolonize the skin in vivo (40). The photokilling potential of tribenzoporphyrazines had been assessed in vitro at 10 μM concentration and light dose of 30 J/cm2, aPDT reduce the growth of Streptococcus pyogenes by over 4.7 log10 and Staphylococcus epidermidis by over 5.7 log10, S. aureus growth by over 5.9 log10, as well as MRSA growth by 5.1 log10 (41). PTT has also been found to assist in the efficient killing of multidrug-resistant bacteria (39, 42). Kun Zhou reported a PS for synergistic aPDT and PTT, and it showed high efficiency in killing MRSA in a concentration-, irradiance-, and irradiative time dependence manner in vitro (25). Six S. aureus strains were treated with aPDT [5 μM photosensitizer 5,10,15,20-tetrakis(1-methylpyridinium-4-yl) porphyrin tetra-iodide and 40 W/m2 irradiance] and highly virulent strains were found to be more susceptible to aPDT than low virulent strains. Two virulence factors, free coagulase and enterotoxins A and C, were affected by aPDT, and the amount of active enterotoxin A and C was reduced at least 68%, indicating that aPDT is not only effective in inactivation of microorganism, but also in the degradation of the external virulence factors after release. Further, resistance did not developed after 10 consecutive cycles of treatment (43).
Trichophyton rubrum is the most common dermatophyte, and aPDT mediated by methylene blue (MB) could inhibit both spores and hyphae of T. rubrum in vitro, and the spores were more sensitive. The antifungal efficacy was positively correlated with the concentration of MB and light dose (44). Photodynamic effects on the viabilities of the biofilm of dermatophytes with employment of 0.1 mM MB and light-emitting diode (LED) (100 mW/cm2 for 10 min, total light dose of 60 J/cm2) were evaluated in vitro, and the colony forming units were reduced up to 4.6 log10 against the biofilms formed by T. rubrum, 4.3 log10 and 4.7 log10 against the biofilms formed by Trichophyton mentagrophytes and Microsporum gypseum. Additionally, the biofilm of dermatophytes became more susceptible to antifungal drugs after aPDT (37). The cell wall of Candida albicans is the main binding site of porphyrin-based PS. The cell membrane becomes permeable after irradiation, and PS can penetrate through these defects and crack into the cytoplasm, leading to cell death. Since the mechanism of action appears to be located in the cell wall and membrane, rather than in the cytoplasm, it is unlikely that C. albicans can provide resistance to aPDT (45). Overall, aPDT is an attractive alternative for fungal infections and is not prone to drug resistance.
Human papillomavirus infection is linked to cervical cancer and genital condyloma acuminatum. It has been reported that 5-aminolaevulinic acid-based PDT (ALA-PDT) inhibited the proliferation of HeLa cells and effectively reduced the viral load in a time-dependent and dose-dependent manner (46). Acanthamoeba is a protist pathogen that can cause blinding keratitis and fatal granulomatous amebic encephalitis. Mannose-conjugated porphyrins selectively targeted the surface membranes of pathogenic Acanthamoeba organisms, resulting in amebicidal effects and blocked excystation in vitro (47). 85 μM curcumin with light dose of 8 J/cm2 (450 nm, 36 mW/cm2 for 222 s) reduced the viability of Leishmania promastigotes, and it caused obvious changes in the cell morphology and induced apoptosis (48). Irrespective of bacteria, fungi, virus or protozoa, aPDT shows excellent anti-infective properties.
The clinical application of PDT as an anti-infective agent is still at a relatively early stage, and it seems to be effective in the treatment of superficial and local infections, including superficial and subcutaneous skin infections, dental diseases, ventilator-associated pneumonia, and gastric infections (49). Wound infection is the most common application. A total of 32 patients who had chronic ulcers were enrolled and randomized into placebo group or aPDT group mediated by PPA904 (3,7-bis (N, N-dibutylamino) phenothiazin-5-ium bromide). As a result, a reduction in bacterial load immediately post-treatment was observed in aPDT group (50). Another compelling application is acne vulgaris. It has been reported that PDT with 8% methyl aminolaevulinate (MAL), illuminated by 635 nm red light at light dose of 37 J/cm2, could effectively reduce the inflammatory lesions of severe acne vulgaris patients within 12 weeks (51). Due to the good clinical efficacy and low recurrence rate, ALA-PDT has been reported as a better choice for the treatment of genital warts (52). It can effectively clear latent or subclinical human papillomavirus infections by reducing viral loads (52, 53). In conclusion, aPDT successfully eliminate various pathogenic microorganisms in vitro, and show excellent anti-infective efficacy in clinical infectious diseases.
The effects of photosensitizer-based therapies on skin cells
Wound healing after skin injury involves complex interactions among multiple cells, primarily keratinocytes, vascular endothelial cells, fibroblasts, recruited immune cells, and the related extracellular matrix (ECM), and all of them are indispensable in the process (Figure 2). The type and concentration of the PS and the light dose affect the effect of PDT on cells (54, 55). With the increase concentration of 5-ALA and energy density, the viability of normal fibroblast cell decreases (56). The light dose of 84 J/cm2 is quite safe for keratinocytes at concentrations ranging from 4 to 161 μM indocyanine green (ICG). Most keratinocytes were damaged at any concentration of ICG when light dose of 252 J/cm2 was used (55). By properly optimizing the energy density and PS concentration, the damage of PDT to related cells can be minimized (55, 56). Thus, researches in the field of oncology therapy involve higher concentration of PS to kill tumor cells, whereas PDT for antimicrobial and wound healing only requires lower dose.
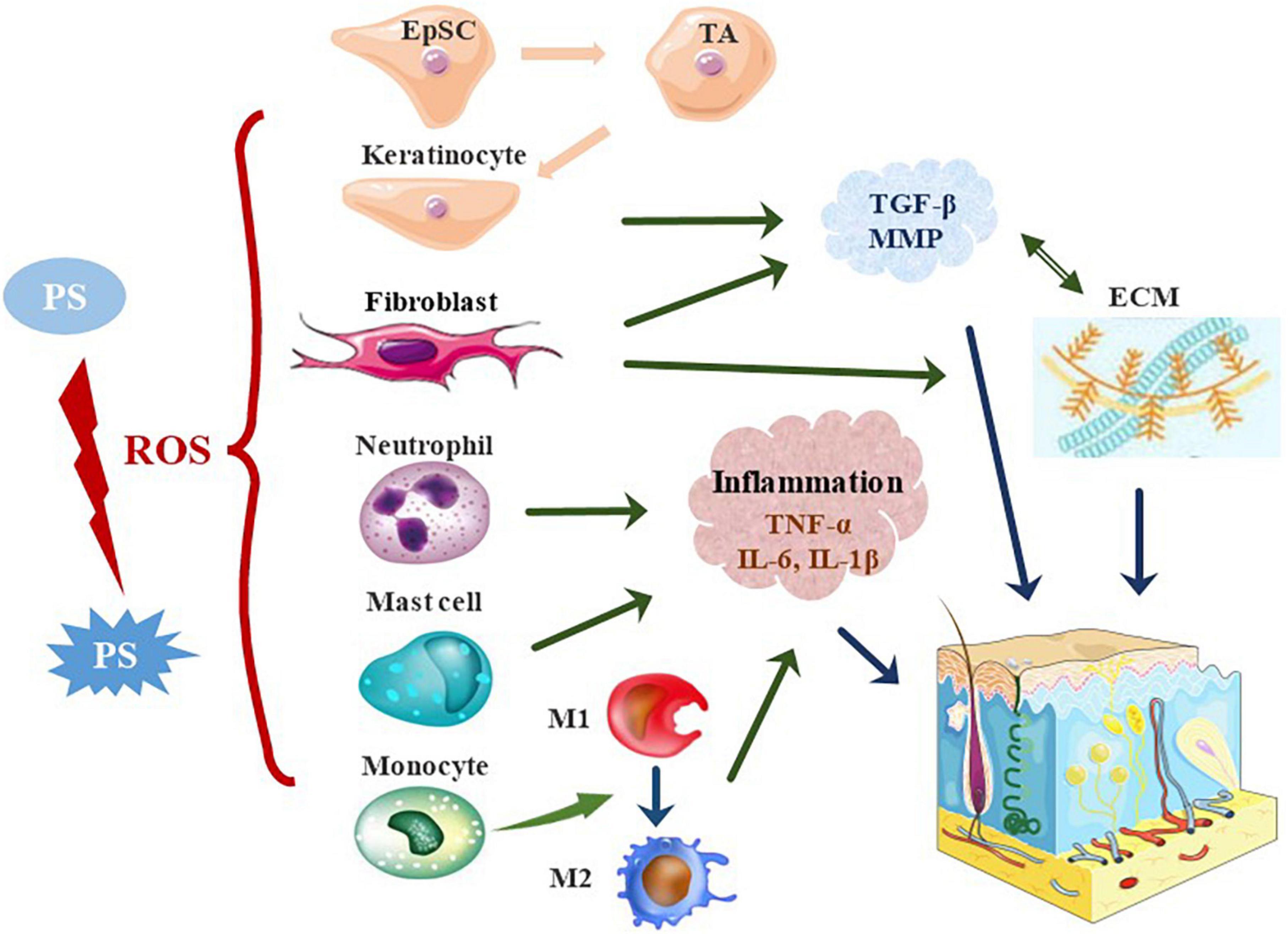
Figure 2. The effects of photosensitizer-based therapies on skin cells. In PDT, PS is activated to generate ROS, which promotes wound healing by stimulating skin resident cells and recruiting immune cells. PDT activates quiescent EpSCs to divide into proliferative TA cells, which promote re-epithelialization by enhancing their proliferation and migration. Besides, PDT stimulates the proliferation of keratinocytes and fibroblasts, and increases the secretion of TGF-β and MMP, thereby stimulating the production of collagen and elastin. PDT alters the local immune state and induces acute inflammatory responses in the early stages of trauma. PDT significantly affects the activation and migration of neutrophils. The neutrophil response is switched to that of monocyte-macrophages in the early stages of wound repair. The pro-inflammatory M1 phenotype is adopted in the early stages of injury and the anti-inflammatory M2 phenotype in the middle and late stages. PDT induces rapid recruitment of mast cells to further degranulate and release inflammatory molecules. EpSC, epidermal stem cells; TA cells, transient amplifying cells; ECM, extracellular matrix.
Resident cells
Under appropriate parameters, PDT could promote wound healing by directly affecting the functional behavior of skin resident cells (57–59). Significant proliferation of keratinocytes and fibroblasts, differentiation into myofibroblasts, and neovascularization after PDT have been observed in a number of studies in vivo and in vitro (60–65). PDT after injury appears to accelerate re-endothelialization and enhance the angiogenic response.
Re-epithelialization is a key event in wound healing, and it involves continuous proliferation, migration and differentiation of epidermal keratinocytes. The process requires the activation of quiescent epidermal stem cells (EpSC), which proliferate and migrate to the injured site and differentiate into stratified epidermis. Transient amplifying cells (TA cells) are the progeny cells of EpSC by asymmetric division with a proliferative capacity. ALA-PDT increased the proportion of TA cells and K14 expression. PDT could accelerate wound closure by enhancing EpSCs differentiation, proliferation and migration, thereby promoting re-epithelialization (66). 0.1 mM MAL irradiated with red light for 10 min (6.2 mW/cm2, 3.72 J/cm2) promoted the proper production of endogenous ROS, thereby effectively stimulating the proliferation of human immortalized keratinocytes, which may adjust the cell cycle process by activating Src kinase (67). PDT-conditioned medium containing interleukin 6 (IL-6) enhanced the migration of keratinocytes (68). ICG is a non-specific activator of the cystic fibrosis transmembrane conductance regulator. ICG-PDT conditioned medium collected from HaCaT cells exposed to 5 J/cm2 near-infrared light at 129 μM ICG activated cystic fibrosis transmembrane conductance regulator and enhanced the migration of HaCaT cells. The enhanced migration may be related to the activation of the cystic fibrosis transmembrane conductance regulator and the focal adhesion kinase pathway (69). Another study showed that ROS stimulated by ALA-PDT can inhibit the fibroblast growth factor receptor-2b pathway in downstream of protein kinase C, resulting in a decrease in IL-1α expression, and ultimately leading to keratinocyte differentiation and proliferation (70). Besides, PDT targeted to lysosomal membranes efficiently induced the differentiation of keratinocyte (71).
Fibroblast dysfunction is the key factor in the non-healing of chronic ulcers (72). Early activation of fibroblasts during PDT in ulcers has been observed (59, 62, 63). PDT at the ALA concentration of 0.1 mM, and light dose of 3 J/cm2 induced the production of intracellular ROS, thus it regulated prolonged extracellular signal-regulated kinase activation, and contributed to the fibroblast proliferation and activation (18). Fibroblast proliferation was enhanced by co-culture with keratinocytes treated with low-dose PDT (1 mM ALA, light dose of 1 J/cm2) (73). PDT with 38 μM ALA at light dose of 1 J/cm2 increased fibroblasts migration and closure of the scratch, which was more obvious in diabetic cells compared to normal cells (56). The proliferation and differentiation of fibroblast cells may be achieved by triggering phosphoinositol 3 kinase, protein kinase B phosphorylation, and extracellular signal-regulated kinase signaling pathways (60). Low-dose ALA-PDT (1 mM ALA, light dose of 1 J/cm2) can stimulate the transforming growth factor-beta1 (TGF-β1) in keratinocytes, which activated the TGF-β pathway in dermal fibroblasts to reshape the collagen in the dermis (73). Fibroblasts are an important source of the wound granulation tissue, and PDT enhances the functional activity of fibroblasts, thereby promoting wound repair.
The synthesis and degradation of the ECM are crucial in the process of wound remodeling. It is a highly dynamic structural network, which composes collagen, elastin, fibronectin, laminin and other glycoproteins. PDT apparently regulated the production of TGF-β during wound remodeling, which is significantly related to the reduction in the wound area (74–76). Skin wound healing model in vitro was used to evaluate the effects of PDT. The synthesis of collagen I and III was increased in the dermis around the healing wound, and the expression of matrix metallopeptidase 3 (MMP3), MMP19, p16, and α-smooth muscle actin (α-SMA) was significantly higher after treatment with 20% ALA and 20 J/cm2 of light dose (77). The increased expression of type I and III procollagen and MMP after ALA-PDT can be mediated not only by the direct effect on fibroblasts, but also by the indirect effect of keratinocyte-derived cytokines such as IL-1α and IL-6 (78, 79). In a photobiological stimulation study, the combined treatment with PS (aluminum phthalocyanine chloride) and light at intermediate dose (140 J/cm2) promoted collagen and elastin formation after 14 days, increasing up to 20% compared to the control sample. In addition, the expression of MMP-2 and MMP-9 increased accordingly (80). PDT induces changes in the ECM and its related molecules, which are essential for tissue repair.
Immune cells
Upon wounding, skin resident cells recognize damage-associated molecular patterns and pathogen-associated molecular patterns, along with a series of signaling and secreted chemokines, leading to further inflammation and recruitment of other immune cells (81, 82). Recent studies indicated that PDT altered the local immune state (74), inducing acute inflammatory response in the early stage of wound healing and reducing chronic inflammation in the late stages (83). More specifically, PDT induced the recruitment of immune cells, such as neutrophils, mast cells, monocytes or macrophages, to the wound site, thereby facilitating the removal of damaged tissue from the wound and inducing pro-inflammatory cytokines.
Neutrophils are the first immune cells to reach the wound bed. PDT significantly affected the activation of neutrophils (84, 85). The infiltration of neutrophils reached a peak at 4 h. At this time, the expression of E-selectin was also increased significantly, and it was correlated strongly with the number of neutrophils (86). MB-PDT can increase the adhesion of neutrophils, but not modify the release of myeloperoxidase (87). Fibroblasts initially responded to PDT by expressing heat shock proteins, triggerring the nuclear factor kappa B pathway, prompting macrophages to secrete pro-inflammatory cytokines [tumor necrosis factor alpha (TNF-α), IL-6, and IL-1β]. The resulting immune response was believed to promote neutrophil migration (88). The activation of inflammatory cells and production of pro-inflammatory cytokines amplified the acute inflammatory response, facilitated wound cleansing and promoted subsequent tissue regeneration (89, 90). In addition to neutrophils, other innate immune cells such as monocytes, macrophages, and mast cells, are also activated and accumulated at the wound site immediately after PDT.
An experimental study observed that the neutrophilic response switched to the reaction of monocyte-macrophage cells in the early stage of wound repair (91). Monocytes translocated to the sites of damage and differentiate into macrophages upon activation. Macrophages play the key role in all stages of wound healing, adopt a pro-inflammatory M1 phenotype at the early stage of injury, while as anti-inflammatory M2 in the middle and later stages (90). A wide variety of cytokines are secreted to bridge the two roles (82, 90). It was found that the change in macrophages was consistent with the proportion of inflammatory factor (IL-1β, TNF-α)/growth factor (TGF-β1, vascular endothelial-derived growth factor) (83).
Mast cells are essential for controlling the bacterial load and wound healing (92). ALA-PDT could induce the rapid recruitment of mast cells, and cause further degranulation and release of inflammatory molecules, thereby stimulating the peripheral nerves to promote healing (93). A controlled study confirmed the important role of mast cells in PDT for ulcers, and immunohistochemical analysis revealed an increase in the number of mast cell, degranulation index, and the expression of the basic fibroblast growth factor (63). The increase of plasmacytoid dendritic cells, MHC-II expression, TNF-α positive mast cell expression, TGF-β expression, and CD4+/CD25+ regulatory T cells was observed on immunofluorescence staining in another study (74). Previous studies have shown that PDT cause beneficial activation of the innate immune system to promote wound healing, but its role in the adaptive immune response remains to be explored.
The effects of the photosensitizer-based therapies on animal models
Recently, the potential of PDT in tissue regeneration and wound healing has attracted the attention of many research groups. Before applying PDT to human clinical trials, many in vivo studies have been carried out using various animal models, including those of burns, excisions, and abrasions (Figure 3). It is worth noting that most of the wounds researched are infected. Bacterial infection will promote inflammation and produce virulence factors, which could aggravate the destruction of local tissue and delay healing (4, 6).
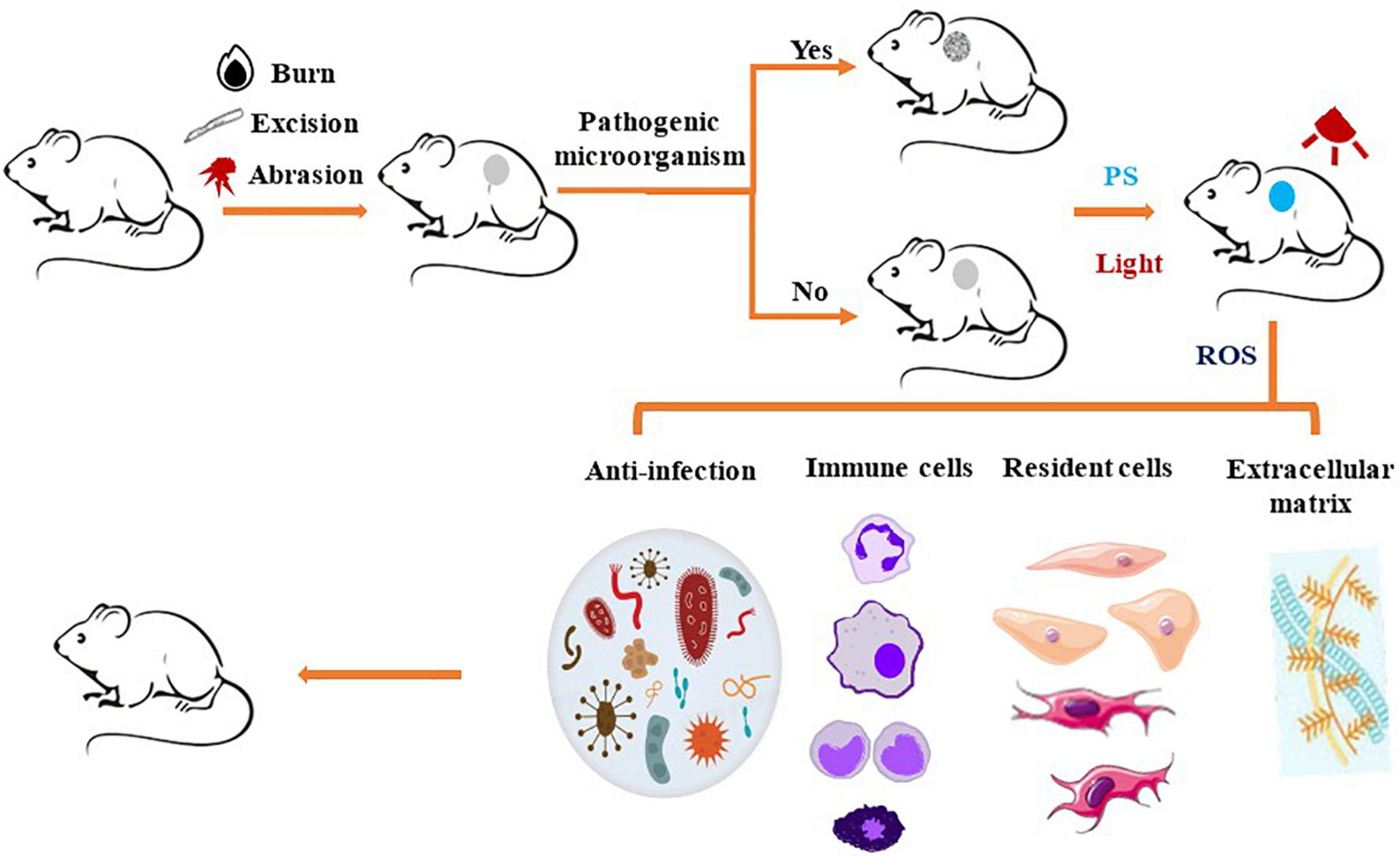
Figure 3. Schematic representation of photosensitizer-based therapies in animal wound model. The animals receive a burn, excision, or abrasion wound, which is uninfected or infected by pathogenic microorganisms. Next, PS is applied to the wound, then irradiated with a light source that transfers energy to generate ROS or heat, which remove various pathogenic microorganisms from wound surface. PDT induced the recruitment of immune cells, such as neutrophils, mast cells, monocytes or macrophages, to the wound site, thereby promoting removal of the damaged tissue from the wound and induction of proinflammatory cytokines. PDT also stimulates skin resident cells to promote re-epithelialization and deposition of extracellular matrix to promote wound healing.
In a burn wound, recent study indicated that local and transient ROS production created by MAL-PDT (light dose of 2.5 J/cm2) could regulate the function of stem cells and tissues and activate cell proliferation in the niche of mouse skin and hair follicle stem cells, thereby promoting hair growth and wound healing (59). A study evaluated the effect of phototherapy on the healing process through clinical and histopathological analysis, and it showed the promotion of red laser, infrared, MB-PDT, and green LED on healing of third-degree burns in rats. Compared with untreated controls, PDT with 1.56 μM MB and 10 J/cm2 light dose stimulated the production and maturation of collagen, and the wound receded more (94). Another histological study in rats proved that PDT mediated by toluidine-O blue acted as a biostimulant adjuvant, balancing the adverse effects of burns during the wound healing process. It mainly acted on the early stage of healing, accelerated inflammation, promoted cell proliferation and chemotaxis, and increased collagen deposition. Besides, the formation of new blood vessels ranging from moderate to strong was also observed (95). The beneficial effect of phototherapy was obvious in the re-epithelialization and collagen matrix deposition, and the presence of PS accelerated this process. Smart hydrogel-based porphyrin photosensitizer sinoporphyrin sodium/basic fibroblast growth factor nanohybrids was developed and used for aPDT in the infected wound. Under mild light dose of 30 J/cm2, 8 μM material (quantified by sinoporphyrin sodium concentration) eradicated almost 99.99% of S. aureus and MRSA in vitro and promoted the healing of burn wound in mice (96). Whether the wound is infected or not, PDT is beneficial to the healing of burn wounds.
Excision is another common type of wound. Acute infection models in mice were established after full-thickness skin excision and then treated separately, it was found that the burden of Pseudomonas aeruginosa was significantly reduced in ALA-PDT group. In addition, further exploration of the mechanism showed that PDT not only killed bacteria, but promoted the healing of skin infection wounds by regulating inflammatory factors, affecting the polarization state of macrophages, and promoting granulation tissue formation, angiogenesis, collagen regeneration and remodeling (83). A study on wounds of diabetic mice showed that PDT with 10% ALA at light dose of 25 J/cm2 accelerated the rate of wound closure (97). After the excellent bactericidal properties of selenoviologen against Escherichia coli and S. aureus were verified in vitro, it was loaded into a hydrogel patch and exposed to 90 mW/cm2 white light for 60 min on infected excised wounds in mice. After 15 days, the wound area was significantly reduced in aPDT group. Researchers believed that the excellent antibacterial property created a crucial local and systemic immune microenvironment for tissue regeneration, thus playing an important role in skin regeneration process (98). Obviously, PDT exerts an anti-bactericidal effect on wound infection, which may promote wound healing.
A mouse model of abrasion inoculated with C. albicans was developed, and aPDT was performed with 400 μM phenothiazinium salt and red light at light irradiance of 32.5 mW/cm2. Whether aPDT initiated at either 30 min or 24 h after infection, the weakening of microbial luminescence and significantly reduced burden of C. albicans could be observed. This indicated that aPDT could be used to prevent and treat superficial C. albicans-infected wounds (99). Another study used MB-aPDT (light dose of 74 J/cm2 in 635 nm), MB-aPDT and daily topical mupirocin in combination or only mupirocin to treat superficial skin infection wounds in mice. Superficial contraction at 24 h was observed after MB-aPDT. Also, a larger area reduction at 48 h, faster crust detachment, fewer scales and no scars were noted. The results showed that MB-aPDT induced the best wound healing. Although the addition of mupirocin to MB-aPDT improved the antibacterial activity, it did not promote wound healing (100). Most studies on abrasion wounds focus on the antibacterial property of PDT and significant sterilization effect is observed after treatment.
Regardless of the type of wound, PDT can effectively promote healing. There was a synergetic effect among light, PS and delivery drug on tissue healing, and PDT will not cause any healing inhibition during the process (101, 102). However, there are some different opinions. Delayed wound re-epithelialization was observed in the second week of PDT treated with 20% MAL and 100 mW/cm2 of light irradiance. Immunohistochemical analysis revealed fewer CD31+ blood vessels after irradiation and no difference in collagen I density between treated and untreated wounds. Therefore, it was concluded that PDT delayed acute wounds healing in mice (103). This difference may be caused by the different power of the irradiation. Different studies used different PS and irradiation. Low-level irradiation was shown to have a stimulating effect, while high-level radiation could have an inhibitory effect on cells (54, 55, 104, 105). This study used a relatively large irradiance. In addition, the observation period was short, only 2 weeks. Other study indicated that PDT was associated with a smaller wound area in the remodeling period after 3 weeks (75). If the observation period could be extended, the results may be different.
The effects of the photosensitizer-based therapies on human skin wounds
In view of the excellent sterilization effect shown by PDT in vitro and in animal studies, as well as the biostimulation of skin cells and promotion of wound healing, it has been applied to the study of human wounds. The clinical studies involve a variety of wounds, including chronic leg ulcers (venous, arterial, mixed, or diabetic), diabetic foot ulcers, acute excised wounds, and refractory ulcerative lesions of other diseases, such as cutaneous leishmaniasis (CL), lipid necrosis (NL), and pemphigus vulgis.
Chronic leg ulcers are common but challenging, and PDT is gradually applied to chronic leg ulcers in clinical practice (Table 1). More than one third of the studies in Table 1 (1st, 2nd, 3rd, and 6th) used 20% ALA as PS. Nineteen patients affected by venous leg ulcer (VLU) were managed with standard of care in addition to weekly ALA-PDT (light dose: 80 J/cm2). Wounds were significantly and gradually reduced after three sessions of treatment, and increased TGF-β was significantly associated with wound reduction (74). After PDT, mast cell degranulation index increased and the expression of neuron mediators increased. It was preliminarily suggested that ALA-PDT may promote the healing of VLU by stimulating quiescent peripheral nerves, possibly following the release of inflammatory molecules through granulated mast cells (93). Ten patients in the sixth study of Table 1 underwent 10 sessions of PDT (light dose: 80 J/cm2) with 20% ALA. At 8-month follow-up, four patients achieved complete remission, and four patients had more than 50% reduction in wound size (Figure 4) (106). To compare the efficacy of PDT using 20% ALA vs. red light alone (630 nm, 80 J/cm2) for chronic skin ulcers infected with P. aeruginosa, treated weekly with standard wet dressings during the treatment and routine care dressings for the rest time. PDT combined with care dressings demonstrated better antibacterial and healing effects (8). PDT containing 0.5% ALA and 0.005% EDTA-2NA (light dose: 10 J/cm2) was used to treat skin ulcers infected with MRSA and P. aeruginosa. Simple daily debridement was also allowed, and continuous PDT treatment for several days resulted in 40% reduction in wound area in six out of seven patients (10). 2% ALA was also applied to three cases of chronic ulcers that failed to respond to conventional treatment and the wounds healed after 1–3 sessions of PDT (20 mW/cm2 for 16.7 min, light dose: 20 J/cm2) without recurrence for more than 29 months (68).
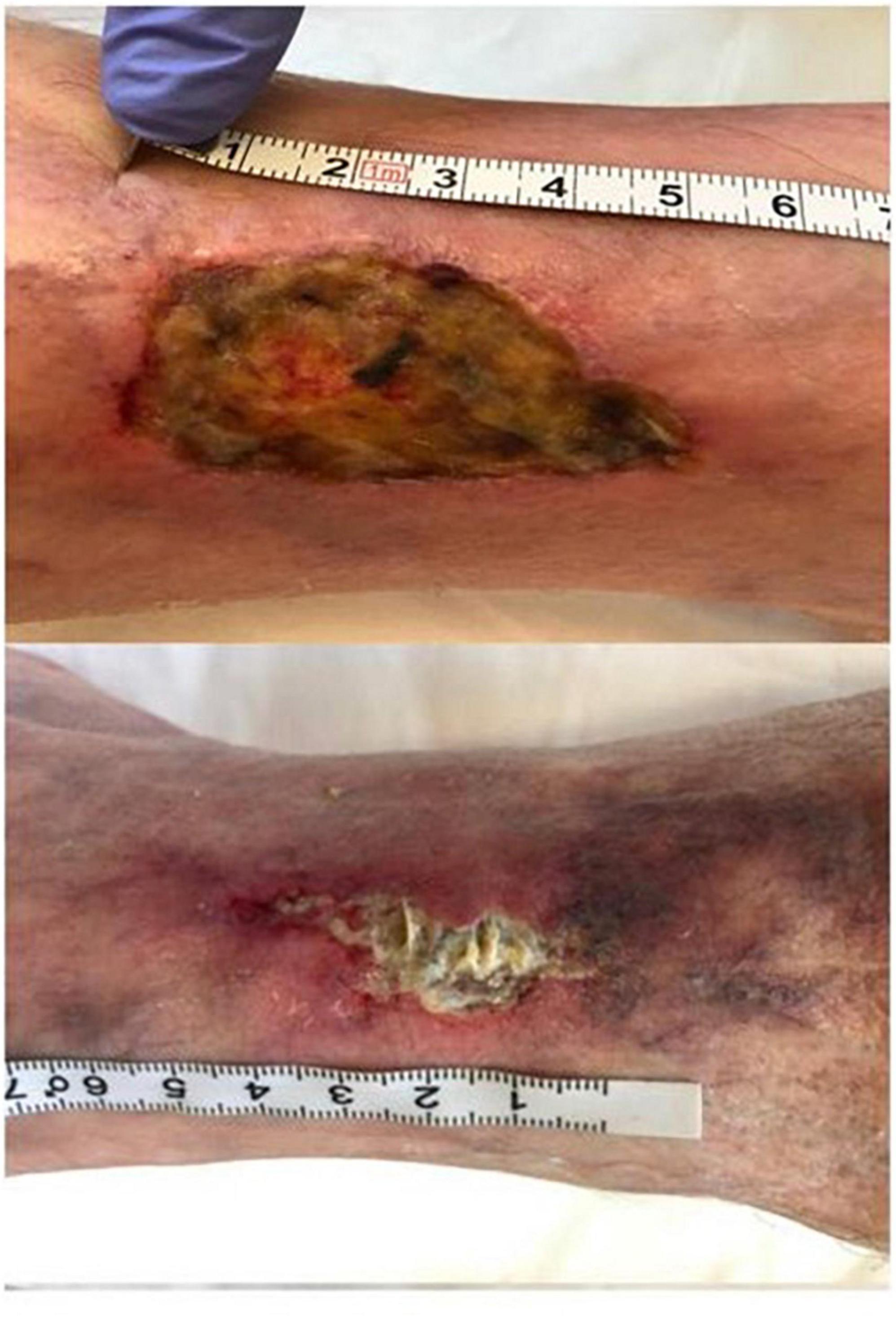
Figure 4. Aminolaevulinic acid-photodynamic therapy (ALA-PDT) for the infected leg ulcer. A patient with chronic foot ulcer before and after 3 cycles of PDT (figure adapted from the reference 107 with no change).
Other types of PS have also been applied to the treatment of chronic leg ulcers. RLP068-PDT (total light dose: 60 J/cm2) was used to treat 36 cases of infected leg ulcer with different pathophysiology. Except for four patients who reported severe pain, other patients were well tolerated. The results showed that PDT could effectively reduce bacterial load without any systemic or local antibiotics, ensuring the smooth progress of follow-up treatment (107). VLU was treated with 4% MAL combined with red light (630 nm, light dose: 18 J/cm2), and PDT was performed at 3-week interval. After treatment, the ulcers were covered with cold water and chamomile-soaked gauze and aloe vera gel twice a day for 10 days. All the patients healed from ulcers completely in a mean of 24 weeks (Figure 5) (108). Macromorphometric assessments using two measurements to track the repair process showed that MB-PDT (0.01% MB, 6 J/cm2) in combination with conventional drug (collagenase/chloramphenicol) accelerated wound healing (109). Combination therapy with PDT based on different PS has beneficial effects on chronic leg ulcers.
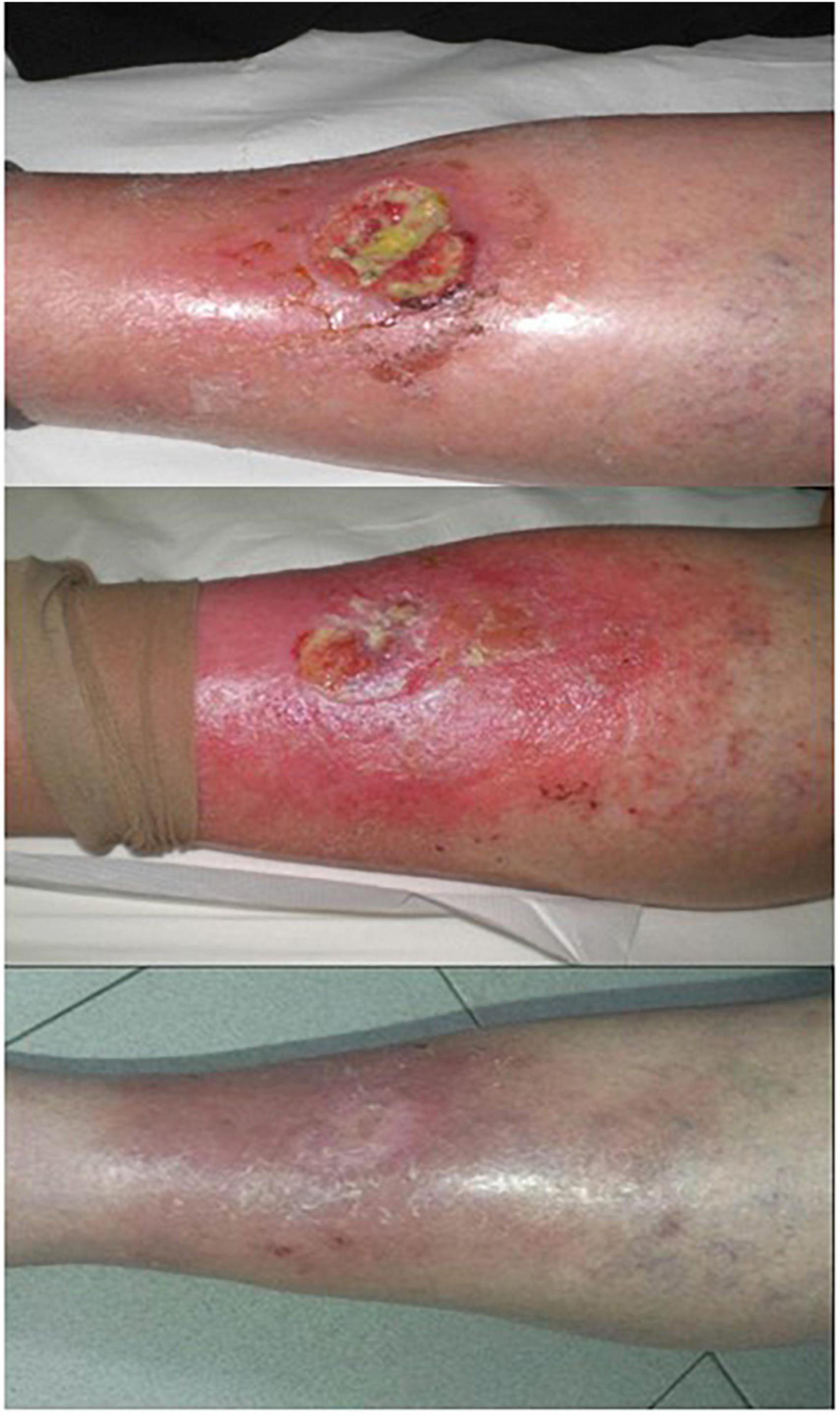
Figure 5. Effective use of PDT on chronic leg ulcer. Up: a 67 years old woman presenting a chronic leg ulcer on the left tibia. Middle: almost complete healing of the ulcer after five treatments (15 weeks). Down: complete healing of the ulcer in 12-month follow-up (figure adapted from the reference 109 with permission of John Wiley and Sons).
Diabetes foot ulcer (DFU) is an increasingly serious public health problem, and the treatment is very difficult. Infection is the most common complication associated with delayed healing and increased risk of amputation (110). The prognosis of DFU treated with PDT combined with systemic antibiotics was better (111). The clinical study of PDT on DFU is shown in Table 2. A randomized, double-blind, placebo-controlled phase IIa trial was conducted in 62 patients with DFU. PDT (light dose: 60 J/cm2) with different concentrations of RLP068 (0.10, 0.30, and 0.50%) was used as a supplement to the systemic treatment of amoxicillin and clavulanic acid. The results showed that RLP068-PDT as add-on to systemic antibiotic treatment reduced the bacterial load, and the total microbial load decreased in a dose-dependent manner (112). In a multicenter study, 64 patients with DFU received repeated PDT (red light irradiation for 8 min, 60 J/cm2) to study the effect of RLP068 on bacterial load and ulcer healing. After a single treatment, the bacterial load was significantly reduced, and the benefit lasted for 2 weeks. During follow-up, even patients infected with gram-negative bacteria or exposed bones had a significant reduction in ulcer area (113). 16 patients with chronic leg ulcer and 16 patients with DFU were recruited into a placebo-controlled, single treatment phase IIa trial. 0.026% PPA904 or placebo was applied topically and immediately irradiated with 50 J/cm2 red light. Both groups received standard bandaging therapy. All patients were well tolerated. Compared with placebo, patients showed a reduction in bacterial load immediately after PDT. After 3 months, 50% patients of PDT group healed completely, while only 12% in placebo group. A significant reduction in bacterial load and a clear trend in wound healing was observed in the study (50). However, Phase IIa clinical studies are mainly exploratory trials of efficacy with a small number of subjects included. No Phase III clinical study with more cases has been reported yet. Further researches in this area need a larger number of patients.
Photodynamic therapy has also been applied to other types of ulcers (Table 3). MAL-PDT (irradiance by 80 mW/cm2 red light for 9 min, total light dose: 37 J/cm2) affected the healing of human skin excision wounds. Resecting the wound during the inflammatory phase, matrix remodeling and cosmetic effects/skin structure showed that wound re-epithelialization delayed at 7 days after MAL-PDT, with smaller wounds at 3 weeks, and greater and more ordered collagen and elastin deposition at 9 months (75). These results suggested that MAL-PDT had a positive stimulation of the inflammatory phase, matrix remodeling, and ultimately producing improved dermal matrix architecture.
The main manifestation of CL was scar skin ulcer. 31 patients with CL received daylight activated PDT (DA-PDT). Fourteen patients were treated in the hospital, coated with 16% MAL, and then exposed to the sun for 2.5 h. Others received self-management treatment at home. The treatment was repeated weekly until clinical and microbiological cure. The total cure rate was 89%, DA-PDT had been proved to be effective in the treatment of CL (114). A 15-year-old boy with leg ulcer received PDT. He was previously resistant to pentavalent antimony, oral fluconazole and local ketoconazole. His ulcer healed completely after repeated ALA-PDT (Figure 6) (115). Compared with systemic therapy, this method should be considered as an attractive antiparasitic treatment, especially for drug-resistant cases. A 44-year-old woman with extensive ulcerative NL received local ALA-PDT (10% ALA, 160 mW/cm2 of light irradiance for 8 min with total light dose of 75 J/cm2) every 2 weeks. At the end of six sessions, complete healing of skin ulcer was observed, and erythema was significantly improved in all treatment sites (116). MAL was applied to the persistent ulcer in the neck of patients with pemphigus vulgaris. After two sessions of PDT (635 nm, light dose: 37 J/cm2), it healed completely (Figure 7) (117). MAL-PDT may be a useful adjunct for the treatment of refractory ulcer in patients with pemphigus vulgaris.
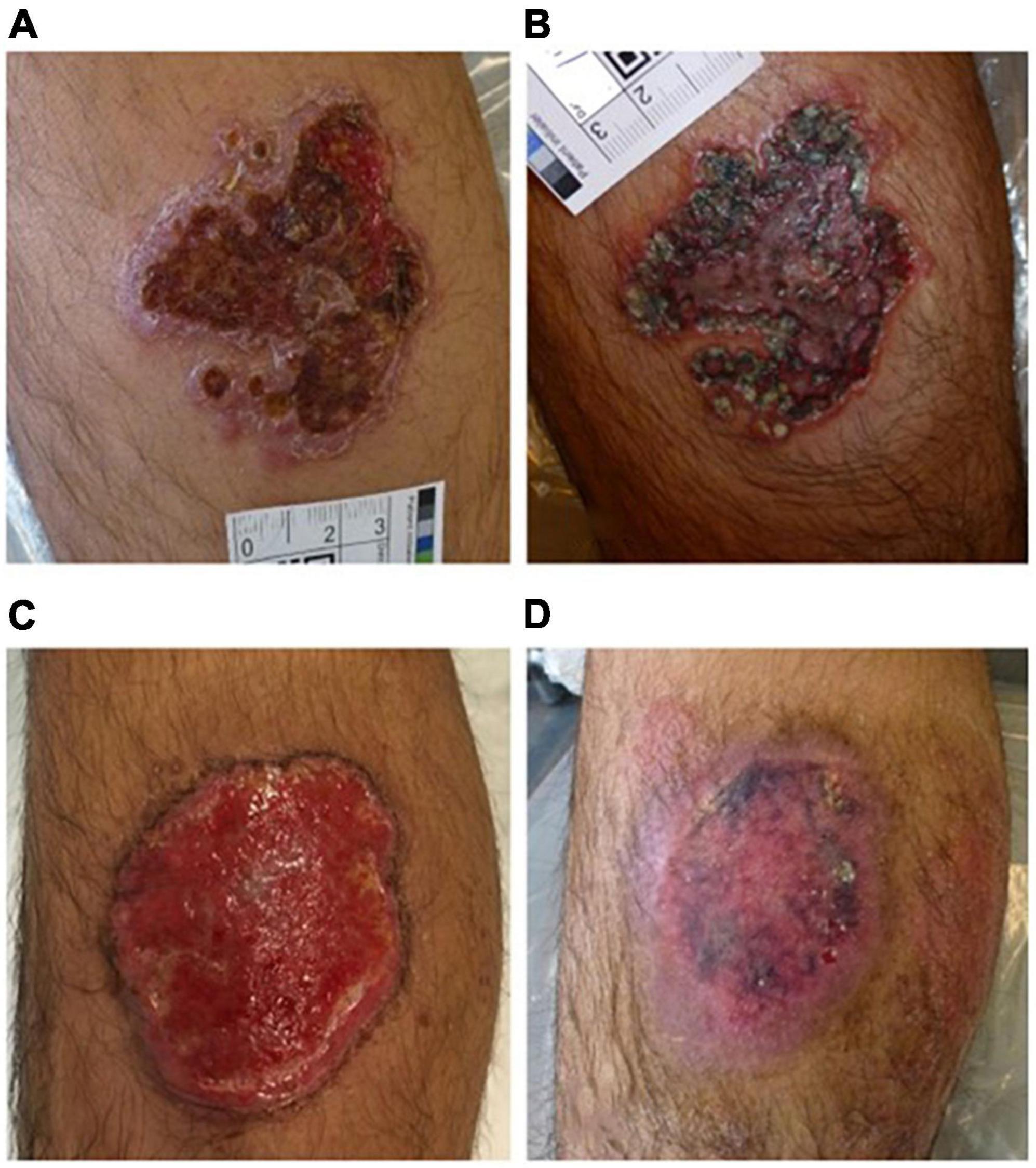
Figure 6. Effective treatment with PDT of cutaneous leishmaniasis. (A) Original lesion before treatment, (B) after oral fluconazole and topical ketoconazole, (C) after 12 PDT treatments, (D) 1 month after PDT treatment (figure adapted from the reference 116 with permission of John Wiley and Sons).
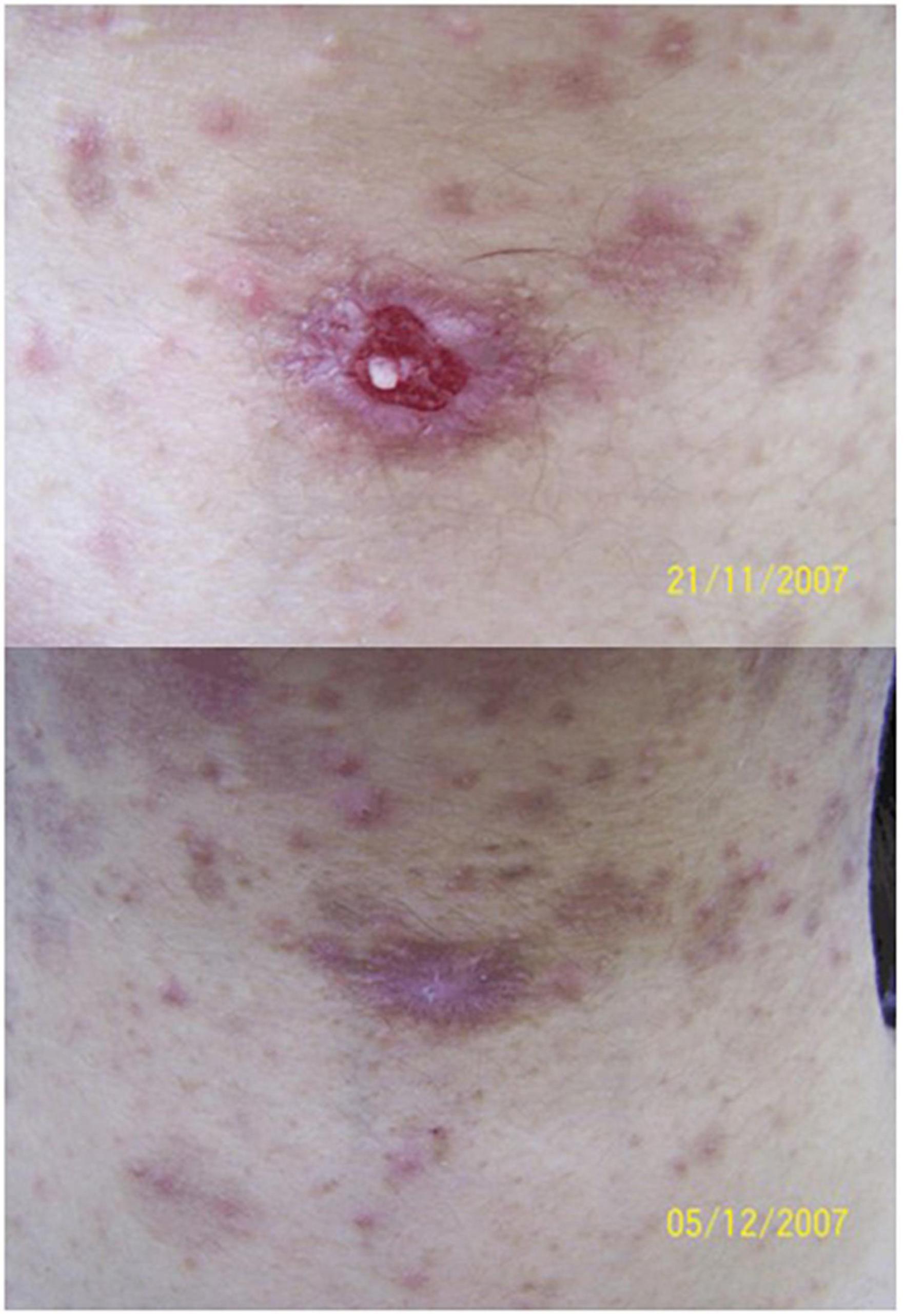
Figure 7. Methyl aminolaevulinate-photodynamic therapy (MAL-PDT) for recalcitrant ulcer in pemphigus vulgaris. Up: recalcitrant ulceration on the cervical region in a patient with pemphigus vulgaris. Down: complete closure of the ulceration 1 week after two sessions of MAL-PDT (figure adapted from the reference 118 with permission of John Wiley and Sons).
The formation of sinuses in chronic skin ulcers complicates the treatment. Seven patients with skin ulcers with sinus tract formation received PDT with 20% ALA every 10 days. They had previously received systemic antibiotic therapy, debridement and dressing change treatment, and there was no significant improvement. The light irradiance was 84 mW/cm2, and the light dose was 100 J/cm2. After 1–5 sessions, six patients completely cured after receiving the combination of ALA-PDT and antibiotics. In another patient, the sinus cured and the ulcer area reduced. There was no serious discomfort during the treatment, and all patients were satisfied with the effect (118). ALA-PDT combined with antibiotics decreases the treatment time for skin ulcers and sinuses, reduces the pain of patients, and it has become a promising method for the treatment of refractory skin ulcers and sinuses.
It is worth noting that all above studies show the benefit of PDT on wound healing, especially for some complex and difficult-to-heal chronic ulcers, which are usually resistant to conventional treatments. However, some limitations should be recognized. The clinical data are not solid. Some studies are only case report or case series. The sample size is small and RCT studies are limited. Different PS are used, such as ALA, MAL, MB, curcumin, etc., the treatment schemes vary, treatment cycles ranging from one to several times. Some studies use PDT in combination with standard care, some with antibiotics, and some apply PDT alone. No standardized treatment plan yet. Lack of comparative study on the efficacy of PDT with different PS. In the future, more large samples and multicenter clinical studies are needed to explore the role of PDT in wound healing.
Conclusion
Besides the excellent sterilization effect, PDT can amplify acute inflammation, stimulate skin resident cells to proliferate, migrate and secrete cytokines, accelerate the proliferation of blood vessels and the deposition of ECM (elastin and collagen), thereby promoting the skin regeneration process. However, various PS have been used in different in vitro experiments and animal experiments, only several PS have been used clinically. In the future, more research is needed to explore the more detailed molecular mechanism of this therapy, develop a wider variety of PS, and explore clinical drug regimens to ensure the maximum efficacy and safety of this effective clinical tool. In view of its excellent antibacterial properties and its role in promoting wound healing, the emergence of PDT will reduce the application of antibiotics, slow down the emergence of drug-resistant strains, and provide a promising weapon for the treatment of refractory skin ulcers.
Author contributions
XN wrote the manuscript and created the figures. WZ and GH revised the manuscript. YX conceived and revised the manuscript. All authors reviewed the manuscript.
Funding
This study was supported by the National Natural Science Foundation of China (Projects Nos. 82173445 and 81172590) and the Innovation Capability Support Plan of Shaanxi Province (No. 2019TD-034).
Conflict of interest
The authors declare that the research was conducted in the absence of any commercial or financial relationships that could be construed as a potential conflict of interest.
Publisher’s note
All claims expressed in this article are solely those of the authors and do not necessarily represent those of their affiliated organizations, or those of the publisher, the editors and the reviewers. Any product that may be evaluated in this article, or claim that may be made by its manufacturer, is not guaranteed or endorsed by the publisher.
Abbreviations
PDT, photodynamic therapy; aPDT, antimicrobial photodynamic therapy; PTT, photothermal therapy; ROS, reactive oxygen species; PS, photosensitizer; MRSA, methicillin-resistant Staphylococcus aureus; MB, methylene blue; LED, light-emitting diode; ALA, aminolaevulinic acid; MAL, methyl aminolaevulinate; ECM, extracellular matrix; ICG, indocyanine green; EpSC, epidermal stem cells; TA cells, transient amplifying cells; MMP, matrix metallopeptidase; IL, interleukin; TGF- β, transforming growth factor- β; α-SMA, α-smooth muscle actin; TNF- α, tumor necrosis factor alpha; CL, cutaneous leishmaniasis; NL, necrobiosis lipoidica; VLU, venous leg ulcer; DFU, diabetic foot ulcer; DA-PDT, daylight-activated photodynamic therapy; RCT, randomized controlled trial.
References
1. Martin P, Nunan R. Cellular and molecular mechanisms of repair in acute and chronic wound healing. Br J Dermatol. (2015) 173:370–8. doi: 10.1111/bjd.13954
2. Castano O, Perez-Amodio S, Navarro-Requena C, Mateos-Timoneda MA, Engel E. Instructive microenvironments in skin wound healing: Biomaterials as signal releasing platforms. Adv Drug Deliv Rev. (2018) 129:95–117. doi: 10.1016/j.addr.2018.03.012
3. Rodrigues M, Kosaric N, Bonham CA, Gurtner GC. Wound healing: A cellular perspective. Physiol Rev. (2019) 99:665–706. doi: 10.1152/physrev.00067.2017
4. Martin JM, Zenilman JM, Lazarus GS. Molecular microbiology: New dimensions for cutaneous biology and wound healing. J Invest Dermatol. (2010) 130:38–48. doi: 10.1038/jid.2009.221
5. Johnson TR, Gomez BI, McIntyre MK, Dubick MA, Christy RJ, Nicholson SE, et al. The cutaneous microbiome and wounds: New molecular targets to promote wound healing. Int J Mol Sci. (2018) 19:2699. doi: 10.3390/ijms19092699
6. Caldwell MD. Bacteria and antibiotics in wound healing. Surg Clin North Am. (2020) 100:757–76. doi: 10.1016/j.suc.2020.05.007
7. Kalan LR, Brennan MB. The role of the microbiome in nonhealing diabetic wounds. Ann N Y Acad Sci. (2019) 1435:79–92. doi: 10.1111/nyas.13926
8. Lei X, Liu B, Huang Z, Wu J. A clinical study of photodynamic therapy for chronic skin ulcers in lower limbs infected with Pseudomonas aeruginosa. Arch Dermatol Res. (2015) 307:49–55. doi: 10.1007/s00403-014-1520-4
9. Mancusi R, Nosso G, Pecoraro S, Barricelli M, Russo A. Photodynamic therapy with RLP068 and 630-nm red led light in foot ulcers in patients with diabetes: A case series. Int J Low Extrem Wounds. (2021) 15347346211053403. doi: 10.1177/15347346211053403 [Epub ahead of print].
10. Shiratori M, Ozawa T, Ito N, Awazu K, Tsuruta D. Open study of photodynamic therapy for skin ulcers infected with MRSA and Pseudomonas aeruginosa. Photodiagnosis Photodyn Ther. (2021) 36:102484. doi: 10.1016/j.pdpdt.2021.102484
11. Vallejo MCS, Moura NMM, Gomes A, Joaquinito ASM, Faustino MAF, Almeida A, et al. The role of porphyrinoid photosensitizers for skin wound healing. Int J Mol Sci. (2021) 22:4121. doi: 10.3390/ijms22084121
12. Daniell MD, Hill JS. A history of photodynamic therapy. Aust N Z J Surg. (1991) 61:340–8. doi: 10.1111/j.1445-2197.1991.tb00230.x
13. Morton CA, Szeimies RM, Basset-Seguin N, Calzavara-Pinton P, Gilaberte Y, Haedersdal M, et al. European dermatology forum guidelines on topical photodynamic therapy 2019 part 1: Treatment delivery and established indications – actinic keratoses, Bowen’s disease and basal cell carcinomas. J Eur Acad Dermatol Venereol. (2019) 33:2225–38. doi: 10.1111/jdv.16017
14. Morton CA, Szeimies RM, Basset-Seguin N, Calzavara-Pinton PG, Gilaberte Y, Haedersdal M, et al. European dermatology forum guidelines on topical photodynamic therapy 2019 part 2: Emerging indications – field cancerization, photorejuvenation and inflammatory/infective dermatoses. J Eur Acad Dermatol Venereol. (2020) 34:17–29. doi: 10.1111/jdv.16044
15. Szeimies RM, Karrer S. [Photodynamic therapy-trends and new developments]. Hautarzt. (2021) 72:27–33. doi: 10.1007/s00105-020-04737-6
16. Qi M, Chi M, Sun X, Xie X, Weir MD, Oates TW, et al. Novel nanomaterial-based antibacterial photodynamic therapies to combat oral bacterial biofilms and infectious diseases. Int J Nanomedicine. (2019) 14:6937–56. doi: 10.2147/IJN.S212807
17. Wen X, Li Y, Hamblin MR. Photodynamic therapy in dermatology beyond non-melanoma cancer: An update. Photodiagnosis Photodyn Ther. (2017) 19:140–52. doi: 10.1016/j.pdpdt.2017.06.010
18. Jang YH, Koo GB, Kim JY, Kim YS, Kim YC. Prolonged activation of erk contributes to the photorejuvenation effect in photodynamic therapy in human dermal fibroblasts. J Invest Dermatol. (2013) 133:2265–75. doi: 10.1038/jid.2013.25
19. Park JY, Jang YH, Kim YS, Sohn S, Kim YC. Ultrastructural changes in photorejuvenation induced by photodynamic therapy in a photoaged mouse model. Eur J Dermatol. (2013) 23:471–7. doi: 10.1684/ejd.2013.2050
20. Park MY, Sohn S, Lee ES, Kim YC. Photorejuvenation induced by 5-aminolevulinic acid photodynamic therapy in patients with actinic keratosis: A histologic analysis. J Am Acad Dermatol. (2010) 62:85–95. doi: 10.1016/j.jaad.2009.06.025
21. Croix J, Burge S, Chwalek J, Gmyrek R, Chapas A. Split-sided chest study of skin rejuvenation comparing low-energy, 1,927-nm thulium fractional laser treatment prior to photodynamic therapy versus photodynamic therapy alone. Lasers Surg Med. (2020) 52:53–60. doi: 10.1002/lsm.23178
22. Jesus P. Low level energy photodynamic therapy for skin processes and regeneration. In: Tanaka Y editor. PhotomediCine-advances in clinical practice. (London: IntechOpen) (2017). p. 75–94. doi: 10.5772/65344
23. Li X, Lovell JF, Yoon J, Chen X. Clinical development and potential of photothermal and photodynamic therapies for cancer. Nat Rev Clin Oncol. (2020) 17:657–74. doi: 10.1038/s41571-020-0410-2
24. Cieplik F, Deng D, Crielaard W, Buchalla W, Hellwig E, Al-Ahmad A, et al. Antimicrobial photodynamic therapy – what we know and what we don’t. Crit Rev Microbiol. (2018) 44:571–89. doi: 10.1080/1040841X.2018.1467876
25. Zhou K, Qiu X, Xu L, Li G, Rao B, Guo B, et al. Poly(selenoviologen)-assembled upconversion nanoparticles for low-power single-NIR light-triggered synergistic photodynamic and photothermal antibacterial therapy. ACS Appl Mater Interfaces. (2020) 12:26432–43. doi: 10.1021/acsami.0c04506
26. Hu X, Huang YY, Wang Y, Wang X, Hamblin MR. Antimicrobial photodynamic therapy to control clinically relevant biofilm infections. Front Microbiol. (2018) 9:1299. doi: 10.3389/fmicb.2018.01299
27. Amos-Tautua BM, Songca SP, Oluwafemi OS. Application of porphyrins in antibacterial photodynamic therapy. Molecules. (2019) 24:2456. doi: 10.3390/molecules24132456
28. Giuliani F, Martinelli M, Cocchi A, Arbia D, Fantetti L, Roncucci G. In vitro resistance selection studies of RLP068/Cl, a new Zn(II) phthalocyanine suitable for antimicrobial photodynamic therapy. Antimicrob Agents Chemother. (2010) 54:637–42. doi: 10.1128/AAC.00603-09
29. Wojewoda K, Gillstedt M, Tovi J, Salah L, Wennberg Larko AM, Sjoholm A, et al. Optimizing treatment of acne with photodynamic therapy (PDT) to achieve long-term remission and reduce side effects. A prospective randomized controlled trial. J Photochem Photobiol B. (2021) 223:112299. doi: 10.1016/j.jphotobiol.2021.112299
30. Brooke RC, Sinha A, Sidhu MK, Watson RE, Church MK, Friedmann PS, et al. Histamine is released following aminolevulinic acid-photodynamic therapy of human skin and mediates an aminolevulinic acid dose-related immediate inflammatory response. J Invest Dermatol. (2006) 126:2296–301. doi: 10.1038/sj.jid.5700449
31. Ibbotson SH, Wong TH, Morton CA, Collier NJ, Haylett A, McKenna KE, et al. Adverse effects of topical photodynamic therapy: A consensus review and approach to management. Br J Dermatol. (2019) 180:715–29. doi: 10.1111/bjd.17131
32. Guarneri C, Vaccaro M. Erosive pustular dermatosis of the scalp following topical methylaminolaevulinate photodynamic therapy. J Am Acad Dermatol. (2009) 60:521–2. doi: 10.1016/j.jaad.2008.09.006
33. Wolfe CM, Hatfield K, Cognetta AB Jr. Cellulitis as a postprocedural complication of topical 5-aminolevulinic acid photodynamic therapy in the treatment of actinic keratosis. J Drugs Dermatol. (2007) 6:544–8.
34. Moseley H, Ibbotson S, Woods J, Brancaleon L, Lesar A, Goodman C, et al. Clinical and research applications of photodynamic therapy in dermatology: Experience of the Scottish PDT Centre. Lasers Surg Med. (2006) 38:403–16. doi: 10.1002/lsm.20369
35. Alves E, Faustino MA, Tome JP, Neves MG, Tome AC, Cavaleiro JA, et al. Nucleic acid changes during photodynamic inactivation of bacteria by cationic porphyrins. Bioorg Med Chem. (2013) 21:4311–8. doi: 10.1016/j.bmc.2013.04.065
36. Awad MM, Tovmasyan A, Craik JD, Batinic-Haberle I, Benov LT. Important cellular targets for antimicrobial photodynamic therapy. Appl Microbiol Biotechnol. (2016) 100:7679–88. doi: 10.1007/s00253-016-7632-3
37. Chen B, Sun Y, Zhang J, Chen R, Zhong X, Wu X, et al. In vitro evaluation of photodynamic effects against biofilms of dermatophytes involved in onychomycosis. Front Microbiol. (2019) 10:1228. doi: 10.3389/fmicb.2019.01228
38. Hall CW, Mah TF. Molecular mechanisms of biofilm-based antibiotic resistance and tolerance in pathogenic bacteria. FEMS Microbiol Rev. (2017) 41:276–301. doi: 10.1093/femsre/fux010
39. Dai X, Zhao Y, Yu Y, Chen X, Wei X, Zhang X, et al. All-in-one NIR-activated nanoplatforms for enhanced bacterial biofilm eradication. Nanoscale. (2018) 10:18520–30. doi: 10.1039/c8nr04748k
40. Schreiner M, Baumler W, Eckl DB, Spath A, Konig B, Eichner A. Photodynamic inactivation of bacteria to decolonize meticillin-resistant Staphylococcus aureus from human skin. Br J Dermatol. (2018) 179:1358–67. doi: 10.1111/bjd.17152
41. Stolarska M, Glowacka-Sobotta A, Mlynarczyk DT, Dlugaszewska J, Goslinski T, Mielcarek J, et al. Photodynamic activity of tribenzoporphyrazines with bulky periphery against wound bacteria. Int J Mol Sci. (2020) 21:6145. doi: 10.3390/ijms21176145
42. Yougbare S, Mutalik C, Krisnawati DI, Kristanto H, Jazidie A, Nuh M, et al. Nanomaterials for the photothermal killing of bacteria. Nanomaterials (Basel). (2020) 10:1123. doi: 10.3390/nano10061123
43. Bartolomeu M, Rocha S, Cunha A, Neves MG, Faustino MA, Almeida A. Effect of photodynamic therapy on the virulence factors of Staphylococcus aureus. Front Microbiol. (2016) 7:267. doi: 10.3389/fmicb.2016.00267
44. Li C, Jia X, Bian Y, Qi D, Wu J. Different susceptibility of spores and hyphae of Trichophyton rubrum to methylene blue mediated photodynamic treatment in vitro. Mycoses. (2021) 64:48–54. doi: 10.1111/myc.13182
45. Voit T, Cieplik F, Regensburger J, Hiller KA, Gollmer A, Buchalla W, et al. Spatial distribution of a porphyrin-based photosensitizer reveals mechanism of photodynamic inactivation of Candida albicans. Front Med (Lausanne). (2021) 8:641244. doi: 10.3389/fmed.2021.641244
46. Xie J, Wang S, Li Z, Ao C, Wang J, Wang L, et al. 5-aminolevulinic acid photodynamic therapy reduces HPV viral load via autophagy and apoptosis by modulating Ras/Raf/MEK/ERK and PI3K/AKT pathways in HeLa cells. J Photochem Photobiol B. (2019) 194:46–55. doi: 10.1016/j.jphotobiol.2019.03.012
47. Aqeel Y, Siddiqui R, Anwar A, Shah MR, Khoja S, Khan NA. Photochemotherapeutic strategy against Acanthamoeba infections. Antimicrob Agents Chemother. (2015) 59:3031–41. doi: 10.1128/AAC.05126-14
48. Marcolino LMC, Pereira AHC, Pinto JG, Mamone LA, Strixino JF. Cellular and metabolic changes after photodynamic therapy in Leishmania promastigotes. Photodiagnosis Photodyn Ther. (2021) 35:102403. doi: 10.1016/j.pdpdt.2021.102403
49. Yin R, Dai T, Avci P, Jorge AE, de Melo WC, Vecchio D, et al. Light based anti-infectives: Ultraviolet c irradiation, photodynamic therapy, blue light, and beyond. Curr Opin Pharmacol. (2013) 13:731–62. doi: 10.1016/j.coph.2013.08.009
50. Morley S, Griffiths J, Philips G, Moseley H, O’Grady C, Mellish K, et al. Phase IIa randomized, placebo-controlled study of antimicrobial photodynamic therapy in bacterially colonized, chronic leg ulcers and diabetic foot ulcers: A new approach to antimicrobial therapy. Br J Dermatol. (2013) 168:617–24. doi: 10.1111/bjd.12098
51. Pariser DM, Eichenfield LF, Bukhalo M, Waterman G, Jarratt M, Group PDTS. Photodynamic therapy with methyl aminolaevulinate 80 mg g(-1) for severe facial acne vulgaris: A randomized vehicle-controlled study. Br J Dermatol. (2016) 174:770–7. doi: 10.1111/bjd.14345
52. Tu P, Zhang H, Zheng H, Gu H, Xu J, Tao J, et al. 5-aminolevulinic photodynamic therapy versus carbon dioxide laser therapy for small genital warts: A multicenter, randomized, open-label trial. J Am Acad Dermatol. (2021) 84:779–81. doi: 10.1016/j.jaad.2019.07.073
53. Hu Z, Li J, Liu H, Liu L, Jiang L, Zeng K. Treatment of latent or subclinical genital hpv infection with 5-aminolevulinic acid-based photodynamic therapy. Photodiagnosis Photodyn Ther. (2018) 23:362–4. doi: 10.1016/j.pdpdt.2018.07.014
54. Liu ZJ, Lin SC, Lee PY, Lin YT, Lai ZL, Chang CC, et al. Dual-acting antibacterial porous chitosan film embedded with a photosensitizer. Sci Technol Adv Mater. (2020) 21:562–72. doi: 10.1080/14686996.2020.1795431
55. Topaloglu N, Kadikoylu G, Onak G, Karaman O. The effect of indocyanine green-based photodynamic therapy on healthy fibroblast and keratinocyte cells. Photodiagnosis Photodyn Ther. (2020) 31:101891. doi: 10.1016/j.pdpdt.2020.101891
56. Khorsandi K, Fekrazad R, Hamblin MR. Low-dose photodynamic therapy effect on closure of scratch wounds of normal and diabetic fibroblast cells: An in vitro study. J Biophotonics. (2021) 14:e202100005. doi: 10.1002/jbio.202100005
57. Yang R, Guo S, Xiao S, Ding Y. Enhanced wound healing and osteogenic potential of photodynamic therapy on human gingival fibroblasts. Photodiagnosis Photodyn Ther. (2020) 32:101967. doi: 10.1016/j.pdpdt.2020.101967
58. Rudenko TG, Shekhter AB, Guller AE, Aksenova NA, Glagolev NN, Ivanov AV, et al. Specific features of early stage of the wound healing process occurring against the background of photodynamic therapy using fotoditazin photosensitizer-amphiphilic polymer complexes. Photochem Photobiol. (2014) 90:1413–22. doi: 10.1111/php.12340
59. Carrasco E, Calvo MI, Blazquez-Castro A, Vecchio D, Zamarron A, de Almeida IJD, et al. Photoactivation of ROS production in situ transiently activates cell proliferation in mouse skin and in the hair follicle stem cell niche promoting hair growth and wound healing. J Invest Dermatol. (2015) 135:2611–22. doi: 10.1038/jid.2015.248
60. Mao C, Xiang Y, Liu X, Cui Z, Yang X, Yeung KWK, et al. Photo-inspired antibacterial activity and wound healing acceleration by hydrogel embedded with Ag/QWdAQWdjbA==/Zno nanostructures. ACS Nano. (2017) 11:9010–21. doi: 10.1021/acsnano.7b03513
61. Xiao F, Cao B, Wang C, Guo X, Li M, Xing D, et al. Pathogen-specific polymeric antimicrobials with significant membrane disruption and enhanced photodynamic damage to inhibit highly opportunistic bacteria. ACS Nano. (2019) 13:1511–25. doi: 10.1021/acsnano.8b07251
62. Sahu K, Sharma M, Dube A, Gupta PK. Topical antimicrobial photodynamic therapy improves angiogenesis in wounds of diabetic mice. Lasers Med Sci. (2015) 30:1923–9. doi: 10.1007/s10103-015-1784-8
63. Corsi A, Lecci PP, Bacci S, Cappugi P, Pimpinelli N. Early activation of fibroblasts during PDT treatment in leg ulcers. G Ital Dermatol Venereol. (2016) 151:223–9.
64. Vallejo MCS, Moura NMM, Ferreira Faustino MA, Almeida A, Goncalves I, Serra VV, et al. An insight into the role of non-porphyrinoid photosensitizers for skin wound healing. Int J Mol Sci. (2020) 22:E234. doi: 10.3390/ijms22010234
65. Adili F, Scholz T, Hille M, Heckenkamp J, Barth S, Engert A, et al. Photodynamic therapy mediated induction of accelerated re-endothelialisation following injury to the arterial wall: Implications for the prevention of postinterventional restenosis. Eur J Vasc Endovasc Surg. (2002) 24:166–75. doi: 10.1053/ejvs.2002.1703
66. Yang Z, Hu X, Zhou L, He Y, Zhang X, Yang J, et al. Photodynamic therapy accelerates skin wound healing through promoting re-epithelialization. Burns Trauma. (2021) 9:tkab008. doi: 10.1093/burnst/tkab008
67. Blazquez-Castro A, Carrasco E, Calvo MI, Jaen P, Stockert JC, Juarranz A, et al. Protoporphyrin IX-dependent photodynamic production of endogenous ros stimulates cell proliferation. Eur J Cell Biol. (2012) 91:216–23. doi: 10.1016/j.ejcb.2011.12.001
68. Lin MH, Lee JY, Pan SC, Wong TW. Enhancing wound healing in recalcitrant leg ulcers with aminolevulinic acid-mediated antimicrobial photodynamic therapy. Photodiagnosis Photodyn Ther. (2021) 33:102149. doi: 10.1016/j.pdpdt.2020.102149
69. Chiu WT, Tran TV, Pan SC, Huang HK, Chen YC, Wong TW. Cystic fibrosis transmembrane conductance regulator: A possible new target for photodynamic therapy enhances wound healing. Adv Wound Care (New Rochelle). (2019) 8:476–86. doi: 10.1089/wound.2018.0927
70. Gozali MV, Yi F, Zhang JA, Liu J, Wu HJ, Xu Y, et al. Photodynamic therapy inhibit fibroblast growth factor-10 induced keratinocyte differentiation and proliferation through ros in fibroblast growth factor receptor-2b pathway. Sci Rep. (2016) 6:27402. doi: 10.1038/srep27402
71. Daugelaviciene N, Grigaitis P, Gasiule L, Dabkeviciene D, Neniskyte U, Sasnauskiene A. Lysosome-targeted photodynamic treatment induces primary keratinocyte differentiation. J Photochem Photobiol B. (2021) 218:112183. doi: 10.1016/j.jphotobiol.2021.112183
72. Wall IB, Moseley R, Baird DM, Kipling D, Giles P, Laffafian I, et al. Fibroblast dysfunction is a key factor in the non-healing of chronic venous leg ulcers. J Invest Dermatol. (2008) 128:2526–40. doi: 10.1038/jid.2008.114
73. Wang P, Han J, Wei M, Xu Y, Zhang G, Zhang H, et al. Remodeling of dermal collagen in photoaged skin using low-dose 5-aminolevulinic acid photodynamic therapy occurs via the transforming growth factor-beta pathway. J Biophotonics. (2018) 11:e201700357. doi: 10.1002/jbio.201700357
74. Grandi V, Bacci S, Corsi A, Sessa M, Puliti E, Murciano N, et al. ALA-PDT exerts beneficial effects on chronic venous ulcers by inducing changes in inflammatory microenvironment, especially through increased tgf-beta release: A pilot clinical and translational study. Photodiagnosis Photodyn Ther. (2018) 21:252–6. doi: 10.1016/j.pdpdt.2017.12.012
75. Mills SJ, Farrar MD, Ashcroft GS, Griffiths CE, Hardman MJ, Rhodes LE. Topical photodynamic therapy following excisional wounding of human skin increases production of transforming growth factor-beta3 and matrix metalloproteinases 1 and 9, with associated improvement in dermal matrix organization. Br J Dermatol. (2014) 171:55–62. doi: 10.1111/bjd.12843
76. Choi JY, Park GT, Na EY, Wi HS, Lee SC, Lee JB. Molecular changes following topical photodynamic therapy using methyl aminolaevulinate in mouse skin. J Dermatol Sci. (2010) 58:198–203. doi: 10.1016/j.jdermsci.2010.03.019
77. Mendoza-Garcia J, Sebastian A, Alonso-Rasgado T, Bayat A. Optimization of an ex vivo wound healing model in the adult human skin: Functional evaluation using photodynamic therapy. Wound Repair Regen. (2015) 23:685–702. doi: 10.1111/wrr.12325
78. Kim SK, Koo GB, Kim YS, Kim YC. Epithelial-mesenchymal interaction during photodynamic therapy-induced photorejuvenation. Arch Dermatol Res. (2016) 308:493–501. doi: 10.1007/s00403-016-1666-3
79. Karrer S, Bosserhoff AK, Weiderer P, Landthaler M, Szeimies RM. Keratinocyte-derived cytokines after photodynamic therapy and their paracrine induction of matrix metalloproteinases in fibroblasts. Br J Dermatol. (2004) 151:776–83. doi: 10.1111/j.1365-2133.2004.06209.x
80. de Jesus PD, Saeki SI, Tedesco AC. An ex vivo study of photobiostimulation in the treatment of skin pathologies. J Biophotonics. (2016) 9:1189–98. doi: 10.1002/jbio.201500288
81. Bdeir K, Gollomp K, Stasiak M, Mei J, Papiewska-Pajak I, Zhao G, et al. Platelet-specific chemokines contribute to the pathogenesis of acute lung injury. Am J Respir Cell Mol Biol. (2017) 56:261–70. doi: 10.1165/rcmb.2015-0245OC
82. Kirchner S, Lei V, MacLeod AS. The cutaneous wound innate immunological microenvironment. Int J Mol Sci. (2020) 21:8748. doi: 10.3390/ijms21228748
83. Yang T, Tan Y, Zhang W, Yang W, Luo J, Chen L, et al. Effects of ALA-PDT on the healing of mouse skin wounds infected with Pseudomonas aeruginosa and its related mechanisms. Front Cell Dev Biol. (2020) 8:585132. doi: 10.3389/fcell.2020.585132
84. Anzengruber F, Avci P, de Freitas LF, Hamblin MR. T-cell mediated anti-tumor immunity after photodynamic therapy: Why does it not always work and how can we improve it? Photochem Photobiol Sci. (2015) 14:1492–509. doi: 10.1039/c4pp00455h
85. Cappugi P, Comacchi C, Torchia D. Photodynamic therapy for chronic venous ulcers. Acta Dermatovenerol Croat. (2014) 22:129–31.
86. Evangelou G, Farrar MD, White RD, Sorefan NB, Wright KP, McLean K, et al. Topical aminolaevulinic acid-photodynamic therapy produces an inflammatory infiltrate but reduces langerhans cells in healthy human skin in vivo. Br J Dermatol. (2011) 165:513–9. doi: 10.1111/j.1365-2133.2011.10433.x
87. Trevisan E, Menegazzi R, Zabucchi G, Troian B, Prato S, Vita F, et al. Effect of methylene blue photodynamic therapy on human neutrophil functional responses. J Photochem Photobiol B. (2019) 199:111605. doi: 10.1016/j.jphotobiol.2019.111605
88. Zulaziz N, Azhim A, Himeno N, Tanaka M, Satoh Y, Kinoshita M, et al. Photodynamic therapy mediates innate immune responses via fibroblast-macrophage interactions. Hum Cell. (2015) 28:159–66. doi: 10.1007/s13577-015-0118-2
89. Raziyeva K, Kim Y, Zharkinbekov Z, Kassymbek K, Jimi S, Saparov A. Immunology of acute and chronic wound healing. Biomolecules. (2021) 11:700. doi: 10.3390/biom11050700
90. Krzyszczyk P, Schloss R, Palmer A, Berthiaume F. The role of macrophages in acute and chronic wound healing and interventions to promote pro-wound healing phenotypes. Front Physiol. (2018) 9:419. doi: 10.3389/fphys.2018.00419
91. Krasnoselsky MV, Simonova LI, Gertman VZ, Pushkar ES, Zavadskaya TS. Tissue immune cells and their role in the healing process of infected radiation ulcers under the impact of photodynamic therapy (experimental study). Probl Radiac Med Radiobiol. (2019) 24:250–60. doi: 10.33145/2304-8336-2019-24-250-260
92. Zimmermann C, Troeltzsch D, Gimenez-Rivera VA, Galli SJ, Metz M, Maurer M, et al. Mast cells are critical for controlling the bacterial burden and the healing of infected wounds. Proc Natl Acad Sci USA. (2019) 116:20500–4. doi: 10.1073/pnas.1908816116
93. Grandi V, Paroli G, Puliti E, Bacci S, Pimpinelli N. Single ALA-PDT irradiation induces increase in mast cells degranulation and neuropeptide acute response in chronic venous ulcers: A pilot study. Photodiagnosis Photodyn Ther. (2021) 34:102222. doi: 10.1016/j.pdpdt.2021.102222
94. de Vasconcelos Catao MH, Nonaka CF, de Albuquerque RL Jr., Bento PM, de Oliveira Costa R. Effects of red laser, infrared, photodynamic therapy, and green led on the healing process of third-degree burns: Clinical and histological study in rats. Lasers Med Sci. (2015) 30:421–8. doi: 10.1007/s10103-014-1687-0
95. Garcia VG, de Lima MA, Okamoto T, Milanezi LA, Junior EC, Fernandes LA, et al. Effect of photodynamic therapy on the healing of cutaneous third-degree-burn: Histological study in rats. Lasers Med Sci. (2010) 25:221–8. doi: 10.1007/s10103-009-0694-z
96. Mai B, Jia M, Liu S, Sheng Z, Li M, Gao Y, et al. Smart hydrogel-based dvdms/bfgf nanohybrids for antibacterial phototherapy with multiple damaging sites and accelerated wound healing. ACS Appl Mater Interfaces. (2020) 12:10156–69. doi: 10.1021/acsami.0c00298
97. Huang J, Guo M, Wu M, Shen S, Shi L, Cao Z, et al. Effectiveness of a single treatment of photodynamic therapy using topical administration of 5-aminolevulinic acid on methicillin-resistant Staphylococcus aureus-infected wounds of diabetic mice. Photodiagnosis Photodyn Ther. (2020) 30:101748. doi: 10.1016/j.pdpdt.2020.101748
98. Zhou K, Tian R, Li G, Qiu X, Xu L, Guo M, et al. Cationic chalcogenoviologen derivatives for photodynamic antimicrobial therapy and skin regeneration. Chemistry. (2019) 25:13472–8. doi: 10.1002/chem.201903278
99. Dai T, Bil de Arce VJ, Tegos GP, Hamblin MR. Blue dye and red light, a dynamic combination for prophylaxis and treatment of cutaneous Candida albicans infections in mice. Antimicrob Agents Chemother. (2011) 55:5710–7. doi: 10.1128/AAC.05404-11
100. Perez M, Robres P, Moreno B, Bolea R, Verde MT, Perez-Laguna V, et al. Comparison of antibacterial activity and wound healing in a superficial abrasion mouse model of Staphylococcus aureus skin infection using photodynamic therapy based on methylene blue or mupirocin or both. Front Med (Lausanne). (2021) 8:673408. doi: 10.3389/fmed.2021.673408
101. Sperandio FF, Simoes A, Aranha AC, Correa L, Orsini Machado de Sousa SC. Photodynamic therapy mediated by methylene blue dye in wound healing. Photomed Laser Surg. (2010) 28:581–7. doi: 10.1089/pho.2009.2601
102. Silva JC, Lacava ZG, Kuckelhaus S, Silva LP, Neto LF, Sauro EE, et al. Evaluation of the use of low level laser and photosensitizer drugs in healing. Lasers Surg Med. (2004) 34:451–7. doi: 10.1002/lsm.20062
103. Sun Y, Tosa M, Takada H, Ogawa R. Photodynamic therapy delays cutaneous wound healing in mice. J Nippon Med Sch. (2020) 87:110–7. doi: 10.1272/jnms.JNMS.2020_87-301
104. Percival SL, Suleman L, Francolini I, Donelli G. The effectiveness of photodynamic therapy on planktonic cells and biofilms and its role in wound healing. Future Microbiol. (2014) 9:1083–94. doi: 10.2217/fmb.14.59
105. Chen Z, Wang Z, Jin T, Shen G, Wang Y, Tan X, et al. Fibrogenic fibroblast-selective near-infrared phototherapy to control scarring. Theranostics. (2019) 9:6797–808. doi: 10.7150/thno.36375
106. Krupka M, Bozek A, Bartusik-Aebisher D, Cieslar G, Kawczyk-Krupka A. Photodynamic therapy for the treatment of infected leg ulcers-a pilot study. Antibiotics (Basel). (2021) 10:506. doi: 10.3390/antibiotics10050506
107. Mosti G, Picerni P, Licau M, Mattaliano V. Photodynamic therapy in infected venous and mixed leg ulcers: A pilot experience. J Wound Care. (2018) 27:816–21. doi: 10.12968/jowc.2018.27.12.816
108. Mavilia L, Cantarella C, Cicero F, Mercuri SR. Effective use of photodynamic therapy on the treatment of chronic leg ulcers: A pilot study. Dermatol Ther. (2015) 28:276–8. doi: 10.1111/dth.12253
109. Carrinho PM, Andreani DIK, Morete VA, Iseri S, Navarro RS, Villaverde AB. A study on the macroscopic morphometry of the lesion area on diabetic ulcers in humans treated with photodynamic therapy using two methods of measurement. Photomed Laser Surg. (2018) 36:44–50. doi: 10.1089/pho.2017.4305
110. Alavi A, Sibbald RG, Mayer D, Goodman L, Botros M, Armstrong DG, et al. Diabetic foot ulcers: Part I. Pathophysiology and prevention. J Am Acad Dermatol. (2014) 70:1.e1–18. doi: 10.1016/j.jaad.2013.06.055
111. Tardivo JP, Adami F, Correa JA, Pinhal MA, Baptista MS. A clinical trial testing the efficacy of PDT in preventing amputation in diabetic patients. Photodiagnosis Photodyn Ther. (2014) 11:342–50. doi: 10.1016/j.pdpdt.2014.04.007
112. Mannucci E, Genovese S, Monami M, Navalesi G, Dotta F, Anichini R, et al. Photodynamic topical antimicrobial therapy for infected foot ulcers in patients with diabetes: A randomized, double-blind, placebo-controlled study–the D.A.N.T.E (Diabetic ulcer Antimicrobial New Topical treatment Evaluation) study. Acta Diabetol. (2014) 51:435–40. doi: 10.1007/s00592-013-0533-3
113. Monami M, Scatena A, Schlecht M, Lobmann R, Landi L, Ricci L, et al. Antimicrobial photodynamic therapy in infected diabetic foot ulcers: A multicenter preliminary experience. J Am Podiatr Med Assoc. (2020) 110:5. doi: 10.7547/18-069
114. Enk CD, Nasereddin A, Alper R, Dan-Goor M, Jaffe CL, Wulf HC. Cutaneous leishmaniasis responds to daylight-activated photodynamic therapy: Proof of concept for a novel self-administered therapeutic modality. Br J Dermatol. (2015) 172:1364–70. doi: 10.1111/bjd.13490
115. Johansen MB, Jemec GBE, Fabricius S. Effective treatment with photodynamic therapy of cutaneous leishmaniasis: A case report. Dermatol Ther. (2019) 32:e13022. doi: 10.1111/dth.13022
116. Borgia F, Vaccaro M, Cantavenera LG, Aragona E, Cannavo SP. Ulcerative necrobiosis lipoidica successfully treated with photodynamic therapy: Case report and literature review. Photodiagnosis Photodyn Ther. (2014) 11:516–8. doi: 10.1016/j.pdpdt.2014.08.002
117. Bakos L, Zoratto G, Brunetto L, Mazzotti N, Cartell A. Photodynamic therapy: A useful adjunct therapy for recalcitrant ulceration in pemphigus vulgaris. J Eur Acad Dermatol Venereol. (2009) 23:599–600. doi: 10.1111/j.1468-3083.2008.02976.x
118. Tan Y, Bai Y, Li F, Chen J, Cheng Q, Wang J, et al. Evaluation of ALA-PDT combined with antibiotics for the treatment of skin ulcers with sinus tract formation: A pilot study. Photodiagnosis Photodyn Ther. (2020) 31:101802. doi: 10.1016/j.pdpdt.2020.101802
119. Clayton TH, Harrison PV. Photodynamic therapy for infected leg ulcers. Br J Dermatol. (2007) 156:384–5. doi: 10.1111/j.1365-2133.2006.07619.x
120. Carbinatto FM, de Aquino AEJ, Coelho VHM, Bagnato VS. Photonic technology for the treatments of venous and arterial ulcers: Case report. Photodiagnosis Photodyn Ther. (2018) 22:39–41. doi: 10.1016/j.pdpdt.2018.02.011
121. Panto F, Adamo L, Giordano C, Licciardello C. Efficacy and safety of photodynamic therapy with RLP068 for diabetic foot ulcers: A review of the literature and clinical experience. Drugs Context. (2020) 9:2020–4. doi: 10.7573/dic.2019-10-3
122. Brocco E, Curci V, Da Ros R, Miranda C, Boschetti G, Barone S, et al. Photodynamic topical antimicrobial therapy for infected diabetic foot ulcers in patients with diabetes: A case series. Int J Low Extrem Wounds. (2020) 21:137–40. doi: 10.1177/1534734620929889
123. Motta S, Monti M. Photodynamic therapy-a promising treatment option for autoimmune skin ulcers: A case report. Photochem Photobiol Sci. (2007) 6:1150–1. doi: 10.1039/b711920h
Keywords: photosensitizer-based therapy, wound repair, skin, anti-infection, immunomodulatory
Citation: Ning X, He G, Zeng W and Xia Y (2022) The photosensitizer-based therapies enhance the repairing of skin wounds. Front. Med. 9:915548. doi: 10.3389/fmed.2022.915548
Received: 08 April 2022; Accepted: 26 July 2022;
Published: 11 August 2022.
Edited by:
Je-Ho Mun, Seoul National University, South KoreaReviewed by:
Marwah Adly Saleh, Cairo University, EgyptMaria Graça De Pinho Morgado Neves, University of Aveiro, Portugal
Maria Amparo Ferreira Faustino, University of Aveiro, Portugal
Irena Pastar, University of Miami, United States
Copyright © 2022 Ning, He, Zeng and Xia. This is an open-access article distributed under the terms of the Creative Commons Attribution License (CC BY). The use, distribution or reproduction in other forums is permitted, provided the original author(s) and the copyright owner(s) are credited and that the original publication in this journal is cited, in accordance with accepted academic practice. No use, distribution or reproduction is permitted which does not comply with these terms.
*Correspondence: Yumin Xia, eGlheXVtaW4xMjAyQDE2My5jb20=
†ORCID: Xiaoying Ning, https://orcid.org/0000-0002-9753-5639; Gang He, https://orcid.org/0000-0002-5319-7084; Weihui Zeng, https://orcid.org/0000-0001-8374-7852; Yumin Xia, https://orcid.org/0000-0002-3493-7198