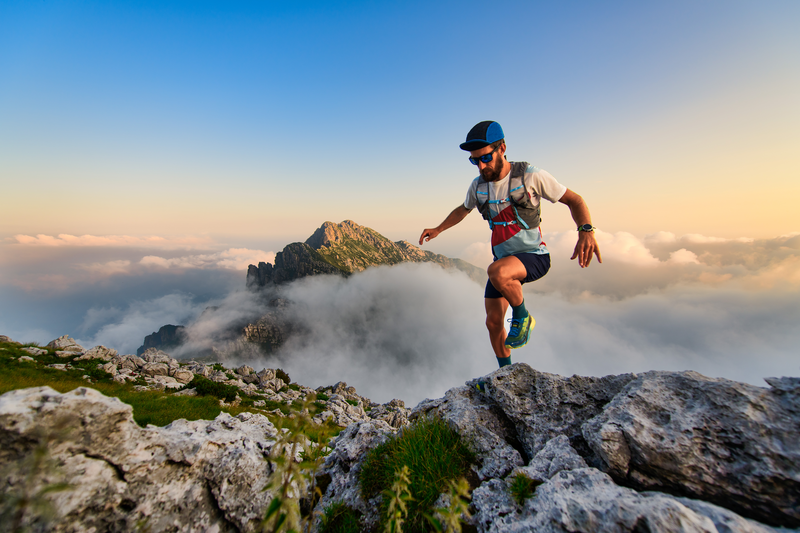
95% of researchers rate our articles as excellent or good
Learn more about the work of our research integrity team to safeguard the quality of each article we publish.
Find out more
REVIEW article
Front. Med. , 08 November 2022
Sec. Dermatology
Volume 9 - 2022 | https://doi.org/10.3389/fmed.2022.1043840
This article is part of the Research Topic Physical Therapy in The Treatment of Skin Diseases and Its Mechanism View all 6 articles
Keloid is a common benign skin tumor in the outpatient department, and patients are often accompanied by itching and pain. Since the pathogenesis is unknown, the effect of single method treatment is unsatisfactory, and therefore the recurrence rate is high. Therefore, comprehensive treatment is mostly used in clinical treatment. Adjuvant radiotherapy is currently one of the most effective treatments for keloid. After long-term clinical practice, brachytherapy and electron beam radiotherapy has increasingly become the gold standard of treatment, because brachytherapy provides more focused radiation treatment to focal tissue to significantly reduce recurrence rate, and better preserve normal tissue. With the development of new radiotherapy techniques, more options for the treatment of keloid. Currently, adjuvant radiotherapy has been widely recognized, but there is no consensus on the optimal protocol for adjuvant radiotherapy for keloids. This review provides a review of published treatment options and new radiotherapy techniques for adjuvant radiotherapy of keloids and gives a comprehensive evaluation for clinical treatment.
Keloid is a dermatofibrosis characterized by excessive fibroblast proliferation and collagen deposition that invades normal tissue beyond its original borders, often secondary to trauma, surgery, and inflammation (1, 2), with an incidence of 5–15% during wound healing (3). Keloid often develops in patients with a family history of the disease or dark-skinned patients (4, 5). Due to the high recurrence rate, this disease remains one of the most pressing clinical challenges. The recurrence rate of keloids in patients who received adjuvant radiation therapy has been reported to be approximately 20%, while the recurrence rate in patients who underwent surgery alone ranged from 50 to 99% (6–8). In addition, Mankowski et al. (9) showed that patients receiving adjuvant radiotherapy also had a lower recurrence rate than those receiving radiation therapy alone (22 and 37%). Therefore, postoperative adjuvant radiotherapy for keloids significantly reduces the recurrence rate (10). Radiotherapy uses radiation to deliver energy to cells to reduce recurrence rates by causing changes in fibroblast structure and activity through direct and indirect effects, thereby killing cells to reduce overproliferation (11). Radiation itself has some carcinogenic potential and therefore the surrounding tissues should be provided with the necessary protection during treatment, especially in children (12, 13). A review of the literature detailed that radiotherapy for keloids induces cancer including fibrosarcoma, basal cell carcinoma, thyroid cancer, and breast cancer, but the probability is extremely low (14). Although radiotherapy has the potential to induce tumors, the risk of surrounding tissue carcinoma from the dose and frequency of radiation used to treat keloids is very low (15, 16). And a survey showed that 78% of oncologic radiologists considered radiotherapy an acceptable treatment for keloids (17). Radiotherapy modalities used to treat keloids include x-ray therapy, electron beam therapy, and brachytherapy (18, 19). This review summarizes the progress of research on different radiation modalities and treatment options in postoperative adjuvant radiotherapy for keloids.
In this review, we conducted a literature search for studies related to keloid scars using PubMed, Web of Science, Embase, and Cochrane. The keywords used in the search were “keloid,” “adjuvant radiotherapy,” and “treatment protocol.” Review articles were used as an initial source of information and, where relevant, information was obtained from primary research papers.
Radiation therapy has been used for the surgical or non-surgical treatment of keloids (8). Currently, the mechanism of radiation therapy for keloids is unclear, but researchers have provided multiple theoretical bases. At the cellular level, postoperative incisions are dominated by naive fibroblasts and unstable collagen fibers, which are more sensitive to radiation, and their damage by ionization can lead to impairment of cell migration, proliferation, and synthetic-secretory functions, thus affecting fibroblast proliferation and collagen synthesis, blocking the cell cycle and inducing apoptosis, and thus inhibiting keloid recurrence (13). A period of a few weeks with premature differentiation and senescence was observed after an immediate cell cycle arrest (20). And after cellular exposure to radiation, the levels of reactive oxygen species such as superoxide (O2-), hydrogen peroxide (H2O2), and hydroxyl radicals (-OH) increase dramatically, leading to damage to macromolecules and DNA (21–24), and thus inhibiting fibroblast proliferation.
However, at the molecular level, radiation inhibited fibroblast proliferation and induced cellular senescence (11). The mRNA and protein expression of senescence-associated genes p16, p21, and p27 were increased in a time-dependent manner after 4 Gy irradiation. This expression is followed by the activation of a dynamic feedback loop by p21, followed by mitochondrial dysfunction and elevated levels of reactive oxygen species, leading to DNA damage and sustained DNA damage reaction (25). Thus, the survival of fibroblasts after radiation-induced cell cycle arrest depends not only on persistent DNA damage and p21 levels but mainly on the cellular CDK2/p21 ratio (26). Tosa et al. (27) combined the results from primary fibroblasts isolated from keloids of five patients who were subjected to irradiation with 15 Gy of electrons, and after 15 min they were compared with unirradiated primary fibroblasts and normal fibroblasts, and the results showed that after irradiation, the expression of 28 genes of keloid fibroblasts was upregulated, accounting for 29.2% of the total number of genes; meanwhile, the expression of 68 genes was downregulated, accounting for the expression of 68 genes was down-regulated, accounting for 70.8% of the total number of genes. Among them, several down-regulated genes were involved in the regulation of cell proliferation and extracellular matrix production, while some up-regulated genes were involved in the regulation of apoptosis and extracellular matrix degradation. Ji et al. (11) found that radiotherapy dysregulated the proliferation and apoptosis of keloid fibroblasts and inhibited the proliferation of keloid fibroblasts compared to normal fibroblasts. Li et al. (28) found that miR-21 was highly expressed in keloid tissues, and its expression level decreased after 15 Gy electron irradiation. Synthesis and this process are affected by electron beam irradiation, therefore, electron beam irradiation can reduce the production of type I collagen by further activating p38 protein through miR-21/Smad7. This study explored the effect of electron beam irradiation on microRNA (miRNA) for the first time, which provides a new idea to discover the molecular mechanism of radiotherapy for keloid treatment. Yan et al. (29) showed that ionizing radiation can inhibit autophagy and promote apoptosis in keloid cells by reducing the expression level of miR-21-5p, and miR-21-5p can regulate this process through the human chromosome 10 deletion phosphatase and tensin homolog (PTEN) and phosphorylated protein kinase B (p-AKT) signaling pathways, thereby preventing local invasion and recurrence of keloids.
Dysregulated long noncoding RNAs (LncRNAs) play an important role in keloid formation by regulating many processes, including fibroblast proliferation and extracellular matrix deposition. Recent studies have shown that LncRNA also has an important role in the formation of keloids (30). The development of keloids is remarkably associated with fibroblast proliferation, invasion, migration, and apoptosis. LncRNA and miRNA can interact and co-regulate with each other. LncRNA is known as a competitive endogenous RNA and can negatively regulate miRNA to influence its activity (31). LncRNA can also prevent miRNA from acting on mRNA and can enhance the translation of mRNA. Over 2,500 LncRNAs were found to be differentially expressed between keloid tissue and normal human skin by microarray and qRT-PCR (32). Of these, 1,731 LncRNAs were upregulated and 782 LncRNAs were downregulated (33). In another study, a total of 2,068 LncRNAs were found to be differentially expressed in earlobe keloids by microarray. Of these, 1,290 LncRNAs were up-regulated and 778 LncRNAs were down-regulated (34). Among them, H19 promotes proliferation by targeting miR-29a and miR-214-5p in keloid fibroblasts (35–37). HOXA11-AS promotes proliferation and migration by targeting miR-124-3p and miR-205-5p in keloid fibroblasts (38–40). CAS1 promotes proliferation, invasion, and migration by targeting miR-205 in keloid fibroblasts and inhibits the apoptotic process (41, 42). LINC01116 promotes proliferation, invasion, and migration by targeting miR-203 and miR-3141 in keloid fibroblasts and produces an extracellular matrix, and inhibits the apoptotic process (43, 44). LncRNA-ATB was upregulated in keloid fibroblasts by targeting miR-200c and ZNF217 to promote their TGF-β2 secretion (45, 46). AC073257.2 was upregulated in keloid fibroblasts by targeting GLI2 to promote their growth and proliferation (47). LINC00937 was upregulated in keloid fibroblasts by targeting miR-28-5p down-regulation in keloid fibroblasts to inhibit their proliferation and extracellular matrix deposition (48). The efficacy of radiotherapy depends mainly on the sensitivity of the irradiated cells to radiotherapy. Some LncRNAs and miRNAs can enhance the sensitivity of cells to radiotherapy, mainly by regulating different genes involved in processes closely related to radiotherapy sensitivity, including affecting DNA damage and inducing cell cycle arrest, regulating the process of DNA damage repair and apoptosis, and activating EGFR signaling (49–56). As radiotherapy research advances, the role of LncRNA for keloid prognosis prediction, treatment process monitoring, and response prediction will certainly become clearer and has the potential to become a potential biomarker for keloids.
Postoperative adjuvant radiotherapy is currently the most effective clinical treatment for keloids (10, 57, 58). Commonly used are external beam radiotherapy (EBRT) and brachytherapy, with EBRT being the earliest radiotherapy method used, consisting mainly of X-ray and electron beam (59). The recurrence rate of high dose rate (HDR) brachytherapy was significantly lower than ERBT, but the recurrence time of keloids was later with EBRT than with brachytherapy, with a mean delay of 2.5 years (60). The relationship between their modes of action, as well as dose and depth, is shown in Figure 1.
Figure 1. Schematic representation of the radiation technique. (A) Process and prognosis of postoperative radiotherapy for keloids, (B) mechanism of X-ray and selection of dose and depth, (C) mechanism of electron radiation and selection of dose and depth, and (D) mechanism of brachytherapy and selection of dose and depth. Reproduced with permission from Journal of Plastic, Reconstructive & Aesthetic Surgery (19).
The X-ray can be produced by X-ray tubes and usually deliver superficial low-dose radiation to the lesion. In 1906, De Bearman and Gourgerot first used X-ray to treat keloids (61). In 1961, Cosman et al. (62) proposed that X-ray treatment be performed immediately after surgical excision of keloids to prevent a recurrence. Studies have shown a recurrence rate of only 21% and a controlled rate of 73–88% for immediate postoperative x-ray treatment (63, 64). Son et al. (65) treated 20 keloids in 15 patients with a single dose of 8 Gy at 50 kV of X-ray and followed up for 6 months, showing a recurrence rate of 6.25%. As revealed in Table 1, the shorter treatment time and the lower dose required for X-ray compared to other radiotherapy modalities resulted in a lower incidence of acute skin complications. However, there are some defects in the treatment of keloids, such as the greater penetration of X-ray and the greater damage to the surrounding normal tissues; the lack of strict radiotherapy protocols, definite histopathological results, and clear consensus on complications; and the lack of follow-up time and the bias of statistical results due to the assessment of efficacy by recurrence rate only, which is rarely used in the treatment of keloids nowadays. Most reports on x-ray are limited to small samples in single-center retrospective studies. The recurrence rates in this literature are within the range of outcomes typically reported for EBRT (6). Despite the low frequency of clinical application of x-ray, the available literature supports its value as an equally effective and safe form of radiotherapy. It may be used when the extent and complexity of the keloid lesion are such that excessive consideration of damage to the surrounding tissue is not allowed.
With advances in radiation technology, X-ray is gradually being replaced by accelerator-generated electron beams. Although some studies have shown that the recurrence rate of postoperative electron beam treatment of keloids is not significantly different from that of x-ray treatment (9, 66). However, the energy is delivered to a depth of 2–5 cm by the accelerator to keloids, and electron beam radiotherapy is more suitable than x-ray therapy for flat and lumpy keloids (67). Compared with X-ray, the more concentrated radiation coverage is more suitable for treating localized superficial lesions such as keloids without causing serious damage to deeper tissues while treating them. Electron beam treatment of keloids often uses energies of 4–6 MeV, and electron beam treatment after surgical excision of keloids can significantly reduce the recurrence rate, and its effectiveness is closely related to the treatment protocol and the site of the keloid (68). Ogawa et al. (69) showed that the local recurrence rate was 5.7% for keloids in the earlobe, 14.3–20% in the chest, deltoid region, and suprapubic region, and 16.7% in other areas. Wang et al. (70) performed electron beam therapy in 58 patients within 4 h after surgery, and the treatment regimen was 12 Gy total dose completed in 4 sessions for keloids in the earlobe and neck, and 16 Gy total dose completed in 4 sessions for keloids in other sites; all patients recovered well with a recurrence rate of 8.6% at 22 months of follow-up. Thus, the choice of electron beam radiotherapy dose depends on the site of the keloid, with keloids in high tension areas (chest, scapular, and suprapubic areas) requiring higher doses for treatment compared to keloids in low tension areas (neck and auricle). As revealed in Table 2, the electron beam therapy produced by accelerators has a stable, controlled, and safe irradiation dose. Electron beam radiotherapy is one of the most common radiation modalities for keloid, and is often chosen when the scar is large and tends to be flat. Currently there are different treatment protocols depending on the site, with 18 Gy in 3 sessions for high recurrence sites, 8 Gy in 1 session for low recurrence sites, and 15 Gy in 2 sessions for other body parts. Keloids on the earlobe are usually adequately controlled with a single session, while keloids on high recurrence areas such as the anterior chest wall are treated with multiple and higher cumulative doses (71).
Brachytherapy was first used for the treatment of keloids in 1967 (72), because high-dose ERBT may damage the normal tissue surrounding the lesion. There was no significant trend in local control with brachytherapy compared to electron beam radiotherapy (86.4% for HDR and 85% for EBRT) (73). However, compared with X-ray or electron beam, brachytherapy significantly reduces the recurrence of keloid (9, 10, 74). Brachytherapy is more effective in postoperative keloids. This therapy allows the radiation source to be placed at the target site, providing more focused radiation therapy to the focal tissue and better preservation of normal tissue (75). Brachytherapy can be divided into interstitial brachytherapy and surface brachytherapy. As shown in Table 3, the overall recurrence rate of HDR brachytherapy was low.
Interstitial brachytherapy is followed by irradiation of the keloid by catheter delivery of the radiation source (inserted at a depth of approximately 5 mm) to avoid damage to the surrounding normal tissue (76). Interstitial brachytherapy can be divided into low and high-dose-rate modalities based on the difference in dose (Table 3). Studies have shown that both LDR and HDR brachytherapy is considered safe and effective (77). Since low-dose-rate brachytherapy patients need to be treated in a lead chamber for 20–72 h, although it can effectively treat keloids, it has been replaced by high-dose-rate brachytherapy due to inconvenience and poor patient compliance. HDR brachytherapy is mostly performed within 24 h after surgery, with short irradiation time (less than 10 min) and high patient compliance, and is suitable for outpatient. Surgery combined with HDR brachytherapy significantly reduces the recurrence of keloids with a high patient satisfaction rate (at 86.9%) (74). For patients who failed surgical or postoperative ERBT, adjuvant HDR brachytherapy had a local control rate of 88% (78). In a study of 24 patients with a total of 32 keloids, patients underwent keloid surgery with three HDR brachytherapy at a dose of 6 Gy and a treatment depth of 5 mm, with a mean follow-up of 29.4 months and a local control rate of 94%(79). Tresoldi et al. (80) reported a study of intraoperative radiation therapy with a postoperative control rate of 90.5%, providing a new perspective on early brachytherapy. Although HDR brachytherapy is highly indicated, it has the disadvantage of catheter dislodgement, which has prompted the emergence of surface brachytherapy.
Surface brachytherapy is a way to treat superficial lesions by uniformly adsorbing radionuclides (32P, 90Sr, or 90Y, etc.) onto filter paper or silver foil, making a special dressing radiator according to the shape and size of the lesion, and applying it close to the surface of the lesion (81). Wagner et al. (82) treated 166 keloids in 139 patients with surgical excision and an adjuvant 90Sr-90Y integrated applicator at a median total dose of 14 (7.5–28.5) Gy. The results showed that the recurrence rate was 20.5% and varied by the site of disease, with the lowest recurrence rate in the face and neck (2%) and the highest recurrence rate in the chest (49%). Surface brachytherapy has been widely used in the treatment of keloids because of its non-invasiveness, ease of operation, low adverse effects, low price, and variability in the shape of the applicator. And the long-term follow-up results showed no secondary malignancies in the irradiated area (82).
HDR interstitial brachytherapy is the primary modality of brachytherapy. After comparing multiple publications on treatment regimen selection, their treatment regimens ranged from 2 to 3 sessions delivering 12–18 Gy. After adjusting for differences in gender, skin type, and keloid characteristics, no differences in recurrence rates emerged (76, 79, 83). HDR resulted in better symptom relief and was convenient and less costly to treat (73). When the keloid is uneven and tends to be curved, brachytherapy is often chosen.
In recent years, heavy ion radiotherapy has been applied in the clinical treatment of refractory and radiation-insensitive tumors, but its application in the postoperative treatment of keloids has been rarely reported. Chen et al. (84) first performed carbon ion therapy after keloid surgery, and 16 patients received a total dose of 16 Gy in 8 sessions, with a mean follow-up of 29.7 months, showing a cure rate of 95%, and none of the patients had any complications. Heavy ion radiotherapy can achieve ideal dose distribution and biological effects by giving precise doses to the tumor site, disrupting the DNA double-strand of tumor cells, with significant cell-killing effects, and significantly reducing damage to normal tissues and endangered organs, with high efficacy and safety. Thus, heavy ion radiotherapy may be a potential clinical treatment modality for keloids.
The multiplication time of normal fibroblasts is 43.5 h. The multiplication time of keloid cells is shortened to 29.5 h, and the rapidly proliferating cells are more sensitive to radiation (85); therefore, adjuvant radiotherapy should be performed as early as possible to obtain better clinical results, and the results of clinical studies show that radiotherapy performed within 24 h after surgery has the best effect (58).
In addition to the choice of radiotherapy timing, the choice of dose and mode is also crucial to the final treatment effect. Too high a dose may damage the normal tissues around the target area and cause serious adverse effects; too low a dose may not achieve the best therapeutic effect. Fractionation therapy allows normal skin cells to recover while keloid cells transition from the radiation-resistant stage to the radiation-sensitive stage. Currently, two segmentation treatment modalities are widely recommended. (1) Multiple splitting mode: a total dose of 17.5–20.0 Gy done in 4 or 5 sessions, each 1 day apart; (2) few splitting mode: a total dose of 18 Gy done in 2 sessions, each 1 week apart. The former is the traditional postoperative radiotherapy modality for keloids, and its safety and efficacy have been proven; however, some investigators believe that the latter is more effective and can be tried for the prevention of postoperative recurrence in refractory and high-recurrence keloids (86). At present, the appropriate radiation dose for the prevention of postoperative keloid recurrence is inconclusive and still needs to be selected by weighing the effectiveness of the treatment against the potential risk of adverse effects.
The potential of radiotherapy as a treatment modality to induce malignancy has been of wide concern. In the X-ray era before 1990, X-ray therapy was reported to have a zero carcinogenic rate (87), which provided supporting evidence for its clinical application. In a study by Preston et al. (88), the probability of developing and dying from skin cancer was 1/7500 in a population aged 18–64 years with whole-body exposure to an irradiation dose of 1 Gy. Based on this study, the postoperative radiotherapy regimen for earlobe keloids reported by Ogawa et al. (89) would expose approximately 0.05% of the patient’s whole body to an 8 Gy dose of irradiation, with an expected morbidity and mortality rate of only 1/2000000 from secondary carcinoma in nearly 4000 patients treated with this regimen. Therefore, the safety of radiotherapy is high.
In the treatment of keloids, radiotherapy may also cause associated complications, which can be divided into early and late complications depending on the time of appearance of symptoms. Early complications include erythema, desquamation, and transient hyperpigmentation, and such adverse effects are seen in all patients within 7–10 days after treatment; (9) late complications are symptoms observed several weeks after treatment, and include permanent hyperpigmentation, capillary dilatation, subcutaneous fibrosis, and chronic ulceration, and the risk of complications after radiotherapy is positively correlated with the irradiation dose (90). In clinical practice, the risk of complications is generally reduced by reducing the fractionated dose while keeping the total dose constant and by lengthening the interval.
Electron beam radiotherapy is high-energy electrons by accelerator-generated. The dose of electrons in the tissue decays rapidly, making electron beam suitable for radiotherapy of skin surface tumors, which minimizes damage to deeper tissues. It can deliver different energies, typically 4–20 MV, and higher energy beams can treat deeper tissue and will have a higher surface dose (91). Although electron beam radiotherapy has advantages in the treatment of superficial lesions, it has complex difficulties, including complicated dose calculations, high costs, and variability of treatment protocols for small lesions (92). Therefore its treatment protocol design in irregular structural lesions is more difficult and prone to computational errors. Because the electron beam used for surface radiotherapy requires high energy maintenance and is generated exclusively by the accelerator, treatment is more expensive.
X-ray differs from electron beam in that it delivers a beam of photons rather than a beam of electrons. The photon beam interacts with the tissue and thus undergoes an ionization reaction to produce secondary electrons. Typically, a lower energy photon beam is produced by an x-ray tube operating in the kilovolt range (89). Compared to electron beam radiotherapy, X-ray has a simpler dose calculation and it is easier to obtain the maximum skin surface dose. The lower energy beam avoids damage to deep structures and can be done on an outpatient basis without the need for hospitalization (93). Since X-ray for the treatment of keloids can be produced by x-ray tubes, the cost is greatly reduced compared to electron beam radiotherapy. However, the low energy of X-ray results in a faster tissue dose and increases the deposition dose in the bone (94). There is less literature on X-ray for keloid treatment (Table 1), and its role in the treatment of skin lesions is not as clear as that of electron beam radiotherapy (95).
In contrast to the two forms of ERBT, brachytherapy is divided into interstitial and surface brachytherapy, depending on the placement of the applicator. Interstitial brachytherapy is further divided into HDR and LDR based on the dose. LDR brachytherapy requires patients to be treated in a lead room for a longer period, usually 20–72 h (96). In contrast, HDR brachytherapy can be performed in an outpatient department because of its short treatment time, proven better patient tolerability, and lower cost (97). And with better symptom relief after HDR treatment (91.7% after HDR vs. 67.9% after LDR, p = 0.03), the use of interstitial brachytherapy in keloid treatment is currently limited to the HDR modality (Table 3) (98). Compared to interstitial brachytherapy, surface brachytherapy reduces the risk of catheter displacement and wound dehiscence, especially in the context of extended treatment periods. Brachytherapy treatment requires multiple treatments in a short period to minimize the risk of wound dehiscence and infection (99). The indications for the application of brachytherapy are similar to the previous indications for ERBT, but because it can be tailored to the shape and size of the keloid and whether it is curved, it can be better adapted to different cases (100). Compared to ERBT, brachytherapy is recommended not to involve more surrounding healthy tissue and requires a lower dose of radiation to achieve the same treatment effect (101). Therefore, despite the similar recurrence rates of external and brachytherapy, brachytherapy is gradually increasing in clinical use due to the convenience and lower cost of treatment leading to high patient compliance.
Heavy ion radiotherapy is a new application in keloid. There are very few literature reports on its efficacy and safety, but based on the only literature available, it has a low recurrence rate and complication rate. As research on keloid treatment continues to advance and clinical translation continues, it is likely to become a new form of radiotherapy for keloid treatment in the future.
X-ray are less commonly used in clinical practice because of the paucity of research literature and the lack of multicenter data on recurrence rates and complications. Currently, HDR brachytherapy and electron beam radiotherapy represent the two main radiation modalities for keloid treatment. For curved, irregularly shaped lesions brachytherapy can be used, while for larger areas, thicker tissues, and recurrence-prone sites electron beam radiotherapy should be combined. Although the differences in efficacy between the different radiotherapy modalities were not statistically significant, they each have their advantages and disadvantages (102), as shown in Table 4.
The review of the literature shows that postoperative adjuvant radiation therapy remains one of the most effective treatments for keloids, with electron beam radiation therapy and HDR brachytherapy being the two main radiation modalities used to adjuvantly treat keloids and provide effective lesion control. Most studies have shown that HDR brachytherapy has better dose coverage and that adjuvant radiation therapy should be administered early (within 24 h), in fewer fractions (usually 4), and at a higher dose (20 Gy) to reduce recurrence rates. Currently, there are numerous studies on postoperative adjuvant radiotherapy for keloids, but no uniform treatment standard has been developed, and future studies should be conducted for the standardized treatment of keloids. Heavy ion therapy may be a potential treatment modality with good future development in the treatment of keloids due to its unique superiority.
WW: conceptualization, data curation, formal analysis, and writing—original draft. JZ: data curation. CZ: visualization. WZ: investigation. MJ: tables organization. YS: project administration, resources, and supervision. All authors read and approved the final version of the submitted manuscript.
The authors declare that the research was conducted in the absence of any commercial or financial relationships that could be construed as a potential conflict of interest.
All claims expressed in this article are solely those of the authors and do not necessarily represent those of their affiliated organizations, or those of the publisher, the editors and the reviewers. Any product that may be evaluated in this article, or claim that may be made by its manufacturer, is not guaranteed or endorsed by the publisher.
1. Li W, Wang Y, Wang X, Liu ZA. Keloid edge precut, preradiotherapy method in large keloid skin graft treatment. Dermatol Surg. (2014) 40:52–7. doi: 10.1111/dsu.12374
2. Gold MH, McGuire M, Mustoe TA, Pusic A, Sachdev M, Waibel J. Updated international clinical recommendations on scar management: part 2—Algorithms for scar prevention and treatment. Dermatol Surg. (2014) 40:825–31. doi: 10.1111/dsu.0000000000000050
3. Wittenberg GP, Fabian BG, Bogomilsky JL, Schultz LR, Rudner EJ, Chaffins ML, et al. Prospective, single-blind, randomized, controlled study to assess the efficacy of the 585-nm flashlamp-pumped pulsed-dye laser and silicone gel sheeting in hypertrophic scar treatment. Arch Dermatol. (1999) 135:1049–55. doi: 10.1001/archderm.135.9.1049
4. Liu S, Yang H, Song J, Zhang Y, Abualhssain ATH, Yang B. Keloid: genetic susceptibility and contributions of genetics and epigenetics to its pathogenesis. Exp Dermatol. (2022). doi: 10.1111/exd.14671
5. Brown JJ, Bayat A. Genetic susceptibility to raised dermal scarring. Br J Dermatol. (2009) 161:8–18. doi: 10.1111/j.1365-2133.2009.09258.x
6. Jones ME, Ganzer CA, Bennett D, Finizio A. Surgical excision of keloids followed by in-office superficial radiation therapy: prospective study examining clinical outcomes. Plast Reconstr Surg Glob Open. (2019) 7:e2212. doi: 10.1097/GOX.0000000000002212
7. Jfri A, Rajeh N, Karkashan EA. Case of multiple spontaneous keloid scars. Case Rep Dermatol. (2015) 7:156–60. doi: 10.1159/000437249
8. Mustoe TA, Cooter RD, Gold MH, Richard Hobbs FD, Ramelet A-A, Shakespeare PG, et al. International clinical recommendations on scar management. Plast Reconstr Surg. (2002) 110:560–71. doi: 10.1097/00006534-200208000-00031
9. Mankowski P, Kanevsky J, Tomlinson J, Dyachenko A, Luc M. Optimizing radiotherapy for keloids: a meta-analysis systematic review comparing recurrence rates between different radiation modalities. Ann Plast Surg. (2017) 78:403–11. doi: 10.1097/SAP.0000000000000989
10. Ogawa R. The most current algorithms for the treatment and prevention of hypertrophic scars and keloids. Plast Reconstr Surg. (2010) 125:557–68. doi: 10.1097/PRS.0b013e3181c82dd5
11. Ji J, Tian Y, Zhu Y, Zhang L, Ji S, Huan J, et al. Ionizing irradiation inhibits keloid fibroblast cell proliferation and induces premature cellular senescence. J Dermatol. (2015) 42:56–63. doi: 10.1111/1346-8138.12702
12. Mazonakis M, Damilakis J. Cancer risk after radiotherapy for benign diseases. Phys Med. (2017) 42:285–91. doi: 10.1016/j.ejmp.2017.01.014
13. Rödel F, Fournier C, Wiedemann J, Merz F, Gaipl US, Frey B, et al. Basics of radiation biology when treating hyperproliferative benign diseases. Front Immunol. (2017) 8:519. doi: 10.3389/fimmu.2017.00519
14. Ogawa R, Yoshitatsu S, Yoshida K, Miyashita T. Is radiation therapy for keloids acceptable? The risk of radiation-induced carcinogenesis. Plast Reconstr Surg. (2009) 124:1196–201. doi: 10.1097/PRS.0b013e3181b5a3ae
15. Recalcati S, Caccialanza M, Piccinno R. Postoperative radiotherapy of auricular keloids: a 26-year experience. J Dermatol Treat. (2011) 22:38–42. doi: 10.3109/09546630903460278
16. McKeown SR, Hatfield P, Prestwich RJ, Shaffer RE, Taylor RE. Radiotherapy for benign disease; assessing the risk of radiation-induced cancer following exposure to intermediate dose radiation. Br J Radiol. (2015) 88:20150405. doi: 10.1259/bjr.20150405
17. Leer JWH, van Houtte P, Davelaar J. Indications and treatment schedules for irradiation of benign diseases: a survey. Radiother Oncol. (1998) 48:249–57. doi: 10.1016/S0167-8140(98)00051-6
18. Gold MH, Berman B, Clementoni MT, Gauglitz GG, Nahai F, Murcia C. Updated international clinical recommendations on scar management: part 1–evaluating the evidence. Dermatol Surg. (2014) 40:817–24. doi: 10.1111/dsu.0000000000000049
19. Liu EK, Cohen RF, Chiu ES. Radiation therapy modalities for keloid management: a critical review. J Plast Reconstr Aesthet Surg. (2022) 75:2455–65. doi: 10.1016/j.bjps.2022.04.099
20. Fournier C, Winter M, Zahnreich S, Nasonova E, Melnikova L, Ritter S. Interrelation amongst differentiation, senescence and genetic instability in long-term cultures of fibroblasts exposed to different radiation qualities. Radiother Oncol. (2007) 83:277–82. doi: 10.1016/j.radonc.2007.04.022
21. Richards SA, Muter J, Ritchie P, Lattanzi G, Hutchison CJ. The accumulation of un-repairable DNA damage in laminopathy progeria fibroblasts is caused by ROS generation and is prevented by treatment with N-acetyl cysteine. Hum Mol Genet. (2011) 20:3997–4004. doi: 10.1093/hmg/ddr327
22. Nathan C, Cunningham-Bussel A. Beyond oxidative stress: an immunologist’s guide to reactive oxygen species. Nat Rev Immunol. (2013) 13:349–61. doi: 10.1038/nri3423
23. Dettmering T, Zahnreich S, Colindres-Rojas M, Durante M, Taucher-Scholz G, Fournier C. Increased effectiveness of carbon ions in the production of reactive oxygen species in normal human fibroblasts. J Radiat Res. (2015) 56:67–76. doi: 10.1093/jrr/rru083
24. Averbeck D, Rodriguez-Lafrasse C. Role of mitochondria in radiation responses: epigenetic, metabolic, and signaling impacts. Int J Mol Sci. (2021) 22:11047. doi: 10.3390/ijms222011047
25. Passos JF, Nelson G, Wang C, Richter T, Simillion C, Proctor CJ, et al. Feedback between p21 and reactive oxygen production is necessary for cell senescence. Mol Syst Biol. (2010) 6:347. doi: 10.1038/msb.2010.5
26. Kollarovic G, Studencka M, Ivanova L, Lauenstein C, Heinze K, Lapytsko A, et al. To senesce or not to senesce: how primary human fibroblasts decide their cell fate after DNA damage. Aging. (2016) 8:158–76. doi: 10.18632/aging.100883
27. Tosa M, Ghazizadeh M, Shimizu H, Hirai T, Hyakusoku H, Kawanami O. Global gene expression analysis of keloid fibroblasts in response to electron beam irradiation reveals the involvement of interleukin-6 pathway. J Invest Dermatol. (2005) 124:704–13. doi: 10.1111/j.0022-202X.2005.23592.x
28. Li S, Liu W, Lei Y, Long J. Regulatory effects of electronic beam irradiation on mir-21/smad7-mediated collagen I synthesis in keloid-derived fibroblasts. Biol Open. (2016) 5:1567–74. doi: 10.1242/bio.018770
29. Yan L, Wang L-Z, Xiao R, Cao R, Pan B, Lv X-Y, et al. Inhibition of microRNA-21-5p reduces keloid fibroblast autophagy and migration by targeting PTEN after electron beam irradiation. Lab Invest. (2020) 100:387–99. doi: 10.1038/s41374-019-0323-9
30. Yu X, Zhu X, Xu H, Li L. Emerging roles of long non-coding RNAs in keloids. Front Cell Dev Biol. (2022) 10:963524. doi: 10.3389/fcell.2022.963524
31. Chen Z, Chu X, Xu J. Detection and analysis of long noncoding RNA expression profiles related to epithelial–mesenchymal transition in keloids. Biomed Eng OnLine. (2022) 21:2. doi: 10.1186/s12938-022-00976-x
32. Zhao Z, Sun W, Guo Z, Zhang J, Yu H, Liu B. Mechanisms of lncRNA/microRNA interactions in angiogenesis. Life Sci. (2020) 254:116900. doi: 10.1016/j.lfs.2019.116900
33. Liang X, Ma L, Long X, Wang X. LncRNA expression profiles and validation in keloid and normal skin tissue. Int J Oncol. (2015) 47:1829–38. doi: 10.3892/ijo.2015.3177
34. Guo L, Xu K, Yan H, Feng H, Chai L, Xu G. Expression profile of long noncoding RNAs in human earlobe keloids: a microarray analysis. BioMed Res Int. (2016) 2016:5893481. doi: 10.1155/2016/5893481
35. Su X, Ma Y, Wang Q, Gao Y. LncRNA HOXA11-AS aggravates keloid progression by the regulation of HOXA11-AS-miR-205-5p-FOXM1 pathway. J Surg Res. (2021) 259:284–95. doi: 10.1016/j.jss.2020.09.035
36. Wan J, He XL, Jian QC, Fan ZF, Shi Y, Luo LF. LINC00937 suppresses keloid fibroblast proliferation and extracellular matrix deposition by targeting the miR-28-5p/MC1R axis. Histol Histopathol. (2021) 36:995–1005. doi: 10.14670/HH-18-372
37. Wang J, Shen J. LncRNA HOXA11-AS aggravates the keloid formation by targeting miR-148b-3p/IGFBP5 axis. Biochem Biophys Res Commun. (2021) 581:60–7. doi: 10.1016/j.bbrc.2021.09.074
38. Wang J, Zhou J, Zhang N, Zhang X, Li Q. A heterocyclic molecule kartogenin induces collagen synthesis of human dermal fibroblasts by activating the smad4/smad5 pathway. Biochem Biophys Res Commun. (2014) 450:568–74. doi: 10.1016/j.bbrc.2014.06.016
39. Wang Z, Feng C, Song K, Qi Z, Huang W, Wang Y. lncRNA-H19/miR-29a axis affected the viability and apoptosis of keloid fibroblasts through acting upon COL1A1 signaling. J Cell Biochem. (2020) 121:4364–76. doi: 10.1002/jcb.29649
40. Wu W, Zhao Y, Gao E, Li Y, Guo X, Zhao T, et al. LncRNA DLEU2 accelerates the tumorigenesis and invasion of non–small cell lung cancer by sponging miR-30a-5p. J Cell Mol Med. (2020) 24:441–50. doi: 10.1111/jcmm.14749
41. Xue M, Jackson CJ. Extracellular matrix reorganization during wound healing and its impact on abnormal scarring. Adv Wound Care. (2015) 4:119–36. doi: 10.1089/wound.2013.0485
42. Xu L, Sun N, Li G, Liu L. LncRNA H19 promotes keloid formation through targeting the miR-769-5p/EIF3A pathway. Mol Cell Biochem. (2021) 476:1477–87. doi: 10.1007/s11010-020-04024-x
43. Ishiwata T. Expression and role of long non-coding RNA H19 in carcinogenesis. Front Biosci. (2018) 23:614–25. doi: 10.2741/4608
44. Yu X, Zheng H, Tse G, Chan MT, Wu WK. Long non-coding RNAs in melanoma. Cell Prolif. (2018) 51:e12457. doi: 10.1111/cpr.12457
45. Yue B, Qiu S, Zhao S, Liu C, Zhang D, Yu F, et al. LncRNA-ATB mediated E-cadherin repression promotes the progression of colon cancer and predicts poor prognosis: LncRNA-ATB mediated E-cadherin repression in colon cancer. J Gastroenterol Hepatol. (2016) 31:595–603. doi: 10.1111/jgh.13206
46. Yuan X, Chen B, Wang X. CircSLC8A1 targets miR-181a-5p/HIF1AN pathway to inhibit the growth, migration and extracellular matrix deposition of human keloid fibroblasts. Burns. (2022). doi: 10.1016/j.burns.2022.04.009
47. Zhang J, Liu CY, Wan Y, Peng L, Li WF, Qiu JX. Long non-coding RNA H19 promotes the proliferation of fibroblasts in keloid scarring. Oncol Lett. (2016) 12:2835–9. doi: 10.3892/ol.2016.4931
48. Zhang Y, Zhu R, Wang J, Cui Z, Wang Y, Zhao Y. Upregulation of lncRNA H19 promotes nasopharyngeal carcinoma proliferation and metastasis in let-7 dependent manner. Artif Cells Nanomedicine Biotechnol. (2019) 47:3854–61. doi: 10.1080/21691401.2019.1669618
49. Carrassa L, Damia G. DNA damage response inhibitors: mechanisms and potential applications in cancer therapy. Cancer Treat Rev. (2017) 60:139–51. doi: 10.1016/j.ctrv.2017.08.013
50. He Y, Jing Y, Wei F, Tang Y, Yang L, Luo J, et al. Long non-coding RNA PVT1 predicts poor prognosis and induces radioresistance by regulating DNA repair and cell apoptosis in nasopharyngeal carcinoma. Cell Death Dis. (2018) 9:235. doi: 10.1038/s41419-018-0265-y
51. Wang S, Pan Y, Zhang R, Xu T, Wu W, Zhang R, et al. Hsa-miR-24-3p increases nasopharyngeal carcinoma radiosensitivity by targeting both the 3’UTR and 5’UTR of Jab1/CSN5. Oncogene. (2016) 35:6096–108. doi: 10.1038/onc.2016.147
52. Wang S, Zhang R, Claret FX, Yang H. Involvement of microRNA-24 and DNA methylation in resistance of nasopharyngeal carcinoma to ionizing radiation. Mol Cancer Ther. (2014) 13:3163–74. doi: 10.1158/1535-7163.MCT-14-0317
53. Li J, Liu X, Nan S, Xu C. Silencing of long non-coding RNA LINC00520 promotes radiosensitivity of head and neck squamous cell carcinoma cells. Free Radic Res. (2020) 54:254–70. doi: 10.1080/10715762.2020.1752373
54. Yi L, Ouyang L, Wang S, Li S, Yang X. Long noncoding RNA PTPRG-AS1 acts as a microRNA-194-3p sponge to regulate radiosensitivity and metastasis of nasopharyngeal carcinoma cells via PRC1. J Cell Physiol. (2019) 234:19088–102. doi: 10.1002/jcp.28547
55. Qu J-Q, Yi H-M, Ye X, Zhu J-F, Yi H, Li L-N, et al. MiRNA-203 reduces nasopharyngeal carcinoma radioresistance by targeting IL8/AKT Signaling. Mol Cancer Ther. (2015) 14:2653–64. doi: 10.1158/1535-7163.MCT-15-0461
56. Marie-Egyptienne DT, Lohse I, Hill RP. Cancer stem cells, the epithelial to mesenchymal transition (EMT) and radioresistance: potential role of hypoxia. Cancer Lett. (2013) 341:63–72. doi: 10.1016/j.canlet.2012.11.019
57. Miles OJ, Zhou J, Paleri S, Fua T, Ramakrishnan A. Chest keloids: effect of surgical excision and adjuvant radiotherapy on recurrence, a systematic review and meta-analysis. ANZ J Surg. (2021) 91:1104–9. doi: 10.1111/ans.16561
58. Xu J, Yang E, Yu N-Z, Long X. Radiation therapy in keloids treatment: history, strategy, effectiveness, and complication. Chin Med J. (2017) 130:1715–21. doi: 10.4103/0366-6999.209896
59. Gold MH, Nestor MS, Berman B, Goldberg D. Assessing keloid recurrence following surgical excision and radiation. Burns Trauma. (2020) 8:tkaa031. doi: 10.1093/burnst/tkaa031
60. Hoang D, Reznik R, Orgel M, Li Q, Mirhadi A, Kulber DA. Surgical excision and adjuvant brachytherapy vs external beam radiation for the effective treatment of keloids: 10-year institutional retrospective analysis. Aesthet Surg J. (2017) 37:212–25. doi: 10.1093/asj/sjw124
62. Cosman B, Crikelair GF, Ju D, Gaulin JC, Lattes R. The surgical treatment of keloids. Plast Reconstr Surg. (1961) 27:335–58.
63. Levy DS, Salter MM, Roth RE. Postoperative irradiation in the prevention of keloids. Am J Roentgenol. (1976) 127:509–10.
64. Kovalic JJ, Perez CA. Radiation therapy following keloidectomy: a 20-year experience. Int J Radiat Oncol Biol Phys. (1989) 17:77–80. doi: 10.1016/0360-3016(89)90373-8
65. Son Y, Phillips EON, Price KM, Rosenberg LZ, Stefanovic B, Wolfe CM, et al. Treatment of keloids with a single dose of low-energy superficial X-ray radiation to prevent recurrence after surgical excision: an in vitro and in vivo study. J Am Acad Dermatol. (2020) 83:1304–14. doi: 10.1016/j.jaad.2020.06.023
66. Hsieh C-L, Chi K-Y, Lin W-Y, Lee LT-J. Timing of adjuvant radiotherapy after keloid excision: a systematic review and meta-analysis. Dermatol Surg. (2021) 47:1438–43. doi: 10.1097/DSS.0000000000003165
67. Hwang N-H, Lee NK, Chae JH, Park S-H, Yoon E-S. The efficacy of CT-based conformal electron beam radiation therapy after keloid excision. Dermatol Surg. (2022) 48:435–40. doi: 10.1097/DSS.0000000000003398
68. Ogawa R, Akaishi S, Dohi T, Kuribayashi S, Miyashita T, Hyakusoku H. Analysis of the surgical treatments of 63 keloids on the cartilaginous part of the auricle: effectiveness of the core excision method. Plast Reconstr Surg. (2015) 135:868–75. doi: 10.1097/PRS.0000000000000962
69. Ogawa R, Miyashita T, Hyakusoku H, Akaishi S, Kuribayashi S, Tateno A. Postoperative radiation protocol for keloids and hypertrophic scars: statistical analysis of 370 sites followed for over 18 months. Ann Plast Surg. (2007) 59:688–91. doi: 10.1097/SAP.0b013e3180423b32
70. Wang Y, Ma J, Zhang Z, Shen H. Combined surgical excision and electron external beam radiation improves the treatment of keloids: a descriptive study. Dermatol Ther. (2020) 33:e13494. doi: 10.1111/dth.13494
71. Papanikolaou N. Handbook of radiotherapy physics: theory and practice. Med Phys. (2008) 35:4281. doi: 10.1118/1.2969650
72. Berman B, Maderal A, Raphael B. Keloids and hypertrophic scars: pathophysiology, classification, and treatment. Dermatol Surg. (2017) 43:S3–18. doi: 10.1097/DSS.0000000000000819
73. De Cicco L, Vischioni B, Vavassori A, Gherardi F, Jereczek-Fossa BA, Lazzari R, et al. Postoperative management of keloids: low-dose-rate and high-dose-rate brachytherapy. Brachytherapy. (2014) 13:508–13. doi: 10.1016/j.brachy.2014.01.005
74. Guix B, Henrıìquez I, Andrés A, Finestres F, Tello JI, Martıìnez A. Treatment of keloids by high-dose-rate brachytherapy: a seven-year study.. Int J Radiat Oncol. (2001) 50:167–72. doi: 10.1016/S0360-3016(00)01563-7
75. Alam M, Nanda S, Mittal BB, Kim NA, Yoo S. The use of brachytherapy in the treatment of nonmelanoma skin cancer: a review. J Am Acad Dermatol. (2011) 65:377–88. doi: 10.1016/j.jaad.2010.03.027
76. Arneja JS, Singh GB, Dolynchuk KN, Murray KA, Rozzelle AA, Jones KD. Treatment of recurrent earlobe keloids with surgery and high-dose-rate brachytherapy. Plast Reconstr Surg. (2008) 121:95–9. doi: 10.1097/01.prs.0000293755.64918.22
77. Renz P, Hasan S, Gresswell S, Hajjar RT, Trombetta M, Fontanesi J. Dose effect in adjuvant radiation therapy for the treatment of resected keloids. Int J Radiat Oncol. (2018) 102:149–54. doi: 10.1016/j.ijrobp.2018.05.027
78. Garg MK, Weiss P, Sharma AK, Gorla GR, Jaggernauth W, Yaparpalvi R, et al. Adjuvant high dose rate brachytherapy (Ir-192) in the management of keloids which have recurred after surgical excision and external radiation. Radiother Oncol. (2004) 73:233–6. doi: 10.1016/j.radonc.2004.04.010
79. Jiang P, Baumann R, Dunst J, Geenen M, Siebert F-A, Niehoff P, et al. Perioperative interstitial high-dose-rate brachytherapy for the treatment of recurrent keloids: feasibility and early results. Int J Radiat Oncol. (2016) 94:532–6. doi: 10.1016/j.ijrobp.2015.11.008
80. Tresoldi MM, Ivaldi GB, Porcu P, Randisi F, Cartocci A, Malovini A, et al. Immediate postoperative treatment of keloids with intraoperative radiation therapy technology: a pilot study. Plast Reconstr Surg Glob Open. (2021) 9:e3738. doi: 10.1097/GOX.0000000000003738
81. Liao Y, Dorafshar AH, Bernard D, Kim T, Camden NB, Wang D. High-dose-rate interstitial brachytherapy vs external beam radiation for the treatment of complex keloids. Med Dosim. (2022) 47:158–60. doi: 10.1016/j.meddos.2022.01.003
82. Wagner W, Alfrink M, Micke O, Schäfer U, Schüller P, Willich N. Results of Prophylactic irradiation in patients with resected keloids: a retrospective analysis. Acta Oncol. (2000) 39:217–20. doi: 10.1080/028418600430806
83. Viani GA, Stefano EJ, Afonso SL, De Fendi LI. Postoperative strontium-90 brachytherapy in the prevention of keloids: results and prognostic factors. Int J Radiat Oncol. (2009) 73:1510–6. doi: 10.1016/j.ijrobp.2008.07.065
84. Chen Y, Feng D, Wang X, Xue J, Chen X. Postoperative carbon ion radiotherapy for keloids: a preliminary report of 16 cases and review of the literature. Wounds. (2014) 26:264–72.
85. Bautista Hernandez Y, Villavicencio Queijero MA, Quezada Bautista AA, Vazquez Tinajero A. Surface brachytherapy in the treatment of keloid scars in Mexico. Rep Pract Oncol Radiother. (2020) 25:133–8. doi: 10.1016/j.rpor.2019.11.002
86. Shen J, Lian X, Sun Y, Wang X, Hu K, Hou X, et al. Hypofractionated electron-beam radiation therapy for keloids: retrospective study of 568 cases with 834 lesions. J Radiat Res. (2015) 56:811–7. doi: 10.1093/jrr/rrv031
87. Borok TL, Bray M, Sinclair I, Plafker J, Labirth L, Rollins C. Role of ionizing irradiation for 393 keloids. Int J Radiat Oncol. (1988) 15:865–70. doi: 10.1016/0360-3016(88)90119-8
88. Preston DL, Ron E, Tokuoka S, Funamoto S, Nishi N, Soda M, et al. Solid cancer incidence in atomic bomb survivors: 1958–1998. Radiat Res. (2007) 168:1–64. doi: 10.1667/RR0763.1
89. Ogawa R, Tosa M, Dohi T, Akaishi S, Kuribayashi S. Surgical excision and postoperative radiotherapy for keloids. Scars Burns Heal. (2019) 5:205951311989111. doi: 10.1177/2059513119891113
90. Bijlard E, Verduijn GM, Harmeling JX, Dehnad H, Niessen FB, Meijer OWM, et al. Optimal high-dose-rate brachytherapy fractionation scheme after keloid excision: a retrospective multicenter comparison of recurrence rates and complications. Int J Radiat Oncol. (2018) 100:679–86. doi: 10.1016/j.ijrobp.2017.10.044
91. Lee SY, Park J. Postoperative electron beam radiotherapy for keloids: treatment outcome and factors associated with occurrence and recurrence. Ann Dermatol. (2015) 27:53. doi: 10.5021/ad.2015.27.1.53
92. Saw CB, Pawlicki T, Korb LJ, Wu A. Effects of extended SSD on electron-beam depth-dose curves. Med Dosim. (1994) 19:77–81. doi: 10.1016/0958-3947(94)90075-2
93. Vyas V, Palmer L, Mudge R, Jiang R, Fleck A, Schaly B, et al. On bolus for megavoltage photon and electron radiation therapy. Med Dosim. (2013) 38:268–73. doi: 10.1016/j.meddos.2013.02.007
94. McGregor S, Minni J, Herold D. Superficial radiation therapy for the treatment of nonmelanoma skin cancers. J Clin Aesthetic Dermatol. (2015) 8:12–4.
95. Martell K, Poirier Y, Zhang T, Hudson A, Spencer D, Jacso F, et al. Radiation therapy for deep periocular cancer treatments when protons are unavailable: is combining electrons and orthovoltage therapy beneficial? J Radiat Res. (2018) 59:593–603. doi: 10.1093/jrr/rry045
96. Pashazadeh A, Boese A, Friebe M. Radiation therapy techniques in the treatment of skin cancer: an overview of the current status and outlook. J Dermatol Treat. (2019) 30:831–9. doi: 10.1080/09546634.2019.1573310
97. Goutos I, Ogawa R. Brachytherapy in the adjuvant management of keloid scars: literature review. Scars Burns Heal. (2017) 3:205951311773548. doi: 10.1177/2059513117735483
98. Escarmant P, Zimmermann S, Amar A, Ratoanina J-L, Moris A, Azaloux H, et al. The treatment of 783 keloid scars by iridium 192 interstitial irradiation after surgical excision. Int J Radiat Oncol. (1993) 26:245–51. doi: 10.1016/0360-3016(93)90204-9
99. van Leeuwen MCE, Stokmans SC, Bulstra A-EJ, Meijer OWM, van Leeuwen PAM, Niessen FB. High-Dose-rate brachytherapy for the treatment of recalcitrant keloids: a unique, effective treatment protocol. Plast Reconstr Surg. (2014) 134:527–34. doi: 10.1097/PRS.0000000000000415
100. Kuribayashi S, Miyashita T, Ozawa Y, Iwano M, Ogawa R, Akaishi S, et al. Post-keloidectomy irradiation using high-dose-rate superficial brachytherapy. J Radiat Res. (2011) 52:365–8. doi: 10.1269/jrr.10159
101. Bryant C, Mendenhall WM. Radiation therapy. In: Dubey SP, Schick B editors. Juvenile Angiofibroma. Cham: Springer International Publishing (2017). p. 225–42. doi: 10.1007/978-3-319-45343-9_18
102. Arno AI, Gauglitz GG, Barret JP, Jeschke MG. Up-to-date approach to manage keloids and hypertrophic scars: a useful guide. Burns. (2014) 40:1255–66. doi: 10.1016/j.burns.2014.02.011
103. Yuan C, Zhang X, Yan Y, Wang B, Li Z, Li L. Sequential therapy with intralesional injections and superficial X-ray therapy in 96 keloids. J Cosmet Dermatol. (2022). doi: 10.1111/jocd.14943
104. Yang X, Shao Y, Yu W, Zhang X, Sun Y, Zhang L, et al. A novel radiotherapy approach for keloids with intrabeam. BioMed Res Int. (2019) 2019:1–7. doi: 10.1155/2019/4693528
105. Petrou IG, Jugun K, Rüegg EM, Zilli T, Modarressi A, Pittet-Cuénod B. Keloid treatment: what about adjuvant radiotherapy? Clin Cosmet Investig Dermatol. (2019) 12:295–301. doi: 10.2147/CCID.S202884
106. Sakamoto T, Oya N, Shibuya K, Nagata Y, Hiraoka M. Dose–response relationship and dose optimization in radiotherapy of postoperative keloids. Radiother Oncol. (2009) 91:271–6. doi: 10.1016/j.radonc.2008.12.018
107. Malaker K, Vijayraghavan K, Hodson I, Al Yafi T. Retrospective analysis of treatment of unresectable keloids with primary radiation over 25 years. Clin Oncol. (2004) 16:290–8. doi: 10.1016/j.clon.2004.03.005
108. Akinbiyi T, Kozak GM, Davis HD, Barrette L-X, Rios-Diaz AJ, Maxwell R, et al. Contemporary treatment of keloids: a 10-year institutional experience with medical management, surgical excision, and radiation therapy. Am J Surg. (2021) 221:689–96. doi: 10.1016/j.amjsurg.2020.07.035
109. Lin Y-F, Shueng P-W, Roan T-L, Chang D-H, Yu Y-C, Chang C-W, et al. Tomotherapy as an alternative irradiative treatment for complicated keloids. J Clin Med. (2020) 9:3732. doi: 10.3390/jcm9113732
110. Liu C-L, Yuan Z-Y. Retrospective study of immediate postoperative electron radiotherapy for therapy-resistant earlobe keloids. Arch Dermatol Res. (2019) 311:469–75. doi: 10.1007/s00403-019-01922-z
111. Hsueh W-T, Hung K-S, Chen Y-C, Huang Y-T, Hsu C-K, Ogawa R, et al. Adjuvant radiotherapy after keloid excision: preliminary experience in Taiwan. Ann Plast Surg. (2019) 82:S39–44. doi: 10.1097/SAP.0000000000001728
112. Sruthi K, Chelakkot P, Madhavan R, Nair R, Dinesh M. Single-fraction radiation: a promising adjuvant therapy to prevent keloid recurrence. J Cancer Res Ther. (2018) 14:1251–5. doi: 10.4103/jcrt.JCRT_20_17
113. Wang L-Z, Ding J-P, Yang M-Y, Chen B. Forty-five cases of chest keloids treated with subcutaneous super-tension-reduction suture combined with postoperative electron-beam irradiation. Dermatol Surg. (2014) 40:1378–84. doi: 10.1097/DSS.0000000000000163
114. Song C, Wu H-G, Chang H, Kim IH, Ha SW. Adjuvant single-fraction radiotherapy is safe and effective for intractable keloids. J Radiat Res. (2014) 55:912–6. doi: 10.1093/jrr/rru025
115. Yamawaki S, Naitoh M, Ishiko T, Muneuchi G, Suzuki S. Keloids can be forced into remission with surgical excision and radiation. Followed by adjuvant therapy. Ann Plast Surg. (2011) 67:402–6. doi: 10.1097/SAP.0b013e31820d684d
116. Speranza G, Sultanem K, Muanza T. Descriptive study of patients receiving excision and radiotherapy for keloids. Int J Radiat Oncol. (2008) 71:1465–9. doi: 10.1016/j.ijrobp.2007.12.015
117. Bischof M, Krempien R, Debus J, Treiber M. Postoperative electron beam radiotherapy for keloids: objective findings and patient satisfaction in self-assessment. Int J Dermatol. (2007) 46:971–5. doi: 10.1111/j.1365-4632.2007.03326.x
118. Ogawa R, Mitsuhashi K, Hyakusoku H, Miyashita T. Postoperative electron-beam irradiation therapy for keloids and hypertrophic scars: retrospective study of 147 cases followed for more than 18 months. Plast Reconstr Surg. (2003) 111:547–53. doi: 10.1097/01.PRS.0000040466.55214.35
119. Maarouf M, Schleicher U, Schmachtenberg A, Ammon J. Radiotherapy in the management of keloids clinical experience with electron beam irradiation and comparison with X-Ray therapy: clinical experience with electron beam irradiation and comparison with X-Ray therapy. Strahlenther Onkol. (2002) 178:330–5. doi: 10.1007/s00066-002-0935-6
120. Deng K, Xiao H, Liu X, Ogawa R, Xu X, Liu Y. Strontium-90 brachytherapy following intralesional triamcinolone and 5-fluorouracil injections for keloid treatment: a randomized controlled trial. PLoS One. (2021) 16:e0248799. doi: 10.1371/journal.pone.0248799
121. Manjunath KN, Venkatesh MS, Alva R, Koushik K, Waiker V, Mohan K, et al. Efficacy of surgical excision and adjuvant high-dose rate brachytherapy in treatment of keloid: our experience. J Cutan Aesthetic Surg. (2021) 14:337–43. doi: 10.4103/JCAS.JCAS_120_16
122. Fuenmayor P, Quiñonez H, Salas R, Pujadas Z. Outcomes of surgical excision and high-dose-rate brachytherapy for earlobe keloids. World J Plast Surg. (2021) 10:78–84. doi: 10.29252/wjps.10.1.78
123. Anderson EM, David J, Phillips T, Demarco J, Reznik RS, Mirhadi AJ, et al. Interstitial high-dose-rate brachytherapy in the treatment of keloids: moving toward a volumetric approach. Brachytherapy. (2021) 20:185–8. doi: 10.1016/j.brachy.2020.07.008
124. Jiang P, Geenen M, Siebert F-A, Bertolini J, Poppe B, Luetzen U, et al. Efficacy and the toxicity of the interstitial high-dose-rate brachytherapy in the management of recurrent keloids: 5-year outcomes. Brachytherapy. (2018) 17:597–600. doi: 10.1016/j.brachy.2017.12.002
125. van Leeuwen MCE, Stokmans SC, Bulstra AEJ, Meijer OWM, Heymans MW, Ket JCF, et al. Surgical excision with adjuvant irradiation for treatment of keloid scars: a systematic review. Plast Reconstr Surg Glob Open. (2015) 3:e440. doi: 10.1097/GOX.0000000000000357
Keywords: keloid, adjuvant radiotherapy, radiation, dose, recurrence
Citation: Wang W, Zhao J, Zhang C, Zhang W, Jin M and Shao Y (2022) Current advances in the selection of adjuvant radiotherapy regimens for keloid. Front. Med. 9:1043840. doi: 10.3389/fmed.2022.1043840
Received: 14 September 2022; Accepted: 20 October 2022;
Published: 08 November 2022.
Edited by:
Feras M. Ghazawi, University of Ottawa, CanadaReviewed by:
Muhammad Ali Shahiman, Rawalpindi Medical University, PakistanCopyright © 2022 Wang, Zhao, Zhang, Zhang, Jin and Shao. This is an open-access article distributed under the terms of the Creative Commons Attribution License (CC BY). The use, distribution or reproduction in other forums is permitted, provided the original author(s) and the copyright owner(s) are credited and that the original publication in this journal is cited, in accordance with accepted academic practice. No use, distribution or reproduction is permitted which does not comply with these terms.
*Correspondence: Ying Shao, c2hhb195aW5nQGpsdS5lZHUuY24=
Disclaimer: All claims expressed in this article are solely those of the authors and do not necessarily represent those of their affiliated organizations, or those of the publisher, the editors and the reviewers. Any product that may be evaluated in this article or claim that may be made by its manufacturer is not guaranteed or endorsed by the publisher.
Research integrity at Frontiers
Learn more about the work of our research integrity team to safeguard the quality of each article we publish.