- Accelerator and Radioisotope Development Team, Korea Atomic Energy Research Institute, Daejeon, South Korea
64Cu and 67Cu are theragnostic pair radionuclides with promising application in the nuclear medicine. 64Cu is PET nuclide for the non-invasive diagnosis and 67Cu is beta emitter for therapy of various cancers. This study discusses optimization efforts in the production of these radioactive coppers carried out with 30 MeV cyclotron. Optimized conditions include target preparation, chemical separation, and quality control. The production routes of 64Cu and 67Cu were studied based on the nuclear reactions of 64Ni(p,n)64Cu and 70Zn(p,α)67Cu. The produced 64Cu and 67Cu have >99.9% of the radionuclidic purity. The yield at the end of bombardment (EOB) of 64Cu and 67Cu is 28.5 MBq/μAh and 67Cu is 0.58 MBq/μAh, respectively.
Introduction
The nuclear medicine field relies on incorporating radioisotopes in small-molecule, nucleic acids, peptides, proteins, antibodies, and drug delivery technologies (1–3) that show high sensitivity for various diseases in order to impart diagnostic and therapeutic effects. Because of the same chemical properties, Copper-64 (64Cu) and (Copper-67) 67Cu can form chemical complexes using the identical labeling protocol, and diagnosis/therapy can be performed simultaneously (4–8). Copper is an essential trace element for the health of all living creatures. In humans, copper is necessary for proper function of organs and metabolic processes (9–13). Therefore, radioactive copper is a promising candidate that can be applied to various diseases. The multipurpose coordination chemistry of copper allows for its radiometallation with various chelators, such as DOTA (1,4,7,10-tetraazacyclododecane-tetraacetic acid), NOTA (1,4,7-triazacyclononane-triacetic acid), TETA (1,4,8,11-tetraazacyclotetraadecane-1,4,8,11-tetraacetic acid), and CB-TE2A (4,11-bis(carboxymethyl)-1,4,8,11-tetraazabicyclo[6.6.2] hexadecane) (14–17), which can be conjugated to various radiopharmaceuticals. 64Cu(T1/2: 12.7 h) is an attractive radioisotope of significant interest for positron emission tomography (PET) with β+(Emax: 653.03 keV and Emean: 278.21 keV) and EC (electron capture: 1675.03 and 1345.77 keV) (18, 19). Furthermore, it has a relatively long half-life compared to fluorine-18 (T1/2: 110 min) and carbon-11 (T1/2: 20.4 min) (19, 20), which corresponds to an adequate half-life for drug requiring long-term follow-up. 67Cu(T1/2 = 61.83 h, β− mean energy = 141 keV) is a radioisotope with significant potential for therapeutic applications in nuclear medicine due to a similar beta mean energy as 134 keV of 177Lu (21–24, 26). Despite its potential, the use of 67Cu for radionuclide therapy has been hindered for decades by its limited supply and low-specific activity. However, the production of 67Cu has been attempted through various nuclear reactions, such as 68Zn(p,2p)67Cu, 70Zn(p,α)67Cu, 67Zn(n,p)67Cu, and 68Zn(γ,p)67Cu (25– 31). In this study, the irradiation, target preparation, chemical separation, and quality control of radioactive copper (64Cu and 67Cu) were verified. The results show that optimized enriched target electrodeposition, proton beam irradiation, separation/purification, and quality control processes can enhance the routine capability of 64Cu and 67Cu production.
Materials and Methods
As shown in Figure 1 below, radioisotopes are produced with a proton beam irradiation process to 64Ni and 70Zn electrodeposited target. In order to maximize the target cooling and the production efficiency of 64Cu and 67Cu when irradiating the target material with a proton beam, a tilted target and a backside water cooling system were applied. The radioactive copper from the target materials was carried out through a solid-phase separation method. The steps of impurities removal, radioactive copper purification, and target material recovery were performed. Finally, 64Cu and 67Cu were verified through quality control of radionuclidic purity and impurity metal content.
Materials and Reagents
Ultra-high purity reagents were used for production of 64Cu and 67Cu in this study. Isotopically enriched 64Ni (58Ni: 0.05%, 60Ni: 0.03%, 61Ni: 0.004%, 62Ni: 0.396%, 64Ni: 99.52%) and 70Zn (64Zn: 0.1%, 66Zn: 0.1%, 67Zn: 0.1%, 68Zn: 2.2%, 70Zn: 97.5%) were supplied from ISOFLEX (San Francisco, CA, USA). The proton beam verification film (Gafchromic Quick Phantom) was obtained from Ashland Company (GafchromicTM Ashland Inc., New Jersey, USA). Substrates of Au-Cu and Ag were received from Doowon Machinery Company (Seoul, South Korea) in a bar shape (1 x 12 cm). Concentrated HCl was purchased from Thermo Fisher Scientific (Waltham, MA, USA); Hydrazine hydrate and sodium hydroxide were purchased from Sigma-Aldrich (St. Louis, MO, USA); CU resin and ZR cartridge were obtained from TRISKEM Company (Bruz, Brittany, France); and AG1X8 anion exchange resin was obtained from Bio-Rad Laboratories (Hercules, CA, USA).
Equipment
The proton beam irradiation studies were performed using a RFT-30 cyclotron (30 MeV, Korea Atomic Energy Research Institute). The apparatus for enriched target material electroplating and dissolution was developed in house. For 64Cu and 67Cu radioactivity measurement, an ionization chamber (AtomlabTM500, BIODEX, New York, USA) was used. The radionuclidic purity was measured by a calibrated high purity germanium gamma detector (HPGe, Oak Ridge, USA). An inductively coupled plasma-mass spectrometry (ICP-MS) system (Agilent 7500, Stevens Creek Blvd, CA, USA) was used to analyze the metallic impurity of the final eluted radioactive copper solution.
Target Preparation
The electroplated target was prepared by an electrodeposition procedure. A typical electroplating target material (64Ni or 70Zn) was dissolved in 10 mL of concentrated HCl. After the target metal was completely dissolved, the target solution was then evaporated to dryness under a vacuum system. The residue was re-dissolved in 600 mL of water, and then 2 mL of hydrazine hydrate was added as an electrolyte to the target solution. The final solution was loaded into the electroplating cell with the substrate. The electroplating was carried out on the substrate at optimized conditions (waveform: square, frequency: 50 Hz, amplitude: 2000, tau: 2, phase: 10 deg, chopping frequency and duty: 100 Hz and 84%, square duty: 60%). After electroplating, the 64Ni and 70Zn targets were examined by measuring their thickness and uniformity.
Proton Beam Irradiation
64Cu and 67Cu were produced at RFT-30 MeV cyclotron by 64Ni(p,n)64Cu and 70Zn(p,α)67Cu nuclear reaction, respectively. The electroplated target was fixed on the solid target station and irradiated with 11.0 MeV (64Cu) and 17.7 MeV (67Cu) protons. The solid target station was fitted with a self-made cradle for the tilted 6° target compared to the beam line. Using Gafchromic film, we optimized the target area of 1175 mm2 with a beam distribution of 90% under the same conditions as employed for 64Cu and 67Cu. Furthermore, the beam currents and cooling system were considered for reducing target thermal damage. The central cooling-water system was connected to the target station (water-pressure: 1.1 MPa, water cooling line: 1/4”). For the production of 64Cu and 67Cu, the beam current was fixed at 30 and 100 μA for 3 and 12 h, respectively.
Separation and Purification
Copper-64
The irradiated 64Ni target (Electroplated target weight: 130 mg) was directly transported to the hot-cell using an automatic target transport system. The irradiated target was dissolved in 7 mL of 8 M HCl with a target dissolving device at 90°C for 1.5 h. The recovered target solution was filtered with a 0.45 μm PVDF syringe filter. To adjust the pH of the dissolved target solution to 2, the solution was evaporated and re-dissolved with water. 64Cu was separated using copper selective CU resin. In brief, 300 mg of CU resin was immersed in water to remove air bubbles and then left in a vacuum for 30 min at 8 mbar. In the wet-packing method, the empty column was filled with immersed CU resin and then the solution was replaced with 0.01 M HCl for the pre-conditioning column. The proton beam irradiated crude 64Ni target solution was loaded on the CU resin pre-packed column at a concentration of 0.01 M HCl. After loading the 64Cu and other radio impurities, the CU resin was washed with 20 mL of 0.01 M HCl (1.0 mL/min) to recover and remove the 64Ni target and impurities. Finally, the 64Cu was eluted with 2 mL of 8 M HCl, and then 64Cu fractions were collected and then evaporated nearly to dryness under a vacuum system.
Copper-67
The separation and purification of the carrier-free 67Cu were performed as follows. After irradiation, the 70Zn target (electroplated target weight: 260 mg) was placed into the target dissolving device. The 70Zn target was dissolved in 7 mL of 9 M HCl at 90°C for 10 min. The dissolved target solution was filtered with a 0.45 μm PVDF syringe filter. We used a two-step separation procedure to remove 66Ga impurities and recover the 70Zn target materials with a ZR cartridge and AG1X8 ion exchange resin. In brief, the target solution was loaded into a ZR cartridge to remove 66Ga and then the cartridge was washed with 9 M HCl for complete recovery of 70Zn and 67Cu. The eluted solution was passed through a wet-packed AG1X8 column (packing height: 7 cm). The column was then washed with 2.5 column volumes of 9 M HCl to eliminate other impurities. The 67Cu was eluted with 8 mL of 2 M HCl, and then 67Cu fractions were collected and then evaporated nearly to dryness under a vacuum system. Finally, enriched 70Zn was completely recovered with 30 mL of 2 M HNO3 and then evaporated for future use.
Quality Control
Metallic Impurity
The impurity metal content (V, Cr, Mn, Fe, Co, Ni, and Zn) of the separated and purified radioactive copper (64Cu and 67Cu) was evaluated via an inductively coupled plasma-mass spectrometer (ICP-MS) to secure biological safety and optimize the radio-labeling yield. The operating conditions were as follows: instrument (Agilent 7500 series), nebulizer (Babington type), spray-chamber (Scott-type), FR generator (frequency: 10 MHz, power 1,300 W), Ar flow rate (plasma 15 L/min, auxiliary 0.9 L/min, nebulizer 1 L/min), sample uptake rate 1 mL/min, and number of replicates (three).
Radionuclidic Purity
Radionuclidic purity was determined using gamma spectroscopy with a high purity germanium detector, multichannel analyzer, and Gamma Vision software. Efficiency and energy calibration was performed with 210Pb (401 Bq/g; 57 keV), 241Am (40 Bq/g; 60 keV), 109Cd (385 Bq/g; 88 keV), 57Co (15 Bq/g; 122 keV), 123mTe (22 Bq/g; 159 keV), 51Cr (491 Bq/g; 320 keV), 113Sn (73 Bq/g; 392 keV), 85Sr (92 Bq/g; 514 keV), 137Cs (65 Bq/g; 662 keV), 88Y (149 Bq/g; 898 and 1,836 keV), and 60Co (77 Bq/g; 1,173 and 1,333 keV). Activity of the multi-nuclide standard source was checked at the day of measurement. The purified samples were fixed on a universal sample holder located 5 cm from the detector window. The gamma spectra were recorded for 86,400 s each for the crude target solution and purified product.
Results
Target Manufacturing
To optimize the production of 64Cu and 67Cu via the nuclear reaction of 64Ni(p,n)64Cu and 70Zn(p,α)67Cu, we prepared 64Ni and 70Zn targets on the substrate by electrodeposition. Gold and silver were used as a cathode and a platinum rod was used as an anode. The bar-shaped substrates were electrodeposited with 64Ni and 70Zn. After cleaning and drying, the weight of the electroplated 64Ni and 70Zn on the substrate was 130 and 260 mg, respectively. The target having a uniform surface was confirmed through an optical microscope (Figure 2; Supplementary Figure 4).
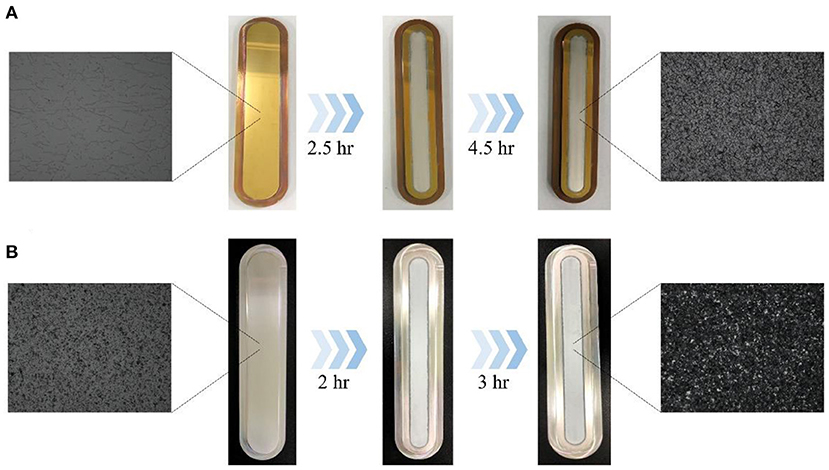
Figure 2. Enriched target electroplated onto substrate (Au-Cu and Ag) (A) 64Ni electrodeposition with Au-Cu substrate (B) 70Zn electrodeposition with silver substrate (magnification under optical microscope X20).
Proton Beam Irradiation
Enriched 64Ni and 70Zn targets were mounted using a self-produced cradle (6° tilted target system) and a target transfer device and then a proton beam was irradiated at 11 and 17.7 MeV incident energy, respectively. We predicted the nuclear reaction cross-section for 64Cu and 67Cu production as 800 and 15 mb at 11 and 17.7 MeV incident energies, respectively, through the NNDC (National nuclear data center) database. The nuclear cross-sections of 64Cu and 67Cu are detailed in the Supplementary Figures 1–5. Based on the theoretical calculation results, the proton beam was irradiated with accumulated current of 90 and 1,200 μAh. The purified 64Cu and 67Cu were produced and isolated from irradiated 64Ni and 70Zn targets; considering the correction due to the decay, at the end of bombardment (EOB) we obtained 64Cu 28.5 MBq/μAh and 67Cu 0.58 MBq/μAh.
Separation and Purification
Preparation of 64Cu
After irradiation for three hours, the 64Cu was completely separated from the enriched 64Ni target solution (Figure 3A). Figure 4A shows the elution profile obtained from the measurement of the fraction activity from the CU resin. To elute 64Cu from CU resin, 64Cu was eluted four times with a volume of 500 μL at a flow rate of 0.5 mL/min using 8 M HCl (64Cu elution yield: 98.6%). The radioactivity of purified 64Cu in each 500 μL fraction and the residual activity on the column were measured by using a dose calibrator. To improve the availability of 64Cu in mild labeling conditions, the purified 64Cu solution was evaporated and re-dissolved in 0.1 M HCl. Finally, 64Ni was collected during the separation and purification procedure for the target recycling.
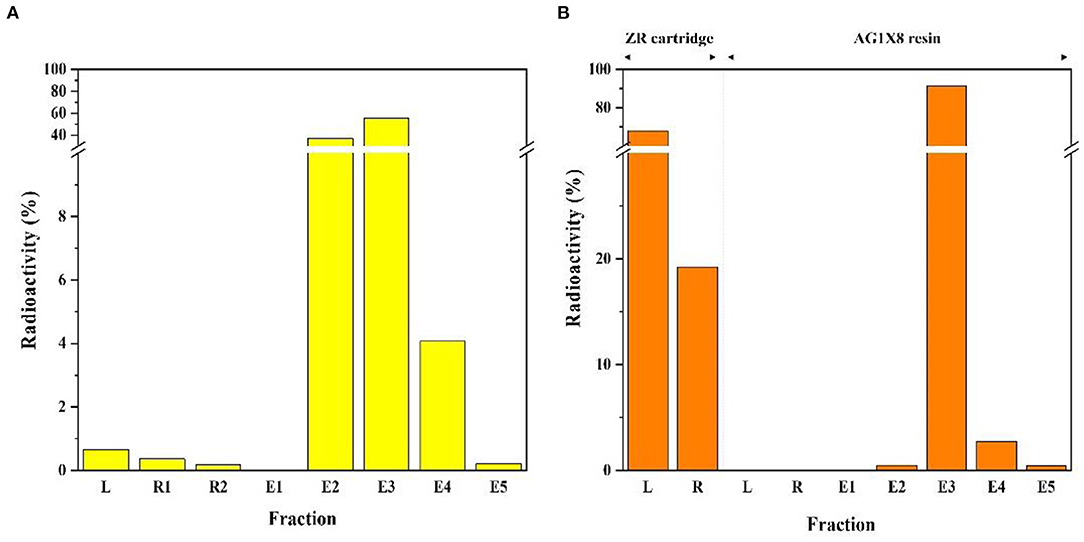
Figure 4. Elution profile of radioactive copper separation from the irradiated target materials (A) 64Cu and (B) 67Cu.
Preparation of 67Cu
After irradiation for 12 h, the enriched 70Zn target was completely dissolved in 9 M HCl. The target solution was chemically processed using two-step separation with a solid-phase resin, specifically, a ZR cartridge and AG1X8 resin (Figure 3B). The target solution was passed through the ZR cartridge to remove 66Ga radio metallic impurities. The 66Ga was completely removed from the crude target solution and then fractions were collected for subsequent separation. During 67Cu production, ~2 days of cooling time was required to remove 66Ga. To maximize the production yield, the method of removing 66Ga was adopted within 20 minutes. Figure 4B shows the elution profile obtained from the measurement of fraction activity from the collected solution. AG1-X8 ion exchange resin was c in water and transferred into an empty column (1 x 10 cm). The target solution was passed through pre-conditioned AG1X8 resin. To elute 67Cu and 70Zn from the AG1X8 resin, 2 M HCl and 2 M HNO3 were used at a 1.0 mL/min flow rate, respectively.
Quality Control
The radionuclidic purity of 64Cu and 67Cu in the separated fraction volume was assessed using a high purity germanium detector. The radioisotope production routes involved radionuclidic impurities that should be removed by chemical separation before use. The gamma energy of the final product must be measured. Figure 5A shows >99% high purity γ-ray spectra of purified 64Cu and 67Cu. In addition, gamma energy spectra of proton beam irradiated target materials described in the Supplementary Figures 1–5. Metallic impurities of the 64Cu and 67Cu elute were determined using ICP-MS to identify metal species that may compete with copper during radio-labeling reactions for radiopharmaceuticals. The content of metallic impurities in the purified 64Cu and 67Cu is <1 ppm, as shown in Figure 5B, and it will not affect the radio-labeling reaction (Supplementary Figure 5).
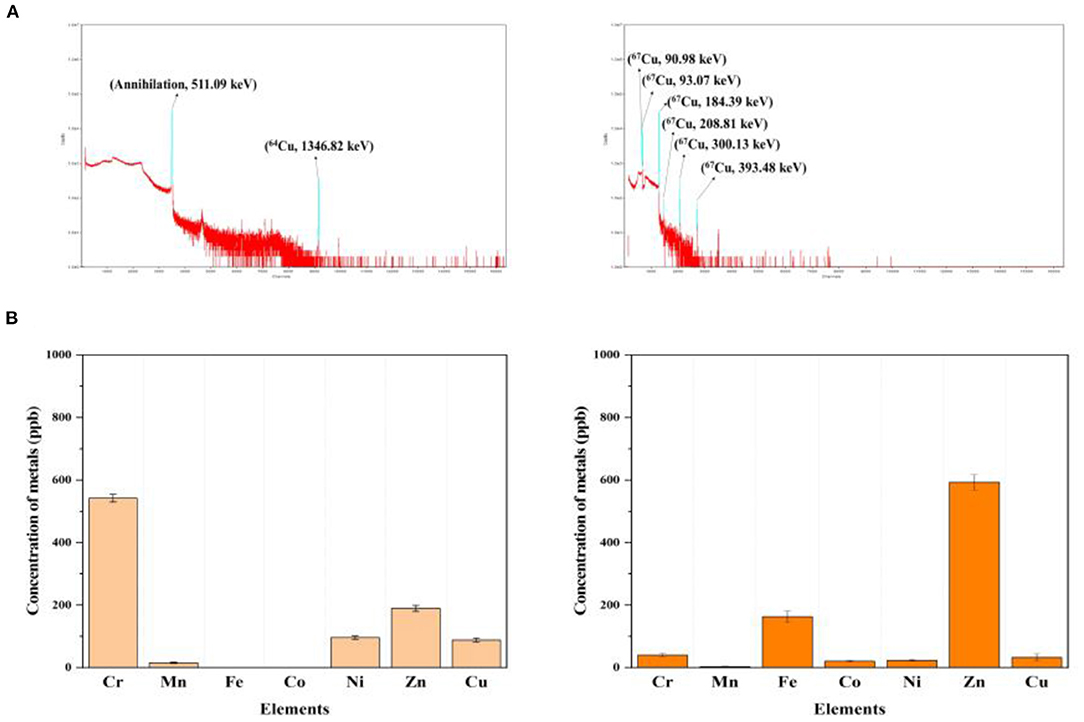
Figure 5. Quality control of pair-radioisotopes (A) gamma energy spectrum of purified 64Cu (left) and 67Cu (right), (B) metallic impurity content in purified final volume of 64Cu (left) and 67Cu (right).
Discussion and Conclusions
The production procedure of pair-radioisotope 64Cu and 67Cu has been studied. This attempt is an imperative study to guarantee the supply and quality of radioisotopes for diagnosis/therapy in the field of nuclear medicine. Each condition was established using RFT-30 cyclotron, equipment, and chemicals to optimize the entire process, including proton beam irradiation, targetry, chemical separation, and quality control of 64Cu and 67Cu. The expected thick target yields of 64Cu and 67Cu were calculated considering the NNDC cross-sections with 11 and 17.7 MeV as incident energy, respectively. The obtained experimental production yields were 2.57 GBq and 696 MBq, respectively. These results have secured sufficiently radioactivity that can be supplied to researchers in the field of nuclear medicine. Furthermore, we will continue to conduct research for mass production. Preparation of electrodeposited target has several advantages including high solidity, density, and heat dissipation efficiency for beam irradiation. Moreover, it is helpful for tilted targets to secure the maximum beam irradiation area. Chemical separation studies were performed using ZR cartridge and AG1X8 resin for 67Cu and CU resin for 64Cu, respectively, considering the copper adsorption capacity, target material recovery, and impurity removal. 64Cu and 67Cu were prepared with >98% separation efficiency, and the final product contains the maximum radioactivity in the minimum volume to introduce the radioactive copper in the radiopharmaceutical under mild reaction conditions, it was evaporated and dried and then re-dissolved in 0.1 M HCl. Furthermore, the radiopharmaceuticals limit the amount of radionuclide contamination allowed in 64Cu and 67Cu solutions for safety and radio-labeling efficiency. The major radionuclide contaminant of radioactive copper solutions is its target material, such as nickel or zinc. Through the quality control procedure, the radionuclidic purity of the final eluted solution was measured as >99% and the metallic impurity content was maintained less than 1 ppm. Further studies on the automation separating apparatus are underway for 64Cu and 67Cu production.
Data Availability Statement
The original contributions presented in the study are included in the article/Supplementary Material, further inquiries can be directed to the corresponding author.
Author Contributions
JYL and JC: conceptualization, data curation, formal analysis, methodology, visualization, writing—original draft, and investigation. JP: funding acquisition and project administration. MH, SY, YK, JL, and JJ: cyclotron operating. JP and SY: validation and writing—review and editing. All authors have read and agreed to the published version of the manuscript.
Funding
This research was supported by the Nuclear R&D Program through the National Research Foundation of Korea, funded by the Ministry of Science, ICT, and Future Planning (2017M2A2A6A05016600 and 2021M2E7A1079112) and NTIS (1711123216), Republic of Korea.
Conflict of Interest
The authors declare that the research was conducted in the absence of any commercial or financial relationships that could be construed as a potential conflict of interest.
Publisher's Note
All claims expressed in this article are solely those of the authors and do not necessarily represent those of their affiliated organizations, or those of the publisher, the editors and the reviewers. Any product that may be evaluated in this article, or claim that may be made by its manufacturer, is not guaranteed or endorsed by the publisher.
Supplementary Material
The Supplementary Material for this article can be found online at: https://www.frontiersin.org/articles/10.3389/fmed.2022.889640/full#supplementary-material
References
1. Man F, Gawne PJ, de Rosales RT. Nuclear imaging of liposomal drug delivery systems: a critical review of radiolabelling methods and applications in nanomedicine. Adv Drug Deliv Rev. (2019) 143:134–60. doi: 10.1016/j.addr.2019.05.012
2. Peltek OO, Muslimov AR, Zyuzin MV, Timin AS. Current outlook on radionuclide delivery systems: from design consideration to translation into clinics. J Nanobiotechnology. (2019) 17:1–34. doi: 10.1186/s12951-019-0524-9
3. Witney TH, Blower PJ. The chemical tool-kit for molecular imaging with radionuclides in the age of targeted and immune therapy. Cancer Imaging. (2021) 21:1–14. doi: 10.1186/s40644-021-00385-8
4. Ferreira CA, Ehlerding EB, Rosenkrans ZT, Jiang D, Sun T, Aluicio-Sarduy E, et al. 86/90Y-Labeled monoclonal antibody targeting tissue factor for pancreatic cancer theranostics. Mol Pharm. (2020) 17:1697–705. doi: 10.1021/acs.molpharmaceut.0c00127
5. McNeil BL, Robertson AK, Fu W, Yang H, Hoehr C, Ramogida CF, et al. Production, purification, and radiolabeling of the 203Pb/212Pb theranostic pair. EJNMMI Radiopharm Chem. (2021) 6:1–18. doi: 10.1186/s41181-021-00121-4
6. Qaim SM, Scholten B, Neumaier B. New developments in the production of theranostic pairs of radionuclides. J Radioanal Nucl Chem. (2018) 318:1493–509. doi: 10.1007/s10967-018-6238-x
7. Cho EH, Lim JC, Lee SY, Park UJ. 44/47Scandium labelled cholecystokinin derivative for cancer theragnostics. Oncol Radiother. (2021) 16:1–5. doi: 10.21203/rs.3.rs-992450/v1
8. Earley DF, Flores JE, Guillou A, Holland JP. Photoactivatable bis (Thiosemicarbazone) derivatives for copper-64 radiotracer synthesis. Dalton Trans. (2022) 51:5041–52. doi: 10.1039/D2DT00209D
9. Chen J, Jiang Y, Shi H, Peng Y, Fan X, Li C. The molecular mechanisms of copper metabolism and its roles in human diseases. Pflügers Arch Eur J Physiol. (2020) 472:1415–29. doi: 10.1007/s00424-020-02412-2
10. Gromadzka G, Tarnacka B, Flaga A, Adamczyk A. Copper dyshomeostasis in neurodegenerative diseases—therapeutic implications. Int J Mol Sci. (2020) 21:9259. doi: 10.3390/ijms21239259
11. Latorre M, Troncoso R, Uauy R. Biological aspects of copper. In: Kerkar N, Roberts EA. Clinical and Translational Perspectives on Wilson Disease. Elsevier (2019). p. 25–31. doi: 10.1016/B978-0-12-810532-0.00004-5
12. Mustafa SK, AlSharif MA. Copper (Cu) an essential redox-active transition metal in living system—a review article. Am J Anal Chem. (2018) 9:15. doi: 10.4236/ajac.2018.91002
13. Shanbhag VC, Gudekar N, Jasmer K, Papageorgiou C, Singh K, Petris MJ. Copper metabolism as a unique vulnerability in cancer. Biochimica et Biophysica Acta Mol Cell Res. (2021) 1868:118893. doi: 10.1016/j.bbamcr.2020.118893
14. Yang H, Gao F, McNeil B, Zhang C, Yuan Z, Zeisler S, et al. Synthesis of dota-pyridine chelates for 64Cu coordination and radiolabeling of αmsh peptide. EJNMMI Radiopharm Chem. (2021) 6:1–16. doi: 10.1186/s41181-020-00119-4
15. Khosravifarsani M, Ait-Mohand S, Paquette B, Sanche L, Guérin B. High cytotoxic effect by combining copper-64 with a nota–terpyridine platinum conjugate. J Med Chem. (2021) 64:6765–76. doi: 10.1021/acs.jmedchem.1c00039
16. Zhu X, Miao X, Qin X, Zhu X. Design of Immunogens: The effect of bifunctional chelator on immunological response to chelated copper. J Pharm Biomed Anal. (2019) 174:263–9. doi: 10.1016/j.jpba.2019.06.001
17. Ocak M, Beaino W, White A, Zeng D, Cai Z, Anderson CJ. 64Cu-labeled phosphonate cross-bridged chelator conjugates of C (Rgdyk) for Pet/Ct imaging of osteolytic bone metastases. Cancer Biother Radiopharm. (2018) 33:74–83. doi: 10.1089/cbr.2017.2419
18. Pettersen NIJ. Novel Production Pathways for the 64, 67Cu Theranostic Pair: Cross Section Measurements for the NatZn (N, X) 64, 67Cu Reactions. (2020).
19. National Nuclear Data Center Brookhaven National Laboratory. (2008). Available online at: https://www.nndc.bnl.gov/nudat2/
20. Welch MJ, Kilbourn MR, Green MA. Radiopharmaceuticals labeled with short-lived positron-emitting radionuclides. Radioisotopes. (1985) 34:170–9. doi: 10.3769/radioisotopes.34.3_170
21. Yano S, Haba H, Shibata S, Komori Y, Takahashi K, Wakitani Y, et al. Specification of 67Cu Produced in the 70Zn (d, αn)67 Cu Reaction.
22. Hao G, Mastren T, Silvers W, Hassan G, Öz OK, Sun X. Copper-67 radioimmunotheranostics for simultaneous immunotherapy and immuno-spect. Sci Rep. (2021) 11:1–11. doi: 10.1038/s41598-021-82812-1
23. Ohya T, Nagatsu K, Suzuki H, Fukada M, Minegishi K, Hanyu M, et al. Small-scale production of 67Cu for a preclinical study via the 64Ni(α,p)67Cu channel. Nucl Med Biol. (2018) 59:56–60. doi: 10.1016/j.nucmedbio.2018.01.002
24. Parrilha GL, dos Santos RG, Beraldo H. Applications of radiocomplexes with thiosemicarbazones and bis (Thiosemicarbazones) in diagnostic and therapeutic nuclear medicine. Coord Chem Rev. (2022) 458:214418. doi: 10.1016/j.ccr.2022.214418
25. Gopalakrishna A, Suryanarayana SV, Naik H, Dixit TS, Nayak BK, Kumar A, et al. Production, separation and supply prospects of 67Cu with the development of fast neutron sources and photonuclear technology. Radiochimica Acta. (2018) 106:549–57. doi: 10.1515/ract-2017-2847
26. Jalilian AR, Gizawy MA, Alliot C, Takacs S, Chakarborty S, Rovais MR, et al. Iaea Activities on 67Cu, 186Re, 47Sc theranostic radionuclides and radiopharmaceuticals. Curr Radiopharm. (2021) 14:306–14. doi: 10.2174/1874471013999200928162322
27. Pupillo G, Mou L, Martini P, Pasquali M, Boschi A, Cicoria G, et al. Production of 67Cu by enriched 70Zn targets: first measurements of formation cross sections of 67Cu, 64Cu, 67Ga, 66Ga, 69mZn and 65Zn in interactions of 70Zn with protons above 45 Mev. Radiochimica Acta. (2020) 108:593–602. doi: 10.1515/ract-2019-3199
28. Qaim SM, Hussain M, Spahn I, Neumaier B. Continuing nuclear data research for production of accelerator-based novel radionuclides for medical use: a mini-review. Front Phys. (2021):16:639290. doi: 10.3389/fphy.2021.639290
29. Medvedev DG, Mausner LF, Meinken GE, Kurczak SO, Schnakenberg H, Dodge CJ, et al. Development of a large scale production of 67Cu from 68Zn at the high energy proton accelerator: closing the 68Zn cycle. Appl Radiat Isotopes. (2012) 70:423–9. doi: 10.1016/j.apradiso.2011.10.007
30. Nigron E, Guertin A, Haddad F, Sounalet T. Is 70Zn(D, X)67Cu the best way to produce 67Cu for medical applications? Front Med. (2021) 8:1059. doi: 10.3389/fmed.2021.674617
Keywords: Copper-64, Copper-67, cyclotron, radioisotope, pair-radioisotope
Citation: Lee JY, Chae JH, Hur MG, Yang SD, Kong YB, Lee J, Ju JS, Choi PS and Park JH (2022) Theragnostic 64Cu/67Cu Radioisotopes Production With RFT-30 Cyclotron. Front. Med. 9:889640. doi: 10.3389/fmed.2022.889640
Received: 04 March 2022; Accepted: 20 April 2022;
Published: 18 May 2022.
Edited by:
Kamil G. Gareev, Saint Petersburg State Electrotechnical University, RussiaReviewed by:
Hans Jurgen Pietzsch, Helmholtz Association of German Research Centers (HZ), GermanyGaia Pupillo, Legnaro National Laboratories (INFN), Italy
Nabanita Naskar, Saha Institute of Nuclear Physics (SINP), India
Izabela Cieszykowska, National Centre for Nuclear Research, Poland
Copyright © 2022 Lee, Chae, Hur, Yang, Kong, Lee, Ju, Choi and Park. This is an open-access article distributed under the terms of the Creative Commons Attribution License (CC BY). The use, distribution or reproduction in other forums is permitted, provided the original author(s) and the copyright owner(s) are credited and that the original publication in this journal is cited, in accordance with accepted academic practice. No use, distribution or reproduction is permitted which does not comply with these terms.
*Correspondence: Jeong Hoon Park, parkjh@kaeri.re.kr