- 1Division of Respiratory Diseases, Department of Medicine, Federal University of São Paulo (UNIFESP), São Paulo, Brazil
- 2Division of Epidemiology and Biostatistics, Department of Global Health, Faculty of Medicine and Health Sciences, Stellenbosch University, Stellenbosch, South Africa
- 3National Health System (NHS), Global Clinical Network, London, United Kingdom
- 4Department of Internal Medicine, University of Kentucky, Lexington, KY, United States
- 5Department of Medicine, Stanford University, Stanford, CA, United States
- 6Division of Pulmonary and Critical Care, National Institute of Health, University of Maryland, College Park, College Park, MD, United States
- 7National Heart and Lung Institute, Imperial College London, London, United Kingdom
- 8Faculty of Medicine, Universidade Eduardo Mondlane, Maputo, Mozambique
- 9Non-communicable Diseases Division, Instituto Nacional de Saúde, Marracuene, Mozambique
- 10College of Medicine, University of Florida, Gainesville, FL, United States
- 11Department of Pulmonology, I.M. Sechenov First Moscow State Medical University (Sechenov University), Moscow, Russia
- 12Infection Prevention and Control-Infectious Disease Service, Foundation University Hospital Campus Bio-Medico, Rome, Italy
- 13Department of Cardiology, Cairo University, Cairo, Egypt
- 14Medway School of Pharmacy, University of Kent at Canterbury, Canterbury, United Kingdom
- 15Division of Pulmonary, Allergy and Critical Care Medicine, Stanford University Medical Center, Stanford, CA, United States
COVID-19 infection primarily targets the lungs, which in severe cases progresses to cytokine storm, acute respiratory distress syndrome, multiorgan dysfunction, and shock. Survivors are now presenting evidence of cardiopulmonary sequelae such as persistent right ventricular dysfunction, chronic thrombosis, lung fibrosis, and pulmonary hypertension. This review will summarize the current knowledge on long-term cardiopulmonary sequelae of COVID-19 and provide a framework for approaching the diagnosis and management of these entities. We will also identify research priorities to address areas of uncertainty and improve the quality of care provided to these patients.
Introduction
The post-COVID-19 syndrome (or long COVID) is defined by the persistence of symptoms with a history of probable or confirmed SARS-CoV-2 infection, usually 3 months from the onset and lasts at least 2 months in the absence of an alternative diagnosis (1). CDC defines long COVID in the context of lingering symptoms that can last greater than 4 weeks and even months in a patient who had the onset of COVID-19 infection 4 weeks prior (2). The main symptoms are fatigue, muscle weakness, dyspnea, sleep, and cognitive disturbances. The proposed hypotheses to explain these findings relate to the viral toxicity itself, systemic inflammatory response, persistent impairment of gas exchange, restrictive lung disease, perfusion abnormality due to micro and macro vascular thrombosis, chronic myocardial dysfunction, corticosteroids use, prolonged hospitalization with immobility, and post-traumatic stress syndrome (3, 4).
Even though the burden of long COVID symptoms correlates with the severity of COVID-19 acute infection (5, 6), patients with milder COVID-19 also have a substantial burden of long COVID symptoms on follow-up. In a study that compared COVID-19 patients who were hospitalized vs. home-isolated patients at 6 months, the persistence of symptoms was noted in 81 vs. 55% of patients respectively (7). In a clinical follow-up of 150 non-critically ill COVID-19 patients after 3 months, 66% of the patients reported symptoms—40% reported asthenia, 30% reported dyspnea, and 23% reported anosmia or dysgeusia (8). In a multistate telephonic survey of 274 patients who had been tested positive for COVID-19 as outpatient, 35% of the patients had not returned to their usual state of health when interviewed 2–3 weeks after being tested positive (9).
A significant economic burden on the healthcare system is expected with the increasing long COVID cases, and further studies exploring this aspect are encouraged. The full spectrum and burden of long COVID in terms of symptoms and cardiopulmonary sequelae such as pulmonary hypertension will be more apparent in the coming years. This narrative review will focus on the most prevalent cardiopulmonary sequelae reported in COVID-19 survivors. We will address the current understanding of the pathobiological mechanisms potentially involved in developing these conditions and focus on areas of uncertainties that should be prioritized in research efforts. We will also review the management strategies for these commonly encountered post-COVID-19 conditions. As the pool of patients recovering from COVID-19 continues to increase, healthcare providers will need to learn to recognize these cardiopulmonary sequelae early and develop a management plan, including focusing on rehabilitation techniques that will prevent further deterioration and improve the quality of life for these patients.
Long-term cardiovascular complications in COVID-19
Cardiovascular complications in COVID-19 infection, including myocardial injury, myocarditis, heart failure, and arrhythmias, have been well-reported during the acute phase since the pandemic’s start (10). The incidence of long-term cardiovascular complications after 30 days of COVID-19 was studied in the US database on a cohort of 153,760 veterans, and an increased risk of strokes, dysrhythmias, myocarditis, ischemic heart diseases, heart failure, cardiac arrest, pulmonary embolism, and deep vein thrombosis was observed (11). The higher risk of cardiovascular complications also extended to COVID-19 survivors who were not hospitalized in this large study (11). These findings were comparable to the results from another large retrospective cohort study (12). In another study of 587 COVID-19 patients, who were followed 1 year after discharge, 11 patients were thought to have died from complications due to COVID-19, including cardiovascular complications such as acute myocardial infarction, acute heart failure and sudden death related to malignant arrhythmia and pulmonary thromboembolism (13). Additionally, new-onset diabetes and major cardiovascular adverse events have also been reported in COVID-19 patients after hospital discharge (14). Regarding the dynamic changes in the ECG or arrhythmias observed during the acute phase of COVID-19, they tend to resolve by 6 months post-COVID-19 (15, 16). However, sinus tachycardia is prevalent in post-COVID-19 survivors (15, 17) and postural orthostatic tachycardia may be the etiology in the setting of persistent complaints of dizziness (18). The cardiovascular impact of COVID-19 infection has also been studied in autopsy studies.
Risk of myocarditis in post-COVID-19 survivors
In an extensive multicenter study screening for myocarditis in 1,597 athletes who underwent cardiac magnetic resonance (CMR) imaging, 2.3% were diagnosed with COVID-19 myocarditis—9 had clinical and 27 had subclinical myocarditis. Follow-up CMR in the 4–14 weeks demonstrated resolution of T2 elevation in all 27 athletes and late gadolinium enhancement in 11 athletes (3). In another observational cohort study following athletes, clinically indicated CMR had a higher yield of myocarditis [15 of 119 (12.6%)] vs. primary screening CMR [6 of 198 (3.0%)]. In post-COVID-19 patients with cardiac symptoms, cardiac edema (54%) and late gadolinium enhancement (31%) on CMR imaging has been reported (15). However, in a study involving 32 patients post-COVID-19 with persistent cardiovascular symptoms, only 3 (9%) met the criteria of acute myocarditis on CMR imaging, and none of those patients met criteria for myocarditis on endomyocardial biopsy (19). In a meta-analysis, the overall prevalence of myocarditis in athletes who had recovered from COVID-19 ranged from 1 to 4% (20). In a study of post-COVID-19 patients (average time since COVID-19 was 4 months) where myocarditis was diagnosed with biopsy in six patients, SARS-CoV-2 was found in four biopsies, lymphocytic myocarditis was found in five, and one patient had giant cell myocarditis (21). The presence of COVID-19 in myocardial tissue months after infection with COVID-19 explains the persistence of chronic inflammation in patients with myocarditis. In a meta-analysis of 277 post-mortem examinations, COVID-19 related histopathological changes such as macro or microvascular thrombi, inflammation, or intraluminal megakaryocytes were common; however, the true prevalence of myocarditis was likely less than 2% based on the pathological analysis. Global uniformity with the use of an autopsy checklist was suggested in reporting cardiovascular pathology findings in COVID-19 (22).
These data indicate that post-COVID-19 survivors can have lingering cardiovascular morbidities in the long-term. Increased burden of cardiovascular complications is also found in COVID-19 patients who may not be hospitalized for COVID-19. During the clinic’s follow-up visits of COVID-19 patients, the possibility of long-term cardiovascular complications should be entertained, especially in the presence of symptoms, and referred to the appropriate specialty clinic when needed. Long-term cardiovascular complications with increased risk in COVID-19 are presented in Table 1.
Right ventricular dysfunction
Prevalence and pathogenesis of right ventricular dysfunction
Right ventricular dysfunction is a known cardiovascular complication of COVID-19 infection observed in 20–31% of cases and associated with increased mortality (23–27). Additionally, worse right ventricular function is associated with elevated troponin and worse clinical presentation (23) and impaired longitudinal strain on echocardiography (27, 28). In a meta-analysis of 1,450 patients, those with right ventricular dysfunction had twofold mortality compared to those without (48.5 vs. 24.7%) (29).
It is known that the thin-walled right ventricle is susceptible to ischemia and dysfunction in response to sudden increases in afterload or coronary occlusion, which in turn may compromise left ventricular function (30). Increased right ventricular afterload in SARS-CoV-2 infection can be secondary to pulmonary parenchymal abnormalities combined with macro- or micro pulmonary vascular disease. In this context, the extent of local tissue damage and the cytokine storm triggered by the host immune response may contribute to the severity of the disease and right ventricular dysfunction (31). Furthermore, activation of the inflammatory cells present in atherosclerotic plaques may lead to coronary plaque rupture and subsequent myocardial ischemia (32). Finally, cardiac CMR imaging findings of patients recovered from COVID-19 infection point to myocardial tissue abnormalities and impaired right ventricular function in otherwise healthy subjects, ultimately suggesting chronic cardiac disease as a consequence of SARS-CoV-2 infection (33). Potential mechanisms of right ventricular dysfunction in COVID-19 are summarized in Figure 1.
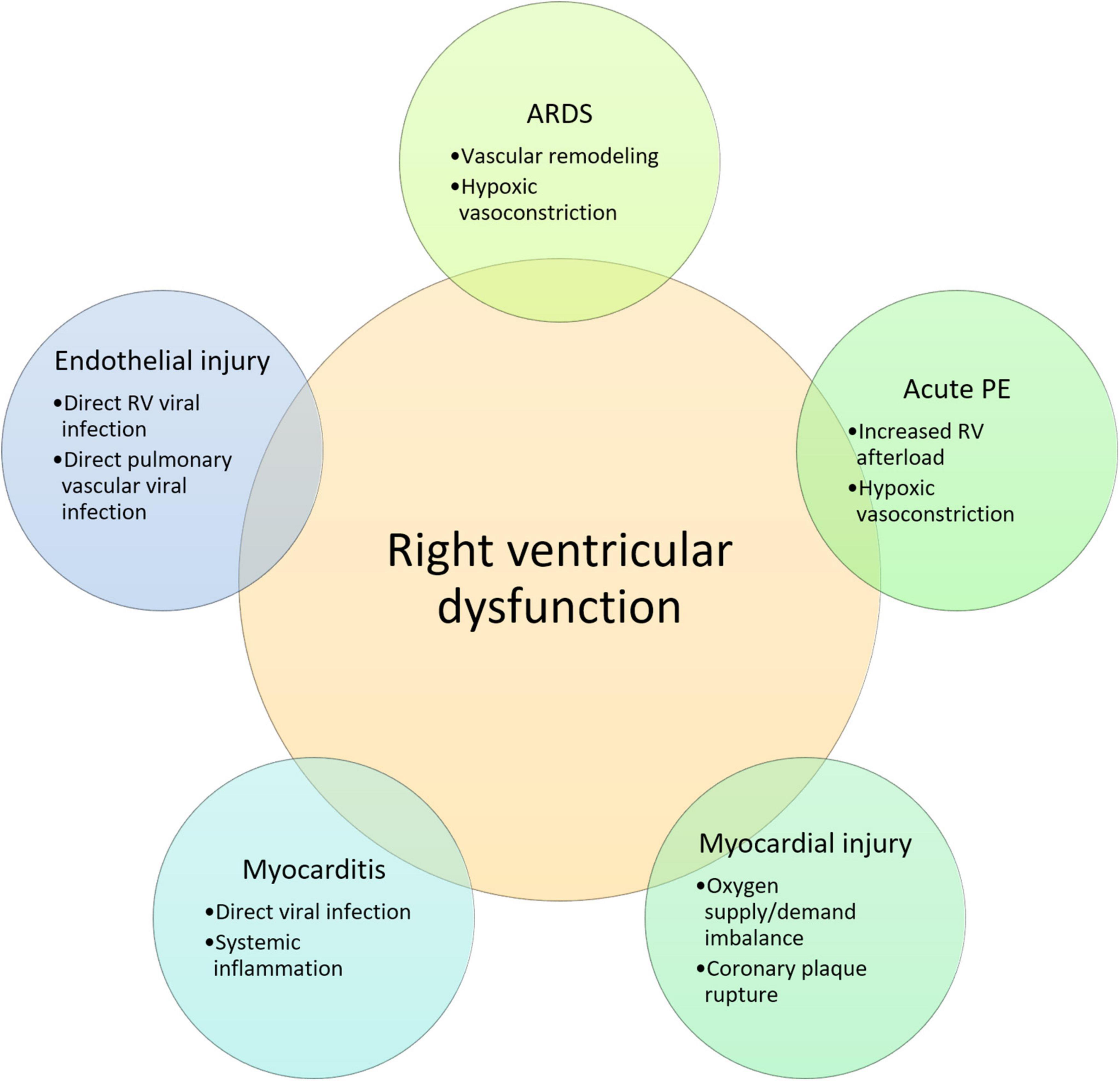
Figure 1. Potential mechanisms of right ventricular dysfunction in COVID-19. RV, right ventricular; ARDS, acute respiratory distress syndrome; PE, pulmonary embolism; IL, interleukin.
Management of right ventricular dysfunction
Management of acute right ventricular dysfunction depends on treating the underlying etiology in addition to the optimization of the intravascular volume, reducing the right ventricular afterload, and enhancing ventricular contractility. Milrinone, a phosphodiesterase-3 inhibitor, enhances right ventricular contractility and reduces pulmonary vascular resistance (34) and may be used instead of dobutamine; however, it might induce hypotension and arrhythmias (35). Inhaled nitric oxide, a selective pulmonary vasodilator, may be beneficial in reducing and stabilizing the pulmonary artery systolic pressure and might reduce the risk of right ventricular failure in COVID-19 (36). Levosimendan, a calcium sensitizer with the advantages of improving the right ventricular contractility and reducing right ventricular afterload with no increase in myocardial oxygen consumption (37), may increase cardiac output and decrease mean pulmonary artery pressure, right atrial pressure, and peripheral systemic resistance in the setting of acute heart failure. Nevertheless, no randomized controlled trials support improved mortality (38–41). Finally, in the presence of severe right ventricular dysfunction, venoarterial, venovenous, venovenous-arterial, or venopulmonary-arterial extracorporeal membrane oxygenation may be required to augment the right ventricle function (42, 43).
In the setting of chronic right heart failure, long-term supplemental oxygen use is recommended for patients with resting or exercise-induced hypoxemia, aiming to reduce hypoxic vasoconstriction and avoid an increase in pulmonary vascular resistance (44). Volume overload should be treated with diuretics. In the setting of isolated right heart failure due to pulmonary embolism, while being cautious of avoiding volume depletion, low-dose diuretics may be used (45). In the setting of decreased left ventricular ejection fraction, goal-directed medical therapy should be employed (46). Whether pulmonary vasodilators can be used or not depends on the primary etiology of pulmonary hypertension, which is further discussed in the section of post-COVID-19 pulmonary hypertension.
Right ventricular dysfunction may not be fully reversible in some COVID-19 survivors, depending on the chronicity of the ischemic insult. In a canine study that involved banding of the pulmonary artery inducing right heart failure, reversibility of the right ventricle was shown to be more profound in those in which pulmonary afterload was reduced early (47). In another canine study, depressed right ventricular function due to severe pulmonary artery obstruction was restored after right coronary artery hyper perfusion (48). However, chronic hypoxia in rats has been shown to induce non-reversible right ventricular dysfunction (49). Additionally, direct or indirect injury of right ventricular myocytes and endothelial cells by SARS-CoV-2 or systemic inflammation might chronically impact right ventricular myocardial and endothelial viability resulting in right ventricular dysfunction. Also, we should point out that the development of chronic thromboembolic pulmonary hypertension (CTEPH) can be accompanied by right ventricular dysfunction (50), which is further discussed in the section of post-COVID-19 pulmonary hypertension. Further studies on the reversibility of right ventricular dysfunction in COVID-19 patients should be pursued and patients with right ventricular dysfunction will require close follow-up for signs of heart failure.
Post-COVID-19 lung fibrosis
Overview of lung function recovery post-COVID-19
A subgroup of post-COVID-19 patients will evolve with persistent chest imaging abnormalities (51) and/or reduced lung diffusion capacity (52, 53). Additionally, post-COVID-19 patients reporting persistent dyspnea, are more likely to have a restrictive pattern, lower carbon monoxide diffusion capacity, reduced functional capacity, and increased exertional desaturation (54). There findings were confirmed by a systematic review of studies assessing pulmonary function post-COVID-19, where altered carbon monoxide diffusion capacity, restrictive pattern, and obstructive pattern were observed in 39, 15, and 7% of patients, respectively, between 1 and 3 months after COVID-19 (55).
In a large study undertaken during the first wave of the COVID-19 epidemic in Wuhan, China, 1,733 of 2,469 discharged patients with COVID-19 were followed up 6 months after hospital discharge. Patients with higher severity scores were found to have lower 6-min walking distance, decreased carbon monoxide diffusion capacity on the pulmonary function test, and worse imaging findings corroborated by high computed tomography (CT) scores (56). Although the pathological characterization of postmortem lung samples from patients who died early after COVID-19 has been extensively reported (57, 58), little is known about the residual pathological changes in the lungs of patients within 2 years of survival of acute COVID-19 (11).
Taken together, these studies suggest that patients with greater severity of acute COVID-19 may have a higher risk for long-term pulmonary complications. These persisting abnormalities are attributed to diffusion impairment and structural pulmonary abnormalities such as pulmonary fibrosis (59).
Evolving pulmonary fibrotic changes in COVID-19
Pulmonary fibrosis is the consequence of aberrant wound healing, which results in a cascade of pathological changes that replaces the lung parenchyma with an extracellular matrix (60). On imaging, pulmonary fibrosis is suggested by the parenchymal bands, reticular opacities, traction bronchiectasis, and honeycombing (61). Pulmonary fibrosis is among the most feared chronic pulmonary complications of COVID-19. It can be challenging to separate the fibrotic changes from the reversible lingering opacities from COVID-19 pneumonia based on the imaging in the post-COVID-19 setting. Often encountered are the imaging findings denoting “grey areas,” which represent evolving immature fibroblastic changes in the background of the diffuse alveolar damage (DAD) remodeling over time (62). It is thus preferred to use the term post-COVID interstitial lung disease (PCILD), which covers a broader spectrum of evolving pulmonary changes seen in patients who have recovered from COVID-19 pneumonia and leaves the prospect of reversibility an open question (62, 63).
Reticular changes suggestive of fibrotic changes were found in half of COVID-19 survivors (23 out of 46) screened at 2 weeks intervals after the onset of severe COVID-19, and these changes persisted at the 4-week follow-up (64). Han et al. showed fibrotic changes in 35% of survivors of severe COVID-19 pneumonia at a 6-month follow-up chest CT (61). According to Vasarmidi et al., the rate of COVID-induced fibrosis may exceed 30% (65, 66). Wu et al. demonstrated that 24% of patients had abnormal CT images at 12 months (67). Similarly, Huang et al. found that at 2 months post-discharge, extensive fibrosis was evident on the CT imaging of 42 out of 81 (52%) patients (68).
The prevalence of PCILD disease varied across all these studies. While interpreting the results of these studies, it is essential to realize that in post-SARS-CoV-2 respiratory syndrome, CT findings suggestive of fibrosis at initial imaging may eventually improve or even resolve with further follow-up (69, 70), but several cases of progressive pulmonary fibrosis have been described in patients with COVID-19 (71–73). Based on the observational studies on SARS-CoV-1, the residual lung damage decreased by the end of the first year (74, 75); however, it persisted after that in the 15 years of follow-up (76). Given that post-COVID-19 pulmonary fibrosis can result in severe chronic hypoxic respiratory failure with significantly debilitating dyspnea, patients with irreversible PCILD after approximately a year may be potential candidates for a lung transplant. Flaifel et al. described the lung pathology of such a population of patients before the lung transplant (77). At 8–11 months after COVID-19 diagnosis, the significant changes noted were described as proliferative and fibrotic phases of DAD, diffuse type 2 pneumocyte hyperplasia, prominent interstitial capillary neo-angiogenesis, and mononuclear cells, specifically macrophages (77).
Risk factors of pulmonary fibrosis in COVID-19
Numerous risk factors have been attributed to the development of PCILD, such as the length of stay in the hospital and the ICU, the use of high-flow nasal oxygen, mechanical ventilation, and the occurrence of acute respiratory distress (66, 78). Acute respiratory distress syndrome (ARDS) is a condition well known for high rates of development of pulmonary fibrosis (79–81). Ventilator-associated lung injury, in the setting of non-adherence to protective lung strategies, can further worsen lung injury (82). Among survivors of severe COVID-19, 20% of non-mechanically ventilated and 72% of mechanically ventilated individuals had fibrotic-like radiographic abnormalities 4 months after hospitalization (83). Greater initial severity of the disease and a longer duration of mechanical ventilation were independent risk factors for the development of fibrosis-like abnormalities. Similar findings were also reported in another study that found most COVID-19 patients with pulmonary fibrosis (81%) during acute COVID-19 infection were admitted to an ICU, and 63% required mechanical ventilation (84).
Patient-related risk factors included male gender, older age, active smoking, persistent breathlessness, and alcohol abuse (68, 85–88). Men are three times more likely to develop PCILD (85). Han et al. identified age >50 years and heart rate >100 beats per minute at admission as independent predictors of fibrotic-like changes in survivors of severe COVID-19 pneumonia at a 6-month follow-up (61). Finally, cytokines such as interleukin-6 and upregulation of other growth factors such as TGF-β1, FGF, and EGF also contribute to the development of pulmonary fibrosis (89, 90). Neutrophil extracellular traps play a key role in the interplay between inflammation and thrombotic changes in the lung. They may have a role in the development of lung fibrosis (91) and therefore can be a potential therapeutic target (92).
Link between idiopathic pulmonary fibrosis and COVID-19
Gene-environment interactions and genetic susceptibility factors may play a role in the development of PCILD. Fadista et al. found a genetic correlation between idiopathic pulmonary fibrosis and COVID-19 severity, pointing several variants associated with both increased idiopathic pulmonary fibrosis risk and increased risk of severe COVID-19 (93). Additionally, genome-wide association studies have identified multiple genetic signals associated with severe COVID-19, including a variant within the DPP9 gene related to increased idiopathic pulmonary fibrosis risk (94). Four genetic association signals showed evidence of a shared causal variant between idiopathic pulmonary fibrosis and at least one COVID-19 phenotype, namely loci at 7q22.1, near MUC5B, near ATP11A, and near DPP9 (94). Finally, shorter blood leukocyte telomere lengths are independent risk factors for developing fibrotic-like abnormalities in COVID-19 (83). Thus, this genomic biomarker may predict increased susceptibility to the development of post-COVID-19 pulmonary fibrosis.
Diagnosis of post-COVID interstitial lung disease
A diagnosis of PCILD should be based on clinical, radiologic, and pathologic findings. While the appropriate timing for the diagnosis of irreversible fibrosis has not yet been established, serial evaluation with lung function test, including assessment for carbon monoxide diffusion capacity and 6-min walk test, can be tailored to the patient’s clinical course, symptoms, and oxygen requirement. Further evaluation may involve the need for a chest CT (90, 95). Earlier in the pandemic, more frequent serial evaluations at 3, 6, 9, and 12 months were recommended, as there was a need for further research studies in this area (95, 96). Although there is no specific finding from laboratory testing, immunohistochemical analysis of TGF-b, IL-1α, and IFN-β may play a role in predicting PCILD. In this context, a recent study found that high IL-1α and TGF-β and low plasma levels of IFN-β could predict an increased relative risk of lung fibrosis-like changes in PCILD (97). More research is needed to confirm the prognostic role of genetic tests such as telomere shortening in PCILD.
Management of post-COVID interstitial lung disease
The role of treatment with antifibrotic and anti-inflammatory drugs (65, 98–100) in improving PCILD symptoms remains unclear and inconclusive. There is still little data on the safety and effectiveness of those treatments in COVID-19 patients with PCILD, and most clinical trials have yet to be completed. Recent evidence has shown that the use of high-dose vs. low-dose prednisolone in a randomized control trial of 130 patients with PCILD did not show significant improvement in symptoms after 6 weeks of follow-up (101). In contrast, three observational studies have reported improvement with glucocorticoids in symptomatic patients with PCILD (102–104). However, these findings should be interpreted cautiously because the two studies had small sample sizes (103, 104). A recent trial found that Pycnogenol® and Centellicum® may improve the residual clinical picture in PCILD patients and reduce the number of subjects progressing to lung fibrosis (100). However, this result should be viewed in the context of small sample size and a poorly designed study. Randomized clinical trials with Pycnogenol® and Centellicum® in PCILD patients are highly recommended. Other suggested therapies include mesenchymal stem cells, cytokine Inhibitors, spironolactone, TGF-β1 Inhibitors, CD147 Inhibitors, poly-(ADP-Ribose) polymerase Inhibitor, galectin-3 (e.g., BIO 300), and Chinese medicine drugs for pulmonary fibrosis in convalescent sequelae of COVID-19 (99, 105).
Presently, the treatment for PCILD remains supportive. Referral to pulmonary rehabilitation programs and evaluation for supplemental oxygen therapy should be considered for patients meeting the criteria. The role of treatment with antifibrotic drugs remains unclear whose lung function continues to deteriorate.
Persisting coagulation abnormalities in COVID-19 survivors
Various coagulation abnormalities such as increased D-dimer, fibrinogen, factor VIII levels, mild thrombocytopenia, and slightly prolonged prothrombin time have been noted in the setting of the inflammatory milieu featured in COVID-19 (106–110). These coagulation abnormalities predispose COVID-19 patients to acute macro and micro thromboembolic events (111). The predisposition to coagulopathy is manifested in the form of microangiopathy with widespread thrombosis observed in COVID-19 autopsied lungs (112). The 90-day incidence rate of venous thromboembolism may range from 0.2 to 0.8% in COVID-19 cases and up to 4.5% in hospitalized patients (113).
Persistent hypercoagulability has been observed in COVID-19 survivors. A study on 208 COVID-19 survivors 2 months after onset, identified significant activation of endothelial cells and in vivo thrombin generation in at least one out of four COVID-19 survivors (114). At 3 months, 203 COVID-19 survivors were found to have increased endothelin-1, thrombin-antithrombin complex, von-Willebrand factor, and inflammatory cytokines (115). Others have identified increased thrombin generation capacity and hypofibrinolytic activity in the setting of increased Factor VIII levels and decreased plasminogen-activator inhibitor 1 (116). In 39 COVID-19 survivors followed for coagulation abnormalities after a year, elevated D-dimer, factor VIII, von-Willebrand factor antigen and interleukin-6 was reported. In a prospective registry study of 4,906 hospitalized patients followed at the mean of 92 days, venous thromboembolic event rates of 1.55% were noted, more than half of which included pulmonary embolism (117). Other smaller studies had thromboembolic events ranging from 0.2 to 2.5% (118–121). Thus, persisting hypercoagulopathy and higher rates of pulmonary thromboembolism have been noted in the post-discharge period in COVID-19 survivors.
Pulmonary hypertension
The vascular remodeling and luminal microthrombi noted in acute SARS-CoV-2 infection raise the suspicion that SARS-CoV-2 infection could be a risk factor for the future development of pulmonary arterial hypertension (122). Thickened pulmonary vascular walls, one of the hallmarks of pulmonary arterial hypertension, were reported in SARS-CoV-2 infection. In an autopsy study, the pulmonary vascular wall thickness was more than twice thicker than those of patients who died from H1N1 influenza (123).
The role of ACE2 in the pathogenesis of pulmonary vascular disease in COVID-19
The vascular endothelium is one of the primary targets of SARS-CoV-2 and the molecular pathways and cellular abnormalities observed in SARS-CoV-2 pulmonary vascular injury are similar to the pathogenesis pathway of pulmonary arterial hypertension (124). Direct viral infection and inflammatory cytokines outbursts are plausible mechanisms for endothelial damage caused by SARS-CoV-2. The host receptor for the virus (ACE2) is widely expressed in endothelial cells. Monteil et al. showed that SARS-CoV-2 can directly infect engineered human blood vessels, which can be inhibited by human recombinant soluble ACE2 (125). Varga et al. demonstrated in an autopsy study that viral particles were present in the endothelial cells of the glomerular capillary loops by electron microscopy of kidney tissue (126).
The presence of ACE2 within normal levels in the lung seems to be essential to combat inflammatory lung disease (127). In PAH, angiotensin II is upregulated and its level correlates with disease severity. Downregulation of ACE2 during SARS-CoV-2 infection can potentially increase angiotensin II circulating levels. Perivascular lymphocytic infiltration has been found in lung biopsies from patients with SARS-CoV-2 infection (125, 126). An autopsy study by Ackermann et al. demonstrated severe endothelial injury in SARS-CoV-2 infection, mediated by the entry of the virus into the endothelial cells, resulting in micro and macrovascular thrombosis. These features are distinct compared to patients who died from ARDS secondary to H1N1 influenza (112). The mechanisms described earlier may also contribute to developing pulmonary arterial hypertension in COVID-19 survivors.
Pulmonary fibrosis and development of pulmonary hypertension in COVID-19
Pulmonary fibrosis can be further complicated by pulmonary hypertension (25), which in turn has been noted to be a major determinant of higher mortality (26). The severity of pulmonary fibrosis is not correlated with the development of pulmonary hypertension, and numerous mechanisms involving dysregulation of molecular pathways resulting in vascular remodeling have been suggested (25). Vascular remodeling is now considered an essential contributor to pulmonary hypertension besides the traditionally known factors of hypoxic vasoconstriction and capillary bed destruction in pulmonary fibrosis. Loss of BMPR2 signaling, upregulation of A2BAR, and endothelial-to-mesenchymal transition have been speculated to be involved in the vascular remodeling process in pulmonary fibrosis (23, 27). The entry of the virus into the endothelial cells of pulmonary capillaries has been implicated in the vascular remodeling in COVID-19. The incidence of pulmonary hypertension in PCILD needs to be studied further. In general, transthoracic echocardiography in chronic lung diseases is insufficient to confirm or rule out pulmonary hypertension (29). Right heart catheterization is considered the gold standard for diagnosing pulmonary hypertension in this population (29). A proposed algorithm for the diagnosis of pulmonary hypertension in PCILD is shown in Figure 2.
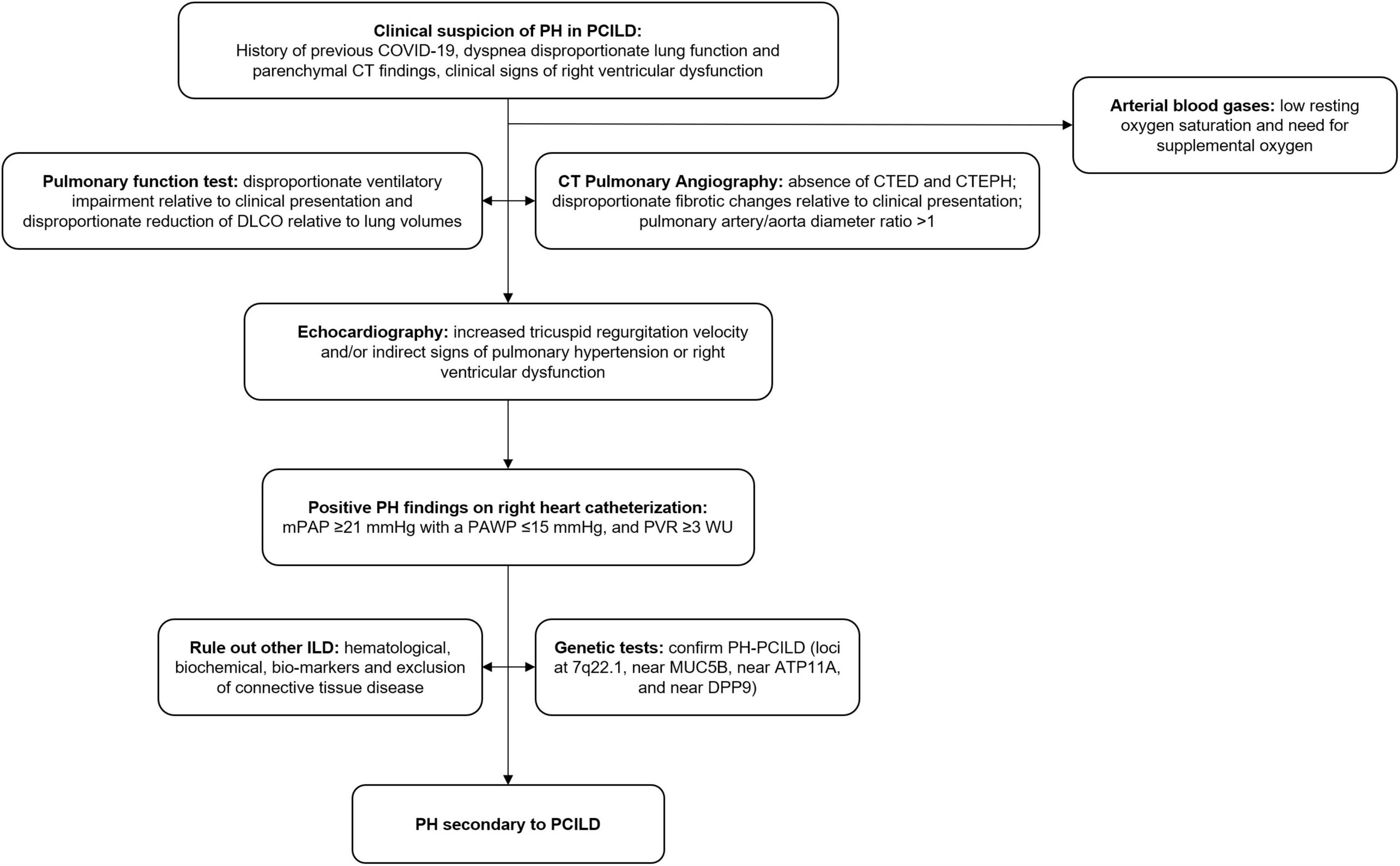
Figure 2. Proposed algorithm for the diagnosis of pulmonary hypertension in PCILD. PH, pulmonary hypertension; PCILD, post-COVID-19 interstitial lung disease; CT, computed tomography; DLCO, diffusion capacity for carbon monoxide; CTED, chronic thromboembolic disease; CTEPH, chronic thromboembolic pulmonary hypertension; mPAP, mean pulmonary arterial pressure; PAWP, pulmonary arterial wedge pressure; PVR, pulmonary vascular resistance; ILD, interstitial lung disease.
Chronic thromboembolic disease and chronic thromboembolic pulmonary hypertension in COVID-19
While most acute pulmonary embolisms and clots resolve with anticoagulation, clot persistence can lead to continued post-embolic symptoms of shortness of breath and the development of chronic thromboembolic disease (CTED). About 30–50% of the patients have persistent defects up to 1 year after diagnosis (128). CTEPH refers to the development of pulmonary hypertension in the setting of CTED. CTEPH is estimated to have a 0.5–5% prevalence after PE (129–132). In the European CTEPH registry, pulmonary endarterectomy mortality rate was 4.7% (133). Additionally, untreated or undiagnosed CTEPH patients have a poor prognosis and a mean pulmonary arterial pressure >30 mmHg is associated with mortality rates >50% in 10 years (134).
In COVID-19 patients, persistent coagulation abnormalities have been noted 4 months after discharge (116, 135), making COVID-19 patients further prone to CTED. Endothelial dysfunction, which can lead to inflammation and thrombosis, is the common pathology in COVID-19 and CTEPH (136). This supports the concept of in situ thrombosis in COVID-19, which is a different phenotype than traditional venous thromboembolism (137). The histological evaluation of lungs in COVID-19 patients showed pulmonary vascular endotheliitis with widespread micro thrombosis (112). Whether severe COVID-19 patients without acute pulmonary embolism and ongoing long-term respiratory symptoms may have developed pulmonary hypertension in the setting of micro-thrombosis needs further evaluation. Dyspnea and prolonged hypoxia being common post-COVID-19 symptoms due to other etiologies such as PCILD, make an early diagnosis of COVID-19-related CTED and CTEPH challenging.
In the absence of scientific evidence specific to COVID-19 for CTED and CTEPH, we recommend standard follow-ups for all patients diagnosed with acute pulmonary embolism during the first 2 years. These patients should be monitored for recurrent thromboembolism and right heart failure symptoms and the optimal timing for initiating diagnostic testing is 3–6 months after acute pulmonary embolism diagnosis (138). Lung ventilation/perfusion scintigraphy (V/Q scan) is the screening test of choice (139). However, residual pulmonary consolidations and fibrosis from post-ARDS changes from COVID-19 might cause abnormalities in ventilation and make interpretation challenging. In such patients, CT pulmonary angiography is a reasonable alternative imaging modality (139, 140). Right heart catheterization is the gold standard confirmation test for CTEPH. Where available, referral to CTEPH centers should be initiated simultaneously with workup once the diagnosis is suspected.
Presently, there are no evidence-based guidelines on the optimal management of CTED and CTEPH in COVID-19 survivors. Most societies recommend a minimum of 3 months of anticoagulation for acute pulmonary embolism and lifelong anticoagulation for CTED or CTEPH (139, 141, 142). If CTEPH is the clinical diagnosis, then pulmonary endarterectomy is the definitive therapy for CTEPH (139). Percutaneous balloon angioplasty shows benefit for inoperable patients and patients with residual CTEPH after endarterectomy (143, 144). For those patients not eligible for endarterectomy or angioplasty, Riociguat is the drug of choice (145). A proposed algorithm for CTED and CTEPH in post-COVID-19 patients is shown in Figure 3.
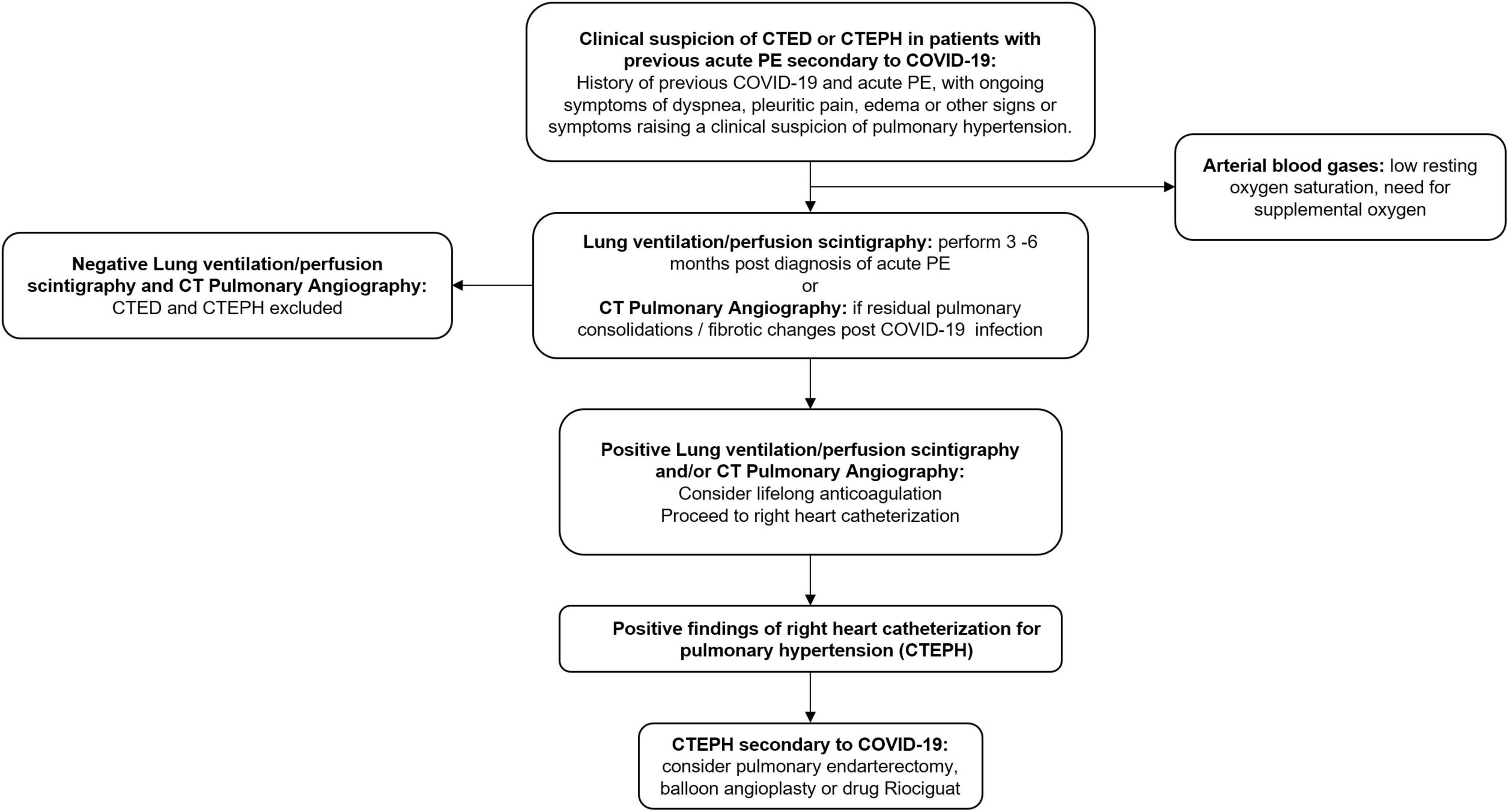
Figure 3. Proposed algorithm for investigation and management of chronic thromboembolic disease and chronic thromboembolic pulmonary hypertension secondary to COVID-19. CTED, chronic thromboembolic disease; CTEPH, chronic thromboembolic pulmonary hypertension; PE, pulmonary embolism; CT, computed tomography.
Post-COVID-19 pulmonary hypertension
Although theoretically, it is expected that post-COVID-19 patients can develop new onset pulmonary hypertension in the setting of interstitial lung disease and pulmonary vasculopathy; however, literature on post-COVID-19 pulmonary hypertension remains scant. We speculate that the complete picture of the burden of post-COVID-19 pulmonary hypertension will be more apparent in the upcoming years, as was the case with HIV and the use of anti-obesity drugs. Additionally, the interaction between parasites such as schistosomiasis in endemic areas and COVID-19 in the development of pulmonary hypertension would be worth exploring further.
Post-COVID-19 pulmonary hypertension has only been mentioned in the setting of case-reports so far. Cueto-Robledo et al. described a case of the development of severe pulmonary hypertension in a patient 3 months post-COVID-19. The finding of pulmonary trunk dilatation on imaging and the echocardiographic results prompted right heart catheterization, which confirmed severe pulmonary hypertension (146). In another case, new pulmonary hypertension was diagnosed on right heart catheterization 6 weeks after discharge from COVID-19 (147). In cases of severe pulmonary hypertension, right heart catheterization will be essential to confirm the pressure readings in the pulmonary vasculature and also to determine further if there is a component of WHO group 1 pulmonary arterial hypertension in addition to WHO group 3 pulmonary hypertension due to PCILD. Pulmonary arterial hypertension-specific therapy or pulmonary arterial vasodilators are rarely indicated in group 3 pulmonary hypertension unless the right heart catheterization findings and pulmonary function testing, demonstrate pulmonary hypertension out of proportion to the chronic lung disease (146, 148). Such patients should be referred to pulmonary hypertension centers for expert-opinion.
The role of pulmonary rehabilitation in post-COVID-19
Pathogenesis of exercise intolerance in post-COVID-19
Most of the evidence regarding the etiology of exercise intolerance in post-COVID syndrome comes from the cardiopulmonary exercise tests, which have been summarized in Table 2. Based on these studies, it has been suggested that exercise intolerance could result from deconditioning, defined as loss of physical fitness due to the inability to maintain an optimal level of physical activity or training (16, 149–158). In cardiopulmonary exercise findings, deconditioning is the reduction of peak oxygen uptake in the absence of known central and peripheral cardiocirculatory diseases. However, post-COVID-19 exercise intolerance may be attributed to impaired peripheral oxygen utilization or extraction due to mitochondrial injury. Evaluating patients with persistent symptoms after COVID-19 infection, Singh et al. elegantly demonstrated through an invasive cardiopulmonary exercise test that oxygen delivery was normal; however, reduced peripheral oxygen extraction and uptake were noted, indicating lower diffusive oxygen delivery to the mitochondria (159). Corroborating peripheral muscle impairment in the setting of myopathy due to inflammatory changes and mitochondrial cellular respiration dysfunction, a case series of 16 patients post-COVID-19 who were evaluated for fatigue and myalgia and who performed muscle biopsy, had muscle fiber atrophy (38%) with signs of regeneration (56%) and mitochondrial and inflammatory changes(62%) (160). This may be the result of myopathy because of viral injury, which may be responsible for the persistence of fatigue in long COVID. Nonetheless, a more recent report demonstrated that in post-COVID-19 patients with fatigue vs. non-fatigue, the only difference in cardiopulmonary exercise test was lower peak oxygen uptake (ml/kg/min), without other noticeable differences in exercise responses (161).
Ventilatory inefficiencies such as an increase in the minute ventilation to carbon dioxide output ratio during exercise or an increase in the dead space to tidal volume observed in the studies may explain the persistent dyspneic symptoms in the long COVID patients (151, 152, 159, 162). These findings can result from increased central chemosensitivity, dysfunctional breathing, and persistent pulmonary or microvascular injury.
Pulmonary rehabilitation in post-COVID syndrome
After hospital discharge, patients need to follow-up with a multidisciplinary approach to control and improve their symptoms and sequelae. For this reason, referring the patient with persistent symptoms after COVID-19 infection to a pulmonary rehabilitation program is crucial to accelerate the improvement in symptoms and health status and allow the patient to return to a productive life. The main goal of the pulmonary rehabilitation program is to restore physical, psychological, and social functions, improve the quality of life in COVID-19 survivors and decrease the incidence of long-term disabilities (163–165). In a large longitudinal cohort in China, within 1 year after acute infection, most hospital survivors with COVID-19 had an excellent physical and functional recovery over the months and had returned to their original work and life (166). Therefore, follow-up evaluations are needed, and rehabilitation might be helpful in post-COVID-19 patients.
Each patient will need a specific program based on education, self-management, and exercise training. However, data on the efficacy of particular rehabilitation approaches in the acute and post-acute phases are still scarce (167–174). An open randomized clinical trial comprising 72 elderly patients more than 6 months after contracting COVID-19 showed an improvement in pulmonary function, exercise capacity, and quality of life with 6-week pulmonary rehabilitation therapy (174). Other cohort studies yielded similar results (175, 176).
In terms of rehabilitation modality, a randomized controlled trial showed that low-intensity aerobic training combined with resistance training has better effects on handgrip strength, kinesiophobia status, and quality of life than high-intensity aerobic training combined with resistance training in post-COVID-19 older adults with sarcopenia. However, the intragroup analysis showed that both groups had significant improvement in the muscle bulk irrespective of exercise intensity (172). With the relocation of health professionals from outpatient activities to hospitals (176, 177), telerehabilitation was used to provide a much-needed resource to address the needs of COVID-19 survivors. In a prospectively randomized program, patients allocated to virtual and home physical therapy had improved outcomes (168). A more robust study was performed to compare supervised home telerehabilitation program with no rehabilitation for post-COVID-19. In this trial, the tele-rehabilitation program was superior to no rehabilitation in terms of functional exercise capacity by six-minute walk test, dyspnea-free symptoms, and physical quality of life. (170).
Before starting rehabilitation therapy, it is essential to evaluate the exercise capacity, which could be done by field tests, such as the 6-min walking test, shuttle walking test, step test, etc., or a more complex test, such as cardiopulmonary exercise testing. Patients should be reevaluated in 6–12 weeks. Although the exercise program is essential, which includes aerobic and strengthening exercises for peripheral and respiratory muscles, it is vital to include breathing retraining, airway clearance, energy conservation techniques, and psychological counseling (163, 165, 178–181). Referring the patient with persistent symptoms after COVID-19 infection to a rehabilitation program is crucial to accelerate the improvement in symptoms and health status and allow the patient to return to a productive life.
Conclusion
COVID-19 survivors may present signs of cardiopulmonary sequelae such as persistent lung fibrosis, chronic thrombosis, right ventricular dysfunction, pulmonary hypertension, and exercise intolerance. Pulmonary fibrosis is among the most feared chronic pulmonary complications of COVID-19. A diagnosis of PCILD should be based on clinical, radiological, and pathological findings. The role of treatment with antifibrotic and anti-inflammatory drugs in improving PCILD symptoms remains inconclusive. A substantial proportion of patients with COVID-19 have coagulation abnormalities. Acute pulmonary embolism in COVID-19 can lead to the development of CTED and CTEPH or even other forms of pulmonary hypertension, which could increase in prevalence over time during the COVID-19 pandemic. Right ventricular dysfunction is prevalent in patients hospitalized with SARS-CoV-2 and is more likely related to systemic consequences rather than direct viral myocardial infection. In terms of mechanisms of exercise intolerance after COVID-19 infection, exercise limitation can be due to a central or peripheral cardiocirculatory origin, decreased oxygen uptake, and with or without ventilatory or gas exchange limitation. Referring the patient with persistent symptoms after COVID-19 infection to a pulmonary rehabilitation program is crucial to accelerate the improvement in symptoms and health status and allow the patient to return to a productive life. We anticipate that as the pool of patients recovering from COVID-19 continues to increase, healthcare providers will need to learn to recognize these long-term cardiopulmonary sequelae early and create a management plan to prevent further deterioration and improve the quality of life of these patients. Furthermore, carefully designed research programs and long-term monitoring of these patients will help clinicians to manage these patients in the long run.
Author contributions
All authors made substantial contributions to the conception or design of the work; or the acquisition, analysis, or interpretation of data for the work, drafted the work or revising it critically for important intellectual content, provided approval for publication of the content, and agreed to be accountable for all aspects of the work in ensuring that questions related to the accuracy or integrity of any part of the work were appropriately investigated and resolved.
Conflict of interest
The authors declare that the research was conducted in the absence of any commercial or financial relationships that could be construed as a potential conflict of interest.
Publisher’s note
All claims expressed in this article are solely those of the authors and do not necessarily represent those of their affiliated organizations, or those of the publisher, the editors and the reviewers. Any product that may be evaluated in this article, or claim that may be made by its manufacturer, is not guaranteed or endorsed by the publisher.
References
1. Soriano JB, Murthy S, Marshall JC, Relan P, Diaz JV, WHO Clinical Case Definition Working Group. A clinical case definition of post-COVID-19 condition by a Delphi consensus. Lancet Infect Dis. (2022) 22:e102–7. doi: 10.1016/S1473-3099(21)00703-9
3. Daniels CJ, Rajpal S, Greenshields JT, Rosenthal GL, Chung EH, Terrin M, et al. Prevalence of clinical and subclinical myocarditis in competitive athletes with recent SARS-CoV-2 infection: results from the big ten COVID-19 cardiac registry. JAMA Cardiol. (2021) 6:1078–87.
4. Choutka J, Jansari V, Hornig M, Iwasaki A. Unexplained post-acute infection syndromes. Nat Med. (2022) 28:911–23. doi: 10.1038/s41591-022-01810-6
5. González J, Zuil M, Benítez ID, de Gonzalo-Calvo D, Aguilar M, Santisteve S, et al. One year overview and follow-up in a post-COVID consultation of critically ill patients. Front Med. (2022) 9:897990. doi: 10.3389/fmed.2022.897990
6. Carfì A, Bernabei R, Landi F, Gemelli Against Covid-19 Post-Acute Care Study Group. Persistent symptoms in patients after acute COVID-19. JAMA. (2020) 324:603–5. doi: 10.1001/jama.2020.12603
7. Blomberg B, Mohn KG-I, Brokstad KA, Zhou F, Linchausen DW, Hansen B-A, et al. Long COVID in a prospective cohort of home-isolated patients. Nat Med. (2021) 27:1607–13. doi: 10.1038/s41591-021-01433-3
8. Carvalho-Schneider C, Laurent E, Lemaignen A, Beaufils E, Bourbao-Tournois C, Laribi S, et al. Follow-up of adults with noncritical COVID-19 two months after symptom onset. Clin Microbiol Infect. (2021) 27:258–63. doi: 10.1016/j.cmi.2020.09.052
9. Tenforde MW, Kim SS, Lindsell CJ, Billig Rose E, Shapiro NI, Files DC, et al. Symptom duration and risk factors for delayed return to usual health among outpatients with COVID-19 in a multistate health care systems network—United States, March–June 2020. Morb Mortal Wkly Rep Cent Dis Control Prev. (2020) 69:993–8. doi: 10.15585/mmwr.mm6930e1
10. Azevedo RB, Botelho BG, de Hollanda JVG, Ferreira LVL, Junqueira de Andrade LZ, Oei SSML, et al. Covid-19 and the cardiovascular system: a comprehensive review. J Hum Hypertens. (2021) 35:4–11. doi: 10.1038/s41371-020-0387-4
11. Xie Y, Xu E, Bowe B, Al-Aly Z. Long-term cardiovascular outcomes of COVID-19. Nat Med. (2022) 28:583–90. doi: 10.1038/s41591-022-01689-3
12. Wang W, Wang C-Y, Wang S-I, Wei JC-C. Long-term cardiovascular outcomes in COVID-19 survivors among non-vaccinated population: a retrospective cohort study from the TriNetX US collaborative networks. EClinicalMedicine (2022) 53:101619. doi: 10.1016/j.eclinm.2022.101619
13. Maestre-Muñiz MM, Arias Á, Mata-Vázquez E, Martín-Toledano M, López-Larramona G, Ruiz-Chicote AM, et al. Long-term outcomes of patients with coronavirus disease 2019 at one year after hospital discharge. J Clin Med MDPI. (2021) 10:2945. doi: 10.3390/jcm10132945
14. Ayoubkhani D, Khunti K, Nafilyan V, Maddox T, Humberstone B, Diamond I, et al. Post-covid syndrome in individuals admitted to hospital with covid-19: retrospective cohort study. BMJ. (2021) 372:n693. doi: 10.1136/bmj.n693
15. Raman B, Bluemke DA, Lüscher TF, Neubauer S. Long COVID: post-acute sequelae of COVID-19 with a cardiovascular focus. Eur Heart J. (2022) 43:1157–72. doi: 10.1093/eurheartj/ehac031
16. Raman B, Cassar MP, Tunnicliffe EM, Filippini N, Griffanti L, Alfaro-Almagro F, et al. Medium-term effects of SARS-CoV-2 infection on multiple vital organs, exercise capacity, cognition, quality of life and mental health, post-hospital discharge. EClinicalMedicine. (2021) 31:100683. doi: 10.1016/j.eclinm.2020.100683
17. Radin JM, Quer G, Ramos E, Baca-Motes K, Gadaleta M, Topol EJ, et al. Assessment of prolonged physiological and behavioral changes associated with COVID-19 infection. JAMA Netw Open. (2021) 4:e2115959. doi: 10.1001/jamanetworkopen.2021.15959
18. Blitshteyn S, Whitelaw S. Postural orthostatic tachycardia syndrome (POTS) and other autonomic disorders after COVID-19 infection: a case series of 20 patients. Immunol Res. (2021) 69:205–11. doi: 10.1007/s12026-021-09185-5
19. Tanacli R, Doeblin P, Götze C, Zieschang V, Faragli A, Stehning C, et al. COVID-19 vs. classical myocarditis associated myocardial injury evaluated by cardiac magnetic resonance and endomyocardial biopsy. Front Cardiovasc Med. (2021) 8:737257. doi: 10.3389/fcvm.2021.737257
20. Modica G, Bianco M, Sollazzo F, Di Murro E, Monti R, Cammarano M, et al. Myocarditis in athletes recovering from covid-19: a systematic review and meta-analysis. Int J Environ Res Public Health MDPI. (2022) 19:4279. doi: 10.3390/ijerph19074279
21. Blagova O, Ainetdinova DH, Lutokhina YUA, Novosadov VM, Rud’ RS, Zaitsev AYU, et al. Post-COVID myocarditis diagnosed by endomyocardial biopsy and/or magnetic resonance imaging 2–9 months after acute COVID-19. Eur Heart J. (2021) 42:1751. doi: 10.1093/eurheartj/ehab724.1751
22. Halushka MK, Vander Heide RS. Myocarditis is rare in COVID-19 autopsies: cardiovascular findings across 277 postmortem examinations. Cardiovasc Pathol. (2021) 50:107300. doi: 10.1016/j.carpath.2020.107300
23. Szekely Y, Lichter Y, Taieb P, Banai A, Hochstadt A, Merdler I, et al. Spectrum of cardiac manifestations in COVID-19: a systematic echocardiographic study. Circulation. (2020) 142:342–53. doi: 10.1161/CIRCULATIONAHA.120.047971
24. Zhou F, Yu T, Du R, Fan G, Liu Y, Liu Z, et al. Clinical course and risk factors for mortality of adult inpatients with COVID-19 in Wuhan, China: a retrospective cohort study. Lancet. (2020) 395:1054–62. doi: 10.1016/S0140-6736(20)30566-3
25. Bhatraju PK, Ghassemieh BJ, Nichols M, Kim R, Jerome KR, Nalla AK, et al. Covid-19 in critically ill patients in the seattle region–case series. N Engl J Med (2020) 382:2012–22. doi: 10.1056/NEJMoa2004500
26. Cao Y, Zhang M, Guo Y, Zhang Y. The overlooked chamber in coronavirus disease 2019. ESC Heart Fail. (2020) 7:3483–6. doi: 10.1002/ehf2.12976
27. Argulian E, Sud K, Vogel B, Bohra C, Garg VP, Talebi S, et al. Right ventricular dilation in hospitalized patients with covid-19 infection. JACC Cardiovasc Imaging. (2020) 13:2459–61. doi: 10.1016/j.jcmg.2020.05.010
28. Li Y, Li H, Zhu S, Xie Y, Wang B, He L, et al. Prognostic value of right ventricular longitudinal strain in patients with COVID-19. JACC Cardiovasc Imaging. (2020) 13:2287–99. doi: 10.1016/j.jcmg.2020.04.014
29. Paternoster G, Bertini P, Innelli P, Trambaiolo P, Landoni G, Franchi F, et al. Right ventricular dysfunction in patients with COVID-19: a systematic review and meta-analysis. J Cardiothorac Vasc Anesth. (2021) 35:3319–24. doi: 10.1053/j.jvca.2021.04.008
30. Park JF, Banerjee S, Umar S. In the eye of the storm: the right ventricle in COVID-19. Pulm Circ. (2020) 10:2045894020936660. doi: 10.1177/2045894020936660
31. Hendren NS, Drazner MH, Bozkurt B, Cooper LT. Description and proposed management of the acute COVID-19 cardiovascular syndrome. Circulation. (2020) 141:1903–14. doi: 10.1161/CIRCULATIONAHA.120.047349
32. Fox SE, Li G, Akmatbekov A, Harbert JL, Lameira FS, Brown JQ, et al. Unexpected features of cardiac pathology in covid-19 infection. Circulation. (2020) 142:1123–5. doi: 10.1161/CIRCULATIONAHA.120.049465
33. Huang L, Zhao P, Tang D, Zhu T, Han R, Zhan C, et al. Cardiac involvement in patients recovered from covid-2019 identified using magnetic resonance imaging. JACC Cardiovasc Imaging. (2020) 13:2330–9. doi: 10.1016/j.jcmg.2020.05.004
34. Rieg AD, Suleiman S, Perez-Bouza A, Braunschweig T, Spillner JW, Schröder T, et al. Milrinone relaxes pulmonary veins in guinea pigs and humans. PLoS One. (2014) 9:e87685. doi: 10.1371/journal.pone.0087685
35. Mathew R, Di Santo P, Jung RG, Marbach JA, Hutson J, Simard T, et al. Milrinone as compared with dobutamine in the treatment of cardiogenic shock. N Engl J Med. (2021) 385:516–25. doi: 10.1056/NEJMoa2026845
36. Feng W-X, Yang Y, Wen J, Liu Y-X, Liu L, Feng C. Implication of inhaled nitric oxide for the treatment of critically ill COVID-19 patients with pulmonary hypertension. ESC Heart Fail. (2021) 8:714–8. doi: 10.1002/ehf2.13023
37. Kerbaul F, Rondelet B, Demester J-P, Fesler P, Huez S, Naeije R, et al. Effects of levosimendan versus dobutamine on pressure load-induced right ventricular failure*. Crit Care Med. (2006) 34:2814–9. doi: 10.1097/01.CCM.0000242157.19347.50
38. Slawsky MT, Colucci WS, Gottlieb SS, Greenberg BH, Haeusslein E, Hare J, et al. Acute hemodynamic and clinical effects of levosimendan in patients with severe heart failure, study investigators. Circulation. (2000) 102:2222–7. doi: 10.1161/01.CIR.102.18.2222
39. Russ MA, Prondzinsky R, Carter JM, Schlitt A, Ebelt H, Schmidt H, et al. Right ventricular function in myocardial infarction complicated by cardiogenic shock: improvement with levosimendan. Crit Care Med. (2009) 37:3017–23. doi: 10.1097/CCM.0b013e3181b0314a
40. Alibaz-Oner F, Gurbuz OZ, Oner E, Yurdakul S, Erguney M. Impact of levosimendan on right ventricular functions by using novel tissue Doppler derived indices in patients with ischaemic left ventricular failure. Kardiol Pol. (2013) 71:1036–41. doi: 10.5603/KP.2013.0258
41. Hansen MS, Andersen A, Nielsen-Kudsk JE. Levosimendan in pulmonary hypertension and right heart failure. Pulm Circ. (2018) 8:204589401879090. doi: 10.1177/2045894018790905
42. Lorusso R, Raffa GM, Heuts S, Lo Coco V, Meani P, Natour E, et al. Pulmonary artery cannulation to enhance extracorporeal membrane oxygenation management in acute cardiac failure. Interact Cardiovasc Thorac Surg. (2020) 30:215–22. doi: 10.1093/icvts/ivz245
43. Mustafa AK, Alexander PJ, Joshi DJ, Tabachnick DR, Cross CA, Pappas PS, et al. Extracorporeal membrane oxygenation for patients with covid-19 in severe respiratory failure. JAMA Surg. (2020) 155:990–2. doi: 10.1001/jamasurg.2020.3950
44. Haddad F, Doyle R, Murphy DJ, Hunt SA. Right ventricular function in cardiovascular disease, part II: pathophysiology, clinical importance, and management of right ventricular failure. Circ Am Heart Assoc. (2008) 117:1717–31. doi: 10.1161/CIRCULATIONAHA.107.653584
45. Arrigo M, Huber LC, Winnik S, Mikulicic F, Guidetti F, Frank M, et al. Right ventricular failure: pathophysiology, diagnosis and treatment. Cardiac Fail Rev. (2019) 5:140. doi: 10.15420/cfr.2019.15.2
46. Ameri P, Inciardi RM, Di Pasquale M, Agostoni P, Bellasi A, Camporotondo R, et al. Pulmonary embolism in patients with COVID-19: characteristics and outcomes in the Cardio-COVID Italy multicenter study. Clin Res Cardiol. (2021) 110:1020–8. doi: 10.1007/s00392-020-01766-y
47. Hsieh C-M, Mishkel GJ, Cardoso PFG, Rakowski H, Dunn SC, Butany J, et al. Production and reversibility of right ventricular hypertrophy and right heart failure in dogs. Ann Thorac Surg. (1992) 54:104–10. doi: 10.1016/0003-4975(92)91152-Y
48. Brooks H, Kirk ES, Vokonas PS, Urschel CW, Sonnenblick EH. Performance of the right ventricle under stress: relation to right coronary flow. Am Soc Clin Investig. (1971) 50:2176–83. doi: 10.1172/JCI106712
49. Bonnet P, Bonnet S, Boissière J, Net J-LL, Gautier M, Dumas de la Roque E, et al. Chronic hypoxia induces nonreversible right ventricle dysfunction and dysplasia in rats. Am J Physiol Heart Circ Physiol. (2004) 287:H1023–8. doi: 10.1152/ajpheart.00802.2003
50. Vonk Noordegraaf A, Westerhof BE, Westerhof N. The relationship between the right ventricle and its load in pulmonary hypertension. J Am Coll Cardiol. (2017) 69:236–43. doi: 10.1016/j.jacc.2016.10.047
51. Solomon JJ, Heyman B, Ko JP, Condos R, Lynch DA. CT of post-acute lung complications of COVID-19. Radiology. (2021) 301:E383–95. doi: 10.1148/radiol.2021211396
52. Froidure A, Mahsouli A, Liistro G, De Greef J, Belkhir L, Gérard L, et al. Integrative respiratory follow-up of severe COVID-19 reveals common functional and lung imaging sequelae. Respir Med. (2021) 181:106383. doi: 10.1016/j.rmed.2021.106383
53. Bellan M, Soddu D, Balbo PE, Baricich A, Zeppegno P, Avanzi GC, et al. Respiratory and psychophysical sequelae among patients with covid-19 four months after hospital discharge. JAMA Netw Open. (2021) 4:e2036142. doi: 10.1001/jamanetworkopen.2020.36142
54. Cortés-Telles A, López-Romero S, Figueroa-Hurtado E, Pou-Aguilar YN, Wong AW, Milne KM, et al. Pulmonary function and functional capacity in COVID-19 survivors with persistent dyspnoea. Respir Physiol Neurobiol. (2021) 288:103644. doi: 10.1016/j.resp.2021.103644
55. Torres-Castro R, Vasconcello-Castillo L, Alsina-Restoy X, Solis-Navarro L, Burgos F, Puppo H, et al. Respiratory function in patients post-infection by COVID-19: a systematic review and meta-analysis. Pulmonology. (2021) 27:328–37. doi: 10.1016/j.pulmoe.2020.10.013
56. Huang C, Huang L, Wang Y, Li X, Ren L, Gu X, et al. 6-month consequences of COVID-19 in patients discharged from hospital: a cohort study. Lancet. (2021) 397:220–32. doi: 10.1016/S0140-6736(20)32656-8
57. De Michele S, Sun Y, Yilmaz MM, Katsyv I, Salvatore M, Dzierba AL, et al. Forty postmortem examinations in covid-19 patients. Am J Clin Pathol. (2020) 154:748–60. doi: 10.1093/ajcp/aqaa156
58. Borczuk AC, Salvatore SP, Seshan SV, Patel SS, Bussel JB, Mostyka M, et al. COVID-19 pulmonary pathology: a multi-institutional autopsy cohort from Italy and New York City. Mod Pathol. (2020) 33:2156–68. doi: 10.1038/s41379-020-00661-1
59. Nalbandian A, Sehgal K, Gupta A, Madhavan MV, McGroder C, Stevens JS, et al. Post-acute COVID-19 syndrome. Nat Med. (2021) 27:601–15. doi: 10.1038/s41591-021-01283-z
60. Yu QY, Tang XX. Irreversibility of pulmonary fibrosis. Aging Dis JKL Int LLC. (2022) 13:73. doi: 10.14336/AD.2021.0730
61. Han X, Fan Y, Alwalid O, Li N, Jia X, Yuan M, et al. Six-month follow-up chest CT findings after severe COVID-19 pneumonia. Radiology. (2021) 299:E177–86. doi: 10.1148/radiol.2021203153
62. Wells AU, Devaraj A, Desai SR. Interstitial lung disease after COVID-19 infection: a catalog of uncertainties. Radiology. (2021) 299:E216–8. doi: 10.1148/radiol.2021204482
63. Garg M, Maralakunte M, Dhooria S. Sequelae of COVID-19 pneumonia: is it correct to label everything as post-COVID lung fibrosis? J Postgrad Med. (2021) 67:224–7. doi: 10.4103/jpgm.jpgm_550_21
64. Hu Q, Guan H, Sun Z, Huang L, Chen C, Ai T, et al. Early CT features and temporal lung changes in COVID-19 pneumonia in Wuhan, China. Eur J Radiol. (2020) 128:109017. doi: 10.1016/j.ejrad.2020.109017
65. Bazdyrev E, Rusina P, Panova M, Novikov F, Grishagin I, Nebolsin V. Lung fibrosis after COVID-19: treatment prospects. Pharmaceuticals (Basel). (2021) 14:807. doi: 10.3390/ph14080807
66. Vasarmidi E, Tsitoura E, Spandidos DA, Tzanakis N, Antoniou KM. Pulmonary fibrosis in the aftermath of the COVID-19 era (Review). Exp Ther Med. (2020) 20:2557–60. doi: 10.3892/etm.2020.8980
67. Wu Q, Zhong L, Li H, Guo J, Li Y, Hou X, et al. Follow-up study of lung function and chest computed tomography at 6 months after discharge in patients with coronavirus disease 2019. Can Respir J. (2021) 2021:6692409. doi: 10.1155/2021/6692409
68. Huang W, Wu Q, Chen Z, Xiong Z, Wang K, Tian J, et al. The potential indicators for pulmonary fibrosis in survivors of severe COVID-19. J Infect. (2021) 82:e5–7. doi: 10.1016/j.jinf.2020.09.027
69. Zou J-N, Sun L, Wang B-R, Zou Y, Xu S, Ding Y-J, et al. The characteristics and evolution of pulmonary fibrosis in COVID-19 patients as assessed by AI-assisted chest HRCT. PLoS One. (2021) 16:e0248957. doi: 10.1371/journal.pone.0248957
70. Li X, Shen C, Wang L, Majumder S, Zhang D, Deen MJ, et al. Pulmonary fibrosis and its related factors in discharged patients with new corona virus pneumonia: a cohort study. Respir Res. (2021) 22:203. doi: 10.1186/s12931-021-01798-6
71. Doane JJ, Hirsch KS, Baldwin JO, Wurfel MM, Pipavath SN, West TE. Progressive pulmonary fibrosis after non-critical covid-19: a case report. Am J Case Rep. (2021) 22:e933458. doi: 10.12659/AJCR.933458
72. Udwadia ZF, Koul PA, Richeldi L. Post-COVID lung fibrosis: the tsunami that will follow the earthquake. Lung India. (2021) 38:S41–7. doi: 10.4103/lungindia.lungindia_818_20
73. Hall DJ, Schulte JJ, Lewis EE, Bommareddi SR, Rohrer CT, Sultan S, et al. Successful lung transplantation for severe post covid-19 pulmonary fibrosis. Ann Thorac Surg. (2021) 114:e17–9. doi: 10.1016/j.athoracsur.2021.10.004
74. Hui DS, Joynt GM, Wong KT, Gomersall CD, Li TS, Antonio G, et al. Impact of severe acute respiratory syndrome (SARS) on pulmonary function, functional capacity and quality of life in a cohort of survivors. Thorax. (2005) 60:401–9. doi: 10.1136/thx.2004.030205
75. Wong K, Antonio GE, Hui DS, Ho C, Chan P, Ng W, et al. Severe acute respiratory syndrome: thin-section computed tomography features, temporal changes, and clinicoradiologic correlation during the convalescent period. J Comput Assist Tomogr. (2004) 28:790–5. doi: 10.1097/00004728-200411000-00010
76. Zhang P, Li J, Liu H, Han N, Ju J, Kou Y, et al. Correction: long-term bone and lung consequences associated with hospital-acquired severe acute respiratory syndrome: a 15-year follow-up from a prospective cohort study. Bone Res. (2020) 8:1–1. doi: 10.1038/s41413-020-00113-1
77. Flaifel A, Kwok B, Ko J, Chang S, Smith D, Zhou F, et al. pulmonary pathology of end-stage covid-19 disease in explanted lungs and outcomes after lung transplantation. Am J Clin Pathol. (2022) 157:908–26. doi: 10.1093/ajcp/aqab208
78. Guler SA, Ebner L, Aubry-Beigelman C, Bridevaux P-O, Brutsche M, Clarenbach C, et al. Pulmonary function and radiological features 4 months after COVID-19: first results from the national prospective observational Swiss COVID-19 lung study. Eur Respir J. (2021) 57:2003690. doi: 10.1183/13993003.03690-2020
79. Zapol WM, Trelstad RL, Coffey JW, Tsai I, Salvador RA. Pulmonary fibrosis in severe acute respiratory failure. Am Rev Respir Dis. (1979) 119:547–54.
80. Marshall R, Bellingan G, Laurent G. The acute respiratory distress syndrome: fibrosis in the fast lane. Thorax. (1998) 53:815–7. doi: 10.1136/thx.53.10.815
81. Thille AW, Esteban A, Fernández-Segoviano P, Rodriguez J-M, Aramburu J-A, Vargas-Errázuriz P, et al. Chronology of histological lesions in acute respiratory distress syndrome with diffuse alveolar damage: a prospective cohort study of clinical autopsies. Lancet Respir Med. (2013) 1:395–401. doi: 10.1016/S2213-2600(13)70053-5
82. Senzi A, Bindi M, Cappellini I, Zamidei L, Consales G. COVID-19 and VILI: developing a mobile app for measurement of mechanical power at a glance. Intensive Care Med Exp. (2021) 9:6. doi: 10.1186/s40635-021-00372-0
83. McGroder CF, Zhang D, Choudhury MA, Salvatore MM, D’Souza BM, Hoffman EA, et al. Pulmonary fibrosis 4 months after COVID-19 is associated with severity of illness and blood leucocyte telomere length. Thorax. (2021) 76:1242–5. doi: 10.1136/thoraxjnl-2021-217031
84. Farghaly S, Badedi M, Ibrahim R, Sadhan MH, Alamoudi A, Alnami A, et al. Clinical characteristics and outcomes of post-COVID-19 pulmonary fibrosis: a case-control study. Medicine (Baltimore). (2022) 101:e28639. doi: 10.1097/MD.0000000000028639
85. Aul DR, Gates DJ, Draper DA, Dunleavy DA, Ruickbie DS, Meredith DH, et al. Complications after discharge with COVID-19 infection and risk factors associated with development of post-COVID pulmonary fibrosis. Respir Med. (2021) 188:106602. doi: 10.1016/j.rmed.2021.106602
86. Jutant E-M, Meyrignac O, Beurnier A, Jaïs X, Pham T, Morin L, et al. Respiratory symptoms and radiologic findings in post-acute COVID-19 syndrome. ERJ Open Res. (2021) 8:00479–2021. doi: 10.1183/23120541.00479-2021
87. Yu M, Liu Y, Xu D, Zhang R, Lan L, Xu H. Prediction of the development of pulmonary fibrosis using serial thin-section CT and clinical features in patients discharged after treatment for covid-19 pneumonia. Korean J Radiol. (2020) 21:746–55. doi: 10.3348/kjr.2020.0215
88. Wallis TJM, Heiden E, Horno J, Welham B, Burke H, Freeman A, et al. Risk factors for persistent abnormality on chest radiographs at 12-weeks post hospitalisation with PCR confirmed COVID-19. Respir Res. (2021) 22:157. doi: 10.1186/s12931-021-01750-8
89. Moodley YP, Scaffidi AK, Misso NL, Keerthisingam C, McAnulty RJ, Laurent GJ, et al. Fibroblasts isolated from normal lungs and those with idiopathic pulmonary fibrosis differ in interleukin-6/gp130-mediated cell signaling and proliferation. Am J Pathol. (2003) 163:345–54. doi: 10.1016/S0002-9440(10)63658-9
90. Vianello A, Guarnieri G, Braccioni F, Lococo S, Molena B, Cecchetto A, et al. The pathogenesis, epidemiology and biomarkers of susceptibility of pulmonary fibrosis in COVID-19 survivors. Clin Chem Lab Med. (2022) 60:307–16. doi: 10.1515/cclm-2021-1021
91. Pandolfi L, Bozzini S, Frangipane V, Percivalle E, De Luigi A, Violatto MB, et al. Neutrophil extracellular traps induce the epithelial-mesenchymal transition: implications in post-COVID-19 fibrosis. Front Immunol. (2021) 12:663303. doi: 10.3389/fimmu.2021.663303
92. Gavillet M, Martinod K, Renella R, Wagner DD, Williams DA. A key role for Rac and Pak signaling in neutrophil extracellular traps (NETs) formation defines a new potential therapeutic target. Am J Hematol. (2018) 93:269–76. doi: 10.1002/ajh.24970
93. Fadista J, Kraven LM, Karjalainen J, Andrews SJ, Geller F, Covid-19 Host Genetics Initiative, et al. Shared genetic etiology between idiopathic pulmonary fibrosis and COVID-19 severity. EBioMedicine. (2021) 65:103277. doi: 10.1016/j.ebiom.2021.103277
94. Allen RJ, Guillen-Guio B, Croot E, Kraven LM, Moss S, Stewart I, et al. Genetic overlap between idiopathic pulmonary fibrosis and COVID-19. medRxiv. (2021) [Preprint]. doi: 10.1183/13993003.03132-2021
95. Raghu G, Wilson KC. COVID-19 interstitial pneumonia: monitoring the clinical course in survivors. Lancet Respir Med. (2020) 8:839–42. doi: 10.1016/S2213-2600(20)30349-0
96. Lee JH, Yim J-J, Park J. Pulmonary function and chest computed tomography abnormalities 6–12 months after recovery from COVID-19: a systematic review and meta-analysis. Respir Res. (2022) 23:1–16. doi: 10.1186/s12931-022-02163-x
97. Colarusso C, Maglio A, Terlizzi M, Vitale C, Molino A, Pinto A, et al. Post-COVID-19 patients who develop lung fibrotic-like changes have lower circulating levels of ifn-β but higher levels of il-1α and TGF-β. Biomedicines. (2021) 9:1931. doi: 10.3390/biomedicines9121931
98. George PM, Wells AU, Jenkins RG. Pulmonary fibrosis and COVID-19: the potential role for antifibrotic therapy. Lancet Respir Med. (2020) 8:807–15. doi: 10.1016/S2213-2600(20)30225-3
99. Rumende CM, Susanto EC, Sitorus TP. The management of pulmonary fibrosis in COVID-19. Acta Med Indones. (2021) 53:233–41.
100. Cesarone MR, Hu S, Belcaro G, Cornelli U, Feragalli B, Corsi M, et al. Pycnogenol®-Centellicum® supplementation improves lung fibrosis and post-COVID-19 lung healing. Minerva Med. (2022) 113:135–40. doi: 10.23736/S0026-4806.20.07225-0
101. Dhooria S, Chaudhary S, Sehgal IS, Agarwal R, Arora S, Garg M, et al. High-dose versus low-dose prednisolone in symptomatic patients with post-COVID-19 diffuse parenchymal lung abnormalities: an open-label, randomised trial (the COLDSTER trial). Eur Respir J. (2022) 59:2102930. doi: 10.1183/13993003.02930-2021
102. Myall KJ, Mukherjee B, Castanheira AM, Lam JL, Benedetti G, Mak SM, et al. Persistent post-COVID-19 interstitial lung disease. An observational study of corticosteroid treatment. Ann Am Thorac Soc. (2021) 18:799–806. doi: 10.1513/AnnalsATS.202008-1002OC
103. Kostorz-Nosal S, Jastrzêbski D, Chyra M, Kubicki P, Zieliñski M, Ziora D. A prolonged steroid therapy may be beneficial in some patients after the COVID-19 pneumonia. Eur Clin Respir J. (2021) 8:1945186. doi: 10.1080/20018525.2021.1945186
104. Segala FV, Sgalla G, Salvati F, Berardini L, Negri M, Nardella E, et al. Adjunctive corticosteroid treatment for organizing pneumonia in COVID-19 patients with persistent respiratory failure. Respir Med. (2021) 187:106571. doi: 10.1016/j.rmed.2021.106571
105. Shen H, Zhang N, Liu Y, Yang X, He Y, Li Q, et al. The interaction between pulmonary fibrosis and COVID-19 and the application of related anti-fibrotic drugs. Front Pharmacol. (2021) 12:805535. doi: 10.3389/fphar.2021.805535
106. Tang N, Li D, Wang X, Sun Z. Abnormal coagulation parameters are associated with poor prognosis in patients with novel coronavirus pneumonia. J Thromb Haemost. (2020) 18:844–7. doi: 10.1111/jth.14768
107. Guan W-J, Ni Z-Y, Hu Y, Liang W-H, Ou C-Q, He J-X, et al. Clinical characteristics of coronavirus disease 2019 in China. N Engl J Med. (2020) 382:1708–20. doi: 10.1056/NEJMoa2002032
108. Naymagon L, Zubizarreta N, Feld J, van Gerwen M, Alsen M, Thibaud S, et al. Admission D-dimer levels, D-dimer trends, and outcomes in COVID-19. Thromb Res. (2020) 196:99–105. doi: 10.1016/j.thromres.2020.08.032
109. Huang C, Wang Y, Li X, Ren L, Zhao J, Hu Y, et al. Clinical features of patients infected with 2019 novel coronavirus in Wuhan, China. Lancet. (2020) 395:497–506. doi: 10.1016/S0140-6736(20)30183-5
110. Panigada M, Bottino N, Tagliabue P, Grasselli G, Novembrino C, Chantarangkul V, et al. Hypercoagulability of COVID-19 patients in intensive care unit: a report of thromboelastography findings and other parameters of hemostasis. J Thromb Haemost. (2020) 18:1738–42. doi: 10.1111/jth.14850
111. Levi M, Thachil J, Iba T, Levy JH. Coagulation abnormalities and thrombosis in patients with COVID-19. Lancet Haematol. (2020) 7:e438–40. doi: 10.1016/S2352-3026(20)30145-9
112. Ackermann M, Verleden SE, Kuehnel M, Haverich A, Welte T, Laenger F, et al. Pulmonary vascular endothelialitis, thrombosis, and angiogenesis in Covid-19. N Engl J Med. (2020) 383:120–8. doi: 10.1056/NEJMoa2015432
113. Burn E, Duarte-Salles T, Fernandez-Bertolin S, Reyes C, Kostka K, Delmestri A, et al. Venous or arterial thrombosis and deaths among COVID-19 cases: a European network cohort study. Lancet Infect Dis. (2022) 8:1142–52. doi: 10.1016/S1473-3099(22)00223-7
114. Gerotziafas GT, Van Dreden P, Sergentanis TN, Politou M, Rousseau A, Grusse M, et al. Persisting Endothelial cell activation and hypercoagulability after COVID-19 recovery–the prospective observational ROADMAP-post COVID-19 study. Hemato. (2022) 3:111–21. doi: 10.3390/hemato3010010
115. Willems LH, Nagy M, Ten Cate H, Spronk HMH, Groh LA, Leentjens J, et al. Sustained inflammation, coagulation activation and elevated endothelin-1 levels without macrovascular dysfunction at 3 months after COVID-19. Thrombos Res. (2022) 209:106–14. doi: 10.1016/j.thromres.2021.11.027
116. von Meijenfeldt FA, Havervall S, Adelmeijer J, Lundström A, Magnusson M, Mackman N, et al. Sustained prothrombotic changes in COVID-19 patients 4 months after hospital discharge. Blood Adv. (2021) 5:756–9. doi: 10.1182/bloodadvances.2020003968
117. Giannis D, Allen SL, Tsang J, Flint S, Pinhasov T, Williams S, et al. Postdischarge thromboembolic outcomes and mortality of hospitalized patients with COVID-19: the CORE-19 registry. Blood. (2021) 137:2838–47. doi: 10.1182/blood.2020010529
118. Roberts LN, Whyte MB, Georgiou L, Giron G, Czuprynska J, Rea C, et al. Postdischarge venous thromboembolism following hospital admission with COVID-19. Blood Am Soc Hematol. (2020) 136:1347–50. doi: 10.1182/blood.2020008086
119. Patell R, Bogue T, Koshy A, Bindal P, Merrill M, Aird WC, et al. Postdischarge thrombosis and hemorrhage in patients with COVID-19. Blood Am Soc Hematol. (2020) 136:1342–6. doi: 10.1182/blood.2020007938
120. Rashidi F, Barco S, Kamangar F, Heresi GA, Emadi A, Kaymaz C, et al. Incidence of symptomatic venous thromboembolism following hospitalization for coronavirus disease 2019: prospective results from a multi-center study. Thromb Res. (2021) 198:135–8. doi: 10.1016/j.thromres.2020.12.001
121. Engelen MM, Vandenbriele C, Balthazar T, Claeys E, Gunst J, Guler I, et al. Venous thromboembolism in patients discharged after COVID-19 hospitalization. Semin Thromb Hemost. (2021) 47:362–71. doi: 10.1055/s-0041-1727284
122. Suzuki YJ, Nikolaienko SI, Shults NV, Gychka SG. COVID-19 patients may become predisposed to pulmonary arterial hypertension. Med Hypotheses. (2021) 147:110483. doi: 10.1016/j.mehy.2021.110483
123. Suzuki YJ, Nikolaienko SI, Dibrova VA, Dibrova YV, Vasylyk VM, Novikov MY, et al. CoV-2 spike protein-mediated cell signaling in lung vascular cells. Vascul Pharmacol. (2021) 137:106823. doi: 10.1016/j.vph.2020.106823
124. Potus XF, Mai V, Lebret M, Malenfant XS, Breton-gagnon E, Lajoie XAC, et al. Novel insights on the pulmonary vascular consequences of COVID-19. Am J Physiol Lung Cell Mol Physiol. (2020) 319:L277–88. doi: 10.1152/ajplung.00195.2020
125. Monteil V, Kwon H, Prado P, Hagelkrüys A, Wimmer RA, Stahl M, et al. Inhibition of SARS-CoV-2 infections in engineered human tissues using clinical-grade soluble human ACE2. Cell. (2020) 181:905.e–13.e. doi: 10.1016/j.cell.2020.04.004
126. Varga Z, Flammer AJ, Steiger P, Haberecker M, Andermatt R, Zinkernagel AS, et al. Endothelial cell infection and endotheliitis in COVID-19. Lancet. (2020) 395:1417–8. doi: 10.1016/S0140-6736(20)30937-5
127. Jia H. Pulmonary angiotensin-converting enzyme 2 (ACE2) and inflammatory lung disease. Shock. (2016) 46:239–48. doi: 10.1097/SHK.0000000000000633
128. Nijkeuter M, Hovens MMC, Davidson BL, Huisman MV. Resolution of thromboemboli in patients with acute pulmonary embolism: a systematic review. Chest. (2006) 129:192–7. doi: 10.1378/chest.129.1.192
129. Klok FA, van Kralingen KW, van Dijk APJ, Heyning FH, Vliegen HW, Huisman MV. Prospective cardiopulmonary screening program to detect chronic thromboembolic pulmonary hypertension in patients after acute pulmonary embolism. Haematologica. (2010) 95:970–5. doi: 10.3324/haematol.2009.018960
130. Pengo V, Lensing AWA, Prins MH, Marchiori A, Davidson BL, Tiozzo F, et al. Incidence of chronic thromboembolic pulmonary hypertension after pulmonary embolism. N Engl J Med. (2004) 350:2257–64. doi: 10.1056/NEJMoa032274
131. Ende-Verhaar YM, Cannegieter SC, Vonk Noordegraaf A, Delcroix M, Pruszczyk P, Mairuhu ATA, et al. Incidence of chronic thromboembolic pulmonary hypertension after acute pulmonary embolism: a contemporary view of the published literature. Eur Respir J. (2017) 49:1601792. doi: 10.1183/13993003.01792-2016
132. Guérin L, Couturaud F, Parent F, Revel M-P, Gillaizeau F, Planquette B, et al. Prevalence of chronic thromboembolic pulmonary hypertension after acute pulmonary embolism. Prevalence of CTEPH after pulmonary embolism. Thromb Haemost. (2014) 112:598–605. doi: 10.1160/TH13-07-0538
133. Pepke-Zaba J, Delcroix M, Lang I, Mayer E, Jansa P, Ambroz D, et al. Chronic thromboembolic pulmonary hypertension (CTEPH): results from an international prospective registry. Circulation. (2011) 124:1973–81. doi: 10.1161/CIRCULATIONAHA.110.015008
134. Riedel M, Stanek V, Widimsky J, Prerovsky I. Longterm follow-up of patients with pulmonary thromboembolism. Late prognosis and evolution of hemodynamic and respiratory data. Chest. (1982) 81:151–8. doi: 10.1378/chest.81.2.151
135. Townsend L, Fogarty H, Dyer A, Martin-Loeches I, Bannan C, Nadarajan P, et al. Prolonged elevation of D-dimer levels in convalescent COVID-19 patients is independent of the acute phase response. J Thromb Haemost. (2021) 19:1064–70. doi: 10.1111/jth.15267
136. Nuche J, Segura de la Cal T, Jiménez López Guarch C, López-Medrano F, Delgado CP, Ynsaurriaga FA, et al. Effect of coronavirus disease 2019 in pulmonary circulation. the particular scenario of precapillary pulmonary hypertension. Diagnostics (Basel). (2020) 10:E548. doi: 10.3390/diagnostics10080548
137. van Dam LF, Kroft LJM, van der Wal LI, Cannegieter SC, Eikenboom J, de Jonge E, et al. Clinical and computed tomography characteristics of COVID-19 associated acute pulmonary embolism: a different phenotype of thrombotic disease? Thromb Res. (2020) 193:86–9. doi: 10.1016/j.thromres.2020.06.010
138. Delcroix M, Torbicki A, Gopalan D, Sitbon O, Klok FA, Lang I, et al. ERS statement on chronic thromboembolic pulmonary hypertension. Eur Respir J. (2021) 57:2002828. doi: 10.1183/13993003.02828-2020
139. Kim NH, Delcroix M, Jais X, Madani MM, Matsubara H, Mayer E, et al. Chronic thromboembolic pulmonary hypertension. Eur Respir J. (2019) 53:1801915. doi: 10.1183/13993003.01915-2018
140. He J, Fang W, Lv B, He J-G, Xiong C-M, Liu Z-H, et al. Diagnosis of chronic thromboembolic pulmonary hypertension: comparison of ventilation/perfusion scanning and multidetector computed tomography pulmonary angiography with pulmonary angiography. Nucl Med Commun. (2012) 33:459–63. doi: 10.1097/MNM.0b013e32835085d9
141. Moores LK, Tritschler T, Brosnahan S, Carrier M, Collen JF, Doerschug K, et al. Prevention, diagnosis, and treatment of VTE in patients with coronavirus disease 2019: CHEST guideline and expert panel report. Chest. (2020) 158:1143–63. doi: 10.1016/j.chest.2020.05.559
143. Brenot P, Jaïs X, Taniguchi Y, Garcia Alonso C, Gerardin B, Mussot S, et al. French experience of balloon pulmonary angioplasty for chronic thromboembolic pulmonary hypertension. Eur Respir J. (2019) 53:1802095. doi: 10.1183/13993003.02095-2018
144. Li W, Yang T, Quan R-L, Chen X-X, An J, Zhao Z-H, et al. Balloon pulmonary angioplasty reverse right ventricular remodelling and dysfunction in patients with inoperable chronic thromboembolic pulmonary hypertension: a systematic review and meta-analysis. Eur Radiol. (2021) 31:3898–908. doi: 10.1007/s00330-020-07481-6
145. Ghofrani H-A, D’Armini AM, Grimminger F, Hoeper MM, Jansa P, Kim NH, et al. Riociguat for the Treatment of Chronic Thromboembolic Pulmonary Hypertension. N Engl J Med. (2013) 369:319–29. doi: 10.1056/NEJMoa1209657
146. Cueto-Robledo G, Porres-Aguilar M, Puebla-Aldama D, del Pilar Barragán-Martínez M, Jurado-Hernández MY, García-César M, et al. Severe pulmonary hypertension: an important sequel after severe post-acute COVID-19 pneumonia. Curr Probl Cardiol. (2022) 47:101004. doi: 10.1016/j.cpcardiol.2021.101004
147. Salcin S, Fontem F. Recurrent SARS-CoV-2 infection resulting in acute respiratory distress syndrome and development of pulmonary hypertension: a case report. Respir Med Case Rep. (2021) 33:101314. doi: 10.1016/j.rmcr.2020.101314
148. Kolb TM, Hassoun PM. Right ventricular dysfunction in chronic lung disease. Cardiol Clin. (2012) 30:243–56. doi: 10.1016/j.ccl.2012.03.005
149. Rinaldo RF, Mondoni M, Parazzini EM, Baccelli A, Pitari F, Brambilla E, et al. Severity does not impact on exercise capacity in COVID-19 survivors. Respir Med. (2021) 187:106577. doi: 10.1016/j.rmed.2021.106577
150. Rinaldo RF, Mondoni M, Parazzini EM, Pitari F, Brambilla E, Luraschi S, et al. Deconditioning as main mechanism of impaired exercise response in COVID-19 survivors. Eur Respir J. (2021) 58:2100870. doi: 10.1183/13993003.00870-2021
151. Skjørten I, Ankerstjerne OAW, Trebinjac D, Brønstad E, Rasch-Halvorsen Ø, Einvik G, et al. Cardiopulmonary exercise capacity and limitations 3 months after COVID-19 hospitalisation. Eur Respir J. (2021) 58:2100996. doi: 10.1183/13993003.00996-2021
152. Motiejunaite J, Balagny P, Arnoult F, Mangin L, Bancal C, Vidal-Petiot E, et al. Hyperventilation as one of the mechanisms of persistent dyspnoea in SARS-CoV-2 survivors. Eur Respir J. (2021) 58:2101578. doi: 10.1183/13993003.01578-2021
153. Debeaumont D, Boujibar F, Ferrand-Devouge E, Artaud-Macari E, Tamion F, Gravier F-E, et al. Cardiopulmonary exercise testing to assess persistent symptoms at 6 months in people with covid-19 who survived hospitalization: a pilot study. Phys Ther. (2021) 101:zab099. doi: 10.1093/ptj/pzab099
154. Clavario P, De Marzo V, Lotti R, Barbara C, Porcile A, Russo C, et al. Cardiopulmonary exercise testing in COVID-19 patients at 3 months follow-up. Int J Cardiol. (2021) 340:113–8. doi: 10.1016/j.ijcard.2021.07.033
155. Acar RD, Sarıbaş E, Güney PA, Kafkas Ç, Aydınlı D, Taşçı E, et al. COVID-19: the new cause of dyspnoea as a result of reduced lung and peripheral muscle performance. J Breath Res. (2021) 15:047103. doi: 10.1088/1752-7163/ac22bb
156. Cassar MP, Tunnicliffe EM, Petousi N, Lewandowski AJ, Xie C, Mahmod M, et al. Symptom Persistence Despite Improvement in Cardiopulmonary Health - Insights from longitudinal CMR, CPET and lung function testing post-COVID-19. EClinicalMedicine. (2021) 41:101159. doi: 10.1016/j.eclinm.2021.101159
157. Vonbank K, Lehmann A, Bernitzky D, Gysan MR, Simon S, Schrott A, et al. Predictors of prolonged cardiopulmonary exercise impairment after COVID-19 Infection: a prospective observational study. Front Med (Lausanne). (2021) 8:773788. doi: 10.3389/fmed.2021.773788
158. Ribeiro Baptista B, d’Humières T, Schlemmer F, Bendib I, Justeau G, Al-Assaad L, et al. Identification of factors impairing exercise capacity after severe COVID-19 pulmonary infection: a 3-month follow-up of prospective COVulnerability cohort. Respir Res. (2022) 23:68. doi: 10.1186/s12931-022-01977-z
159. Singh I, Joseph P, Heerdt PM, Cullinan M, Lutchmansingh DD, Gulati M, et al. Persistent exertional intolerance after COVID-19. Chest. (2021) 161:54–63. doi: 10.1016/j.chest.2021.08.010
160. Hejbøl EK, Harbo T, Agergaard J, Madsen LB, Pedersen TH, Østergaard LJ, et al. Myopathy as a cause of fatigue in long-term post-COVID-19 symptoms: evidence of skeletal muscle histopathology. Eur J Neurol. (2022) 29:2832–41. doi: 10.1111/ene.15435
161. Schaeffer MR, Cowan J, Milne KM, Puyat JH, Voduc N, Corrales-Medina V, et al. Cardiorespiratory physiology, exertional symptoms, and psychological burden in post-COVID-19 fatigue. Respir Physiol Neurobiol. (2022) 302:103898. doi: 10.1016/j.resp.2022.103898
162. Baratto C, Caravita S, Faini A, Perego GB, Senni M, Badano LP, et al. Impact of COVID-19 on exercise pathophysiology: a combined cardiopulmonary and echocardiographic exercise study. J Appl Physiol. (2021) 130:1470–8. doi: 10.1152/japplphysiol.00710.2020
163. Spruit MA, Holland AE, Singh SJ, Tonia T, Wilson KC, Troosters T. COVID-19: interim guidance on rehabilitation in the hospital and post-hospital phase from a European respiratory society and American thoracic society-coordinated international task force. Eur Respir J. (2020) 56:2002197. doi: 10.1183/13993003.02197-2020
164. Li J. Rehabilitation management of patients with COVID-19: lessons learned from the first experience in China. Eur J Phys Rehabil Med. (2020) 56:335–8. doi: 10.23736/S1973-9087.20.06292-9
165. Dixit S, Borghi-Silva A, Bairapareddy KC. Revisiting pulmonary rehabilitation during COVID-19 pandemic: a narrative review. Rev Cardiovasc Med. (2021) 22:315–27. doi: 10.31083/j.rcm2202039
166. Huang L, Yao Q, Gu X, Wang Q, Ren L, Wang Y, et al. 1-year outcomes in hospital survivors with COVID-19: a longitudinal cohort study. Lancet. (2021) 398:747–58. doi: 10.1016/S0140-6736(21)01755-4
167. de Sire A, Andrenelli E, Negrini F, Patrini M, Lazzarini SG, Ceravolo MG, et al. Rehabilitation and COVID-19: a rapid living systematic review by cochrane rehabilitation field updated as of December 31st, 2020 and synthesis of the scientific literature of 2020. Eur J Phys Rehabil Med. (2021) 57:181–8. doi: 10.23736/S1973-9087.21.06870-2
168. Hameed F, Palatulan E, Jaywant A, Said R, Lau C, Sood V, et al. Outcomes of a COVID-19 recovery program for patients hospitalized with SARS-CoV-2 infection in New York City: a prospective cohort study. PM R. (2021) 13:609–17. doi: 10.1002/pmrj.12578
169. Gonzalez-Gerez JJ, Saavedra-Hernandez M, Anarte-Lazo E, Bernal-Utrera C, Perez-Ale M, Rodriguez-Blanco C. Short-term effects of a respiratory telerehabilitation program in confined covid-19 patients in the acute phase: a pilot study. Int J Environ Res Public Health. (2021) 18:7511. doi: 10.3390/ijerph18147511
170. Li J, Xia W, Zhan C, Liu S, Yin Z, Wang J, et al. A telerehabilitation programme in post-discharge COVID-19 patients (TERECO): a randomised controlled trial. Thorax. (2021) 77:697–706. doi: 10.1136/thoraxjnl-2021-217382
171. De Marchi T, Frâncio F, Ferlito JV, Weigert R, de Oliveira C, Merlo AP, et al. Effects of photobiomodulation therapy combined with static magnetic field in severe COVID-19 patients requiring intubation: a pragmatic randomized placebo-controlled trial. J Inflamm Res. (2021) 14:3569–85. doi: 10.2147/JIR.S318758
172. Nambi G, Abdelbasset WK, Alrawaili SM, Elsayed SH, Verma A, Vellaiyan A, et al. Comparative effectiveness study of low versus high-intensity aerobic training with resistance training in community-dwelling older men with post-COVID 19 sarcopenia: a randomized controlled trial. Clin Rehabil. (2022) 36:59–68. doi: 10.1177/02692155211036956
173. Mohamed AA, Alawna M. The effect of aerobic exercise on immune biomarkers and symptoms severity and progression in patients with COVID-19: a randomized control trial. J Bodyw Mov Ther. (2021) 28:425–32. doi: 10.1016/j.jbmt.2021.07.012
174. Liu K, Zhang W, Yang Y, Zhang J, Li Y, Chen Y. Respiratory rehabilitation in elderly patients with COVID-19: a randomized controlled study. Complement Ther Clin Pract. (2020) 39:101166. doi: 10.1016/j.ctcp.2020.101166
175. Güler T, Yurdakul FG, Acar Sivas F, Kiliç Z, Adigüzel E, Yaşar E, et al. Rehabilitative management of post-acute COVID-19: clinical pictures and outcomes. Rheumatol Int. (2021) 41:2167–75. doi: 10.1007/s00296-021-05003-1
176. Bouteleux B, Henrot P, Ernst R, Grassion L, Raherison-Semjen C, Beaufils F, et al. Respiratory rehabilitation for Covid-19 related persistent dyspnoea: a one-year experience. Respir Med. (2021) 189:106648. doi: 10.1016/j.rmed.2021.106648
177. Holland AE, Cox NS, Houchen-Wolloff L, Rochester CL, Garvey C, ZuWallack R, et al. Defining modern pulmonary rehabilitation. An official American thoracic society workshop report. Ann Am Thorac Soc (2021) 18:e12–29. doi: 10.1513/AnnalsATS.202102-146ST
178. Spruit MA, Singh SJ, Garvey C, ZuWallack R, Nici L, Rochester C, et al. An official American thoracic society/European respiratory society statement: key concepts and advances in pulmonary rehabilitation. Am J Respir Crit Care Med (2013) 188:e13–64.
179. Bolton CE, Bevan-Smith EF, Blakey JD, Crowe P, Elkin SL, Garrod R, et al. British thoracic society guideline on pulmonary rehabilitation in adults. Thorax (2013) 68(Suppl. 2):ii1–30. doi: 10.1136/thoraxjnl-2013-203808
180. Radtke T, Crook S, Kaltsakas G, Louvaris Z, Berton D, Urquhart DS, et al. ERS statement on standardisation of cardiopulmonary exercise testing in chronic lung diseases. Eur Respir Rev. (2019) 28:180101. doi: 10.1183/16000617.0101-2018
181. Holland AE, Spruit MA, Troosters T, Puhan MA, Pepin V, Saey D, et al. An official European respiratory society/American thoracic society technical standard: field walking tests in chronic respiratory disease. Eur Respir J. (2014) 44:1428–46. doi: 10.1183/09031936.00150314
182. Gao Y, Chen R, Geng Q, Mo X, Zhan C, Jian W, et al. Cardiopulmonary exercise testing might be helpful for interpretation of impaired pulmonary function in recovered COVID-19 patients. Eur Respir J. (2021) 57:2004265. doi: 10.1183/13993003.04265-2020
183. Liu M, Lv F, Huang Y, Xiao K. Follow-up study of the chest CT characteristics of COVID-19 survivors seven months after recovery. Front Med. (2021) 8:636298. doi: 10.3389/fmed.2021.636298
184. Dorelli G, Braggio M, Gabbiani D, Busti F, Caminati M, Senna G, et al. Importance of cardiopulmonary exercise testing amongst subjects recovering from COVID-19. Diagnostics. (2021) 11:507. doi: 10.3390/diagnostics11030507
185. Barbagelata L, Masson W, Iglesias D, Lillo E, Migone JF, Orazi ML, et al. Cardiopulmonary exercise testing in patients with post-COVID-19 syndrome. Med Clin (Barc). (2021) 159:6–11. doi: 10.1016/j.medcli.2021.07.007
186. Mancini DM, Brunjes DL, Lala A, Trivieri MG, Contreras JP, Natelson BH. Use of cardiopulmonary stress testing for patients with unexplained dyspnea post–coronavirus disease. JACC Heart Fail. (2021) 9:927–37. doi: 10.1016/j.jchf.2021.10.002
187. Szekely Y, Lichter Y, Sadon S, Lupu L, Taieb P, Banai A, et al. Cardiorespiratory abnormalities in patients recovering from coronavirus disease 2019. J Am Soc Echocardiogr (2021) 34:1273–1284.e9. doi: 10.1016/j.echo.2021.08.022
188. de Boer E, Petrache I, Goldstein NM, Olin JT, Keith RC, Modena B, et al. Decreased fatty oxidation and altered lactate production during exercise in post-acute COVID-19 patients. Am J Respir Crit Care Med. (2021) 205:126–9. doi: 10.1164/rccm.202108-1903LE
189. Ladlow P, O’Sullivan O, Houston A, Barker-Davies R, May S, Mills D, et al. Dysautonomia following COVID-19 is not associated with subjective limitations or symptoms but is associated with objective functional limitations. Heart Rhythm. (2022) 19:613–20. doi: 10.1016/j.hrthm.2021.12.005
190. Ambrosino P, Parrella P, Formisano R, Perrotta G, D’Anna SE, Mosella M, et al. Cardiopulmonary exercise performance and endothelial function in convalescent covid-19 patients. J Clin Med. (2022) 11:1452. doi: 10.3390/jcm11051452
Keywords: COVID-19, pulmonary hypertension, interstitial lung disease, thrombosis, right ventricular dysfunction
Citation: Oliveira RKF, Nyasulu PS, Iqbal AA, Hamdan Gul M, Ferreira EVM, Leclair JW, Htun ZM, Howard LS, Mocumbi AO, Bryant AJ, Tamuzi JL, Avdeev S, Petrosillo N, Hassan A, Butrous G and de Jesus Perez V (2022) Cardiopulmonary disease as sequelae of long-term COVID-19: Current perspectives and challenges. Front. Med. 9:1041236. doi: 10.3389/fmed.2022.1041236
Received: 10 September 2022; Accepted: 14 November 2022;
Published: 30 November 2022.
Edited by:
Judit Pongracz, University of Pécs, HungaryReviewed by:
Marco Massari, Independent Researcher, Reggio Emilia, ItalyAshraf Roshdy, Alexandria University, Egypt
Copyright © 2022 Oliveira, Nyasulu, Iqbal, Hamdan Gul, Ferreira, Leclair, Htun, Howard, Mocumbi, Bryant, Tamuzi, Avdeev, Petrosillo, Hassan, Butrous and de Jesus Perez. This is an open-access article distributed under the terms of the Creative Commons Attribution License (CC BY). The use, distribution or reproduction in other forums is permitted, provided the original author(s) and the copyright owner(s) are credited and that the original publication in this journal is cited, in accordance with accepted academic practice. No use, distribution or reproduction is permitted which does not comply with these terms.
*Correspondence: Rudolf K. F. Oliveira, cnVkb2xmLm9saXZlaXJhQHVuaWZlc3AuYnI=
†These authors have contributed equally to this work and share first authorship