- 1School of Civil and Transportation Engineering, Henan University of Urban Construction, Pingdingshan, China
- 2School of Architectural Engineering, Zhongyuan University of Technology, Zhengzhou, China
The failure of ultra-high performance concrete (UHPC) under axial compression has significant brittleness, so it is necessary to use fiber reinforced polymer (FRP) to constrain UHPC for achieving ductile failure. This article mainly summarized the research progress of FRP constrained UHPC columns, analyzed the influence of different variables on the compressive performance of FRP-confined UHPC columns and the coupling effect between variables, compared the axial compressive stress-strain models of FRP-confined UHPC columns proposed by previous scholars, and evaluated the existing models using a large amount of collected experimental data. The evaluation results indicated that the existing models provided relatively accurate predictions for ultimate stress, but further improvement and correction are needed for predicting ultimate strain. Based on the data collected in this study, the models had been modified, resulting in improved accuracy in predicting both ultimate stress and ultimate strain.
1 Introduction
Ultra-high performance concrete (UHPC) is a novel cement-based composite material with high strength, high toughness and high durability, which is of great significance for reducing structural weight and improving the structural safety and durability. It can be used in bridges, municipal engineering, industrial plants with corrosion, super high-rise buildings and military engineering (Cheng et al., 2021; Ma et al., 2021; Liu et al., 2022; Wang, 2022; Zhang et al., 2023a; Mu et al., 2023). Due to the significant brittleness of UHPC under axial compression, which gives an unexpected failure mode and behavior (Dang et al., 2022). In order to improve the brittleness of UHPC, many scholars have added different fibers to UHPC to improve the toughness of the material. Liao et al. (2023a) found that increasing the straight steel fiber (SF) content from 1% to 2% could result in a 24% enhancement in the punching shear capacity. Yu et al. (2018) incorporated polyethylene (PE) fibers into UHPC, and the results showed that the adoption of PE fibers might benefit the punching shear resistance of UHPC slabs. Xu et al. (2019) studied the strengthening effect of steel-polypropylene hybrid fiber on UHPC. The results showed that steel-polypropylene hybrid fiber was able to better enhance the strength and the ductility of UHPC. Existing studies (Wu et al., 2006; Shan and Zhang, 2019; Alwesabi et al., 2022; Zhang et al., 2023b; Mu et al., 2023) have shown that adding a certain amount of fiber can effectively improve the brittleness of concrete, but the concrete post-peak behavior such as the ductility and toughness are improved finitely.
Circumferential constraints are imposed on the periphery of the UHPC column, so that the core UHPC is in a three-way compression state, which can not only improve the bearing capacity of the UHPC column, but also greatly improve its ductility. At present, the most widely used are steel and fiber reinforced polymer (FRP) (Xu et al., 2019; Zhao et al., 2022a; Zhao et al., 2023a; Zhao et al., 2023b; Miao et al., 2023; Zeng et al., 2023). Fiber reinforced polymer has light weight and high strength, and its thermal expansion coefficient is similar to that of concrete, which can be coordinated with the deformation of concrete. It is widely used in repair and reinforcement engineering (Yağar et al., 2022; Wang et al., 2023a), bridge engineering (Davids et al., 2022; De Corte and Uyttersprot, 2022), marine engineering (Chen et al., 2023; Zeng et al., 2023), geotechnical engineering (Giraldo and Rayhani, 2014; Wang et al., 2018a) and various civil buildings (Tbatou and El Youbi, 2020). Lateral confinement of UHPC with FRP can give full play to the advantages of UHPC and FRP, and improve the strength and deformation capacity of core UHPC (Liang et al., 2020).
This paper mainly introduced the research progress of axial compression performance of FRP-confined UHPC columns, and described the failure modes of FRP-confined UHPC columns under axial compression. Furthermore, the effects of different parameters and their coupling effects on the axial compression performance of FRP-confined UHPC columns were summarized. Finally, a database was established by analyzing the collected experimental data, the utilization effects of FRP-confined UHPC column constitutive models proposed by previous scholars were compared and evaluated. Based on the established database, the modified model of the existing constitutive models was proposed.
2 Experimental phenomenon of compression of UHPC columns confined by FRP
2.1 Failure modes
The failure modes of FRP-confined UHPC columns under axial compression are closely related to the confinement levels and steel fiber content. Previous studies have shown (Guler, 2014; Wang et al., 2018b; Liang et al., 2020; Lam et al., 2021; Liao et al., 2021; Dang et al., 2022) that FRP has no constraint effect on specimens at the initial stage of loading. With the increase of load, UHPC begins to expand, which leads to the binding effect of FRP on UHPC. As the load continues to increase, the sound of FRP rupture can be heard (Ma et al., 2022). Until the load reaches 90% of the ultimate strength, the middle part of the FRP breaks horizontally and separates from the UHPC column. At this time, the surface of the UHPC column produces longitudinal shear cracks and penetrates into the entire specimen. Longitudinal shear cracks are generated on the UHPC column surface and penetrate through the entire specimen. Due to the bridging effect of steel fiber, the surface crack of UHPC is effectively suppressed. With the increase of steel fiber content, the bearing capacity of the sample increases, and the energy release at the time of failure also increases, which leads to a wider range of FRP fracture and a louder explosion sound. When the lateral restraint stiffness is insufficient, the shear fracture surface is prone to occur under shear stress. The final failure mode of the sample is typical shear failure, and the fracture of FRP is located near the end of the sample. With the increase of confining stiffness, FRP can provide enough lateral confinement, resulting in the emergence of more shear failure planes on UHPC cylindrical specimens under tri-axial compression. The fracture of FRP usually occurs at the middle height of the specimen. The axial compressive failure modes of two different types of FRP-confined specimens under different confinement strengths are shown in Figures 1, 2.
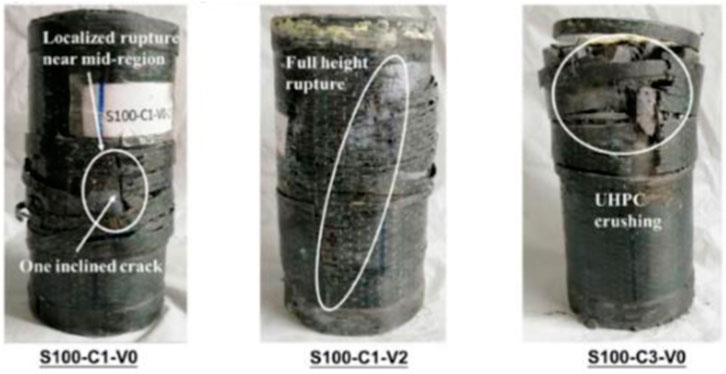
FIGURE 1. Carbon FRP constrained specimen failure morphology (Liao et al., 2021).
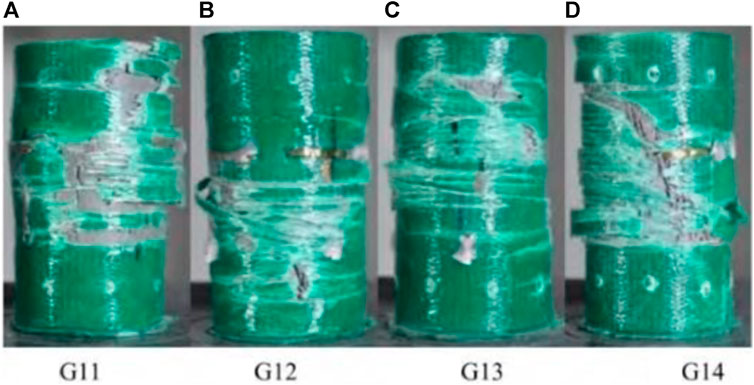
FIGURE 2. Glass FRP constrained specimen failure morphology (Ma et al., 2022). (A) G11, (B) G12, (C) G13, (D) G14.
2.2 Stress-strain relationship
Different from the stress-strain relationship of the bilinear behavior of FRP-constrained normal strength concrete (Lam and Teng, 2003), the stress-strain relationship of FRP-constrained UHPC is similar to that of FRP-constrained high strength concrete, which usually shows strength loss after the first peak value (Fallah Pour et al., 2019). The axial compression stress-strain curve of FRP-confined UHPC can be divided into three stages: linear elastic section, transition section and strengthening section. In the transition section, there are two trends with different constraint strength curves, and the typical stress–strain behaviors are shown in Figure 3.
(i) Linear elastic section. In the early loading phase, the FRP constraint is not activated, and its axial stress-strain relationship is similar to that of unconstrained UHPC (Luo et al., 2020).
(ii) Transition section. With the increase of load, micro cracks begin to appear within UHPC, and the confinement effect of FRP is activated with the expansion of core concrete. In case of sufficient circumferential constraint, the axial stress of the specimen will increase with the increase of axial deformation. In case of insufficient circumferential constraint, the load drops sharply due to the brittleness of UHPC, which results a rapid expansion of longitudinal cracks. Meanwhile, the stress-strain curve shows a strength loss after the first peak.
(iii) Strengthening section. At this stage, as the FRP confinement is fully activated, the stress-strain curve enters the second linear rising stage. The slope of the stress-strain curve depends on the confinement efficiency in this stage.
A large number of these studies (Saleem et al., 2017; Zeng et al., 2020; Liao et al., 2021; Wang et al., 2021) have shown an axial stress–strain response different from the typical “bi-linear” curve which ascends monotonically without stress reduction. Instead, strain softening behavior (i.e., stress reduction) has been frequently reported after the curve exceeds the initial peak point, in most cases the stress reduction is followed by a strain hardening response (i.e., stress recovery). In view of the strength loss, it may have an adverse effect on the performance of the column (such as excessive deformation or instability). Therefore, many scholars have studied the stress reduction-recovery behavior of FRP-confined UHPC short columns. Yuan et al. (2022a) showed that the factors leading to the stress-strain softening curve of FRP confined concrete include constraint stiffness, section type and concrete strength. Wu et al. (2006) studied the conditions and reasons for the softening section of the stress-strain curve of FRP confined concrete. The results showed that the softening section of the stress-strain curve of the confined specimen was mainly related to the lateral restraint strength
3 Analysis of influencing factors on compression properties of FRP-confined UHPC columns
3.1 Steel fiber content
Steel fibers introduced in UHPC column can improve the toughness of UHPC material, while steel fiber is one of the important reasons for the high cost of UHPC. Hence, optimizing the content of steel fiber is of great significance for promoting UHPC (Zhao et al., 2022b). Previous studies (Yoo et al., 2013; Hannawi et al., 2016; Wu et al., 2016; Chen et al., 2024) had found that the initial elastic modulus and compressive strength of unconstrained UHPC can be improved with the addition of steel fiber within the appropriate dosage range. However, when the dosage exceeds the limit, the fluidity and mechanical properties of UHPC tend to decrease. Although the toughness of UHPC can also be improved by adding steel fibers, the improvement effect is limited that the ultimate load-bearing capacity of UHPC members cannot be greatly enhanced in practical engineering (Hoang et al., 2019). Therefore, many scholars began to pay attention to improving the ultimate bearing capacity of UHPC by confining it with FRP. Wang et al. (Wang et al., 2018b) carried out an axial compression test on FRP-confined UHPC, and the results showed that steel fiber could effectively inhibit crack expansion and spalling of concrete. Liao et al. (2021) showed that the addition of steel fiber improved the compressive strength of UHPC, and slightly increased the axial strain corresponding to the compressive strength. Tian et al. (2020) established a finite element model of LS-DYNA and analyzed the effect of steel fiber content on the axial compressive properties of constrained specimens. The results showed that increasing the steel fiber content could limit the development of inclined cracks in core UHPC, but it had little effect on the strength and ductility of the specimens. Liang et al. (2020) studied the influence of steel fiber content on the axial compressive properties of UHPC specimens confined by FRP. The results showed that the strength and toughness of FRP-confined UHPC specimens could be improved by adding proper amount of steel fiber. Ma et al. (2022) studied the axial compression properties of FRP-confined UHPC short columns, and the results showed that steel fiber could improve the brittleness characteristics of FRP-confined UHPC short columns to some extent. The existing literature mostly focuses on the study of single steel fiber content, and lacks the study of steel fiber shape, arrangement and hybrid fiber.
3.2 FRP types
In recent years, the use of FRP composites has greatly increased, among which carbon, glass and aramid fibers are the most popular. Guler, (2014) studied the axial compression properties of UHPC short columns wrapped with carbon FRP (CFRP), glass FRP (GFRP) and aramid FRP (AFRP) respectively. The ultimate strength of UHPC columns wrapped with CFRP increased the most, with an increase of 48%, and that of UHPC columns wrapped with GFRP and AFRP increased by 16.8% and 22.7% respectively. The ultimate strain of UHPC columns wrapped by GFRP increases the most, with an increase of 128%, while the ultimate stress of UHPC columns wrapped by AFRP and CFRP increases by 96.9% and 88.9% respectively. Deng, (2016); Deng and Liu, (2016) conducted axial compression tests on UHPC columns constrained by four different fibers: CFRP, GFRP, AFRP and basalt FRP. The results showed that CFRP had the most obvious effect on improving compressive strength, and GFRP had the most obvious effect on improving ultimate strain. Through a large number of studies, it was found that CFRP and GFRP could improve the axial compression performance of constrained specimens more significantly. Therefore, more and more scholars had carried out research on those two fiber materials. Wang et al. (2018b) believed that GFRP had higher tensile strain capacity than CFRP, so it was more suitable to provide constraints for UHPC. An et al. (2021)), Dang et al. (2022), Ma et al. (2022) compared the two fiber types of CFRP and GFRP. The results showed that CFRP could better improve the ultimate strength of short concrete columns than GFRP, and the number of CFRP layers required for strain hardening phenomenon was less than that of GFRP. Compared with CFRP confined specimens, the transverse strain distribution of GFRP confined specimens was more uniform and the stress redistribution was easier. When the number of FRP layers and the volume of steel fiber were unchanged, the strengthening effect of CFRP on the ultimate strength of circular short columns was obviously better than that of GFRP.
3.3 FRP thickness
Deng and Liu, (2016) thought that the ultimate strength and ultimate strain of confined specimens were related to the confined ratio of specimens. Therefore, the thickness of FRP was the key factor affecting the axial compression performance of specimens. Tian et al. (2019a); Tian et al. (2020) studied the axial compression performance of constrained samples with varying FRP tube thickness. The results showed that the deformation of FRP-confined UHPC specimens with small tube thickness went through three stages: linear elasticity, softening and linear strengthening. However, when the tube thickness was increased to provide sufficient constraints, the deformation of the specimen would directly transition from linear elastic stage to linear strengthening stage. Liang et al. (2020) analyzed the influence of GFRP tube thickness on the axial compression performance of the specimens. The results showed that the thicker the tube, the greater the increase in the ultimate strength, and showed a linear growth relationship. Liao et al. (2021) had studied the influence of the number of CFRP layers on the stress-strain curve. The results showed that the FRP thickness mainly affected the transition section and the strengthening section of the curve. In addition, the increased FRP thickness could also alleviate the sudden stress reduction at the first peak, or even eliminate the stress reduction. Ma et al. (2022), An et al. (2021), Zohrevand and Mirmiran, (2011) studied the axial compression properties of constrained samples with various FRP layers. The results showed that the ultimate compressive strength and ultimate strain of UHPC circular short columns increased with the increase of FRP layers, but the increase of ultimate strain was more substantial.
3.4 Fiber winding angles
When studying FRP-constrained UHPC short columns, most scholars focus primarily on the types and thicknesses of FRP, with less attention given to the winding angles of the FRP fibers. Zhang et al. (2019) conducted the axial compression tests of GFRP-confined concrete short columns with fiber winding angles of ±45, ±60 and ±80°. The results indicated that the winding angles of fibers had a significant impact on the circumferential stiffness and circumferential failure strain of GFRP pipes. Lu, (2020) studied the influence of GFRP-confined UHPC specimens with two different fiber winding angles (60 and 80°) on their mechanical properties. The results showed that the greater the fiber winding angle, the more significant the improvement of compressive strength of the specimen, but it had little effect on the ultimate strain of the specimen. This is consistent with the research results of FRP-confined normal-strength concrete (NSC) (Sadeghian et al., 2010; Vincent and Ozbakkaloglu, 2013). Tian et al. (2019b); Tian et al. (2020) established a micro-finite element model of FRP-confined UHPC cylinder using LS-DYNA finite element analysis software, and studied the influence of fiber winding angles on constrained specimens. The results showed that the ultimate bearing capacity and ductility of specimens increased with the increase of fiber winding angles. Zhu et al. (2023) studied the axial compressive properties of GFRP-confined UHPC with two different fiber winding angles of 45 and 80°. The results showed that, when the axial compression ratio was constant, the ultimate horizontal bearing capacity and equivalent stiffness of the 45° winding tubular composite columns were lower than those of the 80° winding tubular composite columns. However, the former had higher ductility coefficient, better energy dissipation capacity and stronger restraint effect of GFRP tube on UHPC. Vincent and Ozbakkaloglu, (2013) investigated HSC cylinders confined with 3-ply AFRP with different winding angles (i.e., 45°, 60°, 75° and 88°). The results concluded that the confinement effect and ultimate axial stress could decrease when the fiber orientations were further deviated from hoop direction, whereas the ultimate axial strains could increase. Therefore, it is more effective to align the FRP fibers in the hoop direction, which leads to a smaller Poisson’s ratio and thus tighter confinement.
From the results in the above literature, it can be concluded that FRP-confined UHPC columns can significantly improve the ultimate strength and strain of axial compression. Different fiber reinforced composites have different reinforcement effects on UHPC. Among them, CFRP has a significant improvement in the ultimate strength of circular short columns, and GFRP has a significant improvement in the ultimate strain of circular short columns. With the increase of FRP thickness, the lateral restraint stress of the specimen increases, which can more effectively restrain the lateral deformation of UHPC in the core area, thus improving the compressive strength and ultimate strain of the specimen. The ultimate strength and ultimate strain of UHPC round short columns can be improved with the increase of the volume of steel fiber, and the brittleness of the specimens can be improved to a certain extent. However, when the volume of steel fiber is too high, the strength of the structure will be adversely affected. From previous studies, it can be inferred that the steel fiber content should not exceed 3%. For FRP-confined UHPC columns, there is a lack of research on the shape and arrangement of steel fibers. With the increase of fiber winding angle, the limit bearing capacity of constrained samples increases, but it has little effect on the ultimate axial strain. At present, the research on the influence parameters of axial compression performance of FRP confined UHPC short columns is insufficient, such as specimen size, slenderness ratio, steel fiber shape and other parameters. Therefore, it is necessary to carry out relevant tests to verify the influence of these parameters on the axial compression performance of confined specimens.
3.5 Analysis of coupling effect of influencing factors
In many studies, it can be observed that there are often multiple experimental variables, so it is necessary to consider the interaction between variables and the synergistic effects of different variables on experimental results.
Tian et al. (2019a) investigated the coupled effects of FRP thickness and steel fiber content on the ultimate strain and compressive strength of FRP-confined UHPC specimens. The results revealed that the influence of steel fiber content was more obvious in the specimens with thinner walls. When the wall thickness was sufficiently thick, the effect of steel fibers was less significant. Liao et al. (2021) investigated the synergistic effects of specimen dimensions, FRP thickness, and steel fiber content. The results demonstrated that, for larger-sized specimens, increasing the steel fiber content had only a slight enhancement effect on specimen toughness at a relatively low confinement level. However, as the confinement level increased, the strengthening effect of increasing steel fiber content became more pronounced. Conversely, for smaller-sized specimens, the impact of steel fiber content on specimen toughness appeared to be insignificant even at a high confinement level. Yoo et al. (2016) investigated the influence of steel fiber shape on the size effect of specimens. The results indicated that the use of longer steel fibers had a more pronounced mitigating effect on the size effect of the specimens. Ma et al. (2022) studied the effects of steel fiber content, types and layers of FRP on the axial compression properties of constrained specimens. The results showed that CFRP improved the ultimate strength of round short columns significantly better than GFRP when the number of FRP layers or steel fiber content was increased. Wang et al. (2023b) investigated the coupling effect of “dual confinement” of FRP and steel fibers on confined specimens. The results showed that the addition of steel fiber could alleviate the stress reduction effect of UHPC specimens. In the later stage of FRP-confined UHPC loading, the existence of steel fibers had little effect on the FRP constraint effect. Most of the existing studies focus on the influence of a single variable on the axial compression properties of constrained samples, while few studies on the coupling effects of multiple variables. Correspondingly, there is a lack of comparative analysis of axial compression performance under multiple variables.
4 Constitutive model of FRP-confined UHPC columns under axial compression
4.1 Summary of existing models
Currently, many scholars had conducted numerous experimental studies and theoretical analyses on the axial compression performance and stress-strain relationship of FRP-confined ordinary concrete (Ozbakkaloglu et al., 2013; Deng, 2015; Deng and Qu, 2015; Deng and Wang, 2015; Li and Zhou, 2022). Although some constitutive models had been proposed, most of them were based on the modification of the constitutive model of FRP-confined ordinary concrete to make it suitable for FRP-confined UHPC models.
Zohrevand and Mirmiran, (2011) compared the experimental data with Mander model (Mander et al., 1988), Samaan model (Samaan et al., 1998), Toutanji model (Toutanji, 1999) and Lam-Teng model (Lam and Teng, 2003), respectively. The results showed that the Lam-Teng model provided the best fit for the stress-strain response of most specimens. However, all models tended to underestimate the ultimate strength at higher constraint ratios. None of the models can make reasonable predictions for the ultimate strain of UHPC specimens confined by FRP. Zohrevand and Mirmiran, (2013) modified the stress-strain model of unconfined UHPC proposed by Lam and Teng, and the accuracy of the predicted stress-strain curve was significantly improved. Guler, (2014) proposed a predictive model for FRP-confined UHPC and compared it with a model for FRP-confined conventional concrete. The results showed that the proposed model accurately predicted the ultimate strength, consistent with experimental findings. Deng and Wang, (2015) derived predictive formulas for the ultimate strength and ultimate strain of confined specimens through regression analysis, and modified the intercept in the Lam-Teng model. The modified model was more favorable for predicting the ultimate strength. Liang et al. (2020) conducted axial compression tests on GFRP-confined UHPC specimens and derived formulas to calculate the ultimate compressive strength and ultimate strain of UHPC under GFRP confinement based on experimental results. Wang et al. (2018b) evaluated the existing stress-strain models that can be used for FRP-confined UHPC. The results showed that the strength model proposed by Guler, (2014) provided a better prediction of ultimate strength, and the strain model proposed by Cui and Sheikh, (2010) provided a better prediction of ultimate strain. Liao et al. (2021) evaluated four prediction models and the results showed that the equations proposed by Dang et al. (2022) based on the Lam and Teng, (2003) was more accurate in predicting both ultimate strength and ultimate strain. The above models are shown in Table 1.
4.2 Performance evaluation of existing models
Currently, the understanding of the confinement mechanism of FRP-confined UHPC short columns is relatively mature. Many scholars had conducted a large number of experimental studies and proposed many models. For the axial stress-strain models summarized in the above section, their accuracy needs to be considered. In this section, the above-mentioned models will be evaluated through collected experimental data. To ensure a more reliable evaluation, this study selected experimental data from Liao et al. (2021), Lu, (2020) and Wang et al. (2018b) to establish a fitting sample database, which is listed in Table 2.
In this study, the performance of the above stress-strain models was evaluated and the key results predicted by the above models (normalized ultimate axial stress
where
The performance of the selected models is shown in Figure 4. In general, the applicability and accuracy of the selected models are limited. These models can give relatively accurate predictions for the test results of some studies, but cannot provide satisfactory predictions for most test results in the database. It can be observed from Figure 4 that the fitting effect of each model on the test data of Lu, (2020) is poor, mainly due to significant differences in the FRP and UHPC material properties compared to other specimens. For the two models proposed by Liao et al. (2022), they are evaluated only based on the prediction accuracy of ultimate stress and ultimate strain. It is worth noting that the two models proposed by Liao et al. (2022) are suitable for different situations. Because the data of different studies are difficult to coordinate, the accuracy of prediction is affected.
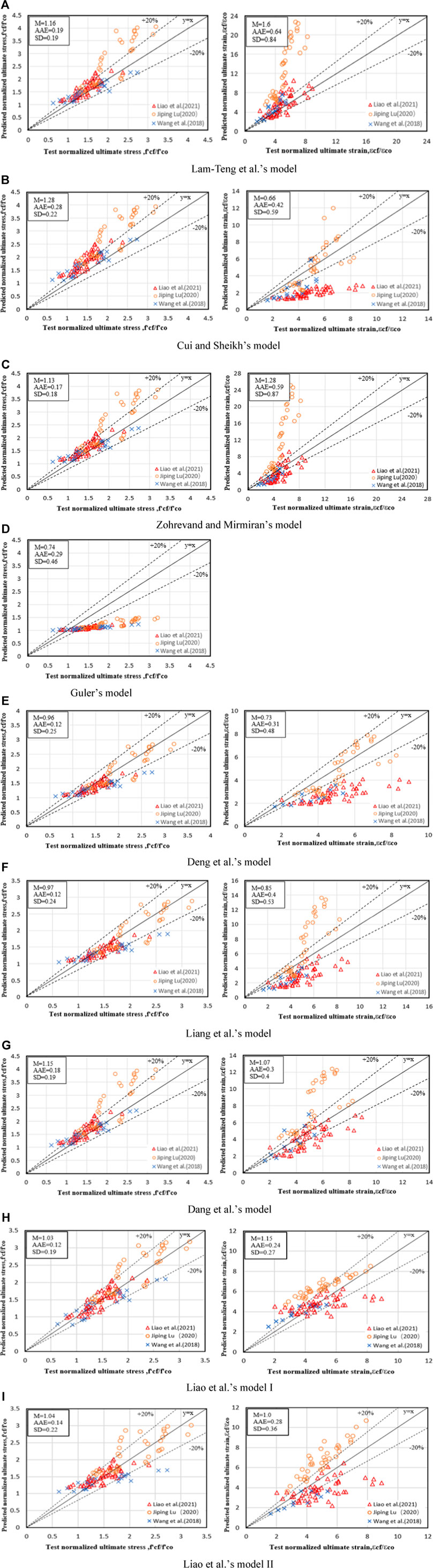
FIGURE 4. Comparisons between model predictions and test results. (A) Lam Teng model, (B) Cui and Sheikh’s model, (C) Zohrevand and Mirmiran’s model, (D) Guler’s model, (E) Deng et al.’s model, (F) Liang et al.’s model, (G) Dang et al.’s model, (H) Liao et al.’s model I, (I) L iao et al.’s model II.
Model I proposed by Liao et al. (2022) is considered as the best model for estimating
The above models have poor performance in estimating extreme strain, among which the model of Liao et al. (2022) has a relatively good estimation of extreme strain, but its AAE is also as high as 24%, and its predictions are relatively discrete. The Teng et al. (2009) model and the modified Lam-Teng model by Zohrevand and Mirmiran, (2013) fit well with the experimental data of Wang et al. (2018b) and Liao et al. (2021), but the predicted results for the test data of Lu (2020) are not satisfactory. The predictions of other models for ultimate strain can only be adapted to their own test results, and cannot provide satisfactory predictions for the majority of test results from other studies.
4.3 Forecasting model modification
Most of the existing prediction models are based on FRP-confined ordinary concrete, and it is believed that the strength confinement efficiency increases with the increase of confinement ratio. Samaan model (Samaan et al., 1998) believed that the relationship between strength and constraint ratio was nonlinear. Deng and Wang, (2015) modified Samaan model and the predicted value was relatively close to the experimental value. Considering the influence of FRP ultimate strain and constraint stiffness, an empirical formula for predicting the compressive strength of FRP-confined UHPC was obtained by regression of the experimental data collected in this study:
Using the regression formula (Eq. 4) for calculations, the predicted values show a good agreement with the experimental values.
On the basis of Zeng et al.’s FRP confined concrete model (Zeng et al., 2020) with the large rupture strain (LRS), Liao et al. obtained the ultimate strain prediction model by regression, and the prediction effect is relatively good. Based on the modified model of Liao et al., the following ultimate strain formula (Eq. 5) was fitted according to the test data collected in this paper, and it exhibits a good predictive performance:
The fitting effect of the revised model was shown in Figure 5. Compared with the model before correction, the prediction accuracy of the revised model has improved both in terms of ultimate stress and ultimate strain. Especially for the prediction of ultimate strain, the mean value is 1.05, with the AAE reduced to 18%, and the degree of dispersion is also improved. However, the prediction results for ultimate stress and ultimate strain are larger than the experimental values.
In order to verify the reliability and applicability of the modified models, the test data of Huang et al. (2021) and Dang et al. (2022) were selected for simulation. The selected test data are based on the axial compression test of FRP-confined UHPC circular short columns. The models verification results are shown in Figure 6. The prediction accuracy of the ultimate stress is high, and its AAE is 6%, indicating that the modified ultimate stress prediction model has good universality. However, the prediction effect of ultimate strain is far less than that of ultimate stress. Similar trends have also been reported in previous studies on FRP-confined NSC Bai et al. (2019); Pimanmas and Saleem, (2019); Yuan et al. (2022b). First, the stress measurement technique is more reliable than the strain measurement technique, and the strain measurement may be affected by the premature failure of the sample. Secondly, different mix proportions of UHPC and different methods of applying FRP constraints (FRP cloth or tube) may also interfere with the strain measurement results.
5 Conclusion and prospect
Through the study of axial compression test of FRP-confined UHPC short columns and the analysis of its stress-strain relationship models, it can be seen that:
(1) With the increase of FRP thickness, the bearing capacity and ductility of the specimens are improved. Steel fiber can improve the brittle characteristics of UHPC columns, and an appropriate amount of steel fiber can also improve the ultimate bearing capacity of the specimens. With the increase of the fiber winding angles, the ultimate bearing capacity of the confined specimens increases, but it has little effect on the ultimate axial strain. Most of the existing studies focus on a single variable, and there is a lack of research on the coupling effect of multiple variables.
(2) Evaluation of existing models suggests that the modified models by Liang et al. and Deng et al. provide the best predictions with high accuracy for ultimate stress, although the existing models show a good prediction performance for ultimate stress, the prediction of ultimate strain remains unsatisfactory.
(3) Based on the data collected in this study, the axial compression stress-strain model was modified. The modified model has improved the prediction accuracy of both ultimate stress and ultimate strain. Research on the axial stress-strain model of FRP-confined UHPC columns is limited, and further experimental research is needed to refine and validate the existing models.
Author contributions
SS: Supervision, Writing–review and editing. BZ: Writing–original draft. PY: Writing–review and editing. XY: Writing–review and editing.
Funding
The author(s) declare financial support was received for the research, authorship, and/or publication of this article. Financial supports to complete this research were provided partially by the Higher Education Youth Key Teacher Training Program Project (No. 2021GGJS142) of Henan Province and the National Natural Science Foundation Youth Fund Project(No.52108207) of China, both at Henan University of Urban Construction, P. R. China.
Conflict of interest
The authors declare that the research was conducted in the absence of any commercial or financial relationships that could be construed as a potential conflict of interest.
Publisher’s note
All claims expressed in this article are solely those of the authors and do not necessarily represent those of their affiliated organizations, or those of the publisher, the editors and the reviewers. Any product that may be evaluated in this article, or claim that may be made by its manufacturer, is not guaranteed or endorsed by the publisher.
References
Alwesabi, E. A. H., Abu Bakar, B. H., Alshaikh, I. M. H., Abadel, A. A., Alghamdi, H., and Wasim, M. (2022). An experimental study of compressive toughness of Steel–Polypropylene hybrid Fibre-Reinforced concrete. Struct. 37, 379–388. doi:10.1016/j.istruc.2022.01.025
An, K. X., Wang, X. Y., and Liu, Z. X. (2021). Study on axial compression performance research on UHPC short columns confined by fiber reinforced polymer. Build. Struct. 51 (11), 129–135. doi:10.19701/j.jzjg.2021.11.021
Bai, Y.-L., Dai, J.-G., Mohammadi, M., Lin, G., and Mei, S. J. (2019). Stiffness-based design-oriented compressive stress-strain model for large-rupture-strain (LRS) FRP-confined concrete. Compos. Struct. 223, 110953. doi:10.1016/j.compstruct.2019.110953
Chen, C. C., Wu, Z. M., and Hu, X. (2024). Influence of steel fiber shape and curing system on strength and toughness of UHPC. Mater. Rep. 38 (15), 23030088. doi:10.11896/cldb.23030088
Chen, Y., Hu, Y., and Wu, Z. M. (2023). Review on the deterioration of FRP reinforced concrete structures subjected to marine environment. Mater. Rep. 37 (18), 83–93. doi:10.11896/cldb.21120052
Cheng, Z., Zhang, Q., Bao, Y., Deng, P., Wei, C., and Li, M. (2021). Flexural behavior of corrugated steel-UHPC composite bridge decks. . Eng. Struct. 246, 113066. doi:10.1016/j.engstruct.2021.113066
Cui, C., and Sheikh, S. A. (2010). Analytical model for circular normal- and high-strength concrete columns confined with FRP. J. Compos. Constr. 14 (5), 562–572. doi:10.1061/(asce)cc.1943-5614.0000115
Dang, Z., Li, Z., and Feng, P. (2022). Axial compressive behavior of UHPC confined by FRP. Compos. Struct. 300, 116110. doi:10.1016/j.compstruct.2022.116110
Davids, W. G., Guzzi, D., and Schanck, A. P. (2022). Development and experimental assessment of friction-type shear connectors for FRP bridge girders with composite concrete decks. Decks. Mater. 15 (9), 3014. doi:10.3390/ma15093014
De Corte, W., and Uyttersprot, J. (2022). FRP bridges in the flanders region: experiences from the C-bridge Project. Appl. Sci. 12 (21), 10897. doi:10.3390/app122110897
Deng, Z., and Qu, J. (2015). The experimental studies on behavior of ultrahigh-performance concrete confined by hybrid fiber-reinforced polymer tubes. Adv. Mater. Sci. Eng. 2015, 1–18. doi:10.1155/2015/201289
Deng, Z. C. (2015). Progress in studies of the constitutive model of FRP-confined concrete columns. Mech. Eng. 37 (1), 33–39. doi:10.6052/1000-0879-14-131
Deng, Z. C. (2016). Effects of thicknesses and types of fiber reinforced polymer tubes on theuniaxial compressive behaviors of confined UHPC specimen. J. Harbin Eng. Univ. 37 (2), 218–222. doi:10.11990/jheu.201409037
Deng, Z. C., and Liu, S. X. (2016). Test and modeling of ultra-high performance concrete confined by fiber reinforced polymer tube. J. Basic Sci. Eng. 24 (4), 792–803. doi:10.16058/j.issn.1005-0930.2016.04.015
Deng, Z. C., and Wang, Y. C. (2015). Axial compression stress-strain model for UHPC cylinders confined by FRP. J. Southwest Jiaot. Univ. 50 (4), 641–647. doi:10.3969/j.issn.0258-2724.2015.04.011
Fallah Pour, A., Gholampour, A., Zheng, J., and Ozbakkaloglu, T. (2019). Behavior of FRP-confined high-strength concrete under eccentric compression: tests on concrete-filled FRP tube columns. Compos. Struct. 220, 261–272. doi:10.1016/j.compstruct.2019.03.031
Giraldo, J., and Rayhani, M. T. (2014). Load transfer of hollow Fiber-Reinforced Polymer (FRP) piles in soft clay. Transp. Geotech. 1 (2), 63–73. doi:10.1016/j.trgeo.2014.03.002
Guler, S. (2014). Axial behavior of FRP-wrapped circular ultra-high performance concrete specimens. Struct. Eng. Mech. 50 (6), 709–722. doi:10.12989/sem.2014.50.6.709
Hannawi, K., Bian, H., Prince-Agbodjan, W., and Raghavan, B. (2016). Effect of different types of fibers on the microstructure and the mechanical behavior of Ultra-High Performance Fiber-Reinforced Concretes. Compos. Part B Eng. 86, 214–220. doi:10.1016/j.compositesb.2015.09.059
Hoang, A. L., Fehling, E., Lai, B., Thai, D. K., and Chau, N. V. (2019). Experimental study on structural performance of UHPC and UHPFRC columns confined with steel tube. Eng. Struct. 187, 457–477. doi:10.1016/j.engstruct.2019.02.063
Huang, L., Xie, J., Li, L., Xu, B., Huang, P., and Lu, Z. (2021). Compressive behaviour and modelling of CFRP-confined ultra-high performance concrete under cyclic loads. Constr. Build. Mater. 310, 124949. doi:10.1016/j.conbuildmat.2021.124949
Lam, L., Huang, L., Xie, J.-H., and Chen, J. F. (2021). Compressive behavior of ultra-high performance concrete confined with FRP. Compos. Struct. 274, 114321. doi:10.1016/j.compstruct.2021.114321
Lam, L., and Teng, J. G. (2003). Design-oriented stress–strain model for FRP-confined concrete. Constr. Build. Mater. 17 (6–7), 471–489. doi:10.1016/s0950-0618(03)00045-x
Li, B., and Zhou, W. (2022). Experimental and finite element analysis on axial compression performance of concrete columns confined with CFRP tubes. . Mater. Rep. 36 (S1), 246–251.
Liang, X. Y., Chi, Y., and Zeng, Y. Q. (2020). Experimental studies on stress-strain relationship of ultra-high performance concrete confined by GFRP tube under uniaxial compression. Eng. J. Wuhan Univ. 53 (6), 498–506. doi:10.1016/j.engstruct.2021.113246
Liao, J., Yang, K. Y., Zeng, J.-J., Quach, W. M., Ye, Y. Y., and Zhang, L. (2021). Compressive behavior of FRP-confined ultra-high performance concrete (UHPC) in circular columns. Eng. Struct. 249, 113246. doi:10.1016/j.engstruct.2021.113246
Liao, J., Zeng, J.-J., Gong, Q.-M., Quach, W. M., Gao, W. Y., and Zhang, L. (2022). Design-oriented stress-strain model for FRP-confined ultra-high performance concrete (UHPC). Constr. Build. Mater. 318, 126200. doi:10.1016/j.conbuildmat.2021.126200
Liao, J., Zeng, J.-J., Lin, X.-C., and Zhuge, Y. (2023a). Punching shear behavior of FRP grid-reinforced ultra-high performance concrete slabs. J. Compos. Constr. 27 (4), 04023031. doi:10.1061/jccof2.cceng-4148
Liao, J., Zeng, J.-J., Zhuge, Y., Zheng, Y., Ma, G., and Zhang, L. (2023b). FRP-confined concrete columns with a stress reduction-recovery behavior: a state-of-the-art review, design recommendations and model assessments. Compos. Struct. 321, 117313. doi:10.1016/j.compstruct.2023.117313
Liu, J., Li, J., Fang, J., Su, Y., and Wu, C. (2022). Ultra-high performance concrete targets against high velocity projectile impact–a-state-of-the-art review. Int. J. Impact Eng. 160, 104080. doi:10.1016/j.ijimpeng.2021.104080
Lu, J. P. (2020). Compression behavior of FRP confined ultra high performance concrete [D]. Bangladesh: Southeast University.
Luo, M., Lin, P. Z., and Yang, Z. J. (2020). Study of mechanical properties and constitutive relations of UHPC under uniaxial compressive loading. Bridge Constr. 50 (5), 62–67. doi:10.3969/j.issn.1003-4722.2020.05.010
Ma, K., Ma, Y., Xing, G., and Liu, B. (2021). Behavior of ultra-high-performance concrete columns subjected to axial compressive load. Adv. Struct. Eng. 24 (16), 3792–3808. doi:10.1177/13694332211038440
Ma, K. Z., Han, X., and He, T. W. (2022). Investigation of FRP confined UHPC circular stub columns under axial compression. J. Southwest Jiaot. Univ. 2022, 1–9. doi:10.3969/j.issn.0258-2724.20220332
Mander, J. B., Priestley, M. J. N., and Park, R. (1988). Theoretical stress-strain model for confined concrete. J. Struct. Eng. 114 (8), 1804–1826. doi:10.1061/(asce)0733-9445(1988)114:8(1804)
Miao, K., Wei, Y., Dong, F., Zheng, K., and Wang, J. (2023). Experimental study on concrete-filled steel tube columns with inner distributed seawater and sea sand concrete-filled fiber-reinforced polymer tubes under axial compression. Compos. Struct. 320, 117181. doi:10.1016/j.compstruct.2023.117181
Mu, R., Chen, J., Chen, X., Diao, C., Wang, X., and Qing, L. (2023). Effect of the orientation of steel fiber on the strength of ultra-high-performance concrete (UHPC). Constr. Build. Mater. 406, 133431. doi:10.1016/j.conbuildmat.2023.133431
Ozbakkaloglu, T., Lim, J. C., and Vincent, T. (2013). FRP-confined concrete in circular sections: review and assessment of stress–strain models. Eng. Struct. 49, 1068–1088. doi:10.1016/j.engstruct.2012.06.010
Pimanmas, A., and Saleem, S. (2019). Evaluation of existing stress–strain models and modeling of PET FRP–confined concrete. J. Mater. Civ. Eng. 31 (12), 04019303. doi:10.1061/(asce)mt.1943-5533.0002941
Sadeghian, P., Rahai, A. R., and Ehsani, M. R. (2010). Effect of fiber orientation on compressive behavior of CFRP-confined concrete columns. J. Reinf. Plastics Compos. 29 (9), 1335–1346. doi:10.1177/0731684409102985
Saleem, S., Hussain, Q., and Pimanmas, A. (2017). Compressive behavior of PET FRP–confined circular, square, and rectangular concrete columns. J. Compos. Constr. 21 (3), 04016097. doi:10.1061/(asce)cc.1943-5614.0000754
Samaan, M., Mirmiran, A., and Shahawy, M. (1998). Model of concrete confined by fiber composites. J. Struct. Eng. 124 (9), 1025–1031. doi:10.1061/(asce)0733-9445(1998)124:9(1025)
Shan, L., and Zhang, L. (2019). Strength and fiber synergy effect of steel-polypropylene hybrid fibre-reinforced concrete. IOP Conf. Ser. Earth Environ. Sci. 304 (5), 052015. doi:10.1088/1755-1315/304/5/052015
Tbatou, T., and El Youbi, M. (2020). Dynamic and structural study of a RC building braced by FRP composite materials. Int. Rev. Civ. Eng. (IRECE) 11 (1), 1. doi:10.15866/irece.v11i1.16991
Teng, J. G., Jiang, T., and Lam, L. (2009). Refinement of a Design-Oriented Stress–Strain Model for FRP-Confined Concrete [J]. Journal of Composites for Construction 13 (4), 269–278. doi:10.1061/(ASCE)CC.1943-5614.0000012
Tian, H., Zhou, Z., Wei, Y., Wang, Y., and Lu, J. (2019b). Experimental investigation on axial compressive behavior of ultra-high performance concrete (UHPC) filled glass FRP tubes. Constr. Build. Mater. 225, 678–691. doi:10.1016/j.conbuildmat.2019.07.204
Tian, H. W., Zhou, Z., and Lu, J. P. (2019a). Effects of steel fiber content on axial compression performance of UHPC filled FRP tubes. J. Southeast Univ. Nat. Sci. Ed. 49 (3), 481–487. doi:10.3969/j.issn.1001-0505.2019.03.011
Tian, H. W., Zhou, Z., and Lu, J. P. (2020). Meso-scale numerical simulation of axial compression performance of fiber reinforced polymer composite-confined ultra-high performance concrete. Acta Mater. Compos. Sin. 37 (7), 1629–1638. doi:10.13801/j.cnki.fhclxb.20190827.001
Toutanji, H. A. (1999). Stress-strain characteristics of concrete columns externally confined with advanced fiber composite sheets. ACI Struct. J. 96 (3), 397–404.
Vincent, T., and Ozbakkaloglu, T. (2013). Influence of fiber orientation and specimen end condition on axial compressive behavior of FRP-confined concrete. Constr. Build. Mater. 47, 814–826. doi:10.1016/j.conbuildmat.2013.05.085
Wang, G., Wei, Y., Shen, C., Huang, Z., and Zheng, K. (2023a). Compression performance of FRP-steel composite tube-confined ultrahigh-performance concrete (UHPC) columns. Thin-Walled Struct. 192, 111152. doi:10.1016/j.tws.2023.111152
Wang, H. (2022). Review of research on ultra-high performance concrete. China Concr. Cem. Prod. (4), 25–28. doi:10.19761/j.1000-4637.2022.04.025.04
Wang, J. J., Zhang, S. S., Nie, X. F., and Yu, T. (2023b). Compressive behavior of FRP-confined ultra-high performance concrete (UHPC) and ultra-high performance fiber reinforced concrete (UHPFRC). Compos. Struct. 312, 116879. doi:10.1016/j.compstruct.2023.116879
Wang, W., Wu, C., Liu, Z., and Si, H. (2018b). Compressive behavior of ultra-high performance fiber-reinforced concrete (UHPFRC) confined with FRP. Compos. Struct. 204, 419–437. doi:10.1016/j.compstruct.2018.07.102
Wang, Y., Feng, J., and Li, J. Y. (2018a). Advance of FRP anchor bolts in geotechnical anchoring. J. Eng. Geol. 26 (3), 776–784. doi:10.13544/j.cnki.jeg.2017-139
Wang, Y., Liu, P., Cao, Q., Chen, G., Wan, B., Wei, Z., et al. (2021). Comparison of monotonic axial compressive behavior of rectangular concrete confined by FRP with different rupture strains. Constr. Build. Mater. 299, 124241. doi:10.1016/j.conbuildmat.2021.124241
Wu, G., Lv, Z. T., and Wu, Z. S. (2006). Strength and ductility of concrete cylinders confined with FRP composites. Constr. Build. Mater. 20, 134–148. doi:10.1016/j.conbuildmat.2005.01.022
Wu, Z., Shi, C., He, W., and Wu, L. (2016). Effects of steel fiber content and shape on mechanical properties of ultra high performance concrete. Constr. Build. Mater. 103, 8–14. doi:10.1016/j.conbuildmat.2015.11.028
Xu, L., Lu, Q., Chi, Y., Yang, Y., Yu, M., and Yan, Y. (2019). Axial compressive performance of UHPC filled steel tube stub columns containing steel-polypropylene hybrid fiber. Constr. Build. Mater. 204, 754–767. doi:10.1016/j.conbuildmat.2019.01.202
Yağar, A. C., İnce, C., and Derogar, S. (2022). FRP strengthening of RC structures: sustainable, environmental and structural evaluations. J. Sustain. Constr. Mater. Technol. 7 (4), 358–374. doi:10.47481/jscmt.1211086
Yoo, D.-Y., Banthia, N., Kang, S.-T., and Yoon, Y. S. (2016). Size effect in ultra-high-performance concrete beams. Eng. Fract. Mech. 157, 86–106. doi:10.1016/j.engfracmech.2016.02.009
Yoo, D.-Y., Lee, J.-H., and Yoon, Y.-S. (2013). Effect of fiber content on mechanical and fracture properties of ultra high performance fiber reinforced cementitious composites. Compos. Struct. 106, 742–753. doi:10.1016/j.compstruct.2013.07.033
Yu, K.-Q., Yu, J.-T., Dai, J.-G., Lu, Z. D., and Shah, S. P. (2018). Development of ultra-high performance engineered cementitious composites using polyethylene (PE) fibers. Constr. Build. Mater. 158, 217–227. doi:10.1016/j.conbuildmat.2017.10.040
Yuan, W.-Y., Han, Q., and Bai, Y.-L. (2022b). A unified stress-strain model for LRS FRP-confined concrete columns with square and circular cross-sections. Eng. Struct. 255, 113900. doi:10.1016/j.engstruct.2022.113900
Yuan, W. Y., Han, Q., and Bai, Y. L. (2022a). A unified confinement model of FRP-wrapped concrete cylinder. China J. Highw. Transp. 35 (2), 146–158. doi:10.19721/j.cnki.1001-7372.2022.02.013
Zeng, J.-J., Ye, Y.-Y., Gao, W.-Y., Smith, S. T., and Guo, Y. C. (2020). Stress-strain behavior of polyethylene terephthalate fiber-reinforced polymer-confined normal-high- and ultra high-strength concrete. J. Build. Eng. 30, 101243. doi:10.1016/j.jobe.2020.101243
Zeng, L., Yu, W., Mo, Z., Huang, S. q., and Yuan, H. (2023). Experimental and numerical studies on sea sand concrete filled stainless steel tube with inner FRP tube subjected to axial compression. China Ocean. Eng. 37 (2), 272–287. doi:10.1007/s13344-023-0023-5
Zhang, B., Wei, W., and Feng, G. S. (2019). Influences of fiber angles on axial compressive behavior of GFRP-confined concrete stub column. J. Build. Struct. 40 (S1), 192–199. doi:10.14006/j.jzjgxb.2019.S1.025
Zhang, S. S., Wang, J. J., Lin, G., Yu, T., and Fernando, D. (2023b). Stress-strain models for ultra-high performance concrete (UHPC) and ultra-high performance fiber-reinforced concrete (UHPFRC) under triaxial compression. Constr. Build. Mater. 370, 130658. doi:10.1016/j.conbuildmat.2023.130658
Zhang, X., Wang, H., Zhang, Y., and Wang, L. (2023a). Corrosion of steel rebars across UHPC joint interface under chloride attack. Constr. Build. Mater. 387, 131591. doi:10.1016/j.conbuildmat.2023.131591
Zhao, P., Huang, Y., Liu, Z., Lu, Y., and Wang, H. (2022a). Experimental study on seismic performance of hybrid steel-polypropylene fiber-reinforced recycled aggregate concrete-filled circular steel tube columns. Constr. Build. Mater. 359, 129418. doi:10.1016/j.conbuildmat.2022.129418
Zhao, Y., Cao, X., and Chen, C. C. (2022b). Effect of steel fiber on properties of ultra-high performance concrete. Jiangsu Build. Mater. (6), 11–13. doi:10.3969/j.issn.1004-5538.2022.06.005
Zhao, Z., Wei, Y., Wang, G., Miao, K., and Zheng, K. (2023b). Exploration on unified calculation of axial compressive load-carrying capacity of square and rectangular concrete-filled steel tubular columns. Constr. Build. Mater. 398, 132546. doi:10.1016/j.conbuildmat.2023.132546
Zhao, Z., Wei, Y., Wang, G., Zhang, Y., and Lin, Y. (2023a). Axial compression performance of square UHPC-filled stainless-steel tubular columns. Constr. Build. Mater. 408, 133622. doi:10.1016/j.conbuildmat.2023.133622
Zhu, M. Q., Tan, X. P., and Liu, W. L. (2023). Pseudostatic experimental study of GFRP tube constraining UHPC column. J. Hunan Univ. Sci. Technol. Nat. Sci. Ed. 38 (1), 25–34. doi:10.13582/j.cnki.1672-9102.2023.01.004
Zohrevand, P., and Mirmiran, A. (2011). Behavior of ultrahigh-performance concrete confined by fiber-reinforced polymers. J. Mater. Civ. Eng. 23 (12), 1727–1734. doi:10.1061/(asce)mt.1943-5533.0000324
Keywords: FRP, axial compression, constitutive model, UHPC, column
Citation: Song S, Zhang B, You P and Yang X (2024) A review study on axial compression properties of UHPC columns confined by FRP. Front. Mater. 10:1339386. doi: 10.3389/fmats.2023.1339386
Received: 16 November 2023; Accepted: 27 December 2023;
Published: 12 January 2024.
Edited by:
Mijia Yang, North Dakota State University, United StatesReviewed by:
Yang Wei, Nanjing Forestry University, ChinaJun-Jie Zeng, Guangdong University of Technology, China
Copyright © 2024 Song, Zhang, You and Yang. This is an open-access article distributed under the terms of the Creative Commons Attribution License (CC BY). The use, distribution or reproduction in other forums is permitted, provided the original author(s) and the copyright owner(s) are credited and that the original publication in this journal is cited, in accordance with accepted academic practice. No use, distribution or reproduction is permitted which does not comply with these terms.
*Correspondence: Shuaiqi Song, 30070507@huuc.edu.cn; Bowen Zhang, z1303431002@163.com