- 1College of Mechanical and Electrical Engineering, Shaanxi University of Science & Technology, Xi’an, China
- 2School of Automation, Beijing University of Posts and Telecommunications, Beijing, China
Shape memory polymers (SMP) are the smart materials that have received the most attention and are the most commonly employed. SMP can be 4D printed to create prefabricated components with transform shapes, properties, or functions under different external excitations. Although smart equipment assembled from these prefabricated components is extensively used in various fields, a systematic overview summary of their design ideas still needs to be provided. This paper briefly analyzes current trends in the design ideas and application of SMP-based prefabricated components. By summarizing two different design ideas: structural combination and material modification, it demonstrates the application of smart equipment assembled from SMP-based prefabricated components in medical, industrial, and aerospace fields. Furthermore, current challenges with both design ideas are discussed.
1 Introduction
Tibbits. (2014); Tibbits. (2016) first proposed the concept of 4D printing technology in 2014, which was innovative and integrated technology based on smart materials, excitation response, and additive manufacturing (Khalid et al., 2022a). Through different printing methods combined with smart materials, prefabricated components with dynamic adaptability under predetermined structural excitation were developed, allowing for more creativity and freedom in the structure design of smart equipment, with numerous advantages over conventional manufacturing (Arif et al., 2022a).
Shape memory polymers (SMP), an excitation-responsive smart material with shape memory properties, could change from a temporary shape to an initial shape in response to external excitation and complete a shape memory cycle (Khalid et al., 2021a). SMP was initially developed by the French company Cdf Chime and was a polynorbornene sheet (Li et al., 2018). SMP has shown great potential in many applications because of its lightweight, adjustable transition temperature, biocompatibility, distinguishable actuating mode, low cytotoxicity, and large deformation, compared with other smart materials, such as shape memory alloys and shape memory ceramics (Khalid et al., 2021b; Khalid et al., 2022b). As a result, many technology prototypes and early commercial practices have been proposed and implemented in medical devices, flexible electronics, textiles, self-healing materials, information carriers, aerospace, soft robotics, and microdrives (Kim et al., 2020; Adam et al., 2021; Demoly et al., 2021; Koch et al., 2021; Schönfeld et al., 2021). Despite its excellent application potential, SMP’s conventional molding process was highly mold-dependent and severely limited by the demolding process (Wu et al., 2017), which caused the prefabricated components with sketchy and low precision. It also made assembling smart equipment with high precision and complexity intricate.
The use of 4D printing technology, based on the SMP properties, and manufacturing of prefabricated components, will effectively overcome the shortcomings of the conventional SMP molding process and bring new opportunities for developing smart equipment. Fused deposition modeling (FDM) (Przekop et al., 2020; Sharma and Rai, 2022), stereo lithography apparatus (SLA) (Al Rashid et al., 2021), digital light processing (DLP) (Nguyen and Kim, 2020), multiphoton lithography (MPL) (Liao et al., 2020), and direct ink writing (DIW) (Wan et al., 2020) were the primary methods for 4D printed SMP. Self-assembling, versatile, pre-programmable, variable stiffness, high precision, and structurally complex prefabricated components could be prepared using diverse 4D printing methods combined with SMP material properties. These prefabricated components could be assembled into more complex, high-precision, and sophisticated smart equipment (see Figure 1).
Although SMP prefabricated components with excitation response properties based on 4D printing have been widely applied in some smart equipment (Arif et al., 2022b). However, an overview of their design ideas was lacking. Therefore, this paper summarized the design ideas based on 4D printed SMP prefabricated components with excitation response properties into the structural combination and material modification, starting from two primary perspectives of material and structure. The design idea of structural combination utilized the inherent properties of SMP and other materials (including other types of smart and non-smart materials) to design prefabricated components with specific deformation patterns or varying degrees of deformation via structure arrangement and combination. For instance, Datta et al. (2020) assembled a hybrid propeller amphibious robot with thermal deformation responsiveness using a hub prepared of SMP and a blade prepared of non-smart resin material. The design idea of material modification utilized improvements in SMP properties to design prefabricated components for unique application scenarios and special functions. For instance, Cui et al. (2022) proposed the introduction of dynamic thiocarbamate bonds into light-cured methacrylate to prepare light-cured 4D printed polyurethane materials (4DP-TPU) with processable, self-healing properties. Because of their unique self-healing qualities, the prefabricated components prepared from 4DP-PTU have a wide range of applications in the assembly of bio-implanted smart equipment.
2 Prefabricated components designed based on structural combination
Some application scenarios required the 4D-printed SMP prefabricated components to produce specific deformation patterns or varying degrees of deformation. These scenarios mostly adopted the design idea of structural combination, which was classified into three types.
The first type was the design idea of considering SMP and non-smart materials as components which were arranged and combined to prepare a whole prefabricated component. By utilizing the property that SMP produces changes under external excitation conditions while non-smart materials do not change or produce only minor changes, 4D-printed SMP prefabricated components with controllable deformation were obtained. For instance, Yang (Yang et al., 2016) demonstrated the 4D-printed variable stiffness robotic finger consisting of a soft pneumatic actuator embedded in an SMP substrate and a pin heater. The significant variation in the elastic modulus of SMP around its glass transition temperature was used to achieve the variable stiffness function of the soft finger, which could potentially be developed as a variable stiffness gripper or hand. Wan et al. (2020); Bai and Bu, 2022) assembled grasping mechanisms using the SMP prefabricated components prepared by laminating paper plates with SMP plates to achieve the function of grasping under external thermal excitation (Figure 2A). Li et al. (2018) demonstrated a self-folding composite variable diameter wheel structure by 4D printing. This structure actuator consisted of a thermoplastic polyurethane elastomer (TPU) embedded in a polylactic acid (PLA) sandwich. This structure merged a control system and a variable diameter mechanical structure into one, enabling drivable self-adjustment of the wheel diameter size ratio under external thermal excitation (Figure 2B).
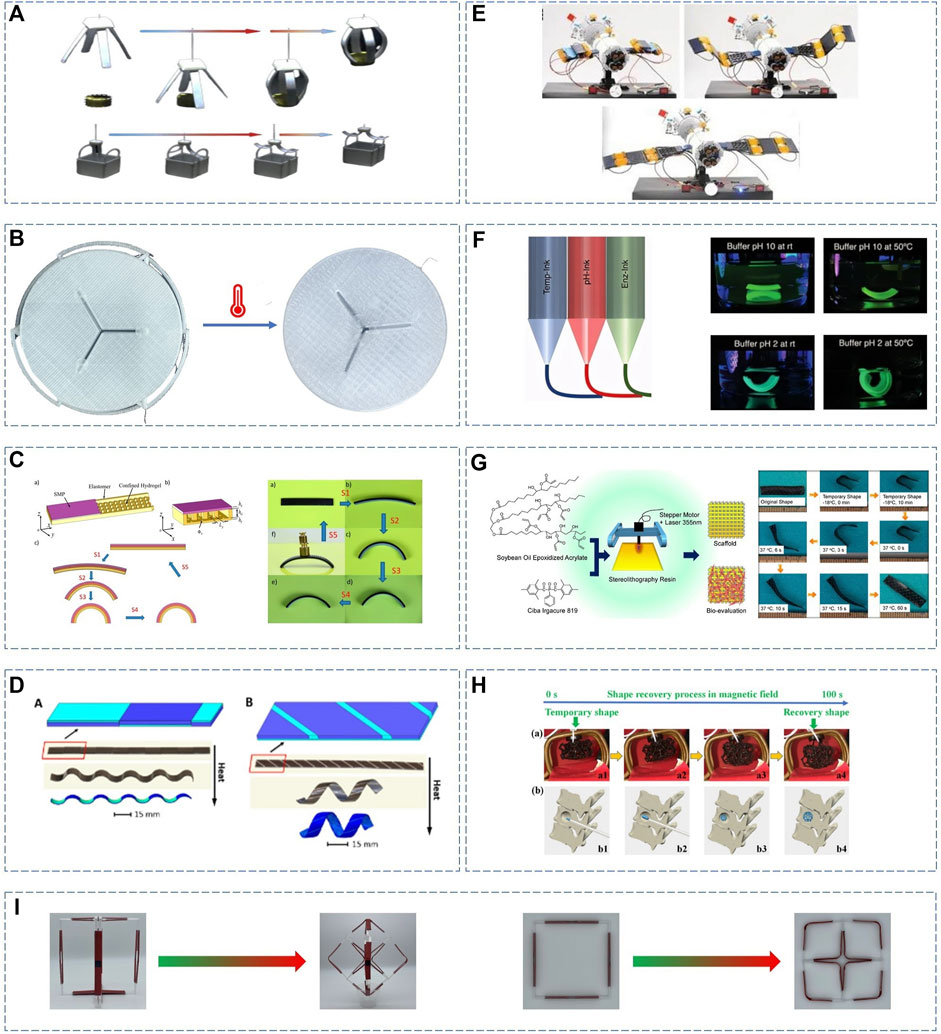
FIGURE 2. (A) 4D printed soft grasping mechanisms (Tolly et al., 2013; Yang et al., 2021) (B) Variable diameter wheel made by Shaanxi University of Science and Technology (C) 4D printed bidirectional reversible deformable prefabricated components (Mao et al., 2016) (D) 4D printing controlled bending and spiral deformation prefabricated components (Ding et al., 2017; Duanling and Wencai, 2022a) (E) 4D printed Satellite solar panel with smart structure (Zhang et al., 2021b) (F) 4D printing of multi-stimuli responsive protein-based hydrogels (Narupai et al., 2021) (G) 4D printed hMSC scaffold (Miao et al., 2016) (H) Mechanistic simulation of 4D printed prefabricated components for bone tissue repair (Zhang et al., 2019) (I) 4D printed spatial and planarmorphology controllable mechanisms (Duanling and Wencai, 2022b).
The second type was the design idea of considering SMP and other types of smart materials as components which were arranged and combined to prepare a whole prefabricated component. By utilizing the property that SMP produces changes under external excitation conditions while other types of smart materials produce different changes under the same or different external excitation conditions, 4D-printed SMP prefabricated components with controllable deformation were obtained. For instance, Mao et al. (2016) used three-layer structures that combined SMP, TPU, and hydrogels in prefabricated components, each layer having a function. It utilized water as the excitation source in the setup and heat as the excitation source in the recovery phase. This combination was developed for bidirectional reversible deformation, distinguishing it from conventional unidirectional irreversible prefabricated components (Figure 2C). Roudaut (Yicong et al., 2020) developed a consisting of SMP and shape memory alloy of a prefabricated component. Based on this component, self-starting, flexible mobile equipment was assembled, which can adjust its shape according to the usage environment to provide better bearing force.
Most controlled deformations of 4D-printed SMP prefabricated components obtained through structural combination were simple bending. Complex deformation patterns such as twists, spirals, and waves were difficult to achieve. Therefore, the third type was the design idea of adjusting the path arrangement and filling gradients in SMP, non-smart materials, or other smart materials components. Then, arranging and combining them into a whole 4D-printed SMP prefabricated component was created to achieve more complex deformation patterns. Yang et al. (2017); Wang et al., 2019) proposed designing 4D-printed prefabricated components with fiber plates compounded with SMP plates by arranging the continuous fiber plate arrangement equations. Varying degrees of bending of these prefabricated components was achieved under the excitation of environment temperature variations. Ding et al. (2017); Feng et al., 2020 proposed a design for straight-through combinations using different filling gradients of elastomeric materials and SMP. Based on the above design, 4D-printed SMP prefabricated components may produce various controlled deformation patterns, such as bending and twisting in the heated excitation (Figure 2D).
3 Prefabricated components designed based on material modification
There were some unique application scenarios, such as high vacuum or ultraviolet (UV) radiation in the space environment and cellular non-toxicity, biodegradability, or body temperature stability in the intra-human environment. These scenarios required the 4D-printed SMP prefabricated components with specific properties, such as specific mechanical properties, biocompatibility, and diverse excitation-response patterns. These scenarios mostly adopted the design idea of material modification.
4D Printing SMP using UV-curable technology could prepare prefabricated components with complex geometries and high resolution. This technology was compatible with 4D printing using DLP technology and had the potential for development in space additive manufacturing. However, UV-curable SMP has limitations in terms of mechanical properties, which significantly constrains their application fields. Zhang et al. (2021a) modified SMP with tert-butyl acrylate (tBA) and aliphatic polyurethane diacrylate (AUD) to make it compatible with DLP-based 4D printing. Using the excellent fatigue resistance and deformability exhibited by this material, the 4D-printed prefabricated components could be applied to unfold the smart structures of satellite solar panels (Figure 2E).
With its stable temperature and complex tissue environment, the human body’s internal environment placed stringent requirements on the medical devices implanted. It was required to have significantly low toxicity and to properly stimulate the body’s appropriate functions in a given application. Miao et al. (2016) modified plant oil polymers with high biocompatibility adapted to the human body temperature to replace the conventional polyethylene glycol diacrylate. This material was used to prepare a 4D-printed prefabricated scaffold with shape memory properties capable of implanting into the human body to support the growth of human bone marrow mesenchymal stem cells (hMSC). Shape-memory analysis showed that the 4D-printed prefabricated scaffold could be fixed into a temporary shape at −18°C and regain its original shape at human body temperature (37°C) (Figure 2G). Cytotoxicity analysis showed that the 4D-printed prefabricated scaffold was non-cytotoxic and significantly affected the adhesion and proliferation of hMSC. Zhou et al. (2022) modified SMP based on bioactive fillers (hydroxyapatite and alendronate) and collagen-dexamethasone (Col-Dex) coating. This material was used to prepare a 4D-printed prefabricated scaffold with shape memory properties capable of implanting into the human body to fill bone defects. In addition, the biological studies demonstrated the effective biological activities and osteogenic effects of the 4D printed prefabricated scaffolds, with potential applications in bone tissue regeneration. Narupai et al. (2021) modified hydrogels based on the 4D printed protein and applied them for prefabricated components changes under the action of temperature, PH, or an enzyme, which is appealing for soft robotics, biomedical devices, drug delivery, and actuators (Figure 2F).
The SMP was modified with magnetic materials to give it a widely functional behavior, and magnetic field excitation-response patterns could be used in scenarios controlled in a contactless method, such as intra-human (Bastola and Hossain, 2021). Moreover, the magnetic field caused less harm to the human body. Therefore, developing SMP prefabricated components with magnetic field excitation response properties provided novel opportunities to assemble medical devices implanted intra-human controlled in a contactless method. Zhang et al. (2019) modified SMP by combining PLA and Fe3O4 nanoparticles in solution and prepared magnetic field excitation-response PLA/Fe3O4 nanocomposites with biodegradability and biocompatibility. When the mass of Fe3O4 nanoparticles in the nanocomposites was 20%, the shape recovery and fixation rate exceeded 95% in a magnetic field of 27.5 kHz, and the response time was reduced to 8 s. Through simulation, 4D-printed prefabricated scaffolds with shape memory properties, prepared using this material, could be implanted in the human body to support bone tissue repair (Figure 2H). Liu et al. (2023) modified SMP based on PLA, TPU, and Fe3O4 particles to provide robustly mechanical properties and magneto-responsive behavior. The modified materials’ high-load capacity, fast magneto-responsive behavior, and high recovery performance have great potential in medical millirobots implanted intra-human controlled in a contactless method.
4 Current challenges
The design ideas based on 4D-printed SMP prefabricated components were summarized into the structural combination based on deformation patterns and material modification based on unique application scenarios. The two design ideas have been gradually developed and partially applied in various fields with promising prospects. However, there were still some challenges and opportunities with both design ideas.
1) Based on structural combination design ideas, 4D-printed SMP prefabricated components have achieved specific deformation patterns (such as unidirectional, bidirectional reversible, and torsional) or varying degrees of deformation. The excitation source was mainly thermal stimuli. They have been preliminarily applied in the fields of industrial robotics and aerospace. Because of excellent features such as lightweight, low-cost, and structure-drive integration, these 4D printed SMP prefabricated components were particularly suitable for use in smart equipment in the aerospace field, such as SMP composite flexible solar array systems (Lan et al., 2020).
However, there were still some challenges in applying this design idea, such as how to achieve more complex topology transformations for 4D printed SMP prefabricated components through design ideas of structure combination. And how to achieve finer drive control for 4D printed SMP prefabricated components through design ideas of structure combination. In order to address the challenges above, the following three progressive dimensions of research and one experimental method will likely provide opportunities for their development. The first dimension will investigate the stimulus-response properties of multiple SMPs. The second dimension will investigate the impact of the print path and fill gradient parameters on the deformation of prefabricated components during the 4D printing of multiple SMPs. The third dimension will investigate the effect of smart equipment structural morphology on the deformation of prefabricated components during the assembly. Furthermore, experimental methods will be used to fit the effect of external excitation on deformation control. For example, by combining traditional mechanical structure design, smart materials, and excitation response experiments, the above research ideas are effectively used to achieve complex and precisely controlled spatial and planar topological transformations of 4D printed SMP prefabricated components (Figure 2I) (Zhongyi et al., 2020; Zhang et al., 2022).
2) Based on material modification design ideas, 4D-printed SMP prefabricated components have adapted to some application-unique scenarios (such as high vacuum or UV radiation and intra-human environment). The excitation sources mainly included temperature, magnetic, chemical, and biological stimuli. They have been preliminarily applied in the field of medicine and aerospace. Because of the variety of excellent non-toxicity and biocompatibility, these 4D printed SMP prefabricated components were particularly suitable for use in smart equipment in the medical field, such as SMP tracheal stents (Zhang et al., 2021b).
However, there were still some challenges in applying this design idea, such as how to respond to diverse excitation sources for 4D printed SMP prefabricated components through design ideas of material modification. And how to enhance the mechanical properties of 4D printed SMP prefabricated components through design ideas of material modification. In order to address the above challenges and in conjunction with the research hotspots and directions in the field of SMP, the nanomaterial-based modified SMP will likely provide opportunities for their development. SMP nanocomposites have high elastic modulus and mechanical properties, which can significantly increase their shape recovery force and obtain new stimulus-response patterns based on Nano effects (Bhanushali et al., 2021). For example, graphene can impart superior electrical conductivity to SMP, and boron nitride nanosheets can improve SMP’s thermal conductivity and shape recovery speed, carbon nanotubes can impart superior mechanical strength and multifunctional stimulus responsiveness to SMP (Kuma and Purohit, 2019; Demoly et al., 2021).
3) Integrate design ideas of structural combinations and material modifications of prefabricated components. By improving SMP material properties to meet the needs of unique application scenarios, complex controlled deformation of prefabricated components was achieved using structural combinations to ensure that it meets the design shape and functional requirements. This integration will likely improve the deformation patterns of 4D-printed SMP prefabricated components and broadens the conditions for their practical applications, which provides new development opportunities for 4D-printed SMP prefabricated components.
Author contributions
WZ and DL carried out the structure of the article and wrote the paper, DL figured out the literature review on applications, WZ finished drafting and analysis and the final version of the manuscript.
Funding
This research was supported by the National Natural Science Foundation of China [grant number 52175019], and the Natural Science Foundation of Beijing Municipality [grant number 3212009].
Conflict of interest
The authors declare that the research was conducted in the absence of any commercial or financial relationships that could be construed as a potential conflict of interest.
Publisher’s note
All claims expressed in this article are solely those of the authors and do not necessarily represent those of their affiliated organizations, or those of the publisher, the editors and the reviewers. Any product that may be evaluated in this article, or claim that may be made by its manufacturer, is not guaranteed or endorsed by the publisher.
References
Adam, G., Benouhiba, A., Rabenorosoa, K., Clévy, C., and Cappelleri, D. J. (2021). 4D printing: Enabling technology for microrobotics applications. Adv. Intell. Syst. 3, 2000216. doi:10.1002/aisy.202000216
Al Rashid, A., Ahmed, W., Khalid, M. Y., and Koç, M. (2021). VAT photopolymerization of polymers and polymer composites: Processes and applications. Addit. Manuf. 47, 102279. doi:10.1016/j.addma.2021.102279
Arif, Z. U., Khalid, M. Y., Ahmed, W., and Arshad, H. (2022a). A review on four-dimensional (4D) bioprinting in pursuit of advanced tissue engineering applications. Bioprinting 27, e00203. doi:10.1016/j.bprint.2022.e00203
Arif, Z. U., Khalid, M. Y., Sheikh, M. F., Zolfagharian, A., and Bodaghi, M. (2022b). Biopolymeric sustainable materials and their emerging applications. J. Environ. Chem. Eng. 10, 108159. doi:10.1016/j.jece.2022.108159
Bai, J., and Bu, G. (2022). Progress in 4D printing technology. J. Adv. Manuf. Sci. Technol. 2, 2022001. doi:10.51393/j.jamst.2022001
Bastola, A. K., and Hossain, M. (2021). The shape–morphing performance of magnetoactive soft materials. Mater. Des. 211, 110172. doi:10.1016/j.matdes.2021.110172
Bhanushali, H., Amrutkar, S., Mestry, S., and Mhaske, S. T. (2021). Shape memory polymer nanocomposite: A review on structure–property relationship. Polym. Bull. 79, 3437–3493. doi:10.1007/s00289-021-03686-x
Cui, C., An, L., Zhang, Z., Ji, M., Chen, K., Yang, Y., et al. (2022). Reconfigurable 4D printing of reprocessable and mechanically strong polythiourethane covalent adaptable networks. Adv. Funct. Mater. 32, 2203720. doi:10.1002/adfm.202203720
Datta, S., Henry, T., Hall, A., and Chattopadhyay, A. (2020). “Carbon nanotube enhanced shape memory polyurethane for improved mechanical properties and shape recovery,” in 2020 AIAA Scitech 2020 Forum, Orlando, Florida, 05 January 2020. doi:10.2514/6.2020-2233
Demoly, F., Dunn, M. L., Wood, K. L., Qi, H. J., and André, J.-C. (2021). The status, barriers, challenges, and future in design for 4D printing. Mater. Des. 212, 110193. doi:10.1016/j.matdes.2021.110193
Ding, Z., Yuan, C., Peng, X., Wang, T., Qi, H. J., and Dunn, M. L. (2017). Direct 4D printing via active composite materials. Sci. Adv. 3, e1602890. doi:10.1126/sciadv.1602890
Duanling, L., and Wencai, Z. (2022a). Form-variable wheel type mechanism based on 4D printing technology. Beijing, China: Chinese Patent. CN113954361A[P].
Duanling, L., and Wencai, Z. (2022b). Spatial variation spherical shear fork deployable mechanism based on 4D printing technology. Beijing, China: Chinese Patent. CN216100445U[P].
Feng, Y., Xu, J., Zeng, S., Gao, Y., and Tan, J. (2020). Controlled helical deformation of programmable bilayer structures: Design and fabrication. Smart Mater. Struct. 29, 085042. doi:10.1088/1361-665x/ab905d
Khalid, M. Y., Al Rashid, A., Arif, Z. U., Ahmed, W., and Arshad, H. (2021a). Recent advances in nanocellulose-based different biomaterials: Types, properties, and emerging applications. J. Mater. Res. Technol. 14, 2601–2623. doi:10.1016/j.jmrt.2021.07.128
Khalid, M. Y., Al Rashid, A., Arif, Z. U., Ahmed, W., Arshad, H., and Zaidi, A. A. (2021b). Natural fiber reinforced composites: Sustainable materials for emerging applications. Results Eng. 11, 100263. doi:10.1016/j.rineng.2021.100263
Khalid, M. Y., Arif, Z. U., and Ahmed, W. (2022b). 4D printing: Technological and manufacturing renaissance. Macromol. Mater. Eng. 307, 2200003. doi:10.1002/mame.202200003
Khalid, M. Y., Arif, Z. U., Noroozi, R., Zolfagharian, A., and Bodaghi, M. (2022a). 4D printing of shape memory polymer composites: A review on fabrication techniques, applications, and future perspectives. J. Manuf. Process. 81, 759–797. doi:10.1016/j.jmapro.2022.07.035
Kim, S. H., Seo, Y. B., Yeon, Y. K., Lee, Y. J., Park, H. S., Sultan, M. T., et al. (2020). 4D-bioprinted silk hydrogels for tissue engineering. Biomaterials 260, 120281. doi:10.1016/j.biomaterials.2020.120281
Koch, H. C., Schmelzeisen, D., and Gries, T. (2021). 4D textiles made by additive manufacturing on pre-stressed textiles-an overview. Actuators 10, 31. doi:10.3390/act10020031
Kuma, P. K., and Purohit, R. (2019). Improved shape memory and mechanical properties of microwave-induced thermoplastic polyurethane/graphene nanoplatelets composites. Sensors Actuators A Phys. 285, 17–24. doi:10.1016/j.sna.2018.10.049
Lan, X., Liu, L. W., Zhang, F. H., Liu, Z. X., Wang, L. L., Li, Q. F., et al. (2020). World’s first spaceflight on-orbit demonstration of a flexible solar array system based on shape memory polymer composites. Sci. China Technol. Sci. 63, 1436–1451. doi:10.1007/s11431-020-1681-0
Li, C., Zhang, F., Wang, Y., Zheng, W., Liu, Y., and Leng, J. (2018). Development of 4D printed shape memory polymers in biomedical field. Sci. China Technol. Sci. 49, 13–25. doi:10.1360/n092018-00153
Liao, C., Wuethrich, A., and Trau, M. (2020). A Material Odyssey for 3D Nano/microstructures: Two photon polymerization-based nanolithography in bioapplications. Appl. Mater. Today 19, 100635. doi:10.1016/j.apmt.2020.100635
Liu, H., Wang, F., Wu, W., Dong, X., and Sang, L. (2023). 4D printing of mechanically robust PLA/TPU/Fe3O4 magneto-responsive shape memory polymers for smart structures. Compos. Part B Eng. 248, 110382. doi:10.1016/j.compositesb.2022.110382
Mao, Y., Ding, Z., Yuan, C., Ai, S., Isakov, M., Wu, J., et al. (2016). 3D printed reversible shape changing components with stimuli responsive materials. Sci. Rep. 6, 24761. doi:10.1038/srep24761
Miao, S., Zhu, W., Castro, N. J., Nowicki, M., Zhou, X., Cui, H., et al. (2016). 4D printing smart biomedical scaffolds with novel soybean oil epoxidized acrylate. Sci. Rep. 6, 27226. doi:10.1038/srep27226
Narupai, B., Smith, P. T., and Nelson, A. (2021). 4D printing of multi-stimuli responsive protein-based hydrogels for autonomous shape transformations. Adv. Funct. Mater. 31, 2011012. doi:10.1002/adfm.202011012
Nguyen, T. T., and Kim, J. (2020). 4D-printing — Fused deposition modeling printing and polyjet printing with shape memory polymers composite. Fibers Polym. 21, 2364–2372. doi:10.1007/s12221-020-9882-z
Przekop, R. E., Kujawa, M., Pawlak, W., Dobrosielska, M., Sztorch, B., and Wieleba, W. (2020). Graphite modified polylactide (PLA) for 3D printed (FDM/FFF) sliding elements. Polymers 12, 1250. doi:10.3390/polym12061250
Schönfeld, D., Chalissery, D., Wenz, F., Specht, M., Eberl, C., and Pretsch, T. (2021). Actuating shape memory polymer for Thermoresponsive Soft Robotic Gripper and programmable materials. Molecules 26, 522. doi:10.3390/molecules26030522
Sharma, A., and Rai, A. (2022). Fused deposition modelling (FDM) based 3D and 4D printing: A state of art review. Mater. Today Proc. 62, 367–372. doi:10.1016/j.matpr.2022.03.679
Tibbits, S. (2014). 4D printing: Multi-material shape change. Archit. Des. 84, 116–121. doi:10.1002/ad.1710
Tibbits, S. (2016). Printing products. 3D Print. Addit. Manuf. 3, 135. doi:10.1089/3dp.2016.29005.sti
Tolley, M. T., Felton, S. M., Miyashita, S., Xu, L., Shin, B., Zhou, M., et al. (2013). “Self-folding shape memory laminates for automated fabrication,” in 2013 IEEE/RSJ International Conference on Intelligent Robots and Systems, Tokyo, Japan, 03-07 November 2013 (IEEE). doi:10.1109/iros.2013.6697068
Wan, X., Luo, L., Liu, Y., and Leng, J. (2020). Direct ink writing based 4D printing of materials and their applications. Adv. Sci. 7, 2001000. doi:10.1002/advs.202001000
Wang, W., Yu, C. Y., Abrego Serrano, P. A., and Ahn, S.-H. (2019). Soft grasping mechanisms composed of shape memory polymer based self-bending units. Compos. Part B Eng. 164, 198–204. doi:10.1016/j.compositesb.2018.10.081
Wu, J. J., Huang, L. M., Zhao, Q., and Xie, T. (2017). 4D printing: History and recent progress. Chin. J. Polym. Sci. 36, 563–575. doi:10.1007/s10118-018-2089-8
Yang, C., Tian, X., Liu, T., Cao, Y., and Li, D. (2017). 3D printing for continuous fiber reinforced thermoplastic composites: Mechanism and performance. Rapid Prototyp. J. 23, 209–215. doi:10.1108/rpj-08-2015-0098
Yang, G. H., Kim, W., Kim, J., and Kim, G. H. (2021). A skeleton muscle model using Gelma-based cell-aligned bioink processed with an electric-field assisted 3D/4D bioprinting. Theranostics 11, 48–63. doi:10.7150/thno.50794
Yang, Y., Chen, Y., Wei, Y., and Li, Y. (2016). Novel design and three-dimensional printing of variable stiffness robotic grippers. J. Mech. Robotics 8. doi:10.1115/1.4033728
Yicong, G., Siyuan, Z., Yixiong, F., Hao, Z., Hao, Q., and Jianrong, T. (2020). Review of Design of programmable morphing composite structures by 4D printing. J. Mech. Eng. 56, 26. doi:10.3901/jme.2020.15.026
Zhang, B., Li, H., Cheng, J., Ye, H., Sakhaei, A. H., Yuan, C., et al. (2021a). Mechanically robust and uv-curable shape-memory polymers for digital light processing based 4D printing. Adv. Mater. 33, 2101298. doi:10.1002/adma.202101298
Zhang, B., Wen, N., Wang, L., Bai, Y., and Leng, J. (2021b). Design of 4D printed shape-changing tracheal stent and remote controlling actuation. Int. J. Smart Nano Mater. 12, 375–389. doi:10.1080/19475411.2021.1974972
Zhang, F., Wang, L., Zheng, Z., Liu, Y., and Leng, J. (2019). Magnetic programming of 4D printed shape memory composite structures. Compos. Part A Appl. Sci. Manuf. 125, 105571. doi:10.1016/j.compositesa.2019.105571
Zhang, W., Ge, Z., and Li, D. (2022). Evolution and emerging trends of 4D printing: A bibliometric analysis. Manuf. Rev. 9, 30. doi:10.1051/mfreview/2022028
Zhongyi, H., Yuguang, Y., Pengyu, L., and Huiling, D. (2020). Motion function transformation and control of amphibious robot based on 4D printing. J. Mech. Eng. Sci. 56, 39. doi:10.3901/jme.2020.15.039
Keywords: smart materials, shape memory polymers, prefabricated components, 4D printing, design ideas
Citation: Zhang W and Li D (2023) Shape memory polymer-based prefabricated components: Design ideas and prospects. Front. Mater. 10:1095384. doi: 10.3389/fmats.2023.1095384
Received: 11 November 2022; Accepted: 05 January 2023;
Published: 12 January 2023.
Edited by:
Yi Ge, Queen’s University Belfast, United KingdomReviewed by:
Mokarram Hossain, Swansea University, United KingdomCopyright © 2023 Zhang and Li. This is an open-access article distributed under the terms of the Creative Commons Attribution License (CC BY). The use, distribution or reproduction in other forums is permitted, provided the original author(s) and the copyright owner(s) are credited and that the original publication in this journal is cited, in accordance with accepted academic practice. No use, distribution or reproduction is permitted which does not comply with these terms.
*Correspondence: Duanling Li, liduanlini@163.com