- 1Escuela Superior Politécnica del Litoral (ESPOL), Facultad de Ingeniería Marítima y Ciencias del Mar, Polytechnic University, Guayaquil, Ecuador
- 2Facultad de Ciencias Naturales, Universidad de Guayaquil, Guayaquil, Ecuador
- 3Subsecretaría de Calidad e Inocuidad, Ministerio de Producción, Guayaquil, Ecuador
- 4School of Ocean and Earth Science, University of Southampton, Southampton, United Kingdom
The Gulf of Guayaquil (GG) is the most important tropical estuarine system of the eastern coast of South America, receiving an average water flow of about 1 650 m3 s-1 from a river basin of approximately 33 700 km2. The city of Guayaquil surrounds the inner coastal lagoon of the Estero Salado (ES) that empties into the GG. This coastal lagoon is of high social, food production, and environmental importance for the city and the GG. However, there is limited high quality data on metal pollution in this zone, no recent information on Hg, and the extent to which sediment metal pollution extends into the GG is presently unknown. As, Cd, Pb, and Hg were analysed in surface sediments from the urban zone and gave average concentrations of 32.3, 2.08, 41.9, and 0.12 mg kg-1 (dry weight), respectively. Additionally, data were obtained for the first time for the El Morro Channel, south of the ES in the GG, which is expected to be a relatively pristine zone; average As, Cd, Pb and Hg concentrations were 6.6, 0.22, 7.9 and 0.02 mg kg-1 (dry weight), well below concentrations seen in the urban ES zone. Estimates of the geo-accumulation index for metal pollution, using the El Morro data as background values, were 1.7 (As), 2.7 (Cd), 1.8 (Pb) and 2.0 (Hg), making the ES class II and a moderately polluted estuary for As, Hg and Pb, but class III and “moderately to heavily polluted” for Cd. If the lowest concentrations of the EM samples are taken the ES is class III for As, IV for Hg and Pb, and V for Cd; id est, the ES would classify as a heavily to extremely polluted estuary regarding these metals. These data show the metal concentrations increase significantly as the main conurbation of Guayaquil is approached from offshore, indicating a strong anthropogenic source of metals from the city, with anticipated negative environmental impacts.
Introduction
There is an extensive scientific literature that shows the importance of estuaries and near-shore waters in providing natural resources, with associated significant impacts on local economies, and ecosystems (e.g., Barbier et al., 2011; Birch, 2017). Estuarine environments nurture large numbers of species at all life stages, and help to sustain and protect them from predators (Dantas et al., 2016). Additionally, estuaries sustain environmental conditions in adjacent coastal waters (Thrush et al., 2013). Nonetheless, these ecosystem services have generally been undetermined (Pinto et al., 2010), and their benefits are not understood or used properly. Lack of planning and infrastructure investment can also lead to pollution and ecosystem damage (Chapman and Wang, 2001; Wang et al., 2014; Yi et al., 2021). Estuaries also sustain a variety of industries, ranging from aquaculture, fisheries, commerce and recreation to port infra-structure (e.g., Niemi et al., 2004; Oi et al., 2011; Yi et al., 2021). Wang et al. (2014) reported that Chinese estuaries had been damaged by both direct and indirect inputs of untreated or partially treated industrial and domestic waste water. Such pollution inputs can negatively impact flora and fauna in pelagic systems as well as migratory species (Birch and Hutson, 2009; Birch et al., 2013). It is well known that the health of residents adjacent to urbanized estuaries and water bodies can be impacted by metal pollution, e.g., Meneses et al. (2022) has reported impacts from Hg contamination.
Particles that form sediments are transported into estuarine and near shore waters through erosion of upstream deposits (Birch et al., 2013; Burton, 2013), surface run off from cities, agriculture, and aquaculture activities. The sediments in either completely or partially urbanized estuaries are effectively a filtering ecosystem for waters going into coastal areas (Larrose et al., 2010; Martin et al., 2012), and may be both sinks and sources of metals from land activities (Barletta et al., 2019; Yi et al., 2021). The inputs of a wide range of anthropogenic chemical elements and compounds have been increasing over recent decades in estuaries (Fan et al., 2020), and their biogeochemistry will change in response to variations in, e.g., dissolved oxygen, redox potential, and pH.
Heavy metals such as Hg, Pb, Cd and the metalloid As, are not required for metabolic processes and so the risk of toxicity is higher than those used in life processes (US EPA, 1999). All these metals are toxic to organisms at elevated concentrations, and are characterized by long residence times, and being bioaccumulated through the food chain (Zhang et al., 2009; Zhao et al., 2019; Ormaza-González et al., 2020). The As is inherent part of agricultural (pesticides), metallurgy, medicine, electronics, etc. industries (e.g., Cheng et al., 2019; Liu et al., 2022) and it is becoming a concern for human health (Zheng et al., 2020) since few years; chronic exposure could lead to risky hearth, ling, kidney ailments as it goes through the food trophic chain (see, Rehman et al., 2021). Because of this potential toxicity they are often studied in estuarine sediments (e.g. Wang et al., 2022), and are under constant scrutiny due to the well-known potentially toxic impact on fauna and flora, as well as on the health of people consuming these organisms (Järup, 2003; Worakhunpiset, 2018; Kolarova and Napiórkowski, 2021). Navarrete-Forero et al. (2019) have reported Hg concentrations in black clams (Anadara spp) and red crabs (Ucides occidentalis) from the Gulf of Guayaquil, that are >1000 times the safe limit for human consumption. Sites included near-shore environments close to or within urban catchments (Martínez and Poleto, 2014), and artisanal gold mining sites (Acquavita et al., 2021).
The Estero Salado (ES) is part of the tropical estuarine system of the Gulf of Guayaquil, which is the largest estuary in the eastern Pacific (Stevenson, 1981; Jiménez, 1983; Montaño-Armijos and Montolío, 2008; Delgado, 2013; Navarrete-Forero et al., 2019; Ormaza-González and Martillo-Bustamante, 2021). It extends over 13 701 km2, of which 11 711 km2 is water and the rest are islands and islets (Stevenson, 1981; CAAM, 1996), and nestled within it is the City of Guayaquil. Over the last 60 years, the ES has accumulated sediments and become heavily contaminated (pesticides, heavy metals, hydrocarbons, oils, fats, pharmaceuticals, etc.), resulting in local fisheries within the urbanized zone disappearing. Ayarza et al. (1993) reported that as early as 1985 most of the commercial species had declined. Recently, Ormaza-González et al. (2022, 2024) have reported the ES is in perennial anoxia, overloaded with fecal coliforms, and is hyper eutrophic, due mainly to unregulated urbanization (Arroyo et al., 2015) and unplanned and unmonitored industrial development (Chalén-Medina et al., 2017) with more than 190 industries discharging their liquid and solid residues (Ministerio del Ambiente, 2012) into the ES. Understanding the impact of metals on mangroves is also required (Kulkarni et al., 2018) as the ES is basically a mangrove system on which important local fisheries have depended in the past, including the red crab (Ucides occidentalis), black-shell clams (Anadara tuberculosa, Anadara similis), black mussel (Mytella strigata), cat fish (Ariopsis guatemalensis), and chame (Dormitator latifrons). Amongst the pollutants present, an improved knowledge of heavy metals in sediments is required.
To assess what sediment metal data may already be available for the ES, an extensive on line and in situ (institutional libraries) literature research was carried out which resulted in 6 820 results. However, there is no peer-reviewed work reported before 2010, although works reviewed within parent Institutes from Arriaga (1976), and Solórzano and Viteri (1993) exist, and Ayarza et al. (1993), gave information from a survey carried out in 1985 and can be considered the first work to provide some data on metals. Ayarza et al. (1993) reported Cd and Hg concentrations at sampling stations close to those used the present work, of up to 0.01 mg kg-1 for Cd and 1.13 to 2.98 mg kg-1 for Hg and these values can be considered the first reported in sediments and fauna for the ES. Post 2010 reports and BSc theses, e.g., Alcívar-Tenorio et al. (2011); Jiménez (2012); Chalén-Medina et al. (2017); Pernía et al. (2018) and Navarrete-Forero et al. (2019), do show high metal concentrations, but varied techniques were applied with very limited quality control and so their accuracy remains unclear. Careful work by Fernández-Cadena et al. (2014), indicated that the surface sediments of the central city part of the Estero Salado are polluted with potentially toxic metals.
Here we provide new high-quality data on the metals Pb, Cd, As, and Hg (not measured by Fernández-Cadena et al., 2014), in sediments from a wider range of sites around the city, as well as in the most southern zone of the ES, the El Morro (EM) Channel. This latter zone includes a wildlife refuge and is expected to be much less polluted than within the city, and the metal data given here are the first for this area. These new data are used to help assess the level of pollution in the city zone and thus provide the evidential background needed to stimulate improved environmental management of this major coastal population centre of the eastern tropical Pacific Ocean.
Materials and methods
Study area
The geographical limits of the ES have not officially been defined, but many authors (e.g., Stevenson (1981); Cruz (1992); Peñafiel et al. (2017)) have proposed, that its boundaries are from the core of the city (Figure 1A) to the southwest as far as the Canal del Morro (hereafter El Morro (EM), Figure 1B). In the present work the ES water body is considered to extend from 2°8.97’ S, 79°54.33’W in the north, to the El Morro channel in the south (2°39.5’S, 80°10.5’W); i.e., about 49km. The Estero Salado has no important natural input of fresh water, and for that reason it is a salty ecosystem (hence the name: “Salado” which in Spanish means “salty”). Rain and legal/illegal discharges of industrial and domestic waters are the only source of freshwater. Average annual rainfall (1961-2017) in Guayaquil is 1755 mm (http://hikersbay.com/climate-conditions/ecuador/guayaquil/), whilst Morales-Estupiñan et al. (2020), reported around 1000 mm. Whatever the average, 60-70% of this rain occurs in January to April. It is possible that groundwater inputs may add to freshwater inflow but no information on this potential input could be found. The water within the ES is primarily replenished by large semidiurnal tidal cycles of up to 4 m in height (Ormaza-González et al., 2022); the ES and Gulf of Guayaquil has the largest tidal regime in the eastern south Pacific (Reynaud et al., 2018). The estimated residence time of water in the Gulf of Guayaquil is over 21 days (Stevenson, 1981), but for the ES this should be much longer. Here we consider the ES as a coastal lagoon (Pérez-Ruzafa et al., 2011; Marques-Figueiredo and Rockwell, 2022) as its natural fresh water input is only that from the city, and water turnover is primarily tidally driven.
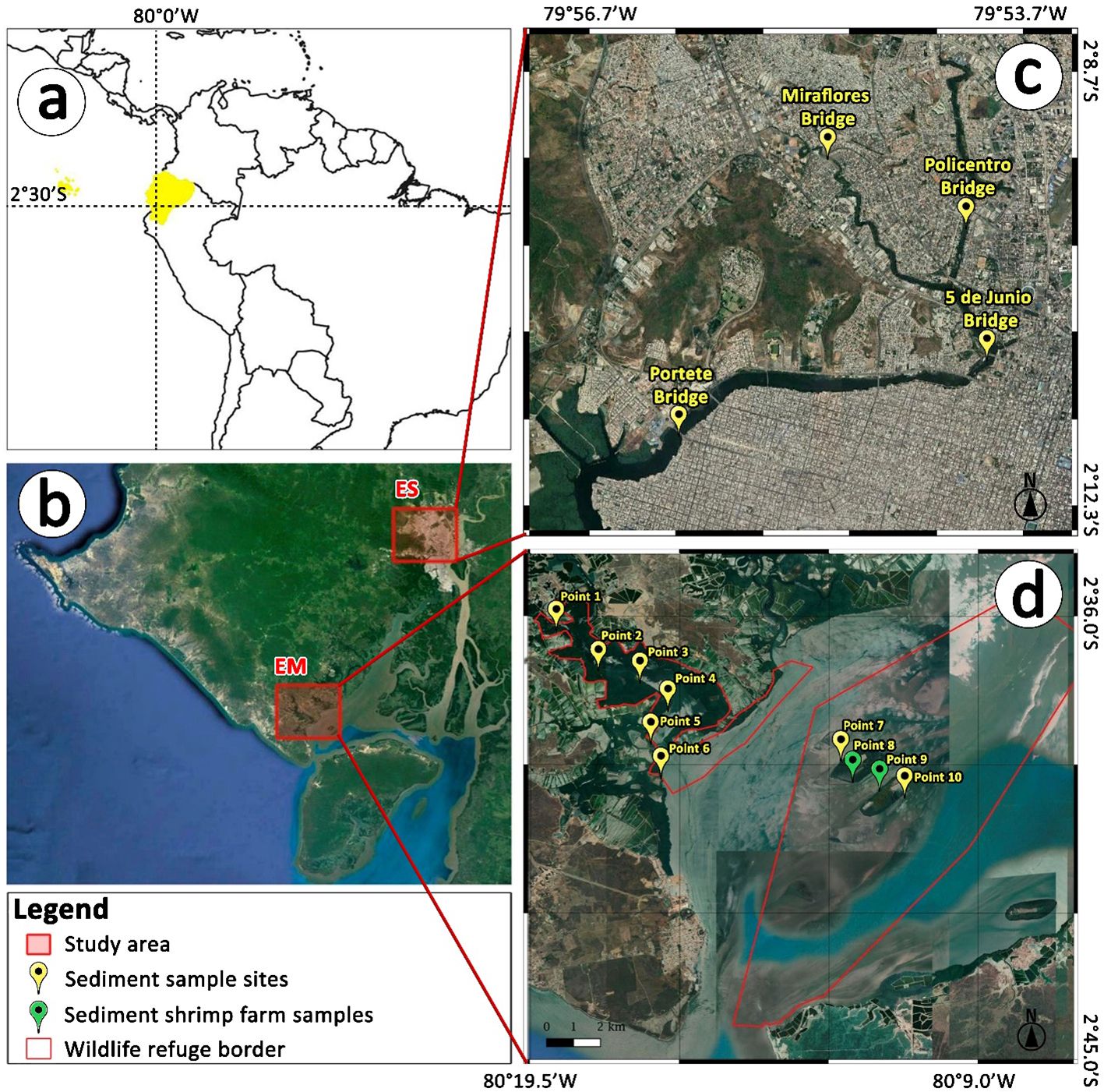
Figure 1. Ecuador (A). The Gulf of Guayaquil, the Estero Salado (ES) and El Morro (EM) shown in panel (B). Sampling sites in the urbanized Estero Salado (yellow dots panel (C)) and El Morro sites (D). Exact locations of sampling sites for the current work are given in Table 1. Figure modified from Google Earth maps.
Major factors leading to the general pollution of the ES are 1) Guayaquil is the economic center of Ecuador (Delgado, 2013) with major growth of a wide range of industries and a large population (2.7 million inhabitants, INEC, 2022). 2) the southern part of the Estero Salado is the navigation channel to the port of Guayaquil (Figure 1), which is the largest in the country and the second busiest container port in the west coast of South America (Wilmsmeier et al., 2021). 3) The generally unplanned growth in population settlements (legal or illegal) has led to overload and collapse of sewage systems, wastewater treatment and disposal of solid waste. Cimentaciones (2000) found that 60% of the discharges to the estuary were domestic and the remaining 40% of industrial origin, and all types of wastewaters have been dumped into the ES without any or only minimal treatment. The city of Guayaquil has >190 registered industries (beverages, food, glass, car batteries, workshops, metallurgy, plastic, textile production, agriculture, etc., MAE, 2023) of which many are sources of polluting agents (Fernández-Cadena et al., 2014). According to the Ministry of Environment (MAE, 2023) only 54 comply with Ecuadorian environmental norms.
Sampling
Figure 1 shows the sampling sites used here, where the four sites within the narrow and urbanized ES (Figure 1C) give a good coverage of the city. Ten sediments samples were taken from the Canal El Morro to the south (Table 1; Figure 1D), including two from active shrimp ponds and mangrove banks. Samples from El Morro are generally considered reference points because of their distance from known contamination sources and they are within a protected wildlife refuge zone. No metal analyses have been reported for sediments from this area.
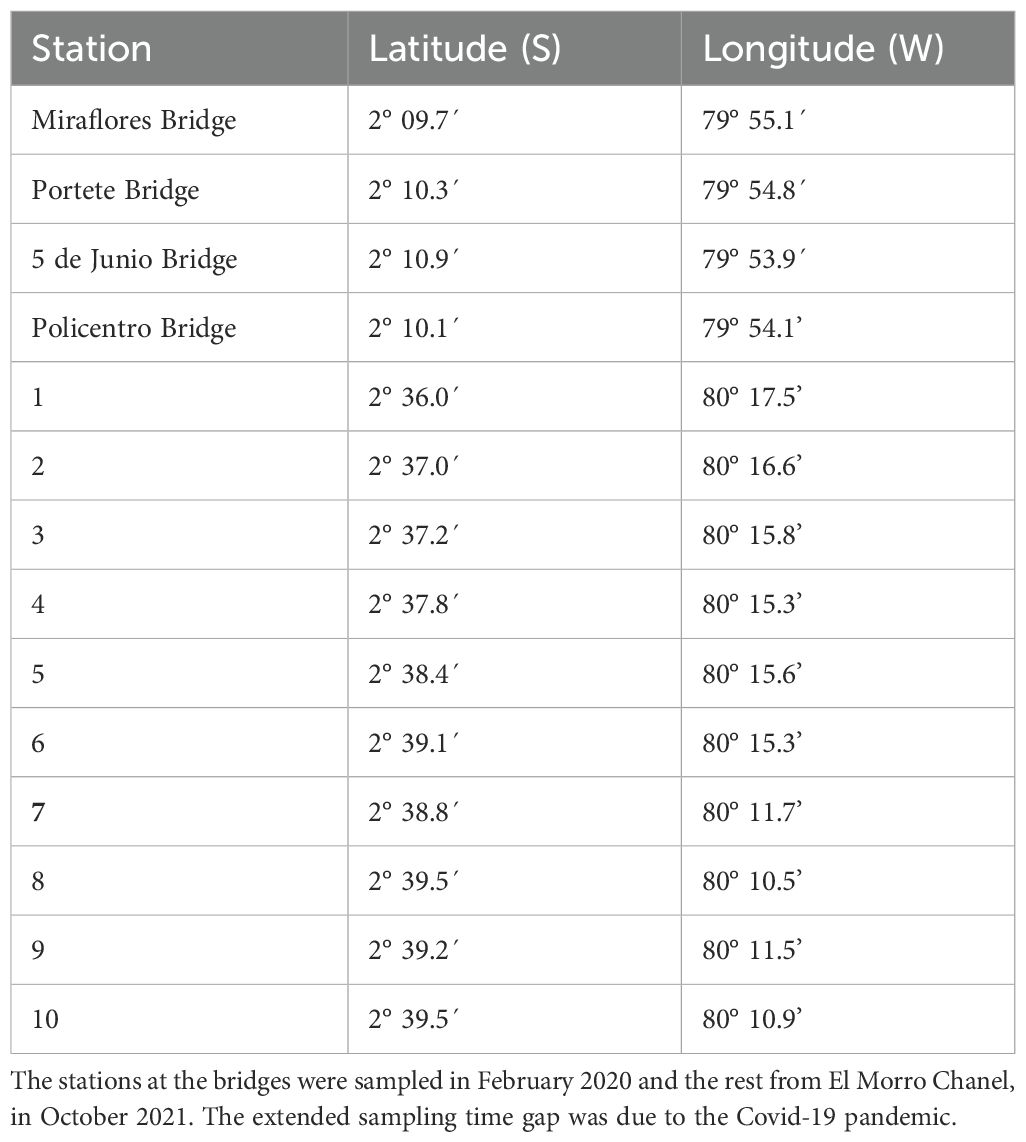
Table 1. Geographical positions of the stations in Figure 1.
Surface sediments (depth 10-15 cm) were collected using a hand-operated portable stainless-steel grab (Van Veen, 250 cm2). These surface sediments were kept in clear low-density polyethylene bags (zipper-top). Then, samples were dried at ~35°C for 72 hours and finely ground (see, Skilbeck et al., 2017).
Metal analysis
For the determination of total Hg concentrations, around 0.25 (± 0.0001) g dry sediment was microwave digested in 10 ml of 65% nitric acid using a Mars Xpress™ system (CEM). After that, samples were diluted into 100 ml with acid solution (HNO3 and H2SO4, 65% and 95-97% strength respectively. Stannous chloride solution was used as the reductant. Cold vapor atomic absorption spectroscopy, with a Varian SpectrAA (model 220FS) coupled to a VGA 77 vapor generation was used. The laboratory is ISO/IEC 17025 certified.
Sample analysis for total Pb, Cd and As in sediment consisted of weighing accurately about 0.30 g dry sample into Teflon vessels, 6 ml of 65% nitric acid were added and samples digested in a microwave system (Mars Xpress™, CEM), samples were diluted to a final volume of 25 ml with ultrapure water. The final solutions were filtered through cellulose filter (Macherey Nagel, 4-12 µm pore size) pre-washed with 1% nitric acid solution. Atomic absorption spectrophotometry by graphite furnace (GFAAS) Varian (model SpectrAA 220Z) and Agilent (model 280Z) with Zeeman background correction were used. Analytical grade reagents were employed throughout analytical procedures.
Calibrations were run for each set of analyses. Hg calibration plot (0.2, 1, 2, 3, 5 and 7 μg [Hg] dm-3), Cd calibration plot (0.2, 0.4, 0.8, 1.6 and 3.2 µg [Cd]. dm-3), Pb calibration plot (1, 5, 9, 14 and 18 μg [Pb] dm-3) and the As calibration plot (10, 20, 30, 40, 50 μg [As] dm-3). Analyte range using cold vapor for Hg is 0.069 to 2.85 mg kg−1, and using GFAAS for Pb, 0.035 to 1.50 mg kg−1: As, 0.20 to 2.80 mg kg−1 and Cd, 0.014 to 2.12 mg kg−1.
Manufacturer’s recommended wavelengths and calibration ranges were used. Reagent blanks and certified reference materials BCR-277R estuarine sediment (CRM-JRC) for Cd, Hg, As and fresh water sediment 016 (Sigma-Aldrich) for Pb were used. Limits of quantification and detection limits are shown in Table 2, and recoveries for CRMs are shown in Table 3. Blanks were run for all sets of analyses.
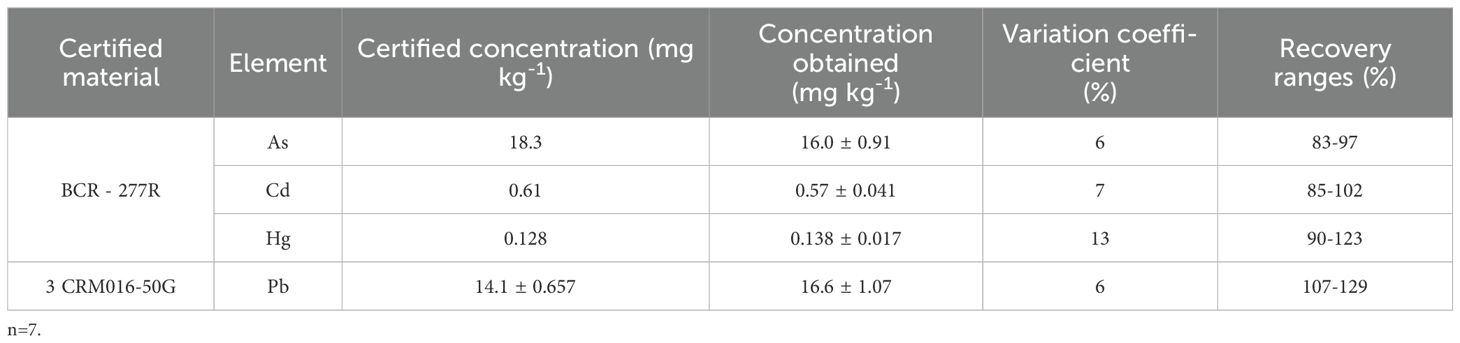
Table 3. Trace elements concentration determined in certified reference materials (estuarine sediment BCR – 277R (https://crm.irmm.jrc.ec.europa.eu/p/40455/40459/By-material-matrix/Soils-sludges-sediment-dust/BCR-277R-ESTUARINE-SEDIMENT/BCR-277R) and fresh water sediment 3 CRM016 [Trace Metals - Fresh Water Sediment 3 certified reference material, pkg of 50 gSigma-Aldrich (sigmaaldrich.com)].
The enrichment factor and the geo-accumulation index
An indication of relative contamination can be obtained from the Enrichment Factor (EF, Li et al., 2014, Equation 1) and Geo-Accumulation Index (Igeo, Zhang et al., 2017, Equation 2) which are amply used since the 60s by European metal studies in urban sediments (Martínez and Poleto, 2014; Barbieri, 2016). Here the average of these metal concentration from the EM is used as the Reference metal.
Where Ci is the actual concentration of the heavy metal i, and Bi is the geological background or the lowest concentration of the heavy metal i. The constant factor of 1.5 is used to account for variation in background values for metals (Muller, 1969: Zhang et al., 2017). For Geo-accumulation Igeo index, class, and classification for metal in estuarine sediments. see Barbieri (2016) and Zhang et al. (2017).
Metal bioavailability
The Reference index ISQG (see, Sediment Quality Guidelines (SQGs): A Review and Their Use in Practice | Geoengineer.org) were used. The concentration is normalized against the empirical threshold level; <1 the adverse effect is very low, whilst >1 would mean metal bioavailability (Krampah et al., 2019). Values for Threshold Effect Levels are: 0.6, 35, 0.17 and 7.3 for Cd, Pb, Hg and As mg kg-1 respectively.
Environmental quality standard – Ecuador
Ecuador has an Environmental quality standard (see TULAS, 2022 or MAE, 2023), which gives upper accepted concentrations of a healthy estuarine sediment.
Sediment grain size
Sediment grain size was measured because of its reported correspondence with trace metal concentrations (e.g. Martincic et al., 1990, Özşeker et al., 2022). Here, size ranges were determined using analytical certified sieves ASTM- E11 (ISO 3310/BS 410), and following recommendations in Blott and Pye (2012).
Results and discussion
Metals in surface sediments
Estero Salado
The present work (Table 4) found averages of 32.3, 0.12, 2.08, and 41.9 mg kg-1 for As, Hg, Cd and Pb respectively. The Canadian International Sediment Quality Guidelines (ISQG) use a strong acid/peroxide leach to determine metal concentrations, and thus their values provide a useful comparator for ES samples here. Average ratios of ES metal concentrations to ISQG values were 4.4, 0.7, 3.5 and 1.2 for As, Hg, Cd and Pb respectively, indicating elevated metal concentrations at the ES bridge sites in the order As>Cd>Pb>Hg. Of particular note is the extremely high concentration of Cd (6.08 mg kg-1) and ISQG ratio of 10 at the Miraflores Bridge station, and a high concentration of As (36.7 mg kg-1), also at this site suggesting a nearby source. Hg and Pb are similar to the ISQG reference values.
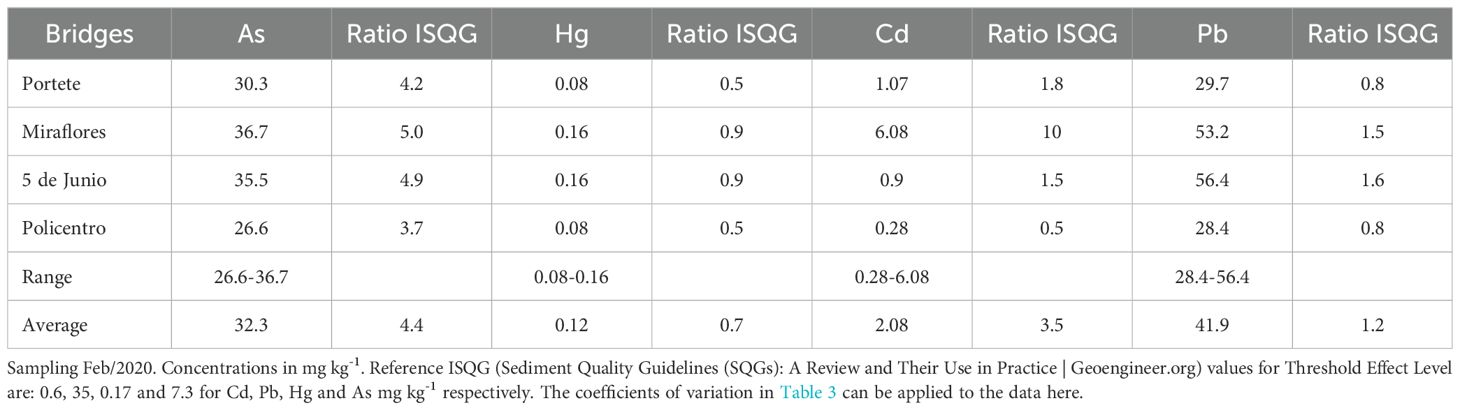
Table 4. Sediment metal measurements at the ES bridges (see Figure 1).
The Miraflores and 5 de Junio bridges have highest metal concentrations, with the Policentro and Portete Bridges being lower. Even though the four sampling sites are relatively close, the Miraflores and 5 Junio bridges are closest to an industrial and urban area of Guayaquil city, where waste inputs since the 1960s have had minimal treatment. Around these two bridges water circulation is restricted, and water volumes small, and so higher levels of metals in sediments might be expected, and water quality generally to be poorer. Recently Ormaza-González et al. (2024) found hyper-eutrophicated and anoxic conditions here. The Portete and Kennedy bridges have a stronger water circulation and greater volume.
The metal measurements reported here generally agree with the careful measurements of Fernández-Cadena et al. (2014) in showing high concentrations of As, Cd and Pb (no Hg measurements reported) around the Miraflores and 5 Junio bridges part of the ES.
In a more regional context, at a pristine estuary (north of Ecuador), which is within a mangrove natural reserve, the Cd sediment concentrations were very high (2.14 mg kg-1) whilst Pb was a low 12.4 mg kg-1 (Romero-Estévez et al., 2020). The high and low concentrations of Cd and Pb respectively are difficult to explain in terms of evident anthropogenic inputs as there is no important population settlement, or any industrial activity close by, as is the case of the ES, and details of the drainage basin are not given. Additional natural and anthropogenic non-point or diffuse inputs (Abessa et al., 2018; Spencer, 2017) including from the atmosphere (e.g., Muñoz and Salamanca, 2003) and groundwater (Li et al., 2024) may have impacted these values, as well as analytical procedures.
The earliest measurements of metals in surface sediments of the ES were in 1985 in the same areas (Figure 1) as examined here by Ayarza et al. (1993). Using similar techniques to those used here, they found Cd and Hg concentration ranges of 0.01-0.03 and 0.1-2.98 mg kg-1 respectively. Cd concentrations were 20-250 times lower than those reported at that time in some other estuaries and coasts (e.g., Katz and Kaplan, 1981) whilst Hg was higher. In relation to 1985 data, Cd has notably increased (138 times), while Hg shows an important decrease (24 times).The most likely reasons for differences in Cd between then and now is the substantial industrial development in Guayaquil with associated discharges. The lower mercury values may reflect a reduction in alluvial gold extraction (Mestanza-Ramón et al., 2021), and the lower quality gasoline and diesel (Won et al., 2007; Jiang et al., 2014) used at that time. Differences in analytical procedures and sampling could also play a role in the variations found, but the data suggests substantial changes in concentrations of Cd and Hg.
El Morro
The sampling sites included two active aquaculture shrimp ponds and the surrounding banks which are fully covered by mangrove. El Morro and part of the surrounding area is a mangrove reserve (Figure 1). Table 5 gives concentration data for all El Morro samples with ISQG ratios, and Figure 2 shows the geographical pattern of metal distributions. There is no previous work in the El Morro channel with which to compare the data shown here.
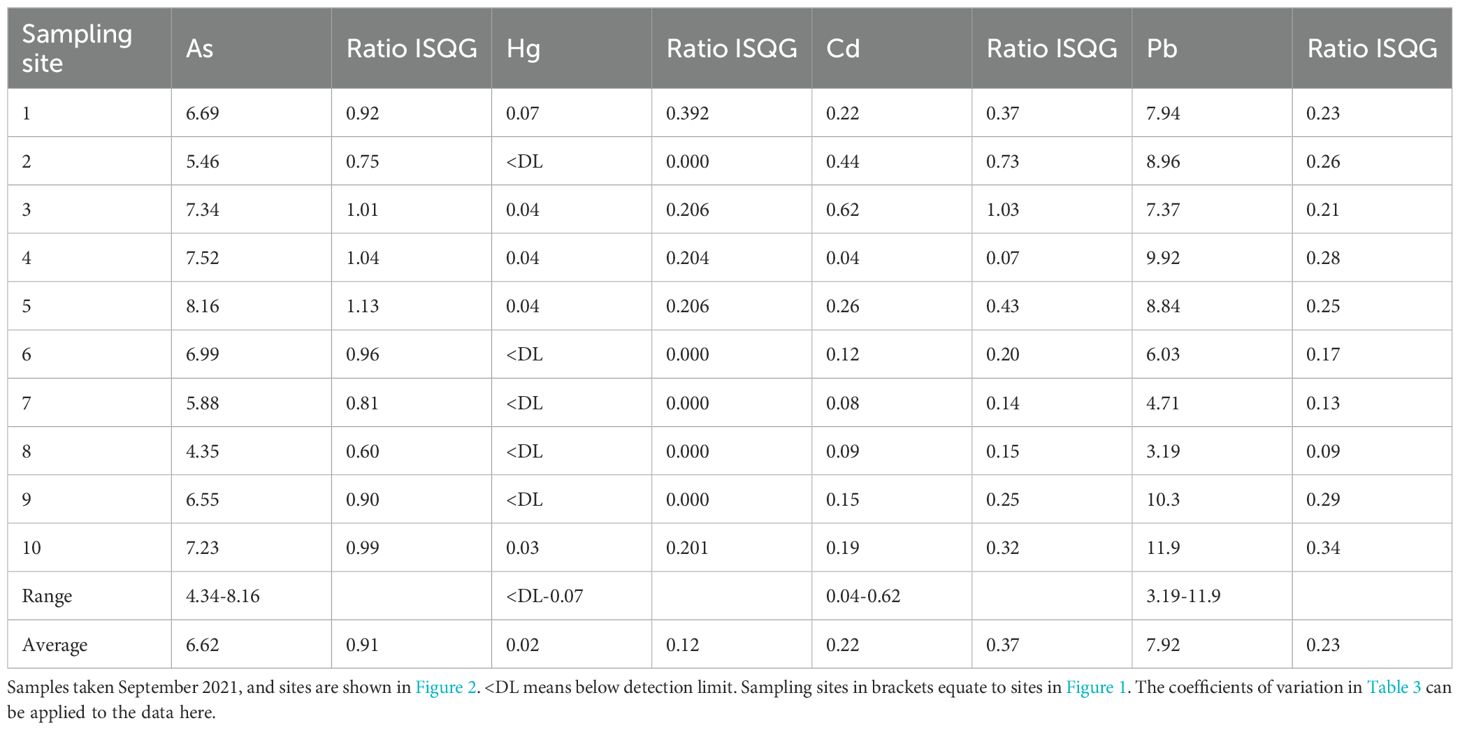
Table 5. Concentration (mg kg-1) of metals in surface sediment of El Morro channel (1-7), shrimp (9-10) pond and mangrove sites (7-8).
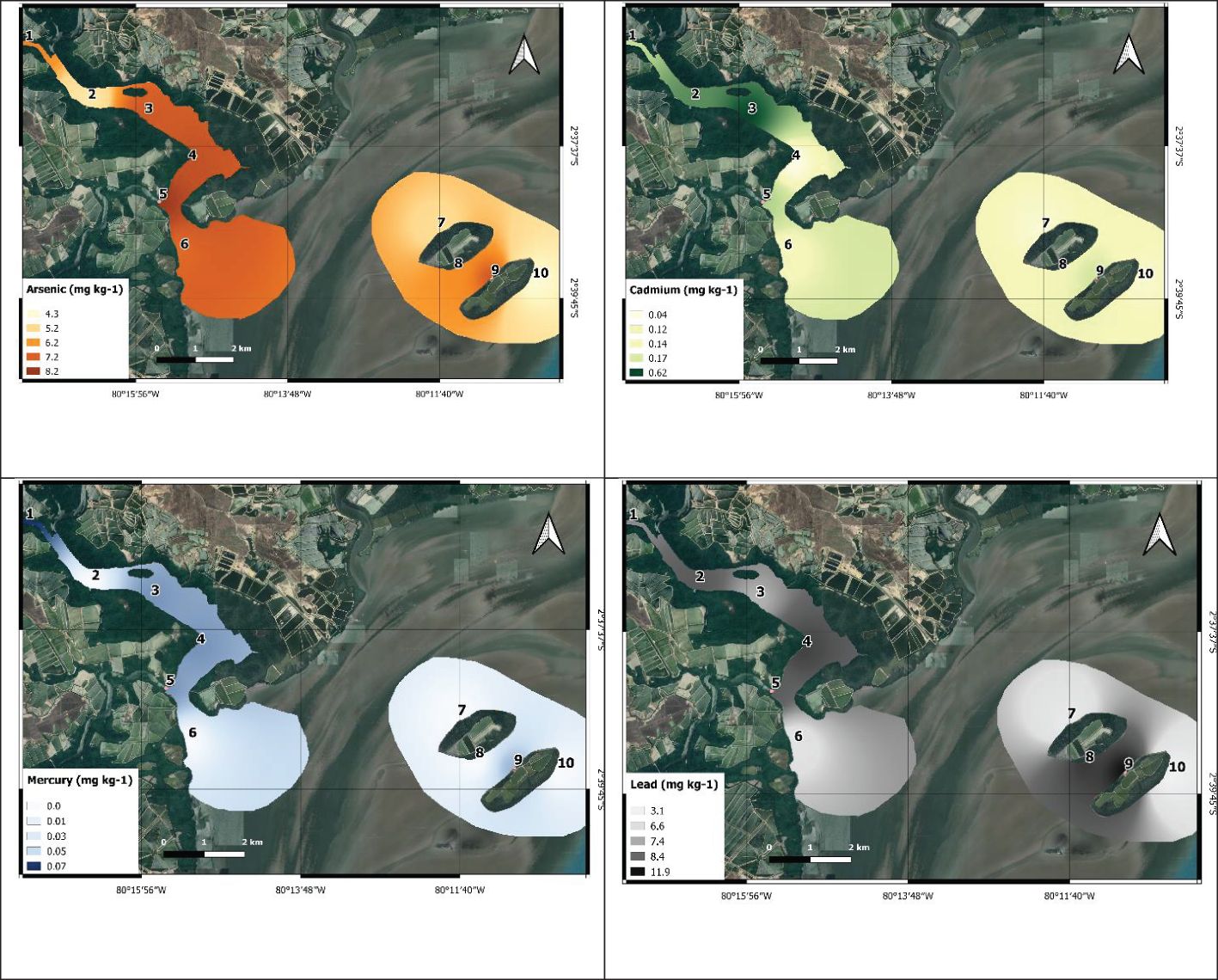
Figure 2. Total As, Cd, Hg and Pb surface distribution in the El Morro Channel. Sampling Oct 2021. Concentration in mg kg-1. Exact positions for the Stations are given in Table 1.
The average values of ratios against ISQG limits are for As, Hg, Cd and Pb respectively, 0.91, 0.12, 0.37 and 0.23. The shrimp ponds ratios were similar or even lower (0.9, 0.16, 0.34 and 0.28 in the same order), and the samples from the mangrove area ISQG ratios were correspondingly even lower, 0.71, 0.0, 0.14 and 0.11. These low values support the view of the EM zone being a relatively metal uncontaminated area.
Grain size and organic carbon content of sediments
The sediments from the ES are mainly sandy silts (see data and curves in SM). The silt fraction is highest at the Miraflores site, whilst the silt is less significant in the other sites. Ayarza et al. (1993) reported that sediments from the ES are mainly fine sand, silt-clay. whilst in the data reported here, they are slightly coarser. Miraflores has more fine fractions than the other sites. Overall, the grain size distribution is quite similar between the ES and EM sites, which is not surprising given the similar Guayas drainage basin source.
The Organic Carbon (OC) content of the ES sediments at the Bridge stations 5 de Junio, Miraflores, Portete (Ayarza et al., 1993); and at the EM stations (Bobadilla-Cordova, 2024) are given in Table 6. Stations closest to the town of El Morro (1-3, Figure 1), have the highest OC content (5.1-6.3%) of the EM stations, which is anticipated as the town has no sewage treatment prior to discharge. Towards the mouth of EM, concentrations were down to 0.7-1.8%, and even lower in the mangrove (<0.3%). At the shrimp farm OC was 0.9-2.0%. Waters at the ES Miraflores bridge site have recently been reported as hyper-eutrophication, frequently anoxic and with low pH (Ormaza-González et al., 2024), and therefore high OC in sediments here is expected. This site also has elevated Cd, Hg and Pb as well as finer sediments, associations that have been found in earlier studies (e.g. Özşeker et al., 2022).
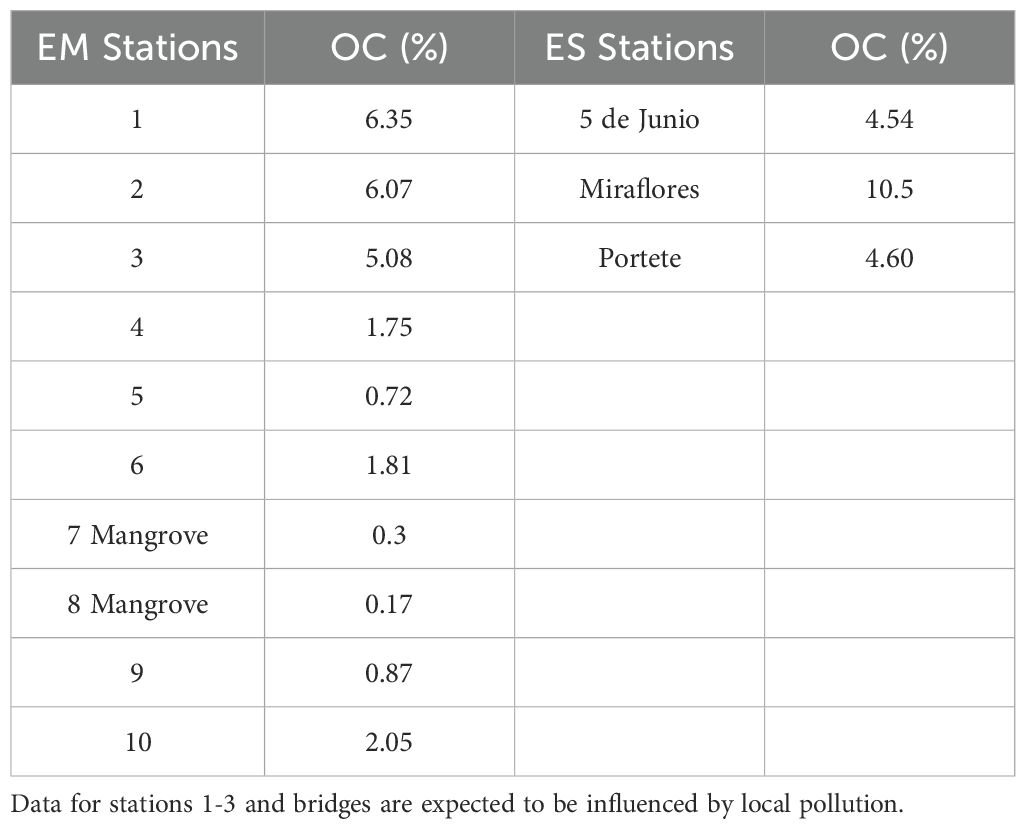
Table 6. Organic carbon content of sediment samples from ES bridge stations (Ayarza et al., 1993) and EM (Bobadilla-Cordova, 2024) sites.
Estimates of metal pollution
Obtaining a measure of pollutant metal content of a sediment relative to background values is very important in assessing potential biological impact (Barbieri, 2016), and assigning a level of pollution to a site. An indication of the magnitude of metal contamination can be obtained using Enrichment Factors (EF) relative to a reference element (RE), but this cannot be applied here as no RE measurements were made. However, if the average values from EM are assumed to be the original concentrations of ES, the average EF for As, Hg, Cd and Pb would be 4.88, 6.00, 9.45, and 5.18 respectively. The four elements are in general enriched at least 5 times, which categorize the EM as extremely enriched according to Li et al. (2014).
Geo-accumulation index
This index is frequently used (Birch, 2017; Barbieri, 2016) and provides a classification of sediment pollution, where the index varies from 0 to >5 with level of pollution increasing with value.
In the original work of Muller (1969) it is reported (Barbieri, 2016; Birch, 2017) that sieved sediment (<2µm) was used and a total measure of metal obtained, and compared to global shale values. Since this pioneering work there have been a multitude of variations used in determining Igeo (Birch, 2017; Barbieri, 2016). Here we do have metal data from a polluted zone (ES surrounded by City), and background sites (El Morro), and thus an estimate of Igeo can be obtained using the ratio-to-reference (RTR) approach (Birch, 2017), or the so-called direct method (Yan et al., 2020), using metal concentrations from a nearby pristine area.
For this Igeo approach to be used, it is important to show that the two sites are not biased by particle size differences. It has been long established that generally finer grained components in sediments contain higher concentrations of metals than coarser fractions as a result of increased surface to volume ratio, and the presence of more adsorptive and absorptive phases (e.g. Martincic et al., 1990; Özşeker et al., 2022). Results of grain-size analysis of samples are shown in Supplementary Material. The sediment grain sizes are similar in both sites, thus making general comparisons between sites in terms of metal concentrations valid and allowing identification of polluted zones easier. Also, it can be assumed that the geological origin of the sediments in the El Morro channel are the same as the urbanized ES, as the whole ES is within the same geological basin of the Gulf of Guayaquil (Ormaza-González and Martillo-Bustamante, 2021), and the origin of the sediments in this coastal lagoon includes the outer Gulf of Guayaquil (Benites, 1975; Barrera Crespo et al., 2019). Therefore, the El Morro Channel and its vicinity can be considered as a suitable site for reference values for sediments closer to anthropogenic sources. Simple ratios of metal concentrations in the urbanized ES to those in El Morro show significant positive values of 4.88 (As), 5.84 (Hg), 9.42 (Cd) and 5.30 (Pb), indicating the ES is highly polluted by these metals.
The geological background Bi used in Igeo calculations was the average values of the 10 samples of the El Morro channel (Table 5), from which the Igeo indexes obtained were 1.7, 1.96, 2.65, and 1.82 for As, Hg, Cd and Pb respectively. Using these values and the categorization in Zhang et al. (2017) the urban ES is class II and moderately polluted zone for As, Hg and Pb, but class III and “moderately to heavily polluted” regarding Cd. However, if we take the lowest Bi of the EM samples (excluding the first 3 values close to the town and its pollution source), the ES is class III for As, IV for Hg and Pb, and V for Cd. These indexes would mainly classify the ES as a heavily to extremely polluted estuary regarding these metals.
Conclusions
The concentrations of As, Hg, Cd and Pb in sediments of the urban ES are high, and when compared to the Canadian interim sediment quality guidelines (ISQG), all except Hg have values greater than 1 indicating contamination and potential for impacts on the environment and its biota. Of particular note is Cd at Miraflores Bridge with an ISQG value of 10. The EF results classify the ES as extremely enriched and above upper limits of National standards. Using the Igeo index approach as applied here, the ES is class II and a moderately polluted estuary for As, Hg and Pb, but class III and “moderately to heavily polluted” for Cd. These approaches therefore overall classify the urbanized ES as a polluted lagoon system for these metals, in agreement for As, Cd and Pb with Fernández-Cadena et al. (2014). The work here shows the ES system is also contaminated with Hg.
High As, Hg, Cd and Pb concentrations lead to concerns over bioaccumulation of metals in benthic organisms with associated reduction in biodiversity and possible toxicity to humans where such species are consumed. In particular demersal and benthic seafood species such as the clam (Anadara spp.) and red crab (Ucides occidentalis) appear to bioaccumulate these metals. The fauna in the upper ES has practically disappeared.
The limited past sediment metal data for the ES from Ayarza et al. (1993), indicate an increase in Cd and decrease in Hg contamination over the past 3 decades in this zone because of changes in industrial activity and, e.g., alluvial gold extraction and gasoline contamination, respectively.
To the south of the ES in the El Morro channel region, the concentrations of the above sediment metals decrease relative to the polluted ES and give ISQG values below 1.0. Even lower ISQG values are found for the mangrove areas. The EM region can therefore be regarded as an overall non-polluted zone for the studied sediment metals.
Whilst the present and some other work indicate significant metal contamination in the ES, further research is needed on their sources, impacts on biota and the local population. Work is also needed on potential remediation methods that have been applied to temperate but not yet tropical estuaries (Oliveira et al., 2024; Patmont and Healy, 2024). The Estero Salado and the less contaminated El Morro zones fall under objectives in the UN initiative launched in 2019 (“Decade on Ecosystem Restoration”) that provides a framework for Sustainable Development.
Data availability statement
The original contributions presented in the study are included in the article/Supplementary Material. Further inquiries can be directed to the corresponding author.
Author contributions
FO: Conceptualization, Formal analysis, Investigation, Validation, Writing – original draft, Writing – review & editing. RC: Formal analysis, Methodology, Validation, Writing – review & editing. AM: Data curation, Methodology, Software, Writing – review & editing. NB: Data curation, Investigation, Methodology, Resources, Writing – review & editing. IR: Investigation, Resources, Supervision, Writing – review & editing. PS: Investigation, Supervision, Validation, Visualization, Writing – original draft, Writing – review & editing.
Funding
The author(s) declare that no financial support was received for the research, authorship, and/or publication of this article.
Acknowledgments
Many people helped in some way or another to carry out this work. We thank support from the ESPOL, University of Guayaquil and Sub-Secretary of Calidad e Inocuidad. This work was originally presented at the ECSA 58 - EMECS 13 Estuaries and coastal seas in the Anthropocene-. 7-11 September 2021 in Hull, UK. Preprint: https://dx.doi.org/10.2139/ssrn.4265697. Oceanographer Pamela Campi for her work on grain size. We are grateful to the reviewers that provided corrections and ideas that improved the manuscript.
Conflict of interest
The authors declare that the research was conducted in the absence of any commercial or financial relationships that could be construed as a potential conflict of interest.
Publisher’s note
All claims expressed in this article are solely those of the authors and do not necessarily represent those of their affiliated organizations, or those of the publisher, the editors and the reviewers. Any product that may be evaluated in this article, or claim that may be made by its manufacturer, is not guaranteed or endorsed by the publisher.
Supplementary material
The Supplementary Material for this article can be found online at: https://www.frontiersin.org/articles/10.3389/fmars.2024.1457548/full#supplementary-material
References
Abessa D. M. S., Albuquerque H. C., Morais L. G., Araújo G. S., Fonseca T. G., Cruz A. C. F., et al. (2018). Pollution status of marine protected areas worldwide and the consequent toxic effects are unknown. Environ. pollut. 243, 1450–1459. doi: 10.1016/j.envpol.2018.09.129
Acquavita A., Floreani F., Covelli S. (2021). Occurrence and speciation of arsenic and mercury in alluvial and coastal sediments. Curr. Opin. Environ. Sci. Health 22, 100272. doi: 10.1016/j.coesh.2021.100272
Alcívar-Tenorio M., Mosquera-Armijo J., Castro-Rendón R. (2011). Concentración de metales pesados (Cr total, Pb, Cd) en agua superficial y sedimentos en el Estero Salado (Guayaquil). Revista Científica de Ciencias Naturales y Ambientales 1, 28–40. Available online at: https://dialnet.unirioja.es/ejemplar/595596.
Arriaga L. (1976). Contaminación en el Océano Pacífico Sur-oriental (Ecuador-Perú-Chile). Rev. Com. Perm. Pacífico Sur. 5), 3–62. Available online at: https://biblioteca.imarpe.gob.pe/opac.
Arroyo A. V., Cayeiro M. L., Bedoya A. M. (2015). Criterios de zonificación ambiental: estudio de caso estero salado de Guayaquil - Ecuador. Rev. Científica Ecociencia 2. Available at: https://www.semanticscholar.org (Accessed December 8, 2024).
Ayarza W., Coello S., Chalen N., Garcés P., García L., García M. L., et al. (1993). Estudios Geobioquímicos de la sección urbana del Estero Salado. Ecuador. Rev. Cienc. Marinas y Limnología 3, 1–20. Available at: https://www.researchgate.net/publication/263964718_Estudios_Geobioquimicos_de_la_seccion_urbana_del_Estero_Salado_Ecuador. (Accessed March 22, 2022).
Barbier E. B., Hacker S. D., Kennedy C., Koch E. W., Stier A. C., Silliman B. R. (2011). The value of estuarine and coastal ecosystem services. Ecol. Monogr. 81, 169 193. doi: 10.1890/10-1510.1
Barbieri M. (2016). The importance of enrichment factor (EF) and Geoaccumulation index (Igeo) to evaluate the soil contamination. J. Geol Geophys 5, 237. doi: 10.4172/2381-8719.100023
Barletta M., Lima A. R., Costa M. F. (2019). Distribution, sources and consequences of nutrients, persistent organic pollutants, metals and microplastics in South American estuaries. Sci. Total Environ. 651, 1199–1218. doi: 10.1016/j.scitotenv.2018.09.276
Barrera Crespo P. D., Mosselman E., Giardino A., Becker A., Ottevanger W., Nabi M., et al. (2019). Sediment budget analysis of the Guayas River using a process-based model. Hydrol. Earth Syst. Sci. 23, 2763–2778. doi: 10.5194/hess-23-2763-2019
Benites S. (1975). Morfología y sedimentos de la Plataforma Continental. Tesis ESPOL, Guayaquil, Ecuador, 112. Available online at: http://www.dspace.espol.edu.ec/xmlui/handle/123456789/32475. (Accessed December 8, 2024).
Birch G. F. (2017). Determination of sediment metal background concentrations and enrichment in marine environments – A critical review. Sci. Total Environ. 580, 813–831. doi: 10.1016/J.SCITOTENV.2016.12.028
Birch G. F., Chang C.-H., Lee J.-H., Churchill L. J. (2013). The use of vintage surficial sediment data and sedimentary cores to determine past and future trends in estuarine metal contamination (Sydney estuary, Australia). Sci. Total Environ. 454–455, 542–561. doi: 10.1016/j.scitotenv.2013.02.072
Birch G. F., Hutson P. (2009). Use of sediment risk and ecological/conservation value for strategic management of estuarine environments: Sydney estuary, Australia. Environ. Manage. 44, 836–850. doi: 10.1007/s00267-009-9362-0
Blott S. J., Pye K. (2012). Particle size scales and classification of sediment types based on particle size distributions: Review and recommended procedures. Sedimentology 59, 2071–2096. doi: 10.1111/j.1365-3091.2012.01335.x
Bobadilla-Córdova D. N. (2024). Estimación de las reservas de carbono en el suelo en zonas de manglar dentro del Refugio de Vida Silvestre Manglares el Morro. BSc Thesis. Guayaquil-Ecuador: Escuela Superior Politécnica del Litoral. 34. pp. Available online at: https://www.espol.edu.ec/en.
Burton G. A. Jr. (2013). Assessing sediment toxicity: Past, present, and future. Environ. Toxicol. Chem. 32, 1438–1440. doi: 10.1002/etc.2250
CAAM. (1996). Sistemas biofísicos y Pesquerías en el Golfo de Guayaquil. 223 p. Available online at: https://books.google.com.ec/books?id=cCQNAAAAYAAJ (Accessed December 8, 2024).
Chalén-Medina J. A., Flor-Chavez G. F., Fun- Sang K. C. (2017). Study of concentrations by heavy metals and hydrocarbons in branch B of Estero Salado. Pol. Con. (Edición núm. 7) 2. doi: 10.23857/pc.v2i6.153. ISSN: 2550 - 682X.
Chapman P. M., Wang F. (2001). Assessing sediment contamination in estuaries. Environ. Toxicol. Chem. 20, 3–22. doi: 10.1002/etc.2250
Cheng Q., Zhou W., Zhang J., Shi L., Xie Y., Li X. (2019). Spatial variations of arsenic and heavy metal pollutants before and after the water-sediment regulation in the wetland sediments of the yellow river estuary, china. Mar. pollut. Bull. 145, 138–147.
Cimentaciones A. L. (2000). Estudios de Prefactibilidad, Factibilidad, y Selección de la mejor alternativa del Plan Integral de la Recuperación del Estero Salado. Informe final. Available online at: https://www.researchgate.net/publication/256547804_CONSULTORIA_CIMENTACIONES-LAHMEYER-_Estudio_Integral_Estero_Salado.
Cruz M. (1992). Estado actual del recurso malacológico (Bivalvos y Gasterópodos) de la zona infralitoral del Golfo de Guayaquil. Acta Oceanográfica del Pacífico 7, 41–68. Available online at: https://www.inocar.mil.ec/web/index.php/publicaciones/actas-oceanograficas/9-acta-oceanografica-del-pacifico-vol-6-n-1-1992.
Dantas D. V., Barletta M., Costa M. F., Barbosa-Cintra S. C. T., Possatto F. E., Ramos J. A. (2016). Movement patterns of catfishes (Ariidae) in a tropical semi-arid estuary. J. Fish Biol. 76, 2540–2557. doi: 10.1111/j.1095-8649.2010.02646.x
Fan H., Chen S., Li Z., Liu P., Xu C., Yang X. (2020). Assessment of heavy metals in water, sediment, and shellfish organisms in typical areas of the Yangtze River Estuary, China. Mar. pollut. Bull. 151, 110864. doi: 10.1016/j.marpolbul.2019.110864
Fernández-Cadena J. C., Andrade S., Silva-Coello C. L., de la Iglesia R. (2014). Heavy metal concentration in mangrove surface sediments from the north-west coast of South America. Mar. pollut. Bull. 82, 221–226. doi: 10.1016/j.marpolbul.2014.03.016
INEC (2022). (Instituto Nacional de Estadística y Censos). Available online at: https://www.Ecuadorencifras.gob.ec/guayaquil-en-cifras/ (Accessed March 2022).
Järup L. (2003). “Hazards of heavy metal contamination,” in British Medical Bulletin, vol. 68. (British Medical Bulletin), 167–182. doi: 10.1093/bmb/ldg032
Jiang X., Teng A. K., Xu W. Z., Liu X. S. (20142014). Distribution and pollution assessment of heavy metals in surface sediments in the Yellow Sea. Mar. pollut. Bull. 83, 366–375. doi: 10.1016/j.marpolbul.2014.03.020
Jiménez R. (1983). Diatomeas y silicoflagelados del fitoplancton del Golfo de Guayaquil. Acta Oceanográfica del Pacífico 2, 193–281. Available online at: https://www.inocar.mil.ec/web/index.php/publicaciones/actas-oceanograficas/4-acta-oceanografica-del-pacifico-vol-2-n-2-1983.
Jiménez D. (2012).Cuantificación de metales pesados (Cd, Cr, Ni y Pb) en agua superficial, sedimentos y organismos (crassostrea columbiensis) ostión de mangle en el puente Portete del Estero Salado (Guayaquil). Available online at: http://repositorio.ug.edu.ec/bitstream/redug/1683 (Accessed January 2, 2019).
Katz A., Kaplan I. R. (1981). Heavy metals behavior in coastal sediments of southern California: a critical review and synthesis. Mar. Chem. 10, 261–299. doi: 10.1016/0304-4203(81)90010-4
Kolarova N., Napiórkowski P. (2021). Trace elements in aquatic environment. Origin, distribution, assessment and toxicity effect for the aquatic biota. Ecohydrology Hydrobiology., 655–668. doi: 10.1016/j.ecohyd.2021.02.002
Krampah F., Nyarko S. Y., Danlogo K., Sanful P. (2019). Application of pollution indices in the assessment of heavy metal contamination of surface sediments of river bonsa, ghana. J. Geosci. Environ. Prot. 7 (02), 176.
Kulkarni R., Deobagkar D., Zinjarde S. (2018). Metals in mangrove ecosystems and associated biota: A global perspective. Ecotoxicology Environ. Saf. 153, 215–228. doi: 10.1016/j.ecoenv.2018.02.021
Larrose A., Coynel A., Schäfer J., Blanc G., Massé L., Maneux E. (2010). Assessing the current state of the Gironde Estuary by mapping priority contaminant distribution and risk potential in surface sediment. Appl. Geochemistry. 25, 1912–1923. doi: 10.1016/j.apgeochem.2010.10.007
Li Y., Zhang M., Mi W., Ji L., He Q., Xie S., et al. (2024). Spatial distribution of groundwater fluoride and arsenic and its related disease in typical drinking endemic regions. Science of The Total Environment 906, 167716.
Li Y., Zhang H., Chen X., Tu C., Luo Y., Christie P. (2014). Distribution of heavy metals in soils of the Yellow River Delta: concentrations in different soil horizons and source identification. J. Soils Sediments 14, 1158–1168. doi: 10.1007/s11368-014-0861-0
Liu Y., Yu J., Sun H., Li T., He X., Lin Z. (2022). Screening and prioritizing substances in groundwater in the beijing–Tianjin–Hebei region of the north china plain based on exposure and hazard assessments. J. Hazardous Materials , 423, 127142. doi: 10.1016/j.jhazmat.2021.127142
MAE (2023). Listado de industrias con descargas al Estero Salado. Available online at: https://www.ambiente.gob.ec/listado-de-industrias-con-descargas-al-estero-salado/ (accessed January 17, 2023).
Marques-Figueiredo P., Rockwell T. K. (2022). “2.17 - application of coastal landforms to active tectonic studies,” in Treatise on Geomorphology, 2nd ed. Ed. Shroder J. (. F. (Academic Press), 443–476, ISBN: ISBN 9780128182352. doi: 10.1016/B978-0-12-818234-5.00163-2
Martin G. D., George R., Shaiju P., Muraleedharan K. R., Nair S. M., Chandramohanakumar N. (2012). Toxic metals enrichment in the surficial sediments of a eutrophic tropical estuary (Cochin backwaters, southwest coast of India. Sci. World J., 2012 17 pages. doi: 10.1100/2012/972839
Martínez L. L. G., Poleto C. (2014). Assessment of diffuse pollution associated with metals in urban sediments using the geoaccumulation index (Igeo). J. Soils Sediments 14, 1251–1257. doi: 10.1007/s11368-014-0871-y
Martinčić D., Kwokal Ž., Branica M. (1990). Distribution of zinc, lead, cadmium and copper between different size fractions of sediments i. the limski kanal (North adriatic sea). Sci. Total Environ. 95, 201–215.
Meneses H., Oliveira-da-Costa M., Basta P. C., Morais C. G., Pereira R. J. B., de Souza S. M. S., et al. (2022). A growing threat to riverine and urban communities in the Brazilian amazon. Int. J. Environ. Res. Public Health 19, 2816. doi: 10.3390/ijerph19052816
Mestanza-Ramón C., Paz-Mena S., López-Paredes C., Jimenez-Gutierrez M., Herrera-Morales G., D’Orio G., et al. (2021). History, current situation and challenges of gold mining in Ecuador’s Litoral region. Land. 10, 1220. doi: 10.3390/land10111220
Ministerio del Ambiente (2012). Listado de industrias con descargas al Estero Salado Ministerio del Ambiente. Available online at: http://www.ambiente.gob.ec/listado-de-industrias-con-descargas-al-estero-salado/ (Accessed December 8, 2024).
Montaño-Armijos M., Montolío S. (2008). Ecosistema Guayas (Ecuador). Medio ambiente y Sostenibilidad. Rev. Tecnológica ESPOL 21, 1–6. Available at: https://portal.issn.org/resource/ISSN/1390-3659 (Accessed December 8, 2024).
Morales-Estupiñan M. J., Recalde S., Orozco K., Ponce W. (2020). “Analysis of heavy metals in Azadirachta indica A,” in Juss Leaves, as Bioindicator for Monitoring Enviromental Pollution in Guayaquil (Proceedings of the 6th World Congress on New Technologies (NewTech'20) Prague, Czech Republic Virtual Conference, Ecuador). Paper No. ICEPR 145. doi: 10.11159/icepr20.145
Muller G. M. (1969). Index of geoaccumulation in sediments of the Rhine River. Geojournal 2, 108–118. Available online at: https://sid.ir/paper/618491/en.
Muñoz P. N., Salamanca M. A. (2003). Input of atmospheric lead to marine sediments in a south-east Pacific coastal area (∼36°S). Mar. Environ. Res. 55, 335–357. doi: 10.1016/S0141-1136(02)00277-5
Navarrete-Forero G., Morales Baren L., Dominguez-Granda L., Pontón Cevallos J., Marín Jarrín J. R. (2019). Heavy metals contamination in the gulf of Guayaquil: even limited data reflects environmental impacts from anthropogenic activity. Rev. Internacional Contaminación Ambiental 35, 731–755. doi: 10.20937/RICA.2019.35.03.18
Niemi G., Wardrop D., Brooks R., Anderson S., Brady V., Paeri H., et al. (2004). Rationale for a new generation of indicators for coastal waters. Environ. Health Perspect. 112, 979–986. doi: 10.1289/ehp.6903
Oi D., SECKIN G., ERSU C. B., YILMAZ T., SARI B. (2011). Heavy metal content and distribution in surface sediments of the Seyhan River, Turkey. J. Environ. Manage 92, 2250–2222. doi: 10.1016/j.jenvman.2011.04.013
Oliveira V. H., Díez S., Dolbeth M., Coelho J. P. (2024). Restoration of degraded estuarine and marine ecosystems: a systematic review of rehabilitation methods in Europe. J. Hazardous Materials 469, 133863. doi: 10.1016/j.jhazmat.2024.133863
Ormaza-González F. I., Caiza-Quinga R., Cárdenas-Condoy J., Intriago-Basurto A., Piguave-Tarira E. J., Ocaña – Balcázar K. D., et al. (2022). Sampling bottles for shallow estuarine waters, constructed using inexpensive recyclable materials. Estuarine Coast. Shelf Science. Estuarine Coast. Shelf Science. 275, 107965. doi: 10.1016/j.ecss.2022.107965
Ormaza-González F. I., Campi-Alvarez P. A., Cárdenas-Condoy J. W., Caiza-Quinga R. J., Statham P. J. (2024). Further evidence for increasing global near-shore eutrophication from the Estero Salado, Guayaquil, Ecuador. Continental Shelf Res. 278, 105271. doi: 10.1016/j.csr.2024.105271
Ormaza-González F. I., Martillo-Bustamante C. (2021). “The Gulf of Guayaquil the largest tropical estuary in the western Americas. Reviewing its geological and oceanographic transcendence,” in Conference: ECSA 58 - EMECS 13 Estuaries and coastal seas in the Anthropocene: Structure, functions, services and management. Hull-UK. ECSA 58 - EMECS 13. Available online at: https://allatlanticocean.org/events/ecsa-58-emecs-13-estuaries-and-coastal-seas-in-the-anthropocene/.
Ormaza-González F. I., Ponce-Villao G. E., Pin-Hidalgo G. M. (2020). Low mercury, cadmium and lead concentrations in tuna products from the eastern Pacific. Heliyon 6, e04576. doi: 10.1016/j.heliyon.2020.e04576
Özşeker K., Erüz C., Terzi Y. (2022). Evaluation of toxic metals in different grain size fractions of sediments of the southeastern black sea. Mar. pollut. Bull. 182, 113959.
Patmont C., Healy R. (2024). Puget Sound sediment cleanup remedy effectiveness retrospective. Integrated Environ. Assess. Manage, 1355–1365. doi: 10.1002/ieam.4890
Peñafiel M., Vallejo A., Chalen J. (2017). Evaluation of the physical-chemical quality in water and sediments of the salt estuary in northwestern Latin America. Pol. Con. (Edition 7) 2, 641–669. doi: 10.23857/pc.v2i6.151
Pérez-Ruzafa A., Marcos C., Pérez-Ruzafa I. M., Pérez-Marcos M. (2011). Coastal lagoons: “transitional ecosystems” between transitional and coastal waters. J. Coast. Conserv. 15, 369–392. doi: 10.1007/s11852-010-0095-2
Pernía B., Mero M., Cornejo X., Ramírez N., Ramírez L., Bravo K., et al. (2018). Determinación de cadmio y plomo en agua, sedimento y organismos bioindicadores en el Estero Salado, Ecuador. Enfoque UTE 9, 89–105. doi: 10.29019/enfoqueute.v9n2.246
Pinto R., Patrício J., Magalhães Neto J., Salas F., Marques J. C. (2010). Assessing estuarine quality under the ecosystem services scope: Ecological and socioeconomic aspects. Ecol. Complexity 7, 389–402. doi: 10.1016/j.ecocom.2010.05.001
Rehman Q., Rehman K., Akash M. S. H. (2021). “Heavy metals and neurological disorders: from exposure to preventive interventions,” in Environmental contaminants and neurological disorders (Cham: Springer International Publishing), 69–87.
Reynaud J.-Y., Witt C., Pazmiño A., Gilces S. (2018). Tide-dominated deltas in active margin basins: Insights from the Guayas estuary, Gulf of Guayaquil, Ecuador. Mar. Geology 403, 165–178. doi: 10.1016/j.margeo.2018.06.002
Romero-Estévez D., Yánez-Jácome G. S., Dazzini Langdon M., Simbaña-Farinango K., Rebolledo Monsalve E., Durán Cobo G and Navarrete H. (2020). An overview of cadmium, chromium, and lead content in bivalves consumed by the community of Santa Rosa Island (Ecuador) and its health risk assessment. Front. Environ. Sci. 8. doi: 10.3389/fenvs.2020.00134
Skilbeck C. G., Trevathan-Tackett S., Apichanangkool P., Macreadie P. I. (2017). Sediment sampling in estuaries: site selection and sampling techniques. In: Weckström K., Saunders K., Gell P., Skilbeck C. (eds) Applications of Paleoenvironmental Techniques in Estuarine Studies. Developments in Paleoenvironmental Research, vol 20. Springer, Dordrecht. doi: 10.1007/978-94-024-0990-1_5
Solórzano L., Viteri G. (1993). Investigación química del Estero Salado. Rev. Cien. Mar. y Limn. 3, 41–48. Available online at: https://www.gob.ec/ipiap.
Spencer K. L. (2017). “Estuarine deposited sediments: sampling and analysis,” in Estuarine and Coastal Hydrography and Sediment Transport. Eds. Uncles R. J., Mitchell S. B. (Cambridge University Press), 153–178.
Stevenson M. (1981). Variaciones Estacionales en el Golfo de Guayaquil, un estuario tropical Vol. IV (Boletín Científico y técnico Guayaquil: Instituto Nacional de Pesca), 133p. Available online at: https://institutopesca.gob.ec/revistas-ciencias-del-mar-limnologia/.
Thrush S. F., Townsend M., Hewitt J. E., Davies K., Lohrer A. M., Lundquist C., et al. (2013).The many uses and values of estuarine ecosy stems. In: Ecosystem services in New Zealand (Lincoln, New Zealand: conditions Manaaki Whenua Press). Available online at: https://www.researchgate.net/publication/281526181_The_many_uses_and_values_of_estuarine_ecosystems (accessed March 11, 2022).
TULAS. (2022). Ministerio de ambiente Ecuador. Anexo 1 del Libro vi del Texto Unificado de Legislacion Secundaria del Ministerio del Ambiente: Norma de Calidad Ambiental y de Descarga de Efluentes al Recurso Agua. Available online at: http://extwprlegs1.fao.org/docs/pdf/ecu155128.pdf (Accessed December 8, 2024).
US EPA. (1999). Office of Air and Radiation (Research Triangle Park, North Carolina: Office of Air Quality Planning and Standards), 225 pp.
Wang Z., Lin K., Liu X. (2022). Distribution and pollution risk assessment of heavy metals in the surface sediment of the intertidal zones of the Yellow River Estuary, China. Mar. pollut. Bull. 174, 113286. doi: 10.1016/j.marpolbul.2021.113286
Wang W.-X., Pan Ke, Tan Q., Guo L., Simpson S. L. (2014). Estuarine pollution of metals in China: science and mitigation. Environ. Sci. Technol. 48, 9975–9976. doi: 10.1021/es503549b
Wilmsmeier G., Monios J., Ballén Farfán A. F. (2021). Port system evolution in Ecuador – Migration, location splitting or specialisation? J. Transport Geogr. 93, 103042. doi: 10.1016/j.jtrangeo.2021.103042
Won J. H., Park J. Y., Lee T. G. (2007). Mercury emissions from automobiles using gasoline, diesel, and LPG. Atmospheric Environ. 41, 7547–7552. doi: 10.1016/j.atmosenv.2007.05.043
Worakhunpiset S. (20182018). Trace elements in marine sediment and organisms in the Gulf of Thailand. Int. J. Environ. Res. Public Health 15, 810. doi: 10.3390/ijerph15040810
Yan Y., Han L., Yu R.-l., Hu G.-r., Zhang W.-f., Cui J.-y., et al. (2020). Background determination, pollution assessment and source analysis of heavy metals in estuarine sediments from Quanzhou Bay, southeast China. Catena 187, 104322. doi: 10.1016/j.catena.2019.104322
Yi J., Lo L. S. H., Liu H., Qian P.-Y., Cheng J. (2021). Study of heavy metals and microbial communities in contaminated sediments along an urban estuary. Front. Mar. Sci. 8. doi: 10.3389/fmars.2021.741912
Zhang G., Bai J., Xiao R., Zhao Q., Jia J., Cui B., et al. (2017). Heavy metal fractions and ecological risk assessment in sediments from urban, rural, and reclamation-affected rivers of the Pearl River Estuary, China. Chemosphere 184, 278–288. doi: 10.1016/j.chemosphere.2017.05.155
Zhang W., Feng H., Chang J., Qu J., Xie H., Yu L. (2009). Heavy metal contamination in surface sediments of yangtze river intertidal zone: an assessment from different indexes. Environ. pollut. 157 (5), 1533–1543.
Zhao M., Wang E., Xia P., Feng A., Chi Y., Sun Y. (2019). Distribution and pollution assessment of heavy metals in the intertidal zone environments of typical sea areas in China. Mar. Pollut. Bull. 138, 397–406. doi: 10.1016/j.marpolbul.2018.11.050
Keywords: Pb, Cd, As, Hg, sediments, coastal lagoon, Estero Salado, Gulf of Guayaquil
Citation: Ormaza-González FI, Castro-Rendón RD, Maridueña-Bravo A, Bobadilla-Cordova N, Ramos-Castañeda I and Statham PJ (2024) Hg, Cd, As, and Pb in surface sediments from the tropical coastal lagoon Estero Salado, Gulf of Guayaquil-Ecuador. Front. Mar. Sci. 11:1457548. doi: 10.3389/fmars.2024.1457548
Received: 30 June 2024; Accepted: 29 November 2024;
Published: 20 December 2024.
Edited by:
Bernardo Duarte, Center for Marine and Environmental Sciences (MARE), PortugalReviewed by:
Sivalingam Periyasamy, National Research Council (CNR), ItalyPedro Brito, Portuguese Institute for Sea and Atmosphere (IPMA), Portugal
Copyright © 2024 Ormaza-González, Castro-Rendón, Maridueña-Bravo, Bobadilla-Cordova, Ramos-Castañeda and Statham. This is an open-access article distributed under the terms of the Creative Commons Attribution License (CC BY). The use, distribution or reproduction in other forums is permitted, provided the original author(s) and the copyright owner(s) are credited and that the original publication in this journal is cited, in accordance with accepted academic practice. No use, distribution or reproduction is permitted which does not comply with these terms.
*Correspondence: Franklin I. Ormaza-González, Zm9ybWF6YUBlc3BvbC5lZHUuZWM=