- 1Instituto de Ciencias Marinas y Limnológicas (ICML), Facultad de Ciencias, Universidad Austral de Chile, Valdivia, Chile
- 2Instituto Milenio Biodiversidad de Ecosistemas Antárticos y Subantárticos (BASE), Ñuñoa, Santiago, Chile
- 3Centro Fondap de Investigación en Dinámica de Ecosistemas Marinos de Altas Latitudes (IDEAL), Universidad Austral de Chile, Valdivia, Chile
- 4Universidad Nacional de Córdoba, Facultad de Ciencias Exactas, Físicas y Naturales, Cátedra de Ecología Marina, Córdoba, Argentina
- 5Consejo Nacional de Investigaciones Científicas y Técnicas (CONICET), Instituto de Diversidad y Ecología Animal (IDEA), Ecosistemas Marinos y Polares (ECOMARES), Córdoba, Argentina
- 6Departamento de Biología Marina, Facultad de Ciencias del Mar, Universidad Católica del Norte, Coquimbo, Chile
- 7Instituto Milenio en Socio-ecología Costera (SECOS), Coquimbo, Chile
- 8Laboratorio de Ecosistemas Marinos Antárticos y Subantárticos, Universidad de Magallanes, Punta Arenas, Chile
- 9Cape Horn International Center (CHIC), Universidad de Magallanes, Punta Arenas, Chile
- 10Centro de Investigación Gaia-Antártica, Universidad de Magallanes, Punta Arenas, Chile
- 11South Atlantic Environmental Research Institute, Falkland Islands, Port Stanley, United Kingdom
- 12School of Biological Sciences (Zoology), University of Aberdeen, Aberdeen, United Kingdom
- 13Instituto de Investigaciones Marinas y Costeras (IIMyC), Facultad de Ciencias Exactas y Naturales (FCEyN), Universidad Nacional de Mar del Plata (UNMdP)-CONICET, CC1260, Mar del Plata, Argentina
- 14Laboratorio de Ecología Molecular (LEM), Departamento de Ciencias Ecológicas, Facultad de Ciencias, Universidad de Chile, Santiago, Chile
Quaternary glaciations severely altered landscape/seascape at high latitudes and had major consequences on species geographical ranges, population sizes, genetic differentiation and speciation rates. The Magellan province in southern South America, constitutes an interesting area to evaluate the effect of glaciations over near-shore marine benthic organisms. Existing data have showed clear signatures of population genetic bottlenecks during glacial maxima followed by recent recolonizations and expansions during the interglacial. Here, we present new population-based analyses in species of the patellogastropod genus Nacella (N. deaurata, N. magellanica, and N. mytilina). The species N. magellanica and N. deaurata inhabit rocky ecosystems while N. mytilina lives closely associated with kelps. The analyzed species exhibit narrow bathymetric ranges and consequently should have been severely affected by recurrent glacial cycles. We performed phylogeographic and demographic analyses in Nacella species including different localities across their respective distributions in the Magellan province including the Falkland/Malvinas Islands (F/M). Genetic analyses showed that all Nacella species exhibited low levels of genetic diversity, the presence of single dominant broadly distributed haplotypes, lack of correlation between geographical and genetic distance, and recent demographic growths, which are evidence supporting rapid postglacial expansions. Such results may have been facilitated by larval and/or rafting-mediated dispersal following the Cape Horn Current System. The three species showed strong and significant differentiation between F/M and southern Pacific margin (SPM) populations including localities across the Strait of Magellan and Cape Horn. Haplotype genealogies and mismatch analyses recognized older and more complex demographic histories in the F/M than in South America. Different glaciological histories between SPM and F/M may be responsible of the marked phylogeographic structure in the analyzed species of Nacella. Alternatively, as previously proposed, the F/M represent a glacial refugium for Nacella species (sink area), as well as a secondary contact zone where endemic haplotypes are found together with recently arrived South American ones. Historical and contemporary processes, contrasting glacial histories between the analyzed areas, as well as life history traits of the analyzed organisms are main factors explaining current phylogeographic patterns of shallow Magellan marine benthic organisms.
Introduction
Ice advances and retreats during the glacial episodes of the Quaternary, and particularly the Last Glacial Maximum (LGM) around 20,000 years ago, generated major landscape/seascape shifts and drastic reduction of available habitats at higher latitudes (Hewitt, 2000; Hewitt, 2004; Fraser et al., 2012). Concomitantly, glacial events affected the distribution and the geographical ranges of species, as well as the demography and populations sizes (Provan and Bennett, 2008; Marko et al., 2010; Allcock and Strugnell, 2012; González-Wevar et al., 2012; González-Wevar et al., 2013; Riesgo et al., 2015; González-Wevar et al., 2018; González-Wevar et al., 2021). Paleontological and palynological evidence from the Northern Hemisphere together with biogeographic and population-based studies provided the empirical basis for the Expansion-Contraction (EC) model of Quaternary biogeography proposed by Provan and Bennett (2008); a relative simple but useful model to describe the geographical response of population and species to past glacial and interglacial periods, which allows one to test demographic and phylogeographic hypotheses (Bennett et al., 1991; Webb and Bartlein, 1992; Williams et al., 1998; Hewitt, 2004; Maggs et al., 2008; Allcock and Strugnell, 2012).
Under a basic EC model, species contracted their distributions into lower latitude glacial refugia throughout glacial maxima while, during interglacials, they expanded their distributions to areas that were previously glaciated (Provan and Bennett, 2008; Marko et al., 2010; Fraser et al., 2012; González-Wevar et al., 2012; González-Wevar et al., 2018; González-Wevar et al., 2021). Accordingly, lower latitude non-glaciated refugial areas are expected to harbor older populations with higher levels of genetic diversity compared to those areas that were heavily impacted by ice and/or recently recolonized ones that are associated with recent postglacial demographic expansions (Marko, 2004; Maggs et al., 2008; Marko et al., 2010; González-Wevar et al., 2013; Fernández Iriarte et al., 2020).
During the LGM, the Pacific margin of the Magellan province in southern South America, from Chiloe Island (42°S) to Cape Horn (56°S), was almost completely covered by the Patagonian Ice Sheet (McCulloch et al., 2000; Hulton et al., 2002; Hein et al., 2010; Rabassa et al., 2011). The availability of shallow sheltered hard-substrate habitats was limited or even absent and hence prevented the survival of most of the near-shore marine species in this Magellan area. During interglacial periods, formerly ice-impacted areas were recolonized following the deglaciation process through dispersal from refugial populations. Recurring rounds of extinctions and recolonization may have enhanced the diversification of near-shore marine invertebrates across this province by the availability of vacant habitats and/or through the survival in multiple refugia which, allowed allopatric divergence (Valdovinos et al., 2003; Kiel and Nielsen, 2010; González-Wevar et al., 2011; González-Wevar et al., 2017). Contrarily to the glacial history recorded across the Pacific Magellan margin, glacial periods of the Quaternary would have affected to a much lesser extent the marine ecosystems across the Atlantic margin of this province (Glasser and Jansson, 2008) and the Falkland/Malvinas Islands (Clapperton and Sugden, 1976; Wilson et al., 2002; Wilson et al., 2008).
Several phylogeographic and population-based studies have been performed in distinct groups of Magellan near-shore marine (Fraser et al., 2010; de Aranzamendi et al., 2011; Ceballos et al., 2012; González-Wevar et al., 2012; de Aranzamendi et al., 2014; Nuñez et al., 2015; Ceballos et al., 2016; González-Wevar et al., 2016a; González-Wevar et al., 2018; Fernández Iriarte et al., 2020) and freshwater (Ruzzante et al., 2006; Zemlak et al., 2008; González-Wevar et al., 2015a; González-Wevar et al., 2015b) organisms. Studies in marine organisms have determined that most of the analyzed taxa exhibited low levels of genetic diversity, absence of genetic structure and strong signals of recent demographic growth, supporting the hypothesis of recent postglacial expansions (Fraser et al., 2010; Macaya and Zuccarello, 2010; de Aranzamendi et al., 2011; González-Wevar et al., 2012; de Aranzamendi et al., 2014; González-Wevar et al., 2017; Pardo-Gandarillas et al., 2018). Such studies have provided evidence of Quaternary glacial refugia on the East side of the Andes, along the Patagonia steppe (Ruzzante et al., 2006; Zemlak et al., 2008), refugia on the West side of the Andes both within (Xu et al., 2009; Nuñez et al., 2011), and outside the limit of the glaciers (Ruzzante et al., 2006; González-Wevar et al., 2015a; González-Wevar et al., 2015b). Moreover, species with high dispersive potential such as the buoyant macroalgae Durvillaea antarctica and Macrocystis pyrifera recolonized the Magellan province following the LGM from geographically distant regions (Macaya and Zuccarello, 2010; Fraser et al., 2012). These studies demonstrate the key role of long-distance dispersal mediated by rafting in the biogeography of sub-Antarctic marine near-shore benthic organisms (Waters, 2008; Fraser et al., 2009; Leese et al., 2010; Nikula et al., 2010; Moon et al., 2017; González-Wevar et al., 2018; González-Wevar et al., 2021). Even when many near-shore marine species are broadly distributed across the whole Magellan province, few genetic studies have been conducted across both the Pacific (PM) and the Atlantic (AM) margins of southern South America (Ceballos et al., 2016; González-Wevar et al., 2012; Trovant et al., 2015; González-Wevar et al., 2016a; González-Wevar et al., 2017; Fernández Iriarte et al., 2020). Moreover, just a couple of population-based studies have included populations from the Falkland/Malvinas Islands (F/M) (González-Wevar et al., 2012; González-Wevar et al., 2016a; González-Wevar et al., 2017; Fernández Iriarte et al., 2020; González-Wevar et al., 2021).
True limpets of the genus Nacella (Patellogastropoda: Nacellidae) are dominant invertebrates of Antarctic and sub-Antarctic intertidal and subtidal rocky ecosystems. Nacella species are gonochoric with external fertilization with a larval lifespan that can extend for more than two months (Bowden et al., 2009; Peck et al., 2016). Currently, the genus includes 12 nominal species that are distributed in different provinces of the Southern Ocean such as maritime Antarctica, southern South America and sub-Antarctic islands (South Georgia, Marion, Kerguelen, Heard, Macquarie and Campbell) (Powell, 1973; Valdovinos and Rüth, 2005; González-Wevar et al., 2019). Biogeographical analyses in Nacella identified the role of recent long-distance dispersal processes associated with the colonization of geographically isolated sub-Antarctic islands (González-Wevar et al., 2016b; González-Wevar et al., 2017). Moreover, the evolution of Nacella includes a recent Quaternary radiation in the Magellan province where four different species (N. deaurata, N. flammea, N. magellanica and N. mytilina) diversified (González-Wevar et al., 2011; González-Wevar et al., 2017, González-Wevar et al., 2019). The species N. magellanica exhibits the broadest distribution with populations along PM between Chiloé Island (42°S) and Diego Ramírez Archipelago (56°S) (González-Wevar et al., 2019). In AM N. magellanica is found from the Río Negro Province (41°S) to Isla de los Estados (55°S) and is also abundant in F/M (González-Wevar et al., 2019). The species N. deaurata and N. mytilina exhibit much narrower distributions from Guarello Island (50°S) to Diego Ramírez Archipelago (56°S) in PM while in AM they are mainly restricted to Tierra del Fuego and F/M (González-Wevar et al., 2019). Population-based studies in N. magellanica recorded a single genetic unit across PM (González-Wevar et al., 2012) and low levels of genetic structure across 2900 km of coast in AM (de Aranzamendi et al., 2014). Also, significant genetic differences have been identified among three main groups PM, AM, and F/M (González-Wevar et al., 2012). Similarly, the species N. mytilina showed absence of genetic differentiation across PM and strong phylogeographic signal between PM and F/M (González-Wevar et al., 2016a).
This study aims to evaluate the impacts of Quaternary glaciations over near-shore marine invertebrates across their distributions in southern South America using as model organisms three species of Nacella (N. magellanica, N. mytilina, and N. deaurata). For this, we performed phylogeographic and population-based analyses using fragments of the mitochondrial cytochrome c subunit I (COI) using previously published data sets in N. magellanica (de Aranzamendi et al., 2009; de Aranzamendi et al., 2011; González-Wevar et al., 2012; de Aranzamendi et al., 2014) and N. mytilina (González-Wevar et al., 2016a) and N. deaurata (de Aranzamendi et al., 2009; de Aranzamendi et al., 2011) as well new data for N. magellanica and N. deaurata. We hypothesize that Quaternary glacial cycles have differently impacted PM, AM and F/M populations of N. magellanica because the extent of habitat eradication would have varied in these areas. Populations across PM are expected to harbor genetic footprints of recent demographic expansions while non-glaciated areas of AM and F/M Islands are expected to show higher levels of genetic diversity because of more stable demographic histories. In those Nacella species with the narrowest geographical distributions (N. mytilina and N. deaurata), specifically those restricted to the southern tip of South America and F/M, we expect to find similar phylogeographic signatures; each should have a low level of genetic diversity structure in a southern South American population (SPM = Strait of Magellan and Cape Horn) and strong genetic structure between this and a population from F/M. Through this study we aim to further understand how key near-shore marine benthic elements survived Quaternary climate shifts in the southern tip of South America in areas that were differentially impacted by ice advances and retreats.
Material and methods
Samplings, DNA extraction and COI amplifications
Individuals of different species of Nacella (N. magellanica, N. mytilina, and N. deaurata) were collected from intertidal and subtidal rocky shore areas between 2010 and 2018 across the Magellan province in three main areas: the Pacific margin (PM), the Atlantic margin (AM) and the Falkland/Malvinas Islands (F/M) (Figure 1; Table 1). We analyzed a total of 18 localities of N. magellanica across the species’ distribution including populations across PM, AM, and F/M. In the case of Nacella species with more restricted distributions such as N. deaurata and N. mytilina we analyzed 8 and 13 localities, respectively, across these species’ distributions in PM including populations from the Strait of Magellan, Cape Horn, and F/M. Nacella specimens were identified following Powell, 1973, Valdovinos and Rüth (2005) and González-Wevar et al. (2011, 2019). All the individuals were fixed in ethanol (95%) and DNAs preparations were done using a standard salt-extraction methodology following Aljanabi and Martinez (1997). A partial fragment of the mitochondrial cytochrome c subunit I gene (COI) was amplified using universal primers LCO1490 and HCO2198 (Folmer et al., 1994) following González-Wevar et al. (2010) and de Aranzamendi et al. (2009). PCR amplification products were purified and sequenced in both directions at Macrogen Inc. (Seoul, South Korea). Forward and reverse sequences were assembled and edited independently for each Nacella species using GENEIOUS (http://www.geneious.com). Multiple sequence alignments were performed using MUSCLE (Edgar, 2004). Nucleotide base compositions were calculated in MEGA 11 (Tamura et al., 2022). Wright’s mitochondrial codon usage was computed using the Effective Number of Codons (ENC) in DnaSP v5 (Librado and Rozas, 2009). New Nacella deaurata COI sequences are available at GenBank under the following Accession Numbers: OQ587619 - OQ587817. Additionally, we included previously published sequences under the GenBank Accession Numbers: EU870927–EU870985, HQ880439–HQ880550; JX262742–JX262797 for N. magellanica; EU870986 – EU0870999; HQ880551–HQ880573 for N. deaurata and KX600474 - KX600492 for N. mytilina (de Aranzamendi et al., 2009; de Aranzamendi et al., 2011; González-Wevar et al., 2012; González-Wevar et al., 2016a).
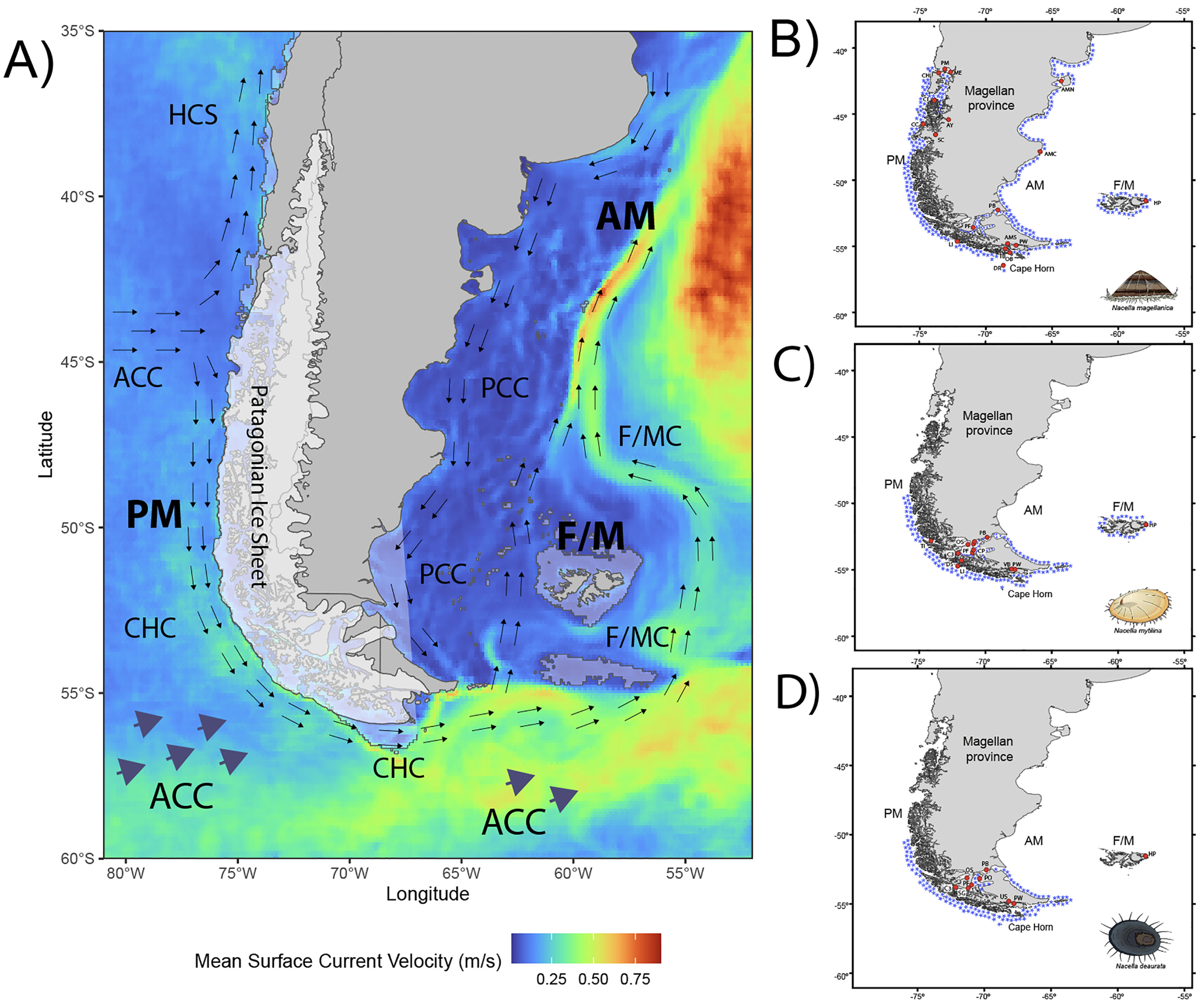
Figure 1 (A) The Magellan province in southern South America showing main oceanographic circulation patterns and directionality, the extension of continental ice and the position of the coastlines during the Last Glacial Maximum (LGM). Current world coastlines shapefile and DEM raster for LGM bathymetry simulation (-150 m) from Natural Earth (public domain). Sampling localities and distribution of (B) Nacella magellanica, (C) Nacella mytilina and (D) Nacella deaurata along the Pacific Magellan margin (PM), the Atlantic Magellan margin (AM) and the Falkland/Malvinas (F/M) Islands. Where: ACC, Antarctic Circumpolar Current; HCS, Humboldt Current System; CHC, Cape Horn Current; F/MC, Falkland/Malvinas Current; PCC, Patagonian Coastal Current. Sampling localities; PM, Puerto Montt; ME, Metri; CHI, Chiloé; CI, Concoto Island; AY, Aysén; CC, Costa Channel; SC, Serrano Channel; LI, London Island; PF, Port Famine; PB, Possession Bay; OB, Orange Bay; TB, Tekenika Bay; PW, Puerto Williams; DR, Diego Ramírez Island; AMN, Atlantic margin north; AMC, Atlantic margin center; AMS, Atlantic margin south; HP, Hookers Point; TI, Tamar Island; DS, Duntze Sound; C3, Carlos III Island; CP, Carrera Point; OS, Otway Sound; CH, Chabunco; LB, Laredo Bay; VB, Virginia Bay; PB, Paula Bay; SG, San Gregorio Bay; US, Ushuaia.
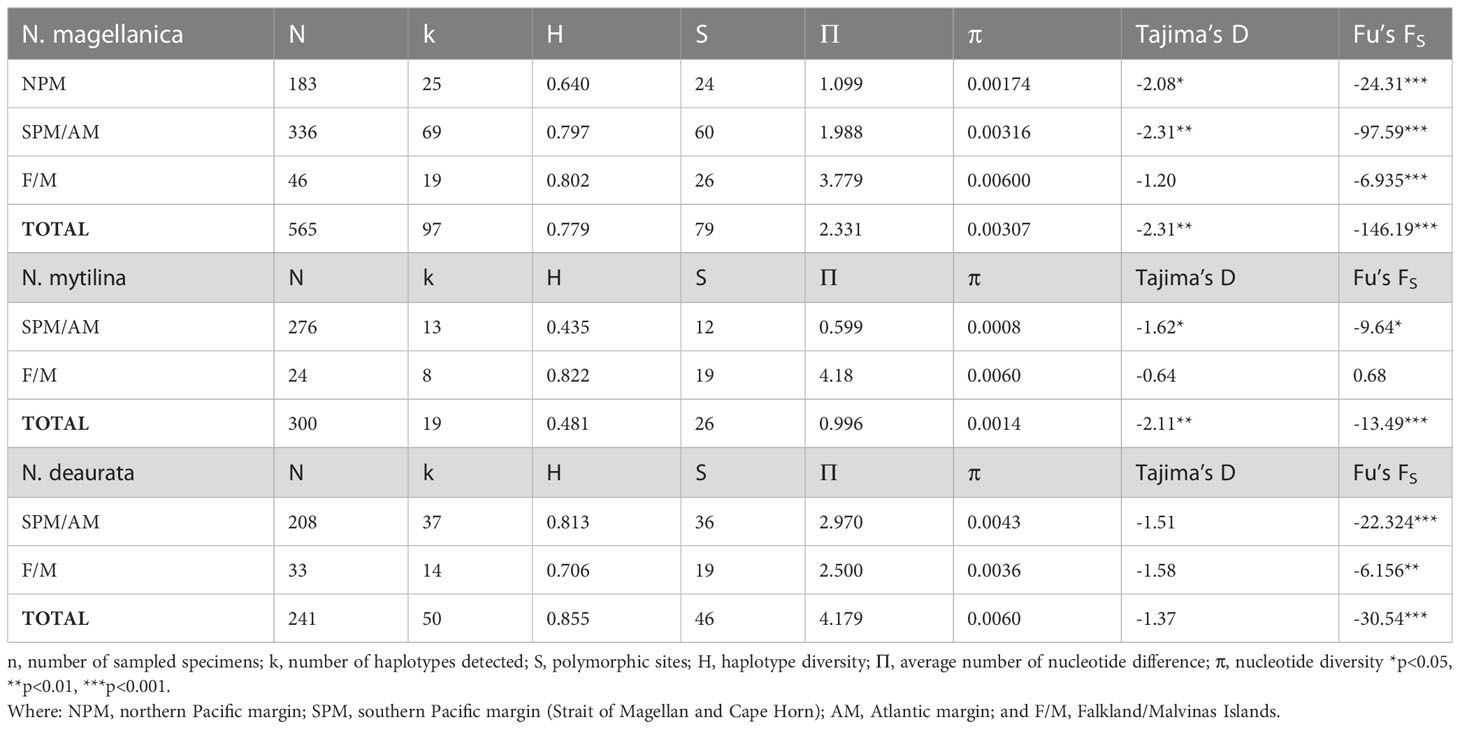
Table 1 Main patterns of genetic diversity and neutrality test in the recognized genetic clusters of A) Nacella magellanica, B) Nacella mytilina and C) Nacella deaurata.
Genetic diversity and population structure in Magellan Nacella species
We performed a DNA saturation analysis following Xia and Xie (2001) to evaluate how saturation of transitions accumulates in relation to nucleotide divergence in each Nacella species COI data set. Moreover, we estimated levels of population mtDNA polymorphism in the three species of Nacella through standard diversity indices including number of haplotypes (k), haplotype diversity (H), and the number of segregating sites (S). We also determined the average number of pairwise differences and the nucleotide diversity across the species distributions using DnaSP (Table 1).
We determined patterns of population differentiation for each Nacella species following Pons and Petit (1996) using haplotype frequencies (GST) and mean pairwise differences (NST) in Arlequin v.3 (Excoffier et al., 2005). The statistical significances of average genetic pairwise differences were calculated using permutation tests (20,000 iterations) and the adjustment for multiple testing was done through False Discovery Rate (FDR) correction (Narum, 2006). Moreover, for each Nacella species we estimated levels of genetic differentiation using the nearest-neighbor statistic (Snn) which measures how often nearest neighbor (in sequence space) sequences are from the same locality in geographic space (Hudson, 2000). The statistical significance of Snn was determined through permutation tests (20,000 iterations) for each Nacella species.
We used two different clustering methods to determine the spatial genetic structure of the analyzed Nacella species. First, we determined the number and the composition of panmictic groups and the spatial boundaries using a Bayesian model computed in GENELAND v.2.0.0 (Guillot et al., 2005) in the R environment (Ihaka and Gentleman, 1996). Through a Markov Chain Monte Carlo (MCMC) this analysis estimates the best clustering of samples considering genetic and geographic information. Geneland analyses were run using 50 x 106 MCMC iterations sampled each 1,000 steps. Assembled scores were graphed against generations in Tracer v.1.5. (Rambaut et al., 2018) to identify stationarity and the number of generations to be discarded as burn-in. A maximum number of clusters for each species (N. magellanica K = 19, N. deaurata K = 9 and N. mytilina K = 14) were run to estimate the model parameters and posterior probabilities of group membership. Second, we determined the spatial genetic structure for each Nacella species. We used Spatial Analysis of Molecular Variance (SAMOVA) (Dupanloup et al., 2002) to estimate the number and composition of groups that were most differentiated based on our sequence data set. This analysis allowed us to partition the genetic variance into i) within populations, ii) among populations within groups and iii) among groups.
Demographic analyses in Magellan Nacella species
Haplotype genealogical relationships in the analyzed Nacella species were reconstructed through median-joining and maximum parsimony networks in Network 10 (Forster et al., 2001) and Hapview (Salzburger et al., 2011), respectively. Moreover, we performed neutrality statistical tests (Tajima’s D and Fu’s FS) using DnaSP for the whole COI data set, for each species, to estimate whether sequences deviate from mutation-drift equilibrium. Population demographic histories were estimated comparing the distribution of pairwise differences between haplotypes (mismatch distribution) for each Nacella species under the sudden expansion growth model of Rogers and Harpending (1992). This analysis rests in the estimation of three parameters: i) τ = the date of growth/decline measured in units of mutational time (τ = 2μt) where t = time in years and μ = mutational rate per sequence per year, ii) initial theta θi = 2 Niμ before the population growth/decline) and iii) final theta θt = 2 Ntμ after population growth/decline. The demographic expansion parameters were estimated using the nonlinear least square approach implemented in Arlequin (Schneider and Excoffier, 1999). The goodness of fit between the observed and expected mismatch distributions was tested using a parametric bootstrap approach that uses the sum of squared deviations as a statistic test implemented in Arlequin. Moreover, we reconstructed past population dynamics through time using a Bayesian skyline plot method in BEAST (Drummond and Rambaut, 2007; Drummond et al., 2012). A strict-clock model was selected as the best fit for COI data in Nacella species and we used a specific population-level mutational rate (1% per million years) previously estimated for COI in Nacellidae limpets (González-Wevar et al., 2010; González-Wevar et al., 2012; González-Wevar et al., 2017). We performed independent Bayesian MCMC runs for each Nacella species recognized genetic groups using the GTR + G model, previously estimated using MrModeltest v.2.3 (http://www.abc-se/~nylander). Two independent runs for each Nacella species’ groups were made for 250 x 106 generations (sampled every 10000 step), discarding a 10% of the trees as burn-in. The convergence of each run was determined using Tracer (Rambaut et al., 2018) ensuring a minimum of 1000 effective sampling for each statistic (ESS). The results of the multiple runs were combined using LogCombiner (Drummond and Rambaut, 2007). The median and corresponding credibility intervals of the Bayesian skylines plots were depicted with Tracer.
Gene flow and connectivity
We compared different models of gene flow between the recorded genetic groups (PM, AM, and F/M) in the analyzed Nacella species to test for distinctive scenarios using the software MIGRATE v.3.5 (Beerli and Felsenstein, 2001). We defined candidate models for each species constraining the directionality of gene flow between the recorded groups. The analyses were performed using a HKY + I + F substitution model and transition/transversion ratio of 10.56432 (N. magellanica), 6.4682 (N. deaurata) and 10.8857 (N. mytilina) as previously estimated by ModelFinder in the program IQ-TREE v.2.2.0 (Kalyaanamoorthy et al., 2017). The specific substitution rate for the selected marker was set to constant following the recommendation of the software developer. Analysis consisted of one long chain with 500,000 recorded parameter steps, a sampling interval of 100 and a burn-in of 10%, running multiplate replicates (10 independent chains). We used a heated scheme (1.0, 1.5, 3.0, 1000000.0) to calculate the marginal likelihoods for model comparisons. Moreover, we used a thermodynamic integration approximation (T.I.) for the log-equivalent Bayes Factor (LBF) considering that this analysis results in LBFs with high repeatability and little variation following Beerli and Palczewski (2010). Higher T.I. values are associated with a better fit of the model than lower ones. The associated probability of each model in relation to others was measured following Kaas and Raftery (1995).
Results
Genetic diversity in Nacella species
The COI data sets for each analyzed Nacella species are summarized in Table 1. No insertion/deletion or stop codons were found in the data sets and sequences were not saturated at any position. Population analyses in Nacella species included specimens of N. deaurata (N = 241), N. magellanica (N = 565) and N. mytilina (N = 300) (Figure 1; Table 2, Tables S1–S3). The COI set in N. magellanica included 630 nucleotide positions coding for 210 amino acids while the COI data sets in N. deaurata and N. mytilina included 687 positions coding for 229 amino acids. In N. magellanica we found two amino acids substitutions at positions 111 (V for I) and 201 (M for L) using the invertebrate mitochondrial table. In the case of N. deaurata we detected three amino acids substitutions at positions 79 (P to R), 186 (L to F), and 197 (M to T) while a single amino acid substitution was found in N. mytilina at position 182 (A to V). Medium levels of genetic diversity characterized populations of N. magellanica with 79 polymorphic sites (12.5%) and 44 of them (55.69%) were parsimoniously informative. Low levels of genetic diversity characterized populations of N. deaurata/N. mytilina with 46/26 polymorphic sites (6.69%/3.78%) and 25/16 of them (54.34%/61.53%) were parsimoniously informative, respectively. Sequences in Magellan Nacella species were A-T rich (60.8% in all the three species) compared to the main G – C content and no evidence of codon bias was detected (ENC N. magellanica = 35.89, ENC N. mytilina = 39.28, and ENC N. deaurata = 35.19).
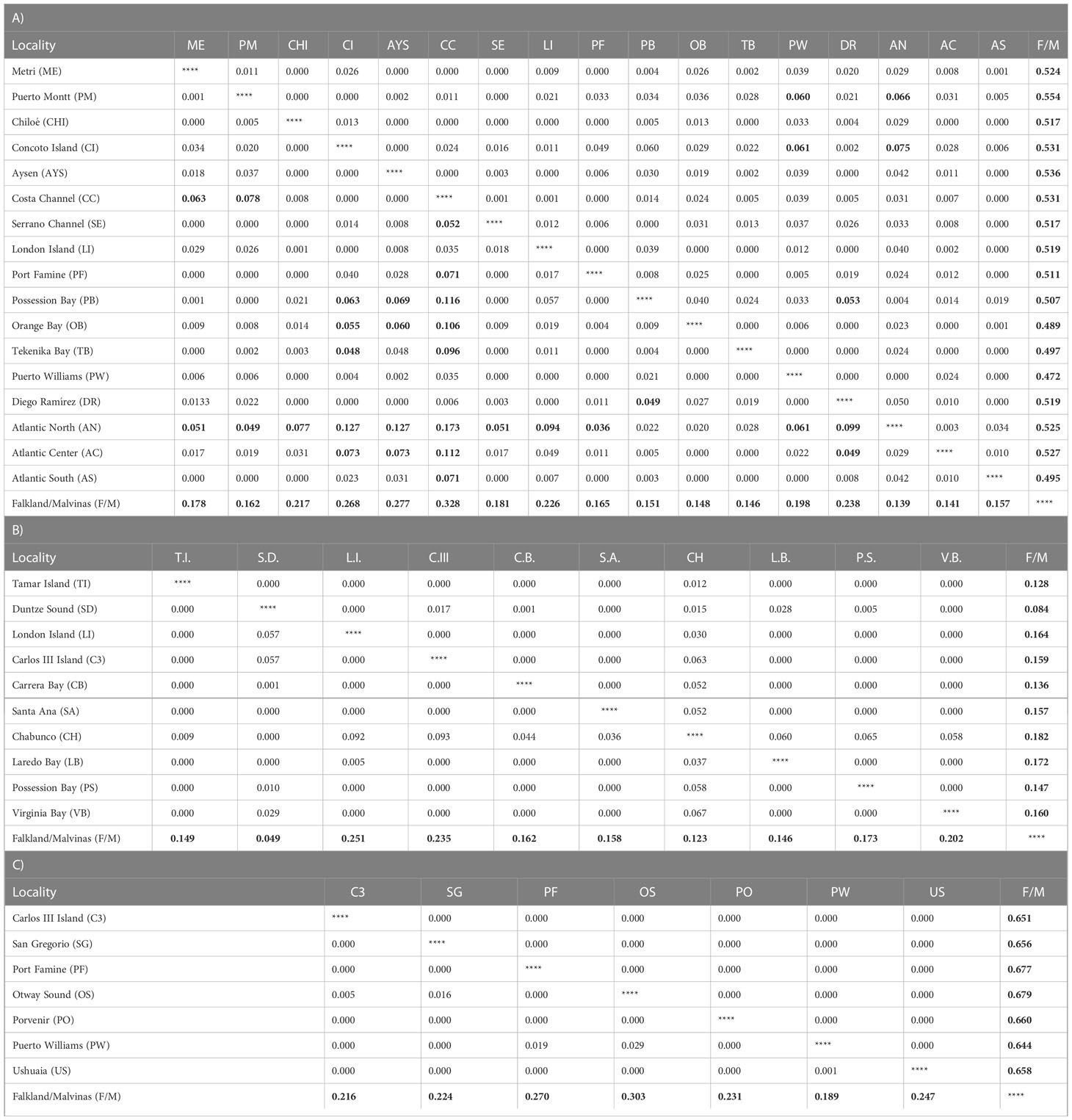
Table 2 Pairwise GST values, based on haplotypic frequencies, (below diagonal) and average number of nucleotide differences between localities NST (above diagonal) in the analyzed Nacella species where A) Nacella magellanica, B) Nacella deaurata and C) Nacella mytilina 20200 iterations, significative values (FDR corrected) are marked in bold.
The haplotype diversity (H) in N. magellanica varied between 0.863 (Atlantic North) and 0.370 (Costa Channel) (Table S1). The number of polymorphic sites (S) in N. magellanica varied between 5 (Costa Channel) and 27 (Atlantic Center). The average number of nucleotide differences (Π) and the mean nucleotide diversity (π) in N. magellanica were low in most of the localities across PM and AM while the diversity of these indices was comparatively higher in the F/M (Table S1). The haplotype diversity (H) in N. deaurata varied between 0.706 (Hookers Point, F/M) and 0.907 (Puerto Williams) (Table S2). The number of polymorphic sites (S) in N. deaurata varied between 10 (Ushuaia) and 22 (Puerto Williams). The average number of nucleotide differences and the mean nucleotide diversity (✓/π) in N. deaurata varied between 2.500/0.00364 (Hookers Point, F/M)) and 3.211/0.00467 (Puerto Williams) (Table S2). In the case of N. mytilina the haplotype diversity (H) varied between 0.284 (Carlos III Island) and 0.822 (Hookers Point, F/M) (Table S3). The number of polymorphic sites (S) in N. mytilina varied between 3 (several localities) and 19 (Hookers Point, F/M). Similarly, the number of haplotypes (k) in N. mytilina varied between 4 (several localities) and 8 (Hookers Point, F/M). The average number of nucleotide differences (✓) and the mean nucleotide diversity (π) in N. mytilina were low in most of the localities across the southern Pacific margin (Strait of Magellan and Cape Horn) while the diversity of these indices was comparatively higher in F/M (Table S3).
Genetic structure in Nacella species across the Magellan province
Mean general values of differentiation estimated in the analyzed Nacella species were low, especially considering average values of GST and NST (Table 2). Most part of the population-level differences were detected in the three species of Nacella when comparing F/M against the rest of the populations from South America. Nevertheless, N. magellanica also showed significant levels of differentiation between northern PM populations (NPM) and those from the Strait of Magellan, Cape Horn (SPM) and AM (Table 2). In the case of N. deaurata and N. mytilina general levels of differentiation across their distributions in the Strait of Magellan and Cape Horn were very low and pairwise populations comparisons did not reveal significant structure in these taxa across these areas. The nearest neighbor statistic (Snn) in Nacella species (Snn N. magellanica = 0.1656; Snn N. deaurata = 0.236 and Snn N. mytilina = 0.09) showed low but significant levels of phylogeographic signal (p < 0.0001) for the whole COI data set. However, when Snn analyses were performed considering the main pattern of genetic differentiation recorded for each species, this statistic became very high (Snn N. magellanica = 0.95, Snn N. deaurata = 0.976 and Snn N. mytilina = 0.9) showing the high degree of phylogeographic signal between South American and F/M populations in the three species of Nacella analyzed here. The patterns of genetic structure found in each Nacella species was supported by the model based on Bayesian clustering algorithm which detected three main clusters in N. magellanica and two main clusters in N. deaurata and N. mytilina, respectively (Figure 2). The first cluster in N. magellanica included northern localities across PM (NPM), the second one encompassed southern localities of PM (SPM = Strait of Magellan and Cape Horn) together with AM sites and the third one the F/M locality (Figure 2A). In the case of N. deaurata (Figure 2B) and N. mytilina (Figure 2C), the first cluster included all the localities across SPM while the second cluster the F/M population, respectively. Values of cluster membership were high for all localities (c.a. P = 0.9). Similarly, SAMOVA analyses in N. magellanica recorded three maximally differentiated groups (NPM, SPM/AM, and F/M) accounting for 32.98% of the total variance (Table 3). In N. deaurata and N. mytilina SAMOVA analyses recorded two maximally differentiated groups (SPM and F/M) accounting for 65.01% (N. deaurata) and 43.09% (N. mytilina) of the total variance (Table 3).
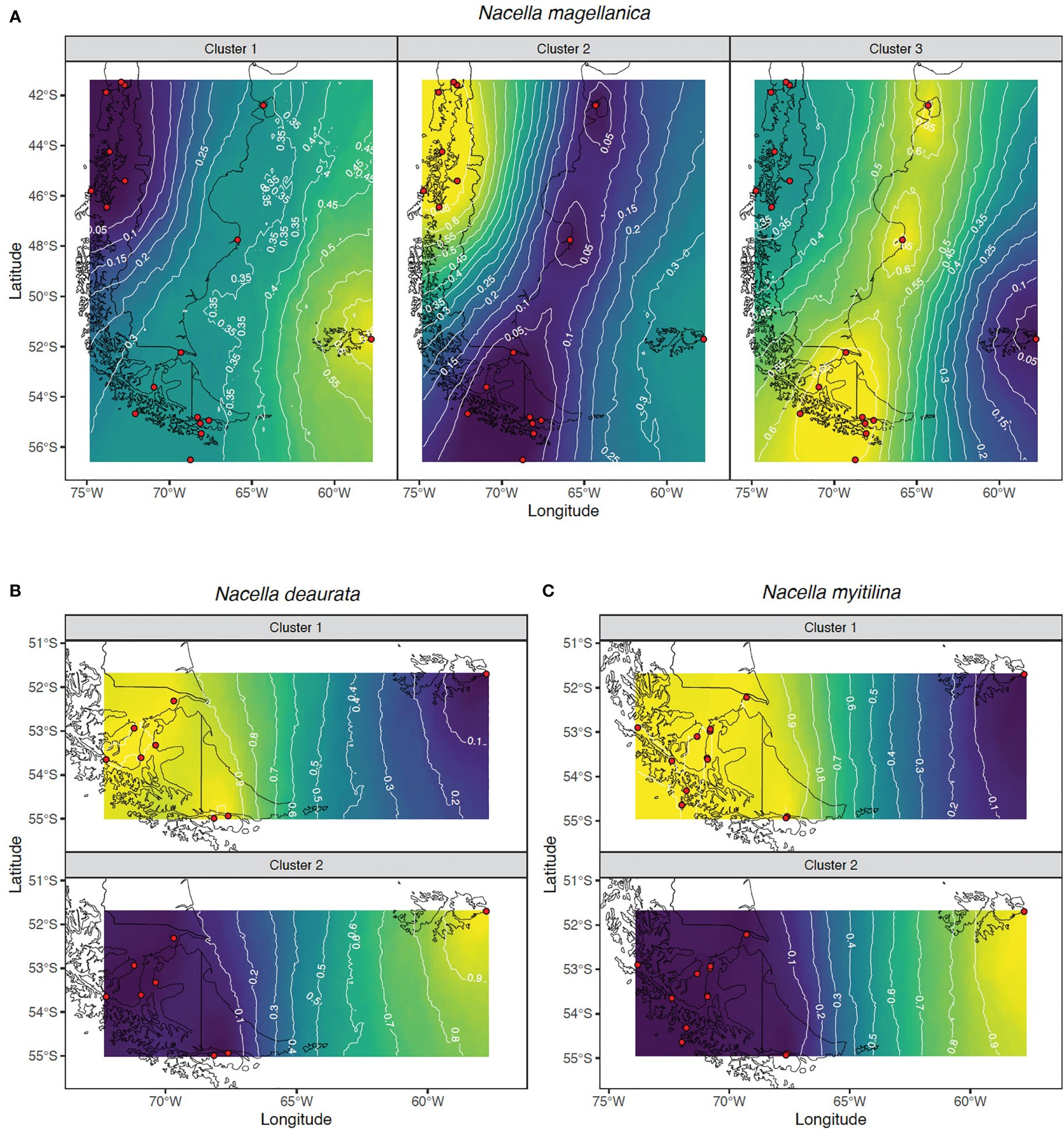
Figure 2 Spatial output from Geneland using all (A) N. magellanica, (B) N. deaurata and (C) N. mytilina populations. Red circles indicate the relative positions of the sampling localities. Darker and lighter shadings are proportional to posterior probabilities of membership to a particular cluster where lighter (yellow areas) show the highest probabilities of cluster. Posterior probabilities of membership were plotted to the shapefiles of the Magellan coastline available in the database GEOdas (NOAA) and filtered using GEOdas Coastline Extractor v.1.1.3.1 (https://www.ngdc.noaa.gov/mgg/geodas/geodas.html).
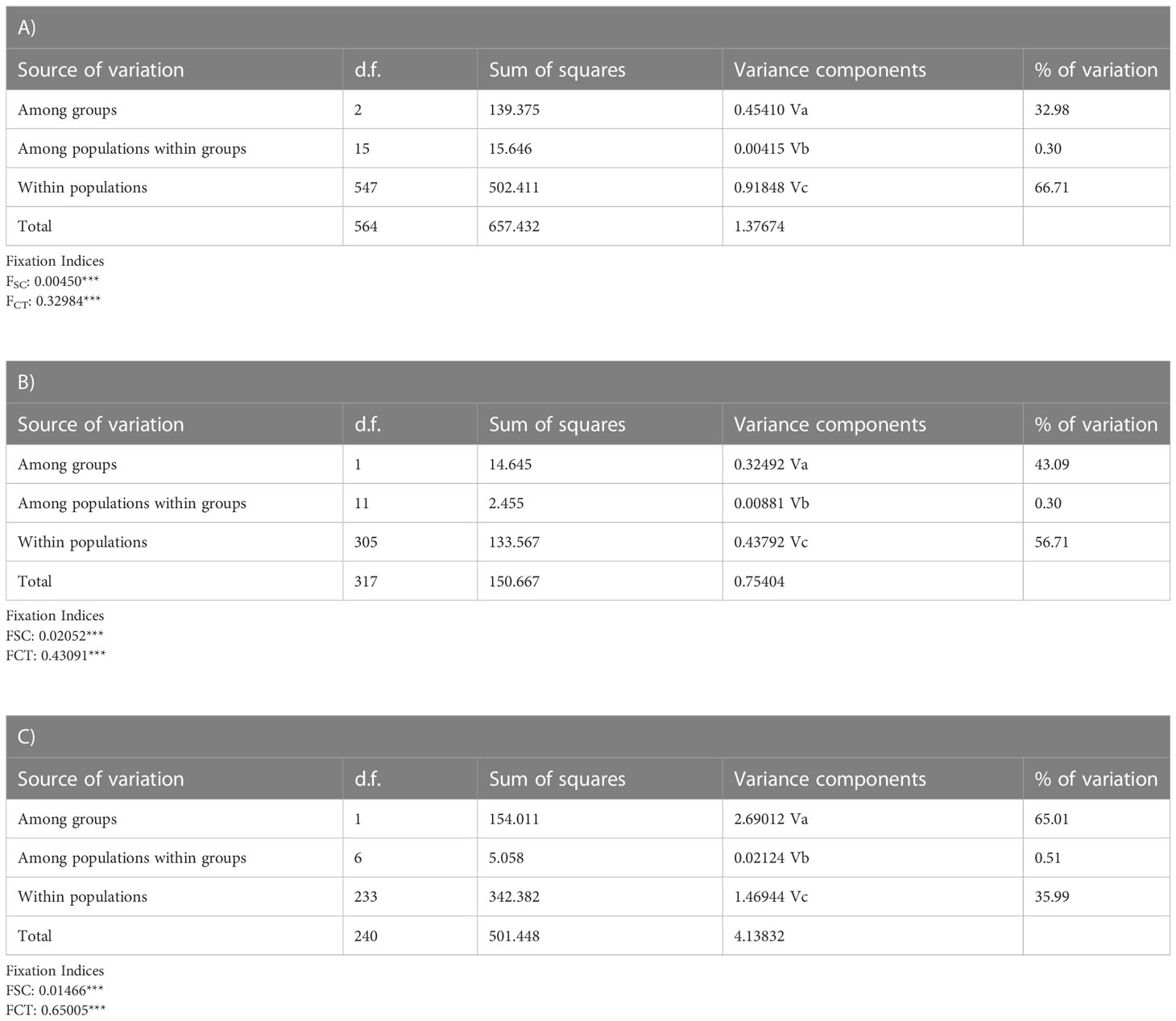
Table 3 Spatial analysis of molecular variance (SAMOVA) in A) Nacella magellanica, B) N. mytilina, and C) N. deaurata depicting the percentage of variation explained among the recognized groups, among populations within groups and within populations. Where FSC = differentiation within populations among groups and FCT = Differentiation among groups (**<p 0.01, *** p<0.001).
Demographic inference in Magellan Nacella species
Network reconstructions: Parsimony network of N. magellanica depicts 79 distinct haplotypes (Figures 3A; S1A) with a typical star-like topology and a short genealogy. The central haplotype (H01) was the most frequent (47.88%) and broadly distributed across the species range, which extends throughout the Magellan province. The most derived haplotype in N. magellanica is related to H01 with a maximum branch length of 9 mutational steps (Figure 3A). A second haplotype (H02) was present in 9.9% of the individuals across the species distribution. Several haplotypes with intermediate frequencies were present in different localities across PM and AM (Figure 3A). The remaining haplotypes occurred at low frequencies and 61 singletons were identified in this species. Several individuals (N = 20) from the F/M shared a dominant haplotype (H03) surrounded by 12 endemic F/M private haplotypes. This dominant F/M haplotype (H03) is separated from the dominant H01 by six mutational steps.
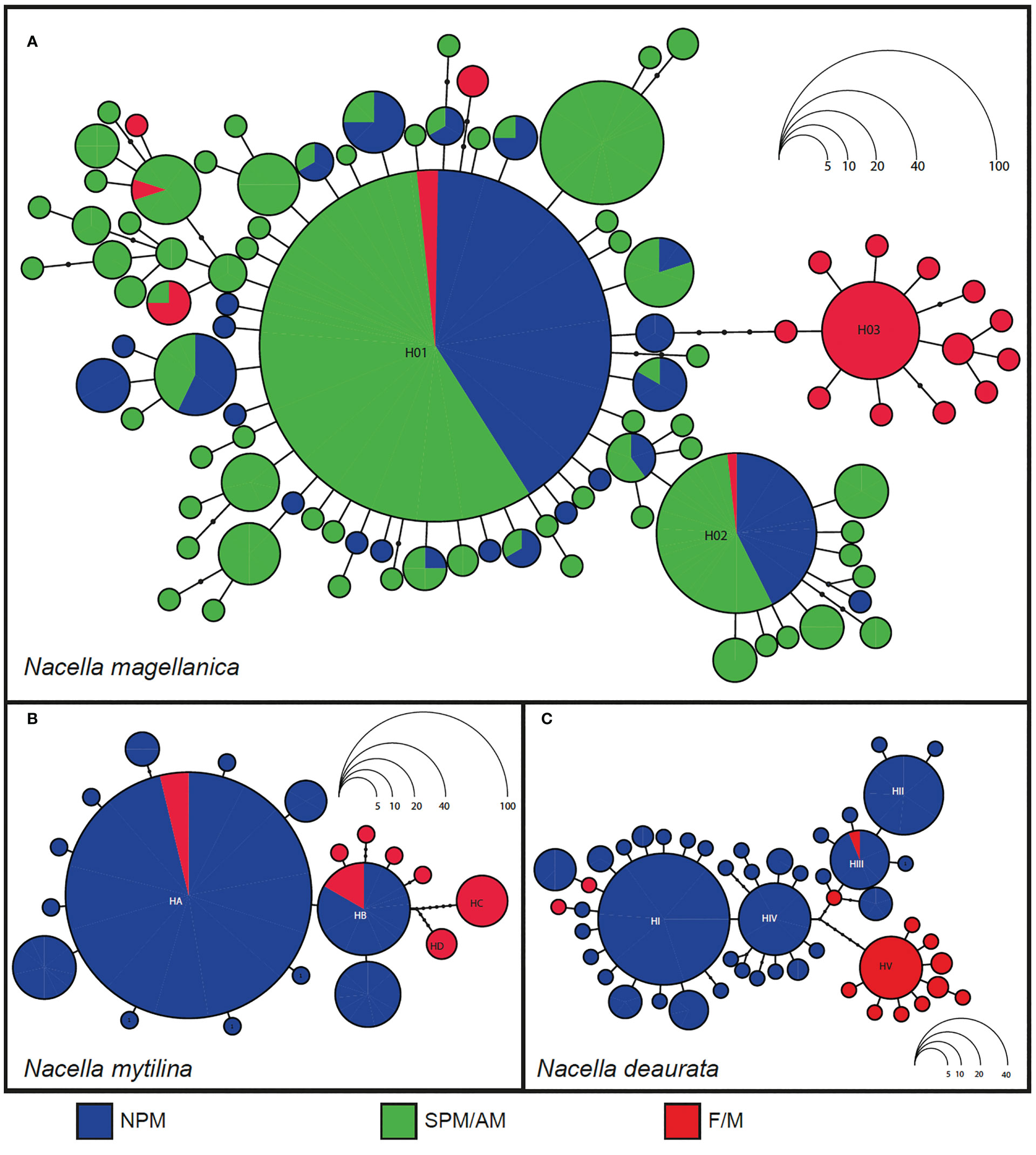
Figure 3 Parsimony mtDNA (COI) haplotype networks in (A) Nacella magellanica, (B) Nacella mytilina, and (C) Nacella deaurata across their distributions in the Magellan Province. Each haplotype is represented by a colored circle indicating the main genetic cluster where it was collected (for each species). Circles sizes are proportional to the frequency of the haplotype in the whole sampling effort. The size of the haplotypes circles (B, C) is proportional to the their frequency. Where NPM, northern Pacific margin; SPM, southern Pacific margin; AM, Atlantic margin; F/M, Falkland/Malvinas Islands.
Maximum parsimony network of N. mytilina included a total of 19 different haplotypes and exhibited a typical star-like topology and a very short genealogy (Figures 3B; S1B). A dominant haplotype (HA) was most frequent one (71%) and was found at all localities (Figure 3B) and the most derived one is related to it with a maximum branch length of 8 mutational steps (HD) (Figure 3B). Another haplotype (HB) of intermediate frequency (10%), was also found in all the analyzed populations (Figure 3B). Several haplotypes were recorded in more than three individuals belonging to different localities across SPM. We identified 10 singletons in N. mytilina, six across SPM and four in F/M (Figure 3B). Several F/M haplotypes are closely related and even shared with the diversity recorded at SPM. Two haplotypes of medium frequency (HC and HD) were only found at F/M and are separated from the dominant H01 by several mutational steps.
Maximum-joining network of N. deaurata included a total of 50 different haplotypes and exhibited a short genealogy with several haplotypes of intermediate frequency (Figures 3C, S1C). A dominant haplotype (HI) showed a frequency of 33.19% and was only recorded at SPM localities from the Strait of Magellan and Cape Horn (Figure 3C). Three medium frequency haplotypes (HII – HIV) were recorded in N. deaurata, two of them (HII and HIV) were also found only in SPM localities while HIII was also found in a single individual from F/M. The most derived haplotype from F/M is related to it with a maximum branch length of 11 mutational steps. Several haplotypes were recorded in more than three individuals belonging to different localities across SPM. We identified 35 singletons in N. deaurata, 25 across PM localities and 10 in F/M (Figure 3C). Four haplotypes in N. deaurata from F/M were closely related and even shared with the diversity recorded in SPM. Nevertheless, a dominant F/M haplotype found in 18 specimens (HV) was separated by eight mutational steps from HI. Again, this dominant F/M haplotype was surrounded by several endemic ones. In contrast to the other analyzed Nacella species, the dominant haplotype found in N. deaurata (HI) was not documented in the F/M.
As expected for a star-like genealogy, global Tajima’s D and Fu’s F neutrality tests were both negative and significant for all the analyzed Magellan Nacella species (Table 1). The distribution of pairwise differences varied among the analyzed Nacella species. The species N. magellanica showed a typical unimodal curve while N. mytilina showed an L-shaped one. In contrast, N. deaurata exhibited a multimodal distribution (Figure 4).
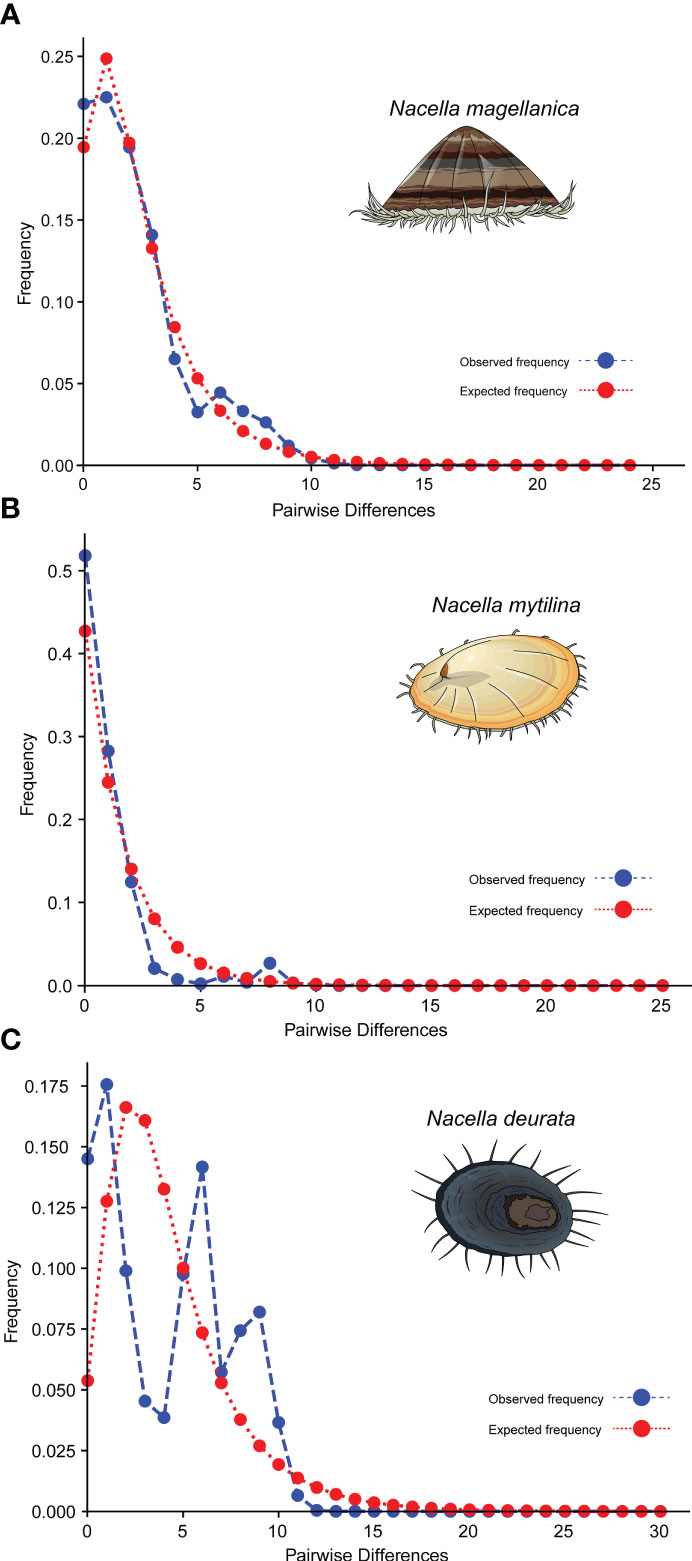
Figure 4 Distribution of pairwise differences for the mtDNA COI gene in (A) Nacella magellanica, (B) Nacella mytilina and (C) Nacella deaurata. X-axis = Pairwise differences and y-axis = frequency.
Demographic reconstructions: Bayesian skyline plot analyses recorded differences in the times of the most recent common ancestor (trmca) and populations expansions between F/M and South American populations in Nacella deaurata and Nacella magellanica (Figure 5). Considering the number of endemic F/M haplotypes of N. mytilina (n = 7), BSP analysis in this population was not conducted. Population expansions of N. magellanica and N. deaurata at the F/M occurred around 15,000 and 14,000 years ago, respectively. Population expansions in N. deaurata and N. mytilina in southern SPM occurred around 9,000 and 7,000 years ago. In the case of N. magellanica, the tmrca of SPM occurred around 18,000 years ago while SAM populations expanded around 15,000 years ago. Results on populations sizes estimations per species/groups are presented in Supplementary Table 4.
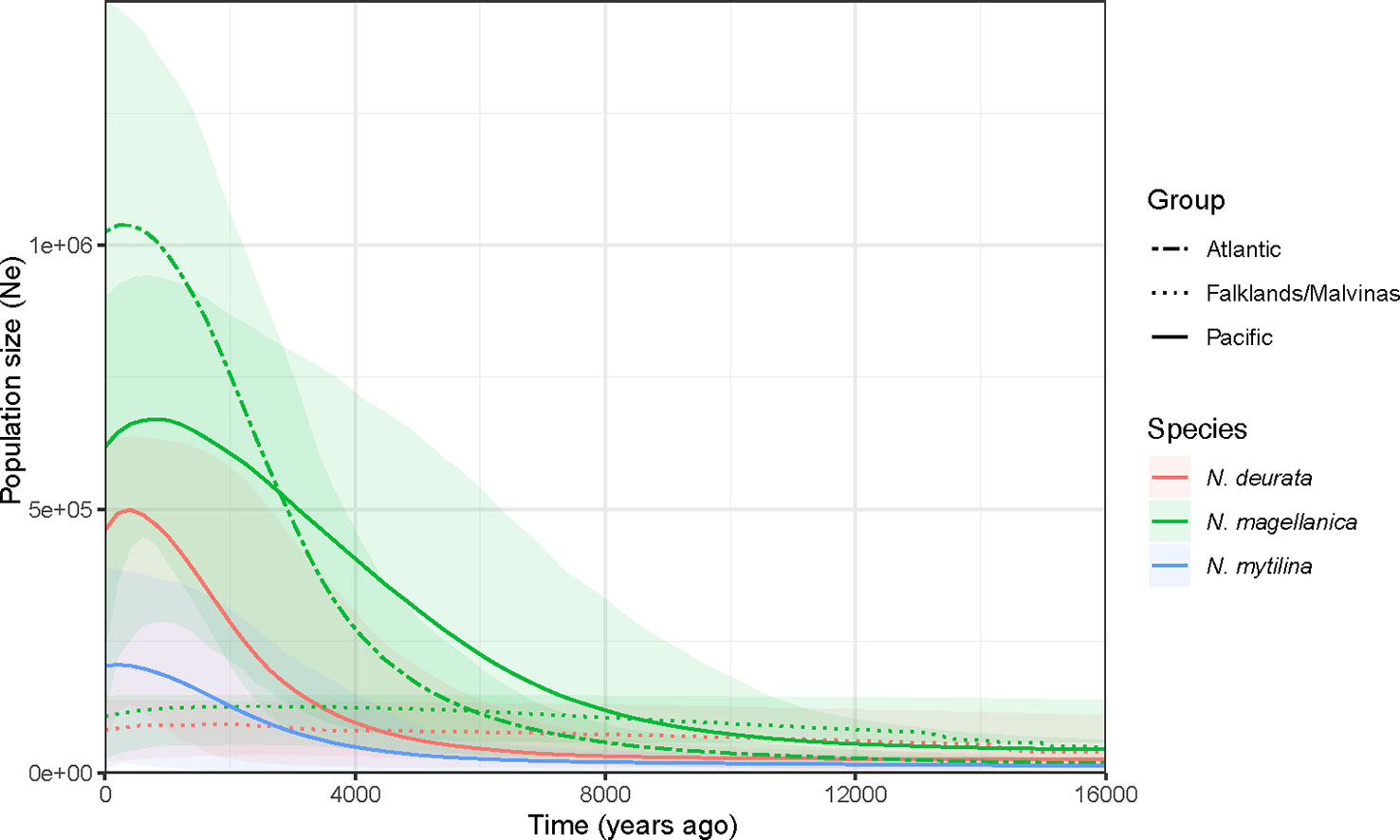
Figure 5 Historical demographic trajectories of the effective population sizes (Ne) constructed using a Bayesian skyline plot approach based on COI haplotypes of N. magellanica (green), N. mytilina (red) and N. deaurata (red) along their distribution in the Magellan province (southern South America and the Falkland/Malvinas Islands). The y-axis is the product of effective population size (Ne) and generation length in a log scale while the x-axis is the time before present. The median estimate are shown for each of the genetic groups recorded in the analyzed species.
Gene flow in Magellan Nacella species
Gene flow analyses using different migration models detected evidence of asymmetrical gene flow from SPM to the F/M in the species N. deaurata and N. mytilina. We chose a stepping-stone model for N. magellanica including either symmetric migration from northern PM localities (NPM) towards SPM/AM (Cluster 2) or asymmetrical migration from SPM/AM towards F/M (Table 4).
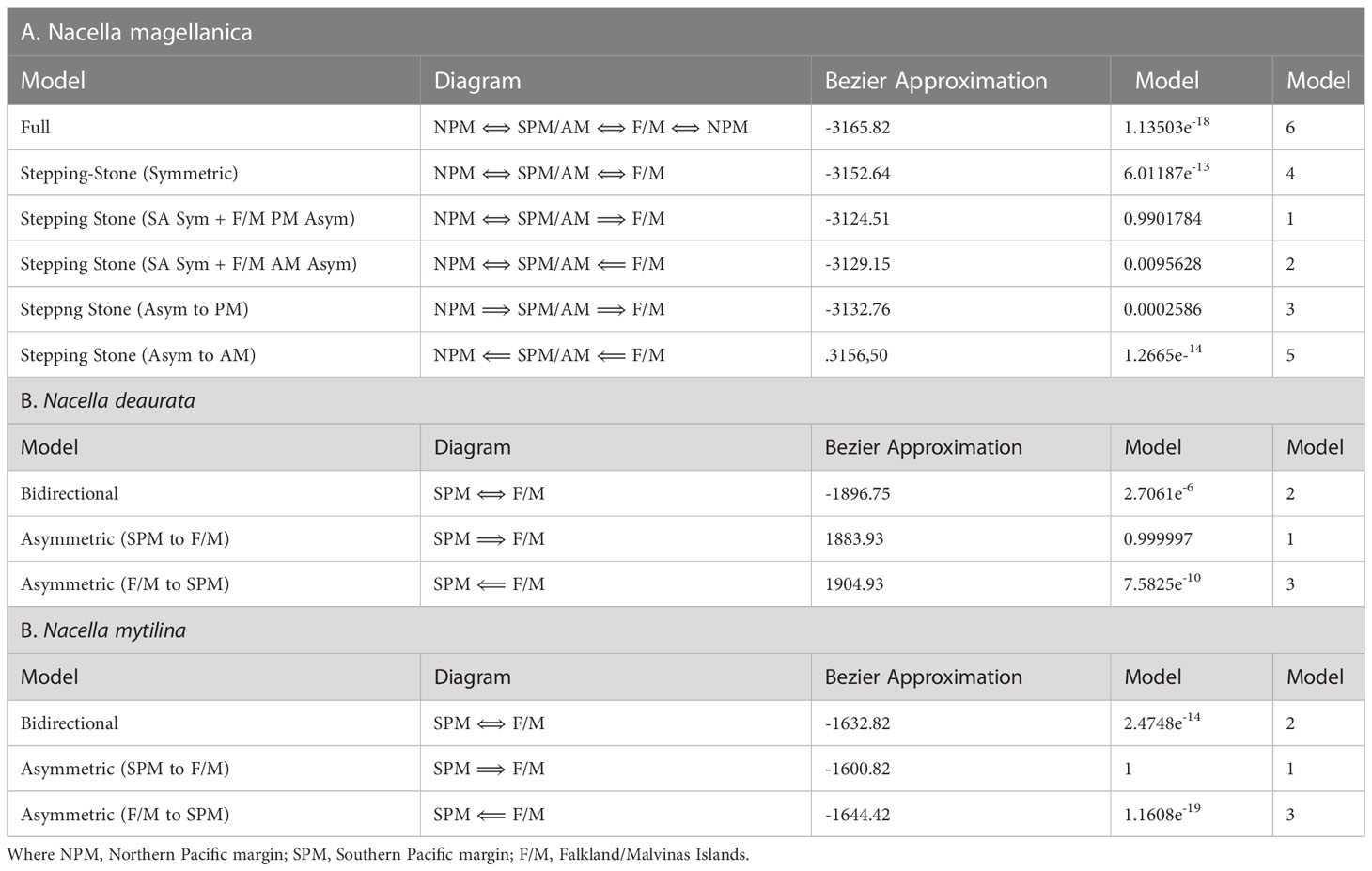
Table 4 Gene flow model selection for the analyzed Nacella species considering their respective genetic clusters across the Magellan province.
Discussion
Reconstructions of how antarctic and sub-antarctic species or ecosystems responded to Quaternary glacial cycles can help understand the evolution and biogeography of this biota during the last million years. These reconstructions also provide key information concerning their potential responses to future climate change (Fraser et al., 2010; Fraser et al., 2012; González-Wevar et al., 2012; González-Wevar et al., 2013; Poulin et al., 2014; Riesgo et al., 2015; González-Wevar et al., 2016a; Moon et al., 2017; Halanych and Mahon, 2018; González-Wevar et al., 2021). Major landscape and seascape changes along the Magellan fjords and channels of southern South America resulted in the sporadic eradication of the associated fauna across these ecosystems (Valdovinos et al., 2003; Zattara and Premoli, 2005; Kiel and Nielsen, 2010). During the last two decades, molecular-based studies have assumed an essential role for unravelling the relationship between Quaternary climate shifts and the distribution and demography of species and populations (Hewitt, 2004; Maggs et al., 2008; Provan and Bennett, 2008; Marko et al., 2010). In this study we performed population-based mtDNA genetic analyses in three Magellan near-shore patellogastropods species of the genus Nacella (N. deaurata, N. magellanica and N. mytilina) to understand the role of Quaternary climatic oscillations over their patterns of genetic diversity and structure. As a general pattern, the three species of Nacella analyzed here showed classical signals of the Expansion-Contraction Quaternary biogeography model with low levels of genetic diversity, absence of genetic structure across broad geographical distances and strong signals of recent post-glacial demographic expansion across their respective distributions. Such results have also been found in other groups of Magellan marine near-shore organisms including macroalgae (Fraser et al., 2010; Macaya and Zuccarello, 2010; Billard et al., 2015), mollusks (Nuñez et al., 2015; González-Wevar et al., 2017; González-Wevar et al., 2018; González-Wevar et al., 2019; Pardo-Gandarillas et al., 2018; Fernández Iriarte et al., 2020; González-Wevar et al., 2021) and fishes (Ceballos et al., 2016; Segovia et al., 2022). Moreover, similar demographic patterns have also been recorded in higher latitude marine invertebrates from Antarctica (Wilson et al., 2009; Allcock and Strugnell, 2012; González-Wevar et al., 2013), the sub-Antarctic (Fraser et al., 2009; Moon et al., 2017; González-Wevar et al., 2019; González-Wevar et al., 2021), New Zealand (Goldstien et al., 2006), Europe (Hewitt, 2000; Hewitt, 2004; Maggs et al., 2008; Villamor et al., 2014) and North America (Marko et al., 2010). Levels of genetic diversity in Magellan Nacella species ranged from medium (N. magellanica) to low (N. deaurata and N. mytilina) compared to temperate (Nakano and Ozawa, 2005; Goldstien et al., 2006; Sá Pinto et al., 2007) and tropical (Bird et al., 2007) patellogastropods but higher (in most of the cases) than in the Antarctic relative N. concinna (González-Wevar et al., 2013; de Aranzamendi et al., 2022). While levels of genetic polymorphism recorded in N. mytilina are lower than those found in the antarctic N. concinna, they are comparable to those in other sub-antarctic nacellid limpets from New Zealand (Reisser et al., 2011) and Marion Island. As proposed by González-Wevar et al. (2016a) exceptionally low levels of mtDNA genetic diversity in this species could be associated with its strong habitat dependency on macroalgae. Post-glacial recolonization of N. mytilina populations would have required the previous settlement of macroalgae. Accordingly, the post-glacial expansion in N. mytilina would have been hindered or delayed compared to the other Magellan species analyzed here. The glacial history of the region together with the larval-mediated dispersal potential of the analyzed species are strongly associated to their respective patterns of genetic diversity and structure. In concert with the dispersal potential, we found evidence of rapid postglacial recolonization processes across a very complex landscape likely related to deglaciation processes across the species’ distributions. As estimated trough BSP analyses, phylogeographic patterns in sub-Antarctic Magellan species of Nacella are probably related to genetic drift processes driven by demographic bottlenecks during glacial maxima, and particularly to the Last Glacial Maximum. After the LGM, Nacella populations suffered postglacial expansions following the deglaciation processes across their respective distributions. Our BSP demographic estimations in Nacella are in basic agreement with the timing of the LGM extent and the onset of the deglaciation process in the Magellan province, a process that occurred synchronously across the region (Hein et al., 2010). The final advances of ice in northern areas of Pacific Patagonia are dated about 18,000 years ago (Heusser et al., 1998; Heusser et al., 1999; Moreno et al., 1999) and the warming began 17,500 years ago (Moreno and León, 2003). The final advances of ice in the Strait of Magellan occurred around 17,000 years ago (McCulloch et al., 2005) while a fast-warming period occurred between 14,000 and 10,000 years ago (De Pol-Holz et al., 2006).
Contrary to our expectations, populations of N. magellanica along temperate areas of the Atlantic (AM), an area considered as less impacted by ice during glacial periods, did not exhibit higher genetic diversity than those populations located inside areas that were heavily impacted by ice across PM and they seem to represent a continuity of the diversity found in the SPM (Strait of Magellan and Cape Horn). A plausible explanation for these results in the Atlantic margin relies in the fact that during the LGM the level of the sea was between 120 and 140 m below its present level (Fleming et al., 1998). During glacial maxima a large portion of the Atlantic Continental Shelf (ACS) was exposed with the development of major plains dominated by depositional environments and sandy environments (Rabassa et al., 2005; Ponce et al., 2011; Rabassa et al., 2011; Violante et al., 2014). As proposed for the intertidal pulmonate limpet Siphonaria lessonii (Fernández Iriarte et al., 2020) and for the mussel Perumytilus purpuratus (Trovant et al., 2015), suitable hard substrate habitat for N. magellanica during glacial maxima would have been strongly reduced south of 46°S in Tierra del Fuego. Accordingly, refuges for N. magellanica would have been more available in the southern tip of the southwest Atlantic, from where the species could have recolonized lower latitudes following the deglaciation process. As proposed by Fernández Iriarte et al. (2020) phylogeographical patterns in broadly distributed near-shore Magellan species are not essentially related to Quaternary glacial continental ice expansions and contractions but also to the availability of habitats for the species.
Each of the analyzed Nacella species are characterized by star-like genealogies with the presence of a dominant and widely distributed haplotype surrounded by several unique, low- and medium-frequency ones. Such demographic patterns are consistent on the one hand of the strong demographic impact of glacial processes resulting in the reduction of their populations sizes through repetitive bottleneck events during the coolest periods of the Quaternary. Moreover, broadly distributed haplotypes recorded in Magellan Nacella species are also consistent with the hypothesis of recent range expansion and high levels of migration. In this context, the absence of structure across large geographical areas, the presence of dominant haplotypes, and the absence of correlation between geographic and genetic distances support the hypothesis of recent expansions of these patellogastropods, probably mediated by their planktonic dispersal as larvae reflecting the general oceanographic circulation patterns of the study area (Escribano et al., 2003; Acha et al., 2004; Alemany et al., 2009; Bustos et al., 2011). Finally, significant negative Tajima’s D and Fu’s FS indices are the result of an excess of low frequency haplotypes, commonly explained by recent demographic processes, as evidenced here in the analyzed Nacella species through Bayesian skyline plots reconstructions.
Main patterns of genetic structure recorded across the Magellan province in marine organisms includes a) absence or moderate genetic differentiation between South American populations and the Falkland/Malvinas Islands (M/F) (Fernández Iriarte et al., 2020; González-Wevar et al., 2021; González-Wevar et al., 2022; Segovia et al., 2022), b) strong phylogeographic signaling between PM and F/M (Macaya and Zuccarello, 2010; González-Wevar et al., 2012; González-Wevar et al., 2016a; González-Wevar et al., 2018) and c) the presence of different species in the F/M (Leese et al., 2008; González-Wevar et al., 2019; González-Wevar et al., 2022). All the Nacella species analyzed here showed strong phylogeographic structure between SPM/AM and F/M supporting previous studies in near-shore Magellan marine organisms. As proposed by Leese et al. (2008), even when the F/M marine biota has often been considered as part of the Magellan inventory, molecular analyses are showing that F/M populations may be strongly differentiated populations and/or even reproductive isolated units. Moreover, F/M populations of N. mytilina and N. magellanica showed higher levels of genetic diversity than PM and AM populations but this pattern was not detected in N. deaurata. As previously proposed in Nacella, the marked differences in diversity and structure between SPM and F/M populations may be associated with the different glaciological trajectories of these areas during the coldest of the Quaternary glaciations (González-Wevar et al., 2012; González-Wevar et al., 2016a; Fernández Iriarte et al., 2020). The SPM was almost completely covered by ice during the LGM and hence the shallow marine ecosystems would have been severely reduced across this area. Thus, marked decline in genetic diversity associated with repeated rounds of bottleneck events and/or founder effects is expected as recorded in several marine near-shore species (González-Wevar et al., 2011; González-Wevar et al., 2012; González-Wevar et al., 2015b; González-Wevar et al., 2016b; González-Wevar et al., 2018; de Aranzamendi et al., 2014; Güller et al., 2015; González-Wevar et al., 2021; González-Wevar et al., 2022; Nuñez et al., 2011; Nuñez et al., 2015; Segovia et al., 2022). In contrast, in the F/M there is little evidence of glacial ice accumulation apart from small cirques and short glacially eroded valleys (Clapperton and Sugden, 1976; Clapperton, 1994; Hodgson et al., 2014). In fact, cosmogenic isotopes surface exposure dates suggest an absence of widespread glaciations at altitude during the LGM and there is no evidence for glaciers extending offshore in these islands (Clark et al., 1998; Wilson et al., 2002; Wilson et al., 2008). Under such glaciological scenarios, our results in Nacella species could be explained through contrasting demographical histories between populations from areas that were dramatically affected by continental ice across PM and those populations located in non-glaciated areas such as F/M. Quaternary genetic models include the prediction that glacial refugia should exhibit a longer demographic history with higher levels of genetic diversity (Provan and Bennett, 2008). Nevertheless, the higher genetic diversity and longer demographic histories recorded in Nacella populations from the F/M may also be a consequence of the asymmetric gene flow from South America towards the F/M. Evidence of these interpretation is provided by the presence of endemic F/M haplotypes that survived the LGM in situ and are found together with recently arrived South American ones. Following this explanation, the F/M could be considered as a glacial refugium for the species analyzed here, comprised of a sink area together with a secondary contact zone (González-Wevar et al., 2012; González-Wevar et al., 2016a).
In marine ecosystems, dispersal plays a key role in providing connectivity of populations, preventing speciation, species persistence despite temporal/local extinctions, and recruitment to potentially favorable environments (Ayre et al., 2009). For benthic organisms with low autonomous mobility the dispersal potential is usually related to the earliest life history stages and the processes that influence developmental modes, types of larvae, larval development, and lifespan (Strathmanns, 1985; Palumbi, 1994; Poulin et al., 2002; Marko, 2004; Cowen and Sponaugle, 2009). Accordingly, in these patellogastropods the duration of the dispersive stages is expected to correlate with the dispersal potential and with the patterns of genetic structure (Paulay et al., 2006; Miller and Ayre, 2008; Ayre et al., 2009; Puritz et al., 2017). Absence of genetic structure across the PM has been found in different marine organisms with dispersal potential (Toro et al., 2004; Ceballos et al., 2012; González-Wevar et al., 2012; González-Wevar et al., 2016a, b; Hüne et al., 2015; González-Wevar et al., 2018; Fernández Iriarte et al., 2020; González-Wevar et al., 2021; Segovia et al., 2022). Currently, there is no information concerning the larval duration in Magellan Nacella species but their developments should be similar to the Antarctic relative N. concinna (Bowden et al., 2009; Peck et al., 2016) and extends for at least six weeks. A main asymmetrical dispersal pattern from South America to F/M found in the Nacella species analyzed here is probably associated with the oceanographical circulation patterns in the Magellan province, as well as by the reproductive biology and the ecology of these species. The Cape Horn Current (CHC), a southward branch of the West Wind Drift, flows around Cape Horn and is responsible for the main oceanographic regime in this province. After surrounding the southern tip of South America, the CHC is divided into two minor branches, one flows east towards South Georgia and the second one flows northward on both sides of the F/M (Knox, 1960; Brattström and Johanssen, 1983; Bastida et al., 1992; Acha et al., 2004). Accordingly, gene flow mediated by larval dispersal, and/or rafting in the case of N. mytilina, may be favored by the main oceanographic conditions in southern South America.
Conclusions
The results of our mtDNA-based phylogeographic analyses for three Magellan Nacella species have provided evidence that historical and contemporary processes have played key roles in explaining the current patterns of genetic diversity, population structure and connectivity by larval dispersal. The main phylogeographic footprints revealed for these Magellan Nacella species include low levels of genetic diversity, the presence of broadly distributed dominant haplotypes and recent post-glacial demographic expansions. Together, these provide further evidence for the Expansion-Contraction (EC) model of Quaternary biogeography proposed by Provan and Bennett (2008). At the same time, the phylogeographic structure patterns revealed marked genetic differences between South American populations and those from the Falkland/Malvinas. Even in the presence of asymmetric levels of gene flow from South America towards the Falkland/Malvinas Islands, the migration rate is not enough to homogenize populations and they constitute different genetic units in all the Nacella species analyzed here. Contrary to our expectations, N. magellanica populations from the Atlantic margin, an area that was less impacted by ice during glacial maxima, did not exhibit higher levels of genetic diversity than heavily ice impacted populations from the Pacific margin. The phylogeographic patterns appear best explained by the interplay of ecological factors including habitat availability, restriction to shallow depths, and obligate planktonic dispersal facilitated by strong but directional currents.
Data availability statement
New COI sequences in Magellan Nacella species have been deposited in GenBank under the following accession numbers: OQ587619–OQ587817. Additionally, we included previously published sequences under the GenBank Accession Numbers: EU870927–EU870985, HQ880439–HQ880550; JX262742–JX262797 for N. magellanica; EU870986–EU0870999; HQ880551–HQ880573 for N. deaurata and KX600474–KX600492 for N. mytilina (de Aranzamendi et al., 2009; de Aranzamendi et al., 2011; González-Wevar et al., 2012; González-Wevar et al., 2016a).
Author contributions
CG-W, MA, EP, CG, RB, CRM, and NS conceived the idea of the study and designed the study. CG-W, SR, EP, PB, MA collected Nacella specimens from different localities across their distribution in the Magellan province. CG-W, SR, CSM, and MA performed molecular experiments. CG-W, NS, SR, CSM, CRM conduced phylogeographic analyses and wrote the manuscript. CG-W, EP, NS, CRM, MA, and SR contributed intellectually to the interpretation and discussion of results. All authors contributed to the article and approved the submitted version.
Funding
This study was funded by Fondecyt Regular Project 1210787, ANID – Millennium Science Initiative Program – ICN2021_002, and Program FONDAP (15150003) to CG-W. Fondecyt Regular Project 1151336 to EP and CG-W and Fondecyt Postdoctoral 3210063 to CSM. Program PR-06-CRN-18 (Universidad de Magallanes) to SR.
Acknowledgments
Many thanks to Angie Díaz, Jaime Ojeda, Mathias Hüne, Javier Naretto, and Karin Gérard, for field assistance and samplings in southern South America. Nacella specimens were captured under the Chilean legislation (Technical Memorandum P.INV N° 258/2021 SUBPESCA). We are mostly grateful to the collaboration of the South Atlantic Environmental Research Institute (SAERI) and the Shallow Marine Surveys Group of the collection of Nacella specimens in the Falkland Islands.
Conflict of interest
The authors declare that the research was conducted in the absence of any commercial or financial relationships that could be construed as a potential conflict of interest.
Publisher’s note
All claims expressed in this article are solely those of the authors and do not necessarily represent those of their affiliated organizations, or those of the publisher, the editors and the reviewers. Any product that may be evaluated in this article, or claim that may be made by its manufacturer, is not guaranteed or endorsed by the publisher.
Supplementary material
The Supplementary Material for this article can be found online at: https://www.frontiersin.org/articles/10.3389/fmars.2023.1154755/full#supplementary-material
References
Acha E. M., Mianzan H. W., Guerrero R. A., Favero M., Bava J. (2004). Marine fronts at the continental shelves of austral south America. J. Mar. Syst. 44, 83–105. doi: 10.1016/j.jmarsys.2003.09.005
Alemany D., Acha E. M., Iribarne O. (2009). The relationship between marine fronts and fish diversity in the Patagonian shelf Large marine ecosystem. J. Biogeogr. 36, 2111–2124. doi: 10.1111/j.1365-2699.2009.02148.x
Aljanabi S. M., Martinez I. (1997). Universal and rapid salt-extraction of high quality genomic DNA for PCR-based techniques. Nucleic Acids Res. 25, 4692–4693. doi: 10.1093/nar/25.22.4692
Allcock A. L., Strugnell J. M. (2012). Southern ocean diversity: new paradigms from molecular ecology. Trends Ecol. Evol. 27, 520–528. doi: 10.1016/j.tree.2012.05.009
Ayre D. J., Minchinton T. E., Perrin C. (2009). Does life history predict past and current connectivity for rocky intertidal invertebrates across a marine biogeographic barrier? Mol. Ecol. 18, 1887–1903. doi: 10.1111/j.1365-294X.2009.04127.x
Bastida R., Roux A., Martínez D. E. (1992). Benthic communities of the Argentine continental shelf. Oceanol. Acta 15, 687–698.
Beerli P., Felsenstein J. (2001). Maximum likelihood estimation of a migration matrix and effective population sizes in n subpopulations by using a coalescent approach. Proc. Natl. Acad. Sci. U.S.A. 98, 4563–4568. doi: 10.1073/pnas.081068098
Beerli P., Palczewski M. (2010). Unified framework to evaluate panmixia and migration direction among multiple sampling locations. Genetics 185, 313–326. doi: 10.1534/genetics.109.112532
Bennett K. D., Tzedakis K., Willis J. (1991). Quaternary refugia of north European trees. J. Biogeogr. 18, 103–115. doi: 10.2307/2845248
Billard E., Reyes J., Mansilla A., Faugeron S., Guillemin M.-L. (2015). Deep genetic divergence between austral populations of the red alga gigartina skottsbergii reveals a cryptic species of endemic to the Antarctic continent. Polar Biol. 38, 2021–2034. doi: 10.1007/s00300-015-1762-4
Bird C. E., Holland B. S., Bowen B. W., Toonen R. J. (2007). Contrasting phylogeography in three endemic Hawaiian limpets (Cellana spp.) with similar life histories. Mol. Ecol. 16, 3173–3186. doi: 10.1111/j.1365-294X.2007.03385.x
Bowden D. A., Clarke A., Peck L. S. (2009). Seasonal variation in the diversity and abundance of pelagic larvae of Antarctic marine invertebrates. Mar. Biol. 156, 2033–2047. doi: 10.1007/s00227-009-1235-9
Brattström H., Johanssen A. (1983). Ecological and regional zoogeography of the marine benthic fauna of Chile. Sarsia 68, 289–339. doi: 10.1080/00364827.1983.10420583
Bustos C. A., Landaeta M. F., Balbontín F. (2011). Ichthyoplankton spatial distribution and its relation with water column stratification in fjords of southern Chile (46°48’- 50°09’S) in austral spring 1996 and 2008. Cont. Shelf Res. 31, 293–303. doi: 10.1016/j.csr.2010.03.013
Ceballos S. G., Lessa E. P., Licandeo R., Fernández D. A. (2016). Genetic relationships between Atlantic and pacific populations of the notothenioid fish Eleginops maclovinus: the footprints of quaternary glaciations in Patagonia. Heredity 116, 372–377. doi: 10.1038/hdy.2015.106
Ceballos S. G., Lessa E. P., Victorio M. F., Fernández D. A. (2012). Phylogeography of the sub-Antarctic notothenioid fish Eleginops maclovinus: evidence of population expansion. Mar. Biol. 159, 499–505. doi: 10.1007/s00227-011-1830-4
Clapperton C. M. (1994). The quaternary glaciation of Chile: a review. Rev. Chil. Hist. Nat. 67, 369–383.
Clapperton C. M., Sugden D. E. (1976). The maximum extent of glaciers in part of west Falkland. J. Glaciol. 17, 73–77. doi: 10.3189/S0022143000030732
Clark R., Huber U. M., Wilson P. (1998). Late pleistocene sediments and environmental change at plaza creek, Falkland islands, south Atlantic. J. Quat. Sci. 13, 95–105. doi: 10.1002/(SICI)1099-1417(199803/04)13:2<95::AID-JQS351>3.0.CO;2-G
Cowen R. K., Sponaugle S. (2009). Larval dispersal and marine population connectivity. Ann. Rev. Mar. Sci. 1, 443–446. doi: 10.1146/annurev.marine.010908.163757
de Aranzamendi M. C., Bastida R., Gardenal C. N. (2014). Genetic population structure in Nacella magellanica: evidence of rapid range expansion throughout the entire species distribution on the Atlantic coast. J. Exp. Mar. Biol. Ecol. 460, 53–61. doi: 10.1016/j.jembe.2014.06.008
de Aranzamendi M. C., Bastidas. R., Gardenal C. (2011). Different evolutionary histories in two sympatric limpets of the genus Nacella (Patellogastropoda) in the south-western Atlantic coast. Mar. Biol. 158, 2405–2418. doi: 10.1007/s00227-011-1742-3
de Aranzamendi M. C., Gardenal C. N., Martin J. P., Bastida R. (2009). Limpets of genus Nacella (Patellogastropoda) from the southwestern Atlantic: species identification based on molecular data. J. Mollusc. Stud. 75, 241–251. doi: 10.1093/mollus/eyp025
de Aranzamendi M. C., Martínez J. J., Held C., Sahade R. (2022). Parallel shape divergence between ecotypes of the limpet nacella concinna along the Antarctic peninsula: a new model species for parallel evolution. Zoology 150, 125983. doi: 10.1016/j.zool.2021.125983
De Pol-Holz R., Ulloa O., Dezileau L., Kaiser J., Lamy F., Hebbeln D. (2006). Melting of the Patagonian ice sheet and deglacial perturbations of the nitrogen cycle in the eastern south pacific. Geophys. Res. Lett. 33, L04704. doi: 10.1029/2005GL024477
Drummond A. J., Rambaut A. (2007). BEAST: Bayesian evolutionary analysis by sampling trees. BMC Evol. Biol. 7, 214. doi: 10.1186/1471-2148-7-214
Drummond A. J., Suchard M. A., Xie D., Rambaut A. (2012). Bayesian Phylogenetics with BEAUti and the BEAST 1.7. Mol. Biol. Evol. 29, 1969–1973. doi: 10.1093/molbev/mss075
Dupanloup I., Schneider S., Excoffier L. (2002). A simulated annealing approach to define the genetic structure of populations. Mol. Ecol. 11, 2571–2581. doi: 10.1046/j.1365-294x.2002.01650.x
Edgar R. C. (2004). MUSCLE: a multiple sequence alignment method with reduced time and space complexity. BMC Bioinf. 5, 113. doi: 10.1186/1471-2105-5-113
Escribano R., Fernández M., Aranis A. (2003). Physical-chemical processes and patterns of diversity of the Chilean eastern boundary pelagic and benthic marine ecosystems: an overview. Gayana 67, 190–205. doi: 10.4067/S0717-65382003000200008
Excoffier L., Laval G., Schneider S. (2005). Arlequin (version 3.0): an integrated software package for population genetics data analysis. Evol. Bioinform. Online 1, 37–50. doi: 10.1177/117693430500100003
Fernández Iriarte P. J., González-Wevar C. A., Segovia N. I., Rosenfeld S., Hüne M., Fainburg L., et al. (2020). Quaternary ice sheets and sea level regression drove divergence in a marine gastropod along Eastern and Western coasts of south America. Sci. Rep. 10, 844. doi: 10.1038/s41598-020-57543-4
Fleming K., Johnston P., Zwartz D., Yokoyama Y., Lambeck K., Chappell J. (1998). Refining the eustatic sea-level curve since the last glacial maximum using far– and intermediate–field sites. Earth Planet. Sci. Lett. 163, 327–342. doi: 10.1016/S0012-821X(98)00198-8
Folmer O., Black M., Hoeh W., Lutz R., Vrijenhoek R. (1994). DNA Primers for amplification of mitochondrial cytochrome c oxidase subunit I from diverse metazoan invertebrates. Mol. Mar. Biotech. 3, 294–299.
Forster P., Torroni A., Renfrew C., Röhl A. (2001). Phylogenetic star contraction applied to Asian and papuan mtDNA evolution. Mol. Biol. Evol. 18, 1864–1881. doi: 10.1093/oxfordjournals.molbev.a003728
Fraser C. I., Nikula R., Ruzzante D. E., Waters J. M. (2012). Poleward bound: biological impacts of southern hemisphere glaciation. Trends Ecol. Evol. 27, 462–471. doi: 10.1016/j.tree.2012.04.011
Fraser C. I., Nikula R., Spencer H. G., Waters J. M. (2009). Kelp genes reveal effects of subantarctic sea during the last glacial maximum. Proc. Natl. Acad. Sci. U.S.A. 106, 3249–3253. doi: 10.1073/pnas.0810635106
Fraser C. I., Thiel M., Spencer H. G., Waters J. M. (2010). Contemporary habitat discontinuity and historic glacial ice drive genetic divergence in Chilean kelp. BMC Evol. Biol. 10, 203. doi: 10.1186/1471-2148-10-203
Glasser N., Jansson K. (2008). The glacial map of southern south America. J. Maps 4, 175–196. doi: 10.4113/jom.2008.1020
Goldstien S. J., Schiel D. R., Gemmel N. J. (2006). Comparative phylogeography of coastal limpets across a marine disjunction in new Zealand. Mol. Ecol. 15, 3259–3268. doi: 10.1111/j.1365-294X.2006.02977.x
González-Wevar C. A., Chown S. L., Morley S., Coria N., Saucéde T., Poulin E. (2016b). Out of Antarctica: quaternary colonization of sub-Antarctic Marion island by the limpet genus Nacella (Patellogastropoda: nacellidae). Polar Biol. 39, 77–89. doi: 10.1007/s00300-014-1620-9
González-Wevar C. A., Hüne M., Cañete J. I., Mansilla A., Nakano T., Poulin E. (2012). Towards a model of postglacial biogeography in shallow marine species along the Patagonian province: lessons from the limpet Nacella magellanica (Gmelin 1791). BMC Evol. Biol. 12, 139. doi: 10.1186/1471-2148-12-139
González-Wevar C. A., Hüne M., Segovia N. I., Nakano T., Spencer H. G., Chown S. L., et al. (2017). Following the Antarctic circumpolar current: patterns and processes in the biogeography of the limpet Nacella (Mollusca: patellogastropoda) across the southern ocean. J. Biogeogr. 44, 861–874. doi: 10.1111/jbi.12908
González-Wevar C. A., Nakano T., Cañete J. I., Poulin E. (2010). Molecular phylogeny and historical biogeography of Nacella (Patellogastropoda: nacellidae) in the southern ocean. Mol. Phylogenet. Evol. 56, 115–124. doi: 10.1016/j.ympev.2010.02.001
González-Wevar C. A., Nakano T., Cañete J. I., Poulin E. (2011). Concerted genetic, morphological and ecological diversification in Nacella limpets in the magellanic province. Mol. Ecol. 20, 1936–1951. doi: 10.1111/j.1365-294X.2011.05065.x
González-Wevar C. A., Rosenfeld S., Segovia N. I., Hüne M., Gérard K., Ojeda J., et al. (2016a). Genetics, gene flow, and glaciation: the case of the south American limpet Nacella mytilina. PloS One 11, e0161963. doi: 10.1371/journal.pone.0161963
González-Wevar C. A., Salinas P., Hüne M., Segovia N. I., Vargas-Chacoff L., Astorga M., et al. (2015b). Phylogeography in Galaxias maculatus (Jenyns 1848) along two biogeographical provinces in the Chilean coast. PloS One 10 (7), e0131289. doi: 10.1371/journal.pone.0131289
González-Wevar C., Salinas P., Hüne M., Segovia N., Vargas-Chacoff L., Oda E., et al. (2015a). Contrasting genetic structure and diversity of Galaxias maculatus (Jenyns 1848) along the Chilean coast: stock identification for fishery management. J. Hered. 106, 439–447. doi: 10.1093/jhered/esv005
González-Wevar C. A., Saucède T., Morley T., Chown S. L., Poulin E. (2013). Extinction and recolonization of maritime Antarctica in the limpet Nacella concinna (Strebel 1908) during the last glacial cycle: toward a model of quaternary biogeography in shallow Antarctic invertebrates. Mol. Ecol. 22, 5221–5236. doi: 10.1111/mec.12465
González-Wevar C. A., Segovia N. I., Rosenfeld S., Maturana C. S., Jeldres V., Pinochet R., et al. (2022). Seven snail species hidden in one: biogeographic diversity in an apparently widespread periwinkle in the southern ocean. J. Biogeogr 49, 1521–1534. doi: 10.1111/jbi.14453
González-Wevar C. A., Gérard K., Rosenfeld S., Saucède T., Naretto J., Díaz A., et al. (2019). Cryptic speciation in Southern Ocean Aequiyoldia eightsii (Jay, 1839): Mio-Pliocene trans-Drake Passage separation and diversification. Prog. Oceanogr 174, 44–54. doi: 10.1016/j.pocean.2018.09.004
González-Wevar C. A., Segovia N. I., Rosenfeld S., Noll D., Maturana C. S., Hüne M., et al. (2021). Contrasting biogeographical patterns in Margarella (Gastropoda: calliostomatidae: margarellinae) across the Antarctic polar front. Mol. Phylogenet. Evol. 156, 107039. doi: 10.1016/j.ympev.2020.107039
González-Wevar C. A., Segovia N. I., Rosenfeld S., Ojeda J., Hüne M., Naretto J., et al. (2018). Unexpected absence of island endemics: long-distance dispersal in higher latitude sub-Antarctic Siphonaria (Gastropoda: euthyneura) species. J. Biogeogr. 45, 874–884. doi: 10.1111/jbi.13174
Guillot G., Mortier F., Estoup A. (2005). GENELAND: a computer package for landscape genetics. Mol. Ecol. Notes 5, 712–715. doi: 10.1111/j.1471-8286.2005.01031.x
Güller M., Zelaya D. G., Ituarte C. (2015). How many siphonaria species (Gastropoda: euthyneura) live in southern south America? J. Mollusc. Stud. 82, 80–96. doi: 10.1093/mollus/eyv036
Hüne M., González-Wevar C. A., Poulin E., Mansilla A., Fernández D. A., Barrera-Oro E. (2015). Low levels of genetic divergence between Harpagifer fish species (Perciformes: notothenioidei) suggests a quaternary colonization of Patagonia from the Antarctic peninsula. Polar Biol. 38, 607–617. doi: 10.1007/s00300-014-1623-6
Halanych K. M., Mahon A. R. (2018). Challenging the dogma concerning biogeographic patterns of Antarctica and the southern ocean. Annu. Rev. Ecol. Evol. Syst. 49, 355–378. doi: 10.1146/annurev-ecolsys-121415-032139
Hein A. S., Hulton N. R. J., Dunai T. J., Sugden D. E., Kaplan M. R., Xu S. (2010). The chronology of the last glacial maximum and deglacial events in central Argentine Patagonia. Quat. Sci. Rev. 29, 1212–1227. doi: 10.1016/j.quascirev.2010.01.020
Heusser C. J. (1998). Deglacial paleoclimate of the American sector of the southern ocean: late glacial–Holocene records from the latitude of canal beagle (55°S), Argentine tierra del fuego. Palaeogeogr. Palaeoclimatol. Palaeoecol. 141, 277–301. doi: 10.1016/S0031-0182(98)00053-4
Heusser C. J., Heusser L. E., Lowell T. V. (1999). Paleoecology of the southern chilean lake district-isla grande de chiloe during the middle-late llanquihue glaciation and deglaciation. Geogr. Ann. A 81, 231–284. doi: 10.1111/j.0435-3676.1999.00058.x
Hewitt G. (2000). The genetic legacy of the quaterary ice ages. Nature 405, 907–913. doi: 10.1038/35016000
Hewitt G. M. (2004). Genetic consequences of climatic oscillations in the quaternary. Phil. Trans. R. Soc Lond. B 359, 183–195. doi: 10.1098/rstb.2003.1388
Hodgson D. A., Graham A. G. C., Roberts S. J., Bentley M. J., Cofaigh C., Verleyen E., et al. (2014). Terrestrial and submarine evidence for the extent and timing of the last glacial maximum and the onset of deglaciation on the maritime-Antarctic and sub-Antarctic islands. Quat. Sci. Rev. 100, 137–158. doi: 10.1016/j.quascirev.2013.12.001
Hudson R. R. (2000). A new statistic for detecting genetic differentiation. Genetics 155, 2011–2014. doi: 10.1093/genetics/155.4.2011
Hulton N. R. J., Purves R. S., McCulloch D. M., Sugden D. E., Bentley M. J. (2002). The last glacial maximum and deglaciation in southern south America. Quat. Sci. Rev. 21, 233–241. doi: 10.1016/S0277-3791(01)00103-2
Ihaka R., Gentleman R. (1996). R: a language for data analysis and graphics. J. Comp. Graph. Stat. 5, 299–314. doi: 10.2307/1390807
Kaas R. E., Raftery A. E. (1995). Bayes factors. J. Am. Stat. Assoc. 90, 773–795. doi: 10.2307/2291091
Kalyaanamoorthy S., Minh B. Q., Wong T. K. F., von Haeseler A., Jermiin L. S. (2017). ModelFinder: fast model selection for accurate phylogenetic estimates. Nat. Methods 14, 587–589. doi: 10.1038/nmeth.4285
Kiel S., Nielsen S. N. (2010). Quaternary origin of the inverse latitudinal diversity gradient among southern Chilean mollusks. Geology 38, 955–958. doi: 10.1130/G31282.1
Knox G. A. (1960). Littoral ecology and biogeography of the southern ocean. Proc. R. Soc Lond. 152, 577–624. doi: 10.1098/rspb.1960.0068
Leese F., Agrawal S., Held C. (2010). Long-distance dispersal hopping without dispersal stages: transportation across major zoogeographic barriers in a southern ocean isopod. Naturwissenshaften 97, 583–594. doi: 10.1007/s00114-010-0674-y
Leese F., Kop A., Wägele J.-W., Held C. (2008). Cryptic speciation in a benthic isopod from Patagonian and Falkland island waters and the impact of glaciations on its population structure. Front. Zool. 5, 19. doi: 10.1186/1742-9994-5-19
Librado P., Rozas J. (2009). DnaSP v5: a software for comprehensive analysis of DNA polymorphism data. Bioinformatics 25, 1451–1452. doi: 10.1093/bioinformatics/btp187
Macaya E. C., Zuccarello G. C. (2010). Genetic structure of the giant kelp Macrocystis pyrifera along the southeastern pacific. Mar. Ecol–Prog. Ser. 420, 103–112. doi: 10.3354/meps08893
Maggs C. A., Castilho R., Foltz D., Henzler C., Jolly M. T., Kelly J., et al. (2008). Evaluating signatures of glacial refugia for north Atlantic benthic marine taxa. Ecology 89, S108–S122. doi: 10.1890/08-0257.1
Marko P. B. (2004). ‘What’s larvae got to do with it?’ disparate patterns of post-glacial population structure in two benthic marine gastropod with identical dispersal potential. Mol. Ecol. 13, 597–611. doi: 10.1046/j.1365-294X.2004.02096.x
Marko P. B., Hoffman J. M., Emme S. A., McGovern T. M., Keever C. C., Cox N. (2010). The ‘Expansion–contraction’ model of pleistocene biogeography: rocky shores suffer a sea change? Mol. Ecol. 19, 146–167. doi: 10.1111/j.1365-294X.2009.04417.x
McCulloch R. R., Bentley M. J., Purves R. S., Hulton N. R. J., Sugden D. E., Clapperton C. M. (2000). Climatic inferences from glacial and paleoecological evidence at the last glacial termination, southern south America. J. Quat. Sci. 15, 409–417. doi: 10.1002/1099-1417(200005)15:4<409::AID-JQS539>3.0.CO;2-%23
McCulloch R. R., Fogwill C. J., Sugden D. E., Bentley M. J., Kubik P. W. (2005). Chronology of the last glaciation in central strait of Magellan and bahía inútil, southernmost south America. Geogr. Ann. A 87, 1–24. doi: 10.1111/j.0435-3676.2005.00260.x
Miller K. J., Ayre D. J. (2008). Population structure is not a simple function of reproductive mode and larval type: insights from tropical corals. J. Anim. Ecol. 77, 713–724. doi: 10.1111/j.1365-2656.2008.01387.x
Moon K. L., Chown S. L., Fraser C. I. (2017). Reconsidering connectivity in the sub-Antarctic. Biol. Rev. 92, 2164–2181. doi: 10.1111/brv.12327
Moreno P. I., León A. L. (2003). Abrupt vegetation changes during the last glacial to Holocene transition in mid-latitude south America. J. Quat. Sci. 18, 787–800. doi: 10.1002/jqs.801
Moreno P. I., Lowell T. V., Jacobson G. L. Jr., Denton G. H. (1999). Abrupt vegetation and climate changes during the last termination in the Chilean lake district: a case study from canal de la puntilla (41°S). Geogr. Ann. A 81, 285–311. doi: 10.1111/j.0435-3676.1999.00059.x
Nakano T., Ozawa T. (2005). Systematic revision of Patelloida pygmaea (Dunker 1860) (Gastropoda: lottiidae), with a description of a new species. J. Molluscan Stud. 71, 357–370. doi: 10.1093/mollus/eyi039
Narum S. R. (2006). Beyond bonferroni: less conservative analyses for conservation genetics. Cons. Genet. 7, 783–787. doi: 10.1007/s10592-005-9056-y
Nikula R., Fraser C. I., Spencer H. G., Waters J. M. (2010). Circumpolar dispersal by rafting in two subantarctic kelp-dwelling crustaceans. Mar. Ecol-Prog. Ser. 405, 221–230. doi: 10.3354/meps08523
Nuñez J. D., Fernández Irirarte P. J., Ocampo E. H., Iudica M., Cledón M. (2015). Deep phylogeographic divergence among populations of limpet Siphonaria lessoni on the east and west coasts of south America. Mar. Biol. 162, 595–605. doi: 10.1007/s00227-014-2607-3
Nuñez J. J., Wood N. K., Rabanal F. E., Fontanella F. M., Sites J. J.W. (2011). Amphibian phylogeography in the antipodes: refugia and postglacial colonization explain mitochondrial haplotype distribution in the Patagonian frog Eupsophus calcaratus (Cycloramphidae). Mol. Phylogenet. Evol. 58, 343–352. doi: 10.1016/j.ympev.2010.11.026
Palumbi S. R. (1994). Genetic divergence, reproductive isolation, and marine speciation. Annu. Rev. Ecol. Syst. 25, 547–572. doi: 10.1146/annurev.es.25.110194.002555
Pardo-Gandarillas M. C., Ibañez C. M., Torres F. I., Sanhueza V., Fabres A., Escobar-Dodero J., et al. (2018). Phylogeography and species distribution modelling reveal the effects of the pleistocene ice ages on an intertidal limpet from the south-eastern pacific. J. Biogeogr. 45, 1751–1767. doi: 10.1111/jbi.13362
Paulay G., Meyer C. (2006). Dispersal and divergence across the greatest ocean region: Do larvae matter? Int. Comp. Biol. 46, 269–281. doi: 10.1093/icb/icj027
Peck L. S., Heiser S., Clark M. (2016). Very slow embryonic and larval development in the Antarctic limpet Nacella polaris. Polar Biol 39, 2273–2280. doi: 10.1007/s00300-016-1894-1
Ponce J. F., Rabassa J., Coronato A., Borromei A. M. (2011). Palaeogeographical evolution of the Atlantic coast of pampa and Patagonia from the last glacial maximum to the middle Holocene. Biol. J. Linn. Soc 103, 363–379. doi: 10.1111/j.1095-8312.2011.01653.x
Pons O., Petit R. J. (1996). Measuring and testing genetic differentiation with ordered versus unordered alleles. Genetics 144, 1237–1245. doi: 10.1093/genetics/144.3.1237
Poulin E., González-Wevar C., Díaz A., Gérard K., Hüne M. (2014). Divergence between Antarctic and south American marine invertebrates: what molecular biology tells us about Scotia arc geodynamics and the intensification of the Antarctic circumpolar current. Global Planet. Change 123, 392–399. doi: 10.1016/j.gloplacha.2014.07.017
Poulin E., Palma A. T., Féral J.-P. (2002). Evolutionary versus ecological success in Antarctic benthic invertebrates. Trends Ecol. Evol. 17, 218–222. doi: 10.1016/S0169-5347(02)02493-X
Powell A. W. B. (1973). The patellid limpets of the world (Patellidae) (New Zealand: Auckland Institute and Museum Auckland), 1–132.
Provan J., Bennett K. D. (2008). Phylogeographic insights into cryptic glacial refugia. Trends Ecol. Evol. 23, 564–571. doi: 10.1016/j.tree.2008.06.010
Puritz J. B., Keever C. C., Addison J. A., Barbosa S. S., Byrne M., Hart M. W., et al. (2017). Life-history predicts past and present population connectivity in two sympatric sea stars. Ecol. Evol. 7, 3916–3930. doi: 10.1002/ece3.2938
Rabassa J., Coronato A., Martínez O. (2011). Late Cenozoic glaciations in Patagonia and tierra del fuego: an updated review. Biol. J. Linn. Soc 103, 316–335. doi: 10.1111/j.1095-8312.2011.01681.x
Rabassa J., Coronato A. M., Salemme M. (2005). Chronology of the late Cenozoic Patagonian glaciations and their correlation with biostratigraphic units of the pampean region (Argentina). J. South Am. Earth. Sci. 20, 81–103. doi: 10.1016/j.jsames.2005.07.004
Rambaut A., Drummond A. J., Xie D., Baele G., Suchard M. A. (2018). Posterior summarization in Bayesian phylogenetics using tracer. Syst. Biol. 67, 901–904. doi: 10.1093/sysbio/syy032
Reisser C. M. O., Wood A. R., Bell J. J., Gardner J. P. A. (2011). Connectivity, small islands and large distances: the Cellana strigilis limpet complex in the southern ocean. Mol. Ecol. 20, 3399–3413. doi: 10.1111/j.1365-294X.2011.05185.x
Riesgo A., Taboada S., Avila C. (2015). Evolutionary patterns in Antarctic marine invertebrates: an update on molecular studies. Mar. Genomics 23, 1–13. doi: 10.1016/j.margen.2015.07.005
Rogers A. R., Harpending H. C. (1992). Population growth makes waves in the distribution of pairwise genetic differences. Mol. Biol. 9, 552–569. doi: 10.1093/oxfordjournals.molbev.a04727
Ruzzante D. E., Walde S. J., Cussac V. E., Dalebout M. L., Seibert J., Ortubay S., et al. (2006). Phylogeography of the percichthyidae (Pisces) in Patagonia: roles of orogeny, glaciation, and volcanism. Mol. Ecol. 15, 2949–2968. doi: 10.1111/j.1365-294X.2006.03010.x
Salzburger W., Ewing G. B., Von Haessler A. (2011). The performance of phylogenetic algorithms in estimating haplotype genealogies with migration. Mol. Ecol. 20, 1952–1963. doi: 10.1111/j.1365-294X.2011.05066.x
Sá Pinto A., Branco M., Sayanda D., Alexandrino P. (2007). Patterns of colonization, evolution and gene flow in species of the genus Patella in the macaronesian islands. Mol. Ecol. 17, 519–532. doi: 10.1111/j.1365-294X.2007.03563.x
Schneider S., Excoffier L. (1999). Estimation of past demographic parameters from the distribution of pairwise differences when the mutation rates vary among sites. application to human mitochondrial DNA. Genetics 152, 1079–1089. doi: 10.1093/genetics/152.3.1079
Segovia N. I., González-Wevar C. A., Naretto J., Rosenfeld S., Brickle P., Hüne M., et al. (2022). The right tool for the right question: contrasting biogeographic patterns in the notothenioid fish harpagifer spp. along the Magellan province. Proc. R. Soc 289, 20212738. doi: 10.1098/rspb.2021.2738
Strathmanns R. R. (1985). Feeding and nonfeeding larval development and life-history evolution in marine invertebrates. Ann. Rev. Ecol. Syst. 16, 339–361. doi: 10.1146/annurev.es.16.110185.002011
Tamura K., Stecher G., Kumar S. (2022). MEGA11: molecular evolutionary genetics analysis version 11. Mol. Biol. Evol. 38, 3022–3027. doi: 10.1093/molbev/msab120
Toro J. E., Ojeda J. A., Vergara A. M. (2004). The genetic structure of Mytilus chilensis (Hupe 1854) populations along the Chilean coast based on RAPDs analysis. Aquat. Res. 35, 1466–1471. doi: 10.1111/j.1365-2109.2004.01172.x
Trovant B., Orenzanz (Lobo) J. M., Ruzzante D. E., Stotz W., Basso N. G. (2015). Scorched mussels (BIVALVIA: MYTILIDAE: BRACHIDONTINAE) from the temperate coasts of south America: phylogenetic relationships, trans-pacific connections and the footprints of quaternary glaciations. Mol. Phylogenet. Evol. 82, 60–74. doi: 10.1016/j.ympev.2014.10.002
Valdovinos C., Navarrete S. A., Marquet P. A. (2003). Mollusk species diversity in the southeastern pacific: why are there more species towards the pole? Ecography 26, 139–144. doi: 10.1034/j.1600-0587.2003.03349.x
Valdovinos C., Rüth M. (2005). Nacellidae limpets of southern south America: taxonomy and distribution. Rev. Chil. Hist. Nat. 78, 497–517. doi: 10.4067/S0716-078X2005000300011
Villamor A., Costantini F., Abbiati M. (2014). Genetic structuring across marine biogeographic boundaries in rocky shore invertebrates. PloS One 9, e101135. doi: 10.1371/journal.pone.0101135
Violante R. A., Paterlini C. M., Marcolini S. I., Costa I. P., Cavalloto J. L., Laprida C., et al. (2014). “The Argentine continental shelf: morphology, sediments, processes and evolution since the last glacial maximum. in: continental shelves of the world: their evolution during the last glacio-eustatic cycle,” in The geological society of London. Eds. Chiocci F. L., Chivas A. R. (UK: Geological Society of London), 55–68. doi: 10.1144/M41.6
Waters J. M. (2008). Driven by the West wind drift? a synthesis of southern temperate marine biogeography, with new directions for dispersalism. J. Biogeogr. 35, 417–427. doi: 10.1111/j.1365-2699.2007.01724.x
Webb T. III, Bartlein P. J. (1992). Global changes during the last 3 million years: climatic controls and biotic responses. Annu. Rev. Ecol. Evol. Syst. 23, 141–173. doi: 10.1146/annurev.es.23.110192.001041
Williams J. W., Summers R. L., Webb III, T. (1998). Applying plant functional types to construct biome maps from eastern north American pollen data. Quat. Sci. Rev. 17, 607–627. doi: 10.1016/S0277-3791(98)00014-6
Wilson P., Bentley M. J., Schnabel C., Clark R., Xu S. (2008). Stone run (block stream) formation in the Falkland islands over several cold stages, deduced from cosmogenic isotope (10Be and 26Al) surface exposure dating. J. Quat. Sci. 23, 461–473. doi: 10.1002/jqs.1156
Wilson P., Clark R., Birnie J., Moore D. M. (2002). Late pleistocene and Holocene landscape evolution and environmental change in the lake sulivan area, Falkland islands, south Atlantic. Quat. Sci. Rev. 21, 1821–1840. doi: 10.1016/S0277-3791(02)00008-2
Wilson N. G., Schrödl M., Halanych K. M. (2009). Ocean barriers and glaciation: evidence for explosive radiation of mitochondrial lineages in the Antarctic sea slug Doris kerguelenensis (Mollusca, nudibranchia). Mol. Ecol. 18, 965–984. doi: 10.1111/j.1365-294X.2008.04071.x
Xia X., Xie Z. (2001). DAMBE: software package for data analysis in molecular biology and evolution. J. Hered. 92, 371–373. doi: 10.1093/jhered/92.4.371
Xu J., Pérez-Losada M., Jara C. G., Crandall K. A. (2009). Pleistocene glaciation leaves deep signature on the freshwater crab Aegla alacalufiin Chilean Patagonia. Mol. Ecol. 18, 904–918. doi: 10.1111/j.1365-294X.2008.04070.x
Zattara E. E., Premoli C. (2005). Genetic structuring in Andean landlocked populations of galaxias maculatus: effects of biogeographic history. J. Biogeogr. 32, 5–14. doi: 10.1111/j.1365-2699.2004.01164.x
Zemlak T. S., Habit E. M., Walde S. J., Battini M. A., Adams E. D. M., Ruzzante D. E. (2008). Across the southern Andes on fin: glacial refugia, drainage reversals and a secondary contact zone revealed by the phylogeographical signal of Galaxias platei in Patagonia. Mol. Ecol. 17, 5049–5061. doi: 10.1111/j.1365-294X.2008.03987.x
Keywords: quaternary, last glacial maximum, population genetics, phylogeography, Nacella, Magellan Province
Citation: González-Wevar CA, de Aranzamendi MC, Segovia NI, Rosenfeld S, Maturana CS, Molina CR, Brickle P, Gardenal CN, Bastida R and Poulin E (2023) Genetic footprints of Quaternary glacial cycles over the patterns of population diversity and structure in three Nacella (Patellogastropoda: Nacellidae) species across the Magellan province in southern South America. Front. Mar. Sci. 10:1154755. doi: 10.3389/fmars.2023.1154755
Received: 31 January 2023; Accepted: 08 May 2023;
Published: 29 May 2023.
Edited by:
Tomoyuki Nakano, Kyoto University, JapanReviewed by:
Douglas Eernisse, California State University, Fullerton, United StatesLijun He, East China Normal University, China
Copyright © 2023 González-Wevar, de Aranzamendi, Segovia, Rosenfeld, Maturana, Molina, Brickle, Gardenal, Bastida and Poulin. This is an open-access article distributed under the terms of the Creative Commons Attribution License (CC BY). The use, distribution or reproduction in other forums is permitted, provided the original author(s) and the copyright owner(s) are credited and that the original publication in this journal is cited, in accordance with accepted academic practice. No use, distribution or reproduction is permitted which does not comply with these terms.
*Correspondence: Claudio A. González-Wevar, Y2xhdWRpby5nb256YWxlekB1YWNoLmNs