- 1Unidad de Zoología Marina, Instituto Cavanilles de Biodiversidad y Biología Evolutiva, Universitat de València, Paterna, Spain
- 2Centre for Ecology and Conservation, College of Life and Environmental Sciences, University of Exeter, Penryn, United Kingdom
- 3Institut de Ciències del Mar, CSIC, Barcelona, Spain
- 4Institute for Marine Biological Resources and Biotechnology, National Research Council, Mazara del Vallo, Italy
- 5Unitat de Zoologia, Universitat Autonoma de Barcelona, Barcelona, Spain
Introduction: Chondrichthyans (sharks, batoids and chimaeras) play key roles in the regulation of marine food webs dynamics. However, more than half of the assessed species in the Mediterranean are threatened, primarily by fishing pressure and compounded by habitat degradation and climate change. Nevertheless, there is an important knowledge gap in identifying the underlying drivers of their community structure and spatial distribution.
Methods and Results: We provide insights into the current bycatch rates of chondrichthyans in the western Mediterranean commercial bottom trawling fishery by accurately depicting the unaltered practices of the local fleet. A total of 17 species were recorded in the studied fishing grounds (ranging from 50 to 800 m deep), including 7 sharks, 9 batoids, and 1 chimaera, although the total catch was dominated by few species. Furthermore, we tested the effect of environmental and fishing-related factors on multiple community descriptors by using analysis of community structure (multidimensional scaling and analysis of similitude) and generalized linear mixed models to further understand the drivers of the chondrichthyan community distribution and structure. This study revealed the importance of combining environmental and anthropogenic drivers to further understand the spatial distribution patterns of chondrichthyan communities. Our results indicate that depth was the main driver of the community structure, with deeper areas within the fishing grounds hosting a higher diversity. Sea bottom temperature and substrate type also influenced the distribution of the community, with substrate effects changing depending on the intensity of fishing pressure. In all cases, density, biomass, and diversity of chondrichthyans were negatively impacted by increasing fishing effort.
Discussion: Understanding the drivers of the structure and distribution of the chondrichthyan community is crucial to understand the potential impacts that increased fishing pressure, habitat loss and global change may entail. The ongoing challenges that the Mediterranean chondrichthyans and their ecosystems are facing highlights the need for continued monitoring and improved chondrichthyan-focused fisheries management.
Introduction
Chondrichthyans (sharks, batoids and chimaeras) play a key role in the regulation of marine ecosystems by controlling the size and dynamics of their prey populations and connecting trophic webs across habitats (Stevens et al., 2000; Sheaves, 2009; Barría et al, 2015a). Their life history traits (slow growth, late maturity, low fecundity, and longevity) make them particularly vulnerable to the anthropogenic pressure which is primarily propitiated by fisheries (for both target and non-target species), followed by habitat loss and degradation and to a lesser extent climate change and pollution (Cortés, 2000; Dulvy et al., 2021). Given their important ecological role, the depletion of chondrichthyans induces changes in the structure and functioning of marine ecosystems (Stevens et al., 2000), ultimately leading to a loss of biodiversity and productivity (Heithaus et al., 2008). Considered as keystone species, some chondrichthyans can be used as indicators to monitor overfishing and ecosystem stability (Stevens et al., 2000; Heithaus et al., 2008).
The global situation for chondrichthyans is highly concerning, constituting the most threatened group of marine animals, with more than one third of the species estimated to be threatened (Dulvy et al., 2021). This trend is even exacerbated in the Mediterranean, with 53% out of the 73 assessed species classified as threatened (Dulvy et al., 2016). Fishing activities are the main cause for the decline of the Mediterranean chondrichthyan community (Follesa et al., 2019; Serena et al., 2020). Chondrichthyans may constitute an important proportion of the catch for multi-specific fisheries, but only a minor part is reported on landings as they are frequently captured as non-target species (bycatch) and discarded at sea (Ferretti et al., 2008; Peristeraki et al., 2020). Despite efforts by the General Fisheries Commission for the Mediterranean (GFCM) to reduce the capture of sharks by prohibiting onboard finning and the sale of the chondrichthyan species listed in the Annex II of the Barcelona Convention (FAO, 2020), the populations of many species are still poorly managed (Walls and Dulvy, 2021; Koehler et al., 2022). Moreover, reliable data on the stock status of chondrichthyans is scarce in many regions.
Monitoring programs on the demersal Mediterranean marine resources started at the beginning of the 20th century describing the regional chondrichthyan community (De Buen, 1935; Maurin, 1968; Aldebert, 1997). In the early 1990s international programs based in fisheries-independent data increased importantly their capacity to monitor the Mediterranean demersal resources. Part of the published research covered large areas of the Mediterranean (e.g. Bertrand et al., 2000; Follesa et al., 2019; Serena et al., 2020), but most occurred at regional scales along the European coasts (e.g. Guijarro et al., 2012; Peristeraki et al., 2020; Geraci et al., 2021), while remaining particularly scarce in the African region (e.g. Ordines et al., 2011; Colloca et al., 2017; Serena et al., 2020). These studies described the regional chondrichthyan communities and their spatial distribution (e.g. Tserpes et al., 2013; Lauria et al., 2015; Porcu et al., 2020). They also elucidated temporal trends for some species (e.g. Damalas et al., 2010; Damalas and Vassilopoulou, 2011; Maravelias et al., 2012), documenting the drastic community composition changes suffered since the early fishing exploitations (e.g. Jukic-Peladic et al., 2001; Ferretti et al., 2013; Barausse et al., 2014). Starting from the 1950s, the north-western Mediterranean trawling grounds were populated with some of the currently most threatened species (Maurin, 1968; Aldebert, 1997), and although after the 2000s certain stability was reached in terms of diversity (Ramírez-Amaro et al., 2020) the abundance of an important part of the species continues to decline (Ligas et al., 2013; Marongiu et al., 2017; Ramírez-Amaro et al., 2020; Walls and Dulvy, 2020; Walls and Dulvy, 2021).
Recently, with the use of spatially explicit modelling approaches, the research efforts have been focused on the effects of the changing environments on the structure and dynamics of these communities (e.g. Follesa et al., 2019; Maioli et al., 2022), and only rarely they have integrated the anthropogenic pressures in the modelling approaches as predictors in shaping the composition, abundance, and distribution of the demersal chondrichthyans in the Mediterranean (Navarro et al., 2016; Peristeraki et al., 2020). From an ecological perspective, it is important to understand how species and the community respond to certain factors. However, from a management standpoint, it may be more useful to quantify how multiple factors interact and combine in space to shape distribution patterns (Melo-Merino et al., 2020). Assessing the combined effects of both environmental and fishing-related drivers on the chondrichthyan community might enhance not only the capacity to determine important areas and conditions for conservation, but also the ability to make predictions where data is scarce or absent (Ovaskainen et al., 2017). This is especially important to build capacity in poorly researched areas, determine thresholds for sustainable practices, and understand the potential effects of increasing fishing pressure, global change and habitat loss (Melo-Merino et al., 2020).
In this study, we updated information on the status of the demersal chondrichthyan community in the Mediterranean trawling grounds from the Northern Spain GFCM geographic subarea (GSA06). First, we collected data from commercial bottom trawling surveys to provide accurate chondrichthyan bycatch rates during regular commercial practices. Then, we assessed the influences of environmental and fishing-related drivers on the structure and distribution of the chondrichthyan community, aiming to improve our understanding of the status of the western Mediterranean chondrichthyan community for a more efficient management.
Materials and methods
Study site and fishery
This work covered diverse bottom trawling grounds across the Mediterranean waters of the eastern Iberian Peninsula, i.e. the “Northern Spain” geographical subarea (GSA06) established by the GFCM (Figure 1), occurring in the soft bottoms of the continental margin between 50 and 800 m deep. The Spanish trawl fleet operating in the GSA06 is censed at 32 home ports ranging from Cartagena to Roses. As in December 2022, the region counts with a fleet of 421 licensed bottom trawlers, although the fleet continues to decrease progressively during the last decades. Surveys were conducted onboard of 20 of these commercial fishing vessels (F/V) from 14 home ports ranging from Cartagena to Roses to cover most of the GSA06 (Figure 1). The surveys were conducted through onboard observation campaigns performed without altering the conventional fishing practices of the F/V nor the location of the conducted hauls, therefore, being these randomly selected. The surveyed hauls were tracked geographically using GPS and bathymetrically using the SCANMAR system (Catch Control Systems, Scanmar AS, Åsgårdstrand, Norway). The sampling protocol accounted with a proportional number of hauls analyzed for the three different métiers (i.e. group of fishing operations targeting a specific assemblage of species) that occur in the region: (1) the “hake métier” targets Merluccius merluccius, Mullus spp., and Parapenaeus longirostris; (2) the “Norway lobster métier” targets Nephrops norvegicus with main accessory species being Micromesistius poutassou, Phycis blennoides and P. longirostris; and (3) the “blue and red shrimp métier” targets Aristeus antennatus, with the main accessory species being Geryon longipes and Plesionika spp. Fishing métiers slightly overlap geographically and bathymetrically but the hake métier mainly occurs in the continental shelf (approximately 50-250 m deep), the Norway lobster métier fishes mainly in the upper continental slope (approximately 200-600 m deep) and the blue and red shrimp métier mostly operates in the lower continental slope (approximately 400-800 m deep). The conducted sampling aimed to accurately represent the normal commercial practices of the local fleet while using a standardized protocol.
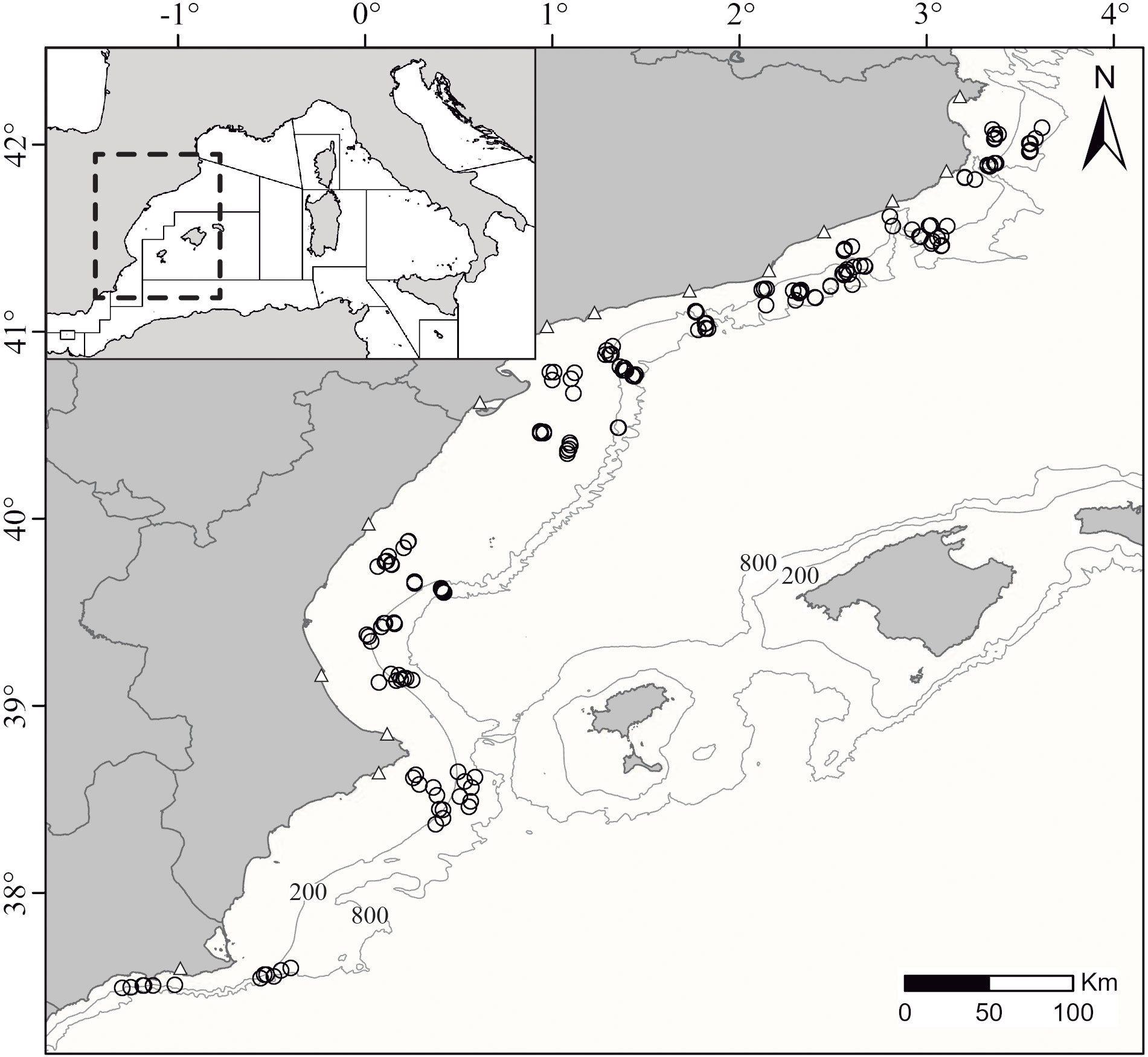
Figure 1 Geographical position of the study area within the western Mediterranean including the respective existent Geographical Sub-Areas (GSAs). The points indicate the position of the 189 fishing hauls analyzed in this study occurring across the GSA06 (Northern Spain) at depths ranging from 50 to 800 m. The triangles denote the location of the home ports included in the study and the isobaths denote 200 and 800 m deep.
Experimental procedures
The data set consisted of 189 hauls conducted by 20 different commercial bottom trawlers (F/V) between June 2020 and January 2022. A consistent stratified-random sampling design was used, as haul locations were randomly selected but a proportional division among the number of analyzed hauls was considered for each port, season, depth range and métier. Data was collected on a seasonal basis at each of the 14 studied ports, accounting with hauls from each of the three previously described métiers and occurring proportionally across the continental margin (continental shelf, upper slope, and lower slope) with minimum and maximum depths ranging from 50 to 737 m. The number of hauls per season and depth range is provided in Table 1. These surveys used F/V of similar capability and fishing gear per each métier to minimize any effects of gear selectivity, although some variation occurred. All fishing gears consisted of a bottom otter trawl design, with the horizontal opening of the net averaging 24.1 ± 7.0 m and fitted with a squared 40 mm cod-end mesh, except in Palamós, where the co-management measures for the blue and red shrimp métier require a 50 mm squared mesh. Each haul was conducted without altering the conventional commercial practices of the F/V. The mean distance covered in each haul averaged 18.0 ± 8.7 km, the effective haul duration was 3.3 ± 1.4 h, and the average towing speed was 2.9 ± 0.4 knots. The depth range covered in each haul varied in average 77.5 ± 59.7 m, but the variation was larger in the upper and lower slope (111.3 ± 68.7 m, 107.1 ± 52.4 m, respectively) than in the shelf (35.2 ± 25.7 m). The raising and lowering of net from and to the sea bottom, as well as the horizontal and vertical openings of the net were estimated using the SCANMAR system. For each haul every chondrichthyan captured was identified and classified and all of them or a representative random sample was counted and measured to the nearest millimeter using total length (TL; tip of the snout to posterior end of caudal fin) for sharks and batoids and pre-supracaudal length (PSCL; tip of the snout to the posterior edge of the supracaudal fin) for chimaeras.

Table 1 Number of hauls analyzed onboard of commercial bottom trawlers per season and depth strata (shelf, 50-200 m deep; upper slope, 200-500 m deep; and lower slope, 500-800 m deep).
Community descriptors
We calculated five descriptive metrics of the chondrichthyan community: (1) density, (2) biomass, (3) diversity, (4) evenness, and (5) richness. Capture per unit of effort (CPUE) was calculated as both density and biomass measures, defined as the number of individuals and kilograms of chondrichthyans, respectively, caught per square kilometer pooling all species together (i.e. inds/km2 and kg/km2, respectively). We estimated the individual weight by using species-specific length-weight relationships presented in previous studies from the study area (Barría et al, 2015b). Species diversity, richness and evenness were calculated using the Shannon index (H’), Margalef’s index (d), and Pielou’s index (J), respectively, accounting not only for the number of species but also with their relative proportion in the catch. Community assemblages were further described using multidimensional scaling (MDS) on the standardized density dataset. Principal Coordinate Analysis (PCoA) was performed on a Bray-Curtis dissimilarity matrix to summarize and visualize patterns in the species assemblages based on their relative density. To meet the assumptions of PCoA, we tested for multivariate normality and applied logarithmic transformations to the data. Analyses were conducted using the package vegan (Oksanen et al, 2022).
Predictor variables
The community descriptor metrics and the principal coordinates (PCoA) axis that accounted for the largest variance in the data were related to different explanatory variables a priori selected including both environmental and fishing-related predictors. Environmental predictors included depth, slope angle, seabed substrate type, and sea bottom temperature (Figure 2). The predictors selected have previously been associated with species diversity and community structure (e.g. Lucifora et al., 2012; Pennino et al., 2013; Lauria et al., 2015). The mean depth of each haul was calculated using the SCANMAR system incorporated in the F/V and was then supported using EMODnet Bathymetry, resulting in a continuous variable ranging from 54 to 685 m. The slope angle was calculated using the raster R package (Hijmans, 2022) from the EMODnet Bathymetry. Substrate data was extracted from the European Marine Observation Data Network (EMODnet) Seabed Habitats project (emodnet.ec.europa.eu/en/seabed-habitats) and categorized as: (1) coarse, (2) sandy, and (3) muddy. Sea bottom temperature (SBT) data was obtained from the Copernicus Marine Environment Monitoring Service (CMEMS) dataset of Mediterranean Sea Physics Reanalysis maps on a 1/24° resolution grid spanning the complete Mediterranean. We extracted monthly sea bottom temperature estimates for the study area from 50 to 800 m deep and we matched the values with each trawl haul, yielding values ranging from 12.9 to 16.6 °C.
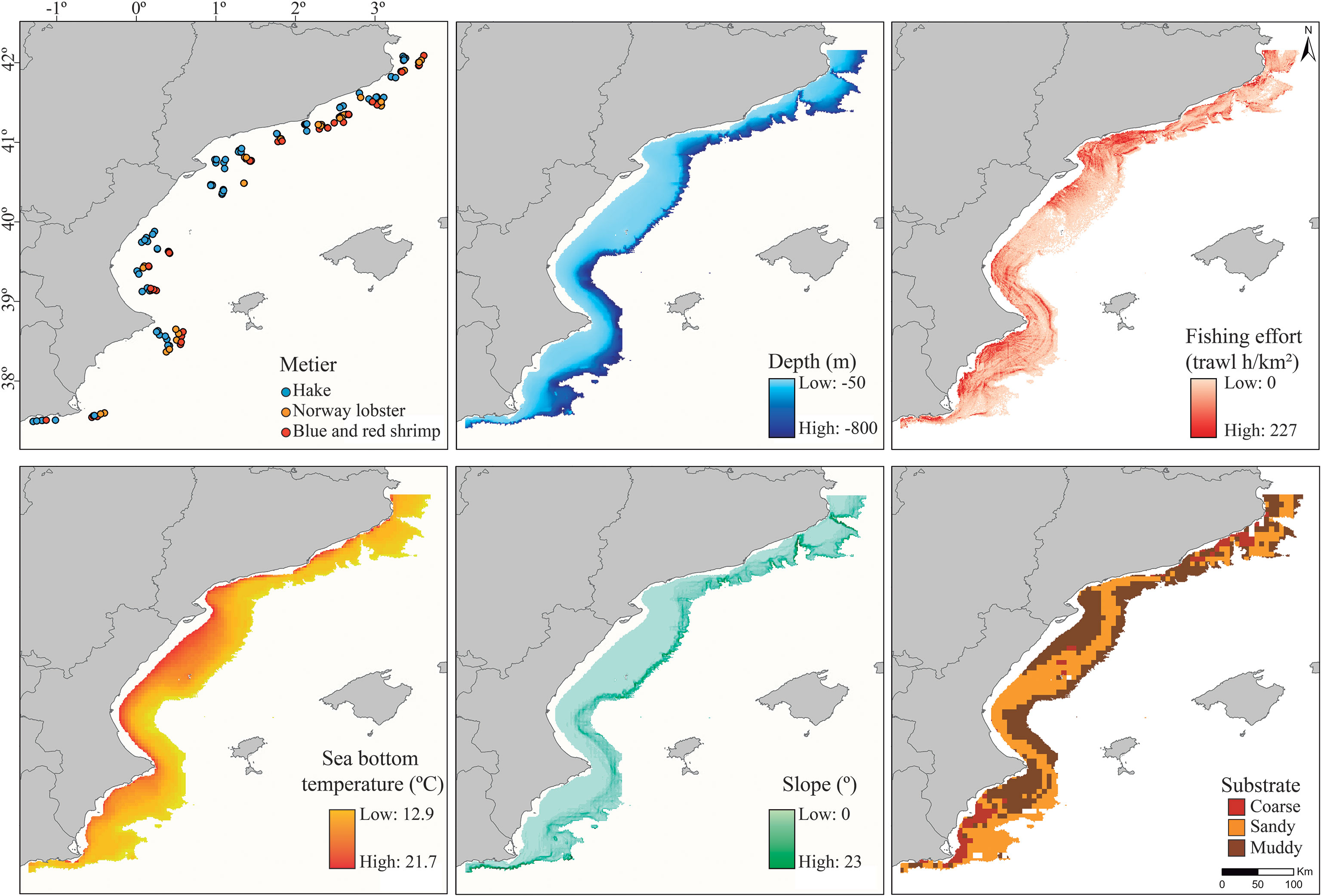
Figure 2 Environmental and fishing-related drivers used as predictors to model the distribution and structure of the chondrichthyan community, including: (A) métier, (B) depth, (C) fishing effort, (D) sea bottom temperature, (E) slope, and (F) seabed substrate. The values for each variable are spanned within the study region (GSA06) from 50 to 800 m deep.
The fishing-related variables included métier and fishing effort (Figure 2). The métier of each haul was classified according to the three categories previously described. Apparent fishing effort was extracted from Global Fishing Watch (globalfishingwatch.org), which uses automatic identification system (AIS) data to identify and track fishing vessels. The apparent effort was calculated as the number of hours trawled within each squared kilometer of the study area for the 2019-2022 period. The time period selected was intended to represent not only the effort conducted during the survey period but the pressure that has been exerted during recent years. This allowed us to highlight areas of high fishing effort, with a maximum effort of 226.8 h/km2 and calculate the average fishing effort in the location where each haul was conducted, ranging from 5.5 to 183.8 h/km2. Fishing effort was higher across the continental shelf (< 200 m deep) and, especially, in areas located near large ports and cities, likely influenced by the market demands, whereas the deepwater fishing grounds (> 200 m deep) were concentrated in certain regions leaving others unfished. Multiple overlapping ring buffers of 5 km2 were used to extract mean values of continuous variables matching to haul locations.
Statistical analyses
We used generalized linear mixed effect models (GLMMs) to analyze the effect of environmental and fishing-related variables on each community descriptor metric and the first principal coordinate (PCoA) axis describing the composition of the community assemblages. The fixed effects included all the previously described predictors (depth, slope, substrate type, sea bottom temperature, fishing effort, and métier). We considered a quadratic form of depth to account for possible nonlinear effects of this predictor variable. We included an interaction term between depth and sea bottom temperature, as the later changes progressively with depth and may affect the response variable differently at different depths. We also included an interaction term between fishing effort and substrate to account for indirect effects of trawling in relation to different substrates (Collie et al., 2017). In addition, we considered F/V (20 levels) as independent random effects to account for the potential heterogeneity between F/V (e.g. skipper behavior and experience).
We created a full model for each response variable including all fixed and random effects (Bolker et al., 2009). We fitted the full models using the package lme4 (Bates et al., 2015) and a Gaussian error distribution and estimated the parameters using restricted maximum likelihood (REML). We standardized the continuous explanatory variables (i.e. transforming them to yield a mean of zero and standard deviation of one) to reduce collinearity effects among variables. We checked for multicollinearity using the variance inflation factor (VIF) and all predictor variables were used for model selection as they were uncorrelated (i.e. VIF < 3.5). Homoscedasticity was examined through the visual evaluation of residual plots across categories and continuous predictors. We tested for normality by visually inspecting residual histograms, normal Q-Q plots, and the Shapiro–Wilk test. Where normality and homoscedasticity assumptions were violated, logarithmic transformations were applied. We first estimated the optimal structure of the random component using the package lmerTest (Kuznetsova et al., 2017). When the random component was not significative, generalized lineal models were fitted instead. Both backwards and forward stepwise approaches based on the Akaike Information Criterion (AIC) were used to select the best model fixed structure (Zuur et al, 2009). The linear term was forced into the model each time the quadratic term was selected in the model (Zurell et al., 2009). Once the optimal model was achieved, we rechecked the assumptions of homoscedasticity and normality. In addition, we examined the presence of spatial autocorrelation (SAC; Dormann, 2007) in the residuals based on Moran’s I test; no SAC was detected in any of the models.
The center of gravity (CoG) was calculated to better understand the influence of bathymetry on the chondrichthyan community structure by determining the average depth at which each species was most commonly found (Stefanescu et al., 1993). In addition, the average density and biomass of each species was calculated for each feature of the continental margin, defined as the continental shelf (50-200 m), upper slope (200-500 m), and lower slope (500-800 m). Finally, analyses of similarities (ANOSIM) and similarity percentages (SIMPER) were conducted on the density Bray-Curtis matrix to determine whether the species assemblages were significantly different among each of the features of the continental margin and to further describe each of them.
Results
Collected data
A total of 189 hauls were examined, covering an area of 53.1 km2 proportionally distributed across the examined geographic and bathymetric range in the GSA06. Chondrichthyan specimens were found in 91.5% of these hauls (173 out of 189), with a total of 11,997 specimens captured. No chondrichthyans were recorded on the remaining 16 hauls (Table 1). Chondrichthyans were present all along the geographical and bathymetrical range.
Community description
A total of 17 chondrichthyan species were caught in the studied area during the surveyed period, including 7 sharks (belonging to 3 orders and 7 families), 9 batoids (belonging to 3 orders and 4 families) and 1 chimaera species (Table 2). The most frequently caught species across the study range were Scyliorhinus canicula, Galeus melastomus, Etmopterus spinax and Torpedo marmorata, which also had the highest levels of abundance. Threatened species were also present in the area with low frequency and abundance, including batoids such as Aetomylaeus bovinus, Myliobatis aquila and Dasyatis pastinaca, found in the continental shelf, and sharks such as Oxynotus centrina, Centrophorus uyato and Dalatias licha mostly occurring in the continental slope. Table 2 summarizes the depth range and center of gravity at which each species was caught, as well as their bycatch rates in terms of frequency of capture and CPUE for the overall studied range, measured as mean density and mean biomass. Additional information on bycatch rates in terms of frequency, density, and biomass of each species at each section of the continental margin (shelf, upper slope and lower slope) is provided in the supplementary material (Supplementary Table S1).
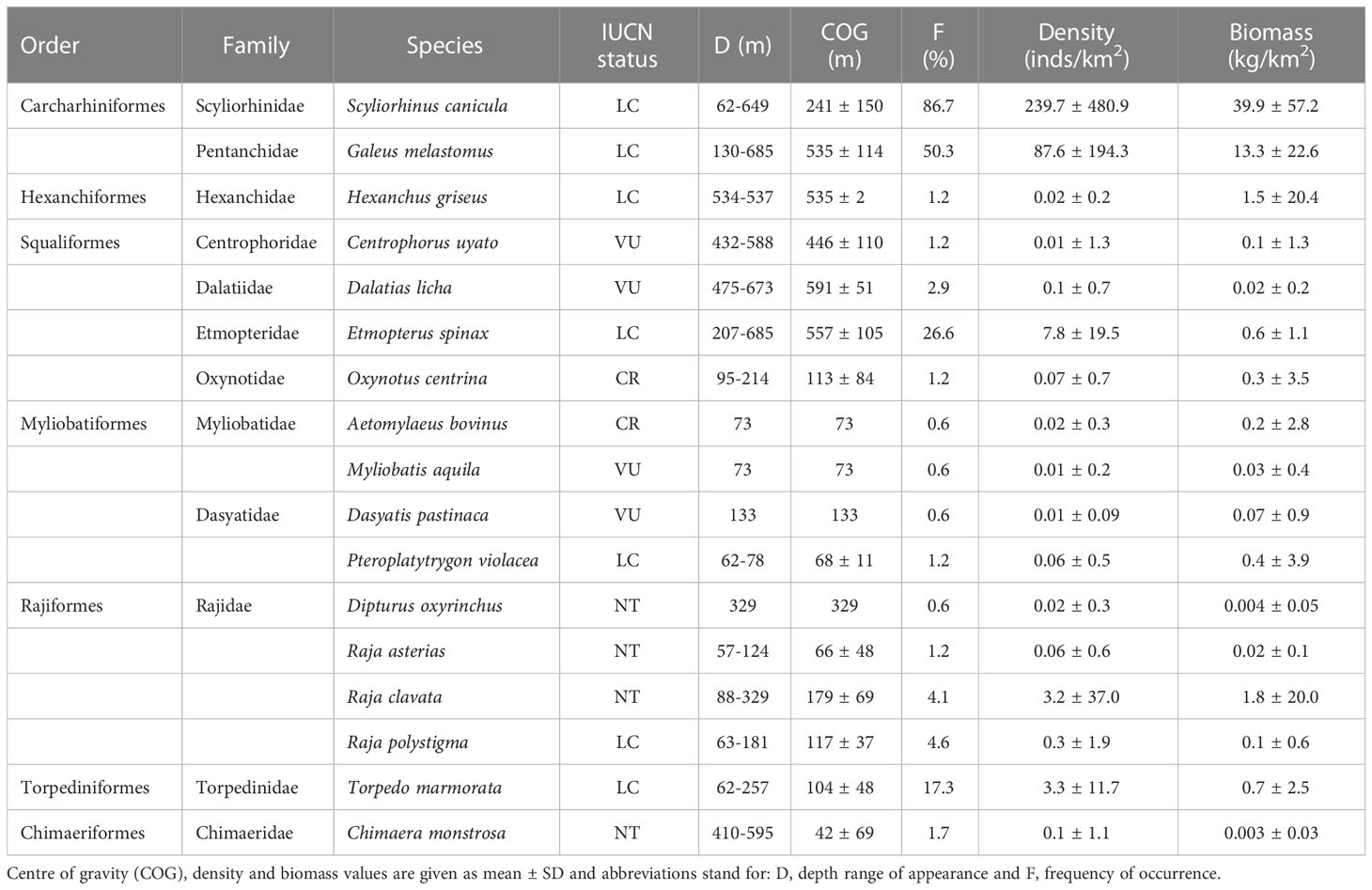
Table 2 List of the chondrichthyan species reported in the surveyed hauls, including their IUCN red list status categories in the Mediterranean according to IUCN (2022), preferred depth ranges and overall bycatch rates.
Environmental and fishing-related drivers of the structure and distribution of the community
The principal coordinates analysis (PCoA) plot based on the relative density of each species revealed dissimilarities in the composition (Figure 3). The first unconstrained index of compositional dissimilarity (PCoA axis 1) explained almost 20% of the total variation in the relative density of the species, grouping them in relation to depth (Table 3; Figure 4). The species assemblage occurring in each of the regions of the continental margin was significantly different (ANOSIM, p < 0.001; R = 0.45). According to the SIMPER analysis, G. melastomus and S. canicula were the main species contributing to this difference, followed by E. spinax, T. marmorata, and D. licha (Table 4). The continental shelf was characterised by high densities of S. canicula, followed by T. marmorata and the skates Raja polystigma and Raja clavata, occurring in >90%, >40, >10 and >5% of the hauls, respectively (Table 4; Supplementary Table S1). Despite their low overall abundance, batoids made up the largest part of the species diversity in the continental shelf fishing grounds, with 8 out of 9 species preferring this bathymetric range. Sharks, on the other hand, constitute the most diverse group in the continental slope. The upper slope is characterised by high abundance levels of S. canicula, followed by G. melastomus and E. spinax, with a frequency of appearance of >90%, >60%, and >20%, respectively. This bathymetric range was also characterized by the occurrence of low densities of C. uyato, Dipturus oxyrinchus, and Chimaera monstrosa, which had their centres of gravity in this range (Table 4; Supplementary Table S1). The lower slope was dominated by G. melastomus with a frequency of appearance of >90%, followed by S. canicula and E. spinax, both appearing in >60% of the hauls conducted in this range. Hexanchus griseus and D. licha also occurred predominantly in this range in low abundance levels (Table 4; Supplementary Table S1).
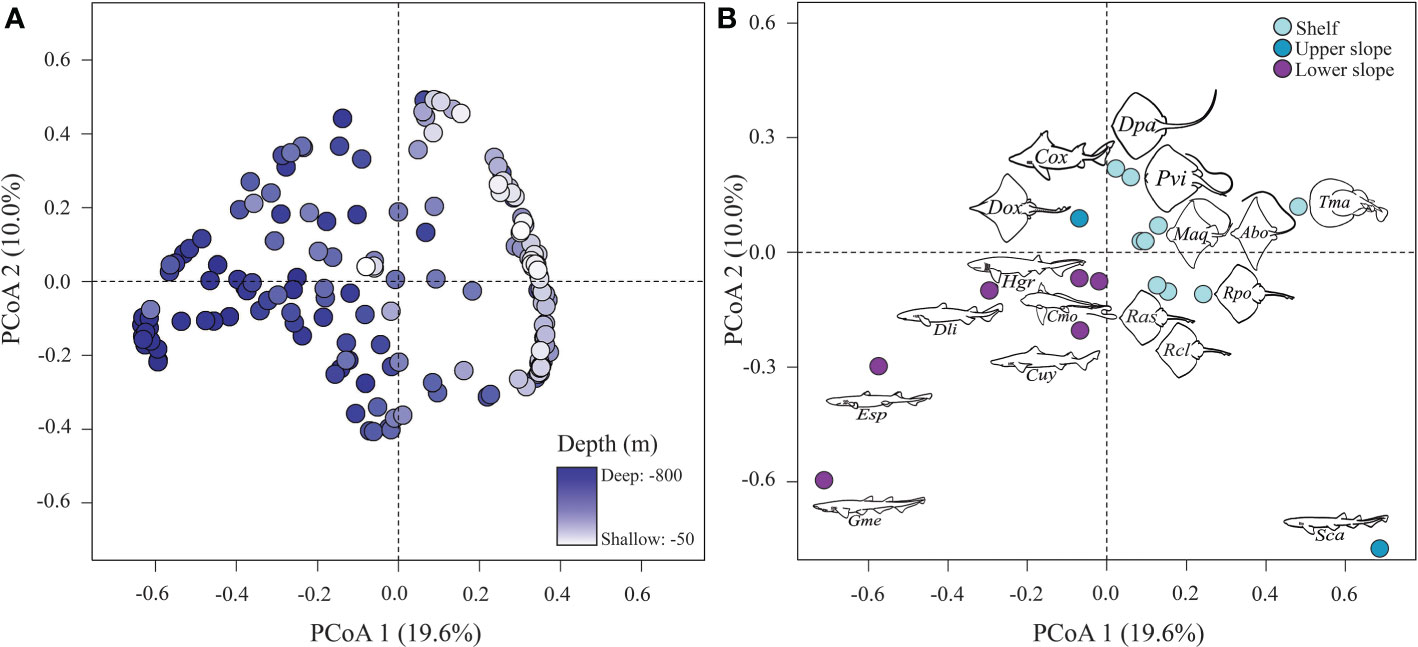
Figure 3 (A) Principal coordinate analysis, where the locations of points reflect compositional differences among hauls based on species-specific density data. The color of the points represents the mean depth of the haul. (B) The same ordination showing scores for each species driving differences in the composition of the community. Each point represent an species and is acompanyed by its ilustration and initials (the first letter for genus and the two other for species). Point colors represents the preferred depth range of the particular species accoding to COG analysis (Table 2).
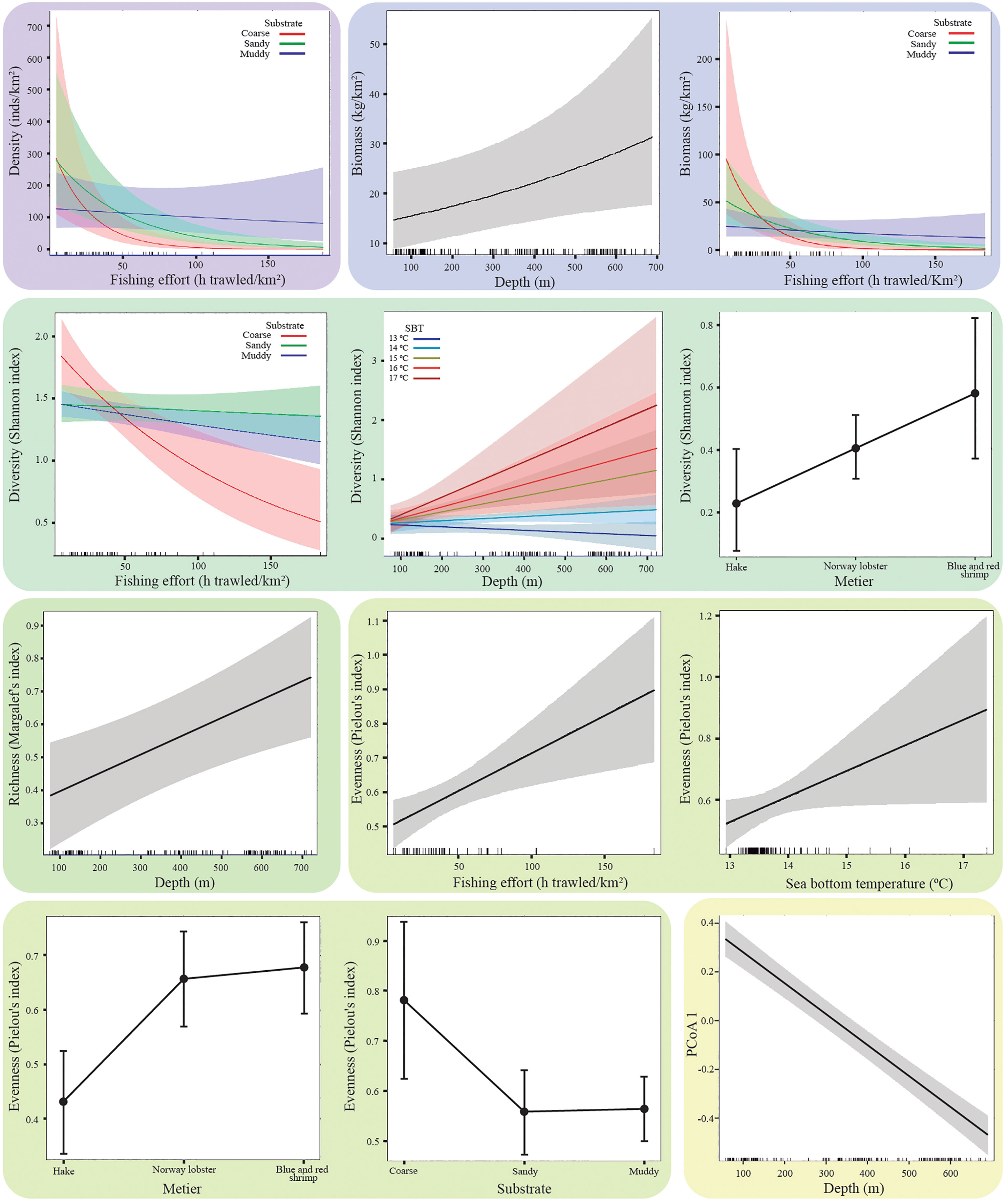
Figure 4 Partial effects of predictor variables included in the selected models for chondrichthyan density (inds/km2), biomass (kg/km2), diversity (Shannon index), richness (Margalef’s index), evenness (Pielou’s index) and the principal coordinate (PCoA) axis. The box of each plot is coloured referring to its respective response variable.
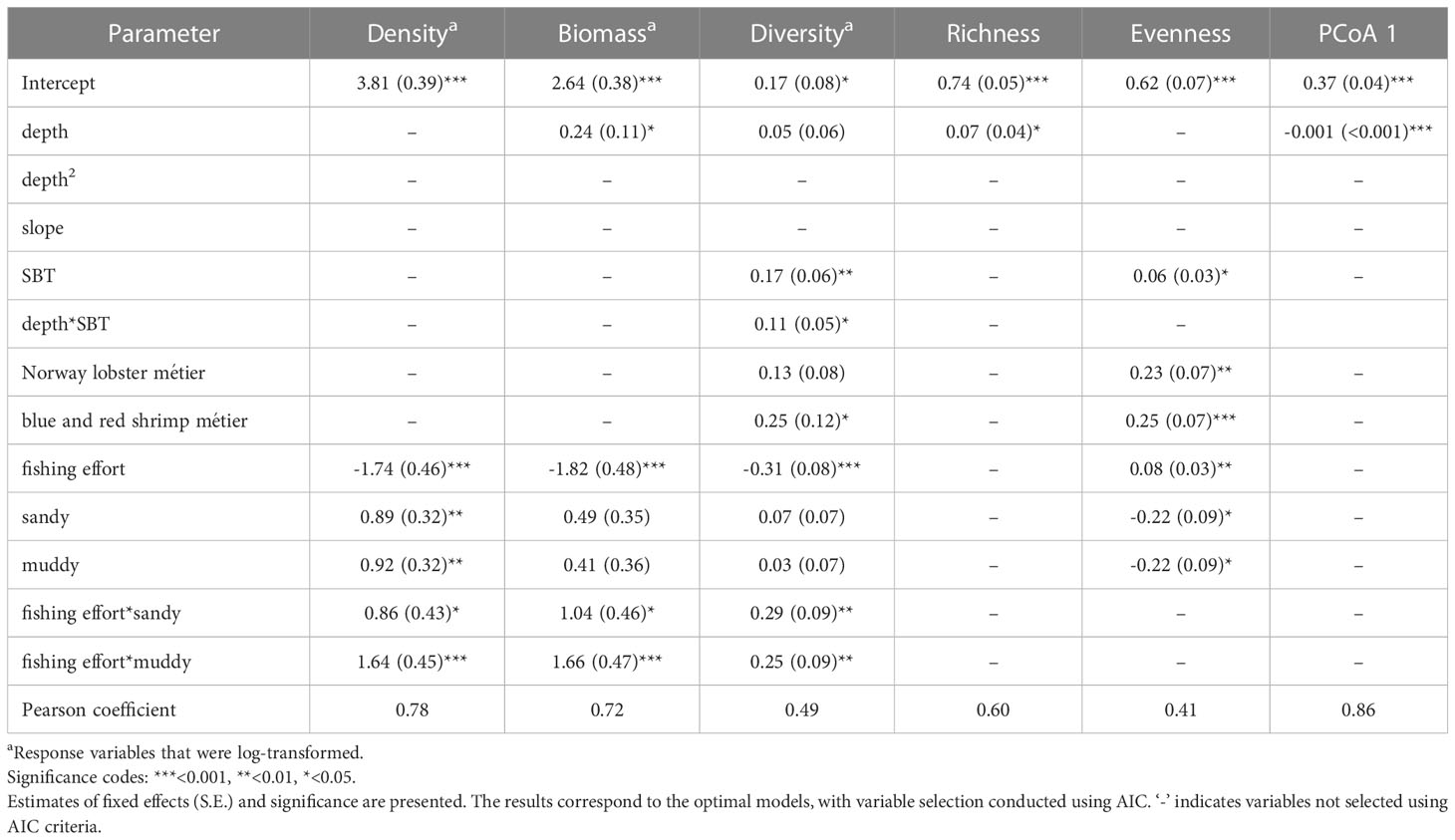
Table 3 Results of the generalized mixed effect models (GLMM) of the five community descriptor metrics and the first principal coordinate (PCoA) axis.
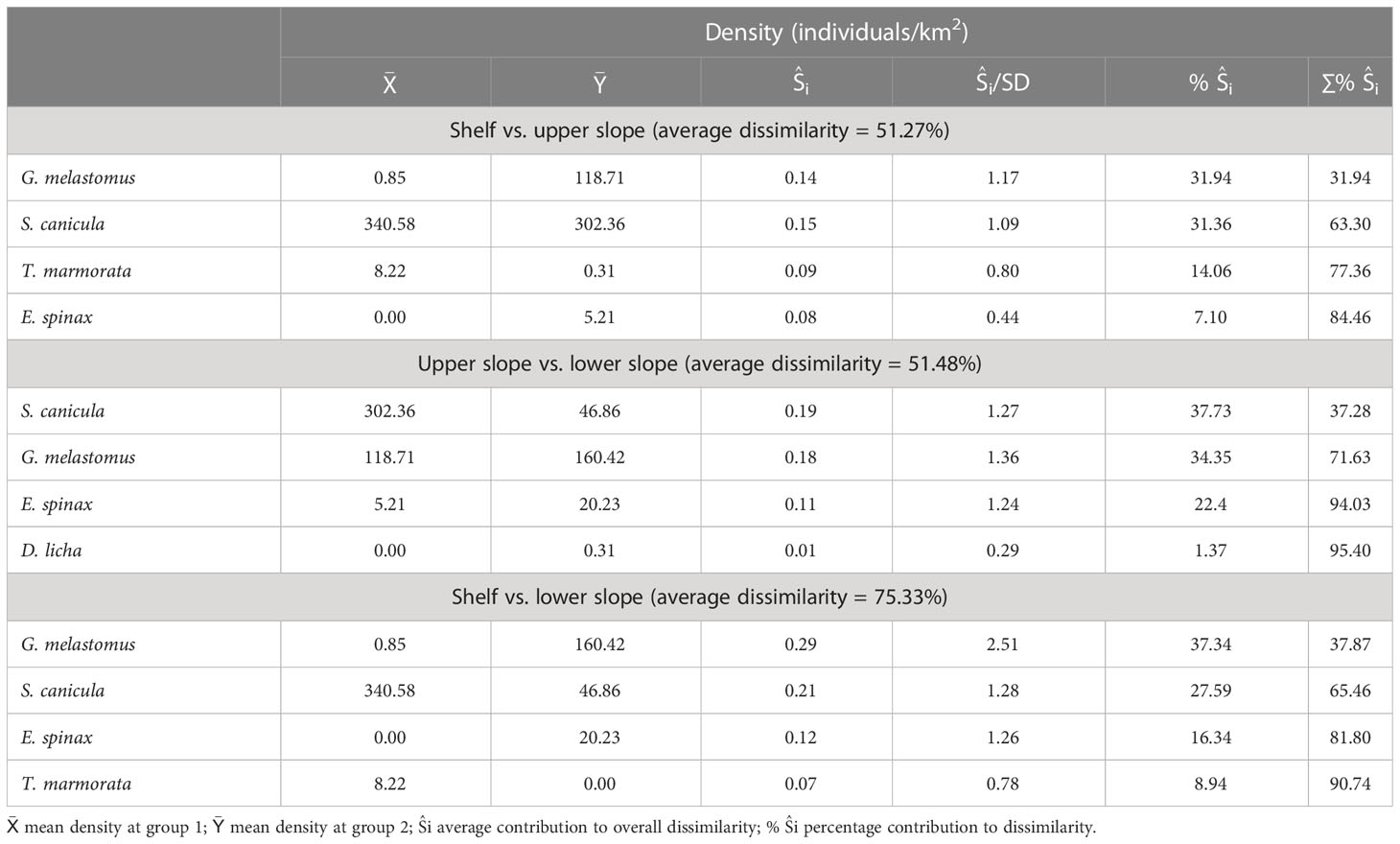
Table 4 Similarity percentages (SIMPER) analysis results for the comparison of the assemblages (continental shelf, upper slope, and lower slope) identified from principal coordinates analysis.
The overall density of chondrichthyan specimens, including all trawls regardless of whether they captured any chondrichthyans, was 252 ± 298 inds/km2. Density values ranged from 0 to 1498 inds/km2. The selected model for chondrichthyan density included the interaction between fishing effort and substrate. The effect of fishing effort on chondrichthyan density varied depending on the substrate, with a stronger negative effect of fishing pressure on sandy and coarse sediments compared to muddy sediments. Larger densities at low fishing effort occurred in sandy and coarse sediments but higher overall mean density occurred in muddy bottoms as density decreased more rapidly in sandy and coarse sediments with increasing fishing effort (Table 3; Figure 4).
The biomass of chondrichthyans averaged 44 ± 52 kg/km2 and ranged from 0 to 323 kg/km2. The selected model for chondrichthyan biomass included the depth and the interaction between fishing effort and substrate. The impact of fishing pressure on chondrichthyan biomass varied depending on the substrate, analogously as for density. In addition, biomass increased significantly with depth (Table 3; Figure 4).
Mean diversity using Shannon index was 0.37 ± 0.32, ranging from 0 to 1.1. The selected model for chondrichthyan diversity included the métier and the interactions between fishing effort and substrate, as well as between sea bottom temperature and depth. The effect of fishing pressure on chondrichthyan diversity varied depending on the substrate, with a stronger negative effect on coarse sediments, while its effect was milder for both sandy and muddy substrates. In all the cases diversity decreased with increasing fishing effort. The effect of sea bottom temperature on diversity also varied depending on depth, with major changes occurring at deeper areas where higher temperatures were associated with higher diversity. Temperature fluctuated more in the continental shelf, ranging from 12.9 to 16.6 °C, whereas in the slope it was more stable, ranging from 12.6 to 13.8 °C. In addition, chondrichthyan diversity varied depending on the métier. Higher diversity of chondrichthyans observed in the catches of the blue and red shrimp métier, followed by the Norway lobster métier, both métiers occurring in the continental slope. The diversity captured in the hake métier was lower, occurring mostly in the continental shelf (Table 3; Figure 4).
Richness (Margalef’s index) values for chondrichthyans in this study ranged from 0 to 1.95, averaging 0.51 ± 0.44, with a maximum of 5 different species found in a single haul. The selected model for chondrichthyan richness only included the predictor depth, having a positive effect (Table 3; Figure 4).
Evenness (Pielou’s index) averaged at 0.59 ± 0.28 and ranged between 0.04 and 1. The optimal model for chondrichthyan evenness included the predictor variables of fishing effort, sea bottom temperature, métier, and substrate. Evenness increased with higher levels of fishing effort and sea bottom temperature. It was lower for the hake métier and in sandy and muddy substrates (Table 3; Figure 4).
Discussion
Our study provides updated information on the status and composition of the chondrichthyan community in the Mediterranean trawling grounds of the eastern Iberian Peninsula (GSA06) and contributes to a deeper understanding of the drivers leading its structure and spatial distribution. A total of 17 species were recorded, including 7 sharks, 9 batoids, and 1 chimaera. The chondrichthyan catch was dominated by few species including S. canicula, G. melastomus, E. spinax and T. marmorata. In this study we provide bycatch rates for all the reported species by accurately depicting the unaltered practices of the local fleet. The bycatch rates provided for each species may aid to understand their current level of interaction with commercial bottom trawling. This information is essential to inform management plans, considering that most of the current research uses fishing-independent methods and most of the chondrichthyan biomass captured is either not landed or labelled incorrectly in fisheries statistics (Barría and Colmenero, 2019; Cashion et al., 2019). The species composition, their relative abundance and the values of the analyzed community descriptors are consistent with what has been described in recent years using fisheries-independent research in the same region (Ramírez-Amaro et al., 2015; Follesa et al., 2019; Ramírez-Amaro et al., 2020). Although, chondrichthyan diversity has been historically considered greatest in the western basin, the demersal community inhabiting in the bottom trawling grounds has been suggested to be higher in the Strait of Sicily and the Aegean Sea, located in the central and eastern Mediterranean, respectively, while little is known about the African Mediterranean (Dulvy et al., 2016; Follesa et al., 2019; Serena et al., 2020). We found that the distribution and structure of the chondrichthyan community is influenced by environmental drivers such as depth, temperature, and seabed substrate, but also by the fishing pressure exerted in the area.
Environmental drivers
Depth was found to be the most important factor in shaping chondrichthyan community composition. Most of the batoids (A. bovinus, M. aquila, Raja asterias, R. clavata, R. polystigma, T. marmorata, D. pastinaca, and Pteroplatytrygon violacea) were found in the continental shelf (50-200 m depth). In contrast, most sharks (G. melastomus, E. spinax, D. licha, and H. griseus), preferred the deepest part of the continental slope (>500 m depth). The skate D. oxyrinchus, the shark C. uyato, and the chimaera C. monstrosa were primarily found in the upper continental slope (200-500 m depth). S. canicula was present all along the studied bathymetrical range although its abundance was higher in the continental shelf and upper slope, with center of gravity at about 250 m depth. Chondrichthyan biomass increased with depth although density did not exhibit any significant trend, indicating that the specimens in deeper areas had a larger body biomass. Diversity and richness indices also increased with depth. Similar patterns have been reported by previous research using fisheries-independent trawling surveys in Northern Spain, finding higher chondrichthyan diversity in the slope, as well as in the Aegean Sea and in the Strait of Sicily, where the diversity in the chondrichthyan community peaked at 600 m deep (Lauria et al., 2015; Ramírez-Amaro et al., 2015; Peristeraki et al., 2020). However, in other subregions the peak in chondrichthyan diversity has been detected in shallower areas as in the Balearic Sea, where it occurs in the continental shelf, condition that has been attributed to a lower fishing pressure occurring in the continental shelf of the Balearic Sea (Gouraguine et al., 2011; Ramírez-Amaro et al., 2015). The changes in the species composing the community in relation to depth is related to the niches available to each species (including habitat and inter and intra-specific competition) depending on its adaptations to the different parameters that vary with depth (e.g. light, temperature, currents, pressure, food, oxygen, substrate) (Gage and Tyler, 1991). Therefore, the higher diversity levels in the slope cannot be disregarded as a result of the more extensive depth range covered by each haul in this region. However, lower diversity levels in the continental shelf may be related to the fishing pressure exerted in the area as suggested in prior research (Aldebert, 1997; Ramírez-Amaro et al., 2015). Fishing pressure in Northern Spain decreases with depth, being higher in the continental shelf, especially in areas located near large ports and cities, likely influenced by the market demands, as in other areas of the Mediterranean (Peristeraki et al., 2020). Additionally, fisheries beyond 1,000 m depth are banned since 2006 as decided by the GFCM. The generally lower fishing pressure in the continental slope may provide deepwater chondrichthyans with certain refuge from anthropogenic pressures, maintaining higher diversity levels (García et al., 2008). However, a shift of the fleet towards deepwaters has occurred in the western Mediterranean during the last decades consequence of technological improvement of fishing activities, the overexploitation status of the continental shelf stocks and the higher economic value of the targets N. norvegicus and A. antennatus (Moranta et al., 2008; Fernandes et al., 2017). It has been suggested that this shift has promoted certain stability for the chondrichthyans inhibiting in the Mediterranean continental shelf (Marongiu et al., 2017; Ramírez-Amaro et al., 2020), but continued elevated fishing pressure may drive to declining population trends for the species dwelling in the slope, as it has been observed for E. spinax and D. oxyrinchus in Northern Spain (Ramírez-Amaro et al., 2020). Special caution must be taken with deepwater species considering their longer turnover times (i.e. slower growth, later age at maturity and higher longevity; Pardo and Dulvy, 2022).
Sea bottom temperature has been previously suggested as a relevant driver of chondrichthyan habitat selection (e.g. Follesa et al., 2019; Maioli et al., 2022). In the Mediterranean, increased shark densities have been previously documented at higher bottom temperatures using fisheries-independent trawling surveys (Follesa et al., 2019), although species-specific patterns and ontogenetic shifts also occur (Damalas et al., 2010; Maioli et al., 2022). As chondrichthyans are ectothermic, changes in the environmental temperature affect their physiological processes (Brown et al., 2004; Savage et al., 2004). Higher temperatures speed chemical and metabolic reactions as hypothesized by metabolic theory, which may lead to a redistribution of chondrichthyans to thermoregulate (Osgood et al., 2021). Temperature decreases with depth and so does light, oxygen, and primary production, and consequently, food availability for chondrichthyans (Gage and Tyler, 1991; Jahnke, 1996; Rex et al., 2006). Thus, the metabolic capacity of chondrichthyans also generally decreases with depth (Seibel and Drazen, 2007; Pardo and Dulvy, 2022), limiting their energetic capabilities to forage in an environment where food availability is generally low. Our study indicates that sea bottom temperature is a significant predictor for chondrichthyan diversity and evenness. Particularly, the effect of bottom temperature upon diversity was remarkable at major depths where diversity is higher in the slightly warmer areas. Although temperature in the slope was fairly stable, it ranged from 12.6 to 13.8 °C. Slightly warmer waters in deeper areas may enable for a higher activity in chondrichthyans, attracting a larger diversity of species (Grady et al., 2019). Additionally, temperature may also affect the availability of prey which in turn affects chondrichthyan distribution (Cotton et al., 2005; Lauria et al., 2015). Our study supports previous insights highlighting the importance of sea bottom temperature on structuring the chondrichthyan community, however, continued research and monitoring on the effects of this driver over extended time periods is essential to understand potential effects of global change. As most species are narrowly thermally adapted, it must be considered that increasing temperatures due to ocean warming may influence species distribution and abundance, potentially shifting their habitat ranges to select deeper and cooler waters in order to enhance physiological processes, potentially entailing important changes in the community dynamics (Sunday et al., 2012; Osgood et al, 2021; Vilmar and Di Santo, 2022).
Habitat preferences of demersal chondrichthyan species have been previously found to be influenced by the type of seabed substrate (e.g. Pennino et al., 2013; Lauria et al., 2015). Additionally, these preferences may change as the species undergoes ontogeny, potentially following the distribution of their preferred prey (Martin et al., 2012). In the Strait of Sicily, higher richness has been documented in coarse sediments using trawling surveys, although certain species including R. clavata, Mustelus mustelus and Squalus blainvillei preferred fine sediments (Lauria et al., 2015). Similarly, in the Adriatic R. clavata, T. marmorata and Scyliorhinus stellaris have been associated to muddy environments, while S. canicula and R. miraletus to sandy substrates (Maioli et al., 2022). In the southern region of the GSA06 the sharks S. canicula, G. melastomus and E. spinax have been documented to prefer sandy substrates rather than muddy bottoms using commercial trawling data (Pennino et al., 2013). Our results indicate that sandy and coarse seabeds in Northern Spain may host a higher abundance and diversity of chondrichthyans at low fishing pressure levels. However, as fishing effort increased in sandy and coarse sediment areas, these levels drop rapidly in comparison to muddy environments, where declining occurred more progressively. This pattern is more marked for abundance than for diversity levels where sandy bottoms exhibited a similar trend to muddy bottoms, but in both cases resulted in larger overall mean values in muddy sediments. As areas with hard sediments are usually not trawlable, they may constitute important de facto refugia for demersal chondrichthyans as it has been previously suggested (Shephard et al., 2012). However, chondrichthyans in these areas may be more abruptly affected by increased fishing effort than muddy bottoms. The use of remotely operated vehicles (ROVs) constitutes a useful tool to acquire a better understanding of the importance of hard substrate areas for chondrichthyans. Certainly, prior research using this technology indicates that hard substrate areas may provide shelter for chondrichthyans and act as nurseries for some demersal species (e.g. Treude et al., 2011; Henry et al., 2013; Micarelli and Reinero, 2021). However, many of these areas remain poorly researched.
The differential effect of muddy sediments with increasing fishing effort may be a consequence of the indirect effects of trawling, which are particularly important in these environments (Collie et al., 2017). Sediment resuspension rates are higher in muddy bottoms, usually found in areas of low natural disturbance where the time to sediment again is generally larger (Duplisea et al., 2001; Kaiser et al., 2006). The physical disturbance caused during trawling in these areas may increase food availability for mesopredators in the short-term, displacing burial biota, increasing carrion, and resuspending organic matter (Link and Almeida, 2002; Collie et al., 2017). Although, the long-term consequences are not well-known and may depend on the species’ feeding habits and the environmental conditions (Collie et al., 2017), chronically trawled sediments in the north-western Mediterranean have experimented significant decreases in organic matter (Pusceddu et al., 2014). Thus, although high fishing effort in muddy environments may lead to depletion, low to medium fishing efforts may retain mesopredators if they are attracted to the short-term pulses of food (Hiddink et al., 2006; Hinz et al., 2009; Collie et al., 2017). A correlation between the abundance of G. melastomus and turbid areas caused by high levels of natural vorticity, has been described in the north-western Mediterranean (Amores et al., 2014). Certainly, chronic trawling along the continental slope of the Northern Spain was reported to cause a similar effect on resuspension and turbidity as natural high levels of vorticity (Paradis et al., 2017; Arjona-Camas et al., 2021). Therefore, we hypothesise that the resuspension effect may mask the direct impact of fishing in muddy areas. However, in all cases higher levels of fishing effort led to changes in the chondrichthyan community structure with declining abundance and diversity and increasing evenness. Nevertheless, it must be considered that trawling surveys are mostly limited to soft substrate, being harder substrate areas not trawlable. Thus, the observed distribution patterns may be partially influenced by limitations in the sampling procedure when using trawling data.
Fishing effects
Our results indicate significant declines in density, biomass, diversity and evenness of chondrichthyans as fishing effort increases. This is in line with previous studies, supporting the idea that fishing effort contributes importantly into shaping the community of chondrichthyans (Navarro et al., 2016; Peristeraki et al., 2020). Fishing effort played an important role in the modification of the community during the early exploitation period (1960-1990). In the north-western Mediterranean, some of the currently most threatened species were commonly caught during the 1950s, including from more to less common: Squalus spp., S. stellaris, Mustelus spp., Leucoraja circularis, Galeorhinus galeus, O. centrina, Leucoraja melitensis, Rhinobatos spp., and Squatina spp. (Maurin, 1968; Aldebert, 1997; Jukic-Peladic et al., 2001). These species are now rare catch in bottom trawling or locally extinct species as their life-history parameters and distribution patterns made them especially susceptible to overexploitation (Walls and Dulvy, 2020; Walls and Dulvy, 2021). After the 2000s, it has been suggested that the GSA06 reached certain stability in terms of diversity (Ramírez-Amaro et al., 2020). Nevertheless, the current species-specific patterns still suggest a trend towards community simplification, with declining population trends generally occurring for species with lower intrinsic rates of population growth (Ligas et al., 2013; Marongiu et al., 2017; Ramírez-Amaro et al., 2020; Walls and Dulvy, 2020; Walls and Dulvy, 2021). Even though most of these chondrichthyan species are discarded due to their low economical value or protection status, their survival rates are likely to be low (Ellis et al., 2017). Further research on post-capture mortality and handling methods to enhance survival are key to inform management plans.
On the other hand, some species such as S. canicula, G. melastomus, T. marmorata and R. clavata exhibit generally increasing population trends in the north-western Mediterranean (Ligas et al., 2013; Marongiu et al., 2017; Ramírez-Amaro et al., 2020). These species can withstand the effects of fishing effort, potentially taking the released niches by accounting with higher rates of intrinsic population growth and post-capture survival (Revill et al., 2005; Ellis et al., 2017; Walls and Dulvy, 2020; Walls and Dulvy, 2021). Additionally, some of them may take advantage of fisheries discards (Olaso et al., 2002). This is in line with the elevated abundance levels that were detected for these species and especially, for S. canicula and G. melastomus, in the fishing grounds studied here. In general, the Mediterranean chondrichthyan communities are shifting towards the predominance of species with lower age at maturity and higher reproductive rates as result of the selection force occasioned by the elevated fishing pressure (Ramírez-Amaro et al., 2020; Maioli et al., 2022). Furthermore, there is evidence of decreasing size at maturity in S. canicula and G. melastomus in the Spanish Mediterranean during recent decades, potentially as adaptative response to overfishing (Ramírez-Amaro et al., 2020). These tendencies indicate that fishing pressure have had an important role in shaping the current structure of the chondrichthyan community, acting as a selective pressure in the Mediterranean and, particularly, in our study region. The results from this study are in line with these insights, revealing that the abundance and diversity of chondrichthyans decreases importantly in areas with increased fishing pressure. Considering that the GSA06 is one of the most exploited sub-areas in the Mediterranean (Armelloni et al., 2021), continued research in this region is essential to monitor changes and inform management measures. Particular attention must be given to species exhibiting decreasing population trends, but also to species that are generally commercialised, such as S. canicula, G. melastomus, and the species of the genus Raja spp. as the overexploitation of many fish stocks may lead to an increase in the catch and retain of species with lower economical interest, including these (Davidson et al., 2016).
Future research may consider both species and ontogenetic-stage specific distribution modelling approaches with additional detail in both environmental and human factors to further understand the drivers of their distribution and the potential effects of increased fishing pressure, global change, and habitat loss. Furthermore, it is crucial to improve chondrichthyan-focused fisheries management in the Mediterranean to halt the declines of these species and prevent regional species extinctions (Colloca et al., 2013; Fernandes et al., 2017; Walls and Dulvy, 2021). Although, there have been diverse suggestions for addressing the overfishing problems in the Mediterranean fisheries, only few have been effectively implemented to protect sharks, rays, and chimaeras (Colloca et al., 2013; Walls and Dulvy, 2021).
Conclusions
This study revealed the importance of combining environmental and fishing-related drivers to understand the spatial distribution patterns of chondrichthyan communities. Our results indicate that depth is the main driver of the composition of the community in the studied bottom trawling fishing grounds (ranging from 50 to 800 m deep). Deeper areas host a higher diversity of chondrichthyans species, potentially related to the higher fishing effort exerted in the continental shelf. The distribution of the chondrichthyan community is also influenced by sea bottom temperature with slight variances in temperature in deeper areas entailing significant changes in diversity, and by substrate type, with different sediment types exhibiting different responses to increased fishing effort. However, in all cases, density, biomass, and diversity of chondrichthyans is importantly affected by the intensity of fishing pressure, with higher pressure leading to declining values. These insights are crucial to further understand the effects of increased fishing effort on the chondrichthyan community as well as the potential effects of global change and habitat loss. Our approach provide capacity to make more accurate predictions when determining thresholds for sustainable practices and estimating the situation for chondrichthyans where data is scarce or absent. This may aid to build management capacity in poorly researched areas and prevent future threats. Additionally, our work highlights the need for continued research and monitoring in this area, given the ongoing challenges and uncertainties that the Mediterranean chondrichthyans and their ecosystems are facing. It is crucial to improve chondrichthyan-focused fisheries management in the Mediterranean to halt the declines of these species and prevent regional species extinctions.
Data availability statement
The raw data supporting the conclusions of this article will be made available by the authors, without undue reservation.
Ethics statement
Ethical review and approval was not required for the animal study because the chondrichthyan specimens analyzed in the present work were obtained from commercial fisheries. The activity was conducted with the observation of the Regulation of the European Parliament and the Council for fishing in the General Fisheries Commission for the Mediterranean (GFCM) Agreement area and amending Council Regulation (EC) No. 1967/2006. This Regulation is de facto the unique authorization needed to conduct this type of activity.
Author contributions
CB, JR, and DR-G conceived and designed the study. JR, DR-G, and CB acquired funding and administrated the project. DR-G, AC, JC, LR, and CB contributed to data collection. DR-G, DM, and FQ performed the statistical analysis. DR-G wrote the draft of the manuscript. All authors contributed to the article and approved the submitted version.
Funding
This research was supported by the Biodiversity Foundation of the Ministry for the Ecological Transition and Demographic Challenge under the project ECEME (CA_BM_2019). This work was supported by the Catalan Institute for Ocean Governance Research (ICATMAR), trawl surveys were financed by European Maritime and Fisheries Fund (EMFF) and the Catalan General Directorate of Marine Policy and Sustainable Fisheries by project and CGCAT (ARP140/20/000006). DR-G was supported by a FPU grant of the Spanish Ministry of Universities (MIU). DM acknowledges support from the CIDEGENT program of the Generalitat Valenciana (CIDEGENT/2021/058).
Acknowledgments
We thank the Conselleria de Agricultura, Desarrollo Rural, Emergencia Climática y Transición Ecológica of the Generalitat Valenciana, the Consejería de Agua, Agricultura, Ganadería, Pesca y Medio Ambiente de la Región de Murcia and the Direcció General de Pesca i Afers Marítims de la Generalitat de Catalunya (Spain). We thank the fisheries associations from Catalonia, El Grao de Castellón, Cullera, Jávea, Calpe and Cartagena and particularly to the skippers and the crew from the F/V participating in the data collection. We thank all ICATMAR technicians who helped with the data collection and Miguel Ángel Ruiz Ramírez for ceding the chondrichthyan illustrations.
Conflict of interest
The authors declare that the research was conducted in the absence of any commercial or financial relationships that could be construed as a potential conflict of interest.
Publisher’s note
All claims expressed in this article are solely those of the authors and do not necessarily represent those of their affiliated organizations, or those of the publisher, the editors and the reviewers. Any product that may be evaluated in this article, or claim that may be made by its manufacturer, is not guaranteed or endorsed by the publisher.
Supplementary material
The Supplementary Material for this article can be found online at: https://www.frontiersin.org/articles/10.3389/fmars.2023.1145176/full#supplementary-material
References
Aldebert Y. (1997). Demersal resources of the gulf of lions (NW mediterranean). impact of exploitation on fish diversity. Vie Milieu. 47, 275–284.
Amores A., Rueda L., Monserrat S., Guijarro B., Pasqual C., Massutí E. (2014). Influence of the hydrodynamic conditions on the accessibility of Aristeus antennatus and other demersal species to the deep water trawl fishery off the Balearic islands (western Mediterranean). J. Mar. Syst. 138, 203–210. doi: 10.1016/j.jmarsys.2013.11.014
Arjona-Camas M., Puig P., Palanques A., Durán R., White M., Paradis S., et al. (2021). Natural vs. trawling-induced water turbidity and suspended sediment transport variability within the palamós canyon (NW Mediterranean). Mar. Geophys. Res. 42, 1–22. doi: 10.1007/s11001-021-09457-7
Armelloni E. N., Tassetti A. N., Ferrà C., Galdelli A., Scanu M., Mancini A., et al. (2021). AIS data, a mine of information on trawling fleet mobility in the Mediterranean Sea. Mar. Policy 129, 104571. doi: 10.1016/j.marpol.2021.104571
Barausse A., Correale V., Curkovic A., Finotto L., Riginella E., Visentin E., et al. (2014). The role of fisheries and the environment in driving the decline of elasmobranchs in the northern Adriatic Sea. ICES J. Mar. Sci. 71, 1593–1603. doi: 10.1093/icesjms/fst222
Barría C., Coll M., Navarro J. (2015a). Unravelling the ecological role and trophic relationships of uncommon and threatened elasmobranchs in the western Mediterranean Sea. Mar. Ecol. Prog. Ser. 539, 225–240. doi: 10.3354/meps11494
Barría C., Colmenero A. I. (2019). La pesca de tiburones y rayas en el noroeste del mediterráneo, una situación compleja. dA. 10, 105–111. doi: 10.5565/rev/da.468
Barría C., Navarro J., Coll M., Fernandez-Arcaya U., Sáez-Liante R. (2015b). Morphological parameters of abundant and threatened chondrichthyans of the northwestern Mediterranean Sea. J. Appl. Ichthyol. 31, 114–119. doi: 10.1111/jai.12499
Bates D., Mächler M., Bolker B., Walker S. (2015). Fitting linear mixed-effects models using lme4. J. Stat. Software 67, 1–48. doi: 10.18637/jss.v067.i01
Bertrand J., Gil-de-Sola-Simarro L., Papakonstantinou C., Relini G., Souplet A. (2000). Contribution on the distribution of elasmobranchs in the Mediterranean (from MEDITS surveys). Biol. Mar. Medit. 7, 1–15.
Bolker B. M., Brooks M. E., Clark C. J., Geange S. W., Poulsen J. R., Stevens M. H. H., et al. (2009). Generalized linear mixed models: a practical guide for ecology and evolution. Trends Ecol. Evol. 24, 127–135. doi: 10.1016/j.tree.2008.10.008
Brown J. H., Gillooly J. F., Allen A. P., Savage V. M., West G. B. (2004). Toward a metabolic theory of ecology. Ecology 85, 1771–1789. doi: 10.1890/03-9000
Cashion M. S., Bailly N., Pauly D. (2019). Official catch data underrepresent shark and ray taxa caught in Mediterranean and black Sea fisheries. Mar. Policy 105, 1–9. doi: 10.1016/j.marpol.2019.02.041
Collie J., Hiddink J. G., van Kooten T., Rijnsdorp A. D., Kaiser M. J., Jennings S., et al. (2017). Indirect effects of bottom fishing on the productivity of marine fish. Fish Fish. 18, 619–637. doi: 10.1111/faf.12193
Colloca F., Cardinale M., Maynou F., Giannoulaki M., Scarcella G., Jenko K., et al. (2013). Rebuilding Mediterranean fisheries: A new paradigm for ecological sustainability. Fish Fish. 14, 89–109. doi: 10.1111/j.1467-2979.2011.00453.x
Colloca F., Scarcella G., Libralato S. (2017). Recent trends and impacts of fisheries exploitation on Mediterranean stocks and ecosystems. Front. Mar. Sci. 4. doi: 10.3389/fmars.2017.00
Cortés E. (2000). Life history patterns and correlations in sharks. Rev. Fish. Sci. 8, 299–344. doi: 10.1080/10408340308951115
Cotton P. A., Sims D. W., Fanshawe S., Chadwick M. (2005). The effects of climate variability on zooplankton and basking shark (Cetorhinus maximus) relative abundance off southwest Britain. Fish. Oceanogr. 14, 151–155. doi: 10.1111/j.1365-2419.2005.00331.x
Damalas D., Maravelias C. D., Katsanevakis S., Karageorgis A. P., Papaconstantinou C. (2010). Seasonal abundance of non-commercial demersal fish in the eastern Mediterranean Sea in relation to hydrographic and sediment characteristics. Estuar. Coast. Shelf Sci. 89, 107–118. doi: 10.1016/j.ecss.2010.06.002
Damalas D., Vassilopoulou V. (2011). Chondrichthyan by-catch and discards in the demersal trawl fishery of the central Aegean Sea (Eastern Mediterranean). Fish. Res. 108, 142–152. doi: 10.1016/j.fishres.2010.12.012
Davidson L. N., Krawchuk M. A., Dulvy N. K. (2016). Why have global shark and ray landings declined: improved management or overfishing? Fish Fish. 17, 438–458. doi: 10.1111/faf.12119
De Buen F. (1935). “Fauna ictiológica,” in Catálogo de los peces ibéricos: de la planicie continental, aguas dulces, pelágicos y de los abismos próximos (Madrid: Instituto Español de Oceanografía: Primera Parte).
Dormann C. F. (2007). Effects of incorporating spatial autocorrelation into the analysis of species distribution data. Global Ecol. Biogeogr. 16, 129–138. doi: 10.1111/j.1466-8238.2006.00279.x
Dulvy N. K., Allen D. J., Ralph G. M., Walls R. (2016). The conservation status of sharks, rays and chimaeras in the Mediterranean Sea [Brochure] (Malaga, Spain: IUCN).
Dulvy N. K., Pacoureau N., Rigby C. L., Pollom R. A., Jabado R. W., Ebert D. A., et al. (2021). Overfishing drives over one-third of all sharks and rays toward a global extinction crisis. Curr. Biol. 31, 4773–4787.e8. doi: 10.1016/j.cub.2021.08.062
Duplisea D. E., Jennings S., Malcolm S. J., Parker R., Sivyer D. B. (2001). Modelling potential impacts of bottom trawl fisheries on soft sediment biogeochemistry in the north Sea. Geochem. Trans. 14, 1–6. doi: 10.1186/1467-4866-2-112
Ellis J. R., McCully Phillips S. R., Poisson F. (2017). A review of capture and post-release mortality of elasmobranchs. J. Fish Biol. 90, 653–722. doi: 10.1111/jfb.13197
FAO (2020). “The state of Mediterranean and black Sea fisheries 2020,” in General fisheries commission for the Mediterranean. (Rome: Food and Agriculture Organization of the United Nations). doi: 10.4060/cb2429en
Fernandes P. G., Ralph G. M., Nieto A., García Criado M., Vasilakopoulos P., Maravelias C. D., et al. (2017). Coherent assessments of europe’s marine fishes show regional divergence and megafauna loss. Nat. Ecol. Evol. 1, 1–9. doi: 10.1038/s41559-017-0170
Ferretti F., Myers R. A., Serena F., Lotze H. K. (2008). Loss of large predatory sharks from the Mediterranean Sea. Conserv. Biol. 22, 952–964. doi: 10.1111/j.1523-1739.2008.00938.x
Ferretti F., Osio G. C., Jenkins C. J., Rosenberg A. A., Lotze H. K. (2013). Long-term change in a meso-predator community in response to prolonged and heterogeneous human impact. Sci. Rep. 3, 1–11. doi: 10.1038/srep01057
Follesa M. C., Marongiu M. F., Zupa W., Bellodi A., Cau A., Cannas R., et al. (2019). Spatial variability of Chondrichthyes in the northern Mediterranean. Sci. Mar. 83, 81–100. doi: 10.3989/scimar.04998.23A
Gage J. D., Tyler P. A. (1991). Deep-Sea biology: A natural history of organisms at the deep-Sea floor (UK: Cambridge University Press).
García V. B., Lucifora L. O., Myers R. A. (2008). The importance of habitat and life history to extinction risk in sharks, skates, rays and chimaeras. Proc. R. Soc B. 275, 83–89. doi: 10.1098/rspb.2007.1295
Geraci M. L., Ragonese S., Scannella D., Falsone F., Gancitano V., et al. (2021). Batoid abundances, spatial distribution, and life history traits in the strait of Sicily (central Mediterranean sea): Bridging a knowledge gap through three decades of survey. Animals 11, 2189. doi: 10.3390/ani11082189
Gouraguine A., Hidalgo M., Moranta J., Bailey D. M., Ordines F., Guijarro B., et al. (2011). Elasmobranch spatial segregation in the western Mediterranean. Sci. Mar. 75, 653–664. doi: 10.3989/scimar.2011.75n4653
Grady J. M., Maitner B. S., Winter A. S., Kaschner K., Tittensor D. P., Record S., et al. (2019). Metabolic asymmetry and the global diversity of marine predators. Science 363, eaat4220. doi: 10.1126/science.aat4220
Guijarro B., Quetglas A., Moranta J., Ordines F., Valls M., González N., et al. (2012). Inter-and intra-annual trends and status indicators of nektobenthic elasmobranchs off the Balearic islands (northwestern Mediterranean). Sci. Mar. 76, 87–96. doi: 10.3989/scimar.03432.22A
Heithaus M. R., Frid A., Wirsing A. J., Worm B. (2008). Predicting ecological consequences of marine top predator declines. Trends Ecol. Evol. 23, 202–210. doi: 10.1016/j.tree.2008.01.003
Henry L. A., Navas J. M., Hennige S. J., Wicks L. C., Vad J., Roberts J. M. (2013). Cold-water coral reef habitats benefit recreationally valuable sharks. Biol. Conserv. 161, 67–70. doi: 10.1016/j.biocon.2013.03.002
Hiddink J. G., Jennings S., Kaiser M. J., Queirós A. M., Duplisea D. E., Piet G. J. (2006). Cumulative impacts of seabed trawl disturbance on benthic biomass, production, and species richness in different habitats. Can. J. Fish. Aquat. Sci. 63, 721–736. doi: 10.1139/f05-266
Hijmans R. (2022). Raster: Geographic data analysis and modeling. R Package version 3., 6–11. Available at: https://cran.r-project.org/web/packages/raster/raster.pdf.
Hinz H., Prieto V., Kaiser M. J. (2009). Trawl disturbance on benthic communities: chronic effects and experimental predictions. Ecol. Appl. 19, 761–773. doi: 10.1890/08-0351.1
IUCN (2022) The IUCN red list of threatened species (Version 2022-2). Available at: https://www.iucnredlist.org (Accessed January 15, 2023).
Jahnke R. A. (1996). The global ocean flux of particulate organic carbon: Areal distribution and magnitude. Global Biogeochem. Cycles 10, 71–88. doi: 10.1029/95GB03525
Jukic-Peladic S., Vrgoc N., Krstulovic-Sifner S., Piccinetti C., Piccinetti-Manfrin G., Marano G., et al. (2001). Long-term changes in demersal resources of the Adriatic Sea: comparison between trawl surveys carried out in 1948 and 1998. Fish. Res. 53, 95–104. doi: 10.1016/S0165-7836(00)00232-0
Kaiser M. J., Clarke K. R., Hinz H., Austen M. C., Somerfield P. J., Karakassis I. (2006). Global analysis of response and recovery of benthic biota to fishing. Mar. Ecol. Prog. Ser. 311, 1–14. doi: 10.3354/meps311001
Koehler L., Giovos I., Lowther J. (2022). The application of precaution in elasmobranch conservation and management in the Mediterranean Sea. Mar. Policy 135, 104830. doi: 10.1016/j.marpol.2021.104830
Kuznetsova A., Brockhoff P. B., Christensen R. H. B. (2017). lmerTest package: Tests in linear mixed effects models. J. Stat. Software 82, 1–26. doi: 10.18637/jss.v082.i13
Lauria V., Gristina M., Attrill M., Garofalo G. (2015). Predictive habitat suitability models to aid conservation of elasmobranch diversity in the central Mediterranean Sea. Sci. Rep. 5, 13245. doi: 10.1038/srep13245
Ligas A., Osio G. C., Sartor P., Sbrana M., De Ranieri S. (2013). Long-term trajectory of some elasmobranch species off the Tuscany coasts (NW Mediterranean) from 50 years of catch data. Sci. Mar. 77, 119–127. doi: 10.3989/scimar.03654.21C
Link J. S., Almeida F. P. (2002). Opportunistic feeding of longhorn sculpin (Myoxocephalus octodecemspinosus): Are scallop fishery discards an important food subsidy for scavengers on georges bank? Fish. Bull. 100, 381–385.
Lucifora L. O., García V. B., Menni R. C., Worm B. (2012). Spatial patterns in the diversity of sharks, rays, and chimaeras (Chondrichthyes) in the southwest Atlantic. Biodivers. Conserv. 21, 407–419. doi: 10.1007/s10531-011-0189-7
Maioli F., Weigel B., Chiarabelli E., Manfredi C., Anibaldi A., Isailović I., et al. (2022)Influence of ecological traits on spatio-temporal dynamics of an elasmobranch community in a heavily exploited basin (Accessed January 15, 2023).
Maravelias C. D., Tserpes G., Pantazi M., Peristeraki P. (2012). Habitat selection and temporal abundance fluctuations of demersal cartilaginous species in the Aegean Sea (eastern Mediterranean). PloS One 7, e35474. doi: 10.1371/journal.pone.0035474
Marongiu M. F., Porcu C., Bellodi A., Cannas R., Cau A., Cuccu D., et al. (2017). Temporal dynamics of demersal chondrichthyan species in the central western Mediterranean Sea: The case study in Sardinia island. Fish. Res. 193, 81–94. doi: 10.1016/j.fishres.2017.04.001
Martin C. S., Vaz S., Ellis J. R., Lauria V., Coppin F., Carpentier A. (2012). Modelled distributions of ten demersal elasmobranchs of the eastern English channel in relation to the environment. J. Exp. Mar. Biol. Ecol. 418, 91–103. doi: 10.1016/j.jembe.2012.03.010
Maurin C. (1968). Ecologie ichthyologique des fonds chalutables atlantiques (de la baie ibéro-marocaine à la mauritanie) et de la méditerranée occidentale. Rev. Trav. Inst. Pêches Marit. 32, 95–124.
Melo-Merino S. M., Reyes-Bonilla H., Lira-Noriega A. (2020). Ecological niche models and species distribution models in marine environments: A literature review and spatial analysis of evidence. Ecol. Modell. 415, 108837. doi: 10.1016/j.ecolmodel.2019.108837
Micarelli P., Reinero F. R. (2021). Preliminary data about nursery survey of Nursehound,Scyliorhinus stellaris (Linnaeus, 1758), around the peninsula of Monte Argentario (Tuscany, Italy) using a newtool: Poseidon R.O.V. (Remote Operative Vehicle). Academia Lett. Article 2013. doi: 10.20935/AL2013
Moranta J., Quetglas A., Massutí E., Guijarro B., Hidalgo M., Diaz P. (2008). Spatio-temporal variations in deep-sea demersal communities of the Balearic islands (western Mediterranean). J. Mar. Syst. 71, 346–366. doi: 10.1016/j.jmarsys.2007.02.029
Navarro J., Cardador L., Fernández Á.M., Bellido J. M., Coll M. (2016). Differences in the relative roles of environment, prey availability and human activity in the spatial distribution of two marine mesopredators living in highly exploited ecosystems. J. Biogeogr. 43, 440–450. doi: 10.1111/jbi.12648
Oksanen J., Simpson G., Blanchet F., Kindt R., Legendre P., Minchin P., et al (2022). Vegan: Community ecology package (R package version 3.0.3). Available at: https://CRAN.R-project.org/package=vegan.
Olaso I., Sánchez F., Rodríguez-Cabello C., Velasco F. (2002). The feeding behaviour of some demersal fish species in response to artificial discarding. Sci. Mar. 66, 301–311. doi: 10.3989/scimar.2002.66n3301
Ordines F., Massutí E., Moranta J., Quetglas A., Guijarro B., Fliti K. (2011). Balearic Islands vs Algeria: Two nearby western Mediterranean elasmobranch assemblages with different oceanographic scenarios and fishing histories. Sci. Mar. 75, 707–717. doi: 10.3989/scimar.2011.75n4707
Osgood G. J., White E. R., Baum J. K. (2021). Effects of climate-change-driven gradual and acute temperature changes on shark and ray species. J. Anim. Ecol. 90, 2547–2559. doi: 10.1111/1365-2656.13560
Ovaskainen O., Tikhonov G., Dunson D., Grøtan V., Engen S., Sæther B., et al. (2017). How are species interactions structured in species-rich communities? a new method for analysing time-series data. Proc. R. Soc B 284, 20170768. doi: 10.1098/rspb.2017.0768
Paradis S., Puig P., Masqué P., Juan-Díaz X., Martín J., Palanques A. (2017). Bottom-trawling along submarine canyons impacts deep sedimentary regimes. Sci. Rep. 7, 1–12. doi: 10.1038/srep43332
Pardo S. A., Dulvy N. K. (2022). Body mass, temperature, and depth shape the maximum intrinsic rate of population increase in sharks and rays. Ecol. Evol. 12, e9441. doi: 10.1002/ece3.9441
Pennino M. G., Muñoz F., Conesa D., López-Quίlez A., Bellido J. M. (2013). Modeling sensitive elasmobranch habitats. J. Sea Res. 83, 209–218. doi: 10.1016/j.seares.2013.03.005
Peristeraki P., Tserpes G., Kavadas S., Kallianiotis A., Stergiou K. I. (2020). The effect of bottom trawl fishery on biomass variations of demersal chondrichthyes in the eastern Mediterranean. Fish. Res. 221, 105367. doi: 10.1016/j.fishres.2019.105367
Porcu C., Marongiu M. F., Olita A., Bellodi A., Cannas R., Carbonara P., et al. (2020). The demersal bathyal fish assemblage of the central-Western Mediterranean: Depth distribution, sexual maturation and reproduction. Deep Sea res. part I oceanogr. Res. Pap. 166, 103394. doi: 10.1016/j.dsr.2020.103394
Pusceddu A., Bianchelli S., Martín J., Puig P., Palanques A., Masqué P., et al. (2014). Chronic and intensive bottom trawling impairs deep-sea biodiversity and ecosystem functioning. PNAS 111, 8861–8866. doi: 10.1073/pnas.1405454111
Ramírez-Amaro S., Ordines F., Esteban A., García C., Guijarro B., Salmerón F., et al. (2020). The diversity of recent trends for chondrichthyans in the Mediterranean reflects fishing exploitation and a potential evolutionary pressure towards early maturation. Sci. Rep. 10, 1–18. doi: 10.1038/s41598-019-56818-9
Ramírez-Amaro S., Ordines F., Terrasa B., Esteban A., García C., Guijarro B., et al. (2015). Demersal chondrichthyans in the western Mediterranean: Assemblages and biological parameters of their main species. Mar. Freshw. Res. 67, 636–652. doi: 10.1071/MF15093
Revill A. S., Dulvy N. K., Holst R. (2005). The survival of discarded lesser-spotted dogfish (Scyliorhinus canicula) in the Western English channel beam trawl fishery. Fish. Res. 71, 121–124. doi: 10.1016/j.fishres.2004.07.006
Rex M. A., Etter R. J., Morris J. S., Crouse J., McClain C. R., Johnson N. A., et al. (2006). Global bathymetric patterns of standing stock and body size in the deep-sea benthos. Mar. Ecol. Prog. Ser. 317, 1–8. doi: 10.3354/meps317001
Savage V. M., Gillooly J. F., Brown J. H., West G. B., Charnov E. L. (2004). Effects of body size and temperature on population growth. Am. Nat. 163, 429–441. doi: 10.1086/381872
Seibel B. A., Drazen J. C. (2007). The rate of metabolism in marine animals: environmental constraints, ecological demands and energetic opportunities. Phil. Trans. R. Soc B 362, 2061–2078. doi: 10.1098/rstb.2007.2101
Serena F., Abella A. J., Bargnesi F., Barone M., Colloca F., Ferretti F., et al. (2020). Species diversity, taxonomy and distribution of Chondrichthyes in the Mediterranean and black Sea. Eur. Zool. J. 87, 497–536. doi: 10.1080/24750263.2020.1805518
Sheaves M. (2009). Consequences of ecological connectivity: The coastal ecosystem mosaic. Mar. Ecol. Prog. Ser. 391, 107–115. doi: 10.3354/meps08121
Shephard S., Gerritsen H., Kaiser M. J., Reid D. G. (2012). Spatial heterogeneity in fishing creates de facto refugia for endangered celtic Sea elasmobranchs. PloS One 7, e49307. doi: 10.1371/journal.pone.0049307
Stefanescu C., Lloris D., Rucabado J. (1993). Deep-sea fish assemblages in the Catalan Sea (western Mediterranean) below a depth of 1000 m. Deep-Sea Res. Part I 40, 695–707. doi: 10.1016/0967-0637(93)90066-C
Stevens J. D., Bonfil R., Dulvy N. K., Walker P. A. (2000). The effects of fishing on sharks, rays, and chimaeras (chondrichthyans), and the implications for marine ecosystems. ICES J. Mar. Sci. 57, 476–494. doi: 10.1006/jmsc.2000.0724
Sunday J. M., Bates A. E., Dulvy N. K. (2012). Thermal tolerance and the global redistribution of animals. Nat. Clim. Change 2, 686–690. doi: 10.1038/nclimate1539
Treude T., Kiel S., Linke P., Peckmann J., Goedert J. L. (2011). Elasmobranch egg capsules associated with modern and ancient cold seeps: A nursery for marine deep-water predators. Mar. Ecol. Prog. Ser. 437, 175–181. doi: 10.3354/meps09305
Tserpes G., Maravelias C. D., Pantazi M., Peristeraki P. (2013). Distribution of relatively rare demersal elasmobranchs in the eastern Mediterranean. Estuar. Coast. Shelf Sci. 117, 48–53. doi: 10.1016/j.ecss.2012.09.020
Vilmar M., Di Santo V. (2022). Swimming performance of sharks and rays under climate change. Rev. Fish Biol. Fish. 32, 765–781. doi: 10.1007/s11160-022-09706-x
Walls R. H., Dulvy N. K. (2020). Eliminating the dark matter of data deficiency by predicting the conservation status of northeast Atlantic and Mediterranean Sea sharks and rays. Biol. Conserv. 246, 108459. doi: 10.1016/j.biocon.2020.108459
Walls R. H. L., Dulvy N. K. (2021). Tracking the rising extinction risk of sharks and rays in the northeast Atlantic ocean and Mediterranean Sea. Sci. Rep. 11, 15397. doi: 10.1038/s41598-021-94632-4
Zurell D., Jeltsch F., Dormann C. F., Schröder B. (2009). Static species distribution models in dynamically changing systems: How good can predictions really be? Ecography 32, 733–744. doi: 10.1111/j.1600-0587.2009.05810.x
Keywords: environmental drivers, fishing pressure, commercial bottom trawling, diversity, elasmobranchs, sharks, rays, modelling
Citation: Ruiz-García D, Raga JA, March D, Colmenero AI, Quattrocchi F, Company JB, Recasens L and Barría C (2023) Spatial distribution of the demersal chondrichthyan community from the western Mediterranean trawl bycatch. Front. Mar. Sci. 10:1145176. doi: 10.3389/fmars.2023.1145176
Received: 15 January 2023; Accepted: 17 February 2023;
Published: 07 March 2023.
Edited by:
Vitor H. Paiva, University of Coimbra, PortugalReviewed by:
Emilio Sperone, University of Calabria, ItalyPanagiota Peristeraki, Hellenic Centre for Marine Research (HCMR), Greece
Copyright © 2023 Ruiz-García, Raga, March, Colmenero, Quattrocchi, Company, Recasens and Barría. This is an open-access article distributed under the terms of the Creative Commons Attribution License (CC BY). The use, distribution or reproduction in other forums is permitted, provided the original author(s) and the copyright owner(s) are credited and that the original publication in this journal is cited, in accordance with accepted academic practice. No use, distribution or reproduction is permitted which does not comply with these terms.
*Correspondence: David Ruiz-García, david.ruiz-garcia@uv.es