- 1Postgraduate and Research Department of Zoology, Pachaiyappa’s College for Men, Chennai, India
- 2Post Graduate and Research Department of Zoology and Aquaculture, Government Arts College for Men (Autonomous), (Affiliated to University of Madras), Chennai, India
- 3Department of Zoology, Ramakrishna Mission Vivekananda College (Autonomous), Chennai, India
- 4eProov Assessment Solution Pvt., Limited, Chennai, India
Introduction
Polycyclic aromatic hydrocarbons (PAHs) are organic pollutants having two or more condensed aromatic rings and are considered the ninth most harmful substance to human health (Jia and Batterman, 2010; Ifegwu and Anyakora, 2015). Because of the large and unregulated exploitation of natural resources, PAH contamination has become a global concern. As far as the aquatic environment is concerned PAHs are found in sediments, benthic fauna, fish, sea birds, and mammals (Baali and Yahyaoui, 2019). Naphthalene is a PAH that is widely found in soil, water, air, and aquatic ecosystems, as well as in petroleum-derived fuels and consumer items (Jia and Batterman, 2010). The most prevalent use of naphthalene in consumer goods is the manufacture of mothballs (Sudakin et al., 2011). Ingesting naphthalene can cause both immediate and delayed toxicity (Kundra, et al., 2015).
The main mechanism of PAH toxicity is that they attach to the hydrophobic regions of macromolecules in the cell, causing molecular and cellular damage and disrupting normal physiology (Honda and Suzuki, 2020). Cytochrome P450 (CYP) enzymes are haeme-containing proteins found in vertebrates, animals, plants, and microbes. These enzymes catalyze the oxidative biotransformation of a wide range of lipophilic xenobiotic and endogenous substances, including steroids, fatty acids, medicines, and organic pollutants (Walker et al., 2001). In addition, CYP enzymes are also well recognized in the detoxification of halogenated and non-halogenated hydrocarbons in the food chain (Shu et al., 2008).
Individual CYP enzyme levels have a crucial role in bioaccumulation and vulnerability to environmental pollutant exposure. The primary hydrocarbon inducible form, CYP1A, has attracted the greatest attention as a potential biomarker of exposure to marine hydrocarbon pollutants (Buhler and Wang-Buhler, 1998). Glutathione S transferase (GST) is a phase II detoxification enzyme that may catalyze the reaction of reduced glutathione (GSH) with xenobiotic or indigenous harmful chemicals, allowing toxins to be expelled quickly from cells (Park et al., 2009). GST has also been used as a sensitive biomarker of exposure to a variety of pollutants, including PAHs and petrochemical compounds, in both field and laboratory research (Gopalakrishnan et al., 2011; Gan et al., 2021). Moreover, the antioxidants play a crucial role in maintaining homeostasis and when antioxidant defenses are compromised, oxidative stress products, particularly reactive oxygen species (ROS), can cause DNA damage, peroxidation of cell components, and enzymatic inactivation. Using the marine green mussel (Perna viridis) as a model species, the current work aims to assess the role of the CYP system in the metabolism of PAHs by marine animals, as well as how these modulations of biotransformation enzymes cause oxidative stress. It is also known that in response to oxidative stresses, marine organisms would be expected to have larger amounts of antioxidant enzymes such as catalase (CAT), glutathione peroxidase (GPx), and superoxide dismutase (SOD). Variations in antioxidant levels can thus be utilized to identify xenobiotic exposure (Gopalakrishnan et al., 2011).
P. viridis is a green-lipped marine mussel that may be found in coastal waters on both the east and west coasts of India. It absorbs pollutants easily and exhibits a variety of physiological and biochemical responses, providing information on the overall state of contamination as well as animal health. P. viridis has been utilized as an environmental bio-indicator in toxicity-related experiments using a range of biomarkers. The majority of bivalves like P. viridis toxicity research has been focused on the animal’s metal bio-accumulation mechanism and the organs involved (Jha et al., 2019; Satheeswaran et al., 2019). There has been very little research on the impact of naphthalene on the physiological processes of bivalves like P. viridis (Bagchi et al., 1998). This is the first research to look at how biotransformation enzymes and DNA damage in P. viridis are affected by chronic long-term exposure to sublethal concentrations of naphthalene. The goal of the study was to see if altering these biotransformation enzymes causes oxidative stress in P. viridis and the resulting antioxidant response.
Methods
Green mussels, P. viridis measuring with range of 7.15- 8.95 cm in length and 15.4-22.1 g in weight were collected and transported to the lab from a coastal location near the sea mouth of the Pulicat Lake, Tamil Nadu, India. The acclimatization and toxicity study procedures were adopted from the protocol of EPA/ROC, 1998 and Gopi et al. (2021). Sixty mussels were used in the present study and the mussels were divided into five groups of six specimens in each tank. Duplicate tank for each concentration was maintained. To ensure the solubility of naphthalene in seawater, acetone was used as a solvent. The mussels in Group I was reared in normal seawater (control group). Group II mussels were reared in a solvent control (Vacetone/Vseawater = 1/20 000), group III mussels were exposed to 1 mg L-1 naphthalene in seawater, group IV mussels were exposed to 2 mg L-1 naphthalene in seawater and group V were exposed to seawater with 4 mg L-1 naphthalene. Mussels were fed with fresh phytoplankton (Chlorella autotrophica) cultured during the experimental periods. The water temperature, salinity, and pH were all maintained at 28 ± 1°C, 33 ± 1 PSU, and 8.1 ± 0.1 respectively, and the tank received a continuous O2 supply. After 28 days of exposure, the animals were analyzed by removing their gills and digestive glands and stored at -20°C for subsequent enzyme and biochemical analysis. Protein concentrations in all tissues were determined using the Bradford (1976) technique.
To study the modulation of biotransformation enzymes due to the sublethal effect of naphthalene, activities of cytochrome c reductase, oxidase and glutathione S transferase and oxidative stress related parameters lipid peroxidation (LPO), DNA damage, reactive oxygen species (ROS) measurement and also the antioxidant parameter including catalase (CAT), super oxide dismutase (SOD), reduced glutathione (GSH) content and glutathione peroxidise (GPX) were analyzed. As a sensitive and practical early warning system for biological exposure to organic contaminants, these parameters have been widely employed.
The activities of cytochrome c reductase were determined spectrophotometrically using Williams and Kamin’s (1962) modified technique. Berry and Trumpower (1987) spectrophotometric approach was used to assess the activity of cytochrome c oxidase. During variations in its oxidation state, the absorbance of cytochrome c oxidase at 550 nm was measured. Shugart’s (1988) alkaline unwinding technique was used to assess DNA strand breakage using an alkaline unwinding assay, in which strand separation is obtained under controlled conditions and the quantities of double and single-stranded DNA after alkaline unwinding were measured using fluorescence at 360 nm excitation and 450 nm emission wavelengths (Ref). Glutathione S-transferase (GST) activity was determined using the 1-chloro-2, 4-dinitrobenzene (CDNB) substrate after conjugating the acceptor substrate with glutathione, as reported by Habig et al. (1974).
LPO was calculated using the protocol of Devasagayam and Tarachand (1987), the color developed was detected at 532 nm, and Malondialdehyde (MDA) level was represented as nmol of MDA generated/mg protein. ROS was assessed using the methods of Driver et al. (2000). CAT activity was measured according to Sinha (1972). SOD activity was determined by measuring the degree of inhibition of pyrogallol auto-oxidation at an alkaline pH using the Marklund and Marklund technique (1974). GPx was determined by measuring the quantity of GSH consumed in the reaction mixture by following the protocol of Rotruck et al. (1973). GSH was calculated by measuring the optical density of the yellow color generated when 5,5’-dithio-bis-(2-nitrobenzoic acid) (DTNB) is reduced by glutathione at 412 nm, as described by Moron et al. (1979).
SPSS software (version 20) was used to perform the statistical analysis. The data were analyzed for significance and reported as the mean ± standard deviation (SD). One-way analysis of variance (ANOVA) was used to analyze the data, followed by Tukey’s multiple-comparison post hoc test. Principal component analysis (PCA) is one of the most effective approaches for decreasing the dimensionality of large datasets while preserving their content (Wunderlin et al., 2001). Strong (> 0.75), moderate (0.75–0.50), and weak (0.50–0.30) factor loadings were classified according to Liu et al. (2003) criteria. To establish sample adequacy, the Kaiser–Meyer–Olkin (KMO) criterion was used. To reduce the impact of discrepancies in measurement units and variance, all parameters were normalized using a Z-scale transformation (mean = 0; variance = 1), which rendered the data dimensionless for PCA. Further, the Pearson correlation coefficient was used to determine the inter-relationship between the parameters. There was no significant difference between the solvent and blank controls, and therefore only the results of the solvent control are shown in the figures.
Data description
The digestive gland of P. viridis showed the maximum activity of cytochrome c reductase at the highest concentration of naphthalene examined, while the mussels subjected to 1mg/L showed the lowest activity, with no significant difference from the control group after 28 d of exposure (Figure 1A). Among the two organs studied, digestive gland exhibited comparatively higher cytochrome c reductase activity than gill samples. However, both the organs showed up to 4-fold increase in cytochrome c reductase activity when compared to the respective control group after 28 d of exposure to 4mg/L of naphthalene, and such increase was statistically significant (p < 0.001). Gills exhibited a dose-dependent increase in cytochrome c reductase activity when the mussels were exposed to different concentrations of naphthalene (Figure 1A). Such increase in this marker enzyme in both the organs could be due animal detoxification mechanism and needs to be elucidated further. Harlocker et al. (1991) opined that gill tissue might not be primarily important for carbon flow control but rather for detoxification reactions as evidenced by alterations to these enzymes in the gill. However, because the enzyme activity trend was strongly associated with other tissues like the digestive gland, this study highlights the fact that mussel gill tissue enzyme might be a biomarker for naphthalene exposure. Furthermore, changes in the activity of the cytochrome c reductase enzyme under naphthalene stress possibly indicate physiological changes in the organisms. These results suggest the possible use of cytochrome c reductase as a biomarker for studying the impact of organic pollutants in mussels.
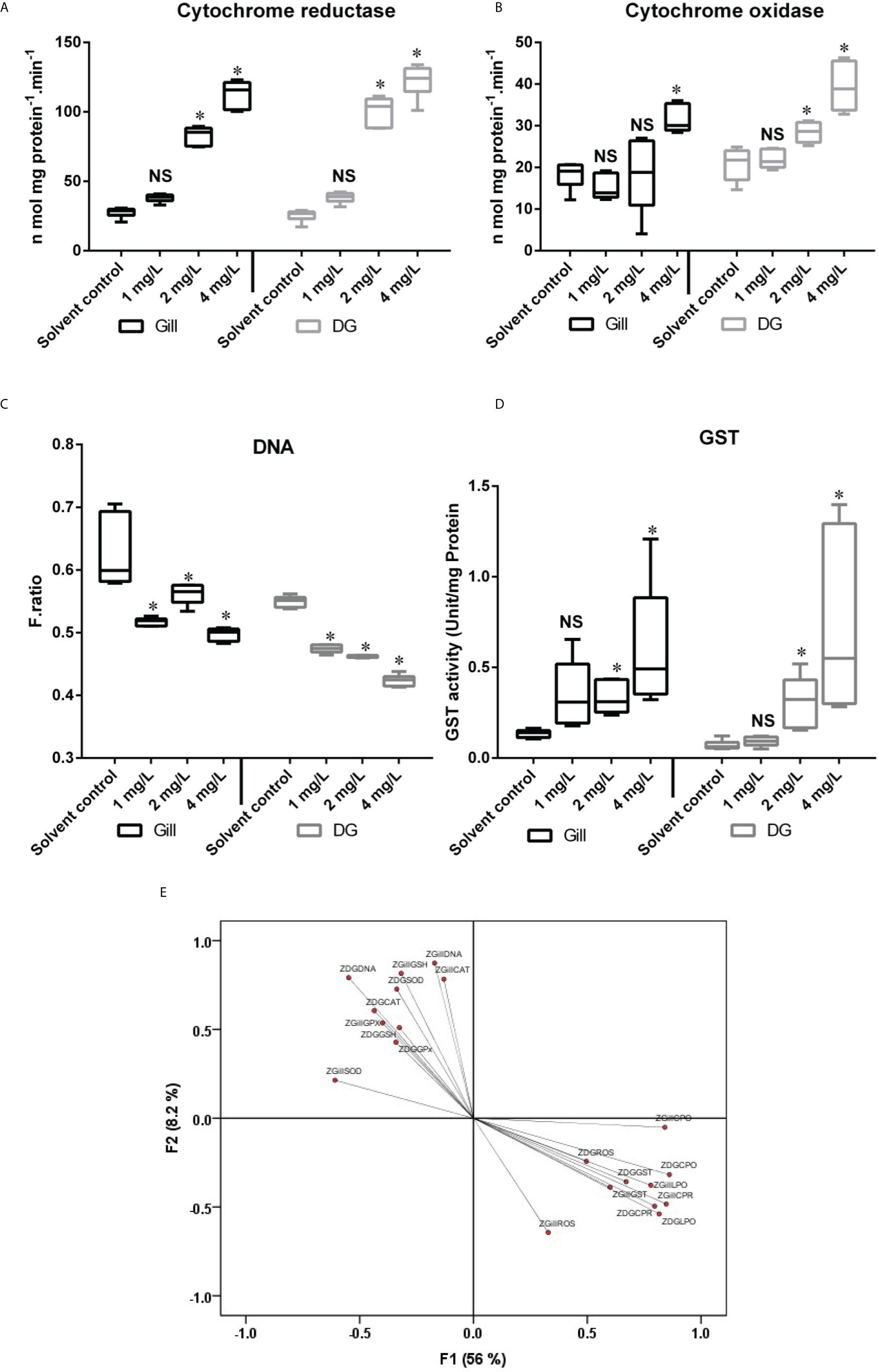
Figure 1 Showing the level of (A) Cytochrome c reductase, (B) Cytochrome c oxidase, (C) DNA damage, (D) GST activity and (E) Factor loading F1 and F2 for different biomarkers analyzed in mussel exposed to different concentration of naphthalene. The line in each box represents median and whiskers represents the upper and lower 95% confidence intervals of the mean of six determinations using samples from different preparations. One-way analysis of variance (ANOVA) followed by Tukey’s post hoc test was used. The significant difference between control and exposure groups were indicated with asterisks (P < 0.05); NS, No significant difference with respective control group. The Z-represent that the data is normalized. DG, Digestive gland.
The activity of cytochrome c oxidase was shown to be increased in mussels exposed to various concentrations of naphthalene over 28 d. The highest level of cytochrome c oxidase enzyme activity in both organs was recorded when the mussels were exposed to the highest concentration of naphthalene (4 mg/L), and the digestive gland showed the highest amount of induction of this biotransformation enzyme. When mussels were exposed to naphthalene, the activity of cytochrome c oxidase in their digestive glands increased in a dose-dependent manner (Figure 1B). Gills on the other hand, showed a significant (p < 0.001) increase in cytochrome c oxidase only when the mussels were exposed to the highest naphthalene (4 mg/L) concentration.
Furthermore, the current study demonstrated that the cytochrome c oxidase activity in the mussel digestive gland was greater than that in the gills. Fontanesi et al. (2006) found that cytochrome c oxidase biogenesis and activity were controlled by changing environmental or physiological factors in the metabolism of adaptive cells. Increased cytochrome c oxidase activity may indicate increased mitochondrial high energy bond Adenosine triphosphate (ATP) production, which is required for highly synthetic processes including DNA synthesis, mitosis, and cellular proliferation (Zorov et al.,2014). When the mitotic index and proliferation (epithelialization) grow dramatically in regenerated tissues, cytochrome c oxidase activity spikes (Saprunova et al., 2008). This represents changes in mitochondria and other sub-cellular components that occur during tissue breakdown (Li et al., 2006). Similar induction of cytochrome c oxidase due to PAH was reported in mussels (Xavier Michel et al., 1994).
DNA damage in the gill and digestive gland was observed when mussels were exposed to naphthalene (Figure 1C). After 28 d of exposure of mussels to 4 mg/L naphthalene, there was a significant reduction (p < 0.001) in the DNA integrity in the gill. A similar trend was observed for DNA integrity in the digestive gland of P. viridis when exposed to naphthalene (Figure 1C). However, the digestive gland had a greater loss in terms of DNA integrity than gill after 28 d exposure and all these changes were statistically significant (p < 0.001). The changes observed in DNA integrity were attributed by Brooks et al. (2009) to variations in the concentration and/or activity of xenobiotic metabolizing enzymes such as cytochrome P450, which are known to alter substantially with seasons and environmental conditions. Ching et al. (2001) reported that mussels exposed to benzo (a) pyrene showed a similar increase in DNA damage.
The GST activity was significantly induced in the digestive gland of mussels after 28 d of exposure to all the naphthalene concentrations. However, such induction was not significant when the mussels were exposed to the lowest naphthalene concentration. A similar increase was also observed in the gill after 28 d of exposure to naphthalene (Figure 1D). Phase II of the metabolic process includes GST activity in mussels in response to an organic contaminant as has been previously reported (Fitzpatrick et al., 1997). According to our findings, GST activity was ineffective in preventing DNA damage after 28 d of naphthalene exposure. This could be due to electrophilic intermediates formed by phase I mixed function oxidase being able to bind to nucleophilic sites of DNA during naphthalene metabolism.
Supplementary Figure 1 depicts changes in antioxidant enzymes, ROS, and LPO levels in the digestive gland and gill of mussels subjected to various naphthalene concentrations. SOD activity in both organs dropped considerably after the mussels were exposed to greater (4 mg/L) naphthalene concentrations for 28 d. Similarly, when mussels were exposed to naphthalene concentrations for 28 d, their CAT activity fell significantly, however it was lower in the gills than in the digestive gland. When mussels were exposed to naphthalene, GSH levels in their gills and digestive glands dropped drastically. Similarly, GPx activity also decreased in mussels exposed, and this reduction was statistically significant (p<0.05) when the mussels were exposed to a greater naphthalene concentration (4 mg/L).
Antioxidant enzyme activities in marine invertebrates are affected by xenobiotic exposure (Cravo et al., 2012; Gopalakrishnan et al., 2011). When toxicant concentrations are low, the modulation of these enzymes is observed and returns to normal, but when the time or dose of toxicant exposure is increased, the modulation of these enzymes remains permanent, and they respond by decreasing their level or becoming blocked in the majority of cases. According to our results, antioxidant enzyme activity in the digestive gland and gills decreased at all dosages, implying an increase in the oxygen-free radical formation and oxidative stress upon naphthalene toxicity (O2 and H2O2). The Pearson correlation matrix calculated for the digestive gland and gill tissues is given in Table 1. The correlation between individual parameters produced similar results to those of PCA and showed a significant (P<0.01; P<0.05) association between the parameters in both the digestive gland and gill studied. There were good correlations between LPO and all other parameters in P. viridis. The correlation coefficients in most cases were greater than 0.50. ROS generated in the digestive gland showed a significant relationship with DNA damage and cytochrome c reductase in the mussels exposed to naphthalene. Similarly, cytochrome c reductase and cytochrome c oxidase showed a correlation with both oxidative stress and corresponding antioxidant response. Cytochrome c reductase in gill showed a significant relation with DNA damage, oxidative stress, and antioxidant responses (Table 1).
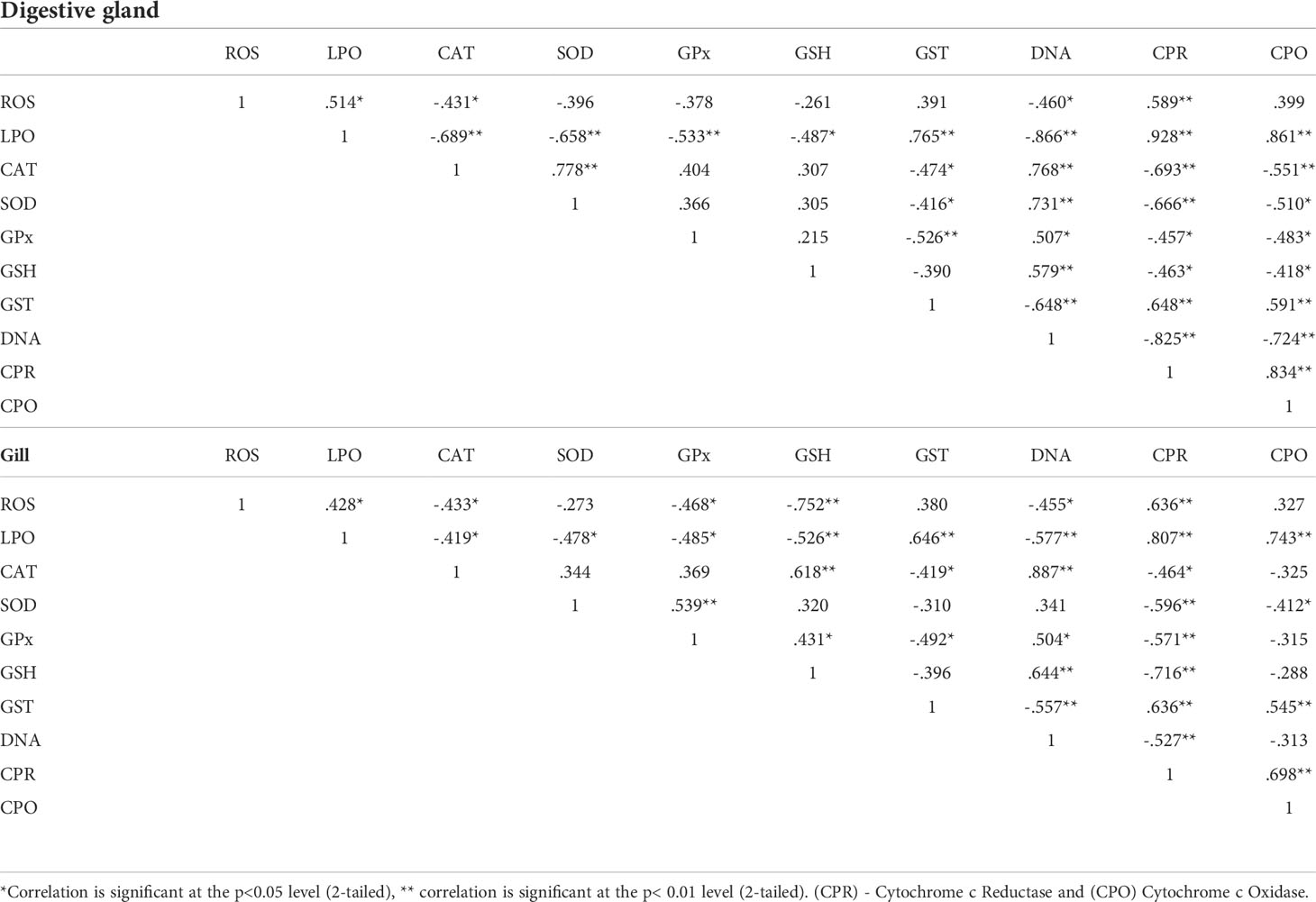
Table 1 Correlation Matrix for measured parameters in digestive gland and gill of Perna viridis exposed to different concentration of Naphthalene.
The KMO criterion values of 0.638 revealed that by factor analysis (FA), a considerable diminution in the dimensionality of the data set has been obtained (Wu et al., 2010). Through FA four significant factors (Eigen value >1) were obtained which elucidating 76.67% of the total variation among the biomarker data in the gill and digestive gland. The factor loadings and communality of the dataset are given in Table S1 (supplemental material). Factor 1 elucidates 56% of the total variance and reveals strong positive loading of DG-LPO (0.815), Gill-LPO (0.779), DG-CPR (0.796), Gill-CPR (0.847), DG-CPO (0.86) and Gill-CPO (0.841) (Figure 1E). It could be attributed to the influence of naphthalene concentrations and the duration of exposure played a major role in modulating the biotransformation enzymes. Factor 2 elucidates 8.2% of the total variance and revealed a strong positive loading of Gill-CAT (0.783), Gill-GSH (0.816), DG-DNA (0.791), and Gill-DNA (0.873). It might be explained by the various metabolites formed from the organic pollutant during biotransformation could impair the physiological system in the mussels. Further based on this finding, we hypothesized that the duration of exposure influenced the antioxidant response and increased oxidative stress, potentially resulting in DNA damage.
In conclusion, cytochrome c reductase and cytochrome c oxidase activities may provide insight into the general health of an organism exposed to PAHs, more specifically to naphthalene as per our study. As indicated by an increase in DNA damage and other oxidative stress indicators, we may deduce that exposing P. viridis to naphthalene elevates reactive oxygen species and oxidative stress in mussels, which may have an inverse influence on antioxidant activity. Together these biomarkers could be vital tools for assessing naphthalene exposure and toxicity in marine animals and can be used in regular coastal and marine environment monitoring programs.
Data availability statement
The original contributions presented in the study are included in the article/Supplementary Material. Further inquiries can be directed to the corresponding authors.
Ethics statement
For the care and use of animals, all applicable international, national, and/or institutional guidelines were followed, since we use the invertebrate and commercial animal ethical approval not necessary.
Author contributions
SR, RT, GJ, PS and RS sample collection, performed experiment and laboratory analysis. KR: conceptualization, experimental design, data validation. TR: manuscript corrections and critical suggestions. GS: statistical analysis of data and manuscript correction. TH and KR: manuscript preparation, reviewing the manuscript and project administration. All authors contributed to the article and approved the submitted version.
Acknowledgments
The authors thank Head, Research department of Zoology and the Principal Government Arts College for men (Autonomous), Chennai-600 035 and Head, Research department of Zoology and also the Principal Pachaiyappa’s College for Men, Chennai- 600 030 India for extending their support through the experiment.
Conflict of interest
Author GS was employed by eProov Assessment Solution Pvt., Limited.
The remaining authors declare that the research was conducted in the absence of any commercial or financial relationships that could be construed as a potential conflict of interest.
Publisher’s Note
All claims expressed in this article are solely those of the authors and do not necessarily represent those of their affiliated organizations, or those of the publisher, the editors and the reviewers. Any product that may be evaluated in this article, or claim that may be made by its manufacturer, is not guaranteed or endorsed by the publisher.
Supplementary material
The Supplementary Material for this article can be found online at: https://www.frontiersin.org/articles/10.3389/fmars.2022.969589/full#supplementary-material
References
Baali A., Yahyaoui A. (2019). Polycyclic Aromatic Hydrocarbons (PAHs) and Their Influence to Some Aquatic Species, Ince M., Ince O. K., Ondrasek G. (Eds.) IntechOpen (Biochemical Toxicology - Heavy Metals and Nanomaterials). doi: 10.5772/intechopen.86213
Bagchi M., Bagchi D., Balmoori J., Ye X., Stohs S. J. (1998). Naphthalene-induced oxidative stress and DNA damage in cultured macrophage J774A.1 cells. Free Radic. Biol. Med. 25 (2), 137–143. doi: 10.1016/s0891-5849(98)00063-x
Berry E. A., Trumpower B. L. (1987). Simultaneous determination of hemes a, b, and c from pyridine hemochrome spectra. Anal. Biochem. 161, 1–15. doi: 10.1016/0003-2697(87)90643-9
Bradford M. M. (1976). A rapid and sensitive method for the quantitation of microgram quantities of protein utilizing the principle of protein-dye binding. Anal. Biochem. 72, 248–254. doi: 10.1006/abio.1976.9999
Brooks S., Lyons B., Goodsir F., Bignell J., Thain J. (2009). Biomarker responses in mussels, an integrated approach to biological effects measurements. J. Toxicol. Environ. Health Part A 72 (3), 196–208. doi: 10.1080/15287390802539038
Buhler D. R., Wang-Buhler J. L. (1998). Rainbow trout cytochrome P450’s: purification, molecular aspects, metabolic activity, induction and role in environmental monitoring. Comp. Biochem. Physiol. 121C, 107–137. doi: 10.1016/S0742-8413(98)10033-6
Ching E. W., Siu W. H., Lam P. K., Xu L., Zhang Y., Richardson B. J., et al. (2001). DNA Adduct formation and DNA strand breaks in green-lipped mussels (Perna viridis) exposed to benzo[a]pyrene: dose- and time-dependent relationships. Mar. pollut. Bull. 42 (7), 603–610. doi: 10.1016/s0025-326x(00)00209-5
Cravo A., Pereira C., Gomes T., Cardoso C., Serafim A., Almeida C., et al. (2012). A multibiomarker approach in the clam ruditapes decussatus to assess the impact of pollution in the ria Formosa lagoon, south coast of Portugal. Mar. Environ. Res. 75, 23–34. doi: 10.1016/j.marenvres.2011.09.012
Devasagayam T. P., Tarachand U. (1987). Decreased lipid peroxidation in the rat kidney during gestation. Biochem. Biophys. Res. Commun. 145, 134–138. doi: 10.1016/0006-291x(87)91297-6
Driver A. S., Kodavanti P. R., Mundy W. R. (2000). Age-related changes in reactive oxygen species production in rat brain homogenates. Neurotoxicol Teratol 22, 175–181. doi: 10.1016/s0892-0362(99)00069-0
EPA/ROC (1998). “Standard guide for conducting acute tests with fishes. static renewal test for common carp,” in NIEA B904.10B, environmental protection administration of the republic of China(Taipei, Taiwan).
Fitzpatrick P. J., Halloran J. O., Sheehan D., Walsh A. R. (1997). Assessment of a glutathione s-transferase and related proteins in the gill and digestive gland of mytilus edulis (L.), as potential organic pollution biomarkers. Biomarkers 2:1, 51–56. doi: 10.1080/135475097231977
Fontanesi F., Soto I. C., Horn D., Barrientos A. (2006). Assembly of mitochondrial cytochrome coxidase, a complicated and highly regulated cellular process. Am. J. Physiol. Cell Physiol. 291, 1129–1147. doi: 10.1152/ajpcell.00233.2006
Gan N., Martin L., Xu W. (2021). Impact of polycyclic aromatic hydrocarbon accumulation on oyster health. Front. Physiol. 12. doi: 10.3389/fphys.2021.734463
Gopalakrishnan S., Nai Z., Thilagam H., Bei C., Ding J., Wang X. H., et al. (2011). Biochemical responses and DNA damage in red sea bream from coastal fujian province, China. Ecotoxicol Environ. Saf. 74 (6), 1526–1535. doi: 10.1016/j.ecoenv.2011.05.007
Gopi J., Nathiya K. R., Sangeetha R., Krishnamurthy R. (2021). Induction of oxidative stress and modulation of antioxidant defense in the haemolymph of green mussel perna viridis exposed to naphthalene. Uttar Pradesh J. Zoology 42 (23), 161–169.
Habig W. H., Pabst M. J., Jakoby W. B. (1974). Glutathione s-transferases. the first enzymatic step in mercapturic acid formation. J. Biol. Chem. 249, 7130–7139. doi: 10.1016/S0021-9258(19)42083-8
Harlocker S. L., Kapper M. A., Greenwalt D. E., Bishop S. H. (1991). Phosphoenolpyruvate carboxykinase from ribbed mussel gill tissue: reactivities with metal ions, kinetics, and action of 3-mercaptopicolinic acid. J. Exp. Zool 257, 285–298. doi: 10.1002/jez.1402570302
Honda M., Suzuki N. (2020). Toxicities of polycyclic aromatic hydrocarbons for aquatic animals. Int. J. Environ. Res. Public Health 17 (4), 1363. doi: 10.3390/ijerph17041363
Ifegwu O. C., Anyakora C. (2015). “Chapter six - polycyclic aromatic hydrocarbons: Part I. exposure,” in Advances in clinical chemistry, vol. 72 . Eds. Gregory S., Makowski (Elsevier), 277–304. doi: 10.1016/bs.acc.2015.08.001
Jha D. K., Ratnam K., Rajaguru S., Dharani G., Devi M. P., Kirubagaran R. (2019). Evaluation of trace metals in seawater, sediments, and bivalves of nellore, southeast coast of India, by using multivariate and ecological tool. Mar. pollut. Bull. 146, 1–10. doi: 10.1016/j.marpolbul.2019.05.044
Jia C., Batterman S. (2010). A critical review of naphthalene sources and exposures relevant to indoor and outdoor air. Int. J. Environ. Res. Public Health 7 (7), 2903–2939. doi: 10.3390/ijerph7072903
Kundra T. S., Bhutatani V., Gupta R., Kaur P. (2015). Naphthalene poisoning following ingestion of mothballs: A case report. J. Clin. Diagn. Res. JCDR 9 (8), UD01–UUD2. doi: 10.7860/JCDR/2015/15503.6274
Li Y., Park J. S., Deng J. H., Bai Y. (2006). Cytochrome c oxidase subunit IV is essential for assembly and respiratory function of the enzyme complex. J. Bioenerg Biomembr. 38, 283–291. doi: 10.1007/s10863-006-9052-z
Liu C. W., Lin K. H., Kuo Y. M. (2003). Application of factor analysis in the assessment of groundwater quality in a blackfoot disease area in Taiwan. Sci. Total Environ. 313, 77–89. doi: 10.1016/S0048-9697(02)00683-6
Marklund S., Marklund G. (1974). Involvement of the superoxide anion radical in the autoxidation of pyrogallol and a convenient assay for superoxide dismutase. Eur. J. Biochem. 47, 469–474. doi: 10.1111/j.1432-1033.1974.tb03714.x
Michel X., Salaün J.-P., Galgani François, Narbonne Jean-François (1994). Benzo(a)pyrene hydroxylase activity in the marine mussel mytilus galloprovincialis: a potential marker of contamination by polycyclic aromatic hydrocarbon-type compounds. Mar. Environ. Res. 38, 257–273. doi: 10.1016/0141-1136(94)90027-2
Moron M. S., Depierre J. W., Mannervik B. (1979). Levels of glutathione, glutathione reductase and glutathione s-transferase activities in rat lung and liver. Biochem. Biophys. Acta 582, 67–78. doi: 10.1016/0304-4165(79)90289-7
Park H., In-Y A., Kim H., Lee J., Chul Shin S. (2009). Glutathione s-transferase as a biomarker in the Antarctic bivalve laternula elliptica after exposure to the polychlorinated biphenyl mixture aroclor 1254. Comp. Biochem. Physiol. Part C: Toxicol. Pharmacol. 150, 4, 528–536. doi: 10.1016/j.cbpc.2009.07.008
Rotruck J. T., Pope A. L., Ganther H. E., Swanson A. B., Hafeman D. G., Hoekstra W. G. (1973). Selenium: biochemical role as a component of glutathione peroxidase. Science 179, 588–590. doi: 10.1126/science.179.4073.588
Saprunova V. B., Solodovnikova I. M., Bakeeva L. E. (2008). Cytochrome c oxidase activity in mitochondria of cardiomyocytes from isolated cardiac tissue incubated under long-term hypoxic conditions. Cell Tissue Biol. 2, 153–157. doi: 10.1134/S1990519X08020089
Satheeswaran T., Purushothaman Y., Palani D., Velmurugan K., Jha D. K., Dharani G., et al. (2019). Assessment of trace metal contamination in the marine sediment, seawater, and bivalves of parangipettai, southeast coast of India. Mar. pollut. Bull. 1492019, 110499. doi: 10.1016/j.marpolbul.2019.110499
Shugart L. R. (1988). Quantitation of chemically induced damage to DNA of aquatic organisms by alkaline unwinding assay. Aquat Toxicol. 13, 43–52. doi: 10.1016/0166-445X(88)90071-9
Shu Y. Z., Johnson B. M., Yang T. J. (2008). Role of biotransformation studies in minimizing metabolism-related liabilities in drug discovery. AAPS J. 10, 178–192. doi: 10.1208/s12248-008-9016-9
Sinha A. K. (1972). Colorimetric assay of catalase. Anal. Biochem. 47, 389–394. doi: 10.1016/0003-2697(72)90132-7
Sudakin D. L., Stone D. L., Power L. (2011). Naphthalene mothballs: Emerging and recurring issues and their relevance to environmental health. Curr. topics Toxicol. 7, 13–19.
Walker C. H., Hopkin S. P., Sibly R. M., Peakall D. B. (2001). Principles of ecotoxicology. 2nd ed (London: Taylor & Francis).
Williams C. H., Kamin H. (1962). Microsomal triphosphopyridine nucleotide-cytochrome c reductase of liver. J. Biol. Chem. 237, 587–595. doi: 10.1016/S0021-9258(18)93967-0
Wunderlin D. A., Diaz M. P., Ame M. V., Pesce S. F., Hued A. C., Bistoni M. (2001). Pattern recognition techniques for the evaluation of spatial and temporal variation in water quality. A Case study: Suquia river basin (Cordoba Argentina). Water Res. 35, 2881–2894. doi: 10.1016/S0043-1354(00)00592-3
Wu M. L., Wang Y. S., Sun C. C., Wang H., Dong J. D., Yin J. P., et al. (2010). Identification of coastal water quality by statistical analysis methods in daya bay, south China Sea. Mar. pollut. Bull. 60, 852–860. doi: 10.1016/j.marpolbul.2010.01.007
Keywords: biotransformation enzymes, DNA damage, oxidative stress, antioxidant, naphthalene, marine, Perna viridis
Citation: Ravi S, Thillainayagam R, Janakiraman G, Subramanyam P, Sivakumar R, Raman T, Singaram G, Harikrishnan T and Rajamanickam K (2022) Modulation of biotransformation enzymes leads to oxidative stress and DNA damage in naphthalene exposed marine bivalve Perna viridis. Front. Mar. Sci. 9:969589. doi: 10.3389/fmars.2022.969589
Received: 15 June 2022; Accepted: 15 July 2022;
Published: 04 August 2022.
Edited by:
Dilip Kumar Jha, National Institute of Ocean Technology, IndiaReviewed by:
Sudarshan Singh Rathore, Central Institute of Mining and Fuel Research, IndiaPankaj Verma, National Institute of Ocean Technology, India
Copyright © 2022 Ravi, Thillainayagam, Janakiraman, Subramanyam, Sivakumar, Raman, Singaram, Harikrishnan and Rajamanickam. This is an open-access article distributed under the terms of the Creative Commons Attribution License (CC BY). The use, distribution or reproduction in other forums is permitted, provided the original author(s) and the copyright owner(s) are credited and that the original publication in this journal is cited, in accordance with accepted academic practice. No use, distribution or reproduction is permitted which does not comply with these terms.
*Correspondence: Thilagam Harikrishnan, dGhpbGFnYW1wYWNoYWl5YXBwYXNAZ21haWwuY29t; Krishnamurthy Rajamanickam, cHJvZmVzc29ya3Jpc2huYW11cnRoeUBnbWFpbC5jb20=
†These authors have contributed equally to this work