- 1Key Laboratory of Mariculture and Stock Enhancement in North China’s Sea, Ministry of Agriculture and Rural Affairs, Dalian Ocean University, Dalian, China
- 2School of Marine Sciences, Ningbo University, Ningbo, China
The sea urchin Strongylocentrotus intermedius is one of the most economically important echinoids harvested from northeast Pacific Ocean coastal waters. The gonads of sea urchins have high nutritional value and are primarily a candidate source of polyunsaturated fatty acids (PUFA). PUFAs are essential for human health, and the biological synthesis and industry production of PUFA have attracted more and more attention from the scientific community. Moreover, PUFAs are important necessary nutrients that determine not only the nutritional value of sea urchins but guarantee their normal growth and reproduction. In this study, we divided 178 sea urchins into three groups (high, medium, and low concentration PUFA groups) according to the concentration of PUFAs, and conducted integrative lipidomics and transcriptomics analyses of different PUFA abundances of S. intermedius to determine the critical genes related to PUFA metabolism in sea urchin gonads. Illumina sequencing generated 894,295,712 clean reads (133.28 Gb) in 18 cDNA libraries. Among all of the unigenes, nine up- and seven down-regulated unigenes were found in a comparison of the “high vs low” concentration PUFA groups of gonads. Interestingly, we found that tudor domain-containing protein 3 (TDRD3) was up-regulated in the high concentration PUFA group, and this gene was significantly related to eicosapentaenoic acid (EPA) in the correlation analysis (p<0.05), and may be used as a candidate marker for EPA biosynthesis and metabolism in the sea urchin. The lipidome and transcriptome information will provide a basic resource for further studies designed to elucidate the molecular mechanism of PUFA metabolism in marine invertebrates, and act as a valuable resource for the practical applications and regulation of the sea urchin aquaculture industry in the future.
Introduction
Strongylocentrotus intermedius, one of the edible and valuable sea urchins, found in the coastal waters of northeastern China, Japan and Korea (Agatsuma, 2013). This species was introduced to China from Japan in the 1980s, and now it is one of the most important aquacultural echinoid species in the northeast coastal areas of the Dalian and Shandong Provinces in China (Wang et al., 2020). Sea urchin gonads, which have high nutritional value, are primarily a candidate source of polyunsaturated fatty acids (PUFA), including eicosapentaenoic fatty acid (EPA), which are essential for human health (Nettleton and Salem, 2019). Marine organism fatty acids, such as PUFA from the sea urchin, could be considered potential targets to develop for the treatment of neurodegenerative or cardiovascular diseases (Murzina et al., 2021), since many epidemiological studies have been conducted on the myriad health benefits of PUFAs in the past few decades (Shahidi and Ambigaipalan, 2018). EPA could improve cellular function through changes in gene expression and show anti-inflammatory effects (Bouwens et al., 2009), and PUFA show their importance in health promotion and disease risk reduction (Swanson et al., 2012). Therefore, the biological synthesis and accumulation of PUFA in sea urchin gonads have attracted more and more attention from the scientific community.
PUFAs are important necessary nutrients that determine not only the nutritional value of sea urchins but guarantee their normal gametogenesis of sea urchin, and PUFAs can be used as a source of energy. Moreover, PUFAs could play an important role in larval development and survival (Raposo et al., 2016). Recently, most studies on the biology and ecology of sea urchins in recent years have evaluated S. intermedius specimens collected from the field in terms of survival, growth, and reproduction (Chi et al., 2021; Yang et al., 2021). Knowledge of the biology and ecology is valuable in that it provides important information related to feeding ecology of the sea urchins, and enhances the ability to carry on nutritional research (Watts et al., 2010). However, nutritional research does not provide information that is adequate for clarifying the biosynthetic and metabolic pathways of PUFA in the sea urchin.
In our previous work, we carried out an untargeted lipidomics study of S. intermedius to highlight the lipid profiles of both sexes of sea urchin gonads, and compared the lipid profiles among samples with different PUFA abundances (Wang et al., 2021). We have detected 11 PUFAs and 1552 lipid molecular species in the gonads, and phosphatidylcholine (42: 11) may be thought as a potential marker for distinguishing high levels of PUFAs in sea urchin individuals. However, this single lipidomics study could only provide a partial clarification of the complexity of the process of lipid metabolic pathways in the sea urchin. Thus, a more intensive and comprehensive method that is capable of characterizing whole lipid changes and associated critical genes is needed to elucidate the molecular mechanisms of PUFA biosynthesis and metabolism. In preliminary work, we sequenced the transcriptomes of S. intermedius at different gonad developmental stages, looking for differentially expressed genes (DEGs) that are related to fatty acid metabolism in sea urchin gonads (Wang et al., 2019). Then, an integrated analysis of untargeted metabolomics and transcriptomics of the same samples was performed to reveal EPA-related DEGs, which belonged to extracellular exosome, intracellular signal transduction, integral component of membrane steroid hydroxylase activity and so on (Wang et al., 2020). However, we did not obtain adequate data to enable an understanding of how to acquire the sea urchin gonads that contain a high accumulation of PUFA, and how DEGs could regulate the lipid in specimens with different PUFA abundances remained unclear, especially in regard to PUFA metabolism.
Therefore, in this study, to ultimately uncover the molecular mechanisms of PUFA biosynthesis and metabolism in marine invertebrates, we first conducted an integrative lipidomics and transcriptomic analysis to reveal the critical genes related to PUFA metabolism in the sea urchin gonad. This important lipid and genetic information will provide a better understanding of the characteristics of the basal differences among S. intermedius specimens with different PUFA abundances and could form the basis for further study on practical applications in the sea urchin aquaculture industry.
Materials and Methods
Animal and Sampling Preparation
Sea urchins (S. intermedius) were collected from Lvshunkou, Dalian, China (38°49’N, 121°26’E), and cultured at the Key Laboratory of Mariculture and Stock Enhancement in the North China Sea, Ministry of Agriculture and Rural Affairs, Dalian Ocean University. The animals were kept in 1,000-L tanks containing aerated sand-filtered seawater under the same conditions (salinity 30‰, pH 8.2). The sea urchins were starved in the laboratory for 7 days. The gonads of 178 sea urchins were sampled and their fatty acid compositions were determined.
According to the results of PUFA analysis, we divided the samples into the following three groups: high concentration of PUFAs (H group, the top 30%, [n = 55], 36600-68500 mg/kg); medium concentration of PUFAs (M group, 30–70%, [n = 68], 28600-36500 mg/kg), and low concentration of PUFAs (L group, the bottom 30%, [n = 55], 15300-28500 mg/kg) (Figure 1A), and there were no significant differences in the characteristics (weight or gonad somatic index= [gonad weight/total tissue weight] × 100, GSI) of the selected samples from the different groups (p>0.05) (Figure 1B). Three male and three female sea urchins were selected from each of the three groups for omics analyses, “HF” - high concentration of PUFA in ovaries of female sea urchins; “HM” - high concentration of PUFA in testes of male sea urchins; “MF” - medium concentration of PUFA in ovaries; “MM” - medium concentration of PUFA in testes; “LF” - low concentration of PUFA in ovaries; “LM” - low concentration of PUFA in testes.
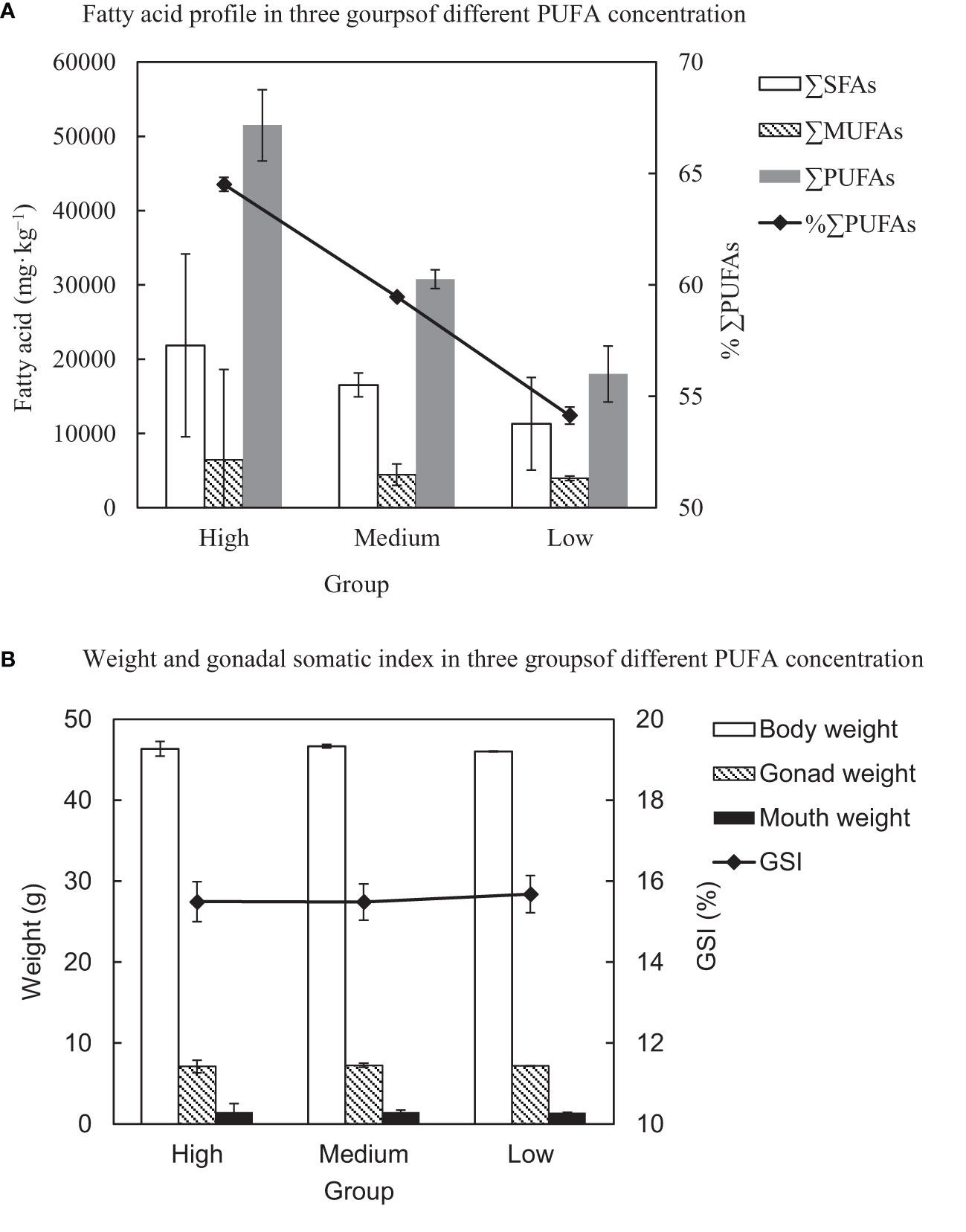
Figure 1 Characteristics of sea urchin Strongylocentrotus intermedius in three groups of different PUFA concentration. (A) Fatty acid profile; (B) weight and gonadal somatic index of sea urchin. The results are expressed as mean ± SEM (n= 3). SFAs, saturated fatty acids; MUFAs, monounsaturated fatty acids; PUFAs, polyunsaturated fatty acids.
Fatty Acid Extraction
The extraction procedure of fatty acids was performed in our previous work (Wang et al., 2021). The fat of sample was extracted into ether and methylated to fatty acid methyl esters (FAMEs), and the fatty acid composition was determined as FAMEs by gas chromatograph (GC). The sample was hydrolyzed by pyrogallic acid, 2 mL 95% ethanol and 10 mL HCl at 70–80°C for 40 min, and extracted with 10 mL 95% ethanol and 50 mL ether. FAMEs were produced by the ether layer extracts with 2 mL sodium hydroxide in methanol (2% NaOH-methanol) and 3 mL boron trifluoride reagent (14% BF3- methanol), heating at 85°C for 30 min. After filtration (0.45-μm filter), the mixture of FAMEs was quantitatively determined by capillary GC.
Lipidomic Analysis
Total lipid extracts were analyzed using an ultra-high-performance liquid chromatography (UHPLC) system (Ultimate 3000; Thermo Scientific, Bremen, Germany) with a Hypersil GOLD C18 column (100×2.1 mm, 1.9 μm; Thermo Scientific). Three biological replicates were analyzed in this study. As we previously described in detail (Wang et al., 2021), a Q-Exactive Plus mass spectrometer (MS) (Thermo Scientific) was used to detect the compounds eluted from the column. The lipid molecular species were identified using Lipid Search 4.1.30 software (Thermo Scientific™) and the principal components that accounted for the majority of the variations within the dataset were identified using SIMCA-P14.1 (Umetrics, Umea, Sweden).
Transcriptomic Analysis
Total RNA was extracted by TRIzol® Reagent (Invitrogen), and its quantity and purity were checked by Nanodrop 2000 with RNA for cDNA library preparation (Supplementary Table S1 and Supplementary Figure S1). The mRNA molecules were purified using poly-T oligo attached magnetic beads (Invitrogen), and the RNA fragments were reverse-transcribed to create the final cDNA library. Samples were sequenced using the Hiseq2000 Truseq SBS Kit v3-HS (Illumina) following the manufacturer’s recommended protocol. De novo assembly was performed with Trinity 2.4.0 software (Grabherr et al., 2011), and unigene annotation was obtained using Blast2GO program (Conesa et al., 2005). The unigenes were annotated by Gene Ontology (GO) (http://www.geneontology.org), Kyoto Encyclopedia of Genes and Genomes (KEGG) pathways (http://www.genome.jp/kegg/), non-redundant (NR) protein database (http://www.ncbi.nlm.nih.gov/), Protein family (PFAM) (http://pfam.sanger.ac.uk/), Search Tool for the Retrieval of Interacting Genes (STRING) (https://string-db.org/) and SWISSPORT (http://www.expasy.ch/sprot/). DEGs were identified by setting criteria of |log2FC|> 1 and FDR < 0.05.
All of the obtained data were submitted to the Short Read Archive (http://www.ncbi.nlm.nih.gov/sra/) at the National Center for Biotechnology Information (NCBI), in Bioproject PRJNA759708 under the accession number SUB8710006.
Lipidomic and Transcriptomic Analysis
For UPLC-MS/MS (lipidomics) and RNA-seq (transcriptomic) analyses, data were converted into vectors to calculate the Spearman’s rank correlation coefficient between the significantly different lipid metabolites and differentially expressed genes (DEGs) profiles among the three groups.
RNA-Seq Differential Gene Expression Analysis
We selected 11 DEGs for real-time quantitative reverse transcription PCR (qRT-PCR) analysis, using the same RNA samples as for the transcriptome profiling. The cDNA was synthesized using Reverse Transcription System (Promega). The analysis was conducted with a LightCycler®96 instrument (Roche Life Science, Basel, Switzerland). The 20-μL qPCR reaction mixture consisted of 10.0 μL FastStart Essential DNA Green Master (Roche), 2.0 μL of template cDNA, and 1 μL (10 μM) each of the forward and reverse primers. The reaction conditions were as follows: 95°C for 30 s, 40 cycles of 95°C for 5 s and 60°C for 60 s, 95°C for 10 s, 60°C for 60 s, 97°C for 1 s and 60°C for 15 s. Three biological replicates were carried out for each group. After normalization to the 18S rRNA gene (Vergara-Amado et al., 2017; Di Natale et al., 2019; Masullo et al., 2021), the relative expressions of 11 selected genes were calculated with the 2−ΔΔCT method (Livak and Schmittgen, 2001). Primers were designed using Primer 5.0 software, and are listed in Supplementary Table S2.
Data Analysis
The evolutionary history was inferred using the UPGMA method, which employed a sequential clustering algorithm, and local topological relationships were identified in order of similarity (Sneath and Sokal, 1973). The tree was drawn to scale, with branch lengths in the same units as those of the evolutionary distances used to infer the phylogenetic tree. The evolutionary distances were computed using the Poisson correction method (Zuckerkandl and Pauling, 1965) and were in units of the number of amino acid substitutions per site. Evolutionary analyses were conducted in Molecular Evolutionary Genetics Analysis (MEGA) X in this work according to the protocol.
Statistical analysis was performed using SPSS software version 19.0 (IBM, Armonk, NY, USA). The data are expressed as the mean ± standard error of the mean (SEM) (n=3). In the results of gene expressions, significant differences (p<0.05) for each variable were first detected using the one-way ANOVA test between different groups, followed by Tukey’s HSD test.
Results
Global Transcriptomic Analysis of S. intermedius Gonads
The transcriptomic analysis of S. intermedius gonads from the three PUFA groups was undertaken. The 18 cDNA libraries (including HF1-3; HM1-3; MF1-3; MM1-3; LF1-3; LM1-3) were sequenced and assembled together to maximize the diversity of transcripts. All of the sequencing data and quality assessment are shown in Table 1. Illumina sequencing generated 894,295,712 clean reads with 133.28 Gb in 18 cDNA libraries. The average length of a unigene was 542.32 bp, and they ranged in size from 201 to 48,802 bp, with N50 and N90 lengths of 622 and 256 bp, respectively (Supplementary Figure 2A and Supplementary Table S3). All of the unigenes of the S. intermedius transcriptome were annotated via six methods, and 23,503, 16,246, 47,042, 15,551, 11,552, and 24,032 unigenes had significant matches in the GO, KEGG, NR, PFAM, STRING, and SWISSPORT databases, respectively (Supplementary Table S4). In total, 107,765 unique sequences were identified through BlastX searches, and the top-hit species distribution of BLAST matches is shown in Supplementary Figure 2B. Approximately 75% of the sequences (30,269 unigenes) had significant matches with the closely related sea urchin, Strongylocentrotus purpuratus (Supplementary Figure 2B). GO assignment was carried out on the unigenes, and GO level 2 analysis was performed their associated biological processes (BP), cellular components (CC), and molecular functions (MF). A more detailed taxonomy of three GO terms was performed by level 2, 3 and 4, and the function of the unigenes was annotated more clearly. At GO level4, “macromolecule metabolic process” (9600), “intracellular” (16920), and “cation binding” (5710) were the most dominant elements among the functional terms of respectively (Figure 2A). Moreover, all of the unigenes were mapped to the reference pathways in the KEGG database, and 559 unigenes were assigned to the “lipid metabolism” pathway (Figure 2B).
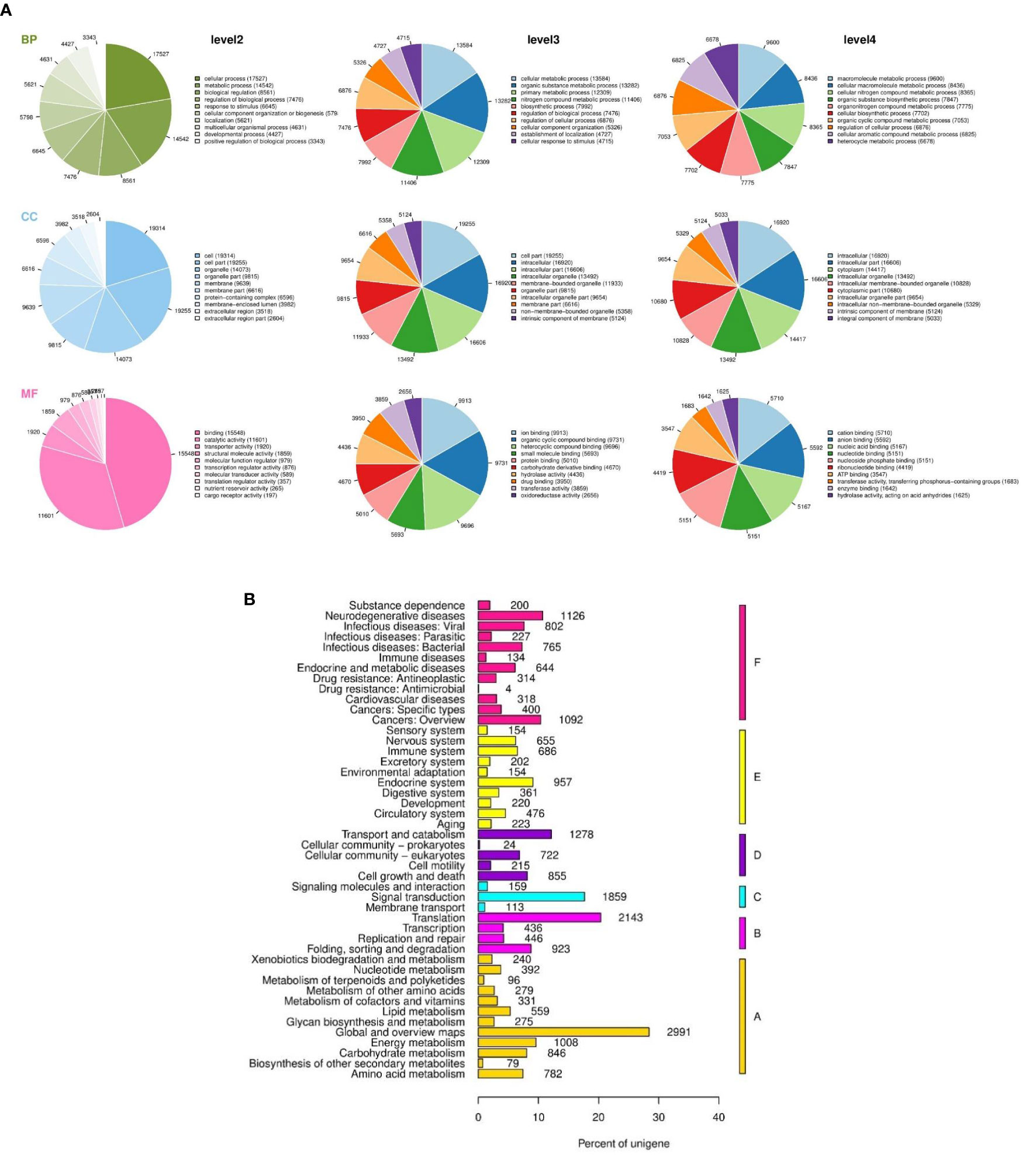
Figure 2 Global transcriptomic analysis of Strongylocentrotus intermedius gonads. (A) GO annotation in level 2, 3 and 4, BP, biological process; CC, cellular component; MF, molecular function. (B) Unigenes homology to KEGG, A: metabolism: B: genetic information processing; C: environmental information processing; D: cellular processes; E: organismal systems; F: human diseases.
Analysis of Differentially Expressed Genes
The number of pairwise comparisons of significantly differentially expressed genes among the different sexes and different PUFA abundance groups are shown in Table 2. Of all of the unigenes, nine up- and seven down-regulated unigenes were found in the comparison of the H vs L groups (Figure 3A), four up- and two down-regulated unigenes in the comparison the H vs M groups (Figure 3B), and four up- and seven down-regulated unigenes in the comparison of the M vs L groups (Figure 3C). Moreover, in the female sea urchins, there were eight, three, and nine DEGs in the comparison of the HF vs LF (Figure 3D), HF vs MF (Figure 3F), and LF vs MF groups (Figure 3G), respectively. In the male sea urchins, there were 21 and 22 DEGs in the comparison of the HM vs LM (Figure 3E) and LM vs MM groups (Figure 3H), with no DEGs in the comparison of the HM vs MM groups. The detailed sequences and names of DEGs are listed in the heat maps from the pairwise comparisons (Table 3 and Supplementary Tables S5–S10).
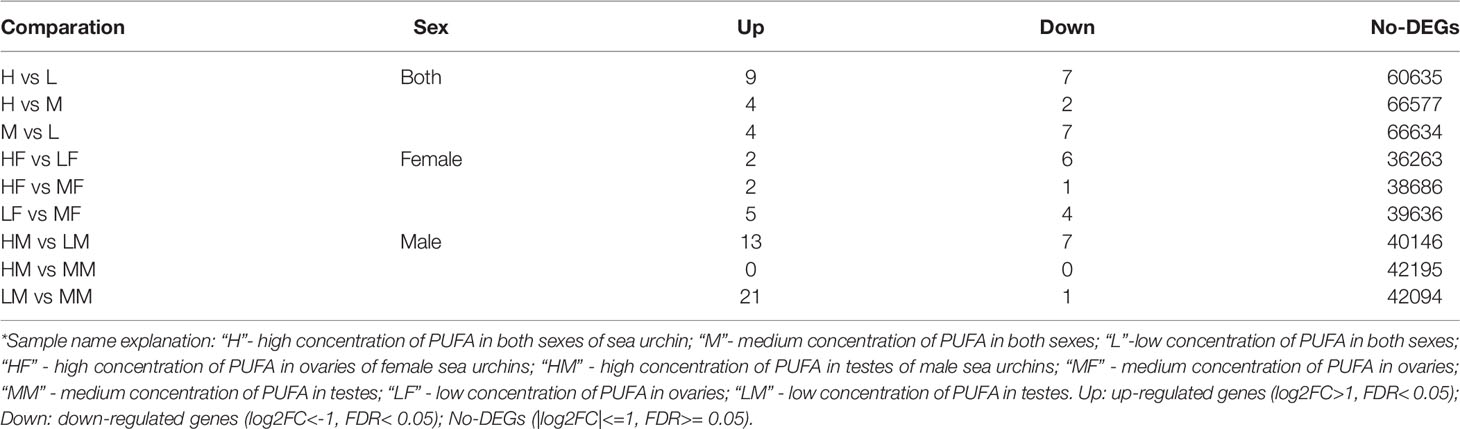
Table 2 Pairwise comparisons of differentially expressed genes in the different sexes and different PUFA abundance groups of Strongylocentrotus intermedius.
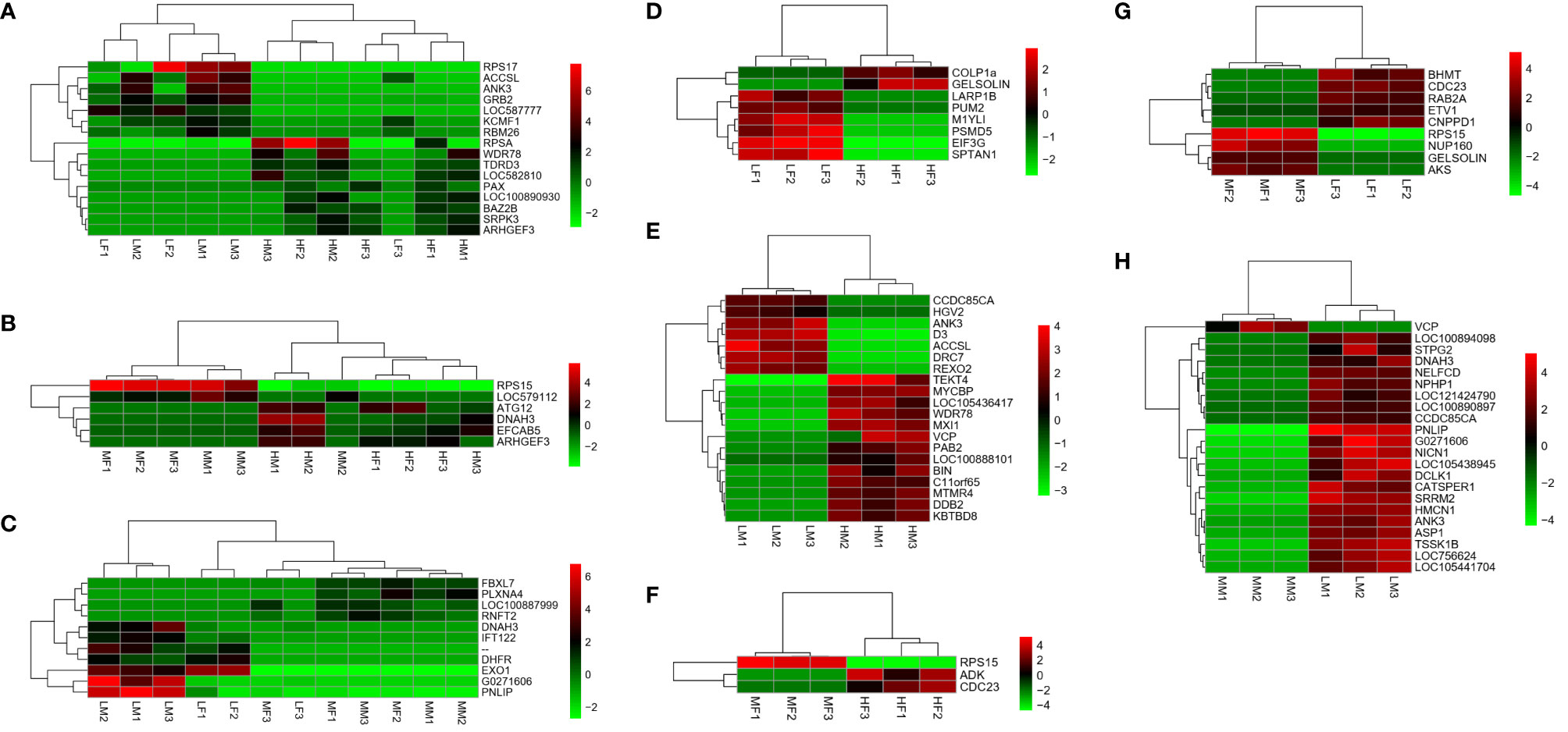
Figure 3 Heatmaps based on differentially expressed genes from pairwise comparisons. Different colors indicate relative expression levels. (A) H vs L; (B) H vs M; (C) M vs L; (D) HF vs LF; (E) HM vs LM; (F) HF vs MF; (G) LF vs MF; (H) LM vs MM. Sample name explanation: H”- high concentration of PUFA in both sexes of sea urchin; “M”- medium concentration of PUFA in both sexes; “L”-low concentration of PUFA in both sexes; “HF” - high concentration of PUFA in ovaries of female sea urchins; “HM” - high concentration of PUFA in testes of male sea urchins; “MF” - medium concentration of PUFA in ovaries; “MM” - medium concentration of PUFA in testes; “LF” - low concentration of PUFA in ovaries; “LM” - low concentration of PUFA in testes. The number (1, 2, 3) means three replicates.
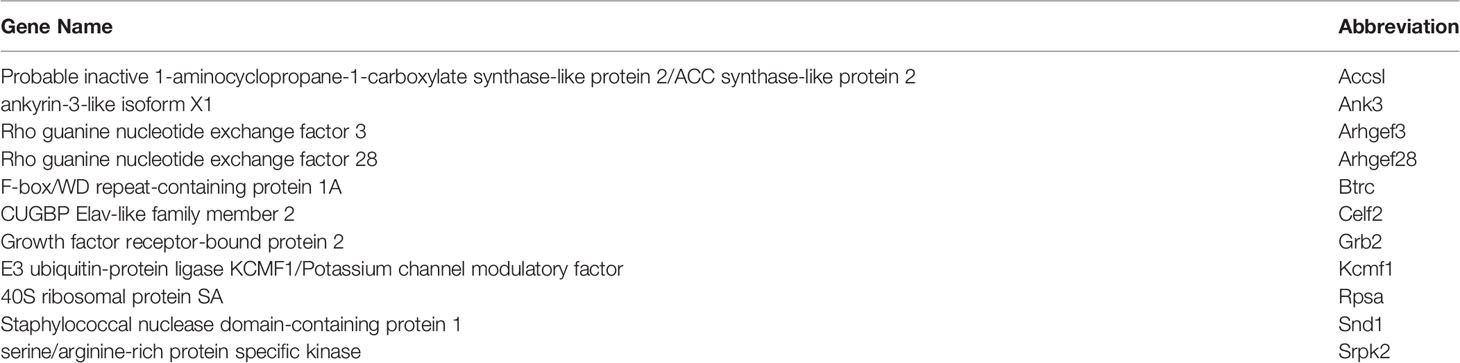
Table 3 Differentially expressed genes related to fatty acid metabolism among different sexes and different PUFA abundance groups of Strongylocentrotus intermedius.
Transcriptomic Data Validation
To verify the validity of the transcriptomic data, 11 selected DEGs (Accsl, Ank3, Arhgef3, Arhgef28, Btrc, Celf2, Grb2, Kcmf1, Rpsa, Snd1, and Srpk2 genes) related to fatty acid metabolism were used to investigate the expression profiles based on qRT-PCR, and the verification results are shown in Figure 4. Gene names and abbreviations of 11 DEGs were shown in Table 3. Their consistency demonstrated the accuracy of the method, which detected the correct gene expression changes in the transcriptomic sequencing. We found that the Btrc, Rpsa, Snd1, and Srpk2 genes were highly expressed in the high PUFA abundance group, and there were several down-regulated genes such as Accsl, Ank3, Celf2, Grb2, and Kcmfl almost in the testis. Arhgef3 and Arhgef28 genes showed high expression in the medium PUFA abundance group.
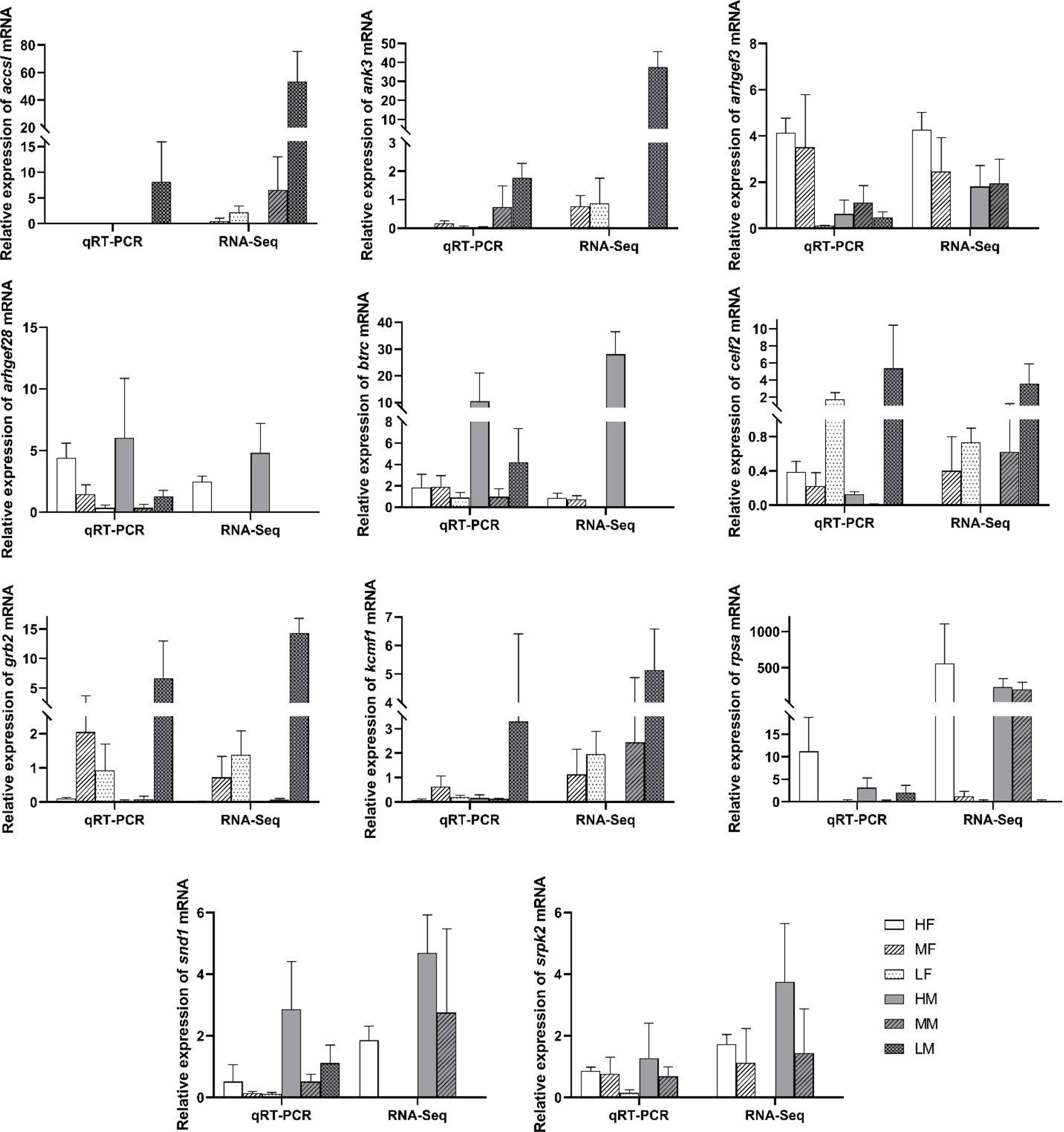
Figure 4 Validation differentially expressed genes (DEGs) in the different sexes and different PUFA abundance groups by qRT-PCR. The relative expression of each gene were normalized to 18 S rRNA gene. Bars represent the mean ± SEM (n=3). Sample name explanation: “HF” - high concentration of PUFA in ovaries of female sea urchins; “HM” - high concentration of PUFA in testes of male sea urchins; “MF” - medium concentration of PUFA in ovaries; “MM” - medium concentration of PUFA in testes; “LF” - low concentration of PUFA in ovaries; “LM” - low concentration of PUFA in testes.
Candidate Genes Related to PUFA Metabolism
To investigate the mechanism of PUFA metabolism in sea urchin gonads, integrated analysis was performed on the lipidomics and transcriptomic levels. The number of DEGs and significantly changed lipid molecules among the different sexes and different PUFA abundance groups are shown in Table 4. There were 16 DEGs and 266 lipids in H vs L comparation, 6 DEGs and 160 lipids in H vs M comparation, 11 DEGs and 129 lipids in M vs L comparation of both sexes of sea urchins, respectively. On the distinction of sea urchin sexes, there were 228 DEGs and 123 lipids, 346 DEGs and 322 lipids in high and low concentration PUFA group, respectively. Then these DEGs and lipids were used to carry out the correlation analysis, and the heat maps performed are shown in Figure 5. Interestingly, in the correlation analysis, we found that the unigene (ID: TRINITY_DN1066_c0_g1) was up-regulated in the high concentration PUFA group, and this gene was significantly related to EPA (C20:5) (p<0.05) (Figure 5A). We used ORF finder to search for the open reading frame of the sequence and identify it in the NCBI database. The putative amino acid sequence had 93.67% identify with the tudor domain-containing protein 3 (TDRD3) of Strongylocentrotus purpuratus, 79.39% with that of Lytechinus variegatus, and 41.24% with that of Patiria miniata, and the phylogenetic tree is shown in Figure 6.
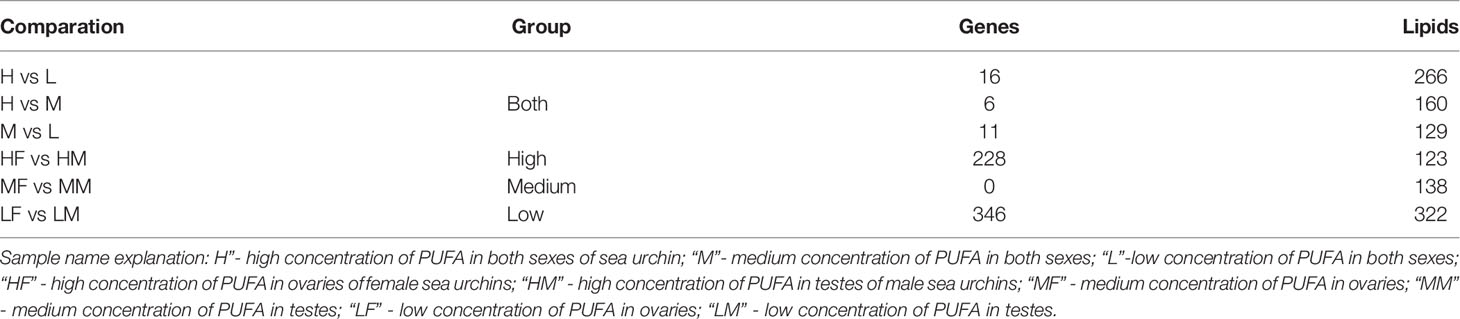
Table 4 The number of differentially expressed genes and significantly changed lipid molecules in transcriptome and lipidome.
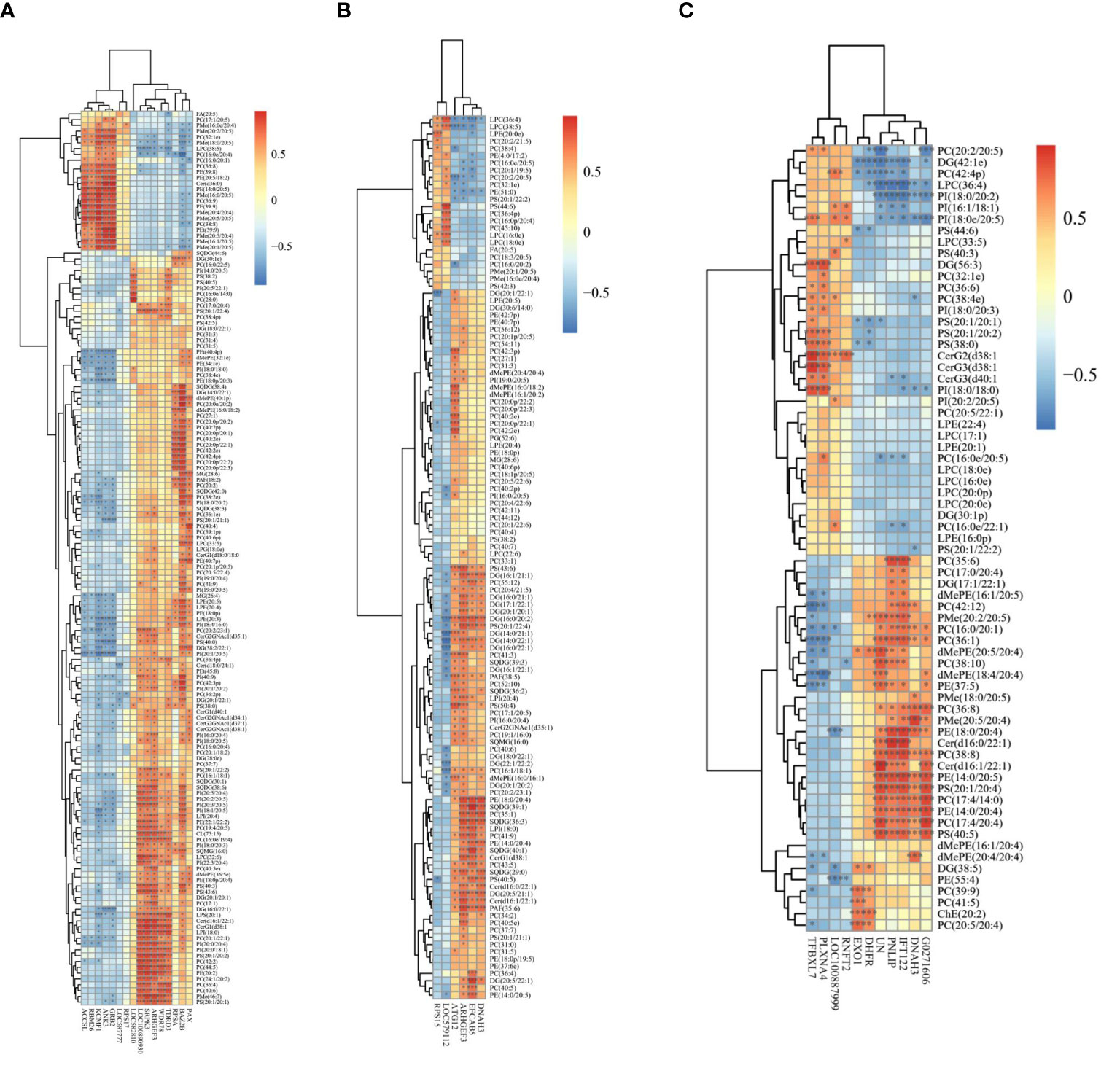
Figure 5 Heatmaps of the correlation analysis based on differentially expressed genes and significantly changed lipid molecules from pairwise comparisons (A) H vs L; (B) H vs M; (C) M vs L.
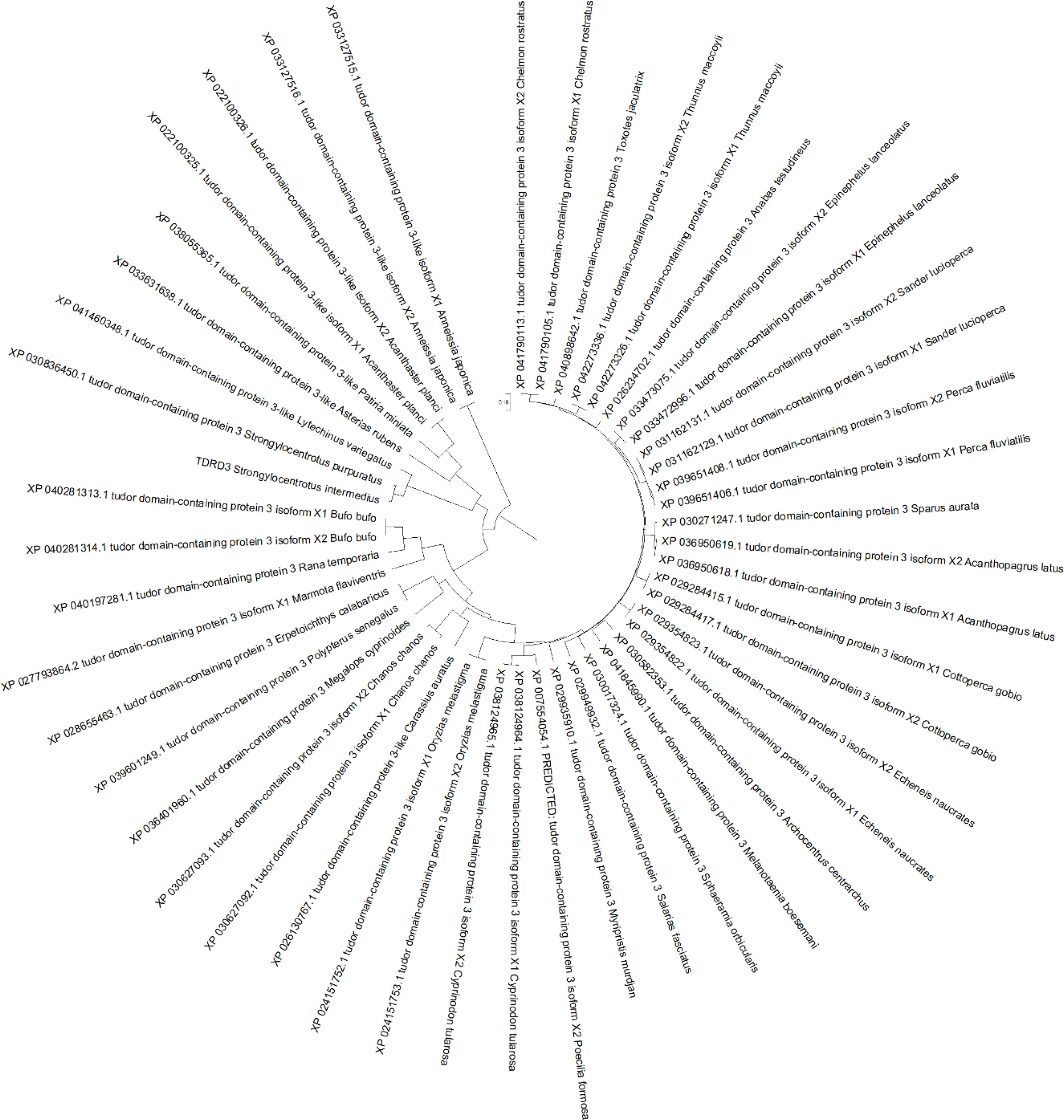
Figure 6 Phylogenetic relationship of tudor domain-containing protein 3 (TDRD3) among Strongylocentrotus intermedius and related species.
Discussion
The lipid content of sea urchin gonads is considered to be highest in mature specimens (Liyana-Pathipana et al., 2002; Zhou et al., 2018), and the gonads as the main lipid depots are considered to support many physiological processes in the sea urchin (Siliani et al., 2016). According to the results for PUFA concentration, we selected samples (both male and female sea urchins) from three groups, and found that there was no significant difference between PUFA concentration and weight (including body, gonad and mouth)/GSI, as shown in Figure 1 (p > 0.05). Therefore, we considered that the multi-omics analysis would be related to PUFA abundance in the sea urchin. Moreover, as mentioned before, the bioprocesses of PUFA biosynthesis and metabolism involve multiple gene expression networks and complex biochemical pathways (Wang et al., 2020). Compared with the results of our previous study, we could narrow down the DEGs list, and focused on much fewer DEGs that are related to lipid metabolism.
The most important finding in this study was that TDRD3 was inferred to be related to the EPA metabolism process. According to the NCBI database, the putative amino acid sequence of S. intermedius TDRD3 has highly homology with that of other animals (Figure 6). TDRD3, as a modular scaffolding protein, could specifically recognize and bind dimethylarginine-containing proteins in mammals (Siaw et al., 2016). TDRD3 could recognize and bind asymmetric dimethylation on histone tails associated with transcriptional activation, and then recruit proteins in the nucleus. Moreover, it also plays a role in the regulation of translation of target mRNAs by binding Arg/Gly-rich motifs and in the assembly/disassembly of mRNA stress granules in the cytoplasm (Morettin et al., 2017; Peng et al., 2020). We believe that this candidate gene will show us a novel way to uncover the mechanism of the EPA metabolism process in the sea urchin.
In this study, the number of significant differentially expressed genes which were related to lipid/PUFA biosynthesis or metabolism was limited (Table 4), and we have selected 11 DEGs from “High PUFA” comparation groups among these genes, while the results of the gene expressions are shown in Figure 4. We found that the Btrc, Rpsa, Snd1, and Srpk2 genes were highly expressed in the high PUFA abundance group. F-box/WD repeat-containing protein 1A is encoded by the Btrc gene, and recognizes and binds to phosphorylated target proteins (Suzuki et al., 1999). The 40S ribosomal protein SA, encoded by the Rpsa gene, is a ribosomal protein and could act as a cell surface receptor in laminins (Jackers et al., 1996). Staphylococcal nuclease domain-containing protein 1, encoded by the Snd1 gene, is a main component of the RNA-induced splicing complex and RISC complex, could play an important role in mediating RNA interference, and it is a promising biomarker in the research of prostate cancer and therapeutic target (Yang et al., 2002; Kuruma et al., 2009). Serine/arginine-rich protein-specific kinase, which is encoded by the Srpk2 gene, could down-regulate HBV replication regulation by reducing the packaging efficiency of the pregenomic RNA and it does not affect the formation of the viral core particles (Sridhara et al., 2017). We have inferred that the expression of these genes might promote PUFA accumulation in sea urchin gonads.
There were several down-regulated genes such as Accsl, Ank3, Celf2, Grb2, and Kcmfl almost in the testis. Probable inactive 1-aminocyclopropane-1-carboxylate synthase-like protein 2/ACC synthase-like protein 2, encoded by the Accsl gene, lacks some residues that are necessary for activity, thus suggesting that lacks enzymatic activity while it has catalytic activity and is related to pyridoxal phosphate binding (Liang et al., 1992). Ankyrin-3, encoded by the Ank3 gene, belongs to the ankyrin family. Ankyrin family plays key roles in the cell motility, proliferation, activation, contact and membrane domains maintenance (Roby, 2017). CUGBP Elav-like family member 2, encoded by the Celf2 gene, as an RNA-binding protein, plays key roles in the regulation of several post-transcriptional events and takes part in pre-mRNA alternative splicing, mRNA translation, mRNA stability, and apoB RNA editing (Nasiri-Aghdam et al., 2021). Moreover, Celf2 mediates glioma cell proliferation, invasion, and apoptosis (Liao et al., 2019), and could increase COX2 mRNA stability and inhibit the translation in epithelial cells after radiation injury (Treiber et al., 2017). Growth factor receptor-bound protein 2, encoded by the Grb2 gene, takes part in signal transduction and cell communication as an adaptor protein. Grb2 could bind the epidermal growth factor receptor as a dominant negative protein, suppressing proliferative signals, and this may induce active programmed cell death (Pao-Chun et al., 2009). E3 ubiquitin-protein ligase KCMF1 (potassium channel modulatory factor), encoded by the Kcmf1 gene, could promote ubiquitination with the intrinsic E3 ubiquitin ligase activity (Jang, 2004). We have inferred that these proteins might inhibit PUFA absorption, accumulation, or biosynthesis processes in sea urchin gonads.
Rho-like GTPases could take part in various cellular processes. They could activate by binding GTP and inactivated by conversion of GTP to GDP. Guanine nucleotide exchange factors could accelerate the GTPase activity of Rho GTPases by catalyzing the release of bound GDP. Rho guanine nucleotide exchange factor 3 (Arhgef3) and factor 28 (Arhgef28) genes encode the guanine nucleotide exchange factors, and they could regulate the major signaling pathways downstream of growth factor receptors and the expression and aggregation of NEFL which plays a role in cancer cell apoptosis and invasion (Schmidt and Debant, 2014). These two genes showed high expression in the medium PUFA abundance group, which might be related to lipid or PUFA biosynthesis or metabolism, but we are unable to conclude that until more verification is done in future research.
Conclusion
In conclusion, the comparative lipidomics and transcriptomic analysis reveal the difference among different PUFA abundances of sea urchin gonads. A total of 894,295,712 clean reads was sequenced in 18 cDNA libraries (high, medium and low PUFA groups in both sexes). We found nine up- and seven down-regulated unigenes were found in the comparation of H vs L group. Interestingly, tudor domain-containing protein 3 (TDRD3) was up-regulated in the high concentration of PUFA group, and this gene was significantly related to eicosapentaenoic acid (EPA) in the correlation analysis which may be used as a candidate marker for EPA biosynthesis and metabolism in sea urchin. Considering the above results, the findings could provide a more thorough understanding of the molecular mechanisms of PUFA biosynthesis and metabolism in S. intermedius, in addition, we wish these could act as a valuable resource for the practical applications and regulation of the sea urchin aquaculture industry.
Data Availability Statement
The datasets presented in this study can be found online at http://www.ncbi.nlm.nih.gov/bioproject/759708.
Author Contributions
Conceptualization, HW and WZ. Methodology, WZ. Software, HW and XL. Validation, HW, YC, and JD. Formal analysis, HW. Investigation, WZ. Resources, RZ. Data curation, LH. Writing—original draft preparation, HW and WZ. Writing—review and editing, HW. Visualization, XL and DG. Supervision, JD. Project administration, JD. Funding acquisition, HW, YC, and JD. All authors contributed to the article and approved the submitted version.
Funding
This research was supported by a grant from National Natural Science Foundation of China [32002414], the National Key Research and Development Program of China [2018YFD0901601], Dalian Youth Science and Technology Star [2020RQ120], Educational Department of Liaoning Province [JL202004], Liaoning Innovative Research Team in University [LT2019003], and Liaoning Province “Xingliao Talents Plan” Project [XLYC2002107].
Conflict of Interest
The authors declare that the research was conducted in the absence of any commercial or financial relationships that could be construed as a potential conflict of interest.
Publisher’s Note
All claims expressed in this article are solely those of the authors and do not necessarily represent those of their affiliated organizations, or those of the publisher, the editors and the reviewers. Any product that may be evaluated in this article, or claim that may be made by its manufacturer, is not guaranteed or endorsed by the publisher.
Acknowledgments
We thank Shanghai Bioprofile Co., Ltd. for helping the initial analysis of raw data of lipidome and transcriptome.
Supplementary Material
The Supplementary Material for this article can be found online at: https://www.frontiersin.org/articles/10.3389/fmars.2022.777341/full#supplementary-material
References
Agatsuma Y. (2013). “Strongylocentrotus Intermedius,” in Developments in Aquaculture and Fisheries Science, Sea Urchins: Biology and Ecology, vol. 38. Ed. Lawrence J. M. (Netherlands: Elsevier: Amsterdam), 437–447.
Bouwens M., Van De Rest O., Dellschaft N., Bromhaar M. G., De Groot L. C., Geleijnse J. M., et al. (2009). Fish-Oil Supplementation Induces Antiinflammatory Gene Expression Profiles in Human Blood Mononuclear Cells. Am. J. Clin. Nutr. 90, 415–424. doi: 10.3945/ajcn.2009.27680
Chi X., Shi D., Ma Z., Hu F., Sun J., Huang X., et al. (2021). Carryover Effects of Long-Term High Water Temperatures on Fitness-Related Traits of the Offspring of the Sea Urchin Strongylocentrotus Intermedius. Mar. Environ. Res. 169, 105371. doi: 10.1016/j.marenvres.2021.105371
Conesa A., Götz S., García-Gómez J. M., Terol J., Talón M., Robles M. (2005). Blast2GO: A Universal Tool for Annotation, Visualization and Analysis in Functional Genomics Research. Bioinformatics 21, 3674–3676. doi: 10.1093/bioinformatics/bti610
Di Natale M., Bennici C., Biondo G., Masullo T., Monastero C., Tagliavia M., et al. (2019). Aberrant Gene Expression Profiles in Mediterranean Sea Urchin Reproductive Tissues After Metal Exposures. Chemosphere 216, 48–58. doi: 10.1016/j.chemosphere.2018.10.137
Grabherr M. G., Haas B. J., Yassour M., Levin J. Z., Thompson D. A., Amit I., et al. (2011). Full-Length Transcriptome Assembly From RNA-Seq Data Without a Reference Genome. Nat. Biotech. 29, 644–652. doi: 10.1038/nbt.1883
Jackers P., Minoletti F., Belotti D., Clausse N., Sozzi G., Sobel M. E., et al. (1996). Isolation From a Multigene Family of the Active Human Gene of the Metastasis-Associated Multifunctional Protein 37LRP/P40 at Chromosome 3p21.3. Oncogene 13, 495–503.
Jang J. H. (2004). FIGC, a Novel FGF-Induced Ubiquitin-Protein Ligase in Gastric Cancers. FEBS Lett. 578, 21–25. doi: 10.1016/j.febslet.2004.10.071
Kuruma H., Kamata Y., Takahashi H., Igarashi K., Kimura T., Miki K., et al. (2009). Staphylococcal Nuclease Domain-Containing Protein 1 as a Potential Tissue Marker for Prostate Cancer. Am. J. Pathol. 174, 2044–2050. doi: 10.2353/ajpath.2009.080776
Liang X., Abel S., Keller J. A., Shen N. F., Theologis A. (1992). The 1-Aminocyclopropane-1-Carboxylate Synthase Gene Family of Arabidopsis Thaliana. P. Natl. Acad. Sci. U. S. A. 89, 11046–11050. doi: 10.1073/pnas.89.22.11046
Liao C., Chen W., Wang J. (2019). MicroRNA-20a Regulates Glioma Cell Proliferation, Invasion, and Apoptosis by Targeting CUGBP Elav-Like Family Member 2. World Neurosurg. 121, e519–e527. doi: 10.1016/j.wneu.2018.09.155
Livak K. J., Schmittgen T. D. (2001). Analysis of Relative Gene Expression Data Using Real-Time Quantitative PCR and the 2(-Delta Delta C(T)) Method. Methods 25, 402–408. doi: 10.1006/meth.2001.1262
Liyana-Pathipana C., Shahidi F., Whittick A., Hooper R. (2002). Lipid and Lipid Soluble Components of Gonads of Green Sea Urchin (Strongylocentrotus Droebachiensis). J. Food Lipids 9, 105–126. doi: 10.1111/j.1745-4522.2002.tb00213.x
Masullo T., Biondo G., Natale M. D., Tagliavia M., Bennici C. D., Musco M., et al. (2021). Gene Expression Changes After Parental Exposure to Metals in the Sea Urchin Affect Timing of Genetic Programme of Embryo Development. Biology 10, 103. doi: 10.3390/biology10020103
Morettin A., Paris G., Bouzid Y., Baldwin R. M., Falls T. J., Bell J. C., et al. (2017). Tudor Domain Containing Protein 3 Promotes Tumorigenesis and Invasive Capacity of Breast Cancer Cells. Sci. Rep. 7, 1–13. doi: 10.1038/s41598-017-04955-4
Murzina S. A., Dgebuadze P. Y., Pekkoeva S. N., Voronin V. P., Mekhova E. S., Thanh N. T. (2021). Lipids and Fatty Acids of the Gonads of Sea Urchin Diadema Setosum (Echinodermata) From the Coastal Area of the Nha Trang Bay, Central Vietnam. Eur. J. Lipid Sci. Technol. 123, 2000321. doi: 10.1002/ejlt.202000321
Nasiri-Aghdam M., Garcia-Garduño T. C., Jave-Suárez L. F. (2021). CELF Family Proteins in Cancer: Highlights on the RNA-Binding Protein/Noncoding RNA Regulatory Axis. Int. J. Mol. Sci. 22, 11056. doi: 10.3390/ijms222011056
Nettleton J. A., Salem J. (2019). International Society for the Study of Fatty Acids and Lipids 2018 Symposium: Arachidonic and Docosahexaenoic Acids in Infant Development. Ann. Nutr. Metab. 74, 83–91. doi: 10.1159/000495906
Pao-Chun L., Chan P. M., Chan W., Manser E. (2009). Cytoplasmic ACK1 Interaction With Multiple Receptor Tyrosine Kinases Is Mediated by Grb2: An Analysis of ACK1 Effects on Axl Signaling. J. Biol. Chem. 284, 34954–34963. doi: 10.1074/jbc.M109.072660
Peng B.L., Li W. J., Ding J. C., He Y. H., Ran T., Xie B. L., et al. (2020). A Hypermethylation Strategy Utilized by Enhancer-Bound CARM1 to Promote Estrogen Receptor α-Dependent Transcriptional Activation and Breast Carcinogenesis. Theranostics 10, 3451. doi: 10.7150/thno.39241
Raposo A., Ramos R., Anjos C. M., Pombo A., Ferreira S. M., Baptista T. M., et al. (2016). “Effect of Three Diets on Gonad Fatty Acid Composition of Reared Sea Urchin Paracentrotus Lividus,” in International Meeting on Marine Research 2016 Abstract (Peniche, Portugal: ESTM - Polytechnic Institute of Leiria, Santuário de Nossa Senhora dos Remédios). doi: 10.3389/conf.FMARS.2016.04.00034
Roby Y. (2017). ANK3 Gene Polymorphisms and Bipolar Disorder: A Meta-Analysis. Psychiat. Genet. 27, 225–235. doi: 10.1097/YPG.0000000000000186
Schmidt S., Debant A. (2014). Function and Regulation of the Rho Guanine Nucleotide Exchange Factor Trio. Small GTPases 5, e983880. doi: 10.4161/sgtp.29769
Shahidi F., Ambigaipalan P. (2018). Omega-3 Polyunsaturated Fatty Acids and Their Health Benefits. Annu. Rev. Food Sci. Technol. 9, 345–381. doi: 10.1146/annurev-food-111317-095850
Siaw G. E. L., Liu I. F., Lin P. Y., Been M. D., Hsieh T. S. (2016). DNA and RNA Topoisomerase Activities of Top3β Are Promoted by Mediator Protein Tudor Domain-Containing Protein 3. P. Natl. Acad. Sci. U. S. A. 113, E5544–E5551. doi: 10.1073/pnas.1605517113
Siliani S., Melis R., Loi B., Guala I., Baroli M., Sanna R., et al. (2016). Influence of Seasonal and Environmental Patterns on the Lipid Content and Fatty Acid Profiles in Gonads of the Edible Sea Urchin Paracentrotus Lividus From Sardinia. Mar. Environ. Res. 113, 124–133. doi: 10.1016/j.marenvres.2015.12.001
Sneath P. H., Sokal R. R. (1973). Numerical Taxonomy. The Principles and Practice of Numerical Classification (San Francisco: W.H. Freeman & Co. Ltd).
Sridhara S. C., Carvalho S., Grosso A. R., Gallego-Paez L. M., Carmo-Fonseca M., de Almeida S. F. (2017). Transcription Dynamics Prevent RNA-Mediated Genomic Instability Through SRPK2-Dependent DDX23 Phosphorylation. Cell Rep. 18, 334–343. doi: 10.1016/j.celrep.2016.12.050
Suzuki H., Chiba T., Kobayashi M., Takeuchi M., Suzuki T., Ichiyama A., et al. (1999). Iκbα Ubiquitination Is Catalyzed by an SCF-Like Complex Containing Skp1, Cullin-1, and Two F-Box/WD40-Repeat Proteins, βtrcp1 and βtrcp2. Biochem. Bioph. Res. Co. 256, 127–132. doi: 10.1006/bbrc.1999.0289
Swanson D., Block R., Mousa S. A. (2012). Omega-3 Fatty Acids EPA and DHA: Health Benefits Throughout Life. Adv. Nutr. 3, 1–7. doi: 10.3945/an.111.000893
Treiber T., Treiber N., Plessmann U., Harlander S., Daiß J. L., Eichner N., et al. (2017). A Compendium of RNA-Binding Proteins That Regulate MicroRNA Biogenesis. Mol. Cell 66, 270–284.e13. doi: 10.1016/j.molcel.2017.03.014
Vergara-Amado J., Silva A. X., Manzi C., Nespolo R. F., Cárdenas L. (2017). Differential Expression of Stress Candidate Genes for Thermal Tolerance in the Sea Urchin Loxechinus Albus. J. Therm. Biol. 68, 104–109. doi: 10.1016/j.jtherbio.2017.03.009
Wang H., Ding J., Ding S., Chang Y. (2019). Transcriptome Analysis to Characterize the Genes Related to Gonad Growth and Fatty Acid Metabolism in the Sea Urchin Strongylocentrotus Intermedius. Genes Genom. 41, 1397–1415. doi: 10.1007/s13258-019-00864-0
Wang H., Ding J., Ding S., Chang Y. (2020). Integrated Metabolomic and Transcriptomic Analyses Identify Critical Genes in Eicosapentaenoic Acid Biosynthesis and Metabolism in the Sea Urchin Strongylocentrotus Intermedius. Sci. Rep. 10, 1697. doi: 10.1016/j.cbd.2021.100900
Wang H., Zhao W., Ding B., Zhang Y., Huang X., Liu X., et al. (2021). Comparative Lipidomics Profiling of the Sea Urchin, Strongylocentrotus Intermedius. Comp. Biochemi. Phys. D 40, 100900. doi: 10.1016/j.cbd.2021.100900
Watts S. A., Lawrence J. M., Lawrence A. L. (2010). “Approaches to the Study of Sea Urchin Nutrition,” in Proceedings of the 12th International Echinoderm Conference. Eds. Harris L. G., Boettger S. A., Walker C. W., Lesser M. P. (New Hampshire: CRC Press/Balkema, Durham), pp. 331–345.
Yang J., Aittomäki S., Pesu M., Carter K., Saarinen J., Kalkkinen N., et al. (2002). Identification of P100 as a Coactivator for STAT6 That Bridges STAT6 With RNA Polymerase II. EMBO J. 21, 4950–4958. doi: 10.1093/emboj/cdf463
Yang M., Hu F., Leng X., Chi X., Yin D., Ding J., et al. (2021). Long-Term Effects of Light Spectra on Fitness Related Behaviors and Growth of the Sea Urchin Strongylocentrotus Intermedius. Aquaculture 537, 736518. doi: 10.1016/j.aquaculture.2021.736518
Zhou X., Zhou D. Y., Lu T., Liu Z. Y., Zhao Q., Liu Y. X., et al. (2018). Characterization of Lipids in Three Species of Sea Urchin. Food Chem. 241, 97–103. doi: 10.1016/j.foodchem.2017.08.076
Keywords: Strongylocentrotus intermedius, polyunsaturated fatty acids, lipidome, transcriptome, integrated analysis, eicosapentaenoic acid, tudor domain-containing protein 3
Citation: Wang H, Zhao W, Liu X, Gang D, Zuo R, Han L, Chang Y and Ding J (2022) Comparative Lipidome and Transcriptome Provide Novel Insight Into Polyunsaturated Fatty Acids Metabolism of the Sea Urchin. Front. Mar. Sci. 9:777341. doi: 10.3389/fmars.2022.777341
Received: 15 September 2021; Accepted: 22 March 2022;
Published: 27 April 2022.
Edited by:
Taewoo Ryu, Okinawa Institute of Science and Technology Graduate University, JapanReviewed by:
Valeria Di Dato, Stazione Zoologica Anton Dohrn Napoli, ItalyYu Zhang, Shenzhen University, China
Copyright © 2022 Wang, Zhao, Liu, Gang, Zuo, Han, Chang and Ding. This is an open-access article distributed under the terms of the Creative Commons Attribution License (CC BY). The use, distribution or reproduction in other forums is permitted, provided the original author(s) and the copyright owner(s) are credited and that the original publication in this journal is cited, in accordance with accepted academic practice. No use, distribution or reproduction is permitted which does not comply with these terms.
*Correspondence: Jun Ding, ZGluZ2p1bjE5NzMxMTE5QGhvdG1haWwuY29t
†These authors have contributed equally to this work