- Key Laboratory of Mariculture and Stock Enhancement in North China’s Sea (Ministry of Agriculture and Rural Affairs), Dalian Ocean University, Dalian, China
This study was carried out to investigate the effects of five formulated feeds with different lipid sources (colza oil (CO), fish oil (FO), linseed oil (LO), soybean oil (SO), or palm oil (PO)) and kelp (Laminaria japonica) on the growth, reproductive performance of broodstock, and early larvae quality of sea urchin (Strongylocentrotus intermedius). The experimental diets were randomly allocated to a total of 48 (eight urchins per experimental group) individually cultured S. intermedius(initial weight 90.51 ± 0.82g) and the feeding period lasted for 12 weeks. The results showed that the weight gain rate of female sea urchins fed kelp was significantly higher than those fed formulated feeds while the gonadosomatic index of spawned female sea urchins fed kelp was significantly lower than those fed formulated feeds.S. intermedius broodstock fed FO showed the largest egg diameter and fecundity, which could be due to the abundant n-3 LC-PUFA deposited in the gonads of this group. Sea urchins fed SO showed the lowest fecundity and hatching rate, and the highest inflammation level. Sea urchins fed CO showed the highest content of oleic acid in the gonads and eggs, but the lowest fertilization rate. The highest hatching rate was observed in the kelp treatment, which was comparable to that in the LO and PO but was significantly higher than that in CO, FO, and SO. Before mouth opening, all prismatic larvae showed no significant differences in survival during the first 2 days post hatchery (DPH). At the 3 DPH, the survival of S. intermedius larvae was highest in the FO group, followed by those in the PO group, with the lowest survival observed in the kelp group. Thus, FO was accepted as the most ideal lipid source based on growth, reproductive performance, and early larval quality. These results could contribute to adopting an efficient feeding strategy to promote the reproductive performance and offspring quality by choosing the optimal lipid source for S. intermedius broodstock.
Introduction
The gonads of sea urchins are highly accepted as luxury seafood products due to their delicious taste and high nutritional value (Xin et al., 2018; Loureno et al., 2019; Wang et al., 2019). In the last decades, the demand for sea urchins has seen a sharp increase worldwide, which directly causes overfishing and destruction of their wild stocks (Prato et al., 2018; Li et al., 2021; Nhan et al., 2020). To mitigate this issue, aquaculture of sea urchins has been attempted globally and has proven to be an effective alternative strategyforf producing gonads with high yield and market acceptance (Phillips et al., 2010; Onomu et al., 2020). Strongylocentrotus intermedius, which is naturally distributed in the coastal areas of North Japan and Far Eastern Russia, was first introduced to China from Japan by Dalian Ocean University in 1989 (Chang et al., 2012). Up to now, it has become one of the most important sea urchin species for aquaculture in the coastal areas of China (Zuo et al., 2018; Wang et al., 2019). At present, fresh kelp Laminaria japonica are used as the main food category for S. intermedius (Zuo et al., 2018; Li et al., 2020). However, the gonad development of S. intermedius was shown to be retarded when they were fed solely macroalgae and could be markedly accelerated after the addition of some mussel Mytilus edulis (Zhou et al., 2013). Thus, it is imperative to quantify the nutritional requirement and formulate specialized formulated feeds for the broodstock of this species.
It has been shown that the quality of broodstock diets and their nutrient levels have profound influences on the gonad maturation, reproductive performance, and subsequent offspring quality of numerous aquatic animals (Izquierdo et al., 2001; Mazorra et al., 2003; Sui et al., 2009; Tercero et al., 2015; Yldz et al., 2020). Lipids are not only one of the main energy sources, but also act as the providers of essential fatty acids, carriers of lipid-soluble vitamins, and the major structural components of cellular membranes (Zhou et al., 2007; Turchini et al., 2010; Wang et al., 2012; Gibbs et al., 2015). The level and fatty acid composition of dietary lipids are considered one of the key factors that affect the spawning performance and larvae quality of aquatic animals (Izquierdo et al., 2001). In particular, the absolute level and specific composition of long-chain polyunsaturated fatty acids (LC-PUFAs) and the ratio of members, such as arachidonic acid (ARA; 20:4n-6), eicosapentaenoic acid (EPA; 20:5n-3), and docosahexaenoic acid (DHA; 22:6n-3), have been proven to be critical factors in regulating the reproductive performance, egg, and larval viability of rainbow trout Oncorhynchus mykiss (Agh et al., 2019; Yldz et al., 2020; Yldz et al., 2021), Nile tilapia Oreochromis niloticus (Ng and Wang, 2011), Yucatan octopus Octopus Maya (Tercero et al., 2015), Chinese mitten crab Eriocheir sinensis (Xiao et al., 2002; Sui et al., 2009), tongue sole Cynoglossus semilaevis (Liang et al., 2014), Pearl gourami Trichogarster leeri (Mobaraki et al., 2020), European eel Anguilla anguilla (Kottmann et al., 2020), and Oriental river prawn Macrobrachium nipponense (Li et al., 2020). Fish oil (FO), as the major source of LC-PUFAs, will not be sufficient and continuous for the expanding aquaculture industry (Turchini and Francis, 2009; Ng and Wang, 2011; Tocher, 2015; Yuan et al., 2019). Vegetable oils (VOs), such as colza oil (CO), soybean oil (SO), linseed oil (LO), and palm oil (PO), are viable alternatives for FO due to their relatively stable supply, abundant PUFA, and low cost (Turchini and Francis, 2009; Gibbs et al., 2015; Cuesta-Gomez et al., 2020). However, little information is available about the effects of VO on the reproductive performance and larvae viability of sea urchins, especially when the requirement for LC-PUFA is guaranteed.
Gonads are the reproductive organ and sole edible part of sea urchins. Thus, different feed formulation should be designed for adult sea urchins to acquire market acceptance or reproductive success (Heflin et al., 2012; Brink-Hull et al., 2022). In a recent study, it was found that PO and CO showed comparable effects to FO for S. intermedius from the perspective of growth performance and gonad market acceptance (Ning et al., 2022). However, to the best of our knowledge, no information is available about the optimal lipid sources for achieving the best reproductive performance of S. intermedius. Thus, this study was conducted to investigate the effects of dietary lipid sources on the gonad development, fatty acid composition, spawning performance of broodstock, and early larvae quality of S. intermedius.
Materials and Methods
Experimental Broodstock Diets
Five isoproteic (25%) and isolipidic (6.0%) formulated feeds and kelp (L. japonica) were used as the experimental diets for sea urchin broodstock in this study. All feed ingredients were ground and passed through a 150 μm mesh. After all the solid ingredients of each formulated feed were thoroughly mixed, one of the following lipid sources, colza oil (CO), fish oil (FO), linseed oil (LO), soybean oil (SO), or palm oil (PO), was added and blended well. At last, about 30% water was mixed with the ingredients above before they were pressed through a die with 2 mm pores by a pellet-making machine (DES-TS1280, Jinan, China). The pellets were then dried at 50°C, tightly packed in sealed bags, and stored at -20° until use. The formulation and nutritional profile of formulated feeds can be found in Tables 1, 2.
Feeding Experiment
Adult S. intermedius with the age of 2.5 years were purchased from a local farm (Changhai County, Dalian, China). Then, sea urchins were reared in a rectangular tank (length: width: depth=180cm: 99cm: 70cm), where they were fed fresh kelp to acclimate the experimental conditions for 10 days. After that, sea urchins of similar size (initial weight 90.51 ± 0.82g) were individually assigned to 48 floating cages (22 cm × 28 cm × 48 cm) with each cage holding one individual. All cages were placed in fiber-reinforced rectangular plastic tanks (length: width: depth=95cm: 65cm: 55cm), with each tank holding eight cages. Each experimental diet was randomly allocated to eight urchins. To avoid feed waste, a Petri dish was placed at the bottom of each cage to prevent the feeds from falling through the gaps.
The experimental animals were handfed every day to a state of apparent satiation. To avoid the detrimental effects on water quality, residual feeds and feces were timely removed out of the tanks by siphoning about 6 hours after each feeding operation. Seawater was sand filtered, aerated, and preheated before they were filled into experimental tanks. Water in each tank was exchanged completely every 3 days. During the whole feeding experiment, the water quality parameters were monitored and recorded daily, with temperature maintained at 15 ± 1°C, salinity 33 ± 1‰, pH 8.0 ± 0.1, and dissolved oxygen >8.0 mg L−1. The feeding period lasted for 12 weeks.
Spawning, Egg, and Gonad Collection
At the end of the feeding experiment, the sea urchins were individually weighed after fasting for 24 h, and then artificially induced for spawning as described by Liu et al. (2005). First, 1 ml KCl (0.5 M) was injected into the coelomic cavity via the peristomial membrane of each sea urchin. Eggs or sperms began to flood out of the genital pores in about 30 min. Then, three female sea urchins and three male sea urchins were chosen out from each dietary group. The eggs of each sea urchin fed the same diet were collected, counted, and divided into three parts. The first part (about 100 eggs) was put into a 1.5 mL tube with 10% formalin for measuring the egg diameter. The second part was placed in 1.5 ml sterile tubes and then stored at -80°C for later analysis of fatty acids. The third part was placed in a sterile beaker with filtered seawater for the larval culture experiment. The collected sperm of broodstock fed the same diet were mixed and diluted with sterile seawater. Then, the diluted sperm were mixed with the eggs of the same dietary group (sperms/eggs ≈ 1000:1).
Fertilized eggs were hatched and incubated according to the method reported by Chang et al. (2012). Briefly, fertilized eggs collected from each female sea urchin were incubated in a 5L plastic tank (15 eggs/mL), where the water temperature was kept at 15°C ± 0.5°C. After hatching, healthy and strong prismatic larvae were carefully gathered by dragging a net across the one-third upper layer of each tank. Among them, some were transferred to a new 5L container for subsequent larval culture with a density of 1.0 individual/mL. The remaining healthy prismatic larvae in each tank were collected, pooled into a 1.5 tube, and stored at -80°C for later analysis of their fatty acids in response to different maternal nutrition. During the larval culture, the water temperature was maintained at 15 ± 1°C, salinity was 33 ± 1‰, pH was 8.0 ± 0.1, and oxygen was above 7 mg/L. The larval container was illuminated by less than 300 lx of incandescent light, with a natural photoperiod. During the larval culture, no feeding was carried out, and the quality of the prismatic larvae was evaluated by a starvation tolerance test (Quintana et al., 2015). Larvae in each tank were daily sampled to monitor the quantity, developmental stages, body length, and width. If four-armed larvae occurred in any tank, the starvation tolerance test was terminated. At this time, larvae within three tanks of each dietary treatment were pooled into one tube, flash frozen, and stored at -80 °C for later fatty acid analysis.
After spawning, three female sea urchins were weighed and then dissected for gonads and digestive tract. First, the digestive tract of each sea urchin was weighed to calculate the digestive tract index and was placed in a 1.5 ml tube and stored at -80°C until they were used for the analysis of related gene transcription. Then, gonads from three urchins were individually weighed to calculate the gonadosomatic index. After that, a small part of the gonad was carefully put in a 5 ml tube with Bouin’s reagent for subsequent histological analysis; the second part was placed in a 1.5 ml tube and stored at -80°C for analysis of gene transcription; the remaining gonads were pooled and then stored at -80°C for later determining the nutritional composition.
Gonadal Histology
The gonad slices were made by following the method of Ning et al. (2022). Briefly, the Bouin’s reagent fixed gonad was dehydrated by sinking them into a graded ethanol series (75%–100%) before they were embedded in paraffin. Tissue sections (4 μm in thickness) were prepared by using a microtome (Leica, RM2016). After that, slices were stained with hematoxylin and eosin, which were observed under an optical microscope (40 ×, Leica, Germany).
Nutritional Composition Analysis
The proximate composition of experimental feeds and gonads was analyzed according to AOAC (1995). Briefly, the contents of crude protein and crude lipids were detected by following the methods of Soxhlet and Kjeldahl, respectively. The content of moisture was determined by calculating the mass loss of the samples which were dried at 105°C.
Fatty acid composition of samples was assayed by following the method of Li et al. (2020). Here, the procedures were introduced briefly. First, the fatty acids in the samples were esterified into methyl esters, which were separated with hexane and transferred to a new tube. Before injection, certain amounts of C19:0 were added to each sample obtained above. Finally, the fatty acids were quantified by using gas chromatography (Thermo Fisher Trace 1310 ISQ). The temperature of the injector and detector was maintained at 290°C. The oven temperature rising procedures were set as follows: 80°C to 200°C at a speed of 10°C/min, 200°C to 250°C at a speed of 5°C/min, and 250°C to 270°C at a speed of 2°C/min. The fatty acid composition (g/kg) was calculated based on their relative peak area.
RNA Extraction and Real-Time Quantitative PCR
The sequences of the specific primers are presented in Table 3. The procedures of RNA extraction and real-time quantitative PCR have been previously described (Zuo et al., 2017). Briefly, total RNA was extracted and was reverse transcribed to cDNA by using a commercial kit (TaKaRa, Beijing, China). Then, the reaction regime (20 μL) was prepared as follows: 2 μL of cDNA, 0.8 μL of forward primer and reverse primer (10 mM), 10 μL of FastStart Essential DNA Green Master, and 6.4 μL of sterile distilled water. The quantitative PCR was performed by using the LightCycler® 96 real-time PCR system with the following reaction conditions: 95°C for 10 min; 95°C for 15 s; and 60°C for 60 s (45 cycles); 95°C for 10 s; 65°C for 60 s; and 97°C for 1 s. The formula of 2−ΔΔCT was used to calculate the relative gene expression of target genes (Kenneth and Thomas, 2002).
Calculations and Statistical Analysis
Survival rate (SR, %) = Nf/Ni ×100
Weight growth rate (WGR, %) = (Wb -Wi)/Wi ×100
Gonadosomatic index (GSI, %) = Wg/Wf ×100
Digestive tract index (DTI, %) = Wd/Wf ×100
Relative fecundity (RF, 103 eggs/g) = Et/Wb
Fertilization rate (FR, %) = E2/Et×100
Hatching rate (HR, %) = Ep/E2×100
Larvae survival rate (LSR, %) = Nd/Nt ×100
where Ni and Nf were the initial and final number of sea urchins in each dietary group. Wi was the initial body weight of each female sea urchin; Wb was the body weight of each female sea urchin before spawning; Wf, Wg and Wd were the body weight, gonad weight, and digestive tract weight of each female sea urchin after spawning. Et was the total number of eggs produced by each female sea urchin; E2 was the number of two-cell stage embryos in the same batch of eggs after fertilization; Ep was the number of healthy prismatic larvae after hatchery. Nt and Nd were the initial number of healthy prismatic larvae and number of live larvae in each tank during the first 3 days post hatchery.
The data were checked for normal distribution and homogeneity of variance before they were statistically analyzed by ANOVA with SPSS 22.0 software. When a significance (P<0.05) was detected, Duncan’s multiple comparison test was used to compare differences in the means between different treatments. The data were presented as means ± S.E.M (standard error of means).
Results
Survival and Growth Performance
No mortality was observed in any dietary group. Before spawning, the WGR of female sea urchins fed kelp was significantly higher than those fed formulated feeds (P<0.05). Among the formulated feed groups, sea urchins fed PO showed a marginally higher WGR than other lipid source groups (P>0.05). After spawning, the body weight of female sea urchins fed PO was comparable to those fed LO (P>0.05) but was significantly higher than those fed CO, FO, SO, and kelp (P<0.05). The gonad weight and GSI of spawned female sea urchins fed kelp were the lowest, which were significantly lower than those fed formulated feeds (P<0.05). Among the formulated feed groups, the highest gonad weight and GSI were observed in the PO group, which were significantly higher than those in the other groups (P<0.05). The DTI of female sea urchins fed FO was comparable to that of sea urchins fed LO and PO (P>0.05) but was significantly higher than those fed CO, SO, and kelp (P<0.05) (Table 4).
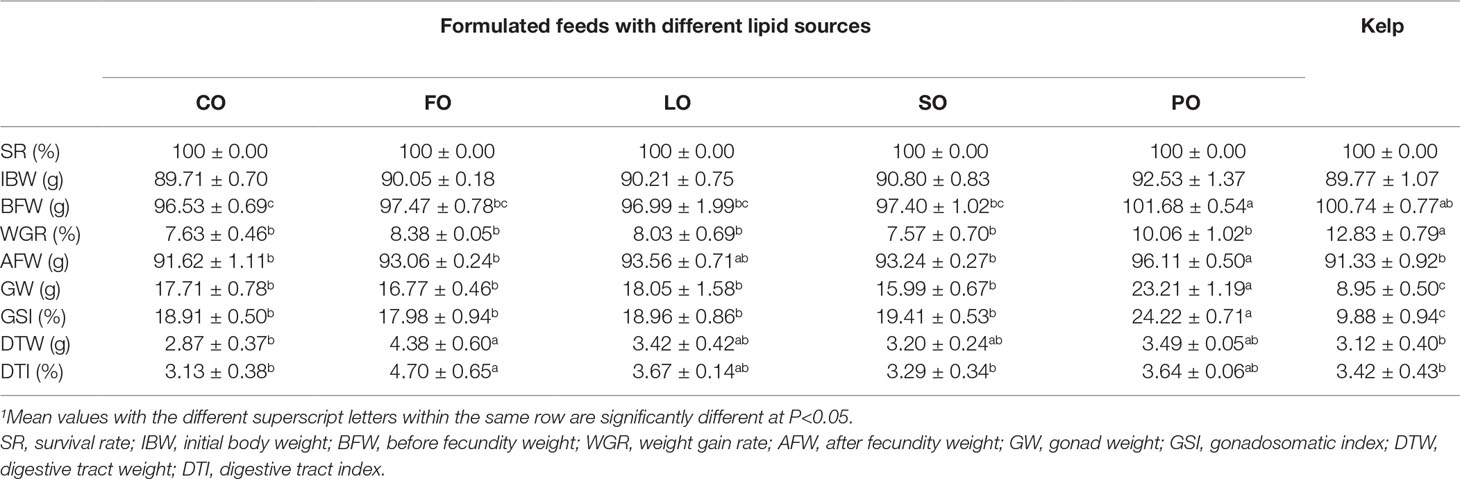
Table 4 Effects of different lipid sources on survival and growth performance of female adult sea urchin (Strongylocentrotus intermedius) (mean ± SEM, n=3)1.
Ovary Histological Observation
A small number of ova were loosely distributed in the space vacated by spawned ova. In the ovary of sea urchins fed kelp, there were still many vitellogenic oocytes that were distributed along the ovarian wall. By contrast, there were only a small number of vitellogenic oocytes observed in the ovaries of sea urchins fed formulated feeds. Generally, sea urchins fed FO showed less vitellogenic oocytes in their ovaries than those fed other lipid sources. The nutritive phagocytes (NP) in the ovary of sea urchins fed the formulated feeds showed a thin and pale meshwork structure, while the nutritive phagocytes in the kelp group showed a dense network structure (Figure 1).
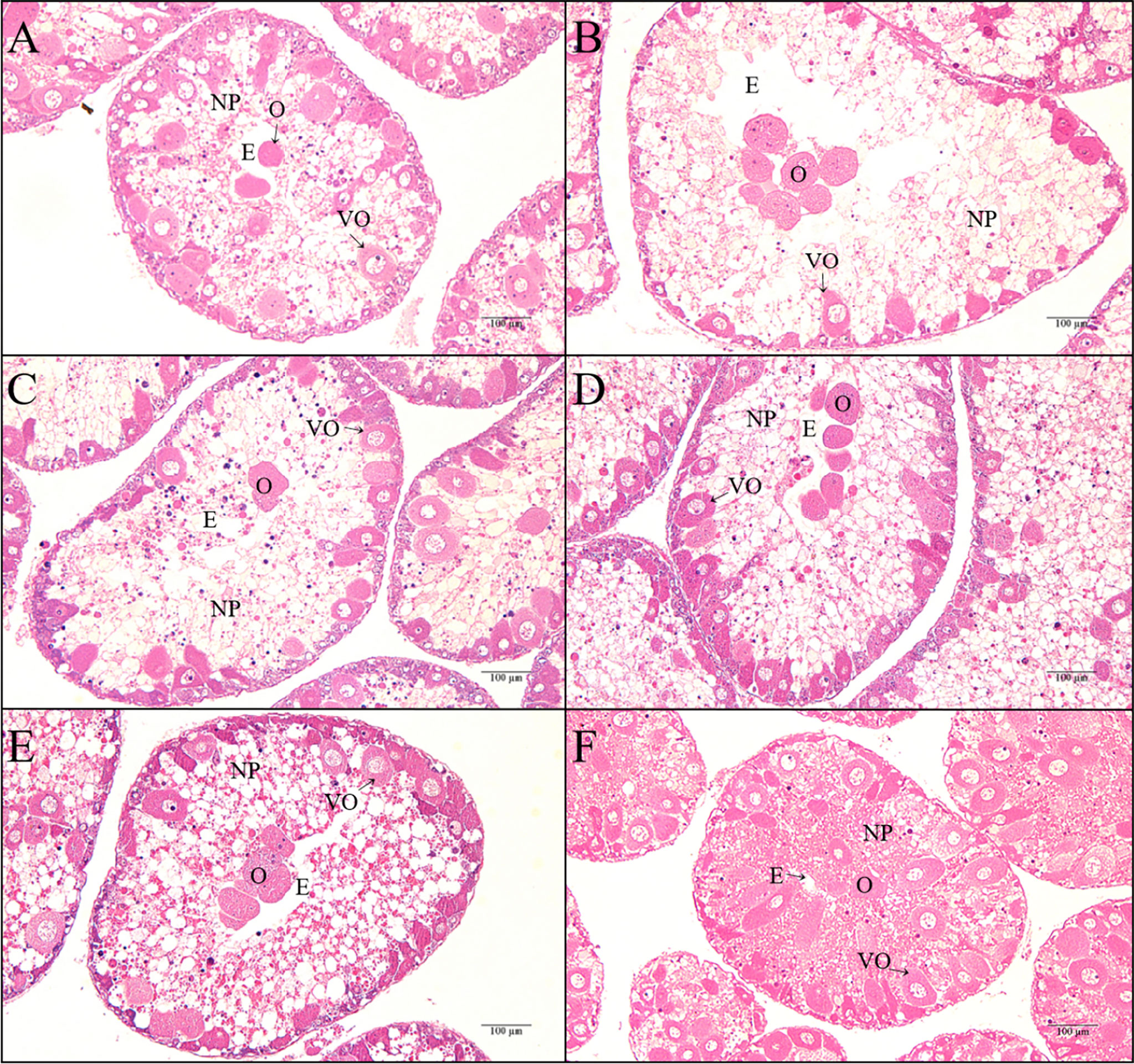
Figure 1 Histology observations in the ovaries of female adult sea urchin (Strongylocentrotus intermedius) fed diets with different lipid sources (colza oil (CO), fish oil (FO), linseed oil (LO), soybean oil (SO), or palm oil (PO)). (A) Colza oil, stage V; (B) Fish oil, stage V; (C) Linseed oil, stage V; (D) Soybean oil, stage V; (E) Palm oil, stage V; (F) Feed kelp, stage V. NP, nutritive phagocyte. VO, vitellogenic oocyte. O, ova. E, empty spaces.
Reproductive Performance
The relative fecundity was not significantly affected by diet categories or lipid sources, with a relatively higher value observed in the FO and kelp groups (P>0.05) (Figure 2A).
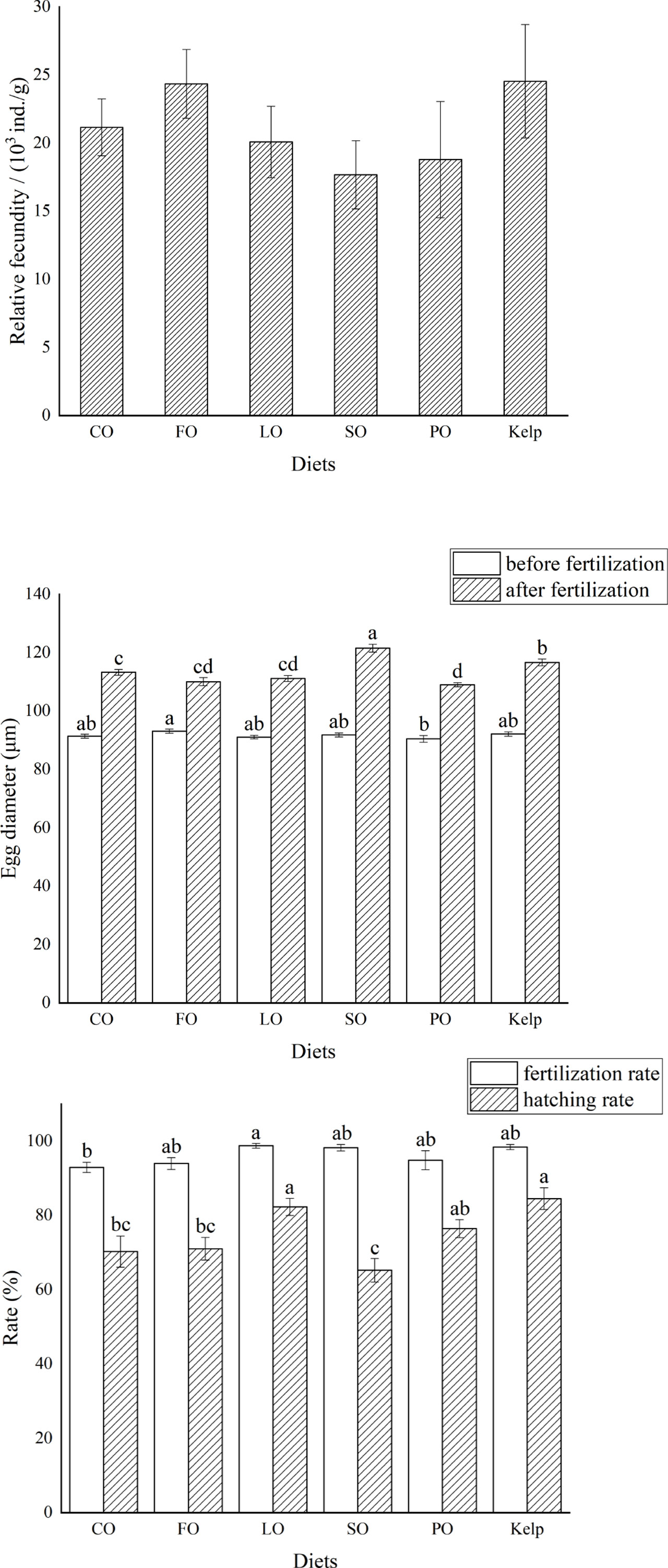
Figure 2 Effects of different lipid sources (colza oil (CO), fish oil (FO), linseed oil (LO), soybean oil (SO), or palm oil (PO)) on the relative fecundity, egg diameter, as well as fertilization rate and hatching rate of sea urchin (Strongylocentrotus intermedius) (mean ± SEM, n=3). Mean value bars within the same parameter of each chart bearing with different lowercase letters are significantly different at P < 0.05.
Before fertilization, the egg diameter in the FO group was significantly larger than that in the PO group (P<0.05). After fertilization, the egg diameter in the SO group was significantly larger than that in the other dietary groups (P<0.05). The lowest egg diameter was observed in the PO group, which was comparable to that in FO and CO groups (P>0.05) but was significantly lower than that in the other groups (P<0.05) (Figure 2).
The fertilization rate was highest in the LO group, which was only significantly higher than that in the CO group (P<0.05). The hatching rate was highest in the kelp group, which was comparable to that in LO and PO (P>0.05) but was significantly higher than that in CO, FO, and SO groups (P<0.05) (Figure 2).
Prismatic Larvae Quality
The body length of prismatic larvae was highest in the treatment of LO, which was only significantly higher than that in CO and SO (P<0.05). The body width of prismatic larvae was highest in the kelp group, which was comparable to that in CO (P>0.05) but was significantly higher than that in FO, LO, SO, and PO groups (P<0.05) (Table 5).

Table 5 Effects of different lipid sources on prismatic larvae size of sea urchin (Strongylocentrotus intermedius) (mean ± SEM, n=3)1..
During the first 2 days post hatching (DPH), no significant difference was observed in the prismatic larvae survival rate (LSR) among dietary groups (P>0.05). On the 3rd DPH, the LSR was significantly decreased in all dietary groups (P<0.05). The LSR in the kelp group was significantly lower than that in the FO group (P<0.05). Among formulated feed groups, LSR was highest in the FO group, followed by PO, CO, SO, and LO groups. However, no significant difference was observed in SR among different formulated feed groups (P>0.05) (Table 6).

Table 6 Effects of different lipid sources on the prismatic larvae survival of sea urchin (Strongylocentrotus intermedius)at different day post hatching (DPH) (mean ± SEM, n=3)1.
Transcription of Proinflammatory Genes
The transcription of COX-2 was highest in the spawned ovary of sea urchins fed SO, which was significantly higher than that in the other groups (P<0.05). The transcription of AIF-1 was highest in the ovary of sea urchins fed SO, but there was no significant difference among all dietary treatments (P>0.05). The transcription of TNF-α was highest in the ovary of sea urchins fed SO, which was comparable to that in CO (P>0.05) but was significantly higher than that in kelp, FO, LO, or PO groups (P<0.05) (Figure 3).
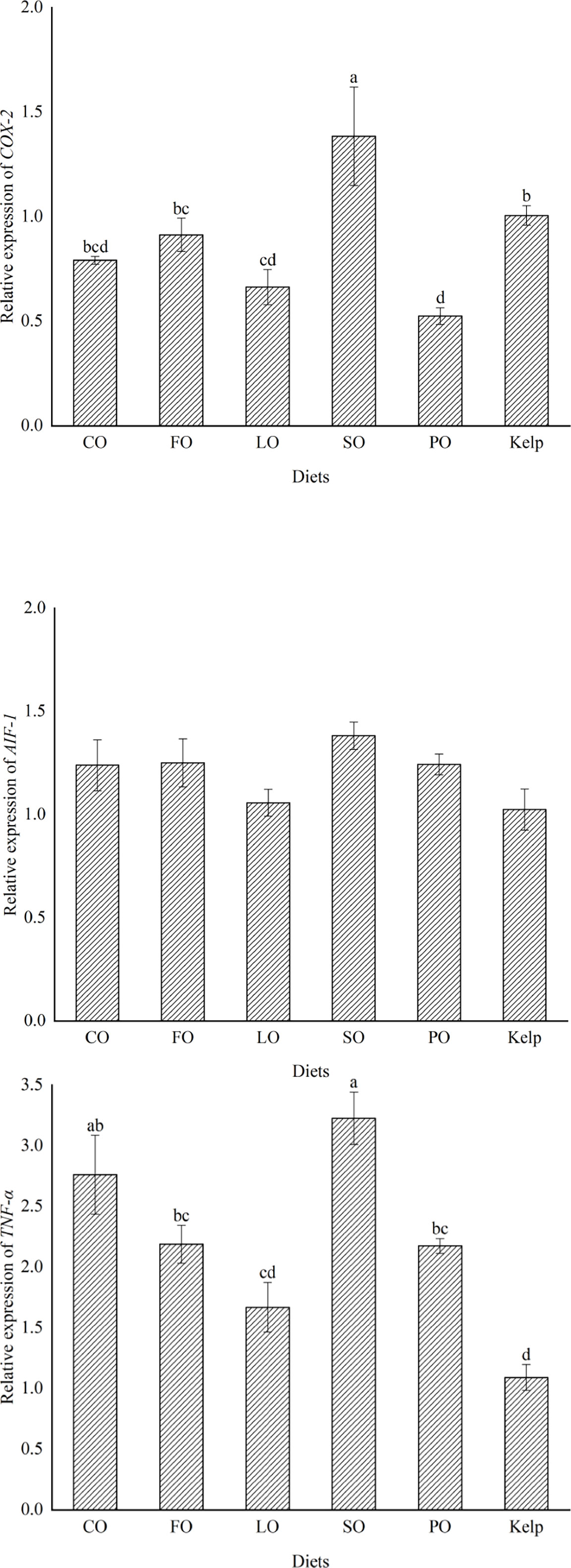
Figure 3 Effects of dietary lipid sources (colza oil (CO), fish oil (FO), linseed oil (LO), soybean oil (SO), or palm oil (PO)) on the inflammatory related gene expression in the ovary of sea urchin (Strongylocentrotus intermedius) (mean ± SEM, n=3). COX-2, cyclooxygenase-2. AIF-1, allograft inflammatory factor-1. TNF-α, tumor necrosis factor-α. Mean value bars bearing with different lowercase letters are significantly different at P < 0.05.
Transcription of Major Yolk Protein (MYP)
The transcription of MYP was highest in the digestive tract of female adult sea urchins fed FO, which was comparable to that in LO (P>0.05) but was significantly higher than that in kelp, CO, SO, or PO groups (P<0.05). The mRNA level of MYP was the highest in the ovary of the FO group, which was approximately 2-fold higher than that in the other dietary groups (P<0.05) (Figure 4).
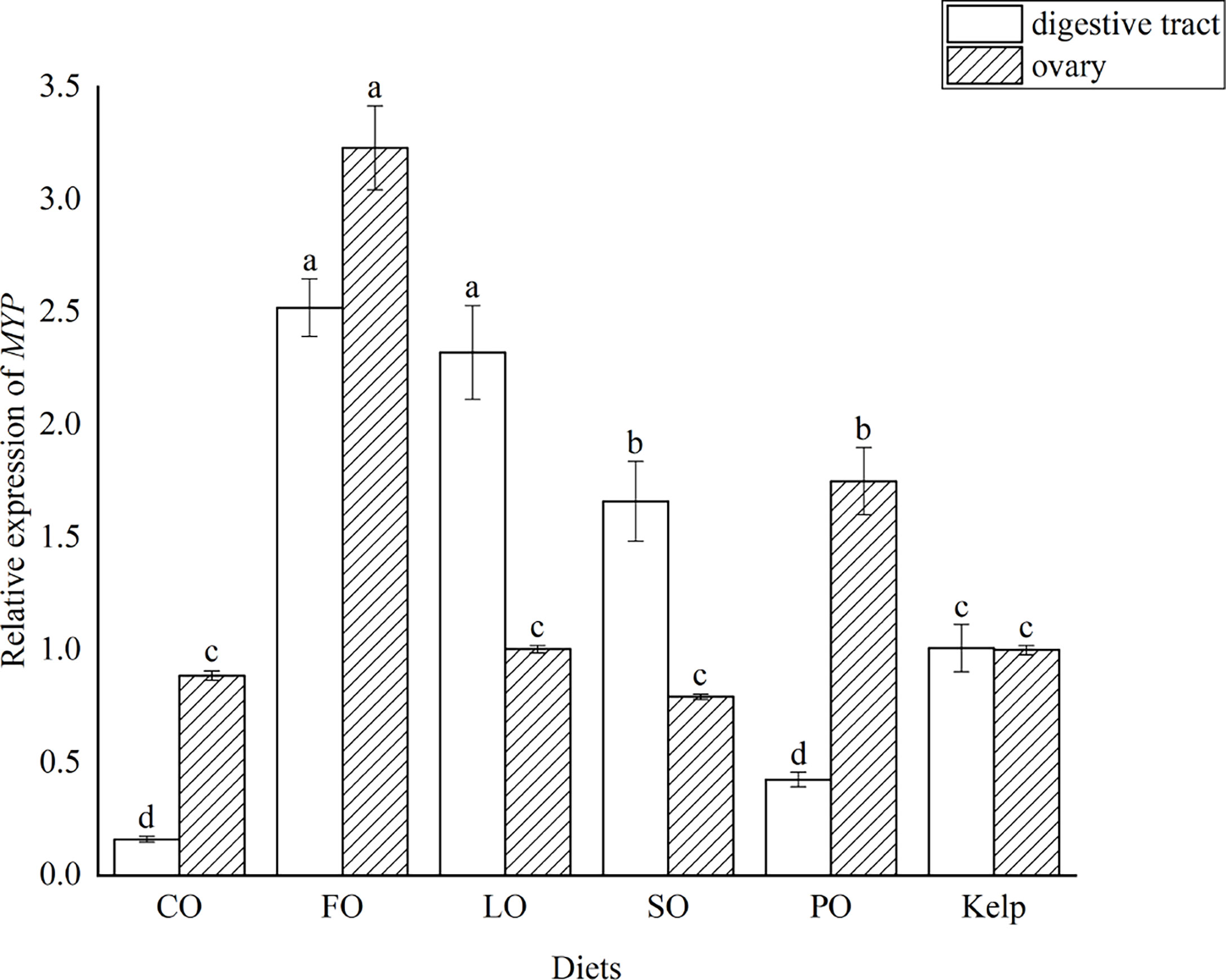
Figure 4 Effects of dietary lipid sources (colza oil (CO), fish oil (FO), linseed oil (LO), soybean oil (SO), or palm oil (PO)) on the major yolk protein (MYP) expression in sea urchin (Strongylocentrotus intermedius) (mean ± SEM, n=3). Mean value bars bearing with different lowercase letters are significantly different at P < 0.05.
Proximate Composition of Ovary
The ovary of sea urchins fed kelp showed significantly lower moisture, but higher protein, than that of sea urchins fed the formulated feeds (P<0.05). Crude lipid in the ovary of sea urchins fed kelp was significantly lower than that of formulated feed groups except for the PO group (P<0.05). Among the formulated feed groups, the moisture was highest in the ovary of sea urchins fed FO and CO, which was only significantly higher than that of sea urchins fed LO (P<0.05). However, there were no significant differences in the protein and lipid among the formulated feed groups (P>0.05) (Table 7).

Table 7 Effects of different lipid sources on the proximate composition (% wet weight) in the ovary of adult sea urchin (Strongylocentrotus intermedius) (mean ± SEM, n=3)1.
Fatty Acid Composition of the Spawned Ovary, Eggs, and Prismatic Larvae
Generally, the spawned ovary of sea urchins showed characteristic fatty acid categories of the ingested lipid sources, with the highest amounts of oleic acid (OA, C18:1n-9), EPA (C20:5n-3) and DHA (C22:6n-3), linolenic acid (LNA, C18:3n-3), linoleic acid (LA, C18:2n-6) and palmitic acid (PA, C16:0) detected in the CO, FO, LO, SO, and PO groups, respectively. Sea urchins fed kelp showed significantly higher EPA and n-3/n-6 PUFA but lower LA in the spawned ovary than those fed formulated feeds (P<0.05). The ARA in the spawned ovary of sea urchins fed CO, FO, and kelp was significantly higher than that in the other groups (P<0.05). ARA/EPA of the spawned ovary was highest in the PO group, which was comparable to that in the CO group (P>0.05) but was significantly higher than the other groups (P<0.05). The lowest ARA/EPA was observed in the spawned ovary of sea urchins fed kelp, which was comparable to that in the FO and LO groups (P>0.05) but was significantly lower than that in the other groups (P<0.05). DHA/EPA was highest in the FO group, followed by the CO group, which were significantly higher than that in the other groups (P<0.05). Sea urchins fed kelp showed the lowest DHA/EPA in the spawned ovary, which was significantly lower than that in the other groups (Table 8).
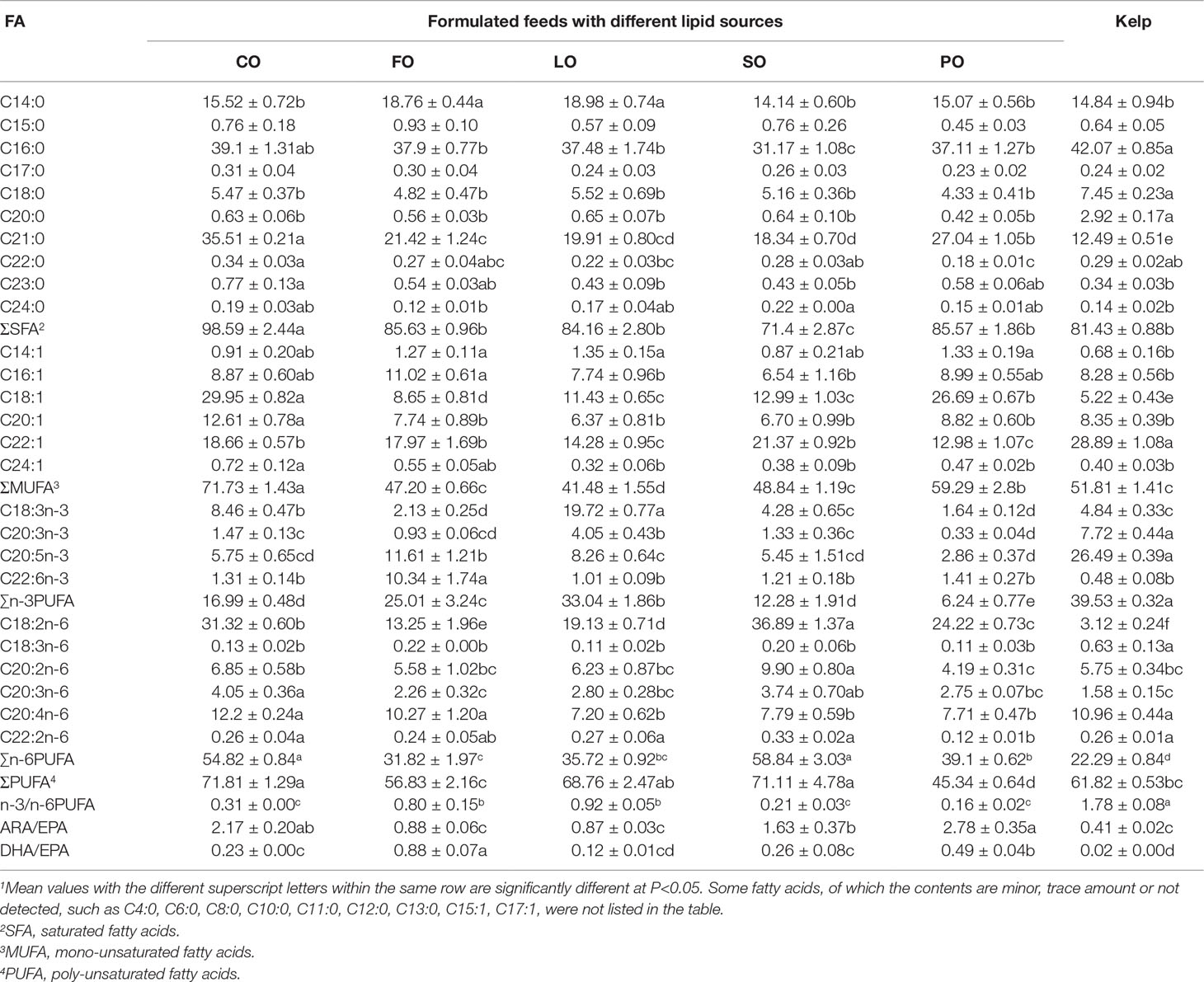
Table 8 Effects of different lipid sources on the fatty acid composition (g/kg dry matter) in the ovary of adult sea urchin (Strongylocentrotus intermedius) (mean ± SEM, n=3)1.
The EPA content in the eggs of sea urchins fed kelp was significantly higher than that in the formulated feed groups (P<0.05). The DHA and ARA contents were highest in the eggs of sea urchins fed FO, which were significantly higher than that in the other dietary groups (P<0.05). However, sea urchins fed LO and CO produced eggs with significantly (P<0.05) higher n-3 PUFA and n-6 PUFA, respectively. The n-3/n-6 PUFA in the eggs of sea urchins fed kelp was significantly higher than that in the formulated feed groups (P<0.05). The ARA/EPA and DHA/EPA showed a similar changing tendency to that of spawned ovary in all dietary groups, with significantly higher ratios observed in the PO and FO, respectively (P<0.05) (Table 9).
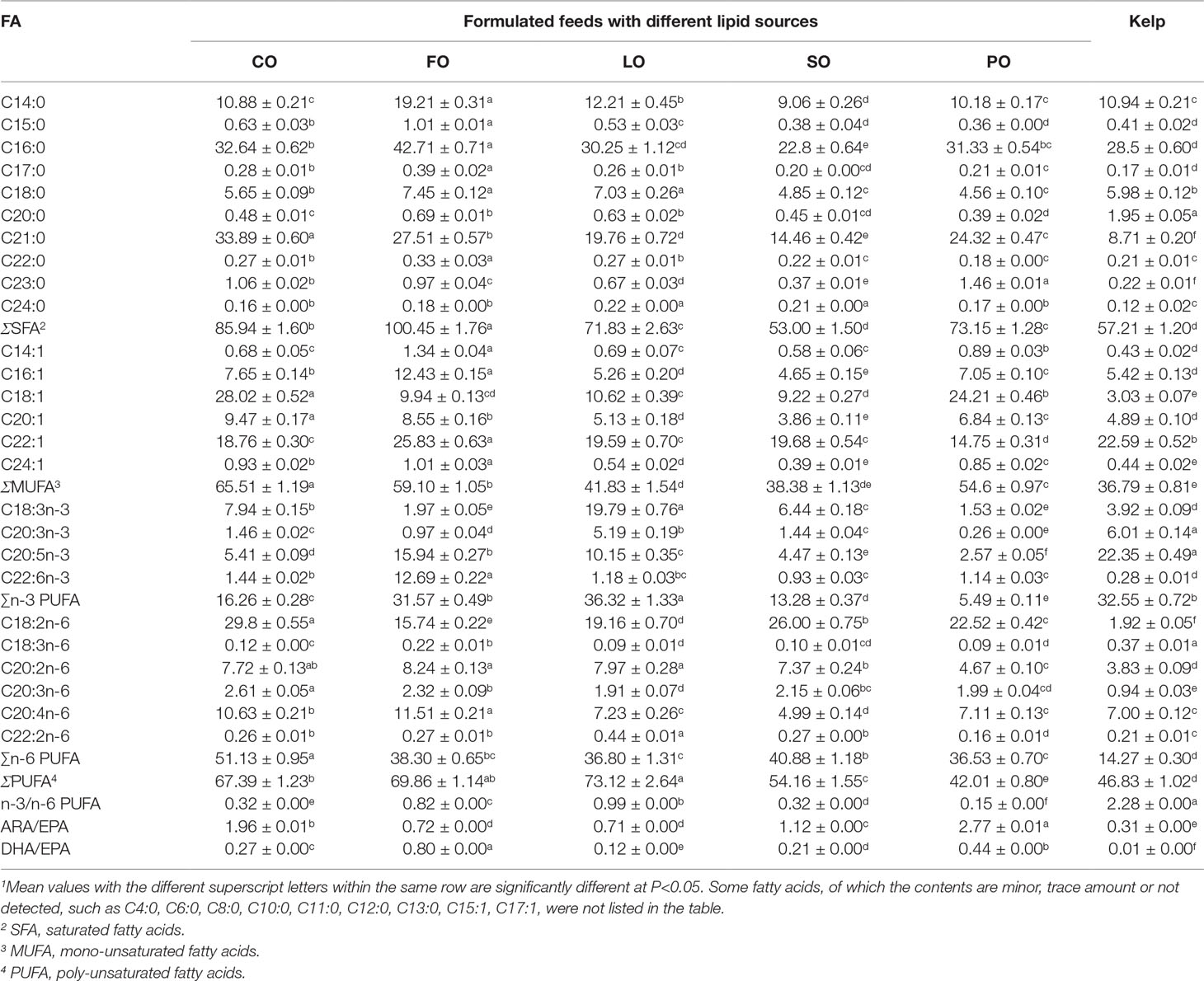
Table 9 Effects of different lipid sources on the fatty acid composition (g/kg dry matter) of eggs of adult sea urchin (Strongylocentrotus intermedius) (mean ± SEM, n=3)1.
The EPA content of the prismatic larvae was highest in the kelp group, followed by that of FO, LO, and CO groups, with the lowest EPA observed in SO and PO groups. Although no statistical analysis was made, DHA content in the prismatic larvae of the FO group was extremely higher than that in the other dietary groups. ARA of prismatic larvae showed comparable levels among dietary groups, with marginally higher value observed in PO and kelp groups. The prismatic larvae in the kelp group showed the lowest n-6 PUFA and the highest n-3 PUFA and n-3/n-6 PUFA among all dietary groups. Among the prismatic larvae of formulated feed groups, the lowest n-6 PUFA and the highest n-3 PUFA and n-3/n-6 PUFA were observed in the FO group. The highest ARA/EPA of prismatic larvae was observed in the PO group, followed by the SO and CO groups. While DHA/EPA of prismatic larvae was highest in the FO group, followed by the PO group, with the lowest ratio observed in the kelp group (Table 10).
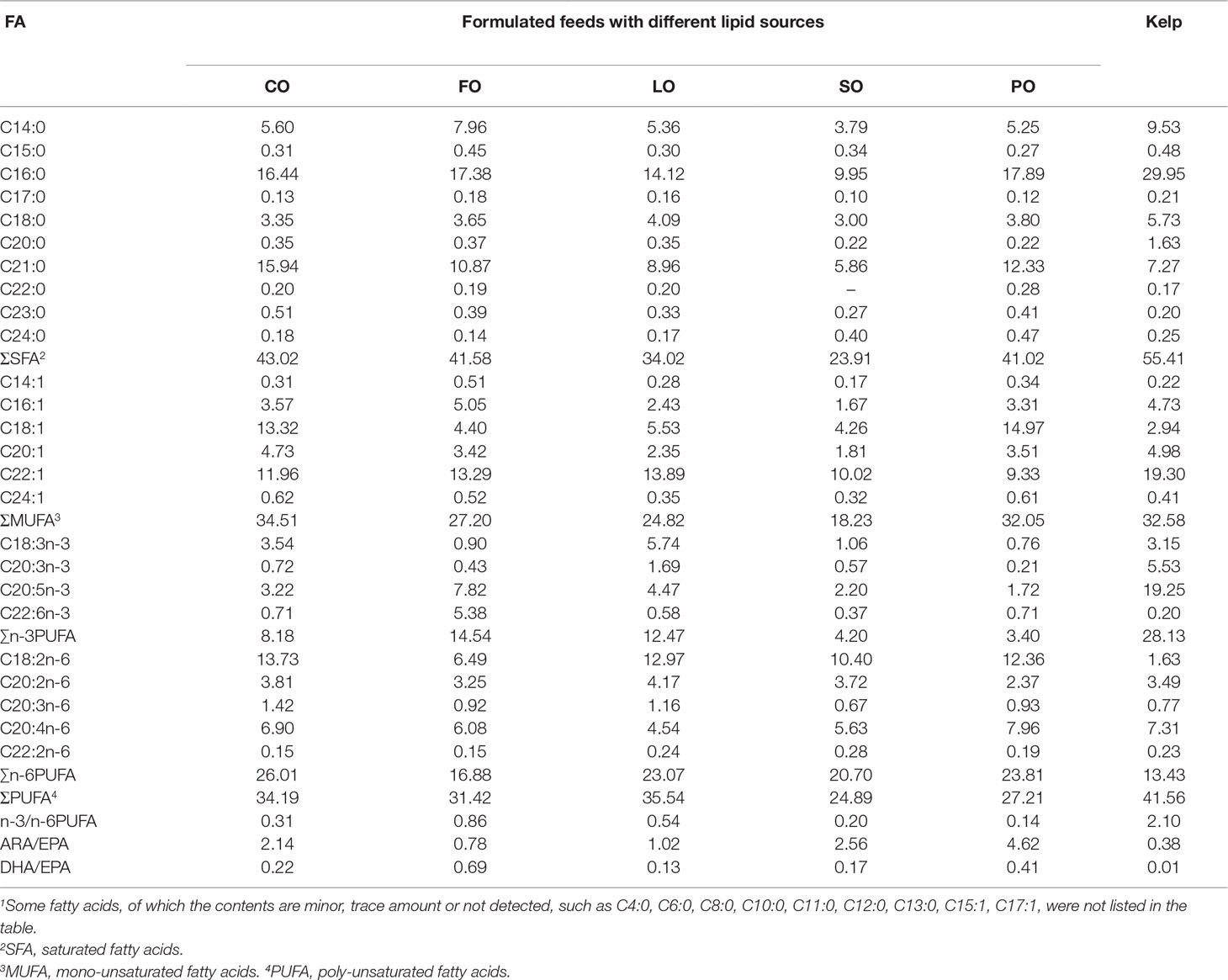
Table 10 Effects of different lipid sources on the fatty acid composition (g/kg dry matter) in the prismatic larvae of sea urchin (Strongylocentrotus intermedius) (n=1)1.
Discussion
In the present study, the WGR of female adult S. intermedius fed kelp was significantly higher than those fed formulated feeds. Similar results have been obtained in the juvenile S. intermedius (Li et al., 2020). This could be due to the presence of unknown immune and growth stimulant substances in the fresh kelp. A low molecular weight fucoidan, obtained from the kelp Laminaria japonica, played an important role in antioxidant and anticoagulant activity (Wang et al., 2010). In the present study, female sea urchins fed SO showed the worst growth performance. Similar results have been reported by Gibbs et al. (2015), who found that the WGR of juvenile Lytechinus variegatus fed SO was lower than that of sea urchins fed FO. Li et al. (2020) reported that the WGR of juvenile S. intermedius fed SO was lower than those fed LO and FO. In a recent study, we also found that the WGR of adult S. intermedius fed SO was lower than those fed PO, CO, and FO (Ning et al., 2022). This indicated that a high amount of linoleic acid (LA, 18:2n-6) had detrimental effects on the growth performance of sea urchins, just as observed in most marine carnivorous fish species (Zuo et al., 2015). The GSI of female S. intermedius fed kelp was significantly lower than those fed formulated feeds, which was consistent with the findings of a variety of previous studies (Ning et al., 2022). Although n-3 LC-PUFA is essential for normal gonad development, results of this study and some previous studies showed that sea urchins seem to have a low n-3 LC-PUFA requirement for sustaining normal gonad development (Lv et al., 2020). In this study, sea urchins fed PO showed higher GSI than those fed other lipid sources. This could be due to the abundant PA in PO, which has also been found in high quantities in the gonads of wild sea urchins (Wang et al., 2019).
Although sea urchins belong to inveterate, they are similar to fish in some physiological processes, including fatty acid metabolism, gonad development, and reproduction (Roöttinger et al., 2008; Silvia et al., 2015; Wang et al., 2021). Fecundity generally depends on the species, age, size, and nutritional conditions of spawners (Izquierdo et al., 2001; Kamler, 2005; Wanke et al., 2017). In the present study, the relative fecundity of sea urchins was not significantly affected by the experimental diets. This was consistent with the findings on rainbow trout Oncorhynchus mykiss, which showed that the relative fecundity of female individuals was not significantly affected by dietary lipid sources (Yldz et al., 2020). However, some studies have shown that dietary lipids can significantly improve the fecundity of broodstock, such as zebrafish Danio rerio (Jaya-Ram et al., 2008), yellowfin sea bream Acanthopagrus latus (Zakeri et al., 2009), Atlantic cod Gadus morhua (Rjbek et al., 2014), and three-spot gourami Trichopodus trichopterus (Berenjestanaki et al., 2014). Egg size (may be expressed as egg diameter) has been shown to be an indicator of evaluating reproductive performance in most aquatic animals (Lund et al., 2008; Wanke et al., 2017; Stuart et al., 2020), with the assumption that larger eggs represent a higher reserve of metabolic energy substrates (Brooks et al., 1997; Kamler, 2005; Nazari et al., 2009; Stuart et al., 2020). In the present study, sea urchins fed FO produced eggs with the largest size. This was consistent with the findings of Xu et al. (2017) who found that the egg diameter of tongue sole Cynoglossus semilaevis was significantly increased by feeding diets enriched with n-3 LC-PUFA. On one hand, the n-3 LC-PUFA could be used for the formation of cell membranes and substances reserved inside the eggs. On the other hand, n-3 LC-PUFA could promote the synthesis, transportation, and deposition of yolk protein in the eggs. Indeed, results of this study showed that the mRNA level of MYP in the ovaries of sea urchins fed FO was approximately 2-fold higher than that in the other groups. However, the egg size of Nile tilapia (Ng and Wang, 2011), flame angelfish Centropyge loriculus (Callan et al., 2014), and three-spot gourami (Berenjestanaki et al., 2014) was not significantly affected by dietary LC-PUFA levels. The discrepancies could be due to differences in oil droplet presence and egg size between species.
In the present study, the fertilization rate and hatching rate of sea urchins fed LO were relatively high, which could be related to the relatively higher ratio of n-3/n-6 PUFA in the eggs of sea urchins. Jaya-Ram et al. (2008) reported that egg quality and hatching rate of zebrafish were positively correlated with dietary n-3/n-6 PUFA. On the other hand, this could be due to the relatively lower inflammation in the ovary of sea urchins fed LO. Crespo et al. (2010) reported that the pro-inflammatory cytokines (e.g., TNF-α) in the ovaries of brown trout Salmo trutta broodstock negatively affected the ovarian function and egg quality. In this study, sea urchin broodstock fed SO had a poor reproductive performance. This could be due to the increased linolic acid derived inflammation, which has been found to be responsible for the infertility. It was previously found that auraptene, as a coumarin derivative with anti-inflammatory properties, was effective in improvement of oocyte maturation and fertilization rate in polycystic ovary syndrome patients (Abizadeh et al., 2020). Furthermore, the sperm quality is important for the success of fertilization. Vassallo-Agius et al. (2001) found that semen obtained from rainbow trout fish fed n-3 PUFA-sufficient showed higher sperm motility rates. Butts et al. (2015) reported that European eel fed diets with deficient n-3 fatty acids showed lower sperm motility. Therefore, the higher fertilization in the LO group of this study could be due to the better sperm quality of sea urchins. However, sperm quality parameters were not analyzed in this study and following studies are needed to clarify this point. It is well known that the steps of fertilization include the acrosome reaction, cell membrane fusion of both gametes, and genetic materials interchange (Pomin, 2015). Previous studies have shown that membrane fluidity plays a critical role in the fusion process of gametes, which is positively correlated with the content of n-3 PUFAs (Asturiano et al., 2001). Despite high n-3 LC-PUFA promoting fertilization, fertilization was lower in the FO group, which was possibly due to oxidation of seminal plasma (Jedrzejczak et al., 2005). This indicated that oxidation levels should be assayed when sea urchin broodstock are conditioned with fish oil to acquire satisfactory fertilization rates.
Larvae survival during a short period of starvation has been widely used as an index of evaluating their viability (Quintana et al., 2015). In the present study, at the 3rd day post hatching (DPH), the larvae survival was the highest in the FO treatment, which paralleled with egg size, n-3 LC-PUFA content, and MYP expression level. Tamada and Iwata (2005) reported that the survival rate of Rhinogobius larvae was positively correlated with the egg size during a period of 72 h starvation. Stuart et al. (2020) reported that the starvation-resistant capacity of California yellowtail Seriola dorsalis larvae was positively correlated with their egg size. It is commonly accepted that larger larvae can live longer under the starvation test (Miller et al., 1998; Kamler, 2005). However, body length and body width of prismatic larvae were almost the highest in the kelp group, which was inconsistent with the poorest survival performance of this group. Thus, in addition to body size, body nutritional composition was also important for the survival of larvae before mouth opening. Carboni et al. (2012) showed that the increasing levels of DHA of sea urchin Paracentrotus lividus larvae fed with Pleurochrysis carterae and Cricosphaera elongata promoted better larval performance. It was previously found that P. lividus larvae has specific dietary requirements for high level of n-3 LC-PUFA, including DHA and EPA, low DHA/EPA and high EPA/ARA (Liu et al., 2007; Carboni et al., 2012). In this study, prismatic larvae with high DHA/EPA showed a higher survival rate. Thus, DHA/EPA could be used as a sensitive indicator of evaluating the survival of early larvae which still relies on endogenous nutrition. Furthermore, survival of larvae in the CO group was the lowest among all dietary groups. This could be due to the high amount of oleic acid (OA, 18:1n-9) in the CO. Samaee (2010) reported that the increasing level of OA in eggs reduced the survival rate of early larvae of common dentex Dentex dentex. Callan et al. (2012) reported that the increasing level of oleic acid in eggs has a negative impact on the egg vitality of flame angelfish Centropyge loriculus.
In conclusion, sea urchins fed PO showed higher GSI than those fed other lipid sources. Egg diameter and fecundity of S. intermedius were largest in the FO group, which could be due to the abundant n-3 LC-PUFA deposited in their gonads. The higher inflammation level could account for lower fecundity and hatching rate of sea urchins fed SO. Sea urchins fed CO showed the highest content of oleic acid in the gonads and eggs, and the lowest fertilization rate. The highest hatching rate was observed in the kelp treatment, which was comparable to that in the LO and PO but was significantly higher than that in CO, FO, and SO. At the 3rd DPH, the survival of S. intermedius larvae was highest in the FO treatment, which was only significantly higher than those in Kelp. Thus, FO was accepted as the most ideal lipid source based on growth, reproductive performance, and early larval quality. These results could contribute to adopting an efficient feeding strategy to promoting the reproductive performance and offspring quality by choosing the optimal lipid source for S. intermedius broodstock.
Data Availability Statement
The datasets presented in this study can be found in online repositories. The names of the repository/repositories and accession number(s) can be found in the article/supplementary material.
Publisher’s Note
All claims expressed in this article are solely those of the authors and do not necessarily represent those of their affiliated organizations, or those of the publisher, the editors and the reviewers. Any product that may be evaluated in this article, or claim that may be made by its manufacturer, is not guaranteed or endorsed by the publisher.
Author Contributions
RZ and YN designed the experiment under the help of JD. YN and RZ performed the feeding experiment. YN, WD, and YH analyzed the data. YN drafted the manuscript. RZ, JD, JS, and YC revised the manuscript. All authors contributed to the article and approved the submitted version.
Funding
This research was supported by the National Key Research and Development Program of China (2018YFD0900400, 2018YFD0901603), National Natural Foundation of China (Grant no.: 41606180), and Young Elite Scientists Sponsorship of China (YESS20150157).
Conflict of Interest
The authors declare that the research was conducted in the absence of any commercial or financial relationships that could be construed as a potential conflict of interest.
References
Abizadeh M., Novin M. G., Amidi F., Ziaei S. A., Abdollahifar M. A., Nazarian H. (2020). Potential of Auraptene in Improvement of Oocyte Maturation, Fertilization Rate, and Inflammation in Polycystic Ovary Syndrome Mouse Model. Reprod. Sci. 27 (1), 1–10. doi: 10.1007/s43032-020-00168-9
Agh N., Jafari F., Jalili R., Noori F., Mozanzadeh M. T. (2019). Replacing Dietary Fish Oil With Vegetable Oil Blends in Female Rainbow Trout Broodstock Does Not Affect Breeding Quality. Lipids 54 (2-3), 149–161. doi: 10.1002/lipd.12138
AOAC (Association of Official Analytical Chemists) (1995). Official Methods of Analysis of Official Analytical Chemists International.16th Edition (Arlington, VA: Assoc. Off. Anal. Chem), 16–26.
Asturiano J. F., Sorbera L. A., Carrillo M., Zanuy S., Ramos J., Navarro J. C., et al. (2001). Reproductive Performance in Male European Sea Bass (Dicentrarchus Labrax, L.) Fed Two Pufa-Enriched Experimental Diets: A Comparison With Males Fed a Wet Diet. Aquaculture 194 (1-2), 173–190. doi: 10.1016/S0044-8486(00)00515-9
Berenjestanaki S. S., Fereidouni A. E., Ouraji H., Khalili K. J. (2014). Influence of Dietary Lipid Sources on Growth, Reproductive Performance and Fatty Acid Compositions of Muscle and Egg in Three-Spot Gourami (Trichopodus Trichopterus) (Pallas 1770). Aquac. Nutr. 20 (5), 494–504. doi: 10.1111/anu.12102
Brink-Hull M., Cyrus M. D., Macey B. M., Rhode C., Hull K. L., Roodt-Wilding R. (2022). Dietary Effects on the Reproductive Performance of the Sea Urchin Tripneustes Gratilla L: Implications for Broodstock Conditioning. Aquaculture 552, 738035. doi: 10.1016/j.aquaculture.2022.738035
Brooks S., Tyler C. R., Sumpter J. P. (1997). Egg Quality Infish: What Makes a Good Egg? Rev. Fish Biol. Fisher. 7 (4), 387–416. doi: 10.1023/A:1018400130692
Butts I., Baeza R., Støtrup J. G., Krüger-Johnsen M., Jacobsen C., Pérez L., et al. (2015). Impact of Dietary Fatty Acids on Muscle Composition, Liver Lipids, Milt Composition and Sperm Performance in European Eel. Comp. Biochem. Phys. A 183, 87–96. doi: 10.1016/j.cbpa.2015.01.015
Callan C. K., Laidley C. W., Forster I. P., Liu K. M., Kling L. J., Place A. R. (2012). Examination of Broodstock Diet Effects on Egg Production and Egg Quality in Flame Angelfish (Centropyge Loriculus). Aquac. Res. 43 (5), 696–705. doi: 10.1111/j.1365-2109.2011.02877.x
Callan C. K., Laidley C. W., Kling L. J., Breen N. E., Rhyne A. L. (2014). The Effects of Dietary Hufa Level on Flame Angelfish (Centropyge Loriculus) Spawning, Egg Quality and Early Larval Characteristics. Aquac. Res. 45 (7), 1176–1186. doi: 10.1111/are.12063
Carboni S., Vignier J., Chiantore M., Tocher D. R., Migaud H. (2012). Effects of Dietary Microalgae on Growth, Survival and Fatty Acid Composition of Sea Urchin Paracentrotus Lividus Throughout Larval Development. Aquaculture 324-325 (12), 250–258. doi: 10.1016/j.aquaculture.2011.10.037
Chang Y. Q., Zhang W. J., Zhao C., Song J. (2012). Estimates of Heritabilities and Genetic Correlations for Growth and Gonad Traits in the Sea Urchin Strongylocentrotus Intermedius. Aquac. Res. 43 (2), 271–280. doi: 10.1111/j.1365-2109.2011.02825.x
Crespo D., Bonnet E., Roher N., Mackenzie S. A., Krasnov A., Goetz F. W., et al. (2010). Cellular and Molecular Evidence for a Role of Tumor Necrosis Factor Alpha in the Ovulatory Mechanism of Trout. Reprod. Biol. Endocrin. 8 (1), 34–34. doi: 10.1186/1477-7827-8-34
Cuesta-Gomez D., Lazo J. P., Sánchez-Saavedra M. (2020). Effects of Dietary Fish Oil and Soya Bean Lecithin on Gonad Index, Colour and Biochemical Composition of the Purple Sea Urchin, Strongylocentrotus Purpuratus (Stimpson 1857). Aquac. Res. 51 (6), 3384–3402. doi: 10.1111/are.14674
Gibbs V. K., Heflin L. E., Jones W. T., Powell M. L., Lawrence A. L., Makowsky R., et al. (2015). Optimizing Dietary Levels of Menhaden and Soybean Oils and Soybean Lecithin for Pre-Gonadal Somatic Growth in Juveniles of the Sea Urchin Lytechinus Variegatus. Aquaculture 446 (1), 198–205. doi: 10.1016/j.aquaculture.2015.05.013
Heflin L. E., Gibbs V. K., Powell M. L., Makowsky R., Lawrence J. M., Lawrence A. L., et al. (2012). Effect of Dietary Protein and Carbohydrate Levels on Weight Gain and Gonad Production in the Sea Urchin (Lytechinus Variegatus). Aquaculture 358-359 (2), 253–261. doi: 10.1016/j.aquaculture.2012.06.009
Izquierdo M. S., Fernández-Palacios H., Tacon A. (2001). Effect of Broodstock Nutrition on Reproductive Performance of Fish. Aquaculture 197 (1-4), 25–42. doi: 10.1016/S0044-8486(01)00581-6
Jaya-Ram A., Kuah M. K., Lim P. S., Kolkovski S., Shu-Chien A. C. (2008). Influence of Dietary HUFA Levels on Reproductive Performance, Tissue Fatty Acid Profile and Desaturase and Elongase mRNAs Expression in Female Zebrafish (Danio Rerio). Aquaculture 277 (3-4), 275–281. doi: 10.1016/j.aquaculture.2008.02.027
Jedrzejczak P., Fraczek M., Szumaa-kakol A., Taszarek-hauke G., Pawelczyk L., Kurpisz M. (2005). Consequences of Semen Inflammation and Lipid Peroxidation on Fertilization Capacity of Spermatozoa in In Vitro Conditions. Int. J. Androl. 28 (5), 275–283. doi: 10.1111/j.1365-2605.2005.00547.x
Kamler E. (2005). Parent-Egg-Progeny Relationships in Teleost Fishes: An Energetics Perspective. Rev. Fish Biol. Fisher. 15 (14), 399–421. doi: 10.1007/s11160-006-0002-y
Kenneth J. L., Thomas D. S. (2002). Analysis of Relative Gene Expression Data Using Real-Time Quantitative PCR and the 2-ΔΔCT Method. Methods 25, 402–408. doi:10.1006/meth.2001
Kottmann J. S., Tomkiewicz J., Butts I. A. E., Lund I., Jacobsen C., Josianne G., et al. (2020). Effects of Essential Fatty Acids and Feeding Regimes on Egg and Offspring Quality of European Eel: Comparing Reproductive Success of Farm-Raised and Wild-Caught Broodstock. Aquaculture 529, 1–16. doi: 10.1016/j.aquaculture.2020.735581
Liang M. Q., Lu Q. C., Qian C., Zheng K. K., Wang X. X. (2014). Effects of Dietary N-3 to N-6 Fatty Acid Ratios on Spawning Performance and Larval Quality in Tongue Sole Cynoglossus Semilaevis. Aquac. Nutr. 20 (1), 79–89. doi: 10.1111/anu.12054
Liu X. L., Chang Y. Q., Xiang J. H., Cao X. B. (2005). Estimates of Genetic Parameters for Growth Traits of the Sea Urchin, Strongylocentrotus Intermedius. Aquaculture 243 (1-4), 27–32. doi: 10.1016/j.aquaculture.2004.10.014
Liu H., Kelly M. S., Cook E. J., Black K., Orr H., Zhu J. X., et al. (2007). The Effect of Diet Type on Growth and Fatty-Acid Composition of Sea Urchin Larvae, I. Paracentrotus Lividus (Lamarck 1816) (Echinodermata). Aquaculture 264 (1), 247–262. doi: 10.1016/j.aquaculture.2006.12.021
Li L., Wang W., Yusuf A., Zhu Y., Zhou Y., Ji P., et al. (2020). Effects of Dietary Lipid Levels on the Growth, Fatty Acid Profile and Fecundity in the Oriental River Prawn, Macrobrachium Nipponense. Aquac Res. 51 (2), 1–10. doi: 10.1111/are.14539
Li M., Zhang F., Ding J., Zuo R., Chang Y. (2021). Effects of Lipid Sources on the Growth Performance, Gonad Development, Fatty Acid Profile and Transcription of Related Genes in Juvenile Sea Urchin (Strongylocentrotus Intermedius). Aquac. Nutr. 27 (1), 28–38. doi: 10.1111/anu.13162
Loureno S., Valente L., Andrade C. (2019). Meta-Analysis on Nutrition Studies Modulating Sea Urchin Roe Growth, Colour and Taste. Rev. Aquacult. 11 (3), 776–781. doi: 10.1111/raq.12256
Lund I., Steenfeldt S. J., Suhr K. I., Hansen B. W. (2008). A Comparison of Fatty Acid Composition and Quality Aspects of Eggs and Larvae From Cultured and Wild Broodstock of Common Sole (Solea soleaL.). Aquac. Nutr. 14 (6), 544–555. doi: 10.1111/j.1365-2095.2007.00560.x
Lv D., Zhang F., Ding J., Chang Y., Zuo R. (2020). Effects of Dietary N-3 LC-PUFA on the Growth Performance, Gonad Development, Fatty Acid Profile, Transcription of Related Genes and Intestinal Microflora in Adult Sea Urchin (Strongylocentrotus Intermedius). Aquac. Res. 52 (5), 1431–1441. doi: 10.1111/are.14997
Mazorra C., Bruce M., Bell J. G., Davie A., Alorend E., Jordan N., et al. (2003). Dietary Lipid Enhancement of Broodstock Reproductive Performance and Egg and Larval Quality in Atlantic Halibut (Hippoglossus Hippoglossus). Aquaculture 227 (1), 21–33. doi: 10.1016/S0044-8486(03)00493-9
Mobaraki M., Kenari A. A., Gorji S. B., Esmaeili M. (2020). Effect of Dietary Fish and Vegetable Oil on the Growth Performance, Body Composition, Fatty Acids Profile, Reproductive Performance and Larval Resistance in Pearl Gourami (Trichogaster Leeri). Aquac. Nutr. 26 (3), 1–14. doi: 10.1111/anu.13048
Nazari R. M., Sohrabnejad M., Ghomi M. R., Modanloo M., Ovissipour M., Kalantarian H. (2009). Correlations Between Egg Size and Dependent Variables Related to Larval Stage in Persian Sturgeon Acipenser Persicus. Mar. Freshw. Behav. Phy. 42 (2), 147–155. doi: 10.1080/10236240902846796
Ng W. K., Wang Y. (2011). Inclusion of Crude Palm Oil in the Broodstock Diets of Female Nile Tilapia, Oreochromis Niloticus, Resulted in Enhanced Reproductive Performance Compared to Broodfish Fed Diets With Added Fish Oil or Linseed Oil. Aquaculture 314 (1-4), 122–131. doi: 10.1016/j.aquaculture.2011.01.034
Nhan H. T., Nhu T. Q., Duc P. M., Liew H. J., Ako H., Jha R. (2020). Effects of Dietary Arachidonic Acid on Final Maturation, Spawning and Composition of Gonad of Black Sea Urchin (Diadema Setosum) (Leske 1778). Aquac. Nutr. 26 (2), 1771–1779. doi: 10.1111/anu.13127
Ning Y. C., Zhang F., Tang L., Song J., Ding J., Chang Y. Q., et al. (2022). Effects of Dietary Lipid Sources on the Growth, Gonad Development, Nutritional and Organoleptic Quality, Transcription of Fatty Acid Synthesis Related Genes and Antioxidant Capacity During Cold Storage in Adult Sea Urchin (Strongylocentrotus Intermedius). Aquaculture 548, 1–14. doi: 10.1016/j.aquaculture.2021.737688
Onomu A. J., Vine N. G., Cyrus M., Macey B. M., Bolton J. J. (2020). The Effect of Fresh Seaweed and a Formulated Diet Supplemented With Seaweed on the Growth and Gonad Quality of the Collector Sea Urchin, Tripneustes Gratilla, Under Farm Conditions. Aquac. Res. 51 (2), 1–16. doi: 10.1111/are.14752
Phillips K., Hamida N., Silcocka P., Sewellb M. A., Barkerc M., Weaverc A., et al. (2010). Effect of Manufactured Diets on the Yield, Biochemical Composition and Sensory Quality of Evechinus Chloroticus Sea Urchin Gonads. Aquaculture 308 (1-2), 49–59. doi: 10.1016/j.aquaculture.2010.07.030
Pomin V. H. (2015). Sulfated Glycans in Sea Urchin Fertilization. Glycoconjugate J. 32 (1-2), 9–15. doi: 10.1007/s10719-015-9573-y
Prato E., Fanelli G., Alberto A., Biandolino F., Piero A. (2018). Influence of a Prepared Diet and a Macroalga (Ulva Sp.) on the Growth, Nutritional and Sensory Qualities of Gonads of the Sea Urchin Paracentrotus Lividus. Aquaculture 493, 240–250. doi: 10.1016/j.aquaculture.2018.05.010
Quintana D., Márquez L., Arévalo J., Lorenzo A., Almansa E. (2015). Relationships Between Spawn Quality and Biochemical Composition of Eggs and Hatchlings of Octopus Vulgaris Under Different Parental Diets. Aquaculture 446 (1), 206–216. doi: 10.1016/j.aquaculture.2015.04.023
Rjbek M. C., Stttrup J. G., Jacobsen C., Tomkiewicz J., Nielsen A., Trippel E. A. (2014). Effects of Dietary Fatty Acids on the Production and Quality of Eggs and Larvae of Atlantic Cod (Gadus Morhua L.). Aquac. Nutr. 20 (6), 654–666. doi: 10.1111/anu.12124
Röttinger E., Saudemont A., Duboc V., Besnardeau L., McClay D., Lepage T. (2008). FGF Signals Guide Migration of Mesenchymal Cells, Control Skeletal Morphogenesis and Regulate Gastrulation During Sea Urchin Development. Development. 135 (2), 353–365. doi: 10.1242/dev.014282
Samaee S. M. (2010). “Quantitative Composition of Egg Protein, Lipid,Fatty Acid, and Free Amino Acid in Common Dentex (Dentex Dentex L.) and Their Relations to Viability and Larval Development,” in Ph.D Dissertation (Austria: Department of Organismic Biology, University of Salzburg), 205pp.
Silvia M., Paolo T., Nobile M., Denise F., Cinta P., Michela S. (2015). Unraveling Estradiol Metabolism and Involvement in the Reproductive Cycle of non-Vertebrate Animals: The Sea Urchin Model. Steroids. 104, 25–36. doi: 10.1016/j.steroids.2015.08.008
Stuart K. R., Armbruster L., Johnson R., Drawbridge M. A. (2020). Egg Diameter as a Predictor for Egg Quality of California Yellowtail (Seriola Dorsalis). Aquaculture 522 (8), 1–6. doi: 10.1016/j.aquaculture.2020.735154
Sui L. Y., Wu X. G., Wille M., Cheng Y. X., Sorgeloos P. (2009). Effect of Dietary Soybean Lecithin on Reproductive Performance of Chinese Mitten Crab Eriocheir Sinensis (H. Milne-Edwards) Broodstock. Aquacult. Int. 17 (1), 45–56. doi: 10.1007/s10499-008-9178-6
Tamada K., Iwata K. (2005). Intra-Specific Variations of Egg Size, Clutch Size and Larval Survival Related to Maternal Size in Amphidromous Rhinogobius Goby. Environ. Biol. Fish. 73 (4), 379–389. doi: 10.1007/s10641-005-2230-8
Tercero J. F., Rosas C., Mascaro M., Poot G., Gallardo P. (2015). Effects of Parental Diets Supplemented With Different Lipid Sources on Octopus Maya Embryo and Hatching Quality. Aquaculture 448, 234–242. doi: 10.1016/j.aquaculture.2015.05.023
Tocher D. R. (2015). Omega-3 Long-Chain Polyunsaturated Fatty Acids and Aquaculture in Perspective. Aquaculture 449, 94–107. doi: 10.1016/j.aquaculture.2015.01.010
Turchini G. M., Francis D. S. (2009). Fatty Acid Metabolism (Desaturation, Elongation and β-Oxidation) in Rainbow Trout Fed Fish Oil- or Linseed Oil-Based Diets. Br. J. Nutr. 102 (1), 69–81. doi: 10.1017/S0007114508137874
Turchini G. M., Mentasti T., Caprino F., Panseri S., Moretti V. M., Valfr F. (2010). Effects of Dietary Lipid Sources on Flavour Volatile Compounds of Brown Trout (Salmo Trutta L.) Fillet. J. Appl. Ichthyol. 20 (1), 71–75. doi: 10.1046/j.0175-8659.2003.00522.x
Vassallo-Agius R., Watanabe T., Yoshizaki G., Satoh S., Takeuchi Y. (2001). Quality of Eggs and Spermatozoa of Rainbow Trout Fed an N-3 Essential Fatty Acid-Deficient Diet and its Effects on the Lipid and Fatty Acid Components of Eggs, Semen and Livers. Fisheries Sci. 67 (5), 818–827. doi: 10.1046/j.1444-2906.2001.00328.x
Wang H., Ding J., Ding S., Chang Y. (2019). Metabolomic Changes and Polyunsaturated Fatty Acid Biosynthesis During Gonadal Growth and Development in the Sea Urchin Strongylocentrotus Intermedius. Comp. Biochem. Phys. D 32, 1–15. doi: 10.1016/j.cbd.2019.100611
Wang X. X., Li Y. J., Hou C. L., Gao Y., Wang Y. Z. (2012). Influence of Different Dietary Lipid Sources on the Growth, Tissue Fatty Acid Composition, Histological Changes and Peroxisome Proliferator-Activated Receptor γ Gene Expression in Large Yellow Croaker (Pseudosciaena Crocea R.). Aquac. Res. 43 (2), 281–291. doi: 10.1111/j.1365-2109.2011.02826.x
Wang H., Zhao W., Ding B., Zhang Y., Huang X., Liu X., et al. (2021). Comparative Lipidomics Profiling of the Sea Urchin, Strongylocentrotus Intermedius. Comp. Biochem. Phys. D. 40, 100900. doi: 10.1016/j.cbd.2021.100900
Wanke T., Brämick U., Mehner T. (2017). High Stock Density Impairs Growth, Female Condition and Fecundity, But Not Quality of Early Reproductive Stages in Vendace (Coregonus Albula). Fish. Res. 186 (1), 159–167. doi: 10.1016/j.fishres.2016.08.028
Xiao B. W., Li Q. C., Zhong L. Z., Ai C. X., Deng G. Y. (2002). Reproduction Response of Chinese Mitten-Handed Crab (Eriocheir Sinensis) Fed Different Sources of Dietary Lipid. Comp. Biochem. Physiol. A Mol. Integr. Physiol. 131 (3), 675–681. doi: 10.1016/S1095-6433(01)00515-3
Xin Z., Zhou D. Y., Lu T., Liu Z. Y., Zhao Q., Liu Y. X., et al. (2018). Characterization of Lipids in Three Species of Sea Urchin. Food Chem. 241 (2), 97–103. doi: 10.1016/j.foodchem.2017.08.076
Xu H. G., Zhao M., Zheng K. K., Wei Y. L., Yan L., Liang M. Q. (2017). Antarctic Krill (Euphausia Superba) Meal in the Diets Improved the Reproductive Performance of Tongue Sole (Cynoglossus Semilaevis) Broodstock. Aquac. Nutr. 23 (6), 1287–1295. doi: 10.1111/anu.12503
Yldz M., Oforic-Mensah S., Arslan M., Yamaner G., Ekici A., Baltaci M. A., et al. (2021). Effects of Different Dietary Lipid Resources on Sperm Quality and Reproductive Success in Rainbow Trout (Oncorhynchus Mykiss). Aquac. Res. 52 (8), 1–11. doi: 10.1111/are.15226
Yldz M., Ofori-Mensah S., Arslan M., Ekici A., Korkmaz F. (2020). Effects of Different Dietary Oils on Egg Quality and Reproductive Performance in Rainbow Trout Oncorhynchus Mykiss. Anim. Reprod. Sci. 221 (2), 1–11. doi: 10.1016/j.anireprosci.2020.106545
Yuan Y., Wang X., Jin M., Jiao L., Zhou Q. (2019). Modification of Nutritional Values and. Flavor Qualities of Muscle of Swimming Crab (Portunus Trituberculatus): Application of a Dietary Lipid Nutrition Strategy. Food Chem. 308, 125607. doi: 10.1016/j.foodchem.2019.125607
Zakeri M., Marammazi J. G., Kochanian P., Savari A., Yavari V., Haghi M. (2009). Effects of Protein and Lipid Concentrations in Broodstock Diets on Growth, Spawning Performance and Egg Quality of Yellowfin Sea Bream (Acanthopagrus Latus). Aquaculture 295 (1-2), 99–105. doi: 10.1016/j.aquaculture.2009.06.026
Zhou H. S., Chang Y. Q., Luo S. B., Tan X. F., Zhang W. J., Zhao C. (2013). Effects of Three Feeding Models on Growth, Gonad Yield and Gonad Quality of the Sea Urchin (Strongylocentrotus Intermedius). J. Fish. China 37 (7), 1050–1057. doi: 10.3724/SP.J.1231.2013.38485
Zhou Q. C., Liu C. W., Chi S. Y., Yang Q. H. (2007). Effects of Dietary Lipid Sources on Growth and Fatty Acid Composition of Juvenile Shrimp, Litopenaeus Vannamei. Aquac. Nutr. 13 (3), 222–229. doi: 10.1111/j.1365-2095.2007.00470.x
Zuo R., Hou S., Wu F., Song J., Zhang W., Zhao C., et al. (2017). Higher Dietary Protein Increases Growth Performance, Anti-Oxidative Enzymes Activity and Expression of Heat Shock Protein 70 in the Juvenile Sea Urchin (Strongylocentrotus Intermedius) Under a Heat Stress. Aquac. Fish. 2 (1), 18–23. doi: 10.1016/j.aaf.2017.01.005
Zuo R., Li M., Ding J., Chang Y. (2018). Higher Dietary Arachidonic Acid Levels Improved the Growth Performance, Gonad Development, Nutritional Value, and Antioxidant Enzyme Activities of Adult Sea Urchin (Strongylocentrotus Intermedius). J. Ocean Univ. China 17 (4), 932–940. doi: 10.1007/s11802-018-3606-7
Keywords: lipid sources, Strongylocentrotus intermedius, growth, reproductive performance, offspring quality
Citation: Zuo R, Ning Y, Di W, Heqiu Y, Song J, Ding J and Chang Y (2022) Effects of Dietary Lipid Sources on the Growth, Gonad Development, Fatty Acid Composition and Spawning Performance of Broodstock, and Early Larvae Quality of Sea Urchin (Strongylocentrotus intermedius). Front. Mar. Sci. 9:927116. doi: 10.3389/fmars.2022.927116
Received: 23 April 2022; Accepted: 14 June 2022;
Published: 22 July 2022.
Edited by:
Luisa MP Valente, ICBAS - Universidade do Porto, PortugalReviewed by:
Kruno Bonačić, University of Dubrovnik, CroatiaM. Virginia Martín, Centro Oceanográfico de Canarias, Instituto Español de Oceanografía (IEO), Spain
Copyright © 2022 Zuo, Ning, Di, Heqiu, Song, Ding and Chang. This is an open-access article distributed under the terms of the Creative Commons Attribution License (CC BY). The use, distribution or reproduction in other forums is permitted, provided the original author(s) and the copyright owner(s) are credited and that the original publication in this journal is cited, in accordance with accepted academic practice. No use, distribution or reproduction is permitted which does not comply with these terms.
*Correspondence: Rantao Zuo, rtzuo@dlou.edu.cn, zuorantao@163.com; Jun Ding, dingjun1119@dlou.edu.cn