- 1South China Sea Fisheries Research Institute, Chinese Academy of Fishery Sciences, Guangzhou, China
- 2Key Laboratory of Marine Ranching, Ministry of Agriculture and Rural Affairs, Guangzhou, China
- 3National Digital Fisheries (Marine Ranching) Innovation Sub-Center, Guangzhou, China
- 4Scientific Observing and Experimental Station of South China Sea Fishery Resources and Environments, Ministry of Agriculture and Rural Affairs, Guangzhou, China
Marine habitat degradation resulting from human activities and environmental pollution has led to serious deterioration of marine fishery resources. To address this issue, countries worldwide are exploring sustainable fishery approaches. Installation of artificial reefs (AR) is rapidly increasing. AR have been widely constructed globally to conserve fishery resources and improve marine habitats. As an important biological group of marine fishery resources, nekton are widely evaluated to determine the effects of ecological restoration and resource conservation. In the current study, we compared the dynamic properties of taxonomic diversity and the eco-exergy of nekton community from 2 to 14 years after AR construction in Dalajia, China. The results indicated that the species number and density of nekton significantly increased after AR construction. Siganus oramin became an absolute dominant fish species in the reef area and its surroundings. The species diversity and evenness of the nekton community decreased, whereas species richness increased. The abundance, biomass, and eco-exergy of the nekton community increased over time because of the dominance of fishes. Our results highlight that AR can increase and conserve fishery resources, improve the structure of the nekton community, and increase ecosystem stability. But, at the same time, the dominance of S. oramin can interfere in the reef community. The explosive growth was quantitatively and qualitatively higher than that of other species, the diversity and evenness indices showed a decreasing trend, although the number of nekton species was significantly higher than that in the background survey and CA habitat during the same period.
1. Introduction
Fishes are the main source of global protein. Marine fisheries offer livelihood support to 8% of the world’s population and are important components of modern agriculture and economy in China (Tong and Xia, 2017; FAO, 2018; Zhang et al., 2019). Because of increasingly complex natural factors and human activities, marine degradation is accelerating at an exponential rate (McCauley et al., 2015; Wang et al., 2019), which has led to the depletion of offshore fishery resources, degradation of marine habitats, and severe trend of ecological desertification. To address these issues, coastal countries are considering approaches for the sustainable management of fisheries. Artificial reefs (AR) are gaining prominence for conserving fishery resources in many coastal countries because of their excellent ecological proliferation effects (Rilov and Benayahu, 1998; Pickering et al., 1999; Fukunaga and Bailey-Brock, 2008; Yu et al., 2015).
AR are human-made or auxiliary structures that are built on the seabed to mimic natural reefs and aimed at protecting and improving the ecological environment to conserve and increase aquatic biological resources (Baine, 2001; Perkol-Finkel et al., 2006; Schroeter et al., 2015). The use of artificial reefs can be traced back to 1795, when Japanese fishermen composed reefs of wooden frames and sandbags and placed them in the sea to increase fish aggregation. This practice was inspired by the aggregation of fish around sunken ships (Chen PM et al., 2019). Japan and the United States were the first countries to recognize the importance of using AR to protect, develop, and utilize fishery resources (Liu, 2003). In 1932, Japan formulated the “coastal fishery revitalization policy”; since September 1945, AR have been installed in its coastal areas annually (Chen et al., 2006). In 1968, the U.S. government proposed building a marine ranch and conducted technical research on AR (Wang and Huang, 2019). At the end of the 1960s, implementation of the 370 km exclusive economic zone allowed pelagic fishery powers to return to and operate in their coastal waters within 200 nautical miles of this zone. Since the 1930s, the use of AR has increased worldwide, particularly in Japan, the United States, China, and Europe (Santos et al., 2011). AR significantly influence the spatial distribution of fishery resources in the sea, which is mainly reflected in the proliferation of fishery resources. Compared to other sea areas, the reef area has a higher density, abundance, and diversity of fishery resources (Steimle and Meier, 1997; Feng et al., 2021). Because the spatial structure of AR provides abundant food sources and suitable shelter for fishery organisms, the spawning, feeding, and predator avoidance behaviors of fish increase and other fishery organisms gradually migrate to the reef area (Liu et al., 2018). Based on differences in the relative abundance of dominant species, the composition of fish assemblages in reef areas and natural sea areas may vary (Folpp et al., 2013; Lowry et al., 2014; Mills et al., 2017). However, the community structure of fishery resources in the AR area and that in surrounding natural reefs differ even after long periods (Perkol-Finkel et al., 2006).
Fishery resources are important components of fishery ecosystem (Su et al., 2006), and changes in fishery resources can reflect the dynamic changes in environmental quality and the fishery ecosystem. The community structure of fishery resources is used to assess ecological restoration or succession in the sea area (Avigdor and Yehiam, 2002; Couto et al., 2010; Wang WD et al., 2010; Jiao et al., 2011; Marchi et al., 2011; Yuan et al., 2017; Wang et al., 2018). Many ecological indicators have been used to evaluate biological community dynamics during restoration or succession. Assessment of the community composition, dominant species, and biodiversity is helpful for determining the impact of human activities on ecosystems; however, these factors can be more comprehensively evaluated by measuring eco-exergy (Chen Q et al., 2019), which is a thermodynamically oriented indicator of the extent to which ecosystems deviate from thermodynamic equilibrium (Jørgensen et al., 2005; Li et al., 2012). Eco-exergy can be used as an index from the perspective of energy ecology to evaluate changes in fishery resources in the sea area after habitat restoration. The eco-exergy value is a thermodynamic index that originated from ecosystem theory. Quantitative and qualitative changes in the ecosystems can be obtained by applying this index. Eco-exergy based on biological complexity information can intuitively reflect the health status of animal communities in the target sea area. Furthermore, eco-exergy determination is more concise, systematic, and comprehensive than biomass and abundance analysis. Therefore, in this study, eco-exergy theory was used to evaluate the structure, function, and composition of the ecosystem after AR construction.
In the present study, based on the fishery resources data surveyed for 14 years (2002–2016) after AR construction, we assessed the effect of AR construction on the community composition, dominant species, taxonomic diversity, and eco-exergy of the nekton community in Dalajia, Guangdong, China. We compare the dynamics of these indicators to answer the following questions: (1) How did the community composition and dominant species of fishery resources change during the 14 years after AR construction? (2) How did the biomass and abundance of fishery resources and taxonomic diversity change during the 14 years after AR construction? (3) How did the eco-exergy of the nekton community change during the 14 years after AR construction? We examined the proliferation of fishery resource in the AR area over a relatively long duration and tracked, observed, and analyzed the sustainable impact of the AR on fishery resource conservation.
2. Materials and methods
2.1. Study area
The study was conducted in the south of Dalajia island, which is in the middle of Daya Bay (22° 33.30’–22° 34.10’ N, 114° 37.90’–114° 39.50’ E; Figure 1). The water depth ranges from 10 to 16 m, mean annual air temperature is 22.0°C, and mean seawater surface temperature is 28.0°C. The seabed surface sediments are fine silty sand–silty sand. Daya Bay has a rich natural ecological environment that includes coral reefs, mangroves, rock reefs, beaches, and mudflats (Wang et al., 2008).
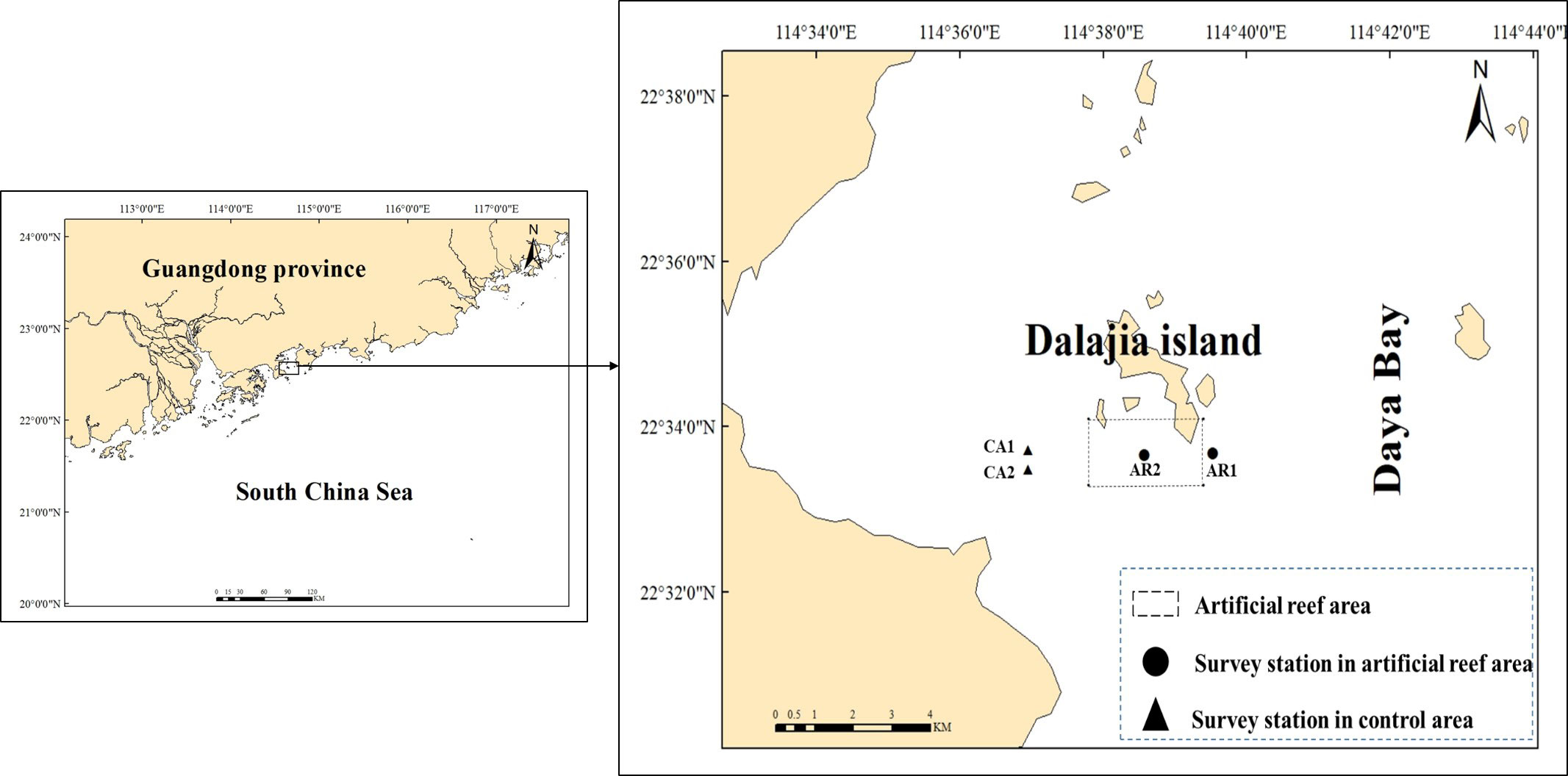
Figure 1 Locations of the two habitats studied in Dalajia, Daya Bay, China. AR, artificial reef habitat; CA, area near AR habitat without artificial reefs.
The northern coastal area of the South China Sea was originally a high-quality fishing ground (Wang et al., 2015). However, since the 1980s, the fishery ecological environment in the coastal area of the South China Sea has deteriorated, with decreased fishery resources, reversion to a younger state, and reduced quality (Chen et al., 2012; Zou, 2021). To restore these resources, 1829 reinforced concrete AR (3 × 3 × 5 m) were installed in the AR area of Dalajia on December 30, 2002 and June 23, 2004 (Figure 2). The volume and area of these concrete reefs were 60,178 m3 and 5.375 km2, respectively (Chen et al., 2007).
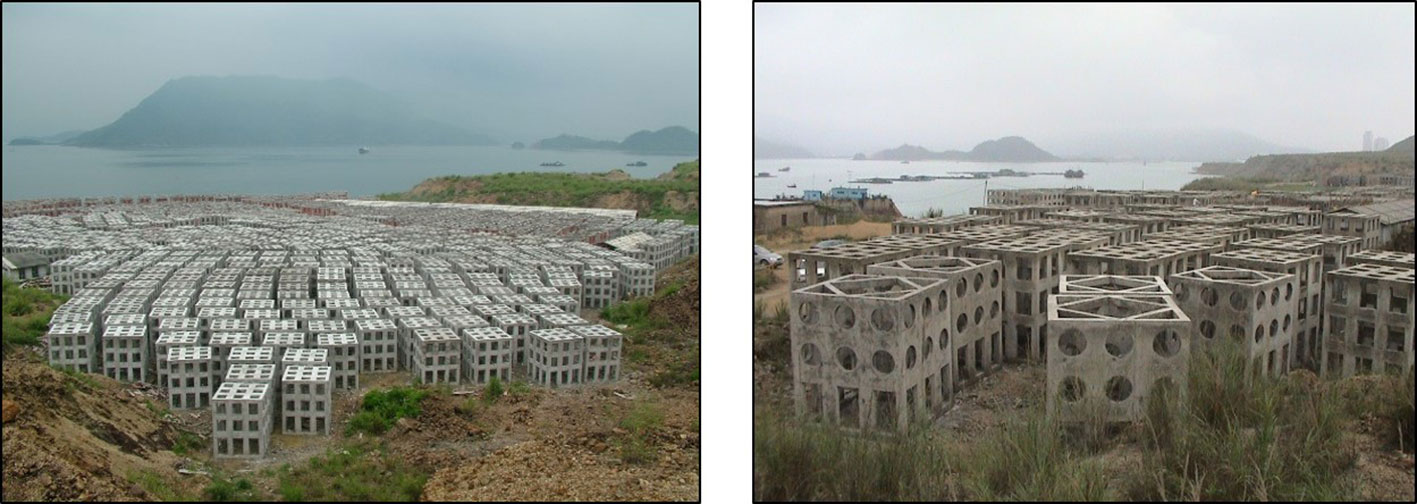
Figure 2 Reinforced concrete artificial reefs (3 × 3 × 5 m) laid on the seafloor in the artificial reef area of Dalajia.
Two types of habitats were selected in the current study: AR and control area (CA). The AR habitat was an inshore area where AR were laid, and two sampling sites (AR1 and AR2) were selected in this habitat. A bottom trawl and gill net were used for sampling at the AR1 and AR2 sampling sites, respectively. Because trawling cannot be performed in the reef area, AR1 was arranged at the edge of the reef area, and AR2 was arranged in the center of the AR area. The CA habitat was near the AR habitat and used to assess the effect of AR construction on the surrounding natural habitats. Similar to AR, two sampling sites (CA1 and CA2) were selected in CA, and a bottom trawl and gill net were used for sampling in CA1 and CA2, respectively (Figure 1). Both the AR and CA habitats were in the same marine space and differed only in whether AR had been constructed.
2.2. Investigation of nekton community
The nekton community was sampled in November 2002 before constructing the AR (background survey). Four tracking surveys were conducted in July 2004, August 2005, November 2006, and January 2016. The nekton community in both AR and CA habitats were sampled simultaneously during these periods.
Bottom trawler sampling was conducted at AR1 and CA1 for 10–15 min and 2.8–3.0 knots. Several gill nets were used to sample at AR2 and CA2 for 1.0–5.0 h. The nekton were identified at the species level using standard taxonomic references. The parameters of nets used in each sampling period are shown in Tables 1, 2.
2.3. Determination of taxonomic indexes
The following equations were used to characterize the nekton communities at each site:
where C is the average trawl net catch per hour (kg/h or individual/h), q is the net capture rate (0.5 for nekton) (Feng et al., 2021), and a is the sampling area (km2).
where c is the average gill net catch per hour (kg/h or individual/h), L is the gill net length (m), and H is the gill net height (m).
where S is the total number of species, Pi is the proportion of individuals in a sample belonging to species i, and N is the total number of individuals.
where F is the frequency of a certain species found at all sampling sites, M is the proportion of the quantity of a certain species compared to the total, W is the proportion of the weight of a certain species compared to the total. When the IRI > 1000, the species was considered as dominant (Wang XH et al., 2010).
where a1 is the number of species in community a, b1 is the number of species in community b, and j is the number of species common to both communities. When 0< Sj ≤ 0.25, the species composition of the two communities was considered as very different; when 0.25< Sj ≤ 0.50, the species composition of the two communities was considered as not similar; when 0.50< Sj ≤ 0.75, the species composition of the two communities was considered as similar; when 0.75< Sj ≤ 1.00, the species composition of the two communities was considered as very similar (Huang et al., 2019).
2.4. Determination of eco-exergy of nekton
The eco-exergy of the nekton community (i.e., eco-exergy of fauna per unit area) was determined using the following equation:
where 18.7 is the mean eco-exergy of detritus or dead organic matter in kJ/g, Ci is the biomass of the ith species (g/m2), and βi is the weighting factor of the genetic information based on Kullbach’s measurement of the genome size and complexity of the ith species (Jørgensen et al., 1995; Fu, 2015; Lu et al., 2015). The reference value for βi is 499 for fish, 230 for crustaceans, and 310 for cephalopods (Jørgensen et al., 2005).
3. Results
3.1. Changes in community composition and dominant species of fishery resources
3.1.1. Community composition of nekton
The results of the trawl net survey showed that nekton species in both the AR and CA habitats exhibited an increasing trend after reef construction, and the number of species in the AR habitat was larger than that in the CA habitat (Figure 3). Based on the four tracking surveys, the number of nekton in the reef area was 1.64-, 1.86-, 1.91-, and 1.68-fold larger than that during the background survey and 1.00-, 1.37-, 1.62-, and 1.37-fold larger than that of the CA in the same period (Table 3). The single sample T-test results showed that after AR construction, the species in both reef zone and contrast zone were significantly higher than those before AR construction (P< 0.05). Paired sample T-test results showed that species in AR was significantly higher than those in CA (P< 0.05). Analysis of the species composition of nekton showed that there was a clear increase in fish, whereas the trends for crustaceans was unclear. Cephalopods newly appeared at the sites during the tracking surveys (Figure 4). The proportion of fish among the total nekton in AR gradually increased from 27.27% during the background survey to 50.00% during the first, 51.22% during the second, 52.38% during the third, and 37.84% during the fourth tracking. The nekton species composition in the CA was similar to that in the AR, although their numbers were lower in the CA.
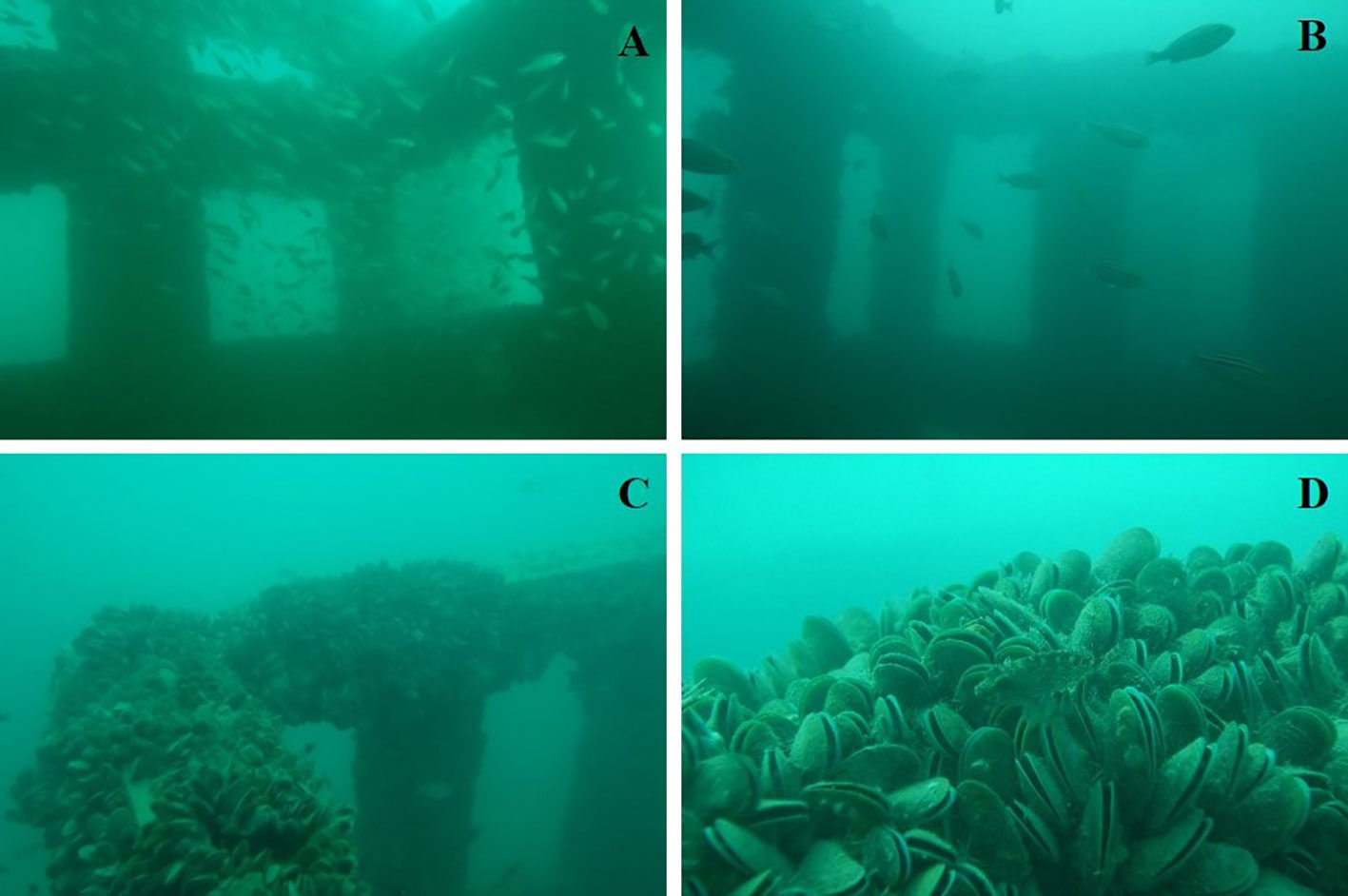
Figure 3 Underwater photos of fish aggregation in artificial reefs (A, B) and living organisms attached to reefs (C, D) in Dalajia, Daya Bay, China.
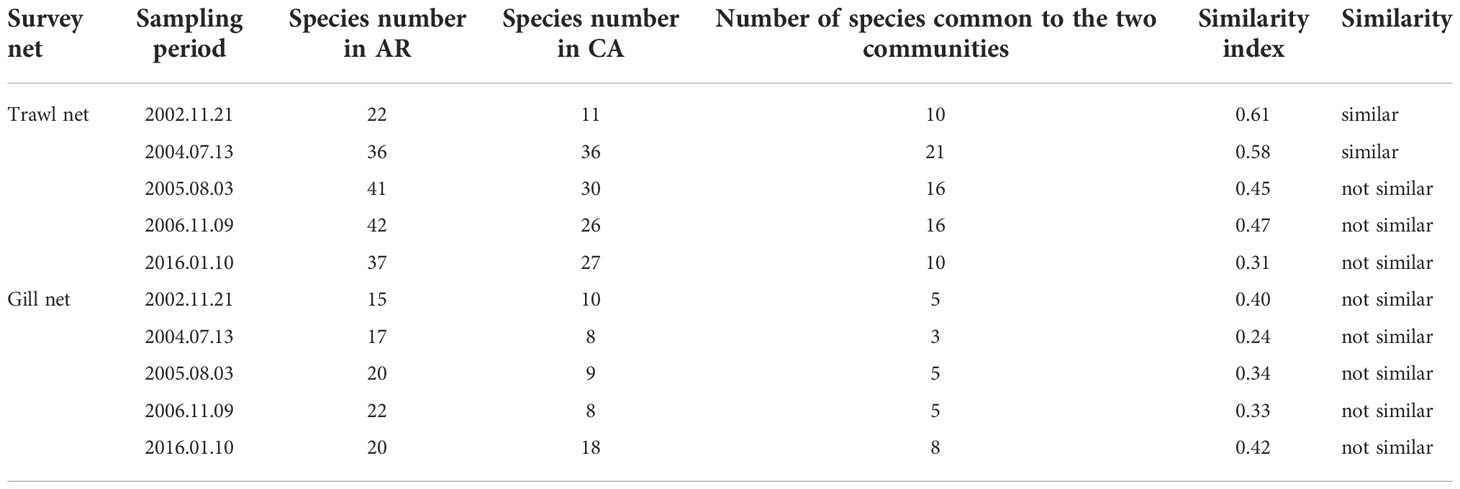
Table 3 Species and similarity index of nekton community in AR and CA habitats surveyed during trawl net and gill net sampling period.
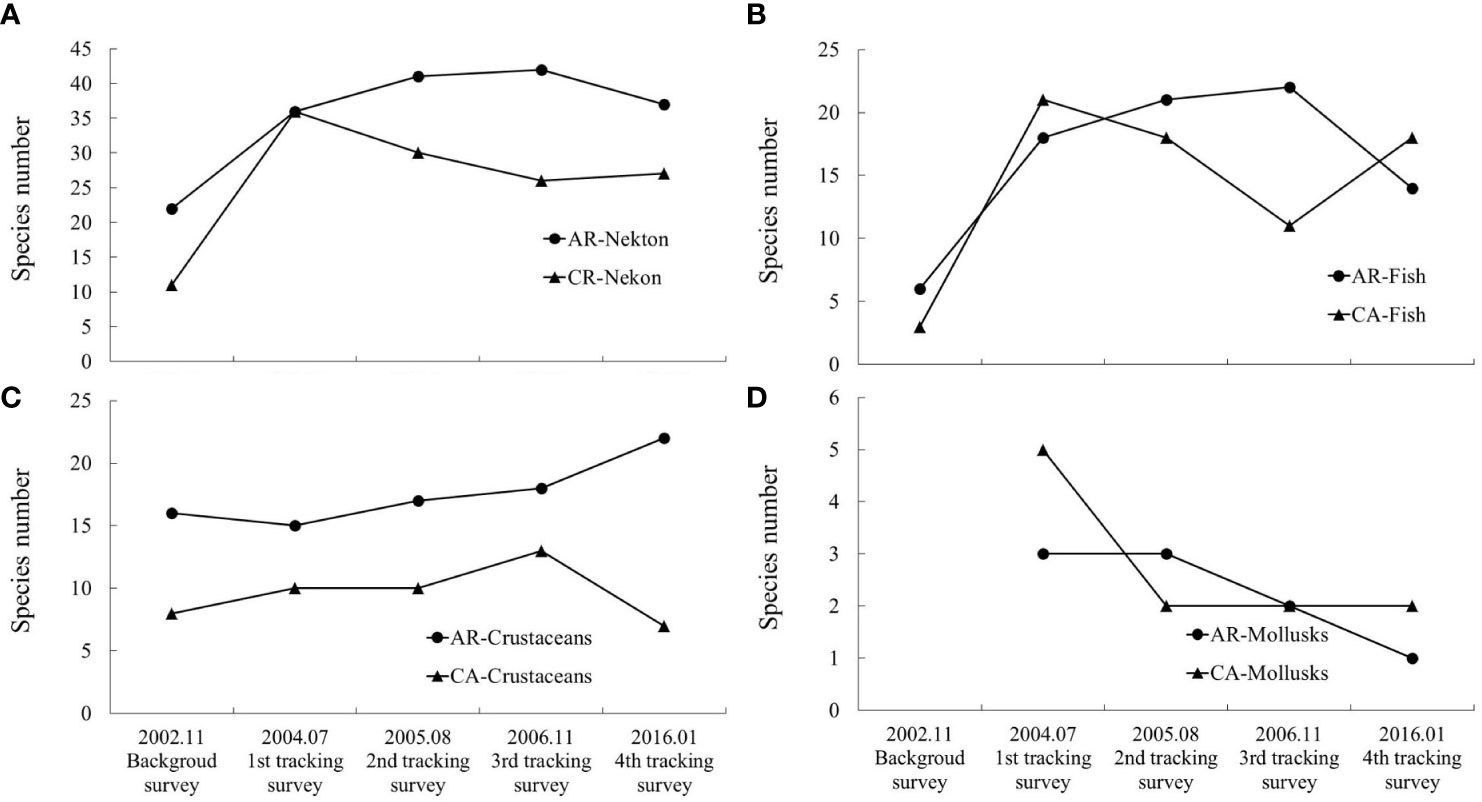
Figure 4 Species composition of nekton (A), fish (B), crustaceans (C) and mollusks (D) collected during each trawl net sampling periods in AR and CA habitats.
The similarity indices of nekton in the AR and CA habitats during the background survey and first tracking survey were 0.61 and 0.58, respectively; the nekton communities in the two habitats were similar. From the second to fourth tracking surveys, the similarity indices of AR and CA were 0.45, 0.47, and 0.31, respectively, and the nekton communities in the two habitats were not similar. Over time, the composition of nekton communities in the AR and CA showed a gradual difference.
The results of the gill net survey were similar to those of the trawl net survey (Figure 5). However, cephalopods did not appear in the catch of the gill net survey, and the similarity between AR and CA was lower (Table 3). The single sample T-test results showed that after AR construction, the species of AR was significantly higher than that before AR construction (P< 0.05), while the species was of CA was not significantly increased (P > 0.05).
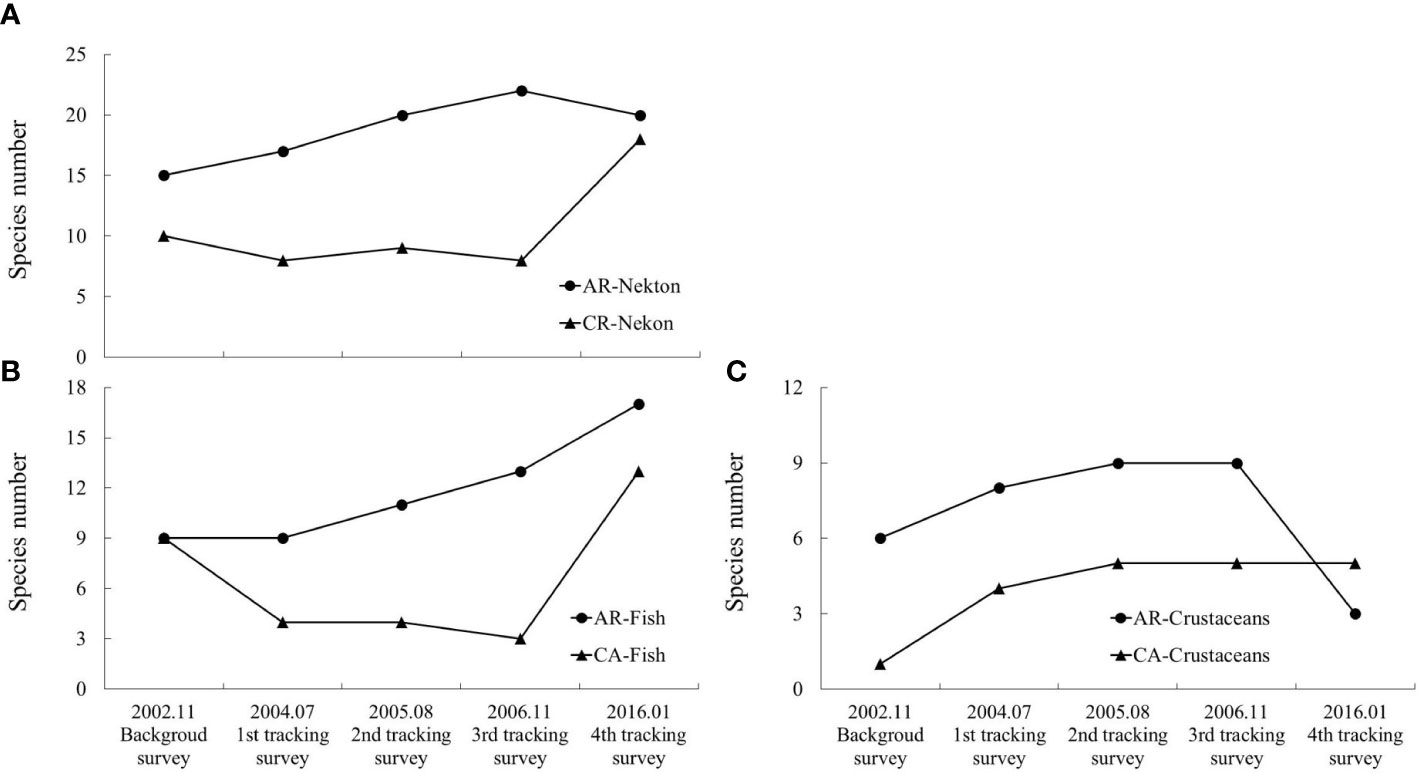
Figure 5 Species composition of nekton (A), fish (B) and crustaceans (C) collected during each gill net sampling periods in AR and CA habitats.
3.1.2. Changes in dominant species
The results of trawl net sampling showed that crustaceans dominated the nekton community in both habitats before AR construction. Among them, the dominant species were Exopalaemon carinicauda in the AR habitat and Harpiosquilla raphidea, Trachypenaeus curvirostris, and Acropoma japonicum in the CA habitat. After AR construction, fish dominated the nekton community in the AR habitat. Among them, the dominant species were Siganus oramin in the AR habitat and Dorippe facchino, Metapenaeopsis barbata, S. oramin, and Charybdis variegata in the CA habitat (Table 4).
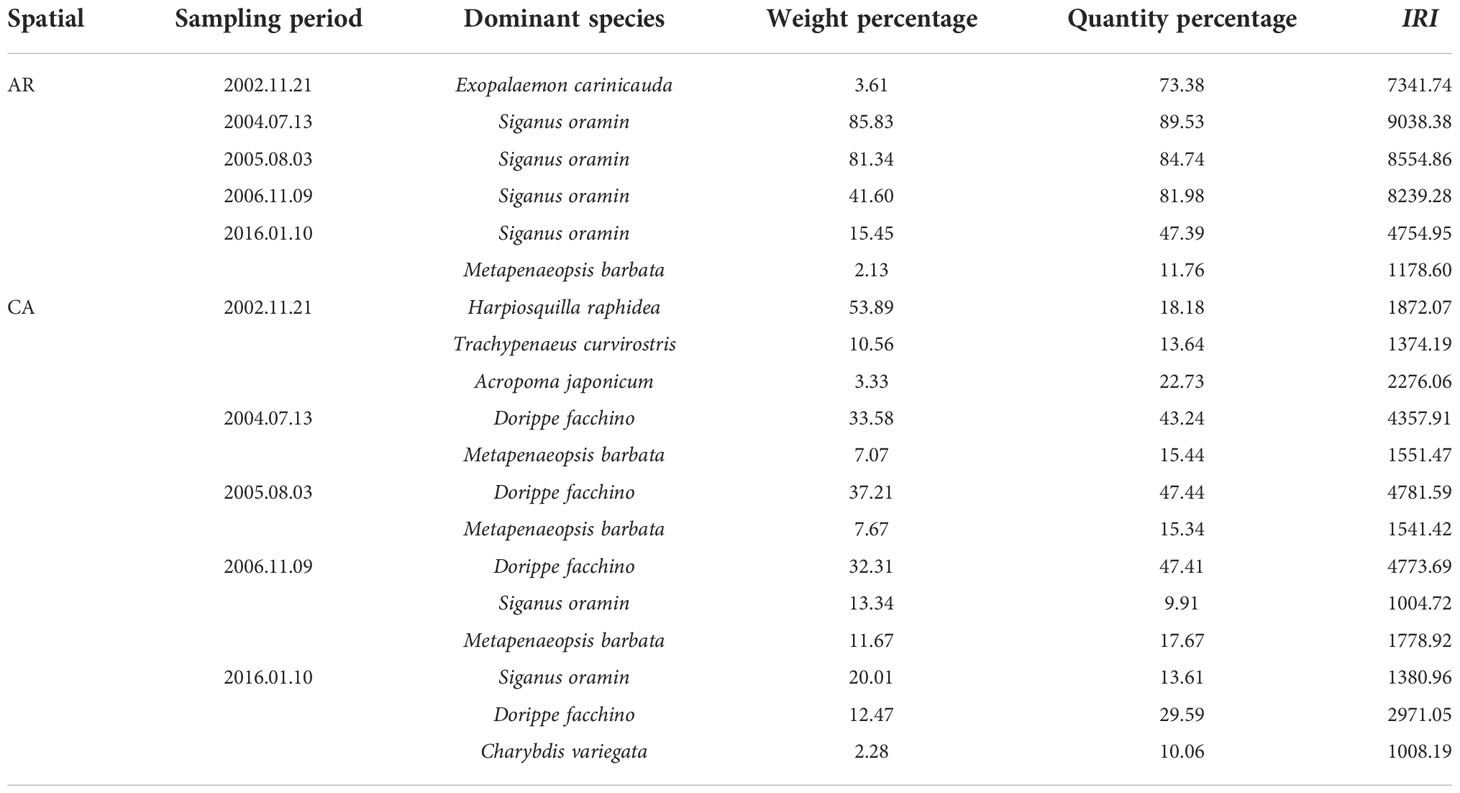
Table 4 Dominant species of nekton community in AR and CA habitat surveyed during trawl net sampling period.
Based on the results of gill net sampling, fish dominated the nekton community in both the AR and CA habitats before AR construction. However, both fish and crustaceans dominated the nekton community in both the AR and CA habitats after AR construction. Four fish and one crustacean species dominated the AR habitat, whereas 11 fish and four crustacean species dominated the CA habitat (Table 5).
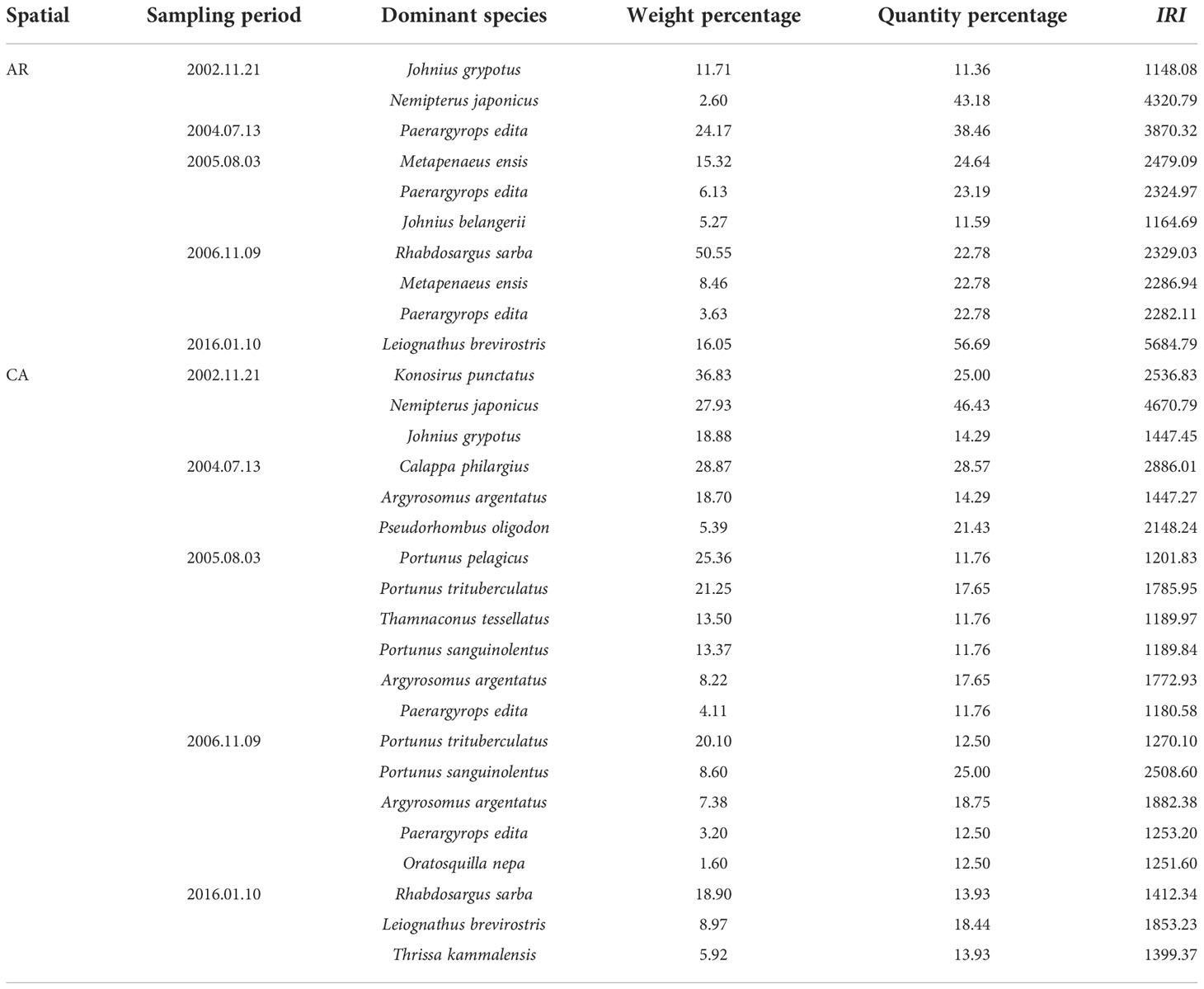
Table 5 Dominant species of nekton community in AR and CA habitat surveyed during gill net sampling period.
Compared with the background survey, after years of recovery and development, fish such as S. oramin, Leiognathus brevirostris, and Paerargyrops edita as well as crustaceans such as M. barbata, and Metapenaeus ensis replaced Johnius grypotus, Nemipterus japonicus, and Exopalaemon carinicauda and became the dominant species in the AR habitat and its adjacent waters.
3.2. Fishery resource density and community biodiversity after artificial reef construction
3.2.1. Biomass and abundance
The results of trawl net sampling showed that the biomass and abundance of nekton were higher both in the AR and CA after AR construction, with higher values observed in the AR than the CA. The biomass and abundance of nekton in the AR during the first to fourth tracking surveys showed an initial increasing trend and then decreased, with biomasses that were 17.74-, 22.22-, 13.13-, and 12.93-fold higher than those during the background survey and abundances that were 4.78-, 5.13-, 2.95-, and 1.63-fold higher than that during the background survey (Figure 6). Single sample T-test results showed that after AR construction, biomass and abundance increased significantly in both AR and CA (P< 0.05). The paired sample T-test showed that the AR biomass and abundance were significantly higher than those in the CA (P< 0.05).
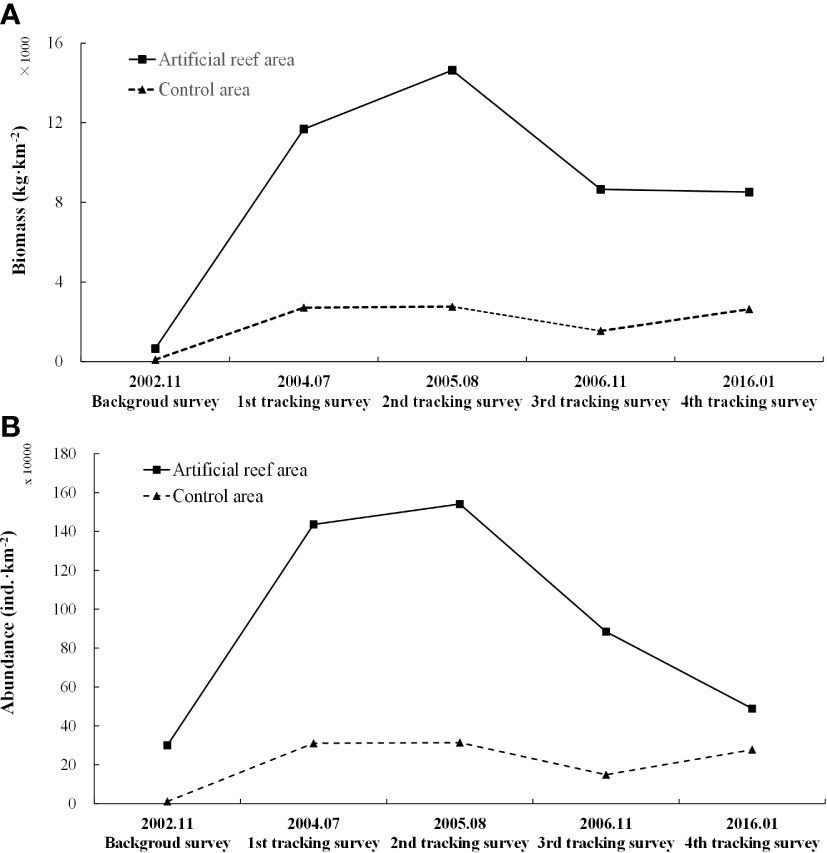
Figure 6 Changes in nekton community biomass (A) and abundance (B) in the AR and CA habitats surveyed during each trawl net sampling period.
According to the results of gill net sampling, the changes in biomass and abundance in both the AR and CA habitats were similar to those of trawl net sampling, showing a greater increase in improvement (Figure 7). Single sample T-test results showed that after AR construction, biomass increased significantly in both AR and CA (P< 0.05), the abundance of AR and CA increased not significantly after AR construction. The Paired sample T-test showed that there were no significant differences in biomass and abundance between AR and CA (P > 0.05).
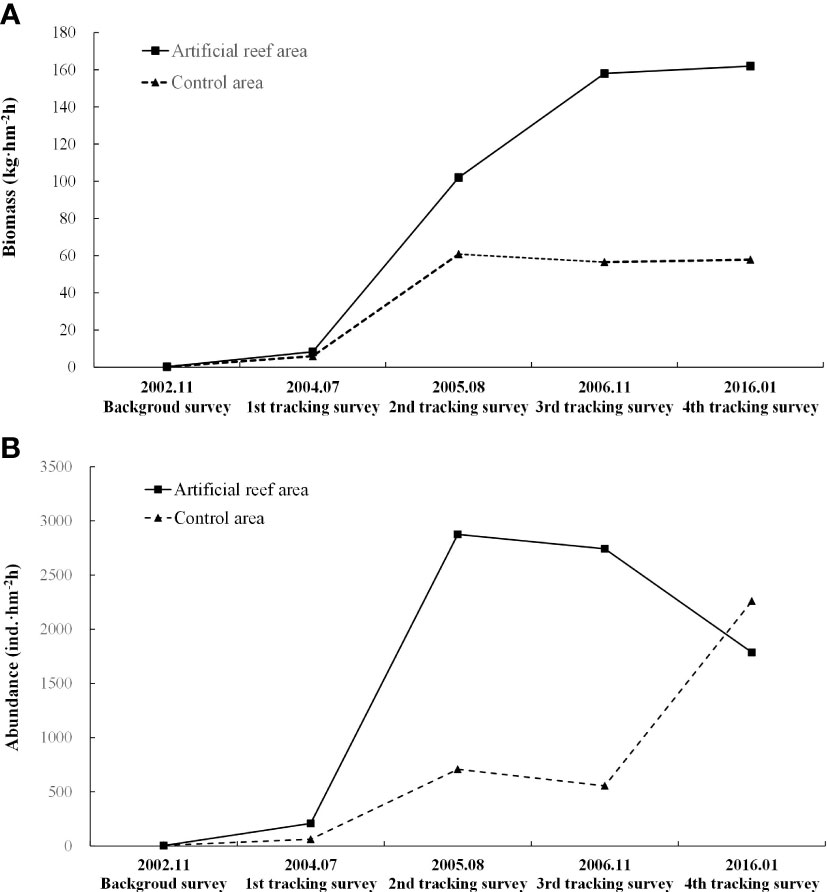
Figure 7 Changes in nekton community biomass (A) and abundance (B) in the AR and CA habitats surveyed during each gill net sampling period.
3.2.2. Taxonomic diversity
From the results of trawl net sampling (Table 6), the Shannon–Weiner diversity and Pielou’s evenness indices of the nekton community decreased initially and then increased, whereas Margalef’s species richness index increased initially and decreased in both the AR and CA habitats after AR construction. Shannon–Weiner diversity and Pielou’s evenness indices of the nekton community in the AR habitat decreased during the first to third tracking surveys compared to those during the background survey and increased during the fourth tracking survey. Margalef’s species richness index in the AR habitat was higher during the first to fourth tracking surveys than that during the background survey. These changes in the Shannon–Weiner diversity, Pielou’s evenness, and Margalef’s species richness indices of the nekton community in the CA habitat were similar to those in the AR habitat.
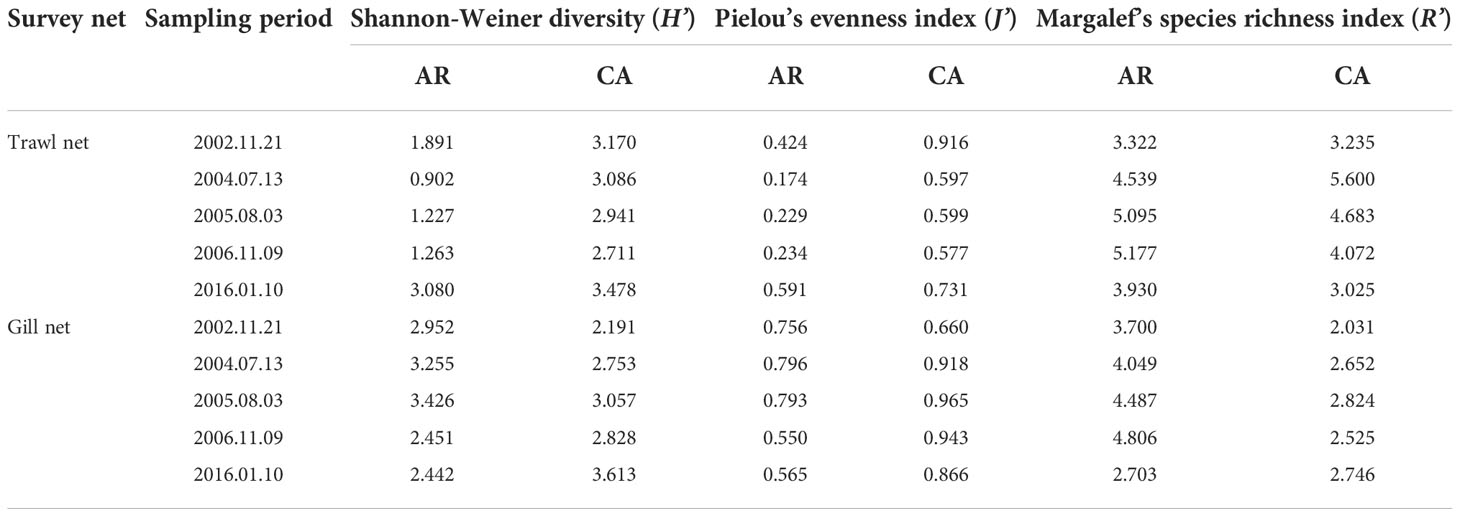
Table 6 Taxonomic diversity indices of nekton community in two habitats survey during trawl net and gill net sampling period.
Based on the results of gill net sampling (Table 6), the Shannon–Weiner diversity, Pielou’s evenness, and Margalef’s species richness indices of the nekton community in the AR habitat increased initially and decreased after AR construction. The Shannon–Weiner diversity and Pielou’s evenness indices of the nekton community in the AR habitat were higher during the first and second tracking surveys and lower during the third and fourth tracking surveys than those during the background survey. Margalef’s species richness index in AR habitat was higher during the first to third tracking surveys and lower during the fourth tracking survey compared to those in the background survey. The Shannon–Weiner diversity, Pielou’s evenness, and Margalef’s species richness indices of the nekton community showed increasing trends during the first to fourth tracking surveys in the CA habitat after AR construction.
3.3. Eco-exergy of the nekton community after artificial reef construction
The eco-exergy of the nekton community increased in both the AR and CA habitats after AR construction and reached a maximum at 3 years after AR construction (Table 7). The eco-exergy of the nekton community in AR habitat increased by 17.97-fold, and the community structure was more orderly and complex at 14 years after AR construction. During the first to fourth tracking surveys, the eco-exergy of the nekton community in the AR habitat was 7.03-, 8.48-, 6.66-, and 3.27-fold higher than those in the CA habitat during the same period, respectively. Fish were the major contributors to the eco-exergy increase by the nekton community; the eco-exergy proportion of fish during the background survey was 26.65%, which increased to 95.66%, 94.09%, 68.18%, and 78.51% during the first to fourth tracking surveys, respectively. The eco-exergy proportion of crustaceans generally showed a decreasing trend, whereas that of the proportion of mollusks generally showed an increasing trend after AR construction, indicating that fish and mollusks play important roles in maintaining the stability and complexity of the nekton community structure in the AR habitat. The eco-exergy proportion of fish and crustaceans showed an increasing trend, whereas that of mollusks showed a decreasing trend in the CA habitat after AR construction.
4. Discussion
4.1. Community composition and dominant species of fishery resources
Changes in fishery resource community compositions is a central concept in research aimed at fishery resource conservation (Brotto and Araujo, 2001; Jan et al., 2003). Many studies have shown that AR play important roles in attracting nekton communities and promoting the aggregation and recovery of biological communities within their effective impact areas. Particularly, fish attraction is important for the aggregation, conservation, and proliferation of fishery resources (Rooker et al., 1997; Liu et al., 2007; Yuan et al., 2011; Dong et al., 2015). In this study, the number of species in the nekton community in both the AR and CA habitats greatly increased over time and were higher in the AR habitat than in the CA habitat during the same period. AR promote habitat changes and influence the surrounding waters of the AR habitat, which is similar to the observations in previous studies (Zhang et al., 2006; Wang et al., 2016).
The number of species in AR habitat was larger than that in CA habitat. This may be attributed to three factors. First, the presence of AR obstructs trawling in the reef area, which protects fishery resources and habitats to some extent. Second, the AR are taller than the seabed, and thus alter the local flow field and produce upwelling effects and wake vortices. Upwelling accelerates the circulation of nutrients in water, causes the rapid growth of plankton, and provides abundant food source for the nekton community such as fish; these factors are conducive to the growth and survival of fishery organisms. The flow field effect produced by the wake vortex causes fish to aggregate. Finally, AR structures on the sea floor provide habitat spaces for the nekton community, including reef fish, to live, feed, and reproduce (Williams-Grove and Szedlmayer, 2017; Jiang et al., 2019).
The similarity between the background survey and first tracking survey of the nekton community was high both in the AR and CA habitats. Over time, the similarity in the nekton community decreased in the two habitats, with gradual differences observed in the community composition in the two regions. The time between the first tracking and background survey was relatively short, and the aggregation effect of AR on nekton was not clear (Wang et al., 2012; Liu et al., 2016). Over time, the sea area tends to self-regulate, and biological changes caused by habitat changes gradually tend to stabilize (Wang ZH et al., 2010). Further, the nekton community adapts to the habitat, gradually reflected by the aggregation and proliferation of nekton surrounding the AR. The species and number of reef-loving fish have greatly increased and gradually become the dominant species. Our survey results showed that S. oramin in the AR habitat was the absolute dominant species, verifying this result.
One limitation of this study was that the number of sampling sites was small; therefore, our results may not completely reflect the situation of the two habitats. Long-term fixed-point observation can be performed to overcome this limitation.
4.2. Biomass, abundance, and taxonomic diversity
In this study, the increases in the biomass and abundance of nekton community after AR construction reflected the effect of the AR in the conservation and proliferation of fishery resources. Based on the changes in the biomass and abundance of the nekton community, the biomass increase was more obvious than that of the abundance in the AR habitat. Thus, the AR clearly protected fishery resources, even 14 years after AR construction. The biomass of the nekton community remained relatively stable from years 4 to 14 after AR construction.
According to the results, compared with the tracking survey in 2006, fish biomass remained stable and abundance showed a downward trend in 2016. Meaning that as the body weight of fish increases, the population decreases when the resource density is similar. Why did the abundance decreased over time? We speculate the reasons are as follows, there was a gap of 10 years between the third tracking survey in 2006 and the fourth tracking survey in 2016. During this period, fish in AR experienced a series of changes, including an increase in quantity and quality. The construction of AR provided a place for fish and other organisms to grow, spawn and reproduce. In the early stage of AR construction, fish gathered in the AR, and the density and abundance of fish resources increased. Then fish spawn in the AR and the supplementary group of fish increased. The growth of fish stocks presents an “S” shaped increase in logistics mode. The supplementary fish groups in the AR gradually increased over time. After reaching a certain number, the number of fish no longer increase due to the restrictions of AR space and food resources, and at the same time, with the growth of individuals, the number even shows a trend of decline. This situation showed that under the premise of limited resources in the AR space, the increase of reef fish resources has reached the optimal state.
Compared with the background survey, variations in the diversity and evenness indices of the AR habitat in tracking surveys by trawl net initially declined and then increased, and was lower than those in the CA habitat during the same period, indicating that the diversity in the AR habitat was not high and distribution of the number of species was uneven. This may be because of improvements in the heterogeneity of the benthic habitats following AR construction (Tews et al., 2004), resulting in explosive growth of reef fish resources, such as S. oramin, in the AR habitat. The explosive growth was quantitatively and qualitatively higher than that of other species, with S. oramin becoming the dominant species in the reef area. In the 2004 trawl survey, the catch and tail number of S. oramin were 93.86% and 95.78% of the total catch, respectively. In 2005, the catch and tail number of S. oramin were 92.04% and 95.94% of the total catch, respectively. In 2006, the catch and tail number of S. oramin were 83.63% and 96.20% of the total catch, respectively. In 2016, the catch and mantissa were 24.34% and 79.66% of the total catch, respectively. The formula for diversity and evenness is based on the proportion of the number of each species to the mantissa of the total catch, When there was only one dominant specie, the results of diversity and low uniformity were low according to the calculation formula. Thus, the diversity and evenness indices showed a decreasing trend, although the number of nekton species was significantly higher than that in the background survey and CA habitat during the same period. Relative abundance or taxonomic diversity alone is not always an appropriate indicator of the ecosystem complexity of a species, and coupled with other indicators of the species can reveal more information in ecosystem functioning.
Compared with the background survey, the species richness indices of the nekton community in the four tracking surveys showed an increasing trend in both the AR and CA habitats, indicating that the number of nekton species and community richness increased in both the AR and CA habitats after AR construction. The ecological effect of artificial fish reefs on the surrounding biological communities is not constant. With changes in the surrounding environmental conditions and topography, ecological communities change and undergo succession, and the ecosystem tends to the structural degree of maximum energy during continuous change (Santos and Monteiro, 1997). Over the long term, AR can increase the biomass, abundance, and species richness of fishery resource communities (Nicoletti et al., 2007; Santos and Monteiro, 2007).
4.3. Eco-exergy of the nekton community
Because of the existence of an absolute dominant species, taxonomic diversity alone is not always an appropriate indicator of ecosystem complexity; however, taxonomic diversity coupled with other indicators of the species can reveal more information on ecosystem function (Hooper et al., 2005). Limited by the complexity of the ecosystem and technical conditions in ecological research, the approximate ecological value of the system is often determined based on the results of observation, providing an approximation of the entire system or selective information on parts of the system (Zhang et al., 2010). Nevertheless, as an important objective function, ecological value has certain advantages in ecosystem research. Four tracking surveys were conducted in the AR and CA habitats in Dalajia from 2002 to 2016. During the 14 years after AR construction, the eco-exergy of nekton community increased in both the AR and CA habitats, indicating that the ecosystems of the AR and CA habitats became increasingly complex, and the structural stability of the system gradually changed to a healthy structure with more species and improved stability. The eco-exergy of the nekton community in the AR habitat was higher than that in the CA habitat during the same period, indicating that the AR habitat has more complex and stable benefits for the nekton community structure, and that the benefits extended to the CA habitat. The results also showed that the biomass of nekton community was most closely related to exergy, which is consistent with other studies (Lu et al., 2015). The change of dominant species along with the AR age might be another responsibility for the changes in eco-exergy of nekton community. Eco-exergy can be used to assess and analyze the trend of the dynamic change of the ecosystem and the expected result. The ecological effects of artificial reef construction on the surrounding biological communities are not invariable. With the change of the surrounding environmental conditions and topography, the ecological community also keeps changing and succeeding, and the ecosystem tends to the structure degree of maximum energy value in the process of constant change. The exergy value of nekton community in Dalijia AR increased continuously, indicating that the reef ecosystem changed from simple to complex, and the stability of the system changed from fragile structure to a healthy structure with increased species and increased stability. Eco-exergy indicators were introduced to explain the role of reef building in restoring fishery resources more comprehensively.
In general, AR construction improved and promoted the diversity and stability of the ecosystem in the AR habitat and surrounding areas. Over time and with further restoration and improvement of the ecosystem, its effects on fishery resource conservation and ecological restoration will become clearer.
A more comprehensive method and longer-term follow-up investigation are needed to further evaluate the impact of AR construction on marine biological resources. To further examine fishery resource conservation and ecological restoration of AR from the perspective of the contribution rate of ecological exergy from the nekton community, continuous follow-up investigations are needed.
5. Conclusions
The number of species and resource density of the nekton community have increased both in the AR and CA habitats, with the AR habitat showing a greater increase than the CA habitat after AR construction in Dalajia. Siganus oramin has become the absolutely dominant species in the AR habitat and its adjacent waters. The diversity and evenness of the nekton community showed a decreasing trend, whereas the species richness improved in the AR habitat and its surrounding areas. Changes in the abundance, biomass, and eco-exergy of the nekton community showed increasing trends over time. AR construction has different effects on the structural changes of fish, crustaceans, and mollusks and it has the greatest benefits on the conservation and proliferation of fish resources. But, at the same time, the dominance of S. oramin can interfere in the reef community. The explosive growth was quantitatively and qualitatively higher than that of other species, the diversity and evenness indices showed a decreasing trend, although the number of nekton species was significantly higher than that in the background survey and CA habitat during the same period. These results highlight that the construction of AR has greatly improved the restoration of nekton community resources in the sea area, improved the structure of nekton community communities, and increased the stability of ecosystems, even at 14 years after AR construction.
Data availability statement
The raw data supporting the conclusions of this article will be made available by the authors, without undue reservation.
Author contributions
HY designed this study, collected and analyzed the data, and drafted the manuscript. PC helped with data analysis. XL carried out the survey of fishery resources. All authors contributed to the article and approved the final manuscript.
Funding
This research was funded by (1) Guangdong Key Areas R&D Program Projects (2020B1111030002), (2) Central Public-interest Scientific Institution Basal Research Fund, CAFS, China (2021SD02, 2020TD06).
Conflict of interest
The authors declare that the research was conducted in the absence of any commercial or financial relationships that could be construed as a potential conflict of interest.
Publisher’s note
All claims expressed in this article are solely those of the authors and do not necessarily represent those of their affiliated organizations, or those of the publisher, the editors and the reviewers. Any product that may be evaluated in this article, or claim that may be made by its manufacturer, is not guaranteed or endorsed by the publisher.
References
Avigdor A., Yehiam S. (2002). Comparison of the development of coral and fish communities on rock-aggregated artificial reefs in eilat, red Sea. ICES J. Mar. Sci. 59, S122–S126. doi: 10.1006/jmsc.2002.1210
Baine M. (2001). Artificial reefs: a review of their design, application, management and performance. Ocean Coast. Manage. 44, 241–259. doi: 10.1016/s0964-5691(01)00048-5
Brotto D. S., Araujo F. G. (2001). Habitat selection by fish in an artificial reef in ilha grande bay, brazil. Brazil. Arch. Biol. Technol. 3, 319–324. doi: 10.1590/S1516-89132001000300015
Chen X., Feng Q. Y., Deng Z. R. (2006). A studying on present situation of man-made reef constructions and its development countermeasures. Natural Sci. J. Hainan Univ. 01, 83–89. doi: 10.3969/j.issn.1004-1729.2006.01.015
Chen Z. Z., Kong X. L., Xu S. N., Qiu Y. S., Huang Z. R. (2012). Dynamic changes of population parameters of nemipterus bathybius in the beibu gulf. J. Fish. China. 04, 584–591. doi: 10.3724/SP.J.1231.2012.27663
Chen Y. H., Li H. Q., Chen P. M., Hong J. Z. (2007). Preliminary evaluation on the construction effect of artificial reef area in the south of dalajia, daya bay. Ocean Fishery. 07, 13–15.
Chen P. M., Shu L. M., Yuan H. R., Feng X., Tong F., Chen Q., et al. (2019). Review on development, definition and classification of marine ranching in domestic and overseas. J. Fish. China. 09, 1851–1869. doi: 10.11964/jfc.20190711887
Chen Q., Yuan H. R., Chen P. M. (2019). Integrated response in taxonomic diversity and eco-exergy of Icrobenthic faunal community to artificial reef construction in daya bay, China. Ecol. Indic. 101, 512–521. doi: 10.1016/j.ecolind.2019.01.049
Couto T., Patrício J., Neto J. M., Ceia F. R., Franco J., Marques J. C. (2010). The influence of mesh size in environmental quality assessment of estuarine Icrobenthic communities. Ecol. Indic. 06, 1162–1173. doi: 10.1016/j.ecolind.2010.03.019
Dong T. W., Huang L. Y., Tang Y. L., Sheng H. X., Liu C. D. (2015). Preliminary evaluation of artificial reef around rizhao qiansan island on the enhancement of fishery resources. Periodical Ocean Univ. China 8, 38–45. doi: 10.16441/j.cnki.hdxb.20140173
FAO Fisheries and Aquaculture Department (2018). Available at: http://www.fao.org/fishery/en.
Feng X., Fan J. T., Sun X., Hong J. Z., Chen P. M. (2021). The stock enhancement effect evaluation of artificial reef in wailingding, zhuhai. J. South. Agric. 12, 3228–3236. doi: 10.3969/j.issn.2095-1191.2021.12.005
Folpp H., Lowry M., Gregson M., Suthers I. M. (2013). Fish assemblages on estuarine artificial reefs: natural rocky-reef mimics or discrete assemblages? PLos One 06, e63505. doi: 10.1371/journal.pone.0063505
Fu F. Y., Lu H. F. (2015). Effects of Urbanization on Soil Community Structure under Subtropical Evergreen Broad-leaved Forests. Ecology and Environmental Sciences 06, 938–46. doi: 10.16258/j.cnki.1674-5906.2015.06.005
Fukunaga A., Bailey-Brock J. H. (2008). Benthic infaunal communities around two artificial reefs in mamala bay, Oahu, Hawaii. Mar. Environ. Res. 3, 250–263. doi: 10.1016/j.marenvres.2007.11.003
Hooper D. U., Chapin F., Ewel J., Hector A., Inchausti P., Lavorel S., et al. (2005). Effects of biodiversity on ecosystem functioning: a consensus of current knowledge. Ecol. Monogr. 1, 3–35. doi: 10.1016/j.drugalcdep.2010.01.006
Huang X. M., Yang S. C., Peng J., Zheng Z. H., Tong S. J. (2019). Analysis of species diversity and community similarity of plant communities in riparian zone of the paihe river: Constructing the model of riparian buffer strips. Environ. Eng. 12, 55–59. doi: 10.13205/j.hjgc.201912010
Jørgensen S. E., Ladegaard N., Debeljak M., Marques J. C. (2005). Calculations of exergy for organisms. Ecol. Modell. 2-4), 165–175. doi: 10.1016/j.ecolmodel.2004.11.020
Jørgensen S. E., Nielsen S. N., Mejer H. (1995). Energy, environ, exergy and ecological modelling. Ecol. Modell. 2-3), 99–109. doi: 10.1016/0304-3800(93)E0080-M
Jan R. Q., Liu Y. H., Chen C. Y., Wang M. C., Song G. S., Lin H. C., et al. (2003). Effects of pile size of artificial reefs on the standing stocks of fishes. Fish. Res. 3, 327–337. doi: 10.1016/S0165-7836(03)00081-X
Jiang Z. Y., Guo Z. S., Zhu L. X., Liang Z. L. (2019). Structural design principle and research progress of artificial reef. J. Fish. China. 9, 1881–1889. doi: 10.11964/jfc.20190711889
Jiao J. J., Pan Y. X., Sun L. Y., Yang B. Q., Qiu S. R. (2011). Effect of artificial reefs on fish multiplication. Fish. Sci. 02, 79–82. doi: 10.3969/j.issn.1003-1111.2011.02.004
Liu T. Y. (2003). Construction of artificial reefs at home and abroad. Fishery Modernization. 2, 36–37. doi: 10.3969/j.issn.1007-9580.2003.02.020
Liu Y. H., Chen Q., Tian T., Chen Y., Yin Z. Q., Liu H. C., et al. (2016). Evaluation and changes in water quality at artificial reef area in zhangzi island in dalian in summer. J. Dalian Ocean Univ. 3, 331–337. doi: 10.16535/j.cnki.dlhyxb.2016.03.018
Liu H. Y., Lü H. B., Zhang P. D., Li W. T., Zhang X. M. (2018). Attraction effect of artificial reef model and macroalgae on juvenile sebastes schlegelii and hexagrammos otakii. J. Fish. China 01, 48–59. doi: 10.11964/jfc.20161210634
Liu S. B., Wang Z. H., Lin L. W., Yang P. H., Zhang S. Y. (2007). Preliminary evaluation on effects of artificial reef project in shengsi. J. Shanghai Fish. Univ. 3, 297–302. doi: 10.3969/j.issn.1004-7271.2007.03.018
Li H., Zeng X. S., Lu H. F. (2012). Eco-exergyanalysis of structural dynamics of Schima wallichii plantation in heshan. Ecol. Environ. Sci. 11, 1822–1829. doi: 10.16258/j.cnki.1674-5906.2012.11.001
Lowry M., Glasby T., Boys C., Folpp H., Suthers I., Gregson M. (2014). Response of fish communities to the deployment of estuarine artificial reefs for fisheries enhancement. Fish. Manage. Ecol. 01, 42–56. doi: 10.1111/fme.12048
Lu H. F., Fu F. Y., Li H., Campbell D. E., Ren H. (2015). Eco-exergy and emergy based self-organization of three forest plantations in lower subtropical China. Sci. Rep. 5, 15047. doi: 10.1038/srep15047
Marchi M., Jørgensen S. E., Bécares E., Corsi I., Marchettini N., Bastianoni S. (2011). Dynamic model of lake chozas (León, NW spain)-decrease in eco-exergy from clear to turbid phase due to introduction of exotic crayfish. Ecol. Modell. 16, 3002–3010. doi: 10.1016/j.ecolmodel.2011.04.016
McCauley D. J., Pinsky M. L., Palumbi S. R., Estes J. A., Joyce F. H., Warner R. R. (2015). Marine defaunation: animal loss in the global ocean. Science. 347 (6219), 1255641. doi: 10.1126/science.1255641
Mills K. A., Hamer P. A., Quinn G. P. (2017). Artificial reefs create distinct fish assemblages. Mar. Ecol. Prog. Ser. 585, 155–173. doi: 10.3354/meps12390
Nicoletti L., Marzialetti S., Paganelli D., Ardizzone G. (2007). Long-term changes in a benthic assemblage associated with artificial reefs. Hydrobiologia. 1, 233–240. doi: 10.1007/s10750-006-0450-3
Perkol-Finkel S., Shashar N., Benayahu Y. (2006). Can artificial reefs mimic natural reef communities? the roles of structural features and age. Mar. Environ. Res. 2, 121–135. doi: 10.1016/j.marenvres.2005.08.001
Pickering H., Whitmarsh D., Jensen A. (1999). Artificial reefs as a tool to aid rehabilitation of coastal ecosystems: Investigating the potential. Mar. pollut. Bull. 37, 505–514. doi: 10.1016/s0025-326x(98)00121-0
Rilov G., Benayahu Y. (1998). Vertical artificial structures as an alternative babitat for coral reef fishes in disturbed environments. Mar. Environ. Res. 45, 431–451. doi: 10.1016/S0141-1136(98)00106-8
Rooker J. R., Dokken Q. R., Pattengill C. V., Holt G. J. (1997). Fish assemblages on artificial and natural reefs in the flower garden banks national marine sanctuary, USA. Coral Reefs 2, 83–92. doi: 10.1007/S003380050062
Santos L. N., García-Berthou E., Agostinho A. A., Latini J. D. (2011). Fish colonization of artificial reefs in a large Neotropical reservoir: material type and successional changes. Ecol. Appl. 1, 251–262. doi: 10.1890/09-1283.1
Santos M. N., Monteiro C. C. (1997). The olhão artificial reef system (south portugal): Fish assemblages and fishing yield. Fish. Res. 1-2, 33–41. doi: 10.1016/S0165-7836(96)00567-X
Santos M. N., Monteiro C. C. (2007). A fourteen-years overview of the fish assemblanges and yield of the two oldest Algarve artificial reefs (Southern Portugal). Hydrobiologia 1, 225–231. doi: 10.1007/s10750-006-0451-2
Schroeter S. C., Reed D. C., Raimondi P. T. (2015). Effects of reef physical structure on development of benthic reef community: A large-scale artificial reef experiment. Mar. Ecol. Progr. Ser. 26, 43–55. doi: 10.3354/meps11483
Steimle F. W., Meier M. H. (1997). What information do artificial reef managers really want from fishery science? Fisheries 4, 6–9.
Su X., Wu L. J., Xu J. M. (2006). Restore and reconstruct the ecosystem of marine fisheries in China to get marine resources sustained. Chin. Fish. Economics 04, 41–44. doi: 10.3969/j.issn.1009-590X.2006.04.014
Tews J., Brose U., Grimm V., Tielbörger K., Wichmann M., Schwager M., et al. (2004). Animal species diversity driven by habitat heterogeneity/diversity: the importance of keystone structures. J. Biogeogr. 1, 79–92. doi: 10.1046/j.0305-0270.2003.00994.x
Tong C. F., Xia F. (2017). Problem and solutions in china’s marine fishery against the background of the supply-side reform. J. Ocean Univ. China (Soc. Sci.) 05, 26–29. doi: 10.16497/j.cnki.1672-335x.2017.05.004
Wang X. H., Du F. Y., Qiu Y. S., Li C. H., Sun D. R., Jia X. P. (2010). Variations of fish species diversity, faunal assemblage, and abundances in daya bay in 1980-2007. Chin. J. Appl. Ecol. 9, 2403–2410. doi: 10.13287/j.1001-9332.2010.0350
Wang Y., Hou C. Q., Wang X. Y., Zhang B. L., Chen W., Xu X. P. (2018). Preliminary evaluation on proliferation effects of artificial reefs in live oyster reef area, tianjin, China. Transact. Oceanol. Limnol. 02, 137–145. doi: 10.13984/j.cnki.cn37-1141.2018.02.019
Wang L. Y., Huang Y. Y. (2019). Research on the monitoring of marine ranching. Technol. Innov. Appl. 06, 71–72. doi: 10.3969/j.issn.2095-2945.2019.06.027
Wang W. D., Liang J., Zhang S. Y. (2010). Influence of artificial reef construction on nutrition and water quality in off - shore area of shengsi, zhejiang. Acta Hydrobiol. Sin. 1, 78–87. doi: 10.3724/SP.J.1035.2010.00078
Wang Y. S., Lou Z. P., Sun C. C., Sun S. (2008). Ecological environment changes in daya bay, China, from 1982 to 2004. Mar. pollut. Bull. 11, 1871–1879. doi: 10.1016/j.marpolbul.2008.07.017
Wang X. H., Qiu Y. S., Du F. Y., Liu W. D., Sun D. R., Chen X., et al. (2019). Roles of fishing and climate change in long-term fish species succession and population dynamics in the outer beibu gulf, south China Sea. Acta Oceanol. Sin. 10, 1–8. doi: 10.1007/s13131-019-1484-5
Wang X. M., Tang Y. L., Sun X. M., Sheng H. X., Wan R. (2016). Catch community structure characteristics and their relationships with environmental factors in the xiao shidao artificial reef sea area of weihai. Mar. Sci. 11, 34–43. doi: 10.11759//hykx20151123001
Wang X., Yu J., Li Y. Z., Chen G. B., Huang M. F. (2015). The relationship between major upwelling and the upwelling fishing grounds in the south China Sea. Mar. Sci. 09, 131–137. doi: 10.11759/hykx20130627001
Wang Z. H., Zhang S. Y., Wang K. (2010). Fish and macroinvertebrates community structure in artificial habitat around sanheng isle, shengsi, China. Acta Ecol. Sin. 08, 2026–2035.
Wang J., Zhang Y., Yuan X. Z., Chen J. F. (2012). Water quality condition and its seasonal variation of the artificial reef waters in shuangdao bay. J. Hydroecol. 6, 90–95. doi: 10.15928/j.1674-3075.2012.06.017
Williams-Grove L. J., Szedlmayer S. T. (2017). Depth preferences and three-dimensional movements of red snapper, lutjanus campechanus, on an artificial reef in the northern gulf of Mexico. Fish. Res. 190, 61–70. doi: 10.1016/j.fishres.2017.01.003
Yuan H. R., Chen P. M., Li H. Q., Li X. G., Tang Z. Z., Qin C. X., et al. (2011). Preliminary evaluation on resources multiplication of artificial reef in wushi leizhou. J. Shanghai Ocean Univ. 6, 883–889.
Yuan X. N., Liang Z. L., Lv Z. B., Wang Y. L., Sun X. H. (2017). The effects of ecological restoration of the artificial reef in weihai offshore. Acta Oceanol. Sin. 10, 54–64. doi: 10.3969/j.issn.0253-4193.2017.10.005
Yu J., Chen P. M., Tang D. L., Qin C. X. (2015). Ecological effects of artificial reefs in daya bay of China observed from satellite and in situ measurements. Adv. Space Res. 09, 2315–2324. doi: 10.1016/j.asr.2015.02.001
Zhang J. J., Gurkan Z., Jorgensen S. E. (2010). Application of eco-exergy for assessment of ecosystem health and development of structurally dynamic models. Ecol. Modell. 4, 693–702. doi: 10.1016/j.ecolmodel.2009.10.017
Zhang S. Y., Zhang H. J., Jiao J. P., Li Y. S., Zhu K. W. (2006). Change of ecological environment of artificial reef waters in haizhou bay. J. Fish. China. 4, 475–480. doi: 10.3321/j.issn:1000-0615.2006.04.007
Zhang S. Y., Zhou X. J., Wang G. K., Lin J., Zhao J., Zhao X., et al. (2019). Review of marine livestock ecological urbanization hypothesis and marine ranching construction key-technology against blue growth background. J. Fish. China. 01, 81–96. doi: 10.11964/jfc.20180211193
Keywords: artificial reef, eco-exergy, fishery development, nekton community, sustainable fishery
Citation: Yuan H, Chen P and Li X (2022) Taxonomic diversity and eco-exergy changes in fishery resources associated with artificial reefs over 14 years in Daya Bay, China. Front. Mar. Sci. 9:1054933. doi: 10.3389/fmars.2022.1054933
Received: 27 September 2022; Accepted: 16 November 2022;
Published: 01 December 2022.
Edited by:
Anning Suo, South China Sea Institute of Oceanology, Chinese Academy of Sciences (CAS), ChinaReviewed by:
Ilana Rosental Zalmon, State University of the North Fluminense Darcy Ribeiro, BrazilThadickal V. Joydas, King Fahd University of Petroleum and Minerals, Saudi Arabia
Copyright © 2022 Yuan, Chen and Li. This is an open-access article distributed under the terms of the Creative Commons Attribution License (CC BY). The use, distribution or reproduction in other forums is permitted, provided the original author(s) and the copyright owner(s) are credited and that the original publication in this journal is cited, in accordance with accepted academic practice. No use, distribution or reproduction is permitted which does not comply with these terms.
*Correspondence: Pimao Chen, Y2hlbnBtQHNjc2ZyaS5hYy5jbg==