- 1National Engineering Research Center of Marine Facilities Aquaculture, Marine Science and Technology College, Zhejiang Ocean University, Zhoushan, China
- 2Institute for Environmental Protection and Research, ISPRA, Livorno, Italy
- 3School of Life Science, Nantong University, Nantong, China
ATP-binding cassette (ABC) transporters play an essential role in xenobiotic resistance through transporting xenobiotics into or out of cells. However, their functional role in mollusk's defense against polycyclic aromatic hydrocarbons (PAHs) remains unclear. In the present study, novel McoABCB1 and McoABCC transporters were identified in the gills of Mytilus coruscus, and their benzo(α)pyrene (Bap) detoxification function was determined by qRT-PCR and efflux assays. McoABCB1 and McoABCC genes were constitutively expressed in all tissue types, suggesting the primary physiological function of these transporters. Bap, a chemical stress agent, could induce the mRNA expression of McoABCB1 and McoABCC in gills. However, at high concentration, Bap inhibited their expression levels at the late stage. Besides, the efflux activities in M. coruscus gills showed a dose-dependent elevation in a Bap pre-exposure group, compared with those in a reversin 205 or MK571 treatment alone group. These results demonstrated the presence of ABCB1 and ABCC transporters in Mytilus coruscus, and their involvement in cellular Bap detoxification.
Introduction
Aquatic organisms are under increasing environmental stress caused by the multiple natural and anthropogenic sources of water pollution. Accumulating evidence indicates that these organisms have developed a multixenobiotic resistance (MXR) system, which can eliminate endogenous and exogenous toxic products and metals during evolution (Kurelec, 1992; Bard, 2000). MXR is a powerful cellular defense mechanism that effluxes a number of xenobiotics and/or their conjugate metabolites, representing an important first line of defense (Smital and Kurelec, 1998). MXR is mediated by transmembrane proteins known as ATP-binding cassette (ABC) transporters, which are mainly responsible for the energy dependent, ATP-driven transport of endogenous and exogenous substrates across plasma membranes (Dean, 2005; Szakàcs et al., 2008). ABC transporters belong to a transmembrane protein superfamily found in both prokaryotes and eukaryotes (Haimeur et al., 2004), which can be sub-categorized into either eight subfamilies (A-H) (Dean et al., 2001) or seven subfamilies (A-G) (Klein et al., 1999), based on their structural and functional categories. Eukaryotic ABC transporters are further divided into two groups: half and full transporters. Half transporters possess one hydrophobic transmembrane domain (TMD) and one nucleotide-binding domain (NBD) and generate a functional pump by forming homo- or heterodimers, while full transporters consist of two TMDs and two NBDS in which ATP hydrolysis occurs (Biemans-Oldehinkel et al., 2006; Hollenstein et al., 2007; Oldham et al., 2008). It is recognized that the major ABC transporters involved in the efflux of toxic substrates are P-glycoprotein (Pgp/MDR1/ABCB1, from ABCB subfamily), multidrug resistance associated proteins 1-5 (MRP1-5/ABCC1-5, from ABCC subfamily), and breast cancer resistance proteins (BCRP/ABCG2, from ABCG subfamily) (Leslie et al., 2005). ABCB1 mainly participates in the extrusion of unmodified exogenous compounds, whereas the substrates of ABCC1-5 and ABCG2 transporters are mostly glucuronide- glutathione- and sulfate-conjugated metabolites (Haimeur et al., 2004).
Bivalve molluscan gills are located at the interface between the body and the external environment and are constantly exposed to xenobiotics in polluted waters, hence representing the first defense system barrier (Luckenbach and Epel, 2008; Luckenbach et al., 2008). Numerous toxicological studies have been focused on the gills of bivalve molluscan. To date, the presence of ABCB and ABCC transporters in gills has been demonstrated in different species of bivalve molluscs, including Mytilus edulis (Lüdeking and Köhler, 2002, 2004), Saccostrea forskali (Kingtong et al., 2007), Mytilus californianus (Luckenbach and Epel, 2008; Luckenbach et al., 2008), Dreissena polymorpha (Faria et al., 2011), Ruditapes philippinarum (Liu et al., 2014), Chlamys farreri (Miao et al., 2014), Prorocentrum lima (Huang et al., 2015), and Mytilus galloprovincialis (Franzellitti et al., 2016). In these bivalve species, ABC efflux transporters play an important role in the selective permeability for chemicals in gills, particularly interfering with xenobiotic infiltration (Faria et al., 2011).
However, there is scarce information on the activated ABC transporters associated with a protective mechanism against polycyclic aromatic hydrocarbons (PAHs), including benzo(α)pyrene (Bap) (Haritash and Kaushik, 2009). PAHs exhibit possible carcinogenicity and mutagenicity, and are involved in oxidative stress production, growth retardation, locomotor impairment, and reproductive health problems. Bap, as a representative compound of PAHs, has been widely used in laboratory ecotoxicological studies in recent years (Juhasz and Naidu, 2000). After exposure to Bap, ABCB1 was downregulated in the gills of C. farreri, while its correspondent was significantly upregulated in those of Ruditapes philippinarum (Liu et al., 2014; Miao et al., 2014). These results, and some other reports, have demonstrated that ABC transporters exert defense mechanisms against Bap exposure, varying with different isoforms, localizations, and even species. For instance, inhibition studies using the chemical inhibitors of ABCB1 and ABCCs showed that Bap did not exhibit any effect on the efflux activities of ABCB1 and ABCC2 in human intestinal Caco-2 cells (Buesen et al., 2002). However, in the fish Nile tilapia, the transcriptional expression levels of ABCC2 in gills as well as those of ABCG2 in the liver and proximal intestine were remarkably up-regulated following 14 days of Bap exposure through water. However, the mRNA levels of ABCB1b and ABCC1 were not significantly changed after exposed to a BAP-containing diet and water (Costa et al., 2012). In the fish Gobiocypris rarus, Bap significantly induced the transcriptional expression levels of three ABC transporters (i.e., ABCC1, ABCC2, and ABCG2) in a dose-dependent manner, while demonstrated no apparent effect on ABCB1 mRNA expression (Yuan et al., 2014). Collectively, ABC transporters have a highly complex detoxifying response to Bap exposure, and the available data are still confusing, especially in mollusks, where the relevant information is very scarce and requires further investigation.
The thick shell mussel Mytilus coruscus, is primarily found in the Chinese Yellow Sea, Japan's Hokkaido coastal regions, and the Korean Peninsula (Xu et al., 2019). Given its high nutritional value, M. coruscus is recognized as one of the most economically significant mariculture species in Zhoushan, Zhejiang, China. The port of Zhoushan-Ningbo is the most active in the world and faces the challenge of frequent oil spills resulting in PAH pollution in local marine water, which has become a serious threat to local mussel culture. Thus, it is relatively critical to perform toxicology research on M. coruscus resistance mechanisms against PAH pollution. Furthermore, M. coruscus is considered a model organism for assessing the biological impacts of marine environmental factors across China's coastal regions, due to its sedentary and filter feeding characteristics (Wang et al., 2015; Liu et al., 2016). In the present study, identification of ABC transporter homologs in M. coruscus, followed by their transcriptional response to Bap exposure, as well as their efflux activities altered by pharmacological inhibitors and Bap, were investigated. This work aims to contribute to a better understanding of the detoxification mechanisms of PAH exposure in M. coruscus via ABC transporters.
Materials and Methods
Chemicals
The fluorescent dye calcein-AM (Ca-AM) was used as a substrate of both ABCB and ABCC (Holl et al., 1996). Reversin 205 (Sharom et al., 1999) and MK571 (Gekeler et al., 1995) were chosen as a specific inhibitor for ABCB1 and ABCC, respectively. Ca-AM was purchased from Solarbio, while Reversin 205, MK571, Bap, and other reagents were supplied by Sigma-Aldrich.
Animals
Mytilus coruscus adults (8.8 ± 0.6 cm shell length) were supplied by Donghe market (Zhoushan, Zhejiang Province, China) and maintained in tanks filled with artificial sterile seawater (ASW) at 25 ± 1°C and 30%0 salinity for 7 days. The ASW was prepared in accordance with the ASTM procedure E 724-98 (ASTM, 2004), and daily renewed.
Bap Exposure and Tissue Sample Collection
Bap was first dissolved in dimethyl sulfoxide (DMSO), and then mixed with ASW to reach a final DMSO concentration of 0.01% (v/v) and different Bap concentrations of 5, 50, and 100 μg/L. The control group was treated with 0.01% (v/v) DMSO alone under similar conditions. The selected Bap conservation in this experiment was performed according to Di et al. (2011) and represented at par with the low, medium, and high concentrations. The concentrations of Bap in water samples were determined by GC-MS method according to Di et al. (2011, 2017). Briefly, an Agilent 5975 GC-MS system was calibrated with commercial Bap standards to generate a standard curve. Then, 500 mL of water samples were extracted with dichloromethane, and the concentrated solution was transferred to glass microvials for GC-MS analysis. Lastly, Bap concentration was determined by comparing with the standard curve. Upon the exposure to 5, 50, and 100 μg/L of Bap, their actual concentrations in water samples were determined to be 4.28, 42.52, and 89.38 μg/L, respectively. Before renewing the ASW at 24 h, the amounts of Bap in the three treatment groups were 1.14, 17.26, and 45.70 μg/L. Meanwhile, Bap was not detectable at either times in the water samples of the control group.
A total of 240 mussels were randomly divided into 3 groups, with 20 replicates in each group. Three mussels were sampled from each replicate at 0, 1, 6, and 12 days post exposure (dpe). Then, their gills were dissected and pooled together to alleviate the individual variability and analyzed as one sample. The samples were kept at −80°C for RNA extraction. Half of the samples in the Bap treatment group at 1 dpe were stored in ASW for efflux assays.
To assess the tissue distribution of ABC transporters, five tissue types, such as gills, gonads (GO), digestive glands (DI), adductor muscle (AD), and mantles (MA), were dissected from 8 healthy adult mussels. The hemocytes (HA) were also withdrawn, and the procedure was conducted according to our previous report (Dong et al., 2017).
RNA Isolation, cDNA Synthesis, and Gene Cloning
Total RNA was extracted by the Trizol reagent (Invitrogen), and its quality and concentration were assessed using a Nanodrop spectrophotometer (Thermo, USA). cDNA synthesis was performed using the M-MLV reverse transcriptase (Promega, USA) containing an oligo-d(T) primer, according to the manufacturer's instructions. The open reading frame (ORF) sequences of two ABC transporter orthologs (termed as McoABCB1 and McoABCC) were acquired by searching through M. coruscus transcriptional database (Dong et al., 2017), and two pairs of specific primers (Table 1) were designed for amplifying the ORF sequences. To amplify the 5′ and 3′ untranslated regions (UTRs) with adaptor specific primers (Table 1), the rapid amplification of cDNA ends (RACE) was carried out by a RACE cDNA amplification kit (Life Technologies, USA) according to the manufacturer's instructions. The cDNA from gills was used as a template.
Sequence Analysis of McoABCB1 and McoABCC
The obtained cDNA sequences of McoABCB1 and McoABCC were analyzed using the Basic Local Alignment Search Tool (BLAST), their conserved domains were predicted by the SMART online tool (http://smart.embl-heidelberg.de/smart/set_mode.cgi), and their physicochemical properties were assessed with the ProtParam tool (http://www.expasy.org). To investigate the genetic relationship between the two newly identified molecules and other known ABC transporters, the complete amino acid sequences of these ABC transporters were retrieved from Genbank, and then aligned using Clustalx software. Subsequently, the aligned data were evaluated by a neighbor-joining approach using MEGA X with 5000 replications of bootstrap (Kumar et al., 2018).
Quantitative Reverse Transcription PCR (qRT-PCR)
A total of 10 μL qRT-PCR mixture was prepared by adding 5 μL 2 × SYBR® Premix Ex Taq™ II (TaKaRa), 0.2 μL ROX II, 0.4 μL forward and reverse primers (Table 1), 0.4 μL cDNA sample (100 ng/μL), and 3.6 μL ddH2O. Quantitative reverse transcription PCR (qRT-PCR) was conducted on a 7500 Real Time PCR System (Applied Biosystems, USA). The relative expression level of each target gene was determined using the 2−ΔΔCt method. β-actin was adopted as the housekeeping gene (Livak and Schmittgen, 2001).
Efflux Assays
Efflux assay was performed based on the capability of ABC transporters to transport specific fluorescent substrates. When ABC transporters are active, the fluorescent substrates are released from the cells, and result in a low fluorescence level in the cells/tissues. Conversely, if the efflux activities are disrupted by transporter inhibiting chemicals, substrates may be accumulated within the cells, and a high fluorescence level is observed in the cells/tissues. In the present study, Ca-AM was used as a fluorescent substance, while Reversin 205 and MK571 were chosen as the specific inhibitors for ABCB and ABCC, respectively. Efflux assays was carried out using gill samples based on the methods published by Luckenbach and Epel (2008) as well as Navarro et al. (2012), with slight modifications. Gill tissues were evenly dissected from the mussels treated with and without Bap, and then incubated separately in 1 mL ASW with 0.5 μM Ca-AM and 10 μM reversin 205 or MK571 at 20°C for 90 min. After incubation, the biopsies were washed with ASW to remove the medium. Then, a microbalance was used to measure the weight of tissue pieces, prior to sonication in hypotonic lysis buffer (10 mM KCl, 10 mM Tris, 1.5 mM MgCl2; pH ~7.4) for 20 s. After centrifuging for 10 min at 8,000 rpm, the supernatant was placed into a 96-well black microplate. The fluorescence intensity of Ca-AM was determined with a Leica TCS SP5 II laser scanning microscopy (Germany) under an excitation/emission wavelength of 480 nm/530 nm. The fluorescence values were presented as fluorescence units per mg of fresh gill tissue weight. All data were expressed as the fold changes in fluorescence intensities relative to the control group (DMSO).
Statistical Analysis
SPSS 17.0 software was employed to perform the statistical analyses. qRT-PCR data were analyzed with a two-way analysis of variance (ANOVA) followed by Tukey's range test. Prior to analysis, fluorescence intensities were log10-transformed to meet the assumptions of normality and homoscedasticity of ANOVA. One-way ANOVA with Fisher's Least Significant Difference (LSD) post-hoc test were used to analyze the normally distributed data, while the non-normally distributed data were analyzed by the Kruskal-Wallis test and Mann-Whitney U test (Chen et al., 2018). Statistical difference was deemed significant at a level of P < 0.05.
Results
Cloning and Molecular Characterization of McoABCB1 and McoABCC
Two novel transporter orthologs McoABCB1 (accession number: MK967812) and McoABCC (accession number: MK967813) were first cloned from M. coruscus for the complete cDNA sequences. The full-length cDNA of McoABCB1 contained 4,547 bp nucleotide residues with 4,092 bp ORF sequence encoding a polypeptide of 1,363 amino acid residues. The McoABCC cDNA contained 4,678 bp nucleotide residues with an ORF sequence of 4,497 bp encoding a putative protein of 1,498 amino acid residues. The molecular masses of McoABCB1 and McoABCC were calculated to be 149.9 and 169.3 kDa, and their isoelectric points were 6.22 and 6.52, respectively. A homologous sequence comparison revealed that McoABCB1 and McoABCC exhibited the highest identity of 97.01 and 97.47, respectively, with their respective counterparts in M. galloprovincialis, a close relative of M. coruscus.
Through analysis of the functional domain with SMART, McoABCB1 and McoABCC were found to comprise two subunits, each with a transmembrane-spanning domain (MSD/ABC_membrane) and a nucleotide-binding domain (NBD/AAA) (Figure 1). In addition, McoABCC possessed five transmembrane regions in the N-terminate (Figure 1). The NBDs of McoABCB1 and McoABCC contained typical motifs conserved in mammalian ABC transporters: Walker A and B, ABC signature, D-, H-, and Q-loops (Figure 2).
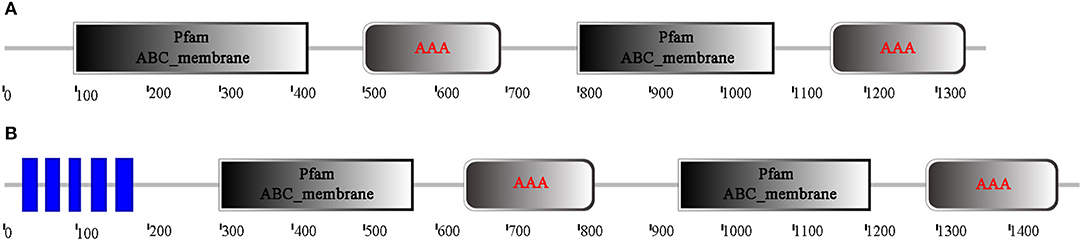
Figure 1. Domain architecture analysis of the deduced amino acid sequences of McoABCB1 (A) and McoABCC (B). McoABCB1 and McoABCC are comprised of two subunits, each with a transmembrane-spanning domain (MSD/ABC_membrane), and a nucleotide-binding domain (NBD/AAA). In addition, McoABCC possesses five transmembrane regions in the N-terminate. (For interpretation of the references to color in this figure legend, the reader is referred to the web version of this article).
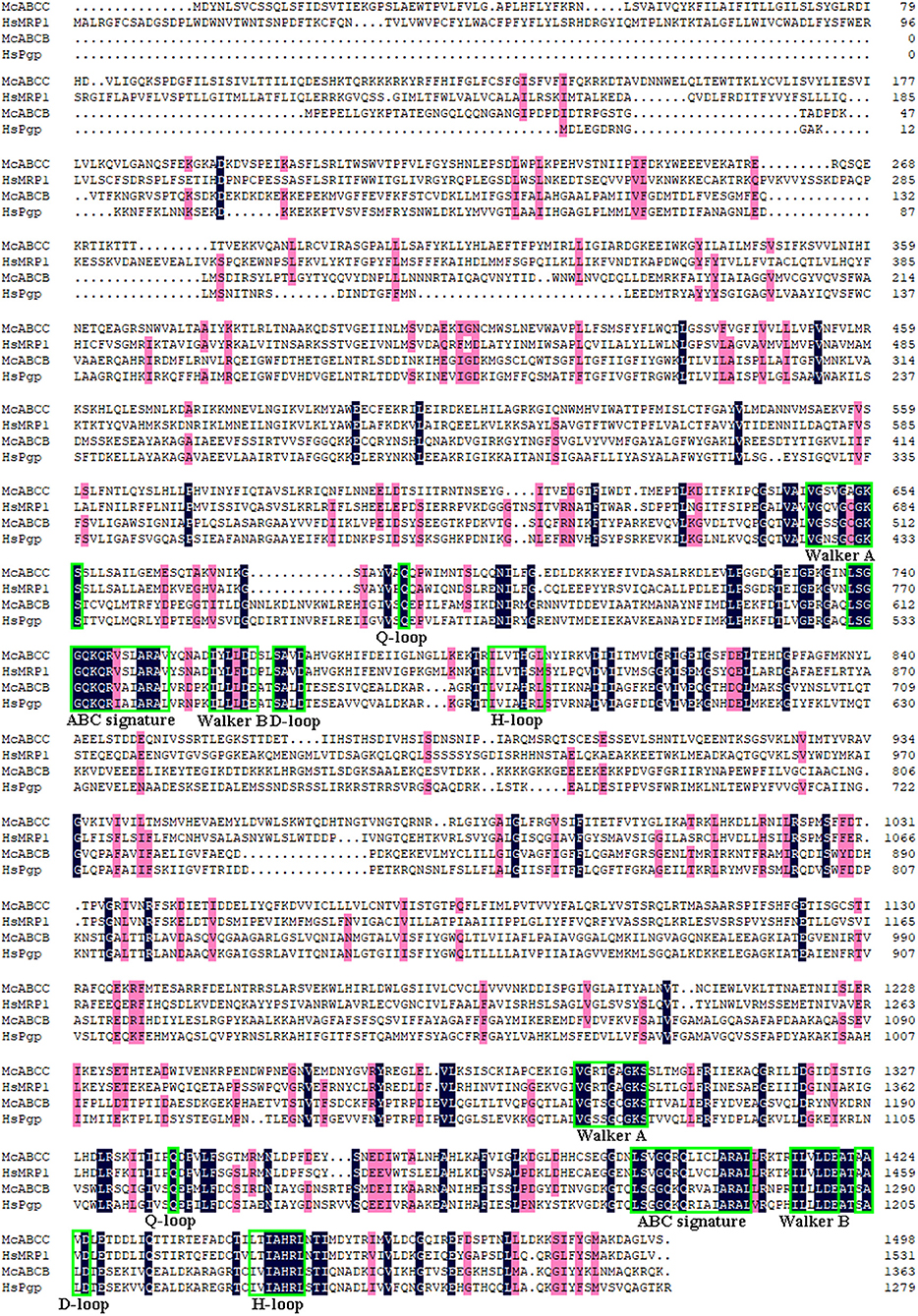
Figure 2. Alignments of McoABCB1 and McoABCC with their correspondents from human (HsABCB1, GenBank accession no. AAI30425.1 and HsABCC, GenBank accession no. ABD72213.1). Identical amino acid residues are represented by dark blue color, while pink color indicates the similarity surpass 75 %. The nucleotide-binding Walker A and B motifs, ABC signature motifs, D-, H- and Q-loops in the sequences are denoted by green rectangle (For interpretation of the references to color in this figure legend, the reader is referred to the web version of this article).
Furthermore, two well-defined branches were demonstrated in the phylogenetic tree, representing ABCB and ABCC transporter families (Figure 3). McoABCC was related to its counterparts from other Mytilus species, i.e., M. galloprovincialis and M. californianus, which clustered into the ABCC family clade (Figure 3). However, in the ABCB branch, M. californianus ABCB1 did not group with Mytilus ABCB1 molecules from M. galloprovincialis and M. coruscus but gathered two other bivalve ABCB1 molecules from Tegillarca granosa and Anadara sativa into one distinct cluster, which distributed on the outermost side of ABCB branch.
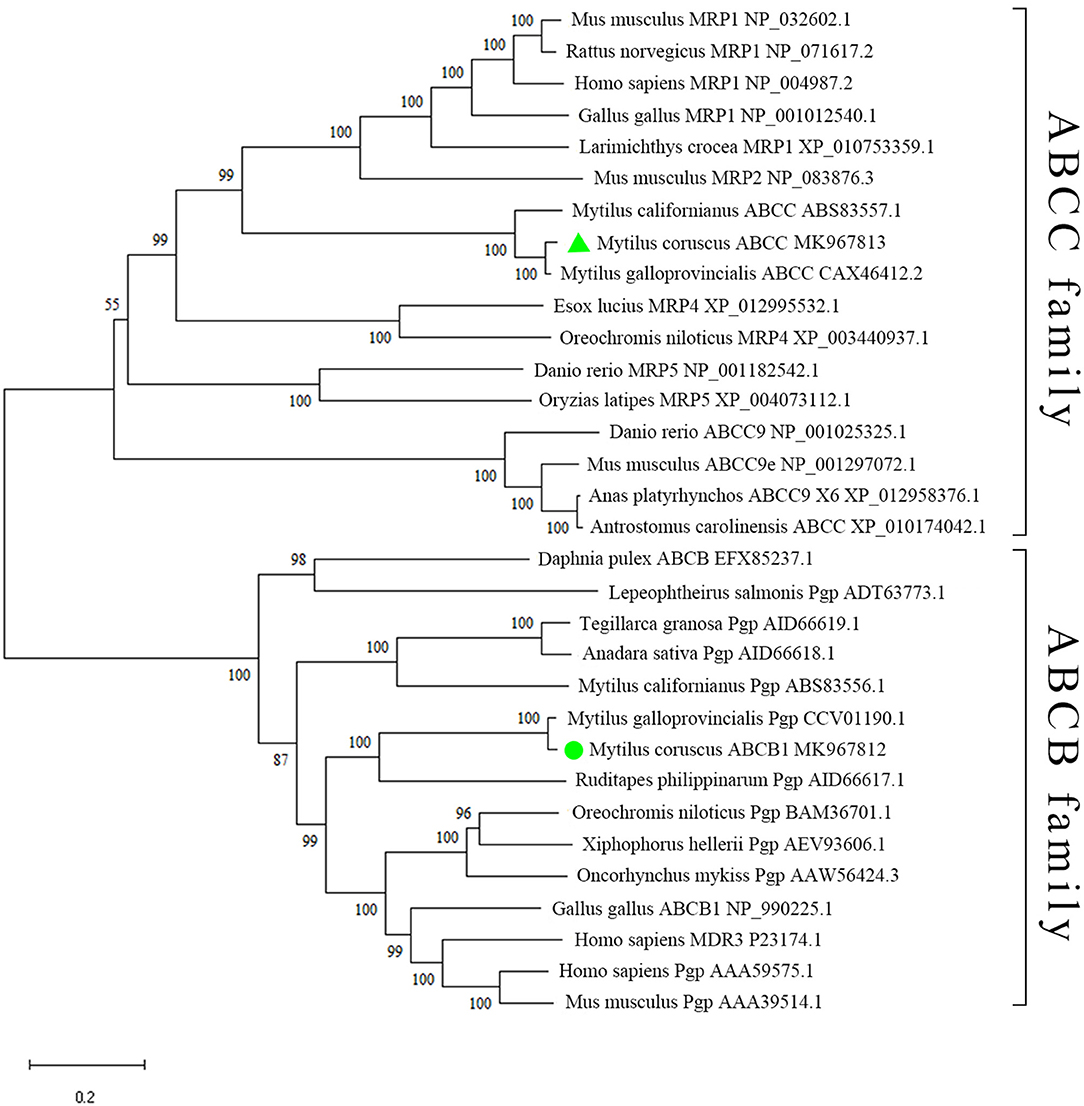
Figure 3. Phylogenetic analysis of McoABCB1 and McoABCC. McoABCB1 and McoABCC are denoted by green circle and green triangle, respectively. The species included in our phylogenetic tree are obtained from Genbank database, and their accession numbers are displayed on the tree (For interpretation of the references to color in this figure legend, the reader is referred to the web version of this article).
McoABCB1 and McoABCC Tissue Distribution
As shown in Figure 4, McoABCB1 and McoABCC transporters were detected in all tissue types. Notably, significant expression levels were observed in DI and gills, followed by HA, AD, and GO, with the lowest expression level found in MA.
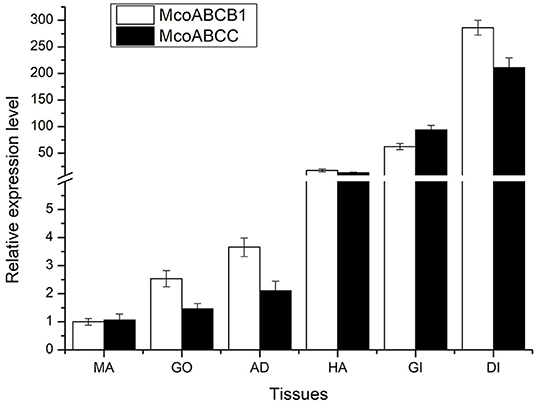
Figure 4. Tissue distribution of McoABCB1 and McoABCC transcripts. The transcriptional expression levels of McoABCB1 and McoABCC are detected in various types, such as gills, GO, DI, AD, MA, and HA, by using qRT-PCR assay. Data are presented as mean ± S.D (n = 8 per group), and β-actin was used as a reference gene.
McoABCB1 and McoABCC Expression in Response to Bap Exposure
After exposure to 100 μg/L Bap, the transcriptional expression levels of McoABCB1 and McoABCC were significantly induced at 1 dpe, followed by a sharp inhibition, and reached significantly decreased levels at 6 and 12 dpe for McoABCB1 and, at 12 dpe for McoABCC, as compared to the control group (Figures 5A,B). Following 50 μg/L Bap exposure, the mRNA expression levels of the two ABC transporters were significantly up-regulated at 1 and 6 dpe, while decreased to control levels at 12 dpe (Figures 5A,B). Furthermore, 5 μg/L Bap exposure increased the transcriptional expression levels of McoABCB1 and McoABCC in a time-response manner and reached the highest expression level at 12 dpe (Figures 5A,B).
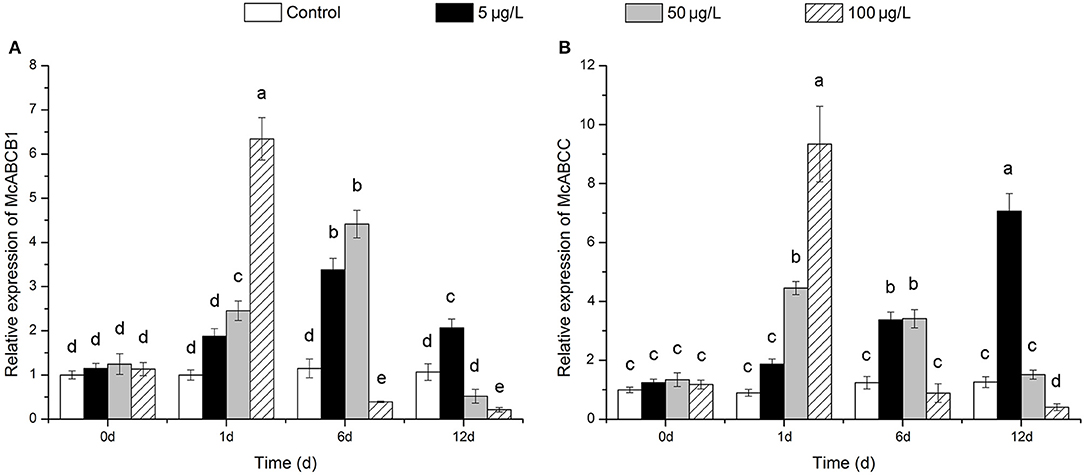
Figure 5. Expression profiles of McoABCB1 (A) and McoABCC (B) in response to Bap exposure in gills. Data are presented as mean ± S.D (n = 3 per group). Different letters indicate significant differences (P < 0.05).
Effects of Bap, reversin 205, and MK571 on Efflux Activity
As shown in Figure 6, Bap reduced the effects of reversin 205 (ABCB1 inhibitor) and MK571 (ABCC inhibitor) in a dose-dependent manner, with the highest effect achieved at 100 μg/L (Figures 6A,B). In addition, after pre-exposure to 50 μg/L Bap, the efflux activity was not significantly altered in reversin 205 group, but it was markedly reduced in MK571 group (Figures 6A,B).
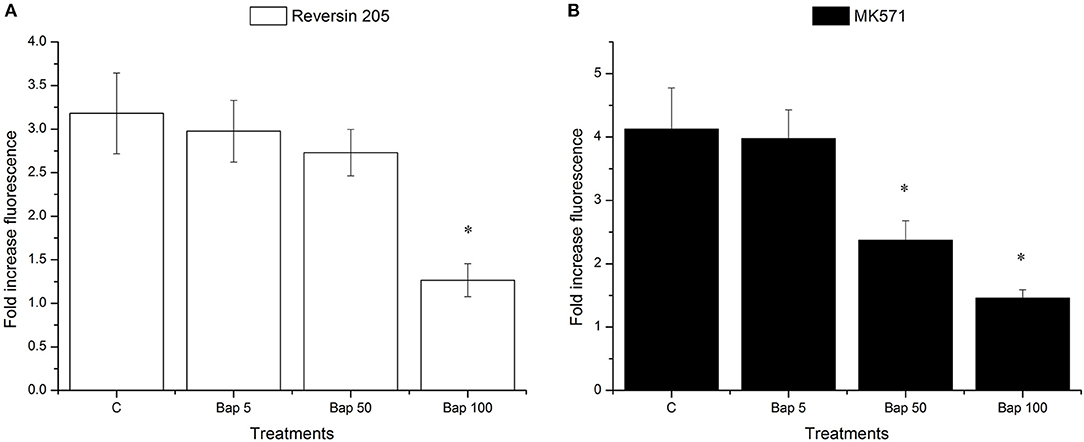
Figure 6. Fold changes in the Ca-AM fluorescence intensities of M. coruscus gills after treatment with ABCB1 inhibitor reversin 205 (A) or ABCC inhibitor MK571 (B). Data are presented as mean ± S.D (n = 3 per group). Each bar represents the fold increase of fluorescence level compared to control group. Asterisks indicate the significant differences in fluorescence intensities between control and treatment groups (*P < 0.05).
Discussion
ABC proteins transport a broad range of toxic substrates by effluxing them out from the cells through an ATP-driven process. Hence, they play an effective role in the detoxification process. Despite their functional significance, the information on ABC transporters in molluscan species is still scarce; in particular, there remains a substantial lack of genetic data regarding the role of ABC transporters in response to PAHs (a large class of organic pollutants in marine water). The aim of this research was to identify the presence of ABC transporters in M. coruscus and evaluate their protective effects on Bap exposure by assessing their transcriptional expression levels and efflux activities in gills.
In the present study, two novel M. coruscus ABC transporters, namely, McoABCB1 and McoABCC, were identified. It has been generally recognized that ABC transporters consist of two subunits, each with a membrane-spanning domain (MSD) and a nucleotide-binding domain (NBD). In NBDs, some typical and highly conserved motifs, such as ABC signatures, Walker A and Walker B, are detected (Ambudkar et al., 2006). The two newly identified ABC transporters contained two subunits, each with an MSD and an NBD. In addition, the conserved functional domains and sites, including Walker A and B, ABC signature, D-, H-, and Q-loops, were identified in McoABCB1 and McoABCC. The results of Blastn showed that McoABCB1 and McoABCC shared the highest sequence identity with their specific counterpart in M. galloprovincialis, suggesting that ABCB1 and ABCCs from the same genus are close relatives. This was further verified by phylogenetic analysis, showing that McoABCB1 initially grouped with MgABCB1 and then clustered into the ABCB family, whereas McoABCC originally grouped with MgABCC and McABCC and then clustered into the ABCC family. Taken together, these results demonstrate that the novel McoABCB1 and McoABCC belong to the ABC transporter family, and may provide a cellular defense against toxicants, as similar to their counterparts in other animals.
Tissue distribution of McoABCB1 and McoABCC transcripts displayed constitutive expression profiles, with the highest expression levels detected in DI and gills. The DI of mussels contains numerous blind-ending epithelial tubules, which are comprised primarily of basophilic and digestive cells (Faggio et al., 2018). The digestive cell type exhibits a well-established endo-lysosomal vacuolar system, such as heterolysosomes, heterophagosomes, and residual bodies, which takes part during the process of intra-cellular digestion (Dimitriadis et al., 2004). Additionally, the lysosomes of digestive cells are not only involved in the intra-cellular digestion of nutrients and antioxidant defenses (Faggio et al., 2016), but also regarded as an important organelle of organic pollutant and metal sequestration and detoxification (Marigómez et al., 2002; Pagano et al., 2016). Besides, the gills of filter-feeding bivalves are generally considered one of the major target organs for accumulating waterborne pollutants, either dissolved in or bound to particulate matter. In the scallop C. farreri, Cf ABCB1 was also highly expressed in DI and gills (Miao et al., 2014). These findings confirmed the existence of ABC transporters in M. coruscus, and these molecules could exert detoxification and antioxidant effects on stress resistance, similar to their counterparts in other animals.
PAHs combine with the aryl hydrocarbon receptor to form receptor-PAH complexes, which translocate into the nucleus and bind to xenobiotic response elements, thus resulting in the up-regulated or down-regulated expression of detoxification genes (Puga et al., 2002). Besides, PAHs can be directly eliminated from cells through multi-xenobiotic resistance proteins (MXRP), including ABCB1 and ABCCs (Xu et al., 2005). The expression levels of ABCB1 expression in some aquatic animals, such as fish [e.g., N. tilapia (Costa et al., 2013) and catfish (Doi et al., 2001)] and bivalves [e.g., C. farreri (Miao et al., 2014; Cai et al., 2016) and M. edulis (Prevodnik et al., 2007)] were remarkably up-regulated in response to Bap exposure. Similarly, a significant high expression of ABCB1 induced by Bap was observed in M. coruscus, suggesting that ABCB1 may be responsible for the transport of excess Bap in M. coruscus. Unfortunately, there are only a few available studies on the role of ABCC in PAHs detoxification in mollusks for establishing comparisons. In the present study, a significant induction of McoABCC was also observed, suggesting that it possibly takes part in Bap scavenging, as ABCB1 does (Costa et al., 2012; Yuan et al., 2014; Kim et al., 2017). Notably, in 100 μg/L Bap group, the mRNA expression levels of McoABCB1 and McoABCC were significantly induced at 1 dpe, sharply decreased over time, and ultimately reached a lower level compared to control group. On the first day of Bap exposure, the ABC transporter system was primarily triggered to eliminate Bap and/or its metabolites from the cells. The detoxification of PAHs is a complex process involving the ABC transporter system, as well as the AhR pathway, phase I, and phase II xenobiotic-metabolizing enzymes (Hall and Grover, 1990; Cavalieri and Rogan, 1992; Penning et al., 1999; Xue and Warshawsky, 2005). With longer exposure time, intracellular Bap concentration can surpass the detoxification potential of ABC transporters, which in turn leads to the loss of transcriptional activity and increased risk of cell damage.
ABC transporter activities induced by environmental stressors can be measured in whole body or through cell-based assay, using fluorescence dyes and inhibitors (Luckenbach et al., 2014; Jeong, 2017). In these assays, the efflux activity of ABC transporters in response to environmental pollutants can be indirectly measured with or without the efflux transporter-specific inhibitors. Specifically, the transport kinetics of fluorescence dyes can be interfered by the addition of specific substrates, leading to an increase in the fluorescence signals of the whole body and/or cells (Jeong, 2017). Here, we focused on the accumulation of fluorescent Ca-AM in M. coruscus gills pre-exposed to Bap followed by treatment with ABC transporter inhibitors. Reversin 205 and MK571 were selected as ABCB1 and ABCC specific inhibitors, respectively. At the previously reported concentration of 10 μM, reversin 205 and MK571 in gills can accumulate Ca-AM within the dynamic range of the dose–response curves (ABC transporter inhibitor concentrations vs. Ca-AM fluorescence intensities) (Navarro et al., 2012). Therefore, the changes in ABC transporter activities can be interpreted as the ratio of Ca-AM fluorescence intensity in the presence and absence of transporter inhibitor (Navarro et al., 2012).
Our data indicated that the efflux activities in M. coruscus gills showed a dose-dependent elevation in the Bap pre-exposure group compared to the reversin 205 and MK571 exposure alone groups, suggesting that Bap can interact with ABC transporters and reduce the inhibitor activities. In this regard, Bap has been demonstrated to be a target of the MDR efflux pump in human hepatocytes (Fardel et al., 1996). Bamdad et al. (1999) performed a flow cytometric assay to investigate the accumulation of Bap in Tetrahymena pyriformis in the presence of verapamil, which is known to be a model inhibitor for ABCB1. Notably, a higher accumulation of Bap was found, suggesting that MDR pumps may promote cellular resistance to PAH toxicity (Bamdad et al., 1999). Contrary to our results, an enhanced accumulation of Ca-AM was observed in marine ciliate Euplotes crassus exposed to Bap, indicating the inhibition of Bap-mediated efflux activity. It is worth noting that the metabolic mechanisms of Bap can be varied in different organisms; mussels may have evolved a more complicated metabolic mechanism in response to Bap than ciliate. In other words, there may be other mechanisms responsible for the accumulation of Bap in mussels, apart from ABC transporters. Furthermore, Bap exposure intensity, such as concentration and duration, may exhibit certain effects on efflux activity. The Bap exposure intensity used in our study was within the range of ABC transporter strength, in order to ensure that these transporters can function properly to transport the substrate out of the cells. However, in previous studies, ABC transporter activity was exceeded, and the prolonged exposure could damage these molecules, thus resulting in substrate accumulation. This was also evidenced by the aforementioned qRT-PCR results, in which the expression levels of McoABCB1 and McoABCC were decreased with increasing exposure time, which suggests the inhibition of ABC transporter expression by Bap exposure.
Conclusions
In the present study, two novel ABC transporters, namely, McoABCB1 and McoABCC, were initially identified in M. coruscus, and their response to Bap in gills were subsequently measured by qRT-PCR and efflux assays. The conserved architecture and close phylogenetic relationship with other ABC transporters indicated that these two newly obtained genes belonged to the ABC superfamily. The transcriptional expression levels of these two transporters were significantly up-regulated in all Bap treatment groups, but down-regulation was observed at higher Bap doses and after prolonged exposure. Pre-treatment of Bap followed by the addition of ABC transporter inhibitors in mussels could lead to a dose-dependent up-regulation of efflux activities in gills, when compared to reversin 205 or MK571 exposure alone. Taken together, our results suggest that these two newly identified ABC transporters are involved in the cellular responses to Bap exposure and may play a detoxification role. In addition, the high-dose exposure to Bap could produce signs of toxicity, thus damaging the detoxification system in mussel cells. These findings shed novel light on the detoxification mechanisms of molluscan ABC transporters in response to PAHs.
Data Availability Statement
The datasets generated for this study can be found in NCBI GenBank, NCBI Accession No.'s MK967812 and MK967813.
Author Contributions
BG was responsible for the efflux assays and prepared the paper. ZX was responsible for the ABC transporters identification and characterization. XY provide the funding. JL provided useful suggestions to this research. CZ and IB reviewed and modified the paper. PQ responsible for the project administration.
Funding
This research was financially supported by the National Natural Science Foundation of China (41976111), the Special Fund for the Key Research and Development Project of Zhejiang Province (2019C02056), and Zhoushan Science and Technology Planning Project (2019F12004).
Conflict of Interest
The authors declare that the research was conducted in the absence of any commercial or financial relationships that could be construed as a potential conflict of interest.
References
Ambudkar, S. V., Kim, I. W., and Sauna, Z.E. (2006). The power of the pump: mechanisms of action of P-glycoprotein (ABCB1). Eur. J. Pharma. Sci. 27, 392–400. doi: 10.1016/j.ejps.2005.10.010
ASTM (2004). “ASTM,” in Standard Guide for Conducting Static Acute Toxicity Tests Starting With Embryos of Four Species of Saltwater Bivalve Molluscs, E724–E798.
Bamdad, M., Brousseau, P., and Denizeau, F. (1999). Identification of a multidrug resistance-like system in Tetrahymena pyriformis: evidence for a new detoxification mechanism in freshwater ciliates. FEBS. Lett. 456, 389–393. doi: 10.1016/S0014-5793(99)00978-3
Bard, S. M. (2000). Multixenobiotic resistance as a cellular defense mechanism in aquatic organisms. Aquat. Toxicol. 48, 357–389. doi: 10.1016/S0166-445X(00)00088-6
Biemans-Oldehinkel, E., Doeven, M. K., and Poolman, B. (2006). ABC transporter architecture and regulatory roles of accessory domains. FEBS. Lett. 580, 1023–1035. doi: 10.1016/j.febslet.2005.11.079
Buesen, R., Mock, M., Seidel, A., Jacob, J., and Lampen, A. (2002). Interaction between metabolism and transport of benzo[a]pyrene and its metabolites in enterocytes. Toxicol. Appl. Pharm. 183, 168–178. doi: 10.1006/taap.2002.9484
Cai, Y., Pan, L., and Miao, J. (2016). Molecular evidence for the existence of an aryl hydrocarbon receptor pathway in scallops Chlamys farreri. Comp. Biochem. Physio. B. 196, 74–84. doi: 10.1016/j.cbpb.2016.02.006
Cavalieri, E. L., and Rogan, E.G. (1992). The approach to understanding aromatic hydrocarbon carcinogenesis. The central role of radical cations in metabolic activation. Pharmacol. Ther. 55, 183–194. doi: 10.1016/0163-7258(92)90015-R
Chen, S., Qu, M., Ding, J., Zhang, Y., Wang, Y., and Di, Y. (2018). BaP-metals co-exposure induced tissue-specific antioxidant defense in marine mussels Mytilus coruscus. Chemosphere 205, 286–296. doi: 10.1016/j.chemosphere.2018.04.109
Costa, J., Reis-Henriques, M. A., Castro, L. F. C., and Ferreira, M. (2012). Gene expression analysis of ABC efflux transporters, CYP1A and GSTα in Nile tilapia after exposure to benzo(a)pyrene. Comp. Biochem. Physiol. C. Toxicol. Pharmacol. 155, 469–482. doi: 10.1016/j.cbpc.2011.12.004
Costa, J., Reis-Henriques, M. A., Wilson, J. M., and Ferreira, M. (2013). P-glycoprotein and CYP1A protein expression patterns in Nile tilapia (Oreochromis niloticus) tissues after waterborne exposure to benzo(a)pyrene (BaP). Environ. Toxicol. Phar. 36, 611–625. doi: 10.1016/j.etap.2013.05.017
Dean, M. (2005). The genetics of ATP-binding cassette transporters. Method. Enzymol. 400, 409–429. doi: 10.1016/S0076-6879(05)00024-8
Dean, M., Rzhetsky, A., and Allikmets, R. (2001). The human ATP-binding cassette (ABC) transporter superfamily. Genome. Res. 11, 1156–1166. doi: 10.1101/gr.GR-1649R
Di, Y. N., Aminot, Y., Schroeder, D. C., Readman, J. W., and Jha, A.N. (2017). Integrated biological responses and tissue-specific expression of p53 and ras genes in marine mussels following exposure to benzo(a)pyrene and C60 fullerenes, either alone or in combination. Mutagenesis 32, 77–90. doi: 10.1093/mutage/gew049
Di, Y. N., Schroeder, D. C., Highfield, A, Readman, J. W., and Jha, A.N. (2011). Tissue-specific expression of p53 and ras genes in response to the environmental genotoxicant benzo(a)pyrene in marine mussels. Environ. Sci. Technol. 45, 8974–8981. doi: 10.1021/es201547x
Dimitriadis, V. K., Domouhtsidou, G. P., and Cajaraville, M.P. (2004). Cytochemical and histochemical aspects of the digestive gland cells of the mussel Mytilus galloprovincialis (L.) in relation to function. J. Mol. Histol. 35, 501–509. doi: 10.1023/B:HIJO.0000045952.87268.76
Doi, A. M., Holmes, E., and Kleinow, K.M. (2001). P-glycoprotein in the catfish intestine: inducibility by xenobiotics and functional properties. Aquat. Toxicol. 55, 157–170. doi: 10.1016/S0166-445X(01)00180-1
Dong, W., Chen, Y., Lu, W., Wu, B., and Qi, P. (2017). Transcriptome analysis of Mytilus coruscus hemocytes in response to Vibrio alginnolyficus infection. Fish. Shellfish. Immunol. 70, 560–567. doi: 10.1016/j.fsi.2017.08.034
Faggio, C., Pagano, M., Alampi, R., Vazzana, I., and Felice, M.R. (2016). Cytotoxicity, haemolymphatic parameters, and oxidative stress following exposure to sublethal concentrations of quaternium-15 in Mytilus galloprovincialis. Aquat. Toxicol. 180, 258–265. doi: 10.1016/j.aquatox.2016.10.010
Faggio, C., Tsarpali, V., and Dailianis, S. (2018). Mussel digestive gland as a model tissue for assessing xenobiotics: an overview. Sci. Total. Environ. 636, 220–229. doi: 10.1016/j.scitotenv.2018.04.264
Fardel, O., Lecureur, V., Corlu, A., and Guillouzo, A. (1996). P-glycoprotein induction in rat liver epithelial cells in response to acute 3-methylcholanthrene treatment. Biochem. Pharmacol. 51, 1427–1436. doi: 10.1016/0006-2952(96)00081-0
Faria, M., Navarro, A., Luckenbach, T., Pina, B., and Barata, C. (2011). Characterization of the multixenobiotic resistance (MXR) mechanism in embryos and larvae of the zebra mussel (Dreissena polymorpha) and studies on its role in tolerance to single and mixture combinations of toxicants. Aquat. Toxicol. 101, 78–87. doi: 10.1016/j.aquatox.2010.09.004
Franzellitti, S., Striano, T., Valbonesi, P., and Fabbri, E. (2016). Insights into the regulation of the mxr response in haemocytes of the mediterranean mussel (Mytilus galloprovincialis). Fish. Shellfish. Immunol, 58, 349–358. doi: 10.1016/j.fsi.2016.09.048
Gekeler, V., Ise, W., Sanders, K. H., Ulrich, W. R., and Beck, J. (1995). The leukotriene LTD4 receptor antagonist MK571 specifically modulates MRP associated multidrug resistance. Biochem. Bioph. Res. 208, 345–352. doi: 10.1006/bbrc.1995.1344
Haimeur, A., Conseil, G., Deeley, R. G., and Cole, S.P.C. (2004). The MRP-related and BCRP/ABCG2 multidrug resistance proteins: biology, substrate specificity and regulation. Curr. Drug. Metab. 5, 21–53. doi: 10.2174/1389200043489199
Hall, M., and Grover, P.L. (1990). “Polycyclic aromatic hydrocarbon: metabolism, activation and tumor initiation,” in Chemical Carcinogenesis and Mutagenesis, vol. 1, eds C. S. Cooper and P. L. Grover (Berlin: Springer-Verlag), 327–372. doi: 10.1007/978-3-642-74775-5_9
Haritash, A. K., and Kaushik, C.P. (2009). Biodegradation aspects of polycyclic aromatic hydrocarbons (PAHs): a review. J. Hazard. Mater. 169, 1–15. doi: 10.1016/j.jhazmat.2009.03.137
Holl, ó Z., Homolya, L., Hegedüs, T., and Sarkadi, B. (1996). Transport properties of multidrug resistance-associated protein (MRP) in human tumour cells. FEBS. Lett. 383, 99–104. doi: 10.1016/0014-5793(96)00237-2
Hollenstein, K., Frei, D. C., and Locher, K.P. (2007). Structure of an ABC transporter in complex with its binding protein. Nature 446, 213–216. doi: 10.1038/nature05626
Huang, L., Liu, S. L., Zheng, J. W., Li, H. Y., Liu, J. S., and Yang, W.D. (2015). P -glycoprotein and its inducible expression in three bivalve species after exposure to Prorocentrum lima. Aquat. Toxicol. 169, 123–132. doi: 10.1016/j.aquatox.2015.10.012
Jeong, C.-B, Kim, H.-S, Kang, H.-M,;, and Lee, J.-S. (2017). ATP-binding cassette (ABC) proteins in aquatic invertebrates: evolutionary significance and application in marine ecotoxicology. Aquat. Toxicol. 185, 29–39. doi: 10.1016/j.aquatox.2017.01.013
Juhasz, A. L., and Naidu, R. (2000). Bioremediation of high molecular weight polycyclic aromatic hydrocarbons: a review of the microbial degradation of benzo[α]pyrene. Int. Biodeterior. Biodegrad. 45, 57–88. doi: 10.1016/S0964-8305(00)00052-4
Kim, H., Yim, B., Kim, J., Kim, H., and Lee, Y.-M. (2017). Molecular characterization of ABC transporters in marine ciliate, Euplotes crassus: identification and response to cadmium and benzo[a]pyrene. Mar. Pollut. Bull 124, 725–735. doi: 10.1016/j.marpolbul.2017.01.046
Kingtong, S., Chitramvong, Y., and Janvilisri, T. (2007). ATP-binding cassette multidrug transporters in Indian-rock oyster Saccostrea forskali and their role in the export of an environmental organic pollutant tributyltin. Aquat. Toxicol. 85, 124–132. doi: 10.1016/j.aquatox.2007.08.006
Klein, I., Sarkadi, B., and Varadi, A. (1999). An inventory of the human ABC proteins. Biochim. Biophys. Acta. 1461, 237–262. doi: 10.1016/S0005-2736(99)00161-3
Kumar, S., Stecher, G., Li, M., Knyaz, C., and Tamura, K. (2018). MEGA X: molecular evolutionary genetics analysis across computing platforms, Mol. Biol. Evol. 35, 1547–1549. doi: 10.1093/molbev/msy096
Kurelec, B. (1992). The multixenobiotic resistance mechanism in aquatic organisms. Crit. Rev. Toxicol. 22, 23–43. doi: 10.3109/10408449209145320
Leslie, E. M., Deeley, R. G., and Cole, S.P.C. (2005). Multidrug resistance proteins: role of P-glycoprotein, MRP1, MRP2, and BCRP (ABCG2) in tissue defense. Toxicol. Appl. Pharm. 204, 216–237. doi: 10.1016/j.taap.2004.10.012
Liu, D., Pan, L., Cai, Y., Li, Z., and Miao, J. (2014). Response of detoxification gene mRNA expression and selection of molecular biomarkers in the clam Ruditapes philippinarum exposed to benzo[a]pyrene. Environ. Pollut. 189, 1–8. doi: 10.1016/j.envpol.2014.02.013
Liu, H., Wu, J., Xu, M., and He, J. (2016). A novel biomarker for marine environmental pollution of HSP90 from Mytilus coruscus. Mar. Pollut. Bull. 111, 428–434. doi: 10.1016/j.marpolbul.2016.07.031
Livak, K. J., and Schmittgen, T.D. (2001). Analysis of relative gene expression data using realtime quantitative PCR and the 2–ΔΔCt method. Methods 25, 402–408. doi: 10.1006/meth.2001.1262
Luckenbach, T., Altenburger, R., and Epel, D. (2008). Teasing apart activities of different types of ABC efflux pumps in bivalve gills using the concepts of independent action and concentration addition. Mar. Environ. Res. 66, 75–76. doi: 10.1016/j.marenvres.2008.02.027
Luckenbach, T., and Epel, D. (2008). ABCB-and ABCC-type transporters confer multixenobiotic resistance and form an environment-tissue barrier in bivalve gills. Am. J. Physiol. Regul. Integr. Comp. Physiol. 294, R1919–R1929. doi: 10.1152/ajpregu.00563.2007
Luckenbach, T., Fischer, S., and Sturm, A. (2014). Current advances on ABC drug transporters in fish. Comp. Biochem. Phys. C. 165, 28–52. doi: 10.1016/j.cbpc.2014.05.002
Lüdeking, A., and Köhler, A. (2002). Identification of six mRNA sequences of genes related to multixenobiotic resistance (MXR) and biotransformation in Mytilus edulis. Mar. Ecol. Prog. Ser. 238, 115–124. doi: 10.3354/meps238115
Lüdeking, A., and Köhler, A. (2004). Regulation of expression of multixenobiotic resistance (MXR) genes by environmental factors in the blue mussel Mytilus edulis. Aquat. Toxicol. 69, 1–10. doi: 10.1016/j.aquatox.2004.03.003
Marigómez, I., Soto, M., Cajaraville, M. P., Angulo, E., and Giamberini, L. (2002). Cellular and subcellular distribution of metals in molluscs. Microsc. Res. Tech. 56, 358–392. doi: 10.1002/jemt.10040
Miao, J., Cai, Y., Pan, L., and Li, Z. (2014). Molecular cloning and characterization of a MXR-related P-glycoprotein cDNA in scallop Chlamys farreri: transcriptional response to benzo(a)pyrene, tetrabromobisphenol A and endosulfan. Ecotoxicol. Environ. Saf. 110, 136–142. doi: 10.1016/j.ecoenv.2014.08.029
Navarro, A., Weißbach, S., Faria, M., Barata, C., Piña, B., and Luckenbach, T. (2012). Abcb and Abcc transporter homologs are expressed and active in larvae and adults of zebra mussel and induced by chemical stress. Aquat. Toxicol. 122, 144–152. doi: 10.1016/j.aquatox.2012.06.008
Oldham, M. L., Davidson, A. L., and Chen, J. (2008). Structural insights into ABC transporter mechanism. Curr. Opin. Struct. Biol. 18, 726–733. doi: 10.1016/j.sbi.2008.09.007
Pagano, M., Capillo, G., Sanfilippo, M., Palato, S., Trischitta, F., Manganaro, A., et al. (2016). Evaluation of functionality and biological responses of Mytilus galloprovincialis after exposure to quaternium-15 (methenamine 3-chloroallylochloride). Molecules 21, 1–12. doi: 10.3390/molecules21020144
Penning, T. M., Burczynski, M. E., Hung, C.-F, McCoull, K. D., Palackal, N. T., and Tsuruda, L.S. (1999). Dihydrodiol dehydrogenase and polycyclic aromatic hydrocarbon activation: generation of reactive and redox active o-quinones. Chem. Res. Toxicol. 12, 1–18. doi: 10.1021/tx980143n
Prevodnik, A., Lilja, K., and Bollner, T. (2007). Benzo[a]pyrene up-regulates the expression of the proliferating cell nuclear antigen (PCNA) and multixenobiotic resistance polyglycoprotein (P-gp) in Baltic Sea blue mussels (Mytilus edulis L.). Comp. Biochem. Physio. C. 145, 265–274. doi: 10.1016/j.cbpc.2006.12.014
Puga, A., Xia, Y., and Elferink, C. (2002). Role of the aryl hydrocarbon receptor in cell cycle regulation. Chem. Biol. Interact. 141, 117–130. doi: 10.1016/S0009-2797(02)00069-8
Sharom, F. J., Yu, X., Lu, P., Liu, R., Chu, J. W., Szabo, K., et al. (1999). Interaction of the P-glycoprotein multidrug transporter (MDR1) with high affinity peptide chemosensitizers inisolated membranes, reconstituted systems, and intact cells. Biochem. Pharmaco. 58, 571–586. doi: 10.1016/S0006-2952(99)00139-2
Smital, T., and Kurelec, B. (1998). The chemosensitizers of multixenobiotic resistance mechanism in aquatic invertebrates: a new class of pollutants. Mutat. Res. 399, 43–53. doi: 10.1016/S0027-5107(97)00265-0
Szakàcs, G., Vàradi, A., Ozvegy-Laczka, C., and Sarkadi, B. (2008). The role of ABC transporters in drug absorption, distribution, metabolism, excretion and toxicity (ADME-Tox). Drug Discov. Today. 13, 379–393. doi: 10.1016/j.drudis.2007.12.010
Wang, Y., Li, L., Hu, M., and Lu, W. (2015). Physiological energetics of the thick shell mussel Mytilus coruscus exposed to seawater acidification and thermal stress. Sci. Total. Environ. 514, 261–272. doi: 10.1016/j.scitotenv.2015.01.092
Xu, C. J., Li, C. Y. T., and Kong, A.N.T. (2005). Induction of phase I, II and III drug metabolism/transport by xenobiotics. Arch. Pharm. Res. 28, 249–268. doi: 10.1007/BF02977789
Xu, K., Zhang, Z., Xu, Z., Tang, Z., Liu, L., Lu, Z., et al. (2019). A novel invertebrate toll-like receptor is involved in TLR mediated signal pathway of thick shell mussel Mytilus coruscus. Dev. Comp. Immunol. 97, 11–19. doi: 10.1016/j.dci.2019.03.012
Xue, W., and Warshawsky, D. (2005). Metabolic activation of polycyclic and heterocyclicaromatic hydrocarbons and DNA damage: a review. Toxicol. Appl. Pharmacol. 206, 73–93. doi: 10.1016/j.taap.2004.11.006
Yuan, L., Lv, B., Zha, J., Wang, W., and Wang, Z. (2014). Basal and benzo[a]pyrene-induced expression profile of phase I and II enzymes and ABC transporter mRNA in the early life stage of Chinese rare minnows (Gobiocypris rarus). Ecotoxicol. Environ. Saf. 106, 86–94. doi: 10.1016/j.ecoenv.2014.04.018
Keywords: Mytilus coruscus, ABC transporters, benzo(α)pyrene, cellular detoxification response, efflux activities
Citation: Guo B, Xu Z, Yan X, Buttino I, Li J, Zhou C and Qi P (2020) Novel ABCB1 and ABCC Transporters Are Involved in the Detoxification of Benzo(α)pyrene in Thick Shell Mussel, Mytilus coruscus. Front. Mar. Sci. 7:119. doi: 10.3389/fmars.2020.00119
Received: 31 October 2019; Accepted: 13 February 2020;
Published: 06 March 2020.
Edited by:
Youji Wang, Shanghai Ocean University, ChinaReviewed by:
Yanan Di, Zhejiang University, ChinaCarlos M. Luquet, National Council for Scientific and Technical Research (CONICET), Argentina
Copyright © 2020 Guo, Xu, Yan, Buttino, Li, Zhou and Qi. This is an open-access article distributed under the terms of the Creative Commons Attribution License (CC BY). The use, distribution or reproduction in other forums is permitted, provided the original author(s) and the copyright owner(s) are credited and that the original publication in this journal is cited, in accordance with accepted academic practice. No use, distribution or reproduction is permitted which does not comply with these terms.
*Correspondence: Pengzhi Qi, qpz2004@vip.sina.com