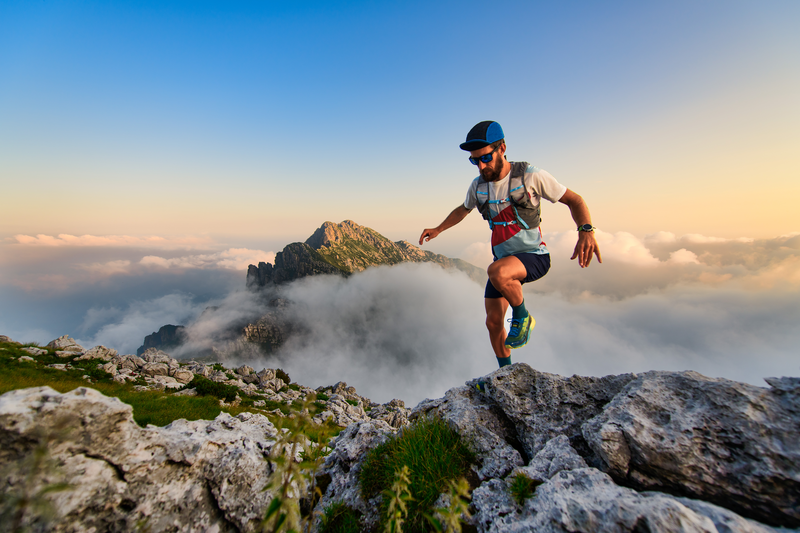
95% of researchers rate our articles as excellent or good
Learn more about the work of our research integrity team to safeguard the quality of each article we publish.
Find out more
REVIEW article
Front. Immunol. , 25 February 2025
Sec. Viral Immunology
Volume 16 - 2025 | https://doi.org/10.3389/fimmu.2025.1540932
This article is part of the Research Topic Deciphering Host-Virus Interactions and Advancing Therapeutics for Chronic Viral Infection View all 4 articles
Elite controllers (ECs) and post-treatment controllers (PTCs) represent important models for achieving a functional cure for HIV. This review synthesizes findings from immunological, genetic, and virological studies to compare the mechanisms underlying HIV suppression in ECs and PTCs. Although ECs maintain viral control without antiretroviral therapy (ART), PTCs achieve suppression following ART discontinuation. Both groups rely on adaptive and innate immunity, host genetic factors, and characteristics of the HIV reservoir; however, they exhibit distinct immune responses and genetic profiles. These differences provide insights into strategies for sustained ART-free remission. Understanding the shared and unique mechanisms in ECs and PTCs can inform the development of novel therapeutic approaches, including immune-based therapies and genome editing, to achieve a functional cure for HIV-1.
Current antiretroviral therapy (ART) effectively suppresses plasma viral RNA to undetectable levels for extended durations, preventing viral evolution. ART is generally prescribed for interventions during the chronic phase of infection. However, in most patients, a rapid rebound in viremia is observed within weeks of ART interruption (1, 2). This dependence poses several challenges, including side effects, drug resistance, stigma, and economic burden. Consequently, researchers are exploring various therapeutic strategies to prevent or delay viral rebound following treatment interruption, aiming for post-treatment remission or a functional cure for HIV (3). A functional cure for HIV refers to a state where patients can cease ART. The main research directions include gene therapy and immunotherapy. These treatment methods aim to fundamentally change the body’s ability to control HIV to achieve long-term virus control without ART (4). Despite substantial progress, achieving sustained ART-free remission remains elusive for the majority of HIV-infected individuals.
Special populations such as elite controllers (ECs) and post-treatment controllers (PTCs) offer invaluable insights into the mechanisms of ART-free HIV remission. ECs, who are able to maintain viral suppression (<50 copies/mL) without initiating ART for prolonged periods (5), provide potential directions for a functional cure (6). The existence of HIV ECs indicates that the goal of ART-free HIV remission is possible, likely because of favorable genetic and immunological profiles (7). However, they represent a very small subset of the population (<1%) (8–10). In contrast, PTCs, identified in therapeutic intervention studies, sustain low or undetectable viremia following ART discontinuation and constitute a larger proportion of patients (5–15% in some studies) (11, 12). The existence of ECs and PTCs demonstrates the feasibility of ART-free remission and inspires efforts to emulate these natural mechanisms of control in broader patient populations.
Although both ECs and PTCs achieve viral suppression without continuous ART, the mechanisms underlying their control may differ. ECs often rely on robust HIV-specific immune responses (13, 14), advantageous host genetic factors (15), and attenuated viral (16) characteristics. On the other hand, PTCs appear to achieve control through a combination of acute ART initiation, reduced HIV reservoir size, and immune-mediated mechanisms (11, 17, 18). Comparing and contrasting these groups provides critical insights into diverse pathways for achieving functional HIV cures.
This review aims to elucidate the overlapping and distinct mechanisms of HIV suppression in ECs and PTCs, with a focus on identifying therapeutic targets and strategies for achieving ART-free remission. By examining these two populations, we hope to provide guidance for future therapeutic approaches, including immune-based therapies and genome-editing strategies. Ultimately, the aim of this study is to contribute to the ongoing effort to achieve a functional cure for HIV-1.
In the early stages of HIV infection, immune activation plays a crucial role in controlling HIV replication and may contribute to limiting the establishment of viral reservoirs (Figure 1). This activation primarily results from the immune system’s response to the presence of the virus. However, prolonged and sustained immune activation, especially in the chronic phase of HIV infection, can impair immune reconstitution, accelerate immune senescence and increase the risk of non-AIDS-related diseases (19). Understanding these complex immune mechanisms is critical, particularly in ECs and PTCs, whose immune responses provide insights into strategies for improving clinical outcomes. This section focuses on the immune characteristics of ECs and PTCs, explores how adaptive immune mechanisms, particularly cellular immunity, contribute to the control of HIV infection and offer potential avenues for immunotherapy (Table 1).
Figure 1. Potential mechanisms of innate immunity and adaptive immunity possibly contributing to the spontaneous control of viremia in HIV controllers. HIV controllers have a more robust and effective immune response, with functional DCs and NKs, highly cytotoxic and activated CD8+ T cells, and CD4+ T cells that can better maintain immune function. (1) DCs from HIV controllers are less permissive to HIV-1 infection but more efficient at capturing HIV-1 particles, and they have distinct phenotypes and functions, such as the presence of a highly functional CD64hi PD-L1hi mDC state, enhanced antigen presentation, and improved sensing of cytosolic HIV-1 replication products, which can trigger stronger T-cell responses. (2) CD4+ T cells, which are the main target of HIV, are severely depleted in AIDS patients. In HIV controllers, CD4+ T cells maintain their numbers and functions, are less sensitive to HIV-1 infection, and have high - affinity TCRs. (3) In HIV controllers, CD8+ T cells show stronger HIV antigen - induced cell proliferation, cytotoxic activity, and can effectively inhibit HIV-1 replication in autologous CD4+ T cells. (4) In HIV controllers, NK cell activity is enhanced. These NK cells secrete higher levels of IFN-γ and TNF-α, express more NKp46/NKG2D-activated receptors, and possess a stronger ADCC capacity.
By exhibiting potent virus-specific responses, CD8+ T cells play a crucial role in the immune surveillance of HIV, especially in ECs (6), by interacting synergistically with HLA genes, restricting viral mutations, and performing versatile immune functions. HIV-1-specific CD8+ T cells are widely regarded as the key mediators of long-term viral suppression in HIV-1 ECs (20). These T lymphocytes recognize and eliminate cells expressing non-self-peptides using major histocompatibility complex (MHC) molecules, which are also referred to as HLAs. Genome-wide association studies (GWASs) have identified specific protective HLA class I alleles, particularly HLA-B* 57/58 and HLA-B* 27, that are strongly associated with CD8+ T-cell responses (7, 34). Consistent with many early cohort studies, the majority of ECs possess these protective HLA alleles (35). Notably, the function of HIV-specific CD8+ T cells in ECs is qualitatively distinct from that of chronic HIV progressors. One major difference is the upregulation of both inflammatory chemokine gene expression and effector functions in ECs. These functional adaptations result in enhanced HIV antigen-induced cell proliferation and robust cytotoxic activity, which collectively contribute to effective viral suppression in ECs (36). In addition to their cytolytic functions, these CD8+ T cells secrete a variety of cytokines and undergo activated degranulation upon HIV peptide presentation (37). For example, CXCR5+ follicular CD8+ T cells release perforin and granzyme B near HIV RNA+ cells in lymphoid follicles (38). Crucially, the HLAs of HIV-specific CD8+ T cells in ECs facilitate the efficient presentation of conserved and highly networked HIV-1 epitopes, thereby enhancing T-cell-mediated immune responses. These findings highlight the central role of HLA class I-restricted CD8+ T-cell responses in the control of HIV-1 replication in ECs. Furthermore, one of the primary functional differences between ECs and HIV progressors lies in the ability of CD8+ T cells in ECs to effectively inhibit HIV-1 replication in autologous CD4+ T cells (39, 40), even when they are infected in vitro (41).
Moreover, other studies suggest that the increased loading of lytic granules within CD8+ T cells in ECs results in a substantial increase in the delivery of granzyme B, which is directed at HIV-infected cells (36, 42, 43). In addition to these cytolytic functions, CD8+ T cells in ECs exhibit HIV-1-specific activation, which is associated with distinct metabolic (44) and transcriptional profiles (45). These profiles include the differential expression or regulation of key transcription factors, further highlighting the complexity of CD8+ T-cell responses in ECs. Additionally, viruses in EC plasma exhibit different mutations compared with those found in resting CD4+ T cells, indicating that CD8+ T cells exert active immune selection pressure on the virus (46–48).
PTCs may exhibit distinct immunological characteristics in contrast to ECs, with features such as attenuated cellular and humoral antiviral responses and increased heterogeneity (21). Compared with ECs, HIV-specific CD8+ T cells in PTCs presented decreased activation levels, lower frequencies, and a reduced ability to inhibit the infection of autologous CD4+ T cells (11). Moreover, research has revealed that PTCs are heterogeneous and that effective HIV-specific CD8+ T-cell-mediated responses can be detected in these patients (49). A previous study revealed that rhesus macaques achieved potential PTC effects after treatment. Rhesus macaques (Macaca mulatta) achieving PTC effects exhibited lower viral DNA levels in deep lymph nodes (e.g., mesenteric and parenteral lymph nodes), correlating with stable virologic suppression. In contrast, rhesus macaques with higher viral DNA levels in superficial lymph nodes (e.g., cervical or axillary lymph nodes) showed stable or unstable viral rebound. Tissues from PTC rhesus macaques demonstrated significantly reduced quantitative viral outgrowth, fewer Programmed Death-1 (PD-1)+ central memory CD4+ T cells, and CD8+ T cells critically contributed to maintaining virologic control efficacy (50).
CD4+ T cells have specific characteristics in controllers. CD4+ T helper cells are required for long-term maintenance of antigen-specific CD8+ T cells. CD4+ T cells play an important role in HIV infection. They are the primary target cells of HIV but also recognize viral antigens and activate other immune cells, such as CD8+ T cells and B cells. In the early stages of infection, the number of CD4+ T cells decreases sharply, resulting in immune system damage. However, in ECs, the number and functions of CD4+ T cells are maintained, which is related to the unique characteristics of CD4+ T cells. CD4+ T cells have high-affinity T-cell antigen receptors (TCRs) that effectively recognize high-avidity T cells and respond to low amounts of HIV viral antigens (22). In addition, the levels of certain cytokines, such as Chemokine (C-C motif) ligand (CCL) 14, CCL21, CCL27, Chemokine (C-X-C motif) ligand (CXCL) 1 and CXCL12, are elevated in ECs, and these cytokines upregulate cell activation, HIV coreceptor expression and the effector functions of CD4+ T cells (51).
Compared with those in HIV-1 progressors and HIV-1-negative individuals, CD4+ T cells from ECs are less sensitive to HIV-1 infection. The intrinsic mechanisms that limit HIV-1 replication in CD4+ T target cells may also play an important role in mediating the resistance of ECs to HIV-1 infection. In the process of HIV infection, CD4+ T-cell number and function are important indicators for assessing disease progression and for determining whether a patient is a controller. ECs (plasma viral load low to undetectable, median percentage of CD4+ T cells> 40%) have normal T-cell and monocyte phenotypes and therefore may have limited benefit from ART (23).
In addition to maintaining CD4+ T-cell functions, ECs also present an increased frequency of helper T (Th) 17 cells and activated regulatory T (Treg) cells compared with ART-treated and HIV-1-negative individuals (52). These subsets are more readily activated and produce higher levels of cytokines, further bolstering the immune response in ECs. Notably, ECs maintain a high Th17/Treg ratio, which has been shown to be beneficial for controlling inflammation and sustaining immune balance (52, 53). Research indicates that HIV-1-specific CD4+ T cells in ECs robustly express genes associated with Th1, Th17, and Th22 subsets of helper T cells, suggesting a multifaceted immune response. Moreover, the expression of cytokines related to mucosal immunity is increased in the HIV-specific CD4+ T cells of ECs, in contrast with the profiles observed in chronic progressors (54).
PTCs exhibit reduced activation of mature CD4+ T cells but more robust Gag-specific CD4+ T-cell activity (18). Compared with noncontrollers (NCs), PTCs subjected to early analytical treatment exhibited significantly elevated levels of Gag-specific CD4+ T cells that produce Interferon-γ (IFN-γ), along with a modest increase in CD4+ T cells that produce Interleukin-2 (IL-2). In addition, CD4+ T cells also have important implications for HIV vaccine research, as they are the main targets of vaccine-induced immune responses (Figure 1).
An increasing number of studies support a role for humoral immunity in controlling HIV infection and replication (55, 56). In particular, ECs exhibit efficient humoral responses that contribute significantly to the natural control of HIV-1, with notably high levels of HIV-specific memory B cells (57). These responses are crucial for long-term viral suppression.
One of the key characteristics of ECs is their production of neutralizing antibodies (NAbs). Although the level of NAbs in ECs may be lower than that in chronic progressors (24, 25), their higher affinity and polyfunctional activity may still allow them to exert strong neutralizing activity. This finding suggests that strong suppression of viral replication limits stimulation and that the maintenance of effective NAb responses and high-titer NAbs are not required for maintenance of viral suppression (58). Although NAbs might not be the primary factor controlling viral replication, they may still play a role in the natural control of HIV-1. For example, bNAbs in ECs are capable of neutralizing multiple strains of HIV, including diverse subtypes, by targeting conserved regions of the virus, such as the CD4 binding site, V3 loop, and gp120/gp41 interface (59).
In addition to bNAbs, ECs present increased levels of nonneutralizing antibodies that engage in Fc-mediated effector functions such as antibody-dependent cellular cytotoxicity (ADCC). In these individuals, ADCC levels correlate positively with CD8+ T-cell function, suggesting that effective CD4+ T-cell support and that CD8-mediated suppression enhance ADCC activity (60). Moreover, these antibodies are thought to contribute to more effective control of viral replication in ECs than chronic progressors (26). For example, negative factor (Nef) proteins in ECs have a diminished ability to downregulate CD4, enhancing the exposure of ADCC-mediated epitopes on the HIV-1 envelope (Env) and increasing the susceptibility of HIV-infected cells to ADCC clearance (61), providing a potential therapeutic strategy.
Memory B-cell responses in ECs also play a critical role in controlling HIV. Research by Rouers et al. revealed that ECs maintain robust memory B-cell compartments and HIV-specific memory B-cell responses (62). The frequency of Env-specific B cells in HLA-B*57+ ECs correlates with the breadth of neutralization observed, suggesting that these memory responses are crucial for maintaining broad neutralization capacities and the natural control of HIV infection.
Some studies have shown that in PTCs, the humoral immune profiles are heterogeneous, mainly affected by virus exposure and dynamics (63). Virally-exposed PTCs produce a functionally coordinated and effective humoral response to HIV-1, which has the potential to generate bNAbs and autologous NAbs. For example, Molinos-Albert et al. identified a bNAb lineage, EPTC112, in a PTC with an elite neutralizer profile, underscoring the potential role of antibodies in long-term HIV control (27, 28). These antibodies demonstrated the ability to neutralize the autologous virus even in the presence of escape mutations, indicating a complex immune response that may support functional remission strategies after ART interruption.
In summary, although NAb levels in ECs may be lower than those in chronic progressors, their higher affinity and greater ADCC activity likely contribute to more effective control of HIV-1. Although bNAbs may not be the decisive factor in regulating viral replication, they may still play an important role in the natural control of HIV infection, especially in individuals not receiving ART. ECs exhibit a multifaceted humoral immune response characterized by strong neutralizing antibody activity, enhanced memory B-cell responses, and potentially structural modifications to the virus that aid in controlling HIV-1 infection without ART. These findings underscore the importance of humoral immunity in HIV-1 control; however, more research is needed to fully understand its role across different patient populations.
Research indicates that HIV-1 immune control is primarily mediated by virus-specific T-cell responses, where increased T-cell polyfunctionality is associated with improved viral control during HIV-1 disease progression. However, innate immune cells are also involved in the natural control of HIV-1 (Figure 1). Innate immunity serves as the first line of defense against HIV and triggers subsequent adaptive immune responses. Pattern recognition receptors recognize HIV, and a series of immune cells are subsequently recruited to induce or activate numerous innate immune-related factors to exert antiviral effects. In HIV-infected EC/PTC populations, dendritic cells (DCs), natural killer (NK) cells, macrophages, and NKT cells are crucial components of innate immunity and play important roles in controlling HIV. A discussion of innate immunity in HIV controllers is provided in the following sections.
Accumulating evidence indicates that NK cells play an important role in HIV control. First, increased NK cell frequency and activity were observed in HIV controllers than in NCs (29). In ECs, NK cells exhibit several distinct characteristics distinguishing them from NCs. Pohlmeyer et al. used mass cytometry to analyze NK cell phenotypes in ECs and viremic non-controllers (VNCs). They revealed that the CD11b+CD57-CD161+Siglec-7+ subpopulations of CD56dimCD16+ NK cells were more abundant in ECs and HIV-negative controls than in VNCs and that the frequency of these cells correlated with HIV DNA levels (64). Certain alleles of killer immunoglobulin receptors (KIRs), such as KIR3DS1, on NK cells are associated with the slow disease progression (65, 66). Marras et al. investigated the effects of NK cell functional characteristics on controllers [ECs and long-term nonprogressors (LTNPs)] and progressor patients. HIV DNA copy numbers (either total or integrated) in circulating CD4+ T cells were negatively correlated with transcriptionally unique NK cell functions. Specifically, the production of induced IFN-γ and the expression of NKp46/NKp30-activating receptors are inversely associated with HIV reservoir size (67). Moreover, in an African green monkey model of nonpathogenic SIV infection, NK cells migrate into follicles and play a major role in controlling the HIV reservoir in lymph nodes (68). These results suggest that some specific phenotypes and functions of NK cells contribute to the control of HIV reservoirs in ECs.
Recent studies of the VISCONTI cohort revealed high levels of specific NK cells in PTCs. In functional studies in vitro, NK cells derived from PTCs also secreted relatively high levels of IFN-γ and showed a strong ability to control HIV infection (30). A proviral landscape study of PTCs revealed higher NK cell activation levels in PTCs, which was associated with lower levels of total and defective proviral genomes (31). Additionally, in an analytical treatment interruption (ATI) study of PTCs from AIDS Clinical Trials Group (ACTG), immunologically, PTCs exhibited stronger NK cell activation and function. PTCs had increased levels of activation markers, including CD38+ CD56+ NK cells and CD69+ NK cells (18). Some studies involving NK cell analysis in controllers revealed that the CD56+/CD16- NK cell subsets of controllers were the same as those of healthy donors and greater than those of chronic patients and that the IFN-γ, Tumor Necrosis Factor-α (TNF-α) and IL-12 levels secreted from the NK cells of controllers were increased (69, 70). Moreover, HIV controllers expressed higher Natural Killer Group 2, Member D (NKG2D) levels than chronic patients, enhancing the susceptibility of infected cells to ADCC (69, 71). Recent studies have treated chronic HIV infection with NK cells coupled with cytokines, indicating the potential of NK cells in controlling HIV (72). These results suggest that preserving the phenotype and function of NK cells at the time of treatment is important for HIV control. Transcriptionally and functionally distinctive NK cell characterization can be used to prospectively identify HIV-infected patients who are highly likely to successfully receive ART simplified to monotherapy or ART interruption (PTCs).
The majority of HIV-1 ECs restrict virus replication by eliciting robust HIV-1-specific T-cell responses, and DCs stand out as the most potent natural antigen-presenting cells, playing a pivotal role in the induction and maintenance of antigen-specific T-cell responses (32, 33). Over the past few years, the role of DCs in HIV-1 controllers has been increasingly appreciated. The DCs derived from HIV controllers are less permissive to HIV-1 infection than cells obtained from healthy donors or HIV-1 patients after ART treatment, but DCs from HIV controllers have a strong ability to capture HIV-1 particles (73). Phenotypically, a highly functional CD64hiPD-L1hi mDC state was found in ECs using single-cell sequencing, with the fractional abundance associated with increased CD4+ T-cell counts and a decreased HIV-1 viral load, and it effectively triggered polyfunctional T-cell responses in vitro (74). Mass cytometry analysis revealed that plasma HIV RNA levels were positively associated with a loss of mDC and pDC subpopulations that displayed high expression of leukocyte immunoglobulin-like receptors (LILRs). A particular subtype of CD1c+ CD32bhi HLA-DRhi mDCs in peripheral blood monouclear cells (PBMCs) was enriched in HIV ECs (75). mDCs from ECs have significantly enhanced antigen presentation characteristics and are able to sensitize allologous T cells more efficiently than those from healthy donors or HIV-1 progressors (76). Cytosolic immunorecognition of HIV-1 in mDCs promotes the initiation and expansion of HIV-1-specific T cells. HIV-1 prevents intracellular immune recognition by mDCs in most infected individuals. However, mDCs from ECs exhibit an improved ability to sense cytosolic HIV-1 replication products. In ECs, HIV-1 replication products in mDCs result in rapid and sustained secretion of endogenous IFN-I from mDCs and the induction of potent HIV-1-specific CD8+ T cells (77, 78). This finding suggests that endogenous cellular IFN-I secretion in DCs plays an important role in inducing potent HIV-1-specific CD8+ T cells and may contribute to eliciting functional T-cell immunity against HIV-1 for prophylactic or therapeutic clinical purposes. The sterile alpha motif and HD domain-containing protein 1 (SAMHD1) restricts HIV-1 infection of mDCs and other myeloid cells (79). SAMHD1 is a host protein that is highly expressed in myeloid cells and limits HIV-1 replication at the reverse transcription level (80) by depleting the intracellular pool of deoxynucleoside triphosphates (81) and directly degrading viral RNA (82). In addition, intracellular immune recognition of HIV-1 by mDCs in ECs involves detection of the replication products of viral RNA and DNA. Therefore, the DNA sensor cyclic GMP-AMP synthase (cGAS) (83), the RNA sensor Retinoic acid-inducible gene I (RIG-I) (84) and cooperation between the two sensing pathways can improve the innate recognition of HIV-1 by mDCs in ECs (85). Despite lower Ag uptake than that of mDC subsets, pDCs efficiently cross-present exogenous Ags to CD8+ T cells (86). pDCs are bone marrow-derived cells that sense HIV via Toll-like receptor (TLR)-7 and TLR-9 and convert this signal to IFN-I (IFN-α, -β, -ϵ, -ω and -κ) production and T-cell activation (87, 88). Compared with VNCs, pDCs from ECs have a greater capacity to reduce HIV production and induce T-cell apoptosis, whereas pDCs from viremic NCs minimally respond to previous TLR-9 stimuli. Additionally, the function of pDCs from ECs is preserved, with similar levels of IFN-α production in healthy donors and higher levels compared to those in VNCs (89, 90). Together, these findings suggest a specific link between innate and adaptive HIV-1 immunity in controllers and that DCs may play an important role in immune defense mechanisms and HIV-1 therapy.
Increasing evidence suggests that immune-mediated effector responses play a crucial role in the establishment of deep viral latency, a state in which the virus remains dormant within cells. The study of immune-mediated effector responses in HIV controllers offers promising insights into the complex interactions between the immune system and HIV-1. HIV controllers are characterized by robust immune responses mediated by a combination of both innate and adaptive immunity and are able to effectively target and eliminate HIV-1-infected cells. The innate immune system is able to quickly recognize and respond to HIV-1, whereas the adaptive immune system generates specific T-cell responses that target and destroy infected cells (Figure 1). As we continue to unravel the immune mechanism of this remarkable subgroup, we hope to identify new approaches to harness the power of the immune system to combat HIV-1.
Susceptibility to HIV infection and the rate of disease progression vary significantly among individuals. After initial infection, the HIV RNA levels reached and the subsequent progression to AIDS differ widely. A growing number of studies suggest that genetic differences may contribute to this variability, influencing not only susceptibility to HIV but also the rate of progression to disease. Additionally, genetic factors may be involved in the risk of developing specific HIV-related complications, such as renal or neurological disorders, as well as non-AIDS conditions such as cardiovascular disease. Furthermore, certain genetic variants have been linked to the recovery of CD4+ T lymphocyte counts following ART. Through candidate gene approaches and GWASs, several key genetic variations associated with both HIV susceptibility and progression have been identified (Table 2). These findings provide valuable insights into HIV control.
C-C chemokine receptor type 5 (CCR5) is a critical coreceptor used by HIV-1 to enter immune cells. Given its key role in the viral entry process, targeting CCR5 has emerged as a promising strategy for preventing and potentially curing HIV-1 infection. Targeting the CCR5 receptor to decrease host cell susceptibility or confer resistance to infection could enhance HIV-1 inhibition, especially when used alongside other anti-HIV-1 strategies (122).
One of the most notable genetic findings in HIV research is the CCR5 Δ32 mutation, which involves a 32-base pair deletion in the CCR5 gene. This mutation leads to a truncated protein that is not expressed on the cell surface, rendering individuals homozygous for the Δ32 allele resistant to HIV-1 infection. These findings were highlighted in the cases of the “Berlin” and “London” patients, where stem cell transplants from CCR5 Δ32 homozygous donors resulted in long-term HIV remission. This has driven interest in exploring CCR5-targeted therapies for broader HIV-1 treatment options (123–125). Recent studies have shown that the downregulation of CCR5 expression in HIV-specific CD4+ T cells leads to the natural ability of ECs to control HIV-1 replication (112–114). This reduced expression limits the ability of the virus to enter and infect these cells, suggesting that inactivating or downregulating the CCR5 gene in a nonfunctional receptor could be key to achieving a functional HIV cure. Recent studies have demonstrated that adoptive cellular therapy using CCR5 knockout in autologous T cells can achieve sustained HIV control in some patients (126, 127).
Human leukocyte antigen (HLA) class I molecules play important roles in the immune response against HIV, particularly in ECs and PTCs. Genetic variants in HLA-B are strongly correlated with both favorable and adverse outcomes in HIV infection patients. Specifically, certain HLA-B alleles, such as HLA-B*57/58 and HLA-B*27, are associated with favorable immune control, whereas others, such as HLA-B*35, are linked to faster disease progression and higher viral loads (7, 34, 91).
HLA-B*57 and HLA-B*27 are considered protective alleles in HIV infection. These alleles are found more frequently in individuals who are classified as LTNPs and ECs. In ECs, specific HLA class I haplotypes, particularly HLA-B*57-01 and HLA-B*27-05, are notably overrepresented. The presence of these alleles is believed to contribute to the lower viral replication observed in these individuals. This control may be partially attributed to the induction of a robust cell-mediated immune response against HIV, including CD8+ T-cell responses that effectively target HIV epitopes (91).
HLAs are associated with the degree of immune control in HIV-infected patients, especially in elite controls. However, unlike those associated with HIV ECs, the proportion of favorable HLA alleles associated with viral control is not high in PTCs (31). In contrast, a number of PTCs have the HLA-B*35 allele, which is associated with faster progression to AIDS and a greater viral load in general. HLA-B35 haplotype enrichment in PTCs might be explained by its association with other genetic signatures. Moreover, not all HLA-B*57+ or HLA-B*27+ patients become HIV controllers when infected. In different cohorts, a significant proportion of HIV controllers do not carry these protective HLA alleles. For example, 15% to 70% of controllers in some cohorts are not HLA-B*57+ (128). This finding indicates that other factors also contribute to the ability to control HIV replication.
Even among HLA-B*57+ controllers, heterogeneity in the ability of CD8+ T cells to suppress viral replication is observed. Some HLA-B57+ individuals are strong viral suppressors, whereas others are weak suppressors. The frequency of HIV-specific CD8+ T cells is highly dependent on the viral burden in HLA-B*57+ patients (128). In those with a lower viral load (weak suppressors), the frequencies of these cells are much lower, suggesting that the level of antigen stimulation also plays a role in the magnitude of the immune response.
One of the most notable features of both ECs and PTCs is the size and composition of their HIV reservoirs. Understanding these reservoirs is crucial for advancing HIV cure strategies given that the size and integrity of the viral reservoirs directly influence the potential for achieving sustained remission without ART (129). ECs are characterized by a very small HIV reservoir, particularly among CD4+ T cells. This is a common feature observed across natural ECs and PTCs, which underscores the importance of reducing the HIV reservoir size in patients undergoing ART as part of HIV cure efforts.
The activation and clearance of HIV-1 viral reservoirs is an important strategy for the functional cure of AIDS (130, 131). In ECs, HIV integrates into the host genome, but the quantity and genetic integrity of the proviruses in their CD4+ T cells are significantly lower than those in chronic progressors and ART-treated patients (132, 133). Studies have shown that proviral reservoirs of ECs usually consist of oligoclonal to near monoclonal clusters of intact proviral sequences (109). These findings suggest that under immune pressure, ECs may favor the persistence of smaller, less inducible viral reservoirs, avoiding the transcription of intact proviruses. This selective process may help limit viral replication and prevent the activation of viral reservoirs, contributing to the spontaneous control of HIV without ART (134).
In ECs, intact proviruses tend to integrate at distinct sites within the human genome. These sites are located primarily in regions that are distant from actively transcribed chromatin and are densely populated with heterochromatin marks. Notably, proviruses in ECs are often integrated into centromeric satellite DNA or specific genes on chromosome 19, which contain zinc finger nucleases (ZNFs) (109, 135). This pattern of integration aligns with the “block and lock” strategy, wherein the proviral genes are silenced by their chromosomal environment, preventing viral expression and replication (136).
PTCs also demonstrate an unusual HIV reservoir profile (137). The size and distribution of the viral reservoir in PTCs are much smaller than those in ART-treated individuals, with some studies showing that the total and intact proviral reservoirs in PTCs are approximately seven times smaller than those in noncontrollers (31). This characteristic is a contributing factor to their ability to maintain HIV control after ART cessation (17, 138). The VISCONTI study reported that PTCs had low levels of HIV DNA that continued to decrease even after the initiation of ART (11). Despite the interruption of ART, PTCs demonstrate a remarkable ability to restrict HIV transcription. And the control mechanisms may occur before treatment interruption (139). These findings suggest that, like ECs, PTCs also achieve viral control through mechanisms of deep latency and transcriptional silencing (49, 140). Research on nonhuman primates also supports the idea that a smaller HIV reservoir is key to achieving post-treatment control of the virus (141). Research on nonhuman primates also supports the idea that a smaller HIV reservoir is key to achieving post-treatment viral control.
Understanding the unique characteristics of HIV reservoirs in ECs and PTCs is critical for the development of new HIV cure strategies. By accurately characterizing the size, distribution, and integrity of these reservoirs, researchers can explore innovative approaches to target and manipulate the reservoirs, potentially leading to a functional cure for HIV. The concept of targeting latent reservoirs through strategies such as “shock and kill” (activating the latent virus and then eliminating it) has been a central focus of HIV cure research. However, the deep latency observed in ECs and PTCs may provide a new avenue for exploring the “block and lock” approach, wherein proviruses are silenced and prevented from being reactivated, thus contributing to long-term viral control. Future studies should focus on improving methods for accurately measuring HIV reservoirs and understanding the mechanisms that contribute to their small size and high integrity in ECs and PTCs. This will allow researchers to identify novel therapeutic targets and refine strategies for achieving sustained remission or even an eventual cure for HIV.
Numerous studies indicate that both the host and virus influence disease progression following HIV-1 infection, with attenuated viruses playing an important role (Table 3). The number of genome-intact and replication-competent proviruses is significantly reduced in the EC (109, 154). Studies have shown that some LTNPs and ECs have large deletions in the nef gene.
HIV-1 Nef is a small (27–35 kDa) accessory protein that is a crucial auxiliary protein for HIV replication and AIDS development (155). Infection with nef-deficient or nef-defective HIV or SIV strains can lead to a slow or nonprogressive disease phenotype (16, 156). This protein is crucial for interacting with the cellular vesicular trafficking system and interfering with cell signaling. Nef engages with proteins related to intracellular trafficking and alters the expression of various cell surface molecules (157). It has diverse in vitro functions that influence pathogenesis (158), such as downregulating CD4 (159, 160) and HLA-I (161), upregulating the HLA class II invariant chain (CD74) (162–164), and enhancing viral infectivity and replication (165, 166). The impairment of these Nef activities has been documented in ECs. Compared with those obtained from individuals with chronic progressive infections, Nef clones from ECs presented a markedly reduced capacity to downregulate CD4 (142, 166, 167). Nef facilitates CD4 downregulation by promoting its internalization into the endosome–lysosome compartment, a conserved function that persists during disease progression, thereby increasing viral infectivity and replication.
In general, EC Nef clones are functional; however, Nef clones of HLA-B* 57-expressing ECs may be influenced by host immune selection pressure, resulting in diminished Nef function and a hallmark of the EC phenotype (167). Research has compared chromosomal integration sites and escape mutations between ECs and AIDS patients who need to receive antiretroviral therapy. The team observed differences in HIV integration sites. Intact and defective HIV proviruses from ECs showed a reduced frequency of escape mutations in cytotoxic T-cell epitopes and antibody contact regions. Approximately 15% of intact HIV proviruses in ECs exhibit a nef deletion, indicating heightened viral susceptibility to host immune responses due to Nef dysfunction (110).
HIV ECs and PTCs represent important models of sustained HIV remission without ART. ECs represent individuals who are able to naturally suppress HIV replication to undetectable levels without ART. PTCs could represent a more concrete objective for research focused on attaining HIV remission even after ART is stopped. Research on these two groups can provide insights into the immune and genetic factors that enable natural HIV control, which is key for developing therapies that replicate this process in other individuals.
This review outlines the immunological characteristics, genetic variations, and HIV reservoirs associated with HIV ECs and PTCs. Recognizing traits linked to virological control can help identify candidates before ART cessation, whereas targeted mechanistic studies may guide the development of HIV remission therapies. Future studies on immune responses in HIV controllers, for example, could offer valuable insights for discovering new targets for the natural control of HIV-1 infection. ART-free remission is a key goal in HIV research, where patients can maintain undetectable viral loads without the need for ART. ECs and PTCs offer a blueprint for what this could look like. Therapies designed to mimic the immune and genetic traits of ECs and PTCs could offer a path to achieve ART-free remission for a broader population, including harnessing the immune system and genome editing methods for effective virus control.
Although the field of HIV remission without ART has great promise, significant challenges remain, particularly in understanding the complex interplay between genetic, immune, and virological factors. Significant variability in how individuals within these groups respond to HIV is noted, making it difficult to pinpoint universal biomarkers or treatment approaches. Another major limitation in this field is the lack of large, well-characterized cohorts of ECs and PTCs, which hampers the identification of common immunological and genetic traits.
Future research should focus on immune mechanisms, genetic variations, and targeted therapies to eradicate HIV reservoirs, which is crucial in advancing treatment strategies and potentially paves the way for a functional cure. The next steps will involve translating the lessons learned from ECs and PTCs into tangible therapies that could benefit the broader HIV-positive population.
ZD: Data curation, Investigation, Writing – original draft. HY: Investigation, Visualization, Writing – original draft. OL: Conceptualization, Validation, Writing – review & editing. CM: Conceptualization, Investigation, Validation, Writing – review & editing. BS: Conceptualization, Data curation, Funding acquisition, Investigation, Project administration, Supervision, Validation, Writing – review & editing.
The author(s) declare that financial support was received for the research, authorship, and/or publication of this article. This work was supported by the National Key R&D Program of China (2023YFE0116000, 2023YFC2308300, 2023YFC2308302, 2021YFC2301900, 2021YFC2301905), the National Natural Science Foundation of China (NSFC, 82472266), the Beijing Natural Science Foundation (Z220018), the High-Level Public Health Specialized Talents Project of Beijing Municipal Health Commission (2022-2-018), the Beijing Key Laboratory for HIV/AIDS Research (BZ0089), and the ANRS (Agence Nationale de Recherches sur le SIDA et les hépatites virales) Investissements d’Avenir program managed by the ANR under reference ANR-10-LABX-77 and EHVA (N°681032, Horizon 2020). The funders had no role in study design, data collection and analysis, decision to publish, or preparation of the manuscript.
The authors declare that the research was conducted in the absence of any commercial or financial relationships that could be construed as a potential conflict of interest.
The author(s) declared that they were an editorial board member of Frontiers, at the time of submission. This had no impact on the peer review process and the final decision.
The author(s) declare that no Generative AI was used in the creation of this manuscript.
All claims expressed in this article are solely those of the authors and do not necessarily represent those of their affiliated organizations, or those of the publisher, the editors and the reviewers. Any product that may be evaluated in this article, or claim that may be made by its manufacturer, is not guaranteed or endorsed by the publisher.
1. Li JZ, Aga E, Bosch RJ, Pilkinton M, Kroon E, MacLaren L, et al. Time to viral rebound after interruption of modern antiretroviral therapies. Clin Infect diseases: an Off Publ Infect Dis Soc America. (2022) 74:865–70. doi: 10.1093/cid/ciab541
2. Sneller MC, Huiting ED, Clarridge KE, Seamon C, Blazkova J, Justement JS, et al. Kinetics of plasma hiv rebound in the era of modern antiretroviral therapy. J Infect Dis. (2020) 222:1655–9. doi: 10.1093/infdis/jiaa270
3. Deeks SG, Archin N, Cannon P, Collins S, Jones RB, de Jong M, et al. Research priorities for an hiv cure: international aids society global scientific strategy 2021. Nat Med. (2021) 27:2085–98. doi: 10.1038/s41591-021-01590-5
4. Davenport MP, Khoury DS, Cromer D, Lewin SR, Kelleher AD, Kent SJ. Functional cure of hiv: the scale of the challenge. Nat Rev Immunol. (2019) 19:45–54. doi: 10.1038/s41577-018-0085-4
5. O’Connell KA, Bailey JR, Blankson JN. Elucidating the elite: mechanisms of control in hiv-1 infection. Trends Pharmacol Sci. (2009) 30:631–7. doi: 10.1016/j.tips.2009.09.005
6. Kwaa AK, Blankson JN. Immune responses in controllers of hiv infection. Annu Rev Immunol. (2024) 42:21–33. doi: 10.1146/annurev-immunol-083122-035233
7. Pereyra F, Jia X, McLaren PJ, Telenti A, de Bakker PI, Walker BD, et al. The major genetic determinants of hiv-1 control affect hla class I peptide presentation. Sci (New York NY). (2010) 330:1551–7. doi: 10.1126/science.1195271
8. Okulicz JF, Marconi VC, Landrum ML, Wegner S, Weintrob A, Ganesan A, et al. Clinical outcomes of elite controllers, viremic controllers, and long-term nonprogressors in the us department of defense hiv natural history study. J Infect Dis. (2009) 200:1714–23. doi: 10.1086/646609
9. Grabar S, Selinger-Leneman H, Abgrall S, Pialoux G, Weiss L, Costagliola D. Prevalence and comparative characteristics of long-term nonprogressors and hiv controller patients in the french hospital database on hiv. AIDS (London England). (2009) 23:1163–9. doi: 10.1097/QAD.0b013e32832b44c8
10. Salgado M, Migueles SA, Yu XG, Martinez-Picado J. Exceptional, naturally occurring hiv-1 control: insight into a functional cure. Med (New York NY). (2024) 5:1071–82. doi: 10.1016/j.medj.2024.06.008
11. Sáez-Cirión A, Bacchus C, Hocqueloux L, Avettand-Fenoel V, Girault I, Lecuroux C, et al. Post-treatment hiv-1 controllers with a long-term virological remission after the interruption of early initiated antiretroviral therapy anrs visconti study. PloS Pathog. (2013) 9:e1003211. doi: 10.1371/journal.ppat.1003211
12. Goujard C, Girault I, Rouzioux C, Lécuroux C, Deveau C, Chaix ML, et al. Hiv-1 control after transient antiretroviral treatment initiated in primary infection: role of patient characteristics and effect of therapy. Antiviral Ther. (2012) 17:1001–9. doi: 10.3851/imp2273
13. Goulder Philip JR, Walker Bruce D. Hiv and hla class I: an evolving relationship. Immunity. (2012) 37:426–40. doi: 10.1016/j.immuni.2012.09.005
14. Brumme ZL, Li C, Miura T, Sela J, Rosato PC, Brumme CJ, et al. Reduced replication capacity of nl4-3 recombinant viruses encoding reverse transcriptase-integrase sequences from hiv-1 elite controllers. J acquired Immune deficiency syndromes (1999). (2011) 56:100–8. doi: 10.1097/QAI.0b013e3181fe9450
15. Shea PR, Shianna KV, Carrington M, Goldstein DB. Host genetics of hiv acquisition and viral control. Annu Rev Med. (2013) 64:203–17. doi: 10.1146/annurev-med-052511-135400
16. Deacon NJ, Tsykin A, Solomon A, Smith K, Ludford-Menting M, Hooker DJ, et al. Genomic structure of an attenuated quasi species of hiv-1 from a blood transfusion donor and recipients. Science. (1995) 270:988–91. doi: 10.1126/science.270.5238.988
17. Cockerham LR, Hatano H, Deeks SG. Post-treatment controllers: role in hiv “Cure” Research. Curr HIV/AIDS Rep. (2016) 13:1–9. doi: 10.1007/s11904-016-0296-x
18. Etemad B, Sun X, Li Y, Melberg M, Moisi D, Gottlieb R, et al. Hiv post-treatment controllers have distinct immunological and virological features. Proc Natl Acad Sci United States America. (2023) 120:e2218960120. doi: 10.1073/pnas.2218960120
19. Yang X, Su B, Zhang X, Liu Y, Wu H, Zhang T. Incomplete immune reconstitution in hiv/aids patients on antiretroviral therapy: challenges of immunological non-responders. J leukocyte Biol. (2020) 107:597–612. doi: 10.1002/JLB.4MR1019-189R
20. Collins DR, Gaiha GD, Walker BD. Cd8(+) T cells in hiv control, cure and prevention. Nat Rev Immunol. (2020) 20:471–82. doi: 10.1038/s41577-020-0274-9
21. Mastrangelo A, Banga R, Perreau M. Elite and posttreatment controllers, two facets of hiv control. Curr Opin HIV AIDS. (2022) 17:325–32. doi: 10.1097/coh.0000000000000751
22. Benati D, Galperin M, Lambotte O, Gras S, Lim A, Mukhopadhyay M, et al. Public T cell receptors confer high-avidity cd4 responses to hiv controllers. J Clin Invest. (2016) 126:2093–108. doi: 10.1172/jci83792
23. Bansal A, Sterrett S, Erdmann N, Westfall AO, Dionne-Odom J, Overton ET, et al. Normal T-cell activation in elite controllers with preserved cd4+ T-cell counts. AIDS (London England). (2015) 29:2245–54. doi: 10.1097/qad.0000000000000860
24. Pereyra F, Addo MM, Kaufmann DE, Liu Y, Miura T, Rathod A, et al. Genetic and immunologic heterogeneity among persons who control hiv infection in the absence of therapy. J Infect Dis. (2008) 197:563–71. doi: 10.1086/526786
25. Lambotte O, Ferrari G, Moog C, Yates NL, Liao HX, Parks RJ, et al. Heterogeneous neutralizing antibody and antibody-dependent cell cytotoxicity responses in hiv-1 elite controllers. AIDS (London England). (2009) 23:897–906. doi: 10.1097/QAD.0b013e328329f97d
26. Madhavi V, Wines B, Wines B, Wines B, Amin J, Emery S, et al. Hiv-1 env- and vpu-specific antibody-dependent cellular cytotoxicity responses associated with elite control of hiv. J Virol. (2017) 91(18):e00700-17. doi: 10.1128/JVI.00700-17
27. Molinos-Albert LM, Baquero E, Bouvin-Pley M, Lorin V, Charre C, Planchais C, et al. Anti-V1/V3-glycan broadly hiv-1 neutralizing antibodies in a post-treatment controller. Cell Host Microbe. (2023) 31:1275–87.e8. doi: 10.1016/j.chom.2023.06.006
28. Molinos-Albert LM, Lorin V, Monceaux V, Orr S, Essat A, Dufloo J, et al. Transient viral exposure drives functionally-coordinated humoral immune responses in hiv-1 post-treatment controllers. Nat Commun. (2022) 13:1944. doi: 10.1038/s41467-022-29511-1
29. Taborda NA, González SM, Alvarez CM, Correa LA, Montoya CJ, Rugeles MT. Higher frequency of nk and cd4+ T-cells in mucosa and potent cytotoxic response in hiv controllers. PloS One. (2015) 10:e0136292. doi: 10.1371/journal.pone.0136292
30. Scott-Algara. D, Didier. C, Arnold. V, Cummings. J-S, Boufassa. F, Lambotte. O, et al. (2015). “Post-treatment controllers have particular nk cells with high anti-hiv capacity: visconti study,” in: Program and Abstracts of CORI 2015. Conference on Retroviruses and Opportunistic Infections. Seattle, Washington: CROI.
31. Sharaf R, Lee GQ, Sun X, Etemad B, Aboukhater LM, Hu Z, et al. Hiv-1 proviral landscapes distinguish posttreatment controllers from noncontrollers. J Clin Invest. (2018) 128:4074–85. doi: 10.1172/jci120549
32. Hor JL, Whitney PG, Zaid A, Brooks AG, Heath WR, Mueller SN. Spatiotemporally distinct interactions with dendritic cell subsets facilitates cd4+ and cd8+ T cell activation to localized viral infection. Immunity. (2015) 43:554–65. doi: 10.1016/j.immuni.2015.07.020
33. López-Bravo M, Ardavín C. In vivo induction of immune responses to pathogens by conventional dendritic cells. Immunity. (2008) 29:343–51. doi: 10.1016/j.immuni.2008.08.008
34. Fellay J, Shianna KV, Ge D, Colombo S, Ledergerber B, Weale M, et al. A whole-genome association study of major determinants for host control of hiv-1. Sci (New York NY). (2007) 317:944–7. doi: 10.1126/science.1143767
35. Migueles SA, Sabbaghian MS, Shupert WL, Bettinotti MP, Marincola FM, Martino L, et al. Hla B*5701 is highly associated with restriction of virus replication in a subgroup of hiv-infected long term nonprogressors. Proc Natl Acad Sci U.S.A. (2000) 97:2709–14. doi: 10.1073/pnas.050567397
36. Migueles SA, Laborico AC, Shupert WL, Sabbaghian MS, Rabin R, Hallahan CW, et al. Hiv-specific cd8+ T cell proliferation is coupled to perforin expression and is maintained in nonprogressors. Nat Immunol. (2002) 3:1061–8. doi: 10.1038/ni845
37. Betts MR, Nason MC, West SM, De Rosa SC, Migueles SA, Abraham J, et al. Hiv nonprogressors preferentially maintain highly functional hiv-specific cd8+ T cells. Blood. (2006) 107:4781–9. doi: 10.1182/blood-2005-12-4818
38. Collins DR, Hitschfel J, Urbach JM, Mylvaganam GH, Ly NL, Arshad U, et al. Cytolytic cd8+T cells infiltrate germinal centers to limit ongoing hiv replication in spontaneous controller lymph nodes. Sci Immunol. (2023) 8(83):eade5872. doi: 10.1126/sciimmunol.ade5872
39. Monel B, McKeon A, Lamothe-Molina P, Jani P, Boucau J, Pacheco Y, et al. Hiv controllers exhibit effective cd8(+) T cell recognition of hiv-1-infected non-activated cd4(+) T cells. Cell Rep. (2019) 27:142–53.e4. doi: 10.1016/j.celrep.2019.03.016
40. Buckheit RW 3rd, Siliciano RF, Blankson JN. Primary cd8+ T cells from elite suppressors effectively eliminate non-productively hiv-1 infected resting and activated cd4+ T cells. Retrovirology. (2013) 10:68. doi: 10.1186/1742-4690-10-68
41. Sáez-Cirión A, Lacabaratz C, Lambotte O, Versmisse P, Urrutia A, Boufassa F, et al. Hiv controllers exhibit potent cd8 T cell capacity to suppress hiv infection ex vivo and peculiar cytotoxic T lymphocyte activation phenotype. Proc Natl Acad Sci United States America. (2007) 104:6776–81. doi: 10.1073/pnas.0611244104
42. Migueles SA, Osborne CM, Royce C, Compton AA, Joshi RP, Weeks KA, et al. Lytic granule loading of cd8+ T cells is required for hiv-infected cell elimination associated with immune control. Immunity. (2008) 29:1009–21. doi: 10.1016/j.immuni.2008.10.010
43. Hersperger AR, Pereyra F, Nason M, Demers K, Sheth P, Shin LY, et al. Perforin expression directly ex vivo by hiv-specific cd8 T-cells is a correlate of hiv elite control. PloS Pathog. (2010) 6:e1000917. doi: 10.1371/journal.ppat.1000917
44. Angin M, Volant S, Passaes C, Lecuroux C, Monceaux V, Dillies MA, et al. Metabolic plasticity of hiv-specific cd8(+) T cells is associated with enhanced antiviral potential and natural control of hiv-1 infection. Nat Metab. (2019) 1:704–16. doi: 10.1038/s42255-019-0081-4
45. Nguyen S, Deleage C, Darko S, Ransier A, Truong DP, Agarwal D, et al. Elite control of hiv is associated with distinct functional and transcriptional signatures in lymphoid tissue cd8(+) T cells. Sci Trans Med. (2019) 11(523):eaax4077. doi: 10.1126/scitranslmed.aax4077
46. Bailey JR, Williams TM, Siliciano RF, Blankson JN. Maintenance of viral suppression in hiv-1-infected hla-B*57+ Elite suppressors despite ctl escape mutations. J Exp Med. (2006) 203:1357–69. doi: 10.1084/jem.20052319
47. Bansal A, Carlson J, Yan J, Akinsiku OT, Schaefer M, Sabbaj S, et al. Cd8 T cell response and evolutionary pressure to hiv-1 cryptic epitopes derived from antisense transcription. J Exp Med. (2010) 207:51–9. doi: 10.1084/jem.20092060
48. Alves E, Al-Kaabi M, Keane NM, Leary S, Almeida CM, Deshpande P, et al. Adaptation to hla-associated immune pressure over the course of hiv infection and in circulating hiv-1 strains. PloS Pathog. (2022) 18:e1010965. doi: 10.1371/journal.ppat.1010965
49. Blazkova J, Gao F, Marichannegowda MH, Justement JS, Shi V, Whitehead EJ, et al. Distinct mechanisms of long-term virologic control in two hiv-infected individuals after treatment interruption of anti-retroviral therapy. Nat Med. (2021) 27:1893–8. doi: 10.1038/s41591-021-01503-6
50. Xue J, Chong H, Zhu Y, Zhang J, Tong L, Lu J, et al. Efficient treatment and pre-exposure prophylaxis in rhesus macaques by an hiv fusion-inhibitory lipopeptide. Cell. (2022) 185:131–44 e18. doi: 10.1016/j.cell.2021.11.032
51. Jacobs ES, Keating SM, Abdel-Mohsen M, Gibb SL, Heitman JW, Inglis HC, et al. Cytokines elevated in hiv elite controllers reduce hiv replication in vitro and modulate hiv restriction factor expression. J Virol. (2017) 91(6):e02051-16. doi: 10.1128/jvi.02051-16
52. Yero A, Bouassa R-SM, Ancuta P, Estaquier J, Jenabian M-AJC, Reviews GF. Immuno-metabolic control of the balance between th17-polarized and regulatory T-cells during hiv infection. Cytokine Growth Factor Rev. (2023) 69:1–13. doi: 10.1016/j.cytogfr.2023.01.001
53. Caetano DG, de Paula HHS, Bello G, Hoagland B, Villela LM, Grinsztejn B, et al. Hiv-1 elite controllers present a high frequency of activated regulatory T and th17 cells. PloS One. (2020) 15:e0228745. doi: 10.1371/journal.pone.0228745
54. Morou A, Brunet-Ratnasingham E, Dubé M, Charlebois R, Mercier E, Darko S, et al. Altered differentiation is central to hiv-specific cd4(+) T cell dysfunction in progressive disease. Nat Immunol. (2019) 20:1059–70. doi: 10.1038/s41590-019-0418-x
55. Su B, Dispinseri S, Iannone V, Zhang T, Wu H, Carapito R, et al. Update on fc-mediated antibody functions against hiv-1 beyond neutralization. Front Immunol. (2019) 10:2968. doi: 10.3389/fimmu.2019.02968
56. Su B, Moog C. Which antibody functions are important for an hiv vaccine? Front Immunol. (2014) 5:289. doi: 10.3389/fimmu.2014.00289
57. Moris A, Pereira M, Chakrabarti L. A role for antibodies in natural hiv control. Curr Opin HIV AIDS. (2019) 14 4:265–72. doi: 10.1097/COH.0000000000000554
58. Ackerman ME, Mikhailova A, Brown EP, Dowell KG, Walker BD, Bailey-Kellogg C, et al. Polyfunctional hiv-specific antibody responses are associated with spontaneous hiv control. PloS Pathog. (2016) 12:e1005315. doi: 10.1371/journal.ppat.1005315
59. Nyanhete TE, Edwards RJ, LaBranche CC, Mansouri K, Eaton A, Dennison SM, et al. Polyclonal broadly neutralizing antibody activity characterized by cd4 binding site and V3-glycan antibodies in a subset of hiv-1 virus controllers. Front Immunol. (2021) 12:670561. doi: 10.3389/fimmu.2021.670561
60. Lambotte O, Pollara J, Boufassa F, Moog C, Venet A, Haynes BF, et al. High antibody-dependent cellular cytotoxicity responses are correlated with strong cd8 T cell viral suppressive activity but not with B57 status in hiv-1 elite controllers. PloS One. (2013) 8:e74855. doi: 10.1371/journal.pone.0074855
61. Alsahafi N, Ding S, Richard J, Markle T, Brassard N, Walker B, et al. Nef proteins from hiv-1 elite controllers are inefficient at preventing antibody-dependent cellular cytotoxicity. J Virol. (2016) 90:2993–3002. doi: 10.1128/jvi.02973-15
62. Rouers A, Klingler J, Su B, Samri A, Laumond G, Even S, et al. Hiv-specific B cell frequency correlates with neutralization breadth in patients naturally controlling hiv-infection. EBioMedicine. (2017) 21:158–69. doi: 10.1016/j.ebiom.2017.05.029
63. Mouquet H. Humoral immunity in hiv-1 post-treatment controllers. Curr Opin HIV AIDS. (2025) 20:80–5. doi: 10.1097/coh.0000000000000893
64. Pohlmeyer CW, Gonzalez VD, Irrinki A, Ramirez RN, Li L, Mulato A, et al. Identification of nk cell subpopulations that differentiate hiv-infected subject cohorts with diverse levels of virus control. J Virol. (2019) 93(7):e01790-18. doi: 10.1128/jvi.01790-18
65. Martin MP, Gao X, Lee JH, Nelson GW, Detels R, Goedert JJ, et al. Epistatic interaction between kir3ds1 and hla-B delays the progression to aids. Nat Genet. (2002) 31:429–34. doi: 10.1038/ng934
66. Martin MP, Qi Y, Gao X, Yamada E, Martin JN, Pereyra F, et al. Innate partnership of hla-B and kir3dl1 subtypes against hiv-1. Nat Genet. (2007) 39:733–40. doi: 10.1038/ng2035
67. Marras F, Casabianca A, Bozzano F, Ascierto ML, Orlandi C, Di Biagio A, et al. Control of the hiv-1 DNA reservoir is associated in vivo and in vitro with nkp46/nkp30 (Cd335 cd337) inducibility and interferon gamma production by transcriptionally unique nk cells. J Virol. (2017) 91(23):e00647-17. doi: 10.1128/jvi.00647-17
68. Huot N, Jacquelin B, Garcia-Tellez T, Rascle P, Ploquin MJ, Madec Y, et al. Natural killer cells migrate into and control simian immunodeficiency virus replication in lymph node follicles in african green monkeys. Nat Med. (2017) 23:1277–86. doi: 10.1038/nm.4421
69. Zhang Z, Zhou Y, Lu J, Chen YF, Hu HY, Xu XQ, et al. Changes in nk cell subsets and receptor expressions in hiv-1 infected chronic patients and hiv controllers. Front Immunol. (2021) 12:792775. doi: 10.3389/fimmu.2021.792775
70. Mavilio D, Lombardo G, Kinter A, Fogli M, La Sala A, Ortolano S, et al. Characterization of the defective interaction between a subset of natural killer cells and dendritic cells in hiv-1 infection. J Exp Med. (2006) 203:2339–50. doi: 10.1084/jem.20060894
71. Alsahafi N, Richard J, Prévost J, Coutu M, Brassard N, Parsons MS, et al. Impaired downregulation of nkg2d ligands by nef proteins from elite controllers sensitizes hiv-1-infected cells to antibody-dependent cellular cytotoxicity. J Virol. (2017) 91(16):e00109-17. doi: 10.1128/jvi.00109-17
72. Miller JS, Davis ZB, Helgeson E, Reilly C, Thorkelson A, Anderson J, et al. Safety and virologic impact of the il-15 superagonist N-803 in people living with hiv: A phase 1 trial. Nat Med. (2022) 28:392–400. doi: 10.1038/s41591-021-01651-9
73. Hamimi C, David A, Versmisse P, Weiss L, Bruel T, Zucman D, et al. Dendritic cells from hiv controllers have low susceptibility to hiv-1 infection in vitro but high capacity to capture hiv-1 particles. PloS One. (2016) 11:e0160251. doi: 10.1371/journal.pone.0160251
74. Martin-Gayo E, Cole MB, Kolb KE, Ouyang Z, Cronin J, Kazer SW, et al. A reproducibility-based computational framework identifies an inducible, enhanced antiviral state in dendritic cells from hiv-1 elite controllers. Genome Biol. (2018) 19:10. doi: 10.1186/s13059-017-1385-x
75. Coindre S, Tchitchek N, Alaoui L, Vaslin B, Bourgeois C, Goujard C, et al. Mass cytometry analysis reveals complex cell-state modifications of blood myeloid cells during hiv infection. Front Immunol. (2019) 10:2677. doi: 10.3389/fimmu.2019.02677
76. Huang J, Burke PS, Cung TD, Pereyra F, Toth I, Walker BD, et al. Leukocyte immunoglobulin-like receptors maintain unique antigen-presenting properties of circulating myeloid dendritic cells in hiv-1-infected elite controllers. J Virol. (2010) 84:9463–71. doi: 10.1128/jvi.01009-10
77. Martin-Gayo E, Buzon MJ, Ouyang Z, Hickman T, Cronin J, Pimenova D, et al. Potent cell-intrinsic immune responses in dendritic cells facilitate hiv-1-specific T cell immunity in hiv-1 elite controllers. PloS Pathog. (2015) 11:e1004930. doi: 10.1371/journal.ppat.1004930
78. Martin-Gayo E, Yu XG. Dendritic cell immune responses in hiv-1 controllers. Curr HIV/AIDS Rep. (2017) 14:1–7. doi: 10.1007/s11904-017-0345-0
79. Su B, Biedma ME, Lederle A, Peressin M, Lambotin M, Proust A, et al. Dendritic cell-lymphocyte cross talk downregulates host restriction factor samhd1 and stimulates hiv-1 replication in dendritic cells. J Virol. (2014) 88:5109–21. doi: 10.1128/jvi.03057-13
80. Yang Z, Greene WC. A new activity for samhd1 in hiv restriction. Nat Med. (2014) 20:808–9. doi: 10.1038/nm.3657
81. Lahouassa H, Daddacha W, Hofmann H, Ayinde D, Logue EC, Dragin L, et al. Samhd1 restricts the replication of human immunodeficiency virus type 1 by depleting the intracellular pool of deoxynucleoside triphosphates. Nat Immunol. (2012) 13:223–8. doi: 10.1038/ni.2236
82. Ryoo J, Choi J, Oh C, Kim S, Seo M, Kim SY, et al. The ribonuclease activity of samhd1 is required for hiv-1 restriction. Nat Med. (2014) 20:936–41. doi: 10.1038/nm.3626
83. Lahaye X, Satoh T, Gentili M, Cerboni S, Conrad C, Hurbain I, et al. The capsids of hiv-1 and hiv-2 determine immune detection of the viral cdna by the innate sensor cgas in dendritic cells. Immunity. (2013) 39:1132–42. doi: 10.1016/j.immuni.2013.11.002
84. Berg RK, Melchjorsen J, Rintahaka J, Diget E, Søby S, Horan KA, et al. Genomic hiv rna induces innate immune responses through rig-I-dependent sensing of secondary-structured rna. PloS One. (2012) 7:e29291. doi: 10.1371/journal.pone.0029291
85. Martin-Gayo E, Gao C, Calvet-Mirabent M, Ouyang Z, Lichterfeld M, Yu XG. Cooperation between cgas and rig-I sensing pathways enables improved innate recognition of hiv-1 by myeloid dendritic cells in elite controllers. Front Immunol. (2022) 13:1017164. doi: 10.3389/fimmu.2022.1017164
86. Tel J, Schreibelt G, Sittig SP, Mathan TS, Buschow SI, Cruz LJ, et al. Human plasmacytoid dendritic cells efficiently cross-present exogenous ags to cd8+ T cells despite lower ag uptake than myeloid dendritic cell subsets. Blood. (2013) 121:459–67. doi: 10.1182/blood-2012-06-435644
87. Colonna M, Trinchieri G, Liu YJ. Plasmacytoid dendritic cells in immunity. Nat Immunol. (2004) 5:1219–26. doi: 10.1038/ni1141
88. Bosinger SE, Utay NS. Type I interferon: understanding its role in hiv pathogenesis and therapy. Curr HIV/AIDS Rep. (2015) 12:41–53. doi: 10.1007/s11904-014-0244-6
89. Barblu L, Machmach K, Gras C, Delfraissy JF, Boufassa F, Leal M, et al. Plasmacytoid Dendritic Cells (Pdcs) from Hiv Controllers Produce Interferon-α and Differentiate into Functional Killer Pdcs under Hiv Activation. J Infect Dis. (2012) 206:790–801. doi: 10.1093/infdis/jis384
90. Machmach K, Leal M, Gras C, Viciana P, Genebat M, Franco E, et al. Plasmacytoid dendritic cells reduce hiv production in elite controllers. J Virol. (2012) 86:4245–52. doi: 10.1128/jvi.07114-11
91. Lunardi LW, Bragatte MAS, Vieira GF. The influence of hla/hiv genetics on the occurrence of elite controllers and a need for therapeutics geotargeting view. Braz J Infect diseases: an Off Publ Braz Soc Infect Dis. (2021) 25:101619. doi: 10.1016/j.bjid.2021.101619
92. Vollmers S, Lobermeyer A, Niehrs A, Fittje P, Indenbirken D, Nakel J, et al. Host kir/hla-C genotypes determine hiv-mediated changes of the nk cell repertoire and are associated with vpu sequence variations impacting downmodulation of hla-C. Front Immunol. (2022) 13:922252. doi: 10.3389/fimmu.2022.922252
93. Zhang X, Lu X, Moog C, Yuan L, Liu Z, Li Z, et al. Kir3dl1-negative cd8 T cells and kir3dl1-negative natural killer cells contribute to the advantageous control of early human immunodeficiency virus type 1 infection in hla-B bw4 homozygous individuals. Front Immunol. (2018) 9:1855. doi: 10.3389/fimmu.2018.01855
94. Habegger de Sorrentino A, Sinchi JL, Marinic K, López R, Iliovich E. Kir-hla-a and B alleles of the bw4 epitope against hiv infection in discordant heterosexual couples in chaco Argentina. Immunology. (2013) 140:273–9. doi: 10.1111/imm.12137
95. Ranasinghe S, Cutler S, Davis I, Lu R, Soghoian DZ, Qi Y, et al. Association of hla-drb1-restricted cd4+ T cell responses with hiv immune control. Nat Med. (2013) 19:930–3. doi: 10.1038/nm.3229
96. Celerino da Silva R, Segat L, Kuhn L, Chies JAB, Crovella S. Association of snps in hla-C and znrd1 genes with hiv-1 mother-to-child transmission in Zambia population. J acquired Immune deficiency syndromes (1999). (2021) 86:509–15. doi: 10.1097/qai.0000000000002584
97. Celerino da Silva R, Moura RR, Victor Campos Coelho A, Arraes LC, Brandão LAC, Crovella S, et al. Hla-C single nucleotide polymorphism associated with increased viral load level in hiv-1 infected individuals from northeast Brazil. Curr HIV Res. (2017) 15:266–72. doi: 10.2174/1570162x15666170511141741
98. Herráiz-Nicuesa L, Hernández-Flórez DC, Valor L, García-Consuegra S, Navarro-Valdivieso JP, Fernández-Cruz E, et al. Impact of the polymorphism rs9264942 near the hla-C gene on hiv-1 DNA reservoirs in asymptomatic chronically infected patients initiating antiviral therapy. J Immunol Res. (2017) 2017:8689313. doi: 10.1155/2017/8689313
99. Le Clerc S, Delaneau O, Coulonges C, Spadoni JL, Labib T, Laville V, et al. Evidence after imputation for a role of mica variants in nonprogression and elite control of hiv type 1 infection. J Infect Dis. (2014) 210:1946–50. doi: 10.1093/infdis/jiu342
100. Blaauw MJT, Cristina Dos Santos J, Vadaq N, Trypsteen W, van der Heijden W, Groenendijk A, et al. Targeted plasma proteomics identifies mica and il1r1 proteins associated with hiv-1 reservoir size. iScience. (2023) 26:106486. doi: 10.1016/j.isci.2023.106486
101. Gao X, Single RM, Karacki P, Marti D, O’Brien SJ, Carrington M. Diversity of mica and linkage disequilibrium with hla-B in two north american populations. Hum Immunol. (2006) 67:152–8. doi: 10.1016/j.humimm.2006.02.009
102. Real LM, Sáez ME, Corma-Gómez A, Gonzalez-Pérez A, Thorball C, Ruiz R, et al. A metagenome-wide association study of hiv disease progression in hiv controllers. iScience. (2023) 26:107214. doi: 10.1016/j.isci.2023.107214
103. Yoon W, Ma BJ, Fellay J, Huang W, Xia SM, Zhang R, et al. A polymorphism in the hcp5 gene associated with hla-B*5701 does not restrict hiv-1 in vitro. AIDS (London England). (2010) 24:155–7. doi: 10.1097/QAD.0b013e32833202f5
104. van Manen D, Kootstra NA, Boeser-Nunnink B, Handulle MA, van’t Wout AB, Schuitemaker H. Association of hla-C and hcp5 gene regions with the clinical course of hiv-1 infection. AIDS (London England). (2009) 23:19–28. doi: 10.1097/QAD.0b013e32831db247
105. Han Y, Lai J, Barditch-Crovo P, Gallant JE, Williams TM, Siliciano RF, et al. The role of protective hcp5 and hla-C associated polymorphisms in the control of hiv-1 replication in a subset of elite suppressors. AIDS (London England). (2008) 22:541–4. doi: 10.1097/QAD.0b013e3282f470e4
106. Catano G, Kulkarni H, He W, Marconi VC, Agan BK, Landrum M, et al. Hiv-1 disease-influencing effects associated with znrd1, hcp5 and hla-C alleles are attributable mainly to either hla-A10 or hla-B*57 alleles. PloS One. (2008) 3:e3636. doi: 10.1371/journal.pone.0003636
107. Ballana E, Senserrich J, Pauls E, Faner R, Mercader JM, Uyttebroeck F, et al. Znrd1 (Zinc ribbon domain-containing 1) is a host cellular factor that influences hiv-1 replication and disease progression. Clin Infect diseases: an Off Publ Infect Dis Soc America. (2010) 50:1022–32. doi: 10.1086/651114
108. An P, Goedert JJ, Donfield S, Buchbinder S, Kirk GD, Detels R, et al. Regulatory variation in hiv-1 dependency factor znrd1 associates with host resistance to hiv-1 acquisition. J Infect Dis. (2014) 210:1539–48. doi: 10.1093/infdis/jiu291
109. Jiang C, Lian X, Gao C, Sun X, Einkauf KB, Chevalier JM, et al. Distinct viral reservoirs in individuals with spontaneous control of hiv-1. Nature. (2020) 585:261–7. doi: 10.1038/s41586-020-2651-8
110. Lian X, Gao C, Sun X, Jiang C, Einkauf KB, Seiger KW, et al. Signatures of immune selection in intact and defective proviruses distinguish hiv-1 elite controllers. Sci Trans Med. (2021) 13:eabl4097. doi: 10.1126/scitranslmed.abl4097
111. Maldarelli F, Wu X, Su L, Simonetti FR, Shao W, Hill S, et al. Hiv latency. Specific hiv integration sites are linked to clonal expansion and persistence of infected cells. Sci (New York NY). (2014) 345:179–83. doi: 10.1126/science.1254194
112. Claireaux M, Robinot R, Kervevan J, Patgaonkar M, Staropoli I, Brelot A, et al. Low ccr5 expression protects hiv-specific cd4+ T cells of elite controllers from viral entry. Nat Commun. (2022) 13(1):521. doi: 10.1038/s41467-022-28130-0
113. Zaunders J, Dyer WB, Churchill M, Munier CML, Cunningham PH, Suzuki K, et al. Possible clearance of transfusion-acquired nef/ltr-deleted attenuated hiv-1 infection by an elite controller with ccr5 Δ32 heterozygous and hla-B57 genotype. J Virus eradication. (2019) 5:73–83. doi: 10.1016/s2055-6640(20)30696-11
114. Nyiro B, Amanya SB, Bayiyana A, Wasswa F, Nabulime E, Kayongo A, et al. Reduced ccr5 expression among Uganda hiv controllers. Retrovirology. (2023) 20:8. doi: 10.1186/s12977-023-00626-7
115. Lafferty MK, Sun L, Christensen-Quick A, Lu W, Garzino-Demo A. Human beta defensin 2 selectively inhibits hiv-1 in highly permissive ccr6+Cd4+ T cells. Viruses. (2017) 9(5):111. doi: 10.3390/v9050111
116. Zhang W, Ambikan AT, Sperk M, van Domselaar R, Nowak P, Noyan K, et al. Transcriptomics and targeted proteomics analysis to gain insights into the immune-control mechanisms of hiv-1 infected elite controllers. EBioMedicine. (2018) 27:40–50. doi: 10.1016/j.ebiom.2017.11.031
117. Limou S, Coulonges C, Herbeck JT, van Manen D, An P, Le Clerc S, et al. Multiple-cohort genetic association study reveals cxcr6 as a new chemokine receptor involved in long-term nonprogression to aids. J Infect Dis. (2010) 202:908–15. doi: 10.1086/655782
118. Picton ACP, Paximadis M, Chaisson RE, Martinson NA, Tiemessen CT. Cxcr6 gene characterization in two ethnically distinct South African populations and association with viraemic disease control in hiv-1-infected black South African individuals. Clin Immunol (Orlando Fla). (2017) 180:69–79. doi: 10.1016/j.clim.2017.04.006
119. Ciccosanti F, Corazzari M, Casetti R, Amendola A, Collalto D, Refolo G, et al. High levels of trim5α Are associated with xenophagy in hiv-1-infected long-term nonprogressors. Cells. (2021) 10(5):1207. doi: 10.3390/cells10051207
120. Amanya SB, Nyiro B, Waswa F, Obura B, Nakaziba R, Nabulime E, et al. Variations in trim5α and cyclophilin a genes among hiv-1 elite controllers and non controllers in Uganda: A laboratory-based cross-sectional study. Retrovirology. (2020) 17:19. doi: 10.1186/s12977-020-00527-z
121. Jäger S, Kim DY, Hultquist JF, Shindo K, LaRue RS, Kwon E, et al. Vif hijacks cbf-β to degrade apobec3g and promote hiv-1 infection. Nature. (2011) 481:371–5. doi: 10.1038/nature10693
122. Sáez-Cirión A, Mamez AC, Avettand-Fenoel V, Nabergoj M, Passaes C, Thoueille P, et al. Sustained hiv remission after allogeneic hematopoietic stem cell transplantation with wild-type ccr5 donor cells. Nat Med. (2024) 30(12):3544–54. doi: 10.1038/s41591-024-03277-z
123. Hütter G, Nowak D, Mossner M, Ganepola S, Müssig A, Allers K, et al. Long-term control of hiv by ccr5 delta32/delta32 stem-cell transplantation. New Engl J Med. (2009) 360:692–8. doi: 10.1056/NEJMoa0802905
124. Gupta RK, Peppa D, Hill AL, Gálvez C, Salgado M, Pace M, et al. Evidence for hiv-1 cure after ccr5δ32/Δ32 allogeneic haemopoietic stem-cell transplantation 30 months post analytical treatment interruption: A case report. Lancet HIV. (2020) 7:e340–e7. doi: 10.1016/s2352-3018(20)30069-2
125. Yukl SA, Boritz E, Busch M, Bentsen C, Chun TW, Douek D, et al. Challenges in detecting hiv persistence during potentially curative interventions: A study of the berlin patient. PloS Pathog. (2013) 9:e1003347. doi: 10.1371/journal.ppat.1003347
126. Tebas P, Stein D, Tang WW, Frank I, Wang SQ, Lee G, et al. Gene editing of ccr5 in autologous cd4 T cells of persons infected with hiv. New Engl J Med. (2014) 370:901–10. doi: 10.1056/NEJMoa1300662
127. Tebas P, Jadlowsky JK, Shaw PA, Tian L, Esparza E, Brennan AL, et al. Ccr5-edited cd4+ T cells augment hiv-specific immunity to enable post-rebound control of hiv replication. J Clin Invest. (2021) 131(7):e144486. doi: 10.1172/jci144486
128. Lécuroux C, Sáez-Cirión A, Girault I, Versmisse P, Boufassa F, Avettand-Fenoël V, et al. Both hla-B*57 and plasma hiv rna levels contribute to the hiv-specific cd8+ T cell response in hiv controllers. J Virol. (2014) 88:176–87. doi: 10.1128/jvi.02098-13
129. Armani-Tourret M, Bone B, Tan TS, Sun W, Bellefroid M, Struyve T, et al. Immune targeting of hiv-1 reservoir cells: A path to elimination strategies and cure. Nat Rev Microbiol. (2024) 22:328–44. doi: 10.1038/s41579-024-01010-8
130. Li S, Moog C, Zhang T, Su B. Hiv reservoir: antiviral immune responses and immune interventions for curing hiv infection. Chin Med J. (2022) 135:2667–76. doi: 10.1097/cm9.0000000000002479
131. Li S, Wang H, Guo N, Su B, Lambotte O, Zhang T. Targeting the hiv reservoir: chimeric antigen receptor therapy for hiv cure. Chin Med J. (2023) 136:2658–67. doi: 10.1097/cm9.0000000000002904
132. Blankson JN, Bailey JR, Thayil S, Yang HC, Lassen K, Lai J, et al. Isolation and characterization of replication-competent human immunodeficiency virus type 1 from a subset of elite suppressors. J Virol. (2007) 81:2508–18. doi: 10.1128/jvi.02165-06
133. Veenhuis RT, Kwaa AK, Garliss CC, Latanich R, Salgado M, Pohlmeyer CW, et al. Long-term remission despite clonal expansion of replication-competent hiv-1 isolates. JCI Insight. (2018) 3(18):e122795. doi: 10.1172/jci.insight.122795
134. Battivelli E, Dahabieh MS, Abdel-Mohsen M, Svensson JP, Tojal Da Silva I, Cohn LB, et al. Distinct chromatin functional states correlate with hiv latency reactivation in infected primary cd4(+) T cells. eLife. (2018) 7:e34655. doi: 10.7554/eLife.34655
135. Gasca-Capote C, Lian X, Gao C, Roseto IC, Jiménez-León MR, Gladkov G, et al. The hiv-1 reservoir landscape in persistent elite controllers and transient elite controllers. J Clin Invest. (2024) 134(8):e174215. doi: 10.1172/jci174215
136. Debyser Z, Vansant G, Bruggemans A, Janssens J, Christ F. Insight in hiv integration site selection provides a block-and-lock strategy for a functional cure of hiv infection. Viruses. (2018) 11(1):12. doi: 10.3390/v11010012
137. Li JZ, Blankson JN. How elite controllers and posttreatment controllers inform our search for an hiv-1 cure. J Clin Invest. (2021) 131(11):e149414. doi: 10.1172/jci149414
138. Conway JM, Perelson AS. Post-treatment control of hiv infection. Proc Natl Acad Sci United States America. (2015) 112:5467–72. doi: 10.1073/pnas.1419162112
139. Charre C, Merad Y, Avettand-Fenoel V. Hiv-1 reservoir landscape of post-treatment control. Curr Opin HIV AIDS. (2025) 20:99–108. doi: 10.1097/coh.0000000000000891
140. Wedrychowski A, Martin HA, Li Y, Telwatte S, Kadiyala GN, Melberg M, et al. Transcriptomic signatures of human immunodeficiency virus post-treatment control. J Virol. (2023) 97:e0125422. doi: 10.1128/jvi.01254-22
141. Strongin Z, Micci L, Fromentin R, Harper J, McBrien J, Ryan E, et al. Virologic and immunologic features of simian immunodeficiency virus control post-art interruption in rhesus macaques. J Virol. (2020) 94(14):e00338-20. doi: 10.1128/jvi.00338-20
142. Toyoda M, Ogata Y, Mahiti M, Maeda Y, Kuang XT, Miura T, et al. Differential ability of primary hiv-1 nef isolates to downregulate hiv-1 entry receptors. J Virol. (2015) 89:9639–52. doi: 10.1128/jvi.01548-15
143. Richard J, Sindhu S, Pham TN, Belzile JP, Cohen EA. Hiv-1 vpr up-regulates expression of ligands for the activating nkg2d receptor and promotes nk cell-mediated killing. Blood. (2010) 115:1354–63. doi: 10.1182/blood-2009-08-237370
144. Mologni D, Citterio P, Menzaghi B, Zanone Poma B, Riva C, Broggini V, et al. Vpr and hiv-1 disease progression: R77q mutation is associated with long-term control of hiv-1 infection in different groups of patients. AIDS (London England). (2006) 20:567–74. doi: 10.1097/01.aids.0000210611.60459.0e
145. Kikuchi T, Iwabu Y, Tada T, Kawana-Tachikawa A, Koga M, Hosoya N, et al. Anti-apobec3g activity of hiv-1 vif protein is attenuated in elite controllers. J Virol. (2015) 89:4992–5001. doi: 10.1128/jvi.03464-14
146. Chen J, Tibroni N, Sauter D, Galaski J, Miura T, Alter G, et al. Modest attenuation of hiv-1 vpu alleles derived from elite controller plasma. PloS One. (2015) 10:e0120434. doi: 10.1371/journal.pone.0120434
147. Apps R, Del Prete GQ, Chatterjee P, Lara A, Brumme ZL, Brockman MA, et al. Hiv-1 vpu mediates hla-C downregulation. Cell Host Microbe. (2016) 19:686–95. doi: 10.1016/j.chom.2016.04.005
148. Barker E, Evans DT. Hla-C downmodulation by hiv-1 vpu. Cell Host Microbe. (2016) 19:570–1. doi: 10.1016/j.chom.2016.04.023
149. Churchill MJ, Chiavaroli L, Wesselingh SL, Gorry PR. Persistence of attenuated hiv-1 rev alleles in an epidemiologically linked cohort of long-term survivors infected with nef-deleted virus. Retrovirology. (2007) 4:43. doi: 10.1186/1742-4690-4-43
150. Ferrando-Martínez S, Casazza JP, Leal M, Machmach K, Muñoz-Fernández M, Viciana P, et al. Differential gag-specific polyfunctional T cell maturation patterns in hiv-1 elite controllers. J Virol. (2012) 86:3667–74. doi: 10.1128/jvi.07034-11
151. Wright JK, Naidoo VL, Brumme ZL, Prince JL, Claiborne DT, Goulder PJ, et al. Impact of hla-B*81-associated mutations in hiv-1 gag on viral replication capacity. J Virol. (2012) 86:3193–9. doi: 10.1128/jvi.06682-11
152. Ojwach DBA, MacMillan D, Reddy T, Novitsky V, Brumme ZL, Brockman MA, et al. Pol-driven replicative capacity impacts disease progression in hiv-1 subtype C infection. J Virol. (2018) 92(19):e00811-18. doi: 10.1128/jvi.00811-18
153. Silver ZA, Dickinson GM, Seaman MS, Desrosiers RC. A highly unusual V1 region of env in an elite controller of hiv infection. J Virol. (2019) 93(10):e00094-19. doi: 10.1128/jvi.00094-19
154. Casado C, Galvez C, Pernas M, Tarancon-Diez L, Rodriguez C, Sanchez-Merino V, et al. Permanent control of hiv-1 pathogenesis in exceptional elite controllers: A model of spontaneous cure. Sci Rep. (2020) 10:1902. doi: 10.1038/s41598-020-58696-y
155. Kestler HW 3rd, Ringler DJ, Mori K, Panicali DL, Sehgal PK, Daniel MD, et al. Importance of the nef gene for maintenance of high virus loads and for development of aids. Cell. (1991) 65:651–62. doi: 10.1016/0092-8674(91)90097-i
156. Kirchhoff F, Greenough TC, Brettler DB, Sullivan JL, Desrosiers RC. Brief report: absence of intact nef sequences in a long-term survivor with nonprogressive hiv-1 infection. N Engl J Med. (1995) 332:228–32. doi: 10.1056/nejm199501263320405
157. Salghetti S, Mariani R, Skowronski J. Human immunodeficiency virus type 1 nef and P56lck protein-tyrosine kinase interact with a common element in cd4 cytoplasmic tail. Proc Natl Acad Sci United States America. (1995) 92:349–53. doi: 10.1073/pnas.92.2.349
158. Peter F. Hiv nef: the mother of all evil? Immunity. (1998) 9:433–7. doi: 10.1016/s1074-7613(00)80626-3
159. Garcia JV, Miller AD. Serine phosphorylation-independent downregulation of cell-surface cd4 by nef. Nature. (1991) 350:508–11. doi: 10.1038/350508a0
160. Schwartz O, Dautry-Varsat A, Goud B, Maréchal V, Subtil A, Heard JM, et al. Human immunodeficiency virus type 1 nef induces accumulation of cd4 in early endosomes. J Virol. (1995) 69:528–33. doi: 10.1128/jvi.69.1.528-533.1995
161. Schwartz O, Maréchal V, Le Gall S, Lemonnier F, Heard JM. Endocytosis of major histocompatibility complex class I molecules is induced by the hiv-1 nef protein. Nat Med. (1996) 2:338–42. doi: 10.1038/nm0396-338
162. Keppler OT, Tibroni N, Venzke S, Rauch S, Fackler OT. Modulation of specific surface receptors and activation sensitization in primary resting cd4+ T lymphocytes by the nef protein of hiv-1. J leukocyte Biol. (2006) 79:616–27. doi: 10.1189/jlb.0805461
163. Ghiglione Y, Rodríguez AM, De Candia C, Carobene M, Benaroch P, Schindler M, et al. Hiv-mediated up-regulation of invariant chain (Cd74) correlates with generalized immune activation in hiv+ Subjects. Virus Res. (2012) 163:380–4. doi: 10.1016/j.virusres.2011.09.011
164. Schindler M, Würfl S, Benaroch P, Greenough TC, Daniels R, Easterbrook P, et al. Down-modulation of mature major histocompatibility complex class ii and up-regulation of invariant chain cell surface expression are well-conserved functions of human and simian immunodeficiency virus nef alleles. J Virol. (2003) 77:10548–56. doi: 10.1128/jvi.77.19.10548-10556.2003
165. Münch J, Rajan D, Schindler M, Specht A, Rücker E, Novembre FJ, et al. Nef-mediated enhancement of virion infectivity and stimulation of viral replication are fundamental properties of primate lentiviruses. J Virol. (2007) 81:13852–64. doi: 10.1128/jvi.00904-07
166. Miller MD, Warmerdam MT, Gaston I, Greene WC, Feinberg MB. The human immunodeficiency virus-1 nef gene product: A positive factor for viral infection and replication in primary lymphocytes and macrophages. J Exp Med. (1994) 179:101–13. doi: 10.1084/jem.179.1.101
Keywords: HIV controllers, functional cure, immune response, genetic polymorphisms, HIV reservoirs
Citation: Deng Z, Yan H, Lambotte O, Moog C and Su B (2025) HIV controllers: hope for a functional cure. Front. Immunol. 16:1540932. doi: 10.3389/fimmu.2025.1540932
Received: 06 December 2024; Accepted: 07 February 2025;
Published: 25 February 2025.
Edited by:
Qian Li, Shenzhen Third People’s Hospital, ChinaReviewed by:
Jun Wang, Jiangnan University, ChinaCopyright © 2025 Deng, Yan, Lambotte, Moog and Su. This is an open-access article distributed under the terms of the Creative Commons Attribution License (CC BY). The use, distribution or reproduction in other forums is permitted, provided the original author(s) and the copyright owner(s) are credited and that the original publication in this journal is cited, in accordance with accepted academic practice. No use, distribution or reproduction is permitted which does not comply with these terms.
*Correspondence: Bin Su, Ymluc3VAY2NtdS5lZHUuY24=
†These authors have contributed equally to this work
Disclaimer: All claims expressed in this article are solely those of the authors and do not necessarily represent those of their affiliated organizations, or those of the publisher, the editors and the reviewers. Any product that may be evaluated in this article or claim that may be made by its manufacturer is not guaranteed or endorsed by the publisher.
Research integrity at Frontiers
Learn more about the work of our research integrity team to safeguard the quality of each article we publish.