- 1Department of Radiation Oncology, The First Medical Center of Chinese PLA General Hospital, Beijing, China
- 2Department of Radiation Oncology, General Hospital of Southern Theatre Command of PLA, Guang Zhou, China
Macrophage plays an important role in homeostasis and immunity, and dysfunctional macrophage polarization is believed to be associated with the pathogenesis of tissue fibrosis and tumor progression. Colony stimulating factor-1 (CSF-1), a polypeptide chain cytokine, through its receptor (CSF-1R) regulates the differentiation of macrophages. Recently, the promising therapeutic potential of CSF-1/CSF-1R signaling pathway inhibition in cancer treatment is widely used. Furthermore, inhibition of CSF-1/CSF-1R signaling combined with radiotherapy has been extensively studied to reduce immunosuppression and promote abscopal effect. In addition, cumulative evidence demonstrated that M2 phenotype macrophage is dominant in tissue fibrosis and the inhibition of CSF-1/CSF-1R signaling pathway ameliorated pulmonary fibrosis, including radiation-induced lung fibrosis. Herein, we provide a comprehensive review of the CSF-1/CSF-1R signaling pathway in radiotherapy, with a focus on advances in macrophage-targeted strategies in the treatment of cancer and pulmonary fibrosis.
1 Introduction
Macrophages exist in all tissues of adult mammals and are considered to be components of resident tissues (1). Due to the important roles that macrophages play in both innate and adaptive immunity, they are involved in various pathological processes such as cancer and tissue repair (2, 3). Two major activated polarized states of macrophages are demonstrated in response to external environmental signals, and this polarized morphology is distinct from the irreversible tissue-specific phenotype of macrophages, which can be reversibly regulated by a variety of cytokines or transcription factors. Generally, macrophage polarization phenotypes can be classified into two types: M1 macrophages and M2 macrophages (4). M1 macrophages secret proinflammatory factors and show proinflammatory functions. M2 macrophages exhibit anti-inflammatory functions and are involved in tissue repair (5). The imbalance of inflammatory and anti-inflammatory macrophage phenotypes is critical in kinds of pathological processes, including the development of tissue fibrosis and tumor (6–8). Thus, modulation of macrophage polarization is a potential therapeutic strategy for a variety of diseases. Macrophage polarization is regulated by several factors, including CSF-1/CSF-1R signaling, which is proved to be critical for shaping the M1/M2 macrophage phenotype (9). CSF-1 promotes the differentiation of myeloid cells into macrophages through its receptor (CSF-1R), and regulates the migration, proliferation, survival and polarization of macrophages.
Radiotherapy has become one of the most important treatments for malignant tumors. According to statistics, about 60% of patients will receive radiotherapy (10). Studies have shown that radiotherapy combined with CSF-1R inhibitors exert synergistic effects through regulating macrophage polarization in the tumor microenvironment (TME), thereby improving tumor local control and enhancing anti-tumor immunity (11, 12). In addition, pulmonary fibrosis is a long-term complication of radiation-induced lung injury, in which M2-dominant phenotype is involved (13). Therefore, targeting polarization of macrophages to explore new strategies for the prevention and treatment of radiation-induced pulmonary fibrosis attract more and more attention in recent years.
Therefore, exploring the physiological and pathological roles of CSF-1/CSF-1R signal transduction in tumor treatment and radiation-induced pulmonary fibrosis is of great significance for the comprehensive treatment of malignant tumors. Here we summarize the biological functions of the CSF-1/CSF-1R signaling pathway and its role in radiotherapy.
2 CSF-1/CSF-1R signaling pathway: structure and biological function
CSF-1, also known as macrophage colony-stimulating factor (M-CSF), is a homologous dimer protein with three different forms: secreted glycoprotein, secreted glycoprotein polysaccharide, and a membrane-bound glycoprotein (14). CSF-1 is expressed in a variety of cells, such as osteoblasts and various cancer cells, which can be used as a potential tumor marker (15, 16). CSF-1R is a tyrosine kinase transmembrane receptor that is activated in an autocrine or paracrine manner. It is encoded by the oncogene c-fms, and its kinase domain contains 20 tyrosine residues, with a highly conserved structure (17). CSF-1R is highly expressed in a variety of tumor cells, including lung cancer, breast cancer, lymphoma, cervical cancer and so on (18). CSF-1 binds to its unique receptor CSF-1R in a hydrophilic manner, which triggers dimerization and phosphorylation of CSF-1R, leading to subsequent biological effects (19). Under physiological conditions, CSF-1 is capable of promoting macrophages polarized into M2 phenotype, which is involved in the latter phases for maintaining tissue repair and homeostasis (20, 21). In cancer, the chronic M2 phenotype or the tumor-associated macrophages (TAMs) inhibits tumor cell apoptosis, and induces angiogenesis under pathological conditions, thus promoting disease progression (22–25).
Accumulating evidence showed that, upregulated CSF-1 promotes the infiltration, survival, and metastasis of TAMs expressing CSF-1R in the tumor microenvironments (11, 26), and blocking the CSF-1/CSF-1R signaling pathway can reduce immunosuppressive TAMs in tumors. CSF-1/CSF-1R inhibitors have been identified as therapeutic targets for a variety of malignant tumors, such as glioma, hepatocellular carcinoma, breast cancer, lung cancer and pancreatic cancer (27), and have broad application prospects in tumor immunotherapy (Table 1).
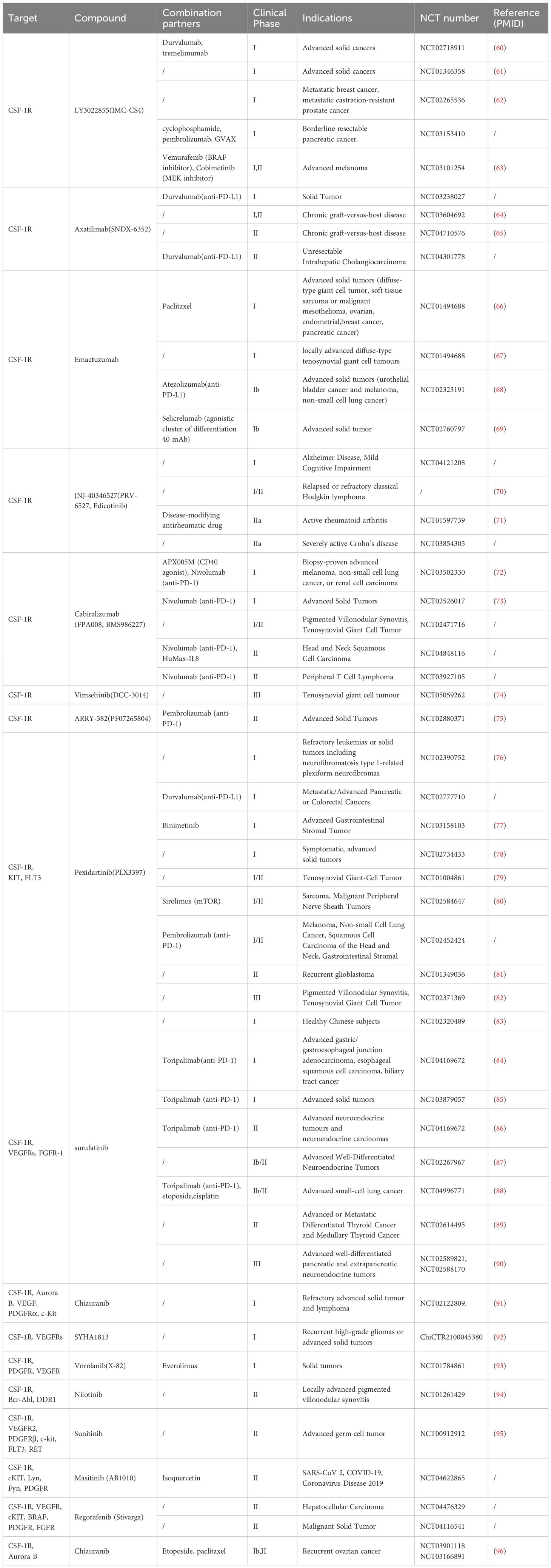
Table 1. Curtent cilincal trails with inhibitors against CSF-1R in inflammatory diseases and cancer.
In addition, aberrant expression of CSF-1/CSF-1R signaling has been reported in kinds of inflammatory diseases (12, 28–31). Related studies have shown that chronic inflammation largely leads to tissue fibrosis, including pulmonary fibrosis, in which M2 macrophages play an important role (32, 33). Previous data indicated that CSF-1 contributes to pulmonary fibrosis in mice. CSF1-/- mice demonstrated less fibrosis in response to bleomycin challenge (34). And M2 macrophages are responsible for pulmonary fibrotic disease in many fibrosis models. Although the pathogenesis of pulmonary fibrosis remains unclear, abnormalities in lung macrophages have been reported to dramatically contribute to the pathogenesis of pulmonary fibrosis. Blocking the CSF-1/CSF-1R signaling pathway may be a potential therapeutic target for pulmonary fibrosis (35, 36).
3 CSF-1/CSF-1R signaling pathway in tumor radiotherapy
TME includes cells such as macrophages, dendritic cells, T cells, endothelial cells and fibroblasts, as well as extracellular matrix (ECM) components, proteases, and cytokines, playing a critical role in tumor evolution and metastasis (37). In the tumor growth and metastasis, immune cells in the tumor microenvironment play very important roles, including T lymphocytes, B lymphocytes, TAMs, bone marrow-derived suppressive cells and other cells. TAMs are the immune cells that infiltrate the most tumor tissue. They are immune regulatory cells that differentiate from peripheral monocytes under the influence of the tumor microenvironment. TAMs are mainly M2-polarized macrophages, which play immunosuppressive roles, promoting tumor growth, metastasis, and angiogenesis (38). TAMs are closely related to CSF-1. When tumor cells proliferate uncontrollably, they secrete a large amount of CSF-1, stimulating the production of a large number of immunosuppressive M2 macrophages (39).
TAMs are associated with radiation resistance, and CSF-1/CSF-1R inhibitors can alter macrophage polarity and show radiation sensitization effects. Radiotherapy can induce adaptive immune responses in tumors, which can be achieved by increasing the expression of MHC class I proteins in tumor cells, enhancing antigen presentation, promoting the release of damaging molecular-related patterns from damaged tumor cells and enhancing the recruitment and activity of antigen-presenting cells (40), and the combination of CSF-1/CSF-1R inhibitors and radiotherapy exert a synergistic effect and enhance the anti-tumor immunity.
Jones et al. demonstrated that radiotherapy combined with macrophage depleting agents enhanced tumor killing and found that colorectal (MC38) and pancreatic (KPC) cell lines produced CSF-1 after irradiation, leading to an increase in M2 macrophages, which were immunosuppressive in tumors. The use of CSF-1 monoclonal antibodies reversed macrophage aggregation and inhibited tumor growth (41). Parsons and colleagues found that the differentiation of hematopoietic stem cells and progenitor cells into M2 macrophages within tumors promoted tumor growth after radiotherapy. Using a non-small cell lung cancer-bearing mouse model, the tumor was irradiated alone or in combination with the CSF-1R inhibitor GW2580 (selective blockade of CSF-1R self-phosphorylation and activation) for 20 Gy. They found that the CSF-1/CSF-1R signaling pathway induced hematopoietic progenitor cells and stem cells derived from bone marrow within the tumor differentiating into M2 tumor-associated macrophages, which contributed to tumor survival and regeneration after radiotherapy. The use of GW2580 improved the tumor-killing ability of radiotherapy and the survival rate of mice (42). Seifert et al. studied the inhibitory immune response of macrophages induced by radiotherapy in a mouse model of pancreatic cancer. Radiation induced an increase in the infiltration of M2 macrophages in pancreatic tumor tissue and reduced the anti-tumor effect mediated by T cells. Combination of radiotherapy with CSF-1 monoclonal antibody changed the phenotype of macrophages in pancreatic tumors, enhanced the effect of T cells, and slowed down the growth of tumor (43). Stafford et al. investigated the effect of inhibiting CSF-1R on bone marrow cell recruitment and polarization to delay the recurrence of glioblastoma after radiotherapy. They constructed a mouse model of intracranial in situ glioblastoma and administered whole brain irradiation at a dose of 12 Gy or in combination with PLX3397 (a small molecule CSF-1R tyrosinase activity inhibitor). They found that combined treatment enhanced the response of intracranial tumors to radiotherapy and blocked the differentiation of mononuclear cells recruited by radiotherapy into immunosuppressive and angiogenic TAMs, thereby delaying tumor recurrence (44). Xu et al. found that blocking CSF-1R signaling pathway inhibited tumor infiltration of bone marrow cells and improved the radiotherapy efficacy of prostate adenocarcinoma. The authors constructed a tumor-bearing animal model of prostate cancer with local irradiation of 15 Gy and found that the expression level of CSF-1 and the recruitment of tumor-infiltrating bone marrow cells were increased. Further mechanism investigation showed that radiotherapy induced the recruitment of kinase ABL1 to the nucleus by DNA damage where it bound to the CSF1 gene promoter and enhanced the transcription of the CSF1 gene. Elevated CSF-1 played a crucial role in the systemic recruitment of primary myeloid cells to the irradiated tumor. Combined with CSF1R inhibitors, it reduced the number of tumor-infiltrating bone marrow cells and inhibited tumor growth (45). In the tumor-bearing model of breast cancer, combination of CSF-1 monoclonal antibody or PLX3397 would lead to depletion of immunosuppressed macrophages, significantly delaying tumor regeneration after radiotherapy (46). In the future, CSF-1/CSF-1R pathway inhibitors combined with radiotherapy are potential targets for the treatment of tumors.
4 CSF-1/CSF-1R signaling pathway in radiation-induced pulmonary fibrosis
60-70% of cancer patients require radiotherapy during treatment, and the most common and severe side effect after thoracic radiation is radiation-induced lung injury. Up to 50% of lung cancer patients develop pneumonia in the high-dose areas of the lungs, and pulmonary fibrosis occurs in 70-80% of patients (47). Radiation-induced pulmonary fibrosis is a progressive, interstitial fibrosis pulmonary disease, which is an important pathological process in the late stage of radiotherapy. Its clinical manifestations are mainly characterized by progressive dyspnea and decreased lung function (48). There are no effective medications for the treatment of radiation-induced pulmonary fibrosis by now (49).
Although the pathogenesis of pulmonary fibrosis is still unclear, recent studies have reported the important role of macrophages as key regulatory factors for fibrosis (50), with M2 polarized macrophages playing an important role in various fibrosis models (51–53). Depletion of macrophages, especially M2-type macrophages, attenuated pulmonary fibrosis (54, 55). In addition, studies showed that CSF-1 was elevated in alveolar lavage in patients with pulmonary fibrosis and stimulated macrophages and fibroblasts to participate in fibrosis formation (56). As an essential factor for macrophage differentiation and proliferation, the inhibition of CSF-1/CSF-1R pathway affects macrophage production and thus attenuates lung fibrosis.
Baran et al. investigated the role of CSF-1 in the pathogenesis of pulmonary fibrosis and found that its expression was elevated in patients with pulmonary fibrosis. In mouse model of bleomycin-induced pulmonary fibrosis, knocking out CSF-1 or usage of CSF-1R inhibitor showed protective effect (56). Zhou et al. investigated the role of IL-34 (a ligand of CSF-1R) and found that IL-34 upregulated IL-6 and IL-8 expression in human lung fibroblasts and these effects were reversed when treated cells with anti-CSF-1R antibody. These data confirmed the inflammatory effect of IL-34 on human lung fibroblasts and suggested that the IL-34/CSF-1R axis may be a novel therapeutic target in pulmonary disease (57). Joshi et al. investigated that monocyte-derived alveolar macrophages in the pulmonary fibrosis microenvironment were regulated by the CSF-1/CSF-1R signaling pathway. In a mouse model of asbestos-induced pulmonary fibrosis, CSF-1 monoclonal antibodies or inhibitor PLX3397 were used to block the CSF-1/CSF-1R signaling pathway to reduce monocyte differentiated alveolar macrophages and the alleviated pulmonary fibrosis was observed (58).
The evidence of CSF-1/CSF-1R signaling pathway in radiation-induced pulmonary fibrosis is limited. Zhang et al. showed that in the middle and late stages of radiation-induced lung injury (RILI), various pro-fibrotic cytokines such as IL-4 and IL-13 promoted macrophage polarization into M2 macrophages, leading to excessive secretion and deposition of extracellular matrix, ultimately resulting in fibrosis and structural changes (52). M2 macrophages can regulate myofibroblast activity in the middle and late stages of RILI through TGF-β/Smad pathway, promoting the progression of radiation-induced pulmonary fibrosis (59). Meziani et al. studied the use of CSF-1R inhibitors to prevent radiation-induced pulmonary fibrosis by depleting pulmonary interstitial macrophages and constructed a 16Gy mouse chest irradiation model. They found that using clodrosomes to deplete alveolar macrophages did not improve pulmonary fibrosis while using CSF-1R monoclonal antibodies to deplete pulmonary interstitial macrophages alleviated radiation-induced pulmonary fibrosis (59). Despite the rapid advances in radiation oncology in plan design and image-guided radiotherapy, normal tissue toxicity remains a dose-limiting factor for optimal local tumor control. The inhibition of the CSF-1/CSF-1R signaling pathway offers a novel therapeutic modality for mitigating radiation-induced pulmonary fibrosis.
5 Summary
In summary, due to the crucial regulatory role of the CSF-1/CSF-1R signaling pathway in tumor development and fibrotic processes, inhibiting the CSF-1/CSF-1R signaling pathway seems to be a promising strategy for cancer treatment and fibrosis (Figure 1). Although different experimental models may yield some controversial results, the accumulation of preclinical evidence has paved the way for the clinical application of CSF-1/CSF-1R inhibitors in tumor radiotherapy and fibrotic diseases. Further researches are needed to achieve further understanding of the interaction between CSF-1/CSF-1R signaling pathway inhibitors and tumor radiotherapy and fibrosis. This will help provide new treatment strategies for precise radiotherapy of tumors and reduce radiotherapy side effects.
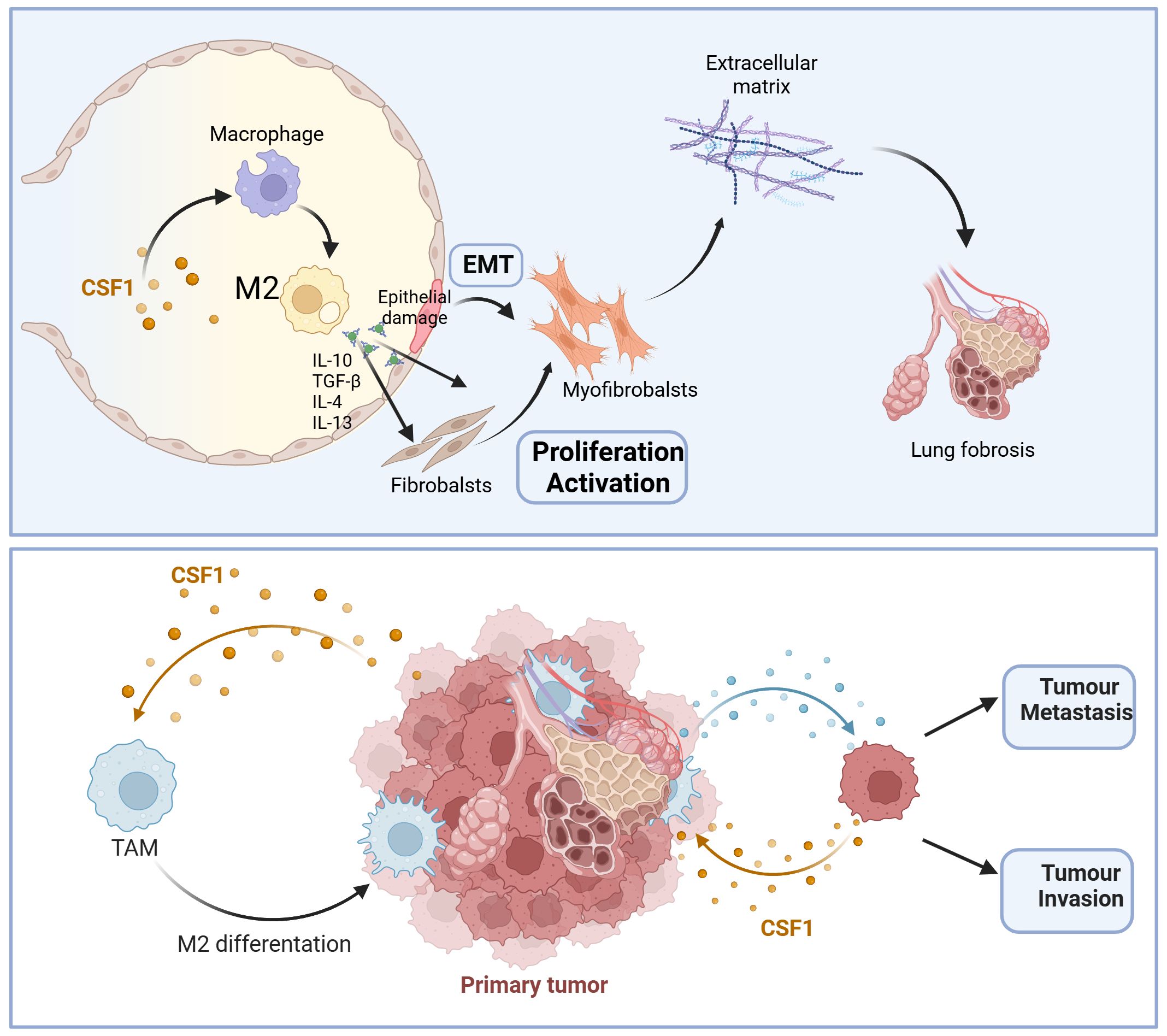
Figure 1. Schematic overview of critical role of CSF-1/CSF-1R signaling pathway in pulmonary fibrosis and tumor progression. IL-10, Interleukin-10; IL-4, Interleukin-4; TGF-β, Transforming growth factor-β; IL-13, Interleukin-13; EMT, Epithelial-mesenchymal transition; M2, alternatively activated macrophages.
Author contributions
QS: Investigation, Writing – original draft. PZ: Conceptualization, Writing – review & editing. XL: Validation, Writing – review & editing. LD: Supervision, Writing – review & editing. BQ: Conceptualization, Supervision, Validation, Writing – review & editing.
Funding
The author(s) declare that no financial support was received for the research, authorship, and/or publication of this article.
Conflict of interest
The authors declare that the research was conducted in the absence of any commercial or financial relationships that could be construed as a potential conflict of interest.
Generative AI statement
The author(s) declare that no Generative AI was used in the creation of this manuscript.
Publisher’s note
All claims expressed in this article are solely those of the authors and do not necessarily represent those of their affiliated organizations, or those of the publisher, the editors and the reviewers. Any product that may be evaluated in this article, or claim that may be made by its manufacturer, is not guaranteed or endorsed by the publisher.
References
1. Yan L, Wang J, Cai X, Liou YC, Shen HM, Hao J, et al. Macrophage plasticity: signaling pathways, tissue repair, and regeneration. MedComm (2020). (2024) 5:e658. doi: 10.1002/mco2.658
2. Xiang C, Li H, Tang W. Targeting CSF-1R represents an effective strategy in modulating inflammatory diseases. Pharmacol Res. (2023) 187:106566. doi: 10.1016/j.phrs.2022.106566
3. Bied M, Ho WW, Ginhoux F, Blériot C. Roles of macrophages in tumor development: a spatiotemporal perspective. Cell Mol Immunol. (2023) 20:983–92. doi: 10.1038/s41423-023-01061-6
4. Li M, Wang M, Wen Y, Zhang H, Zhao GN, Gao Q. Signaling pathways in macrophages: molecular mechanisms and therapeutic targets. MedComm (2020). (2023) 4:e349. doi: 10.1002/mco2.v4.5
5. Wynn TA, Vannella KM. Macrophages in Tissue Repair, Regeneration, and Fibrosis. Immunity. (2016) 44:450–62. doi: 10.1016/j.immuni.2016.02.015
6. Wang N, Liang H, Zen K. Molecular mechanisms that influence the macrophage m1-m2 polarization balance. Front Immunol. (2014) 5:614. doi: 10.3389/fimmu.2014.00614
7. Chen Y, Song Y, Du W, Gong L, Chang H, Zou Z. Tumor-associated macrophages: an accomplice in solid tumor progression. J BioMed Sci. (2019) 26:78. doi: 10.1186/s12929-019-0568-z
8. Vitale I, Manic G, Coussens LM, Kroemer G, Galluzzi L. Macrophages and metabolism in the tumor microenvironment. Cell Metab. (2019) 30:36–50. doi: 10.1016/j.cmet.2019.06.001
9. Pyonteck SM, Akkari L, Schuhmacher AJ, Bowman RL, Sevenich L, Quail DF, et al. CSF-1R inhibition alters macrophage polarization and blocks glioma progression. Nat Med. (2013) 19:1264–72. doi: 10.1038/nm.3337
10. Schaue D, McBride WH. Opportunities and challenges of radiotherapy for treating cancer. Nat Rev Clin Oncol. (2015) 12:527–40. doi: 10.1038/nrclinonc.2015.120
11. Ries CH, Cannarile MA, Hoves S, Benz J, Wartha K, Runza V, et al. Targeting tumor-associated macrophages with anti-CSF-1R antibody reveals a strategy for cancer therapy. Cancer Cell. (2014) 25:846–59. doi: 10.1016/j.ccr.2014.05.016
12. Han J, Chitu V, Stanley ER, Wszolek ZK, Karrenbauer VD, Harris RA. Inhibition of colony stimulating factor-1 receptor (CSF-1R) as a potential therapeutic strategy for neurodegenerative diseases: opportunities and challenges. Cell Mol Life Sci. (2022) 79:219. doi: 10.1007/s00018-022-04225-1
13. Cytlak UM, Dyer DP, Honeychurch J, Williams KJ, Travis MA, Illidge TM. Immunomodulation by radiotherapy in tumour control and normal tissue toxicity. Nat Rev Immunol. (2022) 22:124–38. doi: 10.1038/s41577-021-00568-1
14. Chockalingam S, Ghosh SS. Macrophage colony-stimulating factor and cancer: a review. Tumour Biol. (2014) 35:10635–44. doi: 10.1007/s13277-014-2627-0
15. Chitu V, Stanley ER. Colony-stimulating factor-1 in immunity and inflammation. Curr Opin Immunol. (2006) 18:39–48. doi: 10.1016/j.coi.2005.11.006
16. Hamilton JA. Colony-stimulating factors in inflammation and autoimmunity. Nat Rev Immunol. (2008) 8:533–44. doi: 10.1038/nri2356
17. Sherr CJ, Rettenmier CW, Sacca R, Roussel MF, Look AT, Stanley ER. The c-fms proto-oncogene product is related to the receptor for the mononuclear phagocyte growth factor, CSF-1. Cell. (1985) 41:665–76. doi: 10.1016/S0092-8674(85)80047-7
18. Cersosimo F, Lonardi S, Ulivieri C, Martini P, Morrione A, Vermi W, et al. CSF-1R in Cancer: More than a Myeloid Cell Receptor. Cancers (Basel). (2024) 16(2). doi: 10.3390/cancers16020282
19. Kumari A, Silakari O, Singh RK. Recent advances in colony stimulating factor-1 receptor/c-FMS as an emerging target for various therapeutic implications. BioMed Pharmacother. (2018) 103:662–79. doi: 10.1016/j.biopha.2018.04.046
20. Chen YC, Lai YS, Hsuuw YD, Chang KT. Withholding of M-CSF Supplement Reprograms Macrophages to M2-Like via Endogenous CSF-1 Activation. Int J Mol Sci. (2021) 22(7). doi: 10.3390/ijms22073532
21. Mosser DM, Hamidzadeh K, Goncalves R. Macrophages and the maintenance of homeostasis. Cell Mol Immunol. (2021) 18:579–87. doi: 10.1038/s41423-020-00541-3
22. Kirma N, Hammes LS, Liu YG, Nair HB, Valente PT, Kumar S, et al. Elevated expression of the oncogene c-fms and its ligand, the macrophage colony-stimulating factor-1, in cervical cancer and the role of transforming growth factor-beta1 in inducing c-fms expression. Cancer Res. (2007) 67:1918–26. doi: 10.1158/0008-5472.CAN-06-1991
23. Kacinski BM. CSF-1 and its receptor in breast carcinomas and neoplasms of the female reproductive tract. Mol Reprod Dev. (1997) 46:71–4. doi: 10.1002/(SICI)1098-2795(199701)46:1<71::AID-MRD11>3.0.CO;2-6
24. Skrzypski M, Dziadziuszko R, Jassem E, Szymanowska-Narloch A, Gulida G, Rzepko R, et al. Main histologic types of non-small-cell lung cancer differ in expression of prognosis-related genes. Clin Lung Cancer. (2013) 14:666–73.e2. doi: 10.1016/j.cllc.2013.04.010
25. Achkova D, Maher J. Role of the colony-stimulating factor (CSF)/CSF-1 receptor axis in cancer. Biochem Soc Trans. (2016) 44:333–41. doi: 10.1042/BST20150245
26. Cassetta L, Pollard JW. Tumor-associated macrophages. Curr Biol. (2020) 30:R246–R8. doi: 10.1016/j.cub.2020.01.031
27. Wen J, Wang S, Guo R, Liu D. CSF1R inhibitors are emerging immunotherapeutic drugs for cancer treatment. Eur J Med Chem. (2023) 245:114884. doi: 10.1016/j.ejmech.2022.114884
28. Menke J, Rabacal WA, Byrne KT, Iwata Y, Schwartz MM, Stanley ER, et al. Circulating CSF-1 promotes monocyte and macrophage phenotypes that enhance lupus nephritis. J Am Soc Nephrol. (2009) 20:2581–92. doi: 10.1681/ASN.2009050499
29. Zhou RP, Wu XS, Xie YY, Dai BB, Hu W, Ge JF, et al. Functions of interleukin-34 and its emerging association with rheumatoid arthritis. Immunology. (2016) 149:362–73. doi: 10.1111/imm.2016.149.issue-4
30. Preisser L, Miot C, Le Guillou-Guillemette H, Beaumont E, Foucher ED, Garo E, et al. IL-34 and macrophage colony-stimulating factor are overexpressed in hepatitis C virus fibrosis and induce profibrotic macrophages that promote collagen synthesis by hepatic stellate cells. Hepatology. (2014) 60:1879–90. doi: 10.1002/hep.27328
31. Moon HG, Kim SJ, Lee MK, Kang H, Choi HS, Harijith A, et al. Colony-stimulating factor 1 and its receptor are new potential therapeutic targets for allergic asthma. Allergy. (2020) 75:357–69. doi: 10.1111/all.14011
32. Heukels P, Moor CC, von der Thusen JH, Wijsenbeek MS, Kool M. Inflammation and immunity in IPF pathogenesis and treatment. Respir Med. (2019) 147:79–91. doi: 10.1016/j.rmed.2018.12.015
33. Hou J, Shi J, Chen L, Lv Z, Chen X, Cao H, et al. M2 macrophages promote myofibroblast differentiation of LR-MSCs and are associated with pulmonary fibrogenesis. Cell Commun Signal. (2018) 16:89. doi: 10.1186/s12964-018-0300-8
34. Dakhlallah D, Wang Y, Bobo TA, Ellis E, Mo X, Piper MG, et al. Constitutive AKT Activity Predisposes Lung Fibrosis by Regulating Macrophage, Myofibroblast and Fibrocyte Recruitment and Changes in Autophagy. Adv Biosci Biotechnol. (2019) 10:346–73. doi: 10.4236/abb.2019.1010027
35. Wang Y, Zhang L, Wu GR, Zhou Q, Yue H, Rao LZ, et al. MBD2 serves as a viable target against pulmonary fibrosis by inhibiting macrophage M2 program. Sci Adv. (2021) 7(1). doi: 10.1126/sciadv.abb6075
36. Gibbons MA, MacKinnon AC, Ramachandran P, Dhaliwal K, Duffin R, Phythian-Adams AT, et al. Ly6Chi monocytes direct alternatively activated profibrotic macrophage regulation of lung fibrosis. Am J Respir Crit Care Med. (2011) 184:569–81. doi: 10.1164/rccm.201010-1719OC
37. Fang J, Lu Y, Zheng J, Jiang X, Shen H, Shang X, et al. Exploring the crosstalk between endothelial cells, immune cells, and immune checkpoints in the tumor microenvironment: new insights and therapeutic implications. Cell Death Dis. (2023) 14:586. doi: 10.1038/s41419-023-06119-x
38. Cannarile MA, Weisser M, Jacob W, Jegg AM, Ries CH, Ruttinger D. Colony-stimulating factor 1 receptor (CSF1R) inhibitors in cancer therapy. J Immunother Cancer. (2017) 5:53. doi: 10.1186/s40425-017-0257-y
39. Laoui D, van Overmeire E, de Baetselier P, Van Ginderachter JA, Raes G. Functional Relationship between Tumor-Associated Macrophages and Macrophage Colony-Stimulating Factor as Contributors to Cancer Progression. Front Immunol. (2014) 5:489. doi: 10.3389/fimmu.2014.00489
40. Reits EA, Hodge JW, Herberts CA, Groothuis TA, Chakraborty M, Wansley EK, et al. Radiation modulates the peptide repertoire, enhances MHC class I expression, and induces successful antitumor immunotherapy. J Exp Med. (2006) 203:1259–71. doi: 10.1084/jem.20052494
41. Jones KI, Tiersma J, Yuzhalin AE, Gordon-Weeks AN, Buzzelli J, Im JH, et al. Radiation combined with macrophage depletion promotes adaptive immunity and potentiates checkpoint blockade. EMBO Mol Med. (2018) 10(12). doi: 10.15252/emmm.201809342
42. Parsons TM, Buelow KL, Hanna A, Brake MA, Poma C, Hosch SE, et al. Intratumoural haematopoietic stem and progenitor cell differentiation into M2 macrophages facilitates the regrowth of solid tumours after radiation therapy. Br J Cancer. (2022) 126:927–36. doi: 10.1038/s41416-021-01652-y
43. Seifert L, Werba G, Tiwari S, Giao Ly NN, Nguy S, Alothman S, et al. Radiation Therapy Induces Macrophages to Suppress T-Cell Responses Against Pancreatic Tumors in Mice. Gastroenterology. (2016) 150:1659–72.e5. doi: 10.1053/j.gastro.2016.02.070
44. Stafford JH, Hirai T, Deng L, Chernikova SB, Urata K, West BL, et al. Colony stimulating factor 1 receptor inhibition delays recurrence of glioblastoma after radiation by altering myeloid cell recruitment and polarization. Neuro Oncol. (2016) 18:797–806. doi: 10.1093/neuonc/nov272
45. Xu J, Escamilla J, Mok S, David J, Priceman S, West B, et al. CSF1R signaling blockade stanches tumor-infiltrating myeloid cells and improves the efficacy of radiotherapy in prostate cancer. Cancer Res. (2013) 73:2782–94. doi: 10.1158/0008-5472.CAN-12-3981
46. Shiao SL, Ruffell B, Denardo DG, Faddegon BA, Park CC, Coussens LM. TH2-Polarized CD4(+) T Cells and Macrophages Limit Efficacy of Radiotherapy. Cancer Immunol Res. (2015) 3:518–25. doi: 10.1158/2326-6066.CIR-14-0232
47. Ueki N, Matsuo Y, Togashi Y, Kubo T, Shibuya K, Iizuka Y, et al. Impact of pretreatment interstitial lung disease on radiation pneumonitis and survival after stereotactic body radiation therapy for lung cancer. J Thorac Oncol. (2015) 10:116–25. doi: 10.1097/JTO.0000000000000359
48. Jin H, Yoo Y, Kim Y, Kim Y, Cho J, Lee YS. Radiation-Induced Lung Fibrosis: Preclinical Animal Models and Therapeutic Strategies. Cancers (Basel). (2020) 12(6). doi: 10.3390/cancers12061561
49. Lu L, Sun C, Su Q, Wang Y, Li J, Guo Z, et al. Radiation-induced lung injury: latest molecular developments, therapeutic approaches, and clinical guidance. Clin Exp Med. (2019) 19:417–26. doi: 10.1007/s10238-019-00571-w
50. Zhang L, Wang Y, Wu G, Xiong W, Gu W, Wang CY. Macrophages: friend or foe in idiopathic pulmonary fibrosis? Respir Res. (2018) 19:170. doi: 10.1186/s12931-018-0864-2
51. Wynn TA, Barron L. Macrophages: master regulators of inflammation and fibrosis. Semin Liver Dis. (2010) 30:245–57. doi: 10.1055/s-0030-1255354
52. Zhang H, Han G, Liu H, Chen J, Ji X, Zhou F, et al. The development of classically and alternatively activated macrophages has different effects on the varied stages of radiation-induced pulmonary injury in mice. J Radiat Res. (2011) 52:717–26. doi: 10.1269/jrr.11054
53. Deng X, Yang Q, Wang Y, Zhou C, Guo Y, Hu Z, et al. CSF-1R inhibition attenuates ischemia-induced renal injury and fibrosis by reducing Ly6C(+) M2-like macrophage infiltration. Int Immunopharmacol. (2020) 88:106854. doi: 10.1016/j.intimp.2020.106854
54. Song G, Cai F, Liu L, Xu Z, Peng Y, Yang Z, et al. Liposomal sodium clodronate mitigates radiation-induced lung injury through macrophage depletion. Transl Oncol. (2024) 47:102029. doi: 10.1016/j.tranon.2024.102029
55. Liu B, Jiang Q, Chen R, Gao S, Xia Q, Zhu J, et al. Tacrolimus ameliorates bleomycin-induced pulmonary fibrosis by inhibiting M2 macrophage polarization via JAK2/STAT3 signaling. Int Immunopharmacol. (2022) 113:109424. doi: 10.1016/j.intimp.2022.109424
56. Baran CP, Opalek JM, McMaken S, Newland CA, O'brien JM Jr, Hunter MG, et al. Important roles for macrophage colony-stimulating factor, CC chemokine ligand 2, and mononuclear phagocytes in the pathogenesis of pulmonary fibrosis. Am J Respir Crit Care Med. (2007) 176:78–89. doi: 10.1164/rccm.200609-1279OC
57. Zhou J, Sun X, Zhang J, Yang Y, Chen D, Cao J. IL-34 regulates IL-6 and IL-8 production in human lung fibroblasts via MAPK, PI3K-Akt, JAK and NF-kappaB signaling pathways. Int Immunopharmacol. (2018) 61:119–25. doi: 10.1016/j.intimp.2018.05.023
58. Joshi N, Watanabe S, Verma R, Jablonski RP, Chen CI, Cheresh P, et al. A spatially restricted fibrotic niche in pulmonary fibrosis is sustained by M-CSF/M-CSFR signalling in monocyte-derived alveolar macrophages. Eur Respir J. (2020) 55(1). doi: 10.1183/13993003.00646-2019
59. Meziani L, Mondini M, Petit B, Boissonnas A, Thomas De Montpreville V, Mercier O, et al. CSF1R inhibition prevents radiation pulmonary fibrosis by depletion of interstitial macrophages. Eur Respir J. (2018) 51(3). doi: 10.1183/13993003.02120-2017
60. Falchook GS, Peeters M, Rottey S, Dirix LY, Obermannova R, Cohen JE, et al. A phase 1a/1b trial of CSF-1R inhibitor LY3022855 in combination with durvalumab or tremelimumab in patients with advanced solid tumors. Invest New Drugs. (2021) 39:1284–97. doi: 10.1007/s10637-021-01088-4
61. Dowlati A, Harvey RD, Carvajal RD, Hamid O, Klempner SJ, Kauh JSW, et al. LY3022855, an anti-colony stimulating factor-1 receptor (CSF-1R) monoclonal antibody, in patients with advanced solid tumors refractory to standard therapy: phase 1 dose-escalation trial. Invest New Drugs. (2021) 39:1057–71. doi: 10.1007/s10637-021-01084-8
62. Autio KA, Klebanoff CA, Schaer D, Kauh JSW, Slovin SF, Adamow M, et al. Immunomodulatory Activity of a Colony-stimulating Factor-1 Receptor Inhibitor in Patients with Advanced Refractory Breast or Prostate Cancer: A Phase I Study. Clin Cancer Res. (2020) 26:5609–20. doi: 10.1158/1078-0432.CCR-20-0855
63. Buchbinder EI, Giobbie-Hurder A, Haq R, Ott PA. A phase I/II study of LY3022855 with BRAF/MEK inhibition in patients with Melanoma. Invest New Drugs. (2023) 41:551–5. doi: 10.1007/s10637-023-01374-3
64. Kitko CL, Arora M, Defilipp Z, Zaid MA, Di Stasi A, Radojcic V, et al. Axatilimab for Chronic Graft-Versus-Host Disease After Failure of at Least Two Prior Systemic Therapies: Results of a Phase I/II Study. J Clin Oncol. (2023) 41:1864–75. doi: 10.1200/JCO.22.00958
65. Wolff D, Cutler C, Lee SJ, Pusic I, Bittencourt H, White J, et al. Axatilimab in Recurrent or Refractory Chronic Graft-versus-Host Disease. N Engl J Med. (2024) 391:1002–14. doi: 10.1056/NEJMoa2401537
66. Gomez-Roca CA, Italiano A, Le Tourneau C, Cassier PA, Toulmonde M, D'angelo SP, et al. Phase I study of emactuzumab single agent or in combination with paclitaxel in patients with advanced/metastatic solid tumors reveals depletion of immunosuppressive M2-like macrophages. Ann Oncol. (2019) 30:1381–92. doi: 10.1093/annonc/mdz163
67. Cassier PA, Italiano A, Gomez-Roca CA, Le Tourneau C, Toulmonde M, Cannarile MA, et al. CSF1R inhibition with emactuzumab in locally advanced diffuse-type tenosynovial giant cell tumours of the soft tissue: a dose-escalation and dose-expansion phase 1 study. Lancet Oncol. (2015) 16:949–56. doi: 10.1016/S1470-2045(15)00132-1
68. Gomez-Roca C, Cassier P, Zamarin D, Machiels JP, Perez Gracia JL, Stephen Hodi F, et al. Anti-CSF-1R emactuzumab in combination with anti-PD-L1 atezolizumab in advanced solid tumor patients naive or experienced for immune checkpoint blockade. J Immunother Cancer. (2022) 10.(5) doi: 10.1136/jitc-2021-004076
69. Machiels JP, Gomez-Roca C, Michot JM, Zamarin D, Mitchell T, Catala G, et al. Phase Ib study of anti-CSF-1R antibody emactuzumab in combination with CD40 agonist selicrelumab in advanced solid tumor patients. J Immunother Cancer. (2020) 8(2). doi: 10.1136/jitc-2020-001153
70. von Tresckow B, Morschhauser F, Ribrag V, Topp MS, Chien C, Seetharam S, et al. An Open-Label, Multicenter, Phase I/II Study of JNJ-40346527, a CSF-1R Inhibitor, in Patients with Relapsed or Refractory Hodgkin Lymphoma. Clin Cancer Res. (2015) 21:1843–50. doi: 10.1158/1078-0432.CCR-14-1845
71. Genovese MC, Hsia E, Belkowski SM, Chien C, Masterson T, Thurmond RL, et al. Results from a Phase IIA Parallel Group Study of JNJ-40346527, an Oral CSF-1R Inhibitor, in Patients with Active Rheumatoid Arthritis despite Disease-modifying Antirheumatic Drug Therapy. J Rheumatol. (2015) 42:1752–60. doi: 10.3899/jrheum.141580
72. Weiss SA, Djureinovic D, Jessel S, Krykbaeva I, Zhang L, Jilaveanu L, et al. A Phase I Study of APX005M and Cabiralizumab with or without Nivolumab in Patients with Melanoma, Kidney Cancer, or Non-Small Cell Lung Cancer Resistant to Anti-PD-1/PD-L1. Clin Cancer Res. (2021) 27:4757–67. doi: 10.1158/1078-0432.CCR-21-0903
73. Foster CC, Fleming GF, Karrison TG, Liao CY, Desai A V, Moroney JW, et al. Phase I Study of Stereotactic Body Radiotherapy plus Nivolumab and Urelumab or Cabiralizumab in Advanced Solid Tumors. Clin Cancer Res. (2021) 27:5510–8. doi: 10.1158/1078-0432.CCR-21-0810
74. Gelderblom H, Bhadri V, Stacchiotti S, Bauer S, Wagner AJ, Van De Sande M, et al. Vimseltinib versus placebo for tenosynovial giant cell tumour (MOTION): a multicentre, randomised, double-blind, placebo-controlled, phase 3 trial. Lancet. (2024) 403:2709–19. doi: 10.1016/S0140-6736(24)00885-7
75. Johnson M, Dudek AZ, Sukari A, Call J, Kunk PR, Lewis K, et al. ARRY-382 in Combination with Pembrolizumab in Patients with Advanced Solid Tumors: Results from a Phase 1b/2 Study. Clin Cancer Res. (2022) 28:2517–26. doi: 10.1158/1078-0432.CCR-21-3009
76. Boal LH, Glod J, Spencer M, Kasai M, Derdak J, Dombi E, et al. Pediatric PK/PD Phase I Trial of Pexidartinib in Relapsed and Refractory Leukemias and Solid Tumors Including Neurofibromatosis Type I-Related Plexiform Neurofibromas. Clin Cancer Res. (2020) 26:6112–21. doi: 10.1158/1078-0432.CCR-20-1696
77. Rosenbaum E, Kelly C, D’Angelo SP, Dickson MA, Gounder M, Keohan ML, et al. A Phase I Study of Binimetinib (MEK162) Combined with Pexidartinib (PLX3397) in Patients with Advanced Gastrointestinal Stromal Tumor. Oncologist. (2019) 24:1309–e983. doi: 10.1634/theoncologist.2019-0418
78. Lee JH, Chen TW, Hsu CH, Yen YH, Yang JC, Cheng AL, et al. A phase I study of pexidartinib, a colony-stimulating factor 1 receptor inhibitor, in Asian patients with advanced solid tumors. Invest New Drugs. (2020) 38:99–110. doi: 10.1007/s10637-019-00745-z
79. Tap WD, Wainberg ZA, Anthony SP, Ibrahim PN, Zhang C, Healey JH, et al. Structure-Guided Blockade of CSF1R Kinase in Tenosynovial Giant-Cell Tumor. N Engl J Med. (2015) 373:428–37. doi: 10.1056/NEJMoa1411366
80. Manji GA, van Tine BA, Lee SM, Raufi AG, Pellicciotta I, Hirbe AC, et al. A Phase I Study of the Combination of Pexidartinib and Sirolimus to Target Tumor-Associated Macrophages in Unresectable Sarcoma and Malignant Peripheral Nerve Sheath Tumors. Clin Cancer Res. (2021) 27:5519–27. doi: 10.1158/1078-0432.CCR-21-1779
81. Butowski N, Colman H, de Groot JF, Omuro AM, Nayak L, Wen PY, et al. Orally administered colony stimulating factor 1 receptor inhibitor PLX3397 in recurrent glioblastoma: an Ivy Foundation Early Phase Clinical Trials Consortium phase II study. Neuro Oncol. (2016) 18:557–64. doi: 10.1093/neuonc/nov245
82. Healey JH, Tap WD, Gelhorn HL, Ye X, Speck RM, Palmerini E, et al. Pexidartinib Provides Modest Pain Relief in Patients With Tenosynovial Giant Cell Tumor: Results From ENLIVEN. Clin Orthop Relat Res. (2023) 481:107–16. doi: 10.1097/CORR.0000000000002335
83. Qian H, Wu X, Chen Q, Li T, Wang W, Jia J, et al. Effects of Food on the Pharmacokinetic Properties of Surufatinib: A Phase I, Single-dose, Randomized, Open-label Crossover Study in Healthy Subjects. Clin Ther. (2020) 42:1778–86. doi: 10.1016/j.clinthera.2020.07.010
84. Zhang P, Chen Z, Shi S, Li Z, Ye F, Song L, et al. Efficacy and safety of surufatinib plus toripalimab, a chemotherapy-free regimen, in patients with advanced gastric/gastroesophageal junction adenocarcinoma, esophageal squamous cell carcinoma, or biliary tract cancer. Cancer Immunol Immunother. (2024) 73:119. doi: 10.1007/s00262-024-03677-7
85. Cao Y, Lu M, Sun Y, Gong J, Li J, Lu Z, et al. Surufatinib plus toripalimab in patients with advanced solid tumors: a single-arm, open-label, phase 1 trial. J Cancer Res Clin Oncol. (2023) 149:779–89. doi: 10.1007/s00432-021-03898-8
86. Zhang P, Shi S, Xu J, Chen Z, Song L, Zhang X, et al. Surufatinib plus toripalimab in patients with advanced neuroendocrine tumours and neuroendocrine carcinomas: An open-label, single-arm, multi-cohort phase II trial. Eur J Cancer. (2024) 199:113539. doi: 10.1016/j.ejca.2024.113539
87. Xu J, Li J, Bai C, Xu N, Zhou Z, Li Z, et al. Surufatinib in Advanced Well-Differentiated Neuroendocrine Tumors: A Multicenter, Single-Arm, Open-Label, Phase Ib/II Trial. Clin Cancer Res. (2019) 25:3486–94. doi: 10.1158/1078-0432.CCR-18-2994
88. Zhang Y, Huang Y, Yang Y, Zhao Y, Zhou T, Chen G, et al. Surufatinib plus toripalimab combined with etoposide and cisplatin as first-line treatment in advanced small-cell lung cancer patients: a phase Ib/II trial. Signal Transduct Target Ther. (2024) 9:255. doi: 10.1038/s41392-024-01974-2
89. Chen J, Ji Q, Bai C, Zheng X, Zhang Y, Shi F, et al. Surufatinib in Chinese Patients with Locally Advanced or Metastatic Differentiated Thyroid Cancer and Medullary Thyroid Cancer: A Multicenter, Open-Label, Phase II Trial. Thyroid. (2020) 30:1245–53. doi: 10.1089/thy.2019.0453
90. Li J, Cheng Y, Bai C, Xu J, Shen L, Li J, et al. Health-related quality of life in patients with advanced well-differentiated pancreatic and extrapancreatic neuroendocrine tumors treated with surufatinib versus placebo: Results from two randomized, double-blind, phase III trials (SANET-p and SANET-ep). Eur J Cancer. (2022) 169:1–9. doi: 10.1016/j.ejca.2022.03.027
91. Sun Y, Yang L, Hao X, Liu Y, Zhang J, Ning Z, et al. Phase I dose-escalation study of chiauranib, a novel angiogenic, mitotic, and chronic inflammation inhibitor, in patients with advanced solid tumors. J Hematol Oncol. (2019) 12:9. doi: 10.1186/s13045-018-0695-0
92. Kang Z, Li S, Lin Y, Li Y, Mao Y, Zhang J, et al. A phase I dose-escalation study of SYHA1813, a VEGFR and CSF1R inhibitor, in patients with recurrent High-Grade Gliomas or Advanced Solid Tumors. Invest New Drugs. (2023) 41:296–305. doi: 10.1007/s10637-022-01325-4
93. Pedersen KS, Grierson PM, Picus J, Lockhart AC, Roth B J, Liu J, et al. Vorolanib (X-82), an oral anti-VEGFR/PDGFR/CSF1R tyrosine kinase inhibitor, with everolimus in solid tumors: results of a phase I study. Invest New Drugs. (2021) 39:1298–305. doi: 10.1007/s10637-021-01093-7
94. Gelderblom H, Cropet C, Chevreau C, Boyle R, Tattersall M, Stacchiotti S, et al. Nilotinib in locally advanced pigmented villonodular synovitis: a multicentre, open-label, single-arm, phase 2 trial. Lancet Oncol. (2018) 19:639–48. doi: 10.1016/S1470-2045(18)30143-8
95. Subbiah V, Meric-Bernstam F, Mills GB, Shaw KR, Bailey AM, Rao P, et al. Next generation sequencing analysis of platinum refractory advanced germ cell tumor sensitive to Sunitinib (Sutent(R)) a VEGFR2/PDGFRbeta/c-kit/FLT3/RET/CSF1R inhibitor in a phase II trial. J Hematol Oncol. (2014) 7:52. doi: 10.1186/s13045-014-0052-x
Keywords: CSF-1, macrophage, radiotherapy, radiation-induced pulmonary fibrosis (RIPF), tumor associate macrophages (TAM)
Citation: Shang Q, Zhang P, Lei X, Du L and Qu B (2025) Insights into CSF-1/CSF-1R signaling: the role of macrophage in radiotherapy. Front. Immunol. 16:1530890. doi: 10.3389/fimmu.2025.1530890
Received: 19 November 2024; Accepted: 13 January 2025;
Published: 03 February 2025.
Edited by:
Dening Ma, University of Chinese Academy of Sciences, ChinaReviewed by:
Elisabeth Müller, Oslo University Hospital, NorwayShivam Priya, The Ohio State University, United States
Copyright © 2025 Shang, Zhang, Lei, Du and Qu. This is an open-access article distributed under the terms of the Creative Commons Attribution License (CC BY). The use, distribution or reproduction in other forums is permitted, provided the original author(s) and the copyright owner(s) are credited and that the original publication in this journal is cited, in accordance with accepted academic practice. No use, distribution or reproduction is permitted which does not comply with these terms.
*Correspondence: Baolin Qu, YmFvbGlucXUzMDFAMTYzLmNvbQ==