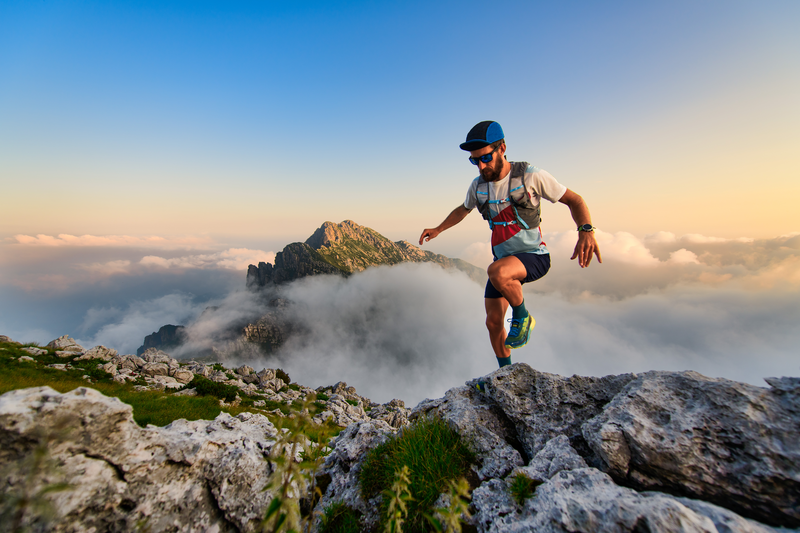
94% of researchers rate our articles as excellent or good
Learn more about the work of our research integrity team to safeguard the quality of each article we publish.
Find out more
REVIEW article
Front. Immunol. , 07 October 2024
Sec. Cancer Immunity and Immunotherapy
Volume 15 - 2024 | https://doi.org/10.3389/fimmu.2024.1469096
Tumor immunotherapy has been widely used in clinical treatment of various cancers. However, some patients of these cancers do not respond to immunotherapy effectively. And H. pylori infection has been considered to be related to the efficacy of immunotherapy. This review aims to summarize the different effects and mechanisms of H. pylori infection on immunotherapy in different kinds of cancers. We searched the relevant literature on H. pylori and tumor immunotherapy, and summarized to form a review. Generally, H. pylori infection plays a role in affecting kinds of cancers’ development, besides gastric cancer. Current evidence suggests that H. pylori infection may reduce the efficacy of immunotherapy for colorectal cancer, non-small cell lung cancer and melanoma, but due to the lack of sufficient evidence, more data is needed to prove that. While for gastric cancer, the effects remain controversial. The H. pylori regulation effects and metabolisms involved in systematic related cancers should be paid attention to. Whether H. pylori should be eradicated when immunotherapy performed may be a critical consideration for some kinds of tumors.
Helicobacter pylori (H. pylori) is a gram-negative, spiral-shaped bacterium that specifically colonizes gastric epithelial cells. It is one of the most common bacterial infections in the world, affecting the gastric mucosa in approximately 50% of the world’s population (1, 2). H. pylori is a well-known risk factor of gastrointestinal disorders including chronic gastritis, peptic ulcer, functional dyspepsia and gastric cancer (3–5). It can induce chronic non-atrophic gastritis, and then progress to intestinal metaplasia and abnormal hyperplasia, and may ultimately develop into gastric cancer. Since 1994, it has been listed as a first-class carcinogen by the World Health Organization. Besides, recent studies have indicated that H. pylori is closely related to the development of some extra-gastric cancers. For example, Ralser A et al. proved that H. pylori is a strong pathogenic factor in the development of colorectal cancer (6). Pulikonda Mounika observed that patients infected with H. pylori have an increased risk of lung cancer (7). The mechanisms have yet to be clarified.
Different from conventional chemotherapy and radiotherapy, cancer immunotherapy is an emerging cancer treatment aiming to elicit an anti-tumor immune response. It can activate immune cells and enhance the ability of the immune system, control and kill cancer cells, and eventually cure cancer (8). Immunotherapy can actually date back to 1891 when William Coley noticed that mixtures of live and inactivated Streptococcus pyogenes and Serratia marcescens could cause tumor regression in sarcoma patients (9), and then first attempted to leverage the immune system to treat cancer. In recent years, cancer treatment has been revolutionized by the advent of immunotherapies, including cancer vaccines, immune checkpoint inhibitors (ICIs), adoptive immune cell therapy, cytokine therapy, and so on. Although some treatments like adoptive cell metastases and ICIs have demonstrated clinical efficacy, their application still remains limited, benefiting only a subset of cancer patients (10). The factors affecting the efficacy of immunotherapy have prompted comprehensive investigations recently.
Tumor micro-environment (TME) is composed of a variety of immune cells, signaling molecules and extracellular matrix components, whose overall state is significantly influenced by tumor cells, and in turn modulates the activity of tumor cells. The gut microbiota constitutes the most extensive and intricate microecosystem in the human body. It can influence TME by secreting small molecule metabolites that can spread from the initial location gut, and modulate both local and systemic anti-tumor immune responses (11). H. pylori can change the cells composition of TME by various virulence factors, such as vacuolating cytotoxin A(VacA), cytotoxin-associated gene A(CagA), and neutrophil activating protein (NAP). Then immune system status and the efficacy of immunotherapy should be affected (12).
Previously, research on the relationship between H. pylori and the efficacy of tumor immunotherapy focused on gastric cancer. An increasing number of studies now point out that H. pylori is also closely related to some extra-gastric cancers. H. pylori infection may have different effects on the efficacy of immunotherapy for cancers such as melanoma, colorectal cancer and non-small cell lung cancer (13–15).
Tumor immunotherapy, particularly immune checkpoint inhibitors, has been widely utilized in clinical treatment. However, its efficacy is affected by numerous factors, which restricts its promotion. A meta-analysis and systematic review of Gong et al. systematically analyzed the effect of H. pylori on tumor immunotherapy, concluded that H. pylori would reduce its efficacy, and suggested the underlying mechanisms (16). To understand the effects and the mechanisms of H. pylori on the efficacy of cancer immunotherapy more comprehensively, we summarized relevant literature recent years. In this review, we show that the effect of H. pylori on the efficacy of cancer immunotherapy is controversial, involving the evidence and mechanisms of both beneficial and adverse effects. In addition, we classify according to different cancer types, which can more clearly reflect the characteristics of systemic manifestations caused by H. pylori infection, and arouse people’s attention to the impact of H. pylori when performing tumor immunotherapy. Through a literature search, we identified four types of cancer: gastric cancer, colorectal cancer, non-small cell lung cancer and melanoma. They are closely related to H. pylori, and there are many articles that have examined the effect of H. pylori on the efficacy of their immunotherapy, so the review will focus on these four cancers.
H. pylori colonizes the gastric mucosa and plays an important role in the occurrence and development of gastric cancer(GC). H. pylori can regulate tumor progression by affecting various cells in the TME, such as tumor-associated macrophages, bone marrow mesenchymal stem cells, cancer-associated fibroblasts, and myeloid-derived suppressor cells (12). H. pylori can regulate tumor-associated macrophage differentiation, disrupt M1/M2(or Mreg) balance, and favor the M2 phenotype to evade immune surveillance (17, 18). H. pylori infection regulates macrophage function by modulating specific microRNAs (19, 20), such as upregulating the expression levels of let-7i-5p, miR-146b-5p, and miR-185-5p (21, 22). In addition, H. pylori may induce the expression of enzymes in macrophages, such as arginase 2 and matrix metalloproteinase, leading to immune evasion (23–25). Chronic inflammation caused by H. pylori infection recruits bone marrow-derived mesenchymal stem cells to stomach, which then undergo malignant transformation because of the clonal dominance. Bone marrow-derived mesenchymal stem cells can also decrease the proportion of T cells that produces IFN-γ and inhibit the proliferation of CD4+ and CD8+ T cells, leading to local and systemic immunosuppression and the development of H. pylori-induced GC (26).
Studies have shown that H. pylori infection upregulates the expression of programmed death protein ligand-1(PD-L1) in gastric mucosa cells, which then affects the prognosis of GC patients immunotherapy (27). Sonic hedgehog(SHH) pathway is considered to be involved in PD-L1 upregulation by H. pylori infection (28). H. pylori virulence factors play critical roles in immune regulation, such as type IV secretion system(T4SS) components that activates the p38 mitogen-activated protein kinase pathway and upregulates PD-L1 expression, thereby inhibiting T cell proliferation (29). Wang et al. pointed out that H. pylori CagA can upregulate the level of exosomal PD-L1 protein in H. pylori-infected GC through the CagA-p53-miR34a-PD-L1 signaling axis, which inhibits CD8+ T cells proliferation and cytokine secretion (30, 31).
Small extracellular vesicles(sEVs) from H. pylori-positive GC cells have been reported to promote lymphangiogenesis and lymphatic remodeling via transferring over-expressed miR-1246 in sEVs to lymphatic endothelial cells (LECs). Then it can lead to hymphatic genesis, stabilize PD-L1 which inducing CD8+T cell apoptosis (32). In conclusion, H. pylori infection can affect the TME, T cell functions and PD-L1 expression of gastric mucosa, which indicates the potential effects of H. pylori infection on immunotherapy.
In addition to gastric adenocarcinoma, there is also a strong association between H. pylori infection and gastric MALT lymphoma. Data show that about 80-90% of patients with gastric MALT lymphoma are infected with H. pylori, of which 60-80% can be relieved by eradication of H. pylori alone (33). Much evidence suggests that most patients with gastric MALT lymphoma achieve complete remission after H. pylori eradication (34–36). Therefore, H. pylori eradication is important in the management of gastric MALT lymphoma.
In addition to having an impact on the treatment of lymphoma, H. pylori infection increases the risk of gastric adenocarcinoma, although cases of primary gastric lymphoma (PGL) complicated by gastric adenocarcinoma are clinically rare. Amiot et al. observed a significantly increased risk of developing a second primary malignancy in patients with gastric MALT lymphoma treated with immunotherapy/chemotherapy, while the incidence was not high in patients receiving H. pylori eradication therapy alone (37). Another study has identified an increased risk of metachronous gastric adenocarcinoma in PGL patients. It may be attributed to factors such as H. pylori infection that exacerbates the damage to the gastric mucosa (38). This potential carcinogenesis promotion deserves attention.
Several studies have observed that H. pylori infection is related to the efficacy of immunotherapy in GC patients. In metastatic GC patients treated with ICI, H. pylori infection was associated with a lower survival (39). Similarly, in another analysis of patients with advanced GC, H. pylori-positive group had a higher risk of nonclinical response to anti-PD-1 therapy. The H. pylori-negative group had a longer overall survival (OS) and progression-free survival (PFS) than the positive group. Multivariate analysis indicated that H. pylori infection was independently associated with PFS, suggesting H. pylori infection is associated with the worse outcome of immunotherapy for advanced GC patients (40). However, some previous studies have come to the opposite conclusion. According to the result of randomized KEYNOTE-062 trial, the large international CheckMate-649 trial and the randomized ATTRACTION-4 trial, Asian patients exhibited better clinical benefits from anti-PD-1/PD-L1 therapy compared with North American and European patients, which is most likely related to a higher rate of H. pylori infection in Asian GC patients (41). And in KEYNOTE-062 trial, pembrolizumab demonstrated clinically meaningful efficacy in patients with a CPS of 10 or higher for PD-L1, with better survival in patients with high PD-L1 expression, indicating that patients with higher levels of PD-L1 expression showed a trend toward better survival (42, 43). A 2021 meta-analysis involving 1870 patients has shown that H. pylori infection was associated with tumor PD-L1 expression, suggesting that H. pylori infection may be a predictor of a favorable response to immunotherapy in GC (44). Another large retrospective study of 10,122 patients with different cancer types in 2024 found that in patients with Epstein-Barr virus-negative microsatellite-stable GC, the H. pylori-positive group had longer immune-related progression-free survival (irPFS) and immune-related overall survival(irOS) than the H. pylori-negative group. And H. pylori-positive patients had higher PD-L1 levels and more unexhausted CD8+ T cells, suggesting that H. pylori infection is a beneficial factor in GC immunotherapy by shaping the thermal tumor microenvironment (15).
Therefore, there is still controversy about the specific effect of H. pylori infection on the efficacy of immunotherapy for GC, and a larger clinical study is needed to confirm the association between them. Meanwhile, the mechanisms of H. pylori affecting the efficacy of immunotherapy for gastric cancer are also being studied.
Infiltrated by a variety of immune cells, TME plays a key role in the immune evasion of tumors by mediating immune tolerance. Therefore, TME-based immunotherapy demonstrates great potential for therapeutic development. Among them, ICIs have attracted extensive attention. It mainly includes two types, anti-cytotoxic lymphocyte-associated protein 4 therapy and anti-PD-1/PD-L1 therapy. At present, ICIs targeting the PD-1/PD-L1 signaling pathway have been applied to patients with various tumors, and have shown good therapeutic effects in malignant melanoma and non-small cell lung cancer (45). However, the efficacy of PD-1 and PD-L1 inhibitors for GC remains controversial (13, 46).
PD-1 and PD-L1 are both transmembrane proteins. As an important immunosuppressive molecule, PD-1 is the receptor of PD-L1 and is mainly expressed in activated T cells, B cells, activated monocytes, natural killer T cells, and dendritic cells (DCs). PD-L1 is more widely expressed than PD-1, appearing on human activated T cells, DCs, monocytes, and some non-hematopoietic cells(e.g., lung cells, vascular endothelium, liver nonparenchymal cells, placental synctiotrophoblasts, and keratinocytes) (47). When PD-L1 binds to PD-1, programmed death of T cells can be initiated. Notably, PD-L1 is also expressed in tumor cells. Therefore, when PD-1 on the surface of activated T cells binds to PD-L1 expressed by tumor cells, the activation, proliferation, survival, cytokine secretion, and cytotoxic activity of T cells are inhibited, resulting in impaired downstream anti-tumor immune responses and ultimately tumor cells immune escape (48). Therefore, the regulation of PD-1 and PD-L1 expression by H. pylori infection may affect the efficacy of checkpoint inhibitors targeting the PD-1/PD-L1 signaling pathway.
Some studies have suggested that up-regulation of PD-L1 expression by H. pylori will weaken the efficacy of tumor immunotherapy. The SHH pathway activated by H. pylori infection induces the expression of PD-L1 and the proliferation of tumor cells in GC, leading to the resistance to immunotherapy (12). GLI1 is a key transcription factor in the SHH pathway, and H. pylori infection reduces the methylation level of the GLI1 promoter region in an m6A-dependent manner, thereby activating the expression of GLI1 and promoting tumor proliferation (49). In addition, the effect of H. pylori upregulating PD-L1 expression is manifested in many cells, such as parietal cells, macrophages, eosinophils, and dendritic cells. Upregulated PD-L1 on these cells competitively bind to PD-1 on T cells, thereby inhibiting tumor killing by T cells, reducing the efficacy of anti-PD-1/PD-L1 therapy (50).
It has been suggested that increased PD-L1 expression might be a favorable factor for ICI therapy in GC (41). CagA is an important virulence factor encoded by H. pylori, and is closely related to the pathogenesis of gastric mucosa (51, 52). The CagA protein is mediated by the H. pylori T4SS and injected into the host gastric epithelial cells. Once it enters the cells, CagA can interact with multiple molecules, disrupt the normal signaling pathway, and cause cytopathy and transformation (53, 54). In addition, CagA is associated with the immune evasion by promoting the expression of PD-L1 in tumor tissues and exosomes via the p53-miR-34a-PD-L1 signaling axis (30). And H. pylori infection induced an increase in SHH and signaling via a CagA-dependent pathway, and elevated Shh signaling mediated H. pylori-induced PD-L1 expression (28). PD-L1 blockade can restore the proliferation and cytokine secretion of CD8+ T cells inhibited by CagA, which is a promising method to improve the efficacy of immunotherapy for H. pylori-infected GC (30). Recently, other factors regulating PD-L1 in H. pylori-infected gastric epithelial cells has also been studied. Among them, miR-140 can directly inhibit PD-L1 expression. In H. pylori-positive GC, miR-140 expression is significantly reduced and PD-L1 expression is significantly increased. It has been suggested that H. pylori infection may influence the efficacy of anti-PD-1/PD-L1 therapy by decreasing the expression level of miR-140 and thereby increasing the level of PD-L1 in cancer cells (55).
In addition to regulating PD-L1 expression, the potential immunotherapeutic value of H. pylori vaccine-activated CD3+ T cells in patients with advanced GC was also revealed. The H. pylori DNA vaccine induces a shift from Th1 to Th2, which mimics the immune status of GC patients with chronic H. pylori infection. Stimulated CD3+ T cells inhibit the growth of human GC cells in vitro, and adoptive transfusion of CD3+ T cells inhibits the growth of GC xenografts in vivo, suggesting the anticancer effects of CD3+ T cells both in vitro and in vivo. This may be due to a greater proportion of CD8+/CD4+ T cell infiltration, decreased regulatory FOXP3+ T cell infiltration, upregulation of Caspase-9/Caspase-3, and enhanced apoptosis induced by downregulation of Survivin (56). Taken together, increased PD-L1 expression and CD3+ T cells may be favorable factors for ICI therapy for GC. Therefore, it is speculated that H. pylori infection may be a novel biological factor that may affect the survival benefit of patients with advanced GC treated with first-line ICIs (41).
Although H. pylori typically colonizes the gastric mucosa and primarily targets the stomach, there are increasing evidences demonstrating the association between H. pylori infection and other extra-gastric diseases, including colorectal cancer (CRC). Data showed that H. pylori infection was associated with a higher risk of colorectal adenoma and CRC, with pooled odds ratios of 1.51 and 1.70, respectively (57, 58). A study of 47,714,750 patients demonstrated an independent positive correlation between CRC risk and history of H. pylori infection (59). Another retrospective study of 96,572 subjects who had been eradicated from H. pylori concluded that the incidence of CRC gradually decreased to a lower level than that of the general population, especially rectal cancer (60). Together, these evidences suggested a strong link between H. pylori and CRC.
A number of studies have also confirmed that H. pylori infection can directly promote the development of CRC in vivo and in vitro. In an employed Apc mutant mouse lines(Apc+/min and Apc+/1638N) as a surrogate model of human CRC, an almost twofold increase in the number of tumors in mice infected with H. pylori was observed, which is consistent with the fact observed in epidemiology that people infected with H. pylori have a nearly twofold increased risk of developing CRC (6). The pro-inflammatory Th17 response induced by H. pylori, especially the differentiation of Treg cells into the Th17 phenotype, may constitute one of the main mechanisms for enhancing tumor development. In addition, the strong pro-inflammatory response induced locally by H. pylori in the small intestine is accompanied by the activation of NF-κB and STAT3 pathways. Activated STAT3 levels in tissues are also related to reduced tumor invasion, tumor, lymph node, metastatic stage, and overall survival in CRC patients (61). H. pylori virulence factors may also affect the development and progression of CRC. CagA increases the levels of the pro-survival factor phosphorylated ERK and the anti-apoptotic protein MCL1, therefore dampening gut epithelial self-renewal by inhibiting apoptosis (62). In addition, H. pylori(CagA+) inhibits the expression of miR-125b-5p in CRC cells and promotes autophagy, contributing to the development and invasion of colon cancer (63). Another virulence factor VacA enters cells and mitochondrial membranes through the formation of chloride(Cl-) channels, resulting in loss of mitochondrial membrane potential, mitochondrial fragmentation, reactive oxygen species formation, autophagy (64). Cl-channel abnormalities are associated with cystic fibrosis, and cystic fibrosis is known to be associated with CRC. Hence, it is suggested that VacA-induced Cl-channel abnormality may be associated with CRC (65).
H. pylori can also affect CRC through indirect pathways. H. pylori is known to affect the local gastric microbiota as well as distant microbiota in the intestine and colon (66). Inflammation-driven intestinal dysbiosis microbiota can promote the formation and progression of colorectal tumors (67). Enrichment of the genera Akkermansia and Ruminococcus was found, and there was an inverse correlation between the presence of Akkermansia and gastrointestinal disease (68). H. pylori infection destroys intestinal mucus integrity in mice models, and a general loss of goblet cells were also observed, which are important for the production of mucin and antimicrobial peptides. The over-expression of certain mucins in tumors is likely to be the reason for the increase in Akkermansia in CRC patients (69). In all, H. pylori infection promotes CRC by altering intestinal homeostasis, including the integrity of intestinal mucosa, the immune barrier, and intestinal microbiota.
In addition, H. pylori can alter gastrin levels in the body and may have an effect on CRC. H. pylori gastritis can cause atrophic gastritis and reduce gastric acid secretion. As a result, the concentration of gastrin in the blood increases. Gastrin causes overgrowth of the large intestine mucosa and is strongly associated with the development of colorectal tumors (70–72). The over-expression of gastrin and gastrin receptors(CCKBR) and cyclooxygenase-2(COX-2) may stimulate tumor growth, angiogenesis, and reduce apoptosis (73). Therefore, gastrin may be a potential factor in the development of CRC in H. pylori infection (74). However, there is still much controversy about whether H. pylori contributes to CRC (75, 76) and further studies are needed to confirm the association.
The traditional treatment methods for CRC are mainly radiotherapy, surgical resection, etc. However, CRC is difficult to detect in the early stage. When patients show clinical symptoms, it is often at an advanced stage and metastasize to distant places, and traditional treatments are not effective at this time (77). Fortunately, ICI-based immunotherapies in patients with CRC have achieved satisfactory results in terms of safety and efficacy. Multiple immunotherapy drugs, including pembrolizumab, nivolumab, and ipilimumab, have been approved for the treatment of advanced CRC. At the same time, new therapies such as CAR-T and cancer vaccines also have good prospects (78). Therefore, elucidating the factors influencing immunotherapy for CRC will benefit more patients.
In mice treated with anti-CTLA4 alone, the tumor volume of H. pylori infected mice was significantly larger than that of uninfected mice. Same results were observed in mice treated with a combination of anti-CTLA4 and anti-PD-L1. It can be concluded that mice infected with H. pylori are less sensitive and responsive to ICIs. In addition, the number of colon tumors in uninfected mice treated with anti-CTLA4 was significantly lower than in H. pylori infected mice (14). Therefore, it is suggested that H. pylori infection appears to play a disruptive role in immunotherapy for CRC.
Clinical data also support that H. pylori has an adverse effect on ICIs for CRC. DNA mismatch repair-deficient(dMMR)/microsatellite instability-high(MSI-H) colorectal adenocarcinoma has a high sensitivity to immunotherapy and has been approved for anti-PD-1/PD-L1 immunotherapy. Based on data from patients with dMMR/MSI-H colorectal adenocarcinoma who received anti-PD-1/PD-L1 immunotherapy, researchers found that the median irPFS of patients with H. pylori-positive cancer was significantly shorter than that of H. pylori-negative patients, and the same discrepancy were observed in irOS. In addition, there is also a trend towards a shortening of irFPS in H. pylori-positive patients with dMMR/MSS colorectal adenocarcinoma (15). These data indicate that H. pylori may reduce the effectiveness of ICIs and is not favorable to patient outcomes.
In conclusion, it has been observed that H. pylori infection can diminish the efficacy of immunotherapy of CRC. Therefore, before administering immunotherapy to patients with CRC, it may be advisable to detect and eradicate H. pylori to attenuate its negative influence on immunotherapy.
H. pylori infection may be relevant to the risk of lung cancer, but the evidence supporting this association remains controversial due to variations in sample size, sample selection criteria, and research methodology. Some data have shown that H. pylori biomarkers, such as VacA, CagA, H. pylori1564, and catalase, were significantly associated with an increased risk of lung cancer (79). Multiple meta-analysis show that patients with H. pylori infection were observed a remarkably increased risk of lung cancer (7, 80). Seroprevalence of H. pylori antibody in lung cancer patients was significantly higher than that in non-lung cancer patients (81). The seroprevalence of H. pylori, especially that expressing CagA, is significantly higher in lung cancers than in healthy controls (82).
Gastric reflux containing H. pylori components through the oral-pharyngeal-laryngeal axis may lead to respiratory colonization of H. pylori (83). H. pylori can also be detected in dental plaque and saliva in human subjects, suggesting that the oral cavity may be an extra-gastric repository for H. pylori (84), which can lead to inflammation of the airways and the development of lung cancer. In fact, the presence of H. pylori and/or its virulence factors in the mucous membranes of the lungs and upper respiratory tract has been identified (83). VacA was identified in surgical lung biopsy tissue samples from patients with pneumonia (85). Inhaled into the lungs, VacA can stimulate lung epithelial cells to secrete inflammatory cytokines and damage lung tissue. Moreover, H. pylori urease protein has also been found to enter the lungs through gastroesophageal reflux disease, providing an antigenic trigger for the development of pulmonary granulomas contributing to proliferation and carcinogenesis of the lung mucosa (83, 86).
Increased gastrin concentrations caused by H. pylori in the stomach may also stimulate lung cell proliferation. In fact, gastrin levels are elevated in lung cancer tissues (82). Studies have shown that H. pylori infection in lung cancer patients is accompanied by significantly elevated gastrin levels in plasma and tumor tissues, as well as increased cyclooxygenase-1(COX-1) and cyclooxygenase-2(COX-2) expression (82). COX-1 and COX-2 are the critical enzymes for the production of prostaglandin E2(PGE2), and the COX-2 has been found overexpressed in many cancers. PGE2 is a prostanoid lipid associated with enhancement of cancer cell survival, growth, migration, invasion, angiogenesis, and immunosuppression (87). Gastrin may contribute to lung cancer by inducing bronchial epithelial mucosal cell proliferation, atrophy, and induction of COX-2. In addition, lipopolysaccharide of H. pylori, may stimulate the production of proinflammatory cytokines like interleukins (7). Chronic inflammatory irritation resulting from this may also be the cause of lung cancer.
In addition, the signaling adaptor protein p130Cas in tumor cell is associated with carcinogenesis and prognosis of lung cancer. Specifically, p130Cas over-expression has been linked to reduced OS in lung cancer patients (88, 89). Therefore, p130Cas, activated by Src kinase following H. pylori infection, may involve in lung carcinogenesis during H. pylori infection (83).
In addition to influencing the occurrence of NSCLC through direct or indirect pathways, H. pylori infection may also be associated with the efficacy of immunotherapy for NSCLC.
The treatment of NSCLC was mainly surgery, radiotherapy, and chemotherapy for an extended period. However, recent studies have shown that NSCLC is one of the most sensitive cancers to ICIs. Many studies have demonstrated that ICIs, including atezolizumab, pembrolizumab and nivolumab, exhibit good efficacy in the treatment of NSCLC (90–92). However, some patients exhibit resistance to immunotherapy. Therefore, it is essential to identify the factors that affect patient prognosis in order to benefit NSCLC patients.
In a cohort of 60(18 H. pylori serology-positive) NSCLC patients in France, H. pylori seropositivity was associated with a significant decrease in survival of NSCLC patients after receiving anti-PD-1 therapy(p = 0.0001) with median survival of 6.7 months and 15.4 months, respectively. A decline in OS was also noted in a cohort of 29 patients(9 H. pylori serology-positive) in Canada(9.3 versus 21.7 months) and PFS was also observed among patients with H. pylori-positive NSCLC. These results showed that H. pylori serology positivity was associated with poor efficacy of anti-PD-1 therapy (14). In addition, the number of monocyte cell lines in H. pylori-positive tumors was significantly reduced compared to H. pylori-negative patients. Additionally, the gene expression induced by type I interferon, IFN-γ, and IL-6 was also decreased. The decrease in monocytes indicates that inhibition of the host innate immune response induced by H. pylori may reduce the efficacy of cancer immunotherapy (14). This may be a mechanism by which H. pylori affects tumor immunotherapy. Further study also confirmed that the reduced efficacy of tumor immunotherapy with H. pylori infection is independent of alterations in the fecal microbiota caused by H. pylori (13).
Up to now, there are still few studies investigating the effect of H. pylori on the efficacy of immunotherapy in NSCLC patients. Existing studies have limitations such as inadequate sample size and H. pylori detection methods, and more comprehensive studies are needed to fill the gaps (46).
Melanoma is one of the most aggressive forms of skin cancer that develops from skin melanocytes located in the basal layer of the epidermis. Ultraviolet radiation is the main cause of cutaneous melanoma (93). Over the past years, there has been a rise in the incidence of melanoma and melanoma-related mortality, which poses a threat to the healthcare system (94). Research on the relationship between H. pylori and melanoma has mainly focused on the application of H. pylori neutrophil activating protein (HP-NAP) in the treatment of melanoma. HP-NAP is a dodecameric protein that stimulates the maturation and differentiation of monocytes and immature dendritic cells. These cells subsequently produce cytokines such as tumor necrosis factor-α(TNF-α), IL-6 and IL-12 (95), which regulate the immune response and potentially influence tumor development. Recent studies have observed that HP-NAP effectively inhibited the growth and metastasis of melanoma and improved the OS rate of zebrafish of melanoma xenograft, which was accompanied by strong recruitment of macrophages with a pro-inflammatory profile at the tumor site (96). In addition, recombinant H. pylori neutrophil activation protein(rMBP-NAP) prevented lung metastasis from melanoma and induced systemic Th1 immune response with anti-angiogenic activity (97). It was also proved that rHP-NAP promoted the maturation of dendritic cell vaccine and enhanced the cytotoxic response. rHP-NAP may serve as an adjuvant against melanoma dendritic cell vaccines (98).
In conclusion, the NAP induced by H. pylori may have a promising future in the treatment to melanoma.
Melanoma is highly sensitive to immunotherapy attributed to its elevated tumor mutational load and high T cell infiltration. In 2011, ipilimumab, the first CTLA-4 immune checkpoint inhibitor, was approved for the treatment of advanced inoperable metastatic melanoma. In the following ten years, multiple monoclonal antibodies have been used to treat melanoma at different stages, such as nivolumab and pembrolizumab, which have achieved good efficacy and significantly improved the survival rate of patients with advanced melanoma (99). Despite advancements, some melanoma patients treated with immunotherapy continue to encounter recurrence and adverse effects (100). Therefore, it is crucial to identity the specific factors that affect their efficacy. Among these factors, the influence of H. pylori has attracted widespread attention.
H. pylori infection may hinder the immunotherapy response in melanoma. Compared with H. pylori-negative patients, H. pylori-positive patients with melanoma had significantly shorter OS(p = 0.02), PFS and objective response rate, suggesting that H. pylori infection affected the efficacy of ICIs (13). In addition, in B16-OVA melanoma model, mice were injected with CpG-adjuvanted OVA peptide, which served as a cancer vaccine. Notably, the tumor volume in H. pylori-uninfected vaccinated mice was significantly smaller than that of H. pylori-infected vaccinated mice. This indicated that the vaccine efficacy was diminished in the presence of H. pylori-positive tumor-bearing mice (14).
The mechanism that H. pylori diminishes the efficacy of melanoma immunotherapy is not yet well understood. But it is certain that it’s independent of the alteration in the fecal microbiota composition induced by H. pylori (13, 14). There were no significant differences in the alpha diversity metrics of fecal microbiota in H. pylori-positive or negative patients. And beta diversity analysis between both groups showed no significant cluster difference following statistical analysis. This indicates that the presence or absence of H. pylori infection does not affect the overall diversity of the human fecal microbiota. Furthermore, the presence of parahaemodhemostreptococcus was associated to resistance against ICIs in melanoma (13). Transplanting the feces of H. pylori-positive mice to H. pylori-negative mice did not reduce the efficacy of cancer vaccines, while transplanting feces from H. pylori-negative mice to positive mice still reduced the efficacy of cancer vaccines (14). More research is needed to elucidate the underlying mechanisms.
In conclusion, H. pylori weakens the efficacy of immunotherapy in experimental models and clinical patients, but the specific mechanisms remain unclear. H. pylori infection should be taken into account in the treatment of melanoma.
This review summarizes current research progress on the effects of H. pylori on the cancer’s development and immunotherapy (Figure 1). Immunotherapy for oncology is developing rapidly and is becoming a new option for patients who can’t benefit from traditional therapies. However, immunotherapy is limited until now. There are many patients who do not respond well to tumor immunotherapy. Identifying the factors affecting the efficacy of immunotherapy is crucial for improving immunotherapy efficacy. Studies have found that H. pylori infection plays a role in both intragastric and extra-gastric diseases, and even has a strong impact on tumor immunotherapy. Numerous clinical investigations have shown that the responsiveness to immunotherapy is related to H. pylori-positive status, such as GC, CRC, NSCLC, and melanoma. The effects of H. pylori on patients with different cancer types may be different, and the mechanisms may be related to the activation on immune cell status, PD-L1 expression level regulation, inflammatory pathway action and so on (6, 7, 13, 39, 101). Based on different effects of H. pylori on different cancers, whether to eradicate H. pylori may be an important consideration in clinical practice to improve immunotherapy efficacy (33, 60). On the contrary, utilizing H. pylori to improve the efficacy of immunotherapy, such as the application of HP-NAP in the treatment of melanoma, may be a promising application (96, 97, 102).
Figure 1. The effect of H. pylori infection on the efficacy of immunotherapy for various cancers and potential mechanisms. NSCLC, non-small cell lung cancer; CagA, cytotoxin-associated gene A; SHH signal pathway, Sonic hedgehog signal pathway; PD-L1, programmed death ligand 1; PD-1, programmed cell death protein 1; CTLA4, cytotoxic T lymphocyte-associated antigen-4; ICIs, immune checkpoint inhibitors; IL-6, interleukin 6; IFN-γ, interferon-γ; HP-NAP, H. pylori neutrophil activating protein; rMBP-NAP, recombinant HP-NAP with the maltose-binding protein of Escherichia coli; rHP-NAP, recombinant H. pylori neutrophil activating protein; DC, dendritic cell. The green arrow indicates a positive effect on tumor immunotherapy, and the red arrow indicates a negative effect on tumor immunotherapy.
In the future, more clinical data and studies are needed to ensure the role of H. pylori in immunotherapy. This will facilitate the identification of more specific mechanisms and targets to effectively select patients who are likely to benefit from immunotherapy, thereby offering insights for the research and development of novel therapeutics.
XZ: Investigation, Methodology, Writing – original draft. HZ: Writing – review & editing. SZ: Writing – review & editing. ZW: Writing – review & editing. YSu: Writing – review & editing. KZ: Project administration, Resources, Supervision, Writing – review & editing. MW: Project administration, Supervision, Validation, Writing – review & editing. YSh: Funding acquisition, Methodology, Project administration, Resources, Supervision, Validation, Writing – review & editing.
The author(s) declare financial support was received for the research, authorship, and/or publication of this article. This work is supported by grants from Beijing Natural Science Foundation of China (J230002), the National Natural Science Foundation of China (81700496), Key Laboratory of Epidemiology of Major Diseases (Peking University), Ministry of Education, the special fund of the Beijing Clinical Key Specialty Construction Program, P. R. China (2021).
The authors declare that the research was conducted in the absence of any commercial or financial relationships that could be construed as a potential conflict of interest.
All claims expressed in this article are solely those of the authors and do not necessarily represent those of their affiliated organizations, or those of the publisher, the editors and the reviewers. Any product that may be evaluated in this article, or claim that may be made by its manufacturer, is not guaranteed or endorsed by the publisher.
1. Mentis A, Lehours P, Mégraud F. Epidemiology and diagnosis of helicobacter pylori infection. Helicobacter. (2015) 20 Suppl 1:1–7. doi: 10.1111/hel.12250
2. Hooi JKY, Lai WY, Ng WK, Suen MMY, Underwood FE, Tanyingoh D, et al. Global prevalence of helicobacter pylori infection: systematic review and meta-analysis. Gastroenterology. (2017) 153:420–9. doi: 10.1053/j.gastro.2017.04.022
3. Manabe N, Matsueda K, Haruma K. Epidemiological review of gastroesophageal junction adenocarcinoma in Asian countries. Digestion. (2022) 103:29–36. doi: 10.1159/000519602
4. Zavros Y, Merchant JL. The immune microenvironment in gastric adenocarcinoma. Nat Rev Gastroenterol Hepatol. (2022) 19:451–67. doi: 10.1038/s41575-022-00591-0
5. Ernst PB, Gold BD. The disease spectrum of helicobacter pylori: the immunopathogenesis of gastroduodenal ulcer and gastric cancer. Annu Rev Microbiol. (2000) 54:615–40. doi: 10.1146/annurev.micro.54.1.615
6. Ralser A, Dietl A, Jarosch S, Engelsberger V, Wanisch A, Janssen KP, et al. Helicobacter pylori promotes colorectal carcinogenesis by deregulating intestinal immunity and inducing a mucus-degrading microbiota signature. Gut. (2023) 72:1258–70. doi: 10.1136/gutjnl-2022-328075
7. Mounika P. Helicobacter pylori infection and risk of lung cancer: A meta-analysis. Lung Cancer Int. (2013) 2013:131869. doi: 10.1155/2013/131869
8. Kumar AR, Devan AR, Nair B, Vinod BS, Nath LR. Harnessing the immune system against cancer: current immunotherapy approaches and therapeutic targets. Mol Biol Rep. (2021) 48:8075–95. doi: 10.1007/s11033-021-06752-9
10. Zhang Y, Zhang Z. The history and advances in cancer immunotherapy: understanding the characteristics of tumor-infiltrating immune cells and their therapeutic implications. Cell Mol Immunol. (2020) 17:807–21. doi: 10.1038/s41423-020-0488-6
11. Lu Y, Yuan X, Wang M, He Z, Li H, Wang J, et al. Gut microbiota influence immunotherapy responses: mechanisms and therapeutic strategies. J Hematol Oncol. (2022) 15:47. doi: 10.1186/s13045-022-01273-9
12. Deng R, Zheng H, Cai H, Li M, Shi Y, Ding S. Effects of helicobacter pylori on tumor microenvironment and immunotherapy responses. Front Immunol. (2022) 13:923477. doi: 10.3389/fimmu.2022.923477
13. Tonneau M, Nolin-Lapalme A, Kazandjian S, Auclin E, Panasci J, Benlaifaoui M, et al. Helicobacter pylori serology is associated with worse overall survival in patients with melanoma treated with immune checkpoint inhibitors. Oncoimmunology. (2022) 11:2096535. doi: 10.1080/2162402x.2022.2096535
14. Oster P, Vaillant L, Riva E, McMillan B, Begka C, Truntzer C, et al. Helicobacter pylori infection has a detrimental impact on the efficacy of cancer immunotherapies. Gut. (2022) 71:457–66. doi: 10.1136/gutjnl-2020-323392
15. Jia K, Chen Y, Xie Y, Wang X, Hu Y, Sun Y, et al. Helicobacter pylori and immunotherapy for gastrointestinal cancer. Innovation (Camb). (2024) 5:100561. doi: 10.1016/j.xinn.2023.100561
16. Gong X, Shen L, Xie J, Liu D, Xie Y, Liu D. Helicobacter pylori infection reduces the efficacy of cancer immunotherapy: A systematic review and meta-analysis. Helicobacter. (2023) 28:e13011. doi: 10.1111/hel.13011
17. Gobert AP, Verriere T, Asim M, Barry DP, Piazuelo MB, de Sablet T, et al. Heme oxygenase-1 dysregulates macrophage polarization and the immune response to helicobacter pylori. J Immunol. (2014) 193:3013–22. doi: 10.4049/jimmunol.1401075
18. Cassetta L, Cassol E, Poli G. Macrophage polarization in health and disease. ScientificWorldJournal. (2011) 11:2391–402. doi: 10.1100/2011/213962
19. Pagliari M, Munari F, Toffoletto M, Lonardi S, Chemello F, Codolo G, et al. Helicobacter pylori affects the antigen presentation activity of macrophages modulating the expression of the immune receptor cd300e through mir-4270. Front Immunol. (2017) 8:1288. doi: 10.3389/fimmu.2017.01288
20. Fabian MR, Sonenberg N. The mechanics of mirna-mediated gene silencing: A look under the hood of mirisc. Nat Struct Mol Biol. (2012) 19:586–93. doi: 10.1038/nsmb.2296
21. Codolo G, Toffoletto M, Chemello F, Coletta S, Soler Teixidor G, Battaggia G, et al. Helicobacter pylori dampens hla-ii expression on macrophages via the up-regulation of mirnas targeting ciita. Front Immunol. (2019) 10:2923. doi: 10.3389/fimmu.2019.02923
22. Coletta S, Battaggia G, Della Bella C, Furlani M, Hauke M, Faass L, et al. Adp-heptose enables helicobacter pylori to exploit macrophages as a survival niche by suppressing antigen-presenting hla-ii expression. FEBS Lett. (2021) 595:2160–8. doi: 10.1002/1873-3468.14156
23. Lewis ND, Asim M, Barry DP, de Sablet T, Singh K, Piazuelo MB, et al. Immune evasion by helicobacter pylori is mediated by induction of macrophage arginase ii. J Immunol. (2011) 186:3632–41. doi: 10.4049/jimmunol.1003431
24. Hardbower DM, Asim M, Murray-Stewart T, Casero RA Jr., Verriere T, Lewis ND, et al. Arginase 2 deletion leads to enhanced M1 macrophage activation and upregulated polyamine metabolism in response to helicobacter pylori infection. Amino Acids. (2016) 48:2375–88. doi: 10.1007/s00726-016-2231-2
25. Krakowiak MS, Noto JM, Piazuelo MB, Hardbower DM, Romero-Gallo J, Delgado A, et al. Matrix metalloproteinase 7 restrains helicobacter pylori-induced gastric inflammation and premalignant lesions in the stomach by altering macrophage polarization. Oncogene. (2015) 34:1865–71. doi: 10.1038/onc.2014.135
26. Lin R, Ma H, Ding Z, Shi W, Qian W, Song J, et al. Bone marrow-derived mesenchymal stem cells favor the immunosuppressive T cells skewing in a helicobacter pylori model of gastric cancer. Stem Cells Dev. (2013) 22:2836–48. doi: 10.1089/scd.2013.0166
27. Aydın EM, Demir TD, Seymen N, Said SS, Oktem-Okullu S, Tiftikci A, et al. The crosstalk between H. Pylori virulence factors and the pd1:Pd-L1 immune checkpoint inhibitors in progression to gastric cancer. Immunol Lett. (2021) 239:1–11. doi: 10.1016/j.imlet.2021.06.009
28. Holokai L, Chakrabarti J, Broda T, Chang J, Hawkins JA, Sundaram N, et al. Increased programmed death-ligand 1 is an early epithelial cell response to helicobacter pylori infection. PloS Pathog. (2019) 15:e1007468. doi: 10.1371/journal.ppat.1007468
29. Lina TT, Alzahrani S, House J, Yamaoka Y, Sharpe AH, Rampy BA, et al. Helicobacter pylori cag pathogenicity island's role in B7-H1 induction and immune evasion. PloS One. (2015) 10:e0121841. doi: 10.1371/journal.pone.0121841
30. Wang J, Deng R, Chen S, Deng S, Hu Q, Xu B, et al. Helicobacter pylori caga promotes immune evasion of gastric cancer by upregulating pd-L1 level in exosomes. iScience. (2023) 26:108414. doi: 10.1016/j.isci.2023.108414
31. Liu Z, Wu X, Tian Y, Zhang W, Qiao S, Xu W, et al. H. Pylori infection induces cxcl8 expression and promotes gastric cancer progress through downregulating klf4. Mol Carcinog. (2021) 60:524–37. doi: 10.1002/mc.23309
32. Lu C, Xie L, Qiu S, Jiang T, Wang L, Chen Z, et al. Small extracellular vesicles derived from helicobacter pylori-infected gastric cancer cells induce lymphangiogenesis and lymphatic remodeling via transfer of Mir-1246. Small. (2023) 20(13):e2308688. doi: 10.1002/smll.202308688
33. Nakamura S, Hojo M. Diagnosis and treatment for gastric mucosa-associated lymphoid tissue (Malt) lymphoma. J Clin Med. (2022) 12(1):20. doi: 10.3390/jcm12010120
34. Zullo A, Hassan C, Cristofari F, Andriani A, De Francesco V, Ierardi E, et al. Effects of helicobacter pylori eradication on early stage gastric mucosa-associated lymphoid tissue lymphoma. Clin Gastroenterol Hepatol. (2010) 8:105–10. doi: 10.1016/j.cgh.2009.07.017
35. Nakamura S, Sugiyama T, Matsumoto T, Iijima K, Ono S, Tajika M, et al. Long-term clinical outcome of gastric malt lymphoma after eradication of helicobacter pylori: A multicentre cohort follow-up study of 420 patients in Japan. Gut. (2012) 61:507–13. doi: 10.1136/gutjnl-2011-300495
36. Nakamura S, Matsumoto T. Helicobacter pylori and gastric mucosa-associated lymphoid tissue lymphoma: recent progress in pathogenesis and management. World J Gastroenterol. (2013) 19:8181–7. doi: 10.3748/wjg.v19.i45.8181
37. Amiot A, Jooste V, Gagniere C, Lévy M, Copie-Bergman C, Dupuis J, et al. Second primary Malignancies in patients treated for gastric mucosa-associated lymphoid tissue lymphoma. Leuk Lymph. (2017) 58:1–11. doi: 10.1080/10428194.2017.1283033
38. Inaba K, Kushima R, Murakami N, Kuroda Y, Harada K, Kitaguchi M, et al. Increased risk of gastric adenocarcinoma after treatment of primary gastric diffuse large B-cell lymphoma. BMC Cancer. (2013) 13:499. doi: 10.1186/1471-2407-13-499
39. Magahis PT, Maron SB, Cowzer D, King S, Schattner M, Janjigian Y, et al. Impact of helicobacter pylori infection status on outcomes among patients with advanced gastric cancer treated with immune checkpoint inhibitors. J Immunother Cancer. (2023) 11(10):e007699. doi: 10.1136/jitc-2023-007699
40. Che H, Xiong Q, Ma J, Chen S, Wu H, Xu H, et al. Association of helicobacter pylori infection with survival outcomes in advanced gastric cancer patients treated with immune checkpoint inhibitors. BMC Cancer. (2022) 22:904. doi: 10.1186/s12885-022-10004-9
41. Zhang MJ, Chen DS, Li S, Chen L, Qi YX, Zhang CJ. Helicobacter pylori infection as a potential favorable factor for immune checkpoint inhibitor therapy for gastric cancer. Invest New Drugs. (2021) 39:1436–8. doi: 10.1007/s10637-021-01122-5
42. Shitara K, Van Cutsem E, Bang YJ, Fuchs C, Wyrwicz L, Lee KW, et al. Efficacy and safety of pembrolizumab or pembrolizumab plus chemotherapy vs chemotherapy alone for patients with first-line, advanced gastric cancer: the keynote-062 phase 3 randomized clinical trial. JAMA Oncol. (2020) 6:1571–80. doi: 10.1001/jamaoncol.2020.3370
43. Tabernero J, Van Cutsem E, Bang YJ, Fuchs CS, Wyrwicz L, Lee KW, et al. Pembrolizumab with or without chemotherapy versus chemotherapy for advanced gastric or gastroesophageal junction (G/gej) adenocarcinoma: the phase iii keynote-062 study. J Clin Oncol. (2019) 37. doi: 10.1200/JCO.2019.37.18_suppl.LBA4007
44. Zhu Y, Zhu F, Ba H, Chen J, Bian X. Helicobacter pylori infection and pd-L1 expression in gastric cancer: A meta-analysis. Eur J Clin Invest. (2023) 53:e13880. doi: 10.1111/eci.13880
45. Kraehenbuehl L, Weng CH, Eghbali S, Wolchok JD, Merghoub T. Enhancing immunotherapy in cancer by targeting emerging immunomodulatory pathways. Nat Rev Clin Oncol. (2022) 19:37–50. doi: 10.1038/s41571-021-00552-7
46. Oster P, Vaillant L, McMillan B, Velin D. The efficacy of cancer immunotherapies is compromised by helicobacter pylori infection. Front Immunol. (2022) 13:899161. doi: 10.3389/fimmu.2022.899161
47. Keir ME, Butte MJ, Freeman GJ, Sharpe AH. Pd-1 and its ligands in tolerance and immunity. Annu Rev Immunol. (2008) 26:677–704. doi: 10.1146/annurev.immunol.26.021607.090331
48. Han Y, Liu D, Li L. Pd-1/pd-L1 pathway: current researches in cancer. Am J Cancer Res. (2020) 10:727–42.
49. Sun J, Zhu W, Luan M, Xing Y, Feng Z, Zhu J, et al. Positive Gli1/Inhba feedback loop drives tumor progression in gastric cancer. Cancer Sci. (2024) 115(7):2301–17. doi: 10.1111/cas.16193
50. Shi Y, Zheng H, Wang M, Ding S. Influence of helicobacter pylori infection on pd-1/pd-L1 blockade therapy needs more attention. Helicobacter. (2022) 27:e12878. doi: 10.1111/hel.12878
51. Salvatori S, Marafini I, Laudisi F, Monteleone G, Stolfi C. Helicobacter pylori and gastric cancer: pathogenetic mechanisms. Int J Mol Sci. (2023) 24(3):2895. doi: 10.3390/ijms24032895
52. Wang H, Zhao M, Shi F, Zheng S, Xiong L, Zheng L. A review of signal pathway induced by virulent protein caga of helicobacter pylori. . Front Cell Infect Microbiol. (2023) 13:1062803. doi: 10.3389/fcimb.2023.1062803
53. Wan X, Liu C. Advances in caga protein and cagamediated pathogenesis of helicobacter pylori-a review. Wei sheng wu xue bao = Acta microbiol Sin. (2016) 56:1821–30. doi: 10.13343/j.cnki.wsxb.20160119
54. Tran SC, Bryant KN, Cover TL. The helicobacter pylori cag pathogenicity island as a determinant of gastric cancer risk. Gut Microbes. (2024) 16:2314201. doi: 10.1080/19490976.2024.2314201
55. Zhao M, Liu Q, Liu W, Zhou H, Zang X, Lu J. Microrna−140 suppresses helicobacter pylori−Positive gastric cancer growth by enhancing the antitumor immune response. Mol Med Rep. (2019) 20:2484–92. doi: 10.3892/mmr.2019.10475
56. Xue LJ, Mao XB, Liu XB, Gao H, Chen YN, Dai TT, et al. Activation of cd3(+) T cells by helicobacter pylori DNA vaccines in potential immunotherapy of gastric carcinoma. Cancer Biol Ther. (2019) 20:866–76. doi: 10.1080/15384047.2019.1579957
57. Ko HJ, Lin YC, Chen CC, Chen MJ, Wu MS, Liu CJ, et al. Helicobacter pylori infection and increased diabetes prevalence were the risks of colorectal adenoma for adults: A systematic review and meta-analysis (Prisma-compliant article). Med (Baltimore). (2021) 100:e28156. doi: 10.1097/md.0000000000028156
58. Zuo Y, Jing Z, Bie M, Xu C, Hao X, Wang B. Association between helicobacter pylori infection and the risk of colorectal cancer: A systematic review and meta-analysis. Med (Baltimore). (2020) 99:e21832. doi: 10.1097/md.0000000000021832
59. Boustany A, Onwuzo S, Almomani A, Asaad I. Epidemiology and risk of colorectal cancer in patients with a history of helicobacter pylori infection: A population-based study. Ann Gastroenterol. (2023) 36:203–7. doi: 10.20524/aog.2023.0783
60. Guo CG, Zhang F, Jiang F, Wang L, Chen Y, Zhang W, et al. Long-term effect of helicobacter pylori eradication on colorectal cancer incidences. Therap Adv Gastroenterol. (2023) 16:17562848231170943. doi: 10.1177/17562848231170943
61. Kusaba T, Nakayama T, Yamazumi K, Yakata Y, Yoshizaki A, Inoue K, et al. Activation of stat3 is a marker of poor prognosis in human colorectal cancer. Oncol Rep. (2006) 15:1445–51. doi: 10.3892/or
62. Mimuro H, Suzuki T, Nagai S, Rieder G, Suzuki M, Nagai T, et al. Helicobacter pylori dampens gut epithelial self-renewal by inhibiting apoptosis, a bacterial strategy to enhance colonization of the stomach. Cell Host Microbe. (2007) 2:250–63. doi: 10.1016/j.chom.2007.09.005
63. Zhong X, Chen O, Zhou T, Lü M, Wan J. Cytotoxin-associated gene a-positive helicobacter pylori promotes autophagy in colon cancer cells by inhibiting mir-125b-5p. Can J Infect Dis Med Microbiol. (2021) 2021:6622092. doi: 10.1155/2021/6622092
64. Rassow J, Meinecke M. Helicobacter pylori vaca: A new perspective on an invasive chloride channel. Microbes Infect. (2012) 14:1026–33. doi: 10.1016/j.micinf.2012.07.002
65. Ponzetto A, Figura N. Colon cancer risk and vaca toxin of helicobacter pylori. Gastroenterology. (2019) 156:2356. doi: 10.1053/j.gastro.2018.11.083
66. Guo Y, Zhang Y, Gerhard M, Gao JJ, Mejias-Luque R, Zhang L, et al. Effect of helicobacter pylori on gastrointestinal microbiota: A population-based study in linqu, a high-risk area of gastric cancer. Gut. (2020) 69:1598–607. doi: 10.1136/gutjnl-2019-319696
67. Uronis JM, Mühlbauer M, Herfarth HH, Rubinas TC, Jones GS, Jobin C. Modulation of the intestinal microbiota alters colitis-associated colorectal cancer susceptibility. PloS One. (2009) 4:e6026. doi: 10.1371/journal.pone.0006026
68. Wang L, Tang L, Feng Y, Zhao S, Han M, Zhang C, et al. A purified membrane protein from akkermansia muciniphila or the pasteurised bacterium blunts colitis associated tumourigenesis by modulation of cd8(+) T cells in mice. Gut. (2020) 69:1988–97. doi: 10.1136/gutjnl-2019-320105
69. Weir TL, Manter DK, Sheflin AM, Barnett BA, Heuberger AL, Ryan EP. Stool microbiome and metabolome differences between colorectal cancer patients and healthy adults. PloS One. (2013) 8:e70803. doi: 10.1371/journal.pone.0070803
70. Chueca E, Lanas A, Piazuelo E. Role of gastrin-peptides in barrett's and colorectal carcinogenesis. World J Gastroenterol. (2012) 18:6560–70. doi: 10.3748/wjg.v18.i45.6560
71. Singh P, Sarkar S, Kantara C, Maxwell C. Progastrin peptides increase the risk of developing colonic tumors: impact on colonic stem cells. Curr Colorectal Cancer Rep. (2012) 8:277–89. doi: 10.1007/s11888-012-0144-3
72. Ryberg B, Axelson J, Hakanson R, Sundler F, Mattsson H. Trophic effects of continuous infusion of [Leu-15]-gastrin-17 in the rat. Gastroenterology. (1990) 98:33–8. doi: 10.1016/0016-5085(90)91287-G
73. Hartwich J, Konturek SJ, Pierzchalski P, Zuchowicz M, Konturek PC, Bielański W, et al. Molecular basis of colorectal cancer - role of gastrin and cyclooxygenase-2. Med Sci Monit. (2001) 7:1171–81.
74. Fujimori S. Progress in elucidating the relationship between helicobacter pylori infection and intestinal diseases. World J Gastroenterol. (2021) 27:8040–6. doi: 10.3748/wjg.v27.i47.8040
75. Luo F, Zhou P, Ran X, Gu M, Zhou S. No evident causal association between helicobacter pylori infection and colorectal cancer: A bidirectional mendelian randomization study. Sci Rep. (2023) 13:18544. doi: 10.1038/s41598-023-45545-x
76. Selgrad M, Bornschein J, Kandulski A, Hille C, Weigt J, Roessner A, et al. Helicobacter pylori but not gastrin is associated with the development of colonic neoplasms. Int J Cancer. (2014) 135:1127–31. doi: 10.1002/ijc.28758
77. Holch JW, Demmer M, Lamersdorf C, Michl M, Schulz C, von Einem JC, et al. Pattern and dynamics of distant metastases in metastatic colorectal cancer. Visc Med. (2017) 33:70–5. doi: 10.1159/000454687
78. Zhao W, Jin L, Chen P, Li D, Gao W, Dong G. Colorectal cancer immunotherapy-recent progress and future directions. Cancer Lett. (2022) 545:215816. doi: 10.1016/j.canlet.2022.215816
79. Yoon HS, Shu XO, Cai H, Zheng W, Wu J, Wen W, et al. Associations of lung cancer risk with biomarkers of helicobacter pylori infection. Carcinogenesis. (2022) 43:538–46. doi: 10.1093/carcin/bgac047
80. Zhuo WL, Zhu B, Xiang ZL, Zhuo XL, Cai L, Chen ZT. Assessment of the relationship between helicobacter pylori and lung cancer: A meta-analysis. Arch Med Res. (2009) 40:406–10. doi: 10.1016/j.arcmed.2009.05.002
81. Behroozian R, Moradkhan E. The assessment of probable relationship between lung cancer and helicobacter pylori infection. Trop Gastroenterol. (2010) 31:34–6.
82. Gocyk W, Nikliński T, Olechnowicz H, Duda A, Bielański W, Konturek PC, et al. Helicobacter pylori, gastrin and cyclooxygenase-2 in lung cancer. Med Sci Monit. (2000) 6:1085–92.
83. Deng B, Li Y, Zhang Y, Bai L, Yang P. Helicobacter pylori infection and lung cancer: A review of an emerging hypothesis. Carcinogenesis. (2013) 34:1189–95. doi: 10.1093/carcin/bgt114
84. Anand PS, Kamath KP, Anil S. Role of dental plaque, saliva and periodontal disease in helicobacter pylori infection. World J Gastroenterol. (2014) 20:5639–53. doi: 10.3748/wjg.v20.i19.5639
85. Nakashima S, Kakugawa T, Yura H, Tomonaga M, Harada T, Hara A, et al. Identification of helicobacter pylori vaca in human lung and its effects on lung cells. Biochem Biophys Res Commun. (2015) 460:721–6. doi: 10.1016/j.bbrc.2015.03.096
86. Herndon B, Quinn T, Wasson N, Nzabi M, Molteni A. Urease and helicobacter spp. Antigens in pulmonary granuloma. J Comp Pathol. (2013) 148:266–77. doi: 10.1016/j.jcpa.2012.06.011
87. Zelenay S, van der Veen AG, Böttcher JP, Snelgrove KJ, Rogers N, Acton SE, et al. Cyclooxygenase-dependent tumor growth through evasion of immunity. Cell. (2015) 162:1257–70. doi: 10.1016/j.cell.2015.08.015
88. Huang W, Deng B, Wang RW, Tan QY, He Y, Jiang YG, et al. Bcar1 protein plays important roles in carcinogenesis and predicts poor prognosis in non-small-cell lung cancer. PloS One. (2012) 7:e36124. doi: 10.1371/journal.pone.0036124
89. Wei L, Yang Y, Zhang X, Yu Q. Anchorage-independent phosphorylation of P130(Cas) protects lung adenocarcinoma cells from anoikis. J Cell Biochem. (2002) 87:439–49. doi: 10.1002/jcb.10322
90. Rittmeyer A, Barlesi F, Waterkamp D, Park K, Ciardiello F, von Pawel J, et al. Atezolizumab versus docetaxel in patients with previously treated non-small-cell lung cancer (Oak): A phase 3, open-label, multicentre randomised controlled trial. Lancet. (2017) 389:255–65. doi: 10.1016/s0140-6736(16)32517-x
91. Herbst RS, Baas P, Kim DW, Felip E, Pérez-Gracia JL, Han JY, et al. Pembrolizumab versus docetaxel for previously treated, pd-L1-positive, advanced non-small-cell lung cancer (Keynote-010): A randomised controlled trial. Lancet. (2016) 387:1540–50. doi: 10.1016/s0140-6736(15)01281-7
92. Horn L, Spigel DR, Vokes EE, Holgado E, Ready N, Steins M, et al. Nivolumab versus docetaxel in previously treated patients with advanced non-small-cell lung cancer: two-year outcomes from two randomized, open-label, phase iii trials (Checkmate 017 and checkmate 057). J Clin Oncol. (2017) 35:3924–33. doi: 10.1200/jco.2017.74.3062
93. Sample A, He YY. Mechanisms and prevention of uv-induced melanoma. Photodermatol Photoimmunol Photomed. (2018) 34:13–24. doi: 10.1111/phpp.12329
94. Rastrelli M, Tropea S, Rossi CR, Alaibac M. Melanoma: epidemiology, risk factors, pathogenesis, diagnosis and classification. In Vivo. (2014) 28:1005–11.
95. Amedei A, Cappon A, Codolo G, Cabrelle A, Polenghi A, Benagiano M, et al. The neutrophil-activating protein of helicobacter pylori promotes th1 immune responses. J Clin Invest. (2006) 116:1092–101. doi: 10.1172/jci27177
96. Codolo G, Facchinello N, Papa N, Bertocco A, Coletta S, Benna C, et al. Macrophage-mediated melanoma reduction after hp-nap treatment in a zebrafish xenograft model. Int J Mol Sci. (2022) 23(3):1644. doi: 10.3390/ijms23031644
97. Wang T, Du M, Ji Z, Ding C, Wang C, Men Y, et al. Recombinant protein rmbp-nap restricts tumor progression by triggering antitumor immunity in mouse metastatic lung cancer. Can J Physiol Pharmacol. (2018) 96:113–9. doi: 10.1139/cjpp-2017-0186
98. Hou M, Wang X, Lu J, Guo X, Ding C, Liang T, et al. Tlr agonist rhp-nap as an adjuvant of dendritic cell-based vaccine to enhance anti-melanoma response. Iran J Immunol. (2020) 17:14–25. doi: 10.22034/iji.2020.80291
99. Garbe C, Amaral T, Peris K, Hauschild A, Arenberger P, Basset-Seguin N, et al. European consensus-based interdisciplinary guideline for melanoma. Part 2: treatment - update 2022. Eur J Cancer. (2022) 170:256–84. doi: 10.1016/j.ejca.2022.04.018
100. Knight A, Karapetyan L, Kirkwood JM. Immunotherapy in melanoma: recent advances and future directions. Cancers (Basel). (2023) 15(4):1106. doi: 10.3390/cancers15041106
101. Cheng Y, Xiao Y, Zhou R, Liao Y, Zhou J, Ma X. Prognostic significance of helicobacter pylori-infection in gastric diffuse large B-cell lymphoma. BMC Cancer. (2019) 19:842. doi: 10.1186/s12885-019-6067-5
Keywords: Helicobacter pylori, immunotherapy, gastric cancer, colorectal cancer, melanoma, non-small cell lung cancer
Citation: Zhong X, Zheng H, Zhao S, Wang Z, Su Y, Zhong K, Wang M and Shi Y (2024) Effects and mechanisms of Helicobacter pylori on cancers development and immunotherapy. Front. Immunol. 15:1469096. doi: 10.3389/fimmu.2024.1469096
Received: 23 July 2024; Accepted: 23 September 2024;
Published: 07 October 2024.
Edited by:
Massimo Fantini, Precision Biologics, Inc., United StatesReviewed by:
Elba Mónica Vermeulen, Instituto de Biología y Medicina Experimental, ArgentinaCopyright © 2024 Zhong, Zheng, Zhao, Wang, Su, Zhong, Wang and Shi. This is an open-access article distributed under the terms of the Creative Commons Attribution License (CC BY). The use, distribution or reproduction in other forums is permitted, provided the original author(s) and the copyright owner(s) are credited and that the original publication in this journal is cited, in accordance with accepted academic practice. No use, distribution or reproduction is permitted which does not comply with these terms.
*Correspondence: Yanyan Shi, MDcyOXhzdEAxNjMuY29t; Mopei Wang, d2FuZ21vcGVpQGJqbXUuZWR1LmNu; Kaili Zhong, emhvbmdrYWlsaTM0NDNAYmpzanRoLmNu
†These authors share first authorship
Disclaimer: All claims expressed in this article are solely those of the authors and do not necessarily represent those of their affiliated organizations, or those of the publisher, the editors and the reviewers. Any product that may be evaluated in this article or claim that may be made by its manufacturer is not guaranteed or endorsed by the publisher.
Research integrity at Frontiers
Learn more about the work of our research integrity team to safeguard the quality of each article we publish.