- 1Universidad de Granada, Departamento de Obstetricia y Ginecología, Granada, Spain
- 2Servicio de Microbiología, Hospital Universitario Clínico San Cecilio, Granada, Spain
- 3Universidad de Granada, Departamento de Medicina Preventiva y Salud Pública, Granada, Spain
- 4Instituto de Investigación Biosanitaria de Granada (ibs.GRANADA), Granada, Spain
- 5Centro de Investigación Biomédica en Red de Epidemiología y Salud Pública (CIBERESP), Madrid, Spain
- 6Centro de Investigación Biomédica en Red de Enfermedades Infecciosas (CIBERINFEC), Madrid, Spain
Introduction: Vaccine-induced immunity against COVID-19 generates antibody and lymphocyte responses. However, variability in antibody titers has been observed after vaccination, and the determinants of a better response should be studied. The main objective of this investigation was to analyze the inflammatory biomarker response induced in healthcare workers vaccinated with BNT162b2, and its association with anti-Spike (a SARS-CoV-2 antigen) antibodies measured throughout a 1-year follow-up.
Methods: Anti-spike antibodies and 92 biomarkers were analyzed in serum, along with socio-demographic and clinical variables collected by interview or exploration.
Results: In our study, four biomarkers (ADA, IL-17C, CCL25 and CD8α) increased their expression after the first vaccine dose; and 8 others (uPA, IL-18R1, EN-RAGE, CASP-8, MCP-2, TNFβ, CD5 and CXCL10) decreased their expression. Age, body mass index (BMI), smoking, alcohol consumption, and prevalent diseases were associated with some of these biomarkers. Furthermore, higher baseline levels of T-cell surface glycoprotein CD6 and hepatocyte growth factor (HGF) were associated with lower mean antibody titers at follow-up, while levels of monocyte chemotactic protein 2 (MCP-2) had a positive association with antibody levels. Age and BMI were positively related to baseline levels of MCP-2 (β=0.02, 95%CI 0.00-0.04, p=0.036) and HGF (β=0.03, 95%CI 0.00-0.06, p=0.039), respectively.
Conclusion: Our findings indicate that primary BNT162b2 vaccination had a positive effect on the levels of several biomarkers related to T cell function, and a negative one on some others related to cancer or inflammatory processes. In addition, a higher level of MCP-2 and lower levels of HGF and CD6 were found to be associated with higher anti-Spike antibody titer following vaccination.
1 Introduction
The coronavirus disease-2019 (COVID-19), an infectious respiratory disease caused by the SARS-CoV-2 virus, continues to place a global burden on morbidity and mortality. By spring 2024, there have been more than 770 millions COVID-19 cases and more than 7 millions deaths worldwide (1).
Several vaccines were developed throughout the year 2020 to provide protection against COVID-19. For the first time, vaccines based on mRNA were used in the general population. These vaccines, i.e. RNA-1273 (Spikevax™, Moderna) and BNT162b2 (Comirnaty®, Pfizer-BioNTech), contain mRNA encoding the Spike (S) protein of SARS-CoV-2 and in clinical trials provided around 95% protection against COVID-19 when two doses were administered (2, 3). They induce a humoral response with high levels of anti-S antibodies that decline after 3 or 6 months (4–6). Besides, the so-called cellular immunity mediated by T cells seems to play a relevant role in disease and vaccine-induced protection, what might be relevant in patients who do not have an adequate humoral response (7–10). Indeed, COVID-19 patients with poor antibody response like those receiving anti-CD20 therapy or cancer patients still induce strong CD4+ and CD8+ T cell responses (8, 9). CD4+T cells display several functions, including regulating the activity of B cells. They also play a role in activating CD8+ cytotoxic T cells, which are responsible for clearing intracellular viral infection.
Despite the effective immune response to BNT162b2 vaccination (11, 12), variability in antibody titers following vaccination has been observed by our group and other laboratories (13–17). Furthermore, some individuals do not develop a humoral response to natural SARS-CoV-2 infection (18, 19), which not necessarily imply a lack of immunity, as T-cells response could provide protection even in the absence of antibodies. Likewise, BNT162b2 vaccination induces a robust response of CD8+ and CD4+T cells that can vary among individuals (10). However, little is known about the immune mediators that are associated with a poorer response to the vaccine.
Understanding the factors that influence vaccine immune response may have important implications for enhancing the immunogenicity of SARS-CoV-2 vaccines. To achieve this goal, we analyzed the effect of BNT16b2 vaccination and sociodemographic and clinical variables on the levels of 92 inflammatory biomarkers in healthcare workers and the association of these biomarkers with anti-S antibodies.
2 Materials and methods
2.1 Study design and participants
An ambispective internal comparison cohort study was carried out. The study was conducted at Hospital Universitario Clínico San Cecilio (HUCSC), in the city of Granada (southern Spain) with a workforce of over 3000 employees. This research is part of a larger project whose main objective was to analyse the neutralizing capacity of antibodies after vaccination with different variants (13). The reference population consisted of healthcare and social-healthcare staff aged less than 65 years vaccinated with BNT162b2 (Cominarty®), as this was the only vaccine dispensed at the hospital for the first dose. After receiving the approval from the Ethic Committee in January 2021, participants were recruited in order of arrival to the vaccination event with no selection criteria restriction, and followed up for a year. A total of 147 participants were finally enrolled and all of them signed the informed consent to participate in the study. In a second part of this project and coinciding with the third vaccine dose administration in December 2021, participants were invited to continue the follow-up for an additional year. Besides, a questionnaire was administered at this timepoint, and permission was requested to analyse biomarkers in the blood samples previously donated. A total of 108 individuals provided their consent to participate in this second part.
2.2 Study samples and data collection
Serum samples were collected at the following times: just before/after the administration of the first dose of the vaccine (t0) and the second dose (t1). The time interval for sample collection was between few minutes before vaccination until a few hours later, being logistics the only reason for not being able to sample before. No record was taken on which participants sampled before or after the vaccination. Samples were also obtained 5 weeks after vaccination (t2), 3 months (t3), 8-9 months (t4) and 11 months (t5) after the first dose administration, the latter coinciding approximately with the administration of the third dose of the vaccine.
Sociodemographic (age, sex, educational level), lifestyle (smoking habit, alcohol consumption, Mediterranean diet adherence), and clinical information (chronic diseases) concerning the time of first vaccine dose administration was collected retrospectively using a self-administered questionnaire at t5 (Supplementary Data Sheet). Anthropometric data (body mass index and waist-hip ratio) was determined at the same visit by a dietician-nutritionist, and participants were asked whether they had changed weight since the first dose.
2.3 Determination of antibodies
Serum IgG antibodies were analyzed in the samples taken at the time points t0, t1, t2, t3, t4 and t5 using COVID-19 VIRCLIA IgG MONOTEST (VIRCELL, S.L., Spain) following manufacturer’s instructions. The assay is a qualitative indirect chemiluminescent immunoassay (CLIA) to test IgG antibodies in SARS-CoV-2 spike (S) and nucleocapsid (N), with a manufacturer declared 98% sensitivity and 99% specificity.
2.4 Determination of biomarkers
The analysis of 92 inflammatory biomarkers was performed on samples taken at the time points t0, t1 and t2. The list with full names and abbreviations of these markers is detailed in Supplementary Table 1. The Proximity Extension Assay technology, manufactured by Olink (Stockholm, Sweden) and performed at Cobiomic Bioscience, (Córdoba, Spain), was used. Briefly, this multiplex technique allows protein assays to be performed in a minimal clinical sample volume, using two matching antibodies labelled with unique DNA barcodes, for each target antigen. The protein in the solution that binds to both antibodies with sufficient stability is subsequently quantified by real-time PCR, providing higher sensitivity than classical immunoassays. The results obtained represent a relative value of protein expression compared to the buffer used for the PCR reaction. For the biomarkers IL-1α, IL-2, IL-4, IL-5, IL-13, IL-24, IL-33, IL-22RA1, beta-NGF and TSLP more than 75% of the samples had a value below the limit of detection and therefore were excluded from further analyses.
2.5 Statistical analysis
The Skewness-Kurtosis test was used to determinate the normal distribution of biomarker levels. Since most of the biomarkers did not exhibit parametric behaviour, the Friedman test was used to compare the levels of the biomarkers among the three timepoints after vaccination (t0, t1 and t2). Considering the large number of biomarkers analysed, a p-value correction for multiple comparisons was performed by means of the Benjamini-Hochberg method and q-values were estimated. As this correction may increase the probability of false type II errors, we also explored the borderline non-significant (q<0.1) results. In addition, a comparison between pairs of groups, namely t1 versus t0, and t2 versus t0, was performed using the Wilcoxon test. Then generalized estimating equations (GEE) were performed to test the association between baseline socio-demographic, lifestyle, anthropometric, and clinical variables (independent variables), and the change of inflammatory biomarker levels over time after vaccination (dependent variable). Of note, for age, BMI and hip-waist ratio, the change in cytokine expression refers to 1-unit increase.
To study the association between baseline biomarkers and antibody levels, the dimensionality of the biomarkers was reduced using a principal component analysis (PCA). It was not possible to perform a single PCA due to the small sample size and a large number of biomarkers. Therefore, it was decided to subdivide the biomarkers into groups according to their biological function (https://insight.olink.com/pathway-browser). The 4 biological pathways mostly covered by the biomarkers in the study were: 1) immune system (51 biomarkers); 2) signal transduction (42 biomarkers); 3) disease (14 biomarkers); and 4) protein metabolism and expression (12 biomarkers) (Supplementary Table 2). In addition, a fifth group was created under the name “Others’’ which included the 9 biomarkers not present in any of the above pathways. Biomarker repetition was allowed in the first 4 clusters. A PCA with no matrix rotation was performed for each of these 5 groups and the Kaiser–Meyer–Olkin (KMO) test, aimed to determine how suited the data are for PCA, was performed. Then, GEE were performed using the two principal components (PC1 and PC2) that contributed the most in the 5 PCAs done as the independent variables, and the repeated measures (t1, t2, t3, t4 and t5) of antibodies, as the dependent variable. For t0, t1 and t2, the samples were collected at practically the same time for all individuals, but for the rest of the timepoints there was variability, and the group mean was assigned to each participant (Supplementary Table 3). The analysis was adjusted for sex and age, as these two variables are well known to modify the immune system (20–22). In addition, the 5 individual biomarkers that contributed most to the PCs with a significant association with antibody levels were selected to evaluate their individual association with antibodies by means of GEEs. Furthermore, to evaluate the association of baseline socio-demographic, lifestyle, anthropometric, and clinical variables (independent variables), and selected baseline inflammatory biomarker levels (dependent variable), linear regression models were estimated.
Statistical significance was stated as p-value or q-value<0.05. Statistical analyses were performed using SPSS (version 28.0.1) and Stata SE 17.0 (Stata Corp, College Station, Texas) statistical softwares and graphs were constructed using GraphPad Prism 9.
3 Results
3.1 Description of the study cohort
The sample consisted of a total of 99 healthcare workers from the HUCSC with available data of both anti-S antibodies and inflammatory biomarkers. The characteristics of this cohort are provided in Table 1. Most part of the participants were female (78.8%) and the mean age was 47.1 years (standard deviation, SD: 11.9). 22.2% of the cohort had some chronic diseases. With regard to lifestyle, the majority were non-smoker (62.1%), and 49,4% did not drink alcohol. The adherence to the Mediterranean diet was high (mean=8.9, SD:1,7). Only 5 participants with available anti-S IgG data reported a SARS-CoV-2 positive diagnostic test between 3 and 10 months previous to vaccination. Antibody titers were similar to those with no previous infection (data not shown).
3.2 Effect of vaccination on biomarker levels
Of the 82 biomarkers finally included in the analysis, the expression of 41 was modified after the vaccination process showing a p-value<0.05 (Figure 1; Supplementary Table 4). However, after adjustment for multiple comparisons, only 11 biomarkers retained the statistical significance: CD8α, UPA, IL-17C, IL-18R1, CD5, CXCL10, EN-RAGE, CASP-8, CCL25, ADA and TNFβ. In addition, the biomarker MCP-2 had a q-value very close to 0.05 and was also considered for further analyses (Figure 1; Supplementary Table 4).
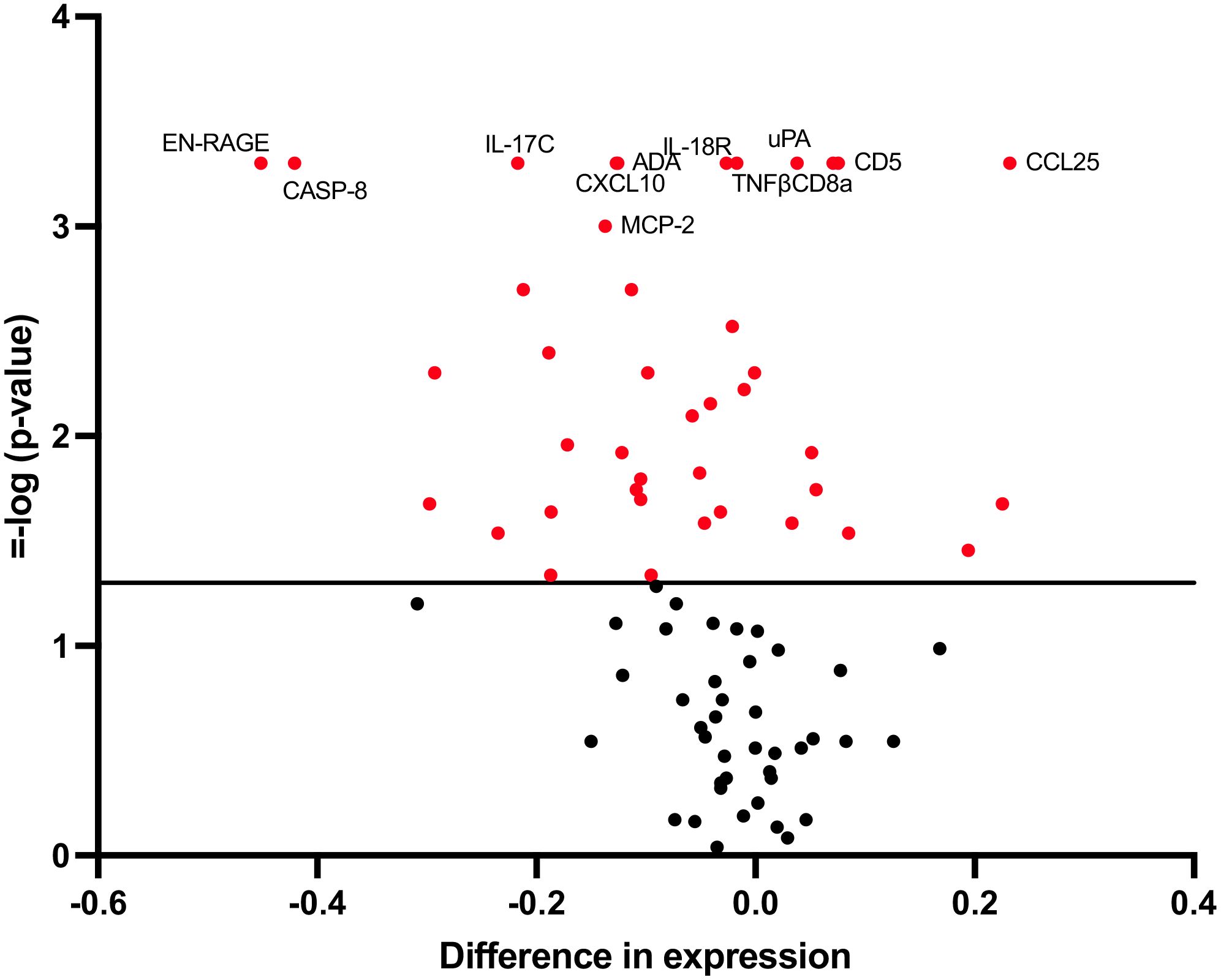
Figure 1. Changes in biomarker levels 5 weeks after vaccine administration. Volcano plots showing changes in biomarker levels comparing baseline versus 5 weeks after vaccination. Each circle represents one biomarker. The difference in expression is represented on the x-axis. The y-axis shows the log10 of the p value of the Friedman test, comparing baseline, 2-week and 5-week timepoints. A p value<0.05 is indicated by a red dot.
The distribution of values for these 12 markers in each timepoint sample as well as the differences between groups are shown in Figure 2. A statistically significant increase was observed 3 weeks after the administration of the first dose of the vaccine (t1, coinciding with the administration of the second dose) in the biomarkers ADA, CCL25, CD8α and IL-17C. This significant increase was maintained 5 weeks later (t2) for CCL25, while a decrease was observed for ADA. On the other hand, a statistically significant decrease was observed 3 weeks after the administration of the first dose in the biomarkers MCP-2, CASP-8, TNFβ, uPA, IL-18R1, EN-RAGE, CD5 and CXCL10. This significant decrease was maintained over the following 5 weeks for MCP-2, CASP-8 and EN-RAGE.
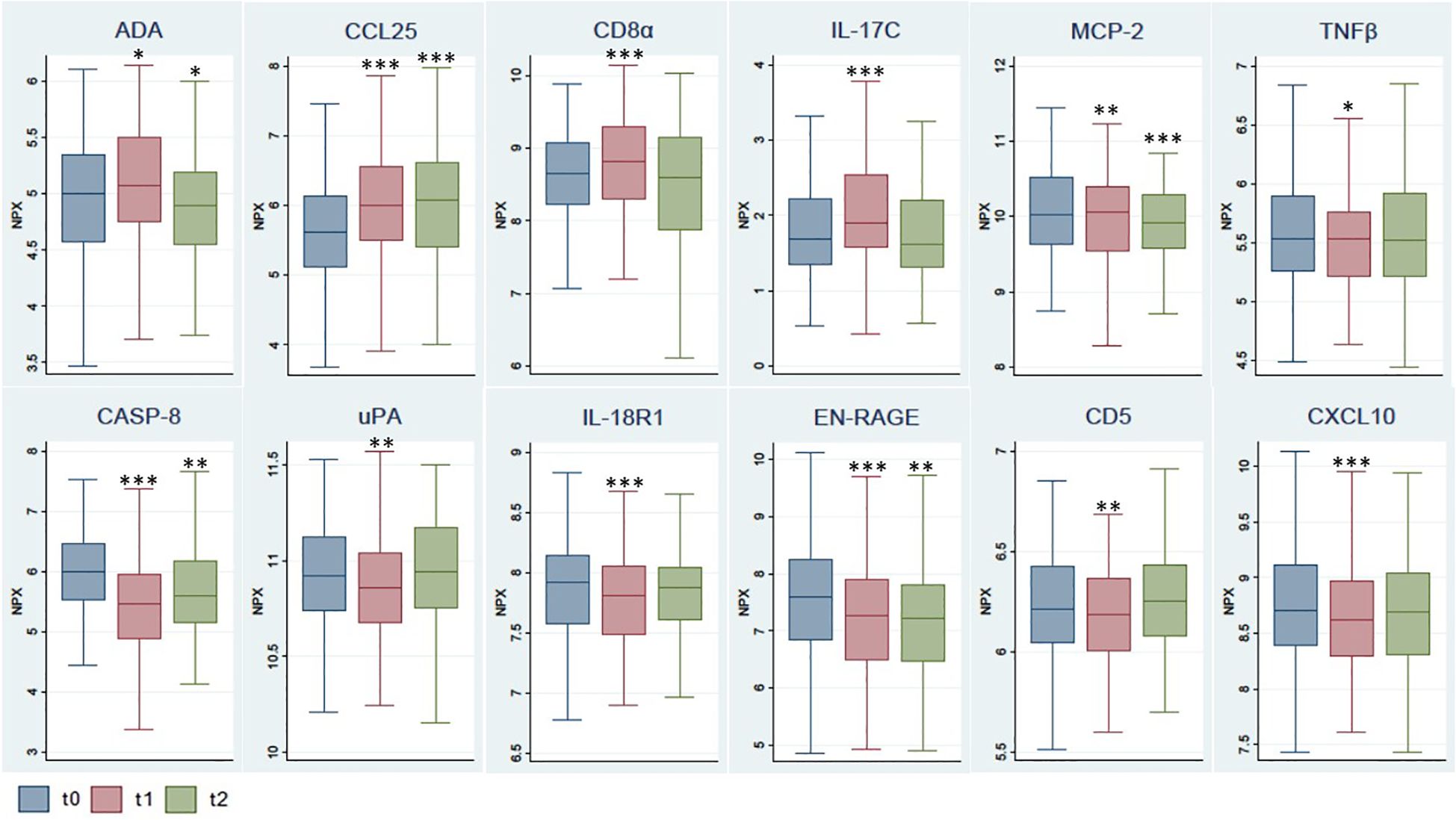
Figure 2. Comparison of selected biomarker levels at different timepoints after vaccination. The box shows the median, and 25th and 75th percentiles. Outliers have not been plotted in the graph. *p<0.05, **p<0.01, ***p<0.001 for Wilcoxon test, with reference t0. NPX, Normalized Protein eXpression.
3.3 Effect of socio-demographic and clinical variables on biomarkers affected by the vaccination
Next, we assessed which baseline socio-demographic and clinical variables may be associated with the mean levels over time of the 12 biomarkers modified after the vaccination process. Older age was significantly associated with a higher mean concentration of CCL25. On the other hand, smoking habit, consumption of high level of alcohol and the presence of any illness were related to a lower mean concentration of CCL25, CD8α and IL-17c, respectively (Table 2).
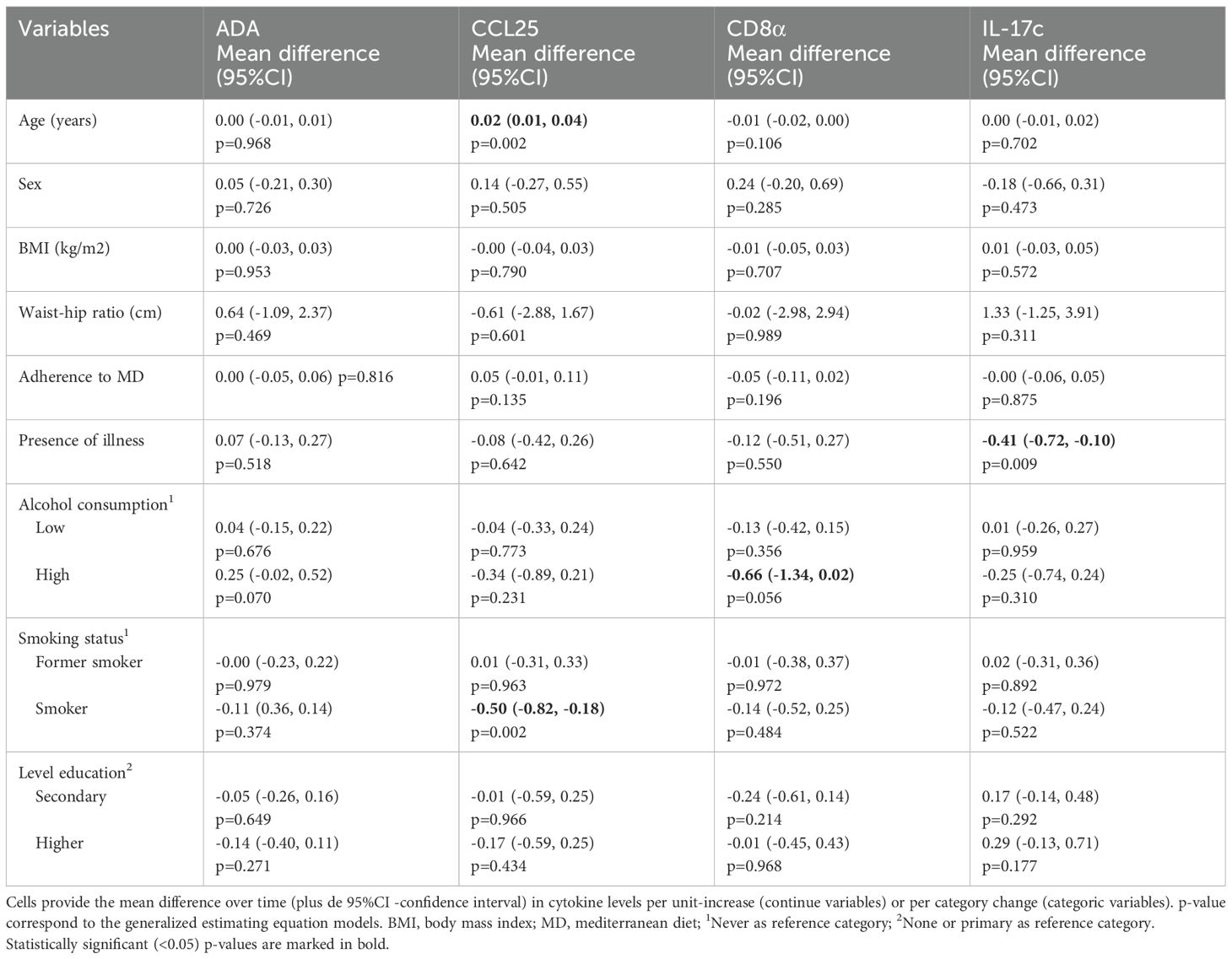
Table 2. Association of socio-demographic and clinical variables with biomarkers with increased levels after 3 weeks of vaccination.
With regards to the biomarkers with decreased changes over time after 3 weeks of vaccination, age was related with increased mean of MCP-2 and CXCL10, and with a decreased mean of IL-18Rl. Higher BMI and waist-hip ratio were associated with IL-18Rl and CD5, respectively. High alcohol consumption was related with a mean increase in MCP-2. Besides, CASP-8 and EN-RANGE had a positive association with the smoking habit and the level of education (Table 3).
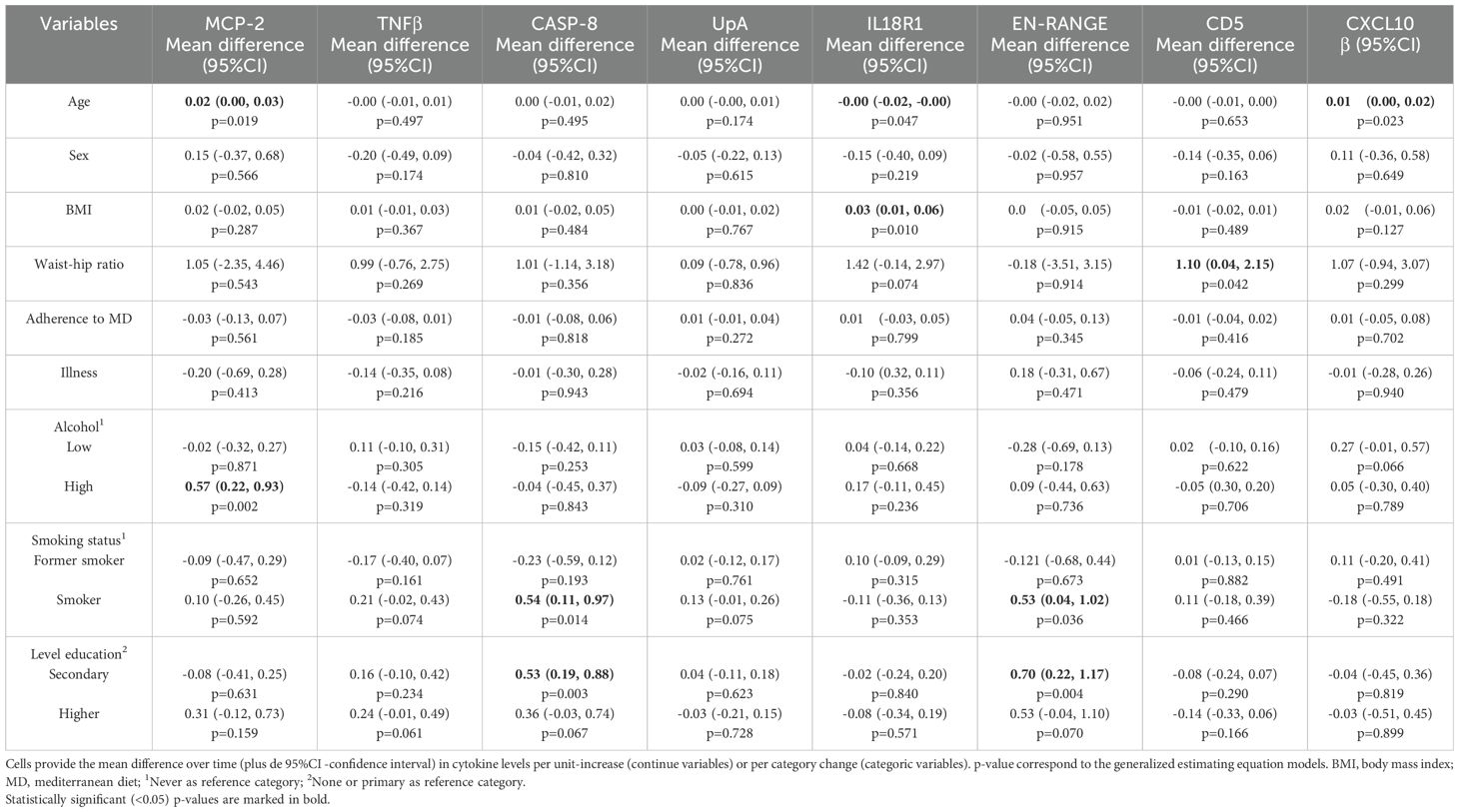
Table 3. Association of socio-demographic and clinical variables with decreased biomarkers levels after vaccination.
3.4 Association of baseline biomarker levels with post-vaccination antibody levels
A PCA was performed for all 5 functional biomarker groups and KMO values above 0.6 were obtained in all cases (Supplementary Tables 5–9). An association of mean antibody levels over follow-up was observed with PC2-Others and PC1-Signal Transduction (Table 4).
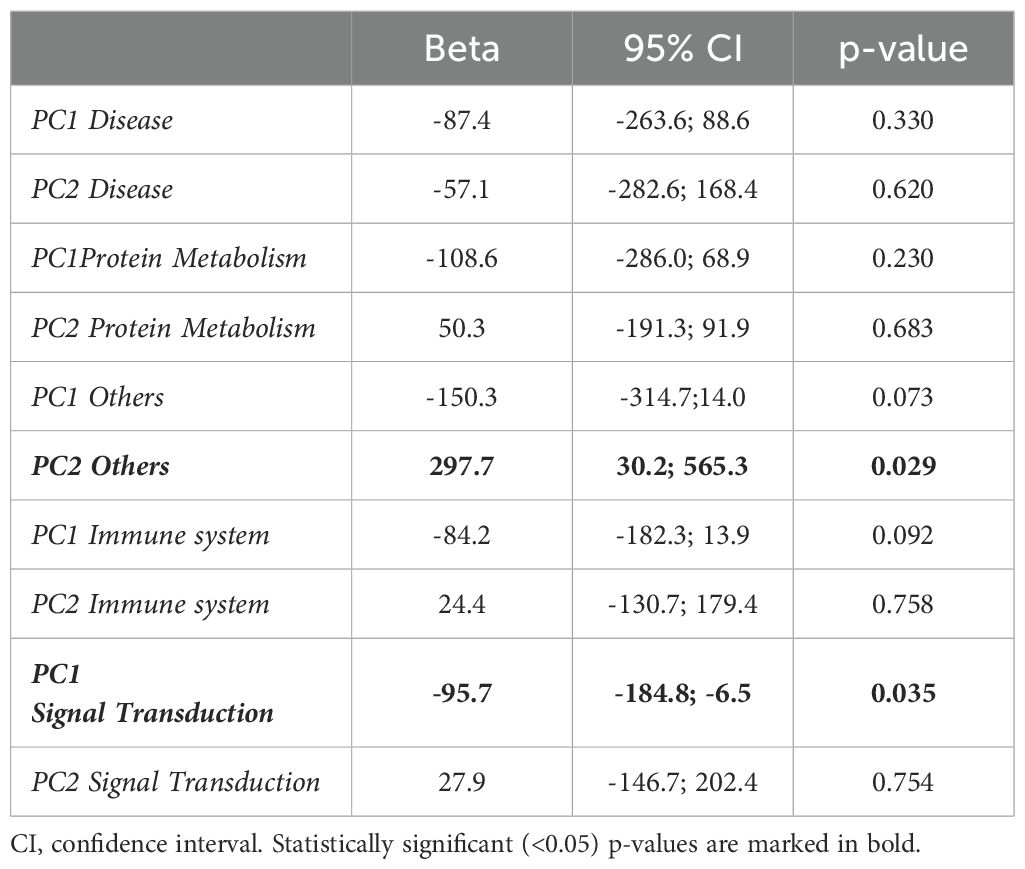
Table 4. Association of PC1 and PC2 of different functional biomarker groups with the mean antibody levels at follow-up.
The biomarkers that contributed most to these PCs (Figure 3; Supplementary Tables 5, 6) were: CD5, CD6, SIRT2, MCP-2 and FGF-21 (PC2 Others); HGF, CXCL1, CXCL11, MCP-1 and MCP-4 (PC1 Signal Transduction). In the analysis of their individual associations with antibody levels, 2 of the biomarkers (CD6 and HGF) showed a significant negative association, and the biomarker MCP-2 obtained a positive association very close to significance (Table 5).
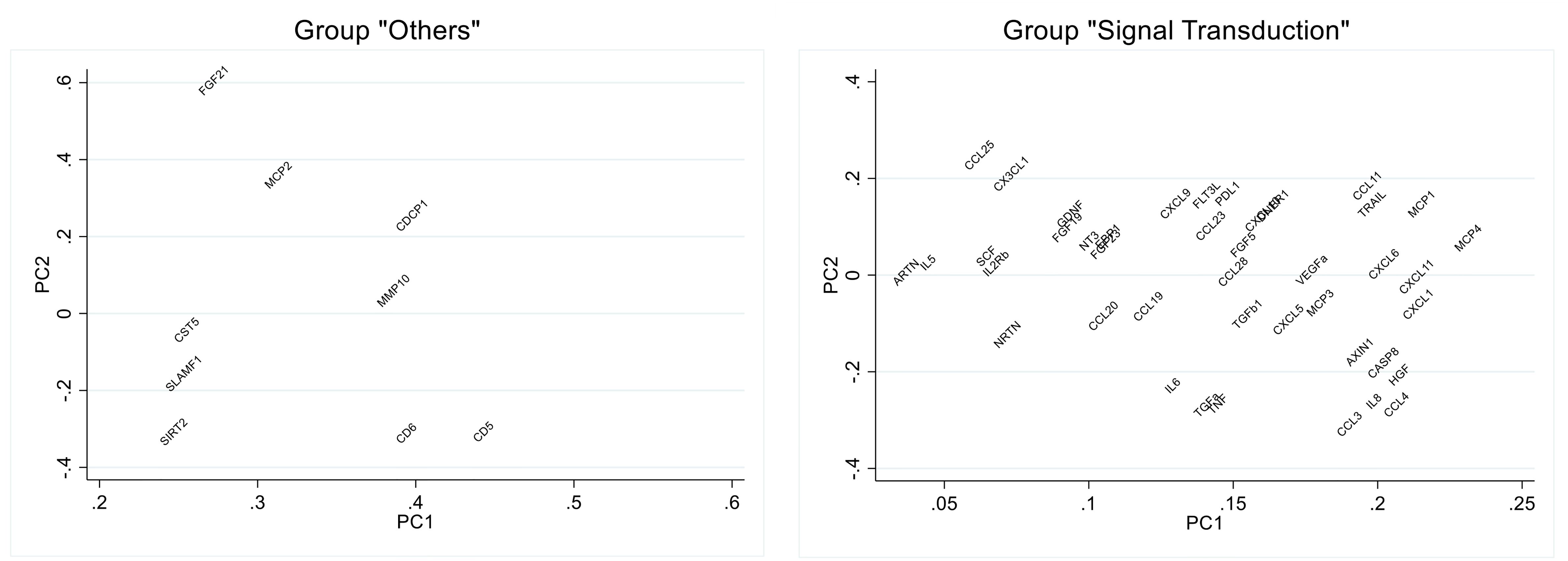
Figure 3. Loading plot of the principal component analysis of selected biomarker groups. Eigen vector values for the principal component (PC) 1 and PC2 are shown in the graph.
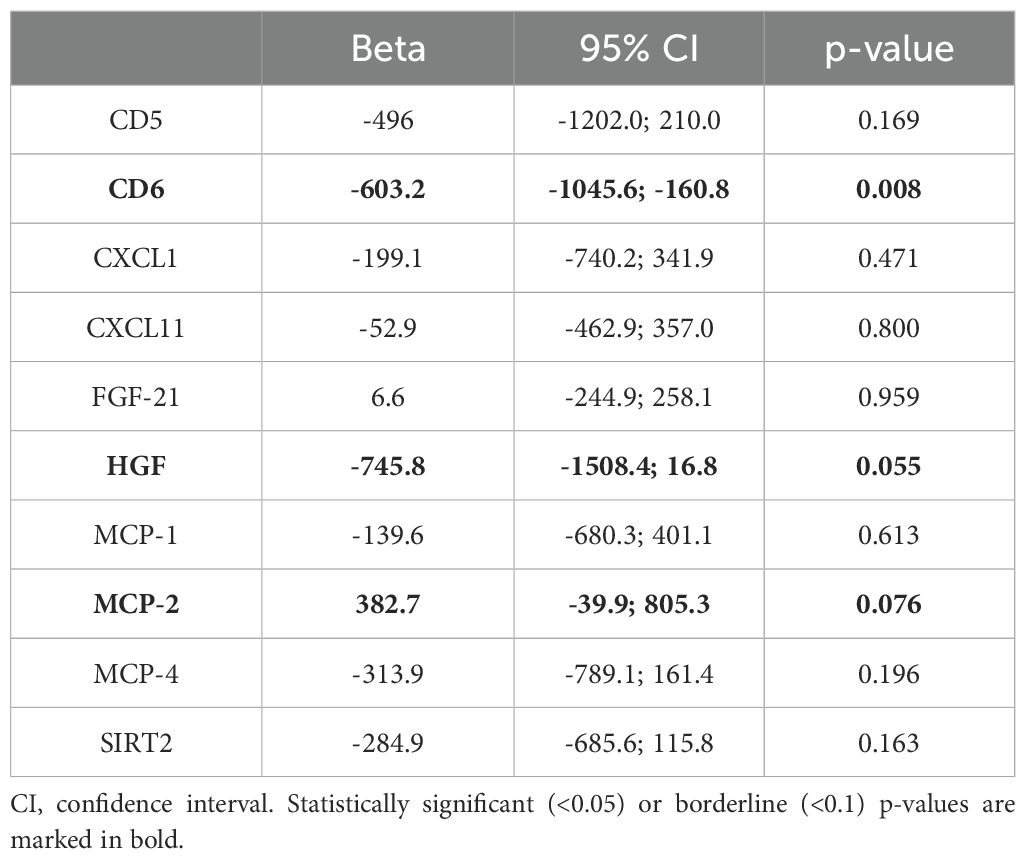
Table 5. Association between baseline individual biomarkers and post-vaccination antibody levels at follow up.
In order to interpret the results more easily, we created a graph for each of these 3 biomarkers categorized by tertiles (Figure 4).
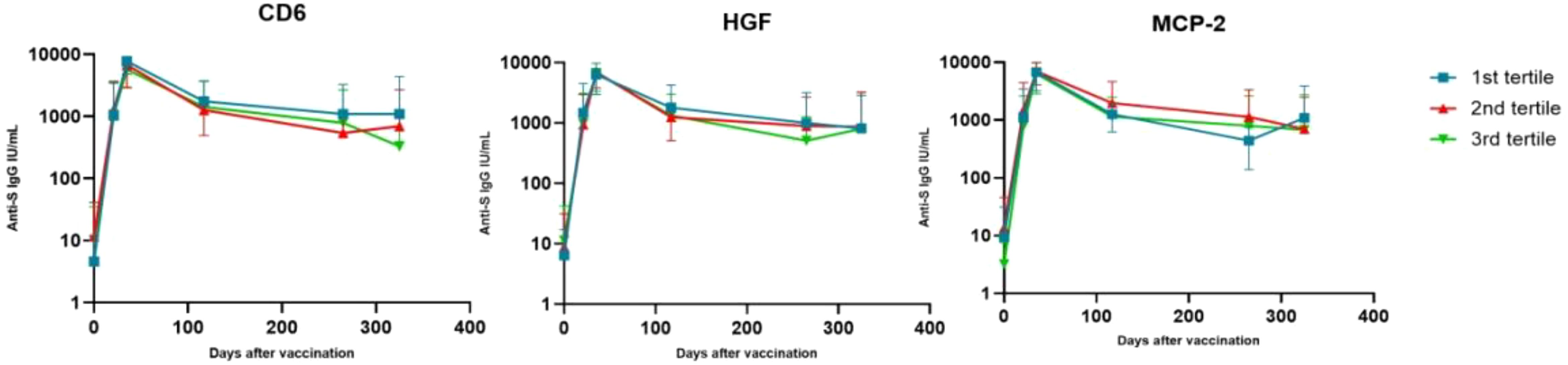
Figure 4. Changes in antibody levels after vaccine administration, stratifying individuals according to their levels of certain biomarkers. Values represent mean and standard deviation. On the x-axis represents the days after vaccination and on the y-axis the mean antibody concentration on a logarithmic scale in base 10.
The highest antibody levels were similar for all individuals (at t2), regardless of their HGF, CD6 or MCP-2 levels. But the drop in antibodies at t4 was more pronounced for those individuals with higher levels (3rd tertile) of CD6 and HGF, and those individuals with lower levels (1st tertile) of MCP-2. This drop continued for CD6 at t5.
3.5 Effect of socio-demographic and clinical variables on baseline biomarkers related to the antibody responses after vaccination
Next, we assessed which socio-demographic and clinical variables may be associated with the levels of those biomarkers related to the antibody response, i.e. CD6, HGF and MCP-2. BMI was positively associated with HGF (β=0.03, 95% CI 0.00, 0.06, p=0.039) and age with MCP-2 (β=0.02, 95% CI 0.00, 0.04, p=0.036, respectively).
4 Discussion
The results show that our study cohort, which was followed up for approximately 1 year, presented changes in 12 inflammatory biomarkers measured after the BNT162b2 vaccination process; 4 of them showed increased expression (ADA, CD8α, IL-17C and CCL25), and 8 others (uPA, IL-18R1, EN-RAGE, CASP-8, MCP-2, TNFβ, CD5 and CXCL10) decreased.
All biomarkers whose expression increased after vaccination are related to T-lymphocyte function. Thus, ADA (adenosine deaminase) is essential for lymphocyte production and its deficiency is a cause of severe combined immunodeficiency (23). Consistent with our findings, upregulation of ADA has been detected after BNT16b2 vaccination (24, 25), as well as after SARS-CoV-2 infection (24). CD8 is a cell surface receptor on cytotoxic T cells, necessary for the recognition of other cells, and CD8+ T cells induced by vaccination may contribute to humoral and long-term immunity (26–29). IL-17C is a member of the IL-17 family that is selectively induced in the epithelium and stimulates Th17 lymphocyte activity (30). Finally, CCL25 is specifically expressed in the thymus and intestinal epithelium, and is involved in T-lymphocyte development (31, 32). Considering its localization in the mucosa, CCL25 may play an important role in attracting T cell and IgA antibody secreting cells to the mucosa (33, 34), and it has been proposed as a viable candidate for mucosal adjuvant in vaccines to boost systemic and mucosal immunity (35). Altogether, these observations highlight the relevance of the T-lymphocyte-mediated cellular response in BNT162b2 vaccine-induced immunity. Of note, unhealthy lifestyles and the presence of a prevalent disease were negatively associated with the expression of some of these biomarkers, suggesting that these conditions may also have a negative impact in the cellular immune response induced by the vaccination.
Some biomarkers whose expression was inhibited after vaccination are related to cancer. Thus, uPA is an urokinase involved in the processes of cancer invasion and metastasis that participates in the degradation of the extracellular matrix and maintains the cohesion of normal cells in tissues (36). Moreover, it modulates inflammation through release pro-inflammatory cytokines. The levels of uPA and its receptor uPAR in the lung have been related to severe outcomes of COVID-19 like the acute respiratory distress syndrome (37–39), lung injury and risk of mortality (40, 41). In addition, CASP-8 plays a key role in regulating cell apoptosis. In consonance with our results showing decreased expression of these biomarkers after vaccination, it has been proposed that one of the mechanisms by which the COVID-19 mRNA vaccine induces lung protection is through the inhibition of apoptosis of epithelial and endothelial cells (42). Another anti-apoptotic effect suggested is mediated PPAR agonists and the upregulation of anti-apoptotic factors such as MCL-1 (43). Other biomarkers inhibited in the weeks following administration of BNT162b2 are related to inflammatory processes, e.g. EN-RAGE, IL-18R1 and CXCL10 (44, 45). Of note, high levels of these biomarkers have been associated with risk of SARS-CoV-2 infection or COVID-19 severity (24, 39, 46–54), what suggests, in line with our results, that these biomarkers hamper the development of either natural or artificial immunity against COVID-19. And in agreement with our results, some studies have shown that CXCL10 levels decrease after COVID-19 vaccination (24, 51, 53, 54). As expected, the expression of some of these pro-inflammatory and pro-cancer markers were positively associated with unhealthy lifestyles or conditions (smoking habit, alcohol consumption or higher BMI).
With respect to biomarkers and their association with the post-vaccination humoral response, higher baseline levels of MCP-2, and, HGF and CD6 were associated with higher and lower mean antibody titers respectively at follow-up. MCP-2, also known as CCL8, is a chemokine produced by a wide variety of cells in response to other cytokines such as IL-1, IFN-gamma, etc. This chemokine, at high concentrations, behaves as a potent activator of eosinophils and basophils (55) and its main function is to attract monocytes, lymphocytes, and other types of inflammatory cells to the site of inflammation or injury. Other functions include cell proliferation and angiogenesis, as well as modulation of the inflammatory response by interacting with macrophages and dendritic cells. Thus, individuals with higher levels of MCP-2 may induce a greater influx of monocytes and lymphocytes to the site of vaccination, ultimately inducing a more durable antibody response. Indeed, a chemokine signature including higher plasma level of MCP-2 has previously been shown in COVID-19 patients compared to healthy controls (52, 56–58). In addition, our analysis showed that MCP-2 was positively associated with age. This would suggest that age may be associated with a stronger antibody response mediated by MCP-2. However, some of our unpublished data and others have shown precisely the opposite, that age is inversely associated with the antibody response after vaccination (59, 60). This controversy may be explained by other factors related to age, like thymic involution or comorbidities that may contribute more strongly to a poor antibody response than MCP-2 production.
HGF, also known as hepatocyte growth factor, is a signaling protein that plays an important role in tissue development regeneration and immune response. In addition, HGF has been found to promote cell survival, tissue protection and regeneration, but restricts fibrosis and inflammation (61). By inhibiting inflammation, HGF may have the opposite effect to MCP-2, not allowing the correct arrival of leukocytes at the site of vaccine administration, which would explain why individuals with higher levels of HGF would eventually experience a drop in antibody levels.
Finally, CD6 is a type I transmembrane glycoprotein, expressed almost entirely by lymphocytes. Its functions are still a matter of study, but some of them include co-stimulation of T cells, thereby enhancing a more effective immune response, or cell adhesion, facilitating interaction between immune cells (62). Given this biological function, it does not seem logical that individuals with the highest CD6 levels would have a worse antibody response at follow-up. However, it should be noted that the physiological effect described above occurs with the membrane marker but in our study, we measured the soluble (serum) biomarker. Further studies are needed to confirm the role of CD6 in the antibody response after vaccination.
This study investigated a large number of biological biomarkers in BNT162b2-vaccinated healthcare workers. The immune response induced by mRNA vaccination has been studied by several researchers (4, 63–65). Cellular and humoral immune memory responses, including T cell activity, have been detected in vaccinated individual (4). Kramer et al., identified a specific population of CD4+ CD8+ ICOS+ CD38+ CXCR5- in response to BNT162b2 vaccine (63). Arunachalam et al., showed a signature of innate antiviral immunity with a higher level of plasma INFγ and CD14+CD16+ inflammatory monocytes (65). Moreover, another study have identified a platelet humoral response with change in proinflammatory cytokines (IL-1β, IFNγ, TNF-α between others) and anti-inflammatory cytokines (IL-10) (64).
Our study has some limitations. Firstly, the study sample is relatively small. In addition, this study was conducted in a single health center and all participants were health professionals, which may limit the representativeness of the population. Finally, there was some variability in the sample timing collection. For t0 and t1, samples were collected the same day but before or after the vaccination, while samples corresponding to t4 and t5 samples were obtained at different days after vaccination. This could potentially lead to a bias towards the null in the analysis. Yet, we did observe some interesting significant results which make our study relevant despite this limitation.
Among the strengths of our study, it is found the follow-up of participants, with six antibody measurements over a one-year period, which allowed us to characterize the post-vaccination immune response properly. Moreover, the large number of biomarkers studied allowed us to examine the expression of molecules that are not usually included in this type of study, and which provide very novel information.
5 Conclusions
In summary, the present study revealed that after primary BNT162b2 vaccination the biomarkers ADA, IL-17C, CCL25 and CD8α, which are related to T-cell function, were found to have increased expression. In contrast, the biomarkers uPA, IL-18R1, EN-RAGE, CASP-8, MCP-2, TNFβ, CD5 and CXCL10, whose functions are related to cancer or inflammatory processes, had their expression inhibited. In addition, higher levels of MCP-2 were associated with higher mean anti-S antibody titers after vaccination, whereas HGF and CD6 levels showed a negative association with mean antibody titers.
Data availability statement
The raw data supporting the conclusions of this article will be made available by the authors, without undue reservation.
Ethics statement
The studies involving humans were approved by the Andalusian Research Ethics Committee (opinions 0670-N-21 for initial recruitment and 2406-N-21 for biomarker measurement). The studies were conducted in accordance with the local legislation and institutional requirements. The participants provided their written informed consent to participate in this study.
Author contributions
EL-D: Supervision, Formal analysis, Writing – original draft. ES-C: Investigation, Methodology, Writing – review & editing. AS-R: Investigation, Methodology, Writing – review & editing. IS-B: Investigation, Methodology, Writing – review & editing. RB-R: Investigation, Methodology, Writing – review & editing. AF: Investigation, Methodology, Writing – review & editing. LV: Investigation, Methodology, Writing – review & editing. FG: Writing – review & editing, Conceptualization, Supervision, Visualization, Funding acquisition. PR: Conceptualization, Supervision, Visualization, Writing – review & editing, Data curation, Funding acquisition, Validation.
Funding
The author(s) declare financial support was received for the research, authorship, and/or publication of this article. This work was supported by the Consejería de Salud, Junta de Andalucía (Spain) under Grant PI-0198-2021.
Acknowledgments
The authors thank all the healthcare professionals in the HUSSC for their help with sample collection, as well as all the participants in the study.
Conflict of interest
The authors declare that the research was conducted in the absence of any commercial or financial relationships that could be construed as a potential conflict of interest.
Publisher’s note
All claims expressed in this article are solely those of the authors and do not necessarily represent those of their affiliated organizations, or those of the publisher, the editors and the reviewers. Any product that may be evaluated in this article, or claim that may be made by its manufacturer, is not guaranteed or endorsed by the publisher.
Supplementary material
The Supplementary Material for this article can be found online at: https://www.frontiersin.org/articles/10.3389/fimmu.2024.1447317/full#supplementary-material
References
1. World Health Organization. WHO COVID-19 dashboard . Available online at: https://data.who.int/dashboards/covid19/cases (Accessed 20 July 2024).
2. Baden LR, El Sahly HM, Essink B, Kotloff K, Frey S, Novak R, et al. Efficacy and safety of the mRNA-1273 SARS-coV-2 vaccine. N Engl J Med. (2021) 384:403–16. doi: 10.1056/NEJMoa2035389
3. Polack FP, Thomas SJ, Kitchin N, Absalon J, Gurtman A, Lockhart S, et al. Safety and efficacy of the BNT162b2 mRNA covid-19 vaccine. N Engl J Med. (2020) 383:2603–15. doi: 10.1056/NEJMoa2034577
4. Goel RR, Painter MM, Apostolidis SA, Mathew D, Meng W, Rosenfeld AM, et al. mRNA vaccines induce durable immune memory to SARS-CoV-2 and variants of concern. Sci (1979). (2021) 374(6572):abm0829. doi: 10.1126/science.abm0829
5. Walsh EE, Frenck RW, Falsey AR, Kitchin N, Absalon J, Gurtman A, et al. Safety and immunogenicity of two RNA-based covid-19 vaccine candidates. N Engl J Med. (2020) 383:2439–50. doi: 10.1056/NEJMoa2027906
6. Favresse J, Bayart JL, Mullier F, Elsen M, Eucher C, Van Eeckhoudt S, et al. Antibody titres decline 3-month post-vaccination with BNT162b2. Emerg Microbes Infect. (2021) 10:1495–8. doi: 10.1080/22221751.2021.1953403
7. Apostolidis SA, Kakara M, Painter MM, Goel RR, Mathew D, Lenzi K, et al. Cellular and humoral immune responses following SARS-CoV-2 mRNA vaccination in patients with multiple sclerosis on anti-CD20 therapy. Nat Med. (2021) 27:1990–2001. doi: 10.1038/s41591-021-01507-2
8. Bange EM, Han NA, Wileyto P, Kim JY, Gouma S, Robinson J, et al. CD8+ T cells contribute to survival in patients with COVID-19 and hematologic cancer. Nat Med. (2021) 27:1280–9. doi: 10.1038/s41591-021-01386-7
9. Oberhardt V, Luxenburger H, Kemming J, Schulien I, Ciminski K, Giese S, et al. Rapid and stable mobilization of CD8+ T cells by SARS-CoV-2 mRNA vaccine. Nature. (2021) 597:268–73. doi: 10.1038/s41586-021-03841-4
10. Sahin U, Muik A, Vogler I, Derhovanessian E, Kranz LM, Vormehr M, et al. BNT162b2 vaccine induces neutralizing antibodies and poly-specific T cells in humans. Nature. (2021) 595:572–7. doi: 10.1038/s41586-021-03653-6
11. Pérez-Then E, Lucas C, Monteiro VS, Miric M, Brache V, Cochon L, et al. Neutralizing antibodies against the SARS-CoV-2 Delta and Omicron variants following heterologous CoronaVac plus BNT162b2 booster vaccination. Nat Med. (2022) 28:481–5. doi: 10.1038/s41591-022-01705-6
12. Li C, Lee A, Grigoryan L, Arunachalam PS, Scott MKD, Trisal M, et al. Mechanisms of innate and adaptive immunity to the Pfizer-BioNTech BNT162b2 vaccine. Nat Immunol. (2022) 23:543–55. doi: 10.1038/s41590-022-01163-9
13. Serrano-Conde E, Leyva A, Fuentes A, de Salazar A, Chueca N, Pérez-Castro S, et al. In vitro neutralizing activity of BNT162b2 mRNA-induced antibodies against full B.1.351 SARS-CoV-2 variant. Transbound Emerg Dis. (2022) 69:2649–55. doi: 10.1111/tbed.14417
14. Perkmann T, Mucher P, Ösze D, Müller A, Perkmann-Nagele N, Koller T, et al. Comparison of five Anti-SARS-CoV-2 antibody assays across three doses of BNT162b2 reveals insufficient standardization of SARS-CoV-2 serology. J Clin Virol. (2023) 158:105345. doi: 10.1016/j.jcv.2022.105345
15. Shrotri M, Fragaszy E, Nguyen V, Navaratnam AMD, Geismar C, Beale S, et al. Spike-antibody responses to COVID-19 vaccination by demographic and clinical factors in a prospective community cohort study. Nat Commun. (2022) 13:5780. doi: 10.1038/s41467-022-33550-z
16. Barbeau DJ, Martin JM, Carney E, Dougherty E, Doyle JD, Dermody TS, et al. Comparative analysis of human immune responses following SARS-CoV-2 vaccination with BNT162b2, mRNA-1273, or Ad26.COV2.S. NPJ Vaccines. (2022) 7:77. doi: 10.1038/s41541-022-00504-x
17. Tai CG, Haviland MJ, Kissler SM, Lucia RM, Merson M, Maragakis LL, et al. Low antibody levels associated with significantly increased rate of SARS-CoV-2 infection in a highly vaccinated population from the US National Basketball Association. J Med Virol. (2024) 96(3):e29505. doi: 10.1002/jmv.29505
18. Petersen LR, Sami S, Vuong N, Pathela P, Weiss D, Morgenthau BM, et al. Lack of antibodies to severe acute respiratory syndrome coronavirus 2 (SARS-coV-2) in a large cohort of previously infected persons. Clin Infect Dis. (2021) 73:e3066–73. doi: 10.1093/cid/ciaa1685
19. Dobaño C, Ramírez-Morros A, Alonso S, Vidal-Alaball J, Ruiz-Olalla G, Vidal M, et al. Persistence and baseline determinants of seropositivity and reinfection rates in health care workers up to 12.5 months after COVID-19. BMC Med. (2021) 19:155. doi: 10.1186/s12916-021-02032-2
20. Shaw AC, Goldstein DR, Montgomery RR. Age-dependent dysregulation of innate immunity. Nat Rev Immunol. (2013) 13:875–87. doi: 10.1038/nri3547
21. Gubbels Bupp MR, Potluri T, Fink AL, Klein SL. The confluence of sex hormones and aging on immunity. Front Immunol. (2018) 9:1269. doi: 10.3389/fimmu.2018.01269
22. Klein SL, Flanagan KL. Sex differences in immune responses. Nat Rev Immunol. (2016) 16:626–38. doi: 10.1038/nri.2016.90
23. Ackeret C, Ploss HJ, Hitzig WH. Hereditary severe combined immunodeficiency and adenosine deaminase deficiency. Pediatr Res. (1976) 10:67–70. doi: 10.1203/00006450-197601000-00013
24. Hu Z, van der Ploeg K, Chakraborty S, Arunachalam PS, Mori DA, Jacobson KB, et al. Early immune markers of clinical, virological, and immunological outcomes in patients with COVID-19: a multi-omics study. Elife. (2022) 11:e77943. doi: 10.7554/eLife.77943.sa2
25. Zhang B, Upadhyay R, Hao Y, Samanovic MI, Herati RS, Blair JD, et al. Multimodal single-cell datasets characterize antigen-specific CD8+ T cells across SARS-CoV-2 vaccination and infection. Nat Immunol. (2023) 24:1725–34. doi: 10.1038/s41590-023-01608-9
26. Oberhardt V, Luxenburger H, Kemming J, Schulien I, Ciminski K, Giese S, et al. Rapid and stable mobilization of CD8+ T cells by SARS-CoV-2 mRNA vaccine. Nature. (2021) 597:268–73. doi: 10.1038/s41586-021-03841-4
27. Painter MM, Mathew D, Goel RR, Apostolidis SA, Pattekar A, Kuthuru O, et al. Rapid induction of antigen-specific CD4+ T cells is associated with coordinated humoral and cellular immunity to SARS-CoV-2 mRNA vaccination. Immunity. (2021) 54:2133–2142.e3. doi: 10.1016/j.immuni.2021.08.001
28. Tarke A, Sidney J, Methot N, Yu ED, Zhang Y, Dan JM, et al. Impact of SARS-CoV-2 variants on the total CD4+ and CD8+ T cell reactivity in infected or vaccinated individuals. Cell Rep Med. (2021) 2:100355. doi: 10.1016/j.xcrm.2021.100355
29. Mateus J, Dan JM, Zhang Z, Rydyznski Moderbacher C, Lammers M, Goodwin B, et al. Low-dose mRNA-1273 COVID-19 vaccine generates durable memory enhanced by cross-reactive T cells. Sci. (2021) 374(6566):eabj9853. doi: 10.1126/science.abj9853
30. Nies JF, Panzer U. IL-17C/IL-17RE: emergence of a unique axis in TH17 biology. Front Immunol. (2020) 11. doi: 10.3389/fimmu.2020.00341
31. Vicari AP, Figueroa DJ, Hedrick JA, Foster JS, Singh KP, Menon S, et al. TECK: A novel CC chemokine specifically expressed by thymic dendritic cells and potentially involved in T cell development. Immunity. (1997) 7:291–301. doi: 10.1016/S1074-7613(00)80531-2
32. Kunkel EJ, Campbell JJ, Haraldsen G, Pan J, Boisvert J, Roberts AI, et al. Lymphocyte cc chemokine receptor 9 and epithelial thymus-expressed chemokine (Teck) expression distinguish the small intestinal immune compartment. J Exp Med. (2000) 192:761–8. doi: 10.1084/jem.192.5.761
33. Hieshima K, Kawasaki Y, Hanamoto H, Nakayama T, Nagakubo D, Kanamaru A, et al. CC chemokine ligands 25 and 28 play essential roles in intestinal extravasation of igA antibody-secreting cells. J Immunol. (2004) 173:3668–75. doi: 10.4049/jimmunol.173.6.3668
34. Bowman EP, Kuklin NA, Youngman KR, Lazarus NH, Kunkel EJ, Pan J, et al. The intestinal chemokine thymus-expressed chemokine (CCL25) attracts igA antibody-secreting cells. J Exp Med. (2002) 195:269–75. doi: 10.1084/jem.20010670
35. Kathuria N, Kraynyak KA, Carnathan D, Betts M, Weiner DB, Kutzler MA. Generation of antigen-specific immunity following systemic immunization with DNA vaccine encoding CCL25 chemokine immunoadjuvant. Hum Vaccin Immunother. (2012) 8:1607–19. doi: 10.4161/hv.22574
36. García Vila L, Merinero Palomares M, Herrera de la Muela M, Argüelles Pinto M, Ojeda Pérez F, López Salvà A. Cáncer de mama: utilidad pronóstica de los nuevos marcadores tumorales. Revista de Senología y Patología Mamaria. J Senology Breast Dis. (2003) 16:58–65. https://www.elsevier.es/es-revista-revista-senologia-patologia-mamaria–131-articulo-cancer-mama-utilidad-pronostica-nuevos-13047769.
37. Yatsenko T, Rios R, Nogueira T, Salama Y, Takahashi S, Tabe Y, et al. Urokinase-type plasminogen activator and plasminogen activator inhibitor-1 complex as a serum biomarker for COVID-19. Front Immunol. (2024) 15:1390698. doi: 10.3389/fimmu.2024.1390698
38. D’Alonzo D, De Fenza M, Pavone V. COVID-19 and pneumonia: a role for the uPA/uPAR system. Drug Discov Today. (2020) 25:1528–34. doi: 10.1016/j.drudis.2020.06.013
39. Acevedo N, Escamilla-Gil JM, Espinoza H, Regino R, Ramírez J, Florez de Arco L, et al. Chronic obstructive pulmonary disease patients have increased levels of plasma inflammatory mediators reported upregulated in severe COVID-19. Front Immunol. (2021) 12:678661. doi: 10.3389/fimmu.2021.678661
40. Nekrasova LA, Shmakova AA, Samokhodskaya LM, Kirillova KI, Stoyanova SS, Mershina EA, et al. The association of PLAUR genotype and soluble suPAR serum level with COVID-19-related lung damage severity. Int J Mol Sci. (2022) 23:16210. doi: 10.3390/ijms232416210
41. Abdellatif HAA, Sultan BO, Nassar HM, Gomaa MEE, Sakr MG, Riad E, et al. Circulating soluble urokinase plasminogen activator receptor as a predictive indicator for COVID-19-associated acute kidney injury and mortality: clinical and bioinformatics analysis. Int J Mol Sci. (2023) 24:7177. doi: 10.3390/ijms24087177
42. Tan Y, Lu S, Wang B, Duan X, Zhang Y, Peng X, et al. Single-cell transcriptome atlas reveals protective characteristics of COVID-19 mRNA vaccine. J Med Virol. (2023) 95(1):e28161. doi: 10.1002/jmv.28161
43. AbdelMassih AF, Menshawey R, Ismail JH, Husseiny RJ, Husseiny YM, Yacoub S, et al. PPAR agonists as effective adjuvants for COVID-19 vaccines, by modifying immunogenetics: a review of literature. J Genet Eng Biotechnol. (2021) 19:82. doi: 10.1186/s43141-021-00179-2
44. Janho dit Hreich S, Hofman P, Vouret-Craviari V. The role of IL-18 in P2RX7-mediated antitumor immunity. Int J Mol Sci. (2023) 24:9235. doi: 10.3390/ijms24119235
45. Lee EY, Lee ZH, Song YW. CXCL10 and autoimmune diseases. Autoimmun Rev. (2009) 8:379–83. doi: 10.1016/j.autrev.2008.12.002
46. Hackney JA, Shivram H, Vander Heiden J, Overall C, Orozco L, Gao X, et al. A myeloid program associated with COVID-19 severity is decreased by therapeutic blockade of IL-6 signaling. iScience. (2023) 26:107813. doi: 10.1016/j.isci.2023.107813
47. Herr C, Mang S, Mozafari B, Guenther K, Speer T, Seibert M, et al. Distinct patterns of blood cytokines beyond a cytokine storm predict mortality in COVID-19. J Inflamm Res. (2021) 14:4651–67. doi: 10.2147/JIR.S320685
48. Arunachalam PS, Wimmers F, Mok CKP, Perera RAPM, Scott M, Hagan T, et al. Systems biological assessment of immunity to mild versus severe COVID-19 infection in humans. Science. (2020) 369:1210–20. doi: 10.1126/science.abc6261
49. Takashima Y, Inaba T, Matsuyama T, Yoshii K, Tanaka M, Matsumoto K, et al. Potential marker subset of blood-circulating cytokines on hematopoietic progenitor-to-Th1 pathway in COVID-19. Front Med (Lausanne). (2024) 11. doi: 10.3389/fmed.2024.1319980
50. Wulandari S, Nuryastuti T, Oktoviani FN, Daniwijaya MEW, Supriyati E, Arguni E, et al. The association between high mobility group box 1 (HMGB1) and Interleukin-18 (IL-18) serum concentrations in COVID-19 inpatients. Heliyon. (2024) 10:e26619. doi: 10.1016/j.heliyon.2024.e26619
51. Bergamaschi C, Terpos E, Rosati M, Angel M, Bear J, Stellas D, et al. Systemic IL-15, IFN-γ, and IP-10/CXCL10 signature associated with effective immune response to SARS-CoV-2 in BNT162b2 mRNA vaccine recipients. Cell Rep. (2021) 36:109504. doi: 10.1016/j.celrep.2021.109504
52. Kovarik JJ, Kämpf AK, Gasser F, Herdina AN, Breuer M, Kaltenecker CC, et al. Identification of immune activation markers in the early onset of COVID-19 infection. Front Cell Infect Microbiol. (2021) 11. doi: 10.3389/fcimb.2021.651484
53. Lozano-Rodríguez R, Avendaño-Ortíz J, Terrón V, Montalbán-Hernández K, Casalvilla-Dueñas J, Bergón-Gutiérrez M, et al. mRNA-1273 boost after BNT162b2 vaccination generates comparable SARS-CoV-2-specific functional responses in naïve and COVID-19-recovered individuals. Front Immunol. (2023) 14:1136029. doi: 10.3389/fimmu.2023.1136029
54. Rosati M, Terpos E, Homan P, Bergamaschi C, Karaliota S, Ntanasis-Stathopoulos I, et al. Rapid transient and longer-lasting innate cytokine changes associated with adaptive immunity after repeated SARS-CoV-2 BNT162b2 mRNA vaccinations. Front Immunol. (2023) 14. doi: 10.3389/fimmu.2023.1292568
55. Proost P, Wuyts A, van Damme J. Human monocyte chemotactic proteins-2 and -3: structural and functional comparison with MCP-1. J Leukoc Biol. (1996) 59:67–74. doi: 10.1002/jlb.59.1.67
56. Blanco-Melo D, Nilsson-Payant BE, Liu WC, Uhl S, Hoagland D, Møller R, et al. Imbalanced host response to SARS-coV-2 drives development of COVID-19. Cell. (2020) 181:1036–1045.e9. doi: 10.1016/j.cell.2020.04.026
57. Thoutam A, Breitzig M, Lockey R, Kolliputi N. Coronavirus: a shift in focus away from IFN response and towards other inflammatory targets. J Cell Commun Signal. (2020) 14:469–70. doi: 10.1007/s12079-020-00574-3
58. Gelzo M, Cacciapuoti S, Pinchera B, De Rosa A, Cernera G, Scialò F, et al. Further findings concerning endothelial damage in COVID-19 patients. Biomolecules. (2021) 11:1368. doi: 10.3390/biom11091368
59. Bag Soytas R, Cengiz M, Islamoglu MS, Borku Uysal B, Yavuzer S, Yavuzer H. Antibody responses to COVID-19 vaccines in older adults. J Med Virol. (2022) 94:1650–4. doi: 10.1002/jmv.27531
60. Collins CP, Longo DL, Murphy WJ. The immunobiology of SARS-CoV-2 infection and vaccine responses: potential influences of cross-reactive memory responses and aging on efficacy and off-target effects. Front Immunol. (2024) 15. doi: 10.3389/fimmu.2024.1345499
61. Sagi Z, Hieronymus T. The impact of the epithelial–mesenchymal transition regulator hepatocyte growth factor receptor/met on skin immunity by modulating langerhans cell migration. Front Immunol. (2018) 9. doi: 10.3389/fimmu.2018.00517
62. Ruth JH, Gurrea-Rubio M, Athukorala KS, Rasmussen SM, Weber DP, Randon PM, et al. CD6 is a target for cancer immunotherapy. JCI Insight. (2021) 6(5):e145662. doi: 10.1172/jci.insight.145662
63. Kramer KJ, Wilfong EM, Voss K, Barone SM, Shiakolas AR, Raju N, et al. Single-cell profiling of the antigen-specific response to BNT162b2 SARS-CoV-2 RNA vaccine. Nat Commun. (2022) 13:3466. doi: 10.1038/s41467-022-31142-5
64. Flego D, Cesaroni S, Romiti GF, Corica B, Marrapodi R, Scafa N, et al. Platelet and immune signature associated with a rapid response to the BNT162b2 mRNA COVID-19 vaccine. J Thromb Haemostasis. (2022) 20:961–74. doi: 10.1111/jth.15648
Keywords: COVID-19, BTN162b2, SARS-CoV-2, anti-spike antibodies, inflammatory biomarker
Citation: Leno-Duran E, Serrano-Conde E, Salas-Rodríguez A, Salcedo-Bellido I, Barrios-Rodríguez R, Fuentes A, Viñuela L, García F and Requena P (2024) Evaluation of inflammatory biomarkers and their association with anti-SARS-CoV-2 antibody titers in healthcare workers vaccinated with BNT162B2. Front. Immunol. 15:1447317. doi: 10.3389/fimmu.2024.1447317
Received: 11 June 2024; Accepted: 31 July 2024;
Published: 23 August 2024.
Edited by:
Geert Leroux-Roels, Ghent University, BelgiumReviewed by:
Valentino D'Onofrio, Ghent University, BelgiumYasutoshi Kido, Osaka City University, Japan
Copyright © 2024 Leno-Duran, Serrano-Conde, Salas-Rodríguez, Salcedo-Bellido, Barrios-Rodríguez, Fuentes, Viñuela, García and Requena. This is an open-access article distributed under the terms of the Creative Commons Attribution License (CC BY). The use, distribution or reproduction in other forums is permitted, provided the original author(s) and the copyright owner(s) are credited and that the original publication in this journal is cited, in accordance with accepted academic practice. No use, distribution or reproduction is permitted which does not comply with these terms.
*Correspondence: Pilar Requena, prequena@ugr.es
†These authors have contributed equally to this work and share first authorship
‡These authors have contributed equally to this work and share last authorship