- 1Institute of Clinical and Experimental Trauma Immunology, Ulm University Medical Center, Ulm, Germany
- 2Department of Orthopaedics, Trauma and Reconstructive Surgery, Rheinisch-Westfälische Technische Hochschule (RWTH) Aachen University, Aachen, Germany
- 3Institute of Orthopaedic Research and Biomechanics, Ulm University Medical Center, Ulm, Germany
- 4Department of Trauma, Hand, and Reconstructive Surgery, University Hospital Frankfurt, Goethe University, Frankfurt/Main, Germany
- 5The Department of Cell Biology-Inspired Tissue Engineering (cBITE), MERLN Institute for Technology-Inspired Regenerative Medicine, Maastricht University, Maastricht, Netherlands
- 6Department of Immunology, Oslo University Hospital, University of Oslo, Oslo, Norway
- 7Research Laboratory, Nordland Hospital Bodø, Bodø, Norway
Background: Polytrauma and hemorrhagic shock can lead to direct and indirect liver damage involving intricate pathophysiologic mechanisms. While hepatic function has been frequently highlighted, there is minimal research on how the receptor activator of the NF-κB (RANK)/RANK ligand (RANKL)/osteoprotegerin (OPG) system is regulated in the liver following trauma. Furthermore, cross-talking complement and toll-like-receptor (TLR) systems can contribute to the posttraumatic response. Therefore, we investigated the hepatic consequences of polytrauma focusing on the RANK-RANKL-OPG axis, and evaluated the effects of a dual blockade of complement factor C5 and TLR-cofactor CD14 on hepatic features.
Methods: The established pig model of polytrauma (PT) and hemorrhagic shock included pulmonary contusion, hepatic dissection, and bilateral femur fractures, surgically addressed either by external fixation (Fix ex) or intramedullary nailing (Nail). Four groups were investigated: 1) sham animals; 2) PT treated by Fix ex (Fix ex); 3) PT by Nail (Nail); or 4) PT by Nail plus combined C5/CD14 inhibition (Nail+Therapy). Serum samples were obtained between 0 - 72 h, and liver samples at 72 h after PT. Liver tissues were histologically scored and subjected to RT-qPCR-analyses, immunohistochemistry and ELISAs to evaluate the posttraumatic hepatic response with a focus on the RANK-RANKL-OPG system.
Results: Following PT, the liver injury score of the Nail+Therapy group was significantly lower than in the Fix ex or Nail group without immunomodulation (p<0.05). Similarly, the degree of necrosis, lobular stasis, and inflammation were significantly reduced when treated with C5/CD14-inhibitors. Compared to the Nail group, AST serum concentrations were significantly decreased in the Nail+Therapy group after 72 h (p<0.05). PCR analyses indicated that RANK, RANKL, and OPG levels in the liver were increased after PT in the Nail group compared to lower levels in the Nail+Therapy group. Furthermore, liver tissue analyses revealed increased RANK protein levels and cellular immunostaining for RANK in the Nail group, both of which were significantly reduced in the case of C5/CD14-inhibition (p<0.05).
Conclusion: Following experimental PT, dual inhibition of C5/CD14 resulted in altered, mainly reduced hepatic synthesis of proteins relevant to bone repair. However, a comprehensive investigation of the subsequent effects on the liver-bone axis are needed.
1 Introduction
Polytrauma is defined as an injury to two or more bodily parts due to violent factors, with at least one or the sum of all injuries being potentially life-threatening (1, 2). In the last decades two main surgical approaches have been established: damage control orthopedic surgery (DCO) with rather minimal invasive but only temporal surgery (e. g. by external bone fixation) and early total care (ETC) with definite but more invasive surgery (e.g. by intramedullary bone nailing), both of which are used in accordance to the overall trauma impact and pattern and patient’s condition (3). As treatment concepts continue to evolve and clinical practice progresses, trauma and its complications remain one of the leading causes of mortality, particularly among young and middle-aged individuals (4). The liver may not only bear the direct impact of trauma, but also - as the largest metabolic organ - become a central actor and target organ for the posttraumatic systemic inflammatory response (5). Various liver injury models with profound shifts in the hepatic transcriptome resulted in differential transcriptomic changes of extrahepatic organs, indicating a metabolite-mediated crosstalk between the liver and distant organs (6), including the bone. Regarding bone, both local and systemic inflammation are known to impair fracture healing (6–8). In our porcine polytrauma model, proteomic analysis of the fracture hematoma at 72 h post injury revealed the presence of coagulation-related, immunomodulatory, and osteogenic proteins, which were influenced by different surgical approaches. ETC using intramedullary nailing activated cellular and fluid components of while reducing the levels of proteins involved in osteogenesis and tissue remodelling. In contrast, DCO using external bone fixation led to elevated concentrations of proteins with anti-inflammatory and pro-regenerative properties within the fracture hematoma (9). Furthermore, severe concomitant trauma impairs fracture healing in mice (10) and men (11). Mechanistically, the initial temporal hypoxia seems to sustainably impact bone healing (12). However, the mechanisms of action of the proposed liver-bone axis after trauma still require further study.
Two crucial innate immunity recognition systems, namely the complement and the Toll-like receptor (TLR) systems, appear to play pivotal roles in trauma-induced inflammation (13). The complement system is vital for the hepatic homeostasis and immune response, influencing the development of various liver diseases, including alcoholic diseases and hepatocellular carcinoma, and hepatic ischemia-reperfusion injury (IRI) (14–17). Several complement inhibition strategies, including a C1- and a C3-inhibitor, as well as minocycline and doxycycline, have demonstrated effective attenuation of IRI to the liver following multiple injuries (18–20). CD14 is a co-receptor for several TLRs, in humans particularly for TLR4 and TLR2, and for mice it has been shown for several others (21). A number of studies on the TLRs and trauma has been published, but the CD14 molecule has barely been investigated, despite its important role as co-factor for several TLRs. One study showed that elevated soluble CD14 (sCD14) was a strong predictor for trauma patients who developed sepsis (22). Moreover, the combined inhibition of complement and CD14 as master alarm and processing systems of the systemic inflammatory response revealed significant anti-inflammatory effects (23).
Moreover, the combined inhibition of complement and toll-like receptor (TLR) as master alarm and processing systems of the systemic inflammatory response revealed significant anti-inflammatory effects (23). It is tempting to speculate, that such a combined blockade could eventually enable the surgeon to perform invasive ETC even when minimal-invasive DCO would be indicated.
However, the molecular mechanism of liver damage, induced either directly or indirectly after polytrauma (e.g. by combined pulmonary contusion, hepatic trauma and bilateral femoral shaft fractures or by the additional presence of a hemorrhagic shock) remain uncertain. In particular, the impact of various inflammatory and regenerative mediators released after liver injury requires further characterization, and especially further clarification to what extent they are dependent on the basic innate immune TLR- and complement system. In this context, it is also essential to elucidate the impact of traumatic liver injury and potential immunomodulation on pivotal factors that regulate bone fracture healing, including the receptor activator of NF-κB (RANK), its ligand (RANKL), and osteoprotegerin (OPG).
In the liver, RANKL and OPG are expressed by multiple cell types, including hepatocytes, Kupffer cells, and liver sinusoidal endothelial cells [3]. Furthermore, the RANK-RANKL-OPG axis represents a pivotal pathway in the context of liver injury and development of fibrosis (24). Additionally, hepatic stellatae cells (HSC) as drivers of fibrosis, also express RANKL, although its expression decreases as these cells activate (25). OPG, acting as a decoy receptor for RANKL, can inhibit the RANKL-RANK interaction, thereby suppressing the activation of HSCs and subsequent fibrotic response (26). The dynamic interplay between these components in the liver is complex and may link to the bone tissue (27) and can vary depending on the stage and type of liver disease. For instance, during the early stages of liver injury, RANKL may promote inflammation and fibrosis, while later stages might reveal a protective role through the inhibition of HSC activation (28). Understanding the RANK-RANKL-OPG axis may potentially offer novel approaches in managing liver injury and its complications, highlighting the importance of further research in this area (29).
Therefore, in the context of trauma, we specifically aimed to characterize the RANK-RANKL-OPG axis in the liver and systemically in a well-defined experimental polytrauma setting in absence or presence of an immunomodulatory therapy. We hypothesised that polytrauma results in an alteration of liver mediators relevant for inflammation and fracture repair; and, furthermore, that this response is improved by an immunomodulation approach targeting the central complement component 5 (C5) and TLR-coreceptor cluster of differentiation 14 (CD14).
2 Materials and methods
2.1 Animal model and group establishment
The study protocol of the hemodynamically instable pig polytrauma (PT) model was approved by the Office for Nature, Environment, and Consumer Protection of the State of North Rhine-Westphalia (LANUV AZ 81-02.04. 2020.A215) (17, 18). All pigs (German Landrace) from a pathogen-free barrier breeding facility, aged 12–16 weeks (weight 35 ± 5 kg), were maintained under a 12-h day/night rhythm for 7 d prior to the experiment to acclimatise to their surroundings.
A total of 25 animals were randomly distributed into four groups: sham (Sham, n=6) in absence of PT; simulated PT plus external fixation (Fix ex, n=8), PT plus internal fixation (Nail, n=7) and PT plus internal fixation plus combined C5/CD14 inhibition therapy (Nail+Therapy, n=4). The combined immunomodulatory therapy involved injecting C5 and CD14 inhibitors intravenously into the femoral vein. A C5 inhibitor (3 mg/kg body weight) was given 30 min after trauma to the animals in the therapy group, followed by a continuous infusion (1.1 mg/kg/h) until 72 h after trauma. The C5 inhibitor dose was based on titration and measured by effect on inhibitory complement activity in 3 pilot pigs observed for 8 hours (unpublished data). The pharmacodynamics of the C5 therapy in pigs in this study, allowing for accurate adjustment for 72 hours has been described in detail previously (30). The anti-CD14 inhibitor (mAb rMIL-2) was given at 5 mg/kg at 30 min, 12 h, and 30 h after trauma and at 2.5 mg/kg at 60 h after trauma. The dose was chosen based previous studies. In a porcine E. coli model, we titrated increasing doses to saturation of the CD14 molecules in blood leukocytes as measured by flow cytometry (14). The recombinant form of the original anti-CD14 (rMIL-2) used in this paper showed the same optimal dose of 5 mg/kg (31), and was used in success in a pig model of polymicrobial sepsis combined with C5 inhibition (32). This dose was therefore used in the present study.
All pigs were harvested 72 h after trauma and intensive care treatment. All data presented in the paper were obtained in the context of a larger study to address the 3R principles. Horst et al. (33) provided a specific description of the preparation and instrumentation. Azaperone (StresnilTM, Janssen, Germany; i.m. injection of 6-8 mg/kg body weight) and Ketamine (Ketanest, Pfizer, New York; 15 mg i.m./kg body weight) were used in combination as pre-anaesthetic agents. In addition, for general anaesthesia, Propofol (Fresenius, Bad Homburg vor der Hoehe, Germany; 2-12 mg/kg body weight/h) and Midazolam (Panpharma GmbH, Trittau, Germany; 0.02 -0.5 mg/kg body weight/h) were used for general anaesthesia and Fentanyl (Panpharma GmbH; 0.5-20 ug/kg body weight/h) was used as a general analgesic. Following intubation, PT was induced by a combination of injuries: blunt chest trauma, laparotomy with hepatic lacerations, haemorrhagic shock and bilateral open femoral shaft fractures. Systemic anaesthesia and analgesia as well as a lung-protective ventilation strategy with a tidal volume of 8–12 ml/kg body weight were applied throughout the experiment. Blunt chest trauma was induced on the right side of the pig’s chest during the inhalation phase using a bolt gun (Dynamit-Nobel, cartridge 9×17; Vienna, Austria) and a pair of steel and lead plates (0.8 and 1.0 cm thickness, respectively). Simulating clinical reality, the proportion of inhaled O2 was set at 21% for the first 90 min after trauma induction and was adjusted continuously thereafter according to real-time O2 saturation. Subsequently, a midline laparotomy was performed to expose the left liver lobe. Two incisions (4.5 cm × 4.5 cm) were made to the liver using a scalpel to simulate abdominal trauma. The bleeding was stopped after 30 s using a sterile gauze packing technique. Subsequently, the femoral shafts were exposed by a 5 cm skin incision. To induce bilateral femoral shaft fractures, a bolt gun and steel stamp were used at a 90° angle to the bone. Concurrently, pressure-controlled haemorrhagic shock was initiated by drawing blood from the femoral vein until a mean arterial pressure (MAP) of 40 ± 5 mmHg was obtained, or the maximum volume of blood drawn reached 45% of the total blood volume. This low MAP was maintained for 90 min. Throughout the 72 h of the experiment, the animals were given fluids (Sterofundin, B. Braun, Germany) at a rate of 0.5-2.0 ml/kg/h and parenteral nutrition (Aminoven, Fresenius Kabi, Germany) of 50-70 ml/kg body weight and day, under monitoring of the fluid balance. If required, norepinephrine was administered i.v. for maintaining the MAP>60 mmHg.
2.2 Drug introduction
UCB Pharma (Brussels, Belgium) provided RA 101295 (2-kDa peptide). This C5 inhibitor inhibits both C5 cleavage and the subsequent formation of the terminal complement complex C5b–9, which presents on the cell surface as the membrane attack complex (MAC).
RMil2 is a recombinant anti-pig CD14 antibody (clone MIL2; IgG2a), made available by Prof. TE Mollnes (Norway), that inhibits CD14-mediated pro-inflammatory cytokine responses. It is effective in porcine sepsis and IRI models when combined with a complement inhibitor (34).
2.3 Liver damage evaluation
Immediately after euthanasia, induced by deepening of the narcosis by 20 ml pentobarbital i.v. and a bolus of 40 ml KCl i.v., liver samples were removed and fixed in 10% formalin at room temperature (RT) for 24 h. Tissues were paraffin-embedded and sectioned onto slides. Ten images from random areas at 20× magnification were obtained from each slide. Morphological changes in haematoxylin and eosin (HE)-stained sections of the liver were examined by two independent blinded observers and scored accordingly. Stasis (appearance and distribution of red blood cells), vacuolation, parenchymal necrosis, and inflammation (appearance and distribution of polymorphonuclear granulocytes [PMNs]) were each graded into four features ranging each from 0 to maximal 3 points (0=none, 1=mild, 2=moderate, 3=severe). Thus, the overall histological liver damage score could range from 0 – maximal 12 points. Assessment of haemorrhage and necrosis was performed with a light microscope (Axio Imager M1, Carl Zeiss, Oberkochen, Germany) at a magnification of 2.5×. The number of liver tissue vacuoles and PMNs were assessed at 10× and 20× magnification.
2.4 Immunohistochemical staining of the liver
Formalin-fixed paraffin-embedded liver slides (4 μm thickness) were deparaffinized and rehydrated in a descending alcohol series. The antigen retrieval was performed in a microwave (700 W, 20 min) using citrate buffer (pH 6.0). The slides were blocked with 10% normal goat serum for 1 h at RT. Subsequently, the slides were incubated with a primary antibody (rabbit anti-pig myeloperoxidase (MPO) polyclonal antibody (Abcam) at a concentration of 1.4 μg/ml at 4°C overnight. MPO detection was based on an alkaline phosphatase method using a DAKO kit (Agilent Technologies). For RANK staining, the liver sections were similarly prepared and incubated overnight at 4°C with RANK primary antibody (Cloud-clone, Wuhan, China) at 1:100 dilution. Following washing with Tween 20 Tris-buffered saline, goat anti-rabbit horse-radish peroxidase-labelled secondary antibody (Abcam, UK) was added at 1:50 dilution at RT for 30 min and developed with DAKO (Agilent) for 20 min. Finally, the sections were visualised using a Zeiss Axio Imager A1 microscope. Seven images at 20× magnification were obtained from each slide and analysed using Image J automated cell-counting.
2.5 Real-time quantitative polymerase chain reaction
Liver tissue homogenates were centrifuged at 300 g for 5 min at 4°C and RNA was isolated according to the manufacturer’s (Qiagen Qiashredder™, Hilden, Germany) protocol. The RNA yield was quantified with a Qubit 2.0 fluorometer using the Qubit RNA BR Assay Kit (Thermo Fisher Scientific). cDNA was generated using the AffinityScript qPCR cDNA synthesis kit (Agilent Technologies) with oligo(dT) primers and stored at −80°C until further use. RT-qPCR was performed using a qPCR cycler Mx3000P (Agilent Technologies) and Brilliant III Ultra-Fast SYBR Green QPCR Master Mix (Agilent Technologies). Primers were purchased from Biomer.net (Ulm, Germany): glyceraldehyde 3-phosphate dehydrogenase (used as a housekeeping gene), OPG, RANK, and RANKL. Relative gene expression was determined by the 2−ΔΔ Ct method (35) and results are reported as fold change compared to unstimulated control cells. Primers used for real-time PCRs are displayed in the Supplementary Table.
2.6 Enzyme linked immunosorbent assay
Serum and liver protein concentrations of RANK, RANKL, and OPG were determined using commercially available Porcine ELISA kits (RANK, RANKL: Mybiosource, San Diego, USA; OPG: Lsbio, Seattle, USA) using the pre-coated kits according to the manufacturers’ instructions.
For C5a measurements, a porcine ELISA (Hycult Biotech, Uden, Netherlands) was applied, strictly following the manufacturers’ protocol.
To determinate the total protein concentrations in serum samples and tissue homogenates, a commercially available bicinchoninic acid protein assay kit (Thermo Scientific, Rockford, USA) was used for protein determination as recommended by the manufacturer.
2.7 Aspartate aminotransferase measurement
Serum AST was analysed using an automated chemical analyser (VITROS 350; Ortho-Clinical Diagnostics, Raritan, NJ).
2.8 Data analysis
To check the normality of the obtained data sets, the Shapiro–Wilk and Kolmogorov-Smirnov tests were performed on each set of data. Unless otherwise noted, data satisfying normal distribution were expressed as the mean ± standard deviation (SD) or in the case of the histological analyses as the median with the 25th/75th percentile. One-way Analysis of variance (ANOVA) followed by Holm-Sidak post-hoc testing was performed for comparisons between multiple groups. Data that did not satisfy a normal distribution were subjected to the rank sum test and post-hoc testing (Dunn’s method). A p<0.05 was considered to be statistically significant.
3 Results
3.1 Liver damage after experimental polytrauma is reduced by synchronic inhibition of complement C5 and CD14
Analysis of the HE-stained liver tissue sections taken 72 h after the polytrauma impact revealed a significant increase of the damage score in both the Fix ex and Nail groups versus the Sham group. The total score was 1.67 ± 0.31 for the Sham group, 8.65 ± 1.01 for the Fix ex group, and 9.19 ± 0.60 for the Nail group. By contrast, the liver injury score of the Nail+Therapy group (5.50 ± 0.44) was significantly lower than in the Nail or Fix ex group without the immunomodulatory therapy (Figure 1A). HE staining of the liver tissue in the PT groups revealed increased signs of disorganisation and congestion of the hepatic lobular structure (Figure 1B) and numbers of vacuoles (Figure 1C) and necrosis of some hepatocytes (Figure 1D) compared to the normal control group. An infiltration of neutrophils in the liver indicated a clear inflammatory response in the liver (Figure 1E). By contrast, the degree of necrosis, hepatic lobular stasis and neutrophil recruitment were significantly reduced in the Nail+Therapy group, which was not the case in the Nail or Fix ex groups without immunomodulation.
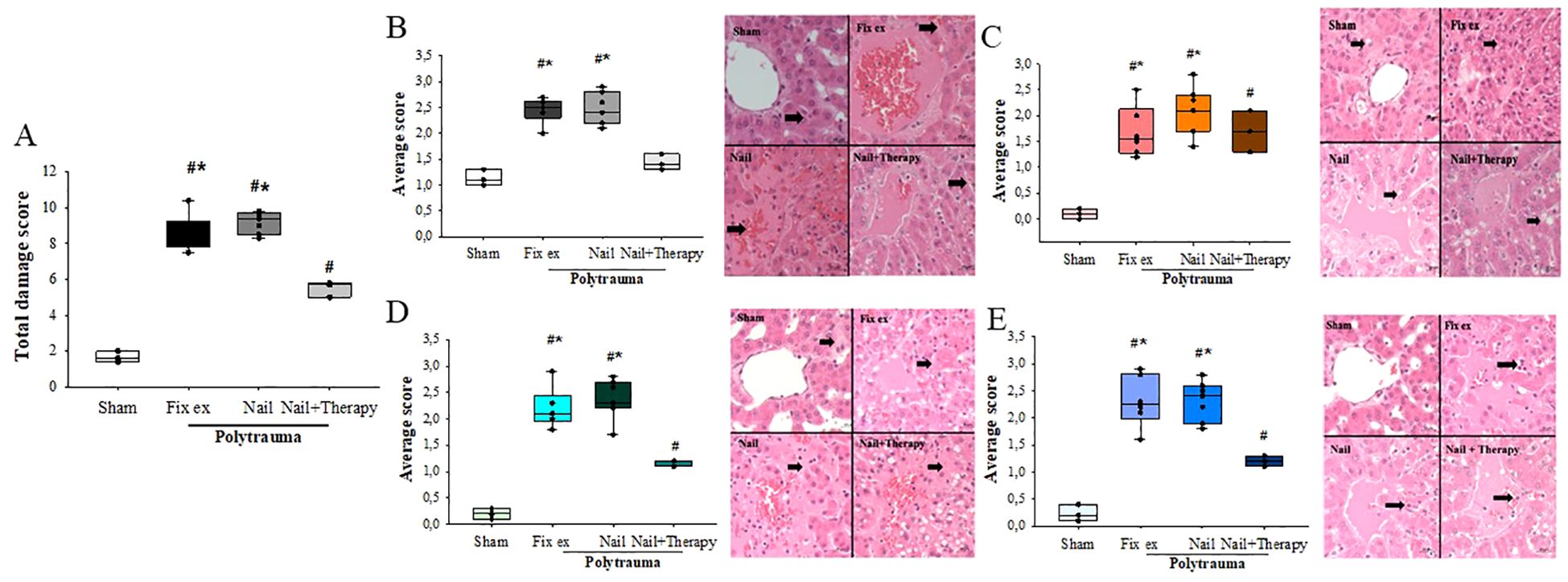
Figure 1. Polytrauma-caused increase in liver injury was improved by C5/CD14-immunomodulation. Liver histology (HE-staining, 400× magnification) was obtained from pigs in each group 72 h post polytrauma including hemorrhagic shock. Total liver damage score (A) for each group assessing four aspects: congestion (B), vacuolation (C), necrosis (D), and inflammation (E). The black arrow indicates the structure of the liver lobules. ANOVA with Holm-Sidak posthoc testing was applied to compare the data between the groups. Median values are displayed with the 25th and 75th percentile, respectively; denotes significant differences vs. Sham group, * denotes significant differences vs. Nail+Therapy group. Fix ex = external fixation, Nail = internal fixation with intramedullary nail, Nail+Therapy = intramedullary nail with combined C5/CD14 inhibition. Sham: n = 6, Fix ex: n = 8, Nail: n = 7, Nail+Therapy: n = 4.
3.2 Effects of C5/CD14-inhibition on enhanced liver enzyme concentrations after polytrauma
Directly after the polytrauma and corresponding surgical intervention (0 h), except in the Nail group, there was no significant increase in serum AST levels (Figure 2). At 1.5 h after polytrauma, the AST level increased by trend in the Fix ex group and significantly in the Nail group compared to the Sham group (Figure 2; p<0.05), suggesting that the liver was significantly injured by 1.5 h after trauma, particularly in case of early total care (Nail). The changes were time dependent, peaking for all groups at 48 h after the trauma with a subsequent decrease (Figure 2). The AST serum concentrations in the Nail group decreased by immunomodulation at least to the AST levels found in the minimal invasive Fix ex group (Figure 2).
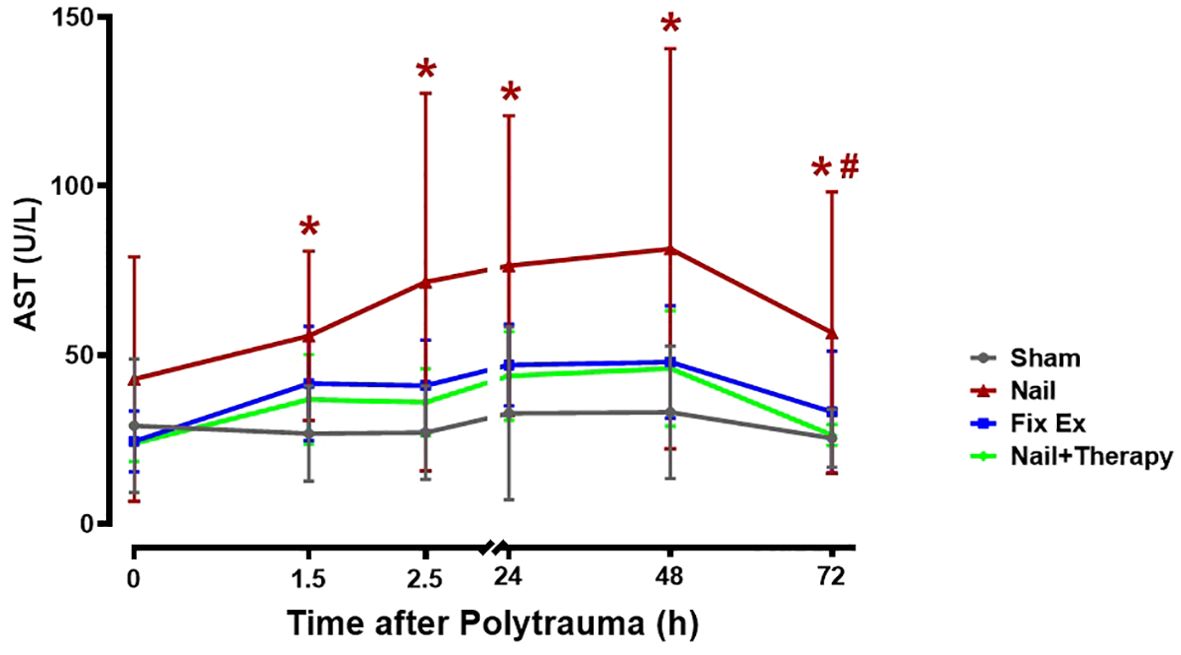
Figure 2. Effects of C5/CD14 blockade on enhanced serum aspartate transaminase (AST) concentrations after polytrauma. Blood samples were obtained from polytraumatized pigs at the indicated time-points. The Nail group displayed a higher overall serum AST concentration than the Nail+Therapy group (p<0.05), the latter group being similar to the Fix ex group without immunomodulation. By trend, AST levels in all groups increased until they peaked 48 h after trauma. AST levels at different time points for each group. Data are shown as the mean ± SD. Fix ex, external fixation; Nail, internal fixation with intramedullary nail; Nail+Therapy, intramedullary nail with combined C5/CD14 inhibitor treatment. Sham: n = 6, Fix ex: n = 8, Nail: n = 7, Nail+Therapy: n = 4. # denotes significant differences vs. Sham group. * denotes significant differences vs. 614 Nail+Therapy group.
3.3 Immunomodulation reduces MPO positive cells in the post polytrauma liver
After immunohistochemical staining, seven observation fields were randomly chosen for each liver tissue sample, and the MPO-positive cells were recorded using Image J to determine the mean number of such cells in each group (Figure 3). Few MPO positive cells were observed in liver tissues of sham pigs, whereas their numbers by trend in the liver tissues of polytraumatised pigs treated by either the Nail or Fix ex. By trend, a decrease in MPO staining with an overall reduced variance was found in livers from the Nail+Therapy versus the Nail only group (Figure 3). Addressing the anaphylatoxin C5a as a potent chemoattractant for inflammatory cells, the liver tissue concentrations of C5a were measured by ELISA, but did not significantly alter between the groups (Supplementary Figure).
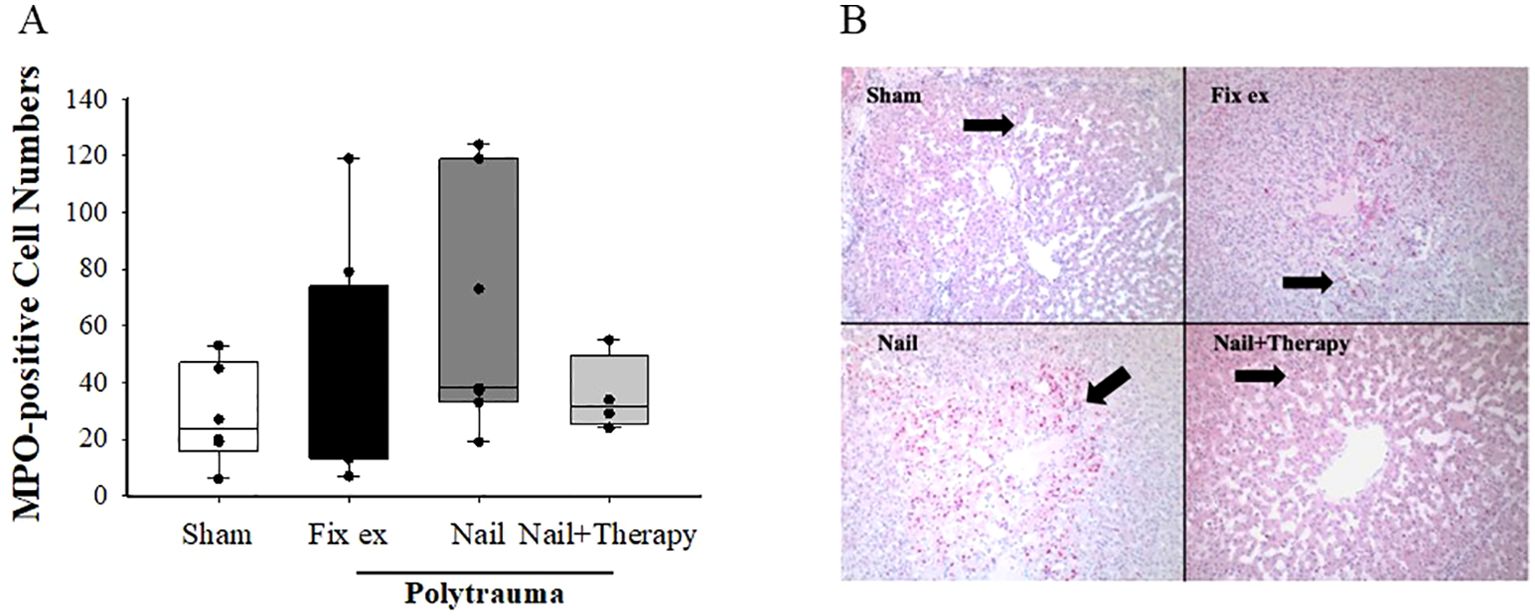
Figure 3. Amelioration of myeloperoxidase (MPO) staining of liver tissues by C5/CD14 inhibition after polytrauma. At 72 h after polytrauma or sham procedure, liver sections were stained for MPO and evaluated. Overall mean score of the number of MPO positive cells in each group (A). ANOVA with Holm-Sidak posthoc testing was applied to compare the data between the groups. Data are displayed as means ± SD, and for the histological analysis as the median with the 25th/75th percentile, denotes significant differences vs. Sham group, Representative histological sections at 100× magnification (B). Black arrow: MPO positive cells in the hepatic lobules. Fix ex, external fixation; Nail, internal fixation with intramedullary nail; Nail+Therapy, intramedullary nail with combined C5/CD14 inhibitor treatment. Sham: n = 6, Fix ex: n = 8, Nail: n = 7, Nail+Therapy: n = 4.
3.4 Polytrauma increases hepatic expression and protein concentration of RANK, which is abolished by C5/CD14 inhibition
Subsequently, the gene expression and protein concentrations of RANK, RANKL, and OPG, as established key regulators of bone resorption and (re)modulation, were determined.
On the protein level, slightly enhanced RANK concentrations were found in the liver (Figure 4A) and in serum (Figure 4B) post polytrauma, which, in the liver, were significantly higher in the Nail group than in the Nail+Therapy group. A similar pattern was found in the liver for the RANK RNA expression (Figure 4C). Immunohistochemical staining indicated some RANK protein staining in hepatocytes, particularly after polytrauma (Figure 4D). The number of RANK positive cells differed between the four groups, with the number of such cells in the Nail group being significantly higher than in the Sham and Nail+Therapy group (p<0.05) (Figure 4E).
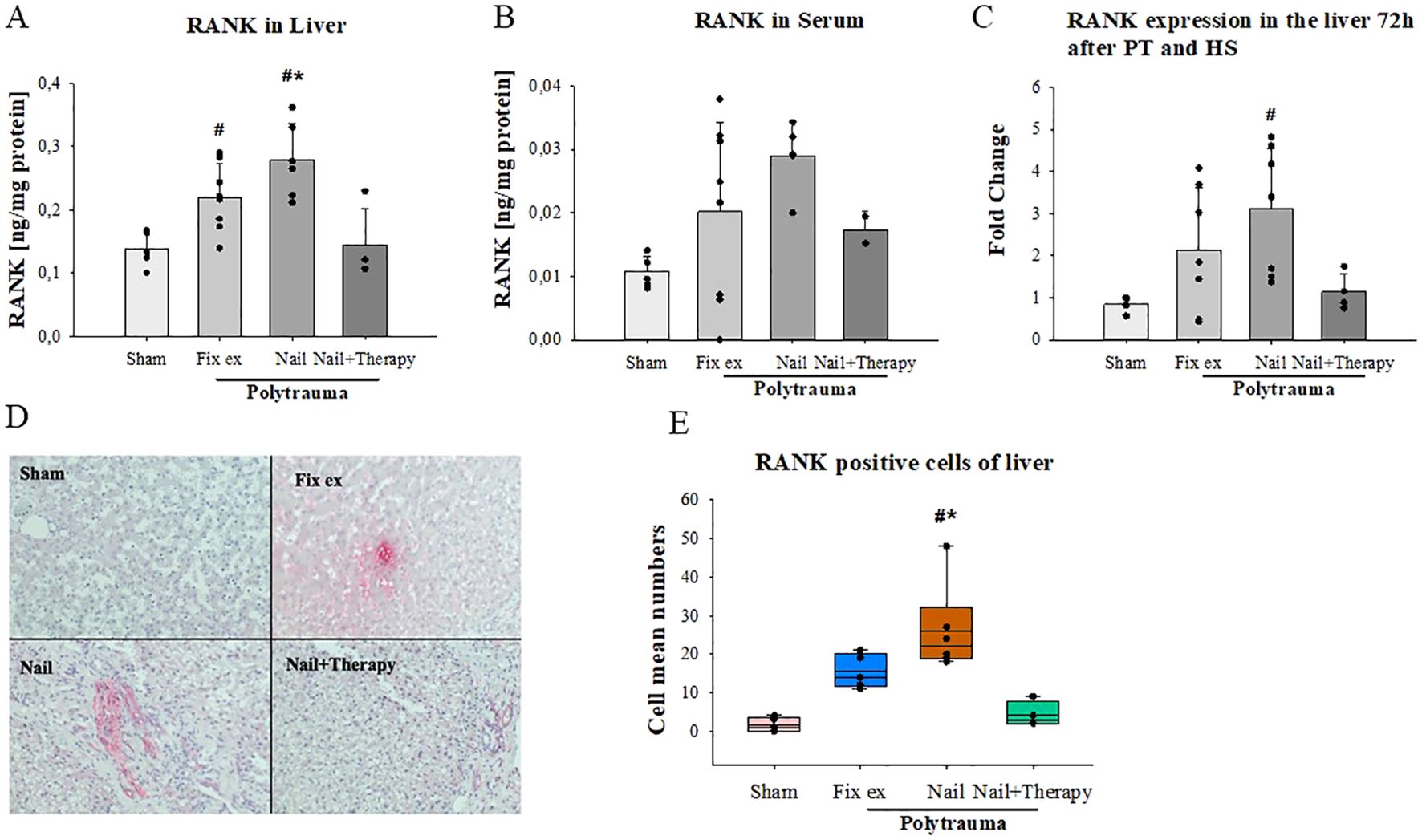
Figure 4. Detection of RANK in liver tissue and serum after polytrauma and reduction by systemic C5/CD14 blockade. RANK protein concentrations in liver tissue homogenates (A) and serum (B) 72 h after polytrauma (determined by ELISA). RANK RNA-expression levels in liver homogenates 72 h post trauma (C). Representative histological liver staining images for RANK (D). Statistical analysis of RANK positive cells in the liver histology (E). Data satisfying a normal distribution were subjected to ANOVA, otherwise the rank sum test was applied. Data are shown as the mean ± SD; denotes significant differences vs. Sham group, * denotes significant differences vs Nail+Therapy group. Fix ex, external fixation; Nail, internal fixation with intramedullary nail; Nail+Therapy, intramedullary nail with combined C5/CD14 inhibitor treatment. Sham: n = 6, Fix ex: n = 8, Nail: n = 7, Nail+Therapy: n = 4.
3.5 Inhibition of C5/CD14 modulates liver and serum RANKL and OPG after polytrauma
Regarding RANKL, liver protein concentrations were by trend slightly but insignificantly higher in the Fix ex group and slightly but significantly higher in the Nail group in comparison to the Sham group (p=0.014) (Figure 5A). The Nail+Therapy group exhibited slightly but significantly increased hepatic RANKL concentrations than in the Sham group (p=0.025). The RANKL serum concentrations were insignificantly altered, with some reduction in the Nail+Therapy group (Figure 5B). RANKL RNA expression was by trend, but insignificantly, enhanced post trauma in the Fix ex and Nail groups, which was abolished upon additional C5/CD14 inhibition (Figure 5C). These findings were associated with the limitation of a broad variance and small n-size in the therapy group.
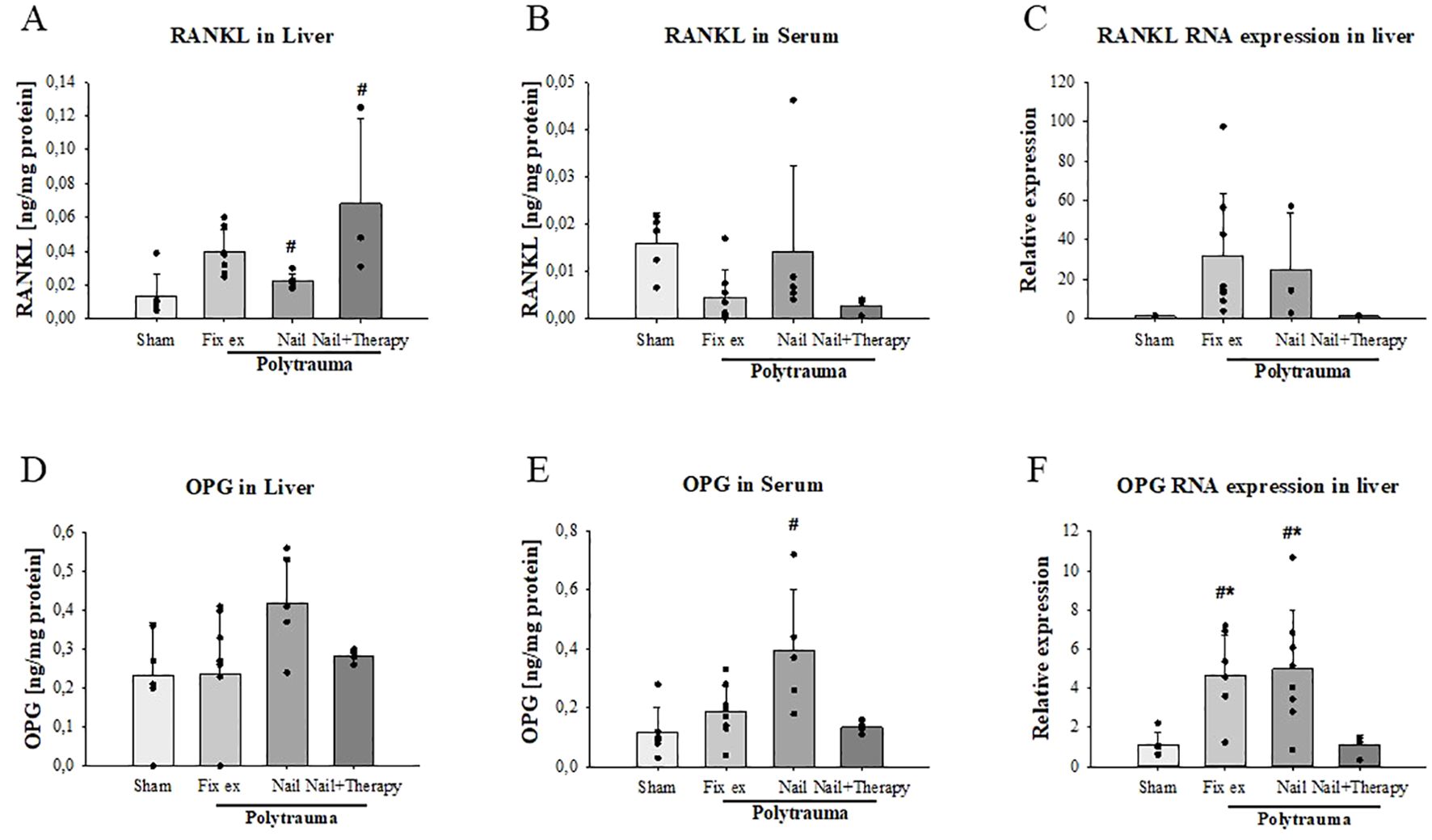
Figure 5. Reduction of polytrauma-induced changes in hepatic and systemic RANKL and OPG by inhibition of C5/CD14 in experimental polytrauma. RANKL and osteoprotegerin (OPG) serum protein concentration and hepatic RNA expression were determined 72 h after polytrauma in the indicated surgical treatment groups with and without immunomodulation of C5 and CD14. RANKL protein concentrations were determined by ELISA in liver tissue homogenates (A) and serum samples (B). RANKL RNA expression levels in liver homogenates (C). OPG protein concentrations in the liver (D) and serum (E) as well as OPG RNA expression in liver homogenates (F). Data satisfying a normal distribution were subjected to ANOVA, otherwise the rank sum test was applied. Data are shown as the mean ± SD; denotes significant differences vs. Sham group, * denotes significant differences vs Nail+Therapy group. Fix ex, external fixation; Nail, internal fixation with intramedullary nail; Nail+Therapy, intramedullary nail with combined C5/CD14 inhibitor treatment. Sham: n = 6, Fix ex: n = 8, Nail: n = 7, Nail+Therapy: n = 4.
Regarding OPG as a decoy receptor of RANKL, OPG protein was by trend higher in the liver (Figure 5D) and significantly higher in the serum after polytrauma and almost back to sham levels when comparing the Nail group in the absence versus the presence of the dual C5/CD14 inhibition (Figure 5E). With respect to the OPG RNA expression, the levels were approximately four times higher in the polytrauma groups without immunomodulation, but was abolished in the case of additional C5/CD14 blockade (Figure 5F).
4 Discussion
In the present study, a well-established, randomised, clinically relevant porcine polytrauma model with haemorrhagic shock was investigated (33). We focused on the liver-bone axis to investigate the effect of a dual immunomodulation.
The drug RA101295 (2-kDa peptide) given in the present study is a broad-spectrum C5 inhibitor. It is characterised by blocking C5 cleavage and the subsequent formation of anaphylatoxin C5a and terminal C5b–9, the latter found in two forms: sC5b–9 in the liquid phase and the MAC on the cell surface. C5a amplifies leukocyte activation and migration, induces basophil/mast cell degranulation, enhances vascular permeability, and therefore can induce all classical signs of inflammation (36). Blockade of the C5a-C5a receptor (C5aR)-interaction significantly improved IRI in the liver, characterised by the inhibition of platelet aggregation in the hepatic microcirculation and high mobility group box 1-release in the early stages of reperfusion. It also inhibited hepatocyte apoptosis by downregulating infiltrating macrophages and neutrophils, cytokine and chemokine release, and reactive oxygen species production (16). In a rodent study of distant organ damage after lower limb IRI, enhanced serum concentrations of lactate dehydrogenase (LDH), alanine transaminase (ALT) and AST and liver tissue tumor necrosis factor (TNF) were all reduced after application of a small peptide C5aR antagonist, indicating a key role of complement activation in the induction of remote liver damage (37). Mechanistically, C5a mediates leukocyte activation and migration during liver injury, ultimately leading to hepatocyte necrosis and apoptosis. In addition, the formed MAC can lyse target cells, promoting the release of further injury-associated molecular patterns, chemokines and other cytotoxins (16).
CD14 as a key molecule of TLRs has hardly been studied in polytrauma, as compared to complement. The TLRs are a major branch of innate immunity recognizing danger molecules, like the complement system. CD14 is a key player as co-receptors for several TLR molecules, in humans documented for TLR4 and TLR-2, and in mice for several others (21). Specific anti-CD14 mAbs to block porcine CD14 are scares. We found that mAb MIL-2 was promising in attenuating the cytokine response in E. coli sepsis (38). However, this antibody of the mouse subclass IgG2b showed some adverse effects due to the Fc part and we therefore genetically engineered a chimeric mouse-human mAb based on the human IgG2/4 Fc part (rMIL-2), avoiding complement activation and binding to Fc-receptors (31), which later have shown to be efficient in pig polymicrobial sepsis both with respect to morbidity and mortality (32). In a recent study, we demonstrated that a combined blockade of C5 and CD14 early in the posttraumatic course with an immune-monitoring-based real-time dose adjustment significantly reduced multiple organ damage (30). Focusing on the liver in the present study, as expected, we found enhanced liver damage scores including increased AST levels after PT surgically addressed by minimal invasive external fixation and even more by early total care with an intra-medullar femur nail. By contrast, a significant decrease of morphological and biochemical signs of liver damage and dysfunction was observed in this polytraumatized group after synchronic and combined C5/CD14 inhibition. Of note, in case of systemic AST concentrations, the immunomodulatory therapy was capable of reducing this liver injury marker in the early total care setting (Nail) to a level found in damage control surgery by the external fixateur (Fix ex), suggesting a promising therapeutic approach which could enable definitive treatment in combination with C5/CD14 inhibition.
Major trauma can cause compromised fracture repair. In a rat model of severe trauma, impaired fracture healing occurred in a surgical group with femoral osteotomy plus blunt thorax trauma compared to a group with femoral osteotomy alone (39). The acute systemic inflammatory response appears to alter the cellular composition and cytokine generation in the fracture haematoma, which substantially reduces bone formation and the mechanical competence of the fracture callus in the later stages of healing (40). On a cellular level, during the early inflammatory phase of fracture healing, C5aR was strongly expressed not only by immune cells, but also by osteoblasts, chondrocytes and osteoclasts in the intramembranous and chondrogenic zones of the fracture callus indicating a modulatory role of activated complement (41).
For fracture healing, the RANK-RANKL-OPG axis is important. Therefore, we investigated in the current study, to our knowledge, for the first time the liver expression of these mediators after PT and hemorrhagic shock (HS). In this context, we also addressed the effects of C5/CD14 inhibition on hepatic expression of these molecules. Our data revealed a consistent trend of enhanced RANK protein expression in the liver and serum 72 h post trauma. The RANKL/RANK interplay activates NF-kB in hepatocytes, leading to inflammatory cytokine production, Kupffer cell activation and increased fat storage (28). In a mouse model of liver IRI, serum RANKL concentrations were increased, peaking after 4 h, while OPG (as decoy receptor of RANKL) increased steadily over the observation period of 8 h after IRI. In the liver, RANK was constitutively expressed in hepatocytes and less in Kupffer cells. Of note, exogenous RANKL application revealed some protective liver effects after murine IRI (42). In our porcine model of polytrauma-induced liver injury, we found sustained protein generation of RANKL, RANK and OPG as late as 72 h post trauma. As a limitation of the study, we did not investigate the dynamics of the histological changes of the liver response. Even so, the C5/CD14 inhibition reduced the hepatic expression levels of RANK and OPG, effects that only can be speculated on to be somehow protective, because reduced OPG levels may lead to enhanced biological activity of RANKL in the liver. Activated T cells and other cells of the pro-inflammatory phenotype, including endothelial cells and lymphocytes are also major sources of RANKL (43, 44), which may contribute to the systemic response in addition to the postulated liver- bone communication. In a murine model of trauma with hemorrhagic shock reduced systemic RANKL levels in plasma at 24 and 72 hours post-trauma were measured, which aligns with our findings (45). However, it remains unclear why C5/CD14 blockade led to a reverse pattern concerning hepatic and systemic RANKL, and to what extent the polytrauma conditions or the various RANKL-generating cell types influence systemic RANKL levels. Further studies are needed to elucidate these mechanisms.
A liver-bone crosstalk was previously described in chronic liver diseases (46), for example, in hepatic osteodystrophy development (47). Despite its crucial function in bone remodelling, the role of the RANK-RANKL-OPG system (28) in the polytrauma pathophysiology remains unclear. In the present study, liver RANK of the PT Nail group was significantly higher than in the PT Nail+Therapy group. Furthermore, PCR results indicated higher OPG and RANKL gene expression in liver samples from the PT Nail group in comparison to the PT Nail+Therapy group.
Regarding the therapeutic approach, experimental studies have shown that inhibition of complement activation before the induction of liver injury results in hepatic protection, manifested by reduced inflammation and cell apoptosis (48–50). The liver is the major source of complement components. However, also osteoblasts can generate and activate complement proteins such as C3 and C5 (51–53). The complement cleavage products C3a and C5a have been shown to stimulate RANKL expression in osteoblasts, thereby increasing osteoclast formation (54). In vivo, increased osteoclast precursor cell recruitment to inflammatory sites may enhance the induction of osteoclast formation due to the chemotactic effects of the anaphylatoxins (55). Immunosuppression of C5a and C3a not only directly affects osteoclast formation, but also regulates osteoblast/osteoclast interactions via RANKL/OPG (54). However, it is noteworthy, that fracture repair also requires the involvement of the terminal complement complex (56). Through the current effective C5/CD14 inhibition, we reduced damaging effects of the inflammatory response on the liver, but at the same time, we also have inhibited C5b-9 generation and thereby potentially compromised bone and tissue regeneration and the clearance of damaged/infected cells (56, 57).
The innovation of the present study is the combined use of C5/CD14 inhibitors to alter inflammation and potentially key bone modulating factors in a clinically relevant long-term model of porcine polytrauma (observation period of 72 h). Through C5/CD14 double blockade, we found significant reduction of polytrauma-caused liver damage and inflammation, not only as assessed morphologically but also biochemically and on a transcription level. While the immunomodulatory approach revealed some protection on the posttraumatic liver response, it also significantly altered the hepatic and systemic RANK-RANKL-OPG axis.
5 Conclusion
In summary, we conclude:
I. I Experimental polytrauma leads to liver injury and hepatic modulation of RANKL, RANK, and OPG;
II. Seventy-two hours after trauma, the combined inhibition of C5/CD14 resulted in reduced polytrauma-induced liver injury and the hepatic generation of mediators, which can influence bone repair;
III. The consequences of the liver-bone-axis on fracture healing requires further investigation.
Data availability statement
The original contributions presented in the study are included in the article/Supplementary Material. Further inquiries can be directed to the corresponding author.
Ethics statement
The animal study was approved by Office for Nature, Environment and Consumer Protection of the State of North Rhine-Westphalia (LANUV AZ 81-02.04. 2020.A215). The study was conducted in accordance with the local legislation and institutional requirements.
Author contributions
YL: Conceptualization, Data curation, Investigation, Visualization, Writing – original draft, Writing – review & editing. KH: Conceptualization, Investigation, Methodology, Project administration, Resources, Supervision, Writing – review & editing. JG: Conceptualization, Investigation, Methodology, Project administration, Resources, Supervision, Writing – review & editing. ÜM: Investigation, Writing – review & editing. LL: Conceptualization, Data curation, Formal Analysis, Investigation, Methodology, Validation, Visualization, Writing – original draft, Writing – review & editing. AP: Supervision, Writing – review & editing. LD: Data curation, Formal Analysis, Investigation, Validation, Visualization, Writing – review & editing. QZ: Investigation, Writing – review & editing. XZ: Supervision, Validation, Writing – review & editing. RH: Supervision, Validation, Writing – review & editing. AI: Supervision, Validation, Writing – review & editing. IM: Supervision, Validation, Writing – review & editing. MG: Conceptualization, Investigation, Methodology, Validation, Writing – review & editing. EB: Data curation, Formal Analysis, Investigation, Methodology, Project administration, Resources, Supervision, Validation, Writing – review & editing, Conceptualization. FH: Conceptualization, Formal Analysis, Funding acquisition, Investigation, Methodology, Project administration, Resources, Supervision, Validation, Writing – review & editing. TM: Conceptualization, Methodology, Resources, Supervision, Writing – review & editing. MH-L: Conceptualization, Data curation, Formal Analysis, Funding acquisition, Methodology, Project administration, Resources, Supervision, Validation, Writing – original draft, Writing – review & editing.
Funding
The author(s) declare financial support was received for the research, authorship, and/or publication of this article. This research was supported by a grant from the German Research Foundation (DFG-Project U1- FOR5417). YL was supported by a grant from the China Scholarship Council (study number: 201906090385). RA101295 was kindly provided by UCB Pharmaceuticals (Brussels, Belgium). This project was also funded by the Deutsche Forschungsgemeinschaft (DFG) - project number 465409392.
Acknowledgments
The authors would like to thank the animal laboratory at the Aachen RWTH Hospital for their help. In addition, the authors would like to thank Anke Schultze, Bettina Berger, and Sonja Braumueller for their excellent assistance and technical support of the experiments. We thank Juchuan Liu, MD student in Internal Medicine II at the University of Ulm for statistical advice.
Conflict of interest
The authors declare that the research was conducted in the absence of any commercial or financial relationships that could be construed as a potential conflict of interest. The authors declared that they were an editorial board member of Frontiers, at the time of submission. This had no impact on the peer review process and the final decision.
Publisher’s note
All claims expressed in this article are solely those of the authors and do not necessarily represent those of their affiliated organizations, or those of the publisher, the editors and the reviewers. Any product that may be evaluated in this article, or claim that may be made by its manufacturer, is not guaranteed or endorsed by the publisher.
Supplementary material
The Supplementary Material for this article can be found online at: https://www.frontiersin.org/articles/10.3389/fimmu.2024.1434274/full#supplementary-material
Supplementary Table | List of applied primers.
Supplementary Figure | Concentration of the complement activation product C5a in liver tissue samples of the corresponding experimental groups: Sham: n = 6, Fix ex: n = 8, Nail: n = 7, Nail+Therapy: n = 4.
References
1. Paffrath T, Lefering R, Flohé S, DGU T. How to define severely injured patients?—An Injury Severity Score (ISS) based approach alone is not sufficient. Injury. (2014) 45:S64–S9. doi: 10.1016/j.injury.2014.08.020
2. Duvigneau JC, Kozlov AV, Zifko C, Postl A, Hartl RT, Miller I, et al. Reperfusion does not induce oxidative stress but sustained endoplasmic reticulum stress in livers of rats subjected to traumatic-hemorrhagic shock. Shock. (2010) 33:289–98. doi: 10.1097/SHK.0b013e3181aef322
3. Pape HC, Grimme K, Van Griensven M, Sott AH, Giannoudis P, Morley J, et al. Impact of intramedullary instrumentation versus damage control for femoral fractures on immunoinflammatory parameters: prospective randomized analysis by the EPOFF Study Group. J Trauma. (2003) 55:7–13. doi: 10.1097/01.TA.0000075787.69695.4E
4. Ciechanowicz D, Samojło N, Kozłowski J, Pakulski C, Żyluk A. Incidence and etiology of mortality in polytrauma patients: an analysis of material from Multitrauma Centre of the University Teaching Hospital no 1 in Szczecin, over a period of 3 years (2017–2019). Polish J Surgery. (2020) 92:1–6. doi: 10.5604/01.3001.0014.1127
5. McGhan LJ, Jaroszewski DE. The role of toll-like receptor-4 in the development of multi-organ failure following traumatic haemorrhagic shock and resuscitation. Injury. (2012) 43:129–36. doi: 10.1016/j.injury.2011.05.032
6. Zheng Y, Cui B, Sun W, Wang S, Huang X, Gao H, et al. Potential crosstalk between liver and extra-liver organs in mouse models of acute liver injury. Int J Biol Sci. (2020) 16:1166. doi: 10.7150/ijbs.41293
7. Bastian O, Pillay J, Alblas J, Leenen L, Koenderman L, Blokhuis T. Systemic inflammation and fracture healing. J leukocyte Biol. (2011) 89:669–73. doi: 10.1189/jlb.0810446
8. Horst K, Greven J, Lüken H, Zhi Q, Pfeifer R, Simon TP, et al. Trauma severity and its impact on local inflammation in extremity injury—Insights from a combined trauma model in pigs. Front Immunol. (2020) 10:3028. doi: 10.3389/fimmu.2019.03028
9. Groven RVM, Kuik C, Greven J, Mert U, Bouwman FG, Poeze M, et al. Fracture haematoma proteomics. Bone Joint Res. (2024) 13:214–25. doi: 10.1302/2046-3758.135.BJR-2023-0323.R1
10. Kaiser K, Prystaz K, Vikman A, Haffner-Luntzer M, Bergdolt S, Strauss G, et al. Pharmacological inhibition of IL-6 trans-signaling improves compromised fracture healing after severe trauma. Naunyn Schmiedebergs Arch Pharmacol. (2018) 391:523–36. doi: 10.1007/s00210-018-1483-7
11. Karladani AH, Granhed H, Karrholm J, Styf J. The influence of fracture etiology and type on fracture healing: a review of 104 consecutive tibial shaft fractures. Arch Orthop Trauma Surg. (2001) 121:325–8. doi: 10.1007/s004020000252
12. Kemmler J, Bindl R, McCook O, Wagner F, Groger M, Wagner K, et al. Exposure to 100% Oxygen abolishes the impairment of fracture healing after thoracic trauma. PloS One. (2015) 10:e0131194. doi: 10.1371/journal.pone.0131194
13. Vijay K. Toll-like receptors in immunity and inflammatory diseases: Past, present, and future. Int immunopharmacology. (2018) 59:391–412. doi: 10.1016/j.intimp.2018.03.002
14. Thorgersen EB, Barratt-Due A, Haugaa H, Harboe M, Pischke SE, Nilsson PH, et al. The role of complement in liver injury, regeneration, and transplantation. Hepatology. (2019) 70:725–36. doi: 10.1002/hep.30508
15. Fan X, McCullough RL, Huang E, Bellar A, Kim A, Poulsen KL, et al. Diagnostic and prognostic significance of complement in patients with alcohol-associated hepatitis. Hepatology. (2021) 73:983–97. doi: 10.1002/hep.31419
16. Kusakabe J, Hata K, Tamaki I, Tajima T, Miyauchi H, Wang Y, et al. Complement 5 inhibition ameliorates hepatic ischemia/reperfusion injury in mice, dominantly via the C5a-mediated cascade. Transplantation. (2020) 104:2065–77. doi: 10.1097/TP.0000000000003302
17. Qian X, Yang Z, Gao L, Liu Y, Yan J. The role of complement in the clinical course of hepatocellular carcinoma. Immunity Inflammation Disease. (2022) 10:e569. doi: 10.1002/iid3.v10.3
18. Cai C, Gill R, Eum H-A, Cao Z, Loughran PA, Darwiche S, et al. Complement factor 3 deficiency attenuates hemorrhagic shock-related hepatic injury and systemic inflammatory response syndrome. Am J Physiology-Regulatory Integr Comp Physiol. (2010) 299:R1175–R82. doi: 10.1152/ajpregu.00282.2010
19. Kholmukhamedov A, Czerny C, Hu J, Schwartz J, Zhong Z, Lemasters JJ. Minocycline and doxycycline, but not tetracycline, mitigate liver and kidney injury after hemorrhagic shock/resuscitation. Shock (Augusta Ga). (2014) 42:256. doi: 10.1097/SHK.0000000000000213
20. Dalle Lucca JJ, Li Y, Simovic M, Pusateri AE, Falabella M, Dubick MA, et al. Effects of C1 inhibitor on tissue damage in a porcine model of controlled hemorrhage. Shock. (2012) 38:82–91. doi: 10.1097/SHK.0b013e31825a3522
21. Lee CC, Avalos AM, Ploegh HL. Accessory molecules for Toll-like receptors and their function. Nat Rev Immunol. (2012) 12:168–79. doi: 10.1038/nri3151
22. Carrillo EH, Gordon L, Goode E, Davis E, Polk HC Jr. Early elevation of soluble CD14 may help identify trauma patients at high risk for infection. J Trauma. (2001) 50:810–6. doi: 10.1097/00005373-200105000-00006
23. Barratt-Due A, Pischke SE, Nilsson PH, Espevik T, Mollnes TE. Dual inhibition of complement and Toll-like receptors as a novel approach to treat inflammatory diseases—C3 or C5 emerge together with CD14 as promising targets. J leukocyte Biol. (2017) 101:193–204. doi: 10.1189/jlb.3VMR0316-132R
24. He XF, Zhang L, Zhang CH, Zhao CR, Li H, Zhang LF, et al. Berberine alleviates oxidative stress in rats with osteoporosis through receptor activator of NF-kB/receptor activator of NF-kB ligand/osteoprotegerin (RANK/RANKL/OPG) pathway. Bosn J Basic Med Sci. (2017) 17:295–301. doi: 10.17305/bjbms.2017.2596
25. Tyrovola JB. The “Mechanostat” Principle and the osteoprotegerin-OPG/RANKL/RANK system PART II. The role of the hypothalamic-pituitary axis. J Cell Biochem. (2017) 118:962–6. doi: 10.1002/jcb.v118.5
26. Guerrini MM, Takayanagi H. The immune system, bone and RANKL. Arch Biochem Biophys. (2014) 561:118–23. doi: 10.1016/j.abb.2014.06.003
27. Monti F, Perazza F, Leoni L, Stefanini B, Ferri S, Tovoli F, et al. RANK-RANKL-OPG axis in MASLD: current evidence linking bone and liver diseases and future perspectives. Int J Mol Sci. (2024) 25:9193. doi: 10.3390/ijms25179193
28. Kiechl S, Wittmann J, Giaccari A, Knoflach M, Willeit P, Bozec A, et al. Blockade of receptor activator of nuclear factor-κB (RANKL) signaling improves hepatic insulin resistance and prevents development of diabetes mellitus. Nat Med. (2013) 19:358–63. doi: 10.1038/nm.3084
29. Lorenzo J. The many ways of osteoclast activation. J Clin Invest. (2017) 127:2530–2. doi: 10.1172/JCI94606
30. Lupu L, Horst K, Greven J, Mert Ü, Ludviksen JA, Pettersen K, et al. Simultaneous C5 and CD14 inhibition limits inflammation and organ dysfunction in pig polytrauma. Front Immunol. (2022) 13:952267. doi: 10.3389/fimmu.2022.952267
31. Lau C, Gunnarsen KS, Hoydahl LS, Andersen JT, Berntzen G, Pharo A, et al. Chimeric anti-CD14 IGG2/4 Hybrid antibodies for therapeutic intervention in pig and human models of inflammation. J Immunol. (2013) 191:4769–77. doi: 10.4049/jimmunol.1301653
32. Skjeflo EW, Sagatun C, Dybwik K, Aam S, Urving SH, Nunn MA, et al. Combined inhibition of complement and CD14 improved outcome in porcine polymicrobial sepsis. Crit Care. (2015) 19:415. doi: 10.1186/s13054-015-1129-9
33. Horst K, Simon TP, Pfeifer R, Teuben M, Almahmoud K, Zhi Q, et al. Characterization of blunt chest trauma in a long-term porcine model of severe multiple trauma. Sci Rep. (2016) 6:1–13. doi: 10.1038/srep39659
34. Islam R, Islam MM, Nilsson PH, Mohlin C, Hagen KT, Paschalis EI, et al. Combined blockade of complement C5 and TLR co-receptor CD14 synergistically inhibits pig-to-human corneal xenograft induced innate inflammatory responses. Acta Biomaterialia. (2021) 127:169–79. doi: 10.1016/j.actbio.2021.03.047
35. Schmittgen TD, Livak KJ. Analyzing real-time PCR data by the comparative CT method. Nat Protoc. (2008) 3:1101–8. doi: 10.1038/nprot.2008.73
36. Sarma JV, Ward PA. New developments in C5a receptor signaling. Cell Health Cytoskeleton. (2012) 4:73. doi: 10.2147/CHC.S27233
37. Woodruff TM, Arumugam TV, Shiels IA, Reid RC, Fairlie DP, Taylor SM. Protective effects of a potent C5a receptor antagonist on experimental acute limb ischemia-reperfusion in rats. J Surg Res. (2004) 116:81–90. doi: 10.1016/j.jss.2003.04.001
38. Thorgersen EB, Pischke SE, Barratt-Due A, Fure H, Lindstad JK, Pharo A, et al. Systemic CD14 inhibition attenuates organ inflammation in porcine Escherichia coli sepsis. Infect Immun. (2013) 81:3173–81. doi: 10.1128/IAI.00390-13
39. Recknagel S, Bindl R, Brochhausen C, Göckelmann M, Wehner T, Schoengraf P, et al. Systemic inflammation induced by a thoracic trauma alters the cellular composition of the early fracture callus. J Trauma Acute Care Surgery. (2013) 74:531–7. doi: 10.1097/TA.0b013e318278956d
40. Recknagel S, Bindl R, Kurz J, Wehner T, Ehrnthaller C, Knöferl MW, et al. Experimental blunt chest trauma impairs fracture healing in rats. J Orthopaedic Res. (2011) 29:734–9. doi: 10.1002/jor.21299
41. Ignatius A, Ehrnthaller C, Brenner RE, Kreja L, Schoengraf P, Lisson P, et al. The anaphylatoxin receptor C5aR is present during fracture healing in rats and mediates osteoblast migration in vitro. J Trauma. (2011) 71:952. doi: 10.1097/TA.0b013e3181f8aa2d
42. Sakai N, Van Sweringen HL, Schuster R, Blanchard J, Burns JM, Tevar AD, et al. Receptor activator of nuclear factor-κB ligand (RANKL) protects against hepatic ischemia/reperfusion injury in mice. Hepatology. (2012) 55:888–97. doi: 10.1002/hep.24756
43. Kawai T, Matsuyama T, Hosokawa Y, Makihira S, Seki M, Karimbux NY, et al. B and T lymphocytes are the primary sources of RANKL in the bone resorptive lesion of periodontal disease. Am J pathology. (2006) 169:987–98. doi: 10.2353/ajpath.2006.060180
44. Collin-Osdoby P, Rothe L, Anderson F, Nelson M, Maloney W, Osdoby P. Receptor activator of NF-κB and osteoprotegerin expression by human microvascular endothelial cells, regulation by inflammatory cytokines, and role in human osteoclastogenesis. J Biol Chem. (2001) 276:20659–72. doi: 10.1074/jbc.M010153200
45. Neunaber C, Yesilkaya P, Putz C, Krettek C, Hildebrand F. Differentiation of osteoprogenitor cells is affected by trauma-haemorrhage. Injury. (2013) 44:1279–84. doi: 10.1016/j.injury.2013.05.011
46. Wang B, Xu H, Li J, Gao HM, Xing YH, Lin Z, et al. Complement depletion with cobra venom factor alleviates acute hepatic injury induced by ischemia−reperfusion. Mol Med Rep. (2018) 18:4523–9. doi: 10.3892/mmr.2018.9484
47. Lu K, Shi T-S, Shen S-Y, Shi Y, Gao H-L, Wu J, et al. Defects in a liver-bone axis contribute to hepatic osteodystrophy disease progression. Cell Metab. (2022) 34:441–57.e7. doi: 10.1016/j.cmet.2022.02.006
48. Arumugam TV, Woodruff TM, Stocks SZ, Proctor LM, Pollitt S, Shiels IA, et al. Protective effect of a human C5a receptor antagonist against hepatic ischaemia-reperfusion injury in rats. J hepatology. (2004) 40:934–41. doi: 10.1016/j.jhep.2004.02.017
49. Marshall KM, He S, Zhong Z, Atkinson C, Tomlinson S. Dissecting the complement pathway in hepatic injury and regeneration with a novel protective strategy. J Exp Med. (2014) 211:1793–805. doi: 10.1084/jem.20131902
50. He S, Atkinson C, Qiao F, Cianflone K, Chen X, Tomlinson S. A complement-dependent balance between hepatic ischemia/reperfusion injury and liver regeneration in mice. J Clin Invest. (2009) 119:2304–16. doi: 10.1172/JCI38289
51. Sato T, Hong MH, Jin CH, Ishimi Y, Udagawa N, Shinki T, et al. The specific production of the third component of complement by osteoblastic cells treated with 1α, 25-dihydroxyvitamin D3. FEBS letters. (1991) 285:21–4. doi: 10.1016/0014-5793(91)80715-F
52. Sato T, Abe E, Jin CH, Hong M, Katagiri T, Kinoshita T, et al. The biological roles of the third component of complement in osteoclast formation. Endocrinology. (1993) 133:397–404. doi: 10.1210/endo.133.1.8319587
53. Jin CH, Shinki T, Hong M, Sato T, Yamaguchi A, Ikeda T, et al. 1 alpha, 25-dihydroxyvitamin D3 regulates in vivo production of the third component of complement (C3) in bone. Endocrinology. (1992) 131:2468–75. doi: 10.1210/endo.131.5.1425444
54. Ignatius A, Schoengraf P, Kreja L, Liedert A, Recknagel S, Kandert S, et al. Complement C3a and C5a modulate osteoclast formation and inflammatory response of osteoblasts in synergism with IL-1β. J Cell Biochem. (2011) 112:2594–605. doi: 10.1002/jcb.v112.9
55. Flierl MA, Schreiber H, Huber-Lang MS. The role of complement, C5a and its receptors in sepsis and multiorgan dysfunction syndrome. J Invest Surgery. (2006) 19:255–65. doi: 10.1080/08941930600778263
56. Mödinger Y, Rapp AE, Vikman A, Ren Z, Fischer V, Bergdolt S, et al. Reduced terminal complement complex formation in mice manifests in low bone mass and impaired fracture healing. Am J Pathology. (2019) 189:147–61. doi: 10.1016/j.ajpath.2018.09.011
Keywords: polytrauma, RANK-RANKL-OPG pathway, immunomodulation, complement, CD14
Citation: Li Y, Horst K, Greven J, Mert Ü, Lupu L, Palmer A, Doerfer L, Zhao Q, Zhang X, Halbgebauer R, Ignatius A, Marzi I, van Griensven M, Balmayor E, Hildebrand F, Mollnes TE and Huber-Lang M (2024) Modulation of the hepatic RANK-RANKL-OPG axis by combined C5 and CD14 inhibition in a long-term polytrauma model. Front. Immunol. 15:1434274. doi: 10.3389/fimmu.2024.1434274
Received: 17 May 2024; Accepted: 30 October 2024;
Published: 21 November 2024.
Edited by:
Hui-Rong Jiang, University of Strathclyde, United KingdomReviewed by:
Olha Lisakovska, Palladin Institute of Biochemistry, NAS, UkraineXinhua Shu, Glasgow Caledonian University, United Kingdom
Copyright © 2024 Li, Horst, Greven, Mert, Lupu, Palmer, Doerfer, Zhao, Zhang, Halbgebauer, Ignatius, Marzi, van Griensven, Balmayor, Hildebrand, Mollnes and Huber-Lang. This is an open-access article distributed under the terms of the Creative Commons Attribution License (CC BY). The use, distribution or reproduction in other forums is permitted, provided the original author(s) and the copyright owner(s) are credited and that the original publication in this journal is cited, in accordance with accepted academic practice. No use, distribution or reproduction is permitted which does not comply with these terms.
*Correspondence: Markus Huber-Lang, TWFya3VzLkh1YmVyLUxhbmdAdW5pa2xpbmlrLXVsbS5kZQ==