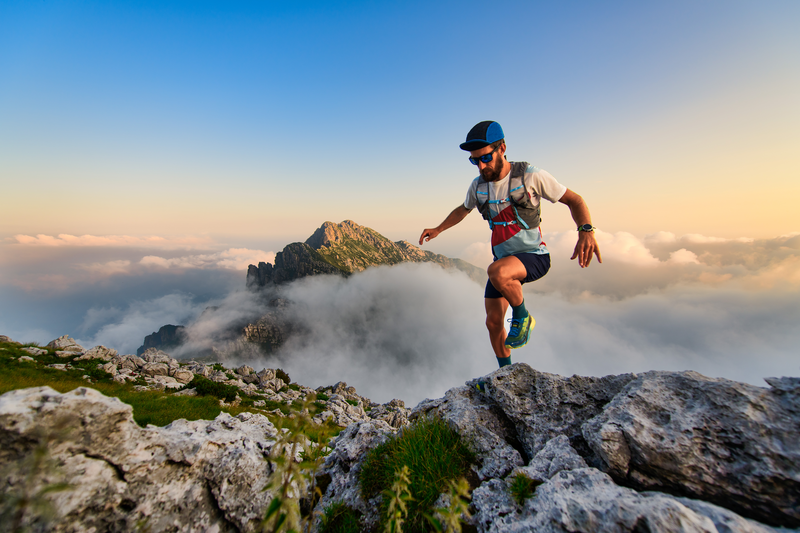
94% of researchers rate our articles as excellent or good
Learn more about the work of our research integrity team to safeguard the quality of each article we publish.
Find out more
PERSPECTIVE article
Front. Immunol. , 08 July 2024
Sec. Cancer Immunity and Immunotherapy
Volume 15 - 2024 | https://doi.org/10.3389/fimmu.2024.1424081
This article is part of the Research Topic Extracellular Vesicles for Immunotherapy View all 9 articles
Exosomes are found in various tissues of the body and carry abundant contents including nucleic acids, proteins, and metabolites, which continuously flow between cells of various tissues and mediate important intercellular communication. In addition, exosomes from different cellular sources possess different physiopathological immunomodulatory effects, which are closely related to the immune regeneration of normal or abnormal organs and tissues. Here, we focus on the mechanistic interactions between exosomes and the human immune system, introduce the immuno-regenerative therapeutic potential of exosomes in common clinical immune-related diseases, such as infectious diseases, autoimmune diseases, and tumors, and reveal the safety and efficacy of exosomes as a novel cell-free immune regenerative therapy.
Extracellular vesicle (EV) is a general term for various types of vesicles secreted by all cells through membrane movement and contain various bioactive substances, including metabolic molecules such as proteins and lipids, and nucleic acids such as DNA and RNA (miRNA, circRNA, lncRNA) that contain genomic information. Specifically, the term exosome refers specifically to an endomembrane-derived intraluminal vesicle (ILV) that forms during the maturation of the multivesicular endosomal system (MEV) by inward fusion (1). These small-diameter vesicles have phospholipid bilayers with the same topological structure with appearance of elliptical or spherical, and will express molecules including CD63, CD81, CD9, Tspan8, compatibility complex (MHCIorII) and heat shock protein family (HSC70,HSP90), which are used as characteristic markers for identifying exosomes (2). According to the data of ExoCarta, as of Jan. 1, 2024, many exosome researches have been discovered and uploaded, including 9769 kinds of proteins, 3408 kinds of mRNAs, 2838 kinds of miRNAs and 1116 kinds of lipid molecules (3). Exosomes are not just a means for cells to renew themselves, but as an additional communication mechanism within and between cells, which participates in a large number of physiological and pathological changes closely related to clinical processes (4). Exosomes can persist in various body fluids besides peripheral blood, including but not limited to saliva, ascites, urine, cerebrospinal fluid, breast milk, etc. (Figure 1A) Therefore, as a cell replacement therapy, vesicles and its engineering derivatives have achieved breakthrough development in recent years, depending on its unique natural advantages, and have been applied to the diagnosis and treatment of clinical diseases, including cancer (5–7), neurological diseases (8–10), cardiovascular diseases (11) and Inflammatory disease (12). (Figure 1B) It is worth noting that engineered vesicle therapy regimens also seem to be making promising progress in the immune field.
Immunotherapy for infectious diseases has become the focus again in recent years: the prevention and treatment of infectious disease like COVID-19. Over the past four years, the novel severe respiratory syndrome coronavirus type 2(SARS-CoV-2), which was born in 2019, has attracted widespread global public attention as a public health issue. Since the epidemic of the BA.5 variant in 2022, the coronavirus outbreak appears to have enjoyed a calmer period. However, data from a variety of sources suggest that we have entered a new wave of covid (13). Excitingly, the alternative strategy of another soluble receptor decoy, which possesses the advantage of ultra-breadth in maintaining neutralization ability against multiple variants of viruses, is expected to be a key strategy for solving the zoonotic coronavirus pandemic challenges in the future (14). There have been some well-established studies on the antiviral applications of engineered ACE2 decoy receptors, and their application in combination with EVs opens up new ideas for achieving superior multifunctional antiviral therapeutic effects. EVs carrying expressed ACE2 protein were extracted and characterized from a human lung epithelial cell line by Cocozza et al. Through in vitro antiviral assays, it was demonstrated that overexpression of full-length ACE2-EV and ACE2-TMPRSS2(Transmembrane protease, serine 2)-EV possessed significant viral inhibitory effects, equivalent to 500-1500-fold soluble recombinant ACE2 levels (15). ACE2 and palmitoyl secretion interact with each other through a strict mechanism; in other words, the presence of palmitoylation directly affects the strength of membrane-anchored ACE2 expression. Meanwhile, EVs containing ACE2 isolated from human plasma or cells have been shown to have a prophylactic capacity against severe acute SARS-CoV-2 that has been assessed to be 60- to 80-fold greater than that of vesiculation-free recombinant ACE2 (16). Moreover, the easily modifiable properties of EVs in terms of structure and function provide great potential for therapeutic performance enhancement, such as affinity and neutralization, of nano-neutralization devices carrying ACE2. Xie and his team used engineering to palmitoylate EVs carrying ACE2 to achieve a breakthrough in affinity for the S protein, as well as a broader neutralization of the virus in the host (17). This novel nano-delivery platform with palmitoylation as the core technology endows natural soluble ACE2 with superior half-life and in vivo stability (18). Kim et al. successfully constructed an engineered extracellular vesicular variant of sACE2 (sACE2.v1) using the CD9ΔTM4 scaffold and demonstrated its ability to cope with the infectious challenge of the WT, D614G, beta- and delta-variant strains in mice, exerting a significant protective effect. CD9ΔTM4 is a truncated form of CD9, serving as a scaffold whose C-terminus forms a splice with SACE2, and overexpression of the scaffold enables overpacking and functionalization of the fusion protein (19). Interestingly, in addition to the good progress in antiviral studies of soluble ACE2 with EVs, this research paradigm seems to be equally valid when applied to the structural domains of the viruses themselves that bind to ACE2, considering the binding of the S proteins to the host’s natural ACE2 receptor as a key prerequisite for viral invasion (20). In cellular experiments, EVs carrying viral spiny proteins were found to be significantly negatively correlated in a dose-dependent manner with antibody-mediated virus neutralization (21). Fu and colleagues successfully doped the receptor-binding domains (RBD) in the spike glycoprotein of SARS-CoV-2 onto extracellular vesicle membranes, and these RBD-labeled EVs were highly specifically targeted to ACE2-enriched tissues of the heart, lungs, and kidneys which were major organs infested by viruses and resulted in significant reductions of viral loads (22). In the field of vaccine development, a novel new crown candidate vaccine based on bacterial outer membrane vesicles (OMV), which is also coupled to the RBD of the viral spike, was shown to be protective in an intranasal inoculation model in mice, and retained better cross-neutralizing activity against WT and Delta for at least 35 days (23). In addition, lung-derived EV mounted messenger ribonucleic acid encoding viral spiking proteins has also been developed as a good room-temperature-stable vaccine product, also delivered by inhalation, which triggers an even better antibody secretion response (24). These findings demonstrate the versatility of the EVs delivery system in therapeutic form, somewhat circumventing the limitations of lung barrier utilization.
The therapeutic potential of EVs is not limited to their use as drug nanocarriers, and their physiological and pathological properties dictate that they play an important role in the process of tissue injury and inflammation, which is evidence for the source of their therapeutic potential in the treatment of SARS-CoV-2: to fight against COVID-19 by mitigating the pathological process of damage to lungs as the main infectious organ. Therefore, given the existence of natural protection of EVs for soluble ACE2 and the favorable properties of its derived therapeutic strategies, such as cell-free, immunologically safe, easily engineered, as well as multi-targeted therapeutic potential conferred by its lipophilicity and high clinical translational value (25, 26), the strategic model of doping ACE2 decoy receptors to antagonize COVID-19 will be of great value to explore.
Mesenchymal stem cells (MSCs) are found in a variety of tissues in the human body and serve as multifunctional stem cells with renewal capacity and differentiation potential (27). A number of studies have shown that the immunomodulatory abilities of MSCs, including inhibition of inflammation progression, promotion of tissue repair, and resistance to pathogenic infections, etc., on immune effector cells as NK cells, B lymphocytes and T lymphocytes, are by means of the paracrine pathway, in which the secretion and communication of exosomes are the key parts (28–30). The treatment of autoimmune diseases has been controversial, and there are no recognized effective technological protocols. However, based on the positive modulatory effects of MSCs-derived exosomes on immunoreactive substances, several studies suggest that this novel cell-free therapy may be a powerful strategy against autoimmune diseases. Multiple sclerosis is the most common dysfunctional autoimmune disease with inflammatory involvement of the central nervous system (31). Microglia play a major immune role in the CNS, on the one hand their neuro-destructive role, represented by the M1 phenotype, and their anti-infective function against invading pathogens. At the same time, the M2 phenotype is responsible for inhibiting excessive pro-inflammatory responses to protect neurons from damage (32). In a study by Zhang et al, increasing the ratio of microglia M2 and M1 phenotypes by MSCs-derived exosomes adjustment was able to suppress neuroinflammatory progression in an animal model of experimental autoimmune encephalomyelitis (EAE). This combined direct and indirect immunomodulatory model significantly ameliorated neurodegenerative progression and optimized cognitive function compared to controls (33). For the powerful therapeutic role of MSCs-derived exosomes in EAE models, Riazifar’s team found that IFNγ-Exo was able to reduce the levels of multiple pro-inflammatory mediators in vitro, including Th1, IL-6, IL-22, and others. Meanwhile, in-depth characterization of exosomes, whose contents contain anti-inflammatory RNAs and a variety of protective neurological anti-inflammatory proteins, provides a molecular evidence base for their therapeutic mechanism (34). In addition, MSC-derived exosomes also have a new and safe prospect in the clinical treatment of Systemic Lupus Erythematosus (SLE). SLE, as a typical chronic autoimmune disease, has a complex and unknown pathogenesis and involved damaged organs, and nephritis is the main cause of disease and death of SLE patients at present (35). Therefore, the treatment of nephritis in patients with SLE is a key aspect of clinical suppression of disease progression. A growing body of research suggests that MSCs are a promising intervention strategy in the immunotherapy of lupus-like diseases. Transplantation of bone marrow-derived MSCs in animal models of lupus significantly reduced the abnormal levels of serum anti-double-stranded DNA antibodies and renal metabolites, which in turn induced potent positive immunomodulatory effects to alleviate the progression of lupus nephritis (36). A clinical trial in patients with drug-resistant systemic lupus erythematosus used a therapeutic strategy of allogeneic mesenchymal stem cell transplantation and followed 87 enrolled patients for an average duration of 27 months. The results showed significant remission of disease activity and clinical symptoms and improved organ function, reflected in an overall survival rate of up to 94%, confirming the excellent long-term safety and efficacy of this promising cell therapy (37). The above evidence suggests a promising application of MSCs in the clinical management of SLE patients, but there are still great challenges in terms of cost and regulation for their further dissemination and application. Interestingly, MSC-derived exosomes are the mainstay of the immunomodulatory effects of this therapy. Therefore, it is not difficult to arrive at the view that MSCs-derived exosomes, a novel cell-free therapy, is expected to break all the limitations in the future for the promotion and application of stem cell therapies in human SLE.
As mentioned previously, MSCs-derived exosomes exert immunomodulatory effects through communication interactions with immune cells, further influencing the expression levels of immunoreactive molecules, and this multifunctional modulatory ability to act simultaneously on both the natural and adaptive immune systems may be able to partially explain their strong therapeutic potential. Macrophages occupy an important position in the innate immune system and can be categorized into M1 pro-inflammatory and M2 anti-inflammatory phenotypes. Recent studies have shown that the anti-inflammatory effects of MSCs-derived exosomes are closely related to macrophage polarization, as evidenced by inhibition of M1 activation and induction of M2 immunosuppressive phenotype (38). Further, reduction of in vivo levels of immunoreactive substances such as IL22 and Th17, along with up-regulation of IL10, ultimately led to inflammatory suppression in macrophages (39, 40). In addition, MSCs-derived exosomes all possess the ability to inhibit the activation and proliferation processes of natural immune cells, such as NK and DC, thus exerting immunosuppressive effects and attenuating toxic inflammatory responses (41, 42). On the other hand, as the main immune cells of the adaptive immune system, both B cells and T cells were regulated by MSCs-derived exosomes, which in turn affected the functions of humoral and cellular immunity. Antibody secretion, activation, and proliferation of B cells were inhibited by MSCs, and interestingly, this regulatory effect could be perfectly inherited by MSCs-derived exosomes (43). At the same time, the secretion level of regulatory B cells, an important functional subpopulation, was significantly upregulated by exosomes, resulting in the release of large amounts of anti-inflammatory factors such as IL-10 (44). T cells are important participating effector immune cells in autoimmune diseases. Exosomes alleviate the progression of most autoimmune diseases by inhibiting T cell activation and proliferation. However, its role in regulating the proportion of helper T cells of different phenotypes is also crucial. The dynamic balance between Th1 and Th2 is closely related to the activation or suppression of inflammation. In addition, the increase in the proportion of Tregs and the induction of apoptosis in activated T cells also make an important contribution to disease prevention and delay in progression (45, 46).
Cancer is a major threat to human life worldwide and has become a crucial challenge that hinders progress in average life expectancy and the maintenance of health (47). One of the main factors that make it difficult to control the number of cancer cases is the lack of potent screening tools for prevention (48). Precision medicine is advancing clinical research based on new biomarkers, with an emphasis on individualized treatment plans for patients (49). It is noted that the importance of intercellular communication of exosomes is not only reflected in physiological regulation, but also in strongly affecting the pathological progress of cells, tissues, organs, and even the biological behavior of tumors, including but not limited to cancer’s origin, metastasis and outcome (50) (Figure 1C). We have previously presented a detailed review of exosomes in immunotherapy of tumors, and we will not repeat it here, but mainly focus on the immunodiagnosis of exosomes in tumors (51).
Figure 1 (A) Exosomes are widely present in the human body and can be extracted from a variety of body fluids, such as: tears, saliva, milk, blood, urine, etc. (B) Exosomes are closely associated with the physiological and pathological activities of the human body, and are involved in the process of immunoregulation in a variety of diseases, like cancers and autoimmune disorders. (C) Nucleic acids, proteins, metabolites and other components contained in exosomes extracted from human blood circulation have great potential to assist in the clinical diagnosis, process analysis and prognosis prediction of tumors and other diseases.
As mentioned earlier, exosome contents are extremely rich, containing a variety of nucleic acids, proteins, metabolites, and other substances that harbor a wealth of genetic information and homeostatic changes. These complex substances and information are valuable tools for overcoming the difficulties of heterogeneous tumor diagnostic barriers. Firstly, exosomes can be potential biomarkers in cancer diagnosis and surveillance for high-risk metastasis. It has been well recognized that the detection of circulating miRNAs transported from cells can more precisely reflect the real-time dynamics of cancer cells for clinical diagnosis. Zhou et al. used Exiqon panel to analyze the miRNA expression profiles of plasma from lung adenocarcinoma(LA) patients and used reverse transcription-polymerase chain reaction (qRT-PCR) for further screening and validation, and successfully identified six miRNA groups that can distinguish LA patients from healthy control individuals by their dysregulation, providing a potential biomarker option for LA detection in Asian populations (52). The diagnostic assistance of extracellular vesicles in lung cancer is being validated in parallel in clinical trials (NCT04529915, NCT03830619). In addition to microRNA, long-stranded RNA in plasma is also a potential biomarker for predicting early cancer. The panel of serum exosomal 1 lncRNA, and 2 mRNA (BCAR4 and MAGEA3,KRTAP5-4) was shown to have unique predictive power in CRC (53). It has been reported that exosomal lnc PTENP1 in tissues and plasma of bladder cancer patients exhibits a strong correlation with tumor pathological features such as tumor morphology, size and weight, and maintains a significant negative correlation with clinical grade of the disease,(P < 0.05). Mechanistically, healthy cells secrete the exosome PTENPI to establish a pathological link with bladder cancer cells and protect PTEN by crosstalk with miR-17, and eventually the tumor malignant invasive behavior is oppressed. Therefore, its ability to frequently silence tumor cell lines allows it to be considered as a tumor suppressor (54). Secondly, different exosomal-biomakers can reflect the real-time treatment feedbacks and drug resistance detection of tumor. The complex dynamics of the tumor microenvironment is determined by the highly heterogeneous and mutagenic nature of cancer cells, whereas exosome biopsies are able to break through spatial and temporal limitations to systematically track dynamic changes in the heterogeneity of almost all cancers and influence the epigenetic characteristics and pathological properties of tumor cells through different regulatory mechanisms during the different clinical stages of conventional treatment of cancer patients (55). Circulating exosomal contents are stripped from primary tissue. Thus, it can serve as an easily accessible biomarker to assess patients’ clinical response to surgery, drugs and radiation therapy (56). Radioresistance is an important obstacle preventing NSCLC patients from achieving full therapeutic benefit. Extracellular miR-1246 was found to significantly inhibit the proliferation of lung cancer lineage tumor cells, while the radiosensitivity of tumor cells in miR-1246 knockdown models was enhanced, demonstrating the potential of in vivo miRNAs to regulate the sensitivity of lung cancer cells to radiation therapy (57). In addition, Alba et al. revealed a correlation between exosomal miRNA expression levels and breast cancer progression. The results confirmed significant differences in miR-21 expression levels between patients with localized and distant metastases of breast cancer(P=0.027), which corroborates the speculation that exosomal miRNAs can be used to predict risk stratification associated with cancer recurrence and metastasis (58). Since exosomes are highly consistent with cancer cells in terms of genetic heterogeneity, they can present comprehensive and macroscopic information as a whole, and also enable precise classification and screening of cell populations with different epigenetic characteristics at the microscopic level. The above results indicate that it is practical to reflect the health status of the organism by detecting the biological information contained in exosomes. In recent years, an increasing number of reports have shown that exosomes hold great promise in the field of clinical diagnosis.
However, there are a number of fundamental and translational issues that need to be considered and addressed before EV therapies can be applied to humans on a large scale. Firstly, in the optimization and screening of EV-sACE2 engineered samples, the relationship between ACE2 loading and inhibitory potency remains controversial in different research reports. In contrast to the generally accepted result that increasing ACE2 loading significantly improves neutralization, improving antiviral performance by increasing ACE2 loading in a certain population of vesicles of a certain size may not be satisfactorily rewarded. For example, in Gunnels’ study, there was no significant difference in potency of specific ACE2 between different types of loaded vesicles, which contradicts the conclusion that ACE2 loading is positively correlated with potency as reported by Xie et al. (17, 59). Therefore, the means of EV purification and size type should be included in platform design considerations to exclude their possible unintended effects on performance. The design of exosome targeting optimization is challenging and some rational targeting strategies have potential. For example, the chemical structure of exosomes can be optimized to increase their permeability and retention and reduce clearance. In addition, investigating more bioactive nanocoated materials to improve exosome targeting and utilization are important design factors (60). In addition, there is no standardized consensus among the many different studies and companies on protocols for isolation and characterization of EVs, which makes it difficult to ultimately reach a consistent concentration and pattern of EVs even when they are derived from the same tissue or cell. For instance, even the most classical differential ultracentrifugation scheme suffers from low productivity and operator randomness for EV separation applications (61). Therefore, a universal economic Good Manufacturing Practices (GMP) guideline is exactly what is urgently needed by research and industry. Finally, given the heterogeneity that exists in different diseases EV therapies need to determine the optimal dose and route of administration to match the specific disease. Specific clinical guidance on exosomes, a novel cell-free therapy, is not yet available in Asia, Europe or the United States, but it is expected that their origin and function will determine the type of regulatory framework to which they are subject (62). In the validation of lung injury models, both the intravenous and inhalation routes possessed better therapeutic efficacy, but the latter may have better organ local targeting in order to seek to achieve precise high-concentration delivery to localized lesions.
Overall, novel EV-based alternative therapies hold good promise in dealing with treatment and diagnosis of clinical immune diseases like viral infections (COVID), autoimmune diseases and cancers because of their excellent maneuverability and safe action properties that penetrate deep into pathophysiological mechanisms. It is foreseeable that EV therapeutics will be a powerful immune weapon for human beings to cope with clinical diseases, after determining the optimal safe pathway for preparation, characterization and application.
The original contributions presented in the study are included in the article/supplementary material. Further inquiries can be directed to the corresponding authors.
GL: Writing – original draft, Writing – review & editing. SZ: Resources, Writing – review & editing. YZ: Investigation, Writing – review & editing. HA: Data curation, Writing – review & editing. XZ: Investigation, Writing – review & editing. KQ: Formal analysis, Writing – review & editing. CL: Writing – review & editing. WF: Writing – review & editing.
The author(s) declare financial support was received for the research, authorship, and/or publication of this article. This study was supported by the National Natural Science Foundation of China (grant numbers 82322055, 82272792, 31970882, 81903140, 82041012 and 92169115); the Shanghai Rising-Star Program (grant number 23QA1405800); The Shanghai Outstanding Academic Leader Program (grant number 23XD1424800); the Shanghai Chenguang Program (grant number 17CG35); the Shanghai Biomedical Technology Support Project (20S11906600) and the Shanghai Key Laboratory of Cell Engineering (14DZ2272300).
The authors declare that the research was conducted in the absence of any commercial or financial relationships that could be construed as a potential conflict of interest.
All claims expressed in this article are solely those of the authors and do not necessarily represent those of their affiliated organizations, or those of the publisher, the editors and the reviewers. Any product that may be evaluated in this article, or claim that may be made by its manufacturer, is not guaranteed or endorsed by the publisher.
ACE2, Angiotensin-converting enzyme 2; EV, Extracellular vesicle; miRNA, MicroRNA; lncRNA, Long non-coding RNA; SARS-CoV-2, severe respiratory syndrome coronavirus type 2; TMPRSS2, Transmembrane protease, serine 2; RBD, receptor-binding domains; OMV, outer membrane vesicles; MSCs, Mesenchymal stem cells ; EAE, experimental autoimmune encephalomyelitis; SLE, Systemic Lupus Erythematosus; LA, lung adenocarcinoma; NSCLC, non-small cell lung cancer; GMP, Good Manufacturing Practices.
1. Raposo G, Stoorvogel W. Extracellular vesicles: exosomes, microvesicles, and friends. J Cell Biol. (2013) 200:373–83. doi: 10.1083/jcb.201211138
2. Kahroba H, Hejazi MS, Samadi N. Exosomes: from carcinogenesis and metastasis to diagnosis and treatment of gastric cancer. Cell Mol Life Sci. (2019) 76:1747–58. doi: 10.1007/s00018-019-03035-2
3. Available online at: http://www.exocarta.org.
4. van Niel G, D’Angelo G, Raposo G. Shedding light on the cell biology of extracellular vesicles. Nat Rev Mol Cell Biol. (2018) 19:213–28. doi: 10.1038/nrm.2017.125
5. Wedge ME, Jennings VA, Crupi MJF, Poutou J, Jamieson T, Pelin A, et al. Virally programmed extracellular vesicles sensitize cancer cells to oncolytic virus and small molecule therapy. Nat Commun. (2022) 13(1):1898. doi: 10.1038/s41467-022-29526-8
6. Pi F, Binzel DW, Lee TJ, Li Z, Sun M, Rychahou P, et al. Nanoparticle orientation to control RNA loading and ligand display on extracellular vesicles for cancer regression. Nat Nanotechnol. (2018) 13(1):82–9. doi: 10.1038/s41565-017-0012-z
7. Fu W, Lei C, Liu S, Cui Y, Wang C, Qian K, et al. CAR exosomes derived from effector CAR-T cells have potent antitumour effects and low toxicity. Nat Commun. (2019) 10(1):4355. doi: 10.1038/s41467-019-12321-3
8. Raffaele S, Gelosa P, Bonfanti E, Lombardi M, Castiglioni L, Cimino M, et al. Microglial vesicles improve post-stroke recovery by preventing immune cell senescence and favoring oligodendrogenesis. Mol Ther. (2021) 29(4):1439–58. doi: 10.1016/j.ymthe.2020.12.009
9. You Y, Muraoka S, Jedrychowski MP, Hu J, McQuade AK, Young-Pearse T, et al. Human neural cell type-specific extracellular vesicle proteome defines disease-related molecules associated with activated astrocytes in Alzheimer’s disease brain. J Extracell Vesicles. (2022) 11(1):e12183. doi: 10.1002/jev2.12183
10. Pan J, Wang Z, Huang X, Xue J, Zhang S, Guo X, et al. Bacteria-derived outer-membrane vesicles hitchhike neutrophils to enhance ischemic stroke therapy. Adv Mater. (2023) 35(38):e2301779. doi: 10.1002/adma.202301779
11. Gao L, Wang L, Wei Y, Krishnamurthy P, Walcott GP, Menasché P, et al. Exosomes secreted by hiPSC-derived cardiac cells improve recovery from myocardial infarction in swine. Sci Transl Med. (2020) 12(567):eaay1318. doi: 10.1126/scitranslmed.aay1318
12. Liu J, Ren H, Zhang C, Li J, Qiu Q, Zhang N, et al. Orally-delivered, cytokine-engineered extracellular vesicles for targeted treatment of inflammatory bowel disease. Small. (2023) 19(50):e2304023. doi: 10.1002/smll.202304023
14. Li G, Qian K, Zhang S, Fu W, Zhao J, Lei C, et al. Engineered soluble ACE2 receptor: Responding to change with change. Front Immunol. (2022) 13:1084331. doi: 10.3389/fimmu.2022.1084331
15. Cocozza F, Névo N, Piovesana E, Lahaye X, Buchrieser J, Schwartz O, et al. Extracellular vesicles containing ACE2 efficiently prevent infection by SARS-CoV-2 Spike protein-containing virus. J Extracell Vesicles. (2020) 10(2):e12050. doi: 10.1002/jev2.12050
16. El-Shennawy L, Hoffmann AD, Dashzeveg NK, McAndrews KM, Mehl PJ, Cornish D, et al. Circulating ACE2-expressing extracellular vesicles block broad strains of SARS-CoV-2. Nat Commun. (2022) 13(1):405. doi: 10.1038/s41467-021-27893-2
17. Xie F, Su P, Pan T, Zhou X, Li H, Huang H, et al. Engineering extracellular vesicles enriched with palmitoylated ACE2 as COVID-19 therapy. Adv Mater. (2021) 33(49):e2103471. doi: 10.1002/adma.202103471
18. Kim OY, Lee J, Gho YS. Extracellular vesicle mimetics: Novel alternatives to extracellular vesicle-based theranostics, drug delivery, and vaccines. Semin Cell Dev Biol. (2017) 67:74–82. doi: 10.1016/j.semcdb.2016.12.001
19. Kim HK, Cho J, Kim E, Kim J, Yang JS, Kim KC, et al. Engineered small extracellular vesicles displaying ACE2 variants on the surface protect against SARS-CoV-2 infection. J Extracell Vesicles. (2022) 11(1):e12179. doi: 10.1002/jev2.12179
20. Lan J, Ge J, Yu J, Shan S, Zhou H, Fan S, et al. Structure of the SARS-CoV-2 spike receptor-binding domain bound to the ACE2 receptor. Nature. (2020) 581(7807):215–20. doi: 10.1038/s41586-020-2180-5
21. Troyer Z, Alhusaini N, Tabler CO, Sweet T, de Carvalho KIL, Schlatzer DM, et al. Extracellular vesicles carry SARS-CoV-2 spike protein and serve as decoys for neutralizing antibodies. J Extracell Vesicles. (2021) 10(8):e12112. doi: 10.1002/jev2.12112
22. Fu Y, Xiong S. Tagged extracellular vesicles with the RBD of the viral spike protein for delivery of antiviral agents against SARS-COV-2 infection. J Control Release. (2021) 335:584–95. doi: 10.1016/j.jconrel.2021.05.049
23. Jiang L, Driedonks TAP, Jong WSP, Dhakal S, Bart van den Berg van Saparoea H, Sitaras I, et al. A bacterial extracellular vesicle-based intranasal vaccine against SARS-CoV-2 protects against disease and elicits neutralizing antibodies to wild-type and Delta variants. J Extracell Vesicles. (2022) 11(3):e12192. doi: 10.1002/jev2.12192
24. Popowski KD, Moatti A, Scull G, Silkstone D, Lutz H, López de Juan Abad B, et al. Inhalable dry powder mRNA vaccines based on extracellular vesicles. Matter. (2022) 5(9):2960–74. doi: 10.1016/j.matt.2022.06.012
25. Kim HY, Kwon S, Um W, Shin S, Kim CH, Park JH, et al. Functional extracellular vesicles for regenerative medicine. Small. (2022) 18(36):e2106569. doi: 10.1002/smll.202106569
26. Zhao X, Wu D, Ma X, Wang J, Hou W, Zhang W. Exosomes as drug carriers for cancer therapy and challenges regarding exosome uptake. BioMed Pharmacother. (2020) 128:110237. doi: 10.1016/j.biopha.2020.110237
27. Ding D-C, Shyu W-C, Lin S-Z. Mesenchymal stem cells. Cell Transplant. (2011) 20. doi: 10.3727/096368910X
28. de Castro LL, Lopes-Pacheco M, Weiss DJ, Cruz FF, Rocco PRM. Current understanding of the immunosuppressive properties of mesenchymal stromal cells. J Mol Med (Berl). (2019) 97:605–18. doi: 10.1007/s00109-019-01776-y
29. Abdulmalek OAAY, Husain KH, AlKhalifa HKAA, Alturani MMAB, Butler AE, Moin ASM. Therapeutic applications of stem cell-derived exosomes. Int J Mol Sci. (2024) 25(6):3562. doi: 10.3390/ijms25063562
30. Shokri M-R, Bozorgmehr M, Ghanavatinejad A, Falak R, Aleahmad M, Kazemnejad S, et al. Human menstrual blood-derived stromal/stem cells modulate functional features of natural killer cells. Sci Rep. (2019) 9(1):10007. doi: 10.1038/s41598-019-46316-3
31. Dendrou CA, Fugger L, Friese MA. Immunopathology of multiple sclerosis. Nat Rev Immunol. (2015) 15:545–58. doi: 10.1038/nri3871
32. Kwon HS, Koh S-H. Neuroinflammation in neurodegenerative disorders: the roles of microglia and astrocytes. Transl Neurodegener. (2020) 9:42. doi: 10.1186/s40035-020-00221-2
33. Zhang J, Buller BA, Zhang ZG, Zhang Y, Lu M, Rosene DL, et al. Exosomes derived from bone marrow mesenchymal stromal cells promote remyelination and reduce neuroinflammation in the demyelinating central nervous system. Exp Neurol. (2022) 347:113895. doi: 10.1016/j.expneurol.2021.113895
34. Riazifar M, Mohammadi MR, Pone EJ, Yeri A, Lässer C, Segaliny AI, et al. Stem cell-derived exosomes as nanotherapeutics for autoimmune and neurodegenerative disorders. ACS Nano. (2019) 13(6):6670–88. doi: 10.1021/acsnano.9b01004
35. Summers SA, Hoi A, Steinmetz OM, O'Sullivan KM, Ooi JD, Odobasic D, et al. TLR9 and TLR4 are required for the development of autoimmunity and lupus nephritis in pristane nephropathy. J Autoimmun. (2010) 35(4):291–8. doi: 10.1016/j.jaut.2010.05.004
36. Hoseinzadeh A, Mahmoudi M, Rafatpanah H, Rezaieyazdi Z, Tavakol Afshari J, Hosseini S, et al. A new generation of mesenchymal stromal/stem cells differentially trained by immunoregulatory probiotics in a lupus microenvironment. Stem Cell Res Ther. (2023) 14(1):358. doi: 10.1186/s13287-023-03578-z
37. Wang D, Zhang H, Liang J, Li X, Feng X, Wang H, et al. Allogeneic mesenchymal stem cell transplantation in severe and refractory systemic lupus erythematosus: 4 years of experience. Cell Transplant. (2013) 22(12):2267–77. doi: 10.3727/096368911X582769c
38. Murray PJ, Allen JE, Biswas SK, Fisher EA, Gilroy DW, Goerdt S, et al. Macrophage activation and polarization: nomenclature and experimental guidelines. Immunity. (2014) 41(1):14–20. doi: 10.1016/j.immuni.2014.06.008
39. Shen Q, Huang Z, Yao J, Jin Y. Extracellular vesicles-mediated interaction within intestinal microenvironment in inflammatory bowel disease. J Adv Res. (2022) 37:221–33. doi: 10.1016/j.jare.2021.07.002
40. Hyvärinen K, Holopainen M, Skirdenko V, Ruhanen H, Lehenkari P, Korhonen M, et al. Mesenchymal stromal cells and their extracellular vesicles enhance the anti-inflammatory phenotype of regulatory macrophages by downregulating the production of interleukin (IL)-23 and IL-22. Front Immunol. (2018) 9:771. doi: 10.3389/fimmu.2018.00771
41. Ko E, Yoon T, Lee Y, Kim J, Park Y-B. ADSC secretome constrains NK cell activity by attenuating IL-2-mediated JAK-STAT and AKT signaling pathway via upregulation of CIS and DUSP4. Stem Cell Res Ther. (2023) 14:329. doi: 10.1186/s13287-023-03516-z
42. Kim H, Lee MJ, Bae EH, Ryu JS, Kaur G, Kim HJ, et al. Comprehensive molecular profiles of functionally effective MSC-derived extracellular vesicles in immunomodulation. Mol Ther. (2020) 28(7):1628–44. doi: 10.1016/j.ymthe.2020.04.020
43. Aloi N, Drago G, Ruggieri S, Cibella F, Colombo P, Longo V. Extracellular vesicles and immunity: at the crossroads of cell communication. Int J Mol Sci. (2024) 25(2):1205. doi: 10.3390/ijms25021205
44. Xie M, Xiong W, She Z, Wen Z, Abdirahman AS, Wan W, et al. Immunoregulatory effects of stem cell-derived extracellular vesicles on immune cells. Front Immunol. (2020) 11:13. doi: 10.3389/fimmu.2020.00013
45. Zhang M, Johnson-Stephenson TK, Wang W, Wang Y, Li J, Li L, et al. Mesenchymal stem cell-derived exosome-educated macrophages alleviate systemic lupus erythematosus by promoting efferocytosis and recruitment of IL-17+ regulatory T cell. Stem Cell Res Ther. (2022) 13(1):484. doi: 10.1186/s13287-022-03174-7
46. Mokarizadeh A, Delirezh N, Morshedi A, Mosayebi G, Farshid AA, Mardani K. Microvesicles derived from mesenchymal stem cells: potent organelles for induction of tolerogenic signaling. Immunol Lett. (2012) 147(1-2):47–54. doi: 10.1016/j.imlet.2012.06.001
47. Bray F, Laversanne M, Weiderpass E, Soerjomataram I. The ever-increasing importance of cancer as a leading cause of premature death worldwide. Cancer. (2021) 127:3029–30. doi: 10.1002/cncr.33587
48. Are C, Rajaram S, Are M, Raj H, Anderson BO, Chaluvarya Swamy R, et al. A review of global cancer burden: trends, challenges, strategies, and a role for surgeons. J Surg Oncol. (2013) 107(2):221–6. doi: 10.1002/jso.23248
49. Kumar-Sinha C, Chinnaiyan AM. Precision oncology in the age of integrative genomics. Nat Biotechnol. (2018) 36:46–60. doi: 10.1038/nbt.4017
50. Li W, Li C, Zhou T, Liu X, Liu X, Li X, et al. Role of exosomal proteins in cancer diagnosis. Mol Cancer. (2017) 16(1):145. doi: 10.1186/s12943-017-0706-8
51. Qian K, Fu W, Li T, Zhao J, Lei C, Hu S. The roles of small extracellular vesicles in cancer and immune regulation and translational potential in cancer therapy. J Exp Clin Cancer Res. (2022) 41(1):286. doi: 10.1186/s13046-022-02492-1
52. Zhou X, Wen W, Shan X, Zhu W, Xu J, Guo R, et al. A six-microRNA panel in plasma was identified as a potential biomarker for lung adenocarcinoma diagnosis. Oncotarget. (2017) 8(4):6513–25. doi: 10.18632/oncotarget.v8i4
53. Dong L, Lin W, Qi P, Xu MD, Wu X, Ni S, et al. Circulating long RNAs in serum extracellular vesicles: their characterization and potential application as biomarkers for diagnosis of colorectal cancer. Cancer Epidemiol Biomarkers Prev. (2016) 25(7):1158–66. doi: 10.1158/1055-9965.EPI-16-0006
54. Zheng R, Du M, Wang X, Xu W, Liang J, Wang W, et al. Exosome-transmitted long non-coding RNA PTENP1 suppresses bladder cancer progression. Mol Cancer. (2018) 17(1):143. doi: 10.1186/s12943-018-0880-3
55. Crowley E, Di Nicolantonio F, Loupakis F, Bardelli A. Liquid biopsy: monitoring cancer-genetics in the blood. Nat Rev Clin Oncol. (2013) 10:472–84. doi: 10.1038/nrclinonc.2013.110
56. Kourembanas S. Exosomes: vehicles of intercellular signaling, biomarkers, and vectors of cell therapy. Annu Rev Physiol. (2015) 77:13–27. doi: 10.1146/annurev-physiol-021014-071641
57. Yuan D, Xu J, Wang J, Pan Y, Fu J, Bai Y, et al. Extracellular miR-1246 promotes lung cancer cell proliferation and enhances radioresistance by directly targeting DR5. Oncotarget. (2016) 7(22):32707–22. doi: 10.18632/oncotarget.v7i22
58. Rodríguez-Martínez A, de Miguel-Pérez D, Ortega FG, García-Puche JL, Robles-Fernández I, Exposito J, et al. Exosomal miRNA profile as complementary tool in the diagnostic and prediction of treatment response in localized breast cancer under neoadjuvant chemotherapy. Breast Cancer Res. (2019) 21(1):21. doi: 10.1186/s13058-019-1109-0
59. Gunnels TF, Stranford DM, Mitrut RE, Kamat NP, Leonard JN. Elucidating design principles for engineering cell-derived vesicles to inhibit SARS-coV-2 infection. Small. (2022) 18:e2200125. doi: 10.1002/smll.202200125
60. Syn NL, Wang L, Chow EK-H, Lim CT, Goh B-C. Exosomes in cancer nanomedicine and immunotherapy: prospects and challenges. Trends Biotechnol. (2017) 35:665–76. doi: 10.1016/j.tibtech.2017.03.004
61. Nordin JZ, Lee Y, Vader P, Mäger I, Johansson HJ, Heusermann W, et al. Ultrafiltration with size-exclusion liquid chromatography for high yield isolation of extracellular vesicles preserving intact biophysical and functional properties. Nanomedicine. (2015) 11(4):879–83. doi: 10.1016/j.nano.2015.01.003
Keywords: exosome (EXO), cancer, immune disease, COVID - 19, nanodrug
Citation: Li G, Zhang S, Zou Y, Ai H, Zheng X, Qian K, Lei C and Fu W (2024) The therapeutic potential of exosomes in immunotherapy. Front. Immunol. 15:1424081. doi: 10.3389/fimmu.2024.1424081
Received: 27 April 2024; Accepted: 24 June 2024;
Published: 08 July 2024.
Edited by:
Bo Li, Southern Medical University, ChinaReviewed by:
Yongsheng Yu, Chinese Academy of Sciences, ChinaCopyright © 2024 Li, Zhang, Zou, Ai, Zheng, Qian, Lei and Fu. This is an open-access article distributed under the terms of the Creative Commons Attribution License (CC BY). The use, distribution or reproduction in other forums is permitted, provided the original author(s) and the copyright owner(s) are credited and that the original publication in this journal is cited, in accordance with accepted academic practice. No use, distribution or reproduction is permitted which does not comply with these terms.
*Correspondence: Changhai Lei, lei@smmu.edu.cn; Wenyan Fu, fuwenyan@fengmed.com
†These authors have contributed equally to this work
Disclaimer: All claims expressed in this article are solely those of the authors and do not necessarily represent those of their affiliated organizations, or those of the publisher, the editors and the reviewers. Any product that may be evaluated in this article or claim that may be made by its manufacturer is not guaranteed or endorsed by the publisher.
Research integrity at Frontiers
Learn more about the work of our research integrity team to safeguard the quality of each article we publish.