- 1Genetics Laboratory, Center of Development and Innovation, Butantan Institute, São Paulo, Brazil
- 2BioDecision Analytics Ltda., São Paulo, Brazil
- 3Post-graduation Program in Structural and Functional Biology, Paulista School of Medicine Escola Paulista de Medicina (EPM), Federal University of São Paulo Universidade Federal de São Paulo (UNIFESP), São Paulo, Brazil
Interleukin-6 (IL-6) is a versatile cytokine crucial for immune response modulation, inflammation regulation, and various physiological processes in the body. Its wide-ranging functions underscore its importance in maintaining health. Dysregulated IL-6 is closely associated with many diseases, making it a key research and therapeutic target. Elevated IL-6 levels in the central nervous system worsen neuroinflammation in neurodegenerative diseases by activating microglia and astrocytes and releasing pro-inflammatory cytokines and neurotoxic molecules. Moreover, dysregulated IL-6 weakens the blood-brain barrier, exacerbating neuroinflammation and neuronal damage by allowing peripheral immune cells and inflammatory mediators to enter the brain. Mesenchymal stem cells (MSCs) show promise in modulating neuroinflammation by regulating IL-6 levels. They effectively suppress pro-inflammatory cytokines, including IL-6, while promoting anti-inflammatory factors. This therapeutic approach highlights the importance of targeting IL-6 and other inflammatory mediators to alleviate neuroinflammation and its adverse effects on neurological disorders. This review provides a comprehensive overview of IL-6’s involvement in neurological disorders, examining endogenous IL-6 and IL-6 derived from MSCs. We explore IL-6’s mechanisms affecting neuronal function, survival, and immune modulation in the central nervous system. Additionally, we discuss the potential of MSC-derived IL-6 in neuroregeneration and neuroprotection. By elucidating IL-6’s interplay with neurological pathologies, this review offers insights into novel therapeutic strategies targeting IL-6 signaling pathways for neurological disorders.
1 Introduction
Interleukin-6 (IL-6) is an indispensable pleiotropic cytokine, that plays a pivotal role in orchestrating the body’s immune response to infection, injury, or inflammation to induce and coordinate the different elements of the acute-phase response (1–3). Its multifaceted actions encompass the stimulation of activation and proliferation of diverse immune cells, including T cells, B cells, and macrophages. Additionally, IL-6 facilitates the differentiation of B cells into plasma cells, which is essential for antibody production (4–8).
Beyond its immunomodulatory functions, IL-6 also influences hematopoiesis, the intricate process of blood cell formation within the bone marrow, by promoting the differentiation of hematopoietic stem cells into various blood cell types, ensuring a balanced and functional immune system (9–12). Furthermore, IL-6 triggers the liver to produce acute- phase proteins essential for inflammation, tissue repair, and immune responses. It also contributes to fever induction during infection or inflammation by interacting with the hypothalamus, the brain region regulating body temperature (13–16).
In summary, IL-6 is a multifaceted cytokine with crucial roles in immune response modulation, inflammation regulation, and diverse physiological processes throughout the body (17). The IL-6 intricate functions underscore its significance in maintaining health and homeostasis. Dysregulation of IL-6 is closely linked to numerous diseases, emphasizing its prominence as a prime target for both research exploration and therapeutic intervention strategies (18, 19).
The clinical significance of IL-6 spans multiple domains (5). In inflammatory disorders, dysregulation of IL-6 signaling is at the core of conditions like rheumatoid arthritis (20–23), inflammatory bowel disease (24), and systemic lupus, exacerbating inflammation, that cause to tissue damage (25, 26). IL-6’s involvement in cancer extends beyond inflammation, since the IL-6 serves as “fuels” to tumor growth, promoting angiogenesis, and facilitating metastasis (27–29). By contrast, the IL-6 deficiency has been shown to exacerbate neurodegenerative disorders (30).
Given its critical role in various diseases, IL-6 and its receptors have emerged as promising therapeutic targets (31–34). However, IL-6 is naturally expressed by different mesenchymal stroma/stem cells (MSCs) populations, since its expression regulates the MSC stemness (35), in vitro proliferation (36) and differentiation (37, 38). Based on this, herein we summarize the IL-6 roles in pathophysiology of neurodegenerative disorders and, discussing the possible IL-6 and MSC-derived IL-6 therapeutic applications for the treatment of these diseases.
2 IL-6 signaling pathways
The IL-6/IL-6R axis is crucial for mediating a wide range of biological processes, including immune response modulation, inflammation regulation, and cellular proliferation (1–3). IL-6 exerts its effects through classic signaling, where it binds to the membrane-bound IL-6 receptor (mIL-6R) on target cells, and trans signaling, where it interacts with the soluble IL-6 receptor (sIL-6R) and the signal transducer gp130 on cells that do not express mIL-6R. This axis plays a pivotal role in the acute-phase response, T-cell differentiation, and B-cell maturation, contributing to both pro-inflammatory and anti-inflammatory effects (39, 40). In the central nervous system, IL-6 can activate glial cells and disrupt the blood-brain barrier, exacerbating neuroinflammation, while also promoting neuronal survival and neurogenesis. IL-6 signaling exerts influence over specific brain regions crucial for cognitive function, motor control, and emotional regulation (41, 42). Dysregulation of IL-6 signaling within these regions, such as the hippocampus, cortex, striatum, and substantia nigra, can significantly affect neurological function and contribute to the manifestation of various symptoms observed in neurological disorders (43–45). Additionally, IL-6 influences metabolic processes and is implicated in conditions like cancer, where it supports tumor growth and survival. Understanding the IL-6/IL-6R axis is essential for developing targeted therapies for a variety of diseases, including autoimmune disorders, neurodegenerative diseases, and metabolic syndromes (28, 39, 46).
IL-6 signaling pathways constitute intricate networks of molecular interactions governing the biological effects of IL-6 (47–49). The initiation of IL-6 signaling commences with IL-6 binding to its specific receptor, the IL-6 receptor (IL-6R), existing in two forms: membrane-bound (mIL-6R) and soluble (sIL-6R). The classical IL-6 signaling pathway primarily operates through membrane-bound IL-6R (mIL-6R) and involves four key steps (Figure 1).
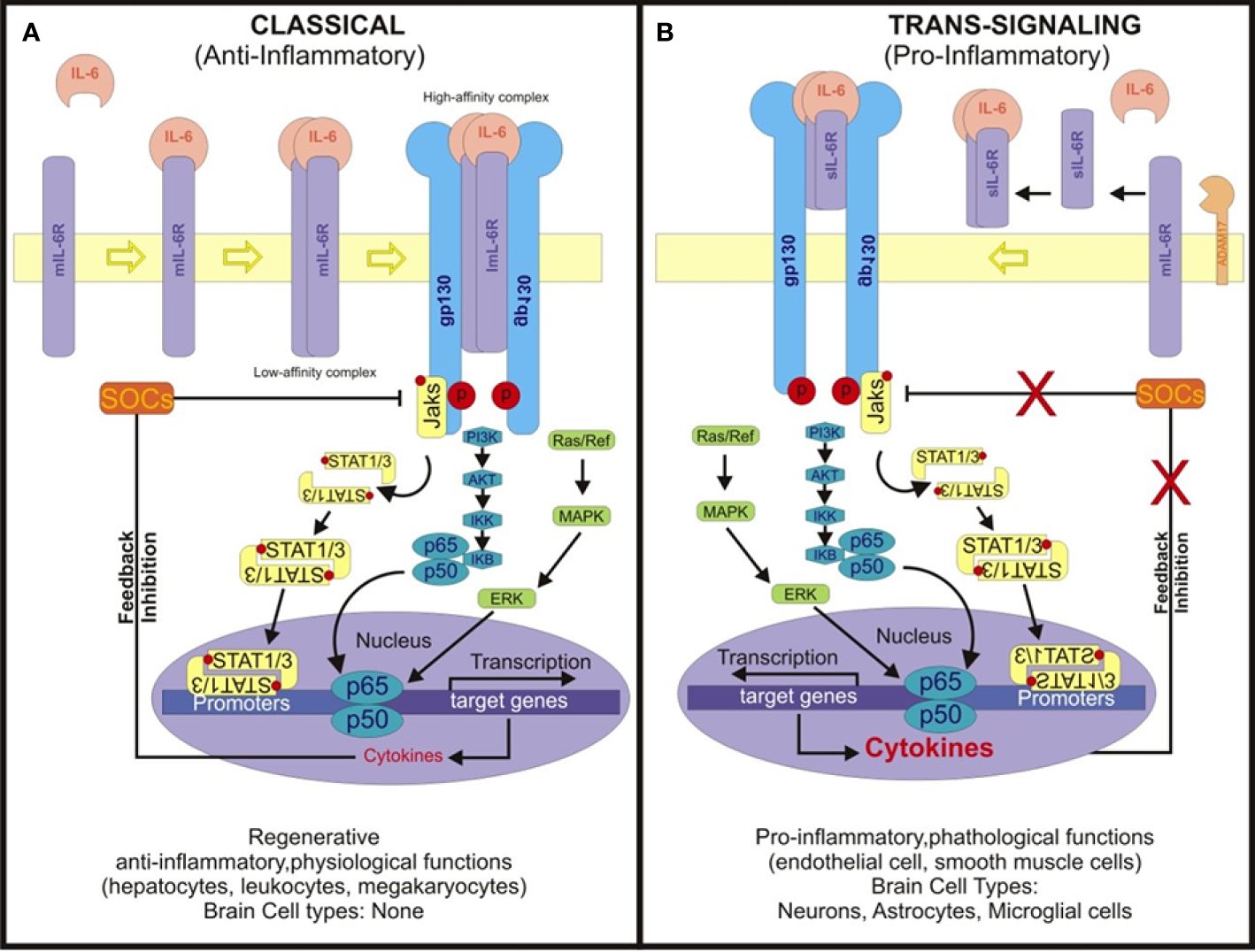
Figure 1 Scheme depicting the classical IL-6 and trans-signaling pathways. (A) In classical IL-6 signaling, IL-6 binds to membrane-boundIL-6 receptor (mIL-6R), initiating complex formation with gp130. This triggers dimerization of IL-6R, assembling a hexameric signaling complex. This complex activates intracellular cascades including JAK/STAT, MAPK/MEK-ERK, and PI3K/AKT pathways. Phosphorylated JAKs recruit and phosphorylate STAT proteins, enabling their nuclear translocation and transcriptional activation. The Classical IL-6 signaling regulates genes involved in inflammation, immune response, proliferation, and differentiation. It primarily occurs in select peripheral cell types. (B) The soluble IL-6 receptor (sIL-6R) assumes a pivotal role in IL-6 trans-signaling by engaging with interleukin-6 (IL-6) in the extracellular milieu. This complex formation ensues subsequent to the cleavage of the membrane-bound IL-6 receptor (mIL-6R) from cell membranes by ADAM17, thereby releasing sIL-6R. Unlike classical signaling, where IL-6 binds exclusively to mIL-6R on specific cell types, trans-signaling via the IL-6/sIL-6R complex can transpire in any cell expressing the glycoprotein 130 (gp130) receptor. Given the ubiquitous expression of gp130, this complex has the capacity to activate a plethora of signaling pathways, including the Janus kinase/signal transducer and activator of transcription (JAK/STAT), mitogen-activated protein kinase (MAPK), and phosphoinositide 3-kinase (PI3K)/Akt pathways. Such broad activation spectrum culminates in diverse cellular responses, notably amplifying JAK/STAT signaling while modulating the MAPK pathway through SOCS suppression and NF-κB, thereby fostering a pro-inflammatory milieu. This mechanism assumes paramount importance in tissues devoid of mIL-6R expression, thereby ensuring that IL-6 signaling retains its capacity to modulate gene expression and immune responses across a diverse array of cell types, including the brain, where it significantly influences inflammation and immune functions.
In addition to the classical pathway, IL-6 can initiate signaling through a trans-signaling mechanism involving soluble IL-6 receptor (sIL-6R) (48, 49). This process begins with the binding of IL-6 to its soluble receptor (sIL-6R), forming a complex. The sIL-6R is generated through proteolytic cleavage of mIL-6R or alternative splicing, releasing the extracellular domain of mIL-6R into circulation. Subsequently, the soluble IL-6/sIL-6R complex circulates freely in the bloodstream (48, 49).
In tissues lacking mIL-6R expression, the soluble IL-6/sIL-6R complex binds to the glycoprotein 130 (gp130) receptor on the cell surface. Gp130 is ubiquitously expressed on various cell types. Binding of the soluble IL-6/sIL-6R complex to Gp130 triggers receptor dimerization and activates intracellular signaling pathways (50). These signaling cascades typically involve the activation of the Janus kinase/signal transducer and activator of transcription (JAK/STAT) pathway, mitogen-activated protein kinase (MAPK) pathway, and phosphoinositide 3-kinase (PI3K)/Akt pathway (51, 52). Activation of these pathways culminates in the modulation of gene expression and cellular responses akin to the classical IL-6 signaling pathway.
To comprehensively understand the functional mechanisms depicted in Figure 1, it’s essential to delineate the roles of each cell type involved in the IL-6 signaling pathways. IL-6-secreting cells produce and release interleukin-6 (IL-6), a pivotal pro-inflammatory cytokine. Cells expressing membrane-bound IL-6 receptor (mIL-6R) facilitate IL-6 binding, forming a complex with glycoprotein 130 (gp130), which transduces downstream signals. ADAM17-expressing cells, such as certain immune cells, cleave mIL-6R to release soluble IL-6 receptor (sIL-6R). In turn, cells lacking mIL-6R but expressing gp130 can still respond to IL-6 via trans-signaling when the IL-6/sIL-6R complex binds to gp130. This intricate interplay among various cell types orchestrates IL-6 signaling, culminating in the activation of downstream pathways and modulation of cellular responses, including inflammation and immune reactions.
While the classic IL-6 signaling pathway is responsible for the anti-inflammatory actions, the IL-6 trans-signaling pathway contribute to pathogenic activities. Thus, they diverge notably in their cellular targets and the breadth of their biological impact across various tissues and organ systems. In the classic pathway, membrane-bound IL-6R is crucial for cell activation, while the trans-signaling pathway exploits soluble IL-6R to broaden the spectrum of target cells and biological responses.
3 Role of IL-6 during development and adult life
During embryonic development, interleukin-6 (IL-6) is produced by diverse cell types, including trophoblasts (53), extraembryonic endoderm (54), embryonic stem cells (ESCs) (55, 56), and mesenchymal stem cells (MSCs) (35, 36, 57), contributing to the intricate orchestration of early developmental processes. Trophoblast cells, forming the outer layer of the blastocyst during early embryonic development, are essential for implantation, placental formation, and nutrient exchange between the developing embryo and the maternal environment. Recognized as IL-6 producers, trophoblasts suggest potential regulatory functions during early embryonic development and implantation processes.
Additionally, the extraembryonic endoderm, derived from the blastocyst’s inner cell mass, significantly contributes to yolk sac formation and placental development. These cells also produce IL-6 during early embryogenesis, implicating its involvement in supporting placental growth and function (11, 58, 59).
ESCs, originating from the blastocyst’s inner cell mass, exhibit remarkable pluripotency, capable of differentiating into cell types representing all three germ layers. Under specific conditions, ESCs have been observed to produce IL-6, particularly during in vitro culture and differentiation procedures, suggesting a potential role for IL-6 in guiding ESC fate and development (60, 61).
Furthermore, MSCs, progenitors of various connective tissues such as bone, cartilage, and muscle, actively participate in organogenesis and tissue remodeling throughout embryonic development. IL-6 production by these cells hints at its regulatory involvement in these processes, potentially influencing tissue differentiation and morphogenesis (35–37, 57).
The diverse production of IL-6 by various cell types during embryonic development underscores its importance in regulating key developmental events and highlights its potential as a critical player in guiding embryonic growth and organogenesis.
In adult life, IL-6 plays multifaceted roles beyond its well-established functions in immune response regulation and inflammation modulation. A crucial aspect of IL-6 activity lies in its contribution to tissue repair and regeneration following injury or damage in adults. This cytokine serves as a key orchestrator in the intricate process of wound healing, facilitating the proliferation and migration of various cell types important for tissue restoration, including fibroblasts, essential for generating connective tissue; endothelial cells crucial for blood vessel formation; and immune cells that aid in tissue remodeling and defense against pathogens (62–64).
Moreover, IL-6’s involvement extends to the regeneration of specific tissues such as muscle and liver. By promoting cellular proliferation and tissue remodeling mechanisms, IL-6 aids in the restoration of structural and functional integrity in these vital organs post-injury or during pathological conditions. After skeletal muscle injury, IL-6 is dynamically released from damaged muscle fibers and promotes infiltration of immune cells within the injury site. Acting as a key regulator of muscle repair, IL-6 serves as a potent stimulator of myoblast proliferation, the precursor cells crucial for initiating muscle regeneration. Additionally, IL-6 promotes the fusion of myoblasts into multinucleated myotubes, a fundamental step in the restoration of muscle fiber integrity post-injury. Furthermore, IL-6 exerts its regenerative influence by stimulating the secretion of growth factors, including insulin-like growth factor-1 (IGF-1), which amplifies the reparative processes within the injured muscle tissue (65). Studies utilizing animal models underscore the indispensable role of IL-6 in muscle regeneration, as mice deficient in IL-6 exhibit delayed and impaired recovery, emphasizing the essential contribution of IL-6 in ordering efficient muscle repair mechanisms. Furthermore, IL-6 exerts a significant influence on neurological functions in adults (64, 66–70).
The diverse roles of IL-6 in tissue repair, neurological function, and disease pathogenesis underscore its importance as a multifunctional cytokine in adult physiology and pathology. Further understanding of its intricate mechanisms of action holds promise for the development of targeted therapeutic interventions for a range of conditions affecting human health.
4 IL-6 in neurological disorders
The classical IL-6 signaling pathway profoundly influences neurodegenerative diseases, playing an essential role in various aspects of their pathophysiology. Similarly, the IL-6 trans-signaling pathway holds significant relevance in these conditions. Consequently, dysregulation of IL-6 emerges as a substantial factor in various neurological disorders, including Alzheimer’s disease (AD), Parkinson’s disease (PD) (71, 72), and Huntington’s disease (HD) (30, 73, 74), Multiple Sclerosis (MS) (75, 76), Amyotrophic Lateral Sclerosis (ALS) (77, 78) an others. HD, AD, and PD are marked by the gradual deterioration of nerve cells in specific brain regions, such as the striatum in HD, the hippocampus and cortex in AD, and the substantia nigra in PD. This degeneration leads to cognitive decline and motor impairment, affecting various aspects of daily life. While they exhibit overlapping symptoms like cognitive deficits and movement issues, each has unique genetic origins and impacts individuals uniquely. Nonetheless, these conditions profoundly impact the well-being of those affected and their families, underscoring the crucial demand for efficacious treatments. These diseases were specifically chosen to exemplify IL-6’s role in the neuroinflammation process.
In Alzheimer’s disease (AD), characterized by amyloid beta (Aβ) plaque accumulation and tau pathology, IL-6 influences Aβ production and clearance. Dysregulated IL-6 signaling disrupts the delicate balance between Aβ production and clearance mechanisms, leading to toxic Aβ species accumulation and neurotoxic plaque formation (79). Another hallmark of AD is the abnormal phosphorylation and aggregation of tau protein into neurofibrillary tangles. IL-6 has also been implicated in tau pathology, affecting tau phosphorylation and aggregation processes (80). Dysregulated IL-6 signaling can contribute to tau pathology by promoting aberrant tau phosphorylation and impairing tau clearance mechanisms, which ultimately exacerbate neuronal dysfunction and degeneration (81).
Moreover, AD is characterized by chronic neuroinflammation, marked by sustained activation of microglia and astrocytes (82). IL-6 exacerbates chronic neuroinflammation, affecting the blood-brain barrier (BBB) and influencing neuronal survival. Dysregulated IL-6 signaling contributes to neuroinflammation, releasing pro-inflammatory cytokines and reactive oxygen species, further damaging neurons, and impairing cognitive function in AD (83). Additionally, IL-6 can affect the integrity of the BBB, which regulates the passage of molecules and immune cells between the bloodstream and the brain. Dysregulated IL-6 signaling compromises BBB integrity, allowing peripheral immune cells and inflammatory mediators to infiltrate the brain, further amplifying neuroinflammation and neuronal damage in AD (42, 84, 85). Furthermore, IL-6 plays a complex role in regulating neuronal survival and synaptic plasticity in the brain. While acute IL-6 signaling may promote neuroprotection and synaptic plasticity, chronic or dysregulated IL-6 signaling can lead to neuronal dysfunction and synaptic loss. This imbalance in IL-6 signaling disrupts the delicate equilibrium between neuronal survival and death, contributing to neurodegeneration in AD (86–89).
In Huntington’s disease (HD), marked by motor dysfunction, cognitive decline, and psychiatric symptoms (90), IL-6 dysregulation (30, 73) leads to several detrimental effects. First, it contributes to excitotoxicity, an overwhelming stimulation of glutamate receptors resulting in neuronal damage. IL-6 enhances glutamate release while impairing its reuptake, exacerbating excitotoxicity in HD (91, 92). Additionally, IL-6 is implicated in the dysregulation of intracellular calcium signaling, further contributing to neuronal dysfunction and cell death (93). Astrocyte dysfunction, increasingly recognized in HD pathology, is also influenced by IL-6 signaling. Dysregulated IL-6 signaling disrupts astrocyte function, impairing their ability to support neuronal health and regulate synaptic activity. This disruption in astrocyte-neuron interactions contributes to neuronal dysfunction and degeneration (94, 95). While acute IL-6 signaling may have neuroprotective and tissue repair roles, chronic or dysregulated IL-6 signaling has detrimental effects. It impairs the brain’s ability to mount effective neuroprotective and repair responses, exacerbating neuronal damage and disease progression in HD (74).
Furthermore, HD presents psychiatric symptoms like depression and anxiety, and IL-6 dysregulation has been implicated in their pathophysiology. Elevated IL-6 levels are associated with depressive symptoms in HD patients, suggesting a potential role for IL-6 in the psychiatric manifestations of the disease (73). Therefore, dysregulated IL-6 signaling plays a significant role in HD progression by fostering neuroinflammation, enhancing excitotoxicity, disrupting astrocyte function, impairing neuroprotective mechanisms, and potentially exacerbating psychiatric symptoms (96–100).
IL-6 has attracted considerable interest within the context of Parkinson’s disease (PD), a progressive neurodegenerative disorder characterized by motor manifestations such as tremors, rigidity, and bradykinesia, coupled with non-motor symptoms encompassing cognitive decline and mood disturbances (80, 101–105). Furthermore, IL-6-induced Chronic Inflammatory Response (CISR) Syndrome precipitates toxic neuronal iron accumulation, thereby contributing to synuclein-induced neurodegeneration (106).
Despite the intricate and multifaceted nature of IL-6’s precise contribution to PD, recent insights suggest its involvement in various facets of the disorder’s pathophysiology. Neuroinflammation plays a pivotal role in PD, with dysregulated IL-6 signaling contributing to the chronic inflammatory state observed in this condition. This is substantiated by elevated levels of IL-6 detected in the cerebrospinal fluid and brain tissue of PD patients (107–110).
Additionally, studies indicate that IL-6 can initiate the demise of dopaminergic neurons through inflammatory pathways and oxidative stress mechanisms, potentially contributing to the progressive loss of these neurons in PD. Furthermore, IL-6’s impact on the integrity of the blood-brain barrier (BBB) is noteworthy, facilitating the entry of peripheral immune cells and inflammatory molecules into the brain (111–115), thereby exacerbating neuroinflammation and neuronal damage in PD, as well as motor and cognitive impairment, and an increased risk of dementia in PD patients (107, 108, 116, 117).
Dysregulated IL-6 signaling significantly contributes to the pathogenesis of neurodegenerative diseases (AD, HD, and PD) by promoting neuroinflammation, exacerbating Aβ accumulation and tau pathology in AD, compromising BBB integrity, and impairing neuronal survival and synaptic plasticity. Targeted interventions aimed at IL-6 signaling pathways offer-promising strategies for managing these conditions and potentially slowing disease progression.
5 Mesenchymal stem cells in IL-6 regulation
Mesenchymal stem cells (MSCs) represent a versatile type of multipotent adult stem cell with the ability to differentiate into osteoblasts, chondrocytes, and adipocytes. Widely distributed in tissues like bone marrow, adipose tissue, umbilical cord blood, and dental pulp, MSCs are valued for their self-renewal capacity, making them promise in regenerative medicine and tissue engineering applications (90, 118).
While MSCs can express and secrete various cytokines, including IL-6, their IL-6 production is generally lower compared to immune cells like macrophages or T cells. However, under specific conditions, such as exposure to inflammatory stimuli or interactions with immune cells, MSCs can produce IL-6. The extent of IL-6 secretion by MSCs depends on factors like their source, culture conditions, and microenvironmental cues, emphasizing a context-dependent regulation of IL-6 production (36–38, 119–122).
MSCs have been extensively studied in clinical trials across various medical conditions, demonstrating potential from musculoskeletal disorders to autoimmune diseases and inflammatory ailments (123, 124). In the context of neuroinflammation, MSCs exhibit promise in modulating IL-6 expression. The downregulation of IL-6 by MSCs involves autocrine and paracrine signaling loops, as well as feedback regulation within the immune system (125–128).
In autocrine regulation, cells producing IL-6 respond to their own secretion, amplifying IL-6 production in response to stimuli. Paracrine signaling involves IL-6 influencing neighboring cells, leading to coordinated IL-6 expression among different cell types. Feedback mechanisms within the immune system, influenced by IL-6 signaling, further regulate IL-6 production (129–133). Additionally, IL-6 expression can be regulated by feedback mechanisms within the immune system, where IL-6 signaling influences the differentiation and activation of immune cells, thereby modulating IL-6 production (134–136).
The intricate influence of MSCs on endogenous IL-6 production is contingent upon cellular interactions and experimental parameters (36). Gu et al. (119) delineated that MSC-induced endogenous IL-6 release resulted in upregulating of IL-6R and p-STAT3 levels in astrocytes subjected to oxygen and glucose deprivation. Notably, a conspicuous elevation in the Bcl-2 to Bax ratio, pivotal downstream factors of the STAT3 signaling pathway, was observed. This investigation elucidated the neuroprotective impact of MSCs transplantation in neonatal hypoxic-ischemic brain damage rats, partly mediated by IL-6, enhancing the anti-apoptotic profile of injured astrocytes via the IL-6/STAT3 signaling pathway. While MSCs have demonstrated their capability to suppress IL-6 production by immune cells such as macrophages and T cells through paracrine signaling and immunomodulatory mechanisms (137, 138), MSC-derived IL-6 has also been shown to stimulate or modulate the activity of other immune cells, thereby influencing endogenous IL-6 levels (139, 140).
MSCs exhibit anti-inflammatory effects, affecting IL-6 levels across various contexts and can downregulate IL-6 production by suppressing the activation of immune cells. This was evident in a study involving inflammatory bowel disease (IBD) in mice, where MSC administration led to diminished levels of pro-inflammatory cytokines, including IL-6, in inflamed colon tissue (141, 142). Mechanistically, MSCs manifest their anti-inflammatory effects through the secretion of factors such as transforming growth factor-beta (TGF-β) and prostaglandin E2 (PGE2), as well as by fostering the generation of regulatory T cells (Tregs) (143, 144).
6 MSCs and IL-6 in neuroinflammation and neuroprotection
In the intricate landscape of neuroinflammation, both MSCs and IL-6 wield substantial influence, jointly shaping the pathophysiology of diverse neurological disorders.
When introduced into neuroinflammatory conditions, MSCs exhibit the capacity to downregulate the expression of IL-6 by activated microglia, astrocytes, and infiltrating immune cells within the central nervous system (CNS), as illustrated in Figure 2. This regulatory effect is achieved through the secretion of anti-inflammatory factors such as IL-10 and TGF-β, which effectively inhibit the synthesis and release of IL-6 by immune cells. Additionally, MSCs foster the generation of Tregs, contributing to an additional layer of suppression on IL-6 production and the attenuation of neuroinflammation (145–147).
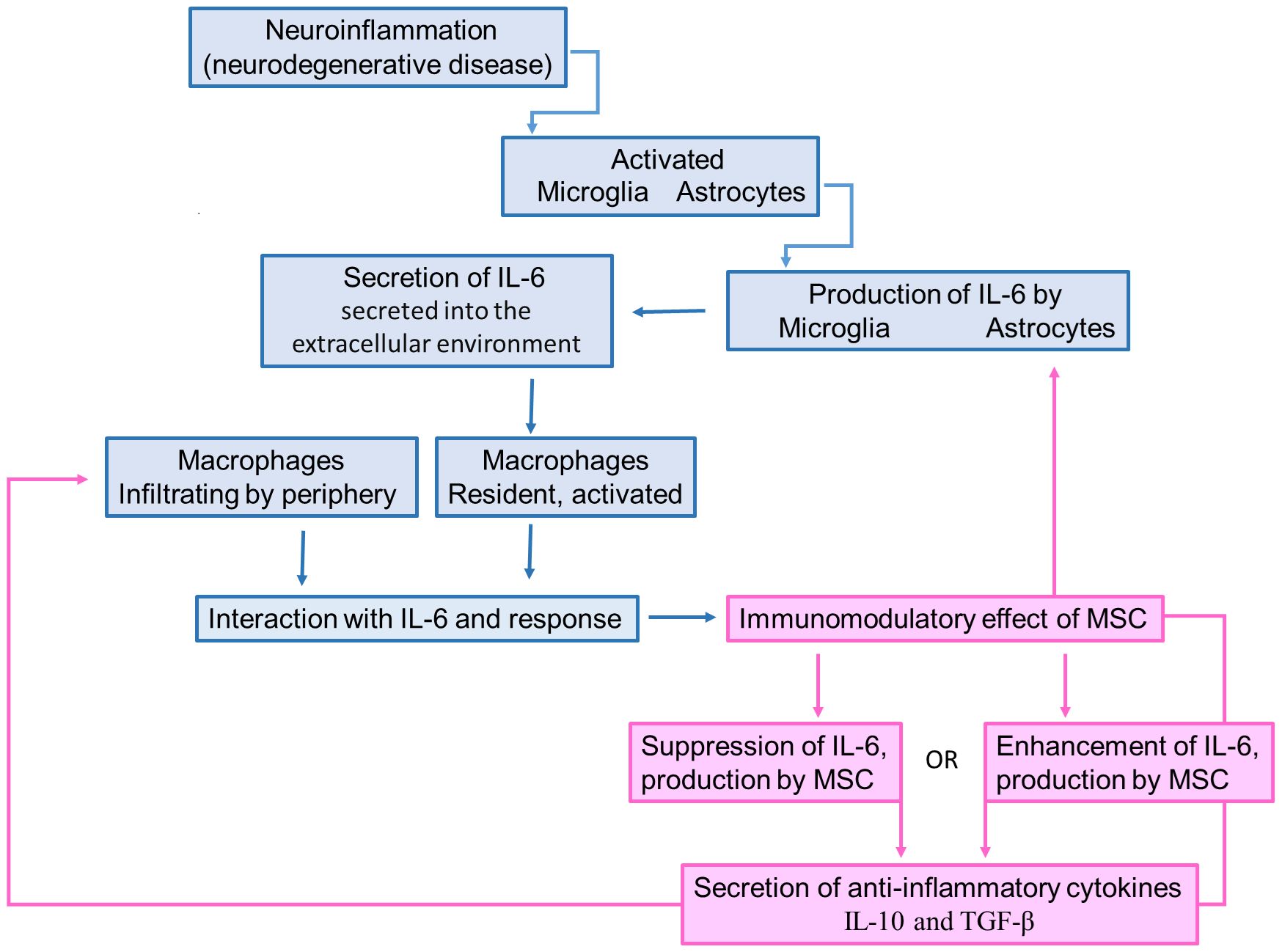
Figure 2 Schematic illustration describing the IL-6 role in neurodegeneration and how the MSC-secreted IL-6 can promote immunomodulation. Neuroinflammatory process caused by the bioaccumulation of misfolded proteins promotes the activation of microglia and astrocytes. Once activated, these cells produce and secrete IL-6, which attracts monocytes to be differentiated into macrophages. However, the MSC transplantation increases the IL-6 levels, leading to the production of IL-10 and TGF-β by macrophages, which suppress the microglia/astrocyte activation.
In the complex realm of immunomodulation, MSCs play a crucial role in balancing pro-inflammatory and anti-inflammatory forces by regulating cytokine production, notably IL-6 (Figure 2). Both the suppression and enhancement of IL-6 production by MSCs influence the secretion of anti-inflammatory cytokines. When MSCs suppress IL-6 production, they create a favorable environment for anti-inflammatory cytokine secretion by dampening the pro-inflammatory response. This displays MSCs’ multifaceted immunomodulatory capabilities, where reduced IL-6 levels pave the way for anti-inflammatory mediators. Conversely, when MSCs enhance IL-6 production, they introduce complexity to immune regulation. Despite IL-6’s pro-inflammatory reputation, it can exhibit anti-inflammatory effects in certain contexts. By increasing IL-6 levels, MSCs may trigger a regulatory response, leading to the secretion of anti-inflammatory cytokines to counteract inflammation. In both scenarios, achieving the desired outcome relies on a delicate balance influenced by cellular signals and contextual cues. MSCs act as pivotal orchestrators in this intricate dance of immunomodulation, guiding the secretion of anti-inflammatory cytokines amidst the dynamic landscape of inflammation and immune response.
Recent research underscores that endogenous MSCs transplantation significantly enhances cognitive function and sustains brain health in neonatal rats with hypoxic-ischemic brain damage (HIBD), owing to the immune-regulating abilities of MSCs (119). Notably, this study reveals a substantial boost in IL-6 release compared to other cytokines upon MSC transplantation. When MSCs with reduced IL-6 expression were used, positive effects on behavior and brain activity were notably diminished correlating with decreased IL-6 levels in the hippocampus. This study sheds light on MSCs enhancing the survival of injured astrocytes through the IL-6/STAT3 signaling pathway, indicating IL-6 as a key mediator in the neuroprotective effects, emphasizing the critical role of IL-6 in the neuroprotective effects of MSCs transplantation in neonatal HIBD rats partly rely on IL-6, promoting the survival of injured astrocytes via the IL-6/STAT3 signaling pathway (119).
Conversely, IL-6 secreted by exogenous cells can influence the immunomodulatory properties of MSCs and influence their behavior within the CNS. Studies suggest that IL-6 enhances the immunosuppressive function of exogenous MSCs and facilitates their migration to inflammatory sites in the CNS (148, 149). However, prolonged exposure to high levels of IL-6 may jeopardize the therapeutic potential of MSCs and compromise their regenerative capacity. IL-6 signaling has the potential to disrupt the equilibrium between pro-inflammatory and anti-inflammatory factors secreted by exogenous MSCs, leading to dysregulated immune responses and persistent neuroinflammation (150).
The modulation of IL-6 signaling pathways, coupled with leveraging the immunomodulatory prowess of MSCs, emerges as a compelling avenue for developing therapeutic strategies aimed at alleviating neuroinflammation and fostering neurological recovery in neurodegenerative diseases and neurological injuries. Current research endeavors, as highlighted by Gu et al. (119), Wang et al. (151), and Kitzberger et al. (122) are diligently exploring the efficacy of MSC-based therapies and IL-6 inhibitors in the realm of neuroinflammatory disorders. These studies seek not only to unravel the therapeutic potential of such interventions but also to refine treatment strategies for patients grappling with these complex conditions.
The therapeutic promise extends to MSC-derived IL-6, operating synergistically with other bioactive factors secreted by MSCs. This collaborative action holds substantial potential for amplifying the effectiveness of MSC-based treatments in neurological disorders. Through mechanisms involving neuroprotection, neuroregeneration, and immunomodulation within the CNS, IL-6 plays a crucial role in enhancing the overall efficacy of MSC therapies. Strategic targeting of IL-6 signaling pathways, as already demonstrated (152–155), introduces innovative dimensions in neuroprotection and neurorepair.
In specific contexts, IL-6 derived from MSCs showcases neuroprotective properties by fortifying neuronal survival and providing a shield against various insults, including oxidative stress, excitotoxicity, and inflammatory cytokines (156, 157). Through intricate intracellular signaling cascades, particularly via the Janus kinase (JAK)-signal transducer and activator of transcription (STAT) pathway, IL-6 actively reinforces neuronal resilience and viability (158, 159).
Beyond these fundamental roles, IL-6 assumes a crucial position in modulating synaptic plasticity, a process essential for learning and memory consolidation. Its regulatory influence on the expression and functionality of neurotransmitter receptors, synaptic proteins, and signaling molecules (160, 161). Additionally, IL-6 actively promotes neurite outgrowth and axonal regeneration, facilitating crucial neuronal connectivity and repair within the injured or diseased CNS (162–164). Furthermore, IL-6 modulates neurotransmitter release and neuronal excitability, underscoring its multifaceted role in neurological function and pathology (165–167).
Thus, IL-6, and MSC-derived IL-6 emerges as a key player in both the pathophysiology of neurological disorders and potential therapeutic interventions. Its involvement in immunomodulation, tissue repair, and neuroprotection emphasizes its therapeutic potential in conditions such as stroke, traumatic brain injury, and neurodegenerative diseases.
7 Conclusions
The collaborative interaction between MSCs and IL-6 in neuroinflammation is crucial for understanding the pathophysiology of neurological disorders and developing therapeutic strategies to mitigate neuroinflammation and promote neurological recovery in various neurodegenerative diseases and neurological injuries.
The diverse roles of IL-6 in immunomodulation, tissue repair, neuroprotection, and synaptic plasticity highlight its promising application in neurological conditions ranging from stroke to traumatic brain injury and neurodegenerative diseases.
Current studies on the efficacy of MSC-based therapies and IL-6 inhibitors aim to elucidate their therapeutic potential and optimize treatment strategies for patients with these conditions.
IL-6 derived from MSCs, along with other bioactive factors secreted by them, promises to enhance the therapeutic efficacy of MSC-based treatments in neurological disorders, contributing to neuroprotection, neuroregeneration, and immunomodulation in the CNS. In this regards, strategic targeting of IL-6 signaling pathways can further amplify the effectiveness of MSC-based interventions, paving the way for innovative approaches in neuroprotection and neurorepair.
Author contributions
AR: Writing – review & editing, Validation, Formal analysis. ÁS: Writing – review & editing, Visualization, Validation, Formal analysis. IK: Writing – review & editing, Writing – original draft, Visualization, Validation, Supervision, Project administration, Methodology, Investigation, Funding acquisition, Formal analysis, Conceptualization.
Funding
The author(s) declare financial support was received for the research, authorship, and/or publication of this article. The authors thank the Butantan Institute and the Butantan Foundation for the financial support provided for this study. The support provided in this study by the Butantan Foundation was facilitated through the cost center BF FCDID 73012.
Conflict of interest
Author Rodrigo Pinheiro Araldi was employed by BioDecision Analytics Ltda.
The remaining authors declare that the research was conducted in the absence of any commercial or financial relationships that could be construed as a potential conflict of interest.
Publisher’s note
All claims expressed in this article are solely those of the authors and do not necessarily represent those of their affiliated organizations, or those of the publisher, the editors and the reviewers. Any product that may be evaluated in this article, or claim that may be made by its manufacturer, is not guaranteed or endorsed by the publisher.
References
1. Xu G-Y, Yu H-A, Hong J, Stahl M, McDonagh T, Kay LE, et al. Solution structure of recombinant human interleukin-6 1 1Edited by P. E. Wright. J Mol Biol. (1997) 268:468–81. doi: 10.1006/jmbi.1997.0933
2. Varghese JN, Moritz RL, Lou M-Z, van Donkelaar A, Ji H, Ivancic N, et al. Structure of the extracellular domains of the human interleukin-6 receptor α-chain. Proc Natl Acad Sci. (2002) 99:15959–64. doi: 10.1073/pnas.232432399
3. Metcalfe RD, Putoczki TL, Griffin MDW. Structural understanding of interleukin 6 family cytokine signaling and targeted therapies: focus on interleukin 11. Front Immunol. (2020) 11:1424. doi: 10.3389/fimmu.2020.01424
4. Redondo-Castro E, Cunningham C, Miller J, Martuscelli L, Aoulad-Ali S, Rothwell NJ, et al. Interleukin-1 primes human mesenchymal stem cells towards an anti-inflammatory and pro-trophic phenotype. vitro Stem Cell Res Ther. (2017) 8:1–11. doi: 10.1186/s13287–017-0531–4
5. Gabay C. Interleukin-6 and chronic inflammation. Arthritis Res Ther. (2006) 8:S3. doi: 10.1186/ar1917
6. Tanaka T, Narazaki M, Kishimoto T. IL-6 in inflammation, immunity, and disease. Cold Spring Harb Perspect Biol. (2014) 6:a016295–a016295. doi: 10.1101/cshperspect.a016295
7. Mauer J, Denson JL, Brüning JC. Versatile functions for IL-6 in metabolism and cancer. Trends Immunol. (2015) 36:92–101. doi: 10.1016/j.it.2014.12.008
8. Velazquez-Salinas L, Verdugo-Rodriguez A, Rodriguez LL, Borca MV. The role of interleukin 6 during viral infections. Front Microbiol. (2019) 10:1057. doi: 10.3389/fmicb.2019.01057
9. Hirano T, Taga T, Matsuda T, Hibi M, Suematsu S, Tang B, et al. Interleukin 6 and its receptor in the immune response and hematopoiesis. Int J Cell Cloning. (1990) 8:155–67. doi: 10.1002/stem.5530080714
10. Peters M, Jacobs S, Ehlers M, Vollmer P, Müllberg J, Wolf E, et al. The function of the soluble interleukin 6 (IL-6) receptor in vivo: sensitization of human soluble IL-6 receptor transgenic mice towards IL-6 and prolongation of the plasma half-life of IL-6. J Exp Med. (1996) 183:1399–406. doi: 10.1084/jem.183.4.1399
11. Tie R, Li H, Cai S, Liang Z, Shan W, Wang B, et al. Interleukin-6 signaling regulates hematopoietic stem cell emergence. Exp Mol Med. (2019) 51:1–12. doi: 10.1038/s12276–019-0320–5
12. Collins A, Mitchell CA, Passegué E. Inflammatory signaling regulates hematopoietic stem and progenitor cell development and homeostasis. J Exp Med. (2021) 218(10):e20201545. doi: 10.1084/jem.20201545
13. Castell JV, Gómez-Lechón MJ, David M, Andus T, Geiger T, Trullenque R, et al. Interleukin-6 is the major regulator of acute phase protein synthesis in adult human hepatocytes. FEBS Lett. (1989) 242:237–9. doi: 10.1016/0014–5793(89)80476–4
14. Cartmell T, Poole S, Turnbull AV, Rothwell NJ, Luheshi GN. Circulating interleukin-6 mediates the febrile response to localised inflammation in rats. J Physiol. (2000) 526:653–61. doi: 10.1111/j.1469-7793.2000.00653.x
15. Jankord R, Zhang R, Flak JN, Solomon MB, Albertz J, Herman JP. Stress activation of IL-6 neurons in the hypothalamus. Am J Physiol Integr Comp Physiol. (2010) 299:R343–51. doi: 10.1152/ajpregu.00131.2010
16. Nilsberth C, Elander L, Hamzic N, Norell M, Lönn J, Engström L, et al. The role of interleukin-6 in lipopolysaccharide-induced fever by mechanisms independent of prostaglandin E2. Endocrinology. (2009) 150:1850–60. doi: 10.1210/en.2008–0806
17. Aliyu M, Zohora FT, Anka AU, Ali K, Maleknia S, Saffarioun M, et al. Interleukin-6 cytokine: An overview of the immune regulation, immune dysregulation, and therapeutic approach. Int Immunopharmacol. (2022) 111:109130. doi: 10.1016/j.intimp.2022.109130
18. Choy E, Rose-John S. Interleukin-6 as a multifunctional regulator: inflammation, immune response, and fibrosis. J Scleroderma Relat Disord. (2017) 2:S1–5. doi: 10.5301/jsrd.5000265
19. Jenkins RH, Hughes STO, Figueras AC, Jones SA. Unravelling the broader complexity of IL-6 involvement in health and disease. Cytokine. (2021) 148:155684. doi: 10.1016/j.cyto.2021.155684
20. Srirangan S, Choy EH. The role of Interleukin 6 in the pathophysiology of rheumatoid arthritis. Ther Adv Musculoskelet Dis. (2010) 2:247–56. doi: 10.1177/1759720X10378372
21. Strand V, Boklage SH, Kimura T, Joly F, Boyapati A, Msihid J. High levels of interleukin-6 in patients with rheumatoid arthritis are associated with greater improvements in health-related quality of life for sarilumab compared with adalimumab. Arthritis Res Ther. (2020) 22:250. doi: 10.1186/s13075–020-02344–3
22. Jarlborg M, Gabay C. Systemic effects of IL-6 blockade in rheumatoid arthritis beyond the joints. Cytokine. (2022) 149:155742. doi: 10.1016/j.cyto.2021.155742
23. Kuwabara T, Ishikawa F, Kondo M, Kakiuchi T. The role of IL-17 and related cytokines in inflammatory autoimmune diseases. Mediators Inflammation. (2017) 2017:1–11. doi: 10.1155/2017/3908061
24. Shahini A, Shahini A. Role of interleukin-6-mediated inflammation in the pathogenesis of inflammatory bowel disease: focus on the available therapeutic approaches and gut microbiome. J Cell Commun Signal. (2023) 17:55–74. doi: 10.1007/s12079-022-00695-x
25. Tackey E, Lipsky PE, Illei GG. Rationale for interleukin-6 blockade in systemic lupus erythematosus. Lupus. (2004) 13:339–43. doi: 10.1191/0961203304lu1023oa
26. Nepal D, Gazeley D. Role of IL-6 and IL-6 targeted therapy in systemic lupus erythematosus. Rheumatology. (2023) 62:3804–10. doi: 10.1093/rheumatology/kead416
27. Kumari N, Dwarakanath BS, Das A, Bhatt AN. Role of interleukin-6 in cancer progression and therapeutic resistance. Tumor Biol. (2016) 37:11553–72. doi: 10.1007/s13277–016-5098–7
28. Rašková M, Lacina L, Kejík Z, Venhauerová A, Skaličková M, Kolář M, et al. The role of IL-6 in cancer cell invasiveness and metastasis—Overview and therapeutic opportunities. Cells. (2022) 11:3698. doi: 10.3390/cells11223698
29. Orange ST, Leslie J, Ross M, Mann DA, Wackerhage H. The exercise IL-6 enigma in cancer. Trends Endocrinol Metab. (2023) 34:749–63. doi: 10.1016/j.tem.2023.08.001
30. Wertz MH, Pineda SS, Lee H, Kulicke R, Kellis M, Heiman M. Interleukin-6 deficiency exacerbates Huntington’s disease model phenotypes. Mol Neurodegener. (2020) 15:29. doi: 10.1186/s13024–020-00379–3
31. Saraiva M, Vieira P, O’Garra A. Biology and therapeutic potential of interleukin-10. J Exp Med. (2020) 217(1):e20190418. doi: 10.1084/jem.20190418
32. Yang Y, Zhang Y, Xing X, Xu G, Lin X, Wang Y, et al. IL-6 translation is a therapeutic target of human cytokine release syndrome. J Exp Med. (2023) 220(11):e20230577. doi: 10.1084/jem.20230577
33. Choy EH, De Benedetti F, Takeuchi T, Hashizume M, John MR, Kishimoto T. Translating IL-6 biology into effective treatments. Nat Rev Rheumatol. (2020) 16:335–45. doi: 10.1038/s41584-020-0419-z
34. Nolde M, Alayash Z, Reckelkamm SL, Kocher T, Ehmke B, Holtfreter B, et al. Downregulation of interleukin 6 signaling might reduce the risk of periodontitis: a drug target Mendelian randomization study. Front Immunol. (2023) 14:1160148. doi: 10.3389/fimmu.2023.1160148
35. Pricola KL, Kuhn NZ, Haleem-Smith H, Song Y, Tuan RS. Interleukin-6 maintains bone marrow-derived mesenchymal stem cell stemness by an ERK1/2-dependent mechanism. J Cell Biochem. (2009) 108:577–88. doi: 10.1002/jcb.22289
36. Dorronsoro A, Lang V, Ferrin I, Fernández-Rueda J, Zabaleta L, Pérez-Ruiz E, et al. Intracellular role of IL-6 in mesenchymal stromal cell immunosuppression and proliferation. Sci Rep. (2020) 10:21853. doi: 10.1038/s41598–020-78864–4
37. Xie Z, Tang S, Ye G, Wang P, Li J, Liu W, et al. Interleukin-6/interleukin-6 receptor complex promotes osteogenic differentiation of bone marrow-derived mesenchymal stem cells. Stem Cell Res Ther. (2018) 9:13. doi: 10.1186/s13287–017-0766–0
38. Viveiros MMH, Viveiros ME de M, Silva MG, Kaneno R, Avelino NP, Rainho CA, et al. Expression of inflammatory cytokines in mesenchymal stem cells derived from proximal humerus fractures. Stem Cell Investig. (2022) 9:3–3. doi: 10.21037/sci-2021–031
39. Grebenciucova E, VanHaerents S. Interleukin 6: at the interface of human health and disease. Front Immunol. (2023) 14:1255533. doi: 10.3389/fimmu.2023.1255533
40. Gupta D, Orehek S, Turunen J, O’Donovan L, Gait MJ, El-Andaloussi S, et al. Modulation of pro-inflammatory IL-6 trans-signaling axis by splice switching oligonucleotides as a therapeutic modality in inflammation. Cells. (2023) 12:2285. doi: 10.3390/cells12182285
41. Rothaug M, Becker-Pauly C, Rose-John S. The role of interleukin-6 signaling in nervous tissue. Biochim Biophys Acta - Mol Cell Res. (2016) 1863:1218–27. doi: 10.1016/j.bbamcr.2016.03.018
42. Yang J, Ran M, Li H, Lin Y, Ma K, Yang Y, et al. New insight into neurological degeneration: Inflammatory cytokines and blood–brain barrier. Front Mol Neurosci. (2022) 15:1013933. doi: 10.3389/fnmol.2022.1013933
43. Lyra e Silva NM, Gonçalves RA, Pascoal TA, Lima-Filho RAS, Resende E de PF, Vieira ELM, et al. Pro-inflammatory interleukin-6 signaling links cognitive impairments and peripheral metabolic alterations in Alzheimer’s disease. Transl Psychiatry. (2021) 11:251. doi: 10.1038/s41398-021-01349-z
44. Hu J, Zhang Y, Huang C, Feng X, He S, Zhang Y, et al. Interleukin-6 trans-signalling in hippocampal CA1 neurones mediates perioperative neurocognitive disorders in mice. Br J Anaesth. (2022) 129:923–36. doi: 10.1016/j.bja.2022.08.019
45. Vai B, Palladini M, Lorenzi C, Zanardi R, Poletti S, Aggio V, et al. Interleukin 6 associates with reduced grey matter volume and resting-state connectivity in the anterior cingulate cortex in bipolar patients. Brain Behav Immun - Heal. (2022) 26:100522. doi: 10.1016/j.bbih.2022.100522
46. Cheteh EH, Sarne V, Ceder S, Bianchi J, Augsten M, Rundqvist H, et al. Interleukin-6 derived from cancer-associated fibroblasts attenuates the p53 response to doxorubicin in prostate cancer cells. Cell Death Discovery. (2020) 6:42. doi: 10.1038/s41420–020-0272–5
47. Rose-John S. The biology of interleukin-6 in the 21st century. Semin Immunol. (2014) 26:1. doi: 10.1016/j.smim.2014.01.012
48. Rose-John S. The soluble interleukin 6 receptor: advanced therapeutic options in inflammation. Clin Pharmacol Ther. (2017) 102:591–8. doi: 10.1002/cpt.782
49. Rose-John S, Jenkins BJ, Garbers C, Moll JM, Scheller J. Targeting IL-6 trans-signalling: past, present and future prospects. Nat Rev Immunol. (2023) 23:666–81. doi: 10.1038/s41577-023-00856-y
50. Reeh H, Rudolph N, Billing U, Christen H, Streif S, Bullinger E, et al. Response to IL-6 trans- and IL-6 classic signalling is determined by the ratio of the IL-6 receptor α to gp130 expression: fusing experimental insights and dynamic modelling. Cell Commun Signal. (2019) 17:46. doi: 10.1186/s12964–019-0356–0
51. Nishikai-Yan Shen T, Kanazawa S, Kado M, Okada K, Luo L, Hayashi A, et al. Interleukin-6 stimulates Akt and p38 MAPK phosphorylation and fibroblast migration in non-diabetic but not diabetic mice. PloS One. (2017) 12:e0178232. doi: 10.1371/journal.pone.0178232
52. Uciechowski P, Dempke WCM. Interleukin-6: A masterplayer in the cytokine network. Oncology. (2020) 98:131–7. doi: 10.1159/000505099
53. Ding J, Yang C, Cheng Y, Wang J, Zhang S, Yan S, et al. Trophoblast-derived IL-6 serves as an important factor for normal pregnancy by activating Stat3-mediated M2 macrophages polarization. Int Immunopharmacol. (2021) 90:106788. doi: 10.1016/j.intimp.2020.106788
54. Wooldridge LK, Ealy AD. Interleukin-6 promotes primitive endoderm development in bovine blastocysts. BMC Dev Biol. (2021) 21:3. doi: 10.1186/s12861-020-00235-z
55. Wooldridge LK, Ealy AD. Interleukin-6 increases inner cell mass numbers in bovine embryos. BMC Dev Biol. (2019) 19:2. doi: 10.1186/s12861-019-0182-z
56. Zhong Y, Choi T, Kim M, Jung KH, Chai YG, Binas B. Isolation of primitive mouse extraembryonic endoderm (pXEN) stem cell lines. Stem Cell Res. (2018) 30:100–12. doi: 10.1016/j.scr.2018.05.008
57. Dedier M, Magne B, Nivet M, Banzet S, Trouillas M. Anti-inflammatory effect of interleukin-6 highly enriched in secretome of two clinically relevant sources of mesenchymal stromal cells. Front Cell Dev Biol. (2023) 11:1244120. doi: 10.3389/fcell.2023.1244120
58. Wu W-L, Hsiao EY, Yan Z, Mazmanian SK, Patterson PH. The placental interleukin-6 signaling controls fetal brain development and behavior. Brain Behav Immun. (2017) 62:11–23. doi: 10.1016/j.bbi.2016.11.007
59. Vilotić A, Nacka-Aleksić M, Pirković A, Bojić-Trbojević Ž, Dekanski D, Jovanović Krivokuća M. IL-6 and IL-8: an overview of their roles in healthy and pathological pregnancies. Int J Mol Sci. (2022) 23:14574. doi: 10.3390/ijms232314574
60. Schmitt RM, Bruyns E, Snodgrass HR. Hematopoietic development of embryonic stem cells in vitro: cytokine and receptor gene expression. Genes Dev. (1991) 5:728–40. doi: 10.1101/gad.5.5.728
61. Seo Y, Shin K-H, Kim HH, Kim H-S. Current advances in red blood cell generation using stem cells from diverse sources. Stem Cells Int. (2019) 2019:1–10. doi: 10.1155/2019/9281329
62. Yao JS, Zhai W, Young WL, Yang G-Y. Interleukin-6 triggers human cerebral endothelial cells proliferation and migration: The role for KDR and MMP-9. Biochem Biophys Res Commun. (2006) 342:1396–404. doi: 10.1016/j.bbrc.2006.02.100
63. Johnson BZ, Stevenson AW, Prêle CM, Fear MW, Wood FM. The role of IL-6 in skin fibrosis and cutaneous wound healing. Biomedicines. (2020) 8:101. doi: 10.3390/biomedicines8050101
64. Li J, Tan J, Martino MM, Lui KO. Regulatory T-cells: potential regulator of tissue repair and regeneration. Front Immunol. (2018) 9:585. doi: 10.3389/fimmu.2018.00585
65. Bakker AD, Jaspers RT. IL-6 and IGF-1 signaling within and between muscle and bone: how important is the mTOR pathway for bone metabolism? Curr Osteoporos Rep. (2015) 13:131–9. doi: 10.1007/s11914–015-0264–1
66. Kami K, Senba E. Localization of leukemia inhibitory factor and interleukin-6 messenger ribonucleic acids in regenerating rat skeletal muscle. Muscle Nerve. (1998) 21:819–22. doi: 10.1002/(SICI)1097–4598(199806)21:6<819::AID-MUS20>3.0.CO;2-M
67. Joe AWB, Yi L, Natarajan A, Le Grand F, So L, Wang J, et al. Muscle injury activates resident fibro/adipogenic progenitors that facilitate myogenesis. Nat Cell Biol. (2010) 12:153–63. doi: 10.1038/ncb2015
68. Zhang C, Li Y, Wu Y, Wang L, Wang X, Du J. Interleukin-6/signal transducer and activator of transcription 3 (STAT3) pathway is essential for macrophage infiltration and myoblast proliferation during muscle regeneration. J Biol Chem. (2013) 288:1489–99. doi: 10.1074/jbc.M112.419788
69. Muñoz-Cánoves P, Scheele C, Pedersen BK, Serrano AL. Interleukin-6 myokine signaling in skeletal muscle: a double-edged sword? FEBS J. (2013) 280:4131–48. doi: 10.1111/febs.12338
70. Kistner TM, Pedersen BK, Lieberman DE. Interleukin 6 as an energy allocator in muscle tissue. Nat Metab. (2022) 4:170–9. doi: 10.1038/s42255–022-00538–4
71. Pons-Espinal M, Blasco-Agell L, Fernandez-Carasa I, Andrés-Benito P, di Domenico A, Richaud-Patin Y, et al. Blocking IL-6 signaling prevents astrocyte-induced neurodegeneration in an iPSC-based model of Parkinson’s disease. JCI Insight. (2024) 9(3):e163359. doi: 10.1172/jci.insight.163359
72. Diaz K, Kohut ML, Russell DW, Stegemöller EL. Peripheral inflammatory cytokines and motor symptoms in persons with Parkinson’s disease. Brain Behav Immun - Heal. (2022) 21:100442. doi: 10.1016/j.bbih.2022.100442
73. Eide S, Misztal M, Feng Z-P. Interleukin-6 as a marker of Huntington’s disease progression: Systematic review and meta-analysis. Brain Behav Immun - Heal. (2023) 30:100635. doi: 10.1016/j.bbih.2023.100635
74. Bensadoun J-C, De Almeida LP, Dréano M, Aebischer P, Déglon N. Neuroprotective effect of interleukin-6 and IL6/IL6R chimera in the quinolinic acid rat model of Huntington’s syndrome. Eur J Neurosci. (2001) 14:1753–61. doi: 10.1046/j.0953-816x.2001.01802.x
75. Petkovic F, Castellano B. The role of interleukin-6 in central nervous system demyelination. Neural Regener Res. (2016) 11:1922. doi: 10.4103/1673–5374.195273
76. Stampanoni Bassi M, Iezzi E, Drulovic J, Pekmezovic T, Gilio L, Furlan R, et al. IL-6 in the cerebrospinal fluid signals disease activity in multiple sclerosis. Front Cell Neurosci. (2020) 14:120. doi: 10.3389/fncel.2020.00120
77. Wosiski-Kuhn M, Caress JB, Cartwright MS, Hawkins GA, Milligan C. Interleukin 6 (IL6) level is a biomarker for functional disease progression within IL6R 358 Ala variant groups in amyotrophic lateral sclerosis patients. Amyotroph Lateral Scler Front Degener. (2021) 22:248–59. doi: 10.1080/21678421.2020.1813310
78. Pronto-Laborinho A, Pinto S, Gromicho M, Pereira M, Swash M, de Carvalho M. Interleukin-6 and amyotrophic lateral sclerosis. J Neurol Sci. (2019) 398:50–3. doi: 10.1016/j.jns.2019.01.026
79. Lin W, Song H, Shen J, Wang J, Yang Y, Yang Y, et al. Functional role of skeletal muscle-derived interleukin-6 and its effects on lipid metabolism. Front Physiol. (2023) 14:1110926. doi: 10.3389/fphys.2023.1110926
80. Spooren A, Kolmus K, Laureys G, Clinckers R, De Keyser J, Haegeman G, et al. Interleukin-6, a mental cytokine. Brain Res Rev. (2011) 67:157–83. doi: 10.1016/j.brainresrev.2011.01.002
81. Kinney JW, Bemiller SM, Murtishaw AS, Leisgang AM, Salazar AM, Lamb BT. Inflammation as a central mechanism in Alzheimer’s disease. Alzheimer’s Dement Transl Res Clin Interv. (2018) 4:575–90. doi: 10.1016/j.trci.2018.06.014
82. Kaur D, Sharma V, Deshmukh R. Activation of microglia and astrocytes: a roadway to neuroinflammation and Alzheimer’s disease. Inflammopharmacology. (2019) 27:663–77. doi: 10.1007/s10787-019-00580-x
83. Shan C, Zhang C, Zhang C. The role of IL-6 in neurodegenerative disorders. Neurochem Res. (2024) 49:834–46. doi: 10.1007/s11064–023-04085–6
84. Huang Z, Wong L-W, Su Y, Huang X, Wang N, Chen H, et al. Blood-brain barrier integrity in the pathogenesis of Alzheimer’s disease. Front Neuroendocrinol. (2020) 59:100857. doi: 10.1016/j.yfrne.2020.100857
85. Takeshita Y, Fujikawa S, Serizawa K, Fujisawa M, Matsuo K, Nemoto J, et al. New BBB model reveals that IL-6 blockade suppressed the BBB disorder, preventing onset of NMOSD. Neurol Neuroimmunol Neuroinflamm. (2021) 8(6):e1076. doi: 10.1212/NXI.0000000000001076
86. März P, Cheng J-G, Gadient RA, Patterson PH, Stoyan T, Otten U, et al. Sympathetic neurons can produce and respond to interleukin 6. Proc Natl Acad Sci. (1998) 95:3251–6. doi: 10.1073/pnas.95.6.3251
87. Islam O, Gong X, Rose-John S, Heese K. Interleukin-6 and neural stem cells: More than gliogenesis. Mol Biol Cell. (2009) 20:188–99. doi: 10.1091/mbc.e08–05-0463
88. Mousa A, Bakhiet M. Role of cytokine signaling during nervous system development. Int J Mol Sci. (2013) 14:13931–57. doi: 10.3390/ijms140713931
89. Kummer KK, Zeidler M, Kalpachidou T, Kress M. Role of IL-6 in the regulation of neuronal development, survival and function. Cytokine. (2021) 144:155582. doi: 10.1016/j.cyto.2021.155582
90. Kerkis I, Araldi R, Wenceslau C, Mendes T. Advances in cellular and cell-free therapy medicinal products for Huntigton’s disease treatment. From Physiopatol to Treat Huntigton’s Dis. (2022), 1–27. doi: 10.5772/55358
91. Palpagama TH, Waldvogel HJ, Faull RLM, Kwakowsky A. The role of microglia and astrocytes in Huntington’s disease. Front Mol Neurosci. (2019) 12:258. doi: 10.3389/fnmol.2019.00258
92. Jia Q, Li S, Li X-J, Yin P. Neuroinflammation in Huntington’s disease: From animal models to clinical therapeutics. Front Immunol. (2022) 13:1088124. doi: 10.3389/fimmu.2022.1088124
93. Qiu Z, Parsons K, Gruol D. Interleukin-6 selectively enhances the intracellular calcium response to NMDA in developing CNS neurons. J Neurosci. (1995) 15:6688–99. doi: 10.1523/JNEUROSCI.15–10-06688.1995
94. Wilton DK, Stevens B. The contribution of glial cells to Huntington’s disease pathogenesis. Neurobiol Dis. (2020) 143:104963. doi: 10.1016/j.nbd.2020.104963
95. Saba J, Couselo FL, Bruno J, Carniglia L, Durand D, Lasaga M, et al. Neuroinflammation in huntington’s disease: A starring role for astrocyte and microglia. Curr Neuropharmacol. (2022) 20:1116–43. doi: 10.2174/1570159X19666211201094608
96. Wild E, Magnusson A, Lahiri N, Krus U, Orth M, Tabrizi SJ, et al. Abnormal peripheral chemokine profile in Huntington’s disease. PloS Curr. (2011) 3:RRN1231. doi: 10.1371/currents.RRN1231
97. Träger U, Andre R, Lahiri N, Magnusson-Lind A, Weiss A, Grueninger S, et al. HTT-lowering reverses Huntington’s disease immune dysfunction caused by NFκB pathway dysregulation. Brain. (2014) 137:819–33. doi: 10.1093/brain/awt355
98. Raper J, Bosinger S, Johnson Z, Tharp G, Moran SP, Chan AWS. Increased irritability, anxiety, and immune reactivity in transgenic Huntington’s disease monkeys. Brain Behav Immun. (2016) 58:181–90. doi: 10.1016/j.bbi.2016.07.004
99. Gómez-Jaramillo L, Cano-Cano F, del Carmen González-Montelongo M, Campos-Caro A, Aguilar-Diosdado M, Arroba AI. A new perspective on huntington’s disease: how a neurological disorder influences the peripheral tissues. Int J Mol Sci. (2022) 23:6089. doi: 10.3390/ijms23116089
100. Jiang A, Handley RR, Lehnert K, Snell RG. From pathogenesis to therapeutics: A review of 150 years of huntington’s disease research. Int J Mol Sci. (2023) 24:13021. doi: 10.3390/ijms241613021
101. Conroy SM, Nguyen V, Quina LA, Blakely-Gonzales P, Ur C, Netzeband JG, et al. Interleukin-6 produces neuronal loss in developing cerebellar granule neuron cultures. J Neuroimmunol. (2004) 155:43–54. doi: 10.1016/j.jneuroim.2004.06.014
102. Ma J, Gao J, Niu M, Zhang X, Wang J, Xie A. P2X4R overexpression upregulates interleukin-6 and exacerbates 6-OHDA-induced dopaminergic degeneration in a rat model of PD. Front Aging Neurosci. (2020) 12:580068. doi: 10.3389/fnagi.2020.580068
103. Dufek M, Rektorova I, Thon V, Lokaj J, Rektor I. Interleukin-6 may contribute to mortality in parkinson’s disease patients: A 4-year prospective study. Parkinsons Dis. (2015) 2015:1–5. doi: 10.1155/2015/898192
104. Scalzo P, Kümmer A, Cardoso F, Teixeira AL. Serum levels of interleukin-6 are elevated in patients with Parkinson’s disease and correlate with physical performance. Neurosci Lett. (2010) 468:56–8. doi: 10.1016/j.neulet.2009.10.062
105. Kozina E, Byrne M, Smeyne RJ. Mutant LRRK2 in lymphocytes regulates neurodegeneration via IL-6 in an inflammatory model of Parkinson’s disease. NPJ Park Dis. (2022) 8:24. doi: 10.1038/s41531–022-00289–9
106. Sterling JK, Kam T-I, Guttha S, Park H, Baumann B, Mehrabani-Tabari AA, et al. Interleukin-6 triggers toxic neuronal iron sequestration in response to pathological α-synuclein. Cell Rep. (2022) 38:110358. doi: 10.1016/j.celrep.2022.110358
107. Müller T, Blum-Degen D, Przuntek H, Kuhn W. Short communication Interleukin-6 levels in cerebrospinal fluid inversely correlate to severity of Parkinson’s disease. Acta Neurol Scand. (2009) 98:142–4. doi: 10.1111/j.1600–0404.1998.tb01736.x
108. Qin X-Y, Zhang S-P, Cao C, Loh YP, Cheng Y. Aberrations in peripheral inflammatory cytokine levels in parkinson disease. JAMA Neurol. (2016) 73:1316. doi: 10.1001/jamaneurol.2016.2742
109. Karpenko MN, Vasilishina AA, Gromova EA, Muruzheva ZM, Bernadotte A. Interleukin-1β, interleukin-1 receptor antagonist, interleukin-6, interleukin-10, and tumor necrosis factor-α levels in CSF and serum in relation to the clinical diversity of Parkinson’s disease. Cell Immunol. (2018) 327:77–82. doi: 10.1016/j.cellimm.2018.02.011
110. Dzamko N. Cytokine activity in Parkinson’s disease. Neuronal Signal. (2023) 7(4):NS20220063. doi: 10.1042/NS20220063
111. de Vries HE, Blom-Roosemalen MCM, van Oosten M, de Boer AG, van Berkel TJC, Breimer DD, et al. The influence of cytokines on the integrity of the blood-brain barrier in vitro. J Neuroimmunol. (1996) 64:37–43. doi: 10.1016/0165–5728(95)00148–4
112. Wong D, Dorovini-Zis K, Vincent SR. Cytokines, nitric oxide, and cGMP modulate the permeability of an in vitro model of the human blood–brain barrier. Exp Neurol. (2004) 190:446–55. doi: 10.1016/j.expneurol.2004.08.008
113. Kortekaas R, Leenders KL, van Oostrom JCH, Vaalburg W, Bart J, Willemsen ATM, et al. Blood–brain barrier dysfunction in parkinsonian midbrain. vivo. Ann Neurol. (2005) 57:176–9. doi: 10.1002/ana.20369
114. Al-Bachari S, Naish JH, Parker GJM, Emsley HCA, Parkes LM. Blood–brain barrier leakage is increased in parkinson’s disease. Front Physiol. (2020) 11:593026. doi: 10.3389/fphys.2020.593026
115. Gray MT, Woulfe JM. Striatal blood–brain barrier permeability in parkinson’S disease. J Cereb Blood Flow Metab. (2015) 35:747–50. doi: 10.1038/jcbfm.2015.32
116. Brodacki B, Staszewski J, Toczyłowska B, Kozłowska E, Drela N, Chalimoniuk M, et al. Serum interleukin (IL-2, IL-10, IL-6, IL-4), TNFα, and INFγ concentrations are elevated in patients with atypical and idiopathic parkinsonism. Neurosci Lett. (2008) 441:158–62. doi: 10.1016/j.neulet.2008.06.040
117. Fu J, Chen S, Liu J, Yang J, Ou R, Zhang L, et al. Serum inflammatory cytokines levels and the correlation analyses in Parkinson’s disease. Front Cell Dev Biol. (2023) 11:1104393. doi: 10.3389/fcell.2023.1104393
118. Wei W, Ao Q, Wang X, Cao Y, Liu Y, Zheng SG, et al. Mesenchymal stem cell–derived exosomes: A promising biological tool in nanomedicine. Front Pharmacol. (2021) 11:590470. doi: 10.3389/fphar.2020.590470
119. Gu Y, He M, Zhou X, Liu J, Hou N, Bin T, et al. Endogenous IL-6 of mesenchymal stem cell improves behavioral outcome of hypoxic-ischemic brain damage neonatal rats by supressing apoptosis in astrocyte. Sci Rep. (2016) 6:18587. doi: 10.1038/srep18587
120. Philipp D, Suhr L, Wahlers T, Choi Y-H, Paunel-Görgülü A. Preconditioning of bone marrow-derived mesenchymal stem cells highly strengthens their potential to promote IL-6-dependent M2b polarization. Stem Cell Res Ther. (2018) 9:286. doi: 10.1186/s13287–018-1039–2
121. Huang P, Zhang C, Delawary M, Korchak JA, Suda K, Zubair AC. Development and evaluation of IL-6 overexpressing mesenchymal stem cells (MSCs). J Tissue Eng Regener Med. (2022) 16:244–53. doi: 10.1002/term.3274
122. Kitzberger C, Shehzad K, Morath V, Spellerberg R, Ranke J, Steiger K, et al. Interleukin-6-controlled, mesenchymal stem cell-based sodium/iodide symporter gene therapy improves survival of glioblastoma-bearing mice. Mol Ther - Oncolytics. (2023) 30:238–53. doi: 10.1016/j.omto.2023.08.004
123. Li N, Hua J. Interactions between mesenchymal stem cells and the immune system. Cell Mol Life Sci. (2017) 74:2345–60. doi: 10.1007/s00018–017-2473–5
124. Lee S, Chae D-S, Song B-W, Lim S, Kim SW, Kim I-K, et al. ADSC-based cell therapies for musculoskeletal disorders: A review of recent clinical trials. Int J Mol Sci. (2021) 22:10586. doi: 10.3390/ijms221910586
125. Hofer HR, Tuan RS. Secreted trophic factors of mesenchymal stem cells support neurovascular and musculoskeletal therapies. Stem Cell Res Ther. (2016) 7:131. doi: 10.1186/s13287–016-0394–0
126. Lopez-Santalla M, Fernandez-Perez R, Garin MI. Mesenchymal stem/stromal cells for rheumatoid arthritis treatment: An update on clinical applications. Cells. (2020) 9:1852. doi: 10.3390/cells9081852
127. Molnar V, Pavelić E, Vrdoljak K, Čemerin M, Klarić E, Matišić V, et al. Mesenchymal stem cell mechanisms of action and clinical effects in osteoarthritis: A narrative review. Genes (Basel). (2022) 13:949. doi: 10.3390/genes13060949
128. Gerami MH, Khorram R, Rasoolzadegan S, Mardpour S, Nakhaei P, Hashemi S, et al. Emerging role of mesenchymal stem/stromal cells (MSCs) and MSCs-derived exosomes in bone- and joint-associated musculoskeletal disorders: a new frontier. Eur J Med Res. (2023) 28:86. doi: 10.1186/s40001–023-01034–5
129. Fisher DT, Appenheimer MM, Evans SS. The two faces of IL-6 in the tumor microenvironment. Semin Immunol. (2014) 26:38–47. doi: 10.1016/j.smim.2014.01.008
130. Chen L, Wang S, Wang Y, Zhang W, Ma K, Hu C, et al. IL-6 influences the polarization of macrophages and the formation and growth of colorectal tumor. Oncotarget. (2018) 9:17443–54. doi: 10.18632/oncotarget.24734
131. Španko M, Strnadová K, Pavlíček AJ, Szabo P, Kodet O, Valach J, et al. IL-6 in the ecosystem of head and neck cancer: possible therapeutic perspectives. Int J Mol Sci. (2021) 22:11027. doi: 10.3390/ijms222011027
132. Alvites R, Branquinho M, Sousa AC, Lopes B, Sousa P, Maurício AC. Mesenchymal stem/stromal cells and their paracrine activity—Immunomodulation mechanisms and how to influence the therapeutic potential. Pharmaceutics. (2022) 14:381. doi: 10.3390/pharmaceutics14020381
133. Quillen D, Hughes TM, Craft S, Howard T, Register T, Suerken C, et al. Levels of soluble interleukin 6 receptor and asp358Ala are associated with cognitive performance and alzheimer disease biomarkers. Neurol Neuroimmunol Neuroinflamm. (2023) 10(3):e200095. doi: 10.1212/NXI.0000000000200095
134. Lee J, Nakagiri T, Oto T, Harada M, Morii E, Shintani Y, et al. IL-6 amplifier, NF-κB–triggered positive feedback for IL-6 signaling, in grafts is involved in allogeneic rejection responses. J Immunol. (2012) 189:1928–36. doi: 10.4049/jimmunol.1103613
135. Nguyen HN, Noss EH, Mizoguchi F, Huppertz C, Wei KS, Watts GFM, et al. Autocrine loop involving IL-6 family member LIF, LIF receptor, and STAT4 drives sustained fibroblast production of inflammatory mediators. Immunity. (2017) 46:220–32. doi: 10.1016/j.immuni.2017.01.004
136. Billing U, Jetka T, Nortmann L, Wundrack N, Komorowski M, Waldherr S, et al. Robustness and information transfer within IL-6-induced JAK/STAT signalling. Commun Biol. (2019) 2:27. doi: 10.1038/s42003–018-0259–4
137. Song N, Scholtemeijer M, Shah K. Mesenchymal stem cell immunomodulation: mechanisms and therapeutic potential. Trends Pharmacol Sci. (2020) 41:653–64. doi: 10.1016/j.tips.2020.06.009
138. Toh WS, Lai RC, Hui JHP, Lim SK. MSC exosome as a cell-free MSC therapy for cartilage regeneration: Implications for osteoarthritis treatment. Semin Cell Dev Biol. (2017) 67:56–64. doi: 10.1016/j.semcdb.2016.11.008
139. Glenn JD. Mesenchymal stem cells: Emerging mechanisms of immunomodulation and therapy. World J Stem Cells. (2014) 6:526. doi: 10.4252/wjsc.v6.i5.526
140. Dabrowska S, Andrzejewska A, Janowski M, Lukomska B. Immunomodulatory and regenerative effects of mesenchymal stem cells and extracellular vesicles: therapeutic outlook for inflammatory and degenerative diseases. Front Immunol. (2021) 11:591065. doi: 10.3389/fimmu.2020.591065
141. Saadh MJ, Mikhailova MV, Rasoolzadegan S, Falaki M, Akhavanfar R, Gonzáles JLA, et al. Therapeutic potential of mesenchymal stem/stromal cells (MSCs)-based cell therapy for inflammatory bowel diseases (IBD) therapy. Eur J Med Res. (2023) 28:47. doi: 10.1186/s40001–023-01008–7
142. Tian C-M, Zhang Y, Yang M-F, Xu H-M, Zhu M-Z, Yao J, et al. Stem cell therapy in inflammatory bowel disease: A review of achievements and challenges. J Inflammation Res. (2023) 16:2089–119. doi: 10.2147/JIR.S400447
143. Han Y, Yang J, Fang J, Zhou Y, Candi E, Wang J, et al. The secretion profile of mesenchymal stem cells and potential applications in treating human diseases. Signal Transduct Target Ther. (2022) 7:92. doi: 10.1038/s41392–022-00932–0
144. Jin Q-H, Kim H-K, Na J-Y, Jin C, Seon J-K. Anti-inflammatory effects of mesenchymal stem cell-conditioned media inhibited macrophages activation in vitro. Sci Rep. (2022) 12:4754. doi: 10.1038/s41598–022-08398–4
145. Negi N, Griffin MD. Effects of mesenchymal stromal cells on regulatory T cells: Current understanding and clinical relevance. Stem Cells. (2020) 38:596–605. doi: 10.1002/stem.3151
146. An N, Yang J, Wang H, Sun S, Wu H, Li L, et al. Mechanism of mesenchymal stem cells in spinal cord injury repair through macrophage polarization. Cell Biosci. (2021) 11:41. doi: 10.1186/s13578-021-00554-z
147. Pang Q-M, Chen S-Y, Fu S-P, Zhou H, Zhang Q, Ao J, et al. Regulatory role of mesenchymal stem cells on secondary inflammation in spinal cord injury. J Inflammation Res. (2022) 15:573–93. doi: 10.2147/JIR.S349572
148. Wang Y, Fang J, Liu B, Shao C, Shi Y. Reciprocal regulation of mesenchymal stem cells and immune responses. Cell Stem Cell. (2022) 29:1515–30. doi: 10.1016/j.stem.2022.10.001
149. Li P, Ou Q, Shi S, Shao C. Immunomodulatory properties of mesenchymal stem cells/dental stem cells and their therapeutic applications. Cell Mol Immunol. (2023) 20:558–69. doi: 10.1038/s41423-023-00998-y
150. Narazaki M, Kishimoto T. Current status and prospects of IL-6–targeting therapy. Expert Rev Clin Pharmacol. (2022) 15:575–92. doi: 10.1080/17512433.2022.2097905
151. Xu Y, Wang N, Shen X, Liu X, Liu H, Liu Y. Persistent lymphocyte reduction and interleukin-6 levels are independently associated with death in patients with COVID-19. Clin Exp Med. (2023) (7):3719-3728. doi: 10.1007/s10238–023-01114–0
152. Huang P, Gebhart N, Richelson E, Brott TG, Meschia JF, Zubair AC. Mechanism of mesenchymal stem cell–induced neuron recovery and anti-inflammation. Cytotherapy. (2014) 16:1336–44. doi: 10.1016/j.jcyt.2014.05.007
153. Shokati A, Naser Moghadasi A, Nikbakht M, Sahraian MA, Mousavi SA, Ai J. A focus on allogeneic mesenchymal stromal cells as a versatile therapeutic tool for treating multiple sclerosis. Stem Cell Res Ther. (2021) 12:400. doi: 10.1186/s13287–021-02477–5
154. Badyra B, Sułkowski M, Milczarek O, Majka M. Mesenchymal stem cells as a multimodal treatment for nervous system diseases. Stem Cells Transl Med. (2020) 9:1174–89. doi: 10.1002/sctm.19–0430
155. Gao F, Chiu SM, Motan DAL, Zhang Z, Chen L, Ji H-L, et al. Mesenchymal stem cells and immunomodulation: current status and future prospects. Cell Death Dis. (2016) 7:e2062–2. doi: 10.1038/cddis.2015.327
156. Bona D, Scapagnini G, Candore G, Castiglia L, Colonna-Romano G, Duro G, et al. Immune-inflammatory responses and oxidative stress in alzheimers disease: therapeutic implications. Curr Pharm Des. (2010) 16:684–91. doi: 10.2174/138161210790883769
157. He J, Liu J, Huang Y, Tang X, Xiao H, Hu Z. Oxidative stress, inflammation, and autophagy: potential targets of mesenchymal stem cells-based therapies in ischemic stroke. Front Neurosci. (2021) 15:641157. doi: 10.3389/fnins.2021.641157
158. Guo Z, Zhong W, Zou Z. miR-98–5p prevents hippocampal neurons from oxidative stress and apoptosis by targeting STAT3 in epilepsy in vitro. Neuropsychiatr Dis Treat. (2023) 19:2319–29. doi: 10.2147/NDT.S415597
159. Hou G, Lv Z, Liu W, Xiong S, Zhang Q, Li C, et al. An aquatic virus exploits the IL6-STAT3-HSP90 signaling axis to promote viral entry. PloS Pathog. (2023) 19:e1011320. doi: 10.1371/journal.ppat.1011320
160. Gruol DL. IL-6 regulation of synaptic function in the CNS. Neuropharmacology. (2015) 96:42–54. doi: 10.1016/j.neuropharm.2014.10.023
161. Hernandez RV, Puro AC, Manos JC, Huitron-Resendiz S, Reyes KC, Liu K, et al. Transgenic mice with increased astrocyte expression of IL-6 show altered effects of acute ethanol on synaptic function. Neuropharmacology. (2016) 103:27–43. doi: 10.1016/j.neuropharm.2015.12.015
162. Wareham LK, Echevarria FD, Sousa JL, Konlian DO, Dallas G, Formichella CR, et al. Interleukin-6 promotes microtubule stability in axons via Stat3 protein–protein interactions. iScience. (2021) 24:103141. doi: 10.1016/j.isci.2021.103141
163. Fischer D. Hyper-IL-6: a potent and efficacious stimulator of RGC regeneration. Eye. (2017) 31:173–8. doi: 10.1038/eye.2016.234
164. Leibinger M, Zeitler C, Gobrecht P, Andreadaki A, Gisselmann G, Fischer D. Transneuronal delivery of hyper-interleukin-6 enables functional recovery after severe spinal cord injury in mice. Nat Commun. (2021) 12:391. doi: 10.1038/s41467–020-20112–4
165. D’arcangelo G, Tancredi V, Onofri F, D’antuono M, Giovedì S, Benfenati F. Interleukin-6 inhibits neurotransmitter release and the spread of excitation in the rat cerebral cortex. Eur J Neurosci. (2000) 12:1241–52. doi: 10.1046/j.1460–9568.2000.00011.x
166. Erta M, Quintana A, Hidalgo J. Interleukin-6, a major cytokine in the central nervous system. Int J Biol Sci. (2012) 8:1254–66. doi: 10.7150/ijbs.4679
Keywords: interleukin-6 (IL-6), mesenchymal stem cells (MSCs), IL-6 dysregulation, neurodegenerative diseases, Huntington disease (HD), stroke
Citation: Kerkis I, Silva ÁPd and Araldi RP (2024) The impact of interleukin-6 (IL-6) and mesenchymal stem cell-derived IL-6 on neurological conditions. Front. Immunol. 15:1400533. doi: 10.3389/fimmu.2024.1400533
Received: 13 March 2024; Accepted: 04 June 2024;
Published: 24 June 2024.
Edited by:
Tomasz Kordula, Virginia Commonwealth University, United StatesReviewed by:
Lutz Graeve, University of Hohenheim, GermanyMasanori A. Murayama, Kansai Medical University, Japan
Copyright © 2024 Kerkis, Silva and Araldi. This is an open-access article distributed under the terms of the Creative Commons Attribution License (CC BY). The use, distribution or reproduction in other forums is permitted, provided the original author(s) and the copyright owner(s) are credited and that the original publication in this journal is cited, in accordance with accepted academic practice. No use, distribution or reproduction is permitted which does not comply with these terms.
*Correspondence: Irina Kerkis, irina.kerkis@butantan.gov.br