- 1Departament of Pharmacology, Faculty of Medicine; Institute of Biomedical Research of Malaga (IBIMA), Regional University Hospital of Malaga, Malaga, Spain
- 2Department of Pharmacology and Pediatry, Faculty of Medicine, University of Malaga, Malaga, Spain
- 3Danish Multiple Sclerosis Center, Department of Neurology, Copenhagen University Hospital, Rigshospitalet, Copenhagen, Denmark
- 4Copenhagen and Department of Clinical Medicine, Faculty of Health and Medical Sciences, University of Copenhagen, Copenhagen, Denmark
- 5Department of Neurorehabilitation Sciences, Multiple Sclerosis Centre Casa di Cura Igea, Milan, Italy
- 6University Vita-Salute San Raffaele, Milan, Italy
- 7Univ. Lille, Inserm U1172 LilNCog, CHU Lille, FHU Precise, Lille, France
- 8Department of Neurology, Medical Faculty, Heinrich-Heine-University, Düsseldorf, Germany
- 9Brain and Mind Center, University of Sydney, Sydney, NSW, Australia
- 10Department of Neurology, Palacky University Olomouc, Olomouc, Czechia
- 11Department of Neurology, Medical University of Vienna, Vienna, Austria
- 12Comprehensive Center for Clinical Neurosciences & Mental Health, Medical University of Vienna, Vienna, Austria
- 13University MS Centre, Hasselt-Pelt, Belgium
- 14Rehabilitation and Multiple Sclerosis (MS), Noorderhart Hospitals, Pelt, Belgium
- 15Department of Neurology, Hospital Clínico Universitario San Carlos, IdISSC, Madrid, Spain
- 16Department of Medicine, Faculty of Medicine, Complutense University of Madrid, Madrid, Spain
Multiple Sclerosis (MS) management in individuals aged 55 and above presents unique challenges due to the complex interaction between aging, comorbidities, immunosenescence, and MS pathophysiology. This comprehensive review explores the evolving landscape of MS in older adults, including the increased incidence and prevalence of MS in this age group, the shift in disease phenotypes from relapsing-remitting to progressive forms, and the presence of multimorbidity and polypharmacy. We aim to provide an updated review of the available evidence of disease-modifying treatments (DMTs) in older patients, including the efficacy and safety of existing therapies, emerging treatments such as Bruton tyrosine kinase (BTKs) inhibitors and those targeting remyelination and neuroprotection, and the critical decisions surrounding the initiation, de-escalation, and discontinuation of DMTs. Non-pharmacologic approaches, including physical therapy, neuromodulation therapies, cognitive rehabilitation, and psychotherapy, are also examined for their role in holistic care. The importance of MS Care Units and advance care planning are explored as a cornerstone in providing patient-centric care, ensuring alignment with patient preferences in the disease trajectory. Finally, the review emphasizes the need for personalized management and continuous monitoring of MS patients, alongside advocating for inclusive study designs in clinical research to improve the management of this growing patient demographic.
1 Introduction
Multiple Sclerosis (MS) is a chronic autoimmune, neurodegenerative disease characterized by inflammation, demyelination and axonal degeneration in the central nervous system (CNS). The number of people affected by the disease is estimated to be 2.9 million worldwide (1–3). Historically, MS has mainly affected younger adults, with first symptoms often presenting between the ages of 20 and 40. However, a growing body of literature underscores an evolving epidemiology with an increasing incidence and prevalence in older individuals (4–6). This changing demographic landscape not only offers an opportunity to understand the disease intricacies within an older population but also mandates an in-depth examination of existing and potential management strategies. In this article, we provide a succinct overview of the epidemiology, symptomatology, and pathophysiological events, and a deeper review of the use of disease-modifying treatment (DMTs) in older MS patients, including data from randomized clinical trials (RCT) and real-world evidence (RWE), and pharmacologic and non-pharmacologic symptomatic treatment, including reference to the important role of MS care units (MSCU). The article concludes by discussing the strategies for planning care in the advanced age and offers some practical insights for neurologists.
A key consideration is the distinction between chronological age (the number of years a person has lived) and biological age (aging-driven biological changes, such as molecular and cellular degradation). In this article, our focus will be on chronological age, as this is the metric employed in the vast majority of clinical studies evaluating management of MS patients.
1.1 Epidemiology: incidence and prevalence of MS in older adults
MS can no longer be regarded as a condition of early adulthood. Several studies have documented an increase in the prevalence of MS among older adults in the recent years (3–5, 7–9). For instance, a study in Canada showed that the peak prevalence moved from 50-54 years in the eighties to 55-59 years in the 2000s (5). Similarly, in the US, the highest age-specific prevalence of MS in 2010 was observed in the 55-64 age group, followed by the 65-74 range (7). Similar results were found in Denmark, where the prevalence peaked at ages 55-59 years for women and 60-64 years for men in 2013 (9). This rise in the prevalence of MS in older adults might be linked to the fact that life expectancy of people living with MS has progressively increased in the last years (10, 11), even if it is still 6-10 years less than in the general population (10, 12, 13). Factors contributing to the extended life expectancy of patients with MS might include early initiation of therapy (14), the introduction of high-efficacy DMTs (heDMTs) and their earlier use (15, 16), advancements in symptomatic treatments (17), the establishment of multidisciplinary care models (18), and lifestyle interventions (19, 20).
Moreover, there is evidence that the age of onset of MS has also shifted forward over the last five decades (21, 22). When the onset of MS occurs at the age of 50 years or older, it is referred to as late-onset MS (LOMS), and when it occurs after 60 it is known as very-late-onset MS (VLOMS). A rise in the incidence of LOMS has also been observed in European countries (3, 22). A study in Italy showed that the percentage of individuals diagnosed with LOMS rose from 1% prior to 1991 to nearly 10% after 2010 (22). The rise in incidence of LOMS might be due to more awareness with this population and improvement of diagnostic tools. However, it does not explain why the increase in incidence in older individuals is seen particularly in women. Moreover, in LOMS and VLOMS, the time to diagnosis is almost double that of adult early-onset MS (AEOMS), indicating notably longer delays in diagnosis for older patients (23).
The rise of prevalence of MS in older patients poses a great burden not only to those affected but also to society as a whole. This concern is particularly pertinent given that the highest levels of life-years lost due to premature death or disability occur predominantly in the sixth decade of life (24). Figure 1 illustrated the age at onset, age at diagnosis, and current age of patients included in the MSBase registry, a renowned international online registry that collects longitudinal, real-world data from people with MS mainly in MS centers. The registry has recorded data of 96,352 patients so far (25), providing updated complementary information of the age distribution of MS.
1.2 Symptomatology and quality of life
The symptoms already present at a young age —spasticity, fatigue, pain, bladder, bowel and sexual dysfunction, dizziness, vertigo, balance issues, sleep and cognitive impairments, and emotional dysregulation — (17, 26–28) persist and often worsen as patients with MS age. In older patients some of these symptoms may be underrecognized or overlooked owing to the presence of comorbidities (28, 29). While some symptoms of MS, such as impairments in walking and bladder function continuously worsen throughout the age spectrum (30, 31), anxiety or depression might remain stable or even decrease with age (30–36). MS symptoms and age symptoms can overlap, complicating the diagnosis and management of MS in the elderly. For example, micturition alterations, is a typical symptom of MS but can also arise from prostate disease, a common condition in older men.
In the case of LOMS, the predominant symptoms encompass motor dysfunctions, sensory disturbances, visual impairments, and cerebellar symptoms (23, 37–39). These patients frequently exhibit a primary progressive phenotype, and, when they present a relapsing-remitting MS (RRMS) phenotype, an earlier conversion to secondary progressive MS (SPMS) compared to those diagnosed at a younger age is observed (37, 38, 40, 41). Onset at age 50 indeed tripled risks of developing SPMS compared to onset at age 20 (40). For equivalent disease duration, LOMS has been associated with higher Expanded Disability Status Scale (EDSS) (41). A study including 3,352 patients with age at onset <50 years and 245 patients with age at onset ≥50 years showed that, once EDSS 4 is achieved, age at disease onset was the only predictor of progression of irreversible disability (42).
The age-related decline in physical health in patients with MS has been estimated to be accelerated by 15–30 years compared to healthy old individuals (43). Pre-existing disability and older age have been identified as the principal risk factors for further disability accumulation (44). The gradual loss of mobility and independence inevitably affect the health-related quality of life (HRQoL) of patients and their families (45–47). Disability, fatigue, pain, spasms, stiffness, depression, and cognitive impairment have been pinpointed as risk factors for poor HRQoL in MS (46, 48, 49), while higher heightened self-esteem, self-efficacy, resilience and social support have demonstrated to be protective (46). In older adults with MS, in particular, physical HRQoL was positively associated with the patient being employed and widowed (likely because they now assume responsibilities previously shared with their spouse), whereas mental HRQoL was negatively associated with a lower level of education (50).
1.3 Underlying pathophysiology
MS manifests differently throughout the lifespan, with inflammation and neurodegeneration being two pivotal processes that shape the course of the disease. In older adults, the patterns of inflammation and neurodegeneration often intertwine differently than in younger populations. The initial phase of MS is dominated by waves of inflammation driven by peripheral immune mechanisms, with relapses and magnetic resonance imaging (MRI) lesions and pathologically areas of demyelination and variable axonal loss which can be already observed in radiologically isolated syndrome (RIS) (51) However, with the increase of disease duration and age, relapse frequency and new inflammatory MRI lesions decline (52–55) and the disease often transitions from the relapsing-remitting phase to the progressive phase. This progressive phase is marked by a compartmentalized inflammation due to a combination of variable neurodegenerative mechanisms with a key role of smoldering plaques, characterized by microglial activation and slow expansion of pre-existing plaques (44, 54, 56–58). In a study that examined changes in immune cell composition and activation levels in the CSF related to age in patients with RRMS and primary progressive (PPMS) an age-dependent decrease in counts of B and T cells, plasma cells and natural killer cells was noted in patients with PPMS, but not in patients with RRMS. These findings suggested an age-related reduction in immune cell penetration into the CSF of PPMS patients (59).
The natural aging of the immune system, known as “immunosenescence”, could be responsible for the shift in MS from an inflammatory to a neurodegenerative nature (60). As we age, the innate and adaptive immune systems undergo numerous changes, including decreased pool of naïve T cells, decreased diversity in T-cell and B-cell receptors, age-related changes in B cell development and function, and accumulation of memory T cells (61, 62). This declining immune functionality, together with other biological processes associated with aging – telomer shortening, DNA mutations, mitochondrial dysfunction, stem cell exhaustion, cellular senescence, or compromised repair capacity of the CNS, among others (63–65) – may contribute to generate the state of chronic low-grade inflammation known as “inflamm-aging”. Inflammaging, coupled with failure of compensatory mechanisms, such as neuroplasticity and remyelination (66), may significantly influence the course of MS and contribute to facilitate a steady neurodegenerative phase in older adults (62). Both inflammaging and immunosenescence can increase susceptibility to infections in older adults (62).
1.4 Multimorbidity and polypharmacy
In the aged population, the presence of comorbidities increases, which impacts the disease course, potentially complicating both symptomatology and treatment approaches in MS. Comorbidities, such as infections, cardiovascular and cerebrovascular diseases, diabetes, and psychiatric disorders have been found to be more prevalent in people with MS than the general population (67–69). Older age at diagnosis has been identified as the only factor significantly associated with comorbidities, whereas other factors such as higher baseline disability, higher diagnostic delay, higher relapse rate in the previous year, and lower education were not associated (70). The occurrence of these comorbidities can exacerbate the prognosis of MS and heighten the risk of mortality (71, 72). Comorbidities play a crucial role in deciphering new symptoms in older MS patients, as clinicians must determine whether a decrease in functionality is due to the progression of MS or to a comorbidity. Furthermore, modifiable risk factors such as obesity and smoking have been shown to influence susceptibility to MS (73–78) and disability worsening (73, 79–84).
As a result of multimorbidity, polypharmacy is common in MS (85). Antidepressants, antihypertensives, sedative hypnotics, antiepileptics, antiplatelets, and drugs for peptic ulcer or gastro-esophageal reflux disease are among the most common comedications in these patients (86–88). The odds of polypharmacy were three times higher in those ≥65 years-old than in patients below 50 (86). Among the negative outcomes of polypharmacy are increased fatigue, subjective cognitive impairment, and diminished HRQoL (85). The occurrence of drug-drug interactions has been associated with older age, together with lower educational level (89). These polypharmacy in older individuals is associated with poorer physical and cognitive functioning, even after adjusting for disease burden (90).
Importantly, as patients age, the benefits and risks associated with medications change considerably. Drug metabolism, excretion, and receptor sensitivity may shift as individuals grow older, potentially altering the therapeutic efficacy and side effect profiles of medications (91). Multimorbidity and polypharmacy increase the complexity of MS management and poses major challenges for clinicians and patients (92), despite still remaining underexplored domain in research.
In addition to the challenges posed by comorbidity and polypharmacy, the impact of menopause on women with MS deserves attention. Menopause, characterized by significant hormonal and immunological changes, modifies disease progression and clinical outcomes (93). Studies have observed reduced relapse rates after menopause, whereas the data are inconsistent regarding disability progression, with some studies reporting disability worsening (94) and others observing a similar rate of disability progression (95). Hormonal fluctuations and immunosenescence contribute to neuroinflammation and neurodegeneration, exacerbating MS-related disability post-menopause (96). The concurrent presentation of menopausal symptoms, such as fatigue, cognitive impairments, mood disturbances, and bladder dysfunction, often overlaps with MS symptoms, posing diagnostic and therapeutic challenges (93, 97).
2 Managing MS in older individuals
2.1 Disease modifying-treatments
2.1.1 Results from clinical trials and real-world evidence
2.1.1.1 Efficacy and effectiveness
The inclusion of older patients in RCT evaluating DMTs for MS has historically been limited, which is a recognized challenge in generalizing trial results to the older MS population. Pivotal trials of the DMTs approved by the European Medicine Agency (EMA) for relapsing MS (RMS) included only patients younger than 55 years (98–108), 50 years (109–112) or even 45 years (113) of age, while studies in SPMS usually include patients up to 60 years of age (114) (Table 1). Nevertheless, several subgroup analyses from phase 3 clinical trials have been conducted to understand the influence of age on the DMT efficacy, mainly comparing those aged 18-40 years to the 41-55 years group (104, 106–114, 123, 124). In these post-hoc analysis, significant reduction of clinical and MRI activity in patients >40 years were found with most of the DMTs, including peginterferon beta-1a (125), cladribine (124), dimethyl fumarate (DMF) (104), ocrelizumab (105), ofatumumab (126), natalizumab (123), alemtuzumab (127) and teriflunomide (≥38 years cut-off) (128), although in some studies the efficacy on disease activity in older group was lower than in the younger group (126, 129). With fingolimod treatment the efficacy on relapse rate in patients aged >40 years was present with the dose of 1.25 mg but not with the dose approved of 0.5 mg (100, 107). As far as disability progression is concerned, a statistically significant (108, 124) or numerical (129) lowering of the risk in patients >40 years was observed with cladribine, ofatumumab, and ocrelizumab (108, 124,129) but not with DMF, teriflunomide, natalizumab, and alemtuzumab (101, 104, 123, 128). The significant results cited here were obtained when comparing the DMT with placebo, except for ofatumumab which were obtained when compared with teriflunomide.
However, it is important to note that these subgroup analyses, in addition to involving patients under 55 years of age, were not powered to detect statistical significance of the differences between the age groups, and therefore these results should be taken with caution. Using a meta-analysis method allows to increase the power to detect differences of DMT efficacy by age. Signori et al. (130) conducted a meta-analysis of six of the above-mentioned RCT with subgroup analysis based on age and found higher reductions of disease activity and disability progression in patients ≤40 years (Table 2). This study also reported a significantly higher benefit from DMT on disease activity, but not on disability progression, in patients with baseline gadolinium-enhanced (Gd+) lesions and lower baseline EDSS.
Two subsequent metanalysis specifically evaluated the role of age in the efficacy of DMTs for MS in clinical trials (131, 132) (Table 2). The first of these meta-analysis, conducted by Weideman et al. (131), assessed whether the efficacy of immunomodulatory DMTs on MS disability progression depended on age. The results showed that DMT efficacy in preventing disability progression strongly decreased with advancing age. Indeed, the model predicted that DMTs had no efficacy on disability after the age of 53 years. Including the EDSS in the model did not change the association between age and DMT efficacy. They also assessed the impact of age in low- and heDMTs, separately, and found that heDMTs were superior to low-efficacy DMTs only in patients younger than 40.5 years. The second meta-analysis adopted the same analytic methods as Weideman et al. but assessed the role of age in the efficacy of DMTs in terms of disease activity (reduction of annualized relapse rate [ARR], new T2 lesions, and Gd+ lesions) instead of disability (132). Here, the authors found no significant association between mean age and the reduction of disease activity, neither clinical nor radiological measures. The authors concluded that despite DMTs being efficient in reducing disease activity regardless of age, patients included in clinical trials were selected based on the presence of baseline disease activity and, therefore, do not represent the real-world patients who have declines in disease activity as they age.
Limitations of these meta-analyses include the absence of access to individual patient data from the trials, with limited information about the age of the participants. Furthermore, there is a notable data gap for patients in their sixth decade and beyond. There is a pressing need for a new meta-analysis that overcomes these shortcomings, aims at assessing both the efficacy of DMTs on disease activity and progression of disability by age. But for that aim, more RCT assessing DMTs in patients older than 55 years need to be conducted.
Indeed, few trials assessing DMTs have included patients older than 55 (114, 133) (Table 3); and these trials did not assess the effect of the DMTs by age. The EXPAND trial demonstrated, in patients with SPMS aged 18-60, that siponimod reduced the risk of disability progression significantly more than placebo (114). In the EVOLVE-MS-1, patients with RRMS aged 18-65 had improvements in ARR when treated with diroximel fumarate compared to prior treatments, with no unexpected safety concerns (133). The ongoing trials, CONSONANCE (134) and LIBERTO (135), are evaluating the effectiveness and safety of ocrelizumab in patients aged 18-65 (with PPMS and SPMS in the CONSONANCE trial and with MS in the LIBERTO). Interim analysis of the CONSONANCE study have showed that, over a 2 year period, half of the patients treated with ocrelizumab had no evidence of progression and no disease activity; around one-third of patients had confirmed improvement in at least one disability measure (EDSS, T25FWT, or 9HPT), and most patients (72%) had stable or improved SDMT scores (136). The estimated study completion date is March 2025 for LIBERTO and December 2026 for CONSONANCE. While it remains uncertain if subgroup analyses in patients over 55 from these trials will be conducted, we strongly advocate for them.
In general, the selection criteria of RWE studies, are more inclusive, encompassing patients across a wider age range (see Figure 1). Studies to evaluate the effect of age on DMT efficacy have been conducted with RWE data. Vollmer et al. re-analyzed data from a retrospective study of MS patients to assess disease activity for oral (DMF and fingolimod) and higher efficacy DMT administered via infusion (natalizumab and rituximab) by age (137). They found that there was a statistically significant difference between oral and infusible DMTs up until the age of 54.2, when disease activity was evaluated according to DMT type (linear model). When disease activity was examined by age subgroup (<45 or >45 years old; binomial model), there was no significant difference in clinical relapses between oral and infusible DMT among those >45 years of age, but patients <45 treated with infusible DMT had significantly lower disease activity. In another RWE study that used linked administrative health data from Canada of over 19,000 patients, treatment with DMT compared with no DMT was associated with a 23% lower hazard of hospitalization in patients <55 year old but not in those over ≥55-years (138). Another Italian retrospective study included RRMS patients with ≥5 years follow-up and ≥3 EDSS stratified by age (≤18 years; 18–49; ≥50). The authors found that sustained exposure to DMT decreased the risk of disability progression; the effectiveness of DMT was lower in LOMS, although still detectable (139). Similar effectiveness has been observed for injectables and oral first-line DMTs in patients with LOMS (RRMS) in terms of first relapse, time to confirmed disability progression (CDP), and time to discontinuation (140).
RWE on the effectiveness of specific DMTs in older patients has also been published (141–145). One study conducted during COVID-19 pandemic aimed at understanding ofatumumab utilization patterns and patient characteristics reported that one-third of patients treated in the real-world were ≥55 years (141). However, the study neither provided the results by age, nor reported effectiveness or safety outcomes.
The effectiveness of interferon beta (IFNβ), a DMT commonly used in older MS patients, have also been assessed in the real world. One prospective 2-year study showed that patients older than 50 years treated with INFβ had decreases in the ARR and stable anxiety and depression (142). Other retrospective study reported no significant association between exposure to IFNβ and disability progression in this population (39).
A recent retrospective study, using data from the Italian MS Registry, examined the effectiveness of ocrelizumab in PPMS patients not meeting or meeting the ORATORIO eligibility criteria (aged 18-55 years, EDSS of 3.0-6.5, a disease duration <15 years if EDSS >5.0 or <10 years if EDSS ≤5.0) (143). Patients in the non-ORATORIO group were further stratified according to age (≤55, 56–64 and ≥65 years), EDSS (≤ 6.5 and >6.5), and disease duration (≤10–15 and >10–15 years). In patients not meeting the ORATORIO criteria, those aged over 65 years at ocrelizumab initiation demonstrated a significantly higher confirmed EDSS worsening of ≥1 point at 12 months and of ≥2 points at both 12 and 24 months, compared to patients between 56-64 years and ≤55 years old. Another retrospective study that included patients older than 55 with PPMS or SPMS at ocrelizumab initiation found that 60% of patients remained stable or improved after 2 years of ocrelizumab treatment (144).
The effectiveness of cladribine, measured as time to evidence of disease activity, in patients aged 50 years and older has been observed to be comparable to that in younger patients (under 50) over a median follow-up period of 12.4 months (145).
2.1.1.2 Safety
Alterations in the immune system due to biological aging heighten susceptibility to infections, neoplasms, and lymphopenia (146–148). These alterations, combined with the changes induced by DMTs on the immune system, have been hypothesized to produce synergistic effects (149). Older individuals are generally more susceptible to severe adverse events (AEs), such as the risk of progressive multifocal leukoencephalopathy (PML) (150). The increased risk in PML is because the prevalence of seropositivity to the John-Cunningham virus (JCV) – which causes PML – grows with age. This condition has been primarily linked to natalizumab (151, 152), but also to the use of other DMTs such as fingolimod (153), especially after previous treatment with natalizumab (154), and DMF (150). Older age has been observed to independently heighten the PML risk, with these patients being more prone to develop PML after fewer natalizumab infusions and exhibiting higher mortality rates (155).
Other infectious complications seem to also be related to aging (156). Older patients were more susceptible to cryptococcal meningitis associated to fingolimod (157), infections requiring hospitalization associated to ocrelizumab (158), and herpes zoster across several DMTs (149). Among MS inpatients with COVID-19, those receiving DMT —except for those treated with anti-CD20 monoclonal antibodies— had reduced odds of 30-day mortality and a lower risk of death (159). Nevertheless, older age, progressive MS, comorbidities and higher disability were associated with worse COVID-19 outcomes in patients on DMTs (159–161). Sustained treatment with anti-CD20 might not be a feasible choice in older MS patients due to a reported heightened risk of severe and opportunistic infections, malignancies, and a diminished vaccine response associated with these treatments (162).
A meta-analysis with meta-regression of 45 RCTs was conducted to investigate if there was an age-related rise in infections and neoplasms among MS patients receiving DMTs with different mechanisms of action regarding the effects on peripheral leukocytes: 1) immunomodulatory (DMF, glatiramer acetate, INFβ, and teriflunomide), 2) sequestrating (fingolimod, natalizumab, ozanimod, and siponimod), and 3) depletive (alemtuzumab, cladribine, and ocrelizumab) (163). The mean age in the clinical trials was 38 years. The investigators found that age alone did not influence the rate of any AEs, but interaction of age with depletive mechanism of action explained approximately 23% of the variance in neoplasm rate. In patients older than 45 years treated with depletive agents, the risk of neoplasms was found to be higher compared to other treatments. Conversely, there was no association between age and the rate of overall infections and herpetic infections.
A prospective real-world study conducted in Germany included patients treated with teriflunomide and analyzed the data based on age subgroups. Results on safety showed that the number of patients with AEs was lowest in patients aged 26–35 years but was not different in the rest of age groups (18–25, 36–45, 46–55, 56–65 and 66+ years). The rate of patients with infections was similar regardless age (≤45 and ≥45 years). However, the rate of discontinuations was significantly higher in patients aged >45 years (62.9%) than in the younger group (37.1%). Further research is needed to determine the safety of specific DMTs in older patients with MS, including those with LOMS (164).
In a real-world study in the United States, patients aged18 to 74 years who switched from high-efficacy infusion DMTs to oral cladribine did not experience any new AEs (165). When comparing older (≥50 years) and younger (<50) patients treated with cladribine, AEs and infections during treatment were numerically more frequent in older patients (145), especially among those who experienced Grade ≥3 lymphopenias (166); however, these differences were not statistically significant (145, 166).
2.1.2 Initiation, de-escalation and discontinuation
Initiating DMTs in individuals with MS older than 55 years has been a subject of debate among clinicians. The decision to start, continue, or switch DMTs in older individuals requires a deep understanding of the benefits and risks in this age group, where scientific evidence is limited, as we have discussed in the prior sections.
Information on prescribing patterns of DMTs among older adults with MS remains scarce. A study conducted in the US included 12,922 older adults with MS showed that around 19% of patients were receiving a DMT (10.5% injectables, 6% orals, and 2.5% injectables). Older adults aged 60-69 years had higher odds of receiving DMT than adults aged ≥70 years. Several factors increased the odds of being prescribed a DMT, such as the presence of symptoms or symptomatic medication (167). Many experts are advocating for the early initiation of heDMT in MS (168–173), based on the increasing evidence supporting the benefits of this strategy in controlling disease activity and progression (15, 174, 175). The strategy seizes the window of opportunity during which the underlying pathophysiology can be altered and influence long-term clinical outcomes (176). Whether heDMT should be use as early as possible in older patients or if moderate efficacy DMT are a better option for this population has not been specifically evaluated.
A Danish study comprised all patients (N=3497) with RRMS registered in The Danish Multiple Sclerosis Registry who began a DMT after 2014. After mutual adjustment for all selected covariates (DMT efficacy, pre-treatment relapse activity, disease duration, EDSS score, and MRI activity), patient age was a strong decisive factor for choosing a heDMT with odds ratio 1.69 for starting a heDMT in patients <40 years compared with patients ≥ 40 years (177).
Some authors have stated that in PPMS patients older than 55 years, with a long disease course (> 15 years) and a high degree of disability (EDSS score > 6.5) therapeutic nihilism (i.e. avoiding DMT use) should be avoided (171).
Two pragmatic clinical trials are currently being conducted to delve deeper into escalation and early heDMT: DELIVER-MS (NCT03535298) and TREAT-MS (NCT03500328). Despite the anticipated valuable insights that these two studies will provide concerning treatment strategies in MS, it is noteworthy that both studies limit their patient cohorts to a maximum age of 60 years. Consequently, while the data derived from these studies will shed light on treatment approaches for patients aged between 55 and 60 years, they leave patients ≥60 uncovered. Further research specifically addressing treatment strategies for MS patient older than 55 years is still needed.
Weideman et al, considering the results of their meta-analysis (after age 53 there was no predicted benefit to receiving DMTs) stated that “a prescribing clinician must consider the possibility that starting or continuing immunomodulatory DMT beyond age 53 will expose an average patient to treatment-associated risks with few, if any, potential benefits”, although they recognized that the model was based on average outcomes, and individual genetic and environmental factors were not considered (131).
The optimal duration of treatment with a DMT still remains under debate, and the available evidence regarding de-escalation or discontinuation is still limited, even if we are now encountering aging patients who have been treated with DMT for decades (178, 179). The DISCOMS was a phase 4 non-inferiority RCT aimed to assess whether it was safe to discontinue DMT in aged MS patients. The study included people with any MS subtype, ≥55 years old, with no relapse within the past 5 years or new MRI lesion in the past 3 years while continuously taking an approved DMT. Participants were randomly assigned (1:1) to either continue or discontinue DMT. Similar numbers of patients had AEs and serious AEs (SAEs), but a higher number of AEs and serious SAEs occurred in the discontinue group. The authors concluded that the null hypothesis could not be rejected, and they could not determine if discontinuing DMTs is equally safe compared to maintaining treatment in patients over 55 years without recent disease activity (180).
Studies from the real-world setting have explored the association between DMT discontinuation and both disability progression and disease activity. A registry-based study, with an average follow-up of 4.6 years, revealed that prior to DMT discontinuation, 72.5% of patients were classified as stable (<1.0 or <0.5 increase if EDSS<6.0 or ≥6.0, respectively). However, after discontinuation, 32.9% of these previously stable MS patients experienced disability progression. Two years after DMT discontinuation, the progression rate was comparable for patients younger (31.1%) and older (25.9%) than 55 years old (181). Another study evaluated disease activity in RRMS patients who discontinued first-line DMT. The study showed that higher age at DMT discontinuation (45-55 vs <45 years) was associated with a lower risk of MRI activity and relapses after discontinuation (182).
Other studies have explored disease activity and disability progression in older adults, but without comparing the results with a group of younger patients. For instance, one study conducted in France in RRMS patients over 50 years with a median follow-up of 7 years after DMT discontinuation showed that discontinuation was not associated with an increased risk of relapse or EDSS progression, but was associated with a higher risk of reaching an EDSS of 6 (183). Another study assessed inflammatory activity after the discontinuation of second and first-line DMT in patients over 45 years. Within a year of discontinuation, they discovered that the likelihood of experiencing a relapse was considerably higher for natalizumab than for first-line DMT or fingolimod (184). Similar findings were obtained in a prior study where discontinuation of natalizumab, but not first-line DMTs, was associated with rebound (185). In patients with SPMS, the ARR remained stable after DMT discontinuation (186). These data suggest that disability progression after DMT discontinuation is not significantly influenced by age, but disease activity might be, depending on the DMT. Thus, when prevention of both relapses and disability worsening is the goal, age is a complex variable to consider.
Fewer studies addressed the de-escalation phase, the transitionary from heDMT to lower efficacy DMT. A recent retrospective study evaluated patients who switched from a heDMT — including any monoclonal antibodies or oral DMTs, except teriflunomide — to a low-efficacy DMT. Using a noninferiority analysis, the study found that de-escalating from a heDMT to a low-efficacy DMT did not worsen ARR or disability, although these findings were not significant; the only significant observation was that the T2 lesion burden did not worsen when switching (187).
There is a lack of evidence-based guidelines for optimal initiation, de-escalation or discontinuation of DMT in MS patients older than 55 years (188, 189). In the absence of evidence-based guidelines and consensus, it is essential to note that the decision to initiate DMTs in older MS patients should be individualized. Some older patients might still have active inflammation and might benefit from treatment. Also, newer DMTs with different mechanisms of action might offer advantages in terms of efficacy and safety profiles for older individuals. Therefore, while age is an essential factor in the decision-making process, the overall clinical picture, including disease activity, progression rate, comorbid conditions, and patient preferences, should guide the choice of initiating, de-escalating, or discontinuing treatment.
Overall, given the safety concerns associated with DMTs in older patients, coupled with the heightened prevalence of comorbidities in this demographic, the primary factor in deciding to use a DMT in older patients should be safety rather than efficacy.
2.1.3 New treatments: BTKs
Despite current DMTs effectively reduce relapses and associated disability worsening, mainly linked to immune cells infiltrating the CNS, they are less effective in slowing overall disability accumulation, possibly due to not targeting inflammation inside the CNS, a key driver of disability. BTK (Bruton tyrosine kinase) is an intracellular signaling molecule involved in the regulation of maturation, survival, migration and activation of B cells and microglia (190). Although BTK inhibitors were originally developed for B-cell malignancies, such as certain types of lymphomas and leukemias, they have been explored as potential treatments for MS due to their ability to modulate B-cell activity and other immune responses. The second-generation BTK inhibitors being explored as potential treatments for MS have improved their safety profile resulting from reduced off-target inhibition of molecules such as Janus kinase 3, epidermal growth factor receptor, and possibly other TEC tyrosine kinases family members (191).
Phase II safety and efficacy data for evobrutinib and tolebrutinib have been published (192, 193), but only the evobrutinib trial included patients up to 65 years old. In the overall population, the common AEs were nasopharyngitis and elevated liver enzymes (192). By week 52, higher doses of evobrutinib had greater AEs rates. An open-label extension following the initial study indicated that most participants continued with the treatment, and the initial data did not raise new safety concerns. No results by age subgroup are available so far. Unfortunately, in the phase III trial comparing evobrutinib and teriflunomide, evobrutinib did not meet their primary endpoints of reducing ARR in people with RMS compared to oral teriflunomide (194). Only some of the ongoing phase II trials of tolebrutinib and fenebrutinib include older patients (up to 60 years [NCT04411641], 65 years [NCT04544449] or 120 years [NCT04742400]).
2.1.4 Treatments focused on remyelination and neuroprotection
The loss of myelin sheath, that characterizes MS, disrupts not only the efficient transmission of nerve impulses but also the preservation of the integrity of the axon (195). Following demyelination, the unprotected axons are vulnerable to energy deficits, potentially leading to neurodegeneration that might be irreversible. Consequently, the regeneration of new myelin sheaths through remyelination after a demyelinating event is crucial to maintain axonal stability and prevent the major pathological change underlying the progressive phase of the disease.
Oligodendrocytes (OL) newly generated from oligodendrocyte precursor cells (OPCs) were proposed to be responsible for remyelination in the adult human CNS (196, 197). Upon receiving signals from a lesion, these OPCs differentiate into OL, which then begin to wrap around the denuded axons, forming new myelin sheaths. Also, recent studies showed that mature OL can regenerate new myelin sheaths after demyelination (198). In individuals with MS, remyelination is often incomplete or insufficient, particularly at older age (199), leading to persistent functional deficits and leaving axons vulnerable to degeneration. The incomplete remyelination in MS can be attributed to various factors (200). With age and disease progression, the reservoir of OPCs can diminish, and their differentiation into myelin-producing OL can be hindered (201, 202). Furthermore, the MS lesion environment, replete with inflammatory molecules and scar tissue, may be hostile to the remyelination process (200).
Strategies for remyelination are diverse and encompass the enhancement of oligodendrocyte progenitor cell mobilization (via semaphorins) and differentiation (through pathways such as Notch, LINGO-1, and Wnt; or agents like vitamin D, thyroid hormone, or activation of the retinoid X receptor). Other tactics involve the stimulation of neuronal activity or the modulation of microglial targets (203). Table 4 presents late-stage clinical trials evaluating agents targeting remyelination that included MS individuals older than 55 years.
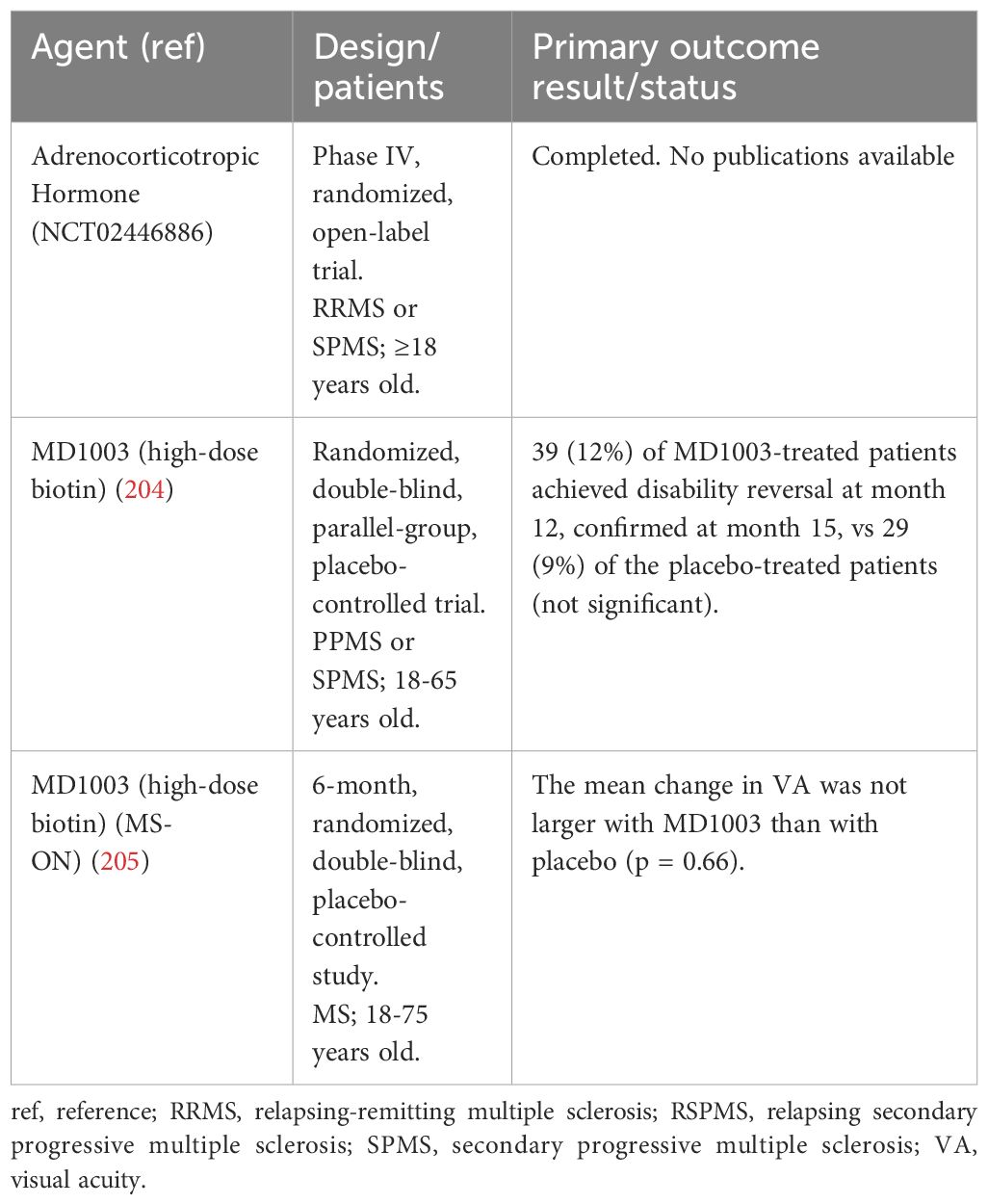
Table 4 Phase III and IV clinical trials of remyelination treatments including patients older than 55 years.
Some agents such as biotin showed promising results in terms of disability reversal in small trials (206), but failed to replicate its efficacy in trials with a higher number of patients and more ambitious endpoints (204). Phase I and II trials have been performed in MS patients older than 55 years evaluating agents such as liothyronine (207), olesoxime (208), clemastine fumarate (209), quetiapine (210), opicinumab (211, 212), rHIgM22 (213), domperidone (214), amiloride, fluoxetine, riluzole (215), and elezanumab (216). Several of these studies did not meet the primary endpoint (208, 210–212, 215) and further phase III trials were not encouraged. In those trials where the study endpoints were met (207, 209, 213), phase 3 trials will need to confirm the efficacy and safety of these agents. The MACSiMiSE-BRAIN is an ongoing phase II trial conducted to assess the neuroprotective and remyelinating effects of metformin in MS patients of 18-70 years. The study is estimated to be completed in 2026 (217). Up to date, new remyelinating and neuroprotective agents have not yet successfully showed results in older MS patients.
2.2 Symptomatic treatments
Symptomatic treatments focus on alleviating symptoms that patients experience throughout the course of their illness. Given the heterogeneity of MS symptomatology, a tailored, patient-centered approach is essential in symptomatic management. Available symptomatic treatment encompasses pharmacological and non-pharmacological interventions, such as physical and occupational therapy, psychotherapy, and lifestyle modifications. Here we present a review of the evidence of the effectiveness of these treatments in studies that included older patients.
2.2.1 Pharmacologic treatment
The pharmacologic treatment approach for each symptom can vary based on the prevalence of symptoms and range of available therapeutic options. Typically, pharmacological therapy are combined with non-pharmacological strategies, as a combined approach often yields better outcomes (218). A comprehensive study from Germany, encompassing both pharmacological and non-pharmacological treatments, assessed 35,755 MS patients. This study showed that most of the patients (up to 80%) with depression, spasticity, pain, and epilepsy were treated. Contrastingly, only a minority (21-36%) received treatment for fatigue and cognitive dysfunctions, a gap attributed by the authors to the scarcity of treatment options (17). Additionally, a Canadian survey reported even higher treatment rates for depression, at 85.7%, covering also both pharmacological and non-pharmacological interventions (219).
Several pharmacological agents are used to treat the symptoms of MS, but for most of these treatments, the evidence supporting their efficacy in patients with MS is weak. Several meta-analyses have been conducted, examining both RCT and uncontrolled studies, to assess the effect of these treatments in MS symptoms. To date, while no meta-analysis has explicitly focused on the impact of these treatments in older patients, each one has incorporated studies with patients aged 55 or over. Table 5 presents pharmacologic treatments that, based in meta-analysis findings, have shown consistent efficacy in alleviating various symptoms. These include fampridine, cannabinoids, botulinum toxin, nabiximols, desmopressin, and amantadine (220–233).
Other symptomatic treatment approaches have reported positive results in MS patients, although they have not been confirmed by meta-analysis. For spasticity, other treatment options include baclofen, tizanidine, and gabapentin as first line (234–236) and dantrolene or diazepam as second-line (236). Consensus documents on therapeutic management of spasticity in patients with MS are available (237).
Pain management has involved the use of tricyclic antidepressants, serotonin noradrenaline reuptake inhibitor (SNRI), carbamazepine, gabapentin, pregabalin, and lamotrigine (235, 238, 239). Duloxetine has been recognized for its potential to treat both depression and fatigue, while fluoxetine and bupropion have been noted for their effects on depression, with the latter also addressing sexual dysfunction (238, 240–242). In treating tremor, agents such as propranolol, primidone, anticholinergics, gabapentin, topimarato and clonazepam are used (243). Bladder dysfunctions has also been addressed using mirabegron, antimuscarinic agents (solifenacin, tolterodine, trospium) and botulinum toxin (244–249).
Also, it is important to note that not all studies of pharmacological symptomatic treatments have demonstrated efficacy in patients with MS. Some studies have reported a lack of positive effects or inconsistent results of some pharmacologic treatments for ataxia or tremor (233, 250). Also, the efficacy of oxybutynin for bladder dysfunction (251) and amantadine, pemoline, modafinil, 4-aminopyridine, and prokarin for fatigue (252, 253) remains contentious. Desipramine and paroxetine showed a trend towards efficacy in treating depression in MS, but the trials assessing these antidepressants had a high number of patients lost to follow-up (254). Also, vortioxetine was effective in reducing the symptoms of depression and anxiety, but it did not improved cognition or fatigue in a recent case series (255). Results from a meta-analysis showed that acetylcholinesterase inhibitors (AChEIs; donepezil and rivastigmine) and stimulants (methylphenidate, modafinil, l-amphetamine sulfate and lisdexamfetamine dimesylate) offered no significant benefits over placebo in cognition – specifically in processing speed, verbal fluency, working memory, verbal and visuospatial memory or executive function – (256). Also, there is evidence that drugs with sedative or psychotropic properties, such as benzodiazepines, cannabinoids and anticholinergics, can potentially compromise cognitive or physical functioning (257–259).
Few studies have investigated the effect of age on the safety of symptomatic treatments in MS. In a large study, it was observed that MS patients aged 50-64 had twice the likelihood, and those 65 and older had over three times the likelihood, of being on multiple medications compared to younger patients (86), which is known to increase the risk of AEs. Post hoc analyses have revealed that older age does not affect response or AEs associated with duloxetine; but combining duloxetine with pregabalin or gabapentin with imipramine, nortriptyline or venlafaxine might offer added benefits over monotherapy, they also pose a heightened risk of AEs (260). Despite studies having included adults of a wide age range, research assessing the effect of these treatments by age is still missing. There is a need for studies that evaluate the safety and efficacy of medications targeting symptoms, particularly when used in combination, in older MS patients.
2.2.1.1 Treating symptoms clusters
Most MS treatments focus on individual symptoms, often leading to polytherapy, which heightens the risk of AEs, drug interactions, and can intensify other MS symptoms. Simplifying MS symptom management would be beneficial for patients. An approach to do this is by pinpointing symptoms with a shared pathological mechanism or those treatable with a single therapy. This reasoning led to the Spasticity-Plus syndrome concept, which groups MS spasticity-related symptoms with similar pathophysiology or treatment response (48). Two major clusters have been identified: spasticity-spasms/cramps-pain, and ataxia-instability-vertigo. Depression, cognitive impairment, and fatigue were clustered by common pathophysiology. A common underlying pathophysiology between spasticity and associated symptoms could explain the fact that pharmacological intervention targeting the cannabinoid system, such as nabiximols, has effects on several spasticity-associated symptoms (48).
2.2.1.2 Vaccination
Given that some DMTs may lead to increased rates of infection-related adverse events and considering that older adults inherently have a higher risk of infections, routine assessment of vaccination status in older patients with MS is very important to allow updating of vaccines as needed. Vaccination against common and potentially severe infections such as hepatitis, diphtheria, tetanus (often combined in the DT vaccine), influenza, COVID-19, and pneumococcal disease is recommended (261).
2.2.2 Non-pharmacologic approaches
In the older population, where comorbidities and polypharmacy are more prevalent, non-pharmacologic interventions offer unique benefits (262–265), often complementing drug treatments. Symptoms such as spasticity, impaired gait, cognitive decline, fatigue, and sexual dysfunction can be intensified by age-related changes in the musculoskeletal, nervous, and sensory systems, leading to a more pronounced decline in overall function and mobility.
2.2.2.1 Physical therapy and exercise
Both physical therapy (PT) and physical exercise play crucial roles in the management of MS patients (48, 265, 266). PT offers tailored interventions that are delivered by trained professionals, and usually targets a specific symptom. Several meta-analyses (Table 6) have concluded that PT interventions are effective for improving gait and balance and addressing spasticity, sexual dysfunction, and bladder issues (268–272, 276, 277). Also, the benefits of PT for improving gait, balance, fatigue and QoL appear to be enhanced when incorporating robot-assisted training or virtual reality tools (267, 268, 275, 278, 289, 290). Moreover, physiotherapy may enhance the benefits of symptomatic drugs, such as botulinum toxin for spasticity (291).
On the other hand, exercise training, including endurance and resistance training, can be part of patients’ daily or weekly routine. Exercise training has been also proved by meta-analyses to reduce fatigue and symptoms of depression (281, 282, 284, 292, 293) and to improve balance and HRQoL (294, 295). Although the studies included in the meta-analyses assessing the effect of PT and exercise did not specifically target patients older than 55 years, most of the studies did not impose an age limit in their selection criteria, leading to a broad age range among participants (Table 6).
Individuals with MS, including older adults, exhibit reduced physical activity levels compared to the general population, underscoring the imperative for targeted physical activity promotion within these patients (296–299). Interventions to increase physical activity behavior among people with MS are efficacious for increasing and sustaining physical activity behavior (300). Resistance training, both in isolation and combined with aerobic exercise, has proven most effective for enhancing muscular fitness, while endurance exercises improve cardiorespiratory fitness (301).. In older adults with MS, targeting moderate-to-vigorous physical activity as an approach for improving cardiorespiratory fitness has been suggested (302).
Importantly, physical exercise has been shown to increase neuroplasticity (303), even in the aging brain (304). A comprehensive meta-analysis revealed that while low and high-intensity exercise improved neuroplasticity, the relationship between exercise intensity and neuroplasticity seems to be present in younger adults but not in older adults or patients with MS, Parkinson’s disease (PD) or stroke (305). Thus, exercise intensity is a crucial factor to adjust the exercise regimen for healthy young individuals, but it may not be as critical for older adults with MS (305). The meta-analysis also concluded that exercise-induced improvements in neuroplasticity were associated with motor changes but not cognitive changes (305).
It is important to note, however, the inconsistent reporting of age in the meta-analyses conducted to assess the effect of physical therapy and physical exercise in MS symptomatology (see Table 6). In some of these meta-analyses, data on age was not reported (267, 268, 270, 273, 274, 277, 284). The lack of standardization in reporting the age of the patients included in the studies poses a challenge to interpreting and comparing findings across studies. Standardizing the reporting of age data would facilitate drawing conclusions from these meta-analyses.
2.2.2.2 Neuromodulation therapies
Neurostimulation, which involves using cutting-edge technologies for stimulation or inhibition of the neural function, has received limited attention in MS, compared to other neurological diseases. Some studies in a wide age range of the MS population have been conducted.
A research study encompassing participants aged between 27-75 demonstrated the long-term efficacy of the intrathecal baclofen pump (ITB) in diminishing symptoms of spasticity, spasms, depression, and pain, while also enhancing overall function. Furthermore, the safety of ITB remained consistent, unaffected by the age (257). Functional electrical stimulation (FES) has shown an initial and persistent benefit on gait speed specifically with short walking evaluations (306). Deep brain stimulation (DBS) has been observed to alleviate MS-related tremor (307, 308). Though DBS has not specifically been studied in older MS patients, results from PD research revealed that DBS improves motor function and reduces medication reliance in PD patients, including those over 70 (309). Transcranial direct current stimulation (tDCS) seems to improve processing speed, mood, pain, and fatigue (310). Also, spinal cord stimulation (SCS) seems beneficial for MS-associated pain and bladder issues but its efficacy for spasticity is mixed (311, 312). Bladder disorders seems to benefit from peripheral tibial nerve stimulation (277). Additionally, repetitive transcranial magnetic stimulation (rTMS) shows preliminary evidence of efficacy in improving spasticity in MS patients (313), though more studies are required to assess its impact on fatigue (314). These insights underscore the potential of diverse neuromodulatory approaches in addressing a range of MS symptoms.
2.2.2.3 Psychotherapy, cognitive rehabilitation and mindfulness
Psychological interventions have demonstrated efficacy in enhancing the mental health, sexual dysfunction and HRQoL of patients with MS (46, 315–318). The efficacy of psychotherapy in MS extends beyond in-person sessions, including sessions conducted via telephone or online platforms (319, 320). Cognitive-behavioral therapy (CBT) is especially prominent due to its targeted approach in helping patients manage their pain and stress and in reducing symptoms of fatigue and depression (315, 321–327), although some researchers have reported CBT not to be efficacious when considered alone (328).
Patients with MS also benefit from cognitive rehabilitation programs (329, 330). Memory, attention, and executive function deficits have showed some improvements with cognitive rehabilitation (330–332). However, the evidence is still limited (330) and most RCT did not properly report information regarding the content or procedures of the intervention (333). There remains a critical need for large-scale, high-quality RCTs that utilize ecologically valid outcome measures and extend assessments to longer-term time points to ensure comprehensive reporting (332).
Furthermore, mindfulness-based interventions (MBI) have gained recognition for their effectiveness in mitigating stress, depression, anxiety and fatigue while enhancing HQRoL and sleep quality among adults with MS (334–344). MBI are not only cost-effective (337) but they are also feasible techniques to adopt by older adults. Once the technique is learnt, it enables individuals to independently engage in the practice. However, the most optimal MBI method and the ideal frequency for achieving sustained long-term benefits continues to be unclear.
2.2.2.4 Other interventions
Occupational therapy has also proved to be effective for improving the functionality of MS patients in tasks such as dressing, bathing, and ambulation (345). Fatigue management programs delivered by occupational therapists are also effective in improving MS symptoms (346), including fatigue, manual dexterity, falls prevention, cognition, depression and QoL (347). Future studies will determine whether the outcomes of occupational therapy vary in relation to the method of delivery (one-on-one versus group) and the setting of the therapy (outpatient, inpatient, or therapy administered at home) (348).
Speech and swallowing therapy is particularly important when MS has affected vocal or swallowing muscles (349, 350), which can reduce HRQoL specially in the last stages of MS (349). Electrical stimulation and botulinum toxin treatment have showed positive effects on dysphagia (351). A survey of 5,289 patients with MS in Sweden showed that 80% of participants experienced at least one speech or communication issue, including symptoms like speech fatigue or unclear articulation, while 25% indicated they faced challenges with swallowing. However, few of these patients received speech and language pathology services (352).
Most MS patients present symptoms when their body temperature is increased. Active and passive cooling methods have been used as a complimentary therapeutic approach to address symptoms sensitive to temperature (353), with no specific cooling garment identified as superior (354). The benefits of cooling therapies have been reported in physical activity and function, fatigue, and the QoL in individuals with MS (354, 355).
Limited research exists evaluating the impact of orthoses on balance in patients with MS, with studies focusing on ankle-foot and foot orthoses yielding inconclusive outcomes. One recent meta-analysis indicates that orthotic interventions appear not to enhance balance in people with MS (251).
Lifestyle modifications, including diet, may help improve MS symptoms and HRQoL (356). This can include vitamin D supplementation (357–359), probiotics (360), or Mediterranean diet (361). The evidence for the benefits of ketogenic diet and fasting in MS patients is still inconclusive (362), and ongoing studies will provide further evidence on their effects not only in MS symptomatology but also on disease progression (363).
Strong social support networks, including family, friends, support groups, or community organizations, can significantly impact the HRQoL and functioning of people with MS. MS symptoms, especially fatigue, hinder patients’ ability to form relationships and engage in social or daily activities (364). Online peer support serves as an alternative for those who cannot reach in-person groups, mitigating social isolation, yet the symptoms of MS might complicate the use of technological devices (365).
2.3 MS care units
As we have extensively reviewed in the prior sections, the landscape of MS treatment encompasses an array of DMTs, most of them with lack of evidence in older adults, coupled with an extensive selection of both pharmacologic and non-pharmacologic symptomatic therapies. This complexity has underscored the necessity for specialized MSCU, as the management of MS patients extend beyond the expertise of family physicians and general neurologists (18).
Central to the function of these MSCU is a multidisciplinary team, which, in addition to MS-specialized neurologists and nurses, would include neuropsychologists, clinical psychologists, physiotherapists, occupational therapists, and administrative support staff (18). In older adults with MS, a collaboration with an internal medicine specialist or geriatrician would ensure a more comprehensive approach to patient care by integrating specialized knowledge on aging-related health issues. Additionally, strong collaboration with social workers might also be key for supporting patients’ functional capacity and connection with the job market, especially as retirement ages rise across Europe. Collaboration with other specialists such as a speech therapist, dietitian, and specialists in pain, spasticity and continence is also recommended (18). Some of these services may, however, be provided by a MS neurologist or MS nurse in the MS Care Unit with special education and interest.
Moreover, the MSCU should ensure the access in a reasonable time at all the investigational procedures for diagnostic and monitoring purposes, including MRI, optical coherence tomography, evoked potentials, CSF and hematological tests. Finally, the services of neurorehabilitation are central to the management of a person with MS. This is particularly true for aged individuals in order to reinforce the mechanisms of plasticity, which efficiency decreases with age.
When the fulfillment of MSCU international criteria has been evaluated in European countries such as Hungary, half of MSCU provided sufficient care for MS patients (366). Local aspects should be considered for the implementation of MSCU worldwide. Some recommendations on the objectives, human and technical resources, and the general functioning of MSCU for specific regions have been made (367).
3 Advance care planning
Fear of the future has been found to be a prevailing worry among patients with MS. Some specific fears were potential further losses of mobility and independence, the prospect of becoming reliant on caregivers, and the possibility of transitioning to a nursing home (368). These insights highlight some challenges that should be addressed, such as implementing interventions that empower older adults with MS to feel more in control of their future, multidisciplinary collaboration to help families impacted by MS, and promoting community support for individuals with MS.
Advance care planning allows patients to plan their future medical care by identifying values and treatment preferences, discussing these with family and healthcare professionals, and appointing a representative to make decisions if they cannot. Discussions with neurologists about treatment restrictions have been observed to start late, usually when significant physical and cognitive decline has occurred (369).
Patients frequently imply desire to engage in conversations about death and dying (370), and it is important for healthcare professionals to recognize and foster these discussions. The European Academy of Neurology (EAN) on palliative care of people with severe progressive MS states that patients should be encouraged to discuss their wishes about future care, including the restriction of interventions and the consideration of accelerated death (371).
4 Final considerations
4.1 MS management: personalization and monitoring
The pathophysiology and immunology of MS in elderly patients, characterized by the complex interplay of the immunosenescence and inflammaging processes with neurodegeneration, highlight the need for a tailored approach to the management of MS in older adults. Taking into account the unique aspects of the disease in this population, the choice of DMT must be approached on a case-by-case basis, particularly in older patients, weighing potential risks against therapeutic benefits. Interactions with other treatments for existing comorbidities, treatment monitoring related to special conditions, the need for pharmacologic and non-pharmacologic symptomatic therapy, and the possibility of DMT discontinuation should be considered and discussed with the patient. This personalized approach underscores the importance of continuous monitoring and adjustment of the management plan to address the needs of older individuals with MS. The evaluation of biological age, in addition to chronological age, may help adapt treatment to each patient in the future. Such a complex approach requires a high level of organization that can be reached with appropriate models of assistance as the MSCU model.
4.2 Study design: inclusion of a wider range of age
We advocate for the inclusion of a broader age range in the design of clinical trials that evaluate the efficacy and safety of DMTs in MS. There are several reasons for this suggestion. Firstly, the demographic shift in MS prevalence towards older age groups requires a better understanding of DMT efficacy and safety in these populations. As the pathophysiology and immunology of MS evolve with age, older patients may respond differently to treatments compared to younger patients. Secondly, older MS patients often present with several comorbidities and a different pharmacokinetic profile, which can influence the efficacy and safety of DMTs. Without adequate representation of older adults in clinical trials, there is a risk of underestimating potential AEs or overestimating the efficacy of DMTs in this group. Thirdly, including a wider age range enhances the generalizability of trial results, making findings more applicable to the broader MS population. We also encourage age-specific trials and real-world studies to better understand the efficacy and safety of DMTs in the older MS population.
Author contributions
ÓF: Conceptualization, Writing – review & editing. PS: Writing – review & editing. GC: Writing – review & editing. PV: Writing – review & editing. H-PH: Writing – review & editing. LL: Writing – review & editing. TB: Writing – review & editing. BVW: Writing – review & editing. CO-G: Conceptualization, Writing – review & editing.
Funding
The author(s) declare financial support was received for the research, authorship, and/or publication of this article. Medical writing was equally funded by the European Charcot Foundation and the ParadigMS Foundation.
Acknowledgments
The authors thank Laura Prieto del Val, PhD of Evidenze Clinical Research for providing medical writing support (including development of first draft, performing initial literature search, incorporation of authors comments, and styling the manuscript per journal requirements), which was equally funded by the European Charcot Foundation and the ParadigMS Foundation in accordance with Good Publication Practice (GPP3) guidelines.
Conflict of interest
Author OF received speaking and/or consulting fees from Allergan, Almirall, Bayer Schering, Biogen, Merck Serono, Novartis, Sanofi, and Teva; compensation for serving as a journal editor, associate editor, or member of an editorial advisory board Revista Española de Esclerosis Múltiple; and research support from Hospital Foundation FIMABIS. Author GC received consulting fees from Actelion, Bayer Schering, Merck Serono, Novartis, Sanofi, and Teva and lecture fees from Bayer Schering, Biogen Dompé, Merck Serono, Novartis, Sanofi, Serono, Symposia International Foundation, and Teva. Author PV has received honoraria and contributions for attending meetings from Biogen, Sanofi-Genzyme, Novartis, Teva, Merck, Roche, Imcyse, AB Science, Janssen, and BMS-Celgene. The author’s research has been supported by grants from Novartis, Sanofi-Genzyme, and Merck. Author H-PH received honoraria for serving on steering, data monitoring or scientific advisory boards from Aurinia Pharma, BMS Celgene, Boehringer Ingelheim, Horizon Therapeutics, Merck, Novartis, Roche, TG Therapeutics. Author BVW has received advisory board and/or speaking fees from Almirall, Biogen, Merck, Novartis, Roche, and Sanofi; research support from Biogen, Merck, Novartis, and Sanofi, and contracted research from Biogen, Merck, Sanofi, Novartis, and Roche. Author PS has received personal compensation for consultations, serving on scientific advisory boards, steering committees, independent data monitoring committees or has received honoraria as speaker from Biogen, Merck, Novartis, TEVA, GlaxoSmithKline, SanofiAventis/Genzyme, and BMS/Celgene. Author TB has participated in meetings sponsored by and received honoraria lectures, advisory boards, consultations from pharmaceutical companies marketing treatments for multiple sclerosis: Almirall, Bayer, Biogen, Biologix, Bionorica, Bristol-Myers-Squibb, Eisai, GW Pharma, Horizon, Janssen-Cilag, MedDay, Merck, Novartis, Octapharma, Roche, Sandoz, Sanofi/Genzyme, TG Pharmaceuticals, TEVA-ratiopharm and UCB. His institution has received financial support in the last 12 months by unrestricted research grants Biogen, Bayer, Bristol-Myers-Squibb, Merck, Novartis, Roche, Sanofi/Genzyme, and TEVA ratiopharm and for participation in clinical trials in multiple sclerosis sponsored by Alexion, Bayer, Biogen, Bristol-Myers-Squibb, Merck, Novartis, Octapharma, Roche, Sanofi/Genzyme, and TEVA. Author CO-G has received speaking and consulting honoraria from Biogen, BMS, Merck, Novartis, Sanofi, Janssen, and Roche.
The remaining author declares that the research was conducted in the absence of any commercial or financial relationships that could be construed as a potential conflict of interest.
The author(s) declared that they were an editorial board member of Frontiers, at the time of submission. This had no impact on the peer review process and the final decisions.
Publisher’s note
All claims expressed in this article are solely those of the authors and do not necessarily represent those of their affiliated organizations, or those of the publisher, the editors and the reviewers. Any product that may be evaluated in this article, or claim that may be made by its manufacturer, is not guaranteed or endorsed by the publisher.
References
1. Walton C, King R, Rechtman L, Kaye W, Leray E, Marrie RA, et al. Rising prevalence of multiple sclerosis worldwide: Insights from the Atlas of MS, third edition. Mult Scler. (2020) 26:1816–21. doi: 10.1177/1352458520970841
2. MS International Federation. 3rd edition of the Atlas of MS . Available online at: https://www.atlasofms.org/map/global/epidemiology/number-of-people-with-ms#about (Accessed September 11, 2023).
3. Koch-Henriksen N, Thygesen LC, Stenager E, Laursen B, Magyari M. Incidence of MS has increased markedly over six decades in Denmark particularly with late onset and in women. Neurology. (2018) 90:e1954–63. doi: 10.1212/wnl.0000000000005612
4. Solaro C, Ponzio M, Moran E, Tanganelli P, Pizio R, Ribizzi G, et al. The changing face of multiple sclerosis: Prevalence and incidence in an aging population. Mult Scler. (2015) 21:1244–50. doi: 10.1177/1352458514561904
5. Marrie RA, Yu N, Blanchard J, Leung S, Elliott L. The rising prevalence and changing age distribution of multiple sclerosis in Manitoba. Neurology. (2010) 74:465–71. doi: 10.1212/WNL.0b013e3181cf6ec0
6. Sorensen PS, Sellebjerg F, Hartung HP, Montalban X, Comi G, Tintoré M. The apparently milder course of multiple sclerosis: changes in the diagnostic criteria, therapy and natural history. Brain. (2020) 143:2637–52. doi: 10.1093/brain/awaa145
7. Wallin MT, Culpepper WJ, Campbell JD, Nelson LM, Langer-Gould A, Marrie RA, et al. The prevalence of MS in the United States: A population-based estimate using health claims data. Neurology. (2019) 92:e1029–40. doi: 10.1212/wnl.0000000000007035
8. Kern DM, Cepeda MS. Treatment patterns and comorbid burden of patients newly diagnosed with multiple sclerosis in the United States. BMC Neurol. (2020) 20:296. doi: 10.1186/s12883-020-01882-2
9. Grytten N, Aarseth JH, Lunde HM, Myhr KM. A 60-year follow-up of the incidence and prevalence of multiple sclerosis in Hordaland County, Western Norway. J Neurol Neurosurg Psychiatry. (2016) 87:100–5. doi: 10.1136/jnnp-2014-309906
10. Lunde HMB, Assmus J, Myhr K-M, Bø L, Grytten N. Survival and cause of death in multiple sclerosis: a 60-year longitudinal population study. J Neurology Neurosurg Psychiatry. (2017) 88:621–5. doi: 10.1136/jnnp-2016-315238
11. Rotstein DL, Chen H, Wilton AS, Kwong JC, Marrie RA, Gozdyra P, et al. Temporal trends in multiple sclerosis prevalence and incidence in a large population. Neurology. (2018) 90:e1435–41. doi: 10.1212/wnl.0000000000005331
12. Kingwell E, van der Kop M, Zhao Y, Shirani A, Zhu F, Oger J, et al. Relative mortality and survival in multiple sclerosis: findings from British Columbia, Canada. J Neurol Neurosurg Psychiatry. (2012) 83:61–6. doi: 10.1136/jnnp-2011-300616
13. Rodríguez-Antigüedad Zarranz A, Mendibe Bilbao M, Llarena González C, Audicana C. Mortality and cause of death in multiple sclerosis: findings from a prospective population-based cohort in Bizkaia, Basque Country, Spain. Neuroepidemiology. (2014) 42:219–25. doi: 10.1159/000359971
14. Chalmer TA, Baggesen LM, Nørgaard M, Koch-Henriksen N, Magyari M, Sorensen PS. Early versus later treatment start in multiple sclerosis: a register-based cohort study. Eur J Neurol. (2018) 25:1262–e110. doi: 10.1111/ene.13692
15. Simonsen CS, Flemmen HØ, Broch L, Brunborg C, Berg-Hansen P, Moen SM, et al. Early high efficacy treatment in multiple sclerosis is the best predictor of future disease activity over 1 and 2 years in a norwegian population-based registry. Front Neurol. (2021) 12:693017. doi: 10.3389/fneur.2021.693017
16. Buron MD, Chalmer TA, Sellebjerg F, Barzinji I, Danny B, Christensen JR, et al. Initial high-efficacy disease-modifying therapy in multiple sclerosis: A nationwide cohort study. Neurology. (2020) 95:e1041–51. doi: 10.1212/wnl.0000000000010135
17. Rommer PS, Eichstädt K, Ellenberger D, Flachenecker P, Friede T, Haas J, et al. Symptomatology and symptomatic treatment in multiple sclerosis: Results from a nationwide MS registry. Multiple Sclerosis J. (2019) 25:1641–52. doi: 10.1177/1352458518799580
18. Soelberg Sorensen P, Giovannoni G, Montalban X, Thalheim C, Zaratin P, Comi G. The multiple sclerosis care unit. Mult Scler. (2019) 25:627–36. doi: 10.1177/1352458518807082
19. Wills OC, Probst YC. Understanding lifestyle self-management regimens that improve the life quality of people living with multiple sclerosis: a systematic review and meta-analysis. Health Qual Life Outcomes. (2022) 20:153. doi: 10.1186/s12955-022-02046-1
20. Amato MP, Derfuss T, Hemmer B, Liblau R, Montalban X, Soelberg Sørensen P, et al. Environmental modifiable risk factors for multiple sclerosis: Report from the 2016 ECTRIMS focused workshop. Mult Scler. (2018) 24:590–603. doi: 10.1177/1352458516686847
21. Romero-Pinel L, Bau L, Matas E, León I, Muñoz-Vendrell A, Arroyo P, et al. The age at onset of relapsing-remitting multiple sclerosis has increased over the last five decades. Mult Scler Relat Disord. (2022) 68:104103. doi: 10.1016/j.msard.2022.104103
22. Prosperini L, Lucchini M, Ruggieri S, Tortorella C, Haggiag S, Mirabella M, et al. Shift of multiple sclerosis onset towards older age. J Neurology Neurosurg Psychiatry. (2022) 93:1137–9. doi: 10.1136/jnnp-2022-329049
23. Zinganell A, Göbel G, Berek K, Hofer B, Asenbaum-Nan S, Barang M, et al. . doi: 10.1007/s00415-023-12041-1
24. Wallin MT, Culpepper WJ, Nichols E, Bhutta ZA, Gebrehiwot TT, Hay SI, et al. Global, regional, and national burden of multiple sclerosis 1990–2016: a systematic analysis for the Global Burden of Disease Study 2016. Lancet Neurol. (2019) 18:269–85. doi: 10.1016/S1474-4422(18)30443-5
25. MSBase Neuro-immunology Registry. Patient demographics . Available online at: https://www.msbase.org/data-and-findings/patient-demographics (Accessed January 9, 2024).
26. Patti F, Vila C. Symptoms, prevalence and impact of multiple sclerosis in younger patients: a multinational survey. Neuroepidemiology. (2014) 42:211–8. doi: 10.1159/000360423
27. Filippi M, Bar-Or A, Piehl F, Preziosa P, Solari A, Vukusic S, et al. Multiple sclerosis. Nat Rev Dis Primers. (2018) 4:43. doi: 10.1038/s41572-018-0041-4
28. Oreja-Guevara C, Alonso M, Calles MC, Cebolla S, Fernández Ó, Gich J, et al. Síntomas Invisibles de la Esclerosis Múltiple. Barcelona: Permanyer (2023).
29. Marrie RA, Horwitz R, Cutter G, Tyry T, Campagnolo D, Vollmer T. Comorbidity delays diagnosis and increases disability at diagnosis in MS. Neurology. (2009) 72:117–24. doi: 10.1212/01.wnl.0000333252.78173.5f
30. Kister I, Bacon T, Cutter GR. How multiple sclerosis symptoms vary by age, sex, and race/ethnicity. Neurol Clin Pract. (2021) 11:335–41. doi: 10.1212/cpj.0000000000001105
31. Kister I, Bacon TE, Chamot E, Salter AR, Cutter GR, Kalina JT, et al. Natural history of multiple sclerosis symptoms. Int J MS Care. (2013) 15:146–58. doi: 10.7224/1537-2073.2012-053
32. Wood B, van der Mei I, Ponsonby A-L, Pittas F, Quinn S, Dwyer T, et al. Prevalence and concurrence of anxiety, depression and fatigue over time in multiple sclerosis. Multiple Sclerosis J. (2013) 19:217–24. doi: 10.1177/1352458512450351
33. Buchanan RJ, Chakravorty BJ, Tyry T, Hatcher W, Vollmer T. Age-related comparisons of people with multiple sclerosis: demographic, disease, and treatment characteristics. NeuroRehabilitation. (2009) 25:271–8. doi: 10.3233/nre-2009-0525
34. Beal CC, Stuifbergen AK, Brown A. Depression in multiple sclerosis: a longitudinal analysis. Arch Psychiatr Nurs. (2007) 21:181–91. doi: 10.1016/j.apnu.2007.02.008
35. Koch MW, Patten S, Berzins S, Zhornitsky S, Greenfield J, Wall W, et al. Depression in multiple sclerosis: a long-term longitudinal study. Mult Scler. (2015) 21:76–82. doi: 10.1177/1352458514536086
36. Peres DS, Rodrigues P, Viero FT, Frare JM, Kudsi SQ, Meira GM, et al. Prevalence of depression and anxiety in the different clinical forms of multiple sclerosis and associations with disability: A systematic review and meta-analysis. Brain Behav Immun Health. (2022) 24:100484. doi: 10.1016/j.bbih.2022.100484
37. Mirmosayyeb O, Brand S, Barzegar M, Afshari-Safavi A, Nehzat N, Shaygannejad V, et al. Clinical characteristics and disability progression of early- and late-onset multiple sclerosis compared to adult-onset multiple sclerosis. J Clin Med. (2020) 9(5):1326. doi: 10.3390/jcm9051326
38. Lotti C. B., Oliveira A. S. B., Bichuetti D. B., de Castro I., Oliveira E. M. L. Late onset multiple sclerosis: concerns in aging patients. Arquivos Neuro-Psiquiatria. (2017) 75 (7). doi: 10.1590/0004-282x20170070
39. Shirani A, Zhao Y, Petkau J, Gustafson P, Karim ME, Evans C, et al. Multiple sclerosis in older adults: the clinical profile and impact of interferon Beta treatment. BioMed Res Int. (2015) 2015:451912. doi: 10.1155/2015/451912
40. Scalfari A, Neuhaus A, Daumer M, Ebers GC, Muraro PA. Age and disability accumulation in multiple sclerosis. Neurology. (2011) 77:1246–52. doi: 10.1212/WNL.0b013e318230a17d
41. Moura J, Duarte S, Oliveira V, Pereira D, Costa D, Samões R, et al. Characterization of a late-onset multiple sclerosis Portuguese cohort. Mult Scler Relat Disord. (2023) 70:104506. doi: 10.1016/j.msard.2023.104506
42. Guillemin F, Baumann C, Epstein J, Kerschen P, Garot T, Mathey G, et al. Older age at multiple sclerosis onset is an independent factor of poor prognosis: A population-based cohort study. Neuroepidemiology. (2017) 48:179–87. doi: 10.1159/000479516
43. Cortese M, Bjornevik K, Chitnis T, Ascherio A, Munger KL. Aging with multiple sclerosis: A longitudinal study of physical function, mental health, and memory in two cohorts of US women. Mult Scler. (2022) 28:121–31. doi: 10.1177/13524585211007739
44. Lublin FD, Häring DA, Ganjgahi H, Ocampo A, Hatami F, Čuklina J, et al. How patients with multiple sclerosis acquire disability. Brain. (2022) 145:3147–61. doi: 10.1093/brain/awac016
45. Hosseini Z, Homayuni A, Etemadifar M. Barriers to quality of life in patients with multiple sclerosis: a qualitative study. BMC Neurol. (2022) 22:174. doi: 10.1186/s12883-022-02700-7
46. Gil-González I, Martín-Rodríguez A, Conrad R, Pérez-San-Gregorio MÁ. Quality of life in adults with multiple sclerosis: a systematic review. BMJ Open. (2020) 10:e041249. doi: 10.1136/bmjopen-2020-041249
47. Pike J, Jones E, Rajagopalan K, Piercy J, Anderson P. Social and economic burden of walking and mobility problems in multiple sclerosis. BMC Neurol. (2012) 12:94. doi: 10.1186/1471-2377-12-94
48. Fernandez O, Costa-Frossard L, Martínez-Ginés ML, Montero P, Prieto-González JM, Ramió-Torrentà L. Integrated management of multiple sclerosis spasticity and associated symptoms using the spasticity-plus syndrome concept: results of a structured specialists’ Discussion using the workmat(®) methodology. Front Neurol. (2021) 12:722801. doi: 10.3389/fneur.2021.722801
49. Williams AE, Vietri JT, Isherwood G, Flor A. Symptoms and association with health outcomes in relapsing-remitting multiple sclerosis: results of a US patient survey. Mult Scler Int. (2014) 2014:203183. doi: 10.1155/2014/203183
50. Buhse M, Banker WM, Clement LM. Factors associated with health-related quality of life among older people with multiple sclerosis. Int J MS Care. (2014) 16:10–9. doi: 10.7224/1537-2073.2012-046
51. Stromillo ML, Giorgio A, Rossi F, Battaglini M, Hakiki B, Malentacchi G, et al. Brain metabolic changes suggestive of axonal damage in radiologically isolated syndrome. Neurology. (2013) 80:2090–4. doi: 10.1212/WNL.0b013e318295d707
52. Gärtner J, Chitnis T, Ghezzi A, Pohl D, Brück W, Häring DA, et al. Relapse rate and MRI activity in young adult patients with multiple sclerosis: A post hoc analysis of phase 3 fingolimod trials. Mult Scler J Exp Transl Clin. (2018) 4:2055217318778610. doi: 10.1177/2055217318778610
53. Tremlett H, Zhao Y, Joseph J, Devonshire V. and Neurologists* tUC, Relapses in multiple sclerosis are age- and time-dependent. J Neurology Neurosurg Psychiatry. (2008) 79:1368–74. doi: 10.1136/jnnp.2008.145805
54. Scalfari A, Lederer C, Daumer M, Nicholas R, Ebers GC, Muraro PA. The relationship of age with the clinical phenotype in multiple sclerosis. Mult Scler. (2016) 22:1750–8. doi: 10.1177/1352458516630396
55. Koch MW, Mostert J, Zhang Y, Wolinsky JS, Lublin FD, Strijbis EMM, et al. Association of age with contrast-enhancing lesions across the multiple sclerosis disease spectrum. Neurology. (2021) 97:e1334–e1342. doi: 10.1212/WNL.0000000000012603
56. Papadopoulos D, Magliozzi R, Mitsikostas DD, Gorgoulis VG, Nicholas RS. Aging, cellular senescence, and progressive multiple sclerosis. Front Cell Neurosci. (2020) 14:178. doi: 10.3389/fncel.2020.00178
57. Frischer JM, Weigand SD, Guo Y, Kale N, Parisi JE, Pirko I, et al. Clinical and pathological insights into the dynamic nature of the white matter multiple sclerosis plaque. Ann Neurol. (2015) 78:710–21. doi: 10.1002/ana.24497
58. Hemmer B, Kerschensteiner M, Korn T. Role of the innate and adaptive immune responses in the course of multiple sclerosis. Lancet Neurol. (2015) 14:406–19. doi: 10.1016/s1474-4422(14)70305-9
59. Eschborn M, Pawlitzki M, Wirth T, Nelke C, Pfeuffer S, Schulte-Mecklenbeck A, et al. Evaluation of age-dependent immune signatures in patients with multiple sclerosis. Neurol Neuroimmunol Neuroinflamm. (2021) 8(6):e1094. doi: 10.1212/nxi.0000000000001094
60. Rommer PS, Bsteh G, Zrzavy T, Hoeftberger R, Berger T. Immunosenescence in neurological diseases-is there enough evidence? Biomedicines. (2022) 10(11):2864. doi: 10.3390/biomedicines10112864
61. Macaron G, Larochelle C, Arbour N, Galmard M, Girard JM, Prat A, et al. Impact of aging on treatment considerations for multiple sclerosis patients. Front Neurol. (2023) 14:1197212. doi: 10.3389/fneur.2023.1197212
62. Perdaens O, van Pesch V. Molecular mechanisms of immunosenescene and inflammaging: relevance to the immunopathogenesis and treatment of multiple sclerosis. Front Neurol. (2021) 12:811518. doi: 10.3389/fneur.2021.811518
63. López-Otín C, Blasco MA, Partridge L, Serrano M, Kroemer G. Hallmarks of aging: An expanding universe. Cell. (2023) 186:243–78. doi: 10.1016/j.cell.2022.11.001
64. Neumann B, Segel M, Chalut KJ, Franklin RJ. Remyelination and ageing: Reversing the ravages of time. Mult Scler. (2019) 25:1835–41. doi: 10.1177/1352458519884006
65. Krysko KM, Henry RG, Cree BAC, Lin J, Caillier S, Santaniello A, et al. Telomere length is associated with disability progression in multiple sclerosis. Ann Neurol. (2019) 86:671–82. doi: 10.1002/ana.25592
66. Kuhlmann T, Moccia M, Coetzee T, Cohen JA, Correale J, Graves J, et al. Multiple sclerosis progression: time for a new mechanism-driven framework. Lancet Neurol. (2023) 22:78–88. doi: 10.1016/s1474-4422(22)00289-7
67. Capkun G, Dahlke F, Lahoz R, Nordstrom B, Tilson HH, Cutter G, et al. Mortality and comorbidities in patients with multiple sclerosis compared with a population without multiple sclerosis: An observational study using the US Department of Defense administrative claims database. Multiple Sclerosis Related Disord. (2015) 4:546–54. doi: 10.1016/j.msard.2015.08.005
68. Murtonen A, Kurki S, Hänninen K, Soilu-Hänninen M, Sumelahti ML. Common comorbidities and survival in MS: Risk for stroke, type 1 diabetes and infections. Mult Scler Relat Disord. (2018) 19:109–14. doi: 10.1016/j.msard.2017.10.019
69. Castelo-Branco A, Chiesa F, Bengtsson CE, Lee S, Minton NN, Niemcryk S, et al. Non-infectious comorbidity in patients with multiple sclerosis: A national cohort study in Sweden. Mult Scler J Exp Transl Clin. (2020) 6:2055217320947761. doi: 10.1177/2055217320947761
70. Laroni A, Signori A, Maniscalco GT, Lanzillo R, Russo CV, Binello E, et al. Assessing association of comorbidities with treatment choice and persistence in MS: A real-life multicenter study. Neurology. (2017) 89:2222–9. doi: 10.1212/wnl.0000000000004686
71. Ostolaza A, Corroza J, Ayuso T. Multiple sclerosis and aging: comorbidity and treatment challenges. Mult Scler Relat Disord. (2021) 50:102815. doi: 10.1016/j.msard.2021.102815
72. Marrie RA, Maxwell C, Mahar A, Ekuma O, McClintock C, Seitz D, et al. Colorectal cancer survival in multiple sclerosis. A Matched Cohort Study. (2021) 97:e1447–56. doi: 10.1212/wnl.0000000000012634
73. GianFrancesco MA, Barcellos LF. Obesity and multiple sclerosis susceptibility: A review. J Neurol Neuromedicine. (2016) 1:1–5. doi: 10.29245/2572.942x/2016/7.1064
74. Schreiner TG, Genes TM. Obesity and multiple sclerosis-A multifaceted association. J Clin Med. (2021) 10(12):2689. doi: 10.3390/jcm10122689
75. Wingerchuk DM. Smoking: effects on multiple sclerosis susceptibility and disease progression. Ther Adv Neurol Disord. (2012) 5:13–22. doi: 10.1177/1756285611425694
76. Manouchehrinia A, Huang J, Hillert J, Alfredsson L, Olsson T, Kockum I, et al. Smoking attributable risk in multiple sclerosis. Front Immunol. (2022) 13:840158. doi: 10.3389/fimmu.2022.840158
77. Kleerekooper I, Chua S, Foster PJ, Trip SA, Plant GT, Petzold A, et al. Associations of alcohol consumption and smoking with disease risk and neurodegeneration in individuals with multiple sclerosis in the United Kingdom. JAMA Netw Open. (2022) 5:e220902. doi: 10.1001/jamanetworkopen.2022.0902
78. Mokry LE, Ross S, Timpson NJ, Sawcer S, Davey Smith G, Richards JB. Obesity and multiple sclerosis: A mendelian randomization study. PloS Med. (2016) 13:e1002053. doi: 10.1371/journal.pmed.1002053
79. Langford A, Langer-Gould A, Zhang J. Are obesity and tobacco smoke risk factors for conversion from clinically isolated syndrome to multiple sclerosis? Neurology. (2013) 80:P05.140–P05.140. doi: 10.1212/WNL.80.7_supplement.P05.140
80. Wu J, Olsson T, Hillert J, Alfredsson L, Hedström AK. Influence of oral tobacco versus smoking on multiple sclerosis disease activity and progression. J Neurology Neurosurg Psychiatry. (2023) 94:589–96. doi: 10.1136/jnnp-2022-330848
81. Lutfullin I, Eveslage M, Bittner S, Antony G, Flaskamp M, Luessi F, et al. Association of obesity with disease outcome in multiple sclerosis. J Neurology Neurosurg Psychiatry. (2023) 94:57–61. doi: 10.1136/jnnp-2022-329685
82. Degelman ML, Herman KM. Smoking and multiple sclerosis: A systematic review and meta-analysis using the Bradford Hill criteria for causation. Mult Scler Relat Disord. (2017) 17:207–16. doi: 10.1016/j.msard.2017.07.020
83. Society M. Review of the evidence on smoking and MS . Available online at: https://www.mssociety.org.uk/sites/default/files/2021-11/Smoking%20and%20MS%20Evidence%20Review.pdf.
84. Almramhi MM, Storm CS, Kia DA, Coneys R, Chhatwal BK, Wood NW. The role of body fat in multiple sclerosis susceptibility and severity: A Mendelian randomisation study. Multiple Sclerosis J. (2022) 28:1673–84. doi: 10.1177/13524585221092644
85. Thelen J, Zvonarev V, Lam S, Burkhardt C, Lynch S, Bruce J. Polypharmacy in multiple sclerosis: current knowledge and future directions. Mo Med. (2021) 118:239–45.
86. Chertcoff A, Ng HS, Zhu F, Zhao Y, Tremlett H. Polypharmacy and multiple sclerosis: A population-based study. Multiple Sclerosis J. (2023) 29:107–18. doi: 10.1177/13524585221122207
87. Marrie RA, Fisk JD, Walld R, Bolton JM, Sareen J, Patten SB, et al. Use of benzodiazepines and Z-drugs in multiple sclerosis. Front Neurol. (2022) 13:874724. doi: 10.3389/fneur.2022.874724
88. Bakirtzis C, Nikolaidis I, Boziki MK, Grigoriadou E, Karakasi MV, Moysiadis T, et al. Epidemiological insights on medication concurrency and polypharmacy in people with multiple sclerosis in Greece. Int J MS Care. (2023) 25:140–4. doi: 10.7224/1537-2073.2022-046
89. Debus JL, Bachmann P, Frahm N, Mashhadiakbar P, Langhorst SE, Streckenbach B, et al. Associated factors of potential drug-drug interactions and drug-food interactions in patients with multiple sclerosis. Ther Adv Chronic Dis. (2022) 13:20406223221108391. doi: 10.1177/20406223221108391
90. Rawle MJ, Cooper R, Kuh D, Richards M. Associations between polypharmacy and cognitive and physical capability: A british birth cohort study. J Am Geriatr Soc. (2018) 66:916–23. doi: 10.1111/jgs.15317
91. Turnheim K. When drug therapy gets old: pharmacokinetics and pharmacodynamics in the elderly. Exp Gerontology. (2003) 38:843–53. doi: 10.1016/S0531-5565(03)00133-5
92. Magyari M, Sorensen PS. Comorbidity in multiple sclerosis. Front Neurol. (2020) 11:851. doi: 10.3389/fneur.2020.00851
93. Bove R, Okai A, Houtchens M, Elias-Hamp B, Lugaresi A, Hellwig K, et al. Effects of menopause in women with multiple sclerosis: an evidence-based review. Front Neurol. (2021) 12:554375. doi: 10.3389/fneur.2021.554375
94. Baroncini D, Annovazzi PO, Rossi ND, Mallucci G, Clerici VT, Tonietti S, et al. Impact of natural menopause on multiple sclerosis: a multicentre study. J Neurology Neurosurg Psychiatry. (2019) 90:1201–6. doi: 10.1136/jnnp-2019-320587
95. Ladeira F, Salavisa M, Caetano A, Barbosa R, Sá F, Correia Ana S. The influence of menopause in multiple sclerosis course: A longitudinal cohort study. Eur Neurol. (2019) 80:223–7. doi: 10.1159/000496374
96. Bridge F, Butzkueven H, van der Walt A, Jokubaitis VG. The impact of menopause on multiple sclerosis. Autoimmun Rev. (2023) 22:103363. doi: 10.1016/j.autrev.2023.103363
97. Lorefice L, D’Alterio MN, Firinu D, Fenu G, Cocco E. Impact of menopause in patients with multiple sclerosis: current perspectives. Int J Women’s Health. (2023) 15:103–9. doi: 10.2147/IJWH.S334719
98. Jacobs LD, Cookfair DL, Rudick RA, Herndon RM, Richert JR, Salazar AM, et al. Intramuscular interferon beta-1a for disease progression in relapsing multiple sclerosis. Ann Neurol. (1996) 39:285–94. doi: 10.1002/ana.410390304
99. Hartung H-P, Gonsette R, Konig N, Kwiecinski H, Guseo A, Morrissey SP, et al. Mitoxantrone in progressive multiple sclerosis: a placebo-controlled, double-blind, randomised, multicentre trial. Lancet. (2002) 360:2018–25. doi: 10.1016/S0140-6736(02)12023-X
100. Kappos L, Radue EW, O’Connor P, Polman C, Hohlfeld R, Calabresi P, et al. A placebo-controlled trial of oral fingolimod in relapsing multiple sclerosis. N Engl J Med. (2010) 362:387–401. doi: 10.1056/NEJMoa0909494
101. Fox RJ, Miller DH, Phillips JT, Hutchinson M, Havrdova E, Kita M, et al. Placebo-controlled phase 3 study of oral BG-12 or glatiramer in multiple sclerosis. New Engl J Med. (2012) 367:1087–97. doi: 10.1056/NEJMoa1206328
102. Cohen JA, Barkhof F, Comi G, Hartung HP, Khatri BO, Montalban X, et al. Oral fingolimod or intramuscular interferon for relapsing multiple sclerosis. N Engl J Med. (2010) 362:402–15. doi: 10.1056/NEJMoa0907839
103. Miller AE, Wolinsky JS, Kappos L, Comi G, Freedman MS, Olsson TP, et al. Oral teriflunomide for patients with a first clinical episode suggestive of multiple sclerosis (TOPIC): a randomised, double-blind, placebo-controlled, phase 3 trial. Lancet Neurol. (2014) 13:977–86. doi: 10.1016/s1474-4422(14)70191-7
104. Gold R, Kappos L, Arnold DL, Bar-Or A, Giovannoni G, Selmaj K, et al. Placebo-controlled phase 3 study of oral BG-12 for relapsing multiple sclerosis. N Engl J Med. (2012) 367:1098–107. doi: 10.1056/NEJMoa1114287
105. Montalban X, Hauser SL, Kappos L, Arnold DL, Bar-Or A, Comi G, et al. Ocrelizumab versus placebo in primary progressive multiple sclerosis. New Engl J Med. (2016) 376:209–20. doi: 10.1056/NEJMoa1606468
106. Hauser SL, Bar-Or A, Comi G, Giovannoni G, Hartung HP, Hemmer B, et al. Ocrelizumab versus interferon beta-1a in relapsing multiple sclerosis. N Engl J Med. (2017) 376:221–34. doi: 10.1056/NEJMoa1601277
107. Comi G, Kappos L, Selmaj KW, Bar-Or A, Arnold DL, Steinman L, et al. Safety and efficacy of ozanimod versus interferon beta-1a in relapsing multiple sclerosis (SUNBEAM): a multicentre, randomised, minimum 12-month, phase 3 trial. Lancet Neurol. (2019) 18:1009–20. doi: 10.1016/S1474-4422(19)30239-X
108. Hauser SL, Bar-Or A, Cohen JA, Comi G, Correale J, Coyle PK, et al. Ofatumumab versus teriflunomide in multiple sclerosis. New Engl J Med. (2020) 383:546–57. doi: 10.1056/NEJMoa1917246
109. The IFNB Multiple Sclerosis Study Group. Interferon beta-1b is effective in relapsing-remitting multiple sclerosis. I. Clinical results of a multicenter, randomized, double-blind, placebo-controlled trial. The IFNB Multiple Sclerosis Study Group. Neurology. (1993) 43:655–61. doi: 10.1212/WNL.43.4.655
110. Polman CH, O’Connor PW, Havrdova E, Hutchinson M, Kappos L, Miller DH, et al. Placebo-controlled trial of natalizumab for relapsing multiple sclerosis. New Engl J Med. (2006) 354:899–910. doi: 10.1056/NEJMoa044397
111. Coles AJ, Compston DA, Selmaj KW, Lake SL, Moran S, Margolin DH, et al. The CAMMS223 trial investigators, alemtuzumab vs. Interferon beta-1a in early multiple sclerosis. New Engl J Med. (2008) 359:1786–801. doi: 10.1056/NEJMoa0802670
112. Cohen JA, Coles AJ, Arnold DL, Confavreux C, Fox EJ, Hartung HP, et al. Alemtuzumab versus interferon beta 1a as first-line treatment for patients with relapsing-remitting multiple sclerosis: a randomised controlled phase 3 trial. Lancet. (2012) 380:1819–28. doi: 10.1016/s0140-6736(12)61769-3
113. Johnson KP, Brooks BR, Cohen JA, Ford CC, Goldstein J, Lisak RP, et al. Copolymer 1 reduces relapse rate and improves disability in relapsing-remitting multiple sclerosis: results of a phase III multicenter, double-blind placebo-controlled trial. Copolymer 1 Multiple Sclerosis Study Group Neurol. (1995) 45:1268–76. doi: 10.1212/wnl.45.7.1268
114. Kappos L, Bar-Or A, Cree BAC, Fox RJ, Giovannoni G, Gold R, et al. Siponimod versus placebo in secondary progressive multiple sclerosis (EXPAND): a double-blind, randomised, phase 3 study. Lancet. (2018) 391:1263–73. doi: 10.1016/S0140-6736(18)30475-6
115. Ebers GC. Randomised double-blind placebo-controlled study of interferon beta-1a in relapsing/remitting multiple sclerosis. PRISMS (Prevention of Relapses and Disability by Interferon beta-1a Subcutaneously in Multiple Sclerosis) Study Group. Lancet. (1998) 352:1498–504.
116. Polman CH, O'Connor PW, Havrdova E, Hutchinson M, Kappos L, Miller DH, et al. A randomized, placebo-controlled trial of natalizumab for relapsing multiple sclerosis. N Engl J Med. (2006) 354:899–910. doi: 10.1056/NEJMoa044397
117. O'Connor P, Wolinsky JS, Confavreux C, Comi G, Kappos L, Olsson TP, et al. Randomized trial of oral teriflunomide for relapsing multiple sclerosis. N Engl J Med. (2011) 365:1293–303. doi: 10.1056/NEJMoa1014656
118. Confavreux C, O'Connor P, Comi G, Freedman MS, Miller AE, Olsson TP, et al. Oral teriflunomide for patients with relapsing multiple sclerosis (TOWER): a randomised, double-blind, placebo-controlled, phase 3 trial. Lancet Neurol. (2014) 13:247–56. doi: 10.1016/s1474-4422(13)70308-9
119. Coles AJ, Compston DA, Selmaj KW, Lake SL, Moran S, Margolin DH, et al. Alemtuzumab vs. Interferon beta-1a early multiple sclerosis. N Engl J Med. (2008) 359:1786–801. doi: 10.1056/NEJMoa0802670
120. Giovannoni G, Comi G, Cook S, Rammohan K, Rieckmann P, Sørensen PS, et al. A placebo-controlled trial of oral cladribine for relapsing multiple sclerosis. New Engl J Med. (2010) 362:416–26. doi: 10.1056/NEJMoa0902533
121. Comi G, Bermel R, Bar-Or A, McGinley M, Arnold D, Henry R, et al. A multicentre, open label, single-arm, phase 3b study (CONSONANCE) to assess the effectiveness and safety of ocrelizumab in patients with primary and secondary progressive multiple sclerosis: year-1 interim analysis (P1-1.Virtual). Neurology. (2022) 98:652. doi: 10.1212/WNL.98.18_supplement.652
122. Kappos L, Fox RJ, Burcklen M, Freedman MS, Havrdová EK, Hennessy B, et al. Ponesimod compared with teriflunomide in patients with relapsing multiple sclerosis in the active-comparator phase 3 OPTIMUM study: A randomized clinical trial. JAMA Neurol. (2021) 78:558–67. doi: 10.1001/jamaneurol.2021.0405
123. Hutchinson M, Kappos L, Calabresi PA, Confavreux C, Giovannoni G, Galetta SL, et al. The efficacy of natalizumab in patients with relapsing multiple sclerosis: subgroup analyses of AFFIRM and SENTINEL. J Neurol. (2009) 256:405–15. doi: 10.1007/s00415-009-0093-1
124. Giovannoni G, Cook S, Rammohan K, Rieckmann P, Sørensen PS, Vermersch P, et al. Sustained disease-activity-free status in patients with relapsing-remitting multiple sclerosis treated with cladribine tablets in the CLARITY study: a post-hoc and subgroup analysis. Lancet Neurol. (2011) 10:329–37. doi: 10.1016/S1474-4422(11)70023-0
125. Newsome SD, Kieseier BC, Arnold DL, Shang S, Liu S, Hung S, et al. Subgroup and sensitivity analyses of annualized relapse rate over 2 years in the ADVANCE trial of peginterferon beta-1a in patients with relapsing-remitting multiple sclerosis. J Neurol. (2016) 263:1778–87. doi: 10.1007/s00415-016-8182-4
126. Cohen JA, Gold R, de Sèze J, Robertson D, Wiendl H, Wray S, et al. (2022). Efficacy of early ofatumumab versus late-switch from teriflunomide: subgroup analysis of the ALITHIOS open-label extension study by prior disease-modifying therapy exposure and age, in: 38th Congress of the European Committee for Treatment and Research in Multiple Sclerosis, Amsterdam, Netherlands, 2022 Oct 26-28.
127. Bass AD, Arroyo R, Boster AL, Boyko AN, Eichau S, Ionete C, et al. Alemtuzumab outcomes by age: Post hoc analysis from the randomized CARE-MS studies over 8 years. Mult Scler Relat Disord. (2021) 49:102717. doi: 10.1016/j.msard.2020.102717
128. Miller AE, O’Connor P, Wolinsky JS, Confavreux C, Kappos L, Olsson TP, et al. Pre-specified subgroup analyses of a placebo-controlled phase III trial (TEMSO) of oral teriflunomide in relapsing multiple sclerosis. Mult Scler. (2012) 18:1625–32. doi: 10.1177/1352458512450354
129. Turner B, Cree BAC, Kappos L, Montalban X, Papeix C, Wolinsky JS, et al. Ocrelizumab efficacy in subgroups of patients with relapsing multiple sclerosis. J Neurol. (2019) 266:1182–93. doi: 10.1007/s00415-019-09248-6
130. Signori A, Schiavetti I, Gallo F, Sormani MP. Subgroups of multiple sclerosis patients with larger treatment benefits: a meta-analysis of randomized trials. Eur J Neurol. (2015) 22:960–6. doi: 10.1111/ene.12690
131. Weideman AM, Tapia-Maltos MA, Johnson K, Greenwood M, Bielekova B. Meta-analysis of the age-dependent efficacy of multiple sclerosis treatments. Front Neurol. (2017) 8:577. doi: 10.3389/fneur.2017.00577
132. Zhang Y, Gonzalez Caldito N, Shirani A, Salter A, Cutter G, Culpepper W. 2nd, et al. Aging efficacy disease-modifying therapies multiple sclerosis: meta-analysis Clin trials. Ther Adv Neurol Disord. (2020) 13:1756286420969016. doi: 10.1177/1756286420969016
133. Wray S, Then Bergh F, Wundes A, Arnold DL, Drulovic J, Jasinska E, et al. Efficacy and safety outcomes with diroximel fumarate after switching from prior therapies or continuing on DRF: results from the phase 3 EVOLVE-MS-1 study. Adv Ther. (2022) 39:1810–31. doi: 10.1007/s12325-022-02068-7
134. Roche H-L. A study to evaluate ocrelizumab treatment in participants with progressive multiple sclerosis (CONSONANCE); NCT03523858 . Available online at: https://classic.clinicaltrials.gov/ct2/show/NCT03523858.
135. Roche H-L. This is an extension study of the roche P-trials to investigate safety and effectiveness of ocrelizumab in participants with multiple sclerosis (MS); NCT03599245 . Available online at: https://classic.clinicaltrials.gov/ct2/show/NCT03599245.
136. Comi G BR, Bar-Or A, McGinley M, Arnold D, Henry R, Benedict R, et al. A multicentre, open-label, single-arm, phase 3b study (CONSONANCE) to assess the effectiveness and safety of ocrelizumab in patients with primary and secondary progressive multiple sclerosis: Year 2 interim analysis, in: 38th Congress of the European Committee for Treatment and Research in Multiple Sclerosis (ECTRIMS 2022), , 26–28 October 2022. Amsterdam, Netherlands.
137. Vollmer BL, Wolf AB, Sillau S, Corboy JR, Alvarez E. Evolution of disease modifying therapy benefits and risks: an argument for de-escalation as a treatment paradigm for patients with multiple sclerosis. Front Neurol. (2021) 12:799138. doi: 10.3389/fneur.2021.799138
138. Ng HS, Graf J, Zhu F, Kingwell E, Aktas O, Albrecht P, et al. Disease-modifying drug uptake and health service use in the ageing MS population. Front Immunol. (2022) 12:794075. doi: 10.3389/fimmu.2021.794075
139. Amato MP, Fonderico M, Portaccio E, Pastò L, Razzolini L, Prestipino E, et al. Disease-modifying drugs can reduce disability progression in relapsing multiple sclerosis. Brain. (2020) 143:3013–24. doi: 10.1093/brain/awaa251
140. Zanghì A, Avolio C, Amato MP, Filippi M, Trojano M, Patti F, et al. First-line therapies in late-onset multiple sclerosis: An Italian registry study. Eur J Neurol. (2021) 28:4117–23. doi: 10.1111/ene.15006
141. Coyle PK, Gorritz M, Wade RL, Zhou Z, Khalid S, Maiese EM, et al. Real-world use of ofatumumab to treat multiple sclerosis 9 months post-FDA approval during COVID-19 pandemic. Multiple Sclerosis Related Disord. (2023) 78:104881. doi: 10.1016/j.msard.2023.104881
142. Patti F, Penaherrera JN, Zieger L, Wicklein E-M. Clinical characteristics of middle-aged and older patients with MS treated with interferon beta-1b: post-hoc analysis of a 2-year, prospective, international, observational study. BMC Neurol. (2021) 21:324. doi: 10.1186/s12883-021-02347-w
143. Chisari CG, Bianco A, Brescia Morra V, Calabrese M, Capone F, Cavalla P, et al. Effectiveness of ocrelizumab in primary progressive multiple sclerosis: a multicenter, retrospective, real-world study (OPPORTUNITY). Neurotherapeutics. (2023) 20(6):1696–706. doi: 10.1007/s13311-023-01415-y
144. Epstein S, Fong KT, De Jager PL, Levine L, Riley C, Wesley S, et al. Evaluation of ocrelizumab in older progressive multiple sclerosis patients. Mult Scler Relat Disord. (2021) 55:103171. doi: 10.1016/j.msard.2021.103171
145. Disanto G, Moccia M, Sacco R, Spiezia AL, Carotenuto A, Brescia Morra V, et al. Monitoring of safety and effectiveness of cladribine in multiple sclerosis patients over 50 years. Mult Scler Relat Disord. (2022) 58:103490. doi: 10.1016/j.msard.2022.103490
146. Grebenciucova E, Berger JR. Immunosenescence: the role of aging in the predisposition to neuro-infectious complications arising from the treatment of multiple sclerosis. Curr Neurol Neurosci Rep. (2017) 17:61. doi: 10.1007/s11910-017-0771-9
147. Goldman MD, Dwyer L, Coleman R, Sohn MW, Stuve O. Patient-specific factors modulate leukocyte response in dimethyl fumarate treated MS patients. PloS One. (2020) 15:e0228617. doi: 10.1371/journal.pone.0228617
148. Vaughn CB, Jakimovski D, Kavak KS, Ramanathan M, Benedict RHB, Zivadinov R, et al. Epidemiology and treatment of multiple sclerosis in elderly populations. Nat Rev Neurol. (2019) 15:329–42. doi: 10.1038/s41582-019-0183-3
149. Schweitzer F, Laurent S, Fink GR, Barnett MH, Reddel S, Hartung HP, et al. Age and the risks of high-efficacy disease modifying drugs in multiple sclerosis. Curr Opin Neurol. (2019) 32:305–12. doi: 10.1097/wco.0000000000000701
150. Jordan AL, Yang J, Fisher CJ, Racke MK, Mao-Draayer Y. Progressive multifocal leukoencephalopathy in dimethyl fumarate-treated multiple sclerosis patients. Mult Scler. (2022) 28:7–15. doi: 10.1177/1352458520949158
151. Prosperini L, Scarpazza C, Imberti L, Cordioli C, De Rossi N, Capra R. Age as a risk factor for early onset of natalizumab-related progressive multifocal leukoencephalopathy. J NeuroVirology. (2017) 23:742–9. doi: 10.1007/s13365-017-0561-9
152. Schwab N, Schneider-Hohendorf T, Hoyt T, Gross CC, Meuth SG, Klotz L, et al. Anti-JCV serology during natalizumab treatment: Review and meta-analysis of 17 independent patient cohorts analyzing anti-John Cunningham polyoma virus sero-conversion rates under natalizumab treatment and differences between technical and biological sero-converters. Multiple Sclerosis J. (2018) 24:563–73. doi: 10.1177/1352458517728814
153. Nakahara J, Tomaske L, Kume K, Takata T, Kamada M, Deguchi K, et al. Three cases of non-carryover fingolimod-PML: Is the risk in Japan increased? Neurol Neuroimmunol Neuroinflamm. (2019) 6:e559. doi: 10.1212/nxi.0000000000000559
154. Berger JR, Cree BA, Greenberg B, Hemmer B, Ward BJ, Dong VM, et al. Progressive multifocal leukoencephalopathy after fingolimod treatment. Neurology. (2018) 90:e1815–21. doi: 10.1212/wnl.0000000000005529
155. Prosperini L, de Rossi N, Scarpazza C, Moiola L, Cosottini M, Gerevini S, et al. Natalizumab-related progressive multifocal leukoencephalopathy in multiple sclerosis: findings from an italian independent registry. PloS One. (2016) 11:e0168376. doi: 10.1371/journal.pone.0168376
156. Jakimovski D, Eckert SP, Zivadinov R, Weinstock-Guttman B. Considering patient age when treating multiple sclerosis across the adult lifespan. Expert Rev Neurother. (2021) 21:353–64. doi: 10.1080/14737175.2021.1886082
157. Grebenciucova E, Reder AT, Bernard JT. Immunologic mechanisms of fingolimod and the role of immunosenescence in the risk of cryptococcal infection: A case report and review of literature. Mult Scler Relat Disord. (2016) 9:158–62. doi: 10.1016/j.msard.2016.07.015
158. Habek M, Piskač D, Gabelić T, Barun B, Adamec I, Krbot Skorić M. Hypogammaglobulinemia, infections and COVID-19 in people with multiple sclerosis treated with ocrelizumab. Mult Scler Relat Disord. (2022) 62:103798. doi: 10.1016/j.msard.2022.103798
159. Fuchs TA, Wattengel BA, Carter MT, El-Solh AA, Lesse AJ, Mergenhagen KA. Outcomes of multiple sclerosis patients admitted with COVID-19 in a large veteran cohort. Mult Scler Relat Disord. (2022) 64:103964. doi: 10.1016/j.msard.2022.103964
160. Simpson-Yap S, Brouwer ED, Kalincik T, Rijke N, Hillert JA, Walton C, et al. Associations of disease-modifying therapies with COVID-19 severity in multiple sclerosis. Neurology. (2021) 97:e1870–85. doi: 10.1212/wnl.0000000000012753
161. Louapre C, Collongues N, Stankoff B, Giannesini C, Papeix C, Bensa C, et al. Clinical characteristics and outcomes in patients with coronavirus disease 2019 and multiple sclerosis. JAMA Neurol. (2020) 77:1079–88. doi: 10.1001/jamaneurol.2020.2581
162. Zecca C, Gobbi C. Long-term treatment with anti-CD20 monoclonal antibodies is untenable because of risk: YES. Multiple Sclerosis J. (2022) 28:1173–1175. doi: 10.1177/13524585221088734
163. Prosperini L, Haggiag S, Tortorella C, Galgani S, Gasperini C. Age-related adverse events of disease-modifying treatments for multiple sclerosis: A meta-regression. Mult Scler. (2021) 27:1391–402. doi: 10.1177/1352458520964778
164. Buscarinu MC, Reniè R, Morena E, Romano C, Bellucci G, Marrone A, et al. Late-onset MS: disease course and safety-efficacy of DMTS. Front Neurol. (2022) 13:829331. doi: 10.3389/fneur.2022.829331
165. Negroski D, Sellers D, Khiabani A, Khiabani D. Real-world experience switching from high-efficacy infusions to cladribine tablets (EP1055). ECTRIMS; 2022. Amsterdam: Multiple Sclerosis J. (2022) 28:692–945. doi: 10.1016/j.msard.2022.104491
166. Giovannoni G, Coyle PK, Vermersch P, Walker B, Aldridge J, Nolting A, et al. Integrated lymphopenia analysis in younger and older patients with multiple sclerosis treated with cladribine tablts. Front Immunol. (2021) 12:763433. doi: 10.3389/fimmu.2021.763433
167. Talwar A, Earla JR, Hutton GJ, Aparasu RR. Prescribing of disease modifying agents in older adults with multiple sclerosis. Mult Scler Relat Disord. (2022) 57:103308. doi: 10.1016/j.msard.2021.103308
168. Filippi M, Amato MP, Centonze D, Gallo P, Gasperini C, Inglese M, et al. Early use of high-efficacy disease−modifying therapies makes the difference in people with multiple sclerosis: an expert opinion. J Neurol. (2022) 269:5382–94. doi: 10.1007/s00415-022-11193-w
169. Hartung H-P, Meuth SG, Thompson AJ. Paradigm shifts: Early initiation of high-efficacy disease-modifying treatment in multiple sclerosis. Multiple Sclerosis J. (2021) 27:1473–6. doi: 10.1177/13524585211033190
170. Freeman L, Longbrake EE, Coyle PK, Hendin B, Vollmer T. High-efficacy therapies for treatment-naïve individuals with relapsing–remitting multiple sclerosis. CNS Drugs. (2022) 36:1285–99. doi: 10.1007/s40263-022-00965-7
171. Wiendl H, Gold R, Zipp F, Berger T, Deisenhammer F, Di Pauli F, et al. Multiple sclerosis therapy consensus group (MSTCG): answers to the discussion questions. Neurological Res Pract. (2021) 3:44. doi: 10.1186/s42466-021-00140-1
172. Trojano M, Kalincik T, Iaffaldano P, Amato MP. Interrogating large multiple sclerosis registries and databases: what information can be gained? Curr Opin Neurol. (2022) 35:271–7. doi: 10.1097/wco.0000000000001057
173. Selmaj K, Cree BAC, Barnett M, Thompson A, Hartung H-P. Multiple sclerosis: time for early treatment with high-efficacy drugs. J Neurol. (2024) 271(1):105–15. doi: 10.1007/s00415-023-11969-8
174. Iaffaldano P, Lucisano G, Caputo F, Paolicelli D, Patti F, Zaffaroni M, et al. Long-term disability trajectories in relapsing multiple sclerosis patients treated with early intensive or escalation treatment strategies. Ther Adv Neurological Disord. (2021) 14:17562864211019574. doi: 10.1177/17562864211019574
175. Buron MD, Chalmer TA, Sellebjerg F, Barzinji I, Bech D, Christensen JR, et al. Initial high-efficacy disease-modifying therapy in multiple sclerosis. A nationwide cohort study. (2020) 95:e1041–51. doi: 10.1212/wnl.0000000000010135
176. Filippi M, Rocca MA. Rethinking multiple sclerosis treatment strategies. Lancet Neurol. (2020) 19:281–2. doi: 10.1016/S1474-4422(20)30063-6
177. Sorensen PS, Kopp TI, Joensen H, Olsson A, Sellebjerg F, Magyari M. Age and sex as determinants of treatment decisions in patients with relapsing-remitting MS. Mult Scler Relat Disord. (2021) 50:102813. doi: 10.1016/j.msard.2021.102813
178. Hartung HP, Meuth SG, Miller DM, Comi G. Stopping disease-modifying therapy in relapsing and progressive multiple sclerosis. Curr Opin Neurol. (2021) 34:598–603. doi: 10.1097/wco.0000000000000960
179. Bsteh G, Feige J, Ehling R, Auer M, Hegen H, Di Pauli F, et al. Discontinuation of disease-modifying therapies in multiple sclerosis - Clinical outcome and prognostic factors. Mult Scler. (2017) 23:1241–8. doi: 10.1177/1352458516675751
180. Corboy JR, Fox RJ, Kister I, Cutter GR, Morgan CJ, Seale R, et al. Risk of new disease activity in patients with multiple sclerosis who continue or discontinue disease-modifying therapies (DISCOMS): a multicentre, randomised, single-blind, phase 4, non-inferiority trial. Lancet Neurol. (2023) 22:568–77. doi: 10.1016/s1474-4422(23)00154-0
181. Jakimovski D, Kavak KS, Vaughn CB, Goodman AD, Coyle PK, Krupp L, et al. Discontinuation of disease modifying therapies is associated with disability progression regardless of prior stable disease and age. Multiple Sclerosis Related Disord. (2022) 57:103406. doi: 10.1016/j.msard.2021.103406
182. Coerver EME, Bourass A, Wessels MHJ, van Kempen ZLE, Jasperse MMS, Tonino BAR, et al. Discontinuation of first-line disease-modifying therapy in relapse onset multiple sclerosis. Mult Scler Relat Disord. (2023) 74:104706. doi: 10.1016/j.msard.2023.104706
183. Kaminsky AL, Omorou AY, Soudant M, Pittion-Vouyovitch S, Michaud M, Anxionnat R, et al. Discontinuation of disease-modifying treatments for multiple sclerosis in patients aged over 50 with disease Inactivity. J Neurol. (2020) 267:3518–27. doi: 10.1007/s00415-020-10029-9
184. Chappuis M, Rousseau C, Bajeux E, Wiertlewski S, Laplaud D, Le Page E, et al. Discontinuation of second- versus first-line disease-modifying treatment in middle-aged patients with multiple sclerosis. J Neurol. (2023) 270:413–22. doi: 10.1007/s00415-022-11341-2
185. Fagius J, Feresiadou A, Larsson EM, Burman J. Discontinuation of disease modifying treatments in middle aged multiple sclerosis patients. First line Drugs vs natalizumab. Mult Scler Relat Disord. (2017) 12:82–7. doi: 10.1016/j.msard.2017.01.009
186. Bonenfant J, Bajeux E, Deburghgraeve V, Le Page E, Edan G, Kerbrat A. Can we stop immunomodulatory treatments in secondary progressive multiple sclerosis? Eur J Neurol. (2017) 24:237–44. doi: 10.1111/ene.13181
187. Goldschmidt CH, Glassman J, Ly B, Harvey T, Hua LE. A Retrospective Study on the Effects of De-Escalation of Disease-Modifying Therapy in Patients With Multiple Sclerosis. Presented at: 2023 CMSC Annual Meeting; May 31 to June 3; Aurora, CO. DMT01.
188. Montalban X, Gold R, Thompson AJ, Otero-Romero S, Amato MP, Chandraratna D, et al. ECTRIMS/EAN guideline on the pharmacological treatment of people with multiple sclerosis. Eur J Neurol. (2018) 25:215–37. doi: 10.1111/ene.13536
189. Rae-Grant A, Day GS, Marrie RA, Rabinstein A, Cree BAC, Gronseth GS, et al. Comprehensive systematic review summary: Disease-modifying therapies for adults with multiple sclerosis. Rep Guideline Development Dissemination Implementation Subcommittee Am Acad Neurol. (2018) 90:789–800. doi: 10.1212/wnl.0000000000005345
190. Krämer J, Bar-Or A, Turner TJ, Wiendl H. Bruton tyrosine kinase inhibitors for multiple sclerosis. Nat Rev Neurol. (2023) 19:289–304. doi: 10.1038/s41582-023-00800-7
191. Ringheim GE, Wampole M, Oberoi K. Bruton's tyrosine kinase (BTK) inhibitors and autoimmune diseases: making sense of BTK inhibitor specificity profiles and recent clinical trial successes and failures. Front Immunol. (2021) 12:662223. doi: 10.3389/fimmu.2021.662223
192. Montalban X, Arnold DL, Weber MS, Staikov I, Piasecka-Stryczynska K, Willmer J, et al. Placebo-controlled trial of an oral BTK inhibitor in multiple sclerosis. N Engl J Med. (2019) 380:2406–17. doi: 10.1056/NEJMoa1901981
193. Reich DS, Arnold DL, Vermersch P, Bar-Or A, Fox RJ, Matta A, et al. Safety and efficacy of tolebrutinib, an oral brain-penetrant BTK inhibitor, in relapsing multiple sclerosis: a phase 2b, randomised, double-blind, placebo-controlled trial. Lancet Neurol. (2021) 20:729–38. doi: 10.1016/S1474-4422(21)00237-4
194. group M. Merck provides update on phase III results for evobrutinib in relapsing multiple sclerosis . Available online at: https://www.merckgroup.com/en/news/evobrutinib-phase-lll.html (Accessed December 18, 2023).
195. Nave K-A. Myelination and the trophic support of long axons. Nat Rev Neurosci. (2010) 11:275–83. doi: 10.1038/nrn2797
196. Duncan ID, Radcliff AB, Heidari M, Kidd G, August BK, Wierenga LA. The adult oligodendrocyte can participate in remyelination. Proc Natl Acad Sci U.S.A. (2018) 115:E11807–e11816. doi: 10.1073/pnas.1808064115
197. Boyd A, Zhang H, Williams A. Insufficient OPC migration into demyelinated lesions is a cause of poor remyelination in MS and mouse models. Acta Neuropathol. (2013) 125:841–59. doi: 10.1007/s00401-013-1112-y
198. Franklin RJM, Frisén J, Lyons DA. Revisiting remyelination: Towards a consensus on the regeneration of CNS myelin. Semin Cell Dev Biol. (2021) 116:3–9. doi: 10.1016/j.semcdb.2020.09.009
199. Cadavid D, Balcer L, Galetta S, Aktas O, Ziemssen T, Vanopdenbosch LJ, et al. Predictors of response to opicinumab in acute optic neuritis. Ann Clin Transl Neurol. (2018) 5:1154–62. doi: 10.1002/acn3.620
200. Heß K, Starost L, Kieran NW, Thomas C, Vincenten MCJ, Antel J, et al. Lesion stage-dependent causes for impaired remyelination in MS. Acta Neuropathol. (2020) 140:359–75. doi: 10.1007/s00401-020-02189-9
201. Sim FJ, Zhao C, Penderis J, Franklin RJ. The age-related decrease in CNS remyelination efficiency is attributable to an impairment of both oligodendrocyte progenitor recruitment and differentiation. J Neurosci. (2002) 22:2451–9. doi: 10.1523/jneurosci.22-07-02451.2002
202. Franklin RJM. Why does remyelination fail in multiple sclerosis? Nat Rev Neurosci. (2002) 3:705–14. doi: 10.1038/nrn917
203. Lubetzki C, Zalc B, Williams A, Stadelmann C, Stankoff B. Remyelination in multiple sclerosis: from basic science to clinical translation. Lancet Neurol. (2020) 19:678–88. doi: 10.1016/S1474-4422(20)30140-X
204. Cree BAC, Cutter G, Wolinsky JS, Freedman MS, Comi G, Giovannoni G, et al. Safety and efficacy of MD1003 (high-dose biotin) in patients with progressive multiple sclerosis (SPI2): a randomised, double-blind, placebo-controlled, phase 3 trial. Lancet Neurol. (2020) 19:988–97. doi: 10.1016/S1474-4422(20)30347-1
205. Tourbah A, Gout O, Vighetto A, Deburghgraeve V, Pelletier J, Papeix C, et al. MD1003 (High-dose pharmaceutical-grade biotin) for the treatment of chronic visual loss related to optic neuritis in multiple sclerosis: A randomized, double-blind, placebo-controlled study. CNS Drugs. (2018) 32:661–72. doi: 10.1007/s40263-018-0528-2
206. Tourbah A, Lebrun-Frenay C, Edan G, Clanet M, Papeix C, Vukusic S, et al. MD1003 (high-dose biotin) for the treatment of progressive multiple sclerosis: A randomised, double-blind, placebo-controlled study. Mult Scler. (2016) 22:1719–31. doi: 10.1177/1352458516667568
207. Newsome SD, Tian F, Shoemaker T, Fitzgerald KC, Cassard SD, Fiol J, et al. A phase 1b, open-label study to evaluate the safety and tolerability of the putative remyelinating agent, liothyronine, in individuals with MS. Neurotherapeutics. (2023) 20:1263–74. doi: 10.1007/s13311-023-01402-3
208. Pelletier J, Ranjeva J-P, Tourbah A, Edan G, Barillot C, Mer SLL, et al. Results of a Phase 1b study to confirm safety and tolerability of olesoxime in multiple sclerosis patients. Neurology. (2015) 84:P7.282. doi: 10.1212/WNL.84.14
209. Green AJ, Gelfand JM, Cree BA, Bevan C, Boscardin WJ, Mei F, et al. Clemastine fumarate as a remyelinating therapy for multiple sclerosis (ReBUILD): a randomised, controlled, double-blind, crossover trial. Lancet. (2017) 390:2481–9. doi: 10.1016/S0140-6736(17)32346-2
210. Metz LM, Cerchiaro G, Almalik Y, Makkawi SO, Zedde A. Quetiapine is not tolerable to people with MS in doses potentially required to enhance myelin repair. Am Acad Neurology. (2020) 94 (15_supplement). doi: 10.1212/WNL.94.15_supplement.2868
211. Cadavid D, Mellion M, Hupperts R, Edwards KR, Calabresi PA, Drulović J, et al. Safety and efficacy of opicinumab in patients with relapsing multiple sclerosis (SYNERGY): a randomised, placebo-controlled, phase 2 trial. Lancet Neurol. (2019) 18:845–56. doi: 10.1016/s1474-4422(19)30137-1
212. Biogen. Efficacy and safety of BIIB033 (Opicinumab) as an add-on therapy to disease-modifying therapies (DMTs) in relapsing multiple sclerosis (MS) (AFFINITY). Available online at: https://clinicaltrials.gov/study/NCT03222973?tab=results.
213. Greenberg BM, Bowen JD, Alvarez E, Rodriguez M, Caggiano AO, Warrington AE, et al. placebo-controlled, single-ascending-dose intravenous infusion study of rHIgM22 in subjects with multiple sclerosis immediately following a relapse. Mult Scler J Exp Transl Clin. (2022) 8:20552173221091475. doi: 10.1177/20552173221091475
214. Metz L. Pilot trial of domperidone in relapsing-remitting multiple sclerosis (RRMS) . Available online at: https://clinicaltrials.gov/study/NCT02493049#collaborators-and-investigators (Accessed October 2, 2023).
215. Chataway J, De Angelis F, Connick P, Parker RA, Plantone D, Doshi A, et al. Efficacy of three neuroprotective drugs in secondary progressive multiple sclerosis (MS-SMART): a phase 2b, multiarm, double-blind, randomised placebo-controlled trial. Lancet Neurol. (2020) 19:214–25. doi: 10.1016/S1474-4422(19)30485-5
216. Cree BA, Ziemann A, Pfleeger K, Schwefel B, Wundes A, Freedman MS, et al. Safety and efficacy of elezanumab in relapsing and progressive forms of multiple sclerosis: results from two phase 2 studies, RADIUS-R and RADIUS-P. Mult Scler J. (2021) 27(2 SUPPL):92–3. doi: 10.1177/13524585211044647
217. Willekens B. Metformin add-on clinical study in multiple sclerosis to evaluate brain remyelination and neurodegeneration. Available online at: https://clinicaltrials.gov/study/NCT05893225?cond=Multiple%20Sclerosis&intr=%E2%80%9Cremyelination%E2%80%9D&rank=3#collaborators-and-investigators (Accessed October 2, 2023).
218. Jones CD, Motl R, Sandroff BM. Depression in multiple sclerosis: Is one approach for its management enough? Multiple Sclerosis Related Disord. (2021) 51:102904. doi: 10.1016/j.msard.2021.102904
219. Raissi A, Bulloch AGM, Fiest KM, McDonald K, Jetté N, Patten SB. Exploration of undertreatment and patterns of treatment of depression in multiple sclerosis. Int J MS Care. (2015) 17:292–300. doi: 10.7224/1537-2073.2014-084
220. Zhang E, Tian X, Li R, Chen C, Li M, Ma L, et al. Dalfampridine in the treatment of multiple sclerosis: a meta-analysis of randomised controlled trials. Orphanet J Rare Dis. (2021) 16:87. doi: 10.1186/s13023-021-01694-8
221. Shi J, Wu X, Chen Y. Study on Dalfampridine in the treatment of Multiple Sclerosis Mobility Disability: A meta-analysis. PloS One. (2019) 14:e0222288. doi: 10.1371/journal.pone.0222288
222. Valet M, Quoilin M, Lejeune T, Stoquart G, Van Pesch V, El Sankari S, et al. Effects of fampridine in people with multiple sclerosis: A systematic review and meta-analysis. CNS Drugs. (2019) 33:1087–99. doi: 10.1007/s40263-019-00671-x
223. Ghorbanpour S, Rahimibarghani S, Rohani S, Rastkar M, Ghajarzadeh M. Fampridine for gait imbalance in patients with multiple sclerosis (MS): a systematic review and meta-analysis. Neurol Sci. (2023) 44:3059–69. doi: 10.1007/s10072-023-06795-9
224. Fu X, Wang Y, Wang C, Wu H, Li J, Li M, et al. A mixed treatment comparison on efficacy and safety of treatments for spasticity caused by multiple sclerosis: a systematic review and network meta-analysis. Clin Rehabil. (2018) 32:713–21. doi: 10.1177/0269215517745348
225. Torres-Moreno MC, Papaseit E, Torrens M, Farré M. Assessment of efficacy and tolerability of medicinal cannabinoids in patients with multiple sclerosis: A systematic review and meta-analysis. JAMA Netw Open. (2018) 1:e183485. doi: 10.1001/jamanetworkopen.2018.3485
226. da Rovare VP, Magalhães GPA, Jardini GDA, Beraldo ML, Gameiro MO, Agarwal A, et al. Cannabinoids for spasticity due to multiple sclerosis or paraplegia: A systematic review and meta-analysis of randomized clinical trials. Complement Ther Med. (2017) 34:170–85. doi: 10.1016/j.ctim.2017.08.010
227. Kleiner D, Horváth IL, Bunduc S, Gergő D, Lugosi K, Fehérvári P, et al. Nabiximols is efficient as add-on treatment for patients with multiple sclerosis spasticity refractory to standard treatment: A systematic review and meta-analysis of randomised clinical trials. Curr Neuropharmacol. (2023) 21:2505–15. doi: 10.2174/1570159x21666230727094431
228. Hajebrahimi S, Darvishi A, HajEbrahimi R, Asadi N, Jafari Shendi Z, Asiaban N, et al. Efficacy and safety of desmopressin in nocturia and nocturnal polyuria control of neurological patients: A systematic review and meta-analysis. Neurourol Urodyn. (2024) 43(1):167–82. doi: 10.1002/nau.25291
229. Perez DQ, Espiritu AI, Jamora RDG. Efficacy and safety of amantadine for the treatment of fatigue in multiple sclerosis: a systematic review and meta-analysis. Neurodegener Dis Manag. (2020) 10:383–95. doi: 10.2217/nmt-2020-0030
230. Yang TT, Wang L, Deng XY, Yu G. Pharmacological treatments for fatigue in patients with multiple sclerosis: A systematic review and meta-analysis. J Neurol Sci. (2017) 380:256–61. doi: 10.1016/j.jns.2017.07.042
231. Motavalli A, Majdi A, Hosseini L, Talebi M, Mahmoudi J, Hosseini SH, et al. Pharmacotherapy in multiple sclerosis-induced cognitive impairment: A systematic review and meta-analysis. Mult Scler Relat Disord. (2020) 46:102478. doi: 10.1016/j.msard.2020.102478
232. Zheng X, Wei W, Liu P, Wu C, Lu L, Tang C. Botulinum toxin type A for hand tremor: a meta-analysis of randomised controlled trials. Neurol Neurochir Pol. (2020) 54:561–7. doi: 10.5603/PJNNS.a2020.0079
233. Pourmohammadi A, Riahi R, Hosseini SM, Adibi I. Pharmacological treatment of tremor in multiple sclerosis; a systematic review. Mult Scler Relat Disord. (2022) 60:103722. doi: 10.1016/j.msard.2022.103722
234. Chou R, Peterson K, Helfand M. Comparative efficacy and safety of skeletal muscle relaxants for spasticity and musculoskeletal conditions: a systematic review. J Pain Symptom Manage. (2004) 28:140–75. doi: 10.1016/j.jpainsymman.2004.05.002
235. Pöllmann W, Feneberg W, Steinbrecher A, Haupts MR, Henze T. [Therapy of pain syndromes in multiple sclerosis – an overview with evidence-based recommendations]. Fortschr Neurol Psychiatr. (2005) 73:268–85. doi: 10.1055/s-2004-830193
236. Otero-Romero S, Sastre-Garriga J, Comi G, Hartung HP, Soelberg Sørensen P, Thompson AJ, et al. Pharmacological management of spasticity in multiple sclerosis: Systematic review and consensus paper. Mult Scler. (2016) 22:1386–96. doi: 10.1177/1352458516643600
237. Oreja-Guevara C, Montalban X, de Andrés C, Casanova-Estruch B, Muñoz-García D, García I, et al. Consensus document on spasticity in patients with multiple sclerosis. Grupo de Enfermedades Desmielinizantes de la Sociedad Española de Neurología. Rev Neurol. (2013) 57:359–73.
238. Vollmer TL, Robinson MJ, Risser RC, Malcolm SK. A randomized, double-blind, placebo-controlled trial of duloxetine for the treatment of pain in patients with multiple sclerosis. Pain Pract. (2014) 14:732–44. doi: 10.1111/papr.12127
239. Prnjavorac B, Kunic S, Pejanovic-Skobic N, Gorana NP, Zirojevic D, Vukas SK, et al. Pregabalin in the treatment of peripheral and central chronic neuropathic pain. Mater Sociomed. (2023) 35:42–7. doi: 10.5455/msm.2023.35.42-47
240. Solaro C, Bergamaschi R, Rezzani C, Mueller M, Trabucco E, Bargiggia V, et al. Duloxetine is effective in treating depression in multiple sclerosis patients: an open-label multicenter study. Clin Neuropharmacol. (2013) 36:114–6. doi: 10.1097/WNF.0b013e3182996400
241. Carta MG, Paribello P, Anastasia A, De Berardis D, Nardi AE, Fornaro M. Pharmacological management of depression in patients with multiple sclerosis. Expert Opin Pharmacotherapy. (2018) 19:1533–40. doi: 10.1080/14656566.2018.1516207
242. Baghbanian SM, Khalili B, Bakhshi A, Azizi H, Ghazaeian M. The effects of bupropion on sexual dysfunction in female patients with multiple sclerosis: A double-blind randomized clinical trial. Mult Scler Relat Disord. (2023) 69:104399. doi: 10.1016/j.msard.2022.104399
243. Schneider SA, Deuschl G. The treatment of tremor. Neurotherapeutics. (2014) 11:128–38. doi: 10.1007/s13311-013-0230-5
244. Panicker JN, Fowler CJ, Kessler TM. Lower urinary tract dysfunction in the neurological patient: clinical assessment and management. Lancet Neurol. (2015) 14:720–32. doi: 10.1016/s1474-4422(15)00070-8
245. Bapir R, Bhatti KH, Eliwa A, García-Perdomo HA, Gherabi N, Hennessey D, et al. Efficacy of overactive neurogenic bladder treatment: A systematic review of randomized controlled trials. Arch Ital Urol Androl. (2022) 94:492–506. doi: 10.4081/aiua.2022.4.492
246. Brucker BM, Jericevic D, Rude T, Enemchukwu E, Pape D, Rosenblum N, et al. Mirabegron versus solifenacin in multiple sclerosis patients with overactive bladder symptoms: A prospective comparative nonrandomized study. Urology. (2020) 145:94–9. doi: 10.1016/j.urology.2020.08.008
247. Horstmann M, Schaefer T, Aguilar Y, Stenzl A, Sievert KD. Neurogenic bladder treatment by doubling the recommended antimuscarinic dosage. Neurourol Urodyn. (2006) 25:441–5. doi: 10.1002/nau.20289
248. Amend B, Hennenlotter J, Schäfer T, Horstmann M, Stenzl A, Sievert K-D. Effective treatment of neurogenic detrusor dysfunction by combined high-dosed antimuscarinics without increased side-effects. Eur Urol. (2008) 53:1021–8. doi: 10.1016/j.eururo.2008.01.007
249. Aharony SM, Lam O, Corcos J. Treatment of lower urinary tract symptoms in multiple sclerosis patients: Review of the literature and current guidelines. Can Urol Assoc J. (2017) 11:E110–e115. doi: 10.5489/cuaj.4059
250. Mills RJ, Yap L, Young CA. Treatment for ataxia in multiple sclerosis. Cochrane Database Syst Rev. (2007) 24(1):Cd005029. doi: 10.1002/14651858.CD005029.pub2
251. Nicholas RS, Friede T, Hollis S, Young CA. Anticholinergics for urinary symptoms in multiple sclerosis. Cochrane Database Syst Rev. (2009) 21(1):Cd004193. doi: 10.1002/14651858.CD004193.pub2
252. Mücke M, Cuhls H, Peuckmann-Post V, Minton O, Stone P, Radbruch L. Pharmacological treatments for fatigue associated with palliative care. Cochrane Database Syst Rev. (2015) 2015):Cd006788. doi: 10.1002/14651858.CD006788.pub3
253. Miller P, Soundy A. The pharmacological and non-pharmacological interventions for the management of fatigue related multiple sclerosis. J Neurol Sci. (2017) 381:41–54. doi: 10.1016/j.jns.2017.08.012
254. Koch MW, Glazenborg A, Uyttenboogaart M, Mostert J, De Keyser J. Pharmacologic treatment of depression in multiple sclerosis. Cochrane Database Syst Rev. (2011) 16(2):Cd007295. doi: 10.1002/14651858.CD007295.pub2
255. Gil-Sanchez A, Canudes M, Valcheva P, Nogueras L, González-Mingot C, Hervás JV, et al. Effects of vortioxetine on cognition and fatigue in patients with multiple sclerosis and depression: a case series study. CNS Neurol Disord Drug Targets. (2024) 23(3):395–401. doi: 10.2174/1871527322666230321093133
256. Cotter J, Muhlert N, Talwar A, Granger K. Examining the effectiveness of acetylcholinesterase inhibitors and stimulant-based medications for cognitive dysfunction in multiple sclerosis: A systematic review and meta-analysis. Neurosci Biobehav Rev. (2018) 86:99–107. doi: 10.1016/j.neubiorev.2018.01.006
257. Seppala LJ, Wermelink A, de Vries M, Ploegmakers KJ, van de Glind EMM, Daams JG, et al. Fall-risk-increasing drugs: A systematic review and meta-analysis: II. Psychotropics. J Am Med Dir Assoc. (2018) 19:371.e11–371.e17. doi: 10.1016/j.jamda.2017.12.098
258. Cruce R, Vosoughi R, Freedman MS. Cognitive impact of anticholinergic medication in MS: Adding insult to injury? Mult Scler Relat Disord. (2012) 1:156–61. doi: 10.1016/j.msard.2012.05.002
259. Meza R, Peña J, García K, Corsi O, Rada G. Are cannabinoids effective in multiple sclerosis? Medwave. (2017) 17:e6865. doi: 10.5867/medwave.2017.6865
260. Selph S, Carson S, Fu R, Thakurta S, Low A, McDonagh M. Drug class reviews, drug class review: neuropathic pain: final update 1 report. [Internet]. Portland (OR): Oregon Health & Science University (2011).
261. Otero-Romero S, Rodríguez-García J, Vilella A, Ara JR, Brieva L, Calles C, et al. Recommendations for vaccination in patients with multiple sclerosis who are eligible for immunosuppressive therapies: Spanish consensus statement. Neurología (English Edition). (2021) 36:50–60. doi: 10.1016/j.nrleng.2020.02.010
262. Byrnes KL, Whillier S. Effects of nonpharmaceutical treatments on symptom management in adults with mild or moderate multiple sclerosis: ;Meta-analysis. J Manipulative Physiol Ther. (2019) 42:514–31. doi: 10.1016/j.jmpt.2018.12.010
263. Asano M, Finlayson ML. Meta-analysis of three different types of fatigue management interventions for people with multiple sclerosis: exercise, education, and medication. Mult Scler Int. (2014) 2014:798285. doi: 10.1155/2014/798285
264. Zielińska-Nowak E, Włodarczyk L, Kostka J, Miller E. New strategies for rehabilitation and pharmacological treatment of fatigue syndrome in multiple sclerosis. J Clin Med. (2020) 9:3592. doi: 10.3390/jcm9113592
265. Gitman V, Moss K, Hodgson D. A systematic review and meta-analysis of the effects of non-pharmacological interventions on quality of life in adults with multiple sclerosis. Eur J Med Res. (2023) 28:294. doi: 10.1186/s40001-023-01185-5
266. Döring A, Pfueller CF, Paul F, Dörr J. Exercise in multiple sclerosis – an integral component of disease management. Epma J. (2011) 3:2. doi: 10.1007/s13167-011-0136-4
267. Binshalan T, Nair KPS, McNeill A. The effectiveness of physiotherapy interventions for mobility in severe multiple sclerosis: A systematic review and meta-analysis. Mult Scler Int. (2022) 2022:2357785. doi: 10.1155/2022/2357785
268. Etoom M, Khraiwesh Y, Lena F, Hawamdeh M, Hawamdeh Z, Centonze D, et al. Effectiveness of physiotherapy interventions on spasticity in people with multiple sclerosis: A systematic review and meta-analysis. Am J Phys Med Rehabil. (2018) 97:793–807. doi: 10.1097/phm.0000000000000970
269. Amedoro A, Berardi A, Conte A, Pelosin E, Valente D, Maggi G, et al. The effect of aquatic physical therapy on patients with multiple sclerosis: A systematic review and meta-analysis. Mult Scler Relat Disord. (2020) 41:102022. doi: 10.1016/j.msard.2020.102022
270. Gopal A, Sydow R, Block V, Allen DD. Effectiveness of physical therapy in addressing sexual dysfunction in individuals with multiple sclerosis: A systematic review and meta-analysis. Int J MS Care. (2021) 23:213–22. doi: 10.7224/1537-2073.2020-039
271. Abou L, Qin K, Alluri A, Du Y, Rice LA. The effectiveness of physical therapy interventions in reducing falls among people with multiple sclerosis: A systematic review and meta-analysis. J Bodyw Mov Ther. (2022) 29:74–85. doi: 10.1016/j.jbmt.2021.09.015
272. García-Muñoz C, Cortés-Vega MD, Heredia-Rizo AM, Martín-Valero R, García-Bernal MI, Casuso-Holgado MJ. Effectiveness of vestibular training for balance and dizziness rehabilitation in people with multiple sclerosis: A systematic review and meta-analysis. J Clin Med. (2020) 9(2):590. doi: 10.3390/jcm9020590
273. Alphonsus KB, Su Y, D’Arcy C. The effect of exercise, yoga and physiotherapy on the quality of life of people with multiple sclerosis: Systematic review and meta-analysis. Complementary Therapies Med. (2019) 43:188–95. doi: 10.1016/j.ctim.2019.02.010
274. Veneri D, Gannotti M, Bertucco M, Fournier Hillman SE. Using the international classification of functioning, disability, and health model to gain perspective of the benefits of yoga in stroke, multiple sclerosis, and children to inform practice for children with cerebral palsy: A meta-analysis. J Altern Complement Med. (2018) 24:439–57. doi: 10.1089/acm.2017.0030
275. Castellano-Aguilera A, Biviá-Roig G, Cuenca-Martínez F, Suso-Martí L, Calatayud J, Blanco-Díaz M, et al. Effectiveness of virtual reality on balance and risk of falls in people with multiple sclerosis: A systematic review and meta-analysis. Int J Environ Res Public Health. (2022) 19(21):14192. doi: 10.3390/ijerph192114192
276. Kajbafvala M, Ashnagar Z, Lucio A, Firoozeh F, Salehi R, Pashazadeh F, et al. Pelvic floor muscle training in multiple sclerosis patients with lower urinary tract dysfunction: A systematic review and meta-analysis. Mult Scler Relat Disord. (2022) 59:103559. doi: 10.1016/j.msard.2022.103559
277. Vecchio M, Chiaramonte R. and P DIB, Management of bladder dysfunction in multiple sclerosis: a systematic review and meta-analysis of studies regarding bladder rehabilitation. Eur J Phys Rehabil Med. (2022) 58:387–96. doi: 10.23736/s1973-9087.22.07217-3
278. Cortés-Pérez I, Osuna-Pérez MC, Montoro-Cárdenas D, Lomas-Vega R, Obrero-Gaitán E, Nieto-Escamez FA. Virtual reality-based therapy improves balance and reduces fear of falling in patients with multiple sclerosis. systematic Rev meta-analysis randomized Controlled trials. J Neuroeng Rehabil. (2023) 20:42. doi: 10.1186/s12984-023-01174-z
279. Learmonth YC, Ensari I, Motl RW. Physiotherapy and walking outcomes in adults with multiple sclerosis: Systematic review and meta-analysis. Phys Ther Rev. (2016) 21:160–72. doi: 10.1080/10833196.2016.1263415
280. Mura G, Carta MG, Sancassiani F, MaChado S, Prosperini L. Active exergames to improve cognitive functioning in neurological disabilities: a systematic review and meta-analysis. Eur J Phys Rehabil Med. (2018) 54:450–62. doi: 10.23736/s1973-9087.17.04680-9
281. Ensari I, Motl RW, Pilutti LA. Exercise training improves depressive symptoms in people with multiple sclerosis: results of a meta-analysis. J Psychosom Res. (2014) 76:465–71. doi: 10.1016/j.jpsychores.2014.03.014
282. Dalgas U, Stenager E, Sloth M, Stenager E. The effect of exercise on depressive symptoms in multiple sclerosis based on a meta-analysis and critical review of the literature. Eur J Neurol. (2015) 22:443–e34. doi: 10.1111/ene.12576
283. Torres-Costoso A, Martínez-Vizcaíno V, Reina-Gutiérrez S, Álvarez-Bueno C, Guzmán-Pavón MJ, Pozuelo-Carrascosa DP, et al. Effect of exercise on fatigue in multiple sclerosis: A network meta-analysis comparing different types of exercise. Arch Phys Med Rehabil. (2022) 103:970–987.e18. doi: 10.1016/j.apmr.2021.08.008
284. Razazian N, Kazeminia M, Moayedi H, Daneshkhah A, Shohaimi S, Mohammadi M, et al. The impact of physical exercise on the fatigue symptoms in patients with multiple sclerosis: a systematic review and meta-analysis. BMC Neurol. (2020) 20:93. doi: 10.1186/s12883-020-01654-y
285. Dauwan M, Begemann MJH, Slot MIE, Lee EHM, Scheltens P, Sommer IEC. Physical exercise improves quality of life, depressive symptoms, and cognition across chronic brain disorders: a transdiagnostic systematic review and meta-analysis of randomized controlled trials. J Neurol. (2021) 268:1222–46. doi: 10.1007/s00415-019-09493-9
286. Gascoyne C, Karahalios A, Demaneuf T, Marck C. Effect of exercise interventions on anxiety in people with multiple sclerosis: A systematic review and meta-analysis. Int J MS Care. (2020) 22:103–9. doi: 10.7224/1537-2073.2019-009R
287. Sánchez-Lastra MA, Martínez-Aldao D, Molina AJ, Ayán C. Pilates for people with multiple sclerosis: A systematic review and meta-analysis. Mult Scler Relat Disord. (2019) 28:199–212. doi: 10.1016/j.msard.2019.01.006
288. Cramer H, Lauche R, Azizi H, Dobos G, Langhorst J. Yoga for multiple sclerosis: a systematic review and meta-analysis. PloS One. (2014) 9:e112414. doi: 10.1371/journal.pone.0112414
289. Munari D, Fonte C, Varalta V, Battistuzzi E, Cassini S, Montagnoli AP, et al. Effects of robot-assisted gait training combined with virtual reality on motor and cognitive functions in patients with multiple sclerosis: A pilot, single-blind, randomized controlled trial. Restor Neurol Neurosci. (2020) 38:151–64. doi: 10.3233/rnn-190974
290. Cortés-Pérez I, Sánchez-Alcalá M, Nieto-Escámez FA, Castellote-Caballero Y, Obrero-Gaitán E, Osuna-Pérez MC. Virtual reality-based therapy improves fatigue, impact, and quality of life in patients with multiple sclerosis. A systematic rev meta-analysis. Sensors (Basel). (2021) 21(21):7389. doi: 10.3390/s21217389
291. Giovannelli M, Borriello G, Castri P, Prosperini L, Pozzilli C. Early physiotherapy after injection of botulinum toxin increases the beneficial effects on spasticity in patients with multiple sclerosis. Clin Rehabil. (2007) 21:331–7. doi: 10.1177/0269215507072772
292. Heine M, van de Port I, Rietberg MB, van Wegen EE, Kwakkel G. Exercise therapy for fatigue in multiple sclerosis. Cochrane Database Syst Rev. (2015) 2015(9):CD009956. doi: 10.1002/14651858.CD009956.pub2
293. Adamson BC, Ensari I, Motl RW. Effect of exercise on depressive symptoms in adults with neurologic disorders: a systematic review and meta-analysis. Arch Phys Med Rehabil. (2015) 96:1329–38. doi: 10.1016/j.apmr.2015.01.005
294. Reina-Gutiérrez S, Cavero-Redondo I, Martínez-Vizcaíno V, Núñez de Arenas-Arroyo S, López-Muñoz P, Álvarez-Bueno C, et al. The type of exercise most beneficial for quality of life in people with multiple sclerosis: A network meta-analysis. Ann Phys Rehabil Med. (2022) 65:101578. doi: 10.1016/j.rehab.2021.101578
295. Faíl LB, Marinho DA, Marques EA, Costa MJ, Santos CC, Marques MC, et al. Benefits of aquatic exercise in adults with and without chronic disease-A systematic review with meta-analysis. Scand J Med Sci Sports. (2022) 32:465–86. doi: 10.1111/sms.14112
296. Kinnett-Hopkins D, Adamson B, Rougeau K, Motl RW. People with MS are less physically active than healthy controls but as active as those with other chronic diseases: An updated meta-analysis. Mult Scler Relat Disord. (2017) 13:38–43. doi: 10.1016/j.msard.2017.01.016
297. Horton S, MacDonald DJ, Erickson K MS. exercise, and the potential for older adults. Eur Rev Aging Phys Activity. (2010) 7:49–57. doi: 10.1007/s11556-010-0062-9
298. Baird JF, Cutter GR, Motl RW. Do physical activity, cardiorespiratory fitness, and subcortical brain structures explain reduced walking performance in older adults with multiple sclerosis? Multiple Sclerosis Related Disord. (2022) 60:103702. doi: 10.1016/j.msard.2022.103702
299. Klaren RE, Sebastiao E, Chiu CY, Kinnett-Hopkins D, McAuley E, Motl RW. Levels and rates of physical activity in older adults with multiple sclerosis. Aging Dis. (2016) 7:278–84. doi: 10.14336/ad.2015.1025
300. Kim Y, Mehta T, Lai B, Motl RW. Immediate and sustained effects of interventions for changing physical activity in people with multiple sclerosis: meta-analysis of randomized controlled trials. Arch Phys Med Rehabil. (2020) 101:1414–36. doi: 10.1016/j.apmr.2020.03.017
301. Reina-Gutiérrez S, Meseguer-Henarejos AB, Torres-Costoso A, Álvarez-Bueno C, Cavero-Redondo I, Núñez de Arenas-Arroyo S, et al. Effect of different types of exercise on fitness in people with multiple sclerosis: A network meta-analysis. Scand J Med Sci Sports. (2023) 33:1916–28. doi: 10.1111/sms.14407
302. Motl RW, Baird JF. Cardiorespiratory fitness and moderate-to-vigorous physical activity in older adults with multiple sclerosis. Mult Scler J Exp Transl Clin. (2021) 7:20552173211057514. doi: 10.1177/20552173211057514
303. Hötting K, Röder B. Beneficial effects of physical exercise on neuroplasticity and cognition. Neurosci Biobehav Rev. (2013) 37:2243–57. doi: 10.1016/j.neubiorev.2013.04.005
304. Colcombe SJ, Kramer AF, Erickson KI, Scalf P, McAuley E, Cohen NJ, et al. Cardiovascular fitness, cortical plasticity, and aging. Proc Natl Acad Sci U.S.A. (2004) 101:3316–21. doi: 10.1073/pnas.0400266101
305. Hortobágyi T, Vetrovsky T, Balbim GM, Sorte Silva NCB, Manca A, Deriu F, et al. The impact of aerobic and resistance training intensity on markers of neuroplasticity in health and disease. Ageing Res Rev. (2022) 80:101698. doi: 10.1016/j.arr.2022.101698
306. Miller L, McFadyen A, Lord AC, Hunter R, Paul L, Rafferty D, et al. Functional electrical stimulation for foot drop in multiple sclerosis: A systematic review and meta-analysis of the effect on gait speed. Arch Phys Med Rehabil. (2017) 98:1435–52. doi: 10.1016/j.apmr.2016.12.007
307. Brandmeir NJ, Murray A, Cheyuo C, Ferari C, Rezai AR. Deep brain stimulation for multiple sclerosis tremor: A meta-analysis. Neuromodulation. (2020) 23:463–8. doi: 10.1111/ner.13063
308. Zali A, Khoshnood RJ, Motavaf M, Salimi A, Akhlaghdoust M, Safari S, et al. Deep brain stimulation for multiple sclerosis tremor: A systematic review and meta-analysis. Mult Scler Relat Disord. (2021) 56:103256. doi: 10.1016/j.msard.2021.103256
309. Hanna JA, Scullen T, Kahn L, Mathkour M, Gouveia EE, Garces J, et al. Comparison of elderly and young patient populations treated with deep brain stimulation for Parkinson's disease: long-term outcomes with up to 7 years of follow-up. J Neurosurg. (2018) 131:807–12. doi: 10.3171/2018.4.Jns171909
310. Hsu W-Y, Cheng C-H, Zanto TP, Gazzaley A, Bove RM. Effects of transcranial direct current stimulation on cognition, mood, pain, and fatigue in multiple sclerosis: A systematic review and meta-analysis. Front Neurol. (2021) 12:626113. doi: 10.3389/fneur.2021.626113
311. Rapisarda A, Ioannoni E, Izzo A, D'Ercole M, Montano N. Is there a place for spinal cord stimulation in the management of patients with multiple sclerosis? A systematic review of the literature. Minim Invasive Surg. (2021) 2021:9969010. doi: 10.1155/2021/9969010
312. Goodwin BJ, Mahmud R, TomThundyil S, Rivera-Colon G, Murray VW, O'Donnell K. The efficacy of spinal cord stimulators in the reduction of multiple sclerosis spasticity: A narrative systematic review. Brain Neurorehabil. (2023) 16:e19. doi: 10.12786/bn.2023.16.e19
313. Lefaucheur JP, Aleman A, Baeken C, Benninger DH, Brunelin J, Di Lazzaro V, et al. Evidence-based guidelines on the therapeutic use of repetitive transcranial magnetic stimulation (rTMS): An update (2014-2018). Clin Neurophysiol. (2020) 131:474–528. doi: 10.1016/j.clinph.2019.11.002
314. Chen X, Yin L, An Y, Yan H, Zhang T, Lu X, et al. Effects of repetitive transcranial magnetic stimulation in multiple sclerosis: A systematic review and meta-analysis. Mult Scler Relat Disord. (2022) 59:103564. doi: 10.1016/j.msard.2022.103564
315. Fiest KM, Walker JR, Bernstein CN, Graff LA, Zarychanski R, Abou-Setta AM, et al. Systematic review and meta-analysis of interventions for depression and anxiety in persons with multiple sclerosis. Multiple Sclerosis Related Disord. (2016) 5:12–26. doi: 10.1016/j.msard.2015.10.004
316. Kidd T, Carey N, Mold F, Westwood S, Miklaucich M, Konstantara E, et al. A systematic review of the effectiveness of self-management interventions in people with multiple sclerosis at improving depression, anxiety and quality of life. PloS One. (2017) 12:e0185931. doi: 10.1371/journal.pone.0185931
317. Ghielen I, Rutten S, Boeschoten RE, Houniet-de Gier M, van Wegen EEH, van den Heuvel OA, et al. The effects of cognitive behavioral and mindfulness-based therapies on psychological distress in patients with multiple sclerosis, Parkinson's disease and Huntington's disease: Two meta-analyses. J Psychosom Res. (2019) 122:43–51. doi: 10.1016/j.jpsychores.2019.05.001
318. Pöttgen J, van de Vis W, van Nunen A, Rose A, Engelbrecht J, Pirard M, et al. Psychobehavioral treatment options for sexual dysfunction in multiple sclerosis: A systematic review. Int J MS Care. (2020) 22:276–84. doi: 10.7224/1537-2073.2020-012
319. Proctor BJ, Moghaddam N, Vogt W, das Nair R. Telephone psychotherapy in multiple sclerosis: A systematic review and meta-analysis. Rehabil Psychol. (2018) 63:16–28. doi: 10.1037/rep0000182
320. Montañés-Masias B, Bort-Roig J, Pascual JC, Soler J, Briones-Buixassa L. Online psychological interventions to improve symptoms in multiple sclerosis: A systematic review: Online psychological interventions in Multiple Sclerosis. Acta Neurol Scand. (2022) 146:448–64. doi: 10.1111/ane.13709
321. Kiropoulos L. Early intervention for depressive symptoms in multiple sclerosis. Lancet Digital Health. (2023) 5:e637–8. doi: 10.1016/S2589-7500(23)00162-0
322. van den Akker LE, Beckerman H, Collette EH, Twisk JW, Bleijenberg G, Dekker J, et al. Cognitive behavioral therapy positively affects fatigue in patients with multiple sclerosis: Results of a randomized controlled trial. Multiple Sclerosis J. (2017) 23:1542–53. doi: 10.1177/1352458517709361
323. van den Akker LE, Beckerman H, Collette EH, Eijssen IC, Dekker J, de Groot V. Effectiveness of cognitive behavioral therapy for the treatment of fatigue in patients with multiple sclerosis: A systematic review and meta-analysis. J Psychosom Res. (2016) 90:33–42. doi: 10.1016/j.jpsychores.2016.09.002
324. Hind D, Cotter J, Thake A, Bradburn M, Cooper C, Isaac C, et al. Cognitive behavioural therapy for the treatment of depression in people with multiple sclerosis: a systematic review and meta-analysis. BMC Psychiatry. (2014) 14:5. doi: 10.1186/1471-244x-14-5
325. Moss-Morris R, Harrison AM, Safari R, Norton S, van der Linden ML, Picariello F, et al. Which behavioural and exercise interventions targeting fatigue show the most promise in multiple sclerosis? A systematic review with narrative synthesis and meta-analysis. Behav Res Ther. (2021) 137:103464. doi: 10.1016/j.brat.2019.103464
326. Taylor P, Dorstyn DS, Prior E. Stress management interventions for multiple sclerosis: A meta-analysis of randomized controlled trials. J Health Psychol. (2020) 25:266–79. doi: 10.1177/1359105319860185
327. Phyo AZZ, Demaneuf T, De Livera AM, Jelinek GA, Brown CR, Marck CH, et al. The efficacy of psychological interventions for managing fatigue in people with multiple sclerosis: A systematic review and meta-analysis. Front Neurol. (2018) 9:149. doi: 10.3389/fneur.2018.00149
328. Sesel AL, Sharpe L, Naismith SL. Efficacy of psychosocial interventions for people with multiple sclerosis: A meta-analysis of specific treatment effects. Psychother Psychosom. (2018) 87:105–11. doi: 10.1159/000486806
329. Klein OA, Drummond A, Mhizha-Murira JR, Mansford L, dasNair R. Effectiveness of cognitive rehabilitation for people with multiple sclerosis: a meta-synthesis of patient perspectives. Neuropsychol Rehabil. (2019) 29:491–512. doi: 10.1080/09602011.2017.1309323
330. das Nair R, Martin KJ, Lincoln NB. Memory rehabilitation for people with multiple sclerosis. Cochrane Database Syst Rev. (2016) 3:Cd008754. doi: 10.1002/14651858.CD008754.pub3
331. Dardiotis E, Nousia A, Siokas V, Tsouris Z, Andravizou A, Mentis AA, et al. Efficacy of computer-based cognitive training in neuropsychological performance of patients with multiple sclerosis: A systematic review and meta-analysis. Mult Scler Relat Disord. (2018) 20:58–66. doi: 10.1016/j.msard.2017.12.017
332. Taylor LA, Mhizha-Murira JR, Smith L, Potter KJ, Wong D, Evangelou N, et al. Memory rehabilitation for people with multiple sclerosis. Cochrane Database Syst Rev. (2021) 10:Cd008754. doi: 10.1002/14651858.CD008754.pub4
333. Mhizha-Murira JR, Drummond A, Klein OA, dasNair R. Reporting interventions in trials evaluating cognitive rehabilitation in people with multiple sclerosis: a systematic review. Clin Rehabil. (2018) 32:243–54. doi: 10.1177/0269215517722583
334. Muñoz San José A, Oreja-Guevara C, Cebolla Lorenzo S, Carrillo Notario L, Rodríguez Vega B, Bayón Pérez C. Psychotherapeutic and psychosocial interventions for managing stress in multiple sclerosis: The contribution of mindfulness-based interventions. Neurología (English Edition). (2016) 31:113–20. doi: 10.1016/j.nrleng.2015.07.003
335. Ulrichsen KM, Kaufmann T, Dørum ES, Kolskår KK, Richard G, Alnæs D, et al. Clinical utility of mindfulness training in the treatment of fatigue after stroke, traumatic brain injury and multiple sclerosis: A systematic literature review and meta-analysis. Front Psychol. (2016) 7:912. doi: 10.3389/fpsyg.2016.00912
336. Simpson R, Posa S, Langer L, Bruno T, Simpson S, Lawrence M, et al. A systematic review and meta-analysis exploring the efficacy of mindfulness-based interventions on quality of life in people with multiple sclerosis. J Neurol. (2023) 270:726–45. doi: 10.1007/s00415-022-11451-x
337. Zhang L, Lopes S, Lavelle T, Jones KO, Chen L, Jindal M, et al. Economic evaluations of mindfulness-based interventions: a systematic review. Mindfulness (N Y). (2022) 13:2359–78. doi: 10.1007/s12671-022-01960-1
338. Han A. Mindfulness- and acceptance-based interventions for symptom reduction in individuals with multiple sclerosis: A systematic review and meta-analysis. Arch Phys Med Rehabil. (2021) 102:2022–2031.e4. doi: 10.1016/j.apmr.2021.03.011
339. Simpson R, Simpson S, Ramparsad N, Lawrence M, Booth J, Mercer SW. Effects of Mindfulness-based interventions on physical symptoms in people with multiple sclerosis - a systematic review and meta-analysis. Mult Scler Relat Disord. (2020) 38:101493. doi: 10.1016/j.msard.2019.101493
340. Carletto S, Cavalera C, Sadowski I, Rovaris M, Borghi M, Khoury B, et al. Mindfulness-based interventions for the improvement of well-being in people with multiple sclerosis: A systematic review and meta-analysis. Psychosom Med. (2020) 82:600–13. doi: 10.1097/psy.0000000000000819
341. Simpson R, Simpson S, Ramparsad N, Lawrence M, Booth J, Mercer SW. Mindfulness-based interventions for mental well-being among people with multiple sclerosis: a systematic review and meta-analysis of randomised controlled trials. J Neurol Neurosurg Psychiatry. (2019) 90:1051–8. doi: 10.1136/jnnp-2018-320165
342. Guarnaccia JB, Njike VY, Dutton A, Ayettey RG, Treu JA, Comerford BP, et al. A pilot, randomized, placebo-controlled study of mindfulness meditation in treating insomnia in multiple sclerosis. BMC Neurol. (2023) 23:263. doi: 10.1186/s12883-023-03309-0
343. Sahar P, Mohsen M, Ali akbar P, Ali akbar F. The effectiveness of mindfulness-integrated cognitive-behavioral therapy on sleep quality, anxiety, and fatigue in patients with multiple sclerosis: A randomized clinical trial. J Sleep Sci. (2019) 4(1-2):1–8.
344. Pagnini F, Cavalera C, Rovaris M, Mendozzi L, Molinari E, Phillips D, et al. Longitudinal associations between mindfulness and well-being in people with multiple sclerosis. Int J Clin Health Psychol. (2019) 19:22–30. doi: 10.1016/j.ijchp.2018.11.003
345. Baker NA, Tickle-Degnen L. The effectiveness of physical, psychological, and functional interventions in treating clients with multiple sclerosis: a meta-analysis. Am J Occup Ther. (2001) 55:324–31. doi: 10.5014/ajot.55.3.324
346. Quinn É, Hynes SM. Occupational therapy interventions for multiple sclerosis: A scoping review. Scandinavian J Occup Ther. (2021) 28:399–414. doi: 10.1080/11038128.2020.1786160
347. De-Bernardi-Ojuel L, Torres-Collado L, García-de-la-Hera M. Occupational therapy interventions in adults with multiple sclerosis or amyotrophic lateral sclerosis: A scoping review. Int J Environ Res Public Health. (2021) 18:1432. doi: 10.3390/ijerph18041432
348. Kos D, Eijssen I, Bekkering GE, De Coninck L, O'Meara C, Koen M, et al. Occupational therapy in multiple sclerosis. Cochrane Database Syst Rev. (2023) 2023:CD015371. doi: 10.1002/14651858.CD015371
349. Aghaz A, Alidad A, Hemmati E, Jadidi H, Ghelichi L. Prevalence of dysphagia in multiple sclerosis and its related factors: Systematic review and meta-analysis. Iran J Neurol. (2018) 17:180–8.
350. Plotas P, Nanousi V, Kantanis A, Tsiamaki E, Papadopoulos A, Tsapara A, et al. Speech deficits in multiple sclerosis: a narrative review of the existing literature. Eur J Med Res. (2023) 28:252. doi: 10.1186/s40001-023-01230-3
351. Alali D, Ballard K, Bogaardt H. Treatment effects for dysphagia in adults with multiple sclerosis: A systematic review. Dysphagia. (2016) 31:610–8. doi: 10.1007/s00455-016-9738-2
352. Johansson K, Schalling E, Hartelius L. Self-reported changes in cognition, communication and swallowing in multiple sclerosis: data from the swedish multiple sclerosis registry and from a national survey. Folia Phoniatrica Logopaedica. (2020) 73:50–62. doi: 10.1159/000505063
353. Kaltsatou A, Flouris AD. Impact of pre-cooling therapy on the physical performance and functional capacity of multiple sclerosis patients: A systematic review. Multiple Sclerosis Related Disord. (2019) 27:419–23. doi: 10.1016/j.msard.2018.11.013
354. Stevens CJ, Singh G, Peterson B, Vargas NT, Périard JD. The effect of cooling garments to improve physical function in people with multiple sclerosis: A systematic review and meta-analysis. Mult Scler Relat Disord. (2023) 78:104912. doi: 10.1016/j.msard.2023.104912
355. Bilgin A, Kesik G, Ozdemir L. The effects of cooling therapies on fatigue, physical activity, and quality of life in multiple sclerosis: A meta-analysis. Rehabil Nurs. (2022) 47:228–36. doi: 10.1097/rnj.0000000000000388
356. Snetselaar LG, Cheek JJ, Fox SS, Healy HS, Schweizer ML, Bao W, et al. Efficacy of diet on fatigue and quality of life in multiple sclerosis: A systematic review and network meta-analysis of randomized trials. Neurology. (2023) 100:e357–66. doi: 10.1212/wnl.0000000000201371
357. McLaughlin L, Clarke L, Khalilidehkordi E, Butzkueven H, Taylor B, Broadley SA. Vitamin D for the treatment of multiple sclerosis: a meta-analysis. J Neurol. (2018) 265:2893–905. doi: 10.1007/s00415-018-9074-6
358. López-Muñoz P, Torres-Costoso AI, Fernández-Rodríguez R, Guzmán-Pavón MJ, de Arenas-Arroyo SN, Basco-López J, et al. Effect of vitamin D supplementation on fatigue in multiple sclerosis: A systematic review and meta-analysis. Nutrients. (2023) 15(13):2861. doi: 10.3390/nu15132861
359. Guerrero Aznar MD, Villanueva Guerrero MD, Cordero Ramos J, Eichau Madueño S, Morales Bravo M, López Ruiz R, et al. Efficacy of diet on fatigue, quality of life and disability status in multiple sclerosis patients: rapid review and meta-analysis of randomized controlled trials. BMC Neurol. (2022) 22:388. doi: 10.1186/s12883-022-02913-w
360. Jiang J, Chu C, Wu C, Wang C, Zhang C, Li T, et al. Efficacy of probiotics in multiple sclerosis: a systematic review of preclinical trials and meta-analysis of randomized controlled trials. Food Funct. (2021) 12:2354–77. doi: 10.1039/D0FO03203D
361. Atabilen B, Akdevelioğlu Y. Effects of different dietary interventions in multiple sclerosis: a systematic review of evidence from 2018 to 2022. Nutr Neurosci. (2023) 26(12):1279–91. doi: 10.1080/1028415x.2022.2146843
362. Abboud M, AlAnouti F, Georgaki E, Papandreou D. Effect of ketogenic diet on quality of life in adults with chronic disease: A systematic review of randomized controlled trials. Nutrients. (2021) 13(12):4463. doi: 10.3390/nu13124463
363. Bahr LS, Bock M, Liebscher D, Bellmann-Strobl J, Franz L, Prüß A, et al. Ketogenic diet and fasting diet as Nutritional Approaches in Multiple Sclerosis (NAMS): protocol of a randomized controlled study. Trials. (2020) 21:3. doi: 10.1186/s13063-019-3928-9
364. Koutsogeorgou E, Chiesi AM, Leonardi M. Social capital components and social support of persons with multiple sclerosis: a systematic review of the literature from 2000 to 2018. Disabil Rehabil. (2020) 42:3437–49. doi: 10.1080/09638288.2019.1597182
365. Gerritzen EV, Lee AR, McDermott O, Coulson N, Orrell M. Online peer support for people with multiple sclerosis: A narrative synthesis systematic review. Int J MS Care. (2022) 24:252–9. doi: 10.7224/1537-2073.2022-040
366. Kokas Z, Sandi D, Fricska-Nagy Z, Füvesi J, Biernacki T, Köves Á, et al. Do Hungarian multiple sclerosis care units fulfil international criteria? PloS One. (2022) 17:e0264328. doi: 10.1371/journal.pone.0264328
367. Cristiano E, Abad P, Becker J, Carrá A, Correale J, Flores J, et al. Multiple sclerosis care units in Latin America: Consensus recommendations about its objectives and functioning implementation. J Neurological Sci. (2021) 429:118072. doi: 10.1016/j.jns.2021.118072
368. Finlayson M. Concerns about the future among older adults with multiple sclerosis. Am J Occup Ther. (2004) 58:54–63. doi: 10.5014/ajot.58.1.54
369. Walter HAW, Seeber AA, Willems DL, de Visser M. The role of palliative care in chronic progressive neurological diseases-A survey amongst neurologists in the Netherlands. Front Neurol. (2018) 9:1157. doi: 10.3389/fneur.2018.01157
370. Golla H, Galushko M, Strupp J, Karbach U, Pfaff H, Ostgathe C, et al. Patients feeling severely affected by multiple sclerosis:Addressing death and dying. OMEGA - J Death Dying. (2016) 74:275–91. doi: 10.1177/0030222815598443
Keywords: multiple sclerosis, aging, management, disease-modifying treatments, symptomatic treatment
Citation: Fernández Ó, Sörensen PS, Comi G, Vermersch P, Hartung H-P, Leocani L, Berger T, Van Wijmeersch B and Oreja-Guevara C (2024) Managing multiple sclerosis in individuals aged 55 and above: a comprehensive review. Front. Immunol. 15:1379538. doi: 10.3389/fimmu.2024.1379538
Received: 31 January 2024; Accepted: 21 March 2024;
Published: 05 April 2024.
Edited by:
Robert Zivadinov, University at Buffalo, United StatesReviewed by:
Myla Goldman, Virginia Commonwealth University, United StatesBerenice Anabel Silva, Italian Hospital of Buenos Aires, Argentina
Copyright © 2024 Fernández, Sörensen, Comi, Vermersch, Hartung, Leocani, Berger, Van Wijmeersch and Oreja-Guevara. This is an open-access article distributed under the terms of the Creative Commons Attribution License (CC BY). The use, distribution or reproduction in other forums is permitted, provided the original author(s) and the copyright owner(s) are credited and that the original publication in this journal is cited, in accordance with accepted academic practice. No use, distribution or reproduction is permitted which does not comply with these terms.
*Correspondence: Óscar Fernández, b3NjYXIuZmVybmFuZGV6LnNzcGFAZ21haWwuY29t
†These authors have contributed equally to this work