- Department of Traditional Chinese Medicine, The Eighth Affiliated Hospital of Sun Yat-Sen University, Shenzhen, China
Atherosclerosis (AS) is recognized as a chronic inflammatory condition characterized by the accumulation of lipids and inflammatory cells within the damaged walls of arterial vessels. It is a significant independent risk factor for ischemic cardiovascular disease, ischemic stroke, and peripheral arterial disease. Despite the availability of current treatments such as statins, proprotein convertase subtilisin/kexin type 9 (PCSK9) inhibitors, and lifestyle modifications for prevention, AS remains a leading cause of morbidity and economic burden worldwide. Thus, there is a pressing need for the development of new supplementary and alternative therapies or medications. Huangqin (Scutellaria baicalensis Georgi. [SBG]), a traditional Chinese medicine, exerts a significant immunomodulatory effect in AS prevention and treatment, with baicalin being identified as one of the primary active ingredients of traditional Chinese medicine. Baicalin offers a broad spectrum of pharmacological activities, including the regulation of immune balance, antioxidant and anti-inflammatory effects, and improvement of lipid metabolism dysregulation. Consequently, it exerts beneficial effects in both AS onset and progression. This review provides an overview of the immunomodulatory properties and mechanisms by which baicalin aids in AS prevention and treatment, highlighting its potential as a clinical translational therapy.
1 Introduction
Atherosclerosis (AS) is a chronic and progressive disease characterized by inflammation within the arterial wall, accompanied by the accumulation of overactive smooth muscle cells and immune cells. This condition forms the pathophysiological basis for cardiovascular disease (CVD) and ischemic stroke, leading to significant mortality and disability worldwide (1–4). Although recent advancements in revascularization techniques and cholesterol-lowering medications (primarily statins and PCSK9 inhibitors) have notably improved AS management (5–7), the incidence of AS-induced acute events, such as acute myocardial infarction and acute ischemic stroke, continues to be alarmingly high over the past decade, contributing to substantial economic burden and mortality rates in both developed and developing countries (8). For instance, in China and many Asian countries, the number of newly diagnosed AS cases has been continuously increasing, and AS-associated cardiovascular disease or thrombosis remains a leading cause of death, which is reported to be as high as 40% (9–11). In light of these challenges, there is a consensus on the necessity for complementary and alternative treatment approaches for AS. Current guidelines strongly recommend pharmacological interventions, yet the effectiveness of traditional Chinese medicine (TCM) has garnered interest from the AS and CVD research community because of its “multiple channels and multiple targets” approach (12–14). Significantly, TCM has a higher acceptance in China and other Asian countries, owing to its strong cultural affinity. Therefore, developing TCM-based therapies for AS prevention and treatment represents a promising direction for novel strategic interventions.
Baicalin, a prominent flavonoid compound extracted from the root of Huangqin (Scutellaria baicalensis Georgi. [SBG]), exhibits a wide range of pharmacological effects such as anti-inflammatory, anti-apoptotic, antioxidant, and anticancer properties as well as improvement of smooth muscle cell function (15–19). In line with these properties, baicalin presents promising opportunities for AS prevention and treatment, as its therapeutic mechanisms and functional targets are likely linked to the pathophysiological processes of AS (20–22). This review draws upon the pathological basis of AS and provides a comprehensive analysis of the latest research on the anti-atherosclerotic effects of baicalin from an immunomodulatory perspective, aiming to offer insights into future directions for developing innovative treatment strategies for AS.
2 Immuno-interaction along with the processes in AS
Immune system plays a crucial role in the initiation and progression of AS. Here, we will shortly introduce the main involvements of innate and adaptive immunity during AS pathophysiological processes (Figure 1).
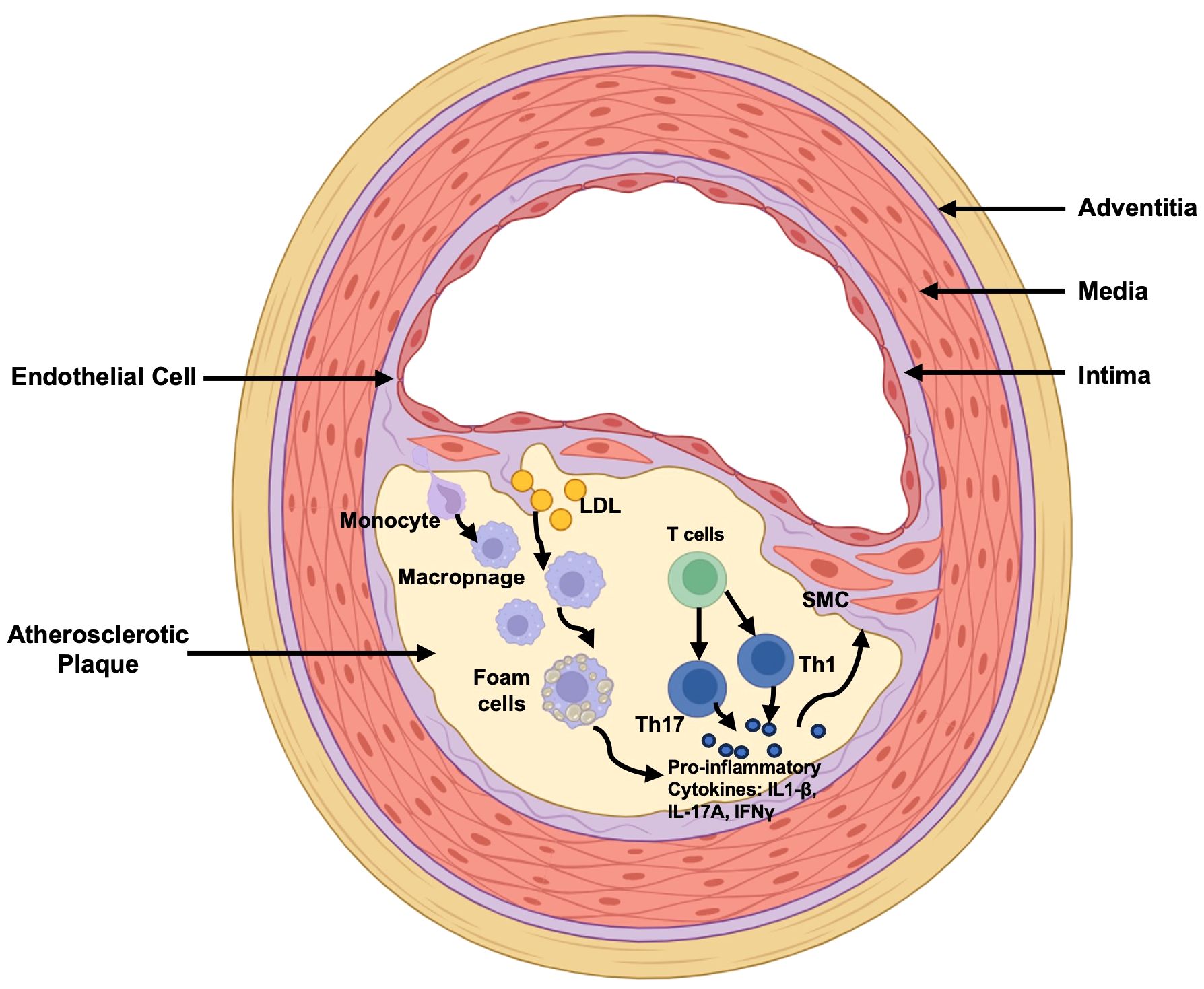
Figure 1 Main Immuno-alteration in Atherosclerosis. LDL retention is the marker of the initiation of atherosclerosis. Monocytes transmigrate into the subendothelial space and differentiate into macrophages, following binding to lipoproteins and forming foam cells which secret pro-inflammatory cytokines IL-1β for down-streaming smooth muscle cell activation for further pro-atherosclerotic processes. In the meantime, T cells are differentiated into Th1 and Th17 cells to secret IFNγ and IL-17A exerting pro-inflammatory effects. LDL, Low-Density Lipoprotein; Th17, T helper cells 17; Th1, T helper cells 1; IL-1β, interleukin (IL)-1β; IL-17A, interleukin (IL)-17A; IFNγ, Interferon-gamma; SMC, Smooth Muscle Cell.
2.1 Innate immunity alteration in AS
In the initial stages of AS, endothelial cell damage triggered by various inflammatory responses and ischemic-hypoxia events leads to the release of adhesion and chemotactic cytokines. These cytokines attract monocytes and other leukocytes to the site of inflammation, where they mature and transition into macrophages (23–25). The intracellular accumulation of cholesterol in macrophages activates surface scavenger receptors, facilitating the binding of macrophages to lipoprotein particles and leading to foam cell formation (26, 27). Additionally, lipid accumulation prompts endothelial cells to secrete inflammatory mediators and cytokines, further accelerating AS onset. Additionally, dendritic cells (DCs) accumulating in atherosclerotic plaques play a crucial role in the disease’s progression by presenting oxidized low-density lipoprotein (ox-LDL)-derived antigens, which activate both humoral and adaptive immune responses (28, 29).
2.2 Adaptive immunity alteration in AS
In the advanced stages of AS, adaptive immunity, especially cellular immunity, plays a role in T-cell infiltration (Figure 1) (30, 31). Although T cells are not as abundant as monocytes, which undergo differentiation into macrophages, they can penetrate the intima to modulate the functions of innate immune cells and smooth muscle cells. T helper cells 1 (Th1) exert a pro-inflammatory role in AS, for example, by secreting interferon-gamma (IFN-γ), which promotes monocyte infiltration, macrophage differentiation, and foam cell formation. T helper cells 2 (Th2), on the other hand, by secreting interleukin (IL)-4 to play a disease prompting role. T helper cells 17 (Th17), activated under pro-atherogenic conditions, have complex effects because of the various roles of IL-17A at different AS stages. However, undoubtedly, the proportion of Th17 cells increases in patients with acute coronary syndrome. Conversely, regulatory T (Treg) cells are present in atherosclerotic plaques at all stages of AS, although in a smaller proportion (32, 33). Animal studies have reported the exacerbating effects of depleting Tregs in AS, while reintroducing Treg cells mitigates and slows disease progression (34). Mechanistically, Treg cells secrete IL-10, playing a crucial role in preventing AS progression. Additionally, Treg cells secrete transforming growth factor-beta (TGF-β) to inhibit inflammation and the proliferation of smooth muscle cells. While the role of humoral immunity in AS development is little known to us since B cells are occasionally detected in atherosclerotic plaques. Studies on B-cell dynamics in atherosclerotic mice have yielded contradictory results: one indicates B cells mitigate AS, while another suggests B cells exacerbate it following B cell depletion (35–38). This discrepancy underscores the need for a comprehensive examination of B cell populations and their specific functions.
Collectively, these findings indicate that the pathological mechanisms of AS involve the participation of endothelial cells, monocyte-derived macrophages, smooth muscle cells, and immune cells. Consequently, understanding the role of immune interactions during AS progression could pave the way for innovative strategies targeting these immune responses. Furthermore, baicalin can modulate immune cells and their actions in AS progression, positioning it as a promising approach for AS prevention and treatment.
3 Immuno-modulatory features of baicalin in AS
Extensive research has confirmed baicalin’s multifaceted pharmacological benefits, including anti-apoptotic effects, enhancement of endothelial cell functions, antioxidant properties, and modulation of host immune homeostasis. These attributes contribute to its protective role in both the initiation and progression of AS (Figure 2). This review focuses on the immunomodulatory effects of baicalin in AS prevention and treatment, underscoring its potential as a significant therapeutic agent.
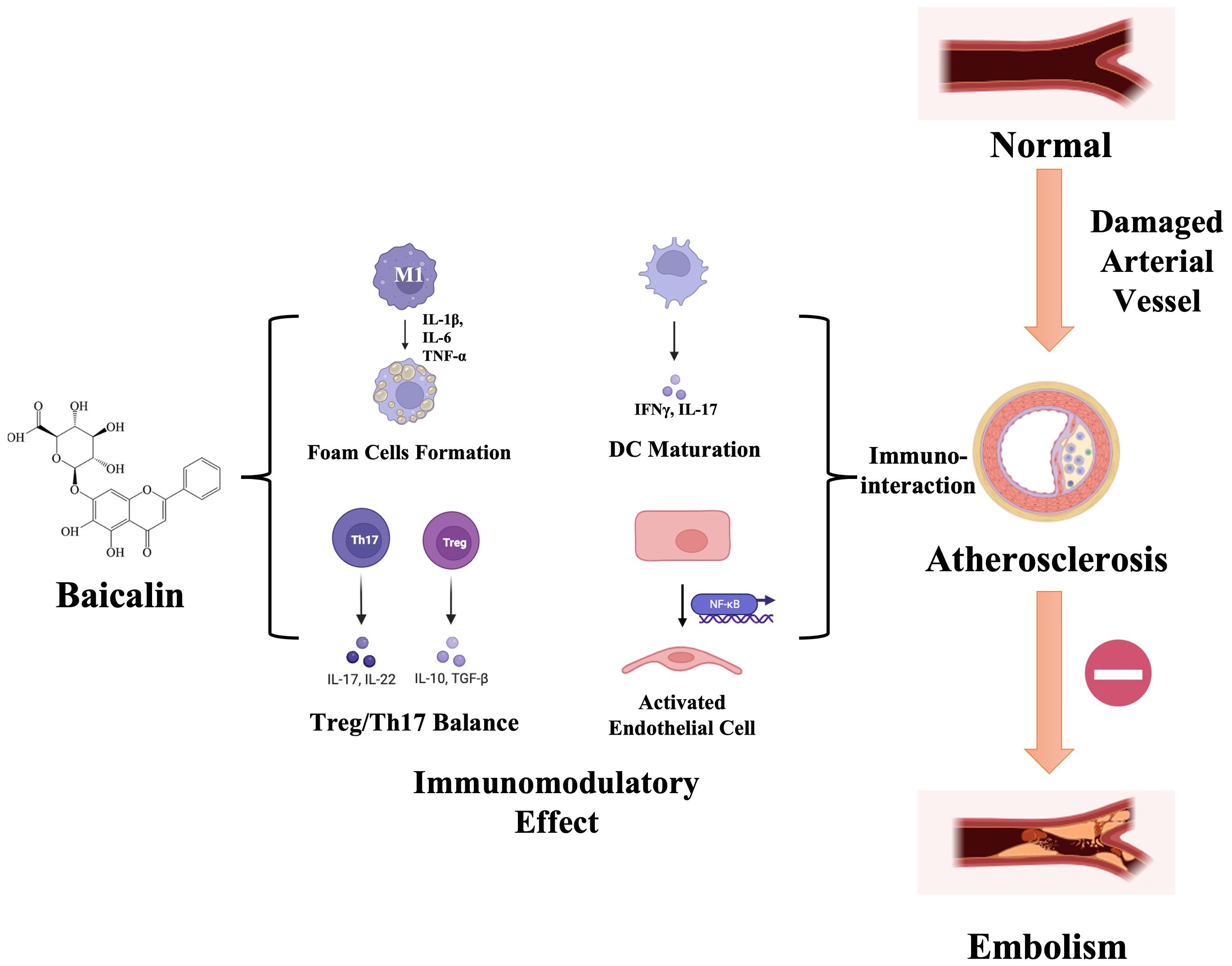
Figure 2 Schematic illustration of baicalin’s immunomodulatory effects during atherosclerosis progression. Upon damage manifestation within arterial vessels, diverse immune cell populations undergo activation and subsequent migration toward the intima, thereby instigating the development of an inflammatory microenvironment. Baicalin, with its immunomodulatory ability, specifically targets the differentiation of macrophage-foam cells, imbalance between Treg/Th17 cells, dendritic cell (DC) maturation, and modulation of inflammatory signaling pathways. This orchestrated modulation serves a dual purpose of impeding the progression of atherosclerosis toward embolism and mitigating the risk of acute ischemic events. IL-17, interleukin (IL)-17; IFNγ, Interferon-gamma; DC, Dendritic Cell; NF-κB, Nuclear Factor-kappa B; IL-1β, interleukin (IL)-1β; IL-6, interleukin (IL)-6; TNF-α, Tumor Necrosis Factor-α; IL-22, interleukin (IL)-22; IL-10, interleukin (IL)-10; TGF-β, Transforming Growth Factor Beta; Th17, T helper cells 17; Treg, regulatory T cells.
3.1 Effect of baicalin on innate immunity in AS
After migrating to the intima, monocytes undergo differentiation into macrophages. These macrophages then engulf large amounts of ox-LDL through scavenger receptors, leading to the formation of foam cells (26). These foam cells play a crucial role in the progression of AS lesions, making the transition of macrophages into foam cells a critical phase in AS development and progression. Consequently, strategies aimed at promoting macrophage apoptosis and preventing of macrophage foam cell formation have emerged as key focus areas in AS prevention and treatment. Baicalin primarily functions by inhibiting macrophage foam cell formation, regulating cholesterol homeostasis, and facilitating macrophage apoptosis. Baicalin triggers macrophage apoptosis by activating three signaling pathways, namely c-Jun N-terminal kinases (JNKs), p38 mitogen activated protein kinase (p38/MAPK), and Steroid Receptor RNA Activator (SRA) (39), in response to lipopolysaccharide (LPS) and acetylated low-density lipoprotein (Ac-LDL), thus preventing foam cell formation in diseased cells. In studies utilizing an LPS-sensitized mouse macrophage model, baicalin demonstrated potential in modulating Protein kinase A (PKA) activity in macrophages, which, in turn, may suppress NLR family pyrin domain containing 3 (NLRP3) inflammasome activation and cell pyroptosis (40). Additionally, Baicalin encourages M2 macrophage polarization and enhances phagocytosis by increasing Mer Tyrosine Kinase (MERTK) receptor expression (41).
Macrophages in AS primarily display two phenotypes: M1 and M2 types. In general, M1 macrophages, also known as classically activated macrophages, are typically involved in the initial pro-inflammatory response. In contrast, M2 macrophages contribute to anti-inflammatory processes and produce anti-inflammatory cytokines. In experiments investigating the effects of baicalin on RAW 264.7 macrophages cell line, it was found that Baicalin inhibited the expression of inflammatory factors IL-1β, IL-6, Tumor Necrosis Factor-α (TNF-α), Cyclooxygenase-2 (COX-2) in macrophage-derived foam cells. This upregulation promoted the expression of immunosuppressive factors TGF-β and IL-10, leading to the polarization of macrophages from M1 to M2 type, which inhibited the inflammatory response during the AS process. Further analysis, combined with relevant literature, revealed a potential association between this mechanism and Baicalin’s activation of the key protein peroxisome proliferator-activated receptor gamma (PPAR-γ) (42, 43). Previous studies have shown that Baicalin exhibits anti-inflammatory activity by inhibiting the activation of the Toll-like Receptor 4 (TLR4)/Myeloid Differentiation primary response 88 (MyD88)/Nuclear Factor-kappa B (NF-κB) cell signaling pathway (44). Recent research has further confirmed that Baicalin inhibits inducible Nitric Oxide Synthase (iNOS) gene functions, providing additional insight into Baicalin’s anti-inflammatory effects in AS (45). Baicalin can also inhibit macrophage proliferation, suppress excessive production of pro-inflammatory cytokines triggered by S100A8/A9, and reduce expression of the NADPH oxidase 2 (NOX2) gene, thereby inhibiting the inflammatory response (46). Despite the increasing understanding of the mechanisms by which Baicalin inhibits macrophage foam formation, further exploration is necessary to investigate its mechanisms for anti-foam cell formation and cholesterol metabolism from multiple perspectives. Additionally, compelling evidence shows that baicalin can reduce CD11c expression on DCs within atherosclerotic plaques, thus inhibiting DC maturation and the development of atherosclerotic lesions (47–49). Baicalin may also promote the transition of conventional DCs into plasmacytoid DCs and alter the expression of functional molecules by blocking the Signal Transducer and Activator of Transcription 5-Inhibitor of DNA binding 2 (STAT5-ID2) pathway, further demonstrating its protective effect against AS (50).
3.2 Effect of baicalin on cellular adaptive immunity in AS
Adaptive immunity plays a crucial role in both the onset and progression of AS (23). Activated T cells, which have encountered antigens before, are present within atherosclerotic plaques. Studies using immunodeficient apo E −/− animal models, which lack both T cells and B cells, have shown fewer atherosclerotic events compared with their immunocompetent counterparts (51, 52). This evidence underscores the pro-atherosclerotic influence of T cells and suggests that immunosuppressive strategies may be beneficial for treating AS. Treg cells, in particular, contribute to the protection against AS development by releasing anti-inflammatory cytokines such as IL-10 and TGF-β. Supporting this notion, baicalin can enhance Forkhead Box P3 (Foxp3) expression, thereby promoting the differentiation of Treg cells within atherosclerotic plaques, and exerting anti-arteriosclerotic effects (22, 53, 54). The underlying mechanism is believed to involve baicalin’s inhibition of the Mammalian Target of Rapamycin (mTOR) pathway, leading to downregulated expression of Ras homolog gene family, member A (RhoA), Rho-Associated Coiled-Coil-Containing Protein Kinase 1 (ROCK1), phosphorylated STAT4 (p-STAT4), and p-STAT3, while simultaneously increasing phosphorylated STAT5 expression. The latter directly interacts with the Foxp3 gene, enhancing Treg cell function. Additionally, baicalin can influence the gut microbiome in an ulcerative colitis animal model, balancing Treg/Th17 ratios (55) and exhibiting anti-inflammatory properties. This suggests potential for further research into baicalin’s effects on the microbiome in AS. Baicalin also inhibits Th17 cell differentiation by downregulating IL-6 and Retinoic Acid Receptor-Related Orphan Receptor Gamma (RORctγ) expression (56). Yet, its effect on the Th1 cell population, which secretes IFN-γ and contributes to AS progression, remains less explored, warranting further investigation. Overall, the evidence supports baicalin’s immunomodulatory benefits in AS, particularly regarding Treg/Th17 balance.
3.3 Effect of baicalin on humoral adaptive immunity in AS
Baicalin’s effect extends beyond adaptive immune cells, also influencing humoral immunity. However, the relationship between humoral immunity and AS presents conflicting evidence, necessitating further research (23). Baicalin is reported to significantly boost B cell proliferation and IL-12 production through its interaction with TLR4 (57). Additionally, by modulating signaling pathways such as NF-κB or Phosphoinositide 3-kinase/Protein Kinase B (PI3K/Akt), which are critical for B cell survival and homeostasis, Baicalin can potentially influence the lifespan and turnover of B cells which eventually affecting the quantity and quality of antibodies produced by B cells. The interplay between innate and adaptive immunity in the onset and progression of AS highlights the potential of baicalin as a therapeutic agent, primarily through its ability to regulate immune homeostasis.
3.4 Effect of baicalin on inflammation responses in AS
Inflammation is a critical driver at all stages of AS, with chemokines, pro-inflammatory cytokines, adhesion molecules, and inflammatory signaling pathways (5) playing pivotal roles. The NF-κB signaling pathway, in particular, promotes AS by increasing the production of pro-inflammatory cytokines and chemokines such as IL-6, IL-1, Intercellular Adhesion Molecule-1 (ICAM-1), Vascular Cell Adhesion Molecule-1 (VCAM-1), and TNF-α. Therefore, inhibiting NF-κB activation is effective in reducing AS incidence. Baicalin can suppress cytokine and chemokine production by inhibiting NF-κB activation in both in vitro and in vivo studies. Baicalin pretreatment to human umbilical vein endothelial cells confers protection from damage induced by vascular inflammation, which is similar to the early stages of AS, by suppressing NF-κB activity (58, 59). Baicalin can enhance SIRT expression in models of cardiovascular AS, acting as an inhibitor of NF-κB. This suppression of NF-κB leads to a reduction in the secretion and activation of downstream inflammatory factors (60). Additionally, baicalin influences the Wnt pathway, a crucial cellular signaling network involved in numerous biological processes, playing a significant anti-atherosclerotic role. Baicalin also promotes the activation of the Wingless-related integration site/Dickkopf-1 (Wnt/DKK1) pathway, potentially mitigating AS progression (61). Baicalin can also decrease the levels of inflammatory factors by promoting the phosphorylation of glycogen synthase kinase 3β (GSK3β), enhancing β-catenin expression and nuclear translocation, which is associated with the Wnt pathway (62). In summary, these findings underscore baicalin’s role in attenuating inflammatory responses, thereby decreasing the degree of AS progression.
4 Future directions of baicalin in AS
Baicalin has shown potential as a novel therapeutic agent for various diseases, but its clinical development and application (63) have been significantly hindered by its low bioavailability. To address this challenge, several innovative strategies, such as nanonization, solid dispersion, inclusion complexes, and micelles, have been designed and developed. These modifications have been proven to enhance the dissolution and solubility of baicalin. For instance, the formation of a baicalin-phospholipid complex through weak interactions under specific conditions can further improve the bioactivity of baicalin, offering enhanced anti-inflammatory effects with faster onset and prolonged duration (64). Furthermore, the advent of various baicalin-loaded liposome technologies, including folate-modified baicalin-loaded liposomes, baicalin-phospholipid nanoparticles, and baicalin-phospholipid prodrugs, has improved baicalin bioavailability in oral drug delivery systems (65). However, the exploration of baicalin’s drug delivery systems in the context of AS therapies remains limited, underscoring the need for further evaluation of suitable delivery vehicles to leverage its promising anti-atherosclerotic effects.
Recent evidence has highlighted the anti-inflammatory effects of mesenchymal stem cells (MSCs) in treating multiple diseases, including AS (66, 67). Intriguingly, baicalin enhances the proliferation and survival of MSCs by modulating the hedgehog signaling pathway (68), addressing the critical challenge of MSC apoptosis in clinical applications. Moreover, baicalin pretreatment can optimize the therapeutic effects of MSC-derived exosomes by inhibiting the nuclear factor erythroid 2–related factor 2 (Nrf2) pathway (69). These findings suggest that a combined approach of baicalin and MSC therapy could offer superior therapeutic benefits over using either treatment alone. This synergy underscores the potential of developing combination strategies that integrate baicalin and MSCs, warranting further investigation.
5 Concluding remarks
Baicalin has been recognized as a promising therapeutic agent for AS prevention and treatment owing to its ability to mitigate inflammation-induced damage, regulate lipid metabolism, promote cell survival, and modulate immune responses. This discussion has focused on the immunomodulatory effects of baicalin in combating AS. Despite extensive experimental evidence supporting baicalin’s immunomodulatory benefits in AS, the specific mechanisms of action, including its precise receptors and downstream signaling pathways, remain elusive. This gap in knowledge, coupled with limited clinical data, has delayed baicalin’s potential clinical applications. Moreover, the physical properties of baicalin still require optimization and technological advancements. Looking forward, initiating appropriate baicalin-based clinical trials is recommended to gather sufficient data, which could enrich our understanding of its mechanisms and enhance its therapeutic potential in AS prevention and treatment.
Author contributions
LW: Funding acquisition, Writing – original draft, Writing – review & editing. SH: Writing – original draft, Writing – review & editing. XL: Resources, Visualization, Writing – original draft. JZ: Investigation, Writing – review & editing. YH: Resources, Writing – review & editing. JH: Visualization, Writing – review & editing. DX: Supervision, Writing – review & editing.
Funding
The author(s) declare financial support was received for the research, authorship, and/or publication of this article. This work was supported by the National Natural Science Foundation of China (81803940); Scientific Research Project of Futian district of Guangdong Province of China (FTWS2022014); Sanming Project of Medicine in Shenzhen (SZZYSM202202012).
Conflict of interest
The authors declare that the research was conducted in the absence of any commercial or financial relationships that could be construed as a potential conflict of interest.
Publisher’s note
All claims expressed in this article are solely those of the authors and do not necessarily represent those of their affiliated organizations, or those of the publisher, the editors and the reviewers. Any product that may be evaluated in this article, or claim that may be made by its manufacturer, is not guaranteed or endorsed by the publisher.
References
1. Doran AC. Inflammation resolution: implications for atherosclerosis. Circ Res. (2022) 130:130–48. doi: 10.1161/CIRCRESAHA.121.319822
2. Hafiane A, Daskalopoulou SS. Targeting the residual cardiovascular risk by specific anti-inflammatory interventions as a therapeutic strategy in atherosclerosis. Pharmacol Res. (2022) 178:106157. doi: 10.1016/j.phrs.2022.106157
3. Kong P, Cui ZY, Huang XF, Zhang DD, Guo RJ, Han M. Inflammation and atherosclerosis: signaling pathways and therapeutic intervention. Signal Transduct Target Ther. (2022) 7:131. doi: 10.1038/s41392-022-00955-7
4. Libby P. The changing landscape of atherosclerosis. Nature.(2021) 592:524–33. doi: 10.1038/s41586-021-03392-8
5. Song B, Bie Y, Feng H, Xie B, Liu M, Zhao F. Inflammatory factors driving atherosclerotic plaque progression new insights. J Transl Int Med. (2022) 10:36–47. doi: 10.2478/jtim-2022-0012
6. Gupta M, Blumenthal C, Chatterjee S, Bandyopadhyay D, Jain V, Lavie CJ, et al. Novel emerging therapies in atherosclerosis targeting lipid metabolism. Expert Opin Investig Drugs. (2020) 29:611–22. doi: 10.1080/13543784.2020.1764937
7. Tokgözoğlu L, Libby P. The dawn of a new era of targeted lipid-lowering therapies. Eur Heart J. (2022) 43:3198–208. doi: 10.1093/eurheartj/ehab841
8. Abbafati C, Abbas KM, Abbasi M, Abbasifard M, Abbasi-Kangevari M, Abbastabar H, et al. Global burden of 369 diseases and injuries in 204 countries and territories, 1990-2019: a systematic analysis for the Global Burden of Disease Study 2019. Lancet. (2020) 396:1204–22. doi: 10.1016/S0140-6736(20)30925-9
9. Hu Q, Chen Z, Yuan X, Li S, Zhang R, Qin X. Common polymorphisms in the RGMa promoter are associated with cerebrovascular atherosclerosis burden in chinese han patients with acute ischemic cerebrovascular accident. Front Cardiovasc Med. (2021) 8:743868. doi: 10.3389/fcvm.2021.743868
10. Huang Y, Liao X, Song Z, Wang L, Xiao M, Zhong S. Evaluation of the influence of etiological factors on the economic burden of ischemic stroke in younger patients in China using the trial of org 10172 in acute stroke treatment (TOAST) classification. Med Sci Monit. (2019) 25:637–42. doi: 10.12659/MSM.913977
11. Xing L, Li R, Zhang S, Li D, Dong B, Zhou H, et al. High burden of carotid atherosclerosis in rural northeast China: A population-based study. Front Neurol. (2021) 12:597992. doi: 10.3389/fneur.2021.597992
12. Jing J, Zhu C, Gong R, Qi X, Zhang Y, Zhang Z. Research progress on the active ingredients of traditional Chinese medicine in the intervention of atherosclerosis: A promising natural immunotherapeutic adjuvant. BioMed Pharmacother.(2023) 159:114201. doi: 10.1016/j.biopha.2022.114201
13. Leung EL, Xu S. Traditional Chinese medicine in cardiovascular drug discovery. Pharmacol Res. (2020) 160:105168. doi: 10.1016/j.phrs.2020.105168
14. Liu H, Zhu L, Chen L, Li L. Therapeutic potential of traditional Chinese medicine in atherosclerosis: A review. Phytother Res. (2022) 36:4080–100. doi: 10.1002/ptr.7590
15. Hu Q, Zhang W, Wu Z, Tian X, Xiang J, Li L, et al. Baicalin and the liver-gut system: Pharmacological bases explaining its therapeutic effects. Pharmacol Res. (2021) 165:105444. doi: 10.1016/j.phrs.2021.105444
16. Jiang M, Li Z, Zhu G. Immunological regulatory effect of flavonoid baicalin on innate immune toll-like receptors. Pharmacol Res. (2020) 158:104890. doi: 10.1016/j.phrs.2020.104890
17. Singh S, Meena A, Luqman S. Baicalin mediated regulation of key signaling pathways in cancer. Pharmacol Res. (2021) 164:105387. doi: 10.1016/j.phrs.2020.105387
18. Wang L, Feng T, Su Z, Pi C, Wei Y, Zhao L. Latest research progress on anticancer effect of baicalin and its aglycone baicalein. Arch Pharm Res. (2022) 45:535–57. doi: 10.1007/s12272-022-01397-z
19. Wen Y, Wang Y, Zhao C, Zhao B, Wang J. The pharmacological efficacy of baicalin in inflammatory diseases. Int J Mol Sci. (2023) 24(11):9317. doi: 10.3390/ijms24119317
20. Baradaran Rahimi V, Askari VR, Hosseinzadeh H. Promising influences of Scutellaria baicalensis and its two active constituents, baicalin, and baicalein, against metabolic syndrome: A review. Phytother Res. (2021) 35:3558–74. doi: 10.1002/ptr.7046
21. Fang P, Yu M, Shi M, Bo P, Gu X, Zhang Z. Baicalin and its aglycone: a novel approach for treatment of metabolic disorders. Pharmacol Rep. (2020) 72:13–23. doi: 10.1007/s43440-019-00024-x
22. Liao P, Liu L, Wang B, Li W, Fang X, Guan S. Baicalin and geniposide attenuate atherosclerosis involving lipids regulation and immunoregulation in ApoE-/- mice. Eur J Pharmacol. (2014) 740:488–95. doi: 10.1016/j.ejphar.2014.06.039
23. Gisterå A, Hansson GK. The immunology of atherosclerosis. Nat Rev Nephrol.(2017) 13:368–80. doi: 10.1038/nrneph.2017.51
24. Koelwyn GJ, Corr EM, Erbay E, Moore KJ. Regulation of macrophage immunometabolism in atherosclerosis. Nat Immunol. (2018) 19:526–37. doi: 10.1038/s41590-018-0113-3
25. Vallejo J, Cochain C, Zernecke A, Ley K. Heterogeneity of immune cells in human atherosclerosis revealed by scRNA-Seq. Cardiovasc Res. (2021) 117:2537–43. doi: 10.1093/cvr/cvab260
26. Yuan Y, Li P, Ye J. Lipid homeostasis and the formation of macrophage-derived foam cells in atherosclerosis. Protein Cell. (2012) 3:173–81. doi: 10.1007/s13238-012-2025-6
27. Chistiakov DA, Melnichenko AA, Myasoedova VA, Grechko AV, Orekhov AN. Mechanisms of foam cell formation in atherosclerosis. J Mol Med (Berl).(2017) 95:1153–65. doi: 10.1007/s00109-017-1575-8
28. Zernecke A. Dendritic cells in atherosclerosis: evidence in mice and humans. Arterioscler Thromb Vasc Biol. (2015) 35:763–70. doi: 10.1161/ATVBAHA.114.303566
29. Manthey HD, Zernecke A. Dendritic cells in atherosclerosis: functions in immune regulation and beyond. Thromb Haemost.(2011) 106:772–8. doi: 10.1160/TH11-05-0296
30. Saigusa R, Winkels H, Ley K. T cell subsets and functions in atherosclerosis. Nat Rev Cardiol. (2020) 17:387–401. doi: 10.1038/s41569-020-0352-5
31. Tabas I, Lichtman AH. Monocyte-macrophages and T cells in atherosclerosis. Immunity.(2017) 47:621–34. doi: 10.1016/j.immuni.2017.09.008
32. Sharma M, Schlegel MP, Afonso MS, Brown EJ, Rahman K, Weinstock A, et al. Regulatory T cells license macrophage pro-resolving functions during atherosclerosis regression. Circ Res. (2020) 127:335–53. doi: 10.1161/CIRCRESAHA.119.316461
33. Wei N, Xu Y, Li Y, Shi J, Zhang X, You Y, et al. A bibliometric analysis of T cell and atherosclerosis. Front Immunol. (2022) 13:948314. doi: 10.3389/fimmu.2022.948314
34. Klingenberg R, Gerdes N, Badeau RM, Gisterå A, Strodthoff D, Ketelhuth DFJ, et al. Depletion of FOXP3+ regulatory T cells promotes hypercholesterolemia a nd atherosclerosis. J Clin Invest. (2013) 123(3):1323–34. doi: 10.1172/JCI63891
35. Xia N, Hasselwander S, Reifenberg G, Habermeier A, Closs EI, Mimmler M, et al. B lymphocyte-deficiency in mice causes vascular dysfunction by inducing neutrophilia. Biomedicines. (2021) 9(11):1686. doi: 10.3390/biomedicines9111686
36. Kyaw T, Tipping P, Bobik A, Toh BH. Opposing roles of B lymphocyte subsets in atherosclerosis. Autoimmunity.(2017) 50:52–6. doi: 10.1080/08916934.2017.1280669
37. Tay C, Liu YH, Hosseini H, Kanellakis P, Cao A, Peter K, et al. B-cell-specific depletion of tumour necrosis factor alpha inhibits atherosclerosis development and plaque vulnerability to rupture by reducing cell death and inflammation. Cardiovasc Res. (2016) 111:385–97. doi: 10.1093/cvr/cvw186
38. Ait-Oufella H, Herbin O, Bouaziz JD, Binder CJ, Uyttenhove C, Laurans L, et al. B cell depletion reduces the development of atherosclerosis in mice. J Exp Med. (2010) 207:1579–87. doi: 10.1084/jem.20100155
39. Paudel KR, Kim D-W. Microparticles-mediated vascular inflammation and its amelioration by antioxidant activity of baicalin. Antioxidants (Basel). (2020) 9(9):890. doi: 10.3390/antiox9090890
40. Li C-G, Yan L, Mai F-Y, Shi Z-J, Xu L-H, Jing Y-Y, et al. Baicalin inhibits NOD-like receptor family, pyrin containing domain 3 inflammasome activation in murine macrophages by augmenting protein ki nase A signaling. Front Immunol. (2017) 8:1409. doi: 10.3389/fimmu.2017.01409
41. Lai Y-S, Putra RBDS, Aui S-P, Chang K-T. M2C Polarization by Baicalin Enhances Efferocytosis via Upr egulation of MERTK Receptor. Am J Chin Med. (2018) 46(8):1899–914. doi: 10.1142/S0192415X18500957
42. He XW, Yu D, Li WL, Zheng Z, Lv CL, Li C, et al. Anti-atherosclerotic potential of baicalin mediated by promoting cholesterol efflux from macrophages via the PPARγ-LXRα-ABCA1/ABCG1 pathway. BioMed Pharmacother.(2016) 83:257–64. doi: 10.1016/j.biopha.2016.06.046
43. Xu B, Huang S, Chen Y, Wang Q, Luo S, Li Y, et al. Synergistic effect of combined treatment with baicalin and emodin on DSS-induced colitis in mouse. Phytother Res. (2021) 35:5708–19. doi: 10.1002/ptr.7230
44. Fu Y-J, Xu B, Huang S-W, Luo X, Deng X-L, Luo S, et al. Baicalin prevents LPS-induced activation of TLR4/NF-κB p65 pathway and inflammation in mice via inhibiting the expression of CD14. Acta Pharmacol Sin. (2021) 42(1):88–96. doi: 10.1038/s41401-020-0411-9
45. Cheng P, Wang T, Li W, Muhammad I, Wang H, Sun X, et al. Baicalin alleviates lipopolysaccharide-induced liver inflammation in C hicken by suppressing TLR4-mediated NF-κB pathway. Front Pharmacol. (2017) 8:547. doi: 10.3389/fphar.2017.00547
46. Yang J, Han F, Wu G, Dong Y, Su H, Xu J, et al. Dysregulated B7H4/JAK2/STAT3 pathway involves in hypertriglyceridemia acute pancreatitis and is attenuated by baicalin. Dig Dis Sci. (2023) 68(2):478–86. doi: 10.1007/s10620-022-07606-5
47. Liu L, Liao P, Wang B, Fang X, Li W, Guan S. Oral administration of baicalin and geniposide induces regression of atherosclerosis via inhibiting dendritic cells in ApoE-knockout mice. Int Immunopharmacol.(2014) 20:197–204. doi: 10.1016/j.intimp.2014.02.037
48. Kim ME, Kim HK, Park HY, Kim DH, Chung HY, Lee JS. Baicalin from Scutellaria baicalensis impairs Th1 polarization through inhibition of dendritic cell maturation. J Pharmacol Sci. (2013) 121:148–56. doi: 10.1254/jphs.12200FP
49. Zhang H, Jiao Q, Gong Q, Zhang Y, Zhang W, Hu Z. Baicalin induced dendritic cell apoptosis in vitro. Front Pharmacol. (2011) 2:15. doi: 10.3389/fphar.2011.00015
50. Lai N, Fu X, Hei G, Song W, Wei R, Zhu X, et al. The role of dendritic cell subsets in recurrent spontaneous abortion and the regulatory effect of baicalin on it. J Immunol Res. (2022) 2022:9693064. doi: 10.1155/2022/9693064
51. Zhou X, Robertson AK, Hjerpe C, Hansson GK. Adoptive transfer of CD4+ T cells reactive to modified low-density lipoprotein aggravates atherosclerosis. Arterioscler Thromb Vasc Biol. (2006) 26:864–70. doi: 10.1161/01.ATV.0000206122.61591.ff
52. Zhou X, Nicoletti A, Elhage R, Hansson GK. Transfer of CD4(+) T cells aggravates atherosclerosis in immunodeficient apolipoprotein E knockout mice. Circulation.(2000) 102:2919–22. doi: 10.1161/01.CIR.102.24.2919
53. Liu P, Xiao Z, Yan H, Lu X, Zhang X, Luo L, et al. Baicalin suppresses Th1 and Th17 responses and promotes Treg response to ameliorate sepsis-associated pancreatic injury via the RhoA-ROCK pathway. Int Immunopharmacol.(2020) 86:106685. doi: 10.1016/j.intimp.2020.106685
54. Yang J, Yang X, Yang J, Li M. Baicalin ameliorates lupus autoimmunity by inhibiting differentiation of Tfh cells and inducing expansion of Tfr cells. Cell Death Dis. (2019) 10:140. doi: 10.1038/s41419-019-1315-9
55. Zhu L, Xu L-Z, Zhao S, Shen Z-F, Shen H, Zhan L-B. Protective effect of baicalin on the regulation of Treg/Th17 balance, gut microbiota and short-chain fatty acids in rats with ulcerative col itis. Appl Microbiol Biotechnol. (2020) 104(12):5449–60. doi: 10.1007/s00253-020-10527-w
56. Yang J, Yang X, Chu Y, Li M. Identification of Baicalin as an immunoregulatory compound by controll ing T(H)17 cell differentiation. PloS One. (2011) 6(2):e17164. doi: 10.1371/journal.pone.0017164
57. Gong S-Q, Sun W, Wang M, Fu Y-Y. Role of TLR4 and TCR or BCR against baicalin-induced responses in T an d B cells. Int Immunopharmacol. (2011) 11(12):2176–80. doi: 10.1016/j.intimp.2011.09.015
58. Wang G, Liang J, Gao LR, Si ZP, Zhang XT, Liang G, et al. Baicalin administration attenuates hyperglycemia-induced malformation of cardiovascular system. Cell Death Dis. (2018) 9:234. doi: 10.1038/s41419-018-0318-2
59. Ku SK, Bae JS. Baicalin, baicalein and wogonin inhibits high glucose-induced vascular inflammation in vitro and in vivo. BMB Rep. (2015) 48:519–24. doi: 10.5483/BMBRep.2015.48.9.017
60. Cai Y, Jiang S, Huang C, Shen A, Zhang X, Yang W, et al. Baicalin inhibits pressure overload-induced cardiac hypertrophy by reg ulating the SIRT3-dependent signaling pathway. Phytomedicine. (2023) 114:154747. doi: 10.1016/j.phymed.2023.154747
61. Wang B, Liao P-P, Liu L-H, Fang X, Li W, Guan S-M. Baicalin and geniposide inhibit the development of atherosclerosis by increasing Wnt1 and inhibiting dickkopf-related protein-1 expression. J Geriatr Cardiol. (2016) 13(10):846–54. doi: 10.11909/j.issn.1671-5411.2016.10.013
62. Xiao Y, Guan T, Yang X, Xu J, Zhang J, Qi Q, et al. Baicalin facilitates remyelination and suppresses neuroinflammation in rats with chronic cerebral hypoperfusion by activating Wnt/β-catenin and inhibiting NF-κB signaling. Behav Brain Res. (2023) 442:114301. doi: 10.1016/j.bbr.2023.114301
63. Huang T, Liu Y, Zhang C. Pharmacokinetics and bioavailability enhancement of baicalin: A review. Eur J Drug Metab Pharmacokinet. (2019) 44(2):159–68. doi: 10.1007/s13318-018-0509-3
64. Wu H, Long X, Yuan F, Chen L, Pan S, Liu Y, et al. Combined use of phospholipid complexes and self-emulsifying microemuls ions for improving the oral absorption of a BCS class IV compound, bai calin. Acta Pharm Sin B. (2014) 4(3):217–26. doi: 10.1016/j.apsb.2014.03.002
65. Wei Y, Guo J, Zheng X, Wu J, Zhou Y, Yu Y, et al. Preparation, pharmacokinetics and biodistribution of baicalin-loaded l iposomes. Int J Nanomedicine. (2014) 9:3623–30. doi: 10.2147/IJN.S66312
66. Lin Y, Zhu W, Chen X. The involving progress of MSCs based therapy in atherosclerosis. Stem Cell Res Ther. (2020) 11:216. doi: 10.1186/s13287-020-01728-1
67. Li F, Guo X, Chen SY. Function and therapeutic potential of mesenchymal stem cells in atherosclerosis. Front Cardiovasc Med. (2017) 4:32. doi: 10.3389/fcvm.2017.00032
68. Jia B, Jiang Y, Yao Y, Xu Y, Wang Y, Li T. Baicalin attenuates dexamethasone-induced apoptosis of bone marrow mes enchymal stem cells by activating the hedgehog signaling pathway. Chin Med J (Engl). (2023) 136(15):1839–47. doi: 10.1097/CM9.0000000000002113
Keywords: baicalin, atherosclerosis, immuno-modulatory, traditional Chinese medicine, anti-inflammatory
Citation: Wang L, Huang S, Liang X, Zhou J, Han Y, He J and Xu D (2024) Immuno-modulatory role of baicalin in atherosclerosis prevention and treatment: current scenario and future directions. Front. Immunol. 15:1377470. doi: 10.3389/fimmu.2024.1377470
Received: 27 January 2024; Accepted: 27 March 2024;
Published: 18 April 2024.
Edited by:
Jiao Xiao, Shenyang Pharmaceutical University, ChinaReviewed by:
Samaneh Abdolmohammadi-Vahid, Shahid Beheshti University of Medical Sciences, IranCopyright © 2024 Wang, Huang, Liang, Zhou, Han, He and Xu. This is an open-access article distributed under the terms of the Creative Commons Attribution License (CC BY). The use, distribution or reproduction in other forums is permitted, provided the original author(s) and the copyright owner(s) are credited and that the original publication in this journal is cited, in accordance with accepted academic practice. No use, distribution or reproduction is permitted which does not comply with these terms.
*Correspondence: Jiangshan He, jiangshanhe.jsh@163.com; Danping Xu, xudp3@mail.sysu.edu.cn
†These authors have contributed equally to this work and share first authorship