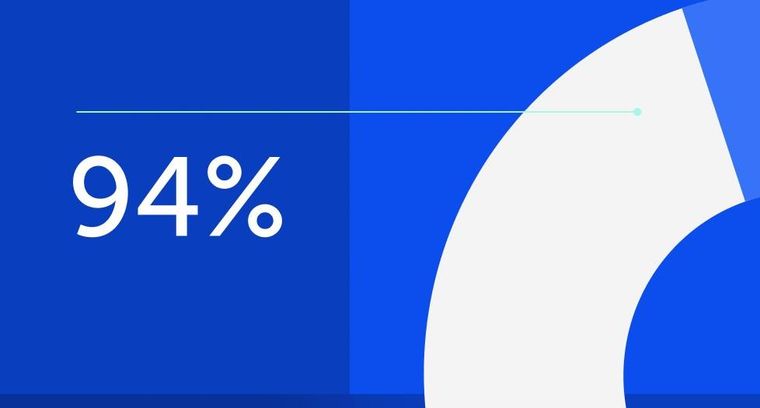
94% of researchers rate our articles as excellent or good
Learn more about the work of our research integrity team to safeguard the quality of each article we publish.
Find out more
MINI REVIEW article
Front. Immunol., 15 February 2024
Sec. T Cell Biology
Volume 15 - 2024 | https://doi.org/10.3389/fimmu.2024.1365843
This article is part of the Research TopicCommunity Series in the Role of CD1- and MR1-restricted T cells in Immunity and Disease, volume IIView all 18 articles
Natural killer T (NKT) cell are members of the innate-like T lymphocytes and recognizes lipid antigens presented by CD1d-expressing cells. Obesity-associated inflammation in adipose tissue (AT) leads to metabolic dysfunction, including insulin resistance. When cellular communication is properly regulated among AT-residing immune cells and adipocytes during inflammation, a favorable balance of Th1 and Th2 immune responses is achieved. NKT cells play crucial roles in AT inflammation, influencing the development of diet-induced obesity and insulin resistance. NKT cells interact with CD1d-expressing cells in AT, such as adipocytes, macrophages, and dendritic cells, shaping pro-inflammatory or anti-inflammatory microenvironments with distinct characteristics depending on the antigen-presenting cells. Additionally, CD1d may be involved in the inflammatory process independently of NKT cells. In this mini-review, we provide a brief overview of the current understanding of the interaction between immune cells, focusing on NKT cells and CD1d signaling, which control AT inflammation both in the presence and absence of NKT cells. We aim to enhance our understanding of the mechanisms of obesity-associated diseases.
Inflammation/immune response control obesity in the adipose tissue (AT) of the body (1, 2). Obesity is a low-grade chronic inflammatory disease that contributes to metabolic dysfunction and insulin resistance (3, 4). Initially, hypertrophied adipocytes secrete inflammatory cytokines and chemokines, thereby recruiting immune cells that promote AT inflammation, including macrophages (Mϕs), T cells, B cells, and neutrophils (5–11). While various immune cells contribute to AT homeostasis and inflammation, natural killer T (NKT) cells play a crucial role in developing obesity and insulin resistance (12). NKT cells are a unique subset of T cells that recognize lipid antigens on MHC class I-like CD1d molecules (13–15). NKT cells are further classified into two subsets depending on their T cell receptor (TCR) expression: type I NKT (invariant NKT; iNKT) cells that harbor an invariant TCR α-chain (Vα14-Jα18 in mice and Vα24-Jα18 in humans) and type II NKT (variant NKT; vNKT) cells that express diverse TCRs. iNKT cells recognize a prototypical ligand, α-galactosylceramide (α-GalCer), whereas vNKT cells recognize various lipid antigens, including sulfatide (16–18). NKT cells secrete various cytokines/chemokines/cytocidal molecules, activating and recruiting immune cells to eliminate target cells (19). Furthermore, iNKT cells can be categorized based on the expression of transcription factors such as T-bet for NKT1 cells, GATA3 and PLZF for NKT2 cells, and RORγt for NKT17 cells (20, 21), which may contribute to the development of inflammatory diseases, including obesity (22–24).
The contribution of CD1d itself to cellular signaling, aside from its role in ligand presentation to NKT cells in inflammatory and immune responses, should be considered. Recent reports have demonstrated that CD1d can modulate critical responses through its cytoplasmic portion (25–30). In this review, we discuss how NKT cells contribute to inflammation through interaction with CD1d-expressing cells in AT and how the intrinsic function of CD1d itself may influence the response in an NKT cell-independent manner.
Numerous studies have revealed that AT-resident and infiltrating immune cells control obesity-associated AT inflammation in a DIO model by feeding mice a high-fat diet (HFD; 60% fat kcal). T helper type 1 (Th1)-immune responses formed by M1-Mϕs, CD8+ T cells, NK cells, and ILC1s exacerbate AT inflammation and insulin resistance (5, 7, 31–34). In contrast, Th2-immune responses induced by M2-Mϕs, regulatory T cells, eosinophils, and ILC2s improve insulin sensitivity (8, 35, 36). Additionally, the ILC2s-eosinophils-M2-Mϕs circuit plays a central role in differentiating beige fat from white fat (beiging), where beige fat expresses thermogenic genes to defend against cold and obesity (37–39). Thus, in the steady state, a lean Th2-immune environment is maintained by the AT-resident cells.
NKT cells appear to play dual roles in the development of obesity. Reports have shown that both iNKT and vNKT cells promote AT inflammation and insulin resistance (40–43), whereas other studies have indicated that iNKT cells play either protective or neutral roles against obesity in DIO experiments using CD1d KO and Jα18 KO mice (44–49) (Table 1). Jα18 KO mice exhibit a significant reduction in T-cell receptor (TCR) diversity (52), thus mucosal-associated invariant T (MAIT) cells which utilize Jα33 are lost (53). Considering that MAIT cells also impact the development of obesity (54–57), Traj18 KO mice (distinct from Jα18 KO mice here) generated by depleting only the Traj18 locus, essentially represent iNKT cell KO mice (42). AT-resident iNKT cells express E4BP4 but not PLZF, reflecting their anti-inflammatory phenotype, and IL-10-producing NKT cells (NKT10) are enriched in subcutaneous white AT (58, 59). Moreover, when the F108Y substitution was artificially induced in TCRβ (Vβ8.2), thereby reducing the interaction of mutated iTCR with CD1d by a partial disruption of the hydrophobic patch formation with TCR α and β chain pairing, it altered iNKT cell development to an E4BP4-expressing, AT-resident-like phenotype (60). Thus, AT-resident iNKT cells may differentiate and exhibit unique functions compared to iNKT cells located in other tissues (61).
NKT cell-deficient mice do not show any pathogenic phenotype in comparison with WT mice, either in an obese or lean state under normal dietary conditions. However, when they are fed an HFD, NKT cells function as either pro-obese or pro-lean (40, 41, 44, 45, 49). This implies that NKT cells in HFD-fed mice are activated by unknown endogenous ligands presented by CD1d+ APCs, including flora-derived ligands (62–64), presumably even during obesity. Schipper et al. showed that CD1d KO mice exhibited adipocyte dysfunction and insulin resistance even under steady-state conditions (46), suggesting that NKT cells function in both obesity and a lean state. Alternatively, the dysfunction may be primarily attributed to the deficiency of CD1d molecules in adipocytes, as discussed later. Moreover, the phenotype of CD1d KO and Jα18 KO mice fed an HFD varied among laboratories (12), ranging from lean to obese, including a neutral state, compared to WT mice. Although contradictory results have been reported, presumably due to differences in the lipid composition of the HFD and intestinal microbiota for different murine strains, the critical reason for the discrepancy remains elusive. Selvanantham et al. have reported that CD1d KO mice exhibit altered gut microbiome profiles, characterized by increase in segmented filamentous bacteria and decrease of Akkermansia, which exacerbate intestinal inflammation (65). Bacteroides fragilis produces the glycosphingolipid α-GalCerBf, which is structurally related to the prototypic ligand α-GalCer or KRN7000 (62), exhibiting regulatory activity by iNKT cells. This indicates that some bacteria in the intestine are involved in the functional modulation of NKT cells, however, whether it indeed affects the function of NKT cells during obesity has not been examined. Meanwhile, the composition and fatty acid concentration in sera, altered and elevated in obese subjects, are determined based on endogenous synthesis rates and dietary fat characteristics (66, 67). Although the ligand for NKT cells in adipocytes remains elusive, it appears possible that dietary-derived lipid components are involved in their ligand production. At least, alteration in serum fatty acid composition affects Mϕs, via TLR4 and other receptors, which may modulate NKT cell activation in an indirect fashion (24). Additionally, single-cell analysis have unveiled distinct subsets of AT-resident iNKT cells, including AT-iNKT10 (induction of Tregs and M2-Mϕs), AT-iNKT1 (killing pathogenic Mϕs via NK cells and clearance of dead adipocytes via Mϕs) and AT-iNKT17 (via induction of adipose stem cell proliferation by amphiregulin) (68, 69). Of significance is the inquiry into how AT-iNKT cells are generated and activated, representing a likely major contribution of the adipose tissue microenvironment. Additionally, from the perspective of the CD1d molecule itself, the activation of the CD1d is differentially regulated by endogenous/exogenous lipid ligands which are biosynthesized during obesity, or through the modulation of the CD1d signaling cascade, as indicated by reports suggesting that endogenous ligands switch on CD1d activation (28, 29). Hence, further investigation is necessary to reconcile this contradiction and establish a therapeutic strategy for obesity-associated diseases.
NKT cells are activated in AT by interacting with CD1d-expressing cells, including Mϕs, dendritic cells, adipocytes, and leukocytes, such as eosinophils. AT functions as an endocrine organ, as adipocytes secrete adipokines and store triglycerides for energy (70). Several studies have demonstrated that adipocytes activate both T and NKT cells through antigen presentation (50, 51, 71–73). CD1d expressed on the surface of adipocytes can induce iNKT cell activation depending on the expression of microsomal triglyceride transfer protein (MTP) and CCAAT/enhancer-binding protein (C/EBP)-β and -δ, even in the absence of exogenous ligands, suggesting that adipocytes express endogenous ligands recognized by NKT cells (71). The inhibition of UDP-glucose ceramide glucosyltransferase (UGCG), the first rate-limiting step in the glucosylceramide biosynthesis pathway, resulted in decreased iNKT cell activity (74). This finding suggests that the amount of glucosylceramide influences the biosynthetic pathway of lipid self-antigen presentation by adipocytes in iNKT cells. Additionally, during their interaction with adipocytes within a lipid-rich microenvironment, the cytokine output of NKT cells skews towards IFN-γ rather than IL-4 (75), indicating that lipid conditions, such as the balance of fatty acids, are important factors for NKT cell activation by adipocytes. Furthermore, the LDL-α-GalCer complex elicits a stronger iNKT cell response than α-GalCer alone; while, LDL receptor mutation impairs their activation. Thus, lipoproteins can form complexes with lipid antigens to facilitate LDL receptor-mediated uptake by APCs, leading to enhanced iNKT cell activation (76).
Our group has demonstrated the role of NKT cell-adipocyte interactions in vivo using adipocyte-specific Cd1d1-deficient (AdipoqCre-Cd1d1f/f) mice during obesity. In comparison to control mice fed a HFD, AdipoqCre-Cd1d1f/f mice exhibited suppressed body weight gain and insulin resistance, suggesting that the interaction between iNKT cells and adipocytes plays a pro-inflammatory role in AT. iNKT cells activated by adipocytes secrete IFN-γ, which enhances the expression of CD1d and CCL2 in adipocytes, thereby promoting a positive loop for AT inflammation (50).
In contrast, other reports have shown that a similar conditional knockout mouse, adipocyte-specific CD1d-KO (CD1dADKO), exhibited reduced IL-4 expression in adipose iNKT cells, resulting in aggravated AT inflammation and insulin resistance in HFD-fed mice. This implies that iNKT cells stimulated by CD1d-expressing adipocytes induce anti-inflammatory responses in the AT (51). Furthermore, the results observed in CD1dADKO mice recapitulated those in whole-body CD1d KO mice, demonstrating the opposite conclusion.
Recently, Xiao et al. re-expressed CD1d by transferring the Cd1d gene into the visceral AT (VAT) of CD1d KO mice using an adeno-associated viral (AAV) vector to investigate the interactions between adipocytes and immune cells. The mice with the Cd1d gene transferred showed massive expansion of CD8+ T cells in the VAT, leading to the dysregulation of adipocyte functions through the activation of the NLRP3 inflammasome (77). Although the transduced mice exhibited a 25% reduction in VAT weight, there was no significant change in other parts of the AT. Since the mice were NKT cell-deficient and CD1d-gene in AAV appeared not to be expressed in either CD4+CD8+ thymocytes or thymic epithelial cells, CD1d expressed on adipocytes served as a neoantigen introduced like an allograft, inducing responses by CD8+ T cells along with an increase in CD4+ and CD4-8- T cells. It would be of an interest whether iNKT cells or vNKT cells respond in CD1d-AAV transduced WT or Jα18 KO mice fed on HFD.
Mϕs are abundantly present in AT and play a crucial role in maintaining AT homeostasis. Mϕs are phenotypically and functionally classified into two types: pro-inflammatory M1 and anti-inflammatory M2, based on gene expression and markers. Adipose iNKT cells have been shown to interact with Mϕ, which act as antigen-presenting cells that polarize Mϕs towards M2 under the influence of IL-10 (44). To examine the role of the interaction between NKT cells and Mϕs during obesity, we utilized myeloid-specific Cd1d1-deficient (LysMCre-Cd1d1f/f) mice. If the interaction is beneficial, its disruption between iNKT cells and Mϕ should result in AT inflammation and obesity. However, LysMCre-Cd1d1f/f mice gained body and VAT weights similar to those of control mice and exhibited enhanced insulin resistance, which was associated with M1-Mϕ and a bias toward NKT1/Th1 in the AT. The insulin resistance result aligns with the findings of a previous study (44), while the weight gain was not significantly greater than that of the control mice. These results may be primarily interpreted as defective M2 polarization due to the lack of interaction between iNKT cells and Mϕ via CD1d. Additionally, CD1d-deficient Mϕs expressed more IL-12p40 than control Mϕs in response to palmitic acid, and the inflammatory phenotype was enhanced by direct contact with iNKT cells (24).
Alternatively, these results may indicate that CD1d-deficient Mϕs themselves exhibit an inflammatory phenotype upon TLR stimulation (palmitic acid as a TLR4 ligand), and the interaction with iNKT cells strengthens the TLR responses, however, the mechanism of interaction remains elusive. A previous report by Zhang et al. suggested that the iNKT cell-Mϕ interactions are important in controlling AT inflammation during obesity. They employed LysMCre-Cd1d1f/f mice as M2-specific CD1d-deleted mice, induced inflammation and insulin resistance during obesity due to the inhibition of M2-Mϕ and iNKT cell interactions (78). M2-Mϕ express more CD1d than M1-Mϕ in murine VAT, facilitating the induction of IL-4 and IL-13 by interacting with iNKT cells (44, 78).
In humans with diabetes, CD11c+ VAT Mϕs laden with several lipids express more CD1d than CD206+ VAT Mϕs, although functional profiling such as M1 or M2 classification is not clear based on surface markers, thus implying that the signatures of human ATMϕ subtypes are unique (79, 80). In the presentation of antigens via CD1d, Th1-biasing glycolipids such as α-GC C26:0 (both Th1+Th2) and α-C-GC C26:0, which stimulate much lower IL-4 and relatively higher and more prolonged IFN-γ secretion, have consistently been observed to form complexes with CD1d that preferentially localize to cholesterol-rich membrane rafts. Contrarily, CD1d with Th2-biasing glycolipids such as α-GC C20:2, α-GC C20:1, α-GC C18:3, and α-GC C10:0, which stimulate strong IL-4 secretion relative to IFN-γ, are more evenly distributed throughout the cell membrane. Neutralization of lysosomal pH enhanced the localization of the CD1d-Th2-biasing glycolipid complex to lipid rafts on the plasma membrane, although the presentation of Th1-biasing glycolipids was drastically reduced. These results suggest that lysosomal pH controls the stability and localization of CD1d-glycolipid complexes in lipid rafts by modulating the cytokine output of iNKT cells (81, 82). Lysosomes accumulate in lipid-laden ATMϕs and appear to be important for lipid metabolism (83). However, it is unclear whether lysosomal activation and pH are involved in M1/M2 Mϕ polarization and obesity development.
In addition to Mϕs, DCs contribute to the control of AT homeostasis and insulin sensitivity. Flt3 KO mice lacking DCs or CD11c+ cell-depleted mice treated with diphtheria toxin failed to induce obesity, insulin resistance, and liver steatosis compared to that in control mice during HFD feeding (84, 85). In contrast, Batf3 KO mice lacking cDC1 showed increased body weight and adiposity during aging, partially mediated by the cDC-iNKT cell axis. However, these data did not show the actual interaction between cDC and iNKT cells (86). In our model, inhibition of the interaction between iNKT cells and DC in CD11cCre-Cd1d1f/f mice demonstrated that insulin sensitivity was improved in obese mice compared to that in control mice (24). iNKT cells activated by adipose DCs produced more IFN-γ than those activated by adipose Mϕs, and different phenotypes were observed between LysMCre-Cd1d1f/f and CD11cCre-Cd1d1f/f mice. These findings suggest that NKT cells play different roles in the development of obesity by interacting with adipose Mϕs or DCs. However, it is unclear whether the lack of CD1d affects the functions of CD1d-expressing cells.
Although the NKT cell-Mϕ interaction seems beneficial in obesity (24), CD1d-deficient Mϕs may exhibit pro-inflammatory functions independent of their lack of cellular interaction with iNKT cells. Given our results that BMMϕs derived from LysMCre-Cd1d1f/f mice express more IL-12p40 in a cell-autonomous manner, it is the deficiency (or downregulation) of CD1d molecules on the cell surface that causes Mϕs to produce more IL-12p40 in response to TLR4 stimulation. The cytoplasmic domain of CD1d transduces signals for the trafficking and regulation of inflammatory responses. The CD1d molecule contains a tyrosine-based signal (YXXZ) (where Y represents tyrosine, X represents any amino acid, and Z is a hydrophobic amino acid) that mediates intracellular trafficking, antigen presentation, NKT cell development (87–90), and a leucine-based basolateral sorting signal in the cytoplasmic tail (91). Another threonine residue (T322) and a serine residue (S323) in the cytoplasmic tail of CD1d control its transport to the cell surface and lysosomal degradation; thus, these motifs can regulate the functional expression of CD1d (92, 93). A few components for sorting CD1d into lysosomes, such as the AP-3 adaptor protein complex and MTP, are necessary to functionally express CD1d (94, 95).
Moreover, phosphorylation of tyrosine residues in the cytoplasmic tail is induced by CD1d crosslinking with an anti-CD1d antibody, which leads to upregulated expression of IL-10 in intestinal epithelial cells (25, 26). Conversely, CD1d crosslinking induces IL-12 production in monocytes and DC via NF-κB activation (27), indicating that the output of the CD1d crosslinking may vary depending on the cell type where CD1d is expressed. Similarly, CD1d in endosomal compartments binds isoglobotrihexosylceramide (iGb3), an endogenous ligand for iNKT cells, induces Tyr332 phosphorylation of the CD1d cytoplasmic domain, and synergizes with TLR signaling in Mϕs and DC (28). Cui et al. have reported that CD1d stimulated with iGb3 induces Ser330 dephosphorylation of CD1d cytoplasmic residue, followed by the downregulation NF-κB activation through the reduction of Peroxiredoxin 1 (PRDX1)-associated AKT-STAT1 phosphorylation in Mϕ (29). The short cytoplasmic tail of CD1d might mediate inhibitory signals for NF-κB activation, depending on Ser330 rather than Tyr332 residue. A similar signaling pathway has been studied as reverse signaling by MHC class Ia in immune and non-immune cells (96).
Notably, a recent study has shown that CD1d-deficient Mϕs amplify TLR signaling by increasing lipid uptake via CD36, independent of CD1d cytoplasmic signaling. This is demonstrated by the fact that the expression of CD1d with a deficient cytoplasmic tail (7 residues) or the tail mutant (332Y -> A) showed similar activity as that of the WT CD1d molecule (30).
According to above reports, the inhibitory effect of CD1d on TLR signaling is weakened in the Mϕs of LysMCre-Cd1d1f/f mice, thereby potentiating the IL-12-IFN-γ circuit leading to AT inflammation (24). However, whether the signaling via CD1d influences only TLR responses or other factors, such as C-type lectins and scavenger receptors, remains unclear. These data suggest that it is difficult to distinguish whether the phenotype observed in CD1d-deficient mice is due to the lack of NKT cells or the CD1d deletion in the respective studies. Caution should be exercised when interpreting the results of studies using mice with either whole-body or conditional deletions of CD1d.
NKT cells control obesity-associated AT inflammation by interacting with CD1d-expressing cells such as adipocytes, Mϕs, and DCs. In the interactions among these cells, unknown endogenous lipid ligands are presented via CD1d. Different ligands are possibly expressed depending on the cell type, leading to the respective immune microenvironment and phenotypes in the development of obesity (Figure 1). Additionally, NKT cells may contribute to both lean and obese phase, and their interaction with the predominant APCs at the time, such as M2-Mϕs in steady state AT or hypertrophied adipocytes in obesity, can affect NKT cell function. However, if CD1d molecules had a regulatory function that either enhances or suppresses the inflammatory process in Mϕs, this pathway could function independently of NKT cells. To understand the chronic inflammatory process during obesity, it will be important to further investigate the regulatory effect of CD1d, endogenous glycolipids, and NKT cells on immune responses in the AT. Thus, we obtained new insights into the strategies for overcoming obesity and obesity-associated diseases.
Figure 1 iNKT cells and CD1d control AT inflammation. Adipose iNKT cells interact with adipocytes, predominantly secreting IFN-γ and activating adipocytes to enhance the expression of CD1d and CCL2. The iNKT-adipocyte interaction forms an inflammatory circuit leading to AT inflammation and insulin resistance. The interaction between M2-Mϕ and iNKT cells induces Th2 cytokines, while the interaction with M1-Mϕ leads to Th1 cytokines, thereby modulating AT microenvironment. CD1d-deficient (or reduced) Mϕs secreted more IL-12 in response to palmitic acid than WT Mϕs to induce IFN-γ production in iNKT cells, because CD1d molecules presumably suppressed TLR responses in WT Mϕs. This scheme indicates that the activation process of adipose iNKT cells is APC-specific and that CD1d molecules contribute to immune responses.
MS: Writing – original draft, Writing – review & editing. KI: Writing – original draft, Writing – review & editing.
The author(s) declare financial support was received for the research, authorship, and/or publication of this article. This work was supported by the Takeda Science Foundation and JSPS KAKENHI Grant Numbers JP22K08679.
The authors declare that the research was conducted in the absence of any commercial or financial relationships that could be construed as a potential conflict of interest.
All claims expressed in this article are solely those of the authors and do not necessarily represent those of their affiliated organizations, or those of the publisher, the editors and the reviewers. Any product that may be evaluated in this article, or claim that may be made by its manufacturer, is not guaranteed or endorsed by the publisher.
1. Hotamisligil GS. Inflammation and metabolic disorders. Nature (2006) 444(7121):860–7. doi: 10.1038/nature05485
2. Gliniak CM, Pedersen L, Scherer PE. Adipose tissue fibrosis: the unwanted houseguest invited by obesity. J Endocrinol (2023) 259(3):e230180. doi: 10.1530/JOE-23-0180
3. Kahn SE, Hull RL, Utzschneider KM. Mechanisms linking obesity to insulin resistance and type 2 diabetes. Nature (2006) 444(7121):840–6. doi: 10.1038/nature05482
4. Kahn CR, Wang G, Lee KY. Altered adipose tissue and adipocyte function in the pathogenesis of metabolic syndrome. J Clin Invest (2019) 129(10):3990–4000. doi: 10.1172/JCI129187
5. Weisberg SP, McCann D, Desai M, Rosenbaum M, Leibel RL, Ferrante AW Jr. Obesity is associated with macrophage accumulation in adipose tissue. J Clin Invest (2003) 112(12):1796–808. doi: 10.1172/JCI19246
6. Weisberg SP, Hunter D, Huber R, Lemieux J, Slaymaker S, Vaddi K, et al. CCR2 modulates inflammatory and metabolic effects of high-fat feeding. J Clin Invest (2006) 116(1):115–24. doi: 10.1172/JCI24335
7. Nishimura S, Manabe I, Nagasaki M, Eto K, Yamashita H, Ohsugi M, et al. CD8+ effector T cells contribute to macrophage recruitment and adipose tissue inflammation in obesity. Nat Med (2009) 15(8):914–20. doi: 10.1038/nm.1964
8. Feuerer M, Herrero L, Cipolletta D, Naaz A, Wong J, Nayer A, et al. Lean, but not obese, fat is enriched for a unique population of regulatory T cells that affect metabolic parameters. Nat Med (2009) 15(8):930–9. doi: 10.1038/nm.2002
9. Winer DA, Winer S, Shen L, Wadia PP, Yantha J, Paltser G, et al. B cells promote insulin resistance through modulation of T cells and production of pathogenic IgG antibodies. Nat Med (2011) 17(5):610–7. doi: 10.1038/nm.2353
10. Talukdar S, Oh DY, Bandyopadhyay G, Li D, Xu J, McNelis J, et al. Neutrophils mediate insulin resistance in mice fed a high-fat diet through secreted elastase. Nat Med (2012) 18(9):1407–12. doi: 10.1038/nm.2885
11. Ouchi N, Parker JL, Lugus JJ, Walsh K. Adipokines in inflammation and metabolic disease. Nat Rev Immunol (2011) 11:85–97. doi: 10.1038/nri2921
12. Satoh M, Iwabuchi K. Role of natural killer T cells in the development of obesity and insulin resistance: insights from recent progress. Front Immunol (2018) 9:1314. doi: 10.3389/fimmu.2018.01314
13. Van Kaer L. NKT cells: T lymphocytes with innate effector functions. Curr Opin Immunol (2007) 19:354–64. doi: 10.1016/j.coi.2007.03.001
14. Bendelac A, Savage PB, Teyton L. The biology of NKT cells. Annu Rev Immunol (2007) 25:297–336. doi: 10.1146/annurev.immunol.25.022106.141711
15. Canchis PW, Bhan AK, Landau SB, Yang L, Balk SP, Blumberg RS. Tissue distribution of the non-polymorphic major histocompatibility complex class I-like molecule, CD1d. Immunology (1993) 80(4):561–5.
16. Godfrey DI, Stankovic S, Baxter AG. Raising the NKT cell family. Nat Immunol (2010) 11:197–206. doi: 10.1038/ni.1841
17. Kawano T, Cui J, Koezuka Y, Toura I, Kaneko Y, Motoki K, et al. CD1d-restricted and TCR-mediated activation of Vα14 NKT cells by glycosylceramides. Science (1997) 278(5343):1626–9. doi: 10.1126/science.278.5343.1626
18. Halder RC, Aguilera C, Maricic I, Kumar V. Type II NKT cell-mediated anergy induction in type I NKT cells prevents inflammatory liver disease. J Clin Invest (2007) 117:2302–12. doi: 10.1172/JCI31602
19. Matsuda JL, Mallevaey T, Scott-Browne J, Gapin L. CD1d-restricted iNKT cells, the 'Swiss-Army knife' of the immune system. Curr Opin Immunol (2008) 20(3):358–68. doi: 10.1016/j.coi.2008.03.018
20. Lee YJ, Wang H, Starrett GJ, Phuong V, Jameson SC, Hogquist KA. Tissue-specific distribution of iNKT cells impacts their cytokine response. Immunity (2015) 43(3):566–78. doi: 10.1016/j.immuni.2015.06.025
21. Kwon DI, Lee YJ. Lineage differentiation program of invariant natural killer T cells. Immune Netw (2017) 17(6):365–77. doi: 10.4110/in.2017.17.6.365
22. Lee B, Jo Y, Kim G, Ali LA, Sohn DH, Lee SG, et al. Specific inhibition of soluble γc receptor attenuates collagen-induced arthritis by modulating the inflammatory T cell responses. Front Immunol (2019) 10:209. doi: 10.3389/fimmu.2019.00209
23. Tumes D, Hirahara K, Papadopoulos M, Shinoda K, Onodera A, Kumagai J, et al. Ezh2 controls development of natural killer T cells, which cause spontaneous asthma-like pathology. J Allergy Clin Immunol (2019) 144(2):549–60.e10. doi: 10.1016/j.jaci.2019.02.024
24. Satoh M, Iizuka M, Majima M, Ohwa C, Hattori A, Van Kaer L, et al. Adipose invariant NKT cells interact with CD1d-expressing macrophages to regulate obesity-related inflammation. Immunology (2022) 165(4):414–27. doi: 10.1111/imm.13447
25. Colgan SP, Hershberg RM, Furuta GT, Blumberg RS. Ligation of intestinal epithelial CD1d induces bioactive IL-10: critical role of the cytoplasmic tail in autocrine signaling. Proc Natl Acad Sci USA (1999) 96(24):13938–43. doi: 10.1073/pnas.96.24.13938
26. Olszak T, Neves JF, Dowds CM, Baker K, Glickman J, Davidson NO, et al. Protective mucosal immunity mediated by epithelial CD1d and IL-10. Nature (2014) 509(7501):497–502. doi: 10.1038/nature13150
27. Yue SC, Shaulov A, Wang R, Balk SP, Exley MA. CD1d ligation on human monocytes directly signals rapid NF-kappaB activation and production of bioactive IL-12. Proc Natl Acad Sci U.S.A. (2005) 102(33):11811–6. doi: 10.1073/pnas.0503366102
28. Liu X, Zhang P, Zhang Y, Wang Z, Xu S, Li Y, et al. Glycolipid iGb3 feedback amplifies innate immune responses via CD1d reverse signaling. Cell Res (2019) 29(1):42–53. doi: 10.1038/s41422-018-0122-7
29. Cui S, Wang C, Bai W, Li J, Pan Y, Huang X, et al. CD1d1 intrinsic signaling in macrophages controls NLRP3 inflammasome expression during inflammation. Sci Adv (2020) 6(43):eaaz7290. doi: 10.1126/sciadv.aaz7290
30. Brailey PM, Evans L, López-Rodríguez JC, Sinadinos A, Tyrrel V, Kelly G, et al. CD1d-dependent rewiring of lipid metabolism in macrophages regulates innate immune responses. Nat Commun (2022) 13(1):6723. doi: 10.1038/s41467-022-34532-x
31. Lee BC, Kim MS, Pae M, Yamamoto Y, Eberlé D, Shimada T, et al. Adipose natural killer cells regulate adipose tissue macrophages to promote insulin resistance in obesity. Cell Metab (2016) 23(4):685–98. doi: 10.1016/j.cmet.2016.03.002
32. Wensveen FM, Jelenčić V, Valentić S, Šestan M, Wensveen TT, Theurich S, et al. NK cells link obesity-induced adipose stress to inflammation and insulin resistance. Nat Immunol (2015) 16(4):376–85. doi: 10.1038/ni.3120
33. Boulenouar S, Michelet X, Duquette D, Alvarez D, Hogan AE, Dold C, et al. Adipose type one innate lymphoid cells regulate macrophage homeostasis through targeted cytotoxicity. Immunity (2017) 46(2):273–86. doi: 10.1016/j.immuni.2017.01.008
34. O'Sullivan TE, Rapp M, Fan X, Weizman OE, Bhardwaj P, Adams NM, et al. Adipose-resident group 1 innate lymphoid cells promote obesity-associated insulin resistance. Immunity (2016) 45(2):428–41. doi: 10.1016/j.immuni.2016.06.016
35. Wu D, Molofsky AB, Liang HE, Ricardo-Gonzalez RR, Jouihan HA, Bando JK, et al. Eosinophils sustain adipose alternatively activated macrophages associated with glucose homeostasis. Science (2011) 332(6026):243–7. doi: 10.1126/science.1201475
36. Sasaki T, Moro K, Kubota T, Kubota N, Kato T, Ohno H, et al. Innate lymphoid cells in the induction of obesity. Cell Rep (2019) 28(1):202–17.e7. doi: 10.1016/j.celrep.2019.06.016
37. Brestoff JR, Kim BS, Saenz SA, Stine RR, Monticelli LA, Sonnenberg GF, et al. Group 2 innate lymphoid cells promote beiging of white adipose tissue and limit obesity. Nature (2015) 519(7542):242–6. doi: 10.1038/nature14115
38. Qiu Y, Nguyen KD, Odegaard JI, Cui X, Tian X, Locksley RM, et al. Eosinophils and type 2 cytokine signaling in macrophages orchestrate development of functional beige fat. Cell (2014) 157(6):1292–308. doi: 10.1016/j.cell.2014.03.066
39. Rao RR, Long JZ, White JP, Svensson KJ, Lou J, Lokurkar I, et al. Meteorin-like is a hormone that regulates immune-adipose interactions to increase beige fat thermogenesis. Cell (2014) 157(6):1279–91. doi: 10.1016/j.cell.2014.03.065
40. Satoh M, Andoh Y, Clingan CS, Ogura H, Fujii S, Eshima K, et al. Type II NKT cells stimulate diet-induced obesity by mediating adipose tissue inflammation, steatohepatitis and insulin resistance. PloS One (2012) 7(2):e30568. doi: 10.1371/journal.pone.0030568
41. Wu L, Parekh VV, Gabriel CL, Bracy DP, Marks-Shulman PA, Tamboli RA, et al. Activation of invariant natural killer T cells by lipid excess promotes tissue inflammation, insulin resistance, and hepatic steatosis in obese mice. Proc Natl Acad Sci USA (2012) 109(19):E1143–52. doi: 10.1073/pnas.1200498109
42. Ren Y, Sekine-Kondo E, Shibata R, Kato-Itoh M, Umino A, Yanagida A, et al. A Novel Mouse Model of iNKT Cell-deficiency Generated by CRISPR/Cas9 Reveals a Pathogenic Role of iNKT Cells in Metabolic Disease. Sci Rep (2017) 7(1):12765. doi: 10.1038/s41598-017-12475-4
43. Subramanian S, Turner MS, Ding Y, Goodspeed L, Wang S, Buckner JH, et al. Increased levels of invariant natural killer T lymphocytes worsen metabolic abnormalities and atherosclerosis in obese mice. J Lipid Res (2013) 54:2831–41. doi: 10.1194/jlr.M041020
44. Lynch L, Nowak M, Varghese B, Clark J, Hogan AE, Toxavidis V, et al. Adipose tissue invariant NKT cells protect against diet-induced obesity and metabolic disorder through regulatory cytokine production. Immunity (2012) 37(3):574–87. doi: 10.1016/j.immuni.2012.06.016
45. Ji Y, Sun S, Xu A, Bhargava P, Yang L, Lam KS, et al. Activation of natural killer T cells promotes M2 Macrophage polarization in adipose tissue and improves systemic glucose tolerance via interleukin-4 (IL-4)/STAT6 protein signaling axis in obesity. J Biol Chem (2012) 287(17):13561–71. doi: 10.1074/jbc.M112.350066
46. Schipper HS, Rakhshandehroo M, van de Graaf SF, Venken K, Koppen A, Stienstra R, et al. Natural killer T cells in adipose tissue prevent insulin resistance. J Clin Invest (2012) 122(9):3343–54. doi: 10.1172/JCI62739
47. Hams E, Locksley RM, McKenzie AN, Fallon PG. Cutting edge: IL-25 elicits innate lymphoid type 2 and type II NKT cells that regulate obesity in mice. J Immunol (2013) 191(11):5349–53. doi: 10.4049/jimmunol.1301176
48. Mantell BS, Stefanovic-Racic M, Yang X, Dedousis N, Sipula IJ, O'Doherty RM. Mice lacking NKT cells but with a complete complement of CD8+ T-cells are not protected against the metabolic abnormalities of diet-induced obesity. PloS One (2011) 6:e19831. doi: 10.1371/journal.pone.0019831
49. Kotas ME, Lee HY, Gillum MP, Annicelli C, Guigni BA, Shulman GI, et al. Impact of CD1d deficiency on metabolism. PloS One (2011) 6(9):e25478. doi: 10.1371/journal.pone.0025478
50. Satoh M, Hoshino M, Fujita K, Iizuka M, Fujii S, Clingan CS, et al. Adipocyte-specific CD1d-deficiency mitigates diet-induced obesity and insulin resistance in mice. Sci Rep (2016) 6:28473. doi: 10.1038/srep28473
51. Huh JY, Park J, Kim JI, Park YJ, Lee YK, Kim JB. Deletion of CD1d in adipocytes aggravates adipose tissue inflammation and insulin resistance in obesity. Diabetes (2017) 66(4):835–47. doi: 10.2337/db16-1122
52. Bedel R, Matsuda JL, Brigl M, White J, Kappler J, Marrack P, et al. Lower TCR repertoire diversity in Traj18-deficient mice. Nat Immunol (2012) 13:705–6. doi: 10.1038/ni.2347
53. Xie J, Pan Y, Tao H, Wang P, Chen Y, Gao J, et al. Deficiency of mucosal-associated invariant T cells in TCRJα18 germline knockout mice. Immunohorizons (2019) 3:203–7. doi: 10.4049/immunohorizons.1900035
54. Toubal A, Kiaf B, Beaudoin L, Cagninacci L, Rhimi M, Fruchet B, et al. Mucosal-associated invariant T cells promote inflammation and intestinal dysbiosis leading to metabolic dysfunction during obesity. Nat Commun (2020) 11(1):3755. doi: 10.1038/s41467-020-17307-0
55. Bergin R, Kinlen D, Kedia-Mehta N, Hayes E, Cassidy FC, Cody D, et al. Mucosal-associated invariant T cells are associated with insulin resistance in childhood obesity, and disrupt insulin signalling via IL-17. Diabetologia (2022) 65:1012–7. doi: 10.1007/s00125-022-05682-w
56. Carolan E, Tobin LM, Mangan BA, Corrigan M, Gaoatswe G, Byrne G, et al. Altered distribution and increased IL-17 production by mucosal-associated invariant T cells in adult and childhood obesity. J Immunol (2015) 194:5775–80. doi: 10.4049/jimmunol.1402945
57. Magalhaes I, Pingris K, Poitou C, Bessoles S, Venteclef N, Kiaf B, et al. Mucosal-associated invariant T cell alterations in obese and type 2 diabetic patients. J Clin Invest (2015) 125:1752–62. doi: 10.1172/JCI78941
58. Sag D, Krause P, Hedrick CC, Kronenberg M, Wingender G. IL-10-producing NKT10 cells are a distinct regulatory invariant NKT cell subset. J Clin Invest (2014) 124(9):3725–40. doi: 10.1172/JCI72308
59. Lynch L, Michelet X, Zhang S, Brennan PJ, Moseman A, Lester C, et al. Regulatory iNKT cells lack expression of the transcription factor PLZF and control the homeostasis of T(reg) cells and macrophages in adipose tissue. Nat Immunol (2015) 16(1):85–95. doi: 10.1038/ni.3047
60. Vieth JA, Das J, Ranaivoson FM, Comoletti D, Denzin LK, Sant'Angelo DB. TCRα-TCRβ pairing controls recognition of CD1d and directs the development of adipose NKT cells. Nat Immunol (2017) 18(1):36–44. doi: 10.1038/ni.3622
61. Aguiar CF, Corrêa-da-Silva F, Gonzatti MB, Angelim MK, Pretti MA, Davanzo GG, et al. Tissue-specific metabolic profile drives iNKT cell function during obesity and liver injury. Cell Rep (2023) 42(1):112035. doi: 10.1016/j.celrep.2023.112035
62. Wieland Brown LC, Penaranda C, Kashyap PC, Williams BB, Clardy J, Kronenberg M, et al. Production of α-galactosylceramide by a prominent member of human gut microbiota. PloS Biol (2013) 11(7):e1001610. doi: 10.1371/journal.pbio.1001610
63. An D, Oh SF, Olszak T, Neves JF, Avci FY, Erturk-Hasdemir D, et al. Sphingolipids from a symbiotic microbe regulate homeostasis of host intestinal natural killer T cells. Cell (2014) 156(1-2):123–33. doi: 10.1016/j.cell.2013.11.042
64. Oh SF, Praveena T, Song H, Yoo JS, Jung DJ, Erturk-Hasdemir D, et al. Host immunomodulatory lipids created by symbionts from dietary amino acids. Nature (2022) 601(7894):E32. doi: 10.1038/s41586-021-04276-7
65. Selvanantham T, Lin Q, Guo CX, Surendra A, Fieve S, Escalante NK, et al. NKT cell-deficient mice harbor an altered microbiota that fuels intestinal inflammation during chemically induced colitis. J Immunol (2016) 197:4464–72. doi: 10.4049/jimmunol.1601410
66. Warensjö E, Risérus U, Vessby B. Fatty acid composition of serum lipids predicts the development of the metabolic syndrome in men. Diabetologia (2005) 48:1999–2005. doi: 10.1007/s00125-005-1897-x
67. Hodson L, Skeaff CM, Fielding BA. Fatty acid composition of adipose tissue and blood in humans and its use as a biomarker of dietary intake. Prog Lipid Res (2008) 47:348–80. doi: 10.1016/j.plipres.2008.03.003
68. LaMarche NM, Kane H, Kohlgruber AC, Dong H, Lynch L, Brenner MB. Distinct iNKT cell populations use IFNγ or ER stress-induced IL-10 to control adipose tissue homeostasis. Cell Metab (2020) 32:243–58.e6. doi: 10.1016/j.cmet.2020.05.017
69. Han SM, Park ES, Park J, Nahmgoong H, Choi YH, Oh J, et al. Unique adipose tissue invariant natural killer T cell subpopulations control adipocyte turnover in mice. Nat Commun (2023) 14:8512. doi: 10.1038/s41467-023-44181-3
70. Tilg H, Moschen AR. Adipocytokines: mediators linking adipose tissue, inflammation and immunity. Nat Rev Immunol (2006) 6(10):772–83. doi: 10.1038/nri1937
71. Rakhshandehroo M, Gijzel SM, Siersbæk R, Broekema MF, de Haar C, Schipper HS, et al. CD1d-mediated presentation of endogenous lipid antigens by adipocytes requires microsomal triglyceride transfer protein. J Biol Chem (2014) 289(32):22128–39. doi: 10.1074/jbc.M114.551242
72. Huh JY, Kim JI, Park YJ, Hwang IJ, Lee YS, Sohn JH, et al. A novel function of adipocytes in lipid antigen presentation to iNKT cells. Mol Cell Biol (2013) 33(2):328–39. doi: 10.1128/MCB.00552-12
73. Deng T, Lyon CJ, Minze LJ, Lin J, Zou J, Liu JZ, et al. Class II major histocompatibility complex plays an essential role in obesity-induced adipose inflammation. Cell Metab (2013) 17(3):411–22. doi: 10.1016/j.cmet.2013.02.009
74. Rakhshandehroo M, van Eijkeren RJ, Gabriel TL, de Haar C, Gijzel SMW, Hamers N, et al. Adipocytes harbor a glucosylceramide biosynthesis pathway involved in iNKT cell activation. Biochim Biophys Acta Mol Cell Biol Lipids (2019) 1864(8):1157–67. doi: 10.1016/j.bbalip.2019.04.016
75. van Eijkeren RJ, Morris I, Borgman A, Markovska A, Kalkhoven E. Cytokine output of adipocyte-iNKT cell interplay is skewed by a lipid-rich microenvironment. Front Endocrinol (Lausanne) (2020) 11:479. doi: 10.3389/fendo.2020.00479
76. Engelen SE, Ververs FA, Markovska A, Lagerholm BC, Kraaijenhof JM, Yousif LI, et al. Lipoproteins act as vehicles for lipid antigen delivery and activation of invariant natural killer T cells. JCI Insight (2023) 8(9):e158089. doi: 10.1172/jci.insight.158089
77. Xiao R, Mansour AG, Huang W, Hassan QN 2nd, Wilkins RK, Komatineni SV, et al. Adipocyte CD1d gene transfer induces T cell expansion and adipocyte inflammation in CD1d knockout mice. J Immunol (2022) 208(9):2109–21. doi: 10.4049/jimmunol.2100313
78. Zhang H, Xue R, Zhu S, Fu S, Chen Z, Zhou R, et al. M2-specific reduction of CD1d switches NKT cell-mediated immune responses and triggers metaflammation in adipose tissue. Cell Mol Immunol (2018) 15(5):506–17. doi: 10.1038/cmi.2017.11
79. Muir LA, Cho KW, Geletka LM, Baker NA, Flesher CG, Ehlers AP, et al. Human CD206+ macrophages associate with diabetes and adipose tissue lymphoid clusters. JCI Insight (2022) 7(3):e146563. doi: 10.1172/jci.insight.146563
80. Wentworth JM, Naselli G, Brown WA, Doyle L, Phipson B, Smyth GK, et al. Pro-inflammatory CD11c+CD206+ adipose tissue macrophages are associated with insulin resistance in human obesity. Diabetes (2010) 59(7):1648–56. doi: 10.2337/db09-0287
81. Arora P, Kharkwal SS, Ng TW, Kunnath-Velayudhan S, Saini NK, Johndrow CT, et al. Endocytic pH regulates cell surface localization of glycolipid antigen loaded CD1d complexes. Chem Phys Lipids (2016) 194:49–57. doi: 10.1016/j.chemphyslip.2015.10.006
82. Morris I, Croes CA, Boes M, Kalkhoven E. Advanced omics techniques shed light on CD1d-mediated lipid antigen presentation to iNKT cells. Biochim Biophys Acta Mol Cell Biol Lipids (2023) 1868(5):159292. doi: 10.1016/j.bbalip.2023.159292
83. Xu X, Grijalva A, Skowronski A, van Eijk M, Serlie MJ, Ferrante AW Jr. Obesity activates a program of lysosomal-dependent lipid metabolism in adipose tissue macrophages independently of classic activation. Cell Metab (2013) 18(6):816–30. doi: 10.1016/j.cmet.2013.11.001
84. Stefanovic-Racic M, Yang X, Turner MS, Mantell BS, Stolz DB, Sumpter TL, et al. Dendritic cells promote macrophage infiltration and comprise a substantial proportion of obesity-associated increases in CD11c+ cells in adipose tissue and liver. Diabetes (2012) 61(9):2330–9. doi: 10.2337/db11-1523
85. Patsouris D, Li PP, Thapar D, Chapman J, Olefsky JM, Neels JG. Ablation of CD11c-positive cells normalizes insulin sensitivity in obese insulin resistant animals. Cell Metab (2008) 8(4):301–9. doi: 10.1016/j.cmet.2008.08.015
86. Hernández-García E, Cueto FJ, Cook ECL, Redondo-Urzainqui A, Charro-Zanca S, Robles-Vera I, et al. Conventional type 1 dendritic cells protect against age-related adipose tissue dysfunction and obesity. Cell Mol Immunol (2022) 19(2):260–75. doi: 10.1038/s41423-021-00812-7
87. Rodionov DG, Nordeng TW, Pedersen K, Balk SP, Bakke O. A critical tyrosine residue in the cytoplasmic tail is important for CD1d internalization but not for its basolateral sorting in MDCK cells. J Immunol (1999) 162(3):1488–95. doi: 10.4049/jimmunol.162.3.1488
88. Roberts TJ, Sriram V, Spence PM, Gui M, Hayakawa K, Bacik I, et al. Recycling CD1d1 molecules present endogenous antigens processed in an endocytic compartment to NKT cells. J Immunol (2002) 168(11):5409–14. doi: 10.4049/jimmunol.168.11.5409
89. Lawton AP, Prigozy TI, Brossay L, Pei B, Khurana A, Martin D, et al. The mouse CD1d cytoplasmic tail mediates CD1d trafficking and antigen presentation by adaptor protein 3-dependent and -independent mechanisms. J Immunol (2005) 174(6):3179–86. doi: 10.4049/jimmunol.174.6.3179
90. Chiu YH, Park SH, Benlagha K, Forestier C, Jayawardena-Wolf J, Savage PB, et al. Multiple defects in antigen presentation and T cell development by mice expressing cytoplasmic tail-truncated CD1d. Nat Immunol (2002) 3(1):55–60. doi: 10.1038/ni740
91. Rodionov DG, Nordeng TW, Kongsvik TL, Bakke O. The cytoplasmic tail of CD1d contains two overlapping basolateral sorting signals. J Biol Chem (2000) 275(12):8279–82. doi: 10.1074/jbc.275.12.8279
92. Liu J, Shaji D, Cho S, Du W, Gervay-Hague J, Brutkiewicz RR. A threonine-based targeting signal in the human CD1d cytoplasmic tail controls its functional expression. J Immunol (2010) 184(9):4973–81. doi: 10.4049/jimmunol.0901448
93. Liu J, Glosson NL, Du W, Gervay-Hague J, Brutkiewicz RR. A Thr/Ser dual residue motif in the cytoplasmic tail of human CD1d is important for the down-regulation of antigen presentation following a herpes simplex virus 1 infection. Immunology (2013) 140(2):191–201. doi: 10.1111/imm.12127
94. Cernadas M, Sugita M, van der Wel N, Cao X, Gumperz JE, Maltsev S, et al. Lysosomal localization of murine CD1d mediated by AP-3 is necessary for NK T cell development. J Immunol (2003) 171(8):4149–55. doi: 10.4049/jimmunol.171.8.4149
95. Sagiv Y, Bai L, Wei DG, Agami R, Savage PB, Teyton L, et al. A distal effect of microsomal triglyceride transfer protein deficiency on the lysosomal recycling of CD1d. J Exp Med (2007) 204(4):921–8. doi: 10.1084/jem.20061568
Keywords: NKT cells, CD1d, macrophages, obesity, insulin resistance, adipose tissue inflammation
Citation: Satoh M and Iwabuchi K (2024) Contribution of NKT cells and CD1d-expressing cells in obesity-associated adipose tissue inflammation. Front. Immunol. 15:1365843. doi: 10.3389/fimmu.2024.1365843
Received: 05 January 2024; Accepted: 30 January 2024;
Published: 15 February 2024.
Edited by:
Raffaele De Palma, University of Genoa, ItalyReviewed by:
Lan Wu, Vanderbilt University Medical Center, United StatesCopyright © 2024 Satoh and Iwabuchi. This is an open-access article distributed under the terms of the Creative Commons Attribution License (CC BY). The use, distribution or reproduction in other forums is permitted, provided the original author(s) and the copyright owner(s) are credited and that the original publication in this journal is cited, in accordance with accepted academic practice. No use, distribution or reproduction is permitted which does not comply with these terms.
*Correspondence: Masashi Satoh, bXNhdG9AbWVkLmtpdGFzYXRvLXUuYWMuanA=
Disclaimer: All claims expressed in this article are solely those of the authors and do not necessarily represent those of their affiliated organizations, or those of the publisher, the editors and the reviewers. Any product that may be evaluated in this article or claim that may be made by its manufacturer is not guaranteed or endorsed by the publisher.
Research integrity at Frontiers
Learn more about the work of our research integrity team to safeguard the quality of each article we publish.