- 1Laboratory Animal Center, the First Affiliated Hospital of Wenzhou Medical University, Wenzhou, Zhejiang, China
- 2Institute of Hematology, Wenzhou Medical University, Wenzhou, Zhejiang, China
- 3Wenzhou Key laboratory of Hematology, Wenzhou, Zhejiang, China
- 4Department of Hematology, The First Affiliated Hospital of Wenzhou Medical University, Wenzhou, Zhejiang, China
- 5Central Laboratory, The First Affiliated Hospital of Wenzhou Medical University, Wenzhou, Zhejiang, China
CD44 is a ubiquitous leukocyte adhesion molecule involved in cell-cell interaction, cell adhesion, migration, homing and differentiation. CD44 can mediate the interaction between leukemic stem cells and the surrounding extracellular matrix, thereby inducing a cascade of signaling pathways to regulate their various behaviors. In this review, we focus on the impact of CD44s/CD44v as biomarkers in leukemia development and discuss the current research and prospects for CD44-related interventions in clinical application.
1 CD44 introduction
1.1 Structural basis of CD44
CD44 is not just a single molecule localized on chromosome 11, but a transmembrane glycoprotein with molecular diversity produced by alternative splicing of multiple exons of a single gene and different post-translational modifications in different cell types (1). Exons located at both ends (1-5 and 16-20) are constitutive exons shared by all members of the CD44 family. In humans, the selective combination of various middle 9 exons during transcription give rise to different isoforms of CD44 precursors, thereby designating these middle 9 exons as variant exons (Figure 1A) (2). In addition to transcriptional shearing to generate different isoforms, CD44 also undergoes post-translational modifications. The most prevalent forms of glycosylation include N-linked and O-linked glycosylation (3). These post-translational modifications of CD44 isoforms have led to a more diverse ability to regulate cellular activities. For instance, following post-translational modification, different CD44 variants (CD44v) exhibit distinct modes of binding with its ligand Hyaluronic acid (HA) (4).
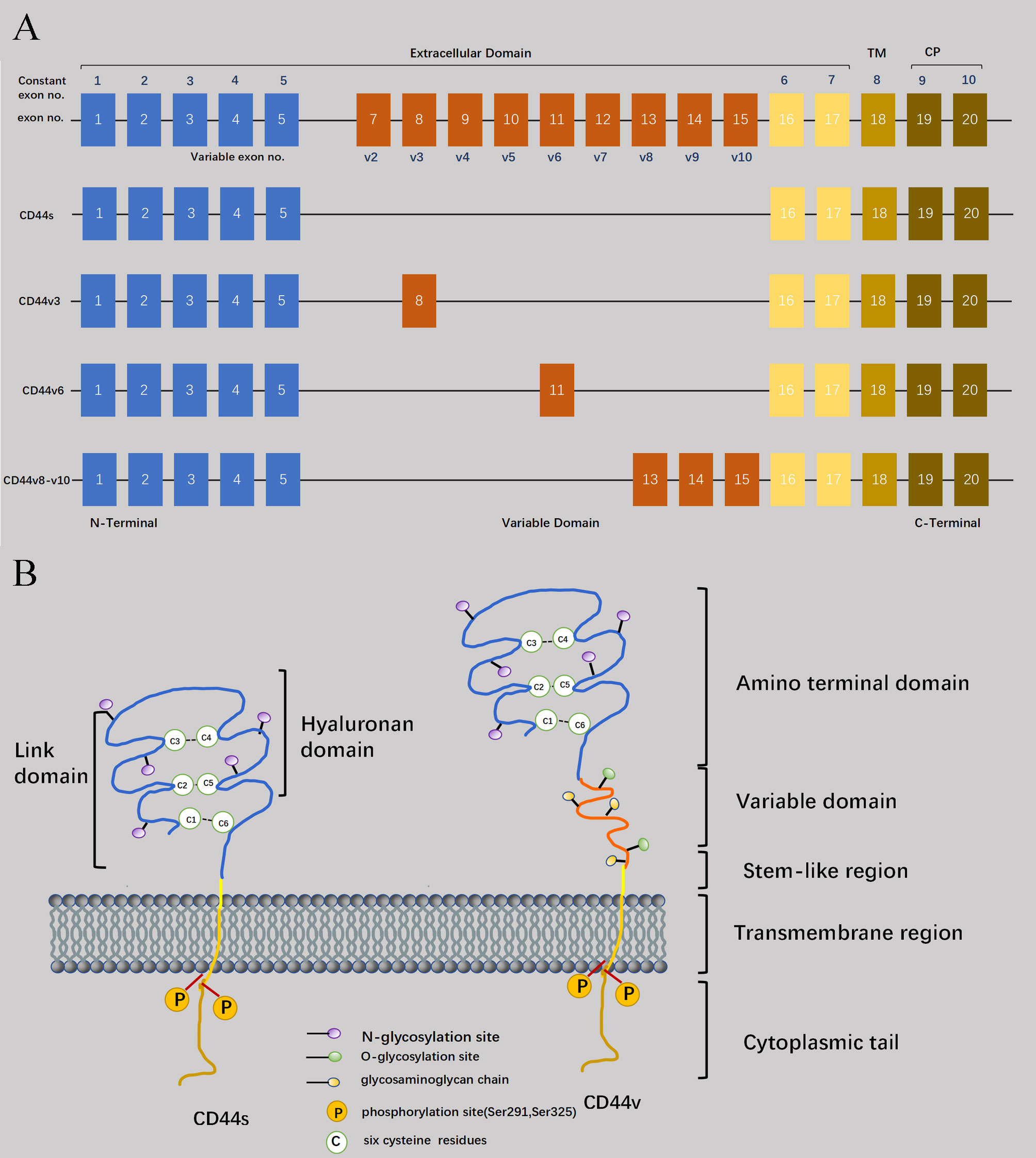
Figure 1 Structural basis of CD44. (A) CD44 is encoded by 20 exons in mice but 19 exons in humans. Exon 6 coding for CD44 variant 1 (CD44v1) is lacking in humans. Except for orange color exons, the rest of exons always expressed as a standard form of CD44 (CD44s), and up to ten exon variants can be inserted by alternative splicing. Full-length CD44, CD44s, CD44v3, CD44v6, and CD44v8-10 are shown schematically. (B) Four domains of CD44 glycoprotein are six cysteine residues (located at the amino acid termini), a stem-like region, transmembrane domain, and cytoplasmic tail. And the variable domain is between six cysteine residues and stem -like region.
Regarding the molecular size, CD44 consists of 363 amino acids (37 kDa). However, due to glycosylation modifications and coding of mutant exons in the CD44 molecule, its actual molecular weight exceeds 37kDa and has been measured to be around 80-90 kDa (5). The extracellular region, transmembrane region, and short intracellular/cytoplasmic region at the C-terminus comprise the key characteristics of CD44 protein (Figure 1B) (6). Specifically, the extracellular domain can be subdivided into a globular structural domain consisting of six cysteine residues and a stem-like region in the extracellular near-membrane portion of the cell (7). As for the transmembrane and intracellular regions, both of them exhibit remarkable conservation in terms of their structure. The transmembrane region facilitates the oligomerization of CD44 at the cell membrane, thereby contributing to its localization in glycolipid-rich micromembrane compartments, as well as serving as a structural basis for interaction with lipid rafts (8).
1.2 CD44 receptor-ligand interaction
The biological characteristics initiated by CD44 are based on its ligand binding specificity and affinity. Commonly utilized ligands include HA, osteopontin (OPN), chondroitin, filaggrin/sulfated proteoglycans, fibronectin, and other compounds (9). Among these ligands, HA and OPN serve as the primary triggers for the majority of CD44-mediated activities.
1.3 Hyaluronic acid
CD44 interacts with HA, a common component of the extracellular matrix (ECM), in two ways (10). Firstly, cell membrane localized CD44 anchors soluble HA to the cell membrane through specific interactions. Secondly, immune or cancer cells expressing CD44 interacts with other cell membrane-expressed or anchored HA to mediate cell adhesion. The CD44 exists in three different states: (1) the resting state, where CD44 is unable to bind to its ligand HA; (2) the inducible and activated state, wherein CD44 requires specific activation by an activator to interact with HA; (3) the activated state, which does not necessitate an activator for interaction with HA (4). The majority of CD44 molecules present on the surface of hematopoietic cells are found in an inducible and activated state. Microstructurally, CD44 interacts with the ligand HA in a 160 amino acid fragment. In the specific subunit CD44 HA-binding domain (HABD) of CD44-bound HA and its corresponding amino acid sequence (The redox environment in cells affects the binding of HA through the disulfide bond formed by Cys77 and Cys97 in CD44), HA binds to CD44 primarily through hydrogen bonding and van der Waals forces rather than electrostatic and aromatic stacking interactions (11). The existence of two primary conformations, A and B, has been established for HABD (12). Notably, the B conformation exhibits a higher affinity compared to the other conformation. These distinct mechanisms can elucidate the varying degrees of conformational effects observed in CD44-HABD. Specifically, upon HA-binding stigmata, a C-terminal fragment undergoes a transition from an ordered state to a disordered state, consequently enhancing flexibility. The other observation is that HA binding causes an orientation change in the loop region of HABD near the arginine site at R41, consequently altering its affinity for HA. In addition, the affinity of CD44 for HA is influenced by the molecular weight of HA (13). Within a specific range, the binding capacity of CD44 to high molecular weight HA surpasses its interaction with low molecular weight HA, potentially due to an abundance of binding sites between high molecular weight HA and CD44. However, the binding affinity between these two molecules decreases as the molecular weight of HA increases, rather than intensifying (13). In addition, numerous other factors can influence the binding ability of CD44 to HA. For instance, the extent of glycosylation in the extracellular domain of CD44 and phosphorylation of specific serine residues at the cytoplasmic end regulates its HA-binding capacity. The regulation also involves cytokines and matrix metalloproteins. Furthermore, TNF-α can also induce HA binding to CD44 on peripheral blood monocytes.
1.4 Osteopontin
The primary roles of OPN encompass serving as an integral constituent of the extracellular matrix and functioning as a cytokine (14). In the C-terminal sequence of OPN, there is also a non-RGD cell adhesion site segment, and OPN acts as a signaling molecule by binding to CD44 on the cell surface in an RGD-independent manner (15). Phosphorylation is an important self-regulatory mechanism for OPN; phosphorylated OPN specifically interacts with cell surface integrin receptors, while the dephosphorylated form selectively binds to the CD44 receptor and elicits distinct functional outcomes (16). The accessibility of the extracellular domain to the compressed core region of OPN is also a pivotal determinant for effective interaction with CD44. Furthermore, glycosylation of OPN may prevent its interaction with CD44. In addition to the extensive diversity of OPN and CD44 proteins resulting from shearing, glycosylation, phosphorylation, etc., their potential co-interacting partners such as heparin, hyaluronan and integrin αVβ3 also give rise to numerous interaction possibilities. Specifically, research evidence suggests that heparin binding on OPN plays a paramount role in its interaction with CD44 (17), which facilitates its interaction with homologous receptors, such as variant exon 3 on CD44 (CD44v3), thereby forming a molecular bridge. The distinct combinations need further investigation to understand how they produce different signals on receptor cells.
2 CD44 related molecular mechanisms
2.1 Molecular mechanisms involved in migration
CD44 is involved in the regulation of cell motility through intracellular structural domains, which constitutes a pivotal mechanism. There are three prevailing mechanisms involved: firstly, phosphorylation of the intracellular terminus of CD44 occurs following its interaction with HA and subsequent activation by protein kinase c (PKC) (18), and then ezrin, radixin, moesin (ERM) proteins serves as a link connecting CD44 and filamentous actin (F-actin) and ultimately influence cellular motility (19). Secondly, c-Src kinase is recruited to the CD44 site and activated through CD44-HA interaction (20). Activated c-Src increases tyrosine phosphorylation of a cytoskeletal protein called Cortactin (21). Consequently, its ability to cross-link F-actin decreases, which regulates cell migration capacity and promotes cell recruitment. Lastly, RhoGTPase triggers two different signaling pathways: firstly, it regulates F-actin via CDC42, thereby modulating the cytoskeleton and facilitating cell recruitment (22, 23). Secondly, it activates Rac1 to modulate the fold structure of the cell membrane in order to regulate cells (24). For example, CD44v3 has been discovered to promote Rac1 signaling by interacting with Tiam1, resulting in the cytoskeleton-mediated breast tumor cell migration (25). CD44-HA binding induces the aggregation of CD44 at the leading edge of cells, where it interacts with the actin cytoskeleton and its cytoplasmic tail (26). Activation of Rac1 through RhoA signaling has been proven to promote a shift towards a migratory phenotype once cells have attached to the endothelium. Rac1 activation plays a pivotal role in CD44-mediated cytoskeletal reorganization. When CD44 binds to c-Src, activated c-Src kinases phosphorylate Vav (mostly Vav1 and Vav2, two guanine exchange factors regulated by Rho GTPases) and consequently increase Rac1 expression (27). Under the help of F-actin, CD44 translocates from the rear end to the leading edge in this process. HA-CD44 binding promotes the combination of RhoA-specific p115RhoGEF with CD44 and further activates the RhoA-ROK signaling pathway. Finally, ROK phosphorylates ankyrin and enhances F-actin linkage (Figure 2) (28).
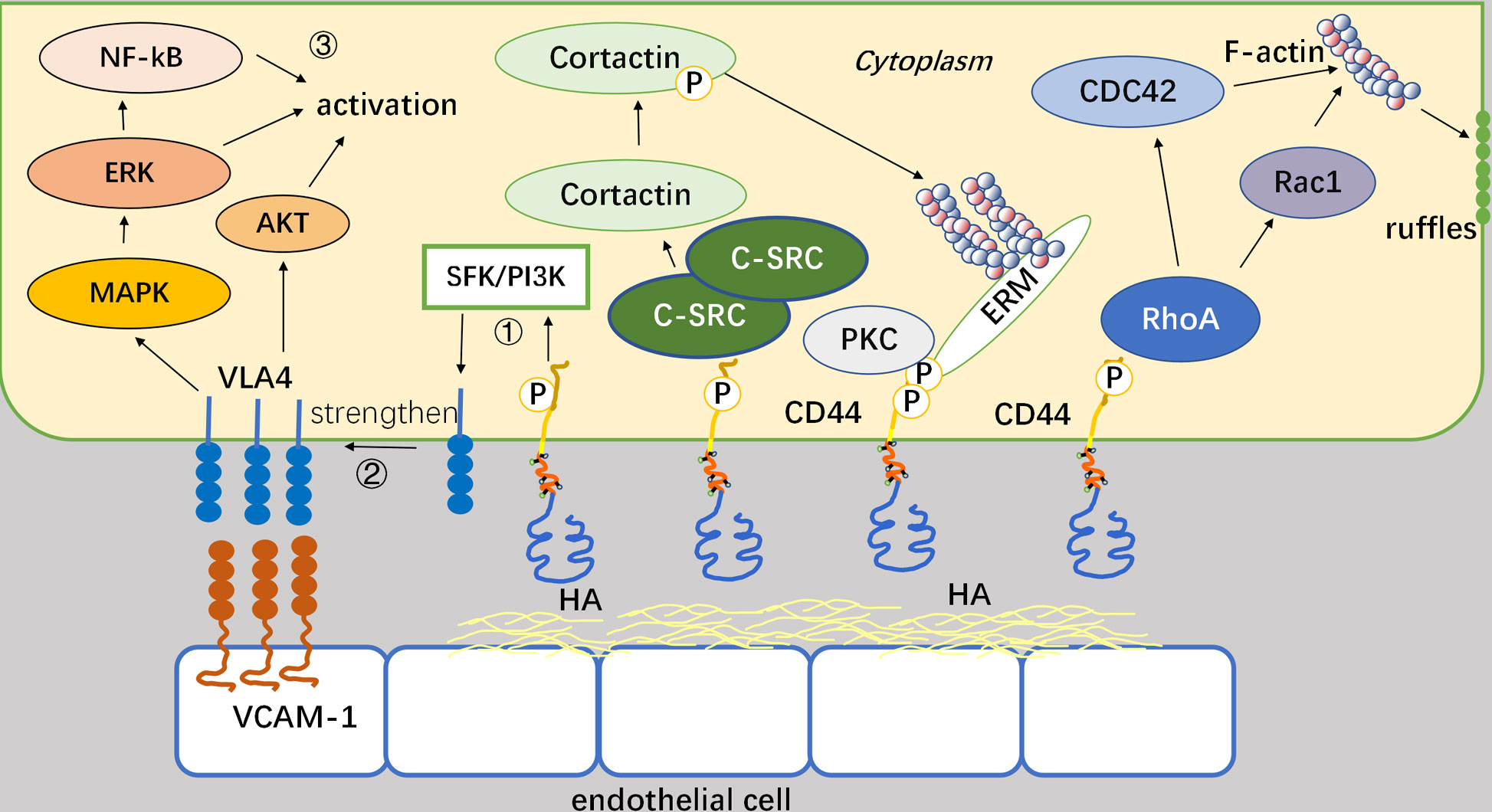
Figure 2 Mechanisms by which CD44 regulates the motility and adhesion. Binding of CD44 to HA can affect the intracellular system of backbone proteins and thus mediate cellular movement. CD44 connects to actin via the ERM as a bridge, and this process requires PKC activation as a prerequisite. It also recruits and activates c-Src, and regulates cell migration capacity by phosphorylating the actin Cortactin cytoskeletal protein tyrosine. After activation of Rho GTPase, two downstream factors, CDC42, and Rac1, regulate the cytoskeleton and cell membrane fold structure, respectively, thereby altering cell motility.
CD44 can synergistically interact with VLA-4 integrins through intracellular signaling pathways, aside from its significant adhesion role (29). VLA-4 is a heterodimer formed by CD49d and CD29 (30). Moreover, as a key coordinator of the interaction between leukemia cells and the bone marrow microenvironment, CD44 plays an important role in mediating cellular homing. In general, VLA-4 often interacts with VCAM-1, and its thermosensitivity and affinity for the corresponding ligands often undergo alterations upon exposure to external stimuli (31). CD44 can act as a regulatory factor in the above process. Specifically speaking, CD44 enhances VLA-4 activity by inducing intracellular molecular pathways through binding to HA or other extracellular matrices, which in turn enhances the adhesion of leukemia cells to VCAM-1. VLA-4 interacts with VCAM-1 to promote Akt, MAPK, NF-κB, and mTOR signaling, while reduce apoptosis in acute myeloid leukemia (AML) cells. Overall, CD44 modulates the affinity of VLA-4 in AML cells for VCAM-1 in peripheral blood and retains AML cells in the bone marrow microenvironment. In contrast to integrin VLA-4, CD44 can interact with integrin α6β4 to form a complex, which activate intracellular cytoskeletal proteins and their signaling pathways, thereby modulating the c-Src and Ras signaling cascade and promoting tumor cell migration (32). Notably, CD44-α6β4 can be delivered to the target region through the paracrine action of exosomes. Moreover, hepatocytes used in the experiments can selectively uptake tumor exosomes with high expression of CD44. Ultimately, this process supports tumor metastasis by stimulating cytokine production, pro-inflammatory factors release, and growth factor secretion for pre-metastatic niche formation. Although the mechanism of action regarding CD44 exosomes on leukemia cells still needs further research, it undoubtedly provides a new approach for targeting CD44 on leukemia.
Lipid rafts are cholesterol- and sphingolipid-rich microstructural domains in the plasma membrane (33). CD44 is predominantly localized in lipid raft clusters. Co-localization of CD44 in specific membrane environments is essential for cell adhesion and migration. The aggregation of CD44 by lipid rafts occurs through two main pathways (34). One type of palmitoylation retains CD44 in the lipid raft structural domain and partially inhibits its interaction with intracellular signaling molecules. However, another lipid knows as phosphatidylinositol-4,5-bisphosphate (PIP2) can expedite the process of CD44-junction complex formation (35). Several factors have been identified to influence lipid rafts. For example, methyl-β-cyclodextrin can deplete cholesterol and reduce CD44 aggregation on lipid rafts, thereby enhancing CD44 binding to HA on T cells. In addition, high levels of cholesterol can promote the entry of CD44 into lipid rafts, resulting in decreased binding between CD44 and Ezrin and subsequently inhibiting the migration and invasion of tumor cells (36).
Paxillin, an adhesive patch adaptor protein, is mainly localized at focal adhesions (FAs) (37). Cell movement and migration require the accumulation of paxillin at nascent FAs at the leading edge to recruit adhesion complexes, including the tyrosine kinases FAK, and elimination of FAs at the rear edge (38). The engagement of CD44 involves promoting tyrosine phosphorylation and activation of FAK under the premise of combination with HA. In the acidic milieu, hyaluronidase-2 and cathepsin B are activated to support ECM degradation and activate ROK, and subsequently induce the phosphorylation of Na+/H+ exchanger 1 (39).
2.2 Mechanisms involved in growth and proliferation survival
CD44 is capable of binding to ligands to activate a range of intracellular signaling pathways that regulate cellular growth, proliferation, survival and various other activities. Specifically, the interaction between CD44 and HA triggers the activation of Rho, which in turn stimulates the PI3K pathway and activates the downstream serine/threonine kinase Akt. Notably, HA establishes a positive feedback loop with Akt by inducing continuous activation of Akt signaling to counteract apoptosis and maintain cellular survival (40). CD44 also enhances cell proliferation by activating the p38 mitogen-activated protein (MAP) kinase (41). Furthermore, extracellular kinases can be involved in regulation, such as extracellular-associated kinases 1 and 2 (ERK1/2), which regulate the activation and proliferation of endothelial cells. Activated ERK2 can promote cell migration and proliferation by phosphorylating EIK-1 (23). CD44v6, as a tumor marker in the CD44 family, forms a trimeric complex with MET and HGF to promote MET activation and activate three downstream pathways: RAS-MAPK activation, PI3K/Akt promotion, and MET transcription enhancement (Figure 3A) (42). Among signaling molecules, ERM and Merlin are commonly recognized as pivotal regulators of cell proliferation, and Merlin and ERM engaging in competitive binding for the CD44 intracellular site (43). Upon activation, Merlin facilitates cytoskeletal reorganization while concurrently suppressing RAS activation and downstream tyrosine kinase signaling. Conversely, phosphorylation-induced inactivation of Merlin impedes its interaction with CD44 (Figure 3B) (44).
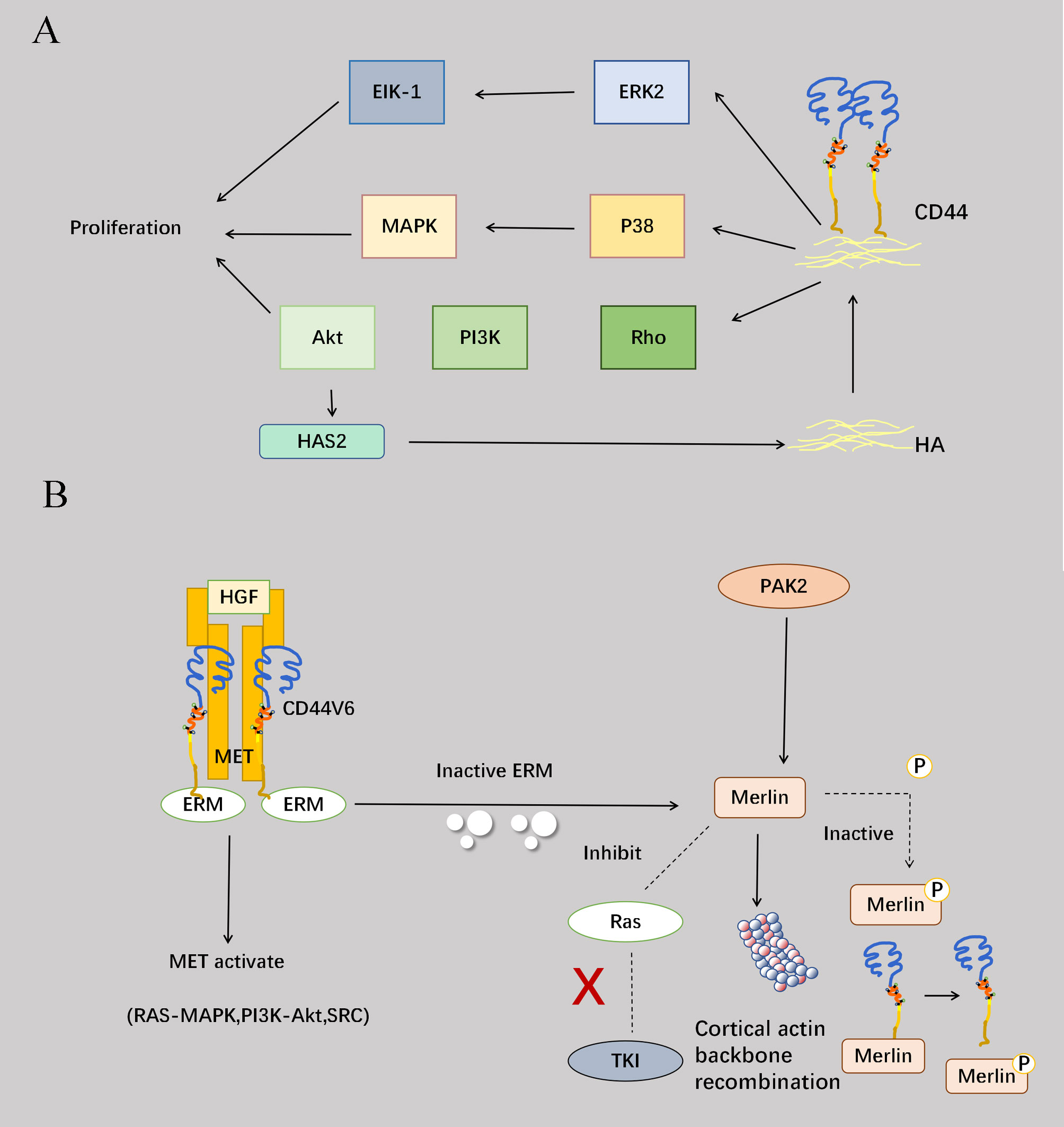
Figure 3 Involvement of CD44 in proliferation. (A) Binding of CD44 to HA can activate the PI3K-Akt pathway through RHO, and the activation of the Akt pathway can promote the increase of HAS2 synthase, which in turn can enhance the effect of CD44 and HA to form a positive cycle, overcoming apoptosis and maintaining cell survival. CD44 also binds to HA and activates the P38-MAPK pathway to enhance cell proliferation. Activation of the extracellular kinase ERK2 phosphorylates EIK-1 and ultimately promotes cell migration and proliferation. (B) CD44v6 forms a trimeric complex with Met and HGF and promotes Met activation. Furthermore, the interaction of the CD44 cytoplasmic tail with ERM proteins is required to activate the Ras-MAPK pathway. CD44v6-ECM binding also promotes PI3K/Akt pathway activation and Met transcription. Another protein, Merlin, competes with ERM at the intracellular end of CD44, and Merlin activation occurs after ERM protein inactivation. Merlin activation causes reorganization of the cortical actin cytoskeleton, prevents Ras activation and Ras-dependent signaling, and inhibits signaling by various receptor tyrosine kinases. The serine/threonine protein kinase PAK2 phosphorylates Merlin, but also leads to Merlin inactivation and inhibits its binding to CD44. The addition of high cell density or high molecular weight HA triggers the dephosphorylation of Merlin, leading to the formation of growth inhibitory complexes that limit cell proliferation. Therefore, ERM and Merlin proteins act as “switches” to control cell proliferation.
2.3 CD44 cleavage
Proteolytic cleavage of CD44 is a key regulatory event dependent on CD44 cell-matrix interactions and signaling pathways. The process of matrix metalloproteinase (MMP)-mediated shearing of the CD44 extracellular structural domain to cleavage of the intracellular structural domain to the final release of intracellular structural domain fragments (ICDs) to act as signaling transcription molecules is strictly sequential (45). After cleavage of the exo-structural domain, the presenilin-dependent-γ-secretase-dependent shearing within the membrane is initiated. Finally, the intramembrane cleavage product CD44-ICD can act as a signal transduction molecule that is translocated to the nucleus and activates transcription. Exo-structural domain cleavage of CD44 correlates with malignancy in human tumors (46). Extracellular soluble CD44 (sCD44) is a competitive inhibitor of endogenous HA-CD44 interaction (47). The main MMPs involved in exon cleavage are MT1-MMP, ADAM10 and ADAM17. Specifically, MT-1-MMP binds to CD44 through the PEX (C-terminal hemopexin-like domain) structural domain and localizes to the ciliary membrane (48). CD44 acts as a linker between MT1-MMP and the actin cytoskeleton in inactive cancer cells. Co-activation of the molecules PKC and RAC induces the aggregation of ADAM17 and CD44 at the leading edge of migrating cells, and accumulation of ADAM17 is activated and undergoes cleavage of the CD44 ecto-structural domains allowing the cells to crawl efficiently on the ECM, with the extended lamellae also inducing mechanical stretching of the cells (49). Extracellular calcium ions flow into the cell through stretch-activated calcium channels. With the accumulation of intracellular calcium ions, ADAM10 is rapidly activated to aid CD44 cleavage. CD44 cleavage promotes attachment of newly synthesized CD44 to the ECM. The release of sCD44 from the C-terminus triggers the intramembrane cleavage of CD44 ectodomain cleavage, a process dependent on presenilin-dependent-γ-secretase. Finally, CD44-ICD translocates to the nucleus and activates the transcription of various genes including CD44. The activation of CD44-associated proteases can facilitate the movement of cells (50). Therefore, profound understanding the underlying mechanisms of CD44 cleavage could develop new therapeutic approaches for cancer cell invasion and metastasis. Elevated levels of sCD44 in plasma are correlated with malignant diseases and immune activation. Additionally, competition between the ectodomain released by the receptor and cell surface CD44 can antagonize the effect of membrane-bound CD44, which might also shed light on CD44-related treatments (Figure 4) (51).
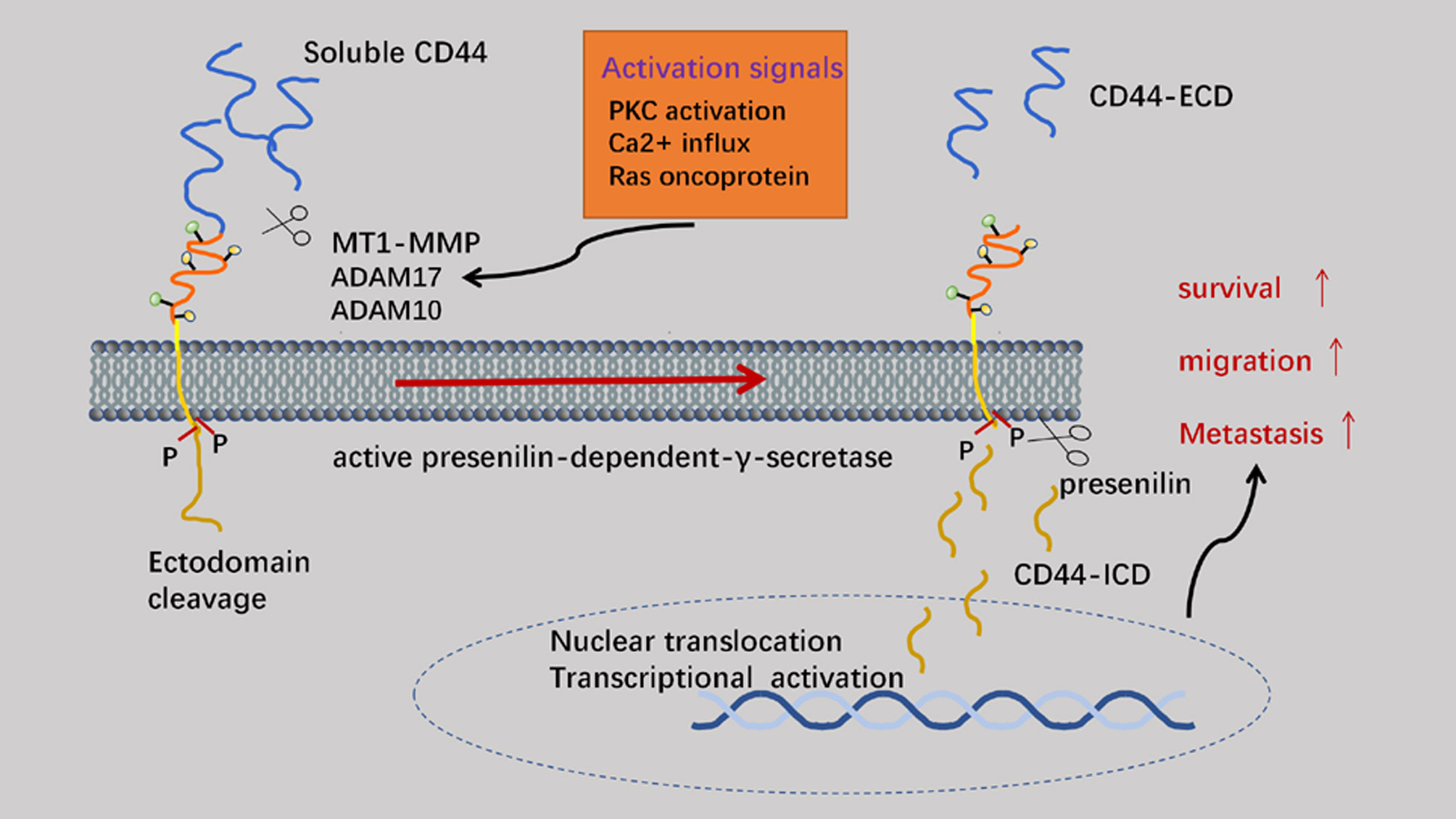
Figure 4 The cleavage of CD44. The ectodomain of CD44 is mainly cleaved by MMP (membrane-associated metalloproteases) to generate CD44 ECD. On the premise of previous process, CD44 ICD can be generated by intramembranous cleavage, which mediated by presenilin (PS)-dependent-γ-secretase. CD44 ICD can act as a signal transduction molecule to activate transcription.
2.4 Molecular mechanisms involved in the metabolism of CD44
CD44 is capable of binding to copper and facilitating its internalization into the cellular interior (52). Cu(II) can exert influence on cellular metabolism and function through diverse pathways, such as involvement in macrophage activation and inflammatory responses. During macrophage activation, upregulation of CD44 leads to an elevation in Cu(II) levels. Additionally, Cu(II) also catalyzes the NAD(H) redox cycle, which promotes metabolic changes and subsequent epigenetic alterations leading to an inflammatory state. This mechanism has been more extensively studied in Wilson and Menkes disease, characterized by impaired copper transporters leading to potential copper accumulation and toxic effects, along with other chronic metabolic diseases (53), further verification is required regarding its precise mode of action on leukemia cells. Moreover, CD44 plays a crucial role in regulating adipogenesis and adipocyte function through PPAR-γ and cell cycle-related pathways. The elimination of CD44 improves adipose tissue inflammation and insulin resistance in obesity. CD44 also regulates glucose metabolism in various cancer cells (such as PC-3 cell line and small cell neuroendocrine carcinoma cells), while also modulating ROS levels and cellular proliferation within neoplastic cells (54). Finally, CD44 is implicated in the regulation of cellular autophagy by downregulating PIK3R4 and PIK3C3 levels through CD44-ICD and disrupting the STAT3-dependent PtdIns3k complex to exert negatively modulate on autophagy (55).
3 The role of CD44 in leukemia
3.1 Expression of CD44 and its variants in leukemia cells
Leukemia is a hematological disorder characterized by diminished levels of normal blood cells and impaired bone marrow function due to uncontrolled proliferation of leukemia cells within the bone marrow. The expression of CD44v is exclusively detected in leukemia cells, while being absent in bone marrow, peripheral blood, and CD34+ hematopoietic stem cells (HSCs). The complexity of CD44v expression in leukemia cells has been associated with low remission rates and high relapse rates during treatment, indicating an unfavorable prognosis. In addition, CD44v expression in leukemia cells is influenced by numerous factors, including inherent individual variations that are common and unavoidable, as well as the specific type of leukemia (e.g. the CD44v7 expression level is lower on lymphocytes than monocytes and granulocytes) (56). Additionally, some leukemia FAB subtypes, specifically AML M3 and M5 subtypes, exhibit elevated levels of CD44v expression in conjunction with genotype variations. For instance, individuals carrying the rs13347 TT and CT genotypes demonstrate higher CD44v expression level compared to those with the rs13347 CC genotype (57). The presence of various complex patterns of CD44 variant exons, particularly exon v6, has been observed in the majority of leukemia samples (58). The combination of CD44v6 and HA enhances cell resistance to apoptosis and activates the PI3K/Akt signaling pathway (42). Upregulation of CD44v6 can also elevate the expression of HA synthase gene. Due to its widespread presence and varying expression levels at each stage of leukemia development and across diverse subtypes of leukemia, CD44 has been considered as a valuable biomarker (Table 1). In the field of leukemia treatment, CD44 can also be applied for universal minimal residual disease (MRD) monitoring, as its high expression levels are always associated with poor prognosis (91). This phenomenon may be related to the reciprocal interaction between CD44 protein expression and oncogenes (92).
3.2 CD44 mediates the rolling of blood cells
Different types of selectins typically act at different stages of cell rolling as important molecules located in endothelial cells for interaction with CD44. For example, P-selectin is known to affect the fast roll of the prophase, while E-selectin mediates CD44 engagement during the slow rolling of neutrophils (93, 94). Furthermore, in various T cell conditions, CD44 collaborates with other ligands like HA and integrins to facilitate the rolling and adhesion of T cells. Moreover, CD44 plays a pivotal role in promoting the recruitment of neutrophils and macrophages to inflammatory sites.
3.3 CD44 correlates with chemoresistance
As an important prognostic indicator, the upregulation of CD44 exhibits statistically significant implications in the cell fate of many cancer cells, including leukemia cells. Moreover, it has been observed that drug-resistant cells tend to display elevated levels of CD44 expression (95). Functionally, CD44 interacts with the extracellular matrix, particularly HA, to activate Nanog through some key signaling pathways, such as the Wnt/β-catenin, and PI3K/Akt (96), the targeted Nanog has been utilized as a mediator to enhance the expression of drug resistance genes. CD44v6 is considered a premetastatic niche of tumor cells and correlates with drug resistance (97). CD44v3 has been implicated in the regulation of chemotherapy resistance through the Oct-Sox2-Nanog signaling pathway (98). It is noteworthy that HA of different sizes has diverse effects on CD44-mediated drug resistance. Low molecular weight HA binding to CD44 tends to induce the internalization of MDR, while high molecular weight HA binding to CD44 promotes the expression of MDR on cell membrane (99).
3.4 CD44 is involved in proliferation and anti-apoptosis of leukemia cells
The prognosis is inversely correlated with the expression levels of CD44 in leukemia cells and their rate of increase; however, further evidence is needed to fully support the underlying mechanism. It has been discovered that CD44 promotes the proliferation of leukemia cells through upregulating expression of anti-apoptotic protein myeloid cell leukaemia-1 (MCL-1) (100). Chang et al. found that the downregulation of CD44 led to a decrease in β-catenin expression, thereby inducing leukemia cell cycle to arrest in the G0/G1 phase, where gene transcription is blocked, and cell proliferation is inhibited (101). Consequently, it can be inferred that CD44 exerts regulatory control over leukemia cells through the Wnt/β-catenin pathway.
4 CD44-related measures applied in clinical treatment
CD44 is frequently overexpressed in the tumor stroma across various malignancies, and both neoplastic cells associated CD44 and HA within the stromal microenvironment have been targeted for anti-cancer therapy. Current perspectives on the treatment of CD44 can be broadly categorized into two domains. One approach focuses on directly targeting CD44 itself (Figure 5A), considering its involved in the self-regulatory activities of tumor cells such as proliferation, anti-apoptosis, drug resistance and other mechanisms. Targeting CD44 hinders relevant signaling pathways associated with these activities. Moreover, as an adhesion molecule, CD44 is involved in cell adhesion and chronic rolling to retain tumor cells in the appropriate microenvironment. By blocking CD44 binding to ligands, it facilitates tumor cells evading the tumor microenvironment for better exposure to drugs and immune cellular strikes - particularly crucial for eliminating residual leukemia stem cells (LSCs) after HSC transplantation to reduce postoperative recurrence (1). The other category involves the utilization of CD44 as a more accurate drug delivery channel and tumor cell localizer, considering its robust affinity for HA and the properties of the HA molecule itself (102). Even in passive immunotherapies including CAR-T cell therapy, CD44v6 is considered a viable T-cell localization molecule due to its high expressivity in AML cells. Specifically, for the first approach to CD44 itself, it is common to develop monoclonal antibodies about CD44 or chemotherapeutic agents that inhibit the binding of CD44 to HA by utilizing sCD44 and specific molecular sizes of HA as inhibitors. Alternatively, drug delivery via CD44-HA has gained significant attention in recent years, particularly regarding the utilization of HA as a special material for nanoparticle modification and its application as an intermediary bridge facilitating communication between the drug and CD44-overexpressing tumor cells (Figure 5B).
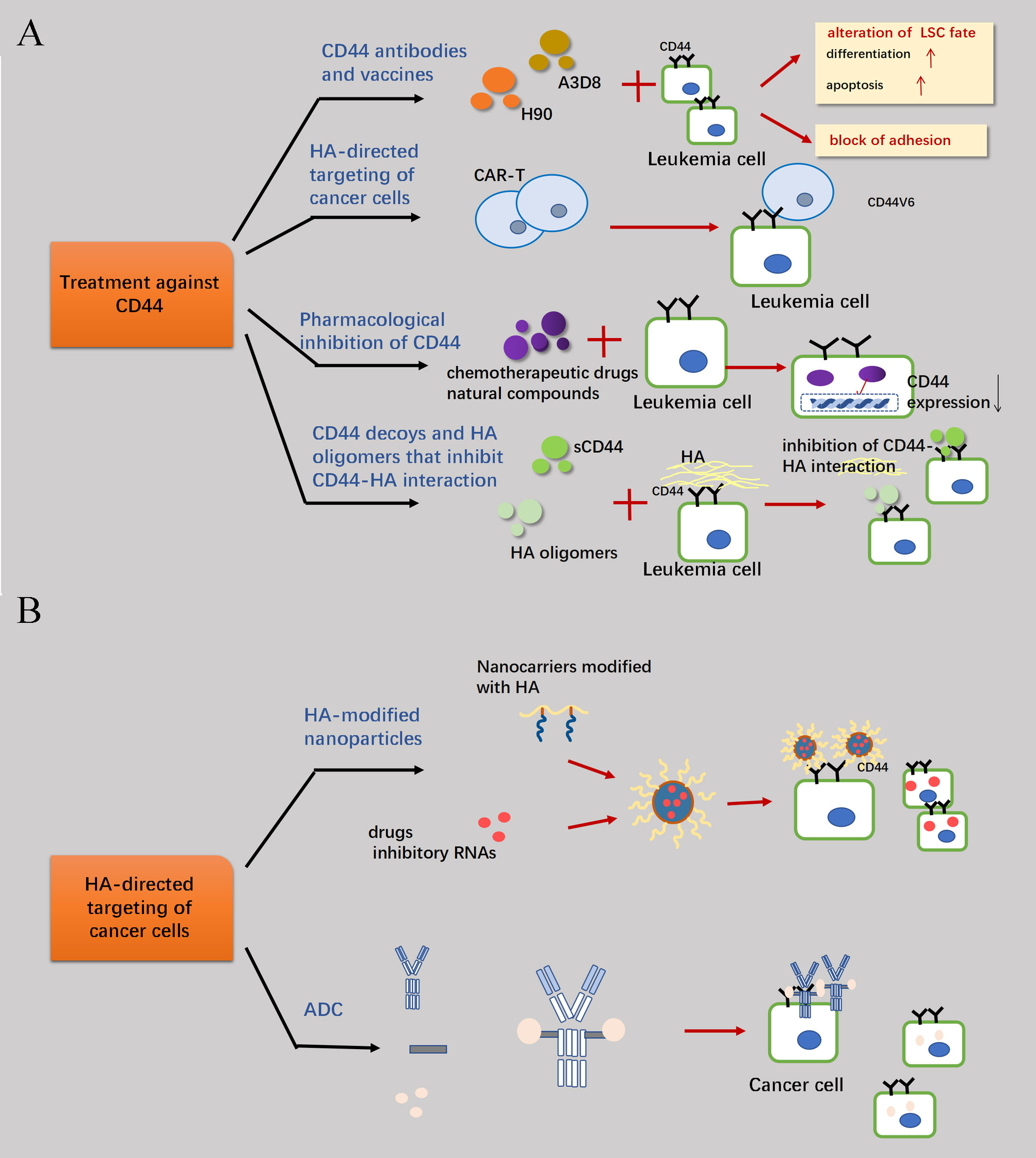
Figure 5 Therapeutic measures concerning CD44. (A) Because of the high expression of CD44 molecules in target cells and their involvement in mechanisms such as anti-apoptosis of tumor cell proliferation, blocking the signaling pathways of relevant cellular activities by combating CD44 is a feasible therapeutic strategy. Primary treatments are the development of CD44-targeted drugs, immune-representative therapies such as the elimination of AML cells through CAR-T cells which associated with CD44v6 and the enhancement of therapeutic efficacy through the screening of drugs that can reduce the transcriptional expression of CD44 in combination with conventional chemotherapeutic agents. Finally, the binding of CD44 to HA can be interfered with by sCD44 and HA oligomers. (B) The high affinity between CD44 and HA can also be an important channel for drug delivery into the cell. Through the HA linkage, CD44 antibodies can be combined with drugs to form antibody-drug conjugates (ADCs), which can more efficiently target and attack highly CD44-expressing tumor cells.
4.1 CD44-targeted drugs
Depending on their chemical structure, CD44-targeted drugs can be classified as monoclonal antibodies (mAbs) or small molecule compounds. The overexpression of CD44 variant isoforms on the surface of tumor cells, coupled with the ability of cellular overexpression of CD44 to increase the secretion of cytosolic HA and create a favorable microenvironment for the tumor cells, positions CD44 as a promising target molecule. CD44 antibodies have two distinct mechanisms of action: alteration of LSC fate, including inhibition of LSC differentiation blockade and disruption of LSC adhesion. The anti-CD44 mAbs H90 and A3D8 not only inhibit the proliferation and self-renewal of LSCs, but also promote leukemia cell differentiation and induce apoptosis. A3D8 mAb induces apoptosis via the engagement of the serine protease-regulated pathway (103). Notably, despite these two antibodies share a common target protein, they exhibit distinct mechanisms of action (104). Furthermore, the combination of A3D8 and H90 effectively induces terminal differentiation of leukemic blasts in AML-M1 to AML-M5 subtypes, which are the most prevalent variants (105). A3D8 inhibited the proliferation of HL-60 cells, and its differentiation mechanism was associated with the up-regulation of p21 cip1 expression and downregulation of cyclin D1 and CDK4 expression (106). The proliferation inhibitory effect of A3D8 on HL-60 cells related to the downregulation of amino acid terminal kinase expression. Another familiar antibody called RG7356 (a recombinant anti-CD44 IgG1 humanized mAb) elicits caspase-dependent apoptosis in leukemic cells (107). In most practical clinical applications, antibodies are utilized in combination with drugs to potentiate their effects. The combined treatment with cyclopamine (a plant-derived steroidal alkaloid) and CD44 antibody effectively enhances the therapeutic effects of inducing the differentiation of leukemia by hindering the Hedgehog signaling pathway (108). The addition of anti-CD44 mAbs provides benefit to “ATRA-FICZ” (FICZ: 6-Formylindolo (3, 2-b) carbazole) enhances ATRA-induced differentiation therapy, especially in APL patients (109). Nonetheless, careful consideration should be given to the sequential administration of antibodies and chemotherapeutics. For example, A3D8 mAb entirely eliminates the ceramide, a lipid second messenger participating in the apoptotic signaling pathway triggered by daunorubicin. Therefore, administering anti-CD44 mAbs after apoptosis-inducing drugs can avoid interfering with their original effects.
Undoubtedly, the development of targeted drugs encounters numerous challenges. The meticulous identification and validation of novel drug targets and their precursors, along with the arduous process of developing targeted drugs with extensive research and development cycles, have contributed to the issue of exorbitant pricing. Furthermore, while targeted drugs are exhibiting efficacy against tumors with high specific expression of certain oncogenes, they demonstrate limited effectiveness against other tumor types. Although the precise mechanisms underlying drug resistance remain incompletely elucidated, it is widely acknowledged that mutations in genes or prolonged drug exposure may cause drug resistance; additionally, epigenetic or cytokine abnormalities might also induce such resistance (110). Furthermore, targeting CD44 will inevitably have some cytotoxicity due to its expression on immune cells, such as NK cells, and CD8+T cells (111). A commonly employed approach to address the aforementioned challenges is protein-targeted chimerism (PROTAC) (112), a method that can transform most non-druggable protein targets into druggable ones. In addition to PROTAC, subsequent advancements have been made in autophagosome-targeted complexes using lysosome-targeted technology and autophagosome-targeted degradation by autophagosome-bound compounds since 2019 (113). In addition, progress has been made in the development of dual-targeted or multi-targeted drugs. Malignant tumors are intricate network regulated by multiple factors with diverse etiologies, merely intervening in one target or inhibiting a pathway following the strategy of a single-target drug triggers activation of another related pathway, leading to suboptimal efficacy of the single target and rapid development of resistance. Therefore, apart from solely targeting CD44, attempts should be made to simultaneously target another highly characterized molecule overexpressed in tumor cells to mitigate the rate of resistance and improve targeting precision. Given the vast number of compounds and target molecules, relying solely on traditional manual screening techniques to ensure efficiency and accuracy becomes challenging. Therefore, leveraging artificial intelligence and big data technologies have proven to be beneficial in this regard. Secondly, regarding the issue of CD44 toxicity, it has been previously mentioned in section 3.1 that distinct subtypes of CD44 are highly expressed by different leukocyte species and different leukemia subtypes. Although NK cells possess the capability to exhibit elevated levels of CD44 expression, and it has been demonstrated that signaling through CD44 enhances cytotoxicity in NK cells. Additionally, expression of CD44-positive liver-resident CD8+ T cells was also found to be critical for immune clearance of hepatitis B virus. However, resting NK cells constitutively express inactive forms of CD44 which do not bind to HA. In addition, pro-inflammatory cytokines play an important role in activating CD44 on NK cells. Although stimulation of resting NK cells with interleukin-12 (IL-12) or IL-18 resulted in increased CD44 expression, only IL-2 or IL-15 led to upregulation and activation of CD44 (111). Cytokine-induced upregulation and activation of CD44 is not associated with NK cell proliferation. That is, HA alone exerts minimal impact on IFN-γ production, whereas low molecular weight HA effectively enhances of IFN-γ production when combined with IL-2, IL-12, or IL-18. Exploiting the unique properties of HA and its association with CD44 holds promise for modifying NK cells and subsequently activating their recognition mechanism to expedite solid tumor treatment, offering a prospective avenue for NK cell-mediated immunotherapy.
4.2 Pharmacological inhibition of CD44
Except for directly targeting the interaction of HA and CD44, several natural compounds or chemotherapeutic drugs have been discovered to indirectly inhibit LSCs through reducing CD44 expression. ATRA and hexamethylene bisacetamide have been proven to induce differentiation while simultaneously downregulate the expression of CD44 (114), suggesting that the decrease of CD44 may participate in the therapeutic effect of chemotherapeutic drugs. Furthermore, it has been observed that certain drugs with similar functions may exert their effects through different mechanisms. The regulation of CD44v6 expression by ATRA differs from that of As2O3 (115). Specifically, in the process of ATRA-induced differentiation, CD44v6 transcript levels are downregulated, while maintain CD44v6 translation levels at their original levels; concurrently, the PI3K/Akt signaling axis is strengthened. Conversely, during As2O3-initiated differentiation, both transcriptional and translational levels of CD44v6 are strikingly downregulated and the PI3K/Akt pathway is blocked. The other natural compounds and chemotherapeutic drugs have been listed in the Table 2.
4.3 The exploration of sCD44 and HA oligomers
The sCD44 present in body fluids functions as a competitive inhibitor, counteracting the effect of membrane-bound CD44 and providing valuable insights for CD44-relevant treatments. Previously, sCD44 was primarily utilized for disease monitoring, especially as a prognostic indicator in AML patients. However, recent studies have implicated that sCD44 is involved in the pathogenesis of B-CLL, and propose inhibition of sCD44 as a potential therapeutic strategy (67).
The use of HA oligomers is an alternative approach to inhibit HA-CD44 interaction. It has been demonstrated that monovalent interaction with small oligomers of HA (6–18 saccharide units of HA) effectively reverse the function exerted by high molecular weight HA. Furthermore, this mechanism efficiently inhibits the CXCL12-regulated CXCR4 signaling pathway (129).
4.4 Effects of CD44 on the hematopoietic niche
The eradication of residual LSCs following chemotherapy or HSC transplantation is pivotal in preventing leukemia relapse, while the protective role of the hematopoietic niche shields leukemia cells from the cytotoxic effects of chemotherapy drugs. Adhesion is a critical part of normal hematopoiesis and helps LSCs to remain sheltered in the bone marrow microenvironment (130). Its involvement in chemotherapy resistance or relapse after remission poses a significant challenge for treatment. Downregulation of cell-surface adhesion molecules can liberate LSCs from the hematopoietic niche and augment the efficacy of chemotherapeutic interventions. The initiation of HA-CD44 binding is responsible for the involvement of CD44 in the increase of additional adhesion molecules (mostly integrins) and the enhanced adhesion of α4β1 (an integrin) to fibronectin and laminin. An in-depth study of the mechanism of action of adhesion molecules would facilitate the identification of a therapeutic agent that impedes aberrant adhesion between LSCs and the hematopoietic niche, thereby promoting their differentiation, proliferation, and migration outwards. Although LSCs and HSCs share the similar adhesion molecules and signaling pathways in most cases, further research reveal that the adhesion mechanisms of HSCs and LSCs do not completely overlap. Several studies have suggested that BCR-ABL1+ LSCs exhibit a greater reliance on selectins and their ligands for homing compared to HSCs (131). Selectin blockade may therefore be a beneficial strategy in the leukemia treatment. CD44 interacts with E- and L- selectin when it is sialo-fucosylated and bears the SleX glycan (132). In vivo administration of H90, an activating mAb directed to CD44, has demonstrated the ability to enhance the adhesion of normal HSCs to HA, while concurrently inhibiting the binding of LSCs to HA, and thereby significantly reduce leukemia repopulation (103).
Abnormal glycosylation is a characteristic of cancer cells, and several changes in glycan structure are associated with cancer progression. Selectin-ligand interactions are involved in cancer cell interactions with platelets, leukocytes, and endothelial cells, and as well as promoting tumor cell dissemination through signal transduction pathways. Moreover, selectin-mediated specific interactions between host cells expressing selectins and ligands on tumor cells can block the microvascular system. Inflammatory cytokines produced by cancer cells have been shown to stimulate high expression of E-selectin during early stages of cancer progression (133). CD44v4 is an E-selectin ligand expressed in metastatic breast cancer, playing a crucial role regulating the interaction between cancer cells and endothelial cells through E-selectin. This regulatory mechanism promotes the trans-endothelial migration of cancer cells. In addition, the loose microenvironment and formation of pre-metastatic niches are reported to be critical for the establishment of metastasis and are the reason why circulating cancer cells can colonize distant organs. Selectins and their respective ligands also help maintain the structural integrity of the pre-metastatic niches.
Relevant methods have been explored, encompassing modulation of selectin-ligand interaction, alteration of selectin expression to modify the biosynthesis or cleavage of selectin ligands. Other strategies can also be considered in conjunction with selectin blockade for targeted attack on cancer cells while minimizing interference with normal HSCs, such as immunophenotype transformation, alteration of cell metabolism, epigenetic regulation, and modification of the cellular microenvironment.
Regarding the potential side effects of such interventions, when selectins mediate metastasis through the activation of the inflammatory cascade and participating in shaping the tumor microenvironment, they also help identifying and eliminating tumor cells. The dual functionality of selectins poses challenges for developing effective inhibitors targeting selectin-ligand interactions in vivo. On one hand, excessively potent inhibitors may exert detrimental effects on the healing process. On the other hand, weak inhibitors may fail to sufficiently intervene in the pathological processes of serious diseases. Thus, an effective therapeutic agent necessitates a delicate balance in selectin-ligand interactions. Furthermore, structural features of the calcium-dependent carbohydrate recognition domain with a relatively shallow surface pose a challenge to the rational design of selectin inhibitors. Numerous sugar-like or non-carbohydrate and polysaccharide inhibitors have been developed to inhibit selectin-ligand interactions, but despite considerable effort, only a few compounds have shown promising results in clinical trials.
Finally, implementing treatment at different stages of treatment can also significantly influences the outcome, with particular emphasis on targeting LSCs during the initial complete remission (CR) phase when they exhibit greater homogeneity. The limited efficacy of LSC-targeted therapy against a vast population of leukemia cells renders it more advantageous in scenarios characterized by lower tumor burden and reduced tumor heterogeneity.
4.5 Precise immunotherapeutic potential of CD44
Immunotherapy refers to the enhancement of therapeutic effects by boosting the anti-tumor immune response and overcoming immune tolerance towards tumor. Moreover, Immunotherapy encompasses not only the manipulation of the tumor microenvironment but also entails regulation of peripheral immune cells. Broadly categorized, immunotherapy can be classified into three types: active immunotherapy, which involves administering low-toxicity tumor vaccines to patients; passive immunotherapy encompassing CAR-T cell therapy or passive delivery of immunosuppressants or anti-tumor cells to patients; and the third type is immunomodulatory site therapy, specifically inhibitors of immune checkpoints or inhibitors of immunomodulatory sites. PD-1 serves as an important immune locus, and inhibition of the PD-1-PD-L1 axis not only blocks immunosuppressive response within tumor cells, but also induces an anti-tumor response in peripheral immune cells (134). CD44 has been proven to promote immune escape in various types of tumor cells, with its high expression being associated with the upregulation of immune escape-related marker proteins (135). In lung cancer, binding of CD44 to Secreted Phosphoprotein1 (SPP1) blocks T-cell proliferation (136). CD44 binds more readily to acetyl HA on antigen-activated T lymphocytes and inflammation-stimulated monocytes (137). Due to its ability to induce immune escape, PD-L1 is targeted by multiple anti-tumor drugs; furthermore, CD44 is positively correlated with the expression of PD-L1 (138). In addition, CD44 may serve as an independent prognostic factor for immune invasion (139). CD44v6 is expressed in a variety of AML cells and is an essential molecule for the proliferation of leukemia cells, while in healthy individuals, HSCs and lymphocytes express minimal levels of CD44v6, which is exclusively found in monocytes. Ex vivo and in vivo experiments have demonstrated that CD44v6 CAR-T cells selectively eliminate AML cells while sparing HSCs but targeting monocytes (140).
4.6 HA-directed targeting of cancer cells
The development of drugs centered on HA is predicated upon its high affinity for CD44, excellent biocompatibility, and biodegradability (141). HA-mediated targeted therapy can be conceptualized as a therapeutic method that capitalizes on the specific binding between HA and CD44. In this strategy, CD44 serves as the target receptor for interaction while drug-conjugated HA acts as the carrier to transport drugs. By exploiting the specific binding capability, the drug-loaded HA facilitates targeted delivery to cancer cells expressing high levels of CD44. Common applications include HA-modified nanoparticles, HA-linked drug conjugates, and HA-liposomes.
The HA-modified nanocarriers based on HA exhibit non-toxic, non-immunogenic and water-soluble attributed to the inherent characteristics of HA itself. Additionally, nanoparticles possess advantages such as small particle size, high drug loading capacity, controlled drug release, efficient tumor targeting ability, and prolonged in vivo circulation time (142). The CD44 receptor specifically binds with high affinity to its natural ligand HA. By considering the chemical structure of the drug itself alone with the specificity of the target site and the affinity towards cell surface molecules, a combination approach is often employed for treating malignancies with overexpression of CD44. The modification of nanoparticles, such as liposomes, carbon nanotubes, and dendritic macromolecules with HA as a surface modification material, can improve the targeting efficiency of nano formulations and relatively prolong the in vivo circulation time of drugs (143).
Furthermore, ongoing research is being conducted on the advancement of nanocell and nanogel technologies. The principle of HA nanomicelles is based on the existence of multiple active functional groups in the chemical structure of HA enabling modification of various hydrophobic chains onto or at the end of the HA skeleton to obtain amphiphilic HA derivatives. These derivatives can self-assembled into solution-phase micelles with a core-shell structure in solution, facilitating encapsulation of poorly water-soluble anti-tumor drugs within the hydrophobic core. This approach significantly enhances drug solubility and stability. Nanogels are prepared by cross-linking sulfhydryl groups in HA-SH to form disulfide bonds. Small interfering RNAs (siRNAs) can be physically encapsulated into the nanogels formed by HA-SH under ultrasonic conditions using the reverse water-in-oil emulsion method. In addition, owing to the presence of disulfide bonds inside the nanogel structure, it enables rapid release of encapsulated siRNAs under high GSH concentration conditions (intra-tumor cell GSH concentration) improving gene silencing efficiency.
HA-drug couplers are synthesized as prodrugs through the formation of cleavable chemical bonds between a drug and HA, which improve the solubility and pharmacokinetic properties of the drug, as well as facilitating its cellular uptake by CD44 receptor mediated endocytosis. The interaction of CD44 and HA can be exploited as a promising therapeutic target for targeted drug delivery due to its high affinity. The typical composition of antibody-drug conjugates (ADCs) include three main components: (1) a mAb with the function of accurately selective location; (2) an effective cytotoxic small molecule; and (3) a linker responsible for the connection between two substances (mainly liposomes, hydrogels, and nanoparticles) which has been proven to promote the therapeutic effects under the same amount of the chemotherapeutic drugs and minimize the systemic toxicities to some extent. The results may be related to the mechanism by which the high affinity between HA-decorated liposomes and specific receptors leads to fully internalization of drugs. Besides, compared with other normal ADCs, HA can be exploited to entrap and transport drugs to CD44-expressing tumors without the requirement of chemical conjugation due to its enormous hydrodynamic domain. The drug-antibody ratio, or the amount of drug molecules attached to a single ADC, is an important indicator for assessing the function and toxicity risk of ADC drugs. High drug loads affect toxicities and pharmacokinetics while low drug loads may diminish potency. In addition, the systemic stability of ADCs after administration encompasses metabolic stability and integrity, which are another important factor determining their efficacy. Consequently, targeted research has focused on stabilizing ADCs through conjugate site selection and joint modification. The selection of attachment sites with larger steric hindrance has been proven to be an effective approach for providing the desired spatial shielding for antibodies. Conversely, introducing proximal steric hindrance around the splice or instability sites of the joint can also effectively improve stability. Other novel ADCs include immunostimulants, such as toll-like receptor agonists, STING agonists or chemokines, which better attract immune cells to tumor cells and further enhance immune cell activities. However, it should be noted that the distribution of cytotoxic drugs is mostly determined by the level of target antigen expression, which can occasionally result in unexpectedly severe “targeted, detumorable” toxicity that is not strictly proportional to the payload. Furthermore, similar to other pharmaceutical agents, the active cytotoxic components carried by ADCs undergo metabolic changes. Thus, the risk of drug interaction and other allergies should be considered, and necessary measures should be taken to eliminate relevant side effects.
5 Conclusion
CD44, a significant biomarker, serves as a platform for intercellular communication and intracellular signaling pathways that play a crucial role in regulating cellular behavior. It is also an established component of the LSC niche, which represents a promising target for anti-cancer therapy. Several strategies have been applied to inhibit CD44 for cancer therapy, including HA nanoparticles, small-molecule inhibitors, and anti-CD44 mAbs. These approaches are showing promising results in preclinical research. Among the various mechanisms reversed by anti-CD44 antibody, the inhibition of LSC differentiation has attracted more interests due to its crucial role in mediating HSC engraftment into the bone marrow niche. As LSCs share most of the same mechanisms as HSCs, finding ways to selectively target LSCs without disrupting HSC function has become a pressing issue. In conclusion, targeting CD44 represents a promising therapeutic strategy for controlling the progression of AML. Therefore, it is imperative to comprehensively elucidate the mechanisms underlying HA-CD44 interaction and signaling in order to identify rational treatments that specifically target CD44 and enhance therapeutic efficacy.
Author contributions
SW: Writing – original draft. YT: Writing – original draft. FL: Writing – review & editing. YH: Writing – review & editing. SZ: Conceptualization, Supervision, Writing – review & editing. XL: Visualization, Writing – review & editing.
Funding
The author(s) declare financial support was received for the research, authorship, and/or publication of this article. This work was supported by National Natural Science Foundation of China (No. 82072354), and Zhejiang Provincial Natural Science Foundation of China under Grant No. LTGD24C040008.
Conflict of interest
The authors declare that the research was conducted in the absence of any commercial or financial relationships that could be construed as a potential conflict of interest.
Publisher’s note
All claims expressed in this article are solely those of the authors and do not necessarily represent those of their affiliated organizations, or those of the publisher, the editors and the reviewers. Any product that may be evaluated in this article, or claim that may be made by its manufacturer, is not guaranteed or endorsed by the publisher.
References
1. Xu H, Niu M, Yuan X, Wu K, Liu A. CD44 as a tumor biomarker and therapeutic target. Exp Hematol Oncol. (2020) 9:36. doi: 10.1186/s40164-020-00192-0
2. Ponta H, Sherman L, Herrlich PA. CD44: from adhesion molecules to signalling regulators. Nat Rev Mol Cell Biol. (2003) 4:33–45. doi: 10.1038/nrm1004
3. Vuorio J, Skerlova J, Fabry M, Veverka V, Vattulainen I, Rezacova P, et al. N-Glycosylation can selectively block or foster different receptor-ligand binding modes. Sci Rep. (2021) 11:5239. doi: 10.1038/s41598-021-84569-z
4. Guo Q, Yang C, Gao F. The state of CD44 activation in cancer progression and therapeutic targeting. FEBS J. (2022) 289:7970–86. doi: 10.1111/febs.16179
5. Dzwonek J, Wilczynski GM. CD44: molecular interactions, signaling and functions in the nervous system. Front Cell Neurosci. (2015) 9:175. doi: 10.3389/fncel.2015.00175
6. Mishra MN, Chandavarkar V, Sharma R, Bhargava D. Structure, function and role of CD44 in neoplasia. J Oral Maxillofac Pathol. (2019) 23:267–72. doi: 10.4103/jomfp.JOMFP_246_18
7. Ma Z, Shi S, Ren M, Pang C, Zhan Y, An H, et al. Molecular mechanism of CD44 homodimerization modulated by palmitoylation and membrane environments. Biophys J. (2022) 121:2671–83. doi: 10.1016/j.bpj.2022.06.021
8. Sun F, Schroer CFE, Palacios CR, Xu L, Luo SZ, Marrink SJ. Molecular mechanism for bidirectional regulation of CD44 for lipid raft affiliation by palmitoylations and PIP2. PloS Comput Biol. (2020) 16:e1007777. doi: 10.1371/journal.pcbi.1007777
9. Senbanjo LT, Chellaiah MA. CD44: A multifunctional cell surface adhesion receptor is a regulator of progression and metastasis of cancer cells. Front Cell Dev Biol. (2017) 5:18. doi: 10.3389/fcell.2017.00018
10. Amorim S, Reis CA, Reis RL, Pires RA. Extracellular matrix mimics using hyaluronan-based biomaterials. Trends Biotechnol. (2021) 39:90–104. doi: 10.1016/j.tibtech.2020.06.003
11. Kim S, Li S, Jangid AK, Park HW, Lee DJ, Jung HS, et al. Surface engineering of natural killer cells with CD44-targeting ligands for augmented cancer immunotherapy. Small. (2023), e2306738. doi: 10.1002/smll.202306738
12. Pall T, Gad A, Kasak L, Drews M, Stromblad S, Kogerman P. Recombinant CD44-HABD is a novel and potent direct angiogenesis inhibitor enforcing endothelial cell-specific growth inhibition independently of hyaluronic acid binding. Oncogene. (2004) 23:7874–81. doi: 10.1038/sj.onc.1208083
13. Kim SJ, Owen SC. Hyaluronic acid binding to CD44S is indiscriminate of molecular weight. Biochim Biophys Acta Biomembr. (2020) 1862:183348. doi: 10.1016/j.bbamem.2020.183348
14. Si J, Wang C, Zhang D, Wang B, Zhou Y. Osteopontin in bone metabolism and bone diseases. Med Sci Monit. (2020) 26:e919159. doi: 10.12659/MSM.919159
15. O’Regan A, Berman JS. Osteopontin: a key cytokine in cell-mediated and granulomatous inflammation. Int J Exp Pathol. (2000) 81:373–90. doi: 10.1046/j.1365-2613.2000.00163.x
16. Gericke A, Qin C, Spevak L, Fujimoto Y, Butler WT, Sorensen ES, et al. Importance of phosphorylation for osteopontin regulation of biomineralization. Calcif Tissue Int. (2005) 77:45–54. doi: 10.1007/s00223-004-1288-1
17. Fnu G, Agrawal P, Kundu GC, Weber GF. Structural constraint of osteopontin facilitates efficient binding to CD44. Biomolecules. (2021) 11:813. doi: 10.3390/biom11060813
18. Legg JW, Lewis CA, Parsons M, Ng T, Isacke CM. A novel PKC-regulated mechanism controls CD44 ezrin association and directional cell motility. Nat Cell Biol. (2002) 4:399–407. doi: 10.1038/ncb797
19. Shao Y, Li L, Liu L, Yang Y, Huang J, Ji D, et al. CD44/ERM/F-actin complex mediates targeted nuclear degranulation and excessive neutrophil extracellular trap formation during sepsis. J Cell Mol Med. (2022) 26:2089–103. doi: 10.1111/jcmm.17231
20. Bourguignon LY, Zhu H, Shao L, Chen YW. CD44 interaction with c-Src kinase promotes cortactin-mediated cytoskeleton function and hyaluronic acid-dependent ovarian tumor cell migration. J Biol Chem. (2001) 276:7327–36. doi: 10.1074/jbc.M006498200
21. Hill A, McFarlane S, Mulligan K, Gillespie H, Draffin JE, Trimble A, et al. Cortactin underpins CD44-promoted invasion and adhesion of breast cancer cells to bone marrow endothelial cells. Oncogene. (2006) 25:6079–91. doi: 10.1038/sj.onc.1209628
22. Mosaddeghzadeh N, Ahmadian MR. The RHO family GTPases: mechanisms of regulation and signaling. Cells. (2021) 10:1831. doi: 10.3390/cells10071831
23. Bourguignon LY, Gilad E, Rothman K, Peyrollier K. Hyaluronan-CD44 interaction with IQGAP1 promotes Cdc42 and ERK signaling, leading to actin binding, Elk-1/estrogen receptor transcriptional activation, and ovarian cancer progression. J Biol Chem. (2005) 280:11961–72. doi: 10.1074/jbc.M411985200
24. Hodge RG, Ridley AJ. Regulating Rho GTPases and their regulators. Nat Rev Mol Cell Biol. (2016) 17:496–510. doi: 10.1038/nrm.2016.67
25. Bourguignon LY, Zhu H, Shao L, Chen YW. CD44 interaction with tiam1 promotes Rac1 signaling and hyaluronic acid-mediated breast tumor cell migration. J Biol Chem. (2000) 275:1829–38. doi: 10.1074/jbc.275.3.1829
26. Skandalis SS. CD44 intracellular domain: A long tale of a short tail. Cancers (Basel). (2023) 15:5041. doi: 10.3390/cancers15205041
27. Bourguignon LY, Zhu H, Zhou B, Diedrich F, Singleton PA, Hung MC. Hyaluronan promotes CD44v3-Vav2 interaction with Grb2-p185(HER2) and induces Rac1 and Ras signaling during ovarian tumor cell migration and growth. J Biol Chem. (2001) 276:48679–92. doi: 10.1074/jbc.M106759200
28. Bourguignon LY, Singleton PA, Zhu H, Diedrich F. Hyaluronan-mediated CD44 interaction with RhoGEF and Rho kinase promotes Grb2-associated binder-1 phosphorylation and phosphatidylinositol 3-kinase signaling leading to cytokine (macrophage-colony stimulating factor) production and breast tumor progression. J Biol Chem. (2003) 278:29420–34. doi: 10.1074/jbc.M301885200
29. Gutjahr JC, Bayer E, Yu X, Laufer JM, Hopner JP, Tesanovic S, et al. CD44 engagement enhances acute myeloid leukemia cell adhesion to the bone marrow microenvironment by increasing VLA-4 avidity. Haematologica. (2021) 106:2102–13. doi: 10.3324/haematol.2019.231944
30. Zucchetto A, Tissino E, Chigaev A, Hartmann TN, Gattei V. Methods for investigating VLA-4 (CD49d/CD29) expression and activation in chronic lymphocytic leukemia and its clinical applications. Methods Mol Biol. (2019) 1881:101–12. doi: 10.1007/978-1-4939-8876-1_8
31. Yusuf-Makagiansar H, Anderson ME, Yakovleva TV, Murray JS, Siahaan TJ. Inhibition of LFA-1/ICAM-1 and VLA-4/VCAM-1 as a therapeutic approach to inflammation and autoimmune diseases. Med Res Rev. (2002) 22:146–67. doi: 10.1002/med.10001
32. Mu W, Xu Y, Gu P, Wang W, Li J, Ge Y, et al. Exosomal CD44 cooperates with integrin α6β4 to support organotropic metastasis via regulating tumor cell motility and target host cell activation. Engineering. (2021) 7:1413–23. doi: 10.1016/j.eng.2020.08.013
33. Codini M, Garcia-Gil M, Albi E. Cholesterol and sphingolipid enriched lipid rafts as therapeutic targets in cancer. Int J Mol Sci. (2021) 22:726. doi: 10.3390/ijms22020726
34. Yang Z, Qin W, Chen Y, Yuan B, Song X, Wang B, et al. Cholesterol inhibits hepatocellular carcinoma invasion and metastasis by promoting CD44 localization in lipid rafts. Cancer Lett. (2018) 429:66–77. doi: 10.1016/j.canlet.2018.04.038
35. Ren M, Zhao L, Ma Z, An H, Marrink SJ, Sun F. Molecular basis of PIP2-dependent conformational switching of phosphorylated CD44 in binding FERM. Biophys J. (2023) 122:2675–85. doi: 10.1016/j.bpj.2023.05.021
36. Vona R, Iessi E, Matarrese P. Role of cholesterol and lipid rafts in cancer signaling: A promising therapeutic opportunity? Front Cell Dev Biol. (2021) 9:622908. doi: 10.3389/fcell.2021.622908
37. Schaller MD. Paxillin: a focal adhesion-associated adaptor protein. Oncogene. (2001) 20:6459–72. doi: 10.1038/sj.onc.1204786
38. Legerstee K, Geverts B, Slotman JA, Houtsmuller AB. Dynamics and distribution of paxillin, vinculin, zyxin and VASP depend on focal adhesion location and orientation. Sci Rep. (2019) 9:10460. doi: 10.1038/s41598-019-46905-2
39. Chang G, Wang J, Zhang H, Zhang Y, Wang C, Xu H, et al. CD44 targets Na(+)/H(+) exchanger 1 to mediate MDA-MB-231 cells’ metastasis via the regulation of ERK1/2. Br J Cancer. (2014) 110:916–27. doi: 10.1038/bjc.2013.809
40. Liu S, Cheng C. Akt signaling is sustained by a CD44 splice isoform-mediated positive feedback loop. Cancer Res. (2017) 77:3791–801. doi: 10.1158/0008-5472.CAN-16-2545
41. Khaldoyanidi S, Moll J, Karakhanova S, Herrlich P, Ponta H. Hyaluronate-enhanced hematopoiesis: two different receptors trigger the release of interleukin-1beta and interleukin-6 from bone marrow macrophages. Blood. (1999) 94:940–9. doi: 10.1182/blood.V94.3.940.415k27_940_949
42. Ma L, Dong L, Chang P. CD44v6 engages in colorectal cancer progression. Cell Death Dis. (2019) 10:30. doi: 10.1038/s41419-018-1265-7
43. McClatchey AI. Merlin and ERM proteins: unappreciated roles in cancer development? Nat Rev Cancer. (2003) 3:877–83. doi: 10.1038/nrc1213
44. Bai Y, Liu YJ, Wang H, Xu Y, Stamenkovic I, Yu Q. Inhibition of the hyaluronan-CD44 interaction by merlin contributes to the tumor-suppressor activity of merlin. Oncogene. (2007) 26:836–50. doi: 10.1038/sj.onc.1209849
45. Nagano O, Saya H. Mechanism and biological significance of CD44 cleavage. Cancer Sci. (2004) 95:930–5. doi: 10.1111/j.1349-7006.2004.tb03179.x
46. Xu H, Tian Y, Yuan X, Wu H, Liu Q, Pestell RG, et al. The role of CD44 in epithelial-mesenchymal transition and cancer development. Onco Targets Ther. (2015) 8:3783–92. doi: 10.2147/OTT.S95470
47. Pure E, Cuff CA. A crucial role for CD44 in inflammation. Trends Mol Med. (2001) 7:213–21. doi: 10.1016/s1471-4914(01)01963-3
48. Terawaki S, Kitano K, Aoyama M, Mori T, Hakoshima T. MT1-MMP recognition by ERM proteins and its implication in CD44 shedding. Genes Cells. (2015) 20:847–59. doi: 10.1111/gtc.12276
49. Kamarajan P, Shin JM, Qian X, Matte B, Zhu JY, Kapila YL. ADAM17-mediated CD44 cleavage promotes orasphere formation or stemness and tumorigenesis in HNSCC. Cancer Med. (2013) 2:793–802. doi: 10.1002/cam4.147
50. Miletti-Gonzalez KE, Murphy K, Kumaran MN, Ravindranath AK, Wernyj RP, Kaur S, et al. Identification of function for CD44 intracytoplasmic domain (CD44-ICD): modulation of matrix metalloproteinase 9 (MMP-9) transcription via novel promoter response element. J Biol Chem. (2012) 287:18995–9007. doi: 10.1074/jbc.M111.318774
51. Cichy J, Pure E. The liberation of CD44. J Cell Biol. (2003) 161:839–43. doi: 10.1083/jcb.200302098
52. Solier S, Muller S, Caneque T, Versini A, Mansart A, Sindikubwabo F, et al. A druggable copper-signalling pathway that drives inflammation. Nature. (2023) 617:386–94. doi: 10.1038/s41586-023-06017-4
53. Ruiz LM, Libedinsky A, Elorza AA. Role of copper on mitochondrial function and metabolism. Front Mol Biosci. (2021) 8:711227. doi: 10.3389/fmolb.2021.711227
54. Thanee M, Dokduang H, Kittirat Y, Phetcharaburanin J, Klanrit P, Titapun A, et al. CD44 modulates metabolic pathways and altered ROS-mediated Akt signal promoting cholangiocarcinoma progression. PloS One. (2021) 16:e0245871. doi: 10.1371/journal.pone.0245871
55. Zhang L, Yang P, Chen J, Chen Z, Liu Z, Feng G, et al. CD44 connects autophagy decline and ageing in the vascular endothelium. Nat Commun. (2023) 14:5524. doi: 10.1038/s41467-023-41346-y
56. Wittig BM, Sabat R, Holzlohner P, Witte-Handel E, Heilmann K, Witte K, et al. Absence of specific alternatively spliced exon of CD44 in macrophages prevents colitis. Mucosal Immunol. (2018) 11:846–60. doi: 10.1038/mi.2017.98
57. Shi J, Duan Y, Pan L, Zhou X. Positive association between CD44 gene rs13347 C>T polymorphism and risk of cancer in Asians: a systemic review and meta-analysis. Onco Targets Ther. (2016) 9:3493–500. doi: 10.2147/OTT.S104734
58. Chen P, Huang HF, Lu R, Wu Y, Chen YZ. Prognostic significance of CD44v6/v7 in acute promyelocytic leukemia. Asian Pac J Cancer Prev. (2012) 13:3791–4. doi: 10.7314/apjcp.2012.13.8.3791
59. Nasu H, Hibi N, Ohyashiki JH, Hara A, Kubono K, Tsukada Y, et al. Serum soluble CD44 levels for monitoring disease states in acute leukemia and myelodysplastic syndromes. Int J Oncol. (1998) 13:525–30. doi: 10.3892/ijo.13.3.525
60. Zittermann SI, Achino BI, Agriello EE, Halperín N, Ramhorst RE, Fainboim L. Modulation of CD44 in acute lymphoblastic leukemia identifies functional and phenotypic differences of human B cell precursors. Eur J Haematol. (2001) 66:377–82. doi: 10.1034/j.1600-0609.2001.066006377.x
61. Yu J, Li Y, Zhang D, Wan D, Jiang Z. Clinical implications of recurrent gene mutations in acute myeloid leukemia. Exp Hematol Oncol. (2020) 9:4. doi: 10.1186/s40164-020-00161-7
62. Horst E, Meijer CJ, Radaskiewicz T, van Dongen JJ, Pieters R, Figdor CG, et al. Expression of a human homing receptor (CD44) in lymphoid Malignancies and related stages of lymphoid development. Leukemia. (1990) 4:383–9. doi: 10.1002/jso.2930440115
63. Khan NI, Cisterne A, Devidas M, Shuster J, Hunger SP, Shaw PJ, et al. Expression of CD44, but not CD44v6, predicts relapse in children with B cell progenitor acute lymphoblastic leukemia lacking adverse or favorable genetics. Leuk Lymphoma. (2008) 49:710–8. doi: 10.1080/10428190701861660
64. Bendall LJ, James A, Zannettino A, Simmons PJ, Gottlieb DJ, Bradstock KF. A novel CD44 antibody identifies an epitope that is aberrantly expressed on acute lymphoblastic leukaemia cells. Immunol Cell Biol. (2003) 81:311–9. doi: 10.1046/j.1440-1711.2003.t01-1-01174.x
65. Coustan-Smith E, Song G, Clark C, Key L, Liu P, Mehrpooya M, et al. New markers for minimal residual disease detection in acute lymphoblastic leukemia. Blood. (2011) 117:6267–76. doi: 10.1182/blood-2010-12-324004
66. Vaskova M, Fronkova E, Starkova J, Kalina T, Mejstrikova E, Hrusak O. CD44 and CD27 delineate B-precursor stages with different recombination status and with an uneven distribution in nonmalignant and Malignant hematopoiesis. Tissue Antigens. (2008) 71:57–66. doi: 10.1111/j.1399-0039.2007.00968.x
67. Samy N, Abd El-Maksoud MD, Mousa TE, El-Mezayen HA, Shaalan M. Potential role of serum level of soluble CD44 and IFN-γ in B-cell chronic lymphocytic leukemia. Med Oncol. (2011) 28 Suppl 1:S471–5. doi: 10.1007/s12032-010-9661-6
68. Zarcone D, De Rossi G, Tenca C, Marroni P, Mauro FR, Cerruti GM, et al. Functional and clinical relevance of CD44 variant isoform expression on B-cell chronic lymphocytic leukemia cells. Haematologica. (1998) 83:1088–98.
69. Buggins AG, Levi A, Gohil S, Fishlock K, Patten PE, Calle Y, et al. Evidence for a macromolecular complex in poor prognosis CLL that contains CD38, CD49d, CD44 and MMP-9. Br J Haematol. (2011) 154:216–22. doi: 10.1111/j.1365-2141.2011.08725.x
70. Bologna C, Buonincontri R, Serra S, Vaisitti T, Audrito V, Brusa D, et al. SLAMF1 regulation of chemotaxis and autophagy determines CLL patient response. J Clin Invest. (2016) 126:181–94. doi: 10.1172/jci83013
71. Molica S, Vitelli G, Levato D, Giannarelli D, Gandolfo GM. Elevated serum levels of soluble CD44 can identify a subgroup of patients with early B-cell chronic lymphocytic leukemia who are at high risk of disease progression. Cancer. (2001) 92:713–9. doi: 10.1002/1097-0142(20010815)92:4<713::aid-cncr1374>3.0.co;2-o
72. Gutjahr JC, Szenes E, Tschech L, Asslaber D, Schlederer M, Roos S, et al. Microenvironment-induced CD44v6 promotes early disease progression in chronic lymphocytic leukemia. Blood. (2018) 131:1337–49. doi: 10.1182/blood-2017-08-802462
73. Casucci M, Nicolis di Robilant B, Falcone L, Camisa B, Norelli M, Genovese P, et al. CD44v6-targeted T cells mediate potent antitumor effects against acute myeloid leukemia and multiple myeloma. Blood. (2013) 122:3461–72. doi: 10.1182/blood-2013-04-493361
74. Wang SW, Yao HX, Rao R, Xia MJ. [Expression of CD44, CD87 and CD123 in acute leukemia and its correlation with cellular immunity]. Zhongguo Shi Yan Xue Ye Xue Za Zhi. (2019) 27:1794–8. doi: 10.19746/j.cnki.issn.1009-2137.2019.06.014
75. Legras S, Günthert U, Stauder R, Curt F, Oliferenko S, Kluin-Nelemans HC, et al. A strong expression of CD44-6v correlates with shorter survival of patients with acute myeloid leukemia. Blood. (1998) 91:3401–13. doi: 10.1182/blood.V91.9.3401.3401_3401_3413
76. Huang X, Wang RH, Li DY, Lai ZL, Chu YT, Zhang Y, et al. [Expression of biomarkers related with bone marrow cells in patients with acute myelogenous leukemia]. Zhongguo Shi Yan Xue Ye Xue Za Zhi. (2014) 22:1193–8. doi: 10.7534/j.issn.1009-2137.2014.05.001
77. Yokota A, Ishii G, Sugaya Y, Nishimura M, Saito Y, Harigaya K. Expression of exon v6-containing CD44 isoforms is related to poor prognosis of acute myelocytic leukemia. Hematol Oncol. (1998) 16:131–41. doi: 10.1002/(sici)1099-1069(199812)16:4<131::aid-hon631>3.0.co;2-k
78. Zhang C, Bai G, Zhu W, Bai D, Bi G. Identification of miRNA-mRNA network associated with acute myeloid leukemia survival. Med Sci Monit. (2017) 23:4705–14. doi: 10.12659/msm.903989
79. Quéré R, Andradottir S, Brun AC, Zubarev RA, Karlsson G, Olsson K, et al. High levels of the adhesion molecule CD44 on leukemic cells generate acute myeloid leukemia relapse after withdrawal of the initial transforming event. Leukemia. (2011) 25:515–26. doi: 10.1038/leu.2010.281
80. Wu H, Deng J, Zheng J, You Y, Li N, Li W, et al. Functional polymorphisms in the CD44 gene and acute myeloid leukemia cancer risk in a Chinese population. Mol Carcinog. (2015) 54:102–10. doi: 10.1002/mc.22078
81. Darwish NH, Sudha T, Godugu K, Elbaz O, Abdelghaffar HA, Hassan EE, et al. Acute myeloid leukemia stem cell markers in prognosis and targeted therapy: potential impact of BMI-1, TIM-3 and CLL-1. Oncotarget. (2016) 7:57811–20. doi: 10.18632/oncotarget.11063
82. Al-Asadi MG, Brindle G, Castellanos M, May ST, Mills KI, Russell NH, et al. A molecular signature of dormancy in CD34(+)CD38(-) acute myeloid leukaemia cells. Oncotarget. (2017) 8:111405–18. doi: 10.18632/oncotarget.22808
83. Liesveld JL, Dipersio JF, Abboud CN. Integrins and adhesive receptors in normal and leukemic CD34+ progenitor cells: potential regulatory checkpoints for cellular traffic. Leuk Lymphoma. (1994) 14:19–28. doi: 10.3109/10428199409049647
84. Ruvolo PP, Hu CW, Qiu Y, Ruvolo VR, Go RL, Hubner SE, et al. LGALS3 is connected to CD74 in a previously unknown protein network that is associated with poor survival in patients with AML. EBioMedicine. (2019) 44:126–37. doi: 10.1016/j.ebiom.2019.05.025
85. Yokota A, Ishii G, Sugaya Y, Nishimura M, Saito Y, Harigaya K. Potential use of serum CD44 as an indicator of tumour progression in acute leukemia. Hematol Oncol. (1999) 17:161–8. doi: 10.1002/(sici)1099-1069(199912)17:4<161::aid-hon646>3.0.co;2-y
86. Reuss-Borst MA, Klein G, Waller HD, Müller CA. Differential expression of adhesion molecules in acute leukemia. Leukemia. (1995) 9:869–74.
87. Reuss-Borst MA, Bühring HJ, Klein G, Müller CA. Adhesion molecules on CD34+ hematopoietic cells in normal human bone marrow and leukemia. Ann Hematol. (1992) 65:169–74. doi: 10.1007/bf01703110
88. Huang X, Li D, Li T, Zhao BO, Chen X. Prognostic value of the expression of phosphatase and tensin homolog and CD44 in elderly patients with refractory acute myeloid leukemia. Oncol Lett. (2015) 10:103–10. doi: 10.3892/ol.2015.3189
89. Loeffler-Ragg J, Steurer M, Ulmer H, Skvortsov S, Kircher B, Herold M, et al. Elevated levels of serum CD44 and E-cadherin predict an unfavourable outcome in myelodysplastic syndromes. Leukemia. (2006) 20:2064–7. doi: 10.1038/sj.leu.2404382
90. Li W, Ji M, Lu F, Pang Y, Dong X, Zhang J, et al. Novel AF1q/MLLT11 favorably affects imatinib resistance and cell survival in chronic myeloid leukemia. Cell Death Dis. (2018) 9:855. doi: 10.1038/s41419-018-0900-7
91. Zuo X, Feng J, Ye L, Xing M, Deng Z, Gu X, et al. The role of CD44 in the assessment of minimal residual disease of multiple myeloma by flow cytometry. J Hematopathol. (2021) 14:299–307. doi: 10.1007/s12308-021-00468-2
92. Rao G, Wang H, Li B, Huang L, Xue D, Wang X, et al. Reciprocal interactions between tumor-associated macrophages and CD44-positive cancer cells via osteopontin/CD44 promote tumorigenicity in colorectal cancer. Clin Cancer Res. (2013) 19:785–97. doi: 10.1158/1078-0432.CCR-12-2788
93. Hellen N, Mashanov GI, Conte IL, le Trionnaire S, Babich V, Knipe L, et al. P-selectin mobility undergoes a sol-gel transition as it diffuses from exocytosis sites into the cell membrane. Nat Commun. (2022) 13:3031. doi: 10.1038/s41467-022-30669-x
94. Yago T, Shao B, Miner JJ, Yao L, Klopocki AG, Maeda K, et al. E-selectin engages PSGL-1 and CD44 through a common signaling pathway to induce integrin alphaLbeta2-mediated slow leukocyte rolling. Blood. (2010) 116:485–94. doi: 10.1182/blood-2009-12-259556
95. Chen C, Zhao S, Zhao X, Cao L, Karnad A, Kumar AP, et al. Gemcitabine resistance of pancreatic cancer cells is mediated by IGF1R dependent upregulation of CD44 expression and isoform switching. Cell Death Dis. (2022) 13:682. doi: 10.1038/s41419-022-05103-1
96. Bourguignon LY, Peyrollier K, Xia W, Gilad E. Hyaluronan-CD44 interaction activates stem cell marker Nanog, Stat-3-mediated MDR1 gene expression, and ankyrin-regulated multidrug efflux in breast and ovarian tumor cells. J Biol Chem. (2008) 283:17635–51. doi: 10.1074/jbc.M800109200
97. Lv L, Liu HG, Dong SY, Yang F, Wang QX, Guo GL, et al. Upregulation of CD44v6 contributes to acquired chemoresistance via the modulation of autophagy in colon cancer SW480 cells. Tumour Biol. (2016) 37:8811–24. doi: 10.1007/s13277-015-4755-6
98. Bourguignon LY, Wong G, Earle C, Chen L. Hyaluronan-CD44v3 interaction with Oct4-Sox2-Nanog promotes miR-302 expression leading to self-renewal, clonal formation, and cisplatin resistance in cancer stem cells from head and neck squamous cell carcinoma. J Biol Chem. (2012) 287:32800–24. doi: 10.1074/jbc.M111.308528
99. Price ZK, Lokman NA, Ricciardelli C. Differing roles of hyaluronan molecular weight on cancer cell behavior and chemotherapy resistance. Cancers (Basel). (2018) 10:482. doi: 10.3390/cancers10120482
100. Sansonetti A, Bourcier S, Durand L, Chomienne C, Smadja-Joffe F, Robert-Lezenes J. CD44 activation enhances acute monoblastic leukemia cell survival via Mcl-1 upregulation. Leuk Res. (2012) 36:358–62. doi: 10.1016/j.leukres.2011.09.022
101. Chang G, Zhang H, Wang J, Zhang Y, Xu H, Wang C, et al. CD44 targets Wnt/beta-catenin pathway to mediate the proliferation of K562 cells. Cancer Cell Int. (2013) 13:117. doi: 10.1186/1475-2867-13-117
102. Li M, Sun J, Zhang W, Zhao Y, Zhang S, Zhang S. Drug delivery systems based on CD44-targeted glycosaminoglycans for cancer therapy. Carbohydr Polym. (2021) 251:117103. doi: 10.1016/j.carbpol.2020.117103
103. Jin L, Hope KJ, Zhai Q, Smadja-Joffe F, Dick JE. Targeting of CD44 eradicates human acute myeloid leukemic stem cells. Nat Med. (2006) 12:1167–74. doi: 10.1038/nm1483
104. Charrad RS, Li Y, Delpech B, Balitrand N, Clay D, Jasmin C, et al. Ligation of the CD44 adhesion molecule reverses blockage of differentiation in human acute myeloid leukemia. Nat Med. (1999) 5:669–76. doi: 10.1038/9518
105. Charrad RS, Gadhoum Z, Qi J, Glachant A, Allouche M, Jasmin C, et al. Effects of anti-CD44 monoclonal antibodies on differentiation and apoptosis of human myeloid leukemia cell lines. Blood. (2002) 99:290–9. doi: 10.1182/blood.v99.1.290
106. Chang GQ, Wang J, Gao W, Wang RJ, Wang LH, Jin WN, et al. [Early apoptosis of HL-60 cells induced by anti-CD44 McAb A3D8 inhibiting ERK1/2-upregulated Bim expression]. Zhongguo Shi Yan Xue Ye Xue Za Zhi. (2011) 19:656–60.
107. Vey N, Delaunay J, Martinelli G, Fiedler W, Raffoux E, Prebet T, et al. Phase I clinical study of RG7356, an anti-CD44 humanized antibody, in patients with acute myeloid leukemia. Oncotarget. (2016) 7:32532–42. doi: 10.18632/oncotarget.8687
108. Takahashi T, Kawakami K, Mishima S, Akimoto M, Takenaga K, Suzumiya J, et al. Cyclopamine induces eosinophilic differentiation and upregulates CD44 expression in myeloid leukemia cells. Leuk Res. (2011) 35:638–45. doi: 10.1016/j.leukres.2010.09.022
109. Amanzadeh A, Molla-Kazemiha V, Samani S, Habibi-Anbouhi M, Azadmanesh K, Abolhassani M, et al. New synergistic combinations of differentiation-inducing agents in the treatment of acute promyelocytic leukemia cells. Leuk Res. (2018) 68:98–104. doi: 10.1016/j.leukres.2018.01.007
110. Assaraf YG, Brozovic A, Goncalves AC, Jurkovicova D, Line A, Machuqueiro M, et al. The multi-factorial nature of clinical multidrug resistance in cancer. Drug Resist Update. (2019) 46:100645. doi: 10.1016/j.drup.2019.100645
111. Sague SL, Tato C, Pure E, Hunter CA. The regulation and activation of CD44 by natural killer (NK) cells and its role in the production of IFN-gamma. J Interferon Cytokine Res. (2004) 24:301–9. doi: 10.1089/107999004323065093
112. Zhao L, Zhao J, Zhong K, Tong A, Jia D. Targeted protein degradation: mechanisms, strategies and application. Signal Transduct Target Ther. (2022) 7:113. doi: 10.1038/s41392-022-00966-4
113. Ahn G, Banik SM, Miller CL, Riley NM, Cochran JR, Bertozzi CR. LYTACs that engage the asialoglycoprotein receptor for targeted protein degradation. Nat Chem Biol. (2021) 17:937–46. doi: 10.1038/s41589-021-00770-1
114. Liu J, Bi G, Wen P, Yang W, Ren X, Tang T, et al. Down-regulation of CD44 contributes to the differentiation of HL-60 cells induced by ATRA or HMBA. Cell Mol Immunol. (2007) 4:59–63. doi: 10.1016/j.cellimm.2007.04.005
115. Huang HF, Chen P, Lu R, Lin ZX, Wu Y, Chen YZ. [Regulation of all-trans retinoic acid and arsenic trioxide on CD44v6 expression in NB4 cells]. Zhongguo Shi Yan Xue Ye Xue Za Zhi. (2012) 20:33–7.
116. Sun D, Zhou JK, Zhao L, Zheng ZY, Li J, Pu W, et al. Novel curcumin liposome modified with hyaluronan targeting CD44 plays an anti-leukemic role in acute myeloid leukemia in vitro and in vivo. ACS Appl Mater Interfaces. (2017) 9:16857–68. doi: 10.1021/acsami.7b02863
117. Zhong Y, Meng F, Deng C, Mao X, Zhong Z. Targeted inhibition of human hematological cancers in vivo by doxorubicin encapsulated in smart lipoic acid-crosslinked hyaluronic acid nanoparticles. Drug Deliv. (2017) 24:1482–90. doi: 10.1080/10717544.2017.1384864
118. Gul-Uludağ H, Valencia-Serna J, Kucharski C, Marquez-Curtis LA, Jiang X, Larratt L, et al. Polymeric nanoparticle-mediated silencing of CD44 receptor in CD34+ acute myeloid leukemia cells. Leuk Res. (2014) 38:1299–308. doi: 10.1016/j.leukres.2014.08.008
119. Darwish NHE, Sudha T, Godugu K, Bharali DJ, Elbaz O, El-Ghaffar HAA, et al. Novel targeted nano-parthenolide molecule against NF-kB in acute myeloid leukemia. Molecules. (2019) 24:2103. doi: 10.3390/molecules24112103
120. Qiu J, Cheng R, Zhang J, Sun H, Deng C, Meng F, et al. Glutathione-sensitive hyaluronic acid-mercaptopurine prodrug linked via carbonyl vinyl sulfide: A robust and CD44-targeted nanomedicine for leukemia. Biomacromolecules. (2017) 18:3207–14. doi: 10.1021/acs.biomac.7b00846
121. Han JM, Kim HL, Jung HJ. Ampelopsin inhibits cell proliferation and induces apoptosis in HL60 and K562 leukemia cells by downregulating AKT and NF-κB signaling pathways. Int J Mol Sci. (2021) 22:4265. doi: 10.3390/ijms22084265
122. Chen Y, Wang T, Du J, Li Y, Wang X, Zhou Y, et al. The critical role of PTEN/PI3K/AKT signaling pathway in shikonin-induced apoptosis and proliferation inhibition of chronic myeloid leukemia. Cell Physiol Biochem. (2018) 47:981–93. doi: 10.1159/000490142
123. Moon HJ, Park SY, Lee SH, Kang CD, Kim SH. Nonsteroidal anti-inflammatory drugs sensitize CD44-overexpressing cancer cells to hsp90 inhibitor through autophagy activation. Oncol Res. (2019) 27:835–47. doi: 10.3727/096504019x15517850319579
124. Moshofsky KB, Cho HJ, Wu G, Romine KA, Newman MT, Kosaka Y, et al. Acute myeloid leukemia-induced T-cell suppression can be reversed by inhibition of the MAPK pathway. Blood Adv. (2019) 3:3038–51. doi: 10.1182/bloodadvances.2019000574
125. Yi HW, Lu XM, Fang F, Wang J, Xu Q. Astilbin inhibits the adhesion of T lymphocytes via decreasing TNF-alpha and its associated MMP-9 activity and CD44 expression. Int Immunopharmacol. (2008) 8:1467–74. doi: 10.1016/j.intimp.2008.06.006
126. Kim E, Hurtz C, Koehrer S, Wang Z, Balasubramanian S, Chang BY, et al. Ibrutinib inhibits pre-BCR(+) B-cell acute lymphoblastic leukemia progression by targeting BTK and BLK. Blood. (2017) 129:1155–65. doi: 10.1182/blood-2016-06-722900
127. El-Gamal D, Williams K, LaFollette TD, Cannon M, Blachly JS, Zhong Y, et al. PKC-β as a therapeutic target in CLL: PKC inhibitor AEB071 demonstrates preclinical activity in CLL. Blood. (2014) 124:1481–91. doi: 10.1182/blood-2014-05-574830
128. Zhang S, Wu CC, Fecteau JF, Cui B, Chen L, Zhang L, et al. Targeting chronic lymphocytic leukemia cells with a humanized monoclonal antibody specific for CD44. Proc Natl Acad Sci USA. (2013) 110:6127–32. doi: 10.1073/pnas.1221841110
129. Fuchs K, Hippe A, Schmaus A, Homey B, Sleeman JP, Orian-Rousseau V. Opposing effects of high- and low-molecular weight hyaluronan on CXCL12-induced CXCR4 signaling depend on CD44. Cell Death Dis. (2013) 4:e819. doi: 10.1038/cddis.2013.364
130. Heath JL, Cohn GM, Zaidi SK, Stein GS. The role of cell adhesion in hematopoiesis and leukemogenesis. J Cell Physiol. (2019) 234:19189–98. doi: 10.1002/jcp.28636
131. Krause DS, Lazarides K, Lewis JB, von Andrian UH, Van Etten RA. Selectins and their ligands are required for homing and engraftment of BCR-ABL1+ leukemic stem cells in the bone marrow niche. Blood. (2014) 123:1361–71. doi: 10.1182/blood-2013-11-538694
132. Li L, Ding Q, Zhou J, Wu Y, Zhang M, Guo X, et al. Distinct binding kinetics of E-, P- and L-selectins to CD44. FEBS J. (2022) 289:2877–94. doi: 10.1111/febs.16303
133. Tvaroška I, Selvaraj C, Koča J. Selectins-the two dr. Jekyll and mr. Hyde faces of adhesion molecules-A review. Molecules. (2020) 25:2835. doi: 10.3390/molecules25122835
134. Ai L, Xu A, Xu J. Roles of PD-1/PD-L1 pathway: signaling, cancer, and beyond. Adv Exp Med Biol. (2020) 1248:33–59. doi: 10.1007/978-981-15-3266-5_3
135. Liu S, Liu Z, Shang A, Xun J, Lv Z, Zhou S, et al. CD44 is a potential immunotherapeutic target and affects macrophage infiltration leading to poor prognosis. Sci Rep. (2023) 13:9657. doi: 10.1038/s41598-023-33915-4
136. Ji X, Liu Y, Mei F, Li X, Zhang M, Yao B, et al. SPP1 overexpression is associated with poor outcomes in ALK fusion lung cancer patients without receiving targeted therapy. Sci Rep. (2021) 11:14031. doi: 10.1038/s41598-021-93484-2
137. DeGrendele HC, Estess P, Siegelman MH. Requirement for CD44 in activated T cell extravasation into an inflammatory site. Science. (1997) 278:672–5. doi: 10.1126/science.278.5338.672
138. Kong T, Ahn R, Yang K, Zhu X, Fu Z, Morin G, et al. CD44 promotes PD-L1 expression and its tumor-intrinsic function in breast and lung cancers. Cancer Res. (2020) 80:444–57. doi: 10.1158/0008-5472.Can-19-1108
139. Hou W, Kong L, Hou Z, Ji H. CD44 is a prognostic biomarker and correlated with immune infiltrates in gastric cancer. BMC Med Genomics. (2022) 15:225. doi: 10.1186/s12920-022-01383-w
140. Haist C, Schulte E, Bartels N, Bister A, Poschinski Z, Ibach TC, et al. CD44v6-targeted CAR T-cells specifically eliminate CD44 isoform 6 expressing head/neck squamous cell carcinoma cells. Oral Oncol. (2021) 116:105259. doi: 10.1016/j.oraloncology.2021.105259
141. Cai S, Alhowyan AA, Yang Q, Forrest WC, Shnayder Y, Forrest ML. Cellular uptake and internalization of hyaluronan-based doxorubicin and cisplatin conjugates. J Drug Target. (2014) 22:648–57. doi: 10.3109/1061186X.2014.921924
142. Sakurai Y, Harashima H. Hyaluronan-modified nanoparticles for tumor-targeting. Expert Opin Drug Deliv. (2019) 16:915–36. doi: 10.1080/17425247.2019.1645115
Keywords: CD44, leukemia, adhesion, migration, homing, differentiation, biomarkers, clinical application
Citation: Wu S, Tan Y, Li F, Han Y, Zhang S and Lin X (2024) CD44: a cancer stem cell marker and therapeutic target in leukemia treatment. Front. Immunol. 15:1354992. doi: 10.3389/fimmu.2024.1354992
Received: 13 December 2023; Accepted: 11 April 2024;
Published: 26 April 2024.
Edited by:
Paula Lam, Independent researcher, Singapore, SingaporeReviewed by:
Turabe M. H. U. Fazil, Nanyang Technological University, SingaporeDongyao Wang, University of Science and Technology of China, China
Copyright © 2024 Wu, Tan, Li, Han, Zhang and Lin. This is an open-access article distributed under the terms of the Creative Commons Attribution License (CC BY). The use, distribution or reproduction in other forums is permitted, provided the original author(s) and the copyright owner(s) are credited and that the original publication in this journal is cited, in accordance with accepted academic practice. No use, distribution or reproduction is permitted which does not comply with these terms.
*Correspondence: Shenghui Zhang, zhangshenghui@wmu.edu.cn; shenghuizhang1@126.com; Xiaofei Lin, luoxiadeyudian@126.com