- 1Medical College, Soochow University, Suzhou, Jiangsu, China
- 2Department of Thoracic Surgery, The Affiliated Wuxi People’s Hospital of Nanjing Medical University, Wuxi People’s Hospital, Wuxi Medical Center, Nanjing Medical University, Wuxi, Jiangsu, China
- 3The First School of Clinical Medicine, Nanjing Medical University. Nanjing, Jiangsu, China
- 4Department of Clinical Laboratory, Changshu Medicine Examination Institute, Changshu, Jiangsu, China
- 5Department of Gastroenterology, The Affiliated Wuxi People’s Hospital of Nanjing Medical University, Wuxi People’s Hospital, Wuxi Medical Center, Nanjing Medical University, Wuxi, Jiangsu, China
At present, cancer is the largest culprit that endangers human health. The current treatment options for cancer mainly include surgical resection, adjuvant radiotherapy and chemotherapy, but their therapeutic effects and long-term prognosis are unsatisfactory. Immunotherapy is an emerging therapy that has completely transformed the therapeutic landscape of advanced cancers, and has tried to occupy a place in the neoadjuvant therapy of resectable tumors. However, not all patients respond to immunotherapy due to the immunological and molecular features of the tumors. Traditional Chinese Medicine (TCM) provides a new perspective for cancer treatment and is considered to have the potential as promising anti-tumor drugs considering its immunoregulatory properties. This review concludes commonly used TCM monomers and compounds from the perspective of immune regulatory pathways, aiming to clearly introduce the basic mechanisms of TCM in boosting cancer immunotherapy and mechanisms of several common TCM. In addition, we also summarized closed and ongoing trials and presented prospects for future development. Due to the significant role of immunotherapy in the treatment of non-small cell lung cancer (NSCLC), TCM combined with immunotherapy should be emphasized in NSCLC.
Highlights
● TCM is a promising cancer treatment with three primary advantages including fewer side effects, personalized treatment, and potential immune system benefits.
● TCM exerts an effect on tumor immunity through multiple modalities of regulation.
● The pertinent studies and mechanisms of several commonly utilized TCM active ingredients were preliminarily reported and unconcealed.
● Immunotherapy in combination with TCM selectively targets cancer cells for suppression, leaving normal cells unharmed.
1 Introduction
Due to its profound impact on public health, the malignant tumor has emerged as a pressing concern in society, characterized by substantial morbidity and mortality rates (1). Presently, surgery and chemotherapy stand as the primary modalities for cancer treatment (2). Despite decades of dedicated research efforts towards unearthing more efficacious approaches, the global incidence of malignant tumors continues to remain alarmingly high. The intricate and heterogeneous nature of tumors has prompted a paradigm shift in cancer management, transitioning from a singular target approach to a multifaceted strategy that encompasses the tumor microenvironment (TME) (2–4).
Tumor immunotherapy, which refers to a type of cancer therapies that aims to harness the natural ability of the immune system to identify and finally eliminate tumor cells, seems to display a promising future as a potential therapeutic option for various kinds of tumors (5, 6). This approach involves the application of various therapeutic agents and strategies that modulate or enhance immune responses against cancer, including agents that activate immune cells such as T cells, antibodies that target specific cancer cells, and cellular therapies that involve the transfer of genetically engineered T cells or other immune cells into cancer patients (7, 8). Unlike traditional cancer therapies such as chemotherapy and radiation, which target both cancerous and normal cells, tumor immunotherapy has an edge of selectively targeting and eliminating cancer cells and minimizing damage to normal tissues. Current drugs have a therapeutic effect on cancer in the short term, but their major side effects can cause serious physical and mental problems for patients. Therefore, there is an urgent need to explore new therapeutic methods that have definite efficacy and mild adverse effects and can be taken for a long time.
For centuries, TCM has been employed as a therapeutic approach for various ailments, with cancer included, boasting a track record of safety, gentleness, and prolonged efficacy (9, 10). TCM adopts a holistic perspective, considering tumors as systemic diseases where treatment should encompass the entire body rather than solely focusing on localized areas. Approaches limited to local therapies often fail to provide comprehensive clinical benefits to cancer patients, thereby contributing to the underlying factors responsible for tumor recurrence and metastasis even after surgical interventions, chemotherapy, or targeted therapies. The advantages of TCM in the tumor immunotherapy can be summarized into three aspects——fewer side effects, personalized treatment, and potential immune system benefits (11–13). Chinese herbal therapy typically takes advantage of natural substances that are less toxic and have fewer side effects compared to chemoradiotherapy drugs (11). Chemoradiotherapy can cause side effects such as nausea, vomiting, hair loss, and fatigue, whereas Chinese herbal therapy is generally well-tolerated and can improve quality of life (12, 13). Chinese herbal therapy is tailored to the individual patient’s needs and can be adjusted based on their response to treatment. Chemoradiotherapy, on the other hand, is a standardized treatment that is prescribed based on the type and stage of cancer, and may not consider the individual differences in patients’ overall health or response to treatment. Chinese herbal therapy can enhance the body’s natural immune system, which can effectively detect and eliminate cancer cells and prevent recurrence. Chemoradiotherapy can weaken the immune system, making the patient more vulnerable to infections and other complications (14, 15). Overall, while there is still much to learn about the potential of TCM for the treatment of tumor, research to date suggests that it may have a role to play in improving outcomes for cancer patients (12, 15).
Currently, research on immunotherapy methods is underway, however, the investigation of possible targets from the perspective of TCM is still in its infancy. Considering this, it is desperately demanded to explore the potential and development direction of TCM as an immunotherapy approach. We will discuss the specific monomers and compounds found in TCM that have been identified to have potential in boosting cancer immunotherapy, as well as the mechanisms by which they work. We will also explore the results of clinical studies that have investigated the application of TCM in combination with cancer immunotherapy, as well as the obstacles and future directions for research in this field.
2 The regulatory effects of TCM monomers and compounds on TME
Immunomodulation means the ability of a substance to modify and regulate the immune response, either by enhancing or suppressing it. Chinese herbal medicine has been uncovered to have both immunostimulatory and immunosuppressive effects, depending on the specific herbs and the context in which they are applied (16–18). The immunoregulatory effects of Chinese medicine include the stimulation of immune cell activity, regulation of cytokine production, inhibition of inflammation, antioxidant activity, modulation of immune cell signaling pathways, etc (16, 19–21). TCM monomers such as baicalin, quercetin, and resveratrol have been reported to modulate the production of cytokines by regulating immune cells (22–25). These monomers can either enhance or suppress cytokine production, relying on the specific immune cells and the specific environment in which they are distributed (26). TCM monomers such as curcumin and berberine have been discovered to have anti-inflammatory effects through suppressing the production of pro-inflammatory cytokines and inhibiting the activation of immune cells such as macrophages. TCM monomers can regulate a variety of signaling pathways involved in immune cell activation and function. For instance, curcumin has been revealed to inhibit the nuclear factor-kappa B (NF-kB) signaling pathway, which plays a crucial role in regulating immune cell activity and inflammation (27, 28). Subsequently, this review expounds upon the potentiality of TCM in the realm of tumor immunotherapy, elucidating the prevailing clinical advancements in the manipulation via immune cells, modulation of cytokine signaling, angiogenesis and vascular normalization, extracellular matrix remodeling, inflammatory and immune responses, targeting immune checkpoints, and the modulation of immunosuppression and immune escape, respectively (Figure 1).
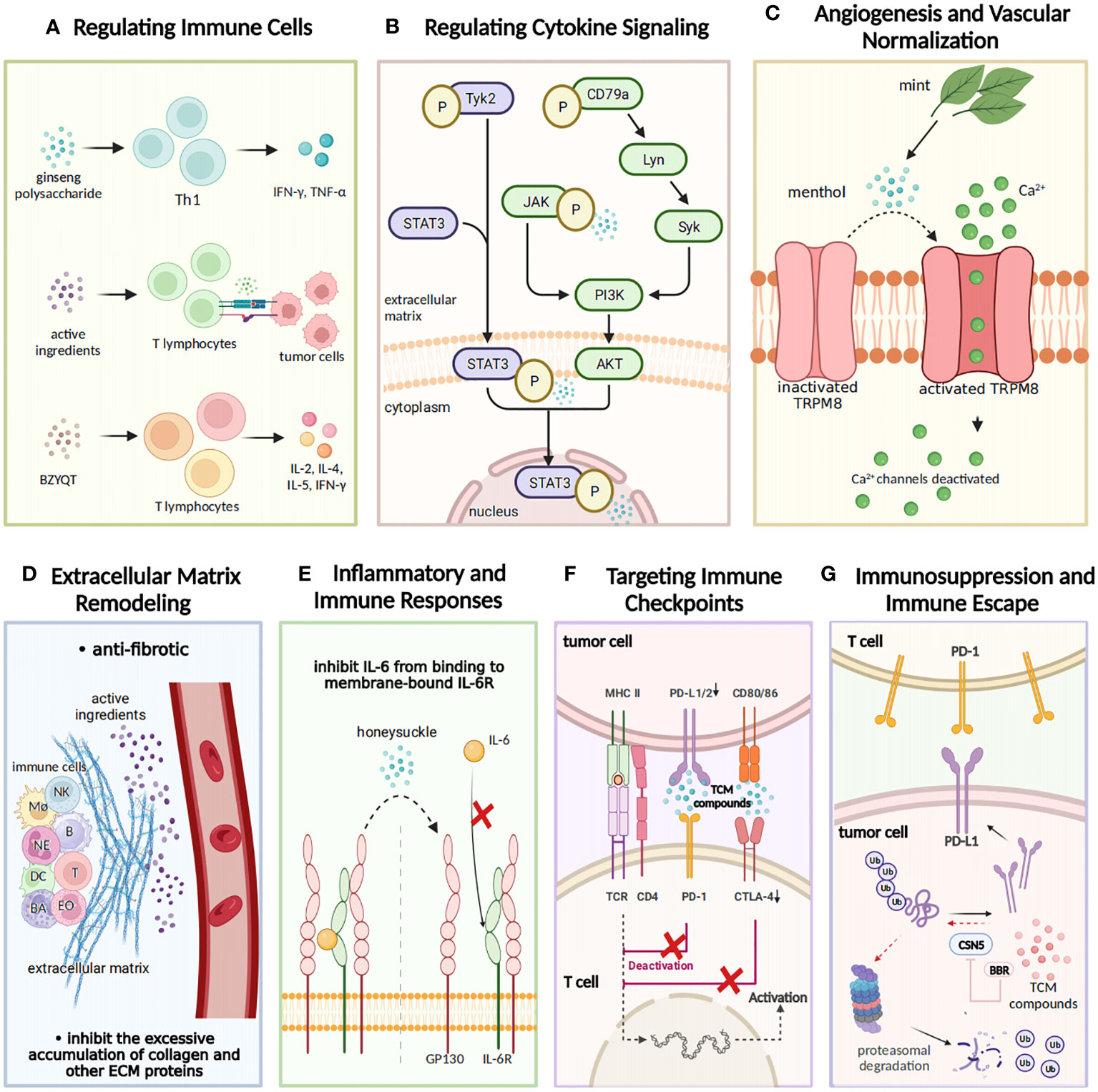
Figure 1 The regulatory effects of TCM on tumor immunity. TCM exerts a regulatory influence on tumor immunity from seven aspects. (A) Regulating immune cells: TCM exhibits immunomodulatory properties by elevating levels of IL-2, IL-8, and TNF-α and facilitating the recognition and binding of T lymphocytes to tumor cells. The TCM prescription Bu-Zhong-Yi-Qi-Tang (BZYQT) stimulates cytokine production from T lymphocytes including IL-2, IL-4, IL-5, and IFN-γ, thus demonstrating its immunomodulatory efficacy. (B) Modulating cytokine signaling: Gansui-Banxia Decoction (GSBXD), a classic TCM formula, exerts anti-tumor immune activity by downregulating AKT/STAT3/ERK signaling pathways and suppressing IL-1β and IFN-γ. (C) Angiogenesis and vascular normalization: Menthol activates angiogenesis by promoting the opening of TRPM8 ion channels and facilitating calcium ion flow. (D) Remodeling of extracellular matrix: TCM compounds regulate the intricate processes of extracellular matrix (ECM) remodeling, including degradation and deposition of ECM components. Additionally, they effectively inhibit excessive accumulation of collagen and other ECM proteins. (E) Inflammatory and immune responses: Honeysuckle inhibits the binding of IL-6 to membrane-bound IL-6R, thereby impeding tumor initiation and progression. (F) Targeting immune checkpoints: TCM compounds disrupt the binding of programmed cell death ligand 1 (PD-L1) and programmed cell death protein 1 (PD-1), impacting their inhibitory effect and promoting normal immune function. (G) Immunosuppression and immune escape: TCM compounds inhibit the expression of immune checkpoint molecules, such as PD-1 and cytotoxic T-lymphocyte associated protein 4 (CTLA-4), hence restoring immune cell functionality and facilitating robust tumor immune responses.
2.1 Modulation of immune cells
Tumor biological behaviors as a hot research topic have long been being investigated, including tumor initiation, progression, invasion, intravasation, dissemination, extravasation, dormancy, activation, and colonization. Immune cells matter a lot in affecting a series of tumor biological behaviors. Recently, TCMs have been reported to inhibit tumor biological behaviors via regulating immune cells. Relevant studies are introduced as follows and summarized in Table 1 (29–46), and an elaborated graph is presented in the form of Taiji to demonstrate the interactions and relationships among tumor cells, immune cells and TCMs (Figure 2).
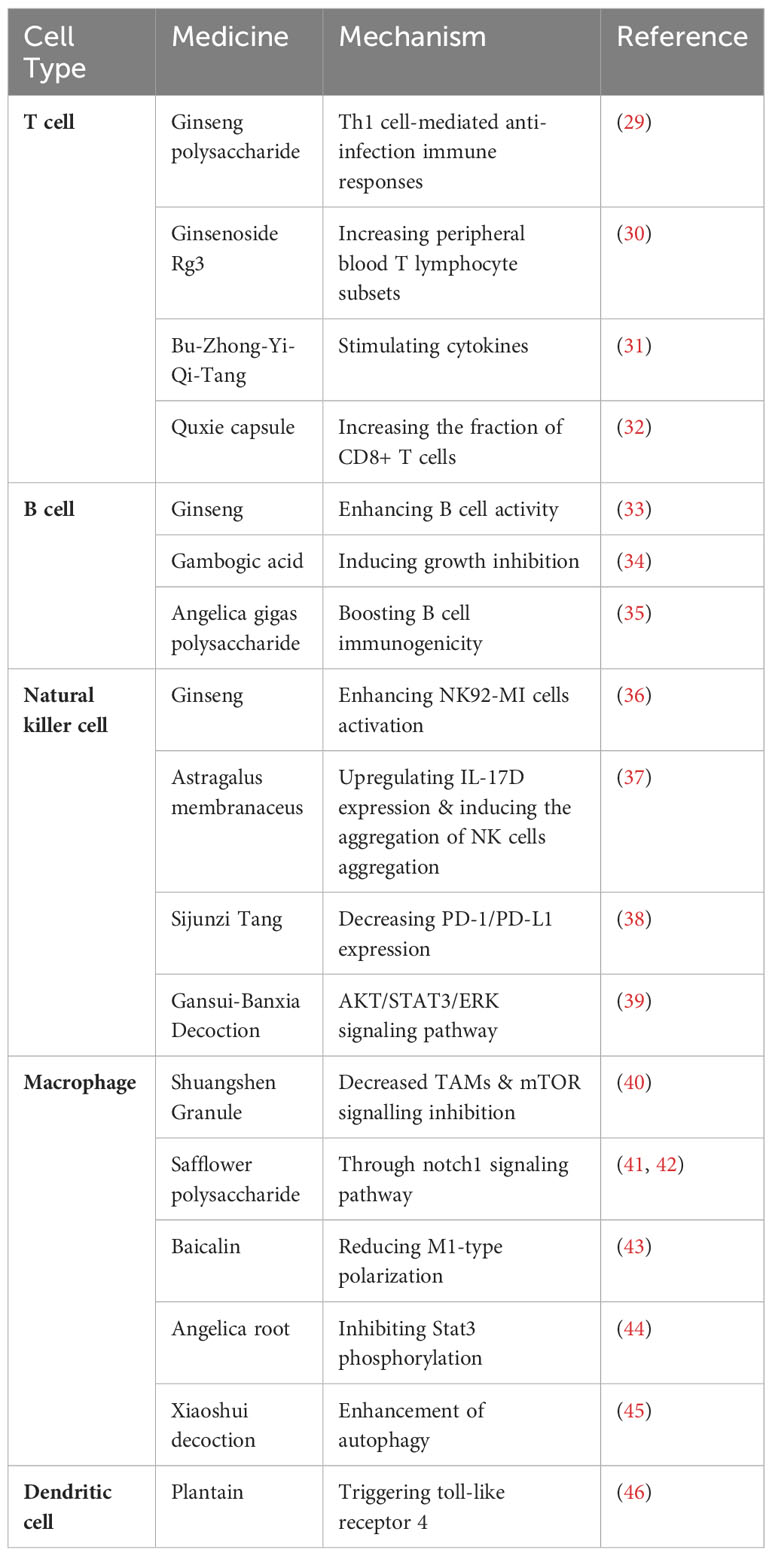
Table 1 mmunological intervention of TCM monomers and compound prescriptions on different cell types.
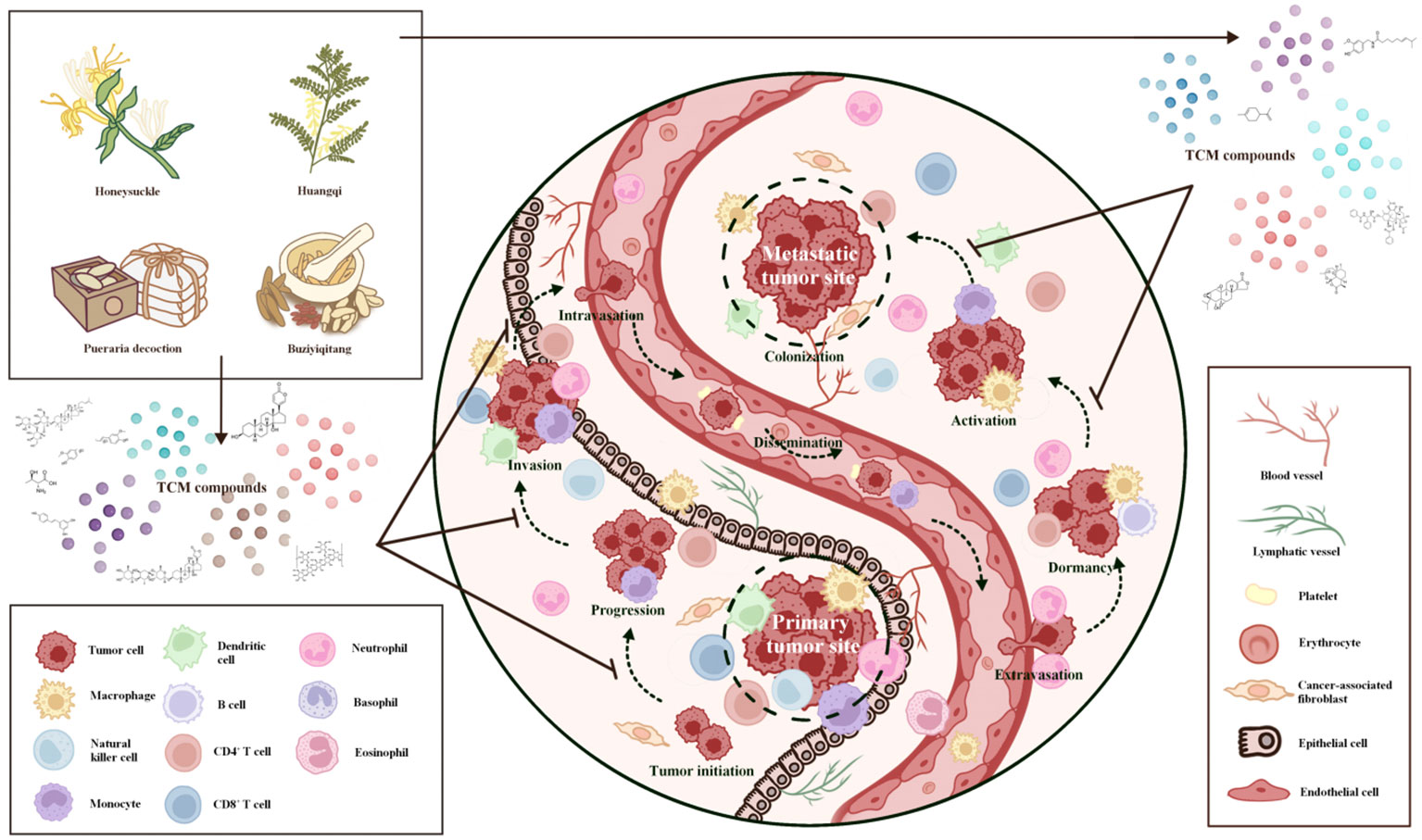
Figure 2 Inhibition of primary and metastatic tumor sites by virtue of traditional Chinese medicine-insights into its therapeutic potential. (Presented in the form of Taiji). TCM demonstrates a multifaceted impact on the suppression of primary and metastatic tumor sites. TCM restrains the growth and expansion of primary tumor cells by regulating cellular proliferation and apoptosis, impeding angiogenesis, and inhibiting tumor-promoting factors. Through its intricate network of bioactive compounds, TCM disrupts key signaling pathways implicated in tumor growth, progression, invasion, and metastasis, ultimately inhibiting tumor biological behaviors. TCM attenuates the formation of pre-metastatic niches by impeding the release of pro-metastatic factors, preventing the colonization and establishment of secondary tumor sites. By interfering with the epithelial-mesenchymal transition process, TCM hinders tumour cells from gaining invasive traits and reduces the likelihood of metastasis. By suppressing the invasive and migratory behavior of tumor cells, TCM curbs their ability to initiate metastasis and invade distant tissues. TCM exerts a modulatory effect on the tumor microenvironment, promoting immune surveillance and enhancing anti-tumor immune responses, which eventually hinders tumor development. These collective actions of TCM underscore its potential as an adjunctive therapy for combating primary and metastatic tumors, holding promise for the advancement of novel therapeutic strategies.
2.1.1 T cells
T cells are a type of leukocytes that play an essential role in the immune system. T cells are responsible for recognizing and attacking foreign pathogens such as viruses, bacteria, and parasites, as well as abnormal or cancerous cells in the body (47). Based on their functions and surface markers, T cells can be categorized into various types, including helper T cells, cytotoxic T cells, regulatory T cells and memory T cells (48). TCM can intervene with T cell activity through various mechanisms. For instance, acidic polysaccharides in ginseng can induce the production of Th1 cells and macrophages, thus synergizing with recombinant interleukin-2 (rIL-2) to generate lymphokine-activated killer cells, potentially contributing to its anti-tumor mechanisms (49). Takei et al. discovered that ginsenosides can promote the transformation of initial T cells into typical Th1 cells, leading to the production of substantial amounts of IFN-γ and IL-4 (50). Additionally, research has indicated that baicalin may achieve the suppression of Th17 cells by reducing RORγt expression, upregulating Foxp3, and decreasing IL-17 expression. Moreover, baicalin can inhibit Th17 cell differentiation through reducing RORγt expression (51). Hence, baicalin holds promise as a therapeutic agent for Th17 cell-mediated diseases. In the tumor microenvironment, immunosuppressive factors secreted by tumor cells lead to increased frequency of CD4+Foxp3+ Tregs and MDSCs, which creates an immunosuppressive environment that reduces CD8 activity. By modulating the immunosuppressive state, Chinese herbal medicine fosters increased CD8+ T cell infiltration while exerting immunomodulatory effects on the tumor microenvironment, ultimately impeding tumor growth (18). For example, ginseng polysaccharide has demonstrated the ability to elevate interleukin-2 (IL-2), interleukin-8 (IL-8), and tumor necrosis factor-alpha (TNF-α) levels, activating Th1 cell-mediated anti-infection immune responses and enhancing immune regulation in the body (29). A meta-analysis has also shown that combining ginsenoside Rg3 with chemotherapy leads to increased peripheral blood T lymphocyte subsets, including CD3, CD4, CD8, and CD4/CD8, in non-small cell lung cancer (NSCLC) patients compared to chemotherapy alone, indicating improved immune system function in these patients (30). Moreover, a study by Liu at al. highlighted the immunomodulatory efficacy of the renowned TCM prescription, Bu-Zhong-Yi-Qi-Tang (BZYQT), which stimulates the production of several cytokines, including IL-2, IL-4, IL-5, and IFN-γ, from T lymphocytes (31). Furthermore, the Quxie capsule has shown its potential to impede intestinal tumorigenesis through the downregulation of pro-inflammatory cytokine expression and the reduction in the proportion of myeloid-derived suppressor cells (MDSCs), while concurrently increasing the fraction of CD8+ T cells within colon tumors (32). Hence, it can be inferred that individual constituents and compounds derived from TCM have the potential to modulate T cell immune function through diverse mechanisms, ultimately manifesting anti-tumor effects.
2.1.2 B cells
B cells, a type of leukocytes, play a crucial role in the immune system by producing antibodies, which are proteins that aid in defending the body against infections. When a foreign pathogen enters the body, B cells recognize the pathogen’s unique antigens and produce antibodies that specifically target and neutralize the pathogen. Also, they are involved in the process of immunological memory, thus being essential for long-term immunity against many infectious diseases. Ginseng, a commonly used Chinese medicine, is believed to possess diverse pharmacological activities, including potential anti-tumor effects. Studies have indicated that ginseng can enhance B cell activity, promote antibody production, and enhance the function of the immune system (33). Shi et al. unearthed the remarkable potential of gambogic acid, a flavonoid compound extracted from the resin of the Garcinia hanburyi, in inducing growth inhibition and apoptosis in activated B-cell-like diffuse large B-cell lymphoma (DLBCL) tumor cells, both in vitro and in vivo (34). Similarly, Kim et al. unveiled the immune-enhancing capabilities of Angelica gigas polysaccharides, which not only boost B-cell immunogenicity but also directly hamper tumor cell adhesion, thereby restraining tumor growth and metastasis (35). Although there are some studies supporting the relevance of TCM to the B cell-related pathway, these studies are still preliminary and more research is demanded to validate and further explore the mechanisms of action of TCM in the anti-tumor field.
2.1.3 Natural killer cells
NK cells are important non-specific immune cells in the body. In the presence of tumor or virus-specific lgG antibodies, NK cells can specifically recognize and eliminate target cells bound to these IgG antibodies. Activated NK cells can also secrete cytokines such as IFN-γ, IL-2 and TNF to play an immunomodulatory role. Wang et al. reported that ginseng polysaccharide can effectively enhance the activation of NK92-MI cells, which then leads to the upregulation of receptors such as NKp30, NKp44, and NKp46, consequently improving the killing ability of NK92-MI cells by increasing the expression of key mediators like perforin and granzyme B (36). Furthermore, Astragalus membranaceus exhibits anti-tumor effects by upregulating IL-17D expression and inducing the aggregation of NK cells in the lung, which plays a vital role in the therapeutic approach for lung cancer (37). The efficacy of Sijunzi Tang, a classic TCM prescription, in impeding the proliferation of colon cancer cells has been substantiated through its ability to decrease the expression of PD-1/PD-L1 while augmenting the activity of NK cells (38). As reported by Feng et al., Gansui-Banxia Decoction (GSBXD) was observed to elevate the proportion of CD3- NK1.1+ NK cells, demonstrating its immunomodulatory potential by inhibiting the AKT/STAT3/ERK signaling pathway and suppressing IL-1β and IFN-γ (39). From the researches above, we can infer that TCM active compounds have a relatively close relationship with the activation of NK cells, then stimulating the natural immune system.
2.1.4 Macrophages
Macrophages are multifunctional non-specific immune cells capable of phagocytosis and destruction of foreign bodies and self-dying cells. In the investigation involving K-Ras G12D spontaneous lung cancer model mice and Lewis lung cancer mice, the intervention of Shuangshen Granule (a combination of Panax quinquefolius L, Cordyceps sinensis, and Panax notoginseng) resulted in a notable reduction in subcutaneous tumor volume and a remarkable decrease in the proportion of F4/80+CD206+ tumor-associated macrophages (TAMs) (40). Further analyses on the genetic level also suggested the effects of Shuangshen Granule on immune microenvironment of lung cancer by modulating bone marrow differentiation through mTOR signalling inhibition (40). A study by Zhang et al. uncovered that safflower polysaccharide induced the polarization of macrophages to M1-type by activating the Notch1 signaling pathway to inhibit the invasion and metastasis of melanocytes (41). Similarly, Ando et al. observed that safflower polysaccharide induced the production of molecules associated with M1-type macrophage polarization in peritoneal macrophages of mice (42). Another study confirmed that baicalin could mitigate acute lung injury in mice by reducing M1-type polarization of U937 macrophages through the inhibition of HMGB-1 (23). Additionally, Angelica polysaccharide was found to suppress tumor metastasis by inhibiting Stat3 phosphorylation in macrophages and preventing their polarization toward the M2 phenotype (43). The TCM compound prescription, Xiaoshui decoction (XSD), has been documented to exhibit a notable capacity in stimulating the proliferation of M1 macrophages and diminishing the presence of M2 macrophages through the enhancement of autophagy. These findings signify the potential of XSD as a promising and innovative therapeutic option for integrated approaches targeting malignant pleural effusion (44). Accordingly, TCM can enhance immune activity by enhancing phagocytosis and killing of macrophages, thus playing the anti-tumor role.
2.1.5 Dendritic cells
Dendritic cells (DCs) act as messengers between the innate and adaptive immune systems. They recognize and capture foreign antigens, such as bacteria, viruses, and tumor cells, and then present them to other immune cells, such as T cells and B cells (16, 45). Moreover, they help prevent the immune system from attacking the body’s own normal cells by presenting self-antigens to immune cells in a way that they do not stimulate an immune response (52). Studies have found that plantain, a perennial plant, plays a positive role in defending against viruses, inhibiting tumor cell proliferation, and enhancing immune function. In addition to whole Psyllium, the polysaccharide extracted from Psyllium is also one of the active components of immune regulation (46). Studies have revealed that the PLP-2 polysaccharide derived from Plantago asiatica L. can promote the phenotypic and functional maturation of dendritic cells and PLP-2 may activate the MAPK and NF-κB pathway by triggering toll-like receptor 4 on DCs (53, 54).
2.2 Modulation of cytokine signaling
Cytokines, essential molecular messengers that orchestrate immune responses, are targets of interest for modulating tumor immunity with TCM compounds. For instance, baicalein, an active ingredient extracted from Scutellaria baicalensis, was reported to play an anti-tumor role by regulating cytokine signaling pathway. It effectively inhibits tumor cell growth, induces apoptosis, and modulates immune cell activity. Notably, it inhibits IL-6 and STAT3 signaling pathways, thus impeding tumor growth and metastasis (55). Sini decoction (SND) is another widely used TCM known for its ability to suppress colorectal cancer liver metastasis and alleviate liver injury in vivo (56). SND achieves this by upregulating IL-2 and IFN-γ and downregulating IL-10 and TGF-β (38). Another traditional prescription, Gansui-Banxia Decoction (GSBXD), created by sage Zhang Zhongjing, is employed to treat stagnation of evil heat and obstruction of qi. Studies by Feng et al. have demonstrated GSBXD’s antitumor immune activity by reducing the accumulation of MDSCs in vivo, possibly achieved through down-regulation of AKT/STAT3/ERK signaling pathways and suppression of IL-1β and IFN-γ (39). Wenjinghuoluo prescription, a TCM compound treatment of rheumatoid arthritis, was discovered to decrease the expression of pro-inflammatory cytokines (TNF-α, IL-1β, IL-6, and IL-17), thus effectively inhibiting bone erosion and osteophyte formation in joints (57). Considering the crucial role of cytokines in tumor occurrence and development, various Chinese medicines hold significant potential for development in regulating cytokine pathways to exert anti-tumor effects.
2.3 Modulation of angiogenesis and vascular normalization
Angiogenesis, the process of forming new blood vessels, is essential for tumor growth and metastasis. Emerging reports indicate that TCM compounds possess anti-angiogenic properties, inhibiting the abnormal and excessive blood vessel formation within the TME (58). These compounds specifically target key molecular pathways involved in angiogenesis, such as the vascular endothelial growth factor (VEGF) signaling. Additionally, TCM compounds have demonstrated the ability to induce vascular normalization, promoting the restoration of a more normal and functional vasculature within the tumor. This normalization can enhance drug delivery, immune cell infiltration, and overall therapeutic efficacy. Notably, β-Elemene stands out as it regulates the expression of crucial molecules implicated in tumor angiogenesis and metastasis, including VEGF, matrix metalloproteinases (MMPs), E-cadherin, N-cadherin, and vimentin (59). Another promising natural phytochemical is Ginsenoside Rg3, which has been extensively studied for its potential in cancer prevention and treatment. Its mechanisms of action encompass a wide range of anti-cancer activities, including apoptosis induction, inhibition of proliferation, metastasis, and angiogenesis, as well as boosting the immune response against cancer cells (30). In the quest for effective anti-angiogenic agents, researchers have looked into other natural compounds such as catechin gallate, astragaloside, and curcumin. These compounds have demonstrated their ability to effectively inhibit angiogenesis, thereby impeding the formation of new blood vessels that are crucial for tumor growth and metastasis (60). Curcumol, another natural substance, has shown promise in restraining cell growth and angiogenesis in non-small cell lung cancer (NSCLC). This potential is attributed to its ability to regulate the SP1/miR-125b-5p/VEGFA pathway, thus suppressing the expression of VEGF and limiting tumor blood vessel formation (61). Additionally, the compound menthol has been found to block the trans-activation of the transient receptor potential vanilloid 1 (TRPV1) by activating the transient receptor potential melastatin subtype 8 (TRPM8) ion channel. This activation inhibits VEGF-induced angiogenesis in specific cancer cells, such as uveal melanoma UM92.1 cells and PC-3 cells, providing a novel approach to target angiogenesis in cancer therapy (62–64). In conclusion, TCM compounds have shown anti-angiogenic properties by targeting key molecular pathways involved in angiogenesis, such as VEGF signaling. They can inhibit abnormal blood vessel formation within the TME and promote vascular normalization. This normalization enhances drug delivery, immune cell infiltration, and overall therapeutic efficacy.
2.4 Modulation of extracellular matrix remodeling
The extracellular matrix (ECM) within the TME plays a crucial role in providing structural support to tumor cells and exerting a significant influence on their behavior. Studies have demonstrated that TCM compounds possess the ability to regulate the intricate processes of ECM remodeling, including the degradation and deposition of ECM components. Notably, certain compounds exhibit compelling anti-fibrotic property, effectively inhibiting the excessive accumulation of collagen and other ECM proteins, which can otherwise impede the infiltration of immune cells (65). Furthermore, TCM compounds display the capacity to modulate the activity of MMPs, a group of enzymes involved in ECM degradation, thus exerting a consequential impact on tumor invasion and metastasis (66). For instance, a notable example is the Kushen injection compound, which has been corroborated to uniformly block the migration and invasion through EMC in colon, brain, and breast cancer cell lines (67). These findings underscore the potential of TCM in regulating the dynamic interactions between tumor cells and the ECM, suggesting their therapeutic relevance in impeding tumor progression and metastasis.
2.5 Modulation of inflammatory and immune responses
Inflammation and immune dysregulation within the TME contribute to tumor growth, immune evasion, and therapy resistance. TCM has been corroborated to exert immunomodulatory effects on tumor cells, influencing inflammatory and immune responses in the TME (68). For instance, extracts derived from Curcuma longa have demonstrated the capacity to modulate immune-inflammatory responses, offering a potential avenue for regulating the occurrence and progression of glioma (69). Furthermore, the extraordinary attributes of honeysuckle and Huangqi have been recognized in their ability to suppress pro-inflammatory cytokines, including IL-6 and TNF-α. These cytokines play pivotal roles in the initiation and progression of tumors (70). The presence of inflammation and immune dysregulation within the TME significantly contributes to tumor growth, immune evasion, and resistance to therapy.
2.6 Targeting immune checkpoints
Immune checkpoints are essential regulatory molecules that help maintain immune homeostasis. However, cancer cells can exploit these checkpoints to evade detection and destruction by the immune system, allowing tumors to evade immune surveillance (71). TCM compounds have shown promise in their ability to modulate immune checkpoint molecules, including PD-1, CTLA-4, and LAG-3 (72). One such example is the Gegen Qinlian decoction (GQD), a classical TCM formula. When used in combination with anti-mouse PD-1 treatment, GQD has been found to downregulate PD-1 expression and increase the levels of IL-2, effectively restoring T-cell functions by suppressing inhibitory checkpoints (73). Berberine (BBR), a well-known anti-inflammatory drug in TCM, has also been identified as a negative regulator of PD-L1 by researchers like Liu et al. Its administration has been shown to enhance the sensitivity of tumor cells to co-cultured T-cells by reducing PD-L1 levels in cancer cells (74). Additionally, Zhang et al. have reported that a compound known as CFF-1 exhibits tumor growth inhibition and prevents lung metastasis by blocking the PD-1/PD-L1 pathway. This action leads to an improved T lymphocyte immune response via the EGFR/JAK1/STAT3 pathway. As a result, CFF-1 shows promise as a potentially effective treatment to counteract tumor immunosuppression, particularly in prostate cancer patients (41). In short, TCM compounds have shown promise in modulating immune checkpoint molecules, such as PD-1, CTLA-4, and LAG-3, which are essential for maintaining immune homeostasis but can be exploited by tumors to evade immune surveillance.
2.7 Immunosuppression and immune escape
TME frequently demonstrates its immunosuppressive characteristics that enable tumors to evade immune surveillance. Extensive research has focused on exploring the potential of TCM compounds to counteract immunosuppression within the TME. One remarkable aspect of TCM compounds is their ability to modulate the activity of immunosuppressive cells, such as regulatory T cells (Tregs) and myeloid-derived suppressor cells (MDSCs). By targeting these cells, TCM compounds effectively reduce their negative impact on the anti-tumor immune response, creating a more conducive environment for fighting cancer (75). Moreover, TCM compounds have demonstrated the ability to inhibit the expression of immune checkpoint molecules, such as PD-1 and CTLA-4, thus reinstating immune cell functionality and facilitating robust tumor immune responses (60, 74, 76). In the pursuit of novel approaches, researchers have explored innovative strategies involving TCM compounds. For instance, co-encapsulation of drugs in nanoparticles has demonstrated encouraging chemo-immunotherapeutic effects in mice with hepatocellular carcinoma (HCC). This approach enhances survival rates without causing significant toxicity, pointing towards a potential breakthrough in cancer treatment (77). Furthermore, specific TCM compounds, like Feiji Recipe, have been identified for their ability to restore T cell functionality within the cancer microenvironment. In non-small cell lung cancer (NSCLC), Feiji Recipe interferes with the indoleamine-2,3-dioxygenase pathway, a critical mediator of immune escape, offering a potential therapeutic avenue to counteract immune evasion in this type of cancer (78). By effectively addressing immunosuppression and reinvigorating the anti-tumor immune response, TCM-based treatments offer a potential complement or alternative to conventional therapies.
3 New findings of TCM natural products and derivatives as immunomodulating agents in cancer treatment
Numerous studies have provided evidence supporting the multifaceted potential of TCM. These properties encompass antibacterial, antioxidant, anti-inflammatory, anti-proliferative, anti-cancer, and various other beneficial effects. In this part, pertinent studies on several typical TCM natural products that have potential anti-cancer property are expounded in the following paragraphs and summarized in Table 2 (39, 79, 80, 82–124, 132). Additionally, some closed and ongoing national clinical trials (NCT) on the association between TCMs and multiple cancer types are also displayed in Table 3.
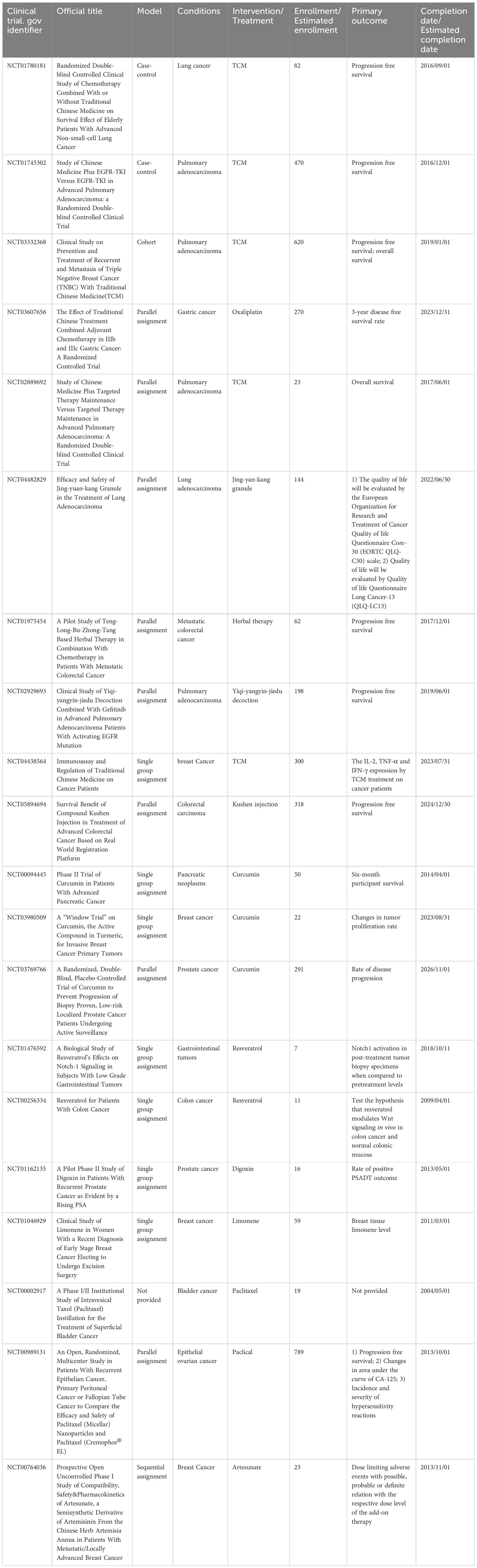
Table 3 Closed and ongoing national clinical trials (NCT) on the association between TCMs and multiple cancer types.
Polyphenols represent a class of naturally occurring compounds abundantly found in multiple plants, encompassing fruits, vegetables, and herbs. These molecules possess distinctive chemical and biological properties owing to their multiple phenol rings. Research has consistently revealed the wide-ranging health benefits of polyphenols, including their remarkable anti-inflammatory, antioxidant, and anti-cancer effects (125, 126). These compounds have been observed to exert modulatory influences on a series of cellular pathways critical to disease development and progression, such as cell cycle regulation, apoptosis, and angiogenesis (125, 127). Curcumin, derived from the dried rhizome of turmeric, is rich in phenols, terpenoids, flavonoids, and other constituents. Specifically categorized as a diphenyl heptane within the phenolic group, curcumin treatment has been discovered to effectively impede the proliferation and promote the apoptosis of colon cancer cells (128). Notably, curcumin treatment elevates the expression of miR-206, which subsequently influences the functional behavior of colon cancer cells. Via augmenting colon cancer cell apoptosis and suppressing PD-L1 expression, miR-206 enhances the cytotoxic effects of T cells on tumor cells. This potentiation is achieved through the inhibition of PD-L1 via suppression of the JAK/STAT3 pathway (57). These compounds, with their distinctive phenol rings, have been consistently shedding light on new horizons in cancer immunotherapy.
Resveratrol (Rsv), another prominent polyphenol compound, is predominantly sourced from plants such as peanuts, grapes, Polygonum cuspidatum, and mulberries (82). Resveratrol exhibits a multitude of pharmacological property, notably its antioxidant, anti-inflammatory, and anti-cancer activities, which render it highly beneficial for the treatment of gastric diseases (83). Resveratrol’s mechanisms of action involve modulating the SLC7A11-HMMR interaction, activating ferroptosis, enhancing CD8+ T cell cytotoxicity, and regulating the tumor immune microenvironment (82). In summary, polyphenols offer a promising avenue in the field of health and disease management, with their diverse chemical properties and potential effects on essential cellular pathways. The specific polyphenols curcumin and resveratrol have shown remarkable impacts on cancer cells and the immune microenvironment, holding significant potential for therapeutic applications.
Cardiac glycosides, natural sterols derived from plants and animals, are known for their cardiotonic properties, enhancing cardiac contractions by targeting the cellular sodium-potassium ATPase pump (85). Extensive evidence suggests the remarkable antitumor property of cardiac glycosides, making them highly recommended for the treatment of diverse cancer types (85, 129, 130). Moreover, mutated or dysregulated transcription factors have emerged as promising therapeutic targets amenable to selective intervention. Neriifolin, a cardiac glycoside, has demonstrated its potential in suppressing malignant characteristics of prostate cancer cells. It achieves this by activating endoplasmic reticulum stress (ERS), which impacts the induction of antitumor inhibitory receptors, thereby enhancing DNA damage and apoptosis (131). Digoxin, a classic cardiac glycoside, exhibits potential in reducing inflammation and fibrosis in a mouse model of pulmonary fibrosis. Its mechanism involves inhibiting the mTORC2/AKT/IRF4 signaling pathway and macrophage M2 polarization (86). Bufalin, a major component of the dried secretion of Bufo, represents another cardiac steroid widely employed for therapeutic purposes. Bufalin exerts anti-breast cancer effects through multiple pathways and targets. It inhibits the PI3K/AKT pathway, induces apoptosis via the mitochondrial pathway, triggers programmed necrosis through the RIP11/RIP3/PARP-1 signaling pathway, and hinders cell proliferation and invasion by influencing cell cycle progression and inhibiting epithelial-mesenchymal transition (EMT) (89, 90, 132). In summation, cardiac glycosides have etched their mark not only in the realm of cardiotonic therapy but also as a burgeoning frontier in the battle against cancer.
Terpenoids, an expansive and diverse class of organic compounds prevalent in numerous plants, including cannabis, contribute significantly to the distinctive aroma and flavor of various botanical specimens. Additionally, terpenoids are renowned for their remarkable medicinal property, with several of them demonstrating potent anti-cancer effects. One such terpenoid, Thymol, can induce an anticancer effect in acute promyelocytic leukemia cells by arresting the cell cycle at G0/G1 phase and mitochondrial depolarization through reactive oxygen species production followed by mitochondrial membrane deterioration (133). Another noteworthy terpenoid, limonene, abundantly found in citrus fruits and specific cannabis strains, has shown anti-cancer effects by inducing apoptosis in breast cancer cells, promoting programmed cell death in the cancerous cells (94). Paclitaxel, a chemotherapy drug derived from the Pacific yew tree, hinders cancer cell division and growth by effectively inhibiting the breakdown of microtubules, cellular structures vital for maintaining cell shape and facilitating cell division. By binding to microtubules, paclitaxel prevents their disassembly, thereby thwarting the ability of cancer cells to divide and proliferate (97). Artemisinin, a compound derived from the sweet wormwood plant and long utilized in TCM for malaria treatment, has recently been discovered to possess anti-cancer property by generating free radicals within cancer cells, leading to oxidative stress and subsequent cell death. Artemisinin has exhibited notable efficacy against breast cancer cells (99, 100). Triptolide, derived from the thunder god vine plant and traditionally employed in Chinese medicine to address many conditions, including inflammation and autoimmune diseases, has also demonstrated potent anti-cancer property inhibiting the NF-kB protein, a crucial regulator of cell survival, and inducing apoptosis in cancer cells (102–104).
Polysaccharides, intricate carbohydrates abundant in plants, fungi, and bacteria, exhibit a diverse array of health benefits, including their remarkable anti-cancer property. Extensive research has demonstrated that polysaccharides possess the ability to impede the growth and proliferation of cancer cells through activating the immune system and promoting the production of cytokines. After the immune system is activated, polysaccharides facilitate the identification and elimination of cancer cells (109). Moreover, polysaccharides showcase direct anti-cancer effects in addition to their immune-boosting attributes. Notably, polysaccharides derived from mushrooms have demonstrated desirable efficacy in inhibiting cancer cell growth by inducing apoptosis or programmed cell death. Furthermore, by inducing apoptosis and inhibiting the formation of blood vessels crucial for cancer cell proliferation and metastasis, mushroom polysaccharides demonstrate potent anti-cancer efficacy (29, 109, 110). For instance, lentinan, a polysaccharide extracted from the shiitake mushroom, has garnered significant attention for its anti-cancer potential. It effectively hinders cancer cell growth and proliferation by activating the immune system and stimulating cytokine production, thus enhancing immune responses against cancer cells (112, 134). Clinical trials evaluating lentinan have shown promising therapeutic capabilities, particularly in gastric cancer and colorectal cancer. Patients treated with lentinan experienced improved survival rates and enhanced quality of life, as the polysaccharide not only combats cancer cells but also alleviates the side effects of chemotherapy and radiation therapy (112). In essence, polysaccharides, with their immune-activating and direct anti-cancer attributes, stand at the forefront of the evolving landscape of cancer therapy.
Saponins, a group of plant glycosides with their distinctive ability to generate abundant foam in aqueous solutions, have garnered attention due to their profound anti-cancer effects (116, 135). These compounds effectively impede cancer cell growth and proliferation by inducing apoptosis. In Chen’s clinical trial, a significant discovery was made regarding the therapeutic potential of combining ginsenoside Rg3 with MF chemotherapy. The findings revealed a notable reduction in serum VEGF concentration, resulting in improved survival rates for patients with advanced gastric cancer (136). Another notable saponin, Saikosaponin D, demonstrated impressive efficacy in enhancing apoptosis mediated by TNF-α in HepG2 cells. This effect was attributed to the inhibition of NF-κB activation and downregulation of target genes associated with cancer cell proliferation, angiogenesis, invasion, and survival (137). Notably, ginsenoside Rg-3 has recently gained recognition in China as a promising anti-angiogenic and anti-cancer drug (116). By activating the immune system and stimulating cytokine production, saponins can identify and suppress the activity of cancer cells. Panax notoginseng Saponins (PNS) have shown cytotoxicity and enhanced chemosensitivity in pancreatic cancer cells treated with Gemcitabine. This effect was achieved through the inhibition of autophagy and promotion of apoptosis, providing a potential treatment option for pancreatic cancer (116). These compounds, by effectively curbing cancer cell growth and proliferation through apoptosis induction, offer a compelling avenue for exploring innovative cancer therapeutics.
Capsaicin, a vanilloid phytochemical found in chili peppers and pepper extracts, exhibits significant anti-cancer properties against various human cancers, including lung, gastric, colon, and breast cancer. Its anti-cancer effects are attributed to its ability to induce apoptosis and inhibit tumor cell proliferation. Additionally, capsaicin exhibits noteworthy anti-inflammatory property, which contribute to its cancer-preventive potential by mitigating chronic inflammation. In specific cancer types, capsaicin has been observed to induce apoptosis in ORL-48 cells through the intrinsic apoptotic pathway, involving disruption of mitochondrial membrane potential and activation of caspase-3, -7, and -9 (138). In bladder cancer cells, capsaicin downregulates tNOX expression, leading to enhanced apoptosis and reduced cell growth. The inhibition of extracellular regulated protein kinases activation by capsaicin results in decreased phosphorylation of paxillin and focal adhesion kinase (FAK), leading to reduced cell migration (119). Thoennissen et al. found that in breast cancer cells, capsaicin modulates the EGFR/HER-2 pathway, suggesting its potential role in breast cancer treatment and prevention (120). Furthermore, Jin et al. reported capsaicin’s profound anti-proliferative effects on colon cancer cells involve cell cycle arrest in the G0/G1 phase and promotion of apoptosis, associated with increased expression of p21, Bax, and cleaved poly ADP-ribose polymerase (PAARP), which play essential roles in regulating cell cycle progression and apoptosis (121). In conclusion, capsaicin, whose impact reverberates across various human cancers, from lung and gastric to colon and breast cancer, offers a promising avenue for novel cancer interventions.
4 Future perspectives
Tumor immunotherapy can activate the immune system to identify and eliminate cancer cells. Accordingly, cancer cells produce suppressive proteins to alleviate immune responses and evade immune surveillance. Immunotherapy agents possess the capability to block these proteins, thereby facilitating the immune system’s efficient recognition and elimination of cancer cells (5, 129, 139). The paramount significance of tumor immunotherapy stems from its capability to harness the formidable potential of the immune system to destroy cancer cells. Diverging from conventional cancer treatments like chemotherapy and radiation therapy, which indiscriminately harm both normal and cancerous cells, immunotherapy selectively targets cancer cells and spares normal cells from harm. Consequently, this approach holds promise as a more potent and less toxic treatment modality for cancer patients (140). Additionally, immunotherapy has displayed promising outcomes in the treatment of multiple cancer types, including melanoma, lung cancer, and bladder cancer (141, 142). It has also demonstrated desirable efficacy in treating cancers that have developed resistance to conventional therapeutic interventions.
Despite its considerable potential benefits, tumor immunotherapy is accompanied by several limitations that necessitate attention and resolution. One primary limitation is the heterogeneous response observed among patients undergoing immunotherapy (143). While some individuals have exhibited remarkable and favorable responses, others have not experienced any discernible benefits. This heterogeneity may arise from variances in patients’ immune system profiles or the distinct characteristics of their specific cancers. Another limitation of immunotherapy pertains to the potential occurrence of side effects (144). Although immunotherapy generally exhibits lower toxicity compared to traditional cancer treatments, it can still induce immune-related side effects such as inflammation, fatigue, and cutaneous manifestations (144, 145). In certain instances, these side effects may severely manifest and urgently demand intervention. The third limitation is its relatively high expenditure. Immunotherapy drugs often incur greater expenses than conventional cancer treatments, thereby posing a financial barrier to access for some cancer patients with low income.
TCM harbors notable potential in the realm of tumor immunity for several compelling reasons. Acknowledging the interconnected nature of the immune system with other bodily systems, TCM endeavors to rectify underlying imbalances, thus enhancing the body’s inherent defense mechanisms, including tumor immunity (146). Secondly, TCM compounds have exhibited immunomodulatory property, influencing the function of immune cells, cytokine signaling, and immune responses. These compounds possess the capacity to augment the activity of immune cells crucial for tumor immunity, while concurrently suppressing immunosuppressive cell populations. Through modulating the immune response, TCM compounds are promising in promoting anti-tumor immune responses and bolstering tumor eradication. They can concurrently target multiple immune checkpoints, cytokines, and cellular pathways, culminating in a comprehensive and multi-faceted approach that augments tumor immunity. Such multimodal effects hold the potential to surmount the limitations associated with therapies focusing on a single target and subsequently enhance therapeutic outcomes. Furthermore, TCM places considerable emphasis on personalized medicine, tailoring treatments to address the specific requirements and imbalances exhibited by each individual patient (147). This personalized approach acknowledges the inherent variability of tumor immunity among patients, attributable to factors such as genetic predisposition, immune status, and the distinctive characteristics of the tumor microenvironment. Lastly, TCM boasts a rich historical heritage, spanning centuries of utilization in China and other Asian nations, thereby accumulating extensive clinical experience in managing a diverse range of health conditions. Traditional herbal formulations and treatment methodologies have been refined over time based on empirical observations and accumulated wisdom. This fusion of historical knowledge with modern scientific investigations presents a distinctive opportunity to harness the potential of TCM in optimizing tumor immunity.
TCM encompasses an extensive repertoire of herbal compounds, possessing infinite potential for immunomodulation. One avenue of progress involves the identification and isolation of specific active compounds present in TCM formulations that exert robust effects on tumor immunity. By isolating these compounds and subjecting them to rigorous scientific scrutiny, their mechanisms of action can be elucidated, enabling a more precise evaluation of their efficacy and safety. Further investigations are warranted to comprehend the underlying mechanisms by which TCM compounds modulate tumor immunity. This necessitates exploring the signaling pathways, cellular interactions, and molecular targets involved in the immunomodulatory effects of TCM compounds. Mechanistic studies offer invaluable insights into the precise actions of TCM compounds on immune cells, cytokine signaling, immune checkpoints, and the tumor microenvironment, thereby guiding the advancement of targeted therapeutic interventions. Given the complexity and heterogeneity of tumor immunity, which varies among individuals and tumor types (148), the development of personalized TCM treatment strategies hinges on the identification of biomarkers capable of predicting treatment responses and patient outcomes. The identification of such biomarkers can facilitate the selection of TCM compounds and formulations tailored to individual patients, optimizing their potential to enhance tumor immunity and improve treatment efficacy (149). By steadfastly pursuing the above exploratory directions, TCM can continuously evolve as a valuable approach for augmenting tumor immunity, ultimately contributing to the advancement of more efficacious and personalized strategies for cancer treatment.
The emergence of precision medicine has fundamentally transformed the landscape of cancer treatment, revolutionizing therapeutic approaches by tailoring interventions to individual patients based on their unique genetic and molecular characteristics (150). In this context, TCM emerges as a highly promising complementary modality, capable of augmenting the efficacy and personalization of cancer immunotherapy. Through the identification of specific TCM compounds and formulations that align with individual tumor and immune profiles, clinicians are more likely to optimize treatment strategies, enhance therapeutic outcomes, and minimize adverse effects. Moreover, the integration of TCM with conventional cancer therapies like chemotherapy and radiation, paves the way for synergistic effects and novel therapeutic avenues. Notably, studies have indicated that TCM compounds can enhance the effectiveness of these treatments by sensitizing tumor cells to cytotoxic agents, modulating the tumor microenvironment, and mitigating treatment-associated side effects (72). Furthermore, the combination of TCM with emerging immunotherapies, such as cancer vaccines and chimeric antigen receptor (CAR) T-cell therapy, embraces a promising future in unleashing robust anti-tumor immune responses and ultimately improving patient outcomes. Finally, the combination of traditional wisdom and modern scientific advancements assumes paramount importance in propelling the progress of TCM within the realm of cancer immunotherapy (151). To this end, rigorous scientific investigations on preclinical and clinical studies are indispensable for elucidating the mechanisms of action, validating efficacy, and ensuring the safety of TCM compounds.
5 Conclusion
The potential of TCM in the realm of anti-cancer immunotherapy is supposed to be cherished, and its immunoregulatory mechanisms should be shed light on. This review summarizes the current state of research, also categorizing and expounding broadly applied TCM approaches that possess anti-cancer property. Additionally, the curative effects and underlying mechanisms of several frequently used Chinese medicines are discussed meticulously. Moreover, the mechanism of several commonly used TCM monomers and compound prescription were explained in detail. Overall, our work presents a comprehensive overview of the progress made in TCM for cancer treatment, providing valuable insights into its advancement in this field.
Author contributions
KM: Resources, Visualization, Writing – original draft. WL: Data curation, Visualization, Writing – original draft. JX: Visualization, Writing – review & editing. ZQ: Conceptualization, Resources, Project administration, Visualization, Writing – review & editing. QZ: Conceptualization, Funding acquisition, Project administration, Visualization, Writing – review & editing.
Funding
The author(s) declare financial support was received for the research, authorship, and/or publication of this article. This work is supported by the Project of Wuxi Health Commission (Q202109) and General Project of Wuxi Medical Center of Nanjing Medical University (WMCG202326).
Conflict of interest
The authors declare that the research was conducted in the absence of any commercial or financial relationships that could be construed as a potential conflict of interest.
Publisher’s note
All claims expressed in this article are solely those of the authors and do not necessarily represent those of their affiliated organizations, or those of the publisher, the editors and the reviewers. Any product that may be evaluated in this article, or claim that may be made by its manufacturer, is not guaranteed or endorsed by the publisher.
Abbreviations
TME, tumor microenvironment; TCM, Traditional Chinese Medicine; NF-kB, nuclear factor-kappa B; IL, interleukin; TNF-α, tumor necrosis factor-alpha; MDSC, myeloid-derived suppressor cell; PSP, pupa polysaccharide; LPS, lipopolysaccharide; ConA, concanavalin A; GSBXD, Gansui-Banxia Decoction; TAM, tumor-associated macrophage; XSD, Xiaoshui decoction; DC, endritic cell; SND, Sini decoction; VEGF, vascular endothelial growth factor; MMP, matrix metalloproteinase; TRPM8, transient receptor potential melastatin subtype 8; TRPV1, transient receptor potential vanilloid 1; ECM, extracellular matrix; GQD, Gegen Qinlian decoction; BBR, Berberine; Treg, regulatory T cell; NSCLC, non-small cell lung cancer; NCT, national clinical trial; ERS, reticulum stress; EMT, epithelial-mesenchymal transition; PNS, Panax notoginseng Saponins; FAK, focal adhesion kinase; CAR, chimeric antigen receptor.
References
1. Zaimy MA, Saffarzadeh N, Mohammadi A, Pourghadamyari H, Izadi P, Sarli A, et al. New methods in the diagnosis of cancer and gene therapy of cancer based on nanoparticles. Cancer Gene Ther (2017) 24:233–43. doi: 10.1038/cgt.2017.16
2. Wang J-J, Lei K-F, Han F. Tumor microenvironment: recent advances in various cancer treatments. Eur Rev Med Pharmacol Sci (2018) 22:3855–64. doi: 10.26355/eurrev_201806_15270
3. Bejarano L, Jordāo MJC, Joyce JA. Therapeutic targeting of the tumor microenvironment. Cancer Discovery (2021) 11:933–59. doi: 10.1158/2159-8290.CD-20-1808
4. Mz J, Wl J. The updated landscape of tumor microenvironment and drug repurposing, Signal Transduct. Targeting Ther (2020) 5(1):166. doi: 10.1038/s41392-020-00280-x
5. Zhang Y, Zhang Z. The history and advances in cancer immunotherapy: understanding the characteristics of tumor-infiltrating immune cells and their therapeutic implications. Cell Mol Immunol (2020) 17:807–21. doi: 10.1038/s41423-020-0488-6
6. Wang D-R, Wu X-L, Sun Y-L. Therapeutic targets and biomarkers of tumor immunotherapy: response versus non-response. Signal Transduction Targeting Ther (2022) 7:331. doi: 10.1038/s41392-022-01136-2
7. Finck AV, Blanchard T, Roselle CP, Golinelli G, June CH. Engineered cellular immunotherapies in cancer and beyond. Nat Med (2022) 28:678–89. doi: 10.1038/s41591-022-01765-8
8. Jogalekar MP, Rajendran RL, Khan F, Dmello C, Gangadaran P, Ahn B-C. CAR T-Cell-Based gene therapy for cancers: new perspectives, challenges, and clinical developments. Front Immunol (2022) 13:925985. doi: 10.3389/fimmu.2022.925985
9. Huang Y, Ma S, Wang Y, Yan R, Wang S, Liu N, et al. The role of traditional Chinese herbal medicines and bioactive ingredients on ion channels: A brief review and prospect. CNS Neurol Disord Drug Targets (2019) 18:257–65. doi: 10.2174/1871527317666181026165400
10. Ma H-D, Deng Y-R, Tian Z, Lian Z-X. Traditional Chinese medicine and immune regulation. Clin Rev Allergy Immunol (2013) 44:229–41. doi: 10.1007/s12016-012-8332-0
11. Liu R, Li X, Huang N, Fan M, Sun R. Toxicity of traditional Chinese medicine herbal and mineral products. Adv Pharmacol San Diego Calif (2020) 87:301–46. doi: 10.1016/bs.apha.2019.08.001
12. Ruhlmann CH, Herrstedt J. New treatments on the horizon for chemoradiotherapy-induced nausea and vomiting, Expert Opin. Pharmacother (2016) 17:1623–9. doi: 10.1080/14656566.2016.1202923
13. Wang Z, Liu W, Zhang J, Chen X, Wang J, Wang K, et al. Antiemetic prophylaxis for chemoradiotherapy-induced nausea and vomiting in locally advanced head and neck squamous cell carcinoma: a prospective phase II trial. Strahlenther. Onkol (2022) 198:949–57. doi: 10.1007/s00066-022-01958-7
14. Rückert M, Flohr A-S, Hecht M, Gaipl US. Radiotherapy and the immune system: More than just immune suppression. Stem Cells Dayt. Ohio (2021) 39:1155–65. doi: 10.1002/stem.3391
15. Rallis KS, Lai Yau TH, Sideris M. Chemoradiotherapy in cancer treatment: rationale and clinical applications. Anticancer Res (2021) 41:1–7. doi: 10.21873/anticanres.14746
16. Li J, Li J, Zhang F. The immunoregulatory effects of Chinese herbal medicine on the maturation and function of dendritic cells. J Ethnopharmacol (2015) 171:184–95. doi: 10.1016/j.jep.2015.05.050
17. Shen C, Li J, Lian Y, Lan H, Pu F, Zhang W, et al. Chinese herbal medicine for incomplete immune reconstruction in patients with AIDS undergoing antiretroviral treatment: A systematic review of randomized trials. J Tradit. Chin Med Sci (2021) 8:291–301. doi: 10.1016/j.jtcms.2021.10.005
18. Xiang M-F, Jin C-T, Sun L-H, Zhang Z-H, Yao J-J, Li L-C. Efficacy and potential mechanisms of Chinese herbal compounds in coronavirus disease 2019: advances of laboratory and clinical studies. Chin Med (2021) 16:130. doi: 10.1186/s13020-021-00542-y
19. Wang S, Long S, Deng Z, Wu W. Positive role of Chinese herbal medicine in cancer immune regulation. Am J Chin Med (2020) 48:1577–92. doi: 10.1142/S0192415X20500780
20. Qi Y, Gao F, Hou L, Wan C. Anti-inflammatory and immunostimulatory activities of astragalosides. Am J Chin Med (2017) 45:1157–67. doi: 10.1142/S0192415X1750063X
21. Hoffman RD, Li C-Y, He K, Wu X, He B-C, He T-C, et al. Chinese herbal medicine and its regulatory effects on tumor related T cells. Front Pharmacol (2020) 11:492. doi: 10.3389/fphar.2020.00492
22. Bai L, Li X, He L, Zheng Y, Lu H, Li J, et al. Antidiabetic potential of flavonoids from traditional Chinese medicine: A review. Am J Chin Med (2019) 47:933–57. doi: 10.1142/S0192415X19500496
23. Tao Y, Zhan S, Wang Y, Zhou G, Liang H, Chen X, et al. Baicalin, the major component of traditional Chinese medicine Scutellaria baicalensis induces colon cancer cell apoptosis through inhibition of oncomiRNAs. Sci Rep (2018) 8:14477. doi: 10.1038/s41598-018-32734-2
24. Bhatia NK, Modi P, Sharma S, Deep S. Quercetin and baicalein act as potent antiamyloidogenic and fibril destabilizing agents for SOD1 fibrils. ACS Chem Neurosci (2020) 11:1129–38. doi: 10.1021/acschemneuro.9b00677
25. Yang D, Wang T, Long M, Li P. Quercetin: its main pharmacological activity and potential application in clinical medicine. Oxid Med Cell Longev (2020) 2020:e8825387. doi: 10.1155/2020/8825387
26. Dong C. Cytokine regulation and function in T cells. Annu Rev Immunol (2021) 39:51–76. doi: 10.1146/annurev-immunol-061020-053702
27. Reuter S, Charlet J, Juncker T, Teiten M-H, Dicato M, Diederich M. Effect of curcumin on nuclear factor kappaB signaling pathways in human chronic myelogenous K562 leukemia cells. Ann N Y. Acad Sci (2009) 1171:436–47. doi: 10.1111/j.1749-6632.2009.04731.x
28. Park J-Y, Sohn H-Y, Koh YH, Jo C. Curcumin activates Nrf2 through PKCδ-mediated p62 phosphorylation at Ser351. Sci Rep (2021) 11:8430. doi: 10.1038/s41598-021-87225-8
29. Kim M, Yi Y-S, Kim J, Han SY, Kim SH, Seo DB, et al. Effect of polysaccharides from a Korean ginseng berry on the immunosenescence of aged mice. J Ginseng Res (2018) 42:447–54. doi: 10.1016/j.jgr.2017.04.014
30. Sun M, Ye Y, Xiao L, Duan X, Zhang Y, Zhang H. Anticancer effects of ginsenoside Rg3 (Review). Int J Mol Med (2017) 39:507–18. doi: 10.3892/ijmm.2017.2857
31. Liu L, Hu L, Yao Z, Qin Z, Idehara M, Dai Y, et al. Mucosal immunomodulatory evaluation and chemical profile elucidation of a classical traditional Chinese formula, Bu-Zhong-Yi-Qi-Tang. J Ethnopharmacol (2019) 228:188–99. doi: 10.1016/j.jep.2018.08.003
32. Zhang Z, Chen Y, Zheng Y, Wang L, Shen S, Yang G, et al. Quxie capsule alleviates colitis-associated colorectal cancer through modulating the gut microbiota and suppressing A. fumigatus-induced aerobic glycolysis. Integr Cancer Ther (2022) 21:15347354221138534. doi: 10.1177/15347354221138534
33. Kachur K, Suntres ZE. The antimicrobial properties of ginseng and ginseng extracts. Expert Rev Anti Infect Ther (2016) 14:81–94. doi: 10.1586/14787210.2016.1118345
34. Shi X, Lan X, Chen X, Zhao C, Li X, Liu S, et al. Gambogic acid induces apoptosis in diffuse large B-cell lymphoma cells via inducing proteasome inhibition. Sci Rep (2015) 5:9694. doi: 10.1038/srep09694
35. Kim SH, Lee SW, Park HJ, Lee SH, Im WK, Kim YD, et al. Anti-cancer activity of Angelica gigas by increasing immune response and stimulating natural killer and natural killer T cells. BMC Complement. Altern. Med (2018) 18:218. doi: 10.1186/s12906-018-2277-7
36. Sun Y, Guo M, Feng Y, Zheng H, Lei P, Ma X, et al. Effect of ginseng polysaccharides on NK cell cytotoxicity in immunosuppressed mice. Exp Ther Med (2016) 12:3773–7. doi: 10.3892/etm.2016.3840
37. Sha X, Xu X, Liao S, Chen H, Rui W. Evidence of immunogenic cancer cell death induced by honey-processed Astragalus polysaccharides in vitro and in vivo. Exp Cell Res (2022) 410:112948. doi: 10.1016/j.yexcr.2021.112948
38. Chen J, Zheng X, Xu G, Wang B, Hu L, Mao J, et al. Sini decoction inhibits tumor progression and enhances the anti-tumor immune response in a murine model of colon cancer. Comb. Chem High Throughput Screen. (2023) 26(14):2517–26. doi: 10.2174/1386207326666230320103437
39. Feng X-Y, Chen B-C, Li J-C, Li J-M, Li H-M, Chen X-Q, et al. Gansui-Banxia Decoction extraction inhibits MDSCs accumulation via AKT /STAT3/ERK signaling pathways to regulate antitumor immunity in C57bl/6 mice. Phytomedicine Int J Phytother. Phytopharm (2021) 93:153779. doi: 10.1016/j.phymed.2021.153779
40. Wei H, Guo C, Zhu R, Zhang C, Han N, Liu R, et al. Shuangshen granules attenuate lung metastasis by modulating bone marrow differentiation through mTOR signalling inhibition. J Ethnopharmacol (2021) 281:113305. doi: 10.1016/j.jep.2020.113305
41. Zhang Y, Wei Y, Jiang S, Dang Y, Yang Y, Zuo W, et al. Traditional Chinese medicine CFF-1 exerts a potent anti-tumor immunity to hinder tumor growth and metastasis in prostate cancer through EGFR/JAK1/STAT3 pathway to inhibit PD-1/PD-L1 checkpoint signaling. Phytomedicine Int J Phytother. Phytopharm (2022) 99:153939. doi: 10.1016/j.phymed.2022.153939
42. de Camargo JBB, Brigatto FA, Zaroni RS, Germano MD, Souza D, Bacurau RF, et al. Does beta-alanine supplementation enhance adaptations to resistance training? A randomized, placebo-controlled, double-blind study. Biol Sport (2023) 40:217–24. doi: 10.5114/biolsport.2023.112967
43. Shen J, Zhang M, Zhang K, Qin Y, Liu M, Liang S, et al. Effect of Angelica polysaccharide on mouse myeloid-derived suppressor cells. Front Immunol (2022) 13:989230. doi: 10.3389/fimmu.2022.989230
44. Jin Z, Shen C, Zhang H, Qi R, Guo Q, Liu R, et al. Chinese medicine Xiaoshui decoction inhibits Malignant pleural effusion in mice and mediates tumor-associated macrophage polarization by activating autophagy. J Ethnopharmacol (2020) 249:112412. doi: 10.1016/j.jep.2019.112412
45. Liu J, Zhang X, Cheng Y, Cao X. Dendritic cell migration in inflammation and immunity. Cell Mol Immunol (2021) 18:2461–71. doi: 10.1038/s41423-021-00726-4
46. Gao J, Zhang Y-N, Cui J, Zhang J, Ming Y, Hao Z, et al. A polysaccharide from the whole plant of Plantago asiatica L. Enhances the antitumor activity of dendritic cell-based immunotherapy against breast cancer. Front Pharmacol (2021) 12:678865. doi: 10.3389/fphar.2021.678865
47. Shepherd FR, McLaren JE. T cell immunity to bacterial pathogens: mechanisms of immune control and bacterial evasion. Int J Mol Sci (2020) 21:6144. doi: 10.3390/ijms21176144
48. Saravia J, Chapman NM, Chi H. Helper T cell differentiation. Cell Mol Immunol (2019) 16:634–43. doi: 10.1038/s41423-019-0220-6
49. Kim KH, Lee YS, Jung IS, Park SY, Chung HY, Lee IR, et al. Acidic polysaccharide from Panax ginseng, ginsan, induces Th1 cell and macrophage cytokines and generates LAK cells in synergy with rIL-2. Planta Med (1998) 64:110–5. doi: 10.1055/s-2006-957385
50. Takei M, Tachikawa E, Umeyama A. Dendritic cells promoted by ginseng saponins drive a potent Th1 polarization. biomark Insights (2008) 3:269–86. doi: 10.4137/bmi.s585
51. Yang J, Yang X, Chu Y, Li M. Identification of baicalin as an immunoregulatory compound by controlling TH17 cell differentiation. PloS One (2011) 6:e17164. doi: 10.1371/journal.pone.0017164
52. Wculek SK, Cueto FJ, Mujal AM, Melero I, Krummel MF, Sancho D. Dendritic cells in cancer immunology and immunotherapy. Nat Rev Immunol (2020) 20:7–24. doi: 10.1038/s41577-019-0210-z
53. Jiang L, Huang D, Nie S, Xie M. Polysaccharide isolated from seeds of Plantago asiatica L. induces maturation of dendritic cells through MAPK and NF-κB pathway. Saudi J Biol Sci (2018) 25:1202–7. doi: 10.1016/j.sjbs.2017.09.011
54. Huang D, Nie S, Jiang L, Xie M. A novel polysaccharide from the seeds of Plantago asiatica L. induces dendritic cells maturation through toll-like receptor 4. Int Immunopharmacol (2014) 18:236–43. doi: 10.1016/j.intimp.2013.11.024
55. Pang H, Wu T, Peng Z, Tan Q, Peng X, Zhan Z, et al. Baicalin induces apoptosis and autophagy in human osteosarcoma cells by increasing ROS to inhibit PI3K/Akt/mTOR, ERK1/2 and β-catenin signaling pathways. J Bone Oncol (2022) 33:100415. doi: 10.1016/j.jbo.2022.100415
56. Wang Y, Zhang X, Li J, Zhang Y, Guo Y, Chang Q, et al. Sini decoction ameliorates colorectal cancer and modulates the composition of gut microbiota in mice. Front Pharmacol (2021) 12:609992. doi: 10.3389/fphar.2021.609992
57. Wu X, Shou Q, Chen C, Cai H, Zhang J, Tang S, et al. An herbal formula attenuates collagen-induced arthritis via inhibition of JAK2-STAT3 signaling and regulation of Th17 cells in mice. Oncotarget (2017) 8:44242–54. doi: 10.18632/oncotarget.17797
58. Dudley AC, Griffioen AW. Pathological angiogenesis: mechanisms and therapeutic strategies. Angiogenesis (2023) 26(3):313–47. doi: 10.1007/s10456-023-09876-7
59. Zhai B, Zhang N, Han X, Li Q, Zhang M, Chen X, et al. Molecular targets of β-elemene, a herbal extract used in traditional Chinese medicine, and its potential role in cancer therapy: A review. Biomed Pharmacother. Biomedecine Pharmacother (2019) 114:108812. doi: 10.1016/j.biopha.2019.108812
60. Ai Y, Zhao Z, Wang H, Zhang X, Qin W, Guo Y, et al. Pull the plug: Anti-angiogenesis potential of natural products in gastrointestinal cancer therapy. Phytother. Res PTR. (2022) 36:3371–93. doi: 10.1002/ptr.7492
61. Ma C, Tang X, Tang Q, Wang S, Zhang J, Lu Y, et al. Curcumol repressed cell proliferation and angiogenesis via SP1/mir-125b-5p/VEGFA axis in non-small cell lung cancer. Front Pharmacol (2022) 13:1044115. doi: 10.3389/fphar.2022.1044115
62. Zhao Y, Pan H, Liu W, Liu E, Pang Y, Gao H, et al. Menthol: An underestimated anticancer agent. Front Pharmacol (2023) 14:1148790. doi: 10.3389/fphar.2023.1148790
63. Zhu G, Wang X, Yang Z, Cao H, Meng Z, Wang Y, et al. Effects of TRPM8 on the proliferation and angiogenesis of prostate cancer PC-3 cells in vivo. Oncol Lett (2011) 2:1213–7. doi: 10.3892/ol.2011.410
64. Walcher L, Budde C, Böhm A, Reinach PS, Dhandapani P, Ljubojevic N, et al. TRPM8 activation via 3-iodothyronamine blunts VEGF-induced transactivation of TRPV1 in human uveal melanoma cells. Front Pharmacol (2018) 9:1234. doi: 10.3389/fphar.2018.01234
65. Li L-C, Kan L-D. Traditional Chinese medicine for pulmonary fibrosis therapy: Progress and future prospects. J Ethnopharmacol (2017) 198:45–63. doi: 10.1016/j.jep.2016.12.042
66. Lihua J, Haodan K, Yuan XU. Efficacy of Buzhong Yiqi decoction on benign prostatic hyperplasia and its possible mechanism. J Tradit. Chin Med Chung Tsa Chih Ying Wen Pan (2023) 43:533–41. doi: 10.19852/j.cnki.jtcm.2023.03.003
67. Nourmohammadi S, Aung TN, Cui J, Pei JV, De Ieso ML, Harata-Lee Y, et al. Effect of compound kushen injection, a natural compound mixture, and its identified chemical components on migration and invasion of colon, brain, and breast cancer cell lines. Front Oncol (2019) 9:314. doi: 10.3389/fonc.2019.00314
68. Zhao H, Wu L, Yan G, Chen Y, Zhou M, Wu Y, et al. Inflammation and tumor progression: signaling pathways and targeted intervention. Signal Transduction Targeting Ther (2021) 6(1):263. doi: 10.1038/s41392-021-00658-5
69. Li H, Li Y. Network pharmacology analysis of molecular mechanism of curcuma longa L. Extracts regulating glioma immune inflammatory factors: implications for precise cancer treatment. Curr Top Med Chem (2022) 22:259–67. doi: 10.2174/1568026621666210910123749
70. Yeh Y-C, Doan LH, Huang Z-Y, Chu L-W, Shi T-H, Lee Y-R, et al. Honeysuckle (Lonicera japonica) and Huangqi (Astragalus membranaceus) Suppress SARS-CoV-2 Entry and COVID-19 Related Cytokine Storm in Vitro. Front Pharmacol (2022) 12:765553. doi: 10.3389/fphar.2021.765553
71. Gaikwad S, Agrawal MY, Kaushik I, Ramachandran S, Srivastava SK. Immune checkpoint proteins: Signaling mechanisms and molecular interactions in cancer immunotherapy. Semin Cancer Biol (2022) 86:137–50. doi: 10.1016/j.semcancer.2022.03.014
72. Yu Y-X, Wang S, Liu Z-N, Zhang X, Hu Z-X, Dong H-J, et al. Traditional Chinese medicine in the era of immune checkpoint inhibitor: theory, development, and future directions. Chin Med (2023) 18(1):59. doi: 10.1186/s13020-023-00751-7
73. Lv J, Jia Y, Li J, Kuai W, Li Y, Guo F, et al. Gegen Qinlian decoction enhances the effect of PD-1 blockade in colorectal cancer with microsatellite stability by remodelling the gut microbiota and the tumour microenvironment. Cell Death Dis (2019) 10:415. doi: 10.1038/s41419-019-1638-6
74. Liu Y, Liu X, Zhang N, Yin M, Dong J, Zeng Q, et al. Berberine diminishes cancer cell PD-L1 expression and facilitates antitumor immunity via inhibiting the deubiquitination activity of CSN5. Acta Pharm Sin B (2020) 10:2299–312. doi: 10.1016/j.apsb.2020.06.014
75. Su L, Zhang F, Liu M, Li H, Li Q, Zhu Y, et al. The Tian-Men-Dong decoction suppresses the tumour-infiltrating G-MDSCs via IL-1β-mediated signalling in lung cancer. J Ethnopharmacol (2023) 313:116491. doi: 10.1016/j.jep.2023.116491
76. Pan J, Yang H, Zhu L, Lou Y, Jin B. Qingfei Jiedu decoction inhibits PD-L1 expression in lung adenocarcinoma based on network pharmacology analysis, molecular docking and experimental verification. Front Pharmacol (2022) 13:897966. doi: 10.3389/fphar.2022.897966
77. Han S, Bi S, Guo T, Sun D, Zou Y, Wang L, et al. Nano co-delivery of Plumbagin and Dihydrotanshinone I reverses immunosuppressive TME of liver cancer. J Control. Release Off J Control. Release Soc (2022) 348:250–63. doi: 10.1016/j.jconrel.2022.05.057
78. Luo B, Que Z-J, Zhou Z-Y, Wang Q, Dong C-S, Jiang Y, et al. Feiji Recipe inhibits the growth of lung cancer by modulating T-cell immunity through indoleamine-2,3-dioxygenase pathway in an orthotopic implantation model. J Integr Med (2018) 16:283–9. doi: 10.1016/j.joim.2018.04.008
79. Hsu K-Y, Ho C-T, Pan M-H. The therapeutic potential of curcumin and its related substances in turmeric: From raw material selection to application strategies. J Food Drug Anal (2023) 31:194–211. doi: 10.38212/2224-6614.3454
80. Jang SY, Kim J, Hong E, Lee K, Na Y, Yeom CH, et al. Curcumin inhibits human cancer cell growth and migration through downregulation of SVCT2. Cell Biochem Funct (2023) 41(6):696–703. doi: 10.1002/cbf.3824
81. Hussain A, Kumar A, Uttam V, Sharma U, Sak K, Saini RV, et al. Application of curcumin nanoformulations to target folic acid receptor in cancer: Recent trends and advances. Environ Res (2023), 116476. doi: 10.1016/j.envres.2023.116476
82. Shan G, Minchao K, Jizhao W, Rui Z, Guangjian Z, Jin Z, et al. Resveratrol improves the cytotoxic effect of CD8 +T cells in the tumor microenvironment by regulating HMMR/Ferroptosis in lung squamous cell carcinoma. J Pharm Biomed Anal (2023) 229:115346. doi: 10.1016/j.jpba.2023.115346
83. Zhang X, Jiang A, Qi B, Ma Z, Xiong Y, Dou J, et al. Resveratrol Protects against Helicobacter pylori-Associated Gastritis by Combating Oxidative Stress. Int J Mol Sci (2015) 16:27757–69. doi: 10.3390/ijms161126061
84. Wu M, Song D, Li H, Ahmad N, Xu H, Yang X, et al. Resveratrol enhances temozolomide efficacy in glioblastoma cells through downregulated MGMT and negative regulators-related STAT3 inactivation. Int J Mol Sci (2023) 24(11):9453. doi: 10.3390/ijms24119453
85. Kumavath R, Paul S, Pavithran H, Paul MK, Ghosh P, Barh D, et al. Emergence of cardiac glycosides as potential drugs: current and future scope for cancer therapeutics. Biomolecules (2021) 11:1275. doi: 10.3390/biom11091275
86. Jia L, Yang M, Tian X, Zhao P, Mei X, Bai Y. [Digoxin alleviates pulmonary fibrosis by regulating phosphatidylinositol-3-kinase/Akt signaling through inhibiting the activation of fibroblast: an in vivo and in vitro experiment]. Zhonghua Wei Zhong Bing Ji Jiu Yi Xue. (2022) 34:1161–6. doi: 10.3760/cma.j.cn121430-20220628-00508
87. Ashbrook AW, Lentscher AJ, Zamora PF, Silva LA, May NA, Bauer JA, et al. Antagonism of the sodium-potassium ATPase impairs chikungunya virus infection. mBio (2016) 7:e00693–16. doi: 10.1128/mBio.00693-16
88. Papale M, Buccarelli M, Mollinari C, Russo MA, Pallini R, Ricci-Vitiani L, et al. Hypoxia, inflammation and necrosis as determinants of glioblastoma cancer stem cells progression. Int J Mol Sci (2020) 21:2660. doi: 10.3390/ijms21082660
89. Yu Z, Li Y, Li Y, Zhang J, Li M, Ji L, et al. Bufalin stimulates antitumor immune response by driving tumor-infiltrating macrophage toward M1 phenotype in hepatocellular carcinoma. J Immunother. Cancer (2022) 10:e004297. doi: 10.1136/jitc-2021-004297
90. Zhang D, Jia T, Chen X, Jiang H, Guo T, Dong J, et al. Bufalin reverses ABCB1-mediated resistance to docetaxel in breast cancer. Heliyon (2023) 9:e13840. doi: 10.1016/j.heliyon.2023.e13840
91. Qian Z, Tian X, Miao Y, Xu X, Cheng X, Wu M, et al. Bufalin inhibits the proliferation of lung cancer cells by suppressing Hippo-YAP pathway. Cell Signal (2023) 109:110746. doi: 10.1016/j.cellsig.2023.110746
92. Miao L, Liu Y, Ali NM, Dong Y, Zhang B, Cui X. Bufalin serves as a pharmaceutic that mitigates drug resistance. Drug Metab Rev (2023) 55(3):195–204. doi: 10.1080/03602532.2023.2206065
93. Shi S, Zhao S, Tian X, Liu F, Lu X, Zang H, et al. Molecular and metabolic mechanisms of bufalin against lung adenocarcinoma: New and comprehensive evidences from network pharmacology, metabolomics and molecular biology experiment. Comput Biol Med (2023) 157:106777. doi: 10.1016/j.compbiomed.2023.106777
94. Thakre A, Zore G, Kodgire S, Kazi R, Mulange S, Patil R, et al. Limonene inhibits Candida albicans growth by inducing apoptosis. Med Mycol (2018) 56:565–78. doi: 10.1093/mmy/myx074
95. Araújo-Filho HGD, Dos Santos JF, Carvalho MTB, Picot L, Fruitier-Arnaudin I, Groult H, et al. Anticancer activity of limonene: A systematic review of target signaling pathways. Phytother. Res (2021) 35:4957–70. doi: 10.1002/ptr.7125
96. Chebet JJ, Ehiri JE, McClelland DJ, Taren D, Hakim IA. Effect of d-limonene and its derivatives on breast cancer in human trials: a scoping review and narrative synthesis. BMC Cancer. (2021) 21:902. doi: 10.1186/s12885-021-08639-1
97. Leung JC, Cassimeris L. Reorganization of paclitaxel-stabilized microtubule arrays at mitotic entry: roles of depolymerizing kinesins and severing proteins. Cancer Biol Ther (2019) 20:1337–47. doi: 10.1080/15384047.2019.1638678
98. Su M, Zhao C, Li D, Cao J, Ju Z, Kim EL, et al. Viriditoxin stabilizes microtubule polymers in SK-OV-3 cells and exhibits antimitotic and antimetastatic potential. Mar Drugs (2020) 18:445. doi: 10.3390/md18090445
99. Wong YK, Xu C, Kalesh KA, He Y, Lin Q, Wong WSF, et al. Artemisinin as an anticancer drug: Recent advances in target profiling and mechanisms of action. Med Res Rev (2017) 37:1492–517. doi: 10.1002/med.21446
100. Tin AS, Sundar SN, Tran KQ, Park AH, Poindexter KM, Firestone GL. Antiproliferative effects of artemisinin on human breast cancer cells requires the downregulated expression of the E2F1 transcription factor and loss of E2F1-target cell cycle genes. Anticancer. Drugs (2012) 23:370–9. doi: 10.1097/CAD.0b013e32834f6ea8
101. Xu G, Huang Y-L, Li P, Guo H-M, Han X-P. Neuroprotective effects of artemisinin against isoflurane-induced cognitive impairments and neuronal cell death involve JNK/ERK1/2 signalling and improved hippocampal histone acetylation in neonatal rats. J Pharm Pharmacol (2017) 69:684–97. doi: 10.1111/jphp.12704
102. He J, Yu S, Zhu H, Wu J, Qin Z. Triptolide inhibits NF-kappaB activation and reduces injury of donor lung induced by ischemia/reperfusion, Acta Pharmacol. Sin (2007) 28:1919–23. doi: 10.1111/j.1745-7254.2007.00701.x
103. Jiang S, Wan F, Lian H, Lu Z, Li X, Cao D, et al. Friend or foe? The dual role of triptolide in the liver, kidney, and heart. Biomed Pharmacother. Biomedecine Pharmacother (2023) 161:114470. doi: 10.1016/j.biopha.2023.114470
104. Liu W, Yang Y, Wang J, Wu S, Chen Z. Triptolide-mediated downregulation of FLIPS in hepatoma cells occurs at the post-transcriptional level independently of proteasome-mediated pathways. Med Oncol Northwood Lond Engl (2022) 40:7. doi: 10.1007/s12032-022-01857-y
105. Ganguly S, Home T, Yacoub A, Kambhampati S, Shi H, Dandawate P, et al. Targeting HSF1 disrupts HSP90 chaperone function in chronic lymphocytic leukemia. Oncotarget (2015) 6:31767–79. doi: 10.18632/oncotarget.5167
106. El-Baba C, Baassiri A, Kiriako G, Dia B, Fadlallah S, Moodad S, et al. Terpenoids’ anti-cancer effects: focus on autophagy. Apoptosis Int J Program. Cell Death (2021) 26:491–511. doi: 10.1007/s10495-021-01684-y
107. Wang G, Guo H, Ren Y, Chen W, Wang Y, Li J, et al. Triptolide enhances carboplatin-induced apoptosis by inhibiting nucleotide excision repair (NER) activity in melanoma. Front Pharmacol (2023) 14:1157433. doi: 10.3389/fphar.2023.1157433
108. Cai J, Zhong M, Xu J, Cheng H, Xu S. Codelivery of triptolide and IFN-γ to boost antitumor immunity for triple-negative breast cancer. Int Immunopharmacol (2023) 120:110346. doi: 10.1016/j.intimp.2023.110346
109. Khan T, Date A, Chawda H, Patel K. Polysaccharides as potential anticancer agents-A review of their progress. Carbohydr. Polym (2019) 210:412–28. doi: 10.1016/j.carbpol.2019.01.064
110. Zhou M, Yue Y, Wang Y, Yan S. Polysaccharides from Chinese herbs as natural weapons against colorectal cancer. Biosci Rep (2023) 43:BSR20230041. doi: 10.1042/BSR20230041
111. Li M, Li H, Fang F, Deng X, Ma S. Astragaloside IV attenuates cognitive impairments induced by transient cerebral ischemia and reperfusion in mice via anti-inflammatory mechanisms. Neurosci Lett (2017) 639:114–9. doi: 10.1016/j.neulet.2016.12.046
112. Ji X, Su L, Zhang P, Yue Q, Zhao C, Sun X, et al. Lentinan improves intestinal inflammation and gut dysbiosis in antibiotics-induced mice. Sci Rep (2022) 12:19609. doi: 10.1038/s41598-022-23469-2
113. You J, Wu Q, Li Y, Li X, Lin Z, Huang J, et al. Lentinan induces apoptosis of mouse hepatocellular carcinoma cells through the EGR1/PTEN/AKT signaling axis. Oncol Rep (2023) 50:142. doi: 10.3892/or.2023.8579
114. Xu H, Qi Z, Zhao Q, Xue J, Zhu J, He Y, et al. Lentinan enhances the antitumor effects of Delta-like 1 via neutrophils. BMC Cancer. (2022) 22:918. doi: 10.1186/s12885-022-10011-w
115. Li M, Du X, Yuan Z, Cheng M, Dong P, Bai Y. Lentinan triggers oxidative stress-mediated anti-inflammatory responses in lung cancer cells. Mol Cell Biochem (2022) 477:469–77. doi: 10.1007/s11010-021-04293-0
116. Yao L-C, Wu L, Wang W, Zhai L-L, Ye L, Xiang F, et al. Panax notoginseng Saponins Promote Cell Death and Chemosensitivity in Pancreatic Cancer through the Apoptosis and Autophagy Pathways. Anticancer Agents Med Chem (2021) 21:1680–8. doi: 10.2174/1871520620999201110191459
117. Xia L, Liu X, Mao W, Guo Y, Huang J, Hu Y, et al. Panax notoginseng saponins normalises tumour blood vessels by inhibiting EphA2 gene expression to modulate the tumour microenvironment of breast cancer. Phytomedicine Int J Phytother. Phytopharm (2023) 114:154787. doi: 10.1016/j.phymed.2023.154787
118. Li S, Pi G, Zeng Y, Ruan C, He X, Xiong X, et al. Notoginsenoside R1 induces oxidative stress and modulates LPS induced immune microenvironment of nasopharyngeal carcinoma. Int Immunopharmacol (2022) 113:109323. doi: 10.1016/j.intimp.2022.109323
119. Lin M-H, Lee Y-H, Cheng H-L, Chen H-Y, Jhuang F-H, Chueh PJ. Capsaicin inhibits multiple bladder cancer cell phenotypes by inhibiting tumor-associated NADH oxidase (tNOX) and sirtuin1 (SIRT1). Mol Basel Switz (2016) 21:849. doi: 10.3390/molecules21070849
120. Thoennissen NH, O’Kelly J, Lu D, Iwanski GB, La DT, Abbassi S, et al. Capsaicin causes cell-cycle arrest and apoptosis in ER-positive and -negative breast cancer cells by modulating the EGFR/HER-2 pathway. Oncogene (2010) 29:285–96. doi: 10.1038/onc.2009.335
121. Jin J, Lin G, Huang H, Xu D, Yu H, Ma X, et al. Capsaicin mediates cell cycle arrest and apoptosis in human colon cancer cells via stabilizing and activating p53. Int J Biol Sci (2014) 10:285–95. doi: 10.7150/ijbs.7730
122. Chen Z-Y, Huang H-H, Li Q-C, Zhan F-B, Wang L-B, Yang C-H, et al. Capsaicin reduces cancer stemness and inhibits metastasis by downregulating SOX2 and EZH2 in osteosarcoma. Am J Chin Med (2023) 51:1–26. doi: 10.1142/S0192415X23500489
123. Wang J, Tian W, Wang S, Wei W, Wu D, Wang H, et al. Anti-inflammatory and retinal protective effects of capsaicin on ischaemia-induced injuries through the release of endogenous somatostatin. Clin Exp Pharmacol Physiol (2017) 44:803–14. doi: 10.1111/1440-1681.12769
124. Que T, Ren B, Fan Y, Liu T, Hou T, Dan W, et al. Capsaicin inhibits the migration, invasion and EMT of renal cancer cells by inducing AMPK/mTOR-mediated autophagy. Chem Biol Interact (2022) 366:110043. doi: 10.1016/j.cbi.2022.110043
125. Yahfoufi N, Alsadi N, Jambi M, Matar C. The immunomodulatory and anti-inflammatory role of polyphenols. Nutrients (2018) 10:1618. doi: 10.3390/nu10111618
126. Rana A, Samtiya M, Dhewa T, Mishra V, Aluko RE. Health benefits of polyphenols: A concise review. J Food Biochem (2022) 46(10):e14264. doi: 10.1111/jfbc.14264
127. Maleki Dana P, Sadoughi F, Asemi Z, Yousefi B. The role of polyphenols in overcoming cancer drug resistance: a comprehensive review. Cell Mol Biol Lett (2022) 27:1. doi: 10.1186/s11658-021-00301-9
128. Tong Q, Wu Z. Curcumin inhibits colon cancer Malignant progression and promotes T cell killing by regulating miR-206 expression. Clin Anat. N Y. N (2023). doi: 10.1002/ca.24057
129. Gao L, Zhao M, Mao Y, Zhang L, Wang X, Li S, et al. Localized microsphere/hydrogel for tumor immunotherapy of cardiac glycoside with minimal toxicity. ACS Appl Mater Interfaces (2023) 15:578–90. doi: 10.1021/acsami.2c18693
130. Bejček J, Jurášek M, Spiwok V, Rimpelová S. Quo vadis cardiac glycoside research? Toxins (2021) 13:344. doi: 10.3390/toxins13050344
131. Zhao W, Li G, Zhang Q, Chen M, He L, Wu Z, et al. Cardiac glycoside neriifolin exerts anti-cancer activity in prostate cancer cells by attenuating DNA damage repair through endoplasmic reticulum stress. Biochem Pharmacol (2023) 209:115453. doi: 10.1016/j.bcp.2023.115453
132. Soumoy L, Ghanem GE, Saussez S, Journe F. Bufalin for an innovative therapeutic approach against cancer. Pharmacol Res (2022) 184:106442. doi: 10.1016/j.phrs.2022.106442
133. Kamran S, Sinniah A, Abdulghani MAM, Alshawsh MA. Therapeutic potential of certain terpenoids as anticancer agents: A scoping review. Cancers (2022) 14:1100. doi: 10.3390/cancers14051100
134. Zhao D, Chen X, Wang L, Zhang J, Lv R, Tan L, et al. Improvement influenza vaccine immune responses with traditional Chinese medicine and its active ingredients. Front Microbiol (2023) 14:1111886. doi: 10.3389/fmicb.2023.1111886
135. Man S, Gao W, Zhang Y, Huang L, Liu C. Chemical study and medical application of saponins as anti-cancer agents. Fitoterapia (2010) 81:703–14. doi: 10.1016/j.fitote.2010.06.004
136. Chen Z-J, Cheng J, Huang Y-P, Han S-L, Liu N-X, Zhu G-B, et al. [Effect of adjuvant chemotherapy of ginsenoside Rg3 combined with mitomycin C and tegafur in advanced gastric cancer]. Zhonghua Wei Chang Wai Ke Za Zhi Chin J Gastrointest. Surg (2007) 10:64–6.
137. Zhou P, Shi W, He X-Y, Du Q-Y, Wang F, Guo J. Saikosaponin D: review on the antitumour effects, toxicity and pharmacokinetics. Pharm Biol (2021) 59:1478–87. doi: 10.1080/13880209.2021.1992448
138. Kamaruddin MF, Hossain MZ, Mohamed Alabsi A, Mohd Bakri M. The antiproliferative and apoptotic effects of capsaicin on an oral squamous cancer cell line of Asian origin, ORL-48. Med Kaunas Lith (2019) 55:322. doi: 10.3390/medicina55070322
139. Baxevanis CN, Perez SA, Papamichail M. Cancer immunotherapy. Crit Rev Clin Lab Sci (2009) 46:167–89. doi: 10.1080/10408360902937809
140. O'Donnell JS, Teng MWL, Smyth MJ. Cancer immunoediting and resistance to T cell-based immunotherapy. Nat Rev Clin Oncol (2019) 16(3):151–67. doi: 10.1038/s41571-018-0142-8
141. Magré L, Verstegen MMA, Buschow S, van der Laan LJW, Peppelenbosch M, Desai J. Emerging organoid-immune co-culture models for cancer research: from oncoimmunology to personalized immunotherapies. J Immunother. Cancer (2023) 11:e006290. doi: 10.1136/jitc-2022-006290
142. Saúde-Conde R, Nguyen D, Hendlisz A. Immunotherapies in non-metastatic gastrointestinal cancers. Curr Opin Oncol (2023) 35(4):334–46. doi: 10.1097/CCO.0000000000000956
143. Budhu A, Pehrsson EC, He A, Goyal L, Kelley RK, Dang H, et al. Tumor biology and immune infiltration define primary liver cancer subsets linked to overall survival after immunotherapy. Cell Rep Med (2023) 4(6):101052. doi: 10.1016/j.xcrm.2023.101052
144. Hu H, Wang K, Jia R, Zeng Z-X, Zhu M, Deng Y-L, et al. Current status in rechallenge of immunotherapy. Int J Biol Sci (2023) 19:2428–42. doi: 10.7150/ijbs.82776
145. Lin M, Sun X, Lv L. New insights and options into the mechanisms and effects of combined targeted therapy and immunotherapy in prostate cancer. Mol Ther Oncolytics (2023) 29:91–106. doi: 10.1016/j.omto.2023.04.007
146. Liu S, Zhu J-J, Li J-C. The interpretation of human body in traditional Chinese medicine and its influence on the characteristics of TCM theory. Anat. Rec. Hoboken NJ (2021) 2007. 304:2559–65. doi: 10.1002/ar.24643
147. Zhang A, Sun H, Wang P, Han Y, Wang X. Future perspectives of personalized medicine in traditional Chinese medicine: a systems biology approach. Complement. Ther Med (2012) 20:93–9. doi: 10.1016/j.ctim.2011.10.007
148. Galon J, Bruni D. Tumor immunology and tumor evolution: intertwined histories. Immunity (2020) 52:55–81. doi: 10.1016/j.immuni.2019.12.018
149. Nicolaides NC, O’Shannessy DJ, Albone E, Grasso L. Co-development of diagnostic vectors to support targeted therapies and theranostics: essential tools in personalized cancer therapy. Front Oncol (2014) 4:141. doi: 10.3389/fonc.2014.00141
150. Tilak T.V.S.V.G.K., Patel A, Kapoor A. Molecular basis and clinical application of targeted therapy in oncology. Med J Armed Forces India. (2023) 79:128–35. doi: 10.1016/j.mjafi.2023.02.001
Keywords: traditional Chinese medicine, natural products, tumor immunity, cancer immunotherapy, immunomodulators, cytokines
Citation: Miao K, Liu W, Xu J, Qian Z and Zhang Q (2023) Harnessing the power of traditional Chinese medicine monomers and compound prescriptions to boost cancer immunotherapy. Front. Immunol. 14:1277243. doi: 10.3389/fimmu.2023.1277243
Received: 14 August 2023; Accepted: 30 October 2023;
Published: 15 November 2023.
Edited by:
Qun Xue, Affiliated Hospital of Nantong University, ChinaReviewed by:
Chengming Li, Shandong University, ChinaLing Bi, Shanghai University of Traditional Chinese Medicine, China
Copyright © 2023 Miao, Liu, Xu, Qian and Zhang. This is an open-access article distributed under the terms of the Creative Commons Attribution License (CC BY). The use, distribution or reproduction in other forums is permitted, provided the original author(s) and the copyright owner(s) are credited and that the original publication in this journal is cited, in accordance with accepted academic practice. No use, distribution or reproduction is permitted which does not comply with these terms.
*Correspondence: Zhengtao Qian, NDY3NTI0MTE4QHFxLmNvbQ==; Qinglin Zhang, emhhbmc1MTcwNjhAMTI2LmNvbQ==
†These authors have contributed equally to this work