- 1Department of Otorhinolaryngology, Head and Neck Surgery, Yantai Yuhuangding Hospital, Qingdao University, Yantai, China
- 2Shandong Provincial Clinical Research Center for Otorhinolaryngologic Diseases, Yantai Yuhuangding Hospital, Yantai, China
- 3Yantai Key Laboratory of Otorhinolaryngologic Diseases, Yantai Yuhuangding Hospital, Yantai, China
- 4Shandong Provincial Innovation and Practice Base for Postdoctors, Yantai Yuhuangding Hospital, Yantai, China
- 5Department of Neurology, Yantai Yuhuangding Hospital, Qingdao University, Yantai, China
In addition to typical respiratory symptoms, patients with asthma are frequently accompanied by cognitive decline, mood disorders (anxiety and depression), sleep disorders, olfactory disorders, and other brain response manifestations, all of which worsen asthma symptoms, form a vicious cycle, and exacerbate the burden on families and society. Therefore, studying the mechanism of neurological symptoms in patients with asthma is necessary to identify the appropriate preventative and therapeutic measures. In order to provide a comprehensive reference for related research, we compiled the pertinent literature, systematically summarized the latest research progress of asthma and its brain response, and attempted to reveal the possible “lung–brain” crosstalk mechanism and treatment methods at the onset of asthma, which will promote more related research to provide asthmatic patients with neurological symptoms new hope.
1 Introduction
Asthma, also known as bronchial asthma, is a chronic inflammatory disease of the airways with a complex etiology, and its pathological process involves multiple aspects, such as the genetic regulation of genes and environmental interference (1, 2). According to a 2022 study, over 334 million individuals are suffering from asthma worldwide, with an incidence of approximately 3.33%, which had risen rapidly because of the synergistic influence of air pollution, climate change, and other factors over the last few decades (3, 4). Asthma primarily affects children and adolescents, and has become a severe global public health issue, imposing a significant economic burden on families and society (5).
Asthma is characterized by non-specific respiratory symptoms such as wheezing, shortness of breath, chest tightness, and episodic cough as the main clinical manifestations, which frequently occur at night and early in the morning, and its main pathogenesis is chronic immune-inflammatory response, airway hyperreactivity, reversible airflow limitation, and airway remodeling (6, 7). Patients with asthma are often accompanied by neurological symptoms such as cognitive dysfunction, depression, anxiety, dysosmia, and sleep disorders, implying a brain response to asthma, which affects their quality of life, increases their economic burden, reduces the treatment sensitivity of asthma, increases the risk of asthma exacerbations, and forms a vicious cycle (8–10). Based on our previous summary of brain response in allergic rhinitis (AR) (11), a “lung–brain” crosstalk in asthma can be observed on the basis of neuro-immune mechanisms, that is, inflammatory factors generated during chronic inflammation in asthma can be transmitted upwards to the central nervous system, thereby stimulating associated brain regions to elicit one or more brain responses, transmitting response commands to peripheral nerves, activating such commands to release mediators such as neuropeptides and neurogenic trophic factors, worsening asthma symptoms through actions such as tracheal smooth muscle contraction, promoting the re-entry of immune inflammatory factors produced in the periphery to the brain, and exacerbating neurological symptoms caused by brain response (12, 13). We have summarized the research on the correlation between asthma and changes in brain activity related to its neurological phenotypes in the past 5 years (Table 1) (14–22).
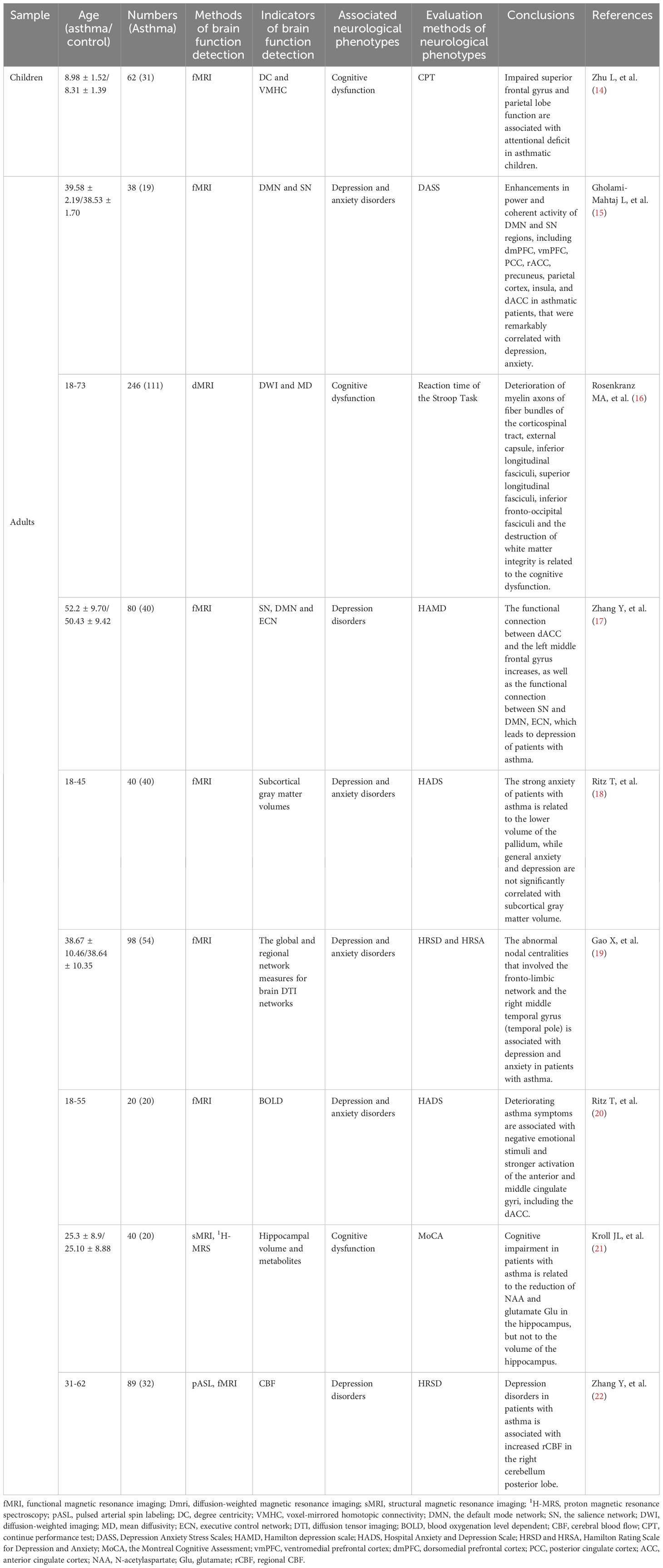
Table 1 Summary of research on the correlation between asthma and changes in brain activity related to its relatedneuropsycological symptoms in the past 5 years.
Despite the abundance of research on asthma and neuroinflammation, the specific brain response mechanism implicated in asthma has not been systematically elucidated. Thus, we aim to sort out the current status and progression of neurological symptoms induced by asthma by summarizing relevant literature to provide a reference for mechanism exploration, prevention, and treatment intervention of asthma-related brain responses. Simultaneously, our summary also complements the “lung–brain” axis theory to a certain extent.
2 Asthma and neuro-immune-mediated “lung–brain” crosstalk
Patients with asthma exhibit altered functional activity in relevant brain regions. Using resting-state functional magnetic resonance imaging (fMRI), Huang et al. scanned the brain regions of patients with asthma and found differences in regional homogeneity values in the cerebellar, frontal, temporal, and occipital lobes (23). Rosenkranz et al. used fMRI to simultaneously measure pulmonary function and inflammatory parameters of induced sputum in patients with asthma and found that changes in insula activity best characterized asthma pathogenesis; such changes were significantly associated with decreases in pulmonary function and increases in eosinophil content of induced sputum (24). Leupoldt et al. used fMRI to investigate changes in brain regions during induced asthma in asthmatic patients and healthy subjects and found that cortical activity in the insula decreased, whereas activity in the periaqueductal gray (PAG) increased when asthma was induced and caused dyspnea. The authors hypothesized that the activity of the insula, a key brain region involved in emotion regulation, may be regulated by PAG, which causes negative mood disorders such as anxiety and depression (25). Dynamic functional connectivity is more sensitive than resting brain function evaluation indicators in MRI, allowing it to disclose the internal heterogeneity and dynamic changes of brain dysfunction (26), of which dynamic voxel-mirrored homotopic connection (dVMHC) plays a role in revealing asthma-induced brain response because of its sensitive and specific quantitative characteristics of neural activity intensity, particularly in indicating emotion-related disorders (27). A clinical study of patients with asthma revealed that dVMHC levels in the lingual gyrus and calcarine sulcus were significantly elevated, and dVMHC values in the medial superior frontal gyrus, cingulate gyrus, and supplementary motor area were considerably lowered; these brain regions have a vital function in mood regulation, indicating that the ability of patients with asthma to regulate their emotions is severely impaired (28). Thus, a “lung–brain” crosstalk based on the neuro-immune mechanisms may be observed when asthma occurs.
2.1 Effects of airway inflammation on the brain in patients with asthma
Prior research on neuro-immune crosstalk in asthma has focused on interactions between inflammatory factors and the peripheral nervous system. Given the increasing number of patients with asthma who develop a variety of neurological symptoms in clinical practice, pertinent research has been conducted, and results show that numerous inflammatory factors produced during chronic asthmatic inflammation can reach the central nervous system via humoral and neural pathways.
Asthma-induced chronic inflammation can stimulate the synthesis and release of prostaglandin mediators and nitric oxide (NO) from cerebrovascular endothelial cells and pericytes that form the blood–brain barrier (BBB), causing endothelial cell injury, tight junction destruction, and structural integrity disruption of the BBB. It can also increase BBB permeability in response to the binding of inflammatory factors to endothelial cell surface receptors (29). In the humoral pathway, after being released into the blood, cellular inflammatory factors can either penetrate the BBB or infiltrate the brain region directly via the periventricular organs without a BBB, thereby activating and damaging neurons, including microglia and astrocytes. Michelle and Parajuli et al. discovered that after eosinophil activation, eotaxin-1 (CCL11) swiftly crossed the BBB and bound to CCL11 receptors on the surface of microglia (30), which increased the amount of nicotinamide adenine dinucleotide phosphate-oxidase 1 (NOX1) in microglia, promoted the development of oxidative stress in microglia, increased the levels of intracellular reactive oxygen species (ROS), and enhanced glutamate-induced neurotoxicity, thereby resulting in neuronal damage and death (31). The pathogenesis of asthma is significantly influenced by oxidative stress. Florentino et al. demonstrated that oxidative stress-mediated inflammation promoted the activation of relevant microglia and the production of inflammatory neuro-mediators after compromising the integrity of the BBB, leading to a series of brain responses (32, 33). In addition, Antunes et al. found that airway inflammation could increase acetylcholinesterase (AchE) activity and decrease Na+, K+-ATPase activity in the brain of asthmatic mice, both of which led to a reduction in acetylcholine (Ach) content and formed a pro-inflammatory humoral immune microenvironment (34, 35).
With regard to neural pathway transmission, after the inflammatory response during asthma attacks activates primary afferent nerves, signals are further emitted to stimulate afferent nerve fibers of the pulmonary vagus nerve, which produce nerve impulses that reach the nucleus tractus solitarius (NTS) and project to multiple brain regions, thereby inducing diverse neurological phenotypes by affecting the functional activity of these brain regions (36, 37). The research of Chen et al. on asthmatic monkeys indicated that protracted exposure to allergens elevated excitability in relevant brain regions (e.g., NTS) that process airway receptor afferent signals, which may be associated with an increase in the plasticity of postsynaptic neurons caused by variations in the amplitude and frequency of nerve impulses transmitted to the NTS by lung receptors (28). Simultaneously, chronic inflammation in asthma increases airway resistance; enhances respiratory reflexes, chemoreceptors, and pulmonary stretch receptors in airways associated with shortness of breath and dyspnea-transmitted nerve impulses to the insula and anterior cingulate cortex (ACC) via the spinothalamic cortex; and completes the regulation of respiration via functional connections formed by the medial prefrontal cortex (mPFC) and brainstem (12, 38). When examining respiratory-related evoked potentials in children with life-threatening asthma (LTA), Davenport et al. found that certain children with LTA had a loss of airway mechanoreceptor-cerebral cortex perception-related circuits, which made their asthma symptoms more difficult to control compared with those of other children (39). The neural pathways implicated in the “lung–brain” axis associated with asthma are intricate and delicate, and they may involve a single brain region, multiple brain regions, or neural circuits, which coordinate and collaborate with humoral pathways to contribute jointly to the effects of peripheral airway inflammation on the brain.
2.2 Brain regulation of airways in asthma
Chronic inflammation resulting from the pathogenesis of asthma is transmitted to the central nervous system via a number of pathways, resulting in the activation of related brain regions and subsequent alterations in brain function and symptoms, during which brain signals are fed back to the periphery in various ways. Asthma occurs when the bronchial smooth muscle contracts and generates various inflammatory factors, triggering an inflammatory response in related brain regions to activate its function, including the amygdala, prefrontal cortex (PFC), and insula. Amygdala stimulation further activates the hypothalamic–pituitary–adrenal (HPA) axis, and glucocorticoids are released into the circulation to bind to glucocorticoid receptors (GR) on the surface of the airway epithelium and immune cells as well as to achieve the central regulation of peripheral inflammation, particularly in the expression of cellular inflammatory factors (such as IL-4, IL-5, and IL-13) involved in the development of asthma (12, 40). The activation of the HPA axis can also lead to the release of non-glucocorticoids (e.g., epinephrine, norepinephrine, and acetylcholine), which, along with glucocorticoids, amplify immune inflammation generated by asthma (41). Bailey et al. identified that the expression level of GR in the lungs of stressed asthmatic mice was drastically downregulated following the activation of the HPA axis, which may be connected to the impaired nuclear translocation of GR in macrophages and other cells, thereby reducing the responsiveness of the body to glucocorticoids, suppressing its anti-inflammatory ability, and deteriorating the airway inflammatory response in patients with asthma (42, 43). Similarly, Pongratz et al. suggested that the hypothalamus triggered the sympathetic nervous system to release norepinephrine, which acts directly on B-cell surface-associated receptors to promote the synthesis and release of immunoglobulin E (IgE), a key factor in asthma (44, 45). Therefore, the distinctive endocrine activation route of the HPA axis is crucial to brain’s modulation of peripheral inflammation.
Furthermore, numerous studies have confirmed that asthma is triggered by psychological stress, negative emotions, and other states (46). After induction, patients experience the activation of brain regions, including the ACC, insula, and limbic system, releasing neurotransmitter molecules represented by substance P(SP), histamine, and neuropeptide Y (NPY) that are transmitted to the airways through specific pathways; interacting with peripheral nerves and immune cells to exacerbate asthma symptoms; and deteriorating asthma symptoms to aggravate the adverse emotions of patients with asthma (24, 47, 48). Miyasaka et al. injected histamine receptor (H1R and H2R) antagonists into the brain ventricles of stressed asthmatic mice and discovered a significant reduction in peripheral airway inflammation, and eosinophil and lymphocyte infiltrations were considerably lowered, which indicated that allergens under stress may increase the severity of the inflammatory response to asthma by stimulating the release of histamine to activate histamine receptors (H1R and H2R) in the brain region (49). Consequently, the regulation of the expression of these neurotransmitter molecules can be used as an effective means to manage asthma symptoms.
3 Asthma-associated brain response phenotypes and mechanisms
3.1 Asthma and cognitive dysfunction
Clinical data indicate that approximately 45% of patients with asthma have varying degrees of cognitive impairment, which is closely related to the duration of asthma, frequency of attacks, and degree of pulmonary function reduction at the onset of asthma, and the risk of cognitive decline in patients with asthma increases by roughly 78% in comparison with the general population (8, 50, 51). Existing research has demonstrated that the precise mechanism by which asthma causes cognitive dysfunction is intricate, which is worthy of further study.
As mentioned previously, asthma can damage the BBB, allowing lung inflammatory factors to easily enter the hippocampus, PFC, and other regions of the brain associated with cognitive function, resulting in cognitive impairment. In addition, the onset of oxidative stress in asthma regulates the Th1/Th2-type immune inflammatory response, activates microglia, and promotes the release of inflammatory factors in cognitively involved brain regions such as the hippocampus by activating the NF-kB signal pathway, which may diminish N-acetylaspartate (NAA) levels in the hippocampus of patients with asthma and ultimately lead to cognitive dysfunction, including learning and memory (21, 52). Nair et al. noticed a high concentration of neurogranin (a protein closely related to synaptic plasticity and consolidation of memory and learning ability) and an elevated level of tau phosphorylation in the cerebrospinal fluid of patients with asthma, indicating that such patients have a pathological basis for cognitive impairment (53).
Given the contraction of bronchial smooth muscle and obstruction of airways, asthma attacks are accompanied by varying degrees of hypoxia symptoms, and the degradation of synaptic structures caused by hypoxia is closely associated with cognitive impairment (54, 55). Ren et al. revealed that the expression level of hypoxia-inducible factor-1α (HIF-1α) and HIF-2α was upregulated in the brain tissue of mice induced with asthma by house dust mites (HDM) along with the decreased synaptic plasticity in the hippocampus, and the learning and memory abilities of asthmatic mice, as measured by the Barnes maze test, were impaired compared with those of the control group (56, 57). In a mouse model of asthma, Guo et al. reported that the synaptic structure was destroyed in the hippocampus, and the maintenance of long-term potential (LTP), which is closely affiliated with learning, was disrupted, but this phenomenon did not affect the occurrence of LTP. In addition, the upregulation of HIF-1α expression and downregulation of c-FOS protein expression were detected in the hippocampus, and c-FOS is known to be a key factor in memory formation, that is, a strong correlation can be observed between hypoxia and cognitive impairment in patients with asthma (58). Moreover, cortisol hormones are used as conventional treatments to control asthma, and chronic oral cortisol hormones have been shown to promote volume reduction in the hippocampus and amygdala of patients with asthma (59). The aforementioned mechanism may be closely linked to cognitive decline in patients with asthma. However, recurrent asthma attacks; poor control often accompanied by sleep disorders, anxiety, depression, and other adverse emotional disorders; and sensitive responses to psychological stress and social stress events will damage patient’s learning and memory ability to varying degrees, which merits our focus.
Interestingly, in considering whether cognitive impairment has a reverse promoting effect on asthma pathogenesis, Wu et al. discovered evidence contradicting the hypothesis that inducing asthma in genetically inherited mice with Alzheimer’s disease (AD) did not exacerbate the severity of asthma, but rather reduced its airway hyperreactivity and airway obstruction, correspondingly increased the number of regulatory immune cells, and decreased the severity of inflammatory response to asthma (60). Contrary to the pathological progression of asthma, the existence of these protective mechanisms merits further investigation.
3.2 Asthma and anxiety disorders
Anxiety disorder is a mental illness characterized by excessive anxiety, worry, or dread. Asthma and anxiety disorders share many common predisposing factors, with an anxiety disorder incidence of approximately 22.7% and a risk nearly three times that of healthy individuals mentioned in a meta-analysis of comorbid anxiety in juvenile asthmatics (61). The co-occurrence of asthma and anxiety disorders diminishes the quality of life of patients; interferes with their ability to study, work, and engage in daily activities; makes asthma control more difficult; and increases the burden of medical care (62, 63).
Existing studies have indicated that the amygdala is a pivotal region of the brain encoding and processing anxiety-like behavior, and its circuit composed of mPFC plays a great part in the body’s anxiety processing network; moreover, the neural activity in this circuit can be directly used to represent the neuro-coding information of anxiety-like behavior (64, 65). Dehdar et al. characterized the immune-inflammatory status of the mPFC and amygdala in an ovalbumin (OVA)-induced asthma rat model by immunofluorescence staining of microglia and astrocytes and found that the activation and proliferation of these two cells were significantly enhanced probably because the immune-inflammatory factors produced during asthma inflammation strengthened the functional activity of local astrocytes via peripheral nerves or through the BBB to reach the paraventricular nucleus of the hypothalamus, stimulated their synthesis and release of gamma-aminobutyric acid, and induced the production of anxiety-like behavior, whereas the activation of microglia may heighten the inflammatory response in the hippocampus and further aggravate asthma-induced anxiety disorders (66–68). Further examination of the electrical activity of the brain in asthmatic rats revealed abnormal changes in the coupling potential between the mPFC and amygdala, such as decreased local delta/theta-gamma phase–amplitude coupling (PAC) in the mPFC, increased local delta-gamma PAC in the amygdala, diminished coupling between the mPFC delta/theta phase and amygdala gamma2 amplitude, enhanced coupling between the amygdala delta/theta phase and mPFC gamma2 amplitude, and improved neural activity in the mPFC and amygdala at low-frequency bands of delta and theta, indicating that chronic inflammation in asthma may achieve the neuromodulation of anxiety by strengthening the functional connectivity of the mPFC–amygdala circuit (69, 70). Dehdar et al. also found that inhaled corticosteroids, such as fluticasone, can improve the functional connectivity of the mPFC–amygdala pathway and attenuate the degree of neuroinflammation in the early stage of asthma pathogenesis, thereby relieving anxiety, which provides a foundation for the subsequent prevention and treatment of negative emotions in patients with asthma (70). More detailed studies have illustrated that ACC, as a subregion of the mPFC, also constitutes circuit connections with the basolateral amygdala (BLA) in a specific manner and plays an essential role in the neuromodulation of anxiety (71). Asthma-induced Th2 inflammatory factors allow type 2 cytokines to reach the ACC, BLA, and other brain regions along specific pathways, and the activation of microglia and astrocytes enhances the frequency of neural oscillations in ACC and BLA brain regions; moreover, the PAC of ACC-BLA circuits is strengthened, leading to heightened functional connectivity of ACC-BLA and inducing the expression of anxiety (72). In addition, anomalous coupling between the amygdala and the respiratory control network has a substantial effect on the development of anxiety in patients with asthma (73, 74). Hence, asthma regulates the development of anxious behavior in patients via numerous abnormal neural network activities.
Additionally, the expression level of type 2 inflammatory factors and corticotropin-releasing hormone was upregulated in the PFC, whereas c-FOS protein was deposited in the hypothalamus and amygdala of OVA-sensitized animal models, in which the activation of the HPA axis by corticotropin-releasing hormone could exacerbate asthma symptoms while modulating negative emotions (68, 75). Bejeshk et al. identified an oxidative/antioxidant imbalance in the hippocampus of asthmatic rats with anxiety, as well as elevated levels of inflammatory factors, and hypothesized that oxidative stress may have significant effects on asthma-related anxiety disorders, whereas the administration of antioxidant substances (e.g., myristicol) can ameliorate anxiety-like behavior by relieving inflammatory responses and oxidative stress in brain regions such as the hippocampus (76).
3.3 Asthma and depression
Epidemiological surveys have indicated that patients with asthmatic are roughly two times as likely as the general population to suffer from depression and that improvement in depressive symptoms contributes to the control of asthma progression (77). Th2-type immune inflammation in the pathogenesis of asthma can serve as a “link” between asthma and depressive disorders.
Accumulating research has verified that the NOD-like receptor family pyrin domain-containing 3 (NLRP3) inflammasome in lung-mediated pathological processes can promote a substantial increase in the serum concentration of pro-inflammatory cytokines (including IL-1β, IL-6, and TNF-α), corresponding activation of the HPA axis, and increased glucocorticoid release in patients with asthma. However, persistently high levels of glucocorticoids can lead to neuronal atrophy, neurogenesis inhibition, and decreased synaptic plasticity in the hippocampus and mPFC, whereas excessive IL-1β further reduces the production of brain-derived neurotrophic factor (BDNF) and neurogenesis in the hippocampus, both of which induce depressive mood in patients (78–80). Notably, Iwata et al. hypothesized that inflammatory signal transduction with the NLRP3 inflammasome as the “link” is biphasic in the development of depressive disorders in patients with asthma, that is, depressive symptoms among patients with asthma can be correspondingly activated by NLRP3 inflammasomes distributed in brain regions such as the hippocampus after pessimistic aggravation of major psychological stress events; the release of inflammatory factors such as IL-1β can transmit such inflammasomes to peripheral organs such as the lung through specific pathways (neural and humoral pathways) to strengthen the immune-inflammatory response of asthma, worsen the relevant symptoms of asthma, and serve as inflammatory factors in brain regions to further strengthen the susceptibility to depression along the abovementioned neural networks (81). However, direct experimental evidence is insufficient to confirm this biphasic regulatory relationship between asthma immune inflammation and neural networks in brain regions. If this hypothesis is validated, then it will have great guiding significance for the prevention and treatment of depressive disorders among patients with asthma.
In addition to inflammatory factors, Kanaya et al. found elevated levels of vascular endothelial growth factor (VEGF) in the blood of asthmatic mice and high concentrations of c-FOS protein, and glial fibrillary acidic protein (GFAP) deposition was detected in their brain regions, which is known to increase the permeability of the BBB. In addition, GFAP is a typical astrocyte injury marker, whereas c-FOS protein expression elevates when mast cells are activated in the brain, thereby indicating that inflammatory factors may infiltrate brain regions through the destruction of the BBB to activate neurons and mast cells in the brain, impair astrocyte function, disrupt mood regulation, and produce depressive disorders (82, 83). Depression and anxiety disorders, as common internalization disorders, involve many overlapping regulatory mechanisms in the pathogenesis of asthma, such as the activation of brain regions by oxidative stress, damage to brain structure by inflammatory factors, and feedback regulation of the HPA axis. Regulating these prevalent pathological processes will be more conducive to our prevention and treatment of asthma-related negative emotions (84).
3.4 Asthma and sleep disorders
Numerous studies suggest that patients with asthma frequently experience varying degrees of sleep disorders, which can manifest as insomnia, nocturnal awakenings, and early morning awakenings; indirectly contribute to daytime sleepiness, difficulty concentrating, diminished learning, and memory abilities; and significantly affect patients’ ability to work (85). What’s more, asthma is an essential risk factor for obstructive sleep apnea (OSA), with an incidence rate of up to 50% in patients with asthma, and the risk of OSA is proportional to the severity of asthma symptoms, the frequency of asthma attacks, and poor asthma control (86–88). Considering that asthma and sleep disorders can be affected by a variety of factors, such as obesity, smoking, rhinitis, and gastroesophageal reflux disease (GORD), the mechanisms involved are complex, and the role of “lung–brain crosstalk” remains unclear (89).
Bonnet et al. discovered significant increases in nocturnal airway hyperresponsiveness and airway resistance in patients with asthma, who tend to be agitated at night and experience more severe symptoms (90). Sensitizer exposure also caused a strong immune inflammatory response to worsen asthma symptoms, and Gervais et al. detected forced expiratory volumes in one second (FEV1) in patients with asthma after inhalation of HDM at different time points and found that FEV1 decreased evidently; moreover, ventilatory dysfunction was most severe after inhalation at 11 pm, which had a small effect at 8 am (91). The onset of asthma has a distinct circadian rhythm, which is closely linked to the development of sleep disorders in patients with asthma; however, the precise molecular mechanism remains unknown (92).
Asthma-related sleep disorders are inextricably linked to the expression of circadian clock-related molecules that modulate the circadian rhythm and activation of pathways (93). Ehlers et al. compared the broncholavage fluid from patients with severe asthma with controls and found that circadian clock-related genes (including BMAL1, PER2, and REV-ERBa) were all decreased and rhythm regulation may be disturbed (94). Zona et al. also discovered a dramatic increase in the number of eosinophils in the blood, the upregulation of the expression level of pro-inflammatory cytokines in the lungs, and a sharp increase in the degree of pulmonary inflammation in OVA-induced asthma in a BMAL1-knockout mouse model, which suggests that the regulation of circadian clock genes on asthma and rhythm may be biphasic (95). More in depth, rhythmic regulatory centers located in the suprachiasmatic nucleus (SCN) of the hypothalamus are stimulated after sensing afferent nerve impulses that activate the HPA axis and autonomic nervous system, and these nerve impulses include peripheral inflammatory molecules produced by asthma, which are transmitted to the brain through pathways such as the BBB, thereby resulting in the activation of brain regions or changes in brain function caused by the provocation of asthma by external stress factors (96, 97). This activity leads to the growing release of glucocorticoids and catecholamines (including epinephrine and norepinephrine), which can be further transmitted to the periphery to participate in the regulation of the immune inflammatory response to asthma, and the specific mechanism has been elaborated previously (98). Consequently, the modulation of circadian rhythm-related neural pathways in patients with asthma can not only reduce nocturnal sleep disorders, but also alleviate symptoms and produce the effect of one arrow and two sculptures.
3.5 Asthma and olfactory disturbances
Common chronic inflammatory diseases of the respiratory system, such as chronic rhinosinusitis (CRS) and AR, have been confirmed by many studies to be accompanied by varying degrees of olfactory dysfunction, which seriously impair the quality of life and mental health of patients, and such diseases are often complicated with asthma. Olfactory dysfunction caused by asthma is mostly induced on the basis of comorbidity with these diseases, and some scholars believe that asthma can be used as an independent risk factor for olfactory disturbance in patients with CRS (99, 100). Rhyou et al. conducted a clinical investigation on olfactory dysfunction in patients with asthma, which indicated that the incidence of olfactory disturbance in patients with CRS or AR associated with asthma was 56.5%, whereas the incidence of olfactory disturbance in patients with asthma alone was only 15.8% (10). Lacking direct research, the pathogenesis of asthma linked to olfactory dysfunction warrants further investigation.
In summary, we have a preliminary understanding of the relevant brain regions and the pathological and physiological changes that occur when asthma patients are accompanied by cognitive dysfunction, anxiety, depression and sleep disorders, as shown in Figure 1. However, there is no unified theory that can fairly and comprehensively reveal its mechanism, which deserves further in-depth consideration.
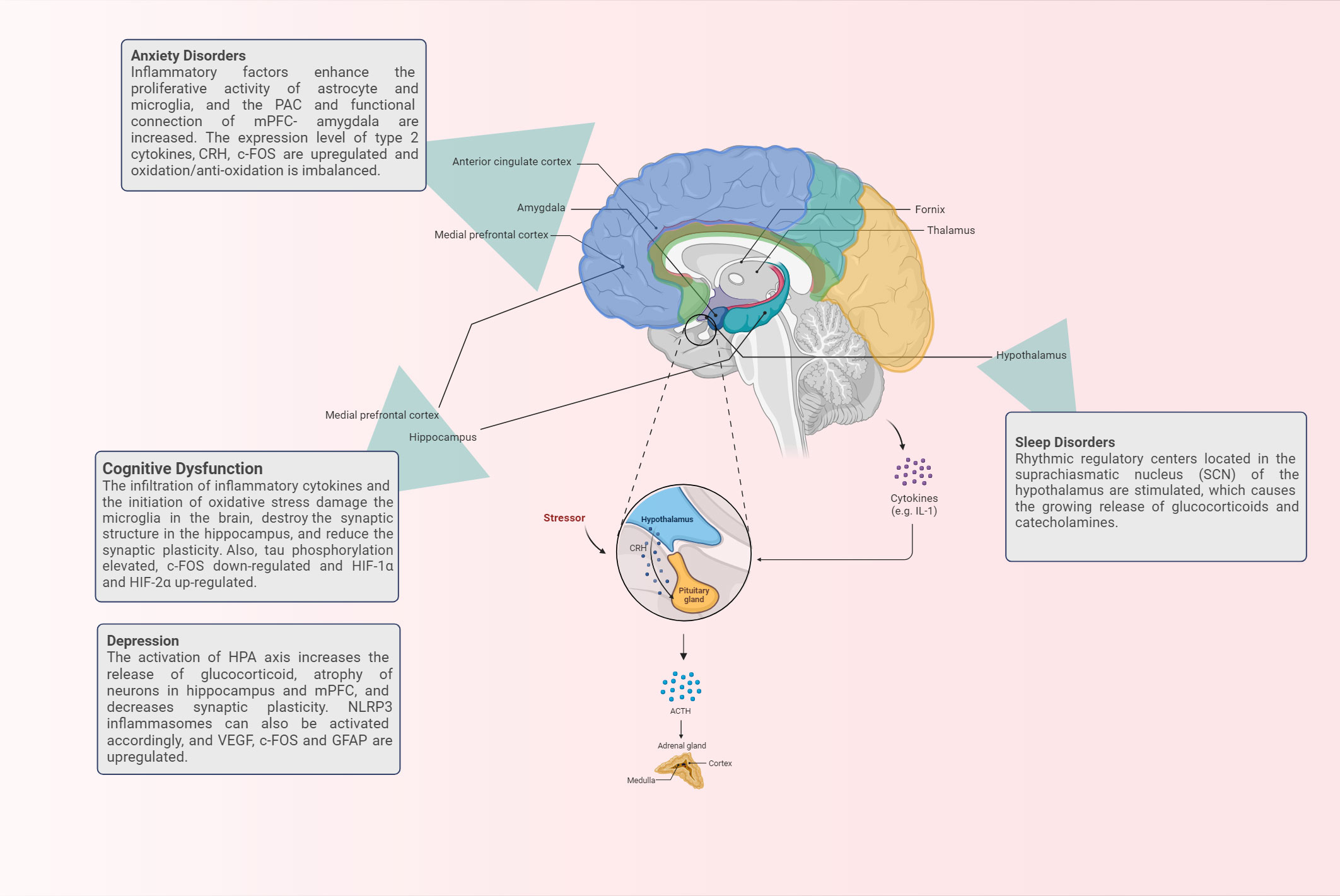
Figure 1 The brain regions and corresponding physiological mechanisms involved in the occurrence of brain response in asthma.
4 Treatment of symptoms associated with brain response in asthma
Asthma causes cognitive impairment, depression, anxiety, and other emotional disorders as well as sleep disorders, olfactory dysfunction, and other neurological phenotypes, which will aggravate the psychological burden and life stress of patients with asthma while deteriorating the clinical symptoms of asthma, thereby resulting in a low quality of life. Consequently, while developing conventional medications for the treatment of asthma, a treatment to alleviate the neurological symptoms associated with brain response in patients with asthma must also be developed. At present, neurocognitive rehabilitation training, psychological intervention, continuous positive airway pressure (CPAP), exercise intervention, family intervention, and drug therapy are widely used and we have provided a brief summary in Figure 2. These interventions can be executed individually or in combination to alleviate each other’s symptoms, but their improvement of neurological asthma symptoms remains to be investigated (101).
Cognitive behavioral therapy (CBT), as one of the most common psychological interventions, is a psychotherapy designed to change a person’s thinking and behavior patterns through talking, which has been demonstrated to have good efficacy in a variety of psychological disorders (including cognitive impairment, anxiety, and depression) (102, 103). A clinical study based on group cognitive behavioral therapy (GCBT) revealed that GCBT can effectively rectify the functional connectivity activity between insular subregions and other brain regions in patients with asthma, which is essential for improving depressive symptoms (104).Of course, other psychological interventions, such as mindfulness-based stress reduction therapy, also have their exclusive effects in alleviating the neurological symptoms of asthma, but the direct effects of these treatments on the central nervous system remain to be studied (101).
Exercise interventions, such as common running, rope skipping, and other exercise modalities, can alleviate airway inflammation by balancing the ratio of Th1/Th2-type cytokines, reduce the response level of oxidative stress in the hippocampus to lighten oxidative damage, upregulate IL-10 levels in the hippocampus to strengthen brain inflammation, promote the induction and maintenance of LTP in the brain, and facilitate the synthesis and release of BDNF to increase synaptic plasticity, thereby relieving asthma-induced cognitive impairment (105–107). Concurrently, exercise can also play a certain role in weight loss. Obesity is known to be a risk factor for comorbid cognitive impairment and sleep disorders in asthma; thus, exercise is essential for neuroprotection (108).
Given its inhibition of GORD, local and systemic anti-inflammatory effects, enhancement of cardiac function, suppression of leptin levels, weight loss, and restoration of sleep patterns, CPAP, as a first-line treatment for OSA, has a substantial impact on the improvement of sleep disturbance in patients with asthma (109). Ninety-nine asthmatic patients with OSA treated with CPAP showed significant improvement in asthma symptoms and sleep disorders after 6 months, but evaluating the efficacy of CPAP treatment was exceedingly difficult because of the absence of a healthy control group (110). In addition, adenoidectomy combined with hormone inhalation, such as montelukast, can alleviate sleep disorders in asthmatic children with OSA (111).
Neurocognitive rehabilitation training refers to the intervention of related brain response to asthma by rehabilitation methods for conventional cardiovascular and cerebrovascular diseases, which only stays at the theoretical level and has not been reliably verified. Family intervention therapy begins with the mental health status of patients’ family members and seeks to alleviate related psychological disorders in patients with asthma by fostering a healthy family environment. Brown et al. suggested that a reduction in melancholy among family caregivers could effectively control the onset and progression of depression in children with asthma (112). By contrast, conventional drug therapy, which includes cortisol hormones, β-adrenergic agonists, etc., may have varying effects on the nervous system because of their specific modes, doses, frequencies, etc., and their benefits and drawbacks contradict the conclusions of the current study. For example, early inhalation of glucocorticoids, as mentioned previously, may alleviate the anxiety symptoms of patients with asthma, but their long-term use will worsen their cognitive impairment. Accordingly, additional multicenter, randomized, double-blind, parallel controlled studies must be conducted to investigate the duality of drug effects in depth.
5 Summary and prospect
Through a systematic exposition of the incidence condition, the inflammatory factors and neural circuits involved, and corresponding treatment methods of neuropsycological symptoms in asthma, we recognize that its pathogenesis is complex and may be the result of a combination of multiple physiological and pathological mechanisms. Furthermore, we summarize its mechanism diagram in Figure 3: (1) A variety of cytokines are produced during the inflammatory response to asthma afferent the nervous system through the humoral pathway or neural pathway, of which the humoral pathway refers to the penetration of inflammatory factors through the BBB or periventricular organs without the BBB into the brain region. In addition, the neural pathway indicates the activation of sympathetic, parasympathetic, and sensory nerves via immune inflammatory factors; the release of multiple neuropeptides and neurotransmitters; and the excitation of the corresponding electrical signals to change the stimulation of the specific brain regions. (2) Inflammatory infiltration in the brain region causes the activation and proliferation of microglia and astrocytes as well as damages neurons, resulting in changes in the corresponding electrical activity of the brain, of which the brain wave amplitude, frequency, PAC between brain regions, and potential are affected. The feedback regulation of the neuroendocrine axis is also initiated accordingly, which in turn acts on related effectors in the lungs and worsens asthma symptoms. (3) Neuroinflammation transmitted to the brain influences the level of oxidative stress in the brain, and the equilibrium between oxidative and antioxidant molecules is disrupted, resulting in functional changes in specific brain regions. (4) Whether the relationship between asthma and olfactory dysfunction is mediated or directly related by nasal diseases lacks relevant evidence. A summary of changes in brain network/activity, mediators, and related brain regions involved in the response of the brain in asthma is shown in Table 2 (12, 21, 31, 34, 37, 42, 44, 49, 53, 56, 58, 59, 68, 70, 72, 74, 78, 82, 97, 113, 114).
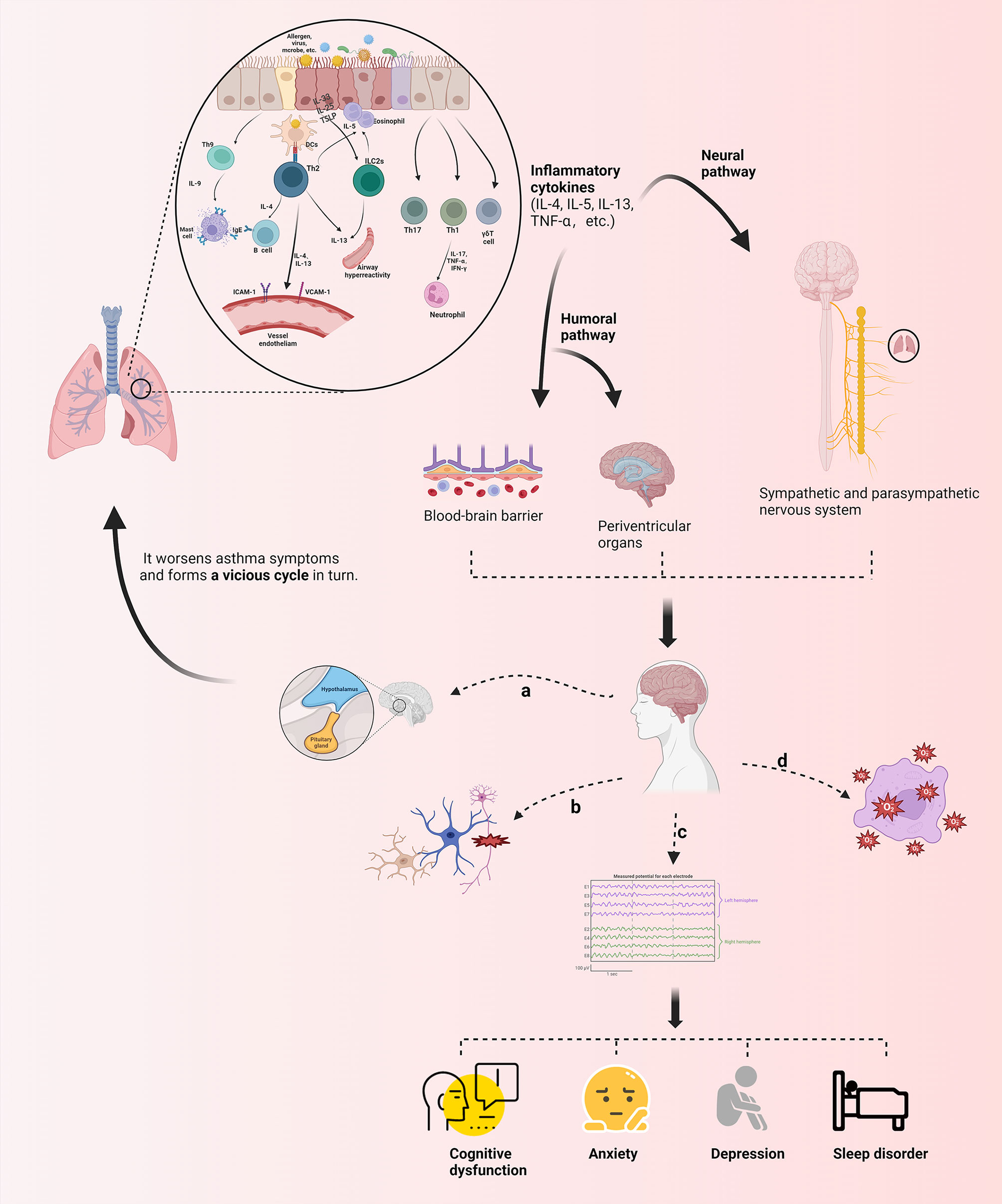
Figure 3 Schematic presentation of the mechanism of neurological phenotypes associated with asthma. Various immune inflammatory factors produced during the onset of asthma can reach the brain through humoral and neural pathways, inducing the production of corresponding brain response through different mechanisms, as follows: (A) Feedback regulation of the neuroendocrine axis (e. g) HPA) is activated; (B) Microglia, astrocyte, etc. are activated and proliferated, and neurons are damaged; (C) Brain wave amplitude, frequency, PAC between brain regions and potential are affected; (D) Imbalance in expression of oxidative and antioxidant molecules leads to changes in oxidative stress levels in brain regions. Theseneuropsycological symptoms further regulate the symptoms of asthma in turn, which forms a cycle. TSLP, the Thymic Stromal Lymphopoietin; ILC2s, group 2 innate lymphoid cells; IFN-γ, interferon-gamma; ICAM-1, intercellular adhesion molecule 1; VCAM-1, vascular cellular adhesion molecule-1 (The figure is created by BioRender.com).
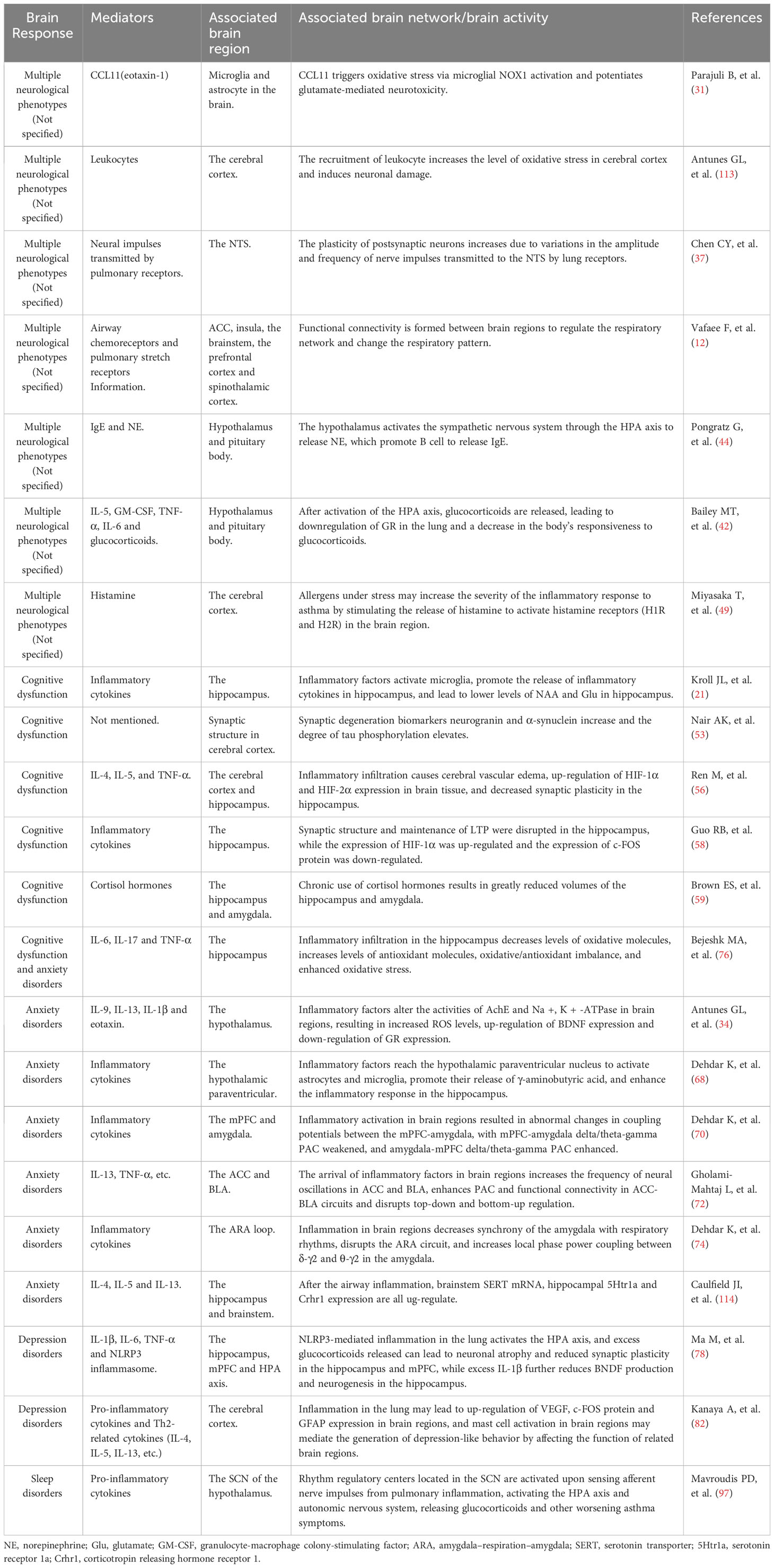
Table 2 Summary of mediators, brain regions, brain networks, and brain activities involved in the development of brain responses to asthma.
It cannot be ignored that not all cognitive dysfunction and mood disorders (anxiety, depression) associated with asthma patients are positively correlated with the level of pulmonary inflammation. For example, asthma in elderly patients was negatively correlated with the degree of cognitive impairment in a study of asthma patients of different ages by Minna et al. and others have found a strong gender bias in the distribution of asthma patients with anxiety, with significantly more males than females and a positive correlation with age (115, 116). More detailed studies have shown that asthma control and the degree of airway obstruction during exacerbations do not significantly associate with cognitive impairment in elderly patients, and we hypothesize that the inflammatory state of the body in asthma patients may be a contributing, rather than a primary, factor in the development of cognitive deficits in the elderly, but there is a lack of direct evidence to prove this (117). In addition, in a study comparing anxiety and depression symptoms in children with asthma before and after hospitalization, it was noted that more than half of the children had a significant increase in anxiety and depression, or even a combination of both, and that nearly 26% of the children showed a persistent increase in adverse moods at discharge follow-up after well controlled of lung symptoms. It does not seem to be fully explained by the theory of the “lung-brain” axis mediated by the neuroimmune crosstalk mechanisms that we have summarized 4and may be associated with the patient’s own degree of sensitivity and tolerance to the perception of symptoms such as wheezing, also known as symptom-related anxiety/depression (118, 119). To some extent, this suggests that the neuropsychological symptoms induced in asthma patients may depend not only on the severity of the asthma attack, which may reflect the level of inflammation in the body, but may also be related to the delayed response of the body to the deterioration of the disease, the stress of hospitalization, and other stressful factors, that is to say, post-traumatic stress disorder (PTSD) cannot be ruled out, especially in the context of the Corona Virus Disease 2019 (COVID-19) pandemic, the influence of the superimposed effect should not be ignored (120). Thus, it is clear that multiple factors other than neuroimmune mechanisms are involved in the development of asthma-associated neuropsychological symptoms, which deserves further investigation.
The “lung–brain” crosstalk phenomenon between asthma and its brain response is governed by a biphasic regulatory mechanism, that is, the inflammatory response caused by asthma is transmitted to the central and peripheral nervous systems through the abovementioned pathways, which stimulates the synthesis and release of neurotransmitters such as neuropeptides, which in turn can further bind to the corresponding receptors on the airway surface to promote inflammatory progression and worsen asthma symptoms. Unique occurrences, such as asthmatic mice, accompanied by cognitive impairment, alleviate the inflammatory response to relieve the symptoms of asthma. This review will encourage more researchers to focus on the brain response and its mechanism in asthma, and more in-depth studies can be conducted on this topic to provide additional evidence for guiding the diagnosis and treatment of asthma using the “lung–brain” axis theory and new hope for enhancing the quality of life of patients with asthma.
Author contributions
YW, Y-KM and H-RW contributed equally to this work. X-CS and CR: conception, design and administrative support. YW, Y-KM and H-RW: provision of study materials. YW, Y-KM and H-RW, X-YS and S-ZW: collection and assembly of data. YW, Y-KM and H-RW, CR and X-CS: data analysis and interpretation. All authors: manuscript writing and final approval of manuscript.
Funding
This work was supported by grants from the National Natural Science Foundation of China (82071021, 82271146); the Key Research and Development Program of Shandong Province (Major Science and Technology Innovation Project) (2020CXGC011302, 2022CXGC020506); the Shandong Provincial Postdoctoral Foundation Project(Innovation Project)(SDCX-ZG-202203077); and the outstanding young talents project in health of Qilu.
Conflict of interest
The authors declare that the research was conducted in the absence of any commercial or financial relationships that could be construed as a potential conflict of interest.
Publisher’s note
All claims expressed in this article are solely those of the authors and do not necessarily represent those of their affiliated organizations, or those of the publisher, the editors and the reviewers. Any product that may be evaluated in this article, or claim that may be made by its manufacturer, is not guaranteed or endorsed by the publisher.
Glossary
References
1. Fahy JV. Type 2 inflammation in asthma–present in most, absent in many. Nat Rev Immunol (2015) 15(1):57–65. doi: 10.1038/nri3786
2. Mims JW. Asthma: definitions and pathophysiology. Int Forum Allergy Rhinol (2015) 5(Suppl 1):S2–6. doi: 10.1002/alr.21609
3. Cao Y, Chen S, Chen X, Zou W, Liu Z, Wu Y, et al. Global trends in the incidence and mortality of asthma from 1990 to 2019: An age-period-cohort analysis using the global burden of disease study 2019. Front Public Health (2022) 10:1036674. doi: 10.3389/fpubh.2022.1036674
4. D’Amato G, Baena-Cagnani CE, Cecchi L, Annesi-Maesano I, Nunes C, Ansotegui I, et al. Climate change, air pollution and extreme events leading to increasing prevalence of allergic respiratory diseases. Multidiscip Respir Med (2013) 8(1):12. doi: 10.1186/2049-6958-8-12
5. Vos T, Flaxman AD, Naghavi M, Lozano R, Michaud C, Ezzati M, et al. Years lived with disability (YLDs) for 1160 sequelae of 289 diseases and injuries 1990-2010: a systematic analysis for the Global Burden of Disease Study 2010. Lancet (2012) 380(9867):628. doi: 10.1016/S0140-6736(12)61729-2
6. Papi A, Brightling C, Pedersen SE, Reddel HK. Asthma. Lancet (2018) 391(10122):783–800. doi: 10.1016/S0140-6736(17)33311-1
7. Barrios RJ, Kheradmand F, Batts L, Corry DB. Asthma: pathology and pathophysiology. Arch Pathol Lab Med (2006) 130(4):447–51. doi: 10.5858/2006-130-447-APAP
8. Rhyou HI, Nam YH. Association between cognitive function and asthma in adults. Ann Allergy Asthma Immunol (2021) 126(1):69–74. doi: 10.1016/j.anai.2020.08.022
9. Wong KO, Hunter Rowe B, Douwes J, Senthilselvan A. Asthma and wheezing are associated with depression and anxiety in adults: an analysis from 54 countries. Pulm Med (2013) 2013:929028. doi: 10.1155/2013/929028
10. Rhyou HI, Bae WY, Nam YH. Association between olfactory function and asthma in adults. J Asthma Allergy (2021) 14:309–16. doi: 10.2147/JAA.S299796
11. Wang Y, Song XY, Wei SZ, Wang HR, Zhang WB, Li YM, et al. Brain response in allergic rhinitis: Profile and proposal. J Neurosci Res (2023) 101(4):480–91. doi: 10.1002/jnr.25159
12. Vafaee F, Shirzad S, Shamsi F, Boskabady MH. Neuroscience and treatment of asthma, new therapeutic strategies and future aspects. Life Sci (2022) 292:120175. doi: 10.1016/j.lfs.2021.120175
13. Kabata H, Artis D. Neuro-immune crosstalk and allergic inflammation. J Clin Invest (2019) 129(4):1475–82. doi: 10.1172/JCI124609
14. Zhu L, Zhao J, Yang Y, Shangguan Q, Chen Y, He Y, et al. Brain network study of attentional cognitive impairment in children with bronchial asthma. Int J Dev Neurosci (2023) 83(2):224–31. doi: 10.1002/jdn.10250
15. Gholami-Mahtaj L, Salimi M, Nazari M, Tabasi F, Bamdad S, Dehdar K, et al. Asthma induces psychiatric impairments in association with default mode and salience networks alteration: A resting-state EEG study. Respir Physiol Neurobiol (2022) 300:103870. doi: 10.1016/j.resp.2022.103870
16. Rosenkranz MA, Dean DC 3rd, Bendlin BB, Jarjour NN, Esnault S, Zetterberg H, et al. Neuroimaging and biomarker evidence of neurodegeneration in asthma. J Allergy Clin Immunol (2022) 149(2):589–598.e6. doi: 10.1016/j.jaci.2021.09.010
17. Zhang Y, Yang Y, Xu X, Yuan Y. Coupling of spatial and directional functional network connectivity reveals a physiological basis for salience network hubs in asthma. Brain Imaging Behav (2022) 16(1):176–85. doi: 10.1007/s11682-021-00490-z
18. Ritz T, Kroll JL, Aslan S, Janssens T, Khan DA, Pinkham AE, et al. Subcortical gray matter volumes in asthma: associations with asthma duration, control, and anxiety. Brain Imaging Behav (2020) 14(6):2341–50. doi: 10.1007/s11682-019-00188-3
19. Gao X, Xiao Y, Lv P, Zhang W, Gong Y, Wang T, et al. Altered brain network integrity in patients with asthma: A structural connectomic diffusion tensor imaging study. Respir Physiol Neurobiol (2019) 266:89–94. doi: 10.1016/j.resp.2019.05.004
20. Ritz T, Kroll JL, Patel SV, Chen JR, Yezhuvath US, Aslan S, et al. Central nervous system signatures of affect in asthma: associations with emotion-induced bronchoconstriction, airway inflammation, and asthma control. J Appl Physiol (1985) (2019) 126(6):1725–36. doi: 10.1152/japplphysiol.01018.2018
21. Kroll JL, Steele AM, Pinkham AE, Choi C, Khan DA, Patel SV, et al. Hippocampal metabolites in asthma and their implications for cognitive function. NeuroImage Clin (2018) 19:213–21. doi: 10.1016/j.nicl.2018.04.012
22. Zhang Y, Yang Y, Wang Z, Bian R, Jiang W, Yin Y, et al. Altered regional cerebral blood flow of right cerebellum posterior lobe in asthmatic patients with or without depressive symptoms. Front Psychiatry (2018) 9:225. doi: 10.3389/fpsyt.2018.00225
23. Huang H, Li SY, Shi L, Huang X, Wang J. Altered spontaneous brain activity in patients with asthma: a resting-state functional MRI study using regional homogeneity analysis. Neuroreport (2021) 32(18):1403–7. doi: 10.1097/WNR.0000000000001736
24. Rosenkranz MA, Busse WW, Sheridan JF, Crisafi GM, Davidson RJ. Are there neurophenotypes for asthma? Functional brain imaging of the interaction between emotion and inflammation in asthma. PloS One (2012) 7(8):e40921. doi: 10.1371/journal.pone.0040921
25. von Leupoldt A, Sommer T, Kegat S, Eippert F, Baumann HJ, Klose H, et al. Down-regulation of insular cortex responses to dyspnea and pain in asthma. Am J Respir Crit Care Med (2009) 180(3):232–8. doi: 10.1164/rccm.200902-0300OC
26. Calhoun VD, Miller R, Pearlson G, Adalı T. The chronnectome: time-varying connectivity networks as the next frontier in fMRI data discovery. Neuron (2014) 84(2):262–74. doi: 10.1016/j.neuron.2014.10.015
27. Liu F, Wang Y, Li M, Wang W, Li R, Zhang Z, et al. Dynamic functional network connectivity in idiopathic generalized epilepsy with generalized tonic-clonic seizure. Hum Brain Mapp (2017) 38(2):957–73. doi: 10.1002/hbm.23430
28. Wang T, Huang X, Wang J. Asthma’s effect on brain connectivity and cognitive decline. Front Neurol (2023) 13:1065942. doi: 10.3389/fneur.2022.1065942
29. Varatharaj A, Galea I. The blood-brain barrier in systemic inflammation. Brain Behav Immun (2017) 60:1–12. doi: 10.1016/j.bbi.2016.03.010
30. Erickson MA, Morofuji Y, Owen JB, Banks WA. Rapid transport of CCL11 across the blood-brain barrier: regional variation and importance of blood cells. J Pharmacol Exp Ther (2014) 349(3):497–507. doi: 10.1124/jpet.114.213074
31. Parajuli B, Horiuchi H, Mizuno T, Takeuchi H, Suzumura A. CCL11 enhances excitotoxic neuronal death by producing reactive oxygen species in microglia. Glia (2015) 63(12):2274–84. doi: 10.1002/glia.22892
32. Florentino D, Della Giustina A, de Souza Goldim MP, Danielski LG, de Oliveira Junior AN, Joaquim L, et al. Early life neuroimmune challenge protects the brain after sepsis in adult rats. Neurochem Int (2020) 135:104712. doi: 10.1016/j.neuint.2020.104712
33. Danielski LG, Giustina AD, Badawy M, Barichello T, Quevedo J, Dal-Pizzol F, et al. Brain barrier breakdown as a cause and consequence of neuroinflammation in sepsis. Mol Neurobiol (2018) 55(2):1045–53. doi: 10.1007/s12035-016-0356-7
34. Antunes GL, Silveira JS, Luft C, Greggio S, Venturin GT, Schmitz F, et al. Airway inflammation induces anxiety-like behavior through neuroinflammatory, neurochemical, and neurometabolic changes in an allergic asthma model. Metab Brain Dis (2022) 37(4):911–26. doi: 10.1007/s11011-022-00907-8
35. Vizi ES, Tŏrŏk T, Seregi A, Serfŏzŏ P, Adam-Vizi V. Na-K activated ATPase and the release of acetylcholine and noradrenaline. J Physiol (Paris) (1982) 78(4):399–406. doi: 10.1007/978-1-4684-8643-8_18
36. Dantzer R, O’Connor JC, Freund GG, Johnson RW, Kelley KW. From inflammation to sickness and depression: when the immune system subjugates the brain. Nat Rev Neurosci (2008) 9(1):46–56. doi: 10.1038/nrn2297
37. Chen CY, Bonham AC, Schelegle ES, Gershwin LJ, Plopper CG, Joad JP. Extended allergen exposure in asthmatic monkeys induces neuroplasticity in nucleus tractus solitarius. J Allergy Clin Immunol (2001) 108(4):557–62. doi: 10.1067/mai.2001.118132
38. Yu L, De Mazancourt M, Hess A, Ashadi FR, Klein I, Mal H, et al. Functional connectivity and information flow of the respiratory neural network in chronic obstructive pulmonary disease. Hum Brain Mapp (2016) 37(8):2736–54. doi: 10.1002/hbm.23205
39. Davenport PW, Cruz M, Stecenko AA, Kifle Y. Respiratory-related evoked potentials in children with life-threatening asthma. Am J Respir Crit Care Med (2000) 161(6):1830–5. doi: 10.1164/ajrccm.161.6.9903077
40. Chen E, Miller GE. Stress and inflammation in exacerbations of asthma. Brain Behav Immun (2007) 21(8):993–9. doi: 10.1016/j.bbi.2007.03.009
41. Miyasaka T, Dobashi-Okuyama K, Takahashi T, Takayanagi M, Ohno I. The interplay between neuroendocrine activity and psychological stress-induced exacerbation of allergic asthma. Allergol Int (2018) 67(1):32–42. doi: 10.1016/j.alit.2017.04.013
42. Bailey MT, Kierstein S, Sharma S, Spaits M, Kinsey SG, Tliba O, et al. Social stress enhances allergen-induced airway inflammation in mice and inhibits corticosteroid responsiveness of cytokine production. J Immunol (2009) 182(12):7888–96. doi: 10.4049/jimmunol.0800891
43. Quan N, Avitsur R, Stark JL, He L, Lai W, Dhabhar F, et al. Molecular mechanisms of glucocorticoid resistance in splenocytes of socially stressed male mice. J Neuroimmunol (2003) 137(1-2):51–8. doi: 10.1016/s0165-5728(03)00042-0
44. Pongratz G, McAlees JW, Conrad DH, Erbe RS, Haas KM, Sanders VM. The level of IgE produced by a B cell is regulated by norepinephrine in a p38 MAPK- and CD23-dependent manner. J Immunol (2006) 177(5):2926–38. doi: 10.4049/jimmunol.177.5.2926
45. Borish L, Chipps B, Deniz Y, Gujrathi S, Zheng B, Dolan CM, et al. Total serum IgE levels in a large cohort of patients with severe or difficult-to-treat asthma. Ann Allergy Asthma Immunol (2005) 95(3):247–53. doi: 10.1016/S1081-1206(10)61221-5
46. Toskala E, Kennedy DW. Asthma risk factors. Int Forum Allergy Rhinol (2015) 5(Suppl 1):S11–6. doi: 10.1002/alr.21557
47. Papathanassoglou ED, Giannakopoulou M, Mpouzika M, Bozas E, Karabinis A. Potential effects of stress in critical illness through the role of stress neuropeptides. Nurs Crit Care (2010) 15(4):204–16. doi: 10.1111/j.1478-5153.2010.00363.x
48. Lu Y, Ho R, Lim TK, Kuan WS, Goh DYT, Mahadevan M, et al. Neuropeptide Y may mediate psychological stress and enhance TH2 inflammatory response in asthma. J Allergy Clin Immunol (2015) 135(4):1061–3. doi: 10.1016/j.jaci.2014.10.036
49. Miyasaka T, Okuyama-Dobashi K, Masuda C, Iwami S, Sato M, Mizoguchi H, et al. The involvement of central nervous system histamine receptors in psychological stress-induced exacerbation of allergic airway inflammation in mice. Allergol Int (2016) 65(Suppl):S38–44. doi: 10.1016/j.alit.2016.05.015
50. Irani F, Barbone JM, Beausoleil J, Gerald L. Is asthma associated with cognitive impairments? A meta-analytic review. J Clin Exp Neuropsychol (2017) 39(10):965–78. doi: 10.1080/13803395.2017.1288802
51. Caldera-Alvarado G, Khan DA, Defina LF, Pieper A, Brown ES. Relationship between asthma and cognition: the Cooper Center Longitudinal Study. Allergy (2013) 68(4):545–8. doi: 10.1111/all.12125
52. Dozor AJ. The role of oxidative stress in the pathogenesis and treatment of asthma. Ann N Y Acad Sci (2010) 1203:133–7. doi: 10.1111/j.1749-6632.2010.05562.x
53. Nair AK, Van Hulle CA, Bendlin BB, Zetterberg H, Blennow K, Wild N, et al. Asthma amplifies dementia risk: Evidence from CSF biomarkers and cognitive decline. Alzheimers Dement (N Y) (2022) 8(1):e12315. doi: 10.1002/trc2.12315
54. Gruneberg D, Montellano FA, Plaschke K, Li L, Marti HH, Kunze R. Neuronal prolyl-4-hydroxylase 2 deficiency improves cognitive abilities in a murine model of cerebral hypoperfusion. Exp Neurol (2016) 286:93–106. doi: 10.1016/j.expneurol.2016.10.001
55. Vargas Becerra MH. Fisiopatología del asma [Physiopathology of asthma]. Rev Alerg Mex (2009) 56(Suppl 1):S24–8.
56. Ren M, Feng M, Long Z, Ma J, Peng X, He G. Allergic asthma-induced cognitive impairment is alleviated by dexamethasone. Front Pharmacol (2021) 12:680815. doi: 10.3389/fphar.2021.680815
57. Pitts MW. Barnes maze procedure for spatial learning and memory in mice. Bio Protoc (2018) 8(5):e2744. doi: 10.21769/bioprotoc.2744
58. Guo RB, Sun PL, Zhao AP, Gu J, Ding X, Qi J, et al. Chronic asthma results in cognitive dysfunction in immature mice. Exp Neurol (2013) 247:209–17. doi: 10.1016/j.expneurol.2013.04.008
59. Brown ES, Vera E, Frol AB, Woolston DJ, Johnson B. Effects of chronic prednisone therapy on mood and memory. J Affect Disord (2007) 99(1-3):279–83. doi: 10.1016/j.jad.2006.09.004
60. Wu Y, Zhao Y, Xu T, You L, Zhang H, Liu F. Alzheimer’s disease affects severity of asthma through methylation control of foxp3 promoter. J Alzheimers Dis (2019) 70(1):121–9. doi: 10.3233/JAD-190315
61. Dudeney J, Sharpe L, Jaffe A, Jones EB, Hunt C. Anxiety in youth with asthma: A meta-analysis. Pediatr Pulmonol (2017) 52(9):1121–9. doi: 10.1002/ppul.23689
62. Braido F, Brusselle G, Guastalla D, Ingrassia E, Nicolini G, Price D, et al. Determinants and impact of suboptimal asthma control in Europe: The international cross-sectional and longitudinal assessment on asthma control (LIAISON) study. Respir Res (2016) 17(1):51. doi: 10.1186/s12931-016-0374-z
63. Stubbs MA, Clark VL, Gibson PG, Yorke J, McDonald VM. Associations of symptoms of anxiety and depression with health-status, asthma control, dyspnoea, dysfunction breathing and obesity in people with severe asthma. Respir Res (2022) 23(1):341. doi: 10.1186/s12931-022-02266-5
64. Bukalo O, Pinard CR, Silverstein S, Brehm C, Hartley ND, Whittle N, et al. Prefrontal inputs to the amygdala instruct fear extinction memory formation. Sci Adv (2015) 1(6):e1500251. doi: 10.1126/sciadv.1500251
65. Liu WZ, Zhang WH, Zheng ZH, Zou JX, Liu XX, Huang SH, et al. Identification of a prefrontal cortex-to-amygdala pathway for chronic stress-induced anxiety. Nat Commun (2020) 11(1):2221. doi: 10.1038/s41467-020-15920-7
66. Shim HS, Park HJ, Woo J, Lee CJ, Shim I. Role of astrocytic GABAergic system on inflammatory cytokine-induced anxiety-like behavior. Neuropharmacology (2019) 160:107776. doi: 10.1016/j.neuropharm.2019.107776
67. Wang YL, Han QQ, Gong WQ, Pan DH, Wang LZ, Hu W, et al. Microglial activation mediates chronic mild stress-induced depressive- and anxiety-like behavior in adult rats. J Neuroinflammation (2018) 15(1):21. doi: 10.1186/s12974-018-1054-3
68. Dehdar K, Mahdidoust S, Salimi M, Gholami-Mahtaj L, Nazari M, Mohammadi S, et al. Allergen-induced anxiety-like behavior is associated with disruption of medial prefrontal cortex - amygdala circuit. Sci Rep (2019) 9(1):19586. doi: 10.1038/s41598-019-55539-3
69. Stujenske JM, Likhtik E, Topiwala MA, Gordon JA. Fear and safety engage competing patterns of theta-gamma coupling in the basolateral amygdala. Neuron (2014) 83(4):919–33. doi: 10.1016/j.neuron.2014.07.026
70. Dehdar K, Mooziri M, Samii Moghaddam A, Salimi M, Nazari M, Dehghan S, et al. Corticosteroid treatment attenuates anxiety and mPFC-amygdala circuit dysfunction in allergic asthma. Life Sci (2023) 315:121373. doi: 10.1016/j.lfs.2023.121373
71. Sellmeijer J, Mathis V, Hugel S, Li XH, Song Q, Chen QY, et al. Hyperactivity of anterior cingulate cortex areas 24a/24b drives chronic pain-induced anxiodepressive-like consequences. J Neurosci (2018) 38(12):3102–15. doi: 10.1523/JNEUROSCI.3195-17.2018
72. Gholami-Mahtaj L, Mooziri M, Dehdar K, Abdolsamadi M, Salimi M, Raoufy MR. ACC-BLA functional connectivity disruption in allergic inflammation is associated with anxiety. Sci Rep (2022) 12(1):2731. doi: 10.1038/s41598-022-06748-w
73. Bondarenko E, Hodgson DM, Nalivaiko E. Amygdala mediates respiratory responses to sudden arousing stimuli and to restraint stress in rats. Am J Physiol Regul Integr Comp Physiol (2014) 306(12):R951–9. doi: 10.1152/ajpregu.00528.2013
74. Dehdar K, Salimi M, Raoufy MR. Allergen disrupts amygdala-respiration coupling. Respir Physiol Neurobiol (2022) 297:103835. doi: 10.1016/j.resp.2021.103835
75. Tonelli LH, Katz M, Kovacsics CE, Gould TD, Joppy B, Hoshino A, et al. Allergic rhinitis induces anxiety-like behavior and altered social interaction in rodents. Brain Behav Immun (2009) 23(6):784–93. doi: 10.1016/j.bbi.2009.02.017
76. Bejeshk MA, Aminizadeh AH, Jafari E, Motamedi S, Zangiabadi I, Ghasemi A, et al. Myrtenol ameliorates recognition memories’ Impairment and anxiety-like behaviors induced by asthma by mitigating hippocampal inflammation and oxidative stress in rats. Neuroimmunomodulation (2023) 30(1):42–54. doi: 10.1159/000528626
77. Rosenkranz MA, Davidson RJ. Affective neural circuitry and mind-body influences in asthma. Neuroimage (2009) 47(3):972–80. doi: 10.1016/j.neuroimage.2009.05.042
78. Ma M, Li G, Qi M, Jiang W, Zhou R. Inhibition of the inflammasome activity of NLRP3 attenuates HDM-induced allergic asthma. Front Immunol (2021) 12:718779. doi: 10.3389/fimmu.2021.718779
79. Sorrells SF, Sapolsky RM. An inflammatory review of glucocorticoid actions?in?the CNS. Brain Behav Immun (2007) 21(3):259–72. doi: 10.1016/j.bbi.2006.11.006
80. Mangan MSJ, Olhava EJ, Roush WR, Seidel HM, Glick GD, Latz E. Targeting the NLRP3 inflammasome in inflammatory diseases. Nat Rev Drug Discovery (2018) 17(9):688. doi: 10.1038/nrd.2018.149
81. Iwata M, Ota KT, Duman RS. The inflammasome: pathways linking psychological stress, depression, and systemic illnesses. Brain Behav Immun (2013) 31:105–14. doi: 10.1016/j.bbi.2012.12.008
82. Kanaya A, Yang M, Emala C, Mikami M. Chronic allergic lung inflammation negatively influences neurobehavioral outcomes in mice. J Neuroinflamm (2022) 19(1):210. doi: 10.1186/s12974-022-02575-y
83. Argaw AT, Asp L, Zhang J, Navrazhina K, Pham T, Mariani JN, et al. Astrocyte-derived VEGF-A drives blood-brain barrier disruption in CNS inflammatory disease. J Clin Invest (2012) 122(7):2454–68. doi: 10.1172/JCI60842
84. Jiang M, Qin P, Yang X. Comorbidity between depression and asthma via immune-inflammatory pathways: a meta-analysis. J Affect Disord (2014) 166:22–9. doi: 10.1016/j.jad.2014.04.027
85. Janson C, De Backer W, Gislason T, Plaschke P, Björnsson E, Hetta J, et al. Increased prevalence of sleep disturbances and daytime sleepiness in subjects with bronchial asthma: a population study of young adults in three European countries. Eur Respir J (1996) 9(10):2132–8. doi: 10.1183/09031936.96.09102132
86. Kong DL, Qin Z, Shen H, Jin HY, Wang W, Wang ZF. Association of obstructive sleep apnea with asthma: A meta-analysis. Sci Rep (2017) 7(1):4088. doi: 10.1038/s41598-017-04446-6
87. Teodorescu M, Broytman O, Curran-Everett D, Sorkness RL, Crisafi G, Bleecker ER, et al. Obstructive sleep apnea risk, asthma burden, and lower airway inflammation in adults in the severe asthma research program (SARP) II. J Allergy Clin Immunol Pract (2015) 3(4):566–75.e1. doi: 10.1016/j.jaip.2015.04.002
88. Wang Y, Liu K, Hu K, Yang J, Li Z, Nie M, et al. Impact of obstructive sleep apnea on severe asthma exacerbations. Sleep Med (2016) 26:1–5. doi: 10.1016/j.sleep.2016.06.013
89. Dixit R, Verma S, Gupta N, Sharma A, Chandran A. Obstructive sleep apnea in bronchial asthma patients: assessment of prevalence and risk factors. J Assoc Physicians India (2018) 66(9):45–8.
90. Bonnet R, Jörres R, Heitmann U, Magnussen H. Circadian rhythm in airway responsiveness and airway tone in patients with mild asthma. J Appl Physiol (1985) (1991) 71(4):1598–605. doi: 10.1152/jappl.1991.71.4.1598
91. Gervais P, Reinberg A, Gervais C, Smolensky M, DeFrance O. Twenty-four-hour rhythm in the bronchial hyperreactivity to house dust in asthmatics. J Allergy Clin Immunol (1977) 59(3):207–13. doi: 10.1016/0091-6749(77)90151-8
92. Kavanagh J, Jackson DJ, Kent BD. Sleep and asthma. Curr Opin Pulm Med (2018) 24(6):569–73. doi: 10.1097/MCP.0000000000000526
93. Paganelli R, Petrarca C, Di Gioacchino M. Biological clocks: their relevance to immune-allergic diseases. Clin Mol Allergy (2018) 16:1. doi: 10.1186/s12948-018-0080-0
94. Ehlers A, Xie W, Agapov E, Brown S, Steinberg D, Tidwell R, et al. BMAL1 links the circadian clock to viral airway pathology and asthma phenotypes. Mucosal Immunol (2018) 11(1):97–111. doi: 10.1038/mi.2017.24
95. Zasłona Z, Case S, Early JO, Lalor SJ, McLoughlin RM, Curtis AM, et al. The circadian protein BMAL1 in myeloid cells is a negative regulator of allergic asthma. Am J Physiol Lung Cell Mol Physiol (2017) 312(6):L855–60. doi: 10.1152/ajplung.00072.2017
96. Wijnen H, Young MW. Interplay of circadian clocks and metabolic rhythms. Annu Rev Genet (2006) 40:409–48. doi: 10.1146/annurev.genet.40.110405.090603
97. Mavroudis PD, Scheff JD, Calvano SE, Androulakis IP. Systems biology of circadian-immune interactions. J Innate Immun (2013) 5(2):153–62. doi: 10.1159/000342427
98. Dickmeis T. Glucocorticoids and the circadian clock. J Endocrinol (2009) 200(1):3–22. doi: 10.1677/JOE-08-0415
99. Katotomichelakis M, Simopoulos E, Zhang N, Tripsianis G, Danielides G, Livaditis M, et al. Olfactory dysfunction and asthma as risk factors for poor quality of life in upper airway diseases. Am J Rhinol Allergy (2013) 27(4):293–8. doi: 10.2500/ajra.2013.27.3903
100. Litvack JR, Fong K, Mace J, James KE, Smith TL. Predictors of olfactory dysfunction in patients with chronic rhinosinusitis. Laryngoscope (2008) 118(12):2225–30. doi: 10.1097/MLG.0b013e318184e216
101. Kroll JL, Ritz T. Asthma, the central nervous system, and neurocognition: Current findings, potential mechanisms, and treatment implications. Neurosci Biobehav Rev (2023) 146:105063. doi: 10.1016/j.neubiorev.2023.105063
102. Thoma N, Pilecki B, McKay D. Contemporary cognitive behavior therapy: A review of theory, history, and evidence. Psychodyn Psychiatry (2015) 43(3):423–61. doi: 10.1521/pdps.2015.43.3.423
103. Zhang Y, Yin Y, Yang Y, Bian R, Hou Z, Yue Y, et al. Group cognitive behavior therapy reversed abnormal spontaneous brain activity in adult asthmatic patients. Psychother Psychosom (2017) 86(3):178–80. doi: 10.1159/000453584
104. Zhang Y, Yang Y, Bian R, Yin Y, Hou Z, Yue Y, et al. Group cognitive behavior therapy reversed insula subregions functional connectivity in asthmatic patients. Front Aging Neurosci (2017) 9:105. doi: 10.3389/fnagi.2017.00105
105. Mokhtari-Zaer A, Hosseini M, Roshan NM, Boskabady MH. Treadmill exercise?ameliorates memory deficits and hippocampal inflammation in ovalbumin-sensitized juvenile rats. Brain Res Bull (2020) 165:40–7. doi: 10.1016/j.brainresbull.2020.09.016
106. Mokhtari-Zaer A, Saadat S, Marefati N, Hosseini M, Boskabady MH. Treadmill exercise restores memory and hippocampal synaptic plasticity impairments in ovalbumin-sensitized juvenile rats: Involvement of brain-derived neurotrophic factor (BDNF). Neurochem Int (2020) 135:104691. doi: 10.1016/j.neuint.2020.104691
107. Jahangiri Z, Gholamnezhad Z, Hosseini M. The effects of exercise on hippocampal inflammatory cytokine levels, brain oxidative stress markers and memory impairments induced by lipopolysaccharide in rats. Metab Brain Dis (2019) 34(4):1157–69. doi: 10.1007/s11011-019-00410-7
108. Alfaro FJ, Gavrieli A, Saade-Lemus P, Lioutas VA, Upadhyay J, Novak V. White matter microstructure and cognitive decline in metabolic syndrome: a review of diffusion tensor imaging. Metabolism (2018) 78:52–68. doi: 10.1016/j.metabol.2017.08.009
109. Alkhalil M, Schulman ES, Getsy J. Obstructive sleep apnea syndrome and asthma: the role of continuous positive airway pressure treatment. Ann Allergy Asthma Immunol (2008) 101(4):350–7. doi: 10.1016/S1081-1206(10)60309-2
110. Serrano-Pariente J, Plaza V, Soriano JB, Mayos M, López-Viña A, Picado C, et al. Asthma outcomes improve with continuous positive airway pressure for obstructive sleep apnea. Allergy (2017) 72(5):802–12. doi: 10.1111/all.13070
111. Cingi C, Gevaert P, Mösges R, Rondon C, Hox V, Rudenko M, et al. Multi-morbidities of allergic rhinitis in adults: European Academy of Allergy and Clinical Immunology Task Force Report. Clin Transl Allergy (2017) 7:17. doi: 10.1186/s13601-017-0153-z
112. Brown ES, Palka JM, Lehman HK, Kulikova A, Khan DA, Lopez J, et al. The impact of caregiver depression on child asthma outcomes: pathways and mechanisms. J Allergy Clin Immunol Pract (2023) 11(1):200–9. doi: 10.1016/j.jaip.2022.09.016
113. Antunes GL, Silveira JS, Kaiber DB, Luft C, Dos Santos TM, Marques EP, et al. Neostigmine treatment induces neuroprotection against oxidative stress in cerebral cortex of asthmatic mice. Metab Brain Dis (2020) 35(5):765–74. doi: 10.1007/s11011-020-00558-7
114. Caulfield JI, Caruso MJ, Michael KC, Bourne RA, Chirichella NR, Klein LC, et al. Peri-adolescent asthma symptoms cause adult anxiety-related behavior and neurobiological processes in mice. Behav Brain Res (2017) 326:244–55. doi: 10.1016/j.bbr.2017.02.046
115. Rusanen M, Ngandu T, Laatikainen T, Tuomilehto J, Soininen H, Kivipelto M. Chronic obstructive pulmonary disease and asthma and the risk of mild cognitive impairment and dementia: a population based CAIDE study. Curr Alzheimer Res (2013) 10(5):549–55. doi: 10.2174/1567205011310050011
116. Kimura M, Ikeda A, Suzuki Y, Maruyama K, Wada H, Tanigawa T. The association between asthma and anxiety in elementary school students in Japan. Pediatr Pulmonol (2020) 55(10):2603–9. doi: 10.1002/ppul.24986
117. Ray M, Sano M, Wisnivesky JP, Wolf MS, Federman AD. Asthma control and cognitive function in a cohort of elderly adults. J Am Geriatr Soc (2015) 63(4):684–91. doi: 10.1111/jgs.13350
118. Morin H, Worsley D, Zhang X, Faerber J, Pettit AR, Kenyon CC, et al. Depression and anxiety symptoms during and after pediatric asthma hospitalization. Hosp Pediatr (2021) 11(11):1272–80. doi: 10.1542/hpeds.2020-000950
119. Mou YK, Wang HR, Zhang WB, Zhang Y, Ren C, Song XC. Allergic rhinitis and depression: profile and proposal. Front Psychiatry (2022) 12:820497. doi: 10.3389/fpsyt.2021.820497
Keywords: asthma, brain response, lung-brain axis, neuro-immune, inflammatory response
Citation: Wang Y, Mou Y-K, Wang H-R, Song X-Y, Wei S-Z, Ren C and Song X-C (2023) Brain response in asthma: the role of “lung-brain” axis mediated by neuroimmune crosstalk. Front. Immunol. 14:1240248. doi: 10.3389/fimmu.2023.1240248
Received: 14 June 2023; Accepted: 09 August 2023;
Published: 24 August 2023.
Edited by:
Man Li, Huazhong University of Science and Technology, ChinaReviewed by:
Jonathan David Turner, Luxembourg Institute of Health, LuxembourgWakiro Sato, National Center of Neurology and Psychiatry (Japan), Japan
Copyright © 2023 Wang, Mou, Wang, Song, Wei, Ren and Song. This is an open-access article distributed under the terms of the Creative Commons Attribution License (CC BY). The use, distribution or reproduction in other forums is permitted, provided the original author(s) and the copyright owner(s) are credited and that the original publication in this journal is cited, in accordance with accepted academic practice. No use, distribution or reproduction is permitted which does not comply with these terms.
*Correspondence: Chao Ren, renchaotg@126.com; Xi-Cheng Song, drxchsong@163.com
†These authors have contributed equally to this work