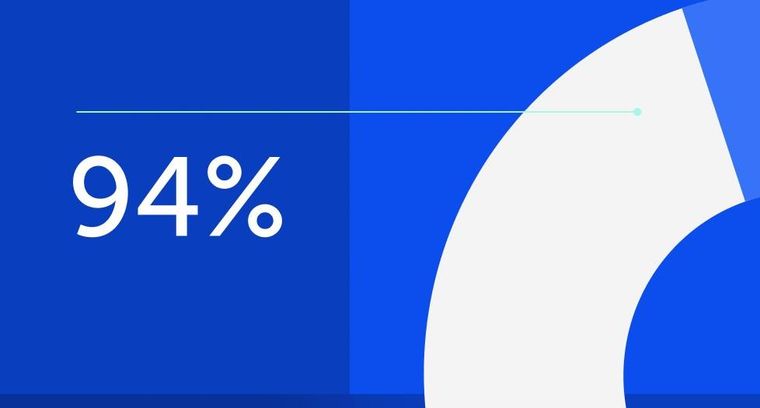
94% of researchers rate our articles as excellent or good
Learn more about the work of our research integrity team to safeguard the quality of each article we publish.
Find out more
REVIEW article
Front. Immunol., 11 September 2023
Sec. Inflammation
Volume 14 - 2023 | https://doi.org/10.3389/fimmu.2023.1215566
This article is part of the Research TopicNeuro-Immune Players of Peripheral Pain SignallingView all 7 articles
Pre-clinical evidence shows that neuropathy is associated with complex neuroimmune responses, which in turn are associated with increased intensity and persistence of neuropathic pain. Routine exercise has the potential to mitigate complications of future nerve damage and persistence of pain through neuroimmune regulation. This systematic review aimed to explore the effect of pre-injury exercise on neuroimmune responses, and other physiological and behavioural reactions following peripheral neuropathy in animals. Three electronic databases were searched from inception to July 2022. All controlled animal studies assessing the influence of an active exercise program prior to experimentally-induced traumatic peripheral neuropathy compared to a non-exercise control group on neuroimmune, physiological and behavioural outcomes were selected. The search identified 17,431 records. After screening, 11 articles were included. Meta-analyses showed that pre-injury exercise significantly reduced levels of IL-1β (SMD: -1.06, 95% CI: -1.99 to -0.13, n=40), but not iNOS (SMD: -0.71 95% CI: -1.66 to 0.25, n=82). From 72 comparisons of different neuroimmune outcomes at different anatomical locations, vote counting revealed reductions in 23 pro-inflammatory and increases in 6 anti-inflammatory neuroimmune outcomes. For physiological outcomes, meta-analyses revealed that pre-injury exercise improved one out of six nerve morphometric related outcomes (G-ratio; SMD: 1.95, 95%CI: 0.77 to 3.12, n=20) and one out of two muscle morphometric outcomes (muscle fibre cross-sectional area; SMD: 0.91, 95%CI: 0.27 to 1.54, n=48). For behavioural outcomes, mechanical allodynia was significantly less in the pre-injury exercise group (SMD -1.24, 95%CI: -1.87 to -0.61) whereas no overall effect was seen for sciatic function index. Post hoc subgroup analysis suggests that timing of outcome measurement may influence the effect of pre-injury exercise on mechanical allodynia. Risk of bias was unclear in most studies, as the design and conduct of the included experiments were poorly reported. Preventative exercise may have potential neuroprotective and immunoregulatory effects limiting the sequalae of nerve injury, but more research in this field is urgently needed.
Regular exercise is associated with less chronic pain (1) and reduces the risk of several chronic conditions, such as cardiovascular disease, diabetes, metabolic syndrome, and even neurodegenerative diseases (2–5). However, for peripheral neuropathic pain conditions, the preventative effects of exercise are less clear. Exercise is recommended for patients with neuropathic pain (6), but research on the effect of exercise on neuropathic pain has mostly focused on exercise prescribed as a treatment after sustaining the injury (7, 8). A recent review and meta-analysis about the effect of exercise on experimentally-induced neuropathic pain in animals included exercise programs that started both before and after peripheral neuropathy induction (9). Interestingly, this review found that the beneficial effect of exercise on mechanical allodynia was related to the timing of the exercise program, alluding to a benefit of exercise prior to sustaining a neuropathy. Unfortunately, underlying working mechanisms of exercise were not synthesised along with the mechanical allodynia results (9).
Animal experimental studies show that nerve injuries result in a cascade of complex neuroimmune responses involving multiple mediators, such as neuropeptides, cytokines, gene expression and hormones (10–13). However, the balance of pro- and anti-inflammatory responses are integral for the recovery/healing process (14, 15). For example, initial pro-inflammatory responses to nerve damage, such as transient neutrophil activation, are normal, and might even be protective against the development of chronic pain (15, 16). On the other hand, pro-inflammatory responses (such as dorsal root ganglion (DRG) macrophage activation (17), dorsal horn microglia activation (18), and pronociceptive cytokines and chemokines (19)) are responsible for predisposing the development of neuropathic pain (hyperalgesia/allodynia) and persistence of the pain. Furthermore, pain resolution after peripheral nerve injury requires regulatory T cells and M2 macrophages [18]. Altogether, a maladaptive inflammatory response to peripheral nerve injury contributes to the generation of persistence of pain (16). Thus, it seems ideal to prevent an excessive inflammatory response and/or promote the anti-inflammatory resolution phase.
Sedentary behaviour, in addition to injury, promotes and prolongs this pro-inflammatory response via alterations in macrophage phenotype (M1) at the site of injury (20). In addition to immune alterations, sedentary behaviour changes the reactivity of the central nervous system promoting greater excitability and less inhibition (21). Exercise, on the other hand, promotes anti-inflammatory responses (22, 23) increased central nociceptive inhibition and decreased central excitability aiding in healing and analgesia (21, 24). The immunoregulatory effects of exercise may be mediated by multiple mechanisms. Muscular contraction induces cytosolic changes (increased calcium ions, activation of p38 MAPK etc.) which leads to activation of transcription factors (e.g., activation protein (AP)-1) leading to the production of myokines (e.g., IL-6). Together with increased sympathoadrenal activity these responses induce an anti-inflammatory environment with each bout of exercise (22, 25, 26). Routine exercise has the potential to mitigate pain through neuroimmune regulation, as pre-injury exercise in animals prevented hyperalgesia resulting from repeated acidic saline injections by promoting anti-inflammatory mechanisms (27, 28). This suggests that exercise primes the neuroimmune system to an anti-inflammatory state and in turn can reduce the complications of future nerve damage and persistence of pain.
The literature on the potential role pre-injury exercise may play in reducing the consequences of peripheral neuropathy through neuroimmune responses has not yet been summarised. More insight is needed in what exercise type, program length, frequency, duration, intensity, and timing are necessary for improvements in pain-related and neuroimmune outcomes. For ethical reasons, invasive experimental research in this domain (e.g., immunohistochemistry of the nervous system) is not possible in humans. Research in animals may provide insight into processes that may be similar in humans. Therefore, the aim of this systematic review was to summarise the evidence on the effect of pre-injury exercise on neuroimmune, physiological and behavioural outcomes following experimentally-induced traumatic peripheral neuropathy in animals.
The review was reported according to the Preferred Reporting Items for Systematic Reviews and Meta-Analysis (PRISMA) guidelines (29) and registered at the International Prospective Register of Systematic Review (PROSPERO), CRD42021245899.
MEDLINE (via PubMed), Web of Science and EMBASE databases were searched from inception until July 5, 2022. The search strategy was developed together with a research librarian (Alice Tillema) and consisted of three components (1) animals, (2) neuropathy and (3) exercise (Appendix A). Reference list of included studies and of relevant reviews were searched to identify other potentially eligible studies.
Two independent researchers (MK, MS) performed the study selection by first screening title and abstracts and then full texts using the Rayyan screening tool (30). Disagreements were first discussed between the two independent researchers. A third reviewer (ILS or GSP) was consulted if a disagreement could not be resolved. Percentage agreement on study inclusion between the two reviewers was calculated. There were no restrictions on language nor publication date.
Studies that met the following criteria were considered for inclusion: (1) Design: (randomised) controlled trials of animal studies; (2) Population: animals with experimentally-induced traumatic peripheral neuropathy; (3) Intervention: active exercise program performed prior to nerve injury; (4) Control: no active exercise. Studies which investigated hereditary neuropathies or neuropathies acquired by disease or toxins (e.g., animal models for diabetes, rheumatoid arthritis, Guillain-Barré syndrome, systemic lupus erythematosus, or chemotherapy-induced peripheral neuropathy), or diseases or injuries to the central nervous system (e.g., Parkinson’s disease, spinal cord injury) were excluded. Studies were also excluded if the exercise intervention was passive (such as neural mobilisations or stretching (31)), consisted of electrical stimulation, or if the exercise was part of a multimodal intervention. Vehicle injections or sham graft injections near the injury site were considered co-interventions and these studies were therefore excluded. The outcome measures included were neuro-immune responses (i.e., neuroimmune responses are defined as processes or substances (such as neuropeptides, cytokines, gene expression and hormones) involved in interactions between the immune system and nervous system.), other physiological reactions (e.g., muscle or nerve morphometrics or behavioural outcomes (e.g., mechanical allodynia).
Data were collected by two reviewers independently (MK, PT). Details regarding bibliographic information (author and year), study design, animal model (species, strain, sex, age and weight), neuropathy model, exercise as an intervention (type, program length, frequency, duration, intensity, and mode) and all types outcomes were extracted from each paper. Outcomes were categorised into primary, i.e., measures related to neuroimmune responses, or secondary outcomes, i.e., other physiological or behavioural outcomes.
For all neuro-immune responses and other outcome measures, continuous data and/or percentages were extracted as mean, standard deviation, and number of animals. If the standardised error of the mean (SEM) was provided, then these values were recalculated to standard deviations. Authors of selected articles were contacted if data could not be extracted directly from the article or if the outcome data was incomplete (e.g., unclear number of animals used). If authors did not respond after two attempts, a digital screen ruler (Universal Digitizer 3.8, AVPSoft.com) was used by two independent review authors (MK, PT) to extract data from the graphs.
The methodological quality of all included studies was evaluated by two independent reviewers (MK, PT) using the Systematic Review Center for Laboratory Animal Experimentation’s (SYRCLE) risk of bias tool for animal studies (32). The tool contains ten items related to selection bias, performance bias, detection bias, attrition bias, reporting bias and other bias. Each item is answered with ‘yes’ (i.e., low risk), ‘no’ (i.e., high risk), and ‘unclear’ (i.e., not enough information to determine the risk of bias). Discrepancies were first discussed between the two reviewers. If agreement could not be reached, a third reviewer (GSP) was consulted. Percentage agreement between the two reviewers was calculated.
The effects of pre-injury exercise on the primary outcome of neuroimmune responses and secondary outcomes of other physiological or behavioural outcomes were analysed using Comprehensive Meta-Analysis (CMA version 3). Standard mean differences (SMD) and 95% confidence intervals (95%CI) for each individual comparison were calculated with Hedges g correction (33). When group size was reported as a range (e.g., 8-10), the median value was used for meta-analysis. When a study contained multiple experimental groups, the control group size was corrected for the number of comparisons made (n/number of comparisons) (34, 35).
Meta-analyses in animal studies are more exploratory compared to those in human research, as direction of the effects is more meaningful than the size (35). In this review, meta-analyses were performed using a random effects model when a specific outcome was measured in at least two independent studies in the same anatomical location. Results from the meta-analyses were presented in forest plots and a pooled estimate was calculated. Neuroimmune outcomes measured in only one study, were also presented on a forest plot but without a pooled estimate. Vote counting was conducted for the neuroimmune outcomes by reporting the number of significant comparisons; results were cautiously interpreted. For repeated measurements at different time points within a study, the overall effect of pre-injury exercise was first presented using the largest recorded effect size, regardless of time, from each study. Statistical heterogeneity between studies was estimated by visual inspection and I2.
Pre-specified subgroup analyses included the comparison of species (e.g., rats versus mice), neuropathic pain model, exercise type (aerobic versus anaerobic). A post-hoc subgroup analysis was performed to explore the effect of timing of measurement post injury (week post injury). Subgroup analyses results were only interpreted if there were at least 10 comparisons per subgroup. To take into account the between study variation in the subgroup analyses, Comprehensive Meta-Analysis (CMA version 3) was used as it estimates the variation across all studies. To assess the publication bias, funnel plots and Trim and Fill analyses were planned for each outcome containing at least 10 studies (36). Lastly, to assess the robustness of the results, sensitivity analyses were planned to examine the effect of including only the low risk of bias compared to the complete analyses.
The flow of study selection is shown in Figure 1. The original search identified 17,431 records. After title and abstract screening, 128 full texts were assessed for eligibility, of which 11 articles originating from 10 experiments were included in the review (37–47). Reference lists of included studies and of relevant reviews (9, 48) did not reveal additional studies. Overall, agreement on study inclusion between the two reviewers before deliberation was 96.1%.
The eleven articles, including a total of 103 independent comparisons and 167 animals, were published between 1998 and 2022 (37–47). All but one study (42) used adult, male rodents; with Wistar rats being the most common species used (6/11 studies) (37, 39, 44–47), followed by Sprague-Dawley rats (3/11 studies) (40, 42, 43) and mice (2/11 studies) (38, 41) All neuropathic pain models were performed by injuring the sciatic nerve, seven included studies used a chronic constriction injury (CCI) model (37, 39–43, 46), while four studies used a nerve crush model (38, 44, 45, 47). The exercise programs administered before the lesion mostly consisted of aerobic exercise (9/11 studies), such as running (38, 40–43, 46) or swimming (37, 39, 47), but also included aquatic resistance training programs (44, 45). The primary outcome, neuroimmune responses, such as cytokines, neurotrophic factors, microglia, and antioxidants, were measured in six studies (38, 40–43, 46). However, one study (41) did not analyse neuroimmune responses in the non-exercise control group, thus was excluded in the analyses for neuroimmune responses. In regards to the secondary outcomes, five studies (37, 38, 40, 44, 45) assessed other physiological outcomes, such as muscle or nerve morphometric. Behavioural outcomes, such as mechanical allodynia measured with von Frey filaments or sciatic function index (SFI), were measured in nine studies (38, 39, 41–47). A summary of the basic characteristics of the eleven included studies is presented in Table 1. All measured outcomes are listed in Appendix B.
Figure 2 summarises the results of the risk of bias assessment for each study. Regarding five of the risk of bias items: random sequence generation, allocation sequence, random housing, random outcome assessment, and selective outcome reporting, all included studies showed an unclear risk of bias, because essential details regarding the methodology was not available. For the rest of the risk of bias items, the percentage marked as ‘unclear risk’ are as follows: baseline characteristics (64%), performance blinding (82%), blinding of outcome assessment (45%), and incomplete outcome data (73%). Low risk of bias was scored for all but one included study for the question, “Was the study apparently free of other problems that could result in high risk of bias? The high risk scored for this item pertained to excluding mice that refused to run. The percentage of agreement between the reviewers was 82%; differences between raters were mainly due to interpretation differences.
Figure 2 Risk of bias summary. Review authors’ judgement for each risk of bias item. The green ‘+’ means low risk, red ‘-’ means high risk, and yellow ‘?’ means unclear.
Five studies (92 rodents) evaluated 72 different neuroimmune responses measured at six different locations (spinal cord, cerebrospinal fluid, dorsal root ganglion, sciatic nerve, muscle and blood/serum) (38, 40, 42, 43, 46). Neuroimmune responses were measured on various days post-injury: 3,7,14, 15 or 26. When a neuroimmune response was measured in the same location in multiple comparisons, a meta-analysis was conducted. Meta-analysis on levels of IL-1β in the spinal cord ranging from 3-15 days post-injury showed that pre-injury exercise compared to non-exercise control significantly reduced levels of IL-1β (pooled data, 2 studies, 3 comparisons, n=40 rodents, SMD: -1.06, 95%CI: -1.99 to -0.13) (38, 43) (Figure 3A). Two other studies (42, 43) showed that pre-injury exercise did not significantly reduce levels of iNOS at the sciatic nerve compared to non-exercise control (pooled data, 2 studies, 4 comparisons, n=82 rodents, SMD: -0.71, 95%CI: -1.66 to 0.25) (Figure 3B). iNOS is an enzyme that produces nitric oxide under stress conditions and is a common marker for M1 macrophage activation (49–51). Due to the low power of these meta-analyses, no strong conclusions can be drawn.
Figure 3 The general effect of pre-injury exercise on various pro-inflammatory and anti-inflammatory neuroimmune outcomes in animal models of experimentally-induced traumatic peripheral neuropathy. (A) Meta-analysis of the effect of pre-injury exercise on IL-1β. (B) Meta-analysis of the effect of prior exercise on iNOS. For neuroimmune outcomes measured in the same study and location, but different timepoints, the day post injury is listed behind study identification in superscript.
Figures 4, 5 provide an overview of all reported neuroimmune responses, for pro-inflammatory (Figure 4) and anti-inflammatory (Figure 5) responses. This forest plot shows the direction of the effect of pre-injury exercise on neuroimmune responses per anatomical location following experimentally-induced traumatic peripheral neuropathy. Vote counting revealed that for the pro-inflammatory neuroimmune responses (Figure 4), pre-injury exercise significantly improved 24 of the 50 comparisons across the six different locations. Of the 22 comparisons evaluating anti-inflammatory neuroimmune responses, pre-injury exercise significantly improved 6 responses across the different locations (Figure 5).
Figure 4 Overview of all reported pro-inflammatory neuroimmune responses. Favours pre-injury exercise implies a reduction in pro-inflammatory neuroimmune markers. Outcomes are categorised by anatomical location. For neuroimmune outcomes measured in the same study and location, but different timepoints, the day post injury is listed behind study identification in superscript. p65, nuclear factor NF-kappa-B p65 subunit; IL-1β, interleukin 1 beta; p38 mitogen-activated protein kinase; P2X4R, P2X4 receptor; BDNF, brain-derived neurotrophic factor; TNF-α, tumor necrosis factor alpha; NLRP3 inflammasome; IL-6R, interleukin-6 receptor; NT, nitro tyrosine; ATF3, activating transcription factor 3; CCL2, chemokine (C-C motif) ligand 2, CD11b (macrophage marker); Iba1, ionized calcium binding adaptor molecule 1 (microglia activation marker); iNOS, inducible nitric oxide synthase; NOX2, NAPDH oxidase 2; HSP, heat shock protein; PBMC, peripheral blood mononuclear cells; CXCL1, chemokine (C-X-C motif) ligand 1.
Figure 5 Overview of all reported anti-inflammatory neuroimmune responses Favours pre-injury exercise implies an increase in anti-inflammatory neuroimmune markers. Outcomes are categorised by anatomical location. For neuroimmune outcomes measured in the same study and location, but different timepoints, the day post injury is listed behind study identification in superscript. GLT-1, glutamate transporter 1; IL-10, interleukin 10; Nrf2, nuclear factor E2-related factor 2 nuclear translocation; Nrf2nuc, nuclear factor E2-related factor 2 nuclear fractions; Arg-1, arginase 1; SOD, superoxide dismutase; HO, heme oxygenase; FRAP, ferric reducing antioxidant power; MDA, malondialdehyde.
Meta-analyses could be performed on two studies (20 rodents) which measured six nerve-related morphometrics (38, 44). Exercise before experimentally-induced traumatic peripheral neuropathy had a favourable effect on the sciatic nerve’s G-ratio (i.e., a measure of the functional and structural index of optimal axonal myelination (52), determined by the inner axon radius relative to the outer, myelinated, axon radius) compared to non-exercise control (pooled data, 2 studies, n=20 rodents, SMD: 1.95, 95%CI: 0.77 to 3.12) (Figure 6.1) (38, 44). Pre-injury exercise was not different than non-exercise control on other nerve-related morphometrics, such as myelin sheath thickness (pooled data, 2 studies, n=20, SMD: 1.94, 95%CI: –2.15 to 6.03), nerve fibre diameter (pooled data, 2 studies, n=20, SMD: 0.81, 95%CI: -0.83 to 2.45), axon diameter (pooled data, 2 studies, n=20, SMD: 0.74, 95%CI: -0.92 to 2.40), nerve fibre density (pooled data, 2 studies, n=20, SMD: 0.65, 95%CI: -0.81 to 2.12), or total number of nerve fibres (pooled data, 2 studies, n=20, SMD: 0.28, 95%CI: -0.87 to 1.43) (Figure 6.2-6) (38, 44).
Figure 6 The effect of pre-injury exercise on six different nerve morphometric outcomes following experimentally-induced traumatic peripheral neuropathy. Favours pre-injury exercise implies an increase in outcome values, except for G-ratio where a reduction in outcome is valued.
There were two muscle morphometric measures that were assessed by two studies (48 rodents). Muscle fibre cross-sectional area of the soleus (37, 45) and plantaris (40) muscle following peripheral neuropathy induction was significantly larger with pre-injury exercise (pooled data, 3 studies, 4 comparisons, n=48 rodents, SMD: 0.91, 95%CI: 0.27 to 1.54) compared to non-exercise control (Figure 7.1). Pre-injury exercise was not different than the non-exercise control in muscle fibre diameter (pooled data, 2 studies, n=28, SMD: 0.76, 95%CI: -0.07 to 1.60) (Figure 7.2) (37, 45).
Figure 7 The effect of pre-injury exercise on two outcomes related to muscle morphometrics following experimentally-induced traumatic peripheral neuropathy. Favours pre-injury exercise implies an increase in outcome values. Artifon et al., 2013 had two experimental groups: the progressive swimming program is labelled “prog” and the regular aerobic swimming is labelled “reg”.
Nine studies (124 rodents) evaluated behavioural outcomes after peripheral neuropathy induction (38, 39, 41–47). Meta-analyses could be performed on two outcomes: mechanical allodynia and SFI measured at the hind paw. Mechanical allodynia scores, measured with von Frey filaments, were significantly better in the pre-injury exercise group compared to and non-exercise control (pooled data, 6 studies, 7 interventions, n=114, SMD -1.24, 95%CI: -1.87 to -0.61) (Figure 8A) (38, 41–43, 45, 46)[38]. There was no difference however, between pre-injury exercise compared to non-exercise control in SFI scores (pooled data, 3 studies, n=36, SMD: 1.05, 95%CI: -1.06 to 3.17) (Figure 8B) (38, 44, 47).
Figure 8 The effect of pre-injury exercise on behavioural outcomes following experimentally-induced traumatic peripheral neuropathy. As both outcomes, mechanical allodynia and sciatic function index (SFI) were repeated measurements taken at multiple time points, only the largest effect per study are presented. Days post injury listed behind study identification in superscript. (A) The effect of prior exercise on mechanical allodynia. Favours pre-injury exercise implies an improvement in mechanical allodynia. Green et al., 2022 had two experimental groups: a short duration program of 3 weeks labelled “short” and a long duration program of 6 weeks labelled “long”. (B) The effect prior exercise on SFI. Favours pre-injury exercise implies an improvement in SFI scores.
Due to the limited number of studies, none of the planned subgroup analysis could be performed. A post-hoc subgroup analysis for mechanical allodynia was performed comparing mechanical allodynia measurements recorded per week post-injury. Comparing the subgroups of mechanical allodynia per week post-injury, pre-injury exercise reduced mechanical allodynia at week 2 but not significantly at week 1 post injury (Figure 9). Sub-groups of mechanical allodynia measured during week 3 and 4 had less than 10 comparisons but results can be found in Appendix C. These results suggests that the timing of outcome measurement may influence the effect of pre-injury exercise on mechanical allodynia. However, due to the low number of studies in each subgroup we have to interpret these findings carefully.
Figure 9 Post hoc subgroup analysis: The effect of pre-injury exercise on mechanical allodynia grouped by week post injury following experimentally-induced traumatic peripheral neuropathy. Negative values favour pre-injury exercise. The error bars represent the 95% confidence intervals. The results from subgroup analysis were only presented when subgroups contained data from at least 10 independent comparisons.
Due to the low number of studies measuring the same outcome, publication bias could not be investigated. Furthermore, proposed sensitivity analyses could not be performed as the risk of bias was unclear for the majority of the included studies in all risk of bias domains.
This systematic review summarises the results of 11 studies that examined the influence of pre-injury exercise on neuroimmune outcomes, physiological responses, and behavioural outcomes following experimentally-induced peripheral neuropathy in animal models. Most studies evaluated aerobic exercise interventions and used a sciatic nerve, chronic constriction injury (CCI) model. Seventy-two different neuroimmune outcomes (mostly pro-inflammatory) were measured in five included papers in different anatomical locations. Meta-analyses could only be conducted for IL-1β (spinal cord) and iNOS (sciatic nerve) and showed exercise prior to experimentally-induced traumatic neuropathy significantly reduced IL-1β levels but not iNOS. All the other neuroimmune outcomes were only measured once. Vote counting revealed a reduction in 23 pro-inflammatory neuroimmune outcomes (such as cytokines, chemokines, and macrophage/microglial markers) and an increase in 6 anti-inflammatory neuroimmune outcomes (such as transcriptional antioxidant response regulator, Nrf2, and glutamate transporter-1). Physiological responses, such as the G-ratio of the nerve and the muscle fibre cross-sectional area of the distally innervated muscle, were improved in the pre-injury exercise group compared to non-exercise control. Behavioural outcomes, such as mechanical allodynia improved in the pre-injury exercise group compared to non-exercise control. The post-hoc subgroup analysis for mechanical allodynia suggests that the timing of outcome measurement may influence the effect of pre-injury exercise on mechanical allodynia, but results from small subgroups. The results of this review suggest a potential neuroprotective and immunoregulatory effect of an exercise regime before sustaining a peripheral neuropathy.
Experimentally-induced traumatic peripheral neuropathy provokes an immune response that requires an intricate balance of pro-inflammatory and anti-inflammatory mechanisms (14, 53). Current evidence suggests that persistent neuropathic pain is the result of the balance being tipped in favour of pro-inflammatory mechanisms (14, 16). Regular exercise is known to induce a transient anti-inflammatory environment, locally within the tissues and systemically (22, 23, 54). In this review, most included studies focused on the reduction of pro-inflammatory responses, such as IL-1β or BDNF related transcription factors (e.g., p65, p38, and P2X4R) other cytokines and chemokines, macrophage/microglia markers (CD11b and Iba1), and neuronal damage factor ATF3. Meta-analyses could only be performed on 2 neuroimmune markers (spinal cord IL-1β and sciatic nerve iNOS). Meta-analysis on levels of IL-1β from two studies (n=40 rodents) showed that pre-injury aerobic exercise significantly reduced levels of IL-1β compared to non-exercise control. A potential explanation for the lack of significant results in the meta-analysis for iNOS could be a result of too few studies (n=2, n=41 rodents) leading to type II error. Pre-injury exercise promoted anti-inflammatory responses including elevated levels of GLT-1 and transcriptional antioxidant response regulator, Nrf2. Activation of Nfr2 seems to be a promising mechanism as it attenuated CCI-induced neuropathic pain via induction of PGC-1α-mediated mitochondrial biogenesis in the spinal cord (55). Additionally, Nrf2 activation reduces oxidative stress and neuroinflammation leading to a reduction in pain and delays the onset of pain in various animal models (56). Thus, pre-injury exercise may influence different neuroimmune signals on both sides of the inflammatory balance to prevent the sustained hyperinflammation as seen in non-exercise animals.
This review also demonstrates that exercise exerts its effects not only locally near the injury site (sciatic nerve), but also in the dorsal root ganglia and spinal cord. Unfortunately, the effect of pre-injury exercise on the brain was not evaluated in the included studies, despite evidence supporting benefits of exercise on the brain, astrocyte function, and specific regions important in pain modulation (24, 57, 58). Then again, the activation of microglia in the dorsal horn of the spinal cord is a critical contributor to the initiation and maintenance of neuropathic pain (11, 17, 59–62). Therefore, it is encouraging that in this review, pre-injury exercise influences several mechanisms in the peripheral and central nervous system involved in the development and repercussions of neuropathic pain.
Unexpectedly, no overall effect was seen for the functional behavioural outcome, SFI. While for the behavioural outcome of mechanical allodynia, the pre-injury exercise group had significantly less sensitivity to touch compared to the non-exercise control. Typically, mechanical allodynia in rodents occurs 3-7 days after CCI and lasts to about 35 days (63–65). In a post hoc subgroup analysis, mechanical allodynia results were grouped by week post injury. The results provide some insight on the sequela of peripheral nerve injury and shows that there might be a time effect but coming from very small groups. By grouping the repeated measures at different time points by time after injury in the post-hoc analyses, the pre-injury exercise group had significantly less mechanical allodynia at week 2, but not week 1. Interestingly, this is the same time period when most neuroimmune outcomes reported in this review were measured. This shared timing is most likely not a coincidence, as the severity of mechanical allodynia was recently correlated with the level of dorsal root ganglion inflammation (65). More research needs to be undertaken to understand the lasting effects of pre-injury exercise, whether its effect also lies in this time window of 1-2 weeks post neuropathic pain induction.
When interpreting the findings of this systematic review and meta-analyses, several limitations should be considered. The first and foremost is that the number of studies that could be analysed per outcome was very low. Thus, increasing the likelihood of imprecise effect estimates which influences the robustness and reliability of the conclusions that can be drawn from this systematic review. Much more research on the neuroimmune responses and neuroprotective effects of pre-injury exercise before the occurrence of traumatic peripheral neuropathy is urgently needed. In addition, the heterogeneity between the studies was large. Studies differed for example, in species used, exercise program duration, and exercise intensity. To account for anticipated heterogeneity, we used a random effects model and explored the suggested causes for between study heterogeneity by means of subgroup analysis.
Thirdly, risk of bias analysis revealed poor reporting of essential details related to the design and conduct of the included experiments. Consequently, in a majority of the studies, risk of bias could not be estimated. The lack of reporting important methodological details raises concerns about bias in the data and skewed results thus, hampering the ability to draw reliable conclusions from the included animal studies. Future preclinical studies should follow ARRIVE guidelines to ensure better transparency in their methodology and reporting (66, 67). The Enhancing Quality in Preclinical Data (EQIPD) framework may also be a tool to help pre-clinical researchers to decide which guidelines to follow (67).
Fourthly, in preclinical pain research the indirectness of the results should be considered (68) – in particular the indirectness of the animal models of neuropathic pain and the outcome measures of pain. The traumatic peripheral neuropathy models (sciatic CCI and nerve crush) used by included studies in this review have the ability to represent some certain neuropathic conditions in humans (e.g., sciatic, radiculopathy, chronic low back pain, and complex regional pain syndrome type II) (69). In terms of the development of pain-related hypersensitivity and underlying pathogenesis, these two models share many features (70). However, some dissimilarities may exist (e.g., different inflammatory reaction severity (70)). Outcomes measured in these neuropathic models such as, biological markers and behavioural tests, are indirect measures of human pain (71). Therefore, translation of the results to the clinical setting is not appropriate. Additionally, the results of this review cannot be extrapolated to female animals as all included studies used male rodents, except one (42). Sex differences are becoming more apparent in neuroimmune responses (72), biological mechanisms of pain maintenance after nerve injury (73), and even in the effectiveness of exercise dependent on the type (74–76). Thus, despite the fact that sex differences in pain sensitivity have been well established for decades (77, 78), the translatability of the results within the preclinical field are limited.
The aforementioned limitations make it difficult to draw conclusions on the mechanisms underlying the effect of pre-injury exercise on recovery following experimentally-induced traumatic peripheral neuropathy. However, this review does provide an overview of the neuroimmune outcomes/mechanisms that have been measured to date. Future research may use this as a guide in selecting neuroimmune targets. Researchers should also keep in mind other factors that could influence neuroimmune responses, for instance forced treadmill and swimming may increase stress biomarkers compared to voluntary exercise (24, 79). The subgroup analyses provide some insight for preclinical researchers analysing mechanical allodynia to be mindful of (or further investigate) the type of animal model used, the intensity of the exercise, the exercise program duration, and the timing of outcome assessment during the recovery process. Similar factors were also suggested in a brief narrative review on the overall effects of exercise on chronic pain (80). More specifically, voluntary exercise paradigms, exercise program duration, and the chronic pain animal model possibly contributed to the effectiveness of pre-injury exercise in rodent models of chronic pain (80).
This is the first review to focus on the effect of pre-injury exercise on recovery in terms of neuroimmune outcomes, physiological response, and behavioural outcomes following experimentally-induced traumatic peripheral neuropathy in animals. The findings in this systematic review suggest a potential neuroprotective and immunoregulatory effect of pre-injury exercise for the recovery after peripheral induced neuropathic pain. However, as most findings were based on single studies, more research is needed to increase the certainty of evidence. Future research could focus on a pro-inflammatory state resulting in a prolonged, exaggerated pain state and susceptibility to chronic pain being the consequence of non-exercise behaviour. On top of the many benefits, regular exercise seems to promote a normal healing process following experimentally-induced traumatic peripheral neuropathy.
All authors contributed substantially to this systematic review, and are listed alphabetically: Conceptualisation, GS-P, MC, MK, and MS-K; methodology, CH, GS-P, IL, MC, MK, and MS-K; data curation CH, GS-P, MK, MS-K, and PT; writing—original draft preparation, MK; writing—review and editing, CH, GS-P, IL, MC, MK, MS-K, and PT; visualisation, CH, GS-P, MC, and MK; supervision, GS-P and MC; project administration, GS-P and MC; funding acquisition, GS-P. All authors contributed to the article and approved the submitted version.
This research and APC was funded by the ZonMw grant Synthesis of Evidence in practice, within the program More Knowledge with Fewer Animals, project number: 114024128.
The authors thank Alice Tillema from the Medical Library of Radboud University Medical Center for her assistance with the literature searches.
The authors declare that the research was conducted in the absence of any commercial or financial relationships that could be construed as a potential conflict of interest.
All claims expressed in this article are solely those of the authors and do not necessarily represent those of their affiliated organizations, or those of the publisher, the editors and the reviewers. Any product that may be evaluated in this article, or claim that may be made by its manufacturer, is not guaranteed or endorsed by the publisher.
The Supplementary Material for this article can be found online at: https://www.frontiersin.org/articles/10.3389/fimmu.2023.1215566/full#supplementary-material
1. Landmark T, Romundstad PR, Borchgrevink PC, Kaasa S, Dale O. Longitudinal associations between exercise and pain in the general population-The HUNT pain study. PloS One (2013) 8:e65279. doi: 10.1371/journal.pone.0065279
2. Lauretta G, Ravalli S, Maugeri G, D’Agata V, Di Rosa M, Musumeci G. The impact of physical exercise on the hippocampus in physiological condition and ageing-related decline: current evidence from animal and human studies. Curr Pharm Biotechnol (2022) 23:180–9. doi: 10.2174/1389201022666210405142611
3. Mcleod JC, Stokes T, Phillips SM. Resistance exercise training as a primary countermeasure to age-related chronic disease. Front Physiol (2019) 1:645. doi: 10.3389/fphys.2019.00645
4. Anderson E, Durstine JL. Physical activity, exercise, and chronic diseases: A brief review. Sports Med Health Sci (2019) 1:3–10. doi: 10.1016/j.smhs.2019.08.006
5. Pedersen BK, Saltin B. Exercise as medicine-evidence for prescribing exercise as therapy in 26 different chronic diseases. Scand J Med Sci Sports (2015) 25:1–72. doi: 10.1111/sms.12581
6. Zhang YH, Hu HY, Xiong YC, Peng C, Hu L, Kong YZ, et al. Exercise for neuropathic pain: A systematic review and expert consensus. Front Med (Lausanne) (2021) 8:756940/FULL. doi: 10.3389/FMED.2021.756940/FULL
7. Leitzelar BN, Koltyn KF. Exercise and neuropathic pain: A general overview of preclinical and clinical research. Sports Med (2021) 7:2021. doi: 10.1186/s40798-021-00307-9
8. Matesanz-García L, Schmid AB, Cáceres-Pajuelo JE, Cuenca-Martínez F, Arribas-ROmano A, González-Zamorano Y, et al. Effect of physiotherapeutic interventions on biomarkers of neuropathic pain: A systematic review of preclinical literature. J Pain (2022) 23:P1833–1855. doi: 10.1016/j.jpain.2022.06.007
9. Guo J, Chen B, Wang Y, Zhu Y, Song G, Yang Z, et al. Meta-analysis of the effect of exercise on neuropathic pain induced by peripheral nerve injury in rat models. Front Neurol (2019) 10:636. doi: 10.3389/fneur.2019.00636
10. Hore Z, Denk F. Neuroimmune interactions in chronic pain – An interdisciplinary perspective. Brain Behav Immun (2019) 79:56–62. doi: 10.1016/j.bbi.2019.04.033
11. Schmid AB, Coppieters MW, Ruitenberg MJ, Mclachlan EM. Local and remote immune-mediated inflammation after mild peripheral nerve compression in rats (2013). Available at: https://academic.oup.com/jnen/article/72/7/662/2917606.
12. Finnerup NB, Kuner R, Jensen TS. Neuropathic pain: From mechanisms to treatment. Physiol Rev (2021) 101:259–301. doi: 10.1152/physrev.00045.2019
13. Kim CF, Moalem-Taylor G. Detailed characterization of neuro-immune responses following neuropathic injury in mice. Brain Res (2011) 1405:95–108. doi: 10.1016/j.brainres.2011.06.022
14. Austin PJ, Moalem-Taylor G. The neuro-immune balance in neuropathic pain: Involvement of inflammatory immune cells, immune-like glial cells and cytokines. J Neuroimmunol (2010) 229:26–50. doi: 10.1016/j.jneuroim.2010.08.013
15. Parisien M, Lima LV, Dagostino C, El-Hachem N, Drury GL, Grant AV, et al. Acute inflammatory response via neutrophil activation protects against the development of chronic pain. Sci Transl Med (2022) 14:eabj9954. doi: 10.1126/SCITRANSLMED.ABJ9954
16. Ellis A, Bennett DLH. Neuroinflammation and the generation of neuropathic pain. Br J Anaesth (2013) 111:26–37. doi: 10.1093/bja/aet128
17. Yu X, Liu H, Hamel KA, Morvan MG, Yu S, Leff J, et al. Dorsal root ganglion macrophages contribute to both the initiation and persistence of neuropathic pain. Nat Commun (2020) 11:1–12. doi: 10.1038/s41467-019-13839-2
18. Bethea JR, Fischer R. Role of peripheral immune cells for development and recovery of chronic pain. Front Immunol (2021) 12:641588. doi: 10.3389/fimmu.2021.641588
19. Clark AK, Old EA, Malcangio M. Neuropathic pain and cytokines: current perspectives. J Pain Res (2013) 2013, 803–814. doi: 10.2147/JPR.S53660
20. Bobinski F, Teixeira JM, Sluka KA, Roberto A, Santos S. IL-4 mediates the analgesia produced by low-intensity exercise in mice with neuropathic pain. Pain (2018) 159:437–50. doi: 10.1097/j.pain.0000000000001109
21. Sluka KA, Frey-Law L, Hoeger Bement M. Exercise-induced pain and analgesia? Underlying mechanisms and clinical translation. Pain (2018) 159:S91–7. doi: 10.1097/j.pain.0000000000001235
22. Gleeson M, Bishop NC, Stensel DJ, Lindley MR, Mastana SS, Nimmo MA. The anti-inflammatory effects of exercise: Mechanisms and implications for the prevention and treatment of disease. Nat Rev Immunol (2011) 11:607–10. doi: 10.1038/nri3041
23. Petersen AMW, Pedersen BK. The anti-inflammatory effect of exercise. J Appl Physiol (2005) 98:1154–62. doi: 10.1152/japplphysiol.00164.2004
24. Lesnak JB, Sluka KA. Mechanism of exercise-induced analgesia: what we can learn from physically active animals. Pain Rep (2020) 5:1–16. doi: 10.1097/PR9.0000000000000850
25. Kistner TM, Pedersen BK, Lieberman DE. Interleukin 6 as an energy allocator in muscle tissue. Nat Metab | (2022) 4:170–9. doi: 10.1038/s42255-022-00538-4
26. Pedersen BK, Febbraio MA. Muscle as an Endocrine Organ: Focus on Muscle-Derived Interleukin-6. Physiol Rev (2008) 88:1379–406. doi: 10.1152/physrev.90100.2007
27. Leung A, Gregory NS, Allen L-AH, Sluka KA. Regular physical activity prevents chronic pain by altering resident muscle macrophage phenotype and increasing IL-10 in mice. Pain (2016) 157:70–9. doi: 10.1097/j.pain.0000000000000312
28. Sluka KA, O’Donnell JM, Danielson J, Rasmussen LA. Regular physical activity prevents development of chronic pain and activation of central neurons. J Appl Physiol (2013) 114:725–33. doi: 10.1152/japplphysiol.01317.2012
29. Page MJ, Mckenzie JE, Bossuyt PM, Boutron I, Hoffmann TC, Mulrow CD, et al. The PRISMA 2020 statement: an updated guideline for reporting systematic reviews. BMJ (2021) 372:n71. doi: 10.1136/bmj.n71
30. Ouzzani M, Hammady H, Fedorowicz Z, Elmagarmid A. Rayyan-a web and mobile app for systematic reviews. Syst Rev (2016) 5:1–10. doi: 10.1186/s13643-016-0384-4
31. Schipholt IJL, Coppieters MW, Meijer OG, Tompra N, De Vries RBM, Scholten-Peeters GGM. Effects of joint and nerve mobilisation on neuroimmune responses in animals and humans with neuromusculoskeletal conditions: a systematic review and meta-analysis. Pain Rep (2021) 9:e927. doi: 10.1097/PR9.0000000000000927
32. Hooijmans CR, Rovers MM, de Vries RB, Leenaars M, Ritskes-Hoitinga M, Langendam MW. SYRCLE’s risk of bias tool for animal studies. BMC Med Res Methodol (2014) 14:43. doi: 10.1186/1471-2288-14-43
34. Hooijmans CR, Draper D, Ergün M, Scheffer GJ. The effect of analgesics on stimulus evoked pain-like behaviour in animal models for chemotherapy induced peripheral neuropathy- a meta-analysis. Sci Rep (2019) 9:1–15. doi: 10.1038/s41598-019-54152-8
35. Hooijmans CR, IntHout J, Ritskes-Hoitinga M, Rovers MM. Meta-analyses of animal studies: An introduction of a valuable instrument to further improve healthcare. ILAR J (2014) 55:418–26. doi: 10.1093/ilar/ilu042
36. Higgins J, Thomas J, Chandler J, Cumpston M, Li T, Page M, et al. Cochrane Handbook for Systematic Reviews of Interventions version 6.2, in: Cochrane (2021). Available at: www.training.cochrane.org/handbook (Accessed February 8, 2022).
37. Artifon EL, Silva LI, de Fátima Chasko Ribeiro L, Meire Costa Brancalhão R, Bertolini GRF. Aerobic training previous to nerve compression: Morphometry analysis of muscle in rats. Rev Bras Med Esporte (2013) 19:66–9. doi: 10.1590/S1517-86922013000100014
38. Bobinski F, Martins DF, Bratti T, Mazzardo-Martins L, Winkelmann-Duarte EC, Guglielmo LGA, et al. Neuroprotective and neuroregenerative effects of low-intensity aerobic exercise on sciatic nerve crush injury in mice. Neuroscience (2011) 194:337–48. doi: 10.1016/j.neuroscience.2011.07.075
39. Bertolini GFR, do Nascimento CM, Artifon EL, Cuhna DM, Meireles A. Swimming training and functional assessment of sciatic nociception in rats. Rev Bras Reumatol (2011) 51:249–59.
40. Campos JC, Baehr LM, Gomes KMS, Bechara LRG, Voltarelli VA, Bozi LHM, et al. Exercise prevents impaired autophagy and proteostasis in a model of neurogenic myopathy. Sci Rep (2018) 8:1–14. doi: 10.1038/s41598-018-30365-1
41. Cobianchi S, Marinelli S, Florenzano F, Pavone F, Luvisetto S. Short- but not long-lasting treadmill running reduces allodynia and improves functional recovery after peripheral nerve injury. Neuroscience (2010) 168:273–87. doi: 10.1016/j.neuroscience.2010.03.035
42. Green-Fulgham SM, Harland ME, Ball JB, Li J, Lacagnina MJ, D’Angelo H, et al. Preconditioning by voluntary wheel running attenuates later neuropathic pain via Nrf2 antioxidant signaling in rats. Pain (2022) 163:1939–51. doi: 10.1097/j.pain.0000000000002589
43. Grace PM, Fabisiak TJ, Green-Fulgham SM, Anderson ND, Strand KA, Kwilasz AJ, et al. Prior voluntary wheel running attenuates neuropathic pain. Pain (2016) 157:2012–23. doi: 10.1097/j.pain.0000000000000607
44. Kakihata CMM, Malanotte JA, Karvat J, Brancalhão RMC, Ribeiro L de FC, Bertolini GRF. The morphological and functional effects of exercise in the aquatic environment, performed before and/or after sciatic nerve compression in Wistar rats. J Exerc Rehabil (2016) 12:393–400. doi: 10.12965/jer.1632670.335
45. Malanotte JA, Kakihata CMM, Karvat J, Brancalhão RMC, Ribeiro L de FC, Bertolini GRF. Jumping in aquatic environment after sciatic nerve compression: nociceptive evaluation and morphological characteristics of the soleus muscle of Wistar rats. Einstein (Sao Paulo) (2017) 15:77–84. doi: 10.1590/S1679-45082017AO3613
46. Safakhah HA, Kor NM, Bazargani A, Bandegi AR, Pourbadie HG, Khoshkholgh-Sima B, et al. Forced exercise attenuates neuropathic pain in chronic constriction injury of male rat: An investigation of oxidative stress and inflammation. J Pain Res (2017) 10:1457–66. doi: 10.2147/JPR.S135081
47. van Meeteren NLU, Brakkee JH, Helders PJM, Gispen WH. The effect of exercise training on functional recovery after sciatic nerve crush in the rat. J Peripheral Nervous System (1998) 3:277–82.
48. Armada-da-Silva PAS, Pereira C, Amado S, Veloso AP. Role of physical exercise for improving posttraumatic nerve regeneration. Int Rev Neurobiol (2013) 109:125–49. doi: 10.1016/B978-0-12-420045-6.00006-7
49. Anavi S, Tirosh O. iNOS as a metabolic enzyme under stress conditions. Free Radic Biol Med (2020) 146:16–35. doi: 10.1016/j.freeradbiomed.2019.10.411
50. Zigmond RE, Echevarria FD. Macrophage biology in the peripheral nervous system after injury. Prog Neurobiol (2019) 173:102–21. doi: 10.1016/j.pneurobio.2018.12.001
51. Naik AK, Tandan SK, Kumar D, Dudhgaonkar SP. Nitric oxide and its modulators in chronic constriction injury-induced neuropathic pain in rats. Eur J Pharmacol (2006) 530:59–69. doi: 10.1016/j.ejphar.2005.11.029
52. Chomiak T, Hu B. What is the optimal value of the g-ratio for myelinated fibers in the rat CNS? A theoretical approach. PloS One (2009) 4:e7754. doi: 10.1371/JOURNAL.PONE.0007754
53. Meacham K, Shepherd A, Mohapatra DP, Haroutounian S. Neuropathic pain: Central vs. peripheral mechanisms. Curr Pain Headache Rep (2017) 21:28. doi: 10.1007/s11916-017-0629-5
54. Svensson M, Lexell J, Deierborg T. Effects of physical exercise on neuroinflammation, neuroplasticity, neurodegeneration, and behavior. Neurorehabil Neural Repair (2015) 29:577–89. doi: 10.1177/1545968314562108
55. Sun J, Li J-Y, Zhang L-Q, Li D-Y, Wu J-Y, Gao S-J, Liu D-Q, Zhou Y-Q. Nrf2 activation attenuates chronic constriction injury-induced neuropathic pain via induction of PGC-1α-mediated mitochondrial biogenesis in the spinal cord. Oxid Med Cell Longev (2021) 2021:9577874. doi: 10.1155/2021/9577874
56. Hendrix J, Nijs J, Ickmans K, Godderis L, Ghosh M, Polli A. The Interplay between oxidative stress, exercise, and pain in health and disease: Potential role of autonomic regulation and epigenetic mechanisms. Antioxidants (Basel) (2020) 9:1166. doi: 10.3390/antiox9111166
57. Cooper MA, Kluding PM, Wright DE. Emerging relationships between exercise, sensory nerves, and neuropathic pain. Front Neurosci (2016) 10:372. doi: 10.3389/fnins.2016.00372
58. Maugeri G, D’Agata V, Trovato B, Roggio F, Castorina A, Vecchio M, et al. The role of exercise on peripheral nerve regeneration: from animal model to clinical application. Heliyon (2021) 7:e08281. doi: 10.1016/j.heliyon.2021.e08281
59. Old EA, Clark AK, Malcangio M. “The role of glia in the spinal cord in neuropathic and inflammatory pain”. Handbook of Experimental Pharmacology. (Berlin, Heidelberg: Springer) (2015) p. 145–170. doi: 10.1007/978-3-662-46450-2_8
60. Dobson JL, Mcmillan J, Li L. Benefits of exercise intervention in reducing neuropathic pain. Front Cell Neurosci (2014) 8:102. doi: 10.3389/fncel.2014.00102
61. Wen YR, Tan PH, Cheng JK, Liu YC, Ji RR. Microglia: A promising target for treating neuropathic and postoperative pain, and morphine tolerance. J Formosan Med Assoc (2011) 110:487–94. doi: 10.1016/S0929-6646(11)60074-0
62. Scholz J, Woolf CJ. The neuropathic pain triad: Neurons, immune cells and glia. Nat Neurosci (2007) 10:1361–8. doi: 10.1038/nn1992
63. Bridges D, Thompson SWN, Rice ASC. Mechanisms of neuropathic pain. Br J Anaesth (2001) 87:12–26. doi: 10.1093/bja/87.1.12
64. Cobos EJ, Nickerson CA, Gao F, Chandran V, Bravo-Caparrós I, González-Cano R, et al. Mechanistic differences in neuropathic pain modalities revealed by correlating behavior with global expression profiling. Cell Rep (2018) 22:1301–12. doi: 10.1016/j.celrep.2018.01.006
65. chul NM, Mikler B, Joy T, Smith PA. Time course of inflammation in dorsal root ganglia correlates with differential reversibility of mechanical allodynia. Neuroscience (2020) 428:199–216. doi: 10.1016/j.neuroscience.2019.12.040
66. Kilkenny C, Browne WJ, Cuthill IC, Emerson M, Altman DG. The ARRIVE guidelines Animal Research: Reporting In Vivo Experiments. Br J Pharmacol (2010) 160(7), 1577–1579. https://doi.org/10.1111/j.1476-5381.2010.00872.x
67. Vollert J, Macleod M, Dirnagl U, Kas MJ, Michel MC, Potschka H, et al. The EQIPD framework for rigor in the design, conduct, analysis and documentation of animal experiments. Nat Methods (2022) 19:1334–1337. doi: 10.1038/s41592-022-01615-y
68. Wei D, Tang K, Wang Q, Estill J, Yao L, Wang X, et al. The use of GRADE approach in systematic reviews of animal studies. J Evid Based Med (2016) 9:98–104. doi: 10.1111/JEBM.12198
69. Abboud C, Duveau A, Bouali-Benazzouz R, Massé K, Mattar J, Brochoire L, et al. Animal models of pain: Diversity and benefits. J Neurosci Methods (2021) 348:108997. doi: 10.1016/j.jneumeth.2020.108997
70. Calvo M, Bennett DLH. The mechanisms of microgliosis and pain following peripheral nerve injury. Exp Neurol (2012) 234:271–82. doi: 10.1016/j.expneurol.2011.08.018
71. Yezierski RP, Hansson P. Inflammatory and neuropathic pain from bench to bedside: What went wrong? J Pain (2018) 19:571–88. doi: 10.1016/j.jpain.2017.12.261
72. Lasselin J, Lekander M, Axelsson J, Karshikoff B. Sex differences in how inflammation affects behavior: What we can learn from experimental inflammatory models in humans. Front Neuroendocrinol (2018) 50:91–106. doi: 10.1016/j.yfrne.2018.06.005
73. Muralidharan A, Ribeiro-da-silva A, Mogil JS, Muralidharan A, Sotocinal SG, Yousefpour N, Akkurt N, Lima L V, Tansley S, Parisien M, et al. Long-term male-specific chronic pain via telomere- and p53 - mediated spinal cord cellular senescence. J Clin Invest (2022) 132:e151817. doi: 10.1172/JCI151817
74. Wood K, Wilhelm J, Sabatier M, Liu K, Gu J, English AW. Sex differences in the effectiveness of treadmill training in enhancing axon regeneration in injured peripheral nerves. Dev Neurobiol (2012) 72:688–98. doi: 10.1002/dneu.20960.Sex
75. Liu C, Ward PJ, English AW. The effects of exercise on synaptic stripping require androgen receptor signaling. PloS One (2014) 9:1–8. doi: 10.1371/journal.pone.0098633
76. Lesnak JB, Fahrion A, Helton A, Rasmussen L, Andrew M, Cunard S, et al. Resistance training protects against muscle pain through activation of androgen receptors in male and female mice. Pain (2022) 163:1879–1891. doi: 10.1097/j.pain.0000000000002638
77. Craft RM, Mogil JS, Aloisi AM. Sex differences in pain and analgesia: the role of gonadal hormones. European Journal of Pain (2004) 8:397–411. doi: 10.1016/j.ejpain.2004.01.003
78. Rosen S, Ham B, Mogil JS. Sex differences in neuroimmunity and pain. J Neurosci Res (2017) 95:500–8. doi: 10.1002/jnr.23831
79. Contarteze RVL, ManChado FDB, Gobatto CA, De Mello MAR. Stress biomarkers in rats submitted to swimming and treadmill running exercises. Comp Biochem Physiol - A Mol Integr Physiol (2008) 151:415–22. doi: 10.1016/j.cbpa.2007.03.005
Keywords: running, prevention, peripheral nerve injury, inflammation, animal model
Citation: Koop MA, Sleijser-Koehorst MLS, Hooijmans CR, Tdlohreg PQ, Lutke Schipholt IJ, Scholten-Peeters GGM and Coppieters MW (2023) The potential protective effects of pre-injury exercise on neuroimmune responses following experimentally-induced traumatic neuropathy: a systematic review with meta-analysis. Front. Immunol. 14:1215566. doi: 10.3389/fimmu.2023.1215566
Received: 02 May 2023; Accepted: 22 August 2023;
Published: 11 September 2023.
Edited by:
Javier Aguilera-Lizarraga, University of Cambridge, United KingdomReviewed by:
Alfredo Ribeiro-da-Silva, McGill University, CanadaCopyright © 2023 Koop, Sleijser-Koehorst, Hooijmans, Tdlohreg, Lutke Schipholt, Scholten-Peeters and Coppieters. This is an open-access article distributed under the terms of the Creative Commons Attribution License (CC BY). The use, distribution or reproduction in other forums is permitted, provided the original author(s) and the copyright owner(s) are credited and that the original publication in this journal is cited, in accordance with accepted academic practice. No use, distribution or reproduction is permitted which does not comply with these terms.
*Correspondence: Michel W. Coppieters, bS5jb3BwaWV0ZXJzQGdyaWZmaXRoLmVkdS5hdQ==
Disclaimer: All claims expressed in this article are solely those of the authors and do not necessarily represent those of their affiliated organizations, or those of the publisher, the editors and the reviewers. Any product that may be evaluated in this article or claim that may be made by its manufacturer is not guaranteed or endorsed by the publisher.
Research integrity at Frontiers
Learn more about the work of our research integrity team to safeguard the quality of each article we publish.