- Department of Hematology, The Second Hospital of Dalian Medical University, Dalian, China
At the turn of the century, researchers discovered a unique subtype of T helper cells that secretes IL-17 and defined it as Th17. The latest study found that Th17 cells play both positive and negative definitive roles in the regulation of antitumor immune responses. Although the function of Th17 in the tumor microenvironment remains poorly understood, more and more studies have shown that this paradoxical dual role is closely related to the plasticity of Th17 cells in recent decades. Further understanding of the characteristics of Th17 cells in the tumor microenvironment could yield novel and useful therapeutic approaches to treat cancer. In this review, we further present the high plasticity of Th17 cells and the function of Th17-producing IL-17 in tumor immunity.
1 Introduction
In terms of tumor immunity, CD8+ T cells, including CTL, directly kill cancer cells and impair tumor growth, while CD4+ T cells act mainly by stimulating the function of other immune cells (1–3). Naïve CD4+ T cells(Th0) exposed to distinctly specific cytokine surroundings have the potential ability to proliferate and effectively differentiate into a variety of epigenetic states, such as Th17, Th1, Treg, Th9, and T follicular helper cells (4–6), each with a distinct function and an individual cytokine profile. In the early 21st century, Park H, Laurie E Harrington, and Annunziato et al. discovered the unique existence of an IL-17-producing CD4+ T cell subtype which we now term Th17 cells. This is the beginning of the research on Th17 cells (7–9). Subsequently, Th17 can be converted into other different subpopulations, mainly Th1 and Treg cells (10–12), but also into Th2 and Tfh under different conditions (13). To a large extent, Th17 cells demonstrate a considerable degree of context-dependent plasticity compared to Th1 and Th2 cells which have more phenotypic stability from the Muranski P’s research findings (11). The observed plasticity of Th17 cells is asymmetric and it occurs only in the direction of Th17 to Th1, as Th1 cells cannot easily convert to Th17 cells (11). It was previously thought that Th17 cells can play an autoimmune suppressive function by secreting IL-17 to induce angiogenesis to promote tumor growth (14). And the follow-up studies demonstrated that Th17 cells can be transdifferentiated into the Treg phenotype to exert a tumor-promoting role relying on this flexibility as well (15–17). By summarizing the studies of previous scholars, we found that this high degree of plasticity is also imperative for the antitumor activity defined for Th17 cells in the development of autoimmunity because Th17 cells can even directly convert to the Th1 phenotype and produce IFN-γ to exert anti-tumor effects. To date, there is a growing body of research indicating that Th17 cells influence the prognosis of cancer patients through their high plasticity and the secretion of inflammatory cytokines like IL-17. In this review, we briefly outline the differentiation and developmental pathways of Th17, Th1, and Treg cells, focus on our existing understanding of the implications of Th17 cell plasticity and functions, and finally summarize the specific mechanisms by which th17 functions in the tumor microenvironment.
2 The differentiation process of CD4+T cells linked to Th17 cell plasticity
CD4+ T cells are both essential regulators of antitumor immunity and critical protectors against invasion by external pathogens. Accumulating evidence currently elucidates the importance of the CD4+ T cell and their polarization status recognizing tumor antigens and resisting tumor cell proliferation in response to cancer immunotherapy (18). Luckheeram RV and colleagues put forward that, after antigen stimulation, differentiation of the naive precursors is initiated due to the interaction of TCR and CD4 as co-receptor with antigen-MHC II complexes presented by professional antigen-presenting cells (APCs) (19, 20). And continued research determined that the Naive CD4+T cells are activated when the TCR recognizes a specific peptide on MHC-II expressed by APCs (21, 22). The flexible differentiation options of Th0 into an individual subpopulation of helper T cells depends on cytokines secreted by APCs and other precursor cells, which induce a network of downstream signaling pathways that lead to cells’ initial activation, proliferation, and eventual differentiation of naive cells into specific effector cells. Different T cell populations that have been characterized, are tightly involved in several cancers and inflammatory diseases (23–25). In the paragraphs below we will characterize the specific differentiation process of naïve CD4+T cells to Th17, Th1, or Treg cells in detail.
2.1 Th17
As a distinct inflammatory lineage of CD4+ helper T cells, Th17 cells are defined by high levels of IL-17 secretion and secrete IL-17A, IL-17F, IL-21, IL-22, and CCL20 (26–29). Th17 cells are dependent on co-stimulation of CD28 and ICOS and are characterized by a master transcription regulator, the orphan nuclear receptor RORγT, which directs the transcription of the genes encoding IL-17 (27, 30, 31). Similarly, the other transcription factor, STAT3, binds to most of the relevant genes playing a crucial role in the differentiation of CD4+ T cells into Th17 cells (32–34).
Joshua et al. showed that individuals with Job’s syndrome (HIES) with definite mutations in STAT3 often fail to express the adequate levels of RORγT and tend to have a concomitant deficiency of Th17 cells, emphasizing the close association between STAT3 and Th17 cells (32, 35–37).
Th17 differentiation is highly dependent on the contribution of the cytokines TGF-β, IL-6, IL-21, and IL-1β (38–42), with subsequent long-term maintenance of the distinct Th17 lineage phenotype in the presence of IL-23 (43–45). In 2020, researchers find that TBK1 produced by intestinal epithelial cells blocked Th17 cell differentiation related to the regulation of IL-1β production, thereby preventing inflammation and tumorigenesis (46). IL-6, IL-21, and IL-23 all activate Stat3 and IRF4-dependent expression of RORγt specifically, which is crucial for Th17 cell differentiation as previously mentioned (47–52). Interestingly, TGF-β acts to upregulate IL-23R expression and confers responsiveness to IL-23 in vivo (39). Several earlier studies denied the large role of TGF-β in human Th17 cell differentiation: in a 2007 study, Sallusto stated that TGF-β even inhibited Th17 cell polarization (53). However, in 2008, experiments with serum-free cultured human neonatal CD4 T cells showed that exogenous addition of TGF-β is necessary for human Th17 cell differentiation (54).To further clarify the role of TGF-β, Annunziato conducted a further study in 2009. It was noted that TGF-β has no direct effect on the differentiation of Th17 cells from their precursors, but can indirectly promote Th17 development by inhibiting T-bet expression and Th1 amplification (55). A recent convincing study demonstrated that TGF-β promotes Th17 cells to differentiate by reversing SKI-SMAD4-mediated inhibition of RORγt expression through inhibition of Rorc gene acetylation (56). Furthermore, TLR2 (a pattern recognition receptor) signaling in T cells promotes Th17 cell proliferation and upregulates Th17-related genes (IL-17, IL-17F, IL-21, and CCR6) (57).
Recent studies have revealed that the heterogeneous Th17 cell subpopulation in humans can be further subdivided into two major categories based on the differential expression of chemokine receptors CCR4 and CXCR3: classical immunomodulatory Th17 and non-classical pro-inflammatory Th17. Classical Th17 is characterized by (CCR4+CXCR3-) indicating high levels of IL-17 and low levels of IFN-γ, whereas non-classical Th17 is characterized by (CCR4-CXCR3, also known as Th17.1 or Th1/Th17) producing low levels of IL-17 and high amounts of IFN -γ, with a phenotype similar to that of Th1 (13).
2.2 Th1
Till now, activated Th1 cells play an important role in coordinating the antitumor immune response, particularly in supporting the normalization of tumor vasculature (12, 13). Besides, Th1 cells are promoted by the critical cytokines IFN-γ (via Stat1) and IL-12(via Stat4), which induce the expression of the transcription factor T-bet and secretion of the signature cytokine IFN-γ protecting the host from intracellular infection (58).
Firstly, STAT1-dependent T-bet, a Th1-specific T box transcription factor, induces IL-12 receptors followed by activation of STAT4. It is a key regulator of Th1 differentiation and IFN-γ production (59–61). Secondly, Th1 cell induction is dependent on IL-12 expression, initiating downstream signaling cascades that induce IFN-γ production by NK cells (62, 63). Interestingly, it has been shown that cells such as Treg cells, which secrete the cytokine IL-10, regulate the life cycle of Th1 cells by preventing DCs from secreting IL-12 (64). And Interferon-gamma (IFN-γ), a major mediator of inflammation and tissue injury, is a fundamental product of activated Th1 cells. Although the particulars are not yet clear, there is convincing evidence provided that Th1-derived IFN-γ may control tumor vasculature through its anti-proliferative effect on endothelial cells and prevent tumor recurrence by keeping tumors in a state of ischemia (65). In addition, Salmonella enterica (Se) or Influenza A virus (IAV) can also influence the life cycle of Th1 cells (61).
2.3 Treg
Regulatory T (Treg) cells, a specialized immunosuppressive lineage, play a crucial role in the induction of tumor-specific immune tolerance (66–70). The antitumor response in B-Cell Non-Hodgkin’s Lymphoma is inhibited by intra-tumor Treg (71). It is also certain that Treg cells play a vital role in autoimmune diseases, infectious diseases, and organ transplantation (72–75).
Similar to differentiation of the Th17 lineage, the development of Treg cells is mediated by selective cytokine signals. Treg cells exert their rapid suppressive effects by secreting inhibitory cytokines such as IL-10 and TGF-β or by inhibitory checkpoint molecules such as TIGIT and CTLA-4 (26, 76, 77). In vivo, developing Treg cells also rely on the expression of IL-2, IL-15, and TGF-β (77, 78). In addition, in recent years, a few research has shown that Treg cells maintain immune homeostasis and support tissue function with the expression of the lineage-specifying transcription factor Foxp3 (66, 79–81). After induction of TCR stimulation, the Foxp3 shapes the identity of regulatory T cells by fine-tuning the activity of other major chromatin remodeling TFs such as TCF1 and modulates related gene expression (79, 82–85). Treg cell epigenetic alterations such as hypomethylation of specific DNA regions are also critical in the Treg cell specification process (86). Thus, therapeutic strategies implementing epigenetic regulatory drugs and gene editing technologies are expected to significantly impact Treg cells to exert antitumor effects (69, 87–89).
3 Role of Th17 in the tumor microenvironment
Recent studies have revealed that Th17 cells infiltrate many types of tumors, such as B cell (non-Hodgkin) cancer, breast cancer, colon cancer, gastric cancer, hepatocellular cancer, melanoma, myeloma, ovarian cancer, pancreatic cancer, and so on, depending on the chemokine receptor interactions (10, 17, 71, 90–98). As demonstrated by the studies of Meng and Yang, Th17 cells retain a high degree of plasticity, allowing for conversion to other lineages under precise stimulation or pathogenic conditions (71, 99). Because of this, we hypothesized that the high plasticity of Th17 cells may contribute to the complex dual function shaping the tumor environment.
In addition, data from Joseph Fabre confirmed the association of IL-17 with the different tumor environments (100). IL-17 is a family of pro-inflammatory cytokines produced by Th17 cells, which contains six cytokines (IL-17A to IL-17F) that are linked to five receptors (IL-17RA through IL-17RE) (14, 101–108). Activation of IL-17 and IL-17F genes by cytokine signaling appears to be functionally linked to histone H3 hyperacetylation (106). Despite the growing evidence for the pathogenic role of IL-17 in cancer, the underlying molecular and cellular mechanisms are still not fully understood. Targeting IL-17 signaling may be helpful in a variety of diseases and cancers (109–111). Here we also discuss the function of the IL-17 depending on cell or tumor type and cytokine properties in the microenvironment, focusing on the balance between the pathogenic and protective roles of IL-17 in antitumor immunity and tumorigenesis.
3.1 Tumor-promoting functions of Th17
3.1.1 Pro-tumor functions of IL-17
Several independent studies have demonstrated that IL-17 is highly expressed in peripheral blood, malignant ascites fluid, and liver, stomach, colon, pancreas, breast, lung, and ovarian tumor tissues, and positively correlates with malignancy aggressiveness (17, 91, 94, 95, 97, 112–121). The mechanisms that we believe could achieve the oncogenic effects of IL-17 have been listed below.
Li evaluates more than 40 HCC specimens by IHC staining and finds that IL-17A levels are significantly higher in HCC specimens that have metastasized than in non-metastasized HCC primary specimens (120). That is, the high frequency of IL-17A-positive cells in tumor tissues is correlated with metastasis and poor prognosis of hepatocellular carcinoma (HCC). Further studies reveal that this correlation is since IL-17A can significantly promote cell migration rate by activating nuclear factor-κB (NF-κB) transcription factors and upregulating matrix metalloproteinase 2 (MMP2) and 9 (MMP9) (120, 122). In a recent article, Gu et al. argued that IL-17 promotes HCC invasion and migration via Akt-dependent IL-6/STAT3 activation and subsequently upregulates its downstream targets IL-8, MMP2, and VEGF (112). IL-17 enhances VEGF and CD31 expression, stimulates the transcription of angiopoietin-2, and promotes tumor growth strongly with high microvessel density (MVD) (80, 89-91). In addition, since EMT plays an important role in tumor metastasis, IL-17-induced EMT promotes lung cancer cell migration and invasion through NF-κB-mediated upregulation of ZEB1 (123, 124).
Another possible mechanism leading to cancer malignancy is that IL-17 promotes cancer stem-like cells (CSLCs) tumorigenic potential. For example, in ovarian tumors, it was found that IL-17 producing cells are located in the niche near the tumor stem cells and IL-17 promotes self-renewal of CD133(+) CSLCs mediated by NF-κB and p38 MAPK signaling pathway (113). IL-17 contributes to the initiation and progression of pancreatic interepithelial neoplasia (PanIN) by increasing the activation of NF-κB and MAPK and the expression of DCLK1 and ALDH1A1 (a marker of embryonic stem cells) (118). IL-17A exerts its pro-tumorigenic activity through IL-17RA which is involved in activating ERK, p38 MAPK, and NF-κB signaling (115). This signals directly promotes the development of early tumor in the mice with a COX2P-NLS Cre recombinase transgene and a loxP-targeted APC allele (115, 125). In Multiple myeloma (MM), IL-17 activates the oncogenic p65 transcription factor, which directly represses the miR-192 gene by binding to the miR-192 promoter and also induced EMT and inhibits cell adhesion to fibronectin and collagen I, promoting cell migration (126). More recently, it was shown that Th17 and IL-17 also accelerate endogenously arising lung tumors proliferation and angiogenesis in part by transducing NF-κB and ERK signaling to induce the expression of G-CSF, Bv8, and VEGF, among others (17). Myeloid derived suppressor cells (MDSC) as an immature form of myeloid cells mostly identified as CD11b and Gr-1 double positive cells in mice are commonly considered that can suppress immunity by arginase-1 (Arg-1), MMP9, and S100A8/A9 in tumor microenvironment (127–130). The researchers discovered that IL-17 is required for the development of MDSC and IL-17R−/− tumor bearing mice expressed lower levels of arginase-1 (Arg-1), MMP9, and S100A8/A9 (127). In IL-17R-deficient mice, MDSC cells decreased as CD8 T cells increased (127). Coincidentally, in a mouse model of lung cancer, in which an oncogenic form of K-ras (K-ras(G12D)), Seon found that Th17 cells preferentially accumulate in tumor tissue and increased Th17 associate with inflammation-promoted lung cancer by recruiting Gr-1CD11b myeloid cells, reducing cytotoxic CD8 T activity and promoting Th17 cell-mediated inflammation (17, 127).
3.1.2 Plasticity of Th17 and Treg
As the constitutive ratio of Tregs and Th17 cells is modified in the tumor microenvironment, human Th17 cells exhibit substantial developmental plasticity and differentiate into Treg cells, an immunosuppressive subset infiltrating the tumor microenvironment (69, 131). The reduction of Treg cells in tumors is associated with a marked increase in survival (72).
Yang et al. found that Foxp3 expression in Treg cells obtained by in vitro induced differentiation was consistently increased by stimulation with TGF-β alone; however, in the presence of TGF-β, IL-6 alone or combination with IL-1 and IL-23 significantly downregulated Foxp3 expression and increased IL-17 production (132). In vitro experiments by Jian Ye’s group confirms this idea: first, they obtained a Th17 subpopulation by stimulating TILs from ovarian and colon cancers with a CD3 monoclonal antibody as well as IL-2; and then, they used flow cytometry to detect the results after amplifying this subpopulation three times and found a significant increase in the FOXP3+ cell subtype with an obvious decrease in the IL-17+ cells, a differentiation that is dependent on TCR stimulation (133, 134). These experiments evidenced that Th17 and Treg are capable of interconversion in vitro.
In biopsy specimens from B-Cell Non-Hodgkin’s lymphoma, malignant B cells induce Foxp3 expression, promote Treg development, and lead to suppression of Th17 cells differentiation, thereby establishing a profoundly inhibitory tumor microenvironment (71). This implies that there is an intimate relationship between Th17 and Treg in the tumor microenvironment.Th17 cells are a source of tumor-induced Treg cells in tumor-bearing mice (135). ID8A ovarian cancer and MC38 colorectal cancer cells were injected intraperitoneally in IL-17ACreR26RReYFP fate reporter mice which allow the visualization of cells that had activated the IL-17 program irrespective of current production of this cytokine, and then researchers calculated the cells from tumor-bearing mice by flow cytometry (135, 136). The study found that a considerable proportion of eYFP+ cells(which represent IL17 producing cells) begin expressing Foxp3 and the percentages of Foxp3+CD4+ T cells(ex-Th17) gradually increases, while the percentage of eYFP+Foxp3neg cells (‘true’ Th17 cells) declines at the later time points (135). In addition, the percentage of tumor-associated Foxp3 cells is significantly reduced in RORγnegID8 tumor-bearing mice (135). Stephanie Downs-Canner et al. suggested that Th17 cell transdifferentiation serves as an important pathway of Treg cell emergence in the tumor microenvironment. Recent articles have discovered that HIF-1α regulates the downstream Th17 genes by directly inducing RORγt transcription to promote Th17 differentiation. Eric V Dang further found that Foxp3 is very sensitive to hypoxia and HIF-1α inhibits Treg differentiation through the glycolytic pathway, allowing Foxp3 protein to be degraded (47, 137). There is increasing evidence that tumor-infiltrating Th17 cells can also differentiate into Tregs due to alter epigenetics and reprogram gene expression profiles, including lineage-specific transcriptional factors and cytokine genes (71, 133).
Immunosuppressive Treg cells are associated with advanced invasion and prognostic exacerbation of malignancies in tumor microenvironment as they inhibit the killing of tumor cells by antigen specific CD8+ T cells. Sadna Budhu et al. used ex vivo three-dimensional collagen-fibrin gel cultures of isolated B16 melanoma to qualitatively measure inhibition. Tumor cells displayed resistance to killing by activated antigen-specific CD8+ T cells, and this resistance was dependent on the binding of TGF-β above resident Treg cells in mice (138). Chen BJ et al. showed that Treg cells ultimately allow tumor escape by rendering T cells dysregulation and by their own immunosuppression (73). Therefore, shifting the balance of Th17/Treg towards Th17 may be beneficial for patients with aggressive tumors (135, 138, 139). The impact of Th17 cell plasticity in the tumor microenvironment is shown in Figure 1.
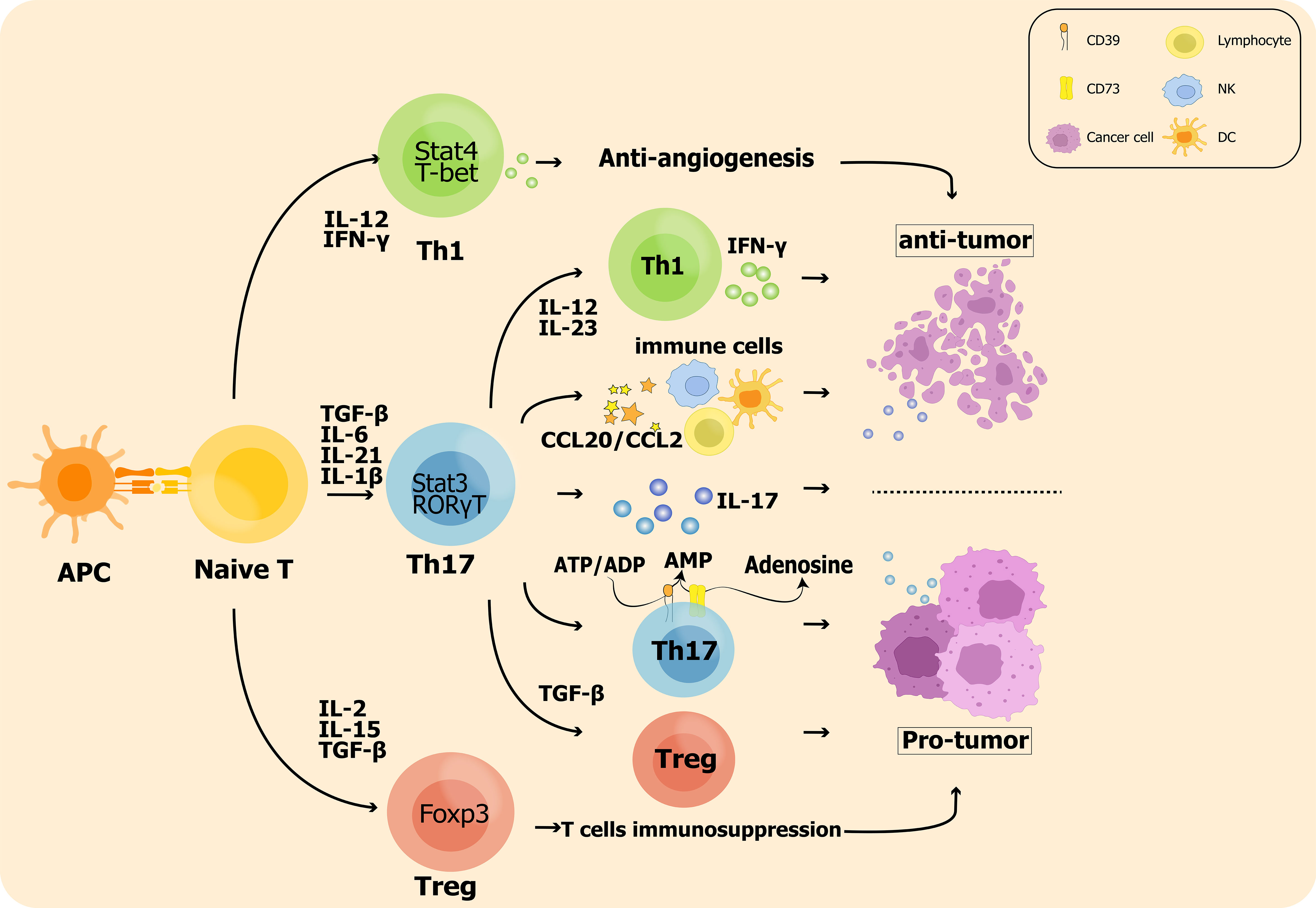
Figure 1 The impact of Th17 cell plasticity in the tumor microenvironment: On the one hand, Th17 cells promote Treg cell polarization leading to enhanced antitumor immunosuppression; on the other hand, Th17 cells are also converted to the Th1 phenotype and produce IFN-γ to exert antitumor effects. In addition, inflammatory hallmark cytokines such as IL-17 produced by Th17 cells are significantly associated with tumor metastasis and prognosis.
3.1.3 CD39 and CD73 define the adenosine production of Th17
Recent studies have confirmed that Th17 cells might also make a difference in immunosuppressive functions through ectonucleotidases CD39 and CD73 to suppress T cell proliferation and cytokine production, causing pro-tumorigenic functions (140–144). The specific possible mechanism is that the enzyme CD39 cleaves ATP or ADP to AMP; meanwhile, CD73 converts AMP to adenosine, the final immunosuppressive molecule (145). Adenosine inhibits both NK and CD8+ T cells mainly by A2A adenosine receptor signaling, promoting tumor immune evasion and escape (146). Chalmin (2012) validated that in vitro Th17 cells generate with the cytokines IL-6 and TGF-β expressed CD39 and CD73, and convert ADP to adenosine, leading to suppression of CD4+ and CD8+ T cell effector functions and subsequent promotion of tumor malignancy (144). In 2014, Aiping Bai’s group presented their novel findings that dual expression of CD39 and CD161 better defines the Th17 profile of human CD4+ T cells, which modulates human Th17 responsiveness by altering the biological activity of acid sphingomyelinase (ASM) and activating downstream signals (including STAT3 and mTOR), as seen in patients with Crohn’s disease (147–149).
However, it was noted that CD73 and CD39 expression was downregulated and exerted anti-tumor immunity when small molecule RORt agonists were applied to induce Th17 differentiation; this experimental result seems to be contradictory with the aforementioned conclusion that Th17 promotes tumors through CD73 and CD39 (150). Th17 exerts pro-tumor effects based on CD39 and CD73 dependent on the conditions of Th17 differentiation. At high concentrations of TGF-β, transcription factor GFi-1 is down-regulated while expression of exonucleases CD73 and CD39 is increased, followed by increased adenosine production, leading to a diminished antitumor effector function (144). However, in the presence of low concentrations of TGFβ with IL-6 and IL-1β, the generated Th17 cells expressed lower levels of CD73 and better antitumor activity (151); Not coincidentally, the application of small-molecule RORt agonists resulted in decreased expression of both CD39 and CD73, th17 which in turn exerted anti-tumor immune effects, regardless of TGF-β concentration (150). In addition, this complex dual role could also be due to the alteration of the balance between th17 and treg under various culture conditions. Most human Treg cells express CD73 with CD39 and exert immunosuppressive effects, and increased Treg infiltration in the tumor microenvironment enhances adenosine-increased mediated immunosuppression. In studies applying RORyT agonists, Treg cell differentiation was inhibited which further affected CD73 & CD39 expression. Interestingly, the differentiation of Th1 cells was not inhibited in this experiment and the expression level of T-bet, the main transcription factor of th1 cells, was extremely similar to RORγT and exerted an anti-tumor immune effect.
3.2 Tumor-protective functions of Th17
It has been shown that the proportion of Th17 cells is decreased in the malignant tumor environment compared to the benign tumor (26, 152, 153).
As evidenced by mouse experiments in vitro, enhancing Th17 cell differentiation by the application of RORγ agonist can increase the expression of the signature 17-type cytokines IL-17A, IL-17F, IL-22, and GM-CSF, and reduce the expression of PD-L1 (150). And then further increase the expression of co-stimulatory receptors, which may provide superior antitumor activity and represent a promising immunotherapeutic approach for the treatment of cancer (150, 154). In other words, Th17 cells affect the prognosis of patients and may even play a powerful role in anti-tumor immunity (90, 155).
3.2.1 Anti-tumor functions of IL-17
While it has been reported that IL-17 promote tumor progression and metastasis by increasing inflammatory angiogenesis, other investigators have pointed out that IL-17 exert antitumor effects by modulating adaptive immune responses via recruiting T lymphocytes, enhancing NK cell activity, and promoting the generation and activation of CTLs (156–162).
For instance, a 50-year-old man with a history of mild psoriasis and Crohn’s disease was treated with a PD-1 blocker (pembrolizumab) for metastatic colon cancer. There was a significant 50% reduction of CEA level in the first two rounds of treatment. However, after the third cycle of pembrolizumab, the patient developed a severe psoriatic rash covering 75% of the body, along with abdominal pain and increased stool frequency. To address the skin manifestations, the patient was treated with an IL-17A blocker (secukinumab) for the treatment of psoriasis (163). Although IL-17 blockade improved psoriasis symptoms and gastrointestinal pain, antitumor activity decreased as serum CEA returned to pre-treatment levels (164). In 2009, Ilona et al. inoculated mice with colon cancer MC38 cells line and found that IL-17-deficient mice had low level of tumor-infiltrating IFN-γ T cells and enhanced tumor growth and subcutaneous and pulmonary metastasis compared to normal wild-type mice (165). And Chen concluded that low amounts of intertumoral IL-17 expression may indicate a poor prognosis for patients with gastric adenocarcinoma (157). Similar results were also obtained in esophageal squamous cell carcinoma (ESCC): the density of IL-17+ cells was inversely correlated with tumor invasion, and the level of IL-17+ cells was positively correlated with the density of CD8+ cytotoxic T lymphocytes (CTLs), CD57+ natural killer (NK) cells and dendritic cells (DCs) by recent studies (156, 166, 167). The levels of IL-17+ TIL and other immune cells involved in antitumor effects, such as CTL, were evaluated in 181 ESCC patients using immunohistochemical methods (167). The results shows that IL-17 induces ESCC tumor cells to produce inflammatory chemokines like CXCL9, CXCL10, and CCL2, CCL20, which are respectively associated with the migration of T cells, NK cells, and DCs (167). The paradoxical effects of IL-17 produced by Th17 cells are illustrated in Figure 2.
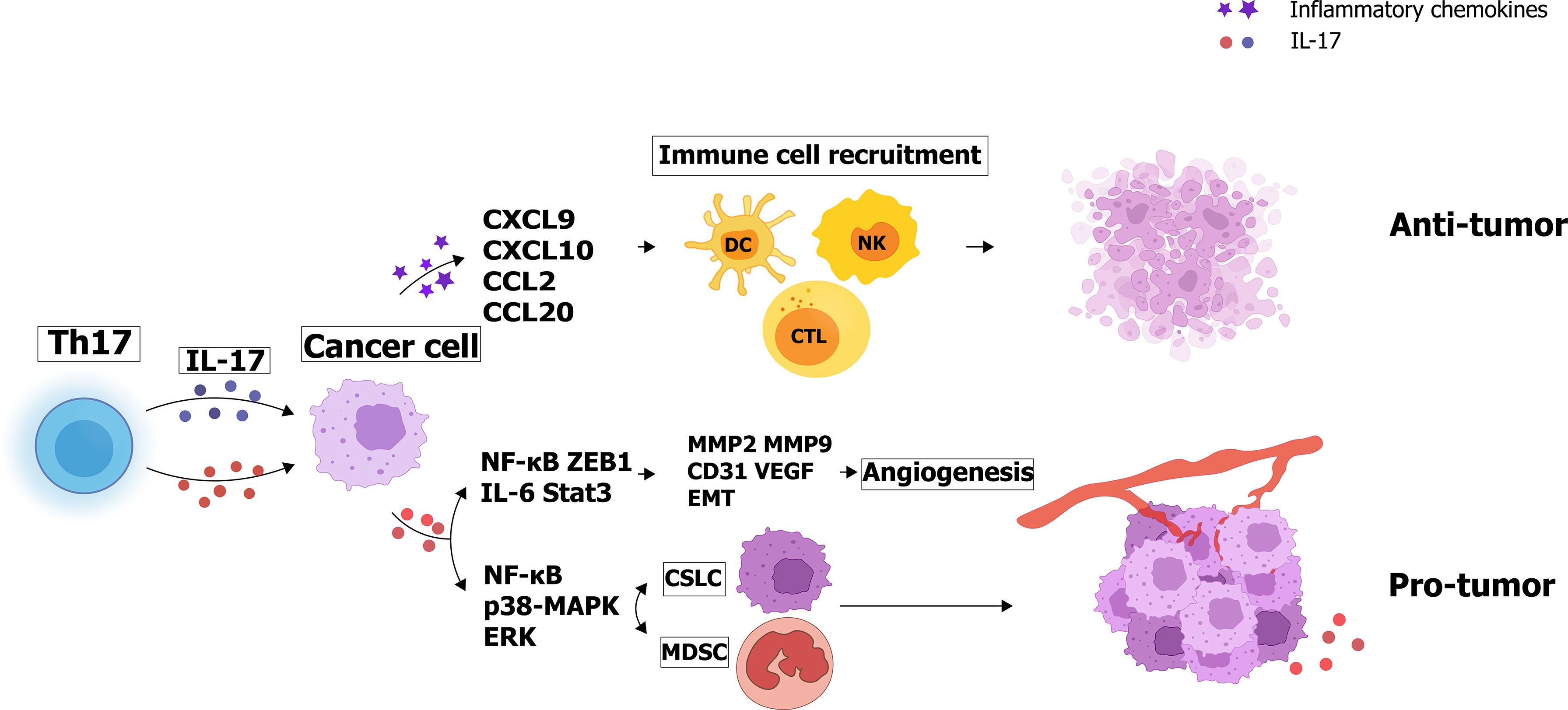
Figure 2 Paradoxical effects of IL-17 produced by Th17 cells: IL-17 secretes angiogenic factors and increases matrix metalloproteinase expression to promote tumor cell proliferation and invasion. Interestingly, IL-17 can also enhance tumor cell apoptosis by recruiting immune cells such as NK cells and CTL cells.
3.2.2 Plasticity of Th17 and Th1
Th17 cells in tumor immunity not only promote the activation of cytotoxic T cells, but also even directly convert to T helper type 1 lineage leading to the production of IFN-γ (10, 168). IFN-γ plays a key role in autoimmune diseases and tumor-protective functions through direct anti-proliferation, pro-apoptosis, and anti-angiogenesis functions (64, 169, 170).
IL-17 producing Th17 cells can switch to effectors with similar characteristics to Th1 cells (known as ex-Th17 or non-classical Th1 cells), which support the ability of antitumor or immunity (11, 170–172). New research shows that the percentage of RORγt cells is similar to that of cells expressing T-bet in tumor-infiltrating lymphocytes (TILs) from different tumor types, such as breast cancer and ovarian cancer (150). In vitro experiments have suggested that in the presence of low levels or total absence of TGF-β, IL-12 and IL-23 cytokines induce conversion of Th17 cells to the Th1 phenotype; while sufficient TGF-β retains Th17 phenotype is retained (12, 63). Interestingly, other researches show that by activating stat4, IL-12 and IL-23 convert Th17 precursors into the Th1-type phenotype, because of their functions of STAT4 activation (63). Furthermore, Meng revealed that Dendritic cells (DCs) expressing Notch ligand DLL4 can promote Th1 and Th17 differentiation by directly activating the transcription factors T-bet and RORγ (99). Moreover, th17-derived cells (ex-Th17 or non-classical Th1 cells) distinguish from classical Th1 cells via unique surface markers, including CD161, CCR6, and IL-17RE (26, 171, 173). Basdeo SA et al. found that these non-classical Th1 cells express significantly higher anti-apoptotic Bcl-2 implying better survivability, and secrete more TNF, IL-2, GM-CSF, and IFN-γ (174).
3.2.3 The recruitment and activation of immunity cells
Th17 cells also drive antitumor immune responses by recruiting immune cells to the tumor, particularly by activating effector CD8+T cells. It was reported that Th17 cells stimulated CD8+ cytotoxic T lymphocytes (CTL) responses via IL-2 and pMHC I and the deterioration of OVA-expressing B16 melanoma can be avoided by this pathway (168, 175). What’s more, Th17 cells were able to stimulate the expression of chemoattractants CCL2 and CCL20 in lung tumor microenvironments and promote the recruitment of various inflammatory leukocytes (DCs, CD4+, and CD8+T cells).It has been shown that Plasmacytoid dendritic cells (pDC) activated by CpG-activated and antigen presentation induced the differentiation and development of Th17 cells, producing large amounts of other inflammatory cytokines such as IFN-γ (176, 177). When pDCs are deficient in MHC II expression, the frequency of infiltrating Th17 in tumor tissue is reduced and the Th17 response is defective, leading to a decrease in the recruitment of immunity cells such as CTL, which ultimately leads to tumor growth (178).
Further understanding of the characteristics of Th17 cells in the tumor microenvironment could generate new therapeutic approaches for cancer treatment. In contrast to Th1 cells, Th17 cells do not exhibit senescence or apoptosis and maintain potent anti-tumor efficacy in vivo (179). Th17 cells offer promise for the next generation of ACT trials because of their stability and persistence (179).
4 Conclusion
It was found that Th17 cells transform into Th1 or Treg cell subtypes under certain conditions, and there are at least two balances in the microenvironment. One is the Treg/Th17 balance: the presence of TGF-β, IL-23, and IL-6 maintain the Th17 phenotype, while TGF-β alone allows a shift in differentiation toward the Treg phenotype (67). The other is the Th1/Th17 balance: IL-12 and IL-23 induce the conversion of Th17 cells to a Th1 cells phenotype in the absence of TGF-β. These two balances are in opposition to each other, shaping the effect of Th17 on tumors. Th17 cells may inhibit T cell proliferation and cytokine production through CD39 and CD73, or promote differentiation of Treg cells, resulting in altered immunosuppressive functions. However, Th17 cells may also promote the activation of cytotoxic T cells or convert to the Th1 phenotype and produce antitumor effects of IFN-γ in tumor immunity. Given the role of protumoral Treg and antitumoral Th1 on tumors, we believe that further understanding of the high plasticity of Th17 cells may yield new therapeutic approaches to treat cancer (180).
In addition, this two-fold effect also depends on IL-17. IL-17 is significantly correlated with tumor development as well as prognosis, and the relationship between prognosis and IL-17 expression level differed among tumor types (181). On the one hand, IL-17 serves to promote tumor cell proliferation or invasion and inhibit tumor cell apoptosis by secreting angiogenic factors and increasing the expression of matrix metalloproteinases (including MMP-2 and MMP-9). On the other hand, IL-17 enhances apoptosis and decreases tumor growth by promoting the activation of NK cells and CTL cells, recruiting neutrophils, NK cells, and CD8+ T cells (182). The effects of Th17 cells are concluded in Table 1.
Since the molecular mechanisms by which Th17 cells function in tumors remain unclear, we believe that further understanding of the relevant mechanisms will facilitate the exploration of novel effective therapeutic approaches and ultimately improve the prognosis of cancer patients.
Author contributions
YP and WY contributed equally to this review. Conceptualization, LL. Investigation, YP and WY. Writing – original draft preparation, YP and WY. Writing – review and editing, BT, XW, QZ, and WL. Supervision, YP and WY. Project administration, LL. All authors contributed to the article and approved the submitted version.
Funding
This work was supported by the National Natural Science Foundation of China (No.81970181).
Conflict of interest
The authors declare that the research was conducted in the absence of any commercial or financial relationships that could be construed as a potential conflict of interest.
Publisher’s note
All claims expressed in this article are solely those of the authors and do not necessarily represent those of their affiliated organizations, or those of the publisher, the editors and the reviewers. Any product that may be evaluated in this article, or claim that may be made by its manufacturer, is not guaranteed or endorsed by the publisher.
References
1. Basu R, Whitlock BM, Husson J, Le Floc’h A, Jin W, Oyler-Yaniv A, et al. Cytotoxic T cells use mechanical force to potentiate target cell killing. Cell (2016) 165(1):100–10. doi: 10.1016/j.cell.2016.01.021
2. Reina-Campos M, Scharping NE, Goldrath AW. CD8(+) T cell metabolism in infection and cancer. Nat Rev Immunol (2021) 21(11):718–38. doi: 10.1038/s41577-021-00537-8
3. Abu N, Rus Bakarurraini NAA. The interweaving relationship between extracellular vesicles and T cells in cancer. Cancer Lett (2022) 530:1–7. doi: 10.1016/j.canlet.2021.12.007
4. Zhu J, Yamane H, Paul WE. Differentiation of effector CD4 T cell populations (*). Annu Rev Immunol (2010) 28:445–89. doi: 10.1146/annurev-immunol-030409-101212
5. Kaplan MH, Hufford MM, Olson MR. The development and in vivo function of T helper 9 cells. Nat Rev Immunol (2015) 15(5):295–307. doi: 10.1038/nri3824
6. Annunziato F, Romagnani S. Do studies in humans better depict Th17 cells? Blood (2009) 114(11):2213–9. doi: 10.1182/blood-2009-03-209189
7. Park H, Li Z, Yang XO, Chang SH, Nurieva R, Wang YH, et al. A distinct lineage of CD4 T cells regulates tissue inflammation by producing interleukin 17. Nat Immunol (2005) 6(11):1133–41. doi: 10.1038/ni1261
8. Harrington LE, Hatton RD, Mangan PR, Turner H, Murphy TL, Murphy KM, et al. Interleukin 17-producing CD4+ effector T cells develop via a lineage distinct from the T helper type 1 and 2 lineages. Nat Immunol (2005) 6(11):1123–32. doi: 10.1038/ni1254
9. Annunziato F, Cosmi L, Santarlasci V, Maggi L, Liotta F, Mazzinghi B, et al. Phenotypic and functional features of human Th17 cells. J Exp Med (2007) 204(8):1849–61. doi: 10.1084/jem.20070663
10. Guéry L, Hugues S. Th17 cell plasticity and functions in cancer immunity. BioMed Res Int (2015) 2015:314620. doi: 10.1155/2015/314620
11. Muranski P, Restifo NP. Essentials of Th17 cell commitment and plasticity. Blood (2013) 121(13):2402–14. doi: 10.1182/blood-2012-09-378653
12. Nistala K, Adams S, Cambrook H, Ursu S, Olivito B, de Jager W, et al. Th17 plasticity in human autoimmune arthritis is driven by the inflammatory environment. Proc Natl Acad Sci USA (2010) 107(33):14751–6. doi: 10.1073/pnas.1003852107
13. Cerboni S, Gehrmann U, Preite S, Mitra S. Cytokine-regulated Th17 plasticity in human health and diseases. Immunology (2021) 163(1):3–18. doi: 10.1111/imm.13280
14. Pelletier M, Maggi L, Micheletti A, Lazzeri E, Tamassia N, Costantini C, et al. Evidence for a cross-talk between human neutrophils and Th17 cells. Blood (2010) 115(2):335–43. doi: 10.1182/blood-2009-04-216085
15. Zhang W, Liu X, Zhu Y, Liu X, Gu Y, Dai X, et al. Transcriptional and posttranslational regulation of Th17/Treg balance in health and disease. Eur J Immunol (2021) 51(9):2137–50. doi: 10.1002/eji.202048794
16. Qianmei Y, Zehong S, Guang W, Hui L, Lian G. Recent advances in the role of Th17/Treg cells in tumor immunity and tumor therapy. Immunol Res (2021) 69(5):398–414. doi: 10.1007/s12026-021-09211-6
17. Chang SH, Mirabolfathinejad SG, Katta H, Cumpian AM, Gong L, Caetano MS, et al. T Helper 17 cells play a critical pathogenic role in lung cancer. Proc Natl Acad Sci USA (2014) 111(15):5664–9. doi: 10.1073/pnas.1319051111
18. Dong C. Cytokine regulation and function in T cells. Annu Rev Immunol (2021) 39:51–76. doi: 10.1146/annurev-immunol-061020-053702
19. Luckheeram RV, Zhou R, Verma AD, Xia B. CD4+T cells: differentiation and functions. Clin Dev Immunol (2012) 2012:925135. doi: 10.1155/2012/925135
20. Nakayama M. Antigen presentation by MHC-dressed cells. Front Immunol (2014) 5:672. doi: 10.3389/fimmu.2014.00672
21. Fooksman DR, Vardhana S, Vasiliver-Shamis G, Liese J, Blair DA, Waite J, et al. Functional anatomy of T cell activation and synapse formation. Annu Rev Immunol (2010) 28:79–105. doi: 10.1146/annurev-immunol-030409-101308
22. Fooksman DR. Organizing MHC class II presentation. Front Immunol (2014) 5:158. doi: 10.3389/fimmu.2014.00158
23. Imam T, Park S, Kaplan MH, Olson MR. Effector T helper cell subsets in inflammatory bowel diseases. Front Immunol (2018) 9:1212. doi: 10.3389/fimmu.2018.01212
24. Hirahara K, Nakayama T. CD4+ T-cell subsets in inflammatory diseases: beyond the Th1/Th2 paradigm. Int Immunol (2016) 28(4):163–71. doi: 10.1093/intimm/dxw006
25. Barbi J, Pardoll D, Pan F. Treg functional stability and its responsiveness to the microenvironment. Immunol Rev (2014) 259(1):115–39. doi: 10.1111/imr.12172
26. Knochelmann HM, Dwyer CJ, Bailey SR, Amaya SM, Elston DM, Mazza-McCrann JM, et al. When worlds collide: Th17 and treg cells in cancer and autoimmunity. Cell Mol Immunol (2018) 15(5):458–69. doi: 10.1038/s41423-018-0004-4
27. Ivanov II, McKenzie BS, Zhou L, Tadokoro CE, Lepelley A, Lafaille JJ, et al. The orphan nuclear receptor RORgammat directs the differentiation program of proinflammatory IL-17+ T helper cells. Cell (2006) 126(6):1121–33. doi: 10.1016/j.cell.2006.07.035
28. Dong C. Diversification of T-helper-cell lineages: finding the family root of IL-17-producing cells. Nat Rev Immunol (2006) 6(4):329–33. doi: 10.1038/nri1807
29. Dong C. TH17 cells in development: an updated view of their molecular identity and genetic programming. Nat Rev Immunol (2008) 8(5):337–48. doi: 10.1038/nri2295
30. Yang XO, Pappu BP, Nurieva R, Akimzhanov A, Kang HS, Chung Y, et al. T Helper 17 lineage differentiation is programmed by orphan nuclear receptors ROR alpha and ROR gamma. Immunity (2008) 28(1):29–39. doi: 10.1016/j.immuni.2007.11.016
31. Suri C, Awasthi A, Asthana S. Crystallographic landscape provides molecular insights into the modes of action of diverse ROR-γt modulators. Drug Discov Today (2022) 27(2):652–63. doi: 10.1016/j.drudis.2021.11.022
32. Durant L, Watford WT, Ramos HL, Laurence A, Vahedi G, Wei L, et al. Diverse targets of the transcription factor STAT3 contribute to T cell pathogenicity and homeostasis. Immunity (2010) 32(5):605–15. doi: 10.1016/j.immuni.2010.05.003
33. Yang XP, Ghoreschi K, Steward-Tharp SM, Rodriguez-Canales J, Zhu J, Grainger JR, et al. Opposing regulation of the locus encoding IL-17 through direct, reciprocal actions of STAT3 and STAT5. Nat Immunol (2011) 12(3):247–54. doi: 10.1038/ni.1995
34. Johnson DE, O’Keefe RA, Grandis JR. Targeting the IL-6/JAK/STAT3 signalling axis in cancer. Nat Rev Clin Oncol (2018) 15(4):234–48. doi: 10.1038/nrclinonc.2018.8
35. Ma CS, Chew GY, Simpson N, Priyadarshi A, Wong M, Grimbacher B, et al. Deficiency of Th17 cells in hyper IgE syndrome due to mutations in STAT3. J Exp Med (2008) 205(7):1551–7. doi: 10.1084/jem.20080218
36. Holland SM, DeLeo FR, Elloumi HZ, Hsu AP, Uzel G, Brodsky N, et al. STAT3 mutations in the hyper-IgE syndrome. N Engl J Med (2007) 357(16):1608–19. doi: 10.1056/NEJMoa073687
37. Milner JD, Brenchley JM, Laurence A, Freeman AF, Hill BJ, Elias KM, et al. Impaired T(H)17 cell differentiation in subjects with autosomal dominant hyper-IgE syndrome. Nature (2008) 452(7188):773–6. doi: 10.1038/nature06764
38. Mailer RK, Joly AL, Liu S, Elias S, Tegner J, Andersson J. IL-1β promotes Th17 differentiation by inducing alternative splicing of FOXP3. Sci Rep (2015) 5:14674. doi: 10.1038/srep14674
39. Mangan PR, Harrington LE, O’Quinn DB, Helms WS, Bullard DC, Elson CO, et al. Transforming growth factor-beta induces development of the T(H)17 lineage. Nature (2006) 441(7090):231–4. doi: 10.1038/nature04754
40. Yang L, Anderson DE, Baecher-Allan C, Hastings WD, Bettelli E, Oukka M, et al. IL-21 and TGF-beta are required for differentiation of human T(H)17 cells. Nature (2008) 454(7202):350–2. doi: 10.1038/nature07021
41. Veldhoen M, Hocking RJ, Atkins CJ, Locksley RM, Stockinger B. TGFbeta in the context of an inflammatory cytokine milieu supports de novo differentiation of IL-17-producing T cells. Immunity (2006) 24(2):179–89. doi: 10.1016/j.immuni.2006.01.001
42. Knochelmann HM, Dwyer CJ, Smith AS, Bowers JS, Wyatt MM, Nelson MH, et al. IL6 fuels durable memory for Th17 cell-mediated responses to tumors. Cancer Res (2020) 80(18):3920–32. doi: 10.1158/0008-5472.CAN-19-3685
43. Stritesky GL, Yeh N, Kaplan MH. IL-23 promotes maintenance but not commitment to the Th17 lineage. J Immunol (2008) 181(9):5948–55. doi: 10.4049/jimmunol.181.9.5948
44. McGeachy MJ, Chen Y, Tato CM, Laurence A, Joyce-Shaikh B, Blumenschein WM, et al. The interleukin 23 receptor is essential for the terminal differentiation of interleukin 17-producing effector T helper cells in vivo. Nat Immunol (2009) 10(3):314–24. doi: 10.1038/ni.1698
45. Chung Y, Dong C. Don’t leave home without it: the IL-23 visa to T(H)-17 cells. Nat Immunol (2009) 10(3):236–8. doi: 10.1038/ni0309-236
46. Yang JY, Jie Z, Mathews A, Zhou X, Li Y, Gu M, et al. Intestinal epithelial TBK1 prevents differentiation of T-helper 17 cells and tumorigenesis in mice. Gastroenterology (2020) 159(5):1793–806. doi: 10.1053/j.gastro.2020.07.047
47. Dang EV, Barbi J, Yang HY, Jinasena D, Yu H, Zheng Y, et al. Control of T(H)17/T(reg) balance by hypoxia-inducible factor 1. Cell (2011) 146(5):772–84. doi: 10.1016/j.cell.2011.07.033
48. Yan S, Golumba-Nagy V, Kotschenreuther K, Thiele J, Refaian N, Shuya D, et al. Membrane-bound IL-6R is upregulated on Th17 cells and inhibits treg cell migration by regulating post-translational modification of VASP in autoimmune arthritis. Cell Mol Life Sci (2021) 79(1):3. doi: 10.1007/s00018-021-04076-2
49. Hirahara K, Ghoreschi K, Laurence A, Yang XP, Kanno Y, O’Shea JJ. Signal transduction pathways and transcriptional regulation in Th17 cell differentiation. Cytokine Growth Factor Rev (2010) 21(6):425–34. doi: 10.1016/j.cytogfr.2010.10.006
50. Zhou L, Littman DR. Transcriptional regulatory networks in Th17 cell differentiation. Curr Opin Immunol (2009) 21(2):146–52. doi: 10.1016/j.coi.2009.03.001
51. Ciofani M, Madar A, Galan C, Sellars M, Mace K, Pauli F, et al. A validated regulatory network for Th17 cell specification. Cell (2012) 151(2):289–303. doi: 10.1016/j.cell.2012.09.016
52. Fiering SN, Ho GW. Speed kills: advancement in Th17 cell adoptive cell therapy for solid tumors. Cancer Res (2020) 80(18):3795–6. doi: 10.1158/0008-5472.CAN-20-2306
53. Acosta-Rodriguez EV, Napolitani G, Lanzavecchia A, Sallusto F. Interleukins 1beta and 6 but not transforming growth factor-beta are essential for the differentiation of interleukin 17-producing human T helper cells. Nat Immunol (2007) 8(9):942–9. doi: 10.1038/ni1496
54. Manel N, Unutmaz D, Littman DR. The differentiation of human T(H)-17 cells requires transforming growth factor-beta and induction of the nuclear receptor RORgammat. Nat Immunol (2008) 9(6):641–9. doi: 10.1038/ni.1610
55. Santarlasci V, Maggi L, Capone M, Frosali F, Querci V, De Palma R, et al. TGF-beta indirectly favors the development of human Th17 cells by inhibiting Th1 cells. Eur J Immunol (2009) 39(1):207–15. doi: 10.1002/eji.200838748
56. Zhang S, Takaku M, Zou L, Gu AD, Chou WC, Zhang G, et al. Reversing SKI-SMAD4-mediated suppression is essential for T(H)17 cell differentiation. Nature (2017) 551(7678):105–9. doi: 10.1038/nature24283
57. Reynolds JM, Pappu BP, Peng J, Martinez GJ, Zhang Y, Chung Y, et al. Toll-like receptor 2 signaling in CD4(+) T lymphocytes promotes T helper 17 responses and regulates the pathogenesis of autoimmune disease. Immunity (2010) 32(5):692–702. doi: 10.1016/j.immuni.2010.04.010
58. Robinson DS, O’Garra A. Further checkpoints in Th1 development. Immunity (2002) 16(6):755–8. doi: 10.1016/S1074-7613(02)00331-X
59. Szabo SJ, Kim ST, Costa GL, Zhang X, Fathman CG, Glimcher LH. A novel transcription factor, T-bet, directs Th1 lineage commitment. Cell (2000) 100(6):655–69. doi: 10.1016/S0092-8674(00)80702-3
60. Fu J, Wang D, Yu Y, Heinrichs J, Wu Y, Schutt S, et al. T-Bet is critical for the development of acute graft-versus-host disease through controlling T cell differentiation and function. J Immunol (2015) 194(1):388–97. doi: 10.4049/jimmunol.1401618
61. Krueger PD, Goldberg MF, Hong SW, Osum KC, Langlois RA, Kotov DI, et al. Two sequential activation modules control the differentiation of protective T helper-1 (Th1) cells. Immunity (2021) 54(4):687–701.e684. doi: 10.1016/j.immuni.2021.03.006
62. Trinchieri G, Pflanz S, Kastelein RA. The IL-12 family of heterodimeric cytokines: new players in the regulation of T cell responses. Immunity (2003) 19(5):641–4. doi: 10.1016/S1074-7613(03)00296-6
63. Lee YK, Turner H, Maynard CL, Oliver JR, Chen D, Elson CO, et al. Late developmental plasticity in the T helper 17 lineage. Immunity (2009) 30(1):92–107. doi: 10.1016/j.immuni.2008.11.005
64. Cope A, Le Friec G, Cardone J, Kemper C. The Th1 life cycle: molecular control of IFN-γ to IL-10 switching. Trends Immunol (2011) 32(6):278–86. doi: 10.1016/j.it.2011.03.010
65. Kammertoens T, Friese C, Arina A, Idel C, Briesemeister D, Rothe M, et al. Tumor ischaemia by interferon-γ resembles physiological blood vessel regression. Nature (2017) 545(7652):98–102. doi: 10.1038/nature22311
66. Campbell C, Rudensky A. Roles of regulatory T cells in tissue pathophysiology and metabolism. Cell Metab (2020) 31(1):18–25. doi: 10.1016/j.cmet.2019.09.010
67. Najafi S, Mirshafiey A. The role of T helper 17 and regulatory T cells in tumor microenvironment. Immunopharmacol Immunotoxicol (2019) 41(1):16–24. doi: 10.1080/08923973.2019.1566925
68. Wing JB, Tanaka A, Sakaguchi S. Human FOXP3(+) regulatory T cell heterogeneity and function in autoimmunity and cancer. Immunity (2019) 50(2):302–16. doi: 10.1016/j.immuni.2019.01.020
69. Tanaka A, Sakaguchi S. Regulatory T cells in cancer immunotherapy. Cell Res (2017) 27(1):109–18. doi: 10.1038/cr.2016.151
70. Whiteside TL. What are regulatory T cells (Treg) regulating in cancer and why? Semin Cancer Biol (2012) 22(4):327–34. doi: 10.1016/j.semcancer.2012.03.004
71. Yang ZZ, Novak AJ, Ziesmer SC, Witzig TE, Ansell SM. Malignant b cells skew the balance of regulatory T cells and TH17 cells in b-cell non-hodgkin’s lymphoma. Cancer Res (2009) 69(13):5522–30. doi: 10.1158/0008-5472.CAN-09-0266
72. Malla RR, Vasudevaraju P, Vempati RK, Rakshmitha M, Merchant N, Nagaraju GP. Regulatory T cells: their role in triple-negative breast cancer progression and metastasis. Cancer (2022). 128(6):1171–83 doi: 10.1002/cncr.34084
73. Chen BJ, Zhao JW, Zhang DH, Zheng AH, Wu GQ. Immunotherapy of cancer by targeting regulatory T cells. Int Immunopharmacol (2022) 104:108469. doi: 10.1016/j.intimp.2021.108469
74. Huang S, Liang C, Zhao Y, Deng T, Tan J, Zha X, et al. Increased TOX expression concurrent with PD-1, Tim-3, and CD244 expression in T cells from patients with acute myeloid leukemia. Cytometry B Clin Cytom (2021) 102(2):143–52. doi: 10.1002/cyto.b.22049
75. Guo J, Zhou X. Regulatory T cells turn pathogenic. Cell Mol Immunol (2015) 12(5):525–32. doi: 10.1038/cmi.2015.12
76. Noack M, Miossec P. Th17 and regulatory T cell balance in autoimmune and inflammatory diseases. Autoimmun Rev (2014) 13(6):668–77. doi: 10.1016/j.autrev.2013.12.004
77. Moreau JM, Velegraki M, Bolyard C, Rosenblum MD, Li Z. Transforming growth factor-β1 in regulatory T cell biology. Sci Immunol (2022) 7(69):eabi4613. doi: 10.1007/s10157-017-1516-4
78. Marshall D, Sinclair C, Tung S, Seddon B. Differential requirement for IL-2 and IL-15 during bifurcated development of thymic regulatory T cells. J Immunol (2014) 193(11):5525–33. doi: 10.4049/jimmunol.1402144
79. van der Veeken J, Glasner A, Zhong Y, Hu W, Wang ZM, Bou-Puerto R, et al. The transcription factor Foxp3 shapes regulatory T cell identity by tuning the activity of trans-acting intermediaries. Immunity (2020) 53(5):971–984.e975. doi: 10.1016/j.immuni.2020.10.010
80. Lio CW, Hsieh CS. A two-step process for thymic regulatory T cell development. Immunity (2008) 28(1):100–11. doi: 10.1016/j.immuni.2007.11.021
81. Li J, Xu B, He M, Zong X, Cunningham T, Sha C, et al. Control of Foxp3 induction and maintenance by sequential histone acetylation and DNA demethylation. Cell Rep (2021) 37(11):110124. doi: 10.1016/j.celrep.2021.110124
82. Ohkura N, Sakaguchi S. Transcriptional and epigenetic basis of treg cell development and function: its genetic anomalies or variations in autoimmune diseases. Cell Res (2020) 30(6):465–74. doi: 10.1038/s41422-020-0324-7
83. Huehn J, Beyer M. Epigenetic and transcriptional control of Foxp3+ regulatory T cells. Semin Immunol (2015) 27(1):10–8. doi: 10.1016/j.smim.2015.02.002
84. Morikawa H, Sakaguchi S. Genetic and epigenetic basis of treg cell development and function: from a FoxP3-centered view to an epigenome-defined view of natural treg cells. Immunol Rev (2014) 259(1):192–205. doi: 10.1111/imr.12174
85. Ohkura N, Hamaguchi M, Morikawa H, Sugimura K, Tanaka A, Ito Y, et al. T Cell receptor stimulation-induced epigenetic changes and Foxp3 expression are independent and complementary events required for treg cell development. Immunity (2012) 37(5):785–99. doi: 10.1016/j.immuni.2012.09.010
86. Ohkura N, Kitagawa Y, Sakaguchi S. Development and maintenance of regulatory T cells. Immunity (2013) 38(3):414–23. doi: 10.1016/j.immuni.2013.03.002
87. Amini L, Greig J, Schmueck-Henneresse M, Volk HD, Bézie S, Reinke P, et al. Super-treg: toward a new era of adoptive treg therapy enabled by genetic modifications. Front Immunol (2020) 11:611638. doi: 10.3389/fimmu.2020.611638
88. Dai E, Zhu Z, Wahed S, Qu Z, Storkus WJ, Guo ZS. Epigenetic modulation of antitumor immunity for improved cancer immunotherapy. Mol Cancer (2021) 20(1):171. doi: 10.1186/s12943-021-01464-x
89. Boardman DA, Levings MK. Emerging strategies for treating autoimmune disorders with genetically modified treg cells. J Allergy Clin Immunol (2022) 149(1):1–11. doi: 10.1016/j.jaci.2021.11.007
90. Zou W, Restifo NP. T(H)17 cells in tumor immunity and immunotherapy. Nat Rev Immunol (2010) 10(4):248–56. doi: 10.1038/nri2742
91. Ortiz ML, Kumar V, Martner A, Mony S, Donthireddy L, Condamine T, et al. Immature myeloid cells directly contribute to skin tumor development by recruiting IL-17-producing CD4+ T cells. J Exp Med (2015) 212(3):351–67. doi: 10.1084/jem.20140835
92. Tosolini M, Kirilovsky A, Mlecnik B, Fredriksen T, Mauger S, Bindea G, et al. Clinical impact of different classes of infiltrating T cytotoxic and helper cells (Th1, th2, treg, th17) in patients with colorectal cancer. Cancer Res (2011) 71(4):1263–71. doi: 10.1158/0008-5472.CAN-10-2907
93. He S, Fei M, Wu Y, Zheng D, Wan D, Wang L, et al. Distribution and clinical significance of Th17 cells in the tumor microenvironment and peripheral blood of pancreatic cancer patients. Int J Mol Sci (2011) 12(11):7424–37. doi: 10.3390/ijms12117424
94. Iida T, Iwahashi M, Katsuda M, Ishida K, Nakamori M, Nakamura M, et al. Tumor-infiltrating CD4+ Th17 cells produce IL-17 in tumor microenvironment and promote tumor progression in human gastric cancer. Oncol Rep (2011) 25(5):1271–7. doi: 10.3892/or.2011.1201
95. Liu J, Duan Y, Cheng X, Chen X, Xie W, Long H, et al. IL-17 is associated with poor prognosis and promotes angiogenesis via stimulating VEGF production of cancer cells in colorectal carcinoma. Biochem Biophys Res Commun (2011) 407(2):348–54. doi: 10.1016/j.bbrc.2011.03.021
96. Tong Z, Yang XO, Yan H, Liu W, Niu X, Shi Y, et al. A protective role by interleukin-17F in colon tumorigenesis. PloS One (2012) 7(4):e34959. doi: 10.1371/journal.pone.0034959
97. Chen X, Wan J, Liu J, Xie W, Diao X, Xu J, et al. Increased IL-17-producing cells correlate with poor survival and lymphangiogenesis in NSCLC patients. Lung Cancer (2010) 69(3):348–54. doi: 10.1016/j.lungcan.2009.11.013
98. Niu Y, Zhou Q. Th17 cells and their related cytokines: vital players in progression of malignant pleural effusion. Cell Mol Life Sci (2022) 79(4):194. doi: 10.1007/s00018-022-04227-z
99. Meng L, Bai Z, He S, Mochizuki K, Liu Y, Purushe J, et al. The notch ligand DLL4 defines a capability of human dendritic cells in regulating Th1 and Th17 differentiation. J Immunol (2016) 196(3):1070–80. doi: 10.4049/jimmunol.1501310
100. Fabre J, Giustiniani J, Garbar C, Antonicelli F, Merrouche Y, Bensussan A, et al. Targeting the tumor microenvironment: the protumor effects of IL-17 related to cancer type. Int J Mol Sci (2016) 17(9). doi: 10.3390/ijms17091433
101. Brevi A, Cogrossi LL, Grazia G, Masciovecchio D, Impellizzieri D, Lacanfora L, et al. Much more than IL-17A: cytokines of the IL-17 family between microbiota and cancer. Front Immunol (2020) 11:565470. doi: 10.3389/fimmu.2020.565470
102. Bastid J, Dejou C, Docquier A, Bonnefoy N. The emerging role of the IL-17B/IL-17RB pathway in cancer. Front Immunol (2020) 11:718. doi: 10.3389/fimmu.2020.00718
103. Nies JF, Panzer U. IL-17C/IL-17RE: emergence of a unique axis in T(H)17 biology. Front Immunol (2020) 11:341. doi: 10.3389/fimmu.2020.00341
104. McGeachy MJ, Cua DJ, Gaffen SL. The IL-17 family of cytokines in health and disease. Immunity (2019) 50(4):892–906. doi: 10.1016/j.immuni.2019.03.021
105. Amatya N, Garg AV, Gaffen SL. IL-17 signaling: the yin and the yang. Trends Immunol (2017) 38(5):310–22. doi: 10.1016/j.it.2017.01.006
106. Akimzhanov AM, Yang XO, Dong C. Chromatin remodeling of interleukin-17 (IL-17)-IL-17F cytokine gene locus during inflammatory helper T cell differentiation. J Biol Chem (2007) 282(9):5969–72. doi: 10.1074/jbc.C600322200
107. Zhang X, Angkasekwinai P, Dong C, Tang H. Structure and function of interleukin-17 family cytokines. Protein Cell (2011) 2(1):26–40. doi: 10.1007/s13238-011-1006-5
108. Wu D, Poholek CH, Majumder S, Liu Q, Revu SK, Mohib K, et al. IL-17-dependent fibroblastic reticular cell training boosts tissue protective mucosal immunity through IL-10-producing b cells. Sci Immunol (2021) 6(66):eaao3669. doi: 10.1126/sciimmunol.aao3669
109. Ivanov S, Lindén A. Interleukin-17 as a drug target in human disease. Trends Pharmacol Sci (2009) 30(2):95–103. doi: 10.1016/j.tips.2008.11.004
110. Wang L, Yi T, Zhang W, Pardoll DM, Yu H. IL-17 enhances tumor development in carcinogen-induced skin cancer. Cancer Res (2010) 70(24):10112–20. doi: 10.1158/0008-5472.CAN-10-0775
111. He D, Li H, Yusuf N, Elmets CA, Athar M, Katiyar SK, et al. IL-17 mediated inflammation promotes tumor growth and progression in the skin. PloS One (2012) 7(2):e32126. doi: 10.1371/journal.pone.0032126
112. Gu FM, Li QL, Gao Q, Jiang JH, Zhu K, Huang XY, et al. IL-17 induces AKT-dependent IL-6/JAK2/STAT3 activation and tumor progression in hepatocellular carcinoma. Mol Cancer (2011) 10:150. doi: 10.1186/1476-4598-10-150
113. Xiang T, Long H, He L, Han X, Lin K, Liang Z, et al. Interleukin-17 produced by tumor microenvironment promotes self-renewal of CD133+ cancer stem-like cells in ovarian cancer. Oncogene (2015) 34(2):165–76. doi: 10.1038/onc.2013.537
114. Grivennikov SI, Wang K, Mucida D, Stewart CA, Schnabl B, Jauch D, et al. Adenoma-linked barrier defects and microbial products drive IL-23/IL-17-mediated tumor growth. Nature (2012) 491(7423):254–8. doi: 10.1038/nature11465
115. Wang K, Kim MK, Di Caro G, Wong J, Shalapour S, Wan J, et al. Interleukin-17 receptor a signaling in transformed enterocytes promotes early colorectal tumorigenesis. Immunity (2014) 41(6):1052–63. doi: 10.1016/j.immuni.2014.11.009
116. Liu X, Jin H, Zhang G, Lin X, Chen C, Sun J, et al. Intratumor IL-17-positive mast cells are the major source of the IL-17 that is predictive of survival in gastric cancer patients. PloS One (2014) 9(9):e106834. doi: 10.1371/journal.pone.0106834
117. McAllister F, Bailey JM, Alsina J, Nirschl CJ, Sharma R, Fan H, et al. Oncogenic kras activates a hematopoietic-to-epithelial IL-17 signaling axis in preinvasive pancreatic neoplasia. Cancer Cell (2014) 25(5):621–37. doi: 10.1016/j.ccr.2014.03.014
118. Zhang Y, Zoltan M, Riquelme E, Xu H, Sahin I, Castro-Pando S, et al. Immune cell production of interleukin 17 induces stem cell features of pancreatic intraepithelial neoplasia cells. Gastroenterology (2018) 155(1):210–223.e213. doi: 10.1053/j.gastro.2018.03.041
119. Nam JS, Terabe M, Kang MJ, Chae H, Voong N, Yang YA, et al. Transforming growth factor beta subverts the immune system into directly promoting tumor growth through interleukin-17. Cancer Res (2008) 68(10):3915–23. doi: 10.1158/0008-5472.CAN-08-0206
120. Li J, Lau GK, Chen L, Dong SS, Lan HY, Huang XR, et al. Interleukin 17A promotes hepatocellular carcinoma metastasis via NF-kB induced matrix metalloproteinases 2 and 9 expression. PloS One (2011) 6(7):e21816. doi: 10.1371/journal.pone.0021816
121. Cui G, Li Z, Florholmen J, Goll R. Dynamic stromal cellular reaction throughout human colorectal adenoma-carcinoma sequence: a role of TH17/IL-17A. BioMed Pharmacother (2021) 140:111761. doi: 10.1016/j.biopha.2021.111761
122. Mehner C, Hockla A, Miller E, Ran S, Radisky DC, Radisky ES. Tumor cell-produced matrix metalloproteinase 9 (MMP-9) drives malignant progression and metastasis of basal-like triple negative breast cancer. Oncotarget (2014) 5(9):2736–49. doi: 10.18632/oncotarget.1932
123. Gu K, Li MM, Shen J, Liu F, Cao JY, Jin S, et al. Interleukin-17-induced EMT promotes lung cancer cell migration and invasion via NF-κB/ZEB1 signal pathway. Am J Cancer Res (2015) 5(3):1169–79.
124. Salazar Y, Zheng X, Brunn D, Raifer H, Picard F, Zhang Y, et al. Microenvironmental Th9 and Th17 lymphocytes induce metastatic spreading in lung cancer. J Clin Invest (2020) 130(7):3560–75. doi: 10.1172/JCI124037
125. Hinoi T, Akyol A, Theisen BK, Ferguson DO, Greenson JK, Williams BO, et al. Mouse model of colonic adenoma-carcinoma progression based on somatic apc inactivation. Cancer Res (2007) 67(20):9721–30. doi: 10.1158/0008-5472.CAN-07-2735
126. Sun Y, Pan J, Mao S, Jin J. IL-17/miR-192/IL-17Rs regulatory feedback loop facilitates multiple myeloma progression. PloS One (2014) 9(12):e114647. doi: 10.1371/journal.pone.0114647
127. He D, Li H, Yusuf N, Elmets CA, Li J, Mountz JD, et al. IL-17 promotes tumor development through the induction of tumor promoting microenvironments at tumor sites and myeloid-derived suppressor cells. J Immunol (2010) 184(5):2281–8. doi: 10.4049/jimmunol.0902574
128. Serafini P, Borrello I, Bronte V. Myeloid suppressor cells in cancer: recruitment, phenotype, properties, and mechanisms of immune suppression. Semin Cancer Biol (2006) 16(1):53–65. doi: 10.1016/j.semcancer.2005.07.005
129. Bronte V, Zanovello P. Regulation of immune responses by l-arginine metabolism. Nat Rev Immunol (2005) 5(8):641–54. doi: 10.1038/nri1668
130. Gebhardt C, Németh J, Angel P, Hess J. S100A8 and S100A9 in inflammation and cancer. Biochem Pharmacol (2006) 72(11):1622–31. doi: 10.1016/j.bcp.2006.05.017
131. Marshall EA, Ng KW, Kung SH, Conway EM, Martinez VD, Halvorsen EC, et al. Emerging roles of T helper 17 and regulatory T cells in lung cancer progression and metastasis. Mol Cancer (2016) 15(1):67. doi: 10.1186/s12943-016-0551-1
132. Yang XO, Nurieva R, Martinez GJ, Kang HS, Chung Y, Pappu BP, et al. Molecular antagonism and plasticity of regulatory and inflammatory T cell programs. Immunity (2008) 29(1):44–56. doi: 10.1016/j.immuni.2008.05.007
133. Ye J, Su X, Hsueh EC, Zhang Y, Koenig JM, Hoft DF, et al. Human tumor-infiltrating Th17 cells have the capacity to differentiate into IFN-γ+ and FOXP3+ T cells with potent suppressive function. Eur J Immunol (2011) 41(4):936–51. doi: 10.1002/eji.201040682
134. Peng G, Wang HY, Peng W, Kiniwa Y, Seo KH, Wang RF. Tumor-infiltrating gammadelta T cells suppress T and dendritic cell function via mechanisms controlled by a unique toll-like receptor signaling pathway. Immunity (2007) 27(2):334–48. doi: 10.1016/j.immuni.2007.05.020
135. Downs-Canner S, Berkey S, Delgoffe GM, Edwards RP, Curiel T, Odunsi K, et al. Suppressive IL-17A(+)Foxp3(+) and ex-Th17 IL-17A(neg)Foxp3(+) t(reg) cells are a source of tumor-associated t(reg) cells. Nat Commun (2017) 8:14649. doi: 10.1038/ncomms14649
136. Hirota K, Duarte JH, Veldhoen M, Hornsby E, Li Y, Cua DJ, et al. Fate mapping of IL-17-producing T cells in inflammatory responses. Nat Immunol (2011) 12(3):255–63. doi: 10.1038/ni.1993
137. Shi LZ, Wang R, Huang G, Vogel P, Neale G, Green DR, et al. HIF1alpha-dependent glycolytic pathway orchestrates a metabolic checkpoint for the differentiation of TH17 and treg cells. J Exp Med (2011) 208(7):1367–76. doi: 10.1084/jem.20110278
138. Budhu S, Schaer DA, Li Y, Toledo-Crow R, Panageas K, Yang X, et al. Blockade of surface-bound TGF-β on regulatory T cells abrogates suppression of effector T cell function in the tumor microenvironment. Sci Signal (2017) 10(494). doi: 10.1126/scisignal.aak9702
139. Komatsu N, Okamoto K, Sawa S, Nakashima T, Oh-hora M, Kodama T, et al. Pathogenic conversion of Foxp3+ T cells into TH17 cells in autoimmune arthritis. Nat Med (2014) 20(1):62–8. doi: 10.1038/nm.3432
140. Moesta AK, Li XY, Smyth MJ. Targeting CD39 in cancer. Nat Rev Immunol (2020) 20(12):739–55. doi: 10.1038/s41577-020-0376-4
141. Schneider E, Winzer R, Rissiek A, Ricklefs I, Meyer-Schwesinger C, Ricklefs FL, et al. CD73-mediated adenosine production by CD8 T cell-derived extracellular vesicles constitutes an intrinsic mechanism of immune suppression. Nat Commun (2021) 12(1):5911. doi: 10.1038/s41467-021-26134-w
142. Chiarella AM, Ryu YK, Manji GA, Rustgi AK. Extracellular ATP and adenosine in cancer pathogenesis and treatment. Trends Cancer (2021) 7(8):731–50. doi: 10.1016/j.trecan.2021.04.008
143. Yang R, Elsaadi S, Misund K, Abdollahi P, Vandsemb EN, Moen SH, et al. Conversion of ATP to adenosine by CD39 and CD73 in multiple myeloma can be successfully targeted together with adenosine receptor A2A blockade. J Immunother Cancer (2020) 8(1). doi: 10.1136/jitc-2020-000610
144. Chalmin F, Mignot G, Bruchard M, Chevriaux A, Végran F, Hichami A, et al. Stat3 and gfi-1 transcription factors control Th17 cell immunosuppressive activity via the regulation of ectonucleotidase expression. Immunity (2012) 36(3):362–73. doi: 10.1016/j.immuni.2011.12.019
145. Young A, Mittal D, Stagg J, Smyth MJ. Targeting cancer-derived adenosine: new therapeutic approaches. Cancer Discov (2014) 4(8):879–88. doi: 10.1158/2159-8290.CD-14-0341
146. Stagg J, Smyth MJ. Extracellular adenosine triphosphate and adenosine in cancer. Oncogene (2010) 29(39):5346–58. doi: 10.1038/onc.2010.292
147. Martin F, Apetoh L, Ghiringhelli F. Controversies on the role of Th17 in cancer: a TGF-β-dependent immunosuppressive activity? Trends Mol Med (2012) 18(12):742–9. doi: 10.1016/j.molmed.2012.09.007
148. Bai A, Robson S. Beyond ecto-nucleotidase: CD39 defines human Th17 cells with CD161. Purinergic Signal (2015) 11(3):317–9. doi: 10.1007/s11302-015-9457-4
149. Takebe N, Nguyen D, Yang SX. Targeting notch signaling pathway in cancer: clinical development advances and challenges. Pharmacol Ther (2014) 141(2):140–9. doi: 10.1016/j.pharmthera.2013.09.005
150. Hu X, Liu X, Moisan J, Wang Y, Lesch CA, Spooner C, et al. Synthetic RORγ agonists regulate multiple pathways to enhance antitumor immunity. Oncoimmunology (2016) 5(12):e1254854. doi: 10.1080/2162402X.2016.1254854
151. Chatterjee S, Thyagarajan K, Kesarwani P, Song JH, Soloshchenko M, Fu J, et al. Reducing CD73 expression by IL1β-programmed Th17 cells improves immunotherapeutic control of tumors. Cancer Res (2014) 74(21):6048–59. doi: 10.1158/0008-5472.CAN-14-1450
152. Punt S, Dronkers EA, Welters MJ, Goedemans R, Koljenović S, Bloemena E, et al. A beneficial tumor microenvironment in oropharyngeal squamous cell carcinoma is characterized by a high T cell and low IL-17(+) cell frequency. Cancer Immunol Immunother (2016) 65(4):393–403. doi: 10.1007/s00262-016-1805-x
153. Haghshenas MR, Khademi B, Faghih Z, Ghaderi A, Erfani N. Immune regulatory cells and IL17-producing lymphocytes in patients with benign and malignant salivary gland tumors. Immunol Lett (2015) 164(2):109–16. doi: 10.1016/j.imlet.2015.02.008
154. Huang M, Bolin S, Miller H, Ng HL. RORγ structural plasticity and druggability. Int J Mol Sci (2020) 21(15). doi: 10.3390/ijms21155329
155. Dong C. Helper T cells and cancer-associated inflammation: a new direction for immunotherapy? J Interferon Cytokine Res (2017) 37(9):383–5. doi: 10.1089/jir.2017.0012
156. Wang B, Li L, Liao Y, Li J, Yu X, Zhang Y, et al. Mast cells expressing interleukin 17 in the muscularis propria predict a favorable prognosis in esophageal squamous cell carcinoma. Cancer Immunol Immunother (2013) 62(10):1575–85. doi: 10.1007/s00262-013-1460-4
157. Chen JG, Xia JC, Liang XT, Pan K, Wang W, Lv L, et al. Intratumoral expression of IL-17 and its prognostic role in gastric adenocarcinoma patients. Int J Biol Sci (2011) 7(1):53–60. doi: 10.7150/ijbs.7.53
158. Jain P, Javdan M, Feger FK, Chiu PY, Sison C, Damle RN, et al. Th17 and non-Th17 interleukin-17-expressing cells in chronic lymphocytic leukemia: delineation, distribution, and clinical relevance. Haematologica (2012) 97(4):599–607. doi: 10.3324/haematol.2011.047316
159. Punt S, van Vliet ME, Spaans VM, de Kroon CD, Fleuren GJ, Gorter A, et al. FoxP3(+) and IL-17(+) cells are correlated with improved prognosis in cervical adenocarcinoma. Cancer Immunol Immunother (2015) 64(6):745–53. doi: 10.1007/s00262-015-1678-4
160. Lin Y, Xu J, Su H, Zhong W, Yuan Y, Yu Z, et al. Interleukin-17 is a favorable prognostic marker for colorectal cancer. Clin Transl Oncol (2015) 17(1):50–6. doi: 10.1007/s12094-014-1197-3
161. Fabre JAS, Giustinniani J, Garbar C, Merrouche Y, Antonicelli F, Bensussan A. The interleukin-17 family of cytokines in breast cancer. Int J Mol Sci (2018) 19(12). doi: 10.3390/ijms19123880
162. Majchrzak K, Nelson MH, Bailey SR, Bowers JS, Yu XZ, Rubinstein MP, et al. Exploiting IL-17-producing CD4+ and CD8+ T cells to improve cancer immunotherapy in the clinic. Cancer Immunol Immunother (2016) 65(3):247–59. doi: 10.1007/s00262-016-1797-6
163. Kolbinger F, Di Padova F, Deodhar A, Hawkes JE, Huppertz C, Kuiper T, et al. Secukinumab for the treatment of psoriasis, psoriatic arthritis, and axial spondyloarthritis: physical and pharmacological properties underlie the observed clinical efficacy and safety. Pharmacol Ther (2022) 229:107925. doi: 10.1016/j.pharmthera.2021.107925
164. Esfahani K, Miller WH Jr. Reversal of autoimmune toxicity and loss of tumor response by interleukin-17 blockade. N Engl J Med (2017) 376(20):1989–91. doi: 10.1056/NEJMc1703047
165. Kryczek I, Wei S, Szeliga W, Vatan L, Zou W. Endogenous IL-17 contributes to reduced tumor growth and metastasis. Blood (2009) 114(2):357–9. doi: 10.1182/blood-2008-09-177360
166. Lv L, Pan K, Li XD, She KL, Zhao JJ, Wang W, et al. The accumulation and prognosis value of tumor infiltrating IL-17 producing cells in esophageal squamous cell carcinoma. PloS One (2011) 6(3):e18219. doi: 10.1371/journal.pone.0018219
167. Lu L, Pan K, Zheng HX, Li JJ, Qiu HJ, Zhao JJ, et al. IL-17A promotes immune cell recruitment in human esophageal cancers and the infiltrating dendritic cells represent a positive prognostic marker for patient survival. J Immunother (2013) 36(8):451–8. doi: 10.1097/CJI.0b013e3182a802cf
168. Martin-Orozco N, Muranski P, Chung Y, Yang XO, Yamazaki T, Lu S, et al. T Helper 17 cells promote cytotoxic T cell activation in tumor immunity. Immunity (2009) 31(5):787–98. doi: 10.1016/j.immuni.2009.09.014
169. Kelchtermans H, Billiau A, Matthys P. How interferon-gamma keeps autoimmune diseases in check. Trends Immunol (2008) 29(10):479–86. doi: 10.1016/j.it.2008.07.002
170. Hu X, Ivashkiv LB. Cross-regulation of signaling pathways by interferon-gamma: implications for immune responses and autoimmune diseases. Immunity (2009) 31(4):539–50. doi: 10.1016/j.immuni.2009.09.002
171. Annunziato F, Cosmi L, Liotta F, Maggi E, Romagnani S. Defining the human T helper 17 cell phenotype. Trends Immunol (2012) 33(10):505–12. doi: 10.1016/j.it.2012.05.004
172. Murphy KM, Stockinger B. Effector T cell plasticity: flexibility in the face of changing circumstances. Nat Immunol (2010) 11(8):674–80. doi: 10.1038/ni.1899
173. Maggi L, Santarlasci V, Capone M, Rossi MC, Querci V, Mazzoni A, et al. Distinctive features of classic and nonclassic (Th17 derived) human Th1 cells. Eur J Immunol (2012) 42(12):3180–8. doi: 10.1002/eji.201242648
174. Basdeo SA, Cluxton D, Sulaimani J, Moran B, Canavan M, Orr C, et al. Ex-Th17 (Nonclassical Th1) cells are functionally distinct from classical Th1 and Th17 cells and are not constrained by regulatory T cells. J Immunol (2017) 198(6):2249–59. doi: 10.4049/jimmunol.1600737
175. Ankathatti Munegowda M, Deng Y, Mulligan SJ, Xiang J. Th17 and Th17-stimulated CD8+ T cells play a distinct role in Th17-induced preventive and therapeutic antitumor immunity. Cancer Immunol Immunother (2011) 60(10):1473–84. doi: 10.1007/s00262-011-1054-y
176. Yu CF, Peng WM, Oldenburg J, Hoch J, Bieber T, Limmer A, et al. Human plasmacytoid dendritic cells support Th17 cell effector function in response to TLR7 ligation. J Immunol (2010) 184(3):1159–67. doi: 10.4049/jimmunol.0901706
177. Xu L, Wang C, Zhou Y, Ren T, Wen Z. CpG oligonucleotides induce the differentiation of CD4(+)Th17 cells by triggering plasmacytoid dendritic cells in adoptively cell transfer immunotherapy. Immunol Lett (2012) 142(1-2):55–63. doi: 10.1016/j.imlet.2011.12.006
178. Guéry L, Dubrot J, Lippens C, Brighouse D, Malinge P, Irla M, et al. Ag-Presenting CpG-activated pDCs prime Th17 cells that induce tumor regression. Cancer Res (2014) 74(22):6430–40. doi: 10.1158/0008-5472.CAN-14-1149
179. Bowers JS, Nelson MH, Majchrzak K, Bailey SR, Rohrer B, Kaiser AD, et al. Th17 cells are refractory to senescence and retain robust antitumor activity after long-term ex vivo expansion. JCI Insight (2017) 2(5):e90772. doi: 10.1172/jci.insight.90772
180. Karpisheh V, Ahmadi M, Abbaszadeh-Goudarzi K, Mohammadpour Saray M, Barshidi A, Mohammadi H, et al. The role of Th17 cells in the pathogenesis and treatment of breast cancer. Cancer Cell Int (2022) 22(1):108. doi: 10.1186/s12935-022-02528-8
181. Ma M, Huang W, Kong D. IL-17 inhibits the accumulation of myeloid-derived suppressor cells in breast cancer via activating STAT3. Int Immunopharmacol (2018) 59:148–56. doi: 10.1016/j.intimp.2018.04.013
Keywords: tumor microenvironment, cancer, Th17, Treg, Th1, IL-17
Citation: Pan Y, Yang W, Tang B, Wang X, Zhang Q, Li W and Li L (2023) The protective and pathogenic role of Th17 cell plasticity and function in the tumor microenvironment. Front. Immunol. 14:1192303. doi: 10.3389/fimmu.2023.1192303
Received: 14 April 2023; Accepted: 19 June 2023;
Published: 29 June 2023.
Edited by:
Varun Sasidharan Nair, Helmholtz Association of German Research Centers (HZ), GermanyReviewed by:
Dhaneshwar Kumar, National Institute of Diabetes and Digestive and Kidney Diseases (NIH), United StatesSung-Min Hwang, Cornell University, United States
Copyright © 2023 Pan, Yang, Tang, Wang, Zhang, Li and Li. This is an open-access article distributed under the terms of the Creative Commons Attribution License (CC BY). The use, distribution or reproduction in other forums is permitted, provided the original author(s) and the copyright owner(s) are credited and that the original publication in this journal is cited, in accordance with accepted academic practice. No use, distribution or reproduction is permitted which does not comply with these terms.
*Correspondence: Li Li, bGlseWRsbXVAaG90bWFpbC5jb20=
†These authors have contributed equally to this work