- 1Department of Sports Rehabilitation, Southwest Medical University, Luzhou, China
- 2Clinical Medical College, Southwest Medical University, Luzhou, China
- 3Department of Specialty Medicine, Ohio University, Athens, OH, United States
- 4Department of Neurosurgery, Wuxi People’s Hospital Affiliated to Nanjing Medical University, Wuxi, China
- 5Division of Basic Biomedical Sciences, The University of South Dakota Sanford School of Medicine, Vermillion, SD, United States
- 6Department of General, Visceral, and Transplant Surgery, Ludwig-Maximilians-University Munich, Munich, Germany
- 7Department of Ophthalmology, Charité – Universitätsmedizin Berlin, Berlin, Germany
- 8Department of Laboratory Medicine, The Affiliated Hospital of Southwest Medical University, Luzhou, China
B cells occupy a vital role in the functioning of the immune system, working in tandem with T cells to either suppress or promote tumor growth within the tumor microenvironment(TME). In addition to direct cell-to-cell communication, B cells and other cells release exosomes, small membrane vesicles ranging in size from 30-150 nm, that facilitate intercellular signaling. Exosome research is an important development in cancer research, as they have been shown to carry various molecules such as major histocompatibility complex(MHC) molecules and integrins, which regulate the TME. Given the close association between TME and cancer development, targeting substances within the TME has emerged as a promising strategy for cancer therapy. This review aims to present a comprehensive overview of the contributions made by B cells and exosomes to the tumor microenvironment (TME). Additionally, we delve into the potential role of B cell-derived exosomes in the progression of cancer.
1 Introduction
Cancer remains a significant global cause of mortality (1). Historically, cancer research solely emphasized studying cancer cells. However, with the introduction of the “seed and soil” concept (2), researchers have redirected their focus towards investigating the development of cancer cells within TME. The TME fosters cancer cell growth and progression through its complex composition of ECM, neuroendocrine cells, immune cells, stromal cells, fibroblasts, and lymphatic networks (3, 4). Immune cells play a significant role in the survival of tumors, as cancer cell metabolites and secretions from specific TME cells can influence the activation, proliferation, differentiation, and function of immune cells (5).
Reports suggest that extracellular vesicles (EVs), particularly exosomes, hold significant potential as a cancer treatment (6). Standard chemotherapy methods may cause harm to normal cells, resulting in detrimental side effects (7, 8). In contrast, Targeted therapy has become a more appealing approach to combatting tumors as it offers greater specificity and can spare adjacent healthy tissues (9). The idea of utilizing exosomes for targeted therapy is intriguing, given that almost all cells produce exosomes (10). Bioengineered exosomes have garnered considerable attention due to their exceptional stability, extensive tissue penetrability, potent targeting capability, and precise drug modulatory properties (11). Although there are no standardized protocols for exosome isolation and purification (12), their potential role in cancer therapy warrants further investigation into their biological functions.
B cells are present in both secondary lymphoid organs (SLOs) and tertiary lymphoid structures(TLSs). TLSs, which represent lymphoid neogenesis sites that occur in most solid tumors (13), primarily consist of B cell follicles and T cell zones, along with mature dendritic cells (DCs) (14, 15). Within these lymphoid structures, there is a rich signaling crosstalk between B cell-derived exosomes and other cells.
2 B cells and the tumor microenvironment
In the past decade, immune cells have gained significant attention in TME research due to their capacity to regulate tumor growth (16). Despite incomplete comprehension of the role of B cells in cancer research, their heterogeneity has been demonstrated as indispensable in the TME (17). B cells are also involved in the formation of TLSs, which has been instrumental in advancing cancer research (18).
2.1 Immunomodulatory functions of B cells
B cells are a component of the adaptive immune system that can differentiate into various subsets when subjected to diverse stimuli and stress conditions (19). The multifaceted nature of B cell subsets, which is primarily due to the absence of a precise definition of their transcription factors, makes comprehending their functions a difficult and complicated process (17).
B cells assume a pivotal role in antibody production and antigen presentation to facilitate effective immune responses among other immune cells (20, 21). After antigen recognition, B cell activation occurs through activation of B cell receptors (BCRs) and Toll-like receptors present on the surface of them. Subsequently, activated B cells differentiate into plasma cells through two distinct pathways. In the first pathway, plasma cells differentiate outside the lymphoid follicle, exhibit lower affinity for antigens, and have shorter lifespans. In the second pathway, B cells migrate into the follicle and establish a germinal center (GC) that differentiates into long-lived plasma cells and memory B cells (22–24). Plasma cells produce IgM antibodies that contribute to humoral immune responses. Moreover, B-cell-associated immunoglobulins, including IgG, IgE, and IgA, have been extensively reviewed in the literature with respect to their subclasses (21). IL-10 promotes plasma cell production through CD40 activation and is superior to IL-4 (25). Recent studies have found that tumour-associated neutrophils in TME rely on TNF-α to recruit B cells and regulate B cell differentiation into plasma cells via the BAFF pathway (26).
Memory B cells exhibit signaling molecules, such as MHC and co-stimulatory molecules, along with cytokines (IL-6, TNF, GM-CSF) on their surface, which stimulate T cells, thereby amplifying the immune response (24). Upon a second exposure to antigens, memory B cells produce high-quality antibodies (27).
B regulatory (Breg) cells are distinct in that their primary function is to suppress the immune system (28). Breg subsets containing IL-10 and IL-35 have the ability to suppress effector T cells (both CD4 and CD8), NK cells, and neutrophils (29). Additionally, Bregs regulate levels of extracellular metabolites such as ATP, ADP, AMP, and adenosine in TME, resulting in the suppression of T and B cell proliferation, forming a complex network (30, 31).
Tumor-infiltrating B cells (TIBs) participate in the development of TLSs, which stimulate an active anti-tumor response via antigen presentation (32, 33). Activated TIBs release enzymes or receptors to kill cancerous cells, leading to their lysis (34). B cells secrete angiogenic factors to stimulate the activation of STAT3 and facilitate angiogenesis (35). In prostate cancer, TIBs may produce lymphotoxin (LT), and high levels of LT can lead to CR-CAP and adversely affect treatment outcomes (36).
2.2 B cells in cancer therapy
Surface markers CD19, CD20, and CD37 are expressed at varying levels by B cells during development. Although studies indicate that targeting these molecules therapeutically holds promise for B-cell cancers (37), their potential efficacy against other cancer types remains largely unexplored. Mediation by CD19 increases antigen presentation by B cells, consequently improving the T cell response (38). Attracting B cells, CXCL13 functions as a chemokine (36, 39). Tumor-induced Bregs (tBregs) serve as a marker of tumor persistence (40). CD20 is expressed on both anti-tumor B cells and tBregs, and targeting CD20 B cells with CXCL13-coupled CpG-ODN can enrich and inactivate tBregs, thereby controlling tumor immune escape (41). This approach minimizes the potential side effects of B-cell depletion methods. In bladder cancer, CXCL13 expression can be used as a surrogate marker for tumor TLSs and correlates with the response to immune checkpoint inhibitors (ICIs) in patients (42). Additionally, in a subset of patients with soft tissue sarcoma, B-cell-enriched TLSs was associated with a better response to anti-PD-1 blockade therapy and increased survival rates (43).
However, the inhibitory mechanisms of tumors mediated by TLSs and B cells in ICI therapy remain poorly understood (44). It is noteworthy that within GCs, B cells are activated to produce antibodies, while Bregs and Tregs produce cytokines such as IL-10, IL-35, and TGF-β to suppress T cell function (18). Furthermore, chemotherapy (45) and vaccination (46) have also demonstrated associations with TLSs.
B cell-enriched TLSs have been found to exhibit tumor-suppressing properties (47). Infiltration of CD19/CD20 B cells has now emerged as a promising target for immunotherapy of hepatocellular carcinoma (48). Thus, combining immunotherapies such as cancer vaccines, cytokine therapies, and immune checkpoint inhibitors (ICIs) with strategies targeting B cells and TLSs may represent promising new anti-tumor approaches.
3 Exosomes and tumor microenvironment
Exosomes facilitate substance transfer between cells, activating signaling pathways (49). The secretion of exosomes by B cells is among the most important ways in which they influence the TME. Additionally, exosomes secreted by other cells and cancer cells also play pivotal roles (50, 51). Therefore, it can be speculated that exosomes hold great potential for advancing the exploration of new therapeutic pathways in cancer (Figure 1A).
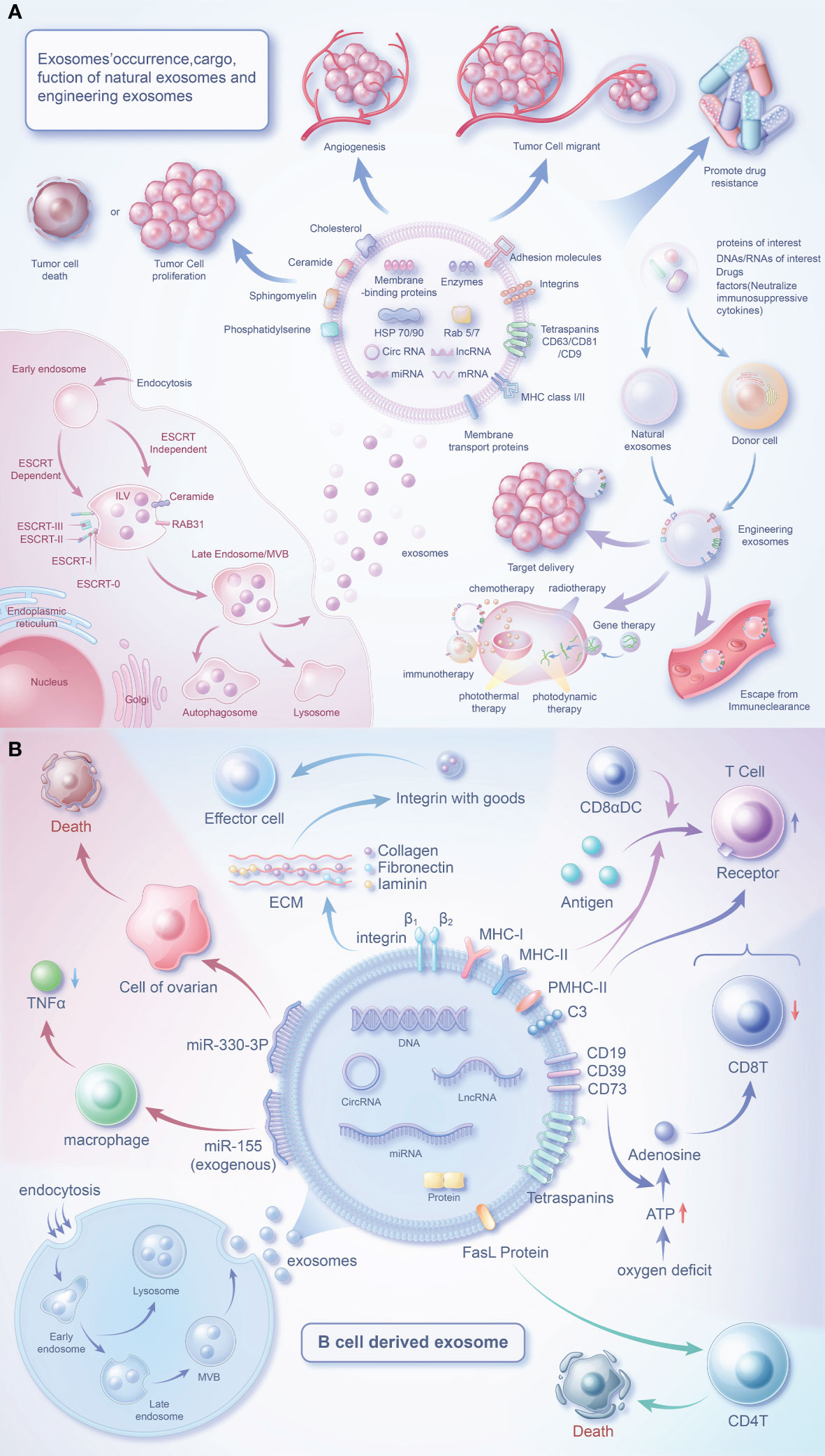
Figure 1 Biology of exosomes and the role of their cargo in the tumor microenvironment. (A) Exosomes are produced in dependence on the ESCRT mechanism or a RAB31-mediated pathway independent of the ESCRT mechanism. Exosomes carry a variety of proteins and effector molecules that can determine the direction of tumour development and tumour metastasis, promote tumour angiogenesis and participate in tumour drug resistance.Engineered exosomes carry cargoes of interest that also have multiple roles closely related to tumours. (B) B cells release exosomes through endocytosis of the plasma membrane, the formation of early endosomes and late endosomes, and fusion of polyvesicular bodies with the plasma membrane. In addition to MHC protein and quadruple transmembrane protein, the surface of B cell-derived exosomes contains special FasL proteins, integrins, C3, CD19, CD39, CD73, etc., which can regulate immune cells (T cells) or affect the survival of tumor cells through special factors.
3.1 The biological characteristics and functions of exosomes
Exosomes, a type of extracellular vesicles, have been extensively studied (52–55). Exosomes are generated through the intracellular process of inward budding of multivesicular bodies, followed by their subsequent release into the extracellular space through fusion events with the plasma membrane (56). The secretion of exosomes is regulated by the RAB family (57, 58). Secretion of exosomes can be divided into an ESCRT mechanism and a RAB31-mediated mechanism independent of ESCRT (59). Despite being previously viewed as waste materials, exosomes are now acknowledged as vital components of the intercellular communication network (60). Exosomes, containing biologically active substances can be found in bodily fluids and are discharged by originating cells (54, 61). Exosomes are structurally more stable than parent cells due to their higher concentration of lipid components (62). CD81 and CD63 are common exosome markers, and along with CD82 and CD37, are highly abundant transmembrane proteins in exosomes. Exosomes also carry a diverse range of membrane signaling proteins (63). These signaling molecules can be delivered to target cells by exosomes (64), making them an integral component of TME communication.
As researchers continue to investigate exosomes, their overall functions have become increasingly evident. In a normal physiological context, exosomes contribute to the maintenance of immune response, cell proliferation, maturation, and homeostasis (65). Exosomes have been identified to play crucial roles in various processes within TME, including but not limited to immune regulation, promotion of cancer cell proliferation, metastasis, drug resistance, and angiogenesis (66, 67).
Exosomes can facilitate crosstalk between B cells and other cells, making them a potent target for cancer treatment (Table 1).
3.2 application of exosomes in cancer therapy
Exosomes are widely recognized as significant biomarkers for cancer diagnosis, prediction, and monitoring (78). Due to their stability and prevalence in circulation, they serve as valuable indicators for liquid biopsy techniques (79), enabling more accurate and less invasive treatments (80). Considering the active cross-talk of exosomes between immune and cancer cells, they can be utilized for immunotherapy to promote (81, 82) or suppress (83, 84) tumor proliferation. Additionally, cancer vaccines based on exosomes have also been developed (85).
Compared to other types of extracellular vesicles (microvesicles, membrane particles, apoptotic bodies), the majority of the biological components, lipids, and proteins of exosomes are relatively clear (86). In comparison to synthetic nanovesicles (polymeric nanoparticles, liposomes, and solid lipid nanoparticles), exosomes possess more membrane proteins, stronger biocompatibility, and a longer circulation half-life naturally (87). They can easily pass through the plasma membrane, blood, and blood-brain barrier (88) to infiltrate tumor tissues (89), which allows for precise and targeted therapeutic interventions (90). Consequently, emerging research is focused on the development and design of bioengineered exosomes.
Bioengineered exosomes can be designed to target specific cells, provide therapeutic cargo, and regulate the immune system (11).
Certain surface-adhesive proteins and carrier ligands allow exosomes to attach to target cell surfaces and efficiently deliver them into the cells (91). Exosomes can efficiently deliver drugs like paclitaxel (92), transport siRNA (93, 94). But the presence of the blood-brain barrier (BBB) limits the access of small molecule drugs to glioma tissue (95). Exosomes derived from brain endothelial cells can transport drugs to BBB, which may become a breakthrough for the treatment of brain cancer (96).
Immune suppressive factors can inhibit the function of immune cells (97–100). By transporting cargo that neutralizes these immune suppressive factors, exosomes can enhance the body’s anti-tumor immune response and improve the efficacy of cancer immunotherapy. An investigation has demonstrated that the T-cell suppressive effects of exosomes can be mitigated by the administration of anti-TGF-β (101). Franz L and colleagues conducted a PD-1 blockade experiment using EV secreted by glioblastoma, which almost reactivated T cells and suppressed tumor progression (102). In the HNSCC microenvironment, the T-cell inhibitory effect of circulating PD-L1 exosomes can be blocked by PD-1 antibodies (103). However, the interference of molecules carried by exosomes in immunotherapy may be related to mechanisms of drug resistance (104, 105).
4 B-cell-derived exosomes and the tumor microenvironment
The investigation of B cell-derived exosomes initiated with the initial characterization of these vesicles in 1996 (106). Through their surface effector molecules, these exosomes can signal to various cells to regulate the TME (Figure 1B).
4.1 The biological components of exosomes released by B cells
Mass spectrometry analysis has uncovered a wealth of constituents in exosomes originating from B cells, including MHC-I, MHC-II, CD20, CD45, and BCR complexes (comprising surface Ig, CD19, and Tetraspanins), as well as chaperones such as heat shock protein 70 and 90, integrins and other proteins (107–109). Furthermore, these exosomes also express cholesterol, sphingomyelin, and ganglioside GM3 (107). Addition of macrophage-derived exosomes to naive monocytes induces cell differentiation (110), however this mechanism is unclear in B cells and B cell exosomes. When B cells are activated, they release a greater amount of exosomes (111, 112), which carry more effector molecules (77, 108, 109). CD45 is a key positive regulator of the BCR-mediated signalling pathway (113, 114) and, interestingly, is absent from T-cell-derived exosomes (115, 116). Triggering the classical NF-κB pathway via downstream of BCR is able to increase HLA expression in B-cell exosomes (111). However, these two regulatory pathways may be linked and need to be explored in further experimental studies.
4.2 Mechanism of involvement of components of B cell-derived exosomes in the tumour process
4.2.1 Proteins
MHC-II molecules in exosomes are predominantly located in SLOs and are expressed exclusively by professional APCs, including B cells (117). The presence of CD20, CD81, and HSC70 is associated with MHC-II on the plasma membrane, which promotes antigen presentation and T cell activation (118). CD20 can form complexes with both MHC-II and CD40 (119), while the R21 monoclonal antibody selectively recognizes CD20 on B cells. Additionally, R21 mAb can induce lfa-1-dependent cell adhesion but inhibits MHC-II-mediated lfa-1-dependent cell adhesion. It’s worth noting that CD40 may interfere with mAb binding to CD20 by competitively binding with it (119).
It is not surprising that exosomes are present in TLSs, as B cells are abundant in these structures (120). CD20 can form complexes with BCR to participate in signal transduction (121), ultimately initiating immune responses. Additionally, CD20 can activate B cells through calcium channels (122), and the resulting stimulated calcium channels may induce the release of a large number of exosomes (108). Some of the mechanisms of CD20 are not yet clear (37). The augmented abundance of CD20-positive B lymphocytes in sentinel lymph nodes is indicative of a favorable prognosis in cases of breast cancer (123). CD20 accumulates at the tumour-liver border in patients with colorectal cancer (124), where it may act as a prognostic marker for the tumour.
These indications suggest that these complexes may be crucial factors in MHC-II or antigen-mediated B cell responses.
B cell-derived exosomes express MHC-II and form a complex (PMHC-II) with peptide antigens. The notion that B-cell exosomal exosomes may transport PMHC-II, proposed more than 20 years ago (106), is confirmed. Exosomes released by most primary B cells express PMHC-II. When antigen-loaded B cells meet specific T cells, B cell activation stimulates exosome release, while stimulating pMHC-II to escape intracellular degradation. pMHC-II interacts with the TCR and activates naive CD4 T cells to initiate an immune response (125). However, exosomes from mature DCs only activate naïve T cells as inefficient APCs (126).
4.2.2 ncRNAs
B-cell exosomes have emerged as viable candidates for the delivery of miRNA-155. When miRNA-155 inhibitor-loaded exosomes were administered to miRNA-155 knockout mice, a notable reduction in TNF-α production was observed in mouse RAW macrophages (77). After treatment with rituximab, a downregulation of miR-155 levels in exosomes was observed (127). This study highlights the potential of B cell-derived exosomes as therapeutic vehicles.
MiR-330-3p has been identified as a key factor in tumor progression. It targets TPX2, which negatively regulates TPX2 expression to inhibit melanoma cell proliferation (128). In addition, miR-330-3p from plasma cell-derived exosomes is a critical regulator of ovarian cancer stroma and promotes tumor metastasis through the JAM2 pathway (74). TPX2 (129) and JAM2 (130, 131) have been described as associated with cancer progression. However, the ability of miR-330-3p to target these proteins may suggest a potentially important role for our B-cell exosome miR-330-3p.
4.3 B cell-derived exosomes are taken up by other cells
4.3.1 Follicular dendritic cells
In vitro isolated B cell-derived exosomes specifically bind follicular dendritic cells (FDC) (132). The fate of B cells in GC depends on the adhesion of the VCAM-1 pathway and is associated with FDC (133). More importantly, FDC themselves do not express MHC-II, but rather pick up peptide-loaded MHC-II on the surface of B cells (134). Exosome binding to FDC may be through interaction with VCAM-1, which then stimulates T helper cells. Additionally, tumor-associated FDCs express CXCL13, which effectively recruits lymphocytes (135). We postulate that in tumor tissues, FDCs may attract B cells, which then bind to the released exosomes, leading to antigen presentation. Therefore, FDCs may represent a physiological target for B cell-derived exosomes (132).
4.3.2 Fibroblasts
Compared to FDCs, fibroblasts exhibit a limited expression of leukocyte adhesion molecules on their surface (132). Treatment of fibroblasts with TNF-α induces the upregulation of ICAM-1 expression on their surface, thereby enhancing the adhesion of B cell-derived exosomes to fibroblast surfaces (136). TNF-α may be a key factor in inducing exosomes to be adsorbed.
4.3.3 Macrophages
In the subcapsular sinus of the lymph node, B-cell-derived exosomes are captured by CD169 macrophages, which then penetrate deep into the paracortex (137). CD169+ cells are present within B-cell follicles (138) and the T-B cell zone boundary of the GC (139). The presentation of antigens by CD169+ cells to CD8 T cells and/or B cells causes them to being activated (140). Activated B cells may release more exosomes. Exosome-induced CTL responses (141) are enhanced in CD169+ mice in cooperation with T and B cells, suggesting that exosomes enter lymphoid organs possibly to reduce the immune response to autoantigens (137).
5 Discussion
In recent years, exosomes have gained increasing attention as important mediators of intercellular communication. While many studies have focused on cancer cell-derived exosomes for diagnosis, prognostic testing, and drug delivery, less is known about exosomes derived from B cells. Fortunately, there are still some relevant studies targeting B-cell-derived exosomes, as outlined in our earlier discussion.
As previously outlined, the effective delivery of miRNA-155 by B cell-derived exosomes has been established. For the delivery of miR-330-3p, researchers may consider the design of a B cell (142) transfected with the V600E mutation. Subsequently, lipid transfection or electroporation can be employed to introduce the miR-330-3p construct into B cells. In addition, the design of a short hairpin RNA (shRNA) or small interfering RNA (siRNA) targeting TPX2 is essential. These molecules should be transfected into B cells along with miR-330-3p for culture, followed by the isolation of exosomes. To enhance the yield of exosomes, researchers can employ additional stimuli, such as TLR3, TLR7, TLR9, and other motifs discussed earlier.It is crucial to emphasize that researchers must continually optimize and thoroughly validate the experimental design at each stage to ensure the exosomes effectively downregulate TPX2 and inhibit melanoma growth. It should be noted that miR-330-3p and TPX2 are merely two components of a complex regulatory network, and their specific roles and effects on tumor growth are contingent upon various factors in the experimental context. Furthermore, although the production of exosomes with multiple engineered cargoes is theoretically feasible, it may induce cellular stress or potentially cytotoxic effects, thereby posing challenges for manipulation.
The study of B-cell-derived exosomes for cancer therapy presents a number of challenges, including inadequate comprehension of their interactions within the complex milieu of TME and insufficient clinical investigations to verify their functionality and potential side effects. We anticipate that with increasing research into B-cell-derived exosomes, their functions and the mechanisms underlying their biological components will soon become clearer.
Author contributions
JX, FY and GT conceived the study. JX, HC and GY drafted the manuscript. JX, SZ, JZ, LT and ZX performed the literature search and collected the data. ZX, FY and GT helped with the final revision of this manuscript. All authors contributed to the article and approved the submitted version.
Funding
This study was supported by grants from the Luzhou Science and Technology Department Applied Basic Research program (No: 2022-JYJ-145), the Sichuan Province Science and Technology Department of foreign (border) high-end talent introduction project (No: 2023JDGD0037), and Sichuan Provincial Medical Association (No: Q22027).
Conflict of interest
The authors declare that the research was conducted in the absence of any commercial or financial relationships that could be construed as a potential conflict of interest.
Publisher’s note
All claims expressed in this article are solely those of the authors and do not necessarily represent those of their affiliated organizations, or those of the publisher, the editors and the reviewers. Any product that may be evaluated in this article, or claim that may be made by its manufacturer, is not guaranteed or endorsed by the publisher.
Supplementary material
The Supplementary Material for this article can be found online at: https://www.frontiersin.org/articles/10.3389/fimmu.2023.1188760/full#supplementary-material
Supplementary Figure 1 | There can be many types of B cells (plasma cells, memory B cells, breg, TIL-B). In their respective ways, they regulate the tumor microenvironment and affect tumor growth.
References
1. Sung H, Ferlay J, Siegel RL, Laversanne M, Soerjomataram I, Jemal A, et al. Global cancer statistics 2020: GLOBOCAN estimates of incidence and mortality worldwide for 36 cancers in 185 countries. CA Cancer J Clin (2021) 71:209–49. doi: 10.3322/caac.21660
2. Paget S. The distribution of secondary growths in cancer of the breast. 1889. Cancer Metastasis Rev (1989) 8:98–101.
3. Chen F, Zhuang X, Lin L, Yu P, Wang Y, Shi Y, et al. New horizons in tumor microenvironment biology: challenges and opportunities. BMC Med (2015) 13:45. doi: 10.1186/s12916-015-0278-7
4. Bejarano L, Jordāo MJC, Joyce JA. Therapeutic targeting of the tumor microenvironment. Cancer Discov (2021) 11:933–59. doi: 10.1158/2159-8290.CD-20-1808
5. Xia L, Oyang L, Lin J, Tan S, Han Y, Wu N, et al. The cancer metabolic reprogramming and immune response. Mol Cancer (2021) 20:28. doi: 10.1186/s12943-021-01316-8
6. Dai J, Su Y, Zhong S, Cong L, Liu B, Yang J, et al. Exosomes: key players in cancer and potential therapeutic strategy. Signal Transduct Target Ther (2020) 5:145. doi: 10.1038/s41392-020-00261-0
7. Qin SY, Zhang AQ, Cheng SX, Rong L, Zhang XZ. Drug self-delivery systems for cancer therapy. Biomaterials (2017) 112:234–47. doi: 10.1016/j.biomaterials.2016.10.016
8. Wei G, Wang Y, Yang G, Wang Y, Ju R. Recent progress in nanomedicine for enhanced cancer chemotherapy. Theranostics (2021) 11:6370–92. doi: 10.7150/thno.57828
9. Ke X, Shen L. Molecular targeted therapy of cancer: the progress and future prospect. Front Lab Med (2017) 1:69–75. doi: 10.1016/j.flm.2017.06.001
10. Choi H, Choi Y, Yim HY, Mirzaaghasi A, Yoo JK, Choi C. Biodistribution of exosomes and engineering strategies for targeted delivery of therapeutic exosomes. Tissue Eng Regener Med (2021) 18:499–511. doi: 10.1007/s13770-021-00361-0
11. Zhang X, Zhang H, Gu J, Zhang J, Shi H, Qian H, et al. Engineered extracellular vesicles for cancer therapy. Adv Mater (2021) 33:e2005709. doi: 10.1002/adma.202005709
12. Yang D, Zhang W, Zhang H, Zhang F, Chen L, Ma L, et al. Progress, opportunity, and perspective on exosome isolation - efforts for efficient exosome-based theranostics. Theranostics (2020) 10:3684–707. doi: 10.7150/thno.41580
13. Dieu-Nosjean MC, Giraldo NA, Kaplon H, Germain C, Fridman WH, Sautès-Fridman C. Tertiary lymphoid structures, drivers of the anti-tumor responses in human cancers. Immunol Rev (2016) 271:260–75. doi: 10.1111/imr.12405
14. Deguchi S, Tanaka H, Suzuki S, Natsuki S, Mori T, Miki Y, et al. Clinical relevance of tertiary lymphoid structures in esophageal squamous cell carcinoma. BMC Cancer (2022) 22:699. doi: 10.1186/s12885-022-09777-w
15. Ruffin AT, Cillo AR, Tabib T, Liu A, Onkar S, Kunning SR, et al. B cell signatures and tertiary lymphoid structures contribute to outcome in head and neck squamous cell carcinoma. Nat Commun (2021) 12:3349. doi: 10.1038/s41467-021-23355-x
16. Yadav D, Puranik N, Meshram A, Chavda V, Lee PC, Jin JO. How advanced are cancer immuno-nanotherapeutics? a comprehensive review of the literature. Int J Nanomed (2023) 18:35–48. doi: 10.2147/IJN.S388349
17. Downs-Canner SM, Meier J, Vincent BG, Serody JS. B cell function in the tumor microenvironment. Annu Rev Immunol (2022) 40:169–93. doi: 10.1146/annurev-immunol-101220-015603
18. Fridman WH, Meylan M, Petitprez F, Sun CM, Italiano A, Sautès-Fridman C. B cells and tertiary lymphoid structures as determinants of tumour immune contexture and clinical outcome. Nat Rev Clin Oncol (2022) 19:441–57. doi: 10.1038/s41571-022-00619-z
19. Ghamlouch H, Ouled-Haddou H, Guyart A, Regnier A, Trudel S, Claisse JF, et al. TLR9 ligand (CpG oligodeoxynucleotide) induces CLL b-cells to differentiate into CD20(+) antibody-secreting cells. Front Immunol (2014) 5:292. doi: 10.3389/fimmu.2014.00292
20. LeBien TW, Tedder TF. B lymphocytes: how they develop and function. Blood (2008) 112:1570–80. doi: 10.1182/blood-2008-02-078071
21. Sharonov GV, Serebrovskaya EO, Yuzhakova DV, Britanova OV, Chudakov DM. B cells, plasma cells and antibody repertoires in the tumour microenvironment. Nat Rev Immunol (2020) 20:294–307. doi: 10.1038/s41577-019-0257-x
22. Kim SS, Sumner WA, Miyauchi S, Cohen EEW, Califano JA, Sharabi AB. Role of b cells in responses to checkpoint blockade immunotherapy and overall survival of cancer patients. Clin Cancer Res (2021) 27:6075–82. doi: 10.1158/1078-0432.CCR-21-0697
23. Palm AE, Henry C. Remembrance of things past: long-term b cell memory after infection and vaccination. Front Immunol (2019) 10:1787. doi: 10.3389/fimmu.2019.01787
24. Cencioni MT, Mattoscio M, Magliozzi R, Bar-Or A, Muraro PA. B cells in multiple sclerosis - from targeted depletion to immune reconstitution therapies. Nat Rev Neurol (2021) 17:399–414. doi: 10.1038/s41582-021-00498-5
25. Rousset F, Peyrol S, Garcia E, Vezzio N, Andujar M, Grimaud JA, et al. Long-term cultured CD40-activated b lymphocytes differentiate into plasma cells in response to IL-10 but not IL-4. Int Immunol (1995) 7:1243–53. doi: 10.1093/intimm/7.8.1243
26. Shaul ME, Zlotnik A, Tidhar E, Schwartz A, Arpinati L, Kaisar-Iluz N, et al. Tumor-associated neutrophils drive b-cell recruitment and their differentiation to plasma cells. Cancer Immunol Res (2021) 9:811–24. doi: 10.1158/2326-6066.CIR-20-0839
27. Packard TA, Cambier JC. B lymphocyte antigen receptor signaling: initiation, amplification, and regulation. F1000Prime Rep (2013) 5:40. doi: 10.12703/P5-40
28. Vitale G, Mion F, Pucillo C. Regulatory b cells: evidence, developmental origin and population diversity. Mol Immunol (2010) 48:1–8. doi: 10.1016/j.molimm.2010.09.010
29. Peng B, Ming Y, Yang C. Regulatory b cells: the cutting edge of immune tolerance in kidney transplantation. Cell Death Dis (2018) 9:109. doi: 10.1038/s41419-017-0152-y
30. Rosser EC, Mauri C. Regulatory b cells: origin, phenotype, and function. Immunity (2015) 42:607–12. doi: 10.1016/j.immuni.2015.04.005
31. Rosser EC, Mauri C. The emerging field of regulatory b cell immunometabolism. Cell Metab (2021) 33:1088–97. doi: 10.1016/j.cmet.2021.05.008
32. Siliņa K, Soltermann A, Attar FM, Casanova R, Uckeley ZM, Thut H, et al. Germinal centers determine the prognostic relevance of tertiary lymphoid structures and are impaired by corticosteroids in lung squamous cell carcinoma. Cancer Res (2018) 78:1308–20. doi: 10.1158/0008-5472.CAN-17-1987
33. Neyt K, Perros F, GeurtsvanKessel CH, Hammad H, Lambrecht BN. Tertiary lymphoid organs in infection and autoimmunity. Trends Immunol (2012) 33:297–305. doi: 10.1016/j.it.2012.04.006
34. Wang SS, Liu W, Ly D, Xu H, Qu L, Zhang L. Tumor-infiltrating b cells: their role and application in anti-tumor immunity in lung cancer. Cell Mol Immunol (2019) 16:6–18. doi: 10.1038/s41423-018-0027-x
35. Yang C, Lee H, Pal S, Jove V, Deng J, Zhang W, et al. B cells promote tumor progression via STAT3 regulated-angiogenesis. PloS One (2013) 8:e64159. doi: 10.1371/journal.pone.0064159
36. Ammirante M, Luo JL, Grivennikov S, Nedospasov S, Karin M. B-cell-derived lymphotoxin promotes castration-resistant prostate cancer. Nature (2010) 464:302–5. doi: 10.1038/nature08782
37. Pavlasova G, Mraz M. The regulation and function of CD20: an “enigma” of b-cell biology and targeted therapy. Haematologica (2020) 105:1494–506. doi: 10.3324/haematol.2019.243543
38. Ding C, Wang L, Marroquin J, Yan J. Targeting of antigens to b cells augments antigen-specific T-cell responses and breaks immune tolerance to tumor-associated antigen MUC1. Blood (2008) 112:2817–25. doi: 10.1182/blood-2008-05-157396
39. Pylayeva-Gupta Y, Das S, Handler JS, Hajdu CH, Coffre M, Koralov SB, et al. IL35-producing b cells promote the development of pancreatic neoplasia. Cancer Discovery (2016) 6:247–55. doi: 10.1158/2159-8290.CD-15-0843
40. He Y, Qian H, Liu Y, Duan L, Li Y, Shi G. The roles of regulatory b cells in cancer. J Immunol Res (2014) 2014:215471. doi: 10.1155/2014/215471
41. Bodogai M, Lee Chang C, Wejksza K, Lai J, Merino M, Wersto RP, et al. Anti-CD20 antibody promotes cancer escape via enrichment of tumor-evoked regulatory b cells expressing low levels of CD20 and CD137L. Cancer Res (2013) 73:2127–38. doi: 10.1158/0008-5472.CAN-12-4184
42. Groeneveld CS, Fontugne J, Cabel L, Bernard-Pierrot I, Radvanyi F, Allory Y, et al. Tertiary lymphoid structures marker CXCL13 is associated with better survival for patients with advanced-stage bladder cancer treated with immunotherapy. Eur J Cancer (2021) 148:181–9. doi: 10.1016/j.ejca.2021.01.036
43. Petitprez F, de Reyniès A, Keung EZ, Chen TW, Sun CM, Calderaro J, et al. B cells are associated with survival and immunotherapy response in sarcoma. Nature (2020) 577:556–60. doi: 10.1038/s41586-019-1906-8
44. Lauss M, Donia M, Svane IM, Jönsson G. B cells and tertiary lymphoid structures: friends or foes in cancer immunotherapy? Clin Cancer Res (2022) 28:1751–8. doi: 10.1158/1078-0432.CCR-21-1130
45. Lu Y, Zhao Q, Liao JY, Song E, Xia Q, Pan J, et al. Complement signals determine opposite effects of b cells in chemotherapy-induced immunity. Cell (2020) 180:1081–1097.e1024. doi: 10.1016/j.cell.2020.02.015
46. Lutz ER, Wu AA, Bigelow E, Sharma R, Mo G, Soares K, et al. Immunotherapy converts nonimmunogenic pancreatic tumors into immunogenic foci of immune regulation. Cancer Immunol Res (2014) 2:616–31. doi: 10.1158/2326-6066.CIR-14-0027
47. Tokunaga R, Naseem M, Lo JH, Battaglin F, Soni S, Puccini A, et al. B cell and b cell-related pathways for novel cancer treatments. Cancer Treat Rev (2019) 73:10–9. doi: 10.1016/j.ctrv.2018.12.001
48. Feng Y, Liu L, Li J, Huang J, Xie JH, Menard L, et al. Systematic characterization of the tumor microenvironment in Chinese patients with hepatocellular carcinoma highlights intratumoral b cells as a potential immunotherapy target. Oncol Rep (2022) 47(2):38. doi: 10.3892/or.2021.8249
49. Gurunathan S, Kang M-H, Jeyaraj M, Qasim M, Kim J-H. Review of the isolation, characterization, biological function, and multifarious therapeutic approaches of exosomes. Cells (2019) 8:307. doi: 10.3390/cells8040307
50. Yan W, Jiang S. Immune cell-derived exosomes in the cancer-immunity cycle. Trends Cancer (2020) 6:506–17. doi: 10.1016/j.trecan.2020.02.013
51. Mashouri L, Yousefi H, Aref AR, Ahadi AM, Molaei F, Alahari SK. Exosomes: composition, biogenesis, and mechanisms in cancer metastasis and drug resistance. Mol Cancer (2019) 18:75. doi: 10.1186/s12943-019-0991-5
52. Samuel M, Gabrielsson S. Personalized medicine and back-allogeneic exosomes for cancer immunotherapy. J Intern Med (2021) 289:138–46. doi: 10.1111/joim.12963
53. Nam GH, Choi Y, Kim GB, Kim S, Kim SA, Kim IS. Emerging prospects of exosomes for cancer treatment: from conventional therapy to immunotherapy. Adv Mater (2020) 32:e2002440. doi: 10.1002/adma.202002440
54. Huang T, Deng CX. Current progresses of exosomes as cancer diagnostic and prognostic biomarkers. Int J Biol Sci (2019) 15:1–11. doi: 10.7150/ijbs.27796
55. Wu M, Wang G, Hu W, Yao Y, Yu XF. Emerging roles and therapeutic value of exosomes in cancer metastasis. Mol Cancer (2019) 18:53. doi: 10.1186/s12943-019-0964-8
56. Zhang L, Yu D. Exosomes in cancer development, metastasis, and immunity. Biochim Biophys Acta Rev Cancer (2019) 1871:455–68. doi: 10.1016/j.bbcan.2019.04.004
57. Ostrowski M, Carmo NB, Krumeich S, Fanget I, Raposo G, Savina A, et al. Rab27a and Rab27b control different steps of the exosome secretion pathway. Nat Cell Biol (2010) 12:19–30. doi: 10.1038/ncb2000
58. Hsu C, Morohashi Y, Yoshimura S, Manrique-Hoyos N, Jung S, Lauterbach MA, et al. Regulation of exosome secretion by Rab35 and its GTPase-activating proteins TBC1D10A-c. J Cell Biol (2010) 189:223–32. doi: 10.1083/jcb.200911018
59. Wei D, Zhan W, Gao Y, Huang L, Gong R, Wang W, et al. RAB31 marks and controls an ESCRT-independent exosome pathway. Cell Res (2021) 31:157–77. doi: 10.1038/s41422-020-00409-1
60. Milane L, Singh A, Mattheolabakis G, Suresh M, Amiji MM. Exosome mediated communication within the tumor microenvironment. J Control Release (2015) 219:278–94. doi: 10.1016/j.jconrel.2015.06.029
61. Tan S, Xia L, Yi P, Han Y, Tang L, Pan Q, et al. Exosomal miRNAs in tumor microenvironment. J Exp Clin Cancer Res (2020) 39:67. doi: 10.1186/s13046-020-01570-6
62. Kalluri R, LeBleu VS. The biology, function, and biomedical applications of exosomes. Science (2020) 367(6478):eaau6977. doi: 10.1126/science.aau6977
63. Pegtel DM, Gould SJ. Exosomes. Annu Rev Biochem (2019) 88:487–514. doi: 10.1146/annurev-biochem-013118-111902
64. Tkach M, Théry C. Communication by extracellular vesicles: where we are and where we need to go. Cell (2016) 164:1226–32. doi: 10.1016/j.cell.2016.01.043
65. Li J, Li S, Guo J, Li Q, Long J, Ma C, et al. Natural product micheliolide (MCL) irreversibly activates pyruvate kinase M2 and suppresses leukemia. J Med Chem (2018) 61:4155–64. doi: 10.1021/acs.jmedchem.8b00241
66. Shao Y, Shen Y, Chen T, Xu F, Chen X, Zheng S. The functions and clinical applications of tumor-derived exosomes. Oncotarget (2016) 7:60736–51. doi: 10.18632/oncotarget.11177
67. Yang K, Zhou Q, Qiao B, Shao B, Hu S, Wang G, et al. Exosome-derived noncoding RNAs: function, mechanism, and application in tumor angiogenesis. Mol Ther Nucleic Acids (2022) 27:983–97. doi: 10.1016/j.omtn.2022.01.009
68. Näslund TI, Gehrmann U, Qazi KR, Karlsson MC, Gabrielsson S. Dendritic cell-derived exosomes need to activate both T and b cells to induce antitumor immunity. J Immunol (2013) 190:2712–9. doi: 10.4049/jimmunol.1203082
69. Segura E, Nicco C, Lombard B, Véron P, Raposo G, Batteux F, et al. ICAM-1 on exosomes from mature dendritic cells is critical for efficient naive T-cell priming. Blood (2005) 106:216–23. doi: 10.1182/blood-2005-01-0220
70. Tay NQ, Lee DCP, Chua YL, Prabhu N, Gascoigne NRJ, Kemeny DM. CD40L expression allows CD8(+) T cells to promote their own expansion and differentiation through dendritic cells. Front Immunol (2017) 8:1484. doi: 10.3389/fimmu.2017.01484
71. Bruno S, Deregibus MC, Camussi G. The secretome of mesenchymal stromal cells: role of extracellular vesicles in immunomodulation. Immunol Lett (2015) 168:154–8. doi: 10.1016/j.imlet.2015.06.007
72. Ye L, Zhang Q, Cheng Y, Chen X, Wang G, Shi M, et al. Tumor-derived exosomal HMGB1 fosters hepatocellular carcinoma immune evasion by promoting TIM-1(+) regulatory b cell expansion. J Immunother Cancer (2018) 6:145. doi: 10.1186/s40425-018-0451-6
73. Schroeder JC, Puntigam L, Hofmann L, Jeske SS, Beccard IJ, Doescher J, et al. Circulating exosomes inhibit b cell proliferation and activity. Cancers (Basel) (2020) 12(8):2110. doi: 10.3390/cancers12082110
74. Yang Z, Wang W, Zhao L, Wang X, Gimple RC, Xu L, et al. Plasma cells shape the mesenchymal identity of ovarian cancers through transfer of exosome-derived microRNAs. Sci Adv (2021) 7(9):eabb0737. doi: 10.1126/sciadv.abb0737
75. Zhang F, Li R, Yang Y, Shi C, Shen Y, Lu C, et al. Specific decrease in b-Cell-Derived extracellular vesicles enhances post-chemotherapeutic CD8(+) T cell responses. Immunity (2019) 50:738–750.e737. doi: 10.1016/j.immuni.2019.01.010
76. Papp K, Végh P, Prechl J, Kerekes K, Kovács J, Csikós G, et al. B lymphocytes and macrophages release cell membrane deposited C3-fragments on exosomes with T cell response-enhancing capacity. Mol Immunol (2008) 45:2343–51. doi: 10.1016/j.molimm.2007.11.021
77. Momen-Heravi F, Bala S, Bukong T, Szabo G. Exosome-mediated delivery of functionally active miRNA-155 inhibitor to macrophages. Nanomedicine (2014) 10:1517–27. doi: 10.1016/j.nano.2014.03.014
78. Zhu L, Sun HT, Wang S, Huang SL, Zheng Y, Wang CQ, et al. Isolation and characterization of exosomes for cancer research. J Hematol Oncol (2020) 13:152. doi: 10.1186/s13045-020-00987-y
79. Ma S, Zhou M, Xu Y, Gu X, Zou M, Abudushalamu G, et al. Clinical application and detection techniques of liquid biopsy in gastric cancer. Mol Cancer (2023) 22:7. doi: 10.1186/s12943-023-01715-z
80. Nikanjam M, Kato S, Kurzrock R. Liquid biopsy: current technology and clinical applications. J Hematol Oncol (2022) 15:131. doi: 10.1186/s13045-022-01351-y
81. Filipazzi P, Bürdek M, Villa A, Rivoltini L, Huber V. Recent advances on the role of tumor exosomes in immunosuppression and disease progression. Semin Cancer Biol (2012) 22:342–9. doi: 10.1016/j.semcancer.2012.02.005
82. Whiteside TL. Immune modulation of T-cell and NK (natural killer) cell activities by TEXs (tumour-derived exosomes). Biochem Soc Trans (2013) 41:245–51. doi: 10.1042/BST20120265
83. Wolfers J, Lozier A, Raposo G, Regnault A, Théry C, Masurier C, et al. Tumor-derived exosomes are a source of shared tumor rejection antigens for CTL cross-priming. Nat Med (2001) 7:297–303. doi: 10.1038/85438
84. Zhang HG, Zhuang X, Sun D, Liu Y, Xiang X, Grizzle WE. Exosomes and immune surveillance of neoplastic lesions: a review. Biotech Histochem (2012) 87:161–8. doi: 10.3109/10520291003659042
85. Zuo B, Zhang Y, Zhao K, Wu L, Qi H, Yang R, et al. Universal immunotherapeutic strategy for hepatocellular carcinoma with exosome vaccines that engage adaptive and innate immune responses. J Hematol Oncol (2022) 15:46. doi: 10.1186/s13045-022-01266-8
86. van der Pol E, Böing AN, Harrison P, Sturk A, Nieuwland R. Classification, functions, and clinical relevance of extracellular vesicles. Pharmacol Rev (2012) 64:676–705. doi: 10.1124/pr.112.005983
87. Mondal J, Pillarisetti S, Junnuthula V, Saha M, Hwang SR, Park IK, et al. Hybrid exosomes, exosome-like nanovesicles and engineered exosomes for therapeutic applications. J Control Release (2023) 353:1127–49. doi: 10.1016/j.jconrel.2022.12.027
88. Liu C, Su C. Design strategies and application progress of therapeutic exosomes. Theranostics (2019) 9:1015–28. doi: 10.7150/thno.30853
89. Prajapati S, Hinchliffe T, Roy V, Shah N, Jones CN, Obaid G. Biomimetic nanotechnology: a natural path forward for tumor-selective and tumor-specific NIR activable photonanomedicines. Pharmaceutics (2021) 13(6):786. doi: 10.3390/pharmaceutics13060786
90. Turturici G, Tinnirello R, Sconzo G, Geraci F. Extracellular membrane vesicles as a mechanism of cell-to-cell communication: advantages and disadvantages. Am J Physiol Cell Physiol (2014) 306:C621–633. doi: 10.1152/ajpcell.00228.2013
91. Batrakova EV, Kim MS. Using exosomes, naturally-equipped nanocarriers, for drug delivery. J Control Release (2015) 219:396–405. doi: 10.1016/j.jconrel.2015.07.030
92. Saari H, Lázaro-Ibáñez E, Viitala T, Vuorimaa-Laukkanen E, Siljander P, Yliperttula M. Microvesicle- and exosome-mediated drug delivery enhances the cytotoxicity of paclitaxel in autologous prostate cancer cells. J Control Release (2015) 220:727–37. doi: 10.1016/j.jconrel.2015.09.031
93. Zhang Y, Li L, Yu J, Zhu D, Zhang Y, Li X, et al. Microvesicle-mediated delivery of transforming growth factor β1 siRNA for the suppression of tumor growth in mice. Biomaterials (2014) 35:4390–400. doi: 10.1016/j.biomaterials.2014.02.003
94. Zhao L, Gu C, Gan Y, Shao L, Chen H, Zhu H. Exosome-mediated siRNA delivery to suppress postoperative breast cancer metastasis. J Control Release (2020) 318:1–15. doi: 10.1016/j.jconrel.2019.12.005
95. Lockman PR, Mittapalli RK, Taskar KS, Rudraraju V, Gril B, Bohn KA, et al. Heterogeneous blood-tumor barrier permeability determines drug efficacy in experimental brain metastases of breast cancer. Clin Cancer Res (2010) 16:5664–78. doi: 10.1158/1078-0432.CCR-10-1564
96. Yang T, Martin P, Fogarty B, Brown A, Schurman K, Phipps R, et al. Exosome delivered anticancer drugs across the blood-brain barrier for brain cancer therapy in danio rerio. Pharm Res (2015) 32:2003–14. doi: 10.1007/s11095-014-1593-y
97. Casey TM, Eneman J, Crocker A, White J, Tessitore J, Stanley M, et al. Cancer associated fibroblasts stimulated by transforming growth factor beta1 (TGF-beta 1) increase invasion rate of tumor cells: a population study. Breast Cancer Res Treat (2008) 110:39–49. doi: 10.1007/s10549-007-9684-7
98. Fridlender ZG, Sun J, Kim S, Kapoor V, Cheng G, Ling L, et al. Polarization of tumor-associated neutrophil phenotype by TGF-beta: “N1” versus “N2” TAN. Cancer Cell (2009) 16:183–94. doi: 10.1016/j.ccr.2009.06.017
99. Chen Y, Zheng X, Wu C. The role of the tumor microenvironment and treatment strategies in colorectal cancer. Front Immunol (2021) 12:792691. doi: 10.3389/fimmu.2021.792691
100. Ren B, Cui M, Yang G, Wang H, Feng M, You L, et al. Tumor microenvironment participates in metastasis of pancreatic cancer. Mol Cancer (2018) 17:108. doi: 10.1186/s12943-018-0858-1
101. Rong L, Li R, Li S, Luo R. Immunosuppression of breast cancer cells mediated by transforming growth factor-β in exosomes from cancer cells. Oncol Lett (2016) 11:500–4. doi: 10.3892/ol.2015.3841
102. Ricklefs FL, Alayo Q, Krenzlin H, Mahmoud AB, Speranza MC, Nakashima H, et al. Immune evasion mediated by PD-L1 on glioblastoma-derived extracellular vesicles. Sci Adv (2018) 4:eaar2766. doi: 10.1126/sciadv.aar2766
103. Theodoraki MN, Yerneni SS, Hoffmann TK, Gooding WE, Whiteside TL. Clinical significance of PD-L1(+) exosomes in plasma of head and neck cancer patients. Clin Cancer Res (2018) 24:896–905. doi: 10.1158/1078-0432.CCR-17-2664
104. Turiello R, Capone M, Morretta E, Monti MC, Madonna G, Azzaro R, et al. Exosomal CD73 from serum of patients with melanoma suppresses lymphocyte functions and is associated with therapy resistance to anti-PD-1 agents. J Immunother Cancer (2022) 10:e004043. doi: 10.1136/jitc-2021-004043
105. Theodoraki MN, Yerneni S, Gooding WE, Ohr J, Clump DA, Bauman JE, et al. Circulating exosomes measure responses to therapy in head and neck cancer patients treated with cetuximab, ipilimumab, and IMRT. Oncoimmunology (2019) 8:1593805. doi: 10.1080/2162402X.2019.1593805
106. Raposo G, Nijman HW, Stoorvogel W, Liejendekker R, Harding CV, Melief CJ, et al. B lymphocytes secrete antigen-presenting vesicles. J Exp Med (1996) 183:1161–72. doi: 10.1084/jem.183.3.1161
107. Wubbolts R, Leckie RS, Veenhuizen PT, Schwarzmann G, Möbius W, Hoernschemeyer J, et al. Proteomic and biochemical analyses of human b cell-derived exosomes. Potential implications their Funct multivesicular body formation. J Biol Chem (2003) 278:10963–72. doi: 10.1074/jbc.M207550200
108. Clayton A, Court J, Navabi H, Adams M, Mason MD, Hobot JA, et al. Analysis of antigen presenting cell derived exosomes, based on immuno-magnetic isolation and flow cytometry. J Immunol Methods (2001) 247:163–74. doi: 10.1016/S0022-1759(00)00321-5
109. Saunderson SC, Schuberth PC, Dunn AC, Miller L, Hock BD, MacKay PA, et al. Induction of exosome release in primary b cells stimulated via CD40 and the IL-4 receptor. J Immunol (2008) 180:8146–52. doi: 10.4049/jimmunol.180.12.8146
110. Ismail N, Wang Y, Dakhlallah D, Moldovan L, Agarwal K, Batte K, et al. Macrophage microvesicles induce macrophage differentiation and miR-223 transfer. Blood (2013) 121:984–95. doi: 10.1182/blood-2011-08-374793
111. Arita S, Baba E, Shibata Y, Niiro H, Shimoda S, Isobe T, et al. B cell activation regulates exosomal HLA production. Eur J Immunol (2008) 38:1423–34. doi: 10.1002/eji.200737694
112. Calvo V, Izquierdo M. Inducible polarized secretion of exosomes in T and b lymphocytes. Int J Mol Sci (2020) 21(7):2631. doi: 10.3390/ijms21072631
113. Penninger JM, Irie-Sasaki J, Sasaki T, Oliveira-dos-Santos AJ. CD45: new jobs for an old acquaintance. Nat Immunol (2001) 2:389–96. doi: 10.1038/87687
114. Hermiston ML, Xu Z, Weiss A. CD45: a critical regulator of signaling thresholds in immune cells. Annu Rev Immunol (2003) 21:107–37. doi: 10.1146/annurev.immunol.21.120601.140946
115. Blanchard N, Lankar D, Faure F, Regnault A, Dumont C, Raposo G, et al. TCR activation of human T cells induces the production of exosomes bearing the TCR/CD3/zeta complex. J Immunol (2002) 168:3235–41. doi: 10.4049/jimmunol.168.7.3235
116. Richmond A, Nf-kappa B. Chemokine gene transcription and tumour growth. Nat Rev Immunol (2002) 2:664–74. doi: 10.1038/nri887
117. Lindenbergh MFS, Stoorvogel W. Antigen presentation by extracellular vesicles from professional antigen-presenting cells. Annu Rev Immunol (2018) 36:435–59. doi: 10.1146/annurev-immunol-041015-055700
118. Buschow SI, van Balkom BW, Aalberts M, Heck AJ, Wauben M, Stoorvogel W. MHC class II-associated proteins in b-cell exosomes and potential functional implications for exosome biogenesis. Immunol Cell Biol (2010) 88:851–6. doi: 10.1038/icb.2010.64
119. Léveillé C, AL-D R, Mourad W. CD20 is physically and functionally coupled to MHC class II and CD40 on human b cell lines. Eur J Immunol (1999) 29:65–74. doi: 10.1002/(SICI)1521-4141(199901)29:01<65::AID-IMMU65>3.0.CO;2-E
120. Munoz-Erazo L, Rhodes JL, Marion VC, Kemp RA. Tertiary lymphoid structures in cancer - considerations for patient prognosis. Cell Mol Immunol (2020) 17:570–5. doi: 10.1038/s41423-020-0457-0
121. Polyak MJ, Li H, Shariat N, Deans JP. CD20 homo-oligomers physically associate with the b cell antigen receptor. dissociation upon receptor engagement and recruitment of phosphoproteins and calmodulin-binding proteins. J Biol Chem (2008) 283:18545–52. doi: 10.1074/jbc.M800784200
122. Bubien JK, Zhou LJ, Bell PD, Frizzell RA, Tedder TF. Transfection of the CD20 cell surface molecule into ectopic cell types generates a Ca2+ conductance found constitutively in b lymphocytes. J Cell Biol (1993) 121:1121–32. doi: 10.1083/jcb.121.5.1121
123. Sato Y, Shimoda M, Sota Y, Miyake T, Tanei T, Kagara N, et al. Enhanced humoral immunity in breast cancer patients with high serum concentration of anti-HER2 autoantibody. Cancer Med (2021) 10:1418–30. doi: 10.1002/cam4.3742
124. Meshcheryakova A, Tamandl D, Bajna E, Stift J, Mittlboeck M, Svoboda M, et al. B cells and ectopic follicular structures: novel players in anti-tumor programming with prognostic power for patients with metastatic colorectal cancer. PloS One (2014) 9:e99008. doi: 10.1371/journal.pone.0099008
125. Muntasell A, Berger AC, Roche PA. T Cell-induced secretion of MHC class II-peptide complexes on b cell exosomes. EMBO J (2007) 26:4263–72. doi: 10.1038/sj.emboj.7601842
126. Knight AM. Regulated release of b cell-derived exosomes: do differences in exosome release provide insight into different APC function for b cells and DC? Eur J Immunol (2008) 38:1186–9. doi: 10.1002/eji.200838374
127. Liao TL, Hsieh SL, Chen YM, Chen HH, Liu HJ, Lee HC, et al. Rituximab may cause increased hepatitis c virus viremia in rheumatoid arthritis patients through declining exosomal MicroRNA-155. Arthritis Rheumatol (2018) 70:1209–19. doi: 10.1002/art.40495
128. Yao Y, Zuo J, Wei Y. Targeting of TRX2 by miR-330-3p in melanoma inhibits proliferation. BioMed Pharmacother (2018) 107:1020–9. doi: 10.1016/j.biopha.2018.08.058
129. Ma Y, Lin D, Sun W, Xiao T, Yuan J, Han N, et al. Expression of targeting protein for xklp2 associated with both malignant transformation of respiratory epithelium and progression of squamous cell lung cancer. Clin Cancer Res (2006) 12:1121–7. doi: 10.1158/1078-0432.CCR-05-1766
130. Arcangeli ML, Frontera V, Bardin F, Thomassin J, Chetaille B, Adams S, et al. The junctional adhesion molecule-b regulates JAM-c-dependent melanoma cell metastasis. FEBS Lett (2012) 586:4046–51. doi: 10.1016/j.febslet.2012.10.005
131. Costa A, Kieffer Y, Scholer-Dahirel A, Pelon F, Bourachot B, Cardon M, et al. Fibroblast heterogeneity and immunosuppressive environment in human breast cancer. Cancer Cell (2018) 33:463–479.e410. doi: 10.1016/j.ccell.2018.01.011
132. Denzer K, van Eijk M, Kleijmeer MJ, Jakobson E, de Groot C, Geuze HJ. Follicular dendritic cells carry MHC class II-expressing microvesicles at their surface. J Immunol (2000) 165:1259–65. doi: 10.4049/jimmunol.165.3.1259
133. Koopman G, Keehnen RM, Lindhout E, Newman W, Shimizu Y, van Seventer GA, et al. Adhesion through the LFA-1 (CD11a/CD18)-ICAM-1 (CD54) and the VLA-4 (CD49d)-VCAM-1 (CD106) pathways prevents apoptosis of germinal center b cells. J Immunol (1994) 152:3760–7. doi: 10.4049/jimmunol.152.8.3760
134. Gray D, Kosco M, Stockinger B. Novel pathways of antigen presentation for the maintenance of memory. Int Immunol (1991) 3:141–8. doi: 10.1093/intimm/3.2.141
135. Vermi W, Lonardi S, Bosisio D, Uguccioni M, Danelon G, Pileri S, et al. Identification of CXCL13 as a new marker for follicular dendritic cell sarcoma. J Pathol (2008) 216:356–64. doi: 10.1002/path.2420
136. Clayton A, Turkes A, Dewitt S, Steadman R, Mason MD, Hallett MB. Adhesion and signaling by b cell-derived exosomes: the role of integrins. FASEB J (2004) 18:977–9. doi: 10.1096/fj.03-1094fje
137. Saunderson SC, Dunn AC, Crocker PR, McLellan AD. CD169 mediates the capture of exosomes in spleen and lymph node. Blood (2014) 123:208–16. doi: 10.1182/blood-2013-03-489732
138. Berney C, Herren S, Power CA, Gordon S, Martinez-Pomares L, Kosco-Vilbois MH. A member of the dendritic cell family that enters b cell follicles and stimulates primary antibody responses identified by a mannose receptor fusion protein. J Exp Med (1999) 190:851–60. doi: 10.1084/jem.190.6.851
139. Martínez-Pomares L, Kosco-Vilbois M, Darley E, Tree P, Herren S, Bonnefoy JY, et al. Fc chimeric protein containing the cysteine-rich domain of the murine mannose receptor binds to macrophages from splenic marginal zone and lymph node subcapsular sinus and to germinal centers. J Exp Med (1996) 184:1927–37. doi: 10.1084/jem.184.5.1927
140. Martinez-Pomares L, Gordon S. CD169+ macrophages at the crossroads of antigen presentation. Trends Immunol (2012) 33:66–70. doi: 10.1016/j.it.2011.11.001
141. Qazi KR, Gehrmann U, Domange Jordö E, Karlsson MC, Gabrielsson S. Antigen-loaded exosomes alone induce Th1-type memory through a b-cell-dependent mechanism. Blood (2009) 113:2673–83. doi: 10.1182/blood-2008-04-153536
Keywords: B cell, B cell-derived exosome, therapy, TME, anti-tumor
Citation: Xiong J, Chi H, Yang G, Zhao S, Zhang J, Tran LJ, Xia Z, Yang F and Tian G (2023) Revolutionizing anti-tumor therapy: unleashing the potential of B cell-derived exosomes. Front. Immunol. 14:1188760. doi: 10.3389/fimmu.2023.1188760
Received: 17 March 2023; Accepted: 22 May 2023;
Published: 05 June 2023.
Edited by:
Harry W. Schroeder, University of Alabama at Birmingham, United StatesReviewed by:
Egest James Pone, University of California, Irvine, United StatesCopyright © 2023 Xiong, Chi, Yang, Zhao, Zhang, Tran, Xia, Yang and Tian. This is an open-access article distributed under the terms of the Creative Commons Attribution License (CC BY). The use, distribution or reproduction in other forums is permitted, provided the original author(s) and the copyright owner(s) are credited and that the original publication in this journal is cited, in accordance with accepted academic practice. No use, distribution or reproduction is permitted which does not comply with these terms.
*Correspondence: Zhijia Xia, WmhpamlhLlhpYUBtZWQudW5pLW11ZW5jaGVuLmRl; Fang Yang, eWFuZy5mYW5nQGNoYXJpdGUuZGU=; Gang Tian, dGlhbmdhbmdAc3dtdS5lZHUuY24=
†These authors have contributed equally to this work