- 1Department of Rheumatology and Immunology, West China Hospital, Sichuan University, Chengdu, China
- 2Rare Diseases Center, West China Hospital, Sichuan University, Chengdu, China
- 3Institute of Immunology and Inflammation, Frontiers Science Center for Disease-related Molecular Network, West China Hospital, Chengdu, China
- 4Department of Respiratory and Critical Care Medicine, Chengdu First People’s Hospital, Chengdu, China
Interstitial lung disease (ILD) is one of the most serious lung complications of connective tissue disease (CTD). The application of proteomics in the past decade has revealed that various proteins are involved in the pathogenesis of each subtype of CTD-ILD through different pathways, providing novel ideas to study pathological mechanisms and clinical biomarkers. On this basis, a multidimensional diagnosis or prediction model is established. This paper reviews the results of proteomic detection of different subtypes of CTD-ILD and discusses the role of some differentially expressed proteins in the development of pulmonary fibrosis and their potential clinical applications.
1 Introduction
Interstitial lung disease (ILD) is a group of common heterogeneous lung diseases characterized by inflammation and fibrosis of lung tissue, which is usually progressive and fatal (1, 2). ILD is one of the most common complications of connective tissue disease (CTD), and studies have shown that approximately 32% of CTD patients have ILD (3). Approximately 30% of ILD cases are associated with CTD, and approximately 15% of ILD patients develop CTD after diagnosis (4). Therefore, the concept of CTD-ILD was proposed to include any diffuse parenchymal lung disease in patients with CTD (5). The CTDs associated with ILD include systemic sclerosis (SSc), rheumatoid arthritis (RA), dermatomyositis (DM), polymyositis (PM), Sjogren’s syndrome (SS), and systemic lupus erythematosus (SLE) (5, 6). ILD in CTD is associated with a number of adverse outcomes, such as reduced quality of life, disability, and death (7–9). Notably, the etiology and pathogenesis of different subtypes of CTD-ILD are not fully understood, hindering accurate diagnosis and precise treatment in clinical practice. Therefore, it is critical to explore the potential pathological mechanism and new diagnostic and therapeutic targets of each CTD-ILD subtype.
Proteomics is a relatively mature “omics” field that refers to the study of protein expression, quantification, localization, function, changes in molecular form, posttranslational modification, conformation, chemical modification, and protein−protein interactions at specific times and under specific conditions (10). In particular, the advent of gel-based and gel-free proteomics techniques and advances in mass spectrometry (MS) allow rapid, unbiased, systematic, and high-throughput identification and quantification of samples of a variety of complex protein mixtures, such as bronchoalveolar lavage fluid (BALF), serum, and lung tissue (11). Therefore, proteomics technology is suitable for exploring the mechanism of complex diseases such as CTD-ILD and helping to clarify pathogenesis and pathological changes, which is vital for establishing accurate clinical diagnosis and prognosis models and identifying therapeutic targets for different CTD-ILD subtypes. Therefore, we summarized the results of proteomic studies on different types of CTD-ILD (Table 1), in addition to the functions of several important proteins and their correlation with CTD-ILD (Table 2), to provide references for future basic research or clinical application of CTD-ILD.
2 Proteomics studies in different CTD-ILD subtypes
2.1 Proteomics studies in RA-ILD
RA is a chronic systemic autoimmune and inflammatory disease characterized by synovitis and vasculitis (33). RA-ILD is a common and serious complication of RA. Approximately 40% to 58% of patients with RA develop ILD (34, 35), and the 5-year mortality rate is estimated at 35% (36). Therefore, it is crucial to determine the etiology, pathogenesis, and prognostic factors of RA-ILD, which can be assisted by proteomics.
To investigate the pathogenesis of RA-ILD, Wu et al. compared the serum proteome of RA patients with or without ILD using SOMA scan analysis and found 234 differentially expressed proteins (DEPs). These DEPs provide a good direction to study the pathogenesis of ILD in patients with RA (13). In clinical practice, RA-ILD is associated with various pulmonary imaging manifestations, such as usual interstitial pneumonia (UIP), nonspecific interstitial pneumonia (NSIP), organizing pneumonia (OP), and lymphocytic interstitial pneumonia (LIP), with each having its treatment and prognosis (37). Suhara et al. analysed the BALF of patients with UIP and OP subtypes of RA-ILD by two-dimensional gel electrophoresis (2-DE) and liquid spectrometry (LC−MS/MS). They found that in BALF, the immunoglobulin k chain level in UIP was significantly higher than that in OP. In addition, α-1 antitrypsin, CRP, haptoglobin β, and surfactant protein A were higher in OP than in UIP. This suggests that these proteins have different pathological mechanisms in different subtypes of RA-ILD. Interestingly, there was no significant difference in gelsolin in BALF, but C-gelsolin and N-gelsolin were significantly increased in UIP (12), which was also observed by Oikonomou et al. in animal models. It is speculated that C-gelsolin and N-gelsolin are gelsolin fragments mediated by caspase-3 (38). Proteomic studies can help to explore the specific molecular mechanism and solve related clinical problems by analyzing RA-ILD with different imaging manifestations.
Proteomics is also of great significance for diagnosing or monitoring RA-ILD. Wu et al. calculated the correlation between DEPs and clinical lung function indicators using a linear regression model and found that two proteins, paired immunoglobulin-like type two receptor-associated neural protein (PIANP) and secretory leukocyte peptidase inhibitor (SLPI), were related to the percentage of carbon monoxide diffusion capacity (13). This finding is significant for the clinical monitoring of disease progression in RA-ILD patients, but its specific practical effect needs to be verified in a large clinical cohort. In addition to this traditional method, Ma et al. used matrix-assisted laser desorption/ionization time-of-flight mass spectrometry (MALDI-TOF-MS) and found an overall change trend of serum protein changes between RA-non-ILD and RA-ILD patients, rather than quantifying the changes in each specific protein. Thirteen protein peaks were detected to be downregulated in RA-ILD patients, and the mass-to-charge value of protein peaks was used to establish the best tree model to distinguish RA-ILD from RA, with a sensitivity of 86.36%, a specificity of 84.09%, and an area under the ROC curve of 0.856 (14). However, the authors did not perform functional analysis of specific DEPs in protein peaks but verified that the model can be established using the overall change trend of proteins to distinguish whether RA patients are complicated with ILD.
In addition, proteomics is also an efficient technology for basic research to explore the treatment of RA-ILD. Wu et al. compared 98 DEPs between IPF and healthy controls. Sixteen proteins increased, while five decreased, and showed similar trends of change in RA-ILD and IPF compared with various control groups. However, four proteins were increased in RA-ILD but not in IPF (13). The results suggest that the pathogenesis of ILD in RA patients is similar to that in IPF patients, but there are also significant differences. These differences in proteins suggest that in the clinical treatment of RA-ILD, in addition to anti-pulmonary fibrosis treatment similar to IPF, other treatments for RA-specific lesions, such as specific autoantibodies and autoinflammation, should also be considered. In the same experiment, gene set enrichment analysis (GSEA) was performed on 234 DEPs between RA and RA-ILD. Signaling receptor binding, extracellular matrix, and negative regulation of proteolysis may play an important role in RA-ILD (13). These may be alternative drug targets for studying RA-ILD patients to strengthen anti-RA-based therapy.
2.2 Proteomics studies in SSc-ILD
SSc is characterized by immune dysregulation leading to inflammation and fibrosis of the skin and multiple internal organs (39). ILD is the most common and serious pulmonary complication of SSc, occurring in 47.0 to 66.4% of SSc patients and accounting for 35% of SSc-related mortality (40, 41). The pathogenesis of SSc-ILD may be multifactorial and is not fully understood.
Rottoli et al. compared the BALF protein composition in SSc-ILD, IPF, and sarcoidosis and found similar changes in many kinds of proteins among the three. Most plasma proteins (complement C3B, transthyretin, A-1-B glycoprotein, and serum retinol-binding protein (SRBP)) were the most highly expressed in sarcoidosis, followed by SSc-ILD, and IPF had the lowest expression. However, locally produced low molecular weight proteins, such as galectin 1, ubiquitin, and thioredoxin peroxidase 2, were more abundant in IPF (15). These findings suggest that the pathological mechanism of SSc-ILD differs from that of IPF. IPF is a generalized fibrotic disease confined to the lungs, while SSc-ILD is a local manifestation of systemic immune-inflammatory disease. In fibrotic lesions, the overexpression of extracellular matrix (ECM) proteins is considered a molecular marker of fibrosis (42). Mullenbrock et al. used LC−MS to specifically detect the protein composition of ECM in the lungs of patients with SSc-ILD and IPF. It was found that the soluble matrix proteins in the ECM of the lungs of SSc-ILD and IPF patients were significantly different compared to those in the healthy control group, but there were no differences in insoluble matrix proteins (25). Interestingly, SSc-ILD and IPF had a high overlap of lung ECM, especially several proteins related to fibrogenesis, such as PLOD2, LUM, POSTN, IGFBP5, and GREM1. The above results suggest that ECM protein signatures may be more reflective of fibrosis and less likely to indicate other SSc-associated pathologies (25).
In addition, Landi’s team found that 14-3-3ϵ was increased in the BALF proteome of SSc-ILD patients compared with nonsmokers (18, 20). In inflammatory and autoimmune diseases, 14-3-3-ϵ, as a component of the TNFR2 complex, restricts the activation of NF-κB through PI3K/Akt/mTOR signaling and stimulates the activation of C/EBP-β, thus guiding the plasticity of macrophages (43). However, studies on 14-3-3ϵ in pulmonary fibrosis, especially in CTD-ILD, are scarce. Similarly, the level of transthyretin in the BALF of patients with SSc-ILD was elevated, as detected by proteomic methods (15, 18, 20). One study found that transthyretin stimulates the production of collagen I and immunoglobulin-binding proteins in fibroblasts, which participate in endoplasmic reticulum stress activation and profibrosis through mitochondrial oxidative stress in cardiac amyloidosis (44). However, its function in CTD-ILD needs further confirmation. In addition to using BALF as a sample, Ryu et al. performed proteomic detection of extracellular vesicles (EVs) precipitated in plasma by LC-MS. The results showed that EVs from SSc-ILD patients contained significantly higher levels of MT-A TP6, and its protein was mainly involved in platelet activation, cell adhesion, and immune responses (23).
Larsen et al. conducted an interesting study on the detection modes. They compared the proteomics results of fibroblasts obtained from BALF and biopsies from SSc-ILD patients and found only three DEPs, indicating that BALF and biopsy fibroblast cultures from SSc-ILD patients were similar in protein composition (17). To some extent, we could use noninvasive BALF to replace biopsied active SSc-ILD-associated proteomic results.
Lung tissue is also an ideal sample type for CTD-ILD proteomic studies. Previous studies have demonstrated a genetic association between connective tissue growth factor (CTGF) and SSc (45, 46). This suggests that subclinical pathologic changes take place in the lungs of SSc patients even without established ILD. In addition, it was found that the response of the proteome of lung fibroblasts in non-ILD SSc patients had an excessive response to CTGF (24). This suggests that subclinical pathologic changes take place in the lungs of SSc patients even without established ILD. In addition to lung tissue or BALF for proteomic study samples from SSc-ILD patients, serum samples with a more extensive and convenient clinical application can be used. Van Bon et al. adopted the SELDI-TOF-MS examination of plasma from SSc patients with and without ILD and showed that S100A8 (calprotectin) levels were significantly increased in patients with SSc-ILD (21), which was consistent with the findings by Fietta et al. using BALF (19). Van Bon’s team also isolated plasmacyte-like dendritic cells from patients’ peripheral blood and used SELDITOF MS to analyze the whole proteome of the supernatant after cell lysis. It was found that SSc patients with higher CXCL4 levels in plasmacyte-like dendritic cell supernatant developed ILD significantly earlier, with a relative decline in forced vital capacity of over 30%, significantly faster decline in lung carbon monoxide diffusion capacity, and bilateral fibrosis on CT (22). Therefore, for long-term follow-up of SSc patients, detecting S100A8 and CXCL4 levels in plasmacytoid dendritic cell supernatant may be an efficient and convenient way to assess the risk of ILD.
The comorbidity rate of ILD in SSc patients is high, but there is still a lack of effective drug treatments, and the therapeutic mechanism of drugs is unclear. Notably, Shirahama et al., through 2-D gel electrophoresis of BALF, found that the number of protein species in SSc patients with ILD was greater than that in patients without ILD. In addition, five proteins were increased, while nine were decreased in patients with SSc-ILD. Specifically, glutathione S-transferase (GST) was increased in each patient with SSc-ILD compared with those without ILD (16). This result is consistent with the findings of Fietta et al. (19). Meanwhile, He et al. reported GST levels in bleomycin-induced pulmonary fibrosis mouse models (47). Strange et al. confirmed that GST contributes to the protection of biological macromolecules from oxidative stress by repairing damage to membrane phospholipids and inhibiting the induction of microsomal peroxidation (48). This point may provide evidence to support the treatment of pulmonary fibrosis with GST inhibitors. Sun et al. decellularized lung tissues from patients with SSc-ILD to obtain the lung cytoskeleton. The protein composition of the cytoskeleton was examined using the bottom-up label-free LC−MS quantitation technique, and periostin in SSc-ILD was found to be similar to previously reported changes in decellularized IPF lungs (49). However, the changes in Fibulin 3, Tinag-like 1, and Elastin were more significant in SSc-ILD (27), and drugs targeting these proteins may be considered SSC-ILD-specific therapies.
2.3 Proteomics studies in idiopathic inflammatory myopathies
Idiopathic inflammatory myopathy (IIM) is an autoimmune disease characterized by skeletal muscle weakness and inflammation, including DM, PM, anti-synthetase syndrome (ASS), and other subtypes. The skeletal muscle is usually affected, and lung changes are represented by ILD, which is the leading cause of death (50). In order to better explore the possible mechanism between the development of ILD in IIM patients, Fernandes-Cerqueira et al. performed proteomic analysis of peripheral serum from IIM and IIM-ILD patients by LC−MS/MS analysis. Among them, the abundance of Fc-agalactosylated glycan of IgG is increased in IIM patients compared to the general population. Intraindividual normalization of the main agalactosylated glycan (FA2) of IgG1 vs FA2-IgG2 was used to distinguish IIM-ILD from IIM-nonILD, which showed that the area under the curve (AUC) of this standard was 88 ± 6%. Moreover, the increase in Fc-agalactosylated glycan was not correlated with other extramuscular manifestations of IIM, further suggesting that the overexpression of Fc-agalactosylated glycan has a lung-specific propensity (28). In the same study, Fernandes-Cerqueira et al. performed a subgroup analysis of IIM with positive anti-Jo1 autoantibodies in the same group of samples and found that the abundance of Fc-glycan contained in JO1-specific IgG was lower than that of total IgG; that is, the proportion of Fc-agalactosylated glycan increased (28). This is consistent with the conclusion of previous studies that anti-Jo1 autoantibodies are common in IIM with ILD (51, 52).
In addition, Passadore et al. used 2-DE and LC−MS/MS to analyze the BALF proteomics of DM/PM patients with ILD. The samples were compared with those of ASS patients with anti-Jo1+ and ILD patients with myositis overlap syndrome. There were 24 specific protein spots among the three groups: nine spots were only present in DM/PM-ILD, three in ASS-ILD, and 12 in myositis overlap syndrome with ILD (29). In this experiment, the authors found that gelsolin was also increased in DM/PM-ILD, which was similar to that found by Suhara et al. in RA-ILD (12). However, Passadore et al. have a different understanding of the role of gelsolin in ILD and are more inclined to believe that gelsolin may maintain the inherent antimicrobial activity of the airway surface (53) and improve airway mucus viscosity by degrading a large amount of filamentous actin released by dead cells during inflammation (54). Similar to the findings of Fietta et al. using BALF of patients with SSc-ILD (19), calgranulin B was also increased in ILD patients with myositis overlap syndrome. Studies have shown that calgranulin B plays a role in endothelial inflammation and participates in the recruitment of white blood cells to the inflammatory site (55, 56), and its expression is related to the inflammatory activity of systemic vasculitis (57). These findings suggest that there may be a common pathological pathway among different CTD-ILD subtypes, but the role of various proteins needs further classification research.
2.4 Proteomics studies in other subtypes of CTD-ILD
Primary Sjögren’s syndrome is a chronic inflammatory autoimmune disease of unknown origin that particularly affects the tear and salivary glands (58). Li et al. also examined serum proteomics in patients with primary Sjogren’s syndrome (pSS) complicated with ILD. Seven protein peaks were significantly different between pSS-ILD and pSS. There were three peaks with ILD high expression and the rest with ILD low expression. Similar to the findings of Ma et al. (14), three differential protein peaks were selected to form a diagnostic model, with a sensitivity of 84% and a specificity of 85.7% (59). These results suggest that searching differential protein peaks using combinations of proteomics and statistical screening can effectively identify alternative diagnostic models of diseases.
Some proteomic studies have not been subdivided into subtypes of CTD-ILD, and the results are of great concern. For instance, cell adhesion molecule 1 (CADM1) was found to be downregulated in CTD-ILD (30). It has been suggested that it is a key adhesion receptor regulating human lung mast cells (HLMCs) and primary human lung fibroblasts (30, 60). SIL1 in DEPs is a nucleotide exchange factor for endoplasmic reticulum (ER) heat shock proteins in eukaryotic cells (61). ER stress is associated with various fibrotic diseases, including cystic fibrosis and idiopathic pulmonary fibrosis (62, 63). N-sulfoglucosamine sulfohydrolase (SGSH) has been reported to be involved in the desulfation of glycosaminoglycan chains on proteoglycans (64). Glycosaminoglycans are important components of lung ECM turnover, and abnormalities in the ECM are one of the main pathologies of pulmonary fibrosis (65). However, the pathological mechanism of the above DEPs in CTD-ILD has not been elucidated. This is also the most important difference between nonoffset proteomics and traditional experiments targeting specific proteins, which can more comprehensively identify the types of proteins that may be involved in the disease and provide more comprehensive and powerful clues for related basic research.
In addition, the correlation between some proteins and the occurrence and development of diseases has been studied more clearly, which can help to diagnose or monitor clinical diseases. Ye et al. detected 132 DEPs in the BALF of CTD-ILD patients using an LC−MS proteomic method. Surfactant protein D (SP-D), a humoral molecule of the congenital immune system, was found (30, 66), and Hant et al. pointed out in their pathological studies that it reflected the status of pulmonary fibrosis and could be used as an alternative indicator to evaluate lung involvement (67). Meanwhile, multiple studies have found that increased SP-D is associated with ILD in patients with SSc, RA, and DM/PM using serum samples (32, 67, 68). The presence of SP-D was also found in the subsynovium and microvascular endothelium of the pannus of the diseased joints in RA patients and was more common than in osteoarthritis patients (69). These findings suggest that the SP-D protein may be involved in the common pathogenesis and development of multiple CTD-ILD, primarily related to fibrosis, and may play a role in extrapulmonary organs. Recently, Bowman et al. explored possible proteins involved in the development of progressive ILD by performing proteomic analysis of peripheral blood from patients with ILD other than IPF, including CTD-ILD. They selected 12 DEPs as biomarkers to construct a risk assessment model for the development of progressive ILD (71). The detection results of CTD-ILD proteomics may not only be used as a guide for targeted research. All DEPs can be divided into a single object for study, and they can also be combined through statistical methods to conduct more direct clinical value transformation.
3 Conclusion
Different CTD-ILD subtypes have different proteomic changes (Figure 1). The development of proteomic detection technology can help obtain relevant data from various samples to the maximum extent, explore specific pathogenesis, and search for clinical diagnosis and treatment biomarkers. However, uncertainties persist about the proteomic detection of many types of CTD-ILD, the changes in the protein composition of CTD-ILD patients before and after treatment, and the specific molecular mechanism of the participation of specific kinds of proteins in CTD-ILD. These questions need to be answered by future proteomic studies based on larger sample cohorts, prospective clinical studies, and sufficient clinical evidence.
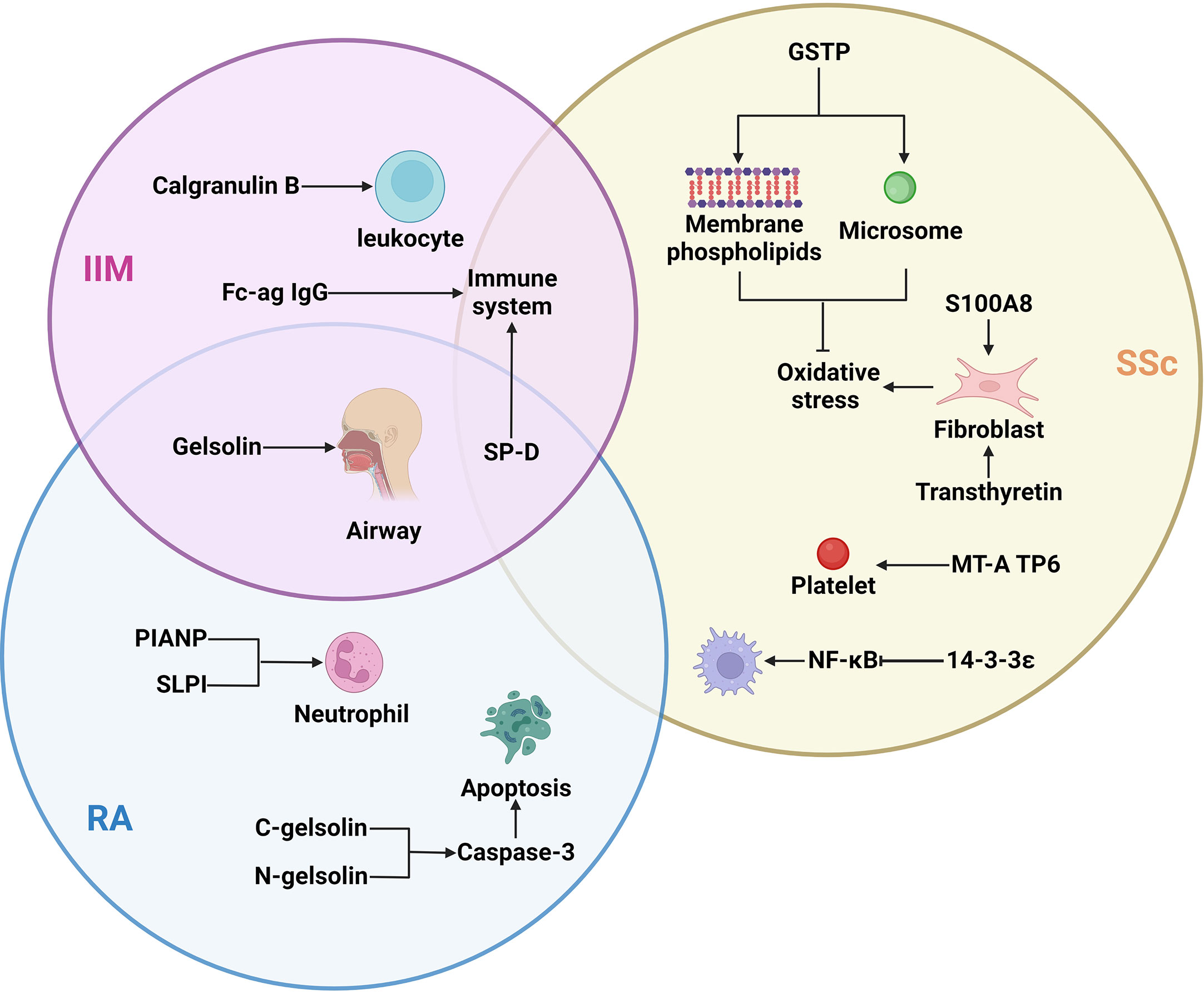
Figure 1 The important proteins of different subtypes of ILD and their target cells/organs: Different subtypes of ILD had different expression proteins, but SP-D differences were found in the three types of ILD, gelsolin differences were found in IIM and RA. IIM, idiopathic inflammatory myopathies; Fc-ag IgG, Fc-agalactosylated glycan of IgG; SP-D, surfactant protein D; SSc, systemic sclerosis; GSTP, glutathione S-transferase P; RA, rheumatoid arthritis; PIANP, paired immunoglobulin-like type two receptor-associated neural protein; SLPI, secretory leukocyte peptidase inhibitor.
Author contributions
YLW and YHL wrote the review. CYT and YL contributed to the theme and structure of the review. YZ, JW, LC, XPL, and TW contributed to the literature search and summary. CYT, YL, and YBL made important modifications to important intellectual content. All authors read and approved the final manuscript.
Funding
This work was supported by the National Natural Science Foundation of China (82001728 and 81973540), 1·3·5 project for Outstanding interdisciplinary project of West China Hospital, Sichuan University (ZYGD18015, ZYJC18003, and ZYJC18024), Sichuan Science and Technology Program (20YYJC3358), and from zero to one Innovative Research Program of Sichuan University (2022SCUH0020).
Conflict of interest
The authors declare that the research was conducted in the absence of any commercial or financial relationships that could be construed as a potential conflict of interest.
Publisher’s note
All claims expressed in this article are solely those of the authors and do not necessarily represent those of their affiliated organizations, or those of the publisher, the editors and the reviewers. Any product that may be evaluated in this article, or claim that may be made by its manufacturer, is not guaranteed or endorsed by the publisher.
Supplementary material
The Supplementary Material for this article can be found online at: https://www.frontiersin.org/articles/10.3389/fimmu.2023.1146904/full#supplementary-material
References
1. Shum AK, Alimohammadi M, Tan CL, Cheng MH, Metzger TC, Law CS, et al. Bpifb1 is a lung-specific autoantigen associated with interstitial lung disease. Sci Trans Med (2013) 5(206):206ra139. doi: 10.1126/scitranslmed.3006998
2. Nakshbandi G, Moor CC, Wijsenbeek MS. Home monitoring for patients with ild and the covid-19 pandemic. Lancet Respir Med (2020) 8(12):1172–4. doi: 10.1016/s2213-2600(20)30452-5
3. Vij R, Strek ME. Diagnosis and treatment of connective tissue disease-associated interstitial lung disease. Chest (2013) 143(3):814–24. doi: 10.1378/chest.12-0741
4. Barnes H, Holland AE, Westall GP, Goh NS, Glaspole IN. Cyclophosphamide for connective tissue disease-associated interstitial lung disease. Cochrane Database systematic Rev (2018) 1(1):Cd010908. doi: 10.1002/14651858.CD010908.pub2
5. Demoruelle MK, Mittoo S, Solomon JJ. Connective tissue disease-related interstitial lung disease. Best Pract Res Clin Rheumatol (2016) 30(1):39–52. doi: 10.1016/j.berh.2016.04.006
6. Singh S, Collins BF, Sharma BB, Joshi JM, Talwar D, Katiyar S, et al. Interstitial lung disease in india. the results of a prospective registry. Am J Respir Crit Care Med (2017) 195(6):801–13. doi: 10.1164/rccm.201607-1484°C
7. Steen VD, Medsger TA. Changes in causes of death in systemic sclerosis, 1972-2002. Ann rheumatic Dis (2007) 66(7):940–4. doi: 10.1136/ard.2006.066068
8. Olson AL, Swigris JJ, Sprunger DB, Fischer A, Fernandez-Perez ER, Solomon J, et al. Rheumatoid arthritis-interstitial lung disease-associated mortality. Am J Respir Crit Care Med (2011) 183(3):372–8. doi: 10.1164/rccm.201004-0622°C
9. Long K, Danoff SK. Interstitial lung disease in polymyositis and dermatomyositis. Clinics chest Med (2019) 40(3):561–72. doi: 10.1016/j.ccm.2019.05.004
10. Scherp P, Ku G, Coleman L, Kheterpal I. Gel-based and gel-free proteomic technologies. Methods Mol Biol (Clifton NJ) (2011) 702:163–90. doi: 10.1007/978-1-61737-960-4_13
11. Aslam B, Basit M, Nisar MA, Khurshid M, Rasool MH. Proteomics: Technologies and their applications. J chromatographic Sci (2017) 55(2):182–96. doi: 10.1093/chromsci/bmw167
12. McInnes IB, Schett G. The pathogenesis of rheumatoid arthritis. New Engl J Med (2011) 365(23):2205–19. doi: 10.1056/NEJMra1004965
13. Spagnolo P, Lee JS, Sverzellati N, Rossi G, Cottin V. The lung in rheumatoid arthritis: Focus on interstitial lung disease. Arthritis Rheumatol (Hoboken NJ) (2018) 70(10):1544–54. doi: 10.1002/art.40574
14. Doyle TJ, Dellaripa PF, Batra K, Frits ML, Iannaccone CK, Hatabu H, et al. Functional impact of a spectrum of interstitial lung abnormalities in rheumatoid arthritis. Chest (2014) 146(1):41–50. doi: 10.1378/chest.13-1394
15. Raimundo K, Solomon JJ, Olson AL, Kong AM, Cole AL, Fischer A, et al. Rheumatoid arthritis-interstitial lung disease in the united states: Prevalence, incidence, and healthcare costs and mortality. J Rheumatol (2019) 46(4):360–9. doi: 10.3899/jrheum.171315
16. Wu X, Jeong Y, Poli de Frías S, Easthausen I, Hoffman K, Oromendia C, et al. Serum proteomic profiling of rheumatoid arthritis-interstitial lung disease with a comparison to idiopathic pulmonary fibrosis. Thorax (2022). doi: 10.1136/thorax-2021-217822
17. Ascherman DP. Interstitial lung disease in rheumatoid arthritis. Curr Rheumatol Rep (2010) 12(5):363–9. doi: 10.1007/s11926-010-0116-z
18. Suhara K, Miyazaki Y, Okamoto T, Ishizuka M, Tsuchiya K, Inase N. Fragmented gelsolins are increased in rheumatoid arthritis-associated interstitial lung disease with usual interstitial pneumonia pattern. Allergology Int (2016) 65(1):88–95. doi: 10.1016/j.alit.2015.08.002
19. Oikonomou N, Thanasopoulou A, Tzouvelekis A, Harokopos V, Paparountas T, Nikitopoulou I, et al. Gelsolin expression is necessary for the development of modeled pulmonary inflammation and fibrosis. Thorax (2009) 64(6):467–75. doi: 10.1136/thx.2008.107946
20. Ma D, Liang N, Zhang L. Establishing classification tree models in rheumatoid arthritis using combination of matrix-assisted laser Desorption/Ionization time-of-Flight mass spectrometry and magnetic beads. Front Med (2021) 8:609773. doi: 10.3389/fmed.2021.609773
21. Cottin V, Brown KK. Interstitial lung disease associated with systemic sclerosis (Ssc-ild). Respir Res (2019) 20(1):13. doi: 10.1186/s12931-019-0980-7
22. Davidsen JR, Miedema J, Wuyts W, Kilpeläinen M, Papiris S, Manali E, et al. Economic burden and management of systemic sclerosis-associated interstitial lung disease in 8 European countries: The buildup Delphi consensus study. Adv Ther (2021) 38(1):521–40. doi: 10.1007/s12325-020-01541-5
23. Tyndall AJ, Bannert B, Vonk M, Airò P, Cozzi F, Carreira PE, et al. Causes and risk factors for death in systemic sclerosis: A study from the eular scleroderma trials and research (Eustar) database. Ann rheumatic Dis (2010) 69(10):1809–15. doi: 10.1136/ard.2009.114264
24. Rottoli P, Magi B, Perari MG, Liberatori S, Nikiforakis N, Bargagli E, et al. Cytokine profile and proteome analysis in bronchoalveolar lavage of patients with sarcoidosis, pulmonary fibrosis associated with systemic sclerosis and idiopathic pulmonary fibrosis. Proteomics (2005) 5(5):1423–30. doi: 10.1002/pmic.200301007
25. Xiao R, Liu FY, Luo JY, Yang XJ, Wen HQ, Su YW, et al. Effect of small interfering rna on the expression of connective tissue growth factor and type I and iii collagen in skin fibroblasts of patients with systemic sclerosis. Br J Dermatol (2006) 155(6):1145–53. doi: 10.1111/j.1365-2133.2006.07438.x
26. Mullenbrock S, Liu F, Szak S, Hronowski X, Gao B, Juhasz P, et al. Systems analysis of transcriptomic and proteomic profiles identifies novel regulation of fibrotic programs by mirnas in pulmonary fibrosis fibroblasts. Genes (2018) 9(12). doi: 10.3390/genes9120588
27. Landi C, Bargagli E, Carleo A, Refini RM, Bennett D, Bianchi L, et al. Bronchoalveolar lavage proteomic analysis in pulmonary fibrosis associated with systemic sclerosis: S100a6 and 14-3-3ϵ as potential biomarkers. Rheumatol (Oxford England) (2019) 58(1):165–78. doi: 10.1093/rheumatology/key223
28. Landi C, Bargagli E, Bianchi L, Gagliardi A, Carleo A, Bennett D, et al. Toward a functional proteomics approach to the comprehension of idiopathic pulmonary fibrosis, sarcoidosis, systemic sclerosis and pulmonary langerhans cell histiocytosis. J Proteomics (2013) 83:60–75. doi: 10.1016/j.jprot.2013.03.006
29. Fu W, Hu W, Yi YS, Hettinghouse A, Sun G, Bi Y, et al. Tnfr2/14-3-3ϵ signaling complex instructs macrophage plasticity in inflammation and autoimmunity. J Clin Invest (2021) 131(16). doi: 10.1172/jci144016
30. Martínez-Martínez E, Fernández-Irigoyen J, Santamaría E, Nieto ML, Bravo-San Pedro JM, Cachofeiro V. Mitochondrial oxidative stress induces cardiac fibrosis in obese rats through modulation of transthyretin. Int J Mol Sci (2022) 23(15). doi: 10.3390/ijms23158080
31. Ryu C, Walia A, Ortiz V, Perry C, Woo S, Reeves BC, et al. Bioactive plasma mitochondrial DNA is associated with disease progression in scleroderma-associated interstitial lung disease. Arthritis Rheumatol (Hoboken NJ) (2020) 72(11):1905–15. doi: 10.1002/art.41418
32. Larsen K, Malmström J, Wildt M, Dahlqvist C, Hansson L, Marko-Varga G, et al. Functional and phenotypical comparison of myofibroblasts derived from biopsies and bronchoalveolar lavage in mild asthma and scleroderma. Respir Res (2006) 7(1):11. doi: 10.1186/1465-9921-7-11
33. Fonseca C, Lindahl GE, Ponticos M, Sestini P, Renzoni EA, Holmes AM, et al. A polymorphism in the ctgf promoter region associated with systemic sclerosis. New Engl J Med (2007) 357(12):1210–20. doi: 10.1056/NEJMoa067655
34. Zhao W, Yue X, Liu K, Zheng J, Huang R, Zou J, et al. The status of pulmonary fibrosis in systemic sclerosis is associated with Irf5, Stat4, Irak1, and ctgf polymorphisms. Rheumatol Int (2017) 37(8):1303–10. doi: 10.1007/s00296-017-3722-5
35. Bogatkevich GS, Ludwicka-Bradley A, Singleton CB, Bethard JR, Silver RM. Proteomic analysis of ctgf-activated lung fibroblasts: Identification of Iqgap1 as a key player in lung fibroblast migration. Am J Physiol Lung Cell Mol Physiol (2008) 295(4):L603–11. doi: 10.1152/ajplung.00530.2007
36. van Bon L, Cossu M, Loof A, Gohar F, Wittkowski H, Vonk M, et al. Proteomic analysis of plasma identifies the toll-like receptor agonists S100a8/A9 as a novel possible marker for systemic sclerosis phenotype. Ann rheumatic Dis (2014) 73(8):1585–9. doi: 10.1136/annrheumdis-2013-205013
37. Fietta A, Bardoni A, Salvini R, Passadore I, Morosini M, Cavagna L, et al. Analysis of bronchoalveolar lavage fluid proteome from systemic sclerosis patients with or without functional, clinical and radiological signs of lung fibrosis. Arthritis Res Ther (2006) 8(6):R160. doi: 10.1186/ar2067
38. van Bon L, Affandi AJ, Broen J, Christmann RB, Marijnissen RJ, Stawski L, et al. Proteome-wide analysis and Cxcl4 as a biomarker in systemic sclerosis. New Engl J Med (2014) 370(5):433–43. doi: 10.1056/NEJMoa1114576
39. Shirahama R, Miyazaki Y, Okamoto T, Inase N, Yoshizawa Y. Proteome analysis of bronchoalveolar lavage fluid in lung fibrosis associated with systemic sclerosis. Allergology Int Off J Japanese Soc Allergology (2010) 59(4):409–15. doi: 10.2332/allergolint.10-OA-0176
40. He N, Bai S, Huang Y, Xing Y, Chen L, Yu F, et al. Evaluation of glutathione s-transferase inhibition effects on idiopathic pulmonary fibrosis therapy with a near-infrared fluorescent probe in cell and mice models. Analytical Chem (2019) 91(8):5424–32. doi: 10.1021/acs.analchem.9b00713
41. Strange RC, Jones PW, Fryer AA. Glutathione s-transferase: Genetics and role in toxicology. Toxicol Lett (2000) 112-113:357–63. doi: 10.1016/s0378-4274(99)00230-1
42. Booth AJ, Hadley R, Cornett AM, Dreffs AA, Matthes SA, Tsui JL, et al. Acellular normal and fibrotic human lung matrices as a culture system for in vitro investigation. Am J Respir Crit Care Med (2012) 186(9):866–76. doi: 10.1164/rccm.201204-0754°C
43. Sun H, Zhu Y, Pan H, Chen X, Balestrini JL, Lam TT, et al. Netrin-1 regulates fibrocyte accumulation in the decellularized fibrotic sclerodermatous lung microenvironment and in bleomycin-induced pulmonary fibrosis. Arthritis Rheumatol (Hoboken NJ) (2016) 68(5):1251–61. doi: 10.1002/art.39575
44. Jee AS, Parker MJS, Bleasel JF, Troy LK, Lau EM, Jo HE, et al. Diagnosis of myositis-associated interstitial lung disease: Utility of the myositis autoantibody line immunoassay. Respir Med (2021) 187:106581. doi: 10.1016/j.rmed.2021.106581
45. Fernandes-Cerqueira C, Renard N, Notarnicola A, Wigren E, Gräslund S, Zubarev RA, et al. Patients with anti-Jo1 antibodies display a characteristic igg fc-glycan profile which is further enhanced in anti-Jo1 autoantibodies. Sci Rep (2018) 8(1):17958. doi: 10.1038/s41598-018-36395-z
46. Ingegnoli F, Lubatti C, Ingegnoli A, Boracchi P, Zeni S, Meroni PL. Interstitial lung disease outcomes by high-resolution computed tomography (Hrct) in anti-Jo1 antibody-positive polymyositis patients: A single centre study and review of the literature. Autoimmun Rev (2012) 11(5):335–40. doi: 10.1016/j.autrev.2011.09.007
47. Liu Y, Luo H, Wang L, Li C, Liu L, Huang L, et al. Increased serum matrix metalloproteinase-9 levels are associated with anti-Jo1 but not anti-Mda5 in myositis patients. Aging Dis (2019) 10(4):746–55. doi: 10.14336/ad.2018.1120
48. Passadore I, Iadarola P, Di Poto C, Giuliano S, Montecucco C, Cavagna L, et al. 2-de and lc-Ms/Ms for a comparative proteomic analysis of balf from subjects with different subsets of inflammatory myopathies. J Proteome Res (2009) 8(5):2331–40. doi: 10.1021/pr800943t
49. Weiner DJ, Bucki R, Janmey PA. The antimicrobial activity of the cathelicidin Ll37 is inhibited by f-actin bundles and restored by gelsolin. Am J Respir Cell Mol Biol (2003) 28(6):738–45. doi: 10.1165/rcmb.2002-0191°C
50. Candiano G, Bruschi M, Pedemonte N, Caci E, Liberatori S, Bini L, et al. Gelsolin secretion in interleukin-4-Treated bronchial epithelia and in asthmatic airways. Am J Respir Crit Care Med (2005) 172(9):1090–6. doi: 10.1164/rccm.200409-1185°C
51. Li C, Li S, Jia C, Yang L, Song Z, Wang Y. Low concentration of S100a8/9 promotes angiogenesis-related activity of vascular endothelial cells: Bridges among inflammation, angiogenesis, and tumorigenesis? Mediators Inflammation (2012) 2012:248574. doi: 10.1155/2012/248574
52. Vogl T, Ludwig S, Goebeler M, Strey A, Thorey IS, Reichelt R, et al. Mrp8 and Mrp14 control microtubule reorganization during transendothelial migration of phagocytes. Blood (2004) 104(13):4260–8. doi: 10.1182/blood-2004-02-0446
53. Viemann D, Strey A, Janning A, Jurk K, Klimmek K, Vogl T, et al. Myeloid-related proteins 8 and 14 induce a specific inflammatory response in human microvascular endothelial cells. Blood (2005) 105(7):2955–62. doi: 10.1182/blood-2004-07-2520
54. Stefanski AL, Tomiak C, Pleyer U, Dietrich T, Burmester GR, Dörner T. The diagnosis and treatment of sjögren's syndrome. Deutsches Arzteblatt Int (2017) 114(20):354–61. doi: 10.3238/arztebl.2017.0354
55. Li YH, Sun XL, He J, Jia RL, Yang DY, Zhang XW, et al. [Screening for serum specific biomarkers in patients with primary sjögren's syndrome and interstitial lung disease using proteomic fingerprint techniques]. Beijing da xue xue bao Yi xue ban = J Peking Univ Health Sci (2012) 44(2):240–3.
56. Ye J, Liu P, Li R, Liu H, Pei W, Ma C, et al. Biomarkers of connective tissue disease-associated interstitial lung disease in bronchoalveolar lavage fluid: A label-free mass spectrometry-based relative quantification study. J Clin Lab Anal (2022) 36(5):e24367. doi: 10.1002/jcla.24367
57. Moiseeva EP, Roach KM, Leyland ML, Bradding P. Cadm1 is a key receptor mediating human mast cell adhesion to human lung fibroblasts and airway smooth muscle cells. PloS One (2013) 8(4):e61579. doi: 10.1371/journal.pone.0061579
58. Ichhaporia VP, Kim J, Kavdia K, Vogel P, Horner L, Frase S, et al. Sil1, the endoplasmic-Reticulum-Localized bip Co-chaperone, plays a crucial role in maintaining skeletal muscle proteostasis and physiology. Dis Models Mech (2018) 11(5). doi: 10.1242/dmm.033043
59. Ruan J, Liang D, Yan W, Zhong Y, Talley DC, Rai G, et al. A small-molecule inhibitor and degrader of the Rnf5 ubiquitin ligase. Mol Biol Cell (2022) 33(13):ar120. doi: 10.1091/mbc.E22-06-0233
60. Dobrinskikh E, Hennessy CE, Kurche JS, Kim E, Estrella AM, Cardwell J, et al. Epithelial er stress enhances the risk of Muc5b associated lung fibrosis. Am J Respir Cell Mol Biol (2022) 68(1):62–74. doi: 10.1165/rcmb.2022-0252°C
61. Harmatz P, Muenzer J, Ezgü F, Dalén P, Huledal G, Lindqvist D, et al. Chemically modified recombinant human sulfamidase (Sobi003) in mucopolysaccharidosis iiia patients: Results from an open, non-controlled, multicenter study. Mol Genet Metab (2022) 136(4):249–59. doi: 10.1016/j.ymgme.2022.06.008
62. Caird R, Williamson M, Yusuf A, Gogoi D, Casey M, McElvaney NG, et al. Targeting of glycosaminoglycans in genetic and inflammatory airway disease. Int J Mol Sci (2022) 23(12). doi: 10.3390/ijms23126400
63. Lin Z, Thorenoor N, Wu R, DiAngelo SL, Ye M, Thomas NJ, et al. Genetic association of pulmonary surfactant protein genes, Sftpa1, Sftpa2, sftpb, sftpc, and sftpd with cystic fibrosis. Front Immunol (2018) 9:2256. doi: 10.3389/fimmu.2018.02256
64. Hant FN, Ludwicka-Bradley A, Wang HJ, Li N, Elashoff R, Tashkin DP, et al. Surfactant protein d and kl-6 as serum biomarkers of interstitial lung disease in patients with scleroderma. J Rheumatol (2009) 36(4):773–80. doi: 10.3899/jrheum.080633
65. Avouac J, Cauvet A, Steelandt A, Shirai Y, Elhai M, Kuwana M, et al. Improving risk-stratification of rheumatoid arthritis patients for interstitial lung disease. PloS One (2020) 15(5):e0232978. doi: 10.1371/journal.pone.0232978
66. Lyu W, Zhou Y, Zhuang Y, Liu Y, Cao M, Xin X, et al. Surfactant protein d is associated with 3-month mortality of anti-Mda5 antibody-interstitial lung disease. Clin Exp Rheumatol (2020) 38(6):1068–74. doi: 10.1164/ajrccm-conference.2020.201.1_MeetingAbstracts.A1093
67. Christensen AF, Sorensen GL, Junker K, Revald PH, Varnum C, Sorensen FB, et al. Localization of surfactant protein-d in the rheumatoid synovial membrane. APMIS Acta pathologica microbiologica immunologica Scandinavica (2018) 126(1):9–13. doi: 10.1111/apm.12785
68. Bowman WS, Newton CA, Linderholm AL, Neely ML, Pugashetti JV, Kaul B, et al. Proteomic biomarkers of progressive fibrosing interstitial lung disease: A multicenter cohort analysis. Lancet Respir Med (2022) 10(6):593–602. doi: 10.1016/s2213-2600(21)00503-8
Keywords: proteomics, interstitial lung disease, connective tissue diseases-interstitial lung disease, differentially expressed proteins, rheumatoid arthritis, systemic sclerosis, idiopathic inflammatory myopathies
Citation: Wu Y, Li Y, Luo Y, Zhou Y, Liang X, Cheng L, Wu T, Wen J, Tan C and Liu Y (2023) Proteomics: Potential techniques for discovering the pathogenesis of connective tissue diseases-interstitial lung disease. Front. Immunol. 14:1146904. doi: 10.3389/fimmu.2023.1146904
Received: 18 January 2023; Accepted: 17 March 2023;
Published: 29 March 2023.
Edited by:
José Delgado Alves, New University of Lisbon, PortugalReviewed by:
Preeti Singh Chauhan, Children’s Hospital of Philadelphia, United StatesFilipe Seguro Paula, Nova University of Lisbon, Portugal
Joana Caetano, Hospital Prof. Doutor Fernando Fonseca, EPE, Portugal
Copyright © 2023 Wu, Li, Luo, Zhou, Liang, Cheng, Wu, Wen, Tan and Liu. This is an open-access article distributed under the terms of the Creative Commons Attribution License (CC BY). The use, distribution or reproduction in other forums is permitted, provided the original author(s) and the copyright owner(s) are credited and that the original publication in this journal is cited, in accordance with accepted academic practice. No use, distribution or reproduction is permitted which does not comply with these terms.
*Correspondence: Chunyu Tan, YW5uYXF1aW50ZXNzZW5jZUAxNjMuY29t; Yi Liu, eWlsaXU4OTk5QHdjaHNjdS5jbg==
†These authors have contributed equally to this work