- 1Department of the Second Medical Oncology, The Third Affiliated Hospital of Kunming Medical University, Kunming, Yunnan, China
- 2Department of Emergency Medicine, The University of Texas MD Anderson Cancer Center, Houston, Texas, TX, United States
Immune checkpoint inhibitors (ICIs) are approved as the first-line drug for treating many cancers and has shown significant survival benefits; however, it also causes immune-related adverse events (irAEs) while activating the immune system, involving multiple organs. Among them, cardiovascular immune-related adverse events (CV-irAE) are rare, but common causes of death in ICIs treated cancer patients, which manifest as myocardial, pericardial, vascular and other cardiovascular toxicities. Therefore, it is important that irAEs, especially CV-irAE should be carefully recognized and monitored during the whole ICIs treatment because early detection and treatment of CV-irAE can significantly reduce the mortality of such patients. Consequently, it is urgent to fully understand the mechanism and management strategies of CV-irAE. The effects of ICIs are multifaceted and the exact mechanism of CV-irAE is still elusive. Generally, T cells identify tumor cell antigens as well as antigen in cardiomyocytes that are the same as or homologous to those on tumor cells, thus causing myocardial damage. In addition, ICIs promote formation of cardiac troponin I (cTnI) that induces cardiac dysfunction and myocardial dilatation; moreover, ICIs also increase the production of cytokines, which promote infiltration of inflammation-linked molecules into off-target tissues. Currently, the management and treatment of cardiovascular toxicity are largely dependent on glucocorticoids, more strategies for prevention and treatment of CV-irAE, such as predictive markers are being explored. This review discusses risk factors, potential pathophysiological mechanisms, clinical manifestations, and management and treatment of CV-irAE, guiding the development of more effective prevention, treatment and management strategies in the future.
1 Introduction
During tumorigenesis, tumor cells inhibit the activation and effector process of T cells by hijacking immune checkpoints molecules, then evade the surveillance and attack of the immune system. Thus, immune checkpoint related to the regulation of T-cell activity is an important target for anti-tumor therapy (1). Tumor microenvironmental factors also modify the anti-tumor immune response, such as T-cell infiltration and expression of immune checkpoint proteins (2). Currently, the main immune checkpoints include cytotoxic T-lymphocyte antigen 4 (CTLA-4), programmed cell death receptor 1 (PD-1), programmed cell death ligand 1 (PD-L1) and lymphocyte activation gene 3 protein (LAG3). Immune checkpoint inhibitors (ICIs) are now approved for treating many malignancies and significantly prolonged the survival of cancer patients (3–6). At the beginning of ICIs application, reports of immune-related adverse events (irAEs) were rare and did not attract broad attention. However, with the rapidly increased use of ICIs and the improvement of patients’ survival, the importance of cardiovascular immune-related adverse events (CV-irAE) therapy has come to the forefront. Despite its low incidence, immune-related adverse events (CV-irAEs) require high attention from clinicians (7). Therefore, through exploring the underlying mechanisms of CV-irAE, we developed more effective prevention, treatment, and management strategies, thus improving the quality of life and patients’ survival. Herein, we review the pharmacological mechanisms of ICIs, current research progression in CV-irAEs epidemiology, risk factors, potential pathophysiological mechanisms as well as clinical manifestation, the management and treatment of CV-irAEs mentioned in guidelines and literatures. The above statements are gross generalizations based on our synthesis of the current litereature.Some statements are not accepted by all, but most of them are based on guidelines published by prestigious professional organizations.
2 Epidemiology
Current reports about epidemiology of CV-irAE are limited because of its low incidence (8). CV-irAEs occur as early as a few days after ICIs initiation, but may also present late until one year after ICIs treatment, the median onset time of CV-irAE was 34 days after starting ICIs (9, 10). In a Danish national study, patients with lung cancer and malignant melanoma had a higher risk rate of CV-irAE in patients treated with ICIs than those who did not receive ICIs therapy (11). Wang et al. (12) performed a retrospective analysis of published irAEs queried in the pharmacovigilance database (Vigilyze) and found that myocarditis had the highest fatality rate among all CV-irAEs (39.7%). Rubio et al. analyzed 1265 papers published before August 31, 2020 and found the total incidence of CV-irAE was about 1.3%, among them myocarditis was the most common irAE, accounting for 50.8%. Notably, a high mortality rate of 24.6% of patients died due to CV-irAE (13). In this study, ICIs included ipilimumab, tremelimumab, nivolumab, pembrolizumab, atezolizumab, durvalumab and avelumab. In addition to these ICIs, there are emerging ICIs, which may also occur CV-irAEs such as relatlimab, a emerging monoclonal antibody that targets LAG-3, relatlimab had a higher incidence in myocarditis (14, 15). Since relatlimab has been approved soon, relatlimab related cardiotoxicity needs to be further explored. The incidence of CV-irAE appears to increase in recent years, probably due to the increased scope and frequency of use of ICIs and the heightened awareness of cardiotoxicity (16–18). However, the real-world prevalence of CV-irAE may be higher than expected, and we currently lack the support of large-sample clinical studies that could offer further in-depth investigation (9, 17).
3 Risk factors for CV-irAE
The risk factors of CV-irAE need further investigation, dual ICIs combination therapy is the greatest risk factor for CV-irAE over other risk factors such as autoimmune diseases (19). Several investigations have also confirmed that dual ICI leads to a higher incidence of CV-irAE than monotherapy or ICI plus chemotherapy (19, 20). A meta-analysis of CV-irAE concluded that the incidence was 3.1% for ICI monotherapy, 2.5% for ICI plus chemotherapy and 5.8% for dual ICIs treatment (anti-PD-1 plus anti-CTLA-4/anti-PD-1 plus anti-PD-L1) (13). The emerging bispecific antibody also causes CV-irAE. The incidence of CV-irAE is 0.9% in 458 patients treated with Cadonilimab (anti-PD-1/CTLA-4) (21). Cardiotoxicity of AK112 (NCT04047290)—anti-PD-1/VEGF and IBI318(NCT03875157)—anti-PD-1/PD-L1 has not been reported.
It was demonstrated that the PD-1 modulates radiation-induced cardiotoxicity in an animal model, acute toxicity was increased with anti-PD-1 treatment in mice with radiotherapy, but further research is needed to get a deep insight (22). Osaka Medical School in Japan established a mouse model of experimental autoimmune myocarditis (EAM) by administration of PD-1 antibodies in mice (23). The study indicated that ICIs-induced autoimmune myocarditis may be related to autoimmunity prior to ICIs administration (23). CV-irAE is more frequently reported in patients diagnosed with autoimmune diseases (24). In a retrospective case-match control study comparing 251 ICI-treated patients who had autoimmune diseases with 251 ICI-treated patients who did not have autoimmune diseases, the risk of CV-irAEs was higher in patients with autoimmune diseases than those without (hazard ratio:1.77) (25).
In addition, the observation of sporadic ICIs-associated myocarditis cases revealed that patients with diabetes were more common in these cases (9, 26). In addition, the patients’ pre-existing cardiovascular risk factors (age ≥80 years, hypertension, diabetes mellitus and chronic kidney disease) and the presence of cardiovascular toxicity caused by previous anti-neoplastic drugs should also be brought to our attention (27). Comparing 35 patients who had ICIs-related myocarditis with 105 ICIs-treated patients who did not have ICIs-related myocarditis, 34% of patients with ICIs-related myocarditis had pre-existing diabetes but only 13% of ICIs-treated patients without myocarditis had diabetes (28).
4 Mechanism of CV-irAE
4.1 Pharmacological mechanism of ICIs
The immune system plays an important role in the surveillance and wiping malignant cells. T cells undergo positive and negative selection in thymic to ensure self-tolerance and specific recognition of abnormal cells (including cancer cells) (29). Tumor cells presenting or releasing tumor antigens are engulfed by antigen presenting cells (APCs), which process tumor antigens and present MHC-I and MHC-II molecular complexes to CD8+ T-cell and CD4+ T-cell receptors then accurately identify cancer cells. A combination of B7, on the surface of APCs, and CD28, on the surface of T cells, constitute synergistic signals in T cells activation, the combination of CD28-B7 lead to cytoskeleton remodelling, cytokines secretion and T cells differentiation. Activated CD4+ T cells secrete cytokines to stimulate CD8+ T cells proliferation in lymph nodes. Activated CD8+ T cells can reach the tumor through circulation, recognize the MHC-I molecular complex on the tumor cells, and kill tumor cells (30–33). Activated CTLA-4, PD-1 and LAG-3 to protect the host from self-attack by abnormally activated T cells (6, 34, 35). CTLA-4, a CD28 homolog, has stronger affinity than CD28, and can induce trans-endocytosis of B7 ligands to reduce the co-stimulatory signal (36–38). PD-1, combined with PD-L1, negatively mediates T cell proliferation and activation (39, 40). CTLA-4 not only competes with CD28 for B7 but also induces regulatory T cells (Treg, inhibitory immune cells) to death, leading to unbalance between Treg and cytotoxic T cells (41, 42). CTLA-4 monoclonal antibody clears Treg in tumor effectively through FcR mediated ADCC (antibody-dependent cell-mediated cytotoxicity), thus relieving immunosuppressive of Treg to achieve anti-tumor (42–44). However, Treg cells are important in peripheral tolerance (45). Reducing peripheral Treg cells lead to the immune system attacking organism, resulting in serious side effects (45, 46). PD-1 plays an important role in T-cell homeostasis and inflammatory inhibition in peripheral tissues (34, 47). Lymphocyte activation gene 3 protein (LAG3) is a negative immunomodulator that regulates the function of T cells and dendritic cells (DC) by binding with MHC-II (6). LAG-3 has an intracellular short tail domain that inhibits the function of LAG-3 in effector CD4+ T cells and an extracellular domain similar to CD4 but possess higher affinity to combine with MHC-II than CD4 (6, 48). FGL1, the ligand of LAG-3, expressed on the surface of cancer cells. When FGL1 combines with LAG-3 on the surface of T cells, immune system mistake cancer cells as normal, contributing to immune-escape of tumor cells (49). After immunoediting (50–52), tumor cells would also express immune checkpoint, so ICIs are designed to reactivate anti-tumor immune response by targeting specific immune checkpoint (Figure 1). Therefore, CTLA-4, PD-1, PD-L1and LAG3 inhibitors have been approved for clinical treatment in several cancer types by Food and Drug Administration (FDA) (1, 53). In addition, new-type ICIs through targeting inhibitory receptors [e.g,. T cell immunoglobulin domain and mucin domain-3 (TIM-3), T cell Ig and ITIM domain (TIGIT) and BTLA (CD272)] and ligand of the B7 family [e.g., V-domain Ig suppressor of T cell activation (VISTA), B7-H3] are being actively investigating and developed for clinical trials in increasing numbers (54–57).
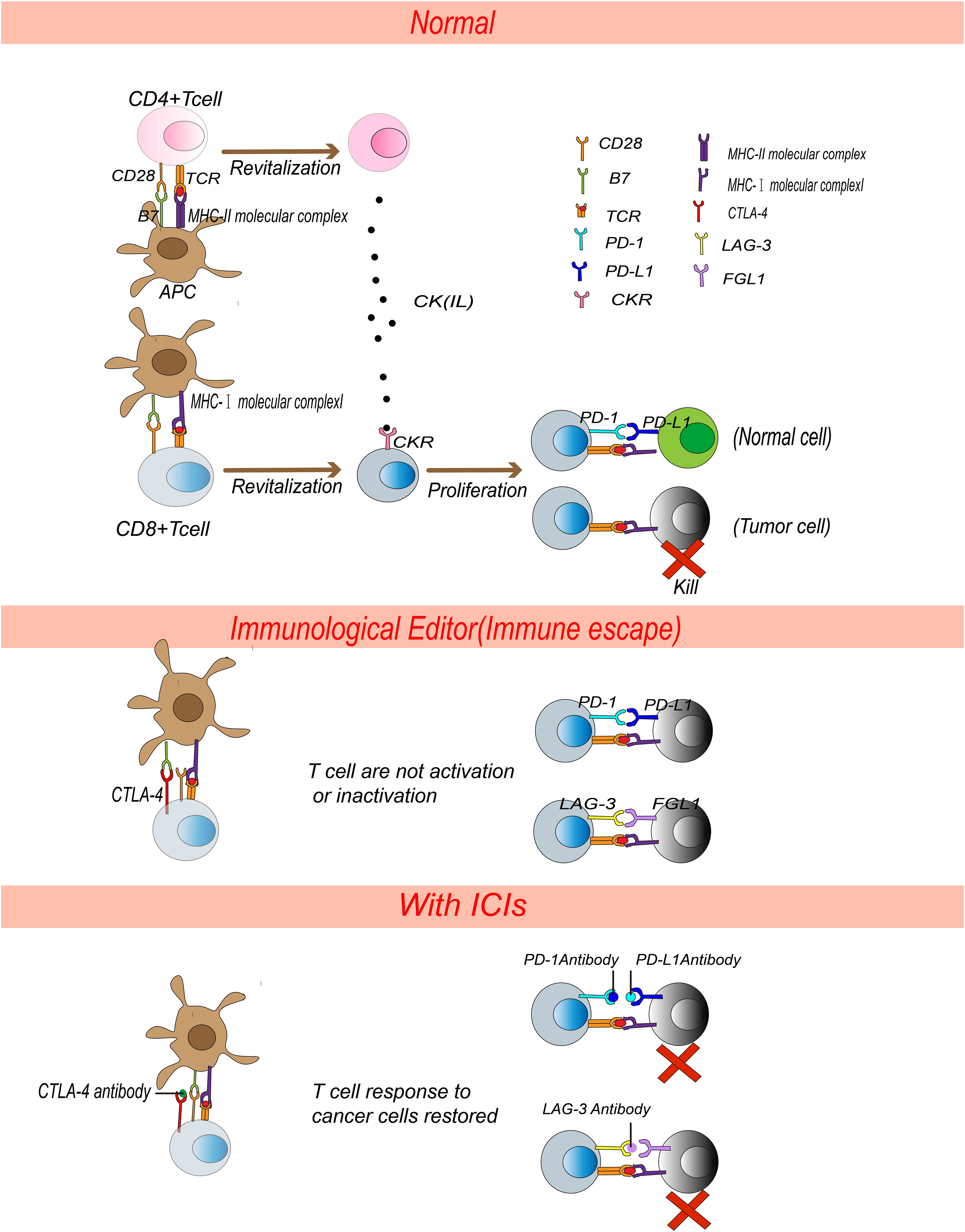
Figure 1 Pharmacological mechanism of ICIs. APCs present MHC molecular complexes to TCR on T cells and activate T cells. CD4+ T cells secrete cytokines and stimulate CD8+ T cells proliferation. Activated CD8+ T cells kill tumor cells precisely. Normally, PD-L1 binds to PD-1, FGL-1 binds to LAG-3, inactivating CD8+ T cells and leading to autoimmune tolerance. After immunoediting, tumor cells express PD-L1 and FGL-1 and T cells express CTLA-4 and LAG-3, receptors on T cells bound with ligands on tumor cells or APCs, which will inactivate T cells. ICIs devitalized the PD-1/PD-L1, LAG-3/FGL-1 and CTLA-4/B7 signals and reactivated T cells to kill tumor cells.
4.2 Potential pathophysiological mechanisms of CV-irAEs
The mechanism of CV-irAE might be ICIs disrupt the autoimmune tolerance of myocardial cell (58). irAEs are reversible in most cases treated appropriately; however, heart is a vital organ so CV-irAE can be fatal (20, 59). Though the effects of ICIs are multifaceted, the exact mechanisms of CV-irAE are still elusive (52) (Figure 2).
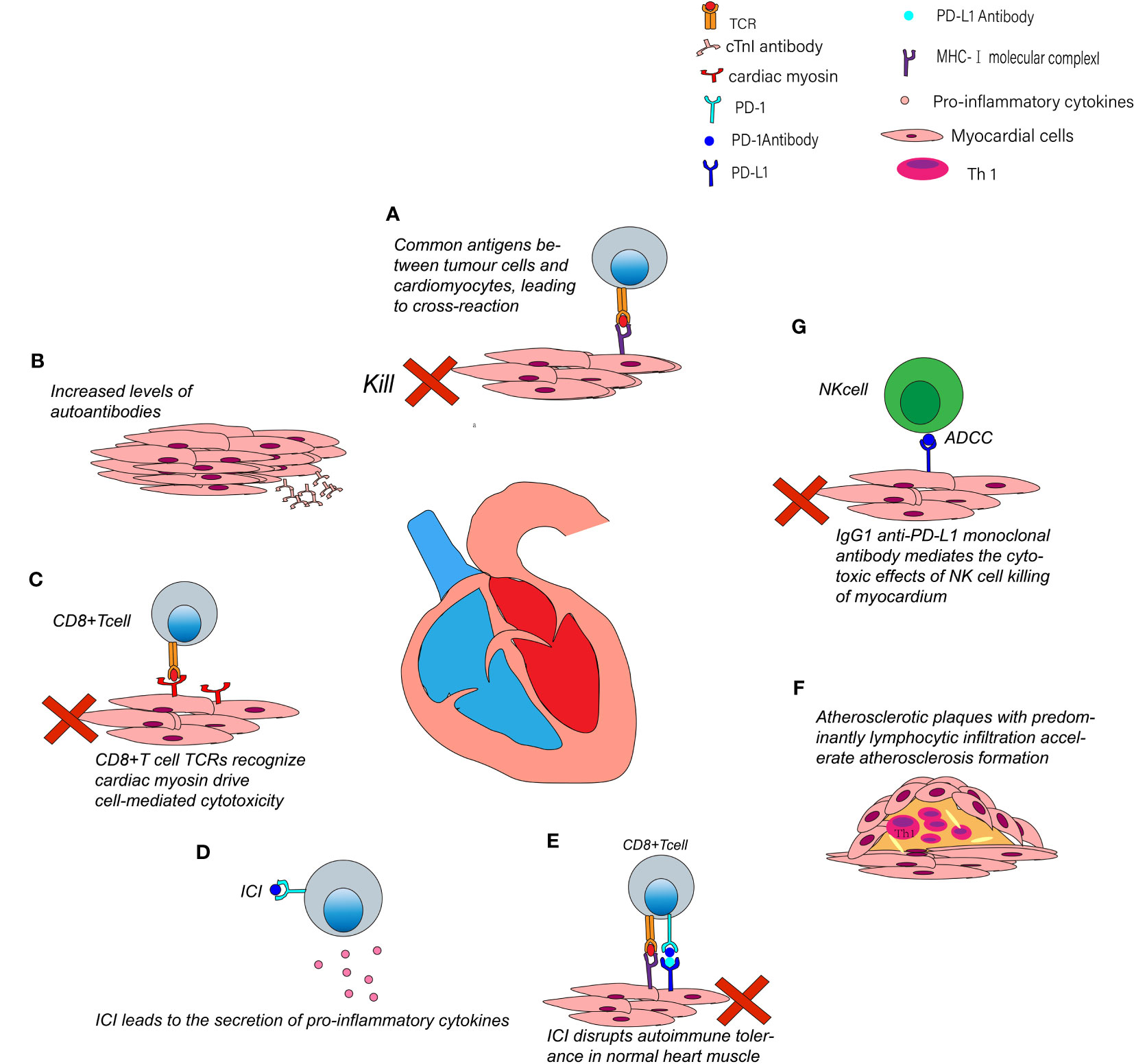
Figure 2 Possible mechanisms of CV-irAE. (A) Activated T cells not only attack tumor cells but also cross-reactivate with cardiac muscle. (B) Cardiac myocytes secrete cTnI antibodies after using ICIs. (C) Myosin-specific T cells TCRs can recognize myosin and drive cytotoxic T-cell-mediated killing. (D) ICIs can lead to increased levels of pro-inflammatory cytokines. (E) Systemic application of ICI may disrupt immune homeostasis between cytotoxic T cells and Tregs. (F) ICIs may contribute to plaques progression and coronary events. (G) Anti-PD-L1 monoclonal antibodies may mediate NK cells killing cardiomyocytes through the ADCC pathway.
4.2.1 The common antigens in tumor cells and cardiomyocytes leading to cross-reaction
T cells identify tumor cell antigens as well as antigen in cardiomyocytes same with or homologous to those on tumor cells simultaneously. In two cases of fulminant myocarditis caused by ICIs, postmortem found that T cell marker (CD3) was positive in myocardial and skeletal muscle infiltrating cells. T cells receptor sequence revealed that patients had high frequency of shared T cell receptor sequences in cardiac and skeletal muscle and tumor infiltrating cells (20). Taken together, these suggest that activated T cells not only attacked tumor cells but also caused cross-reaction with common antigens on skeletal and cardiac muscles, but the specific antigen was not identified in the study. T cells-mediated immune responses in the heart may cause abnormal heart electrical rhythm and irreparable damage to myocardium (58).
4.2.2 Increase of autoantibody
ICIs promote the formation of autoantibodies. Lack of PD-1 caused autoimmune dilated cardiomyopathy in mouse model with Pdcd1 gene knockout, and high titers of circulating immunoglobulins (IgGs) deposited on surface of mouse cardiomyocytes (60). Subsequent experiments showed that the autoantibodies are against cTnI. cTnI induced cardiac dysfunction and myocardial dilatation by means of chronically stimulating influx of calcium ions in cardiomyocytes (61).
4.2.3 Cardiac myosin drive cell-mediated cytotoxicity
Won et al. (62) used anti-PD-1 monoclonal antibodies to induce the development of myocarditis in mice and they found that myosin-specific T cells were increased in such mice. Axelrod et al. (63) has established Pdcd1-/-/Ctla4+/- mouse model to characterize ICIs-related myocarditis. Single-cell RNA and T cell receptor (TCR) sequencing were arranged and found increasing CD8+T cells in ICIs-related myocarditis. They subsequently found that specific TCRs recognize α-myosin, suggesting α-myosin may drive cytotoxic T-cell-mediated killing.
4.2.4 High level of cytokines
Cytokines that recruit immune cells to tumor microenvironment are significant modulators for immune response (58). ICIs lead to increased pro-inflammatory cytokines, which activate T-cells proliferation and result in anti-tumor immune response (64–66). Tarhini et al. (64) found that restraining immune checkpoints result in higher circulating pro-inflammatory cytokines [interferon (IFN)-γ, tumor necrosis factor (TNF)-α, interleukin (IL), and granulocyte macrophage colony-stimulating factor (GM-CSF)]. Those cytokines contribute to ICIs penetration into non-target organs (including cardiovascular cells) (64, 65, 67, 68).
4.2.5 Immune tolerance
Immune checkpoints inhibit T cells activation is called immune tolerance. For example, the PD-1/PD-L1 pathway prevents T cells overactivation to maintain immune balance. Blocking PD1/PD-L1will not only promote anti-tumor immunity but also inhibit Treg cells and Forkhead Box P3 (FOXP3) expression, leading to loss of self-tolerance (69). Treg cells have an effective role in keeping peripheral tolerance. Systemic application of ICIs may disrupt immune homeostasis between cytotoxic T cells and Treg cells in normal myocardial tissue, causing the development of cardiotoxicity (70, 71).
4.2.6 Atherosclerosis
Atherosclerosis is the inflammation of large arteries (72). PD-1 and CTLA-4 restrain formation of atherosclerosis. PD-1 deficient bone marrow progenitor cells up-regulate genes involved in cholesterol synthesis and ingestion, leading to elevated cholesterol (73). Blockading CTLA-4 increases T cells abundance in plaques and exacerbates atherosclerosis in mouse model (74). Banerjee et al. (75) found that senescence-associated secretory phenotype (SASP) are intersections of cancer and cardiovascular events, and SASP can aggravate atherosclerosis. More importantly, ICIs can lead to therapy-induced SASP and accelerate atherosclerosis, so atherosclerosis should be monitored while using ICIs ( (75, 76). A matched cohort study (77) showed that patients treated with ICIs have a 3-fold increase risk for cardiovascular events (77). Autopsies were performed on tumor decedents who received ICIs and those who did not, and the result showed that the ratio of CD3/CD68 was significantly elevated in atherosclerotic plaques among patients undergoing ICIs (78). After treated with ICIs, inflammation in atherosclerotic plaques was dominated by lymphocytes rather than macrophages, which is usually primary cell of atherosclerosis (78). Lymphocytes have a significant effect on the development of atherosclerosis, and in mouse model Th1 cells promote the development of atherosclerosis by secreting IFN-γ (79–81). In summary, this evidence suggests that ICIs may contribute to plaques and coronary events by altering the type of inflammation in atherosclerotic plaques (78).
4.2.7 ADCC(antibody-dependent cell-mediated cytotoxicity)
ICIs interact with proteins expressed on myocardial tissue, such as CTLA-4, FGL1, LAG-3, PD-1 and PD-L1, resulting in complement-mediated tissue injury. The Fc region of human IgG1 monoclonal antibodies binds to receptors on natural killer (NK) cells mediating ADCC. Therefore, most immune checkpoint monoclonal antibodies are IgG4 that do not mediate ADCC; however, avelumab is a human IgG1 anti-PD-L1 monoclonal antibody. Theoretically, the antibodies, bind to PD-L1 on surface of cardiomyocytes, and may mediate killing of cardiomyocytes by NK cells through ADCC (82–84).
5 Clinical manifestations of CV-irAE
CV-irAE may appear as symptoms from the myocardial, pericardial and vascular system of the body (71, 85).
5.1 Myocardial disease
5.1.1 Myocarditis
Myocarditis appears as early as 2 weeks after ICIs, and the median time is 65 days (86, 87). Myocarditis is the most frequent CV-irAE, possibly shown as asymptomatic myocarditis with an increase of cardiac biomarkers, or could be severe cardiac damage, even break out fulminant or life-threatening manifestations such as cardiogenic shock, heart failure, arrhythmias, advanced atrioventricular block or ventricular tachycardia (9, 20, 86, 88). Progression of ICIs-associated myocarditis is fulminant but can also be doubted by clinical symptoms, electrocardiography and biomarkers [troponin, brain natriuretic peptide (BNP)] and imaging (17). Myocardial biopsy is the definitive standard to identify myocarditis. The typical myocarditis clinical symptoms include palpitations, chest pain, heart failure and a range of other manifestations (89).
5.1.2 Takotsubo syndrome
Takotsubo syndrome usually appears between 15 weeks to 8 months after ICIs; however, due to its low incidence, epidemiological data are lacking and the literatures are still limited to only case reports (87). Takotsubo syndrome is an acute and transient syndrome of regional left ventricular insufficiency (90). It was first identified in Japan and characterized by myocardium dilating like a balloon and may lead to several dangerous symptoms. It was usually caused by severe stress. For clinical examination, echocardiograph shows apical or mid-left ventricular dyskinesia and troponin and NT-proBNP will elevate (91–93). A melanoma patient present takotsubo syndrome after ICIs combination therapy, and echocardiograph showed apical motion with ballooning, electrocardiogram showed V2-V6 ST elevation 1-2 mm. Cardiac MRI showed that left ventricular ejection fraction (LVEF) and systolic function returned to normal after corticosteroid treatment (94).
5.1.3 Dilated cardiomyopathy
Activated T cells result in an immune response in vessels and myocardium lead to development of dilated cardiomyopathy (95). Similarly, epidemiological data on ICIs-induced dilated cardiomyopathy is insufficient due to its low incidence. Nishimura et al. (60) found that PD-1 knockout mice developed severe dilated cardiomyopathy. Subsequently, they found that cTnI can induce cardiac dysfunction and myocardial dilatation in cardiomyocytes. Although the clinical manifestation of Takotsubo syndrome and dilated cardiomyopathy is similar, the echocardiogram of dilated cardiomyopathy does not have apical ballooning syndrome (96). There is a dilated cardiomyopathy patient after Nivolumab treatment. Echocardiography shows diffuse hypokinesis and 20% Left Ventricular Ejection Fractions (LVEF), and myocardial biopsy found inflammatory cells and interstitial fibrosis, which did not consistent with myocarditis (96).
5.2 Pericardium
ICIs related pericardium include pericarditis and pericardial effusion (97, 98). In a retrospective study, the median onset time was 40 days for pericardial effusion in 6.7% of patients treated with ICIs (99). However, it can also occur very late after the start of ICIs. In a case of advanced non-small cell lung cancer, after Nivolumab the patient developed pericardial thickening and effusion after 18 months (100). Pericarditis and pericardial effusion may be asymptomatic or mild and life-threatening symptoms may also occur when hemodynamic is unstable (101). Breathlessness is the predominant symptom and is followed by tachycardia and chest pain (102). At the time of diagnosis, the effusion should be distinguished between tumor progression related pericarditis and CV-irAE by TTF-1 immunohistochemical staining (101).
5.3 Vascular diseases
5.3.1 Vasculitis
Vasculitis caused by self-immune disorder can occur in vessels of all sizes (103). The incidence of ICIs-associated vasculitis is lower than 1%, and there was no clear epidemiological data on the median time (104). In a retrospective analysis of 1215 patients treated with ICIs, cardiovascular events occurred in approximately 1% of patients, and the median time to event was 97 days after ICIs (105, 106). Currently, irAE about vasculitis are reported mainly about large vessel and neurological vasculitis (107). ICIs lead to the activation of T cells and NK cells and the secretion of pro-inflammatory cytokines, resulting in inflammation of the vessel wall, revascularization and even vascular occlusion (108, 109). CT or MR can diagnose vasculitis that is characterized by diffuse peripheral thickening of the vessel wall, enhanced wall thickness, or thrombosis (104). Daxini et al. (107) reviewed 20 case reports that met the criteria by searching multiple medical databases, and the results showed that the most common types of ICIs-related vasculitis were macrovasculitis, such as giant cell arteritis (GCA). GCA is an inflammation of blood vessels that occurs in people older than 50 years and primarily affects the great and middle arteries, especially the extracranial branches of the aorta and external carotid arteries (108). The manifestations of GCA are various based on the vessels, leading to blindness, stroke and aneurysms (110). GCA can develop into vascular occlusion, leading to tissue ischemia and should be considered in patients with lately reported headache, visual impairment, claudication of the jaw and polymyositis rheumatica (PMR) symptoms (110). Atherosclerosis is an inflammation of the large arteries, and the primary outcome of accelerated atherosclerosis after ICIs was the occurrence of cardiovascular events (defined as a combination of myocardial infarction, coronary revascularization, and ischemic stroke) (77). A previous study found that atherosclerotic plaque can be ameliorated by the concomitant use of corticosteroids and statins (77).
6 Management and treatment of CV-irAE
6.1 Screening of baseline cardiovascular disease and risk factor
Prior to ICIs, physicians need to assess the potential cardiotoxicity of ICIs and educate patients to report suspicious symptoms to medical personnel in time (27). According to the European Society of Cardiology recommendations, risk factors of baseline include pre-existing cardiovascular disease, elevated cardiac biomarkers, and previous cardiotoxic antineoplastic drugs history (27). Baseline assessment includes physical examination and auxiliary examination, such as an electrocardiogram (ECG), echocardiogram and cardiac troponin and natriuretic peptide etc. Individualized baseline monitoring improves the survival of patients. Patients with abnormal baseline examination results (ECG, cardiac biomarkers) require therapy under the guidance of an integrated oncology and cardiology team (111, 112).
6.2 Monitoring of toxicity
Toxicity monitoring is performed through the process of ICIs, especially in patients with prior cardiac injury. Physicians should assess the possibility of CV-irAE at each follow-up visit. Monitoring of toxicity includes electrocardiogram, echocardiograms, myocardial markers, troponin and NT-proBNP: (1) electrocardiogram is routinely performed before each cycle of treatment, (2) patients are advised to follow-up regularly for echocardiograms and myocardial markers every 2-4 cycles and 6/12 months after ending using ICIs (86, 111, 113), (3) As recommended by 2021 American Society of Clinical Oncology (ASCO) guideline, there is no clear recommendation on the frequency of troponin and NT-proBNP (114). But a literature recommended testing troponin and NT-proBNP at baseline and 2-4 cycles (28).Toxicity monitoring may detect abnormal biomarkers prior to symptoms of CV-irAE. When troponin is elevated, physicians should look out for potential triad myositis-myositis, muscle weakness, and myocarditis. For patients suspicious of myositis, not only creatine kinase (CK) but also lactate dehydrogenase (LDH) should be tested because cardiotoxicity, myositis and myalgia may happen in the same patient. Once the patient appears suspicious clinical symptoms, a cardiology specialist should immediately be consulted (16, 17, 108, 111).
6.3 Diagnosis of CV-irAE
Diagnosis of CV-irAE is a challenge because there are many manifestations of CV-irAEs (115). The clinical presentation is similar to viral myocarditis which may confuse the diagnosis. The evaluation should include telemetry monitoring, serum marker (e.g., cardiac markers, CK, LDH), electrocardiogram and cardiac magnetic resonance (CMR) (116). Myocardial and vascular biopsies are the standard for diagnosing CV-irAE. Finally, diagnosis of CV-irAE should be integrated by a multidisciplinary cardio-oncology team (117).
6.4 Management and treatment of CV-irAE
6.4.1 Grade and management
Management and treatment of CV-irAE mainly depend on toxicity grading, based on the dose and dosage of given immunosuppressants. ASCO, National Comprehensive Cancer Network (NCCN) and Chinese Society of Clinical Oncology (CSCO) have classified CV-irAE in detail (Table 1).
6.4.2 Similarities and differences between guidelines
Although the incidence of CV-irAE is low, ESMO/ASCO/NCCN/CSCO guidelines all consider CV-irAE as a disease characterized by diverse manifestations, rapid progression and high mortality. However, different recommended doses for glucocorticoid were given. ASCO guidelines recommended methylprednisolone 1-2 mg/kg•d, NCCN guidelines recommended pulsed methylprednisolone 1 g/d, and ESMO/CSCO guidelines recommend 500 to 1000mg/d (114, 116, 118) (Table 2).
6.4.3 Steroid refractory CV-irAE
Other immunosuppressive agents (e.g., gammaglobulin, anti-thymocyte globulin, infliximab and morte-macrolimus) can be added if glucocorticoid mono-treatment fails after 24 hours. However, it should be noted that high-dose infliximab is forbidden if the patients have moderate to serious heart failure. Pacemakers can be installed in patients with arrhythmias if necessary, and mechanical hemodynamic support should be given promptly in critical patients (111, 118–120). All guidelines’ recommendations are based on high levels of evidence and recommended high doses of glucocorticoids. The different doses of glucocorticoid in guidelines maybe due to differences in panel references and reference areas. NCCN/ASCO have published many clinical practice guidelines with high level of evidence which have been recognized and followed by clinicians worldwide. The CSCO guidelines include a large number of toxicity data from China, and is more suitable for Chinese.
6.4.4 Re-challenge of ICIs
ASCO guidelines recommended to terminate the use of ICIs in all patients with CV-irAE, while NCCN/CSCO guidelines recommend patients with grade 1-2 cardiotoxicity restart ICIs after symptom remission.
7 Emerging predictive markers
When patients show symptoms of CV-irAE, myocardial damage already exists. In addition to conventional markers, more sensitive predictive markers are needed to prevent myocardial damage in advance. Few studies of toxicity prediction of myocarditis have been reported, but a promising toxicity prediction marker of CV-irAE need to be further explored. Drobni et al. (121) conducted a case-control study in patients with ICIs myocarditis or without CV-irAE after ICIs treatment, showing that significantly higher neutrophil/lymphocyte ratio (NLR) was found in patients with ICI related myocarditis (121). Another study compared echocardiographic global longitudinal strain (GLS) in patients with ICIs myocarditis or without CV-irAE after ICIs treatment. They found that GLS is lower in patients with ICI related myocarditis and suggested a poor prognosis (122). In summary, NLR and GLS are potential makers of immune-mediated myocarditis.
8 Discussion
CV-irAE is lethal, so we expect to detect abnormalities before irreversible myocardial damage happens; therefore, more sensitive and reliable makers are urgently needed (123–125). Although ICIs have been widely used in treating cancer and achieved good results, a series of adverse events may happen after the application of ICIs. Cardiovascular toxicities are rare but usually fatal when it occurs. Therefore, we should continually explore the mechanism of CV-irAE, summarizing the cases that have occurred, strengthening awareness of prevention and improving the management of CV-irAE, and introducing of a new surveillance strategy.
Author contributions
RY conceived the idea, XL wrote the manuscript, WP and JW revised the content and grammar of the manuscript. RY and S-CY reviewed the structure and content of the manuscript in the revision. All authors provided critical feedback and analysis and manuscript, and contributed to the final manuscript.
Funding
This work was supported by the National Natural Science Foundation of China [82072622 and 81860488 to RY; 82202888 to JW]. The work was funded by “Xing Dian Ying Cai given to RY” and Mong-Hong Lee expert workstation [202205AF150027].
Conflict of interest
The authors declare that the research was conducted in the absence of any commercial or financial relationships that could be construed as a potential conflict of interest.
Publisher’s note
All claims expressed in this article are solely those of the authors and do not necessarily represent those of their affiliated organizations, or those of the publisher, the editors and the reviewers. Any product that may be evaluated in this article, or claim that may be made by its manufacturer, is not guaranteed or endorsed by the publisher.
References
1. Vaddepally RK, Kharel P, Pandey R, Garje R, Chandra AB. Review of indications of FDA-approved immune checkpoint inhibitors per NCCN guidelines with the level of evidence. Cancers (Basel) (2020) 12(3):738. doi: 10.3390/cancers12030738
2. Han X, Wei Q, Lv Y, Weng L, Huang H, Wei Q, et al. Ginseng-derived nanoparticles potentiate immune checkpoint antibody efficacy by reprogramming the cold tumor microenvironment. Mol Ther (2022) 30(1):327–40. doi: 10.1016/j.ymthe.2021.08.028
3. Weber JS, Yang JC, Atkins MB, Disis ML. Toxicities of immunotherapy for the practitioner. J Clin Oncol (2015) 33(18):2092–9. doi: 10.1200/JCO.2014.60.0379
4. Thompson JA. New NCCN guidelines: Recognition and management of immunotherapy-related toxicity. J Natl Compr Canc Netw (2018) 16(5S):594–6. doi: 10.6004/jnccn.2018.0047
5. Haslam A, Prasad V. Estimation of the percentage of US patients with cancer who are eligible for and respond to checkpoint inhibitor immunotherapy drugs. JAMA Netw Open (2019) 2(5):e192535. doi: 10.1001/jamanetworkopen.2019.2535
6. Solinas C, Migliori E, De Silva P, Willard-Gallo K. LAG3: The biological processes that motivate targeting this immune checkpoint molecule in human cancer. Cancers (Basel) (2019) 11(8):1213. doi: 10.3390/cancers11081213
7. Sun JY, Qu Q, Lou YX, Hua Y, Sun GZ, Sun W, et al. Cardiotoxicity in cancer immune-checkpoint therapy: Mechanisms, clinical evidence, and management strategies. Int J Cardiol (2021) 344:170–8. doi: 10.1016/j.ijcard.2021.09.041
8. Agostinetto E, Ceppi M, Bruzzone M, Lambertini M, de Azambuja E. Response to letter entitled: Re: Cardiotoxicity of immune checkpoint inhibitors: A systematic review and meta-analysis of randomised clinical trials. Eur J Cancer (2021) 155:303–6. doi: 10.1016/j.ejca.2021.06.042
9. Mahmood SS, Fradley MG, Cohen JV, Nohria A, Reynolds KL, Heinzerling LM, et al. Myocarditis in patients treated with immune checkpoint inhibitors. J Am Coll Cardiol (2018) 71(16):1755–64. doi: 10.1016/j.jacc.2018.02.037
10. Matsuo K, Ishiguro T, Najama T, Shimizu Y, Kobayashi Y, Mutou M. Nivolumab-induced myocarditis successfully treated with corticosteroid therapy: A case report and review of the literature. Intern Med (2019) 58(16):2367–72. doi: 10.2169/internalmedicine.2596-18
11. Souza M, Nielsen D, Svane IM, Iversen K, Rasmussen PV, Madelaire C, et al. The risk of cardiac events in patients receiving immune checkpoint inhibitors: a nationwide Danish study. Eur Heart J (2021) 42(16):1621–31. doi: 10.1093/eurheartj/ehaa884
12. Wang DY, Salem JE, Cohen JV, Chandra S, Menzer C, Ye F, et al. Fatal toxic effects associated with immune checkpoint inhibitors: A systematic review and meta-analysis. JAMA Oncol (2018) 4(12):1721–8. doi: 10.1001/jamaoncol.2018.3923
13. Rubio-Infante N, Ramírez-Flores YA, Castillo EC, Lozano O, García-Rivas G, Torre-Amione G. Cardiotoxicity associated with immune checkpoint inhibitor therapy: a meta-analysis. Eur J Heart Fail (2021) 23(10):1739–47. doi: 10.1002/ejhf.2289
14. Larkin J, Chiarion-Sileni V, Gonzalez R, Grob JJ, Cowey CL, Lao CD, et al. Combined nivolumab and ipilimumab or monotherapy in untreated melanoma. N Engl J Med (2015) 373(1):23–34. doi: 10.1056/NEJMoa1504030
15. Tawbi HA, Schadendorf D, Lipson EJ, Ascierto PA, Matamala L, Castillo Gutiérrez E, et al. Relatlimab and nivolumab versus nivolumab in untreated advanced melanoma. N Engl J Med (2022) 386(1):24–34. doi: 10.1056/NEJMoa2109970
16. Moslehi JJ, Salem JE, Sosman JA, Lebrun-Vignes B, Johnson DB. Increased reporting of fatal immune checkpoint inhibitor-associated myocarditis. Lancet (2018) 391(10124):933. doi: 10.1016/S0140-6736(18)30533-6
17. Salem JE, Manouchehri A, Moey M, Lebrun-Vignes B, Bastarache L, Pariente A, et al. Cardiovascular toxicities associated with immune checkpoint inhibitors: an observational, retrospective, pharmacovigilance study. Lancet Oncol (2018) 19(12):1579–89. doi: 10.1016/S1470-2045(18)30608-9
18. Zamami Y, Niimura T, Okada N, Koyama T, Fukushima K, Izawa-Ishizawa Y, et al. Factors associated with immune checkpoint inhibitor-related myocarditis. JAMA Oncol (2019) 5(11):1635–7. doi: 10.1001/jamaoncol.2019.3113
19. Pirozzi F, Poto R, Aran L, Cuomo A, Galdiero MR, Spadaro G, et al. Cardiovascular toxicity of immune checkpoint inhibitors: Clinical risk factors. Curr Oncol Rep (2021) 23(2):13. doi: 10.1007/s11912-020-01002-w
20. Johnson DB, Balko JM, Compton ML, Chalkias S, Gorham J, Xu Y, et al. Fulminant myocarditis with combination immune checkpoint blockade. N Engl J Med (2016) 375(18):1749–55. doi: 10.1056/NEJMoa1609214
21. Keam SJ. Cadonilimab: First approval. Drugs (2022) 82(12):1333–9. doi: 10.1007/s40265-022-01761-9
22. Du S, Zhou L, Alexander GS, Park K, Yang L, Wang N, et al. PD-1 modulates radiation-induced cardiac toxicity through cytotoxic T lymphocytes. J Thorac Oncol (2018) 13(4):510–20. doi: 10.1016/j.jtho.2017.12.002
23. Tsuruoka K, Wakabayashi S, Morihara H, Matsunaga N, Fujisaka Y, Goto I, et al. Exacerbation of autoimmune myocarditis by an immune checkpoint inhibitor is dependent on its time of administration in mice. Int J Cardiol (2020) 313:67–75. doi: 10.1016/j.ijcard.2020.04.033
24. Xie W, Huang H, Xiao S, Fan Y, Deng X, Zhang Z. Immune checkpoint inhibitors therapies in patients with cancer and preexisting autoimmune diseases: A meta-analysis of observational studies. Autoimmun Rev (2020) 19(12):102687. doi: 10.1016/j.autrev.2020.102687
25. Lee C, Drobni ZD, Zafar A, Gongora CA, Zlotoff DA, Alvi RM, et al. Pre-existing autoimmune disease increases the risk of cardiovascular and noncardiovascular events after immunotherapy. JACC Cardio Oncol (2022) 4(5):660–9. doi: 10.1016/j.jaccao.2022.11.008
26. Tocchetti CG, Galdiero MR, Varricchi G. Cardiac toxicity in patients treated with immune checkpoint inhibitors: It is now time for cardio-Immuno-Oncology. J Am Coll Cardiol (2018) 71(16):1765–7. doi: 10.1016/j.jacc.2018.02.038
27. Lyon AR, Dent S, Stanway S, Earl H, Brezden-Masley C, Cohen-Solal A, et al. Baseline cardiovascular risk assessment in cancer patients scheduled to receive cardiotoxic cancer therapies: a position statement and new risk assessment tools from the cardio-oncology study group of the heart failure association of the European society of cardiology in collaboration with the international cardio-oncology society. Eur J Heart Fail (2020) 22(11):1945–60. doi: 10.1002/ejhf.1920
28. Lyon AR, Yousaf N, Battisti N, Moslehi J, Larkin J. Immune checkpoint inhibitors and cardiovascular toxicity. Lancet Oncol (2018) 19(9):e447–447e458. doi: 10.1016/S1470-2045(18)30457-1
29. Waliany S, Lee D, Witteles RM, Neal JW, Nguyen P, Davis MM, et al. Immune checkpoint inhibitor cardiotoxicity: Understanding basic mechanisms and clinical characteristics and finding a cure. Annu Rev Pharmacol Toxicol (2021) 61:113–34. doi: 10.1146/annurev-pharmtox-010919-023451
30. Chen DS, Irving BA, Hodi FS. Molecular pathways: next-generation immunotherapy–inhibiting programmed death-ligand 1 and programmed death-1. Clin Cancer Res (2012) 18(24):6580–7. doi: 10.1158/1078-0432.CCR-12-1362
31. Sharma P, Allison JP. The future of immune checkpoint therapy. Science (2015) 348(6230):56–61. doi: 10.1126/science.aaa8172
32. Wei SC, Duffy CR, Allison JP. Fundamental mechanisms of immune checkpoint blockade therapy. Cancer Discovery (2018) 8(9):1069–86. doi: 10.1158/2159-8290.CD-18-0367
33. Chen DS, Mellman I. Elements of cancer immunity and the cancer-immune set point. Nature (2017) 541(7637):321–30. doi: 10.1038/nature21349
34. Schildberg FA, Klein SR, Freeman GJ, Sharpe AH. Coinhibitory pathways in the B7-CD28 ligand-receptor family. Immunity (2016) 44(5):955–72. doi: 10.1016/j.immuni.2016.05.002
35. Krummel MF, Allison JP. CTLA-4 engagement inhibits IL-2 accumulation and cell cycle progression upon activation of resting T cells. J Exp Med (1996) 183(6):2533–40. doi: 10.1084/jem.183.6.2533
36. Qureshi OS, Zheng Y, Nakamura K, Attridge K, Manzotti C, Schmidt EM, et al. Trans-endocytosis of CD80 and CD86: a molecular basis for the cell-extrinsic function of CTLA-4. Science (2011) 332(6029):600–3. doi: 10.1126/science.1202947
37. Walunas TL, Bakker CY, Bluestone JA. CTLA-4 ligation blocks CD28-dependent T cell activation. J Exp Med (1996) 183(6):2541–50. doi: 10.1084/jem.183.6.2541
38. Bluestone JA, Anderson M. Tolerance in the age of immunotherapy. N Engl J Med (2020) 383(12):1156–66. doi: 10.1056/NEJMra1911109
39. Freeman GJ, Long AJ, Iwai Y, Bourque K, Chernova T, Nishimura H, et al. Engagement of the PD-1 immunoinhibitory receptor by a novel B7 family member leads to negative regulation of lymphocyte activation. J Exp Med (2000) 192(7):1027–34. doi: 10.1084/jem.192.7.1027
40. Keir ME, Butte MJ, Freeman GJ, Sharpe AH. PD-1 and its ligands in tolerance and immunity. Annu Rev Immunol (2008) 26:677–704. doi: 10.1146/annurev.immunol.26.021607.090331
41. Curiel TJ, Coukos G, Zou L, Alvarez X, Cheng P, Mottram P, et al. Specific recruitment of regulatory T cells in ovarian carcinoma fosters immune privilege and predicts reduced survival. Nat Med (2004) 10(9):942–9. doi: 10.1038/nm1093
42. Liu Y, Zheng P. How does an anti-CTLA-4 antibody promote cancer immunity. Trends Immunol (2018) 39(12):953–6. doi: 10.1016/j.it.2018.10.009
43. Du X, Tang F, Liu M, Su J, Zhang Y, Wu W, et al. A reappraisal of CTLA-4 checkpoint blockade in cancer immunotherapy. Cell Res (2018) 28(4):416–32. doi: 10.1038/s41422-018-0011-0
44. Waight JD, Chand D, Dietrich S, Gombos R, Horn T, Gonzalez AM, et al. Selective FcγR Co-engagement on APCs modulates the activity of therapeutic antibodies targeting T cell antigens. Cancer Cell (2018) 33(6):1033–47.e5. doi: 10.1016/j.ccell.2018.05.005
45. Ferrara AL, Liotti A, Pezone A, De Rosa V. Therapeutic opportunities to modulate immune tolerance through the metabolism-chromatin axis. Trends Endocrinol Metab (2022) 33(7):507–21. doi: 10.1016/j.tem.2022.04.002
46. Ferreira L, Muller YD, Bluestone JA, Tang Q. Next-generation regulatory T cell therapy. Nat Rev Drug Discovery (2019) 18(10):749–69. doi: 10.1038/s41573-019-0041-4
47. Agata Y, Kawasaki A, Nishimura H, Ishida Y, Tsubata T, Yagita H, et al. Expression of the PD-1 antigen on the surface of stimulated mouse T and b lymphocytes. Int Immunol (1996) 8(5):765–72. doi: 10.1093/intimm/8.5.765
48. Anderson AC, Joller N, Kuchroo VK. Lag-3, Tim-3, and TIGIT: Co-inhibitory receptors with specialized functions in immune regulation. Immunity (2016) 44(5):989–1004. doi: 10.1016/j.immuni.2016.05.001
49. Maruhashi T, Okazaki IM, Sugiura D, Takahashi S, Maeda TK, Shimizu K, et al. LAG-3 inhibits the activation of CD4(+) T cells that recognize stable pMHCII through its conformation-dependent recognition of pMHCII. Nat Immunol (2018) 19(12):1415–26. doi: 10.1038/s41590-018-0217-9
50. Bhatia A, Kumar Y. Cellular and molecular mechanisms in cancer immune escape: a comprehensive review. Expert Rev Clin Immunol (2014) 10(1):41–62. doi: 10.1586/1744666X.2014.865519
51. Tumeh PC, Harview CL, Yearley JH, Shintaku IP, Taylor EJ, Robert L, et al. PD-1 blockade induces responses by inhibiting adaptive immune resistance. Nature (2014) 515(7528):568–71. doi: 10.1038/nature13954
52. Zhang JC, Chen WD, Alvarez JB, Jia K, Shi L, Wang Q, et al. Cancer immune checkpoint blockade therapy and its associated autoimmune cardiotoxicity. Acta Pharmacol Sin (2018) 39(11):1693–8. doi: 10.1038/s41401-018-0062-2
53. Chocarro L, Bocanegra A, Blanco E, Fernández-Rubio L, Arasanz H, Echaide M, et al. Cutting-edge: Preclinical and clinical development of the first approved lag-3 inhibitor. Cells (2022) 11(15):2351. doi: 10.3390/cells11152351
54. Curigliano G, Gelderblom H, Mach N, Doi T, Tai D, Forde PM, et al. Phase I/Ib clinical trial of sabatolimab, an anti-TIM-3 antibody, alone and in combination with spartalizumab, an anti-PD-1 antibody, in advanced solid tumors. Clin Cancer Res (2021) 27(13):3620–9. doi: 10.1158/1078-0432.CCR-20-4746
55. Niu J, Maurice-Dror C, Lee DH, Kim DW, Nagrial A, Voskoboynik M, et al. First-in-human phase 1 study of the anti-TIGIT antibody vibostolimab as monotherapy or with pembrolizumab for advanced solid tumors, including non-small-cell lung cancer. Ann Oncol (2022) 33(2):169–80. doi: 10.1016/j.annonc.2021.11.002
56. Yarchoan M, Cope L, Ruggieri AN, Anders RA, Noonan AM, Goff LW, et al. Multicenter randomized phase II trial of atezolizumab with or without cobimetinib in biliary tract cancers. J Clin Invest (2021) 131(24):e152670. doi: 10.1172/JCI152670
57. Aggarwal C, Prawira A, Antonia S, Rahma O, Tolcher A, Cohen RB, et al. Dual checkpoint targeting of B7-H3 and PD-1 with enoblituzumab and pembrolizumab in advanced solid tumors: interim results from a multicenter phase I/II trial. J Immunother Cancer (2022) 10(4):e004424. doi: 10.1136/jitc-2021-004424
58. Grabie N, Lichtman AH, Padera R. T Cell checkpoint regulators in the heart. Cardiovasc Res (2019) 115(5):869–77. doi: 10.1093/cvr/cvz025
59. Hodi FS, O’Day SJ, McDermott DF, Weber RW, Sosman JA, Haanen JB, et al. Improved survival with ipilimumab in patients with metastatic melanoma. N Engl J Med (2010) 363(8):711–23. doi: 10.1056/NEJMoa1003466
60. Nishimura H, Okazaki T, Tanaka Y, Nakatani K, Hara M, Matsumori A, et al. Autoimmune dilated cardiomyopathy in PD-1 receptor-deficient mice. Science (2001) 291(5502):319–22. doi: 10.1126/science.291.5502.319
61. Okazaki T, Tanaka Y, Nishio R, Mitsuiye T, Mizoguchi A, Wang J, et al. Autoantibodies against cardiac troponin I are responsible for dilated cardiomyopathy in PD-1-deficient mice. Nat Med (2003) 9(12):1477–83. doi: 10.1038/nm955
62. Won T, Kalinoski HM, Wood MK, Hughes DM, Jaime CM, Delgado P, et al. Cardiac myosin-specific autoimmune T cells contribute to immune-checkpoint-inhibitor-associated myocarditis. Cell Rep (2022) 41(6):111611. doi: 10.1016/j.celrep.2022.111611
63. Axelrod ML, Meijers WC, Screever EM, Qin J, Carroll MG, Sun X, et al. T Cells specific for α-myosin drive immunotherapy-related myocarditis. Nature (2022) 611(7937):818–26. doi: 10.1038/s41586-022-05432-3
64. Tarhini AA, Zahoor H, Lin Y, Malhotra U, Sander C, Butterfield LH, et al. Baseline circulating IL-17 predicts toxicity while TGF-β1 and IL-10 are prognostic of relapse in ipilimumab neoadjuvant therapy of melanoma. J Immunother Cancer (2015) 3:39. doi: 10.1186/s40425-015-0081-1
65. Lim SY, Lee JH, Gide TN, Menzies AM, Guminski A, Carlino MS, et al. Circulating cytokines predict immune-related toxicity in melanoma patients receiving anti-PD-1-Based immunotherapy. Clin Cancer Res (2019) 25(5):1557–63. doi: 10.1158/1078-0432.CCR-18-2795
66. Baik AH, Oluwole OO, Johnson DB, Shah N, Salem JE, Tsai KK, et al. Mechanisms of cardiovascular toxicities associated with immunotherapies. Circ Res (2021) 128(11):1780–801. doi: 10.1161/CIRCRESAHA.120.315894
67. Varricchi G, Galdiero MR, Tocchetti CG. Cardiac toxicity of immune checkpoint inhibitors: Cardio-oncology meets immunology. Circulation (2017) 136(21):1989–92. doi: 10.1161/CIRCULATIONAHA.117.029626
68. Tsuruda T, Yoshikawa N, Kai M, Yamaguchi M, Toida R, Kodama T, et al. The cytokine expression in patients with cardiac complication after immune checkpoint inhibitor therapy. Intern Med (2021) 60(3):423–9. doi: 10.2169/internalmedicine.5317-20
69. Kumar P, Bhattacharya P, Prabhakar BS. A comprehensive review on the role of co-signaling receptors and treg homeostasis in autoimmunity and tumor immunity. J Autoimmun (2018) 95:77–99. doi: 10.1016/j.jaut.2018.08.007
70. Alissafi T, Hatzioannou A, Legaki AI, Varveri A, Verginis P. Balancing cancer immunotherapy and immune-related adverse events: The emerging role of regulatory T cells. J Autoimmun (2019) 104:102310. doi: 10.1016/j.jaut.2019.102310
71. Kumar P, Saini S, Prabhakar BS. Cancer immunotherapy with check point inhibitor can cause autoimmune adverse events due to loss of treg homeostasis. Semin Cancer Biol (2020) 64:29–35. doi: 10.1016/j.semcancer.2019.01.006
72. Lutgens E, Seijkens T. Cancer patients receiving immune checkpoint inhibitor therapy are at an increased risk for atherosclerotic cardiovascular disease. J Immunother Cancer (2020) 8(1):e000300. doi: 10.1136/jitc-2019-000300
73. Strauss L, Mahmoud M, Weaver JD, Tijaro-Ovalle NM, Christofides A, Wang Q, et al. Targeted deletion of PD-1 in myeloid cells induces antitumor immunity. Sci Immunol (2020) 5(43):eaay1863. doi: 10.1126/sciimmunol.aay1863
74. Seijkens T, van Tiel CM, Kusters P, Atzler D, Soehnlein O, Zarzycka B, et al. Targeting CD40-induced TRAF6 signaling in macrophages reduces atherosclerosis. J Am Coll Cardiol (2018) 71(5):527–42. doi: 10.1016/j.jacc.2017.11.055
75. Dominic A, Banerjee P, Hamilton DJ, Le NT, Abe JI. Time-dependent replicative senescence vs. disturbed flow-induced pre-mature aging in atherosclerosis. Redox Biol (2020) 37:101614. doi: 10.1016/j.redox.2020.101614
76. Banerjee P, Kotla S, Reddy Velatooru L, Abe RJ, Davis EA, Cooke JP, et al. Senescence-associated secretory phenotype as a hinge between cardiovascular diseases and cancer. Front Cardiovasc Med (2021) 8:763930. doi: 10.3389/fcvm.2021.763930
77. Drobni ZD, Alvi RM, Taron J, Zafar A, Murphy SP, Rambarat PK, et al. Association between immune checkpoint inhibitors with cardiovascular events and atherosclerotic plaque. Circulation (2020) 142(24):2299–311. doi: 10.1161/CIRCULATIONAHA.120.049981
78. Newman JL, Stone JR. Immune checkpoint inhibition alters the inflammatory cell composition of human coronary artery atherosclerosis. Cardiovasc Pathol (2019) 43:107148. doi: 10.1016/j.carpath.2019.107148
79. Gupta S, Pablo AM, Xc J, Wang N, Tall AR, Schindler C. IFN-gamma potentiates atherosclerosis in ApoE knock-out mice. J Clin Invest (1997) 99(11):2752–61. doi: 10.1172/JCI119465
80. Zhou X, Robertson AK, Rudling M, Parini P, Hansson GK. Lesion development and response to immunization reveal a complex role for CD4 in atherosclerosis. Circ Res (2005) 96(4):427–34. doi: 10.1161/01.RES.0000156889.22364.f1
81. Zhou X, Robertson AK, Hjerpe C, Hansson GK. Adoptive transfer of CD4+ T cells reactive to modified low-density lipoprotein aggravates atherosclerosis. Arterioscler Thromb Vasc Biol (2006) 26(4):864–70. doi: 10.1161/01.ATV.0000206122.61591.ff
82. Boyerinas B, Jochems C, Fantini M, Heery CR, Gulley JL, Tsang KY, et al. Antibody-dependent cellular cytotoxicity activity of a novel anti-PD-L1 antibody avelumab (MSB0010718C) on human tumor cells. Cancer Immunol Res (2015) 3(10):1148–57. doi: 10.1158/2326-6066.CIR-15-0059
83. Fujii R, Friedman ER, Richards J, Tsang KY, Heery CR, Schlom J, et al. Enhanced killing of chordoma cells by antibody-dependent cell-mediated cytotoxicity employing the novel anti-PD-L1 antibody avelumab. Oncotarget (2016) 7(23):33498–511. doi: 10.18632/oncotarget.9256
84. Khanna S, Thomas A, Abate-Daga D, Zhang J, Morrow B, Steinberg SM, et al. Malignant mesothelioma effusions are infiltrated by CD3(+) T cells highly expressing PD-L1 and the PD-L1(+) tumor cells within these effusions are susceptible to ADCC by the anti-PD-L1 antibody avelumab. J Thorac Oncol (2016) 11(11):1993–2005. doi: 10.1016/j.jtho.2016.07.033
85. Hu JR, Florido R, Lipson EJ, Naidoo J, Ardehali R, Tocchetti CG, et al. Cardiovascular toxicities associated with immune checkpoint inhibitors. Cardiovasc Res (2019) 115(5):854–68. doi: 10.1093/cvr/cvz026
86. Escudier M, Cautela J, Malissen N, Ancedy Y, Orabona M, Pinto J, et al. Clinical features, management, and outcomes of immune checkpoint inhibitor-related cardiotoxicity. Circulation (2017) 136(21):2085–7. doi: 10.1161/CIRCULATIONAHA.117.030571
87. Chen DY, Huang WK, Chien-Chia Wu V, Chang WC, Chen JS, Chuang CK, et al. Cardiovascular toxicity of immune checkpoint inhibitors in cancer patients: A review when cardiology meets immuno-oncology. J Formos Med Assoc (2020) 119(10):1461–75. doi: 10.1016/j.jfma.2019.07.025
88. Palaskas N, Lopez-Mattei J, Durand JB, Iliescu C, Deswal A. Immune checkpoint inhibitor myocarditis: Pathophysiological characteristics, diagnosis, and treatment. J Am Heart Assoc (2020) 9(2):e013757. doi: 10.1161/JAHA.119.013757
89. Bonaca MP, Olenchock BA, Salem JE, Wiviott SD, Ederhy S, Cohen A, et al. Myocarditis in the setting of cancer therapeutics: Proposed case definitions for emerging clinical syndromes in cardio-oncology. Circulation (2019) 140(2):80–91. doi: 10.1161/CIRCULATIONAHA.118.034497
90. Serzan M, Rapisuwon S, Krishnan J, Chang IC, Barac A. Takotsubo cardiomyopathy associated with checkpoint inhibitor therapy: Endomyocardial biopsy provides pathological insights to dual diseases. JACC Cardio Oncol (2021) 3(2):330–4. doi: 10.1016/j.jaccao.2021.02.005
91. Tsuchihashi K, Ueshima K, Uchida T, Oh-mura N, Kimura K, Owa M, et al. Transient left ventricular apical ballooning without coronary artery stenosis: a novel heart syndrome mimicking acute myocardial infarction. Angina Pectoris-Myocardial Infarction Investigations Japan J Am Coll Cardiol (2001) 38(1):11–8. doi: 10.1016/s0735-1097(01)01316-x
92. El-Hussein MT, Kilfoil L. The story of a broken heart: Takotsubo cardiomyopathy. J Emerg Nurs (2021) 47(4):635–42. doi: 10.1016/j.jen.2020.12.014
93. Heinzerling L, Ott PA, Hodi FS, Husain AN, Tajmir-Riahi A, Tawbi H, et al. Cardiotoxicity associated with CTLA4 and PD1 blocking immunotherapy. J Immunother Cancer (2016) 4:50. doi: 10.1186/s40425-016-0152-y
94. Oldfield K, Jayasinghe R, Niranjan S, Chadha S. Immune checkpoint inhibitor-induced takotsubo syndrome and diabetic ketoacidosis: rare reactions. BMJ Case Rep (2021) 14(2):e237217. doi: 10.1136/bcr-2020-237217
95. Herzum M, Maisch B. Humoral and cellular immune response in human myocarditis and dilated cardiomyopathy. Pathol Immunopathol Res (1988) 7(4):240–50. doi: 10.1159/000157120
96. Samejima Y, Iuchi A, Kanai T, Noda Y, Nasu S, Tanaka A, et al. Development of severe heart failure in a patient with squamous non-small-cell lung cancer during nivolumab treatment. Intern Med (2020) 59(16):2003–8. doi: 10.2169/internalmedicine.4550-20
97. Amiri-Kordestani L, Moslehi J, Cheng J, Tang S, Schroeder R, Sridhara R, et al. Cardiovascular adverse events in immune checkpoint inhibitor clinical trials: A US food and drug administration pooled analysis. J Clin Oncol (2018) 36(15_suppl):3009–9. doi: 10.1200/JCO.2018.36.15_suppl.3009
98. Zhu H, Ivanovic M, Nguyen A, Nguyen PK, Wu SM. Immune checkpoint inhibitor cardiotoxicity: Breaking barriers in the cardiovascular immune landscape. J Mol Cell Cardiol (2021) 160:121–7. doi: 10.1016/j.yjmcc.2021.07.006
99. Canale ML, Camerini A, Casolo G, Lilli A, Bisceglia I, Parrini I, et al. Incidence of pericardial effusion in patients with advanced non-small cell lung cancer receiving immunotherapy. Adv Ther (2020) 37(7):3178–84. doi: 10.1007/s12325-020-01386-y
100. Moriyama S, Fukata M, Tatsumoto R, Kono M. Refractory constrictive pericarditis caused by an immune checkpoint inhibitor properly managed with infliximab: a case report. Eur Heart J Case Rep (2021) 5(1):ytab002. doi: 10.1093/ehjcr/ytab002
101. Saade A, Mansuet-Lupo A, Arrondeau J, Thibault C, Mirabel M, Goldwasser F, et al. Pericardial effusion under nivolumab: case-reports and review of the literature. J Immunother Cancer (2019) 7(1):266. doi: 10.1186/s40425-019-0760-4
102. Zarogoulidis P, Chinelis P, Athanasiadou A, Tsiouda T, Trakada G, Kallianos A, et al. Possible adverse effects of immunotherapy in non-small cell lung cancer; treatment and follow-up of three cases. Respir Med Case Rep (2017) 22:101–5. doi: 10.1016/j.rmcr.2017.07.004
103. Lindner AK, Gruenbacher G, Schachtner G, Thurnher M, Pichler R. Rare, but severe: Vasculitis and checkpoint inhibitors. Eur Urol Focus (2020) 6(3):609–12. doi: 10.1016/j.euf.2019.04.014
104. Gosangi B, McIntosh L, Keraliya A, Irugu D, Baheti A, Khandelwal A, et al. Imaging features of toxicities associated with immune checkpoint inhibitors. Eur J Radiol Open (2022) 9:100434. doi: 10.1016/j.ejro.2022.100434
105. Bar J, Markel G, Gottfried T, Percik R, Leibowitz-Amit R, Berger R, et al. Acute vascular events as a possibly related adverse event of immunotherapy: a single-institute retrospective study. Eur J Cancer (2019) 120:122–31. doi: 10.1016/j.ejca.2019.06.021
106. Schiffer WB, Deych E, Lenihan DJ, Zhang KW. Coronary and aortic calcification are associated with cardiovascular events on immune checkpoint inhibitor therapy. Int J Cardiol (2021) 322:177–82. doi: 10.1016/j.ijcard.2020.08.024
107. Daxini A, Cronin K, Sreih AG. Vasculitis associated with immune checkpoint inhibitors-a systematic review. Clin Rheumatol (2018) 37(9):2579–84. doi: 10.1007/s10067-018-4177-0
108. Samson M, Corbera-Bellalta M, Audia S, Planas-Rigol E, Martin L, Cid MC, et al. Recent advances in our understanding of giant cell arteritis pathogenesis. Autoimmun Rev (2017) 16(8):833–44. doi: 10.1016/j.autrev.2017.05.014
109. Hid Cadena R, Abdulahad WH, Hospers G, Wind TT, Boots A, Heeringa P, et al. Checks and balances in autoimmune vasculitis. Front Immunol (2018) 9:315. doi: 10.3389/fimmu.2018.00315
110. Schmidt J, Warrington KJ. Polymyalgia rheumatica and giant cell arteritis in older patients: diagnosis and pharmacological management. Drugs Aging (2011) 28(8):651–66. doi: 10.2165/11592500-000000000-00000
111. Brahmer JR, Lacchetti C, Schneider BJ, Atkins MB, Brassil KJ, Caterino JM, et al. Management of immune-related adverse events in patients treated with immune checkpoint inhibitor therapy: American society of clinical oncology clinical practice guideline. J Clin Oncol (2018) 36(17):1714–68. doi: 10.1200/JCO.2017.77.6385
112. Thompson JA, Schneider BJ, Brahmer J, Andrews S, Armand P, Bhatia S, et al. NCCN guidelines insights: Management of immunotherapy-related toxicities, version 1.2020. J Natl Compr Canc Netw (2020) 18(3):230–41. doi: 10.6004/jnccn.2020.0012
113. Puzanov I, Diab A, Abdallah K, Bingham CO 3rd, Brogdon C, Dadu R, et al. Managing toxicities associated with immune checkpoint inhibitors: consensus recommendations from the society for immunotherapy of cancer (SITC) toxicity management working group. J Immunother Cancer (2017) 5(1):95. doi: 10.1186/s40425-017-0300-z
114. Schneider BJ, Naidoo J, Santomasso BD, Lacchetti C, Adkins S, Anadkat M, et al. Management of immune-related adverse events in patients treated with immune checkpoint inhibitor therapy: ASCO guideline update. J Clin Oncol (2021) 39(36):4073–126. doi: 10.1200/JCO.21.01440
115. Arangalage D, Degrauwe N, Michielin O, Monney P, Özdemir BC. Pathophysiology, diagnosis and management of cardiac toxicity induced by immune checkpoint inhibitors and BRAF and MEK inhibitors. Cancer Treat Rev (2021) 100:102282. doi: 10.1016/j.ctrv.2021.102282
116. Thompson JA, Schneider BJ, Brahmer J, Achufusi A, Armand P, Berkenstock MK, et al. Management of immunotherapy-related toxicities, version 1.2022, NCCN clinical practice guidelines in oncology. J Natl Compr Canc Netw (2022) 20(4):387–405. doi: 10.6004/jnccn.2022.0020
117. Ammirati E, Cipriani M, Moro C, Raineri C, Pini D, Sormani P, et al. Clinical presentation and outcome in a contemporary cohort of patients with acute myocarditis: Multicenter Lombardy registry. Circulation (2018) 138(11):1088–99. doi: 10.1161/CIRCULATIONAHA.118.035319
118. Haanen J, Carbonnel F, Robert C, Kerr KM, Peters S, Larkin J, et al. Management of toxicities from immunotherapy: ESMO clinical practice guidelines for diagnosis, treatment and follow-up. Ann Oncol (2017) 28(suppl_4):iv119–119iv142. doi: 10.1093/annonc/mdx225
119. Kwon HJ, Coté TR, Cuffe MS, Kramer JM, Braun MM. Case reports of heart failure after therapy with a tumor necrosis factor antagonist. Ann Intern Med (2003) 138(10):807–11. doi: 10.7326/0003-4819-138-10-200305200-00008
120. Dueck AC, Mendoza TR, Mitchell SA, Reeve BB, Castro KM, Rogak LJ, et al. Validity and reliability of the US national cancer Institutes patient-reported outcomes version of the common terminology criteria for adverse events (PRO-CTCAE). JAMA Oncol (2015) 1(8):1051–9. doi: 10.1001/jamaoncol.2015.2639
121. Drobni ZD, Zafar A, Zubiri L, Zlotoff DA, Alvi RM, Lee C, et al. Decreased absolute lymphocyte count and increased Neutrophil/Lymphocyte ratio with immune checkpoint inhibitor-associated myocarditis. J Am Heart Assoc (2020) 9(23):e018306. doi: 10.1161/JAHA.120.018306
122. Awadalla M, Mahmood SS, Groarke JD, Hassan M, Nohria A, Rokicki A, et al. Global longitudinal strain and cardiac events in patients with immune checkpoint inhibitor-related myocarditis. J Am Coll Cardiol (2020) 75(5):467–78. doi: 10.1016/j.jacc.2019.11.049
123. Li Y, Ju L, Hou Z, Deng H, Zhang Z, Wang L, et al. Screening, verification, and optimization of biomarkers for early prediction of cardiotoxicity based on metabolomics. J Proteome Res (2015) 14(6):2437–45. doi: 10.1021/pr501116c
124. Hopkins AM, Rowland A, Kichenadasse G, Wiese MD, Gurney H, McKinnon RA, et al. Predicting response and toxicity to immune checkpoint inhibitors using routinely available blood and clinical markers. Br J Cancer (2017) 117(7):913–20. doi: 10.1038/bjc.2017.274
Keywords: cardiotoxicity, immune checkpoint inhibitors, immune-related adverse events, Myocarditis, Pericarditis, Vasculitis
Citation: Li X, Peng W, Wu J, Yeung S-CJ and Yang R (2023) Advances in immune checkpoint inhibitors induced-cardiotoxicity. Front. Immunol. 14:1130438. doi: 10.3389/fimmu.2023.1130438
Received: 23 December 2022; Accepted: 13 February 2023;
Published: 23 February 2023.
Edited by:
Yeonseok Chung, Seoul National University, Republic of KoreaReviewed by:
Jessica Crystal, University of Miami, United StatesGuoqiang Gu, Second Hospital of Hebei Medical University, China
Copyright © 2023 Li, Peng, Wu, Yeung and Yang. This is an open-access article distributed under the terms of the Creative Commons Attribution License (CC BY). The use, distribution or reproduction in other forums is permitted, provided the original author(s) and the copyright owner(s) are credited and that the original publication in this journal is cited, in accordance with accepted academic practice. No use, distribution or reproduction is permitted which does not comply with these terms.
*Correspondence: Runxiang Yang, eXJ4X3Jlc2VhcmNoQDE2My5jb20=