- 1Department of Biology, University of Padova, Padova, Italy
- 2Department of Experimental and Clinical Medicine, University of Firenze, Firenze, Italy
The miniferritin HP-NAP of Helicobacter pylori was originally described as a neutrophil-activating protein because of the capacity to activate neutrophils to generate oxygen radicals and adhere to endothelia. Currently, the main feature for which HP-NAP is known is the ability to promote Th1 responses and revert the immune suppressive profile of macrophages. In this review, we discuss the immune modulating properties of the protein regarding the H. pylori infection and the evidence that support the potential clinical application of HP-NAP in allergy and cancer immunotherapy.
Introduction
Bacteria have two types of ferritin-like molecules, the heme binding bacterioferritins (Bfr) and the non-heme binding bacterial ferritins (Ftn) (1, 2). Both are composed of 24 identical or similar subunits that form a roughly spherical protein containing a large hollow centre that acts as an iron-storage cavity with the capacity to accommodate up to 4000 iron atoms.
In 1992, Almirón and colleagues discovered a starvation-inducible protein that was strongly bound to chromosomal DNA in starved cultures of Escherichia coli. The protein was called Dps, as in DNA-binding protein from starved cells (3). Later, in vivo, and in vitro assays showed that Dps protected DNA during oxidative stress, by sequestering iron and by physically binding the DNA (4), although the latter activity was not demonstrated for all Dps, subsequently identified (5). Dps proteins are ubiquitous in bacteria and, to date, 76 members have been discovered in 57 organisms (6). Their sequence closeness to members of the bacterial ferritin family (7) suggested that Dps represent a new type of ferritin that takes part in a general prokaryotic approach for tackling oxidative stress. In 1998 the first crystal structure of a Dps protein was published (8). The structure proved that Dps is an analogue of ferritins. Dps monomers have essentially the same protein fold (four helix bundle) as the ferritin monomer, and they pack in a dodecameric hollow sphere which closely resembles the packing of ferritin monomers. According to their size which is smaller than that of Bfr and Ftn, Dps can store 500 atoms of iron (9).
Several names and abbreviations have been used to describe miniferritins, depending on the biochemical feature that was being studied: the most common are Dps, for their DNA-binding properties, which is often used interchangeably with miniferritin, and NAP (from neutrophil-activating protein), a term used for the first time referring to the miniferritin of Helicobacter pylori because of its capacity to activate neutrophils to produce oxygen radicals and adhere to endothelia (10).
The discovery that miniferritins had an impact on the function of host immune cells besides their role in protecting bacterial DNA from oxidizing radicals, has given the impetus to numerous studies on Dps proteins produced by pathogenic bacteria, such as Borrelia burgdorferi (NapA), Treponema pallidum (TpF1), Helicobacter cinaedi (CAIP). What emerged is that Dps proteins are major determinants in the pathogenesis of chronic inflammatory diseases because of a robust immune modulatory activity (11–14). Among the miniferritins produced by pathogenic bacteria, the most studied is certainly NAP, also called HP-NAP, produced by H. pylori.
This minireview summarizes the current state of knowledge on HP-NAP. We address the biological features of this Dps, highlighting the ability of promoting inflammation and dictating the profile of the adaptive immune response, as crucial in the pathogenesis of H. pylori-associated diseases. On the other hand, we also emphasize that it is because of its powerful and specific action on the immune system that HP-NAP has a significant potential utility in clinical practice.
HP-NAP in H. pylori Infection
H. pylori infection is mostly acquired during childhood and often persists for life in the infected host. Depending on geographical region and economic development, the prevalence of H. pylori infection in adults has been found to range from 24% to 73% among populations, with a global prevalence estimate of around 50% (15). Although most infected individuals remain asymptomatic, bacteria colonization of the gastric mucosa may cause the development of various clinical conditions such as peptic ulcers, chronic gastritis and gastric adenocarcinomas, and mucosa-associated lymphoid tissue lymphomas (16). The common feature that underlies H. pylori-associated disorders is the generation of an inflammatory milieu that the bacterial infection elicits in the gastric mucosa. The strong recruitment of neutrophils, monocytes/macrophages, but most of all, T helper 1 (Th1) lymphocytes whose homing in the inflamed tissue is needed to potentiate the killing potential of macrophages, one would expect to be the best arsenal to fight the bacterium. On the contrary, if left untreated, the infection persists and the inflammatory status that becomes chronic lays the foundation for the development of severe diseases.
Among several virulence factors which cooperate in promoting and maintaining inflammation, HP-NAP is probably the most active. Released by the bacterium in proximity to the gastric epithelial monolayer, HP-NAP can cross the epithelium and activate monocytes/macrophages and mast cells which represent the first line of defense, to release pro-inflammatory cytokines, i.e. TNF-α, IL-6, IL-12 and IL-23 (17, 18). HP-NAP also increases the synthesis of tissue factor (TF) and the secretion of the inhibitor-2 of the plasminogen activator in mononuclear cells (19). The coordinate expression of pro-coagulant and antifibrinolytic activities is expected to favor fibrin deposition and contribute to the inflammatory reaction elicited by H. pylori in the gastric mucosa. Once in the stomach wall, HP-NAP directly promotes the recruitment of leukocytes with a path resembling that adopted by the chemokine CXCL8 (20): following transcytosis through endothelial cells, a sizable amount of HP-NAP remains bound to the luminal face of the endothelium (Figure 1). How the luminal surface presentation of the protein occurs remains an open issue, but in this form HP-NAP encounters rolling leukocytes, up-regulates the expression of β2 integrins and induces a conformational change of these adhesion receptors, resulting in an increased affinity of them for the endothelial partner (21). This event, which is crucial for the tight adhesion of leukocytes to the endothelium, precedes extravasation. Under HP-NAP stimulation, recruited cells release pro-inflammatory cytokines and chemokines that contribute to the maintenance of inflammation by further recruiting additional neutrophils, monocytes, and lymphocytes (18, 22, 23). Several studies suggest that HP-NAP may interact with at least two receptors on the plasma membrane of leukocytes. The engagement of Toll-like receptor (TLR)-2 (18) is crucial for the production of cytokines, whereas the interaction with a G protein-coupled receptor is mainly linked to burst activation, adhesion, and chemotaxis of leukocytes (22). The evidence that the latter effects are abrogated by inhibiting p38-MAPK, suggested a role for the kinase in the signaling cascade (21, 24).
Despite the pro-inflammatory role of HP-NAP is established, the deletion of the napA gene does not abrogate the capacity of H. pylori to stimulate the production of TNF-α, IL-6, and CXCL8 by mononuclear cells, suggesting that other factors than HP-NAP are involved. On the contrary, bacteria which do not produce HP-NAP are unable to elicit the release of the Th1-polarizing cytokine IL-12 by the same cells, an event that occurs following the engagement of TLR-2 by the miniferritin (18).
In vivo in the antrum H. pylori infection causes a predominant activation of Th1 cells with production of IFN-γ and elevated expression of IL-12, IL-18, IL-17 and TNF-α (25–28). A considerable number of Th cells in the stomach mucosa of H. pylori-infected individuals display significant proliferation in response to HP-NAP (18, 25). According to the evidence that HP-NAP can create an IL-12-rich environment, antigen-specific gastric Th cells produce large amounts of IFN- γ and TNF-α and have a powerful cytotoxic activity in response to HP-NAP stimulation, indicating a polarized Th1/T cytotoxic 1 (Tc1) effector phenotype (18).
Collectively, these findings show that the in vitro and in vivo actions of HP-NAP are highly correlated and identify the bacterial protein as responsible for driving the Th response in the gastric antrum of patients affected by H. pylori. The skewing of the gastric T-cell response towards a Th1 profile, characterized by huge IFN-γ production and activation of a cytolytic program, is expected to lead to gastric damage (Figure 1). Moreover, the high levels of TF, IFN-γ, and TNF-α might result in procoagulant activity and in gastric functional alteration, such as increased gastrin secretion and pepsinogen release, respectively (25).
HP-NAP as Therapeutic Tool
In view of the evidence that HP-NAP possesses a unique capacity to modulate the immune response, numerous researchers have been motivated to verify the application potential of the miniferritin as therapeutic agent. In vivo studies using a recombinant form of HP-NAP has been carried out in mouse model of diseases where a Th2 response is detrimental or where the induction of a Th1 and Tc1 cytotoxic immune response is beneficial, such as allergy and cancer.
Th2 Responses
Allergic disorders, (i.e., allergic rhinitis, asthma and atopic dermatitis-AD) are Th2-mediated inflammatory diseases characterized by local infiltration of eosinophils and elevated allergen-specific IgE serum level (29, 30).
The administration of HP-NAP in a mouse model of ovalbumin (OVA)-induced allergic asthma, revealed the potent inhibitory effect of the protein on the airway eosinophil infiltration and on the Th2 bronchial inflammation, resulting in a great reduction of total serum IgE paralleled by the increase of IL-12 plasma levels (31). A similar effect was achieved in the same mouse model by injecting a plasmid encoding a protein chimera formed by HP-NAP and a soluble form of IL-4 receptor a chain, working as decoy receptor to block the IL-4 released by eosinophils and Th2 cells (32), and after orally administrating spores of Bacillus subtilis as a vehicle to deliver HP-NAP fused to the cholera toxin B subunit, widely used to induce peripheral immunological tolerance to co-administered antigens (33).
The capability of HP-NAP to counteract the Th2 immune responses was confirmed in a mouse model of AD (34). AD is characterized by an imbalance between Th1 and Th2 cells which results in increased production of IL-4 and IgE, and local recruitment of eosinophils (35). Intra peritoneal injection of HP-NAP significantly attenuated the secretion of IgE and IL-4 and alleviated the AD symptoms, such as erythema and swelling (Figure 2A).
Th2 cells not only regulate allergic disorders but are also involved in the immune response to helminth infections (36). Treatment of mice infected with the intestinal parasite Trichinella spiralis with HP-NAP resulted in a consistent reduction of the type 2 immune response, as revealed by the reduced eosinophil infiltration and the drop of IgE serum levels (37).
Cancer
Cancer immunotherapy has revolutionized the field of oncology by prolonging survival of patients with rapidly fatal cancers (38). Among the variety of strategies that have become routine in the clinical practice there is the induction of Th1/Tc1 immune response with massive IFN-γ production (39). Based on the capacity to generate an IL-12-enriched environment promoting the differentiation of Th1 cells, the possibility that HP-NAP might be able to elicit an anti-tumor response, was worth investigating.
The first study, carried out in an orthotopic model of bladder cancer, revealed that the local administration of HP-NAP, by eliciting a potent Th1/Tc1 response, counteracted tumor growth and reduced vascularization of the mass due to the anti-angiogenic activity of IFN-γ (40). Notably, while the administration of Bacillus Calmette-Guérin (BCG), gold standard treatment for non-muscle-invasive bladder cancer, resulted in a strong hematuria, a condition often associated with the therapy, none of the HP-NAP-treated animals showed a macroscopic alteration of the urine aspect. Similar results were obtained in mouse models of hepatoma and sarcoma in which the protein was administered as chimera, fused with the maltose binding protein (rMBP-NAP) (41). The evidence that IFN-γ+ T cells were not produced, and tumor growth was not inhibited in TLR-2-knock-out mice following administration of HP-NAP (40) or by co-administrating rMBP-NAP and a TLR-2 blocking antibody (41), confirmed the in vitro finding suggesting the essential role of the immune receptor for the HP-NAP activity (18).
In a work by Mohabati Mobarez et al. (42), HP-NAP was loaded in chitosan nanoparticles (Chi-rNAP) and applied in a mouse model of breast cancer. The Chi-rNAP formulation strongly affected tumor growth, with an efficacy superior to that of the recombinant protein alone, in accordance to the fact that chitosan nanoparticles, by activating the antigen presenting cells, act as adjuvants (43).
Due to the ability to link the innate with the adaptive immune response, TLR agonists are highly promising as adjuvants in vaccines against life-threatening and complex diseases such as cancer. The possibility of using HP-NAP as adjuvant for cancer treatment was explored by some studies in which the protein was expressed in oncolytic viruses (OVs). The capacity of OVs to selectively replicate in tumor cells leading to cell death makes OVs promising agents for cancer therapy (44). In a neuroendocrine cancer mouse model, the intratumoral injection of OVs expressing HP-NAP improved the animal survival and increased the plasma level of the p40 subunit of IL-12 (45). Using an adenoviral vector encoding HP-NAP, it was demonstrated that the protein promotes the maturation of dendritic cells, both in vitro and in vivo. Dendritic cells matured by vector-encoded HP-NAP secrete high level of IL-12, and in accordance have the capacity to induce antigen-specific T cell expansion with a predominant Th1 profile. In the same line of evidence it was shown that HP-NAP per se promotes the maturation of dendritic cells and the activation and proliferation of cytotoxic T cells towards melanoma cells (46, 47).
An attenuated measles virus strain and vaccinia virus were engineered to express HP-NAP and both were effective in counteracting tumor growth and in improving the survival of animals with breast cancer and neuroblastoma, respectively (48, 49). CAR T cells engineering to produce HP-NAP turned out to be a very promising approach for treating solid tumors that are difficult to completely eradicate with conventional CAR T cells, due to heterogeneity in antigen expression. In mouse models of cancer, injection of CAR(NAP) T cells slowed tumor growth and increased survival rates compared to standard mice CAR T cells, regardless of target antigen or tumor type. The evidence on the safety of this approach in mice bode well for its clinical application (50).
All these studies have converged on the notion that the anti-tumor potential of HP-NAP relies on the activation and shaping of the adaptive immune response, but the possibility that HP-NAP might counteract tumor growth due to the modulation of mononuclear cells, regardless of the participation of the adaptive immunity, remained unexplored. Codolo and colleagues, taking advantage of the zebrafish model, examined the therapeutic efficacy of HP-NAP against metastatic human melanoma, limiting the observational window to 9 days post-fertilization, well before the maturation of the adaptive immunity. The study disclosed a new property of the miniferritin, namely the capacity of reverting the immune suppressive profile of macrophages, so as to counteract the tumor growth even in the absence of the acquired immune system (51).
Concluding Remarks
Since its discovery in 1995, HP-NAP, the miniferritin produced by H. pylori has been under intense focus because of its remarkable ability to modulate the human immune response. H. pylori infection leads to an intense inflammatory response in the gastric mucosa, characterized by the infiltration of polymorphonuclear and mononuclear cells. It is assumed that HP-NAP, by cooperating to the recruitment of inflammatory cells but especially by generating a pro-inflammatory Th1 skewing environment (Figure 1) can make a substantial contribution to the gastric damage caused by H. pylori infection. In accordance, HP-NAP is one of the antigens included in the vaccine formulations currently under investigation (52).
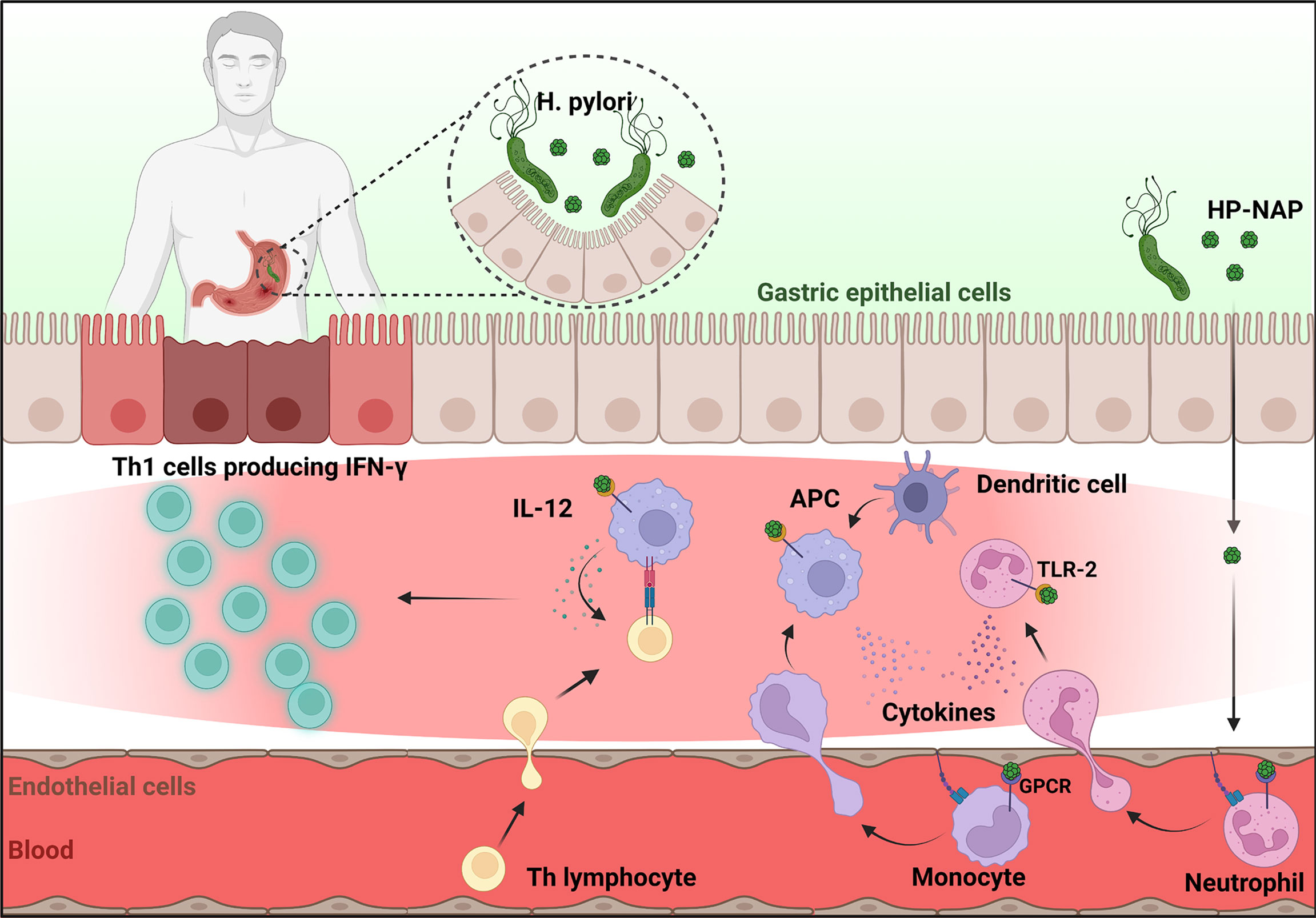
Figure 1 HP-NAP activity in the context of H. pylori infection. Once released by H. pylori in the stomach lumen, HP-NAP crosses the gastric epithelial cell layer and the endothelium. Bound to the luminal face of the latter, it directly stimulates leukocytes to adhere and extravasate. In addition, HP-NAP activates recruited neutrophils and monocytes to secrete cytokines that further promote inflammation, and stimulates monocytes/macrophages and dendritic cells (antigen presenting cells, APC) to release of IL-12 which drives the differentiation of T helper cells towards the IFN-γ producing Th1 phenotype. Figure created with BioRender.com.
On the other hand, the immune modulating activity of HP-NAP makes it an excellent candidate for developing new therapeutic strategies aimed at preventing and treating allergic disorders, such as bronchial asthma, rhinitis, conjunctivitis and, most importantly, at fighting malignant tumors (Figures 2A, B).
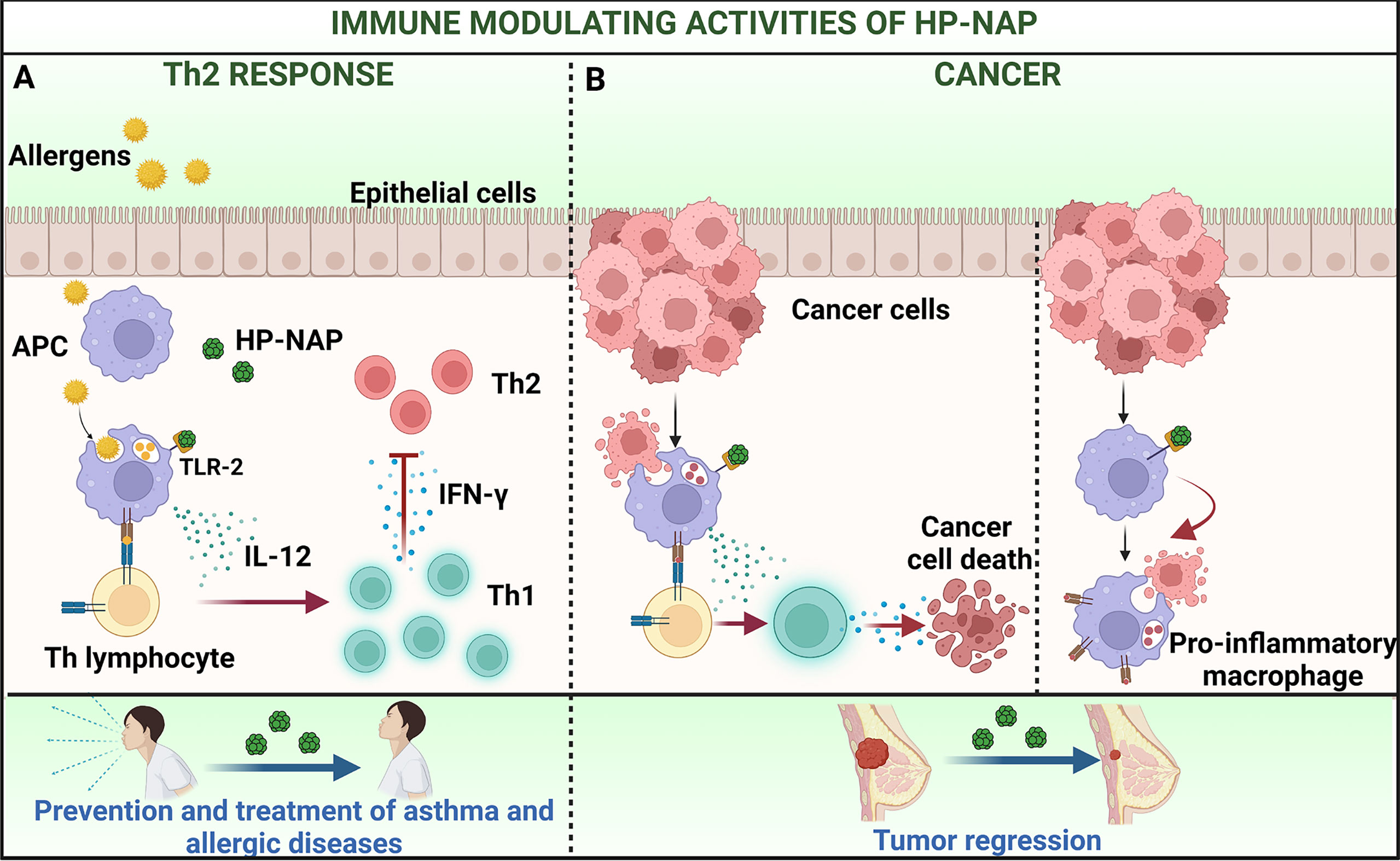
Figure 2 Immune modulating activities of HP-NAP applied to the treatment of allergy and cancer. (A) The delivery of pollen allergens to sub-epithelial APC that initiates the priming of T helper 2 (Th2) cells is a key step in the immunopathology of allergy. Treatment with HP-NAP stimulates APC to secrete IL-12 which mediate the skewing of Th2 lymphocytes towards a Th1 profile. This impacts on the allergic cascade and ameliorates the subsequent symptoms. (B) HP-NAP can potentiate weak natural Th1 responses, that per se are unable to exert protection against tumors (left) and shift the profile of macrophages from pro-oncogenic to pro-inflammatory and anti-tumoral (right). This results in a regression of tumor mass. Figure created with BioRender.com.
Whether the iron-binding ability of HP-NAP is related to the pathogenesis of H. pylori infection or to the immune modulating properties of the miniferritin is not clear. The bacterial protein is constitutively expressed under iron-depletion, and its expression is not regulated by the presence or absence of iron and it has no part in the metal resistance of H. pylori (53). Probably HP-NAP protects the bacterium from the oxidative stress produced in ferrous ion-mediated Fenton reactions, since the degree of DNA damage is much higher in the napA knock-out mutant strain than that in the wild-type strain (54). Moreover, iron plays an important role in generation of the quaternary structure of HP-NAP by promoting stable dimers that are crucial for the ensuing dodecamer structure (55), that is likely to be essential for the immune modulatory properties.
Although more pre-clinical studies are mandatory, the evidence of the clinical potential of HP-NAP are promising and strongly support the possibility of adopting HP-NAP as immunomodulatory agent. The immunostimulatory activity of the bacterial protein could also enhance the immunogenicity of poor immunogens, thus HP-NAP could be used as an adjuvant to be included in vaccines formulations.
Author Contributions
MdB and MMDE conceived the article content. GC prepared the first draft. SC provided a critical review and prepared the figures. All authors contributed to the article and approved the submitted version.
Conflict of Interest
The authors declare that the research was conducted in the absence of any commercial or financial relationships that could be construed as a potential conflict of interest.
Publisher’s Note
All claims expressed in this article are solely those of the authors and do not necessarily represent those of their affiliated organizations, or those of the publisher, the editors and the reviewers. Any product that may be evaluated in this article, or claim that may be made by its manufacturer, is not guaranteed or endorsed by the publisher.
Acknowledgments
We thank the Italian Ministry of University and Research, the University of Padua, the University of Florence, and the Associazione Piccoli Punti, for supporting our studies.
References
1. Andrews SC. “Iron Storage in Bacteria”. In: Advances in Microbial Physiology. (Amsterdam: Elsevier) (1998). p. 281–351. doi: 10.1016/S0065-2911(08)60134-4
2. Smith JL. The Physiological Role of Ferritin-Like Compounds in Bacteria. Crit Rev Microbiol (2004) 30:173–85. doi: 10.1080/10408410490435151
3. Almiron M, Link AJ, Furlong D, Kolter R. A Novel DNA-Binding Protein With Regulatory and Protective Roles in Starved Escherichia Coli. Genes Dev (1992) 6:2646–54. doi: 10.1101/gad.6.12b.2646
4. Martinez A, Kolter R. Protection of DNA During Oxidative Stress by the Nonspecific DNA-Binding Protein Dps. J Bacteriol (1997) 179:5188–94. doi: 10.1128/jb.179.16.5188-5194.1997
5. Davis MM, Brock AM, DeHart TG, Boribong BP, Lee K, McClune ME, et al. The Peptidoglycan-Associated Protein NapA Plays an Important Role in the Envelope Integrity and in the Pathogenesis of the Lyme Disease Spirochete. PloS Pathog (2021) 17:e1009546. doi: 10.1371/journal.ppat.1009546
6. Guerra JPL, Jacinto JP, Tavares P. Miniferritins: Small Multifunctional Protein Cages. Coordination Chem Rev (2021) 449:214187. doi: 10.1016/j.ccr.2021.214187
7. Evans DJ, Evans DG, Lampert HC, Nakano H. Identification of Four New Prokaryotic Bacterioferritins, From Helicobacter pylori, Anabaena variabilis, Bacillus subtilis and Treponema pallidum by Analysis of Gene Sequences. Gene (1995) 153:123–7. doi: 10.1016/0378-1119(94)00774-M
8. Grant RA, Filman DJ, Finkel SE, Kolter R, Hogle JM. The Crystal Structure of Dps, a Ferritin Homolog That Binds and Protects DNA. Nat Struct Mol Biol (1998) 5:294–303. doi: 10.1038/nsb0498-294
9. Ilari A, Stefanini S, Chiancone E, et al. The Dodecameric Ferritin from Listeria innocua Contains a Novel Intersubunit Iron-Binding Site. Nat Struct Mol Biol (2000) 7:38–43. doi: 10.1038/71236
10. Evans DJ, Evans DG, Takemura T, Nakano H, Lampert HC, Graham DY, et al. Characterization of a Helicobacter Pylori Neutrophil-Activating Protein. Infect Immun (1995) 63:2213–20. doi: 10.1128/iai.63.6.2213-2220.1995
11. Codolo G, Bossi F, Durigutto P, Bella CD, Fischetti F, Amedei A, et al. Orchestration of Inflammation and Adaptive Immunity in Borrelia Burgdorferi -Induced Arthritis by Neutrophil-Activating Protein a. Arthritis Rheumatism (2013) 65:1232–42. doi: 10.1002/art.37875
12. Babolin C, Amedei A, Ozoliņš D, Žileviča A, D’Elios MM, de Bernard M. TpF1 From Treponema Pallidum Activates Inflammasome and Promotes the Development of Regulatory T Cells. JI (2011) 187:1377–84. doi: 10.4049/jimmunol.1100615
13. Pozzobon T, Facchinello N, Bossi F, Capitani N, Benagiano M, Di Benedetto G, et al. Treponema Pallidum (Syphilis) Antigen TpF1 Induces Angiogenesis Through the Activation of the IL-8 Pathway. Sci Rep (2016) 6:18785. doi: 10.1038/srep18785
14. D’Elios MM, Vallese F, Capitani N, Benagiano M, Bernardini ML, Rossi M, et al. The Helicobacter Cinaedi Antigen CAIP Participates in Atherosclerotic Inflammation by Promoting the Differentiation of Macrophages in Foam Cells. Sci Rep (2017) 7:40515. doi: 10.1038/srep40515
15. Hooi JKY, Lai WY, Ng WK, Suen MMY, Underwood FE, Tanyingoh D, et al. Global Prevalence of Helicobacter Pylori Infection: Systematic Review and Meta-Analysis. Gastroenterology (2017) 153:420–9. doi: 10.1053/j.gastro2017.04.022
16. Martin-Nuñez GM, Cornejo-Pareja I, Clemente-Postigo M, Tinahones FJ. Gut Microbiota: The Missing Link Between Helicobacter Pylori Infection and Metabolic Disorders? Front Endocrinol (2021) 12:639856. doi: 10.3389/fendo.2021.639856
17. Montemurro P, Nishioka H, Dundon WG, de Bernard M, Del Giudice G, Rappuoli R, et al. The Neutrophil-Activating Protein (HP-NAP) of Helicobacter Pylori is a Potent Stimulant of Mast Cells. Eur J Immunol (2002) 32:671. doi: 10.1002/1521-4141(200203)32:3<671::AID-IMMU671>3.3.CO;2-X
18. Amedei A, Cappon A, Codolo G, Cabrelle A, Polenghi A, Benagiano M, et al. The Neutrophil-Activating Protein of Helicobacter Pylori Promotes Th1 Immune Responses. J Clin Invest (2006) 116:1092–101. doi: 10.1172/JCI27177
19. Montemurro P, Barbuti G, Dundon WG, Del Giudice G, Rappuoli R, Colucci M, et al. Helicobacter pylori Neutrophil-Activating Protein Stimulates Tissue Factor and Plasminogen Activator Inhibitor–2 Production by Human Blood Mononuclear Cells. J Infect Dis (2001) 183:1055–62. doi: 10.1086/319280
20. Middleton J, Neil S, Wintle J, Clark-Lewis I, Moore H, Lam C, et al. Transcytosis and Surface Presentation of IL-8 by Venular Endothelial Cells. Cell (1997) 91:385–95. doi: 10.1016/S0092-8674(00)80422-5
21. Polenghi A, Bossi F, Fischetti F, Durigutto P, Cabrelle A, Tamassia N, et al. The Neutrophil-Activating Protein of Helicobacter Pylori Crosses Endothelia to Promote Neutrophil Adhesion. vivo. J Immunol (2007) 178:1312–20. doi: 10.4049/jimmunol.178.3.1312
22. Satin B, Del Giudice G, Della Bianca V, Dusi S, Laudanna C, Tonello F, et al. The Neutrophil-Activating Protein (Hp-Nap) of Helicobacter Pylori Is a Protective Antigen and a Major Virulence Factor. J Exp Med (2000) 191:1467–76. doi: 10.1084/jem.191.9.1467
23. de Bernard M, D’Elios MM. The Immune Modulating Activity of the Helicobacter Pylori HP-NAP: Friend or Foe? Toxicon (2010) 56:1186–92. doi: 10.1016/j.toxicon.2009.09.020
24. Nishioka H, Baesso I, Semenzato G, Trentin L, Rappuoli R, Del Giudice G, et al. The Neutrophil-Activating Protein of Helicobacter Pylori (HP-NAP) Activates the MAPK Pathway in Human Neutrophils. Eur J Immunol (2003) 33:840–9. doi: 10.1002/eji.200323726
25. D’Elios MM, Manghetti M, De Carli M, Costa F, Baldari CT, Burroni D, et al. T Helper 1 Effector Cells Specific for Helicobacter Pylori in the Gastric Antrum of Patients With Peptic Ulcer Disease. J Immunol (1997) 158:962–7.
26. Bamford KB, Fan X, Crowe SE, Leary JF, Gourley WK, Luthra GK, et al. Lymphocytes in the Human Gastric Mucosa During Helicobacter Pylori Have a T Helper Cell 1 Phenotype. Gastroenterology (1998) 114:482–92. doi: 10.1016/S0016-5085(98)70531-1
27. Luzza F, Parrello T, Monteleone G, Sebkova L, Romano M, Zarrilli R, et al. Up-Regulation of IL-17 Is Associated With Bioactive IL-8 Expression in Helicobacter Pylori -Infected Human Gastric Mucosa. J Immunol (2000) 165:5332–7. doi: 10.4049/jimmunol.165.9.5332
28. Tomita T, Jackson AM, Hida N, Hayat M, Dixon MF, Shimoyama T, et al. Expression of Interleukin-18, a Th1 Cytokine, in Human Gastric Mucosa Is Increased in Helicobacter Pylori Infection. J Infect Dis (2001) 183:620–7. doi: 10.1086/318541
29. Walker JA, McKenzie ANJ. TH2 Cell Development and Function. Nat Rev Immunol (2018) 18:121–33. doi: 10.1038/nri.2017.118
30. León B, Ballesteros-Tato A. Modulating Th2 Cell Immunity for the Treatment of Asthma. Front Immunol (2021) 12:637948. doi: 10.3389/fimmu.2021.637948
31. Codolo G, Mazzi P, Amedei A, Del Prete G, Berton G, D’Elios MM, et al. The Neutrophil-Activating Protein of Helicobacter Pylori Down-Modulates Th2 Inflammation in Ovalbumin-Induced Allergic Asthma. Cell Microbiol (2008) 10:2355–63. doi: 10.1111/j.1462-5822.2008.01217.x
32. Liu X, Fu G, Ji Z, Huang X, Ding C, Jiang H, et al. A Recombinant DNA Plasmid Encoding the sIL-4r-NAP Fusion Protein Suppress Airway Inflammation in an OVA-Induced Mouse Model of Asthma. Inflammation (2016) 39:1434–40. doi: 10.1007/s10753-016-0375-6
33. Dong H, Huang Y, Yao S, Liang B, Long Y, Xie Y, et al. The Recombinant Fusion Protein of Cholera Toxin B and Neutrophil-Activating Protein Expressed on Bacillus Subtilis Spore Surface Suppresses Allergic Inflammation in Mice. Appl Microbiol Biotechnol (2017) 101:5819–29. doi: 10.1007/s00253-017-8370-x
34. Guo X, Ding C, Lu J, Zhou T, Liang T, Ji Z, et al. HP-NAP Ameliorates OXA-Induced Atopic Dermatitis Symptoms in Mice. Immunopharmacol Immunotoxicology (2020) 42:416–22. doi: 10.1080/08923973.2020.1806869
35. Thangam EB, Jemima EA, Singh H, Baig MS, Khan M, Mathias CB, et al. The Role of Histamine and Histamine Receptors in Mast Cell-Mediated Allergy and Inflammation: The Hunt for New Therapeutic Targets. Front Immunol (2018) 9:1873. doi: 10.3389/fimmu.2018.01873
36. Harris NL, Loke P. Recent Advances in Type-2-Cell-Mediated Immunity: Insights From Helminth Infection. Immunity (2017) 47:1024–36. doi: 10.1016/j.immuni.2017.11.015
37. Del Prete G, Chiumiento L, Amedei A, Piazza M, D’Elios MM, Codolo G, et al. Immunosuppression of TH2 Responses in Trichinella Spiralis Infection by Helicobacter Pylori Neutrophil-Activating Protein. J Allergy Clin Immunol (2008) 122:908–913.e5. doi: 10.1016/j.jaci.2008.08.016
38. Waldman AD, Fritz JM. Lenardo Mj. A guide to Cancer immunotherapy: T Cell basic Sci to Clin practice. Nat Rev Immunol (2020) 20:651–68. doi: 10.1038/s41577-020-0306-5
39. Velcheti V, Schalper K. Basic Overview of Current Immunotherapy Approaches in Cancer. Am Soc Clin Oncol Educ Book (2016) 35:298–308. doi: 10.1200/EDBK_156572
40. Codolo G, Fassan M, Munari F, Volpe A, Bassi P, Rugge M, et al. HP-NAP Inhibits the Growth of Bladder Cancer in Mice by Activating a Cytotoxic Th1 Response. Cancer Immunol Immunother (2012) 61:31–40. doi: 10.1007/s00262-011-1087-2
41. Wang T, Liu X, Ji Z, Men Y, Du M, Ding C, et al. Antitumor and Immunomodulatory Effects of Recombinant Fusion Protein rMBP-NAP Through TLR-2 Dependent Mechanism in Tumor Bearing Mice. Int Immunopharmacol (2015) 29:876–83. doi: 10.1016/j.intimp.2015.08.027
42. Mohabati Mobarez A, Soleimani N, Esmaeili S-A, Farhangi B. Nanoparticle-Based Immunotherapy of Breast Cancer Using Recombinant Helicobacter Pylori Proteins. Eur J Pharmaceutics Biopharmaceutics (2020) 155:69–76. doi: 10.1016/j.ejpb.2020.08.013
43. Ruenraroengsak P, Cook JM, Florence AT. Nanosystem Drug Targeting: Facing Up to Complex Realities. J Control Release (2010) 141:265–76. doi: 10.1016/j.jconrel.2009.10.032
44. Kaufman HL, Kohlhapp FJ, Zloza A. Oncolytic Viruses: A New Class of Immunotherapy Drugs. Nat Rev Drug Discovery (2015) 14:642–62. doi: 10.1038/nrd4663
45. Ramachandran M, Yu D, Wanders A, Essand M, Eriksson F. An Infection-Enhanced Oncolytic Adenovirus Secreting H. pylori Neutrophil-activating Protein with Therapeutic Effects on Neuroendocrine Tumors. Mol Ther (2013) 21:2008–18. doi: 10.1038/mt.2013.153
46. Ramachandran M, Jin C, Yu D, Eriksson F, Essand M. Vector-Encoded Helicobacter Pylori Neutrophil-Activating Protein Promotes Maturation of Dendritic Cells With Th1 Polarization and Improved Migration. JI (2014) 193:2287–96. doi: 10.4049/jimmunol.1400339
47. Hou M, Wang X, Lu J, Guo X, Ding C, Liang T, et al. TLR Agonist rHP-NAP as an Adjuvant of Dendritic Cell-Based Vaccine to Enhance Anti-Melanoma Response. IranJImmunol (2020) 17:14–25. doi: 10.22034/iji.2020.80291
48. Iankov ID, Allen C, Federspiel MJ, Myers RM, Peng KW, Ingle JN, et al. Expression of Immunomodulatory Neutrophil-Activating Protein of Helicobacter Pylori Enhances the Antitumor Activity of Oncolytic Measles Virus. Mol Ther (2012) 20:1139–47. doi: 10.1038/mt.2012.4
49. Ma J, Jin C, Čančer M, Wang H, Ramachandran M, Yu D. Concurrent Expression of HP-NAP Enhances Antitumor Efficacy of Oncolytic Vaccinia Virus But Not for Semliki Forest Virus. Mol Ther - Oncolytics (2021) 21:356–66. doi: 10.1016/j.omto.2021.04.016
50. Jin C, Ma J, Ramachandran M, Yu D, Essand M. CAR T Cells Expressing a Bacterial Virulence Factor Trigger Potent Bystander Antitumour Responses in Solid Cancers. Nat BioMed Eng (2022). doi: 10.1038/s41551-022-00875-5
51. Codolo G, Facchinello N, Papa N, Bertocco A, Coletta S, Benna C, et al. Macrophage-Mediated Melanoma Reduction After HP-NAP Treatment in a Zebrafish Xenograft Model. IJMS (2022) 23:1644. doi: 10.3390/ijms23031644
52. Guo L, Yang H, Tang F, Yin R, Liu H, Gong X, et al. Oral Immunization With a Multivalent Epitope-Based Vaccine, Based on NAP, Urease, HSP60, and HpaA, Provides Therapeutic Effect on H. pylori Infection Mongolian gerbils. Front Cell Infect Microbiol (2017) 7:349. doi: 10.3389/fcimb.2017.00349
53. Dundon WG, Nishioka H, Polenghi A, Papinutto E, Zanotti G, Montemurro P, et al. The Neutrophil-Activating Protein of Helicobacter Pylori. Int J Med Microbiol (2001) 291:545–50. doi: 10.1078/1438-4221-00165
54. Wang G, Hong Y, Olczak A, Maier SE, Maier RJ. Dual Roles of Helicobacter Pylori NapA in Inducing and Combating Oxidative Stress. Infect Immun (2006) 74:6839–46. doi: 10.1128/IAI.00991-06
55. Kottakis F, Papadopoulos G, Pappa EV, Cordopatis P, Pentas S, Choli-Papadopoulou T. Helicobacter Pylori Neutrophil-Activating Protein Activates Neutrophils by its C-Terminal Region Even Without Dodecamer Formation, Which Is a Prerequisite for DNA Protection - Novel Approaches Against Helicobacter Pylori Inflammation: DNA Protection and Neutrophil Activation by HP-NAP. FEBS J (2008) 275:302–17. doi: 10.1111/j.1742-4658.2007.06201.x
Keywords: HP-NAP, Helicobacter pylori, inflammation, allergy, cancer, therapy
Citation: Codolo G, Coletta S, D’Elios MM and de Bernard M (2022) HP-NAP of Helicobacter pylori: The Power of the Immunomodulation. Front. Immunol. 13:944139. doi: 10.3389/fimmu.2022.944139
Received: 14 May 2022; Accepted: 31 May 2022;
Published: 29 June 2022.
Edited by:
Laurel L. Lenz, University of Colorado, United StatesReviewed by:
Mohanraj Ramachandran, Uppsala University, SwedenQiaozhen Kang, Zhengzhou University, China
Copyright © 2022 Codolo, Coletta, D’Elios and de Bernard. This is an open-access article distributed under the terms of the Creative Commons Attribution License (CC BY). The use, distribution or reproduction in other forums is permitted, provided the original author(s) and the copyright owner(s) are credited and that the original publication in this journal is cited, in accordance with accepted academic practice. No use, distribution or reproduction is permitted which does not comply with these terms.
*Correspondence: Marina de Bernard, bWFyaW5hLmRlYmVybmFyZEB1bmlwZC5pdA==; Mario Milco D’Elios, bWFyaW9taWxjby5kZWxpb3NAdW5pZmkuaXQ=